- Coastal Plains Soil, Water, and Plant Research Center (USDA-ARS), Florence, SC, United States
To maintain the waste treatment capacity of anaerobic swine lagoons, excessive accumulation of sludge in the bottom of the lagoon is traditionally removed by dredging and pumping followed by dewatering prior to land application. A widespread and low-cost method of dewatering this lagoon sludge in U.S. farms is the use of geotextile bag filtration. Because of typically high phosphorus (P) contents in lagoon sludge, the dewatered sludge is eventually hauled to distant fields to reduce the environmental risk of excess P in land nearby the lagoon. The inclusion of a P recovery approach, called Quick Wash (QW), along with the geotextile bag technology could eliminate or reduce hauling costs of dewatered sludge and produce a valuable P product for use as fertilizer. The QW process uses a novel combination of acid, base, and organic polyelectrolytes to selectively extract and recover P from manure solids. The objective of this study was to evaluate the potential advantage and technical feasibility of combining the QW process and geotextile dewatering in a system to extract and recover P from lagoon sludge. Laboratory tests results showed that the amount of recoverable P from lagoon sludge depends on the pH level obtained by acidification. The highest release of P in solution, 83% of total initial P, was obtained when the lagoon sludge was acidified to pH 3. The amount of P recovered as a precipitate with lime addition at pH 10 was about 79% of the initial total P in the sludge. A mass flow balance confirmed that about 20% of the total P remained in the dewatered sludge solids. The effluent contained extremely low soluble P concentrations. The calcium phosphate product was identified as amorphous calcium phosphate (ACP) with very low heavy metal content. The recovered ACP had P grades (33.2–35.5% P2O5), higher than rock phosphate, with the advantage that there is no need for additional chemical processing for its use as fertilizer. Results of this study support the technical feasibility of using the QW process in combination with geotextile bag filtration for the economic recovery of P from anaerobic swine lagoons.
Introduction
With a total inventory of 8.9 million heads, North Carolina is the second largest swine (Sus scrofa L.) producer in the United States (NASS, 2018). This intensive swine production generates large amounts of manure which is typically treated in anaerobic lagoons (Barker, 1996). One challenging aspect of managing anaerobic swine lagoons is the accumulation of sludge in the bottom of the lagoon. Eventually, the excessive accumulation of sludge reduces the liquid storage volume of the lagoon and the ability of the lagoon to treat waste (Hamilton, 2010). As part of the lagoon management, excess lagoon sludge is removed by pumping after mechanical agitation or dredging. Once the sludge is removed from the lagoon, it is land applied at agronomic rates according to its nutrient content (Schmidt, 2013). However, nutrient management plans may limit P application to crop or pastureland near the lagoon because lagoon sludge usually has a high phosphorus (P) content. Indeed, more than 90% of the total mass of P in lagoons is found concentrated in the solid fraction of the sludge (Bicudo et al., 1999; Szogi and Vanotti, 2016). Therefore, to reduce the risks of excess soil P losses via leaching and runoff into water resources, the dewatering of lagoon sludge is a critical step to facilitate long distance transportation of sludge and offset local soil P imbalances. In North Carolina's swine farms, a low-cost and widespread method of dewatering lagoon sludge is the use of geotextile bag filtration with injection of a flocculant polymer (Spearman, 2017). The polymer is added to increase retention of the small particulate solids by the dewatering bag. The dewatered sludge collected in the geotextile bags is eventually hauled to distant locations for land application. The inclusion of a P recovery approach along with the geotextile bag technology could: (1) eliminate or reduce hauling costs of dewatered sludge, and (2) produce a valuable concentrated P product for use as fertilizer. Since P is a finite mining resource being depleted due to its global demand for agricultural production (Gilbert, 2009; Karunanithi et al., 2015), P recovery is essential to close the P loop in production agriculture and reduce the P pollution risks due to soil leaching and runoff P losses into aquatic environments (Keyzer, 2010; Szogi et al., 2015b; Jurgilevich et al., 2016).
A number of technologies for P recovery are already operational at full-scale in industrial, municipal, and agricultural treatment systems (Karunanithi et al., 2015; Kataki et al., 2016). Most technologies have the purpose to recover phosphate at various points in the treatment process from liquid phase, sludge, or sludge ash (Cieślik and Konieczka, 2017). Technologies for phosphate recovery from the liquid phase consist of feeding P-rich wastewater into a precipitation or crystallization tank, in which calcium (Ca) or magnesium (Mg) salts are added to recover phosphate as Ca phosphate or struvite (Desmidt et al., 2015). Technologies for chemical recovery of P from municipal sludge, biosolids, and incinerator ashes were reported to extract P by acid leaching or washing with recovery of P as a Ca or Mg precipitate (Takahashi et al., 2001; Franz, 2008; Cieślik and Konieczka, 2017). For animal waste, combined acidification and precipitation processes were reported for recovery of P from incinerated poultry litter (Kaikake et al., 2009). A novel chemical P recovery process using a combination of acid, base, and organic polyelectrolytes called “Quick Wash” (QW) was used to selectively extract and recover P from both raw poultry litter and fresh swine manure solids (Szogi et al., 2008, 2015a). The QW is a patented treatment process (U.S. Patent 8,673,046; first filed Feb. 5, 2008) developed for rapid acid wet extraction of P from solid manure and P recovery in a solid concentrated form (Szogi et al., 2014).
The inclusion of the QW process in a lagoon sludge management plan offers an opportunity to recover P as a valuable fertilizer byproduct. The objective of this study was to evaluate the potential advantage and technical feasibility of combining the QW process with a geotextile dewatering technology to extract and recover P from lagoon sludge. In order to provide process data to operate a field scale system, laboratory experiments were performed to determine: (1) acid rates for optimal release of soluble P from swine lagoon sludge; (2) mesh size for effective dewatering of acidified sludge with geotextile bags; and (3) P recovery efficiency. In addition, the study included elemental composition of the products (washed sludge solids and recovered phosphate) and a mass flow balance.
