- Department of Food Technology, Agrotecnio Centre, University of Lleida, Lleida, Spain
This review aims at presenting recent advancements on the design of novel emulsion-based nanostructures for an effective incorporation of active ingredients into foods. The specific characteristics of nanoemulsions, solid lipid nanoparticles, and double emulsions are highlighted. Nanoemulsions are oil-in-water emulsion with droplet sizes below 200 nm that are known to present high stability over time. Moreover, due to their reduced droplet size and therefore maximized active surface area, they are capable of enhancing the interactions with biological systems, such as foods. For instance, nanoemulsions present an improved functionality of active ingredients and a fast digestibility under gastrointestinal conditions leading to an optimal absorption of bioactive ingredients contained within them. Solid lipid nanoparticles are particularly effective for protecting lipophilic active ingredients in their lipid inner core, since the crystalline state of the lipid droplets can reduce the oxidative and degradation processes occurring in aqueous systems. Nevertheless, their formulation should be carefully selected in order to obtain the desired effect. Lastly, double emulsions are defined as emulsions of an emulsion. Therefore, their formation consists on the formation of a primary water-in-oil emulsion and its subsequent further emulsification in a secondary aqueous system. This allows the encapsulation of hydrophilic active compounds in the inner aqueous phase. Their stabilization remains as a scientific challenge yet they present promising applications. The main key aspects for the formation, stabilization and their behavior during gastrointestinal conditions are addressed.
Introduction
Consumers and society are nowadays more and more aware of the relationship between food and well-being. On the one hand, there is an ongoing demand for safe food products with longer shelf life yet with similar characteristics to the fresh ones. Actually, a tendency to reject highly processed foods and the presence of synthetic ingredients or additives and to consume more natural food products is raising. On the other hand, the interest for food products fortified with health-related bioactive compounds with proven benefits is growing among consumers. Therefore, a new generation development of safer and healthier foods is a growing trend. In this context, nanotechnology applied to food science and technology opens a new window for the formulation of delivery systems at the nanoscale containing functional ingredients (Weiss et al., 2006). In this regard, natural antimicrobial agents' incorporation for food preservation or bioactive compounds for food fortification may be effectively improved by using emulsion-based delivery systems. It has been recently described that the fact of reducing the droplet size down to the nanometer range can enhance the activity of functional ingredients due to their higher active surface area (Salvia-Trujillo et al., 2013). Functional compounds used in foods may be hydrophobic or hydrophilic. Therefore, different strategies may be required for their encapsulation and incorporation of active ingredients in foods depending on their nature. This review aims at presenting emulsion-based nanostructures for the protection and delivery of active ingredients in foods by introducing the main key aspects for their formulation. Moreover, the proven advantages of their use and limitations for their incorporation in complex foods will be discussed.
Emulsion-Based Nanostructures
There are several types of emulsion-based nanostructures described as delivery systems of functional ingredients. In general, they consist on lipid droplets dispersed in aqueous media. In the case of lipophilic active ingredients, they are solubilized within the oil core of the dispersed droplets. Depending on the state of the lipid core, whether it is a liquid oil or a solid fat, nanoemulsions or solid lipid nanoparticles can be formed, respectively (Figure 1; Weiss et al., 2008; McClements and Rao, 2011). Hydrophilic active ingredients may be included in a primary aqueous phase, which may be dispersed in the lipid phase, and subsequently emulsified, thus forming double emulsions (Figure 1; Muschiolik and Dickinson, 2017). The proper selection of the type of emulsion-based nanostructured system and its formulation is of crucial importance depending on the type of encapsulated active compound and the desired functionality.
