Fertilizing Potential of Separated Biogas Digestates in Annual and Perennial Biomass Production Systems
- Department Biobased Products and Energy Crops, Institute of Crop Science, University of Hohenheim, Stuttgart, Germany
Digestates produced by the increasing number of biogas plants require appropriate treatment or recycling. This study investigates the fertilizing potential of separated biogas digestates. These contain valuable nutrients and can be used in agriculture to close the nutrient cycle. Multi-year field experiments were established at two challenging sites in south-west Germany in 2010; results from 6 years are shown here. The objectives were to determine (1) whether separated digestates can complement or substitute mineral fertilizers and (2) their effect on long-term yield performance in different biomass cropping and fertilization systems. The fertilizing performance was assessed in a split-plot design with four replications using three cropping systems: (1) perennial grassland; (2) intercropping of triticale and clover grass; (3) silage maize. Five N fertilization treatments were applied, each at 150 kg N ha−1:
• mineral fertilizer (calcium ammonium nitrate)
• combined solid digestate fraction and mineral fertilizer
• solid digestate fraction
• combined liquid digestate fraction and mineral fertilizer
• liquid digestate fraction.
The influences of site, cropping system, year and fertilization treatment were highly significant.The mineral fertilizer and combination “liquid digestate fraction + mineral fertilizer” mostly led to the highest quantitative biomass yields in all cropping systems at both sites. Fertilization with solid digestate fraction produced lowest yields in all fertilized plots, with results very often not significantly different from the untreated control. Maize achieved relatively high yields in years with favorable weather conditions; unfavorable conditions led to low yields. The grassland and intercropping systems were less susceptible to weather conditions, producing a more constant biomass supply irrespective of site, treatment and year. The separated biogas digestates were found to have a comparable effect to mineral fertilizer on biomass yield, but this varied with cropping system. In the intercropping system, complete substitution was possible. The solid fraction is more likely to contribute positively to soil humus in annual systems. In general, the combined application of digestate and mineral fertilizer is highly recommendable to meet crops' short- and long-term N demand, even on challenging sites. In this study, it allowed a mineral fertilizer input reduction of 66%.
Introduction
Power and heat generated from biogas provide a significant contribution to the increasing amount of bioenergy produced in Europe. Here, more than 17,300 biogas plants were counted in 2015 (EBA, 2016) with a primary production of 654 petajoules (Eurostat, 2015). The biogas sector has experienced a strong impetus in Germany in particular, supported by the German Renewable Energy Sources Act, which was introduced in 2000 and has since been modified several times. In 2017, there were more than 9,300 agricultural biogas plants operating in Germany alone (German Biogas Association, 2017), producing 116 petajoules electric power (BMWi, 2017) and an estimated 65.5 million cubic meters of biogas digestates (Möller and Müller, 2012).
Biogas digestates are the residues left from the anaerobic fermentation of organic matter, such as animal manure and plant biomass specifically grown for this purpose. Through the production of biogas (CH4 and CO2) in the fermentation process, the amount of carbon is significantly (>50%) reduced (Tambone et al., 2009). Depending on the operating system (including pH and temperature) of the biogas plant, N can also be lost (as NH3) to a certain extent (Reinhold et al., 2004). However, most of the N and all other mineral elements contained in the input substrates remain in the biogas digestates (Vaneeckhaute et al., 2017). These include major plant nutrients such as phosphorus, potassium and calcium. Therefore, it is common practice to use biogas digestates as organic fertilizers (Alburquerque et al., 2012a), which at the same time saves costs for both mineral fertilizer and potential disposal of the digestates (up to 25 € t−1, Rolink, 2013). The good fertilizing value of biogas digestates in comparison to mineral fertilizer has been confirmed in several studies (Formowitz and Fritz, 2010; Gunnarsson et al., 2010; Walsh et al., 2012; Barbosa et al., 2014). Also, the remaining carbon bound in the organic matter helps to maintain or even increase soil organic matter (Möller, 2015), which is particularly valuable in marginal soils (Nabel et al., 2014). This effect can be considerable in annual cropping systems.
Although the use of biogas digestates as organic fertilizer seems an efficient way of closing nutrient cycles in agriculture and reducing external inputs of mineral fertilizer, several potential drawbacks need to be considered in order to optimize the efficiency and environmental performance of biomass production systems.
The first is the distribution of digestates. They accumulate at biogas plants and their high water content (>90%) limits their ability to be stored and transported. For this reason, many farms separate the digestates on site in order to reduce the water content and volume and increase transportability (Hjorth et al., 2010). The processing is mostly done using screw press separators, a robust and simple on-farm technology. The separated liquid fraction is characterized by high N (mainly in the form of directly plant-available ammonium) and potassium contents and a total solids content of below 5% (Gutser et al., 2005; Möller et al., 2009; Nkoa, 2014). The solid fraction contains approx. 20% of the total N, a third of the total phosphorus and 15% of the potassium and up to 35% total solids (Rolink, 2013; Vaneeckhaute et al., 2017). Farmers often collaborate with the (more or less) neighboring farms supplying them with biomass in order to optimize operation capacity, particularly in larger biogas plants. The biomass suppliers receive digestates in return, thus helping to manage any oversupply.
Second, the composition of biogas digestates can vary due to variations in substrate supply and, as described above, differs between the solid and liquid fractions. For this reason, farmers are often unsure about the performance of digestates as organic fertilizers and various studies have shown that their fertilizing effect is not always as predictable as that of mineral fertilizer (Möller, 2009; Hjorth et al., 2010; Odlare et al., 2011). Some have reported that such variation in organic fertilizers can lead to fluctuation and/or reduction in biomass yield (e.g. Alburquerque et al., 2012b; Sieling et al., 2013). In order to guarantee biomass yield stability, the yield effect of biogas digestates and their liquid and solid fractions needs to be assessed. One option for overcoming this shortcoming of organic fertilizers may be the use of digestates in combination with mineral fertilizer or gradual supplementation of mineral fertilizers by digestates. To test this, two combinations of digestates and mineral fertilizer were included in this study.
Third, decomposition of the organic matter during the fermentation process leads to an enrichment of NH4-N in biogas digestates (Reinhold et al., 2004). This increases the probability of gaseous N being lost during storage and application. To avoid such losses, field applications of digestates should be timed to meet the crops' nutrient demand and low-emission application techniques should be used. Nutrient demands and optimal fertilization systems very much depend on the type of cropping system. Application techniques and timing, and also the fertilizing effects of organic fertilizers all differ between annual cropping systems, such as maize, and perennial cropping systems, such as grassland (Svoboda et al., 2013). In grassland for example, the immediate effect of an organic fertilizer is usually not very pronounced due to the high organic matter content of the soil (Conant et al., 2017).
In farming practice, the nutrient-rich biogas digestates are generally applied as fertilizer to crops grown for biomass to be used as biogas feedstock. Three such crops are considered in this study: silage maize, grass and winter triticale. Silage maize has been by far the most important biogas crop in Central Europe (Herrmann, 2013), especially Germany (73%, FNR, 2017), for quite some time now. There are several reasons for this including high biomass and methane yields, relatively simple production system, good availability of the required technical equipment and low demands for plant protection (Herrmann et al., 2017). Another aspect is the availability of a wide range of varieties for various site conditions and applications. In Germany however, the proportion of maize in biogas substrate has been limited to a maximum of currently 50% (Bundesministerium der Justiz und für Verbraucherschutz, 2017). This stems from ecological concerns, for example the fact that maize is often cultivated in large-scale monocultures (and unfortunately often also in combination with poor farming practices), leading to an anticipated increase in pests in the future as well as landscape image issues. In addition, experience has shown that maize cultivation is highly susceptible to N losses (via leaching and gaseous emissions) and soil erosion (Taube and Herrmann, 2009; Svoboda et al., 2013). This has led to a call for alternatives to maize as biogas substrate and for diversification in crop rotations (von Cossel et al., 2017). As a result, alternative and more environmentally benign biomass supply systems are currently being sought, including semi- to fully perennial cropping systems.