Materials and Methods
Process Description
Figure 1 show first the individual technologies: basic QW process (Figure 1A) and geotextile bag dewatering (Figure 1B), and their combination in one single system, hereafter called “QW retrofit” (Figure 1C). The basic QW process includes three steps (Szogi et al., 2014): (1) selective P extraction, (2) P recovery, and (3) P recovery enhancement (Figure 1A). In the first step, manure solids or slurry are mixed with an acidic solution to form a washed solid residue and a liquid P extract. In step 2, P is precipitated under alkaline conditions by adding calcium (Ca) or magnesium (Mg) to the liquid extract, and in the final step (step 3) the addition of an organic anionic polymer, polyacrylamide (PAM), enhances the formation and recovery of a P-rich precipitate. In the geotextile bag filtration system alone (Figure 1B), polymer (cationic PAM) is injected to enhance filtration and dewatering of solids retained in the geotextile bag, producing an effluent low in solids. In the QW retrofit (Figure 1C), the sludge is first acidified and then cationic PAM is injected to flocculate and retain solids in the geotextile bag (QW step 1). The acidic liquid flowing out of the geotextile bag is then collected in a settling tank to recover the P precipitate after reaction with hydrated lime and anionic PAM (QW steps 2 and 3). The P is recovered as a precipitate from the bottom of the settling tank and further dewatered. The effluent from the settling tank, low in solids and P, is then sent to the lagoon or eventually recycled as dilution water.
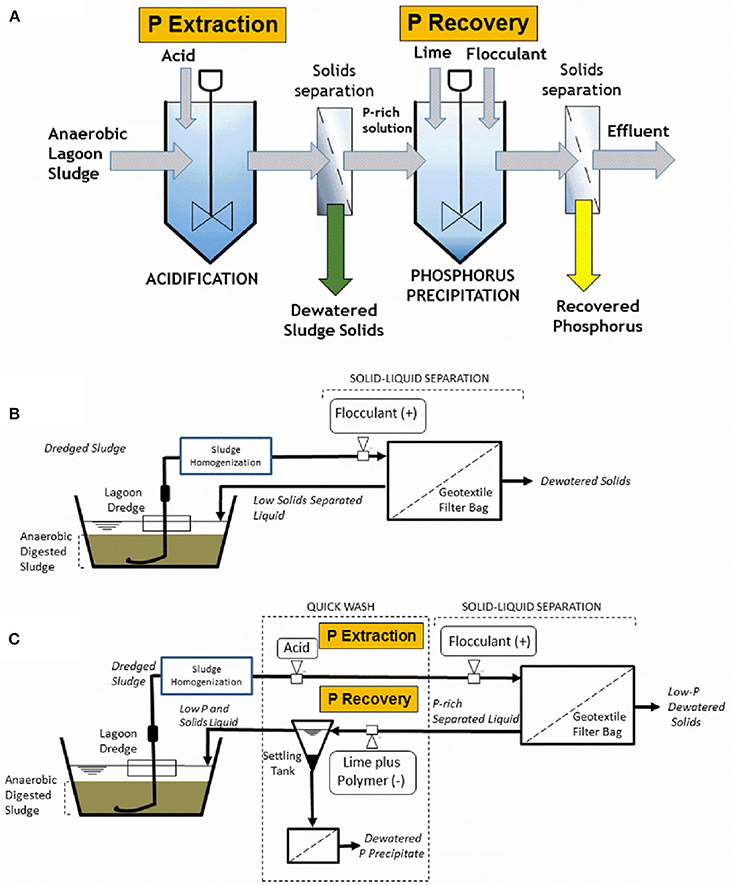
Figure 1. Schematic diagrams of the basic Quick Wash process (A), the geotextile bag filtration for dewatering of sludge (B), and the combination of the Quick Wash process and the geotextile bag filtration into one system for P recovery from sludge (C).
Phosphorus Extraction (Experiment 1)
The P extraction was conducted by filling 20 mL of lagoon sludge into 50-mL centrifuge tubes. Sulfuric acid (4.1 M) was added to the tubes at 10 rates ranging from 20 to 200 mmol L−1 (equivalent to a concentrated acid rate of 2.1–20.8 g H2SO4 L−1, 18M, 1.84 g cm−3) plus one tube with no addition of acid (control). The tubes with the mixture of sludge and acid were capped and shaken on a reciprocating shaker (135 oscillations min−1) for 20 min at ambient temperature (23°C). Subsequently, the tubes were uncapped and pH was measured using a pH meter (Denver Instruments, Arvada, CO). After measuring the pH, the acid-sludge mixtures were filtered through 0.2-μm filters. The collected clear filtrate was used for analysis of soluble inorganic P. The extraction experiment was carried out in duplicate.
Dewatering of Washed Solids (Experiment 2)
A filtration test was used to determine the effect of acidification and mesh size of geotextile bag on sludge dewatering. A preliminary flocculation study was performed to determine the PAM rate (WERF, 1993). The polymer was a cationic PAM (0.05% solution) added at a single rate of 133 mg PAM L−1 which was within the optimum rate of 100–200 mg L−1 to flocculate swine manure slurries (Vanotti and Hunt, 1999). Prior to the filtration test, a set of 150-mL samples were previously acidified to pH 5.0, 4.0, and 3.0 with H2SO4 and conditioned with cationic PAM. Duplicate gravity filtration tests were carried out through 250-, 400-, and 600-μm mesh monofilament nylon filters (NMO, Krystil Klear Filtration, IN). The selected mesh size of the filters were in the range of opening sizes in geotextiles tubes used for dewatering manure and sludges (Szogi et al., 2006; Cantrell et al., 2008; Worley et al., 2008). Total suspended solids (TSS) were determined in the filtrates, and their corresponding moisture content. The solid-liquid separation removal performance for every filter mesh was determined by the difference between the TSS concentration in the filtrate and the TSS in the original sample before acidification and PAM applications and filtration.
Phosphorus Precipitation and Recovery (Experiment 3)
Based on the results of the acidification and dewatering experiments, a 1.0-L sample of sludge was stirred for 20 min using an over-head mixer while adding H2SO4 to reach pH 3.0 and then conditioned with cationic PAM. The acidified sludge was gravity filtered through a 400-μm mesh filter. The separated washed solids retained in the filter were rinsed with distilled water and freeze dried prior to elemental analysis. The filtrate (acidified extract) was used in P precipitation experiments. The same filtration procedures were used to obtain acidified extracts at pH 4.0 and 5.0. From each acid extract, a set of 35-mL aliquots were placed in 50-mL centrifuge tubes and hydrated lime [10% w/v Ca(OH)2] was added to pH endpoints of 8.0, 9.0, and 10.0 units. To facilitate the dewatering and recovery of P, an anionic PAM (0.05% solution) was added at a single rate of about 0.4 mg L−1 to thicken the precipitated material (Szogi et al., 2015a). Subsequently, the samples were centrifuged at 2,000 g for 10 min. The supernatant was poured from the centrifuge tubes into plastic vials and immediately analyzed. The P precipitates were washed with known volumes of de-ionized distilled water, centrifuged again at 2,000 g for 10 min, and decanted. The recovered P precipitates were freeze-dried prior to analysis.