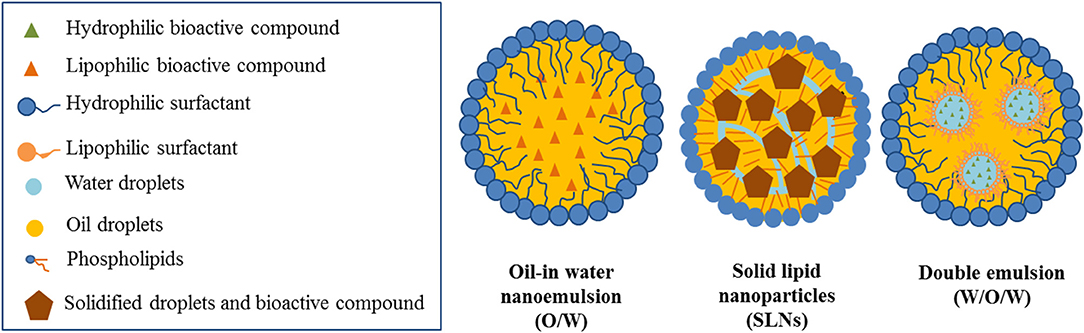
Figure 1. Emulsion-based nanostructured systems as potential carriers of active ingredients in food systems.
Nanoemulsions
Nanoemulsions are defined as oil-in-water emulsions with oil droplets with diameters below 200 nm (McClements, 2011). Due to their reduced oil droplet size, they present unique physicochemical characteristics. On the one hand, they present higher kinetic stability than conventional emulsions and are optically transparent. On the other hand, due to their higher active surface area, they may interact in a higher extent with biological surfaces than emulsions with larger droplet size (Salvia-Trujillo et al., 2017a). This confers them high efficiency as delivery systems of active ingredients in foods as presented in Table 1.
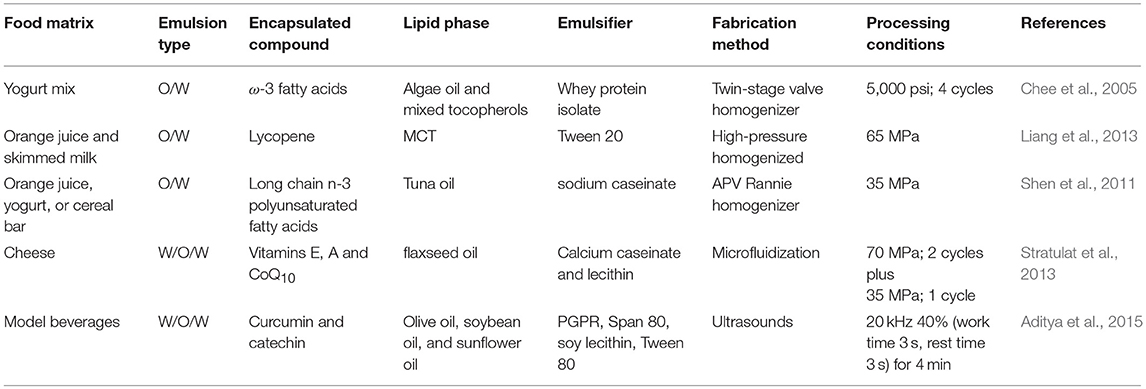
Table 1. Review of the published works reporting emulsion-based nanostructures application in food matrices.
Formation
Nanoemulsions can be formed by the use of high-energy or low energy methods. However, high-energy methods are generally more extended as they have shown to effectively reduce the oil droplet size in wide range of formulations and are regarded as easier methods to scale-up at industrial level. On the one hand, ultrasounds have been reported to produce nanoemulsions with average oil droplet sizes smaller than 100 nm (Ziani et al., 2011). The main factors affecting the fabrication of nanoemulsions by sonication are frequency, amplitude, and treatment time, which determines the intensity of the cavitation phenomena and therefore droplet disruption (Kentish et al., 2008; Abbas et al., 2013). On the other hand, high-pressure homogenizers, such as valve-homogenizers or microfluidizers, are widely used to produce nanoemulsions. In general, the higher the homogenization pressure and number of cycles, the smaller the droplet size, being reported the fabrication of nanoemulsions with particle sizes smaller than 50 nm (Solans et al., 2005; Qian and McClements, 2011).