Permanent grassland is a fully perennial cropping system and a frequent form of land use, especially in agriculturally disadvantaged regions (Huyghe et al., 2014). Cool temperatures and/or a limited vegetation period render them less productive for maize cultivation. Those with a good water supply are very suitable for forage cropping. On such sites, grassland can achieve top yields, comparable to or sometimes even outperforming those of silage maize (Hartmann and Sticksel, 2010). The biomass from grassland (and also clover grass) can be used as animal feed. Any that is not used for feed, e.g., the second and potential following cuts, can be ensiled and digested in a biogas plant (Hartmann et al., 2011). At 12%, grass silage is the second most used biogas crop substrate in Germany (FNR, 2017).
Whole-crop cereals, notably winter triticale, are the third most frequently used biogas crop substrate (8%, FNR, 2017). Winter triticale has a high biomass yield potential and, as a winter cereal crop, can form a valuable part of the crop rotation (Sticksel, 2010). Its ability to resist unfavorable biotic and abiotic environmental factors allows good yields even at marginal sites (Martinek et al., 2008). In our study, it was harvested as whole green crop in early summer. This harvest time makes it difficult to grow a second crop in the same year (Sticksel, 2010). Thus, when grown in an intercropping system, it is most efficient to establish clover grass by undersowing in spring. In this study, the intercropping of triticale and clover grass is considered a “semi-perennial system.” It has positive effects on soil erosion control and N use efficiency due to the year-round soil coverage and the integration of legumes in the crop rotation.
The objectives of this study were to determine whether separated digestates can complement or substitute mineral fertilizers and whether/how they affect the long-term yield performance in different biomass cropping systems.
The research approach was set up to test the following hypotheses:
- The influence of mineral fertilizer and separated biogas digestates on biomass yield is comparable.
- The fertilization effects are stronger in annual cropping systems (with tillage) than in perennial cropping systems.
- The fertilizing effects are influenced by site factors, particularly in the case of organic fertilizers.
These hypotheses were tested by means of a multi-factorial, long-term field experiment allowing a comparison of different fertilization treatments in three cropping systems at two sites. For this purpose, three typical biogas substrate cropping systems (maize; intercropping of winter triticale with clover grass; and grassland) were established on two locations close to a biogas plant. These were chosen to represent an annual, a semi-perennial and a perennial system, respectively. The sites are located at the base and the top of the mountainous region of the Swabian Alb in south-west Germany, both of which display agriculturally challenging conditions (soil quality/growing season, respectively). The fertilizing effects of biogas digestates on these cropping systems were tested using the separated liquid and solid digestate fractions alone and also in combination with mineral fertilizer.
Materials and Methods
Site Description
In 2010, two multi-year field experiments were established on marginal sites belonging to the field research station of the University of Hohenheim in south-west Germany: one at the base (“Valley,” 48.47° latitude, 9.27° longitude, approximately 480 m above sea level, average annual air temperature 10.0°C, average annual rainfall 779 mm) and the other at the top (“Hill,” 48.47° latitude, 9.30° longitude, approximately 700 m above sea level, 7.1°C, 935 mm) of the mountainous region of the Swabian Alb; approximately 35 km south of Stuttgart.
The soil at the “Valley” site is classified as lithoidal clay rendzina with a depth of approximately 0.6 m. The soil at the “Hill” site is a silty clayey loam with a depth of over 1.0 m. The climate data relevant for the field study (2012–2017) are shown in Figure 1. Data for the “Valley” site are taken from the nearest weather station at Metzingen, 48.55° latitude, 9.30° longitude, 391 m above sea level.
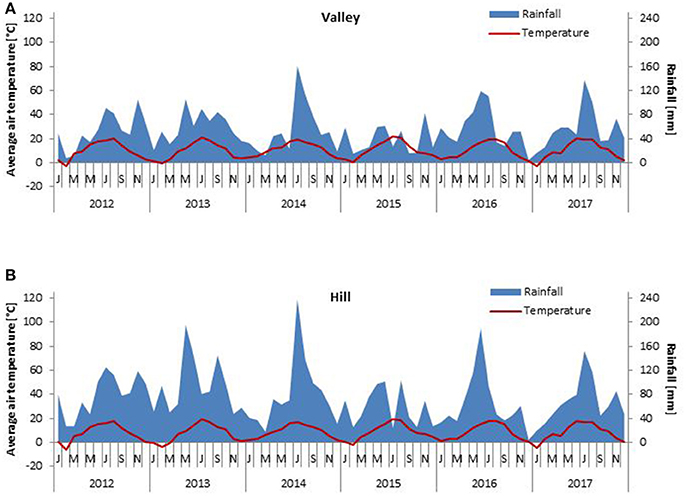
Figure 1. Monthly weather data for field sites “Valley” (A) and “Hill” (B) for years 2012–2017. Average air temperature was measured 2 m above soil surface (Center for Agricultural Technology Augustenberg 2017).
Experimental Approach
The fertilizing performance of separated biogas digestates was assessed using three cropping systems: (1) perennial grassland; (2) intercropping of winter triticale and clover grass; (3) silage maize.
The grassland plots were established in April 2010 using a grassland seed mixture for 3–4 cuts per year (28% Lolium perenne, 19% Festuca pratensis, 19% Phleum pretense, 13% Poa pratensis, 6% Festuca rubra, 6% Dactylis glomerata, 9% Trifolium repens; LAZBW Aulendorf, Germany) sown at a rate of 32 kg ha−1. Reseeding was carried out in August 2014 at a rate of 23 kg ha−1 using a mixture specifically designed for less favorable areas (32% Lolium perenne, 20% Phleum pratense, 16% Poa pratensis, 16% Dactylis glomerata, 4% Alopecurus pratensis, 12% Trifolium repens; LAZBW Aulendorf, Germany) with the aim of maintaining grass cover and counteracting increasing gaps.
The winter triticale (x Triticosecale var. “Tarzan”) plots were generally sown in the first week of October at a rate of 300 seeds m−2. Clover grass was undersown in the triticale in March/April of the years 2013, 2015 and 2017 at a rate of 30 kg ha−1 using a mixture consisting of 83% Italian ryegrass (Lolium multiflorum L. var. “Tarandus”) and 17% red clover (Trifolium pratense L. var. “Titus”). For reasons of clarity, it is referred to as “clover grass” instead of “clover grass mixture.” After the last clover grass cut, plots were cultivated with a rotary hoe (8 cm) and chisel plow (16 cm) and then prepared for sowing triticale by rotary harrow (12 cm).
The maize (Zea mays L.) plots were sown after seedbed preparation with a rotary harrow (12 cm) at a rate of 13 seeds m−2 in rows 0.75 m apart as soon as reasonable, mostly in the first half of May. Varieties were selected according to the vegetation period at each site: “Ronaldinio” (FAO 240) for “Valley” and “Amadeo” (FAO 220) for “Hill.” From 2015 onwards, these were switched to newer varieties with the same FAO numbers, respectively (“Frederico” for “Valley” and “Colisee” for “Hill”). Maize seeds were provided by KWS Saat SE, Einbeck, Germany. Soil tillage included stubble cultivation with a chisel plow (16–18 cm) immediately after harvest and plowing (20 cm) later on.
The three crops were fertilized with separated biogas digestates in four different variants (Table 1). The digestates were obtained from a 355 kilowattelectric biogas plant at the research station, fed mainly with animal manure and maize silage. Solid/liquid separation was performed with a screw press separator. A mineral fertilizer and an untreated control were included for comparison. All treatments except the control were applied at 150 kg N ha−1; amounts and timing are summarized in Table 2. Residual plant-available nitrogen (Nmin), phosphorus, potassium, calcium, magnesium and the pH in the soil were measured every spring and fall to be used for subsequent research analysis (for methods, see Ehmann et al., 2017). Results from the initial soil sampling (0–30 cm) are summarized in Table 3.