Chemical Analysis
Sludge analyses and effluents from the QW process were performed according to Standard Methods for Examination of Water and Wastewater (APHA, 1998). The pH was determined electrometrically (Method 4500-H+ B). Alkalinity was determined by acid titration to the bromocresol green endpoint (pH 4.5) and expressed as mg CaCO3 L−1 (Method 2320 B). TSS were determined according to Method 2540E. Total Kjeldahl nitrogen (TKN) was determined using acid digestion and the automated ascorbic acid and phenate methods adapted to digested extracts (Technicon Instruments Corp, 1977). Concentrations of total phosphorus (TP), and metals were determined in liquid and solids using nitric acid/peroxide block digestion (Peters, 2003) and inductively coupled plasma analysis (Method 3125 A). Ammonium-nitrogen (NH4-N), nitrate-nitrogen (NO3-N) and soluble P (PO4-P) were determined by chemically suppressed ion chromatography using ASTM Standards D6919-09 and D4327-11 (ASTM International, 2009, 2011) after filtration through a 0.20-μm membrane filter.
Both washed sludge solids and P recovered precipitates were analyzed for carbon (C) and N by dry combustion (Elementar, Mt. Laurel, NJ). Minerals in the P recovered precipitates were identified by X-ray powder diffraction using an Olympus Terra (Waltham, MA) field-portable X-ray diffractometer (XRD) system operating at 30 kV and 0.3 mA with a Cu radiation tube. Data were collected from 5 to 55° 2θ in steps of 0.05° 2θ for qualitative XRD analysis including standards of amorphous calcium phosphate (ACP) and calcium carbonate (CaCO3) supplied by Sigma-Aldrich (St. Louis, MO). In addition, carbonates in the recovered P precipitates were determined according to (Horváth et al., 2005).
Extraction and recovery efficiencies of P were determined using mass balances that included the sludge volume and P concentrations before and after treatment as well as the mass and concentration of P in precipitated materials. Data results were statistically analyzed by mean and standard deviation using PROC MEANS procedure in SAS (Statistical Analysis System, Cary, NC). Analysis of variance for the effect of acid extraction treatment on composition of washed sludge solids, lime addition treatments to acid extract on recovered P material and effluents was conducted using the General Linear Model (GLM) in SAS. Difference between means of any two treatments was considered significant when it was larger than the least square difference (LSD) with a probability of t < 0.05.
Origin and Characteristics of the Lagoon Sludge
The sludge was collected during the course of dredging an anaerobic swine lagoon used for storing and treating manure in a swine finishing farm located in North Carolina, USA. A sample of the dredged sludge was pumped into a 20-L plastic container, transported to the USDA-ARS laboratory in Florence, and stored at 4°C until use. The sludge was homogenized using a mechanical stirrer before taking samples for the experiments. On average, the sludge contained pH 7.65, alkalinity 11,290 mg L−1, TSS 57.5 g L−1, TKN 2,513 mg L−1, NH4-N 323 mg L−1, NO3-N 0 mg L−1, K 606 mg L−1, TP 4,070 mg L−1, PO4-P 82 mg L−1, Ca 5,553 mg L−1, Mg 1,278 mg L−1, Al 216 mg L−1, Fe 511 mg L−1, Cu 24 mg L−1, and Zn 179 mg L−1.
Results
Phosphorus Extraction
Previous research on applying mineral acid to poultry litter and fresh swine manure has shown that over 60% of the initial TP was released into solution between pH 5.0 and 3.0 (Szogi et al., 2008, 2015a). At pH lower than 3.0, the acid extract becomes corrosive, and unnecessary oxidation of organic compounds can occur (Szogi et al., 2015a). In this study, the acid requirement to decrease the pH of the sludge and increase release of P from the solid fraction into a soluble fraction is shown in Figure 2A. Prior to acid addition, at pH 7.7, the soluble P concentration was 99 mg PO4-P representing only about 2% of the initial 4,070 TP mg L−1 in the sludge. With addition of the acid, the pH declined with a significant increase of soluble PO4-P concentration in the acid extract. Since the concentration of soluble PO4-P strongly depends on the acid dose, Figure 2B shows the percent of PO4-P extracted with respect to the initial TP at increasing acid dose (H2SO4). At pH 2.8, the acid extract had a concentration of 3,793 mg L−1 of PO4-P (Figure 2A) or 93% of the TP that was in the original sludge (Figure 2B).
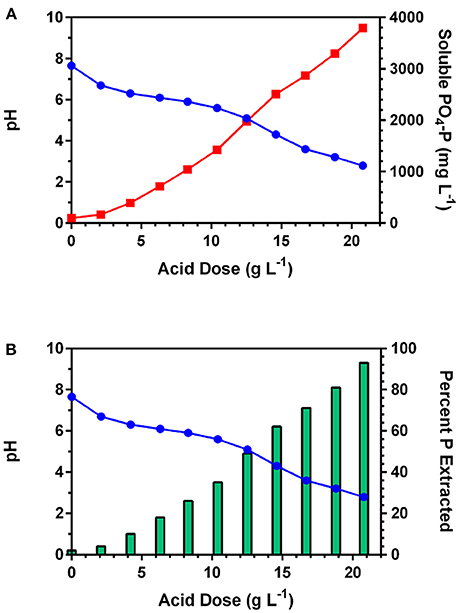
Figure 2. Phosphorus extraction from anaerobic lagoon sludge at increasing concentration levels of sulfuric acid: (A) effect of decreasing pH (blue line) on soluble P concentration (red line); and (B) percent soluble P with respect to initial total P in the anaerobic lagoon sludge (bers).
Dewatering of Acidified Sludge
Dewatering technologies use solid-liquid separation methods to separate the liquid and solid fractions of sludge by gravity, mechanical, and chemical processes. Usually, dewatering efficiencies of mechanical or gravity separators are <60% for removal of solids. Whereas chemical applications of flocculants have been shown to enhance the dewatering efficiency to more than 90% solids removal for liquid manure (Vanotti and Hunt, 1999; Garcia et al., 2009). Furthermore, Cocolo et al. (2016) reported that acidification of pig manure slurry to pH 5.3 followed by cationic polymer addition promoted a faster dewatering of solids than a non-acidified control. In our study, we observed that the addition of acid and cationic PAM to lagoon sludge quickly produced firm, large flocs with rapid settling, and a clear supernatant liquid. The effects of mesh size and pH on TSS concentration in the filtrate and filtration efficiency of sludge conditioned with PAM are presented in Table 1. At the same acidification treatment (pH 5.0, 4.0, or 3.0), the filter with 250 μm mesh size produced filtrates with the lowest TSS concentrations when compared to filtrates obtained with 400 and 600 μm mesh size. Nevertheless, all filtration tests had a high efficiency of >98% retention of solids, and the separated solids had a narrow range moisture content of 86–89%. From these results we concluded that commercially available geotextile filtration bags with a 400-μm openings could be used effectively for solid-liquid separation of acidified sludge conditioned with cationic PAM. The results reported for P recovery in the next section were obtained using the filtrates of the acidified sludge passed through the selected 400-μm mesh filter.