Besides the fabrication method and processing parameters, the formation of nanoemulsions highly depends on the type of oil used as carrier of active ingredients. It has been observed that oils with long chain triglycerides, such as many vegetable oils, render nanoemulsions with larger oil droplets than those with medium or short chain triglycerides (Wooster et al., 2008). In fact, this has been related to the lipid phase viscosity, since long chain triglycerides have a higher viscosity, and therefore it reduces the efficiency of high-pressure homogenizers at droplet disruption (Qian and McClements, 2011).
Stability
Stability of nanoemulsions, and in general of emulsion-based nanostructures, is mainly determined by the presence of surface active molecules. Surfactants are able to adsorb at the oil/water interface, thus reducing the interfacial tension of both immiscible liquids, and also help stabilize the newly formed droplets during emulsification processes by creating an interfacial layer of adsorbed molecules (Walstra, 1993). In general, surfactants are amphiphilic molecules with both hydrophobic and hydrophilic regions, which can adsorb at the oil/water interface. Their emulsifying capacity depends on the Hydrophilic-Lipophilic Balance (HLB), which is a numerical factor to describe the ratio of hydrophilic or lipophilic groups. High HLB numbers (>10) correspond to hydrophilic surfactants which are used to stabilize oil droplets, whereas lipophilic surfactants (HLB < 10) are used in the stabilization of aqueous droplets. Thus, small molecule non-ionic surfactants, such as polysorbates or sodium dodecyl sulfate (SDS), have been reported to render nanoemulsions with smaller particle sizes than other surfactants such as lecithin or proteins (Ozturk et al., 2014). In this regard, nanoemulsions are reported to be kinetically stable systems, thus maintaining their droplet size and physicochemical characteristics during long-term storage (56 days; Guerra-Rosas et al., 2016). In addition to emulsifiers, emulsion stabilizers may be used in order to preserve nanoemulsion properties during time. In this regard, hydrocolloids are often used as thickening agents of the aqueous phase, thus conferring high viscosity of the continuous phase and preventing oil droplet destabilization by retarding their movement (Dickinson, 2009). In this regards, several food-grade hydrocolloids may be used, such as alginate, gum Arabic, chitosan, pectin, among others.
Digestibility
The digestibility of emulsions and in general emulsion-based nanostructures largely takes place in the small intestine, where pancreatic lipases adsorb at the oil/water interface and hydrolyze triacylglycerols into monoacylglycerols and free fatty acids (McClements, 2018). Due to their small oil droplet size, nanoemulsions are potentially digested at a higher speed than conventional emulsions with larger droplet size (Troncoso et al., 2012; Salvia-Trujillo et al., 2013). In fact, this has been attributed to the higher interfacial area exposed for the adsorption of pancreatic lipases (Reis et al., 2009). In turn, their higher digestibility may have an impact on the release of the lipophilic bioactive compounds entrapped within the lipid core. The higher digestibility kinetics of the oil droplets in nanoemulsions may lead to a faster release of the lipophilic bioactive compounds. In turn, it may result in a higher bioaccessibility due to the solubilization of lipophilic bioactive compounds in mixed micelles formed from lipid digestion products (Salvia-Trujillo et al., 2017b). Thus, nanoemulsion may potentially enhance the nutritional value of lipophilic bioactive compounds.
Solid Lipid Nanoparticles
Solid lipid nanoparticles (SLN) are emulsion-based nanostructures consisting of a crystallized lipid droplets as carrier of lipophilic active ingredients dispersed within an aqueous continuous phase (Muller et al., 2000). SLN may exhibit certain protection against oxidation and/or degradation phenomena of lipophilic active compounds since the solid lipid droplets may contribute in retarding the diffusion processes between the lipid core and the bulk aqueous phase (Tikekar and Nitin, 2011). Therefore, SLN may be a promising strategy for the protection of labile lipophilic active compounds to be incorporated in foods. Unfortunately, the number of studies to evaluate the SLN stability in commercial food is limited as the high temperatures achieved during their preparation may lead to bioactive chemical degradation (Sagalowicz and Leser, 2010).