Before each application, the content of the digestates was determined to take account of slight variations over time. Each time, two subsamples were taken; one was analyzed directly using a Quantofix N volumeter (Van Kessel and Reeves, 2000), the other was stored at −18°C and analyzed later in the lab (DIN 38406-E5-2) to validate the first measurement.
Table 4 shows the average concentrations of the digestates (values for 2012–2017), together with concentrations of other nutrients and pH (values for 2013–2015).
Applications were split into 2-3 portions to suit the crops' requirements as optimally as possible (Table 2). In grassland and clover grass, the initial portion was usually applied in spring and the subsequent portions after cutting. Where possible, the digestates were incorporated immediately after application using a harrow (10 cm) to minimize N losses. In the combined treatments, digestates and mineral fertilizer were applied approximately 1 week apart from each other.
The experiments were established in a split-plot design with four replications, resulting in 72 plots (32 m2) at each site. Main plots were the cropping systems and subplots the fertilization treatments. Treatments were randomized for each site separately.
Herbicides and fungicides were only applied when necessary and then according to good agricultural practice.
The grassland plots were cut three (in 2016 two) times per year according to good agricultural practice. The last sparse growth of each year was cut and removed from the plots, but not included in the yield.
For the intercropping plots, the harvesting regime was as follows: in the years 2012, 2014, and 2016, the clover grass was harvested three to four times; in 2013, 2015, and 2017 the winter triticale was harvested wholecrop around the early dough stage (BBCH 83) and the undersown clover grass in September or October.
The maize was harvested wholecrop with a plot-size field chopper around the stage of silage ripeness (BBCH 85) and a dry matter content of 30–35% TS when weather conditions were suitable.
Samples were taken from each cut and the dry matter biomass yield (DMY) determined by drying at 60°C to constant weight.
Statistical Analysis
A mixed model was developed for all traits using the following equation (Piepho et al., 2004):
where C and F denote effects of the treatments “cropping system” and “fertilization,” R, L, and Y denote effects of “replicate,” “site,” and “year,” respectively. Interactions between the treatments “site” and “year” are denoted by a dot between the corresponding main effects. “R • Y • L+C • R • Y • L+C • F • R • Y • L” denotes replicate effects and effects of main and subplot error in each combination of site and year. Effects from different years are repeated measurements, therefore a first-order autocorrelation was fitted to them. Crop-by-fertilizer-specific variances were assumed but only fitted to sub-plot errors to avoid convergence problems. Fixed effects are given before the colon. To achieve homogeneous residual variances and normality of residuals, data were log-transformed. Both pre-requirements were checked graphically. Where an F-test revealed significant effects, a multiple t-test (α = 0.05) was performed. To create the letter display, the %mult macro (Piepho, 2012) was used.
Furthermore, cumulated system-by-site-by-fertilizer treatment estimates across years and their standard errors were calculated as a sum of single-year BLUPs (best linear unbiased prediction), or its standard errors, for each combination of system, site and fertilizer treatment. A single-year BLUP here refers to the sum of the least square estimate for one system-by-site-by-fertilizer treatment mean and the corresponding random year main effect and its interaction effects. Yield data was logarithmically transformed, therefore presented values are interpreted as medians. Thus, cumulated yield estimates were also made from the given model.
The data analysis was carried out with SAS software version 9.3 (SAS Institute Inc., Cary, NC, USA).
Results
Cumulative yields from the 6 years are presented here to compare the long-term yield performance of the cropping systems. Table 5 shows the results of the statistical analysis of the main effects; these all had a significant influence except the interaction “site*system.”
Perennial Grassland
At the “Valley” site, the highest DMY was obtained with mineral fertilizer, followed by the two combination treatments. The liquid digestates only and solid digestates only treatments were not significantly different from the combination treatments or the control (Figure 2). At the “Hill” site, the treatments appeared to be more efficient than at the “Valley” site. This is visible from the difference in DMY between control and treatments, both in cumulative as well as annual DMY. All treatments led to a higher DMY than in the control. The highest DMY was obtained with the two treatments containing solids, which were both significantly better than “liquid” only (Figure 2). As to be expected, the first of the usual three cuts made up the largest share of the annual yield.
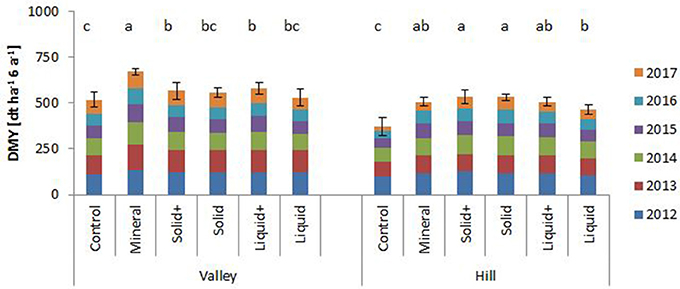
Figure 2. Mean dry matter yield (DMY) of perennial grassland at the sites “Valley” and “Hill” from 2012–2017 fertilized with different treatments of separated biogas digestates and mineral fertilizer in comparison to unfertilized control. Error bars indicate standard deviation of accumulated yields. Means with identical letters are not significantly different from each other (n = 4, p ≤ 0.05). For explanation of treatments, see Table 1.
It was noticeable that in 2017 the DMY was considerably lower at the “Hill” than at the “Valley” site. Here, the effect of decreasing DMY over the years becomes especially visible in the control plots (from 114 dt ha−1 in 2012 down to 49 dt ha−1 in 2017), whereas the “solid+” (average 89 dt ha−1) and “solid” (average 88 dt ha−1) plots were most stable. There were no particular fluctuations visible between the years. At the “Valley” site, the “mineral” plots showed the highest tendency toward decreasing yields.
This general tendency toward declining yields over the years was observed at both sites. It was most likely due to gaps in the grass cover as a consequence of aging plots on the one hand and an infestation with field mice on the other. These gaps increased in frequency and size over time. Although reseeding was performed in August 2014, the plots did not recover satisfactorily as it was too dry during the following weeks. It was observed that the higher-value grass species in the initial seed mix (e.g., including perennial ryegrass Lolium perenne L., meadow fescue Festuca pratensis L., and timothy Phleum pratense L.) disappeared over time and were replaced by species of inferior quality. At the “Valley” site, this was predominantly rough bluegrass (Poa trivialis L.). In addition, the occurrence of broad-leaved dock (Rumex obtusifolius) reduced the quality of botanical composition at this site. Even the frequent cutting did not displace this persistent weed. The plots at the “Valley” site were also invaded by moss. At the “Hill” site, the initially established perennial ryegrass (Lolium perenne L.) was mainly replaced by cocksfoot (Dactylis glomerata L.). In general, the patchiness of the grass cover was less pronounced at the “Hill” than at the “Valley” site.
The dry matter content (DMC) of the grass samples was homogeneous and relatively low. At the “Valley” site, the average DMC of all samples was 20% (cut 1) and 23% (cuts 2–3); at the “Hill” site, 21 and 24%, respectively.
Intercropping of Winter Triticale and Clover Grass
The DMY of the intercropping system was fairly homogeneous at both sites. This was particularly the case at the “Valley” site, where all treatments performed equally well and, with the exception of “solid,” resulted in significantly higher DMY than the control (Figure 3).