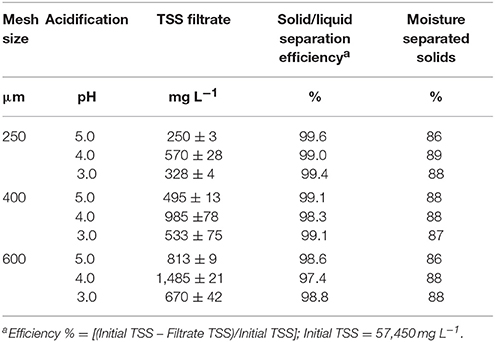
Table 1. Effect of mesh size and acidification of anaerobic lagoon sludge conditioned with cationic polymer on filtrate quality, solid/liquid separation efficiency and moisture of solids (Experiment 2).
P Recovery and Mass Flow Balance
In the QW process, the recovery of P can be done either by addition of either Mg or Ca salts to form a phosphate precipitate under alkaline pH conditions (Szogi et al., 2014). Hao et al. (2013), indicated that efforts for recovery of P from waste streams should be aimed at forms of phosphate compounds acceptable by the fertilizer industry such as Ca phosphates. The potential use of recovered Ca phosphates from waste streams by the fertilizer industry led us to develop the QW process for recovery of Ca phosphates from various waste streams: poultry litter, manure solids (Szogi et al., 2008, 2015a), and lagoon sludge solids presented here. The laboratory results on P recovery and mass flow balances for the QW retrofit are presented in Table 2. The mass results are shown separately for the first step and second step of the QW process. The extraction of P (Step 1) was based on the acid extracts obtained by filtration through a 400-μm mesh of acidified sludge conditioned with cationic PAM. The P recovery (Steps 2 and 3) includes the results of the combined additions of lime and anionic PAM to enhance P precipitation from the acid extracts (Figures 1A,C).
In Table 2, the acid P extraction (Step 1) shows that with decreasing extraction pH from 5.0 to 3.0 there was an increase in soluble P from 2,022 to 3,398 mg L−1, respectively. On a mass basis, from 50, 67, and 83% of the initial TP mass was in solution in the acid extracts at pH 5.0, 4.0, and 3.0, respectively. Following, the P recovery (steps 2 and 3) section of Table 2 shows the pH increase in three steps (pH 8.0, 9.0, and 10.0) by addition of hydrated lime to each of the three acid P extracts, resulting in nine doses of applied lime. Hydrated lime addition was effective to remove large amounts P from each acid extract by precipitating P. Overall, the amount of recovered P in the precipitates was strongly predisposed by the concentration of soluble P in the acid extracts. For instance, the highest concentration of soluble P was obtained at pH 3.0; subsequent addition of hydrate lime to a target pH of 10.0 removed 3,219 mg P L−1 from the extract allowing the recovery of 79% of the initial mass of TP in the sludge. The removal of P from all acid extracts resulted in low TP concentrations in the effluents and represented <1% of the initial mass of TP in the sludge. Figure 3 summarizes the mass flows of solids and P for all three steps of the QW retrofit system when lagoon sludge was acidified at pH 3.0 and P recovered at pH 10.0. While 99% of the mass of TSS was retained in the filter as washed solids, the P mass split in two fractions: (1) 21% of P was retained in the filter with the washed solids (first split); and (2) 79% of the P was recovered as a precipitate. Overall, <1% of the mass of P was in the treated effluent.
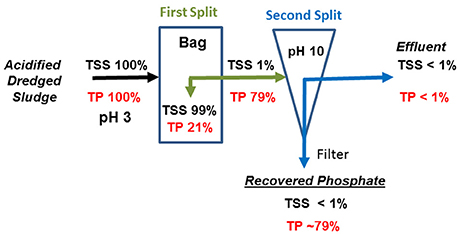
Figure 3. Mass flow balance of total P (TP) and solids (TSS) through the Quick Wash-geotextile bag filtration system.
Washed Solids
The original composition of the solid fraction of the sludge was significantly influenced by the acidification step of the QW process (Table 3). A significant increase in C, N, and S with a significant decrease in P concentration occurred in all acid washed solid residues when compared to the untreated sludge solids. The difference in P content were all highly significant between the untreated sludge solids and each of the wash solids after acid treatment. At pH 3, the P concentration in the wash solid was 4.5-fold smaller (15.8 g kg−1) than the untreated sludge (70.8 g kg−1). This result is consistent with the mass balance in Figure 3 that about 79% of the P was removed from sludge solids allowing the subsequent recovery and off-farm export of P. The selective acid dissolution of P with respect N is represented by the increase in the original N:P ratio of the sludge before treatment from 0.33 to 2.3 after acidification at pH 3. Since P in the sludge solids was mostly in inorganic form, the release of soluble P under acidic conditions was accompanied by significant dissolution of K, Ca, Mg, and Na. It is important to notice the acidification step increased the concentration of heavy metals Cd, Cu, Ni, Pb, and Zn in the washed solids. Heavy metals remained in the washed solids most likely because effective dissolution of these elements requires stronger acidic conditions at pH levels lower than 2.0 (Scott and Horlings, 1975). However, the heavy metals concentrations in the acid washed solids were low enough to meet the U.S. EPA regulatory ceiling concentration limits of 85 mg Cd kg−1, 4,300 mg Cu kg−1, 420 mg Ni kg−1, 840 mg Pb kg−1, and 7,500 mg Zn kg−1 for land application of biosolids (Metcalf Eddy Inc., 2003).
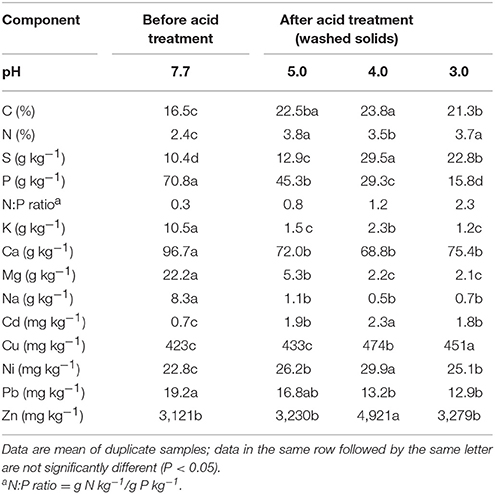
Table 3. Chemical composition of anaerobic swine lagoon sludge solids before chemical extraction of phosphorus (no-acid), and after acid extraction treatment (washed solids) on a dry basis.