Formation
Typically, the formation of SLN is similar to that proposed for the formation of nanoemulsions yet maintaining the temperature during the homogenization process well above the crystallization temperature, which is called hot homogenization. Afterwards, the nanoemulsion is cooled down in order to induce recrystallization of the lipid phase (Weiss et al., 2008). The higher the temperature used during the homogenization step the smaller the particle size of SLN due to the lower viscosity of the lipid phase at higher temperatures (Mehnert and Mäder, 2001). The hot homogenization technique has been reported to form submicron particle sizes depending on the homogenization device and the processing conditions.
In addition, the characteristics of SLN and their encapsulation potential are highly related to the crystallization pattern of the lipid phase. The higher the compactness of the fat crystals, the lower the encapsulation efficiency of the lipid droplets, since the bioactive lipid compound might be expelled from the lipid inner core (Pan et al., 2016). The fatty acid composition and saturation degree of the lipid phase will determine the structure of the inner lipid core. For instance, lipids such as tripalmitin or tristearin have shown to produce SLN with a more compact crystalline structure than other lipids, such as cocoa butter or coconut oil (Qian et al., 2013).
Stability
For the stabilization of SLN, the colloidal stability of the lipid particles in suspension needs to be taken into account, as well as, the chemical degradation of the encapsulated active compounds within the lipid matrix (Wissing et al., 2004).
On the one hand, the colloidal stability of SLN is determined by the type and concentration of surfactant used, as well as, the lipid state. In this regard, similar criteria to that described for nanoemulsions can be applied for the stabilization of SLN. In general, small molecule surfactants, such as Tween 80 or SDS have proven to be very effective for SLN stabilization, producing SLN as small as 40 nm (Helgason et al., 2009, 2015). In addition, the recrystallization during the cooling step after homogenization also determines the compactness of the fat crystals and in turn the stability of SLN. Slower cooling rates have shown to form lipid particles in α-form, which are spherical and more stable (6 weeks) than lipid particles in β-form, which undergo particle aggregation. Lipid particles in β-form are more amorphous, thus being more prone to suffer aggregation and destabilization phenomena (Awad et al., 2008).
On the other hand, the chemical stability of the encapsulated active compound might be determined by the lipid crystalline state. In general, SLN have shown to better protect active compounds, such as β-carotene, than their respective liquid lipid form, which can be attributed to a decreased oxidative degradation (Nik et al., 2012a,b). Nevertheless, the potential of entrap and protect the encapsulated active compound is determined by the crystallization behavior of the lipid phase, which in turn might be modulated by the presence of surfactants at the interface (Nik et al., 2012a,b). In general, lipids crystallized in α or β′ form are able to protect lipophilic active compounds with a higher efficiency than lipid with β crystals. This is due to that lipid crystals evolve from α and β′ forms, which are wider structures, toward β, which are more compact, thus having lower space to accommodate the functional compound. Subsequently, functional compounds might be expelled from the interior of the lipid core, thus showing a higher degradation over time. For instance, SLN, which lipid phase consisted on a mixture of cocoa butter and hydrogenated palm oil (1:1), exhibited a faster degradation of β-carotene with respective to a conventional nanoemulsion (Qian et al., 2013). Therefore, in order to guarantee the protection of the encapsulated active compounds, the proper selection of the lipid type is of crucial importance for the design of SLN.