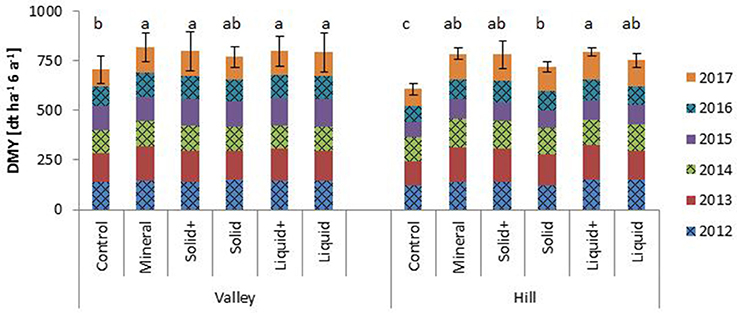
Figure 3. Mean dry matter yield (DMY) of an intercropping system with winter triticale and undersown clover grass grown at the sites “Valley” and “Hill” from 2012–2017 fertilized with different treatments of separated biogas digestates and mineral fertilizer in comparison to unfertilized control. Checked parts of the columns indicate years in which only clover grass was harvested (2012, 2014, 2016). Error bars indicate standard deviation of accumulated yields. Means with identical letters are not significantly different from each other (n = 4, p ≤ 0.05). For explanation of treatment, see Table 1.
At the “Hill” site, all treatments increased the yield compared to the control. The highest DMY was obtained with the “liquid+” treatment. This was significantly higher than with the “solid” treatment (Figure 3).
The highest yields were obtained at both sites in 2012 and 2013. After this, the yields decreased, but remained at a more or less constant level (120 dt ha−1 at the “Valley” site, 112 dt ha−1 at the “Hill” site). As expected, the yield difference between control and treatments was larger for triticale than for clover grass, indicating a more prominent fertilizing effect.
In 2015, the triticale DMY was reduced at the “Hill” site due to infestation with yellow rust (Puccinia striiformis).
Figure 4 shows that the majority of the triticale plots were harvested too late and the DMC was higher than the optimal value, particularly at the “Valley” site. The DMC varied considerably more at the “Valley” than at the “Hill” site. In 2017, the average DMC at the “Valley” site was 63% with individual maximal values of more than 80%, whereas in the other years values were more within the normal range (46% in 2013, 36% in 2015).
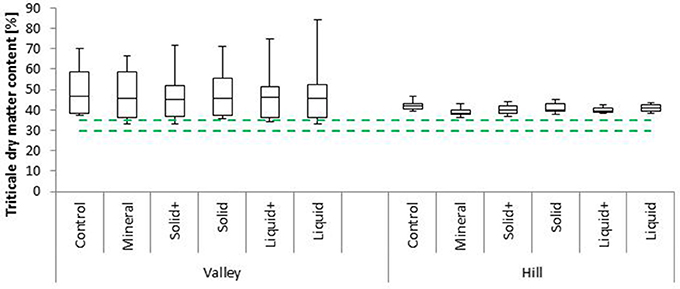
Figure 4. Dry matter contents of triticale grown at the sites “Valley” and “Hill” in 2013, 2015, and 2017 fertilized with different treatments of separated biogas digestates and mineral fertilizer in comparison to unfertilized control (n = 4). The green lines indicate the optimal dry matter content range. For explanation of treatments, see Table 1.
Silage Maize
At both sites, the highest maize DMY was obtained with mineral fertilizer; however, this was only significant at the “Hill” site (Figure 5).
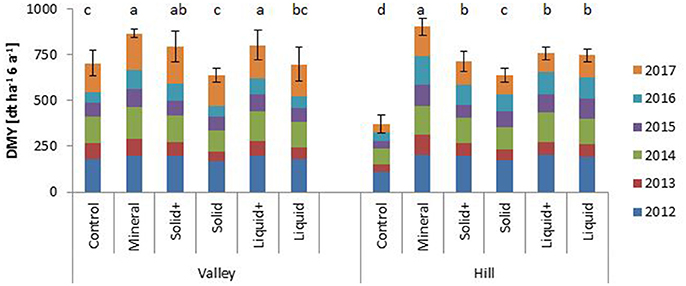
Figure 5. Mean dry matter yield (DMY) of silage maize grown at the sites “Valley” and “Hill” from 2012–2017 fertilized with different treatments of separated biogas digestates and mineral fertilizer in comparison to unfertilized control. Error bars indicate standard deviation of accumulated yields. Means with identical letters are not significantly different from each other (n = 4, p ≤ 0.05). For explanation of treatments, see Table 1.
At the “Valley” site, both combination treatments performed as well as the mineral fertilizer.
At the “Hill” site, all treatments with digestates except “solid” were comparable to each other and resulted in the second highest DMY after mineral fertilizer.
In general, the DMY standard deviations were higher and fluctuated more at the “Valley” than at the “Hill” site. This is likely due to the relatively high heterogeneity of the field conditions. In addition, problems with regrowth from the preceding crop Jerusalem artichoke (Helianthus tuberosus) led to massive yield reductions in certain plots, in one replication in particular, despite frequent manual weeding and the occasional herbicide application. It is interesting that the lowest standard deviation at the “Valley” site was found with mineral fertilizer indicating a reliable fertilizing effect, independent of external influences.
The effect of year was clearly recognizable. 2013 and 2015 were not good years for maize cultivation: wet and cold conditions in spring delayed sowing and/or germination; drought periods with high temperatures in summer months negatively influenced growth (see also Figure 1 for weather data). In those years, the mineral fertilizer showed the best performance of all treatments. In years with weather conditions favorable for maize cultivation (2014, 2016, 2017) most digestate treatments worked equally well as mineral fertilizer.
The year 2016 was exceptional in that the spring was cold and wet, but there was a short favorable time slot which could be used for sowing. This was followed by a lot of rain in early summer before a dry, hot period set in. At the “Valley” site, this resulted in low DMY (on average 83 dt ha−1 for fertilized plots), but a very satisfactory yield at the “Hill” site (118 dt ha−1). Here, the higher altitude and thus lower average temperature, together with the deep soil, were an advantage. Consequently, the water supply lasted longer during the heatwave, ensuring better growth than at the “Valley” site.
The separated solid digestate variant led to the lowest yields of all treatments at both sites (Figure 5). This was visible in most years, but also for the accumulated yields. At the “Valley” site, it resulted in yields comparable to the control and to the “liquid” treatment (or even lower in absolute values). At the “Hill” site, it had a DMY higher than the control, but lower than the other treatments.
Figure 6 shows that the majority of the maize plots were harvested with a dry matter content (DMC) within the optimal range of 30 to 35% TS. In general, DMC was lower and fluctuated less at the “Hill” site.
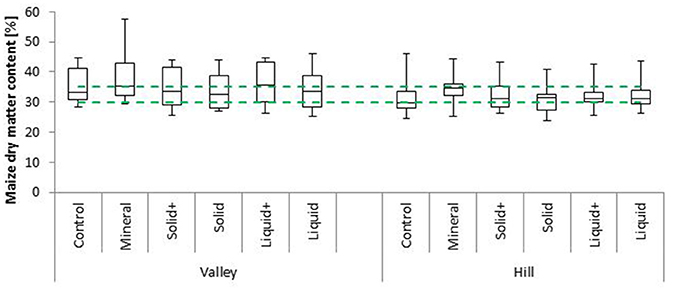
Figure 6. Dry matter contents of silage maize grown at the sites “Valley” and “Hill” from 2012–2017 fertilized with different treatments of separated biogas digestates and mineral fertilizer in comparison to unfertilized control (n = 4). The green lines indicate the optimal dry matter content range. For explanation of treatments, see Table 1.
Discussion
Significant differences in yield performance were found between the annual, intercropping and perennial cropping systems subjected to the treatment variants. Interactions with site and year effects were also observed.
The highest and most stable biomass yields were found in the intercropping system with triticale and clover grass, irrespective of the site, treatment and year. This was followed by perennial grassland, which also proved to be relatively stable with regard to treatment and year, but provided lower DMY. Maize only produced high yields in years with favorable climatic conditions. Particularly in years with unfavorable conditions, the best maize yields were achieved with mineral fertilizer, whereas in normal years the DMY difference between treatments was small. Thus the influence of the year effect also varied between cropping systems.