Recovered Phosphates
The chemical composition of selected P precipitates are presented in Table 4. They were obtained at pH 8.0, 9.0, and 10.0 after hydrated lime addition to acidified extracts (pH 3.0). On a dry matter basis, differences in elemental composition of the precipitates were not significant across the three hydrated lime treatments with the exception of N and K concentrations. The total C content in the precipitates (1.4–1.5%) was low. It was partitioned into inorganic C (0.5–0.9%) and organic C (0.6–0.9%) forms. The organic C content was estimated by the difference in released CO2 from total combusted C and carbonate measurements (2.3–4.3% as ). Only 2.3–5.7% of the initial N on a mass basis was recovered such that N concentrations (0.2–0.4 N%) in the P precipitates were low as compared to the initial lagoon sludge (2.4% N, Table 3). The recovered K, S, and Na were minor components in the precipitates since they remained mostly in solution after lime addition. Both P and Ca were the major components of the phosphate precipitates, whereas Mg was a third component with concentrations much lower than Ca (Table 4). The recovered Ca phosphates had mean P contents within the range of 33.2–35.5% P2O5. On average, they were higher than rock phosphate from U.S. mines in North Carolina (29.8% P2O5) and Central Florida (32.5% P2O5) (FAO, 2004). The only two heavy metals detected in the recovered phosphate precipitates were Ni and Zn (Table 4), with concentrations of both heavy metals below 13 mg Ni kg−1 and 74 mg Zn kg−1 found in commercial P fertilizers (USEPA, 1999).
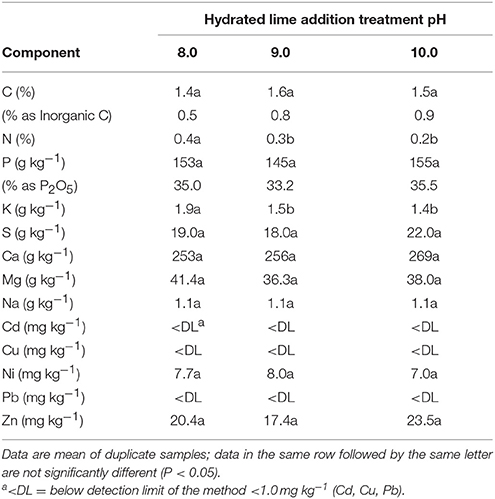
Table 4. Chemical composition of the recovered phosphorus precipitates obtained from anaerobic swine lagoon sludge acid extract (pH 3) after lime addition to precipitate phosphorus at pH to 8.0, 9.0, and 10.0.
Several Ca-Mg phosphate minerals such as hydroxylapatite and struvite can co-exist in crystalline form contributing to phosphate recovered from waste streams (Zhang et al., 2010; Muster et al., 2013). However, our XRD analysis revealed that the presence of small peaks in all recovered P precipitate samples did not correlate with any known crystalline phosphate species (Figures 4A–C). For comparison, XRD analysis of standards of ACP and CaCO3 as calcite were included in Figures 4D,E. Since the content of carbonates in the precipitates was in the low range of 2.3–4.3%, the intense characteristic peak (2θ = 30°) of calcite is not shown clearly in the XRD spectra of the recovered P precipitates. Magnesium had low concentration values but its consistent presence in the elemental composition of recovered P precipitates, hints that Mg was a component of the ACP as reported by Fernandes et al. (2012). However, Mg could be in the form of struvite which requires presence of three soluble ions (Mg2+, , and ). The precipitation of struvite (MgNH4PO4.6H2O) is favored by raising the pH above 8.0 and Mg:P molar ratios between 1:1 and 1:3 (Muster et al., 2013). Although the three soluble ions required for struvite were in the acid extract at pH 3 (Table 5), the Mg:P molar ratio of 0.6:1 was not the most favorable for struvite precipitation. In addition, the presence of Ca in solution was reported to inhibit the crystal growth of struvite, leading to an amorphous material rather than crystalline struvite (Le Corre et al., 2005). In our study, the pH of the acid extract was raised with hydrated lime resulting in supersaturation of Ca at pH 8.0–10.0, and most probably inhibiting the crystal growth of struvite. On the other hand, small amounts of Mg were reported to be essential for the non-crystalline ACP to become thermodynamically stable at room temperature (Posner et al., 1984). Due to its amorphous nature, ACP does not have a fixed molecular formula. Therefore, ACP precipitates are distinguished only by their wide range of Ca/P molecular ratios (1.0–2.2); lower Ca/P ratios indicate higher contents of phosphate (Dorozhkin, 2010). From Table 4, the Ca/P ratios were estimated from the total Ca concentration by subtracting the Ca in the carbonates. Under this assumption, the Ca/P ratio of the P precipitates obtained at pH 8.0, 9.0, and 10.0 were 1.23, 1.28, and 1.25, respectively. These ratios are consistent with the lower range of Ca/P ratio for ACP and the high grade phosphate (33.2–35.5% P2O5) of the recovered P precipitates (Table 4). From the XRD spectra and chemical analysis results we concluded that the recovered phosphates were similar in composition to ACP.
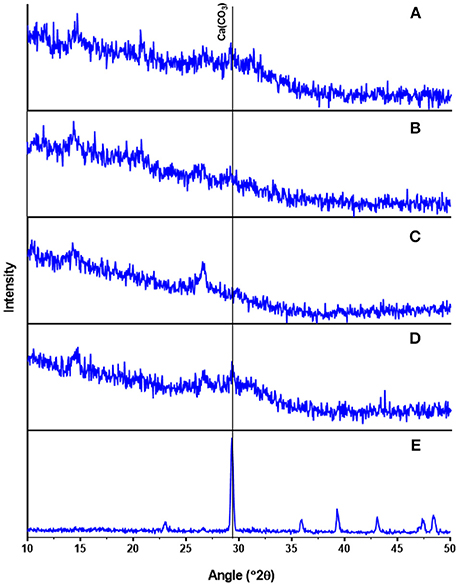
Figure 4. Mineralogical characterization (x-ray diffraction) of precipitates obtained after lime addition to liquid extract of swine lagoon sludge (pH 3): (A) pH 8 precipitate; (B) pH 9 precipitate; (C) pH 10 precipitate; (D) amorphous calcium phosphate (ACP) standard; (E) calcium carbonate (CaCO3) standard.
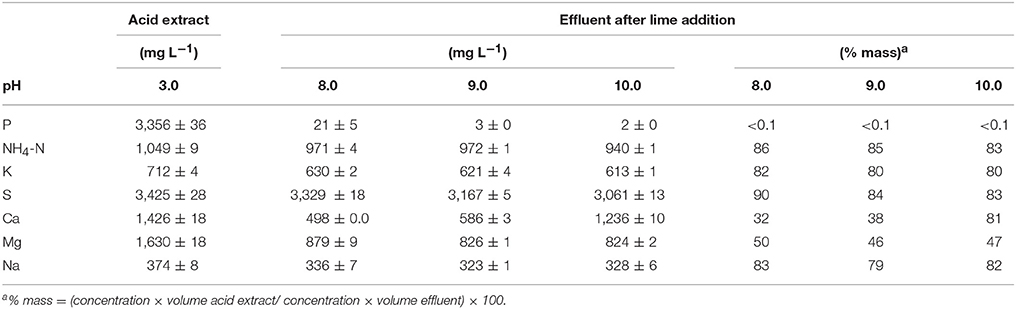
Table 5. Chemical composition of the liquid acid extract at pH 3, the effluent obtained after lime addition to the acid extract at pH of 8, 9, and 10 and settling, and mass percentage of each constituent remaining in the effluent with respect to its mass in the acid extract.
Effluent
Very low TP concentrations (2–21 mg L−1) with respect to the initial acid extract (3,356 mg L−1) were found in the final effluent (Table 5). As a result of lime addition to the acid extract and subsequent formation of P precipitates, the P removal efficiency was about 100% with <0.1% P mass remaining in the final effluent. Although, NH4-N concentrations remained high in the final effluent, they declined with respect to the initial acid extract (1,049 mgL−1) at the increasing alkaline pH (971–940 mg L−1). On a mass basis, the NH4-N in the effluent was 83–86% of the initial NH4-N mass in the acid extract at pH 3. The decline of NH4-N in the effluent was likely due to NH3 losses by volatilization under the alkaline pH of the effluent. In spite of the alkaline pH, K, and Na remained in solution in sufficient high concentrations such that over 79% of the total initial mass of K and Na turn out in the effluents. Similarly, 83–90% of S mass remained in solution in the final effluents since it was added with the H2SO4 to acidify the sludge. On the other hand, the concentration and mass of Ca remaining in the final effluent increased from 32 to 97% with increasing alkaline pH due to addition of hydrated lime whereas the mass of Mg was about half (46–50%) of what was initially in the acid extract. The very low TP concentrations suggests that these effluents can be recycled into the QW retrofit for use as dilution water during acid mixing with the dredged sludge. At, the same time, this recycling of the effluent could help to reduce NH3 emissions. As an alternative to minimize gaseous NH3 losses according to Vanotti and Szogi (2015), a gas-permeable membrane system can be included in the P recovery step (settling tank, Figure 1C) of the QW retrofit to capture and recover NH4-N from the effluents in a concentrated form.
Economic Considerations
The cost to recover P from lagoon sludge using the QW retrofit was calculated on the basis of processing one m3 of sludge. Geotextile filtration bags are available commercially in 9- to 25-m circumference and practically any length, but typically 30.5-m (Worley et al., 2003). We assumed that the QW retrofit would use a 14-m circumference by 30.5-m length geotextile filtration bag with 425-μm mesh and a capacity to treat 1,500 m3 of sludge. This is a volume of sludge estimated to be generated by 3,000 pigs in 5 years according to a sludge generation standard for North Carolina's swine lagoons of 0.125 m3 per animal of 57 kg per year (33 gallons per animal of 125 libs per year) reported by Sheffield et al. (2000). Treatment parameters used in calculating the processing cost are based on our laboratory study with the following conditions:
• Dredged sludge contains 4.1 g P L−1 or 9.4 kg P2O5 m−3.
• The solid/liquid volume fraction is 0.506, obtained from data of our sludge filtration study.
• The P recovery efficiency is 70%.
• The geotextile filtration bag cannot be reused once it is opened to remove the dewatered solids.
• The dewatered solids were applied on-farm.
The costs of chemicals are presented in Table 6 with a total cost of U$10,473 to treat 1,500 m3 of dredged sludge. Additional materials and labor costs include the geotextile filtration bag at US$ 2,500, the cost of site preparation (US$ 340), and 30 h of labor at US$ 30 h−1 (US$ 900). The resulting cost for treating 1,500 m3 of lagoon sludge would be U$14,213, which represents a cost of US$ 9.48 m−3 of processed lagoon sludge.
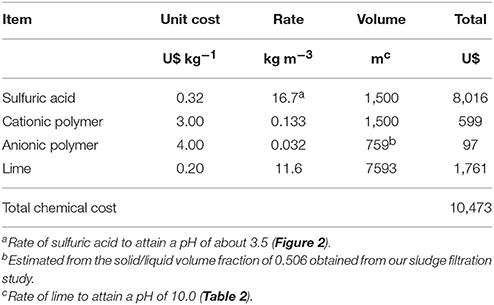
Table 6. Costs of chemicals for recovering P from 1,500 cubic meters of sludge using the Quick Wash—geotextile filtration system.
The amount of P potentially recovered in the precipitates is about 6.57 kg P2O5 m−3 of processed sludge with a fertilizer value of US$ 6.17 (US$ 0.94 kg−1 P2O5). In addition, the combined fertilizer value of both N and P remaining in the washed solids is about US$ 3.89 m−3 of processed sludge. A complete economic evaluation for deployment of this technology to recover P from lagoons per year still requires taking into account the annualized energy and capital or rental cost of the equipment (dredge, pumps, injection equipment, and tanks) and potential additional income from nutrient trading programs (Shortle, 2013).
Conclusions
This laboratory study showed that the amount of recoverable P from lagoon sludge depended on the acidic pH reached after application of sulfuric acid. The highest release of P in solution, 83% of total P, was obtained when the lagoon sludge was acidified to pH 3.0. The P released in the acidic extract was recovered as a calcium phosphate product using hydrated lime. The amount of P recovered at pH 10 was about 80% of the initial total P. The extremely low amounts of P in the effluent (2–3 mg L−1) and a mass flow balance confirmed that about 20% of the total initial P remained in the dewatered sludge solids. The calcium phosphate product was identified as ACP. The recovered ACP had higher P grades (33.2–35.5% P2O5) than rock phosphate and the advantages of low heavy metal content and no need for additional chemical process for use as fertilizers. Results of this study support the technical feasibility of using the QW process in combination with geotextile bag filtration for economic recovery of P from anaerobic swine lagoons. Further tests at pilot scale are needed to determine: (1) solid-liquid separation efficiency of geotextile bags for treatment of acidified sludge; (2) confirm laboratory P recovery efficiencies; and (3) automation of the process. In addition, field trials will be needed to demonstrate the agronomic value of both washed solids and recovered phosphate products.