In general, the “Valley” site had higher DMY, but the “Hill” site provided better conditions for growth during hot, dry periods due to the lower average temperature and longer water supply. We also observed that the treatments were more effective in terms of yield at the “Hill” site, as the DMY was significantly higher than the control on all fertilized plots in all three systems here.
Effect of Fertilizer Treatments on Yield Performance of the Three Cropping Systems
The annual system responded most sensitively to influences of treatments, site and year. The yields were significantly influenced by all these factors. As a C4 crop, maize reacts relatively strongly to temperature fluctuations and requires favorable temperatures and sufficient water supply for germination and good establishment, especially if sown in late spring (Maton et al., 2007). In this study, the highest maize yields were achieved with mineral fertilizers, particularly in cooler years and at the cooler “Hill” site. This can be explained by the fact that mineral fertilizer application can be timed to provide plant-available N to coincide with the crop's requirements (Möller, 2009). The N availability of mineral fertilizer is also less dependent on climatic conditions, especially temperature and water supply, than organic fertilizer (Agehara and Warncke, 2005) and the share of mineral N is of course higher than in the digestates (Table 4). After sowing, maize first needs to build its root system and is highly dependent on rapidly plant-available N at exactly the right time (Plénet and Lemaire, 1999). This can best be steered by the application of easily soluble mineral fertilizer.
The effect of more rapid N availability from mineral than from organic fertilizers was particularly evident in the years 2013 and 2015 when temperatures were lower than the long-term average and 2015 also had less than average rainfall during the growing season. In these years, the mineral fertilizer had significantly better results, especially at the “Hill” site, and the solids had a lower performance. In 2013 at the “Valley” site, the solids even resulted in a lower DMY than the unfertilized control. This may have been caused by initial N immobilization, which often occurs after the application of organic matter (Gutser et al., 2005).
Mineral fertilizer had the best effect on maize yield, in terms of both amount and stability, over the years. This was the case for application of mineral fertilizer alone as well as in combination with digestates. The crop's short-term demand for plant-available N was met through the mineral fertilizer and later—once the maize had established—N from the digestate had been mineralized and could provide the maize with a sufficient supply. In addition, the combinations provided at least a certain amount of organic matter (OM). This may be valuable as maize leaves a limited amount of crop residues and its cultivation tends to reduce soil organic matter and humus (Karpenstein-Machan, 2013; Komainda et al., 2018). Several studies have suggested that the combination of organic and mineral fertilizers can improve the regulation of N supply and enhance the effect of the two fertilizer types. As such, it is the most effective way of achieving both high yields and at the same time a build-up of soil organic matter (SOM) (Rauhe, 1987; Körschens et al., 1998; Svensson et al., 2004; Gutser et al., 2005; Möller, 2009). However, as simultaneous application can temporarily immobilize mineral N and increase the risk of N2O emissions, it is recommended that digestates and mineral fertilizer are applied with a time delay (Möller et al., 2009). We followed this recommendation in our field experiments.
In addition, the effect of combined mineral and organic fertilizers versus the application of mineral fertilization alone depends on site conditions. At the cooler “Hill” site, where the soil only warms up slowly in spring, the mineral treatment worked significantly better than the combinations. By contrast, at the “Valley” site, the mineral fertilizer and the combinations had comparable effects.
As expected, and observed at both sites, the yield effects of the different fertilizer types were less pronounced in permanent grassland than in the annual cropping system. As grassland is characterized by year-round soil cover, it can better exploit the long-term fertilizing effects of the organic treatments than the other two systems. These long-term effects result from the more continuous N release as well as better water retention and other factors improving soil fertility. However, grassland proved to be the system with the lowest total yields over 6 years. In addition, the aging effect of the plots in this system needs to be considered. For this reason, it is difficult to assess the effects of fertilizer treatment and system separately, as the system itself degrades over time (increasing gaps, reduction/loss of valuable grass species) and yields subsequently decrease. Therefore, the aging effect on yields may mask the fertilizer effects.
For permanent grasslands, there were also clear site effects. At the warmer “Valley” site, mineral fertilizer resulted in the significantly highest DMY. In contrast, at the “Hill” site, both treatments with solids led to the highest DMY during the experimental period. This was somewhat unexpected as the solids treatments had lower yields in the annual and intercropping systems at both sites. At the beginning of the experiment, we had assumed that organic fertilizers would be less effective the more marginal the site conditions are. As N mineralization and OM turnover are influenced by temperature (Davidson and Janssens, 2006), it was surprising to find the good performance of the treatments with solids at the site with lower average temperature and limited vegetation period. This result was undoubtedly a consequence of an interaction between system, treatment and site, but cannot be sufficiently explained by the data collected in this study. Repeated application of solid digestates could have increased the soil pH at this site, which was relatively low at the beginning of the experiment (5.5). However, an intermediate soil analysis in fall 2014 showed that the pH had decreased to 5.3 on average on all grassland plots. The smallest decrease was found on plots treated with solids (5.4). Nabel et al. (2017) found that the comparative advantage of digestate fertilization over mineral NPK fertilization on biomass yield became increasingly pronounced over time and explained this through the crucial role of soil carbon content for plant growth. This obviously applies more to perennial systems where the soil is not disturbed and becomes more important the more marginal the soil is. This may serve as an explanation for the surprising performance of the solids at the “Hill” site. However, our hypothesis is that the proportion of nutrient supply provided by OM turnover increases with time and thus renders the grassland system increasingly independent of the direct nutrient effect of the fertilizers.
The intercropping system (here two crops grown in rotation) proved to be a stable and robust system that provided constantly high yields. In this system, the soil was almost always covered (except during early development stages of triticale). Unlike maize, generally all fertilizer treatments worked equally well independent of the site or crop. The yields in the intercropping system appeared to profit from the crop rotation effect, mainly from the biological fixation of atmospheric N2 by the clover in the mixture (not quantified). This is intended to ensure a more constant N supply independent of fertilizer applications, for example during periods of low N availability due to insufficient amounts of mineralized N. The leguminous component of this system differentiates it from the others. Grassland also contains some clover, but in the intercropping system clover is sown afresh every other year resulting in a higher proportion of legumes in the sward and consequently a higher N fixation rate.
The clover grass and triticale both developed intensive root systems; thus the intercropping system produced a considerable amount of crop residues which additionally contributed to the build-up of SOM and the residual supply of mineralized N (Fouda et al., 2013).
In this study, we focused on the effects of the treatments on biomass yield of the cropping systems and mainly limited the explanation of different fertilizer effects to differences in the timeliness of N availability and the capacity of the various fertilizer types to contribute to SOM production. Another aspect that was considered in explaining differences in yield effects of the various fertilizer types was their interaction with the three cropping systems tested here. All cropping systems have their growth peaks at different times, which clearly affects the nutrient demand and uptake during the vegetation period (Herrmann et al., 2017).
Implications of Different Fertilization Systems
When assessing the suitability of biogas digestates as fertilizers, other aspects in addition to the yield effect need to be considered. Clearly, a farmer who produces biogas needs to dispose of the digestates. In practice, biogas digestates are often separated and used as fertilizer on the farm. However, when other feedstock streams, such as slurry, are co-digested in the biogas plant, the nutrients in the digestates constitute an oversupply at farm level. Therefore, digestates are often transported to other farms. Alternatively, they can be further processed to bio-based mineral fertilizers (Vaneeckhaute et al., 2017). For example, nutrients can be recovered from the liquid fraction by precipitation and filtered off as a mixture of phosphate salts, including struvite (Bilbao et al., 2017; Ehmann et al., 2017). Since this process is costly, the extent to which digestates are directly applied as organic fertilizer or, especially in the case of the liquid fraction, are processed into mineral fertilizer should be carefully considered on a case-by-case basis.
Mineral fertilizer use is always accompanied by the highest costs and environmental impacts, irrespective of whether it is produced chemically (N), from mining (P) or through recovery from biogas digestates (N and P). From a farming practice point of view, mineral fertilizers have the advantage of more predictable N supply on the one hand and easier applicability on the other. The latter is particularly relevant for permanent cropping systems. One major environmental benefit of digestates is that they can help save on mineral N fertilizer, either by complete or partial substitution. In good agricultural practice, gaseous emissions during and after digestate application are kept to a minimum, which was not ensured with the liquid manure spreader used in this study. Application techniques near the soil surface including trailing hoses, trailing shoes and injection would of course reduce gaseous losses (especially in systems and at stages where incorporation is not possible) and at the same time increase the plant-usable N (Möller et al., 2008). The solid fraction should ideally be incorporated into the soil to avoid gaseous N losses (Holly et al., 2017), allow for nutrient release through decomposition and avoid a layer of organic matter remaining on the crop. The application of solids is even more laborious in systems which require multiple cuts over the vegetation period. Although our results showed that solids significantly increased grassland yields, at least at the less favorable “Hill” site, the practicability of solid application remains limited. For this reason, only the liquid fraction is recommended for grassland due to its good infiltration, and also its high N and K but low P contents which correspond well with the nutrient removal by the crops (Messner, 2014).
The application of solid digestates thus appears more appropriate in cropping systems with frequent soil cultivation and on sites where a benefit from OM can be expected. Soil tillage increases the turnover of OM from digestates and crop residues (Blair et al., 2006; Sarker et al., 2018). Although solids were actually not recommendable for maize in terms of their fertilizing effect, their regular application is considered beneficial here for OM replacement (Nkoa, 2014). A study by Nabel et al. (2017) showed that organic fertilization with digestates had a positive influence on soil properties (e.g. increased soil respiration and enhanced water-holding capacity), particularly on marginal sites. The supply of nutrients other than N, including P, K and various microelements, is a further advantage over mineral fertilizer (Risberg, 2015).
In this study, we divided the fertilizer and digestate applications into several doses. In farming practice, this effort may be lowered by reducing the number of fertilizer doses. In grassland, the majority of the N dose would be applied in late winter or early spring, followed by only one more dose later on (Möller et al., 2009). In maize for example, the solids could be applied in one dose before sowing. This may even be possible for the liquids, primarily in the combinations. Lavandier et al. (2011) fertilized silage maize with up to 170 kg N ha−1, applied in form of liquid digestate in one dose and found that this did not lead to increased Nmin values.
In this study, grassland proved to be the system with lowest yields and highest workload. Nevertheless, permanent grassland is considered the most environmentally friendly way of producing energy crops (Rippel, 2008) and provides a suitable opportunity to maintain ecologically valuable grasslands that are no longer used for fodder production. This is particularly the case when mineral fertilizer is replaced with digestates, because the grassland productivity can be maintained with lower environmental impact (Walsh et al., 2012).
Conclusion
The first hypothesis underlying this study, that the influence of mineral fertilizer and separated biogas digestates on biomass yield is comparable, was confirmed. However, the recommendations that can be deduced from this vary depending on cropping system and site. All three systems tested revealed their own specific strengths and weaknesses; the same applies to the treatments. For perennial or intercropping systems, separated digestates can be fully recommended. In the intercropping of triticale and clover grass, separated digestates were able to substitute mineral fertilizer completely. Contrary to our expectations, the solids performed very well in terms of yield in interaction with grassland at the “Hill” site. However, it was seen that the use of solids in permanent grassland does not exploit their full potential. A higher benefit from solids is expected from application in annual systems where they can contribute positively to the build-up of OM. Any short-term N demand of crops is better met by a combination of digestates (liquid preferable to solid, due to high content of plant-available ammonia-N) and mineral fertilizer. The combinations performed equally well as mineral fertilizer alone in most of the systems, sites and years and allowed mineral fertilizer input to be reduced by 66%.
The second hypothesis, that fertilization effects are stronger in annual cropping systems (with tillage) than in perennial cropping systems, could be partly confirmed. If the objective is to maximize yield performance, the preferred option is the use of mineral fertilizer alone or in combination with digestates. Since the application of solid digestates and their incorporation into soil is most difficult in perennial systems, the best balance between the goals of high biomass yield and maintenance/increase of SOM content on the one hand, and the practicability of applying solid digestates on the other, can be achieved in the intercropping system.
The third hypothesis, that fertilizing effects are influenced by site factors, particularly in the case of organic fertilizers, could also be confirmed. The effect of organic fertilizer was found to be unpredictable, especially on cooler sites. To avoid yield fluctuations and N losses on such sites, perennial systems are recommended, as they capture N released at different times in the vegetation period. For these sites, the positive effect of solid biogas digestates on soil fertility and SOM can help improve the long-term stability of biomass production.
In summary, the combined application of organic and mineral fertilizer is the best approach to implement the multiple aims in terms of high yields, low-cost farming and minimal negative environmental impacts. The good performance of the combinations, together with reduced expenses for mineral fertilizer, can help improve farmers' acceptance of organic fertilizers.
Author Contributions
AE: carried out the experiments, was involved in sample analysis and analyzed the data. UT and IL: were responsible for the original idea for the research and initial concept of the experiment. All authors contributed to the preparation of the manuscript.
Conflict of Interest Statement
The authors declare that the research was conducted in the absence of any commercial or financial relationships that could be construed as a potential conflict of interest.
Acknowledgments
AE was supported by a scholarship from the Faculty of Agricultural Sciences, University of Hohenheim. The authors are grateful to Peter Weckherlin for the excellent maintenance and management of the field experiments and to Sven Schabel and Anja Neuberdt for their assistance with fieldwork. Particular thanks go to Nicole Gaudet for proofreading the manuscript and to Jens Möhring for statistical advice. Weather data were kindly provided by the Center for Agricultural Technology Augustenberg, Karlsruhe, Germany; maize seeds by KWS Saat SE, Einbeck, Germany; and Triticale seeds ‘Tarzan’ by Pflanzenzucht Oberlimpurg, Schwäbisch Hall, Germany.
Selected yield data from the years 2012 and 2013 were presented at the International Conference Progress in Biogas III, 10 to 11 September 2014, Stuttgart, Germany.
References
Agehara, S., and Warncke, D. D. (2005). Soil moisture and temperature effects on nitrogen release from organic nitrogen sources. Soil Sci. Soc. Am. J. 69:1844. doi: 10.2136/sssaj2004.0361
Alburquerque, J. A., de La Fuente, C., and Bernal, M. P. (2012a). Chemical properties of anaerobic digestates affecting C and N dynamics in amended soils. Agric. Ecosyst. Environ. 160, 15–22. doi: 10.1016/j.agee.2011.03.007
Alburquerque, J. A., La Fuente, C., de Campoy, M., Carrasco, L., Nájera, I., Baixauli, C., et al. (2012b). Agricultural use of digestate for horticultural crop production and improvement of soil properties. Eur. J. Agron. 43, 119–128. doi: 10.1016/j.eja.2012.06.001
Barbosa, D., Nabel, M., and Jablonowski, N. D. (2014). Biogas-digestate as nutrient source for biomass production of Sida hermaphrodita, Zea mays L. and Medicago sativa L. Energy Procedia 59, 120–126. doi: 10.1016/j.egypro.2014.10.357
Bilbao, J., Campos, A., Mariakakis, I., Mack, S., Egner, S., Bach, I. M., et al. (2017). “Fertilizers and soil improvers recovered from digestate in the biogas process chain,” in Proceedings International Conference Progress in Biogas IV (Stuttgart; Hohenheim).