Author Contributions
All authors listed have made a substantial, direct and intellectual contribution to the work, and approved it for publication.
Conflict of Interest Statement
The authors declare that the research was conducted in the absence of any commercial or financial relationships that could be construed as a potential conflict of interest.
Acknowledgments
This research was part of USDA-ARS National Program 212: Soil and Air; ARS Project 6082-12630-001-00D Improvement of Soil Management Practices and Manure Treatment/Handling Systems of the Southern Coastal Plain. Mention of trade names or commercial products in this article is solely for the purpose of providing specific information and does not imply recommendation or endorsement by the U.S. Department of Agriculture.
References
APHA (1998). Standard Methods for Examination of Water and Wastewater, 20th Edn. Washington, DC: American Public Health Association.
ASTM International (2009). Determination of Dissolved Alkali and Alkaline Earth Cations and Ammonium in Water and Wastewater by Ion Chromatography. West Conshohocken, PA: ASTM International; ASTM Standard D6919-09.
ASTM International (2011). Anions in Water by Suppressed Ion Chromatography. ASTM Standard D4327-11. West Conshohocken, PA: ASTM International.
Barker, J. C. (1996). Lagoon Design and Management for Livestock Waste Treatment and Storage. Raleigh, NC: North Carolina Cooperative Extension Service; Pub. EBAE 103-83.
Bicudo, J. R., Safley, L. M., and Westerman, P. W. (1999). Nutrient and sludge volumes in single-cell recycle anaerobic swine lagoons in North Carolina. Trans. ASAE 53, 529–536.
Cantrell, K. B., Chastain, J. P., and Moore, K. P. (2008). Geotextile filtration performance for lagoon sludges and liquid animal manures dewatering. Trans. ASABE 51, 1067–1076. doi: 10.13031/2013.24526
Cieślik, B., and Konieczka, P. (2017). A review of phosphorus recovery methods at various steps of wastewater treatment and sewage sludge management. The concept of “no solid waste generation” and analytical methods. J. Clean. Prod. 142, 1728–1740. doi: 10.1016/j.jclepro.2016.11.116
Cocolo, G., Hjorth, M., Zarebska, A., and Orivolo, G. (2016). Effect of acidification on solid-liquid separtion of pig slurry. Biosyst. Eng. 143, 20–27. doi: 10.1016/j.biosystemseng.2015.11.004
Desmidt, E., Ghyselbrecht, K., Zhang, Y., Pinoy, L., Van der Bruggen, B., Verstraete, W., et al. (2015). Global phosphorus scarcity and full-scale P-recovery techniques: a review. Crit. Rev. Environ. Sci. Technol. 45, 336–384. doi: 10.1080/10643389.2013.866531
Dorozhkin, S. (2010). Amorphous calcium (ortho)phosphates. Acta Biomater. 6, 4457–4475. doi: 10.1016/j.actbio.2010.06.031
FAO (2004). Use of Phosphate Rocks for Sustainable Agriculture. Rome: Food and Agriculture Organization - United Nations. Available online at: http://www.fao.org/docrep/007/y5053e/y5053e00.htm#Contents (Accessed April 20, 2018).
Fernandes, G. W., Kunz, A., Steinmetz, R. L. R., Szogi, A., Vanotti, M., Flores, E. M. M., et al. (2012). Chemical phosphorus removal: a clean strategy for piggery wastewater management in Brazil. Environ. Technol. 33, 1677–1683. doi: 10.1080/09593330.2011.642896
Franz, M. (2008). Phosphate fertilizer from sewage sludge ash (SSA). Waste Manage. 28, 1809–1818. doi: 10.1016/j.wasman.2007.08.011
Garcia, M. C., Szogi, A. A., and Vanotti, M. B. (2009). Enhanced solid-liquid separation of dairy manure with natural flocculants. Bioresour. Technol. 100, 5417–5423. doi: 10.1016/j.biortech.2008.11.012
Hamilton, D. W. (2010). Sludge accumulation in two anaerobic/facultative lagoons treating swine manure from breeding farms in Oklahoma. Trans. ASABE 42, 1087–1093. doi: 10.13031/2013.29567
Hao, A., Wang, C., Loosdrecht, M. C. M., and Hu, Y. (2013). Looking beyond struvite for P-recovery. Environ. Sci. Technol. 47, 4965–4966. doi: 10.1021/es401140s
Horváth, B., Opara-Nadi, O., and Beese, F. (2005). A simple method for measuring the carbonate content of soils. Soil Sci. Soc. Am. J. 69, 1066–1068. doi: 10.2136/sssaj2004.0010
Jurgilevich, A., Birge, T., Kentala-Lehtonen, J., Korhonen-Kurki, K., Pietikäinen, J., Saikku, L., et al. (2016). Transition towards circular economy in the food system. Sustainability 8:69. doi: 10.3390/su8010069
Kaikake, K., Sekito, T., and Dote, Y. (2009). Phosphate recovery from phosphorus-rich solution obtained from chicken manure incineration ash. Waste Manage. 29, 1084–1088. doi: 10.1016/j.wasman.2008.09.008
Karunanithi, R., Szogi, A. A., Bolan, N., Naidu, R., Loganathan, P., Hunt, P. G., et al. (2015). Phosphorus recovery and reuse from waste streams. Adv. Agron. 131, 173–250. doi: 10.1016/bs.agron.2014.12.005
Kataki, S., West, H., Clarke, M., and Baruah, D. C. (2016). Phosphorus recovery as struvite from farm, municipal and industrial waste: feedstock suitability, methods and pre-treatments. Waste Manage. 49, 437–454. doi: 10.1016/j.wasman.2016.01.003
Keyzer, M. (2010). Towards a closed phosphorus cycle. De Econ. 158, 411–425. doi: 10.1007/s10645-010-9150-5
Le Corre, K. S., Valsami-Jones, E., Hobbs, P., and Parsons, S. A. (2005). Impact of calcium on struvite crystal size, shape and purity. J. Cryst. Growth. 283, 514–522. doi: 10.1016/j.jcrysgro.2005.06.012
Metcalf and Eddy Inc. (2003). Wastewater Engineering. Treatment and Reuse, 4th Edn. New York, NY: McGraw Hill.
Muster, T. H., Douglas, G. B., Sherman, N., Seeber, A., Wright, N., and Güzükara, Y. (2013). Towards effective phosphorus recycling from wastewater: quantity and quality. Chemosphere 91, 676–684. doi: 10.1016/j.chemosphere.2013.01.057
NASS (2018). Quarterly Hogs and Pig Inventory. United States Department of Agriculrue – Agriculture Counts. Available online at: http://usda.mannlib.cornell.edu/MannUsda/viewDocumentInfo.do?documentID=1086
Peters, J. (2003). Recommended Methods of Manure Analysis (A3769). Madison, WI: University of Wisconsin-Madison.
Posner, A. S., Blumenthal, N. C., and Foster, B. (1984). “Chemistry and structure of precipitated hydroxyapatites,” in Phosphate Minerals, eds. J. O. Nriagu and P. B. Moore (Heidelberg; Berlin: Springer-Verlag), 330–350.
Schmidt, A. M. (2013). Sludge Management for Anaerobic Lagoons and Runoff Holding Ponds. UNL Extension Pub. G1371. Lincoln, NE: Institute of Agriculture and Natural Resources, University of Nebraska-Lincoln Extension.
Scott, D. S., and Horlings, H. (1975). Removal of phosphates and metals from sewage sludges. Environ. Sci. Technol. 9, 849–855. doi: 10.1021/es60107a011
Sheffield, R. E., Barker, J. C., and Shaffer, K. A. (2000). Sludge Management and Closure Procedures for Anaerobic Lagoons. North Carolina Cooperative Extension AG-604. Raleigh, NC: North Carolina State University.
Shortle, J. (2013). Economics and environmental markets: lessons from water-quality trading. Agr. Resour. Econ. Rev. 42, 57–54. doi: 10.1017/S1068280500007619
Spearman, B. (2017). Sludge Bags or Geotextiles Tubes to Dewater Sludge. North Carolina Cooperative Extension. Available online at: https://animalwaste.ces.ncsu.edu/2017/03/sludge-bags-or-geotextile-tubes-to-dewater-sludge
Szogi, A. A., and Vanotti, M. B. (2016). Decline of phosphorus, copper, and zinc in anaerobic swine lagoon columns receiving pretreated influent. Sci. Agr. 73, 417–423. doi: 10.1590/0103-9016-2015-0249
Szogi, A. A., Vanotti, M. B., and Hunt, P. G. (2006). Dewatering of phosphorus extracted from liquid swine waste. Bioresour. Technol. 97, 183–190. doi: 10.1016/j.biortech.2005.02.001
Szogi, A. A., Vanotti, M. B., and Hunt, P. G. (2008). Phosphorus recovery from poultry litter. Trans. ASABE 51, 1727–1734. doi: 10.13031/2013.25306
Szogi, A. A., Vanotti, M. B., and Hunt, P. G. (2014). Process for Removing and Recovering Phosphorus from Animal Waste. U.S. Patent No. 8,673,046. Washington, DC: U.S. Patent and Trademark Office.
Szogi, A. A., Vanotti, M. B., and Hunt, P. G. (2015a). Phosphorus recovery from pig manure prior to land application. J. Environ. Manage. 157, 1–7. doi: 10.1016/j.jenvman.2015.04.010
Szogi, A. A., Vanotti, M. B., and Ro, K. S. (2015b). Methods for treatment of animal manures to reduce nutrient pollution prior to soil application. Curr. Pollut. Rep. 1, 47–56. doi: 10.1007/s40726-015-0005-1
Takahashi, M., Kato, S., Shima, H., Sarai, E., Ichioka, T., Hatyakawa, S., et al. (2001). Technology for recovering phosphorus from incinerated wastewater treatment sludge. Chemosphere 44, 23–29. doi: 10.1016/S0045-6535(00)00380-5
Technicon Instruments Corp, (1977). Individual/Simultaneous Determination of Nitrogen and/or Phosphorus in BD Acid Digests (Dialyzer): Industrial Method 337-74W/B. Tarrytown, NY: Technicon Instrument Corp.
USEPA (1999). Background Report on Fertilizer Use: Contaminants and Regulations. EPA 747-R-98-003, Office of Pollution Prevention and Toxics. Washington, DC: U.S. Environmental Protection Agency.
Vanotti, M. B., and Hunt, P. G. (1999). Solids and nutrient removal from flushed swine manure using polyacrylamides. Trans. ASAE 42, 1833–1840. doi: 10.13031/2013.13347
Vanotti, M. B., and Szogi, A. A. (2015). Systems and methods for reducing ammonia emissions and for recovering the ammonia liquid effluents. U.S. Patent No. 9,005,333. Washington, DC: U.S. Patent and Trademark Office.
WERF (1993). Guidance Manual for Polymer Selection in Wastewater Treatment Plants. Project 91-ISP-5. Alexandria, VA: Water Environment Research Foundation.
Worley, J. W., Bass, T. M., and Vendrell, P. F. (2003). “Geotextile tube dewatering of lagoon solids,” in Proceedings of 9th International Symposium on Animal Agricultural and Food Processing Wastes, ed R. T. Burns (St. Joseph, MI: ASABE), 480–486.
Worley, J. W., Bass, T. M., and Vendrell, P. F. (2008). Use of geotextile tubes with chemical amendments to dewater dairy lagoon solids. Bioresour. Technol. 99, 4451–4459. doi: 10.1016/j.biortech.2007.08.080
Keywords: phosphate, phosphorus, nutrient recovery, anaerobic lagoon, dredging, sludge, solid-liquid separation, pig manure
Citation: Szogi AA, Vanotti MB and Shumaker PD (2018) Economic Recovery of Calcium Phosphates From Swine Lagoon Sludge Using Quick Wash Process and Geotextile Filtration. Front. Sustain. Food Syst. 2:37. doi: 10.3389/fsufs.2018.00037
Received: 27 April 2018; Accepted: 20 June 2018;
Published: 11 July 2018.
Edited by:
Airton Kunz, Embrapa Suínos e Aves, BrazilReviewed by:
Albert Magrí, Universitat Politecnica de Catalunya, SpainValeria Reginatto, Universidade de São Paulo, Brazil
Copyright © 2018 Szogi, Vanotti and Shumaker. This is an open-access article distributed under the terms of the Creative Commons Attribution License (CC BY). The use, distribution or reproduction in other forums is permitted, provided the original author(s) and the copyright owner(s) are credited and that the original publication in this journal is cited, in accordance with accepted academic practice. No use, distribution or reproduction is permitted which does not comply with these terms.
*Correspondence: Ariel A. Szogi, ariel.szogi@ars.usda.gov