Blair, N., Faulkner, R. D., Till, A. R., Korschens, M., and Schulz, E. (2006). Long-term management impacts on soil C, N and physical fertility: Part II: Bad Lauchstadt static and extreme FYM experiments. Soil Tillage Res. 91, 39–47. doi: 10.1016/j.still.2005.11.001
BMWi Bundesministerium für Wirtschaft und Energie (2017). Erneuerbare Energien in Zahlen. Nationale und Internationale Entwicklung im Jahr 2016. Available online at: https://www.bmwi.de/Redaktion/DE/Publikationen/Energie/erneuerbare-energien-in-zahlen-2016.pdf?__blob=publicationFile&v=8 (Accessed January 26, 2018).
Bundesministerium der Justiz und für Verbraucherschutz (2017). Gesetz für den Ausbau erneuerbarer Energien (Erneuerbare- Energien-Gesetz-EEG 2017).
Conant, R. T., Cerri, C. E., Osborne, B. B., and Paustian, K. (2017). Grassland management impacts on soil carbon stocks: a new synthesis. Ecol. Appl. 27, 662–668. doi: 10.1002/eap.1473
Davidson, E. A., and Janssens, I. A. (2006). Temperature sensitivity of soil carbon decomposition and feedbacks to climate change. Nature 440, 165–173. doi: 10.1038/nature.04514
E. B. A European Biogas Association (2016). EBA Launches 6th Edition of the Statistical Report of the European Biogas Association. Available online at: http://european-biogas.eu/2016/12/21/eba-launches-6th-edition-of-the-statistical-report-of-the-european-biogas-association/ (Accessed Jan 26, 2018).
Ehmann, A., Bach, I.-M., Laopeamthong, S., Bilbao, J., and Lewandowski, I. (2017). Can phosphate salts recovered from manure replace conventional phosphate fertilizer? Agriculture 7:1. doi: 10.3390/agriculture7010001
Eurostat (2015). Supply, Transformation and Consumption of Renewable Energies - Annual Data. Available online at: http://appsso.eurostat.ec.europa.eu/nui/submitViewTableAction.do (Accessed Jan 26, 2018).
FNR (2017). Massebezogener Substrateinsatz nachwachsender Rohstoffe in Biogasanlagen. Available online at: https://mediathek.fnr.de/grafiken/daten-und-fakten/bioenergie/biogas/massebezogener-substrateinsatz-nachwachsender-rohstoffe-in-biogasanlagen.html (Accessed Jan 26, 2018).
Formowitz, B., and Fritz, M. (2010). “Biogas digestates as organic fertilizer in different crop rotations,” in Proceedings of the 18th European Biomass Conference and Exhibition (Lyon).
Fouda, S., Tucher, S., von Lichti, F., and Schmidhalter, U. (2013). Nitrogen availability of various biogas residues applied to ryegrass. Z. Pflanzenernähr. Bodenk. 176, 572–584. doi: 10.1002/jpln.201100233
German Biogas Association (2017). Biogas market data in Germany 2016/2017. Available onlinne at: https://www.biogas.org/edcom/webfvb.nsf/id/DE_Branchenzahlen/$file/17-10-13_Biogasindustryfigures-2016-2017.pdf (Accessed Jan 26, 2018).
Gunnarsson, A., Bengtsson, F., and Caspersen, S. (2010). Use efficiency of nitrogen from biodigested plant material by ryegrass. J. Plant Nutr. Soil Sci. 173, 113–119. doi: 10.1002/jpln.200800250
Gutser, R., Ebertseder, T., Weber, A., Schraml, M., and Schmidhalter, U. (2005). Short-term and residual availability of nitrogen after long-term application of organic fertilizers on arable land. J. Plant Nutr. Soil Sci. 168, 439–446. doi: 10.1002/jpln.200520510
Hartmann, S., Diepolder, M., and Lichti, F. (2011). Grünland als Biogassubstrat. Bayerische Landesanstalt für Landwirtschaft, Institut für Pflanzenbau und Pflanzenzüchtung. Freising.
Hartmann, S., and Sticksel, E. (2010). Kleegras als Biogassubstrat. Bayerische Landesanstalt für Landwirtschaft, Institut für Pflanzenbau und Pflanzenzüchtung. Freising.
Herrmann, A. (2013). Biogas production from maize: current state, challenges and prospects. 2. agronomic and environmental aspects. Bioenerg. Res. 6, 372–387. doi: 10.1007/s12155-012-9227-x
Herrmann, A., Kage, H., Taube, F., and Sieling, K. (2017). Effect of biogas digestate, animal manure and mineral fertilizer application on nitrogen flows in biogas feedstock production. Eur. J. Agron. 91, 63–73. doi: 10.1016/j.eja.2017.09.011
Hjorth, M., Christensen, K. V., Christensen, M. L., and Sommer, S. G. (2010). Solid-liquid separation of animal slurry in theory and practice. A review. Agron. Sustain. Dev. 30, 153–180. doi: 10.1051/agro/2009010
Holly, M. A., Larson, R. A., Powell, J. M., Ruark, M. D., and Aguirre-Villegas, H. (2017). Greenhouse gas and ammonia emissions from digested and separated dairy manure during storage and after land application. Agric. Ecosyst. Environ. 239, 410–419. doi: 10.1016/j.agee.2017.02.007
Huyghe, C., Vliegher, A., de van Gils, B., and Peeters, A. (2014). Grasslands and Herbivore Production in Europe and Effects of Common Policies. Versailles: Editions Quae.
Karpenstein-Machan, M. (2013). “Integrative energy crop cultivation as a way to a more nature-orientated agriculture,” in Sustainable Bioenergy Production - An Integrated Approach, eds H. Ruppert, M. Kappas, and J. Ibendorf (Dordrecht: Springer Netherlands), 143–180.
Komainda, M., Taube, F., Kluß, C., and Herrmann, A. (2018). The effects of maize (Zea mays L.) hybrid and harvest date on above- and belowground biomass dynamics, forage yield and quality – A trade-off for carbon inputs? Eur. J. Agron. 92, 51–62. doi: 10.1016/j.eja.2017.10.003
Körschens, M., Weigel, A., and Schulz, E. (1998). Turnover of soil organic matter (SOM) and long-term balances-tools for evaluating sustainable productivity of soils. J. Plant Nutr. Soil Sci. 161, 409–424. doi: 10.1002/jpln.1998.3581610409
Lavandier, P., Riexinger, J., Müller, T., Schulz, R., Huchler, G., and Ehrhart, E. (2011). “Einmalige Gülle-Düngung zu Silomais mit Biogasgülle bzw. angereicherter Biogasgülle als Gülledepot mit Grubber oder Scheibenegge ausgebracht,” in Gülle- und Gärrestdüngung auf Grünland, eds M. Elsäßer, M. Diepolder, O. Huguenin-Elie, E. Pötsch, H. Nußbaum, and J. Messner (Aulendorf: LAZBW), 112–115.
Martinek, P., Vinterová, M., Burešová, I., and Vyhnánek, T. (2008). Agronomic and quality characteristics of triticale (X Triticosecale Wittmack) with HMW glutenin subunits 5+10. J. Cereal Sci. 47, 68–78. doi: 10.1016/j.jcs.2007.02.003
Maton, L., Bergez, J.-E., and Leenhardt, D. (2007). Modelling the days which are agronomically suitable for sowing maize. Eur. J. Agron. 27, 123–129. doi: 10.1016/j.eja.2007.02.007
Messner, J. (2014). Separierung kostet mindestens 2 Euro/m3. Badische Bauernzeitung. Available online at: http://www.badische-bauern-zeitung.de/separierung-kostet-mindestens-2-euro/m3 (Accessed Jan 30, 2018).
Möller, K. (2009). Influence of different manuring systems with and without biogas digestion on soil organic matter and nitrogen inputs, flows and budgets in organic cropping systems. Nutr. Cycl. Agroecosyst. 84, 179–202. doi: 10.1007/s10705-008-9236-5
Möller, K. (2015). Effects of anaerobic digestion on soil carbon and nitrogen turnover, N emissions, and soil biological activity. A review. Agron. Sustain. Dev. 35, 1021–1041. doi: 10.1007/s13593-015-0284-3
Möller, K., and Müller, T. (2012). Effects of anaerobic digestion on digestate nutrient availability and crop growth: a review. Eng. Life Sci. 12, 242–257. doi: 10.1002/elsc.201100085
Möller, K., Schulz, R., and Müller, T. (2009). Mit Gärresten richtig Düngen. Aktuelle Informationen für Berater. Institute of Plant Nutrition, University of Hohenheim.
Möller, K., Stinner, W., Deuker, A., and Leithold, G. (2008). Effects of different manuring systems with and without biogas digestion on nitrogen cycle and crop yield in mixed organic dairy farming systems. Nutr. Cycl. Agroecosyst. 82, 209–232. doi: 10.1007/s10705-008-9196-9
Nabel, M., Barbosa, D. B., Horsch, D., and Jablonowski, N. D. (2014). Energy crop (Sida hermaphrodita) fertilization using digestate under marginal soil conditions: a dose-response experiment. Energy Procedia 59, 127–133. doi: 10.1016/j.egypro.2014.10.358
Nabel, M., Schrey, S. D., Poorter, H., Koller, R., and Jablonowski, N. D. (2017). Effects of digestate fertilization on Sida hermaphrodita: boosting biomass yields on marginal soils by increasing soil fertility. Biomass Bioenergy 107, 207–213. doi: 10.1016/j.biombioe.2017.10.009
Nkoa, R. (2014). Agricultural benefits and environmental risks of soil fertilization with anaerobic digestates: a review. Agron. Sustain. Dev. 34, 473–492. doi: 10.1007/s13593-013-0196-z
Odlare, M., Arthurson, V., Pell, M., Svensson, K., Nehrenheim, E., and Abubaker, J. (2011). Land application of organic waste – Effects on the soil ecosystem. Appl. Energy 88, 2210–2218. doi: 10.1016/j.apenergy.2010.12.043
Piepho, H. P. (2012). A SAS macro for generating letter displays of pairwise mean comparisons. Commun. Biometry Crop Sci. 7, 4–13.
Piepho, H. P., Büchse, A., and Richter, C. (2004). A mixed modelling approach for randomized experiments with repeated measures. J. Agron. Crop Sci. 190, 230–247. doi: 10.1111/j.1439-037X.2004.00097.x
Plénet, D., and Lemaire, G. (1999). Relationships between dynamics of nitrogen uptake and dry matter accumulation in maize crops. Determination of critical N concentration. Plant Soil 216, 65–82. doi: 10.1023/A:1004783431055
Rauhe, K. (1987). “Effects of organic manuring and cropping on soil humus and fertility,” in Proceedings of the 4th International CIEC Symposium Agricultural Waste Management and Environmental Protection (Braunschweig), 11–14.
Reinhold, G., König, V., and Herold, L. (2004). “Auswirkungen der Biogaserzeugung auf die Eigenschaften der Gärsubstrate,” in Proceedings 116. VDLUFA-Kongress (Rostock), 13–17.
Rippel, R. (2008). Umweltwirkungen eines zunehmenden Energiepflanzenanbaus. Bayerische Landesanstalt für Landwirtschaft, Institut für Agrarökologie, Ökologischen Landbau und Bodenschutz Freising. Available online at: https://www.lfl.bayern.de/mam/cms07/publikationen/daten/schriftenreihe/p_33149.pdf (Accessed Jan 30, 2018).
Risberg, K. (2015). Quality and Function of Anaerobic Digestion Residues. Doctoral thesis, Uppsala: Swedish University of Agricultural Sciences.
Sarker, J. R., Singh, B. P., Dougherty, W. J., Fang, Y., Badgery, W., Hoyle, F. C., et al. (2018). Impact of agricultural management practices on the nutrient supply potential of soil organic matter under long-term farming systems. Soil Tillage Res. 175, 71–81. doi: 10.1016/j.still.2017.08.005
Sieling, K., Herrmann, A., Wienforth, B., Taube, F., Ohl, S., Hartung, E., et al. (2013). Biogas cropping systems: short term response of yield performance and N use efficiency to biogas residue application. Eur.J. Agron. 47, 44–54. doi: 10.1016/j.eja.2013.01.002
Sticksel, E. (2010). Wintergetreide zur Erzeugung von Ganzpflanzensilage als Biogassubstrat. Bayerische Landesanstalt für Landwirtschaft, Institut für Pflanzenbau und Pflanzenzüchtung. Freising, Germany. Available online at: https://www.lfl.bayern.de/mam/cms07/ipz/dateien/leitfaden_2010-02_biogasforum.pdf (Accessed Jan 26, 2018).
Svensson, K., Odlare, M., and Pell, M. (2004). The fertilizing effect of compost and biogas residues from source separated household waste. J. Agric. Sci. 142, 461–467. doi: 10.1017/S0021859604004514
Svoboda, N., Taube, F., Klu,ß, C., Wienforth, B., Kage, H., Ohl, S., et al. (2013). Crop production for biogas and water protection—A trade-off? Agric. Ecosyst. Environ. 177, 36–47. doi: 10.1016/j.agee.2013.05.024
Tambone, F., Genevini, P., D'Imporzano, G., and Adani, F. (2009). Assessing amendment properties of digestate by studying the organic matter composition and the degree of biological stability during the anaerobic digestion of the organic fraction of MSW. Bioresour. Technol. 100, 3140–3142. doi: 10.1016/j.biortech.2009.02.012
Taube, F., and Herrmann, A. (2009). Relative benefit of maize and grass under conditions of climatic change. Landbauforsch. Agric. For. Res. Sonderh. 331, 115–126.
Vaneeckhaute, C., Lebuf, V., Michels, E., Belia, E., Vanrolleghem, P. A., Tack Filip, M. G., et al. (2017). Nutrient recovery from digestate: systematic technology review and product classification. Waste Biomass Valor 8, 21–40. doi: 10.1007/s12649-016-9642-x
Van Kessel, J. S., and Reeves, J. B. (2000). On-farm quick tests for estimating nitrogen in manure. J. Dairy Sci. 1837–1844. doi: 10.3168/jds.S0022-0302(00)75054-5
von Cossel, M., Möhring, J., Kiesel, A., and Lewandowski, I. (2017). Methane yield performance of amaranth (Amaranthus hypochondriacus L.) and its suitability for legume intercropping in comparison to maize (Zea mays L.). Ind. Crops Prod. 103, 107–121. doi: 10.1016/j.indcrop.2017.03.047
Keywords: biogas digestates, fertilization, cropping systems, bioenergy, alternative biogas substrates, nutrient cycles
Citation: Ehmann A, Thumm U and Lewandowski I (2018) Fertilizing Potential of Separated Biogas Digestates in Annual and Perennial Biomass Production Systems. Front. Sustain. Food Syst. 2:12. doi: 10.3389/fsufs.2018.00012
Received: 31 January 2018; Accepted: 06 April 2018;
Published: 24 April 2018.
Edited by:
Tom Misselbrook, Rothamsted Research (BBSRC), United KingdomReviewed by:
M. Angeles de la Rubia, Universidad Autonoma de Madrid, SpainLaura Zavattaro, Università degli Studi di Torino, Italy
Copyright © 2018 Ehmann, Thumm and Lewandowski. This is an open-access article distributed under the terms of the Creative Commons Attribution License (CC BY). The use, distribution or reproduction in other forums is permitted, provided the original author(s) and the copyright owner are credited and that the original publication in this journal is cited, in accordance with accepted academic practice. No use, distribution or reproduction is permitted which does not comply with these terms.
*Correspondence: Andrea Ehmann, a.ehmann@uni-hohenheim.de