Digestibility
The digestibility of SLN is highly determined by the melting profile of the lipid particles at human body temperature (37°C). Depending on the Solid Fat Content (SFC) at human body temperature, SLN may present a similar digestion pattern with regards to liquid lipids or present slower lipid hydrolysis (Nik et al., 2012a,b). Lipids that were fully melted during digestive conditions presented similar digestibility than liquid oils while lipids that remained partially or fully solidified presented significantly slower lipolysis (Guo et al., 2017). This has been mainly attributed to the differences in spatial organization of triacylglycerols at the oil/water interface, altering the lipase ability to adsorb and initiate lipid hydrolysis. The presence of solidified lipids at the particle surface might lead to more rigid and amorphous-like interfaces, thus hindering lipase adsorption, thus retarding lipid hydrolysis (Pan et al., 2016). Hence, lipid digestion may be slowed down by using SLN as carriers of active ingredients, thus delaying the release of encapsulated bioactive compounds (Bonnaire et al., 2008).
Double Emulsions
Double emulsions, or multiple emulsions, are emulsions in which the dispersed phase is an emulsion itself (Dickinson, 2011). Specifically, water-in-oil-in-water (W1/O/W2) emulsions consist of an oil-in-water (O/W2) emulsion, in which the oil phase has water droplets within it (W1). This type of emulsion-based nanostructures may present potential advantages for the encapsulation of both hydrophilic compounds in the inner aqueous phase and lipophilic compounds in the oil phase (Table 1; Márquez et al., 2007). Nevertheless, the formation and stabilization of such emulsion-based nanostructures remains as a challenge.
Formation
The formation of double emulsions is generally achieved with a two-step emulsification procedure in which first a primary water-in-oil emulsion (W1/O) is formed, and is subsequently emulsified to form a secondary emulsion (W1/O/W2) (Muschiolik and Dickinson, 2017). In order to form the primary emulsion, the use of a lipophilic surfactant to stabilize the interface between the water droplets within the oil phase is required. Polyglycerol polyricinoleate (PGPR) at a 4–6% concentration is often used as lipophilic surfactant to stabilize double emulsions. However, it is possible to reduce its concentration when used in combination with other surfactants, such as lecithin (Altuntas et al., 2017). To emulsify the primary emulsion, several homogenization techniques, such as high-pressure homogenization, sonication or high-speed blending have been reported to render water droplets between 200 and 600 nm depending on the processing conditions (Márquez et al., 2007). The formation of the secondary emulsion implies the use of a hydrophilic surfactant to stabilize the newly created oil/water interface. In this regard, the most commonly used surfactants are Tweens, lecithin, and several proteins (Andrade and Corredig, 2016). The emulsification of the primary W1/O into the W2 phase determined the overall double emulsion formation since too intense disruption forces may lead to the breakdown of the primary emulsion. Therefore, gentle high-speed blenders are often used to produce the secondary emulsion, leading to droplet sizes typically between 5 and 50 μm (Yamanaka et al., 2017).
Stability
The stabilization of double emulsions is more difficult than in the case of simple O/W emulsions due to the multiple interfaces created. There are several types of reported instability in double emulsions. First, the coalescence of the outer oil droplets might occur. Second, the inner water droplets might coalesce, without having any change in the oil droplet. Third, there might be coalescence of the inner water droplets with the outer oil interface, resulting in the transfer of some internal phase to the continuous external phase. And fourth, there might be shrinkage or swelling of the inner water droplets due to diffusive transport between the internal and external water phases though the oil phase (Dickinson, 2011). In order to avoid double emulsion destabilization, maintaining the osmotic balance between the two aqueous phases is required. Therefore, the use of small quantities of electrolytes, sugars or polysaccharides is a common practice to preserve the stability of double emulsions (Mezzenga, 2007). In addition, gelling agents, such as proteins or other hydrocolloids, have been used to avoid osmotic imbalance between the aqueous phases by inducing gelation of the inner water phase (Andrade et al., 2018).
Digestibility
Recently, the use of double emulsions has arisen as a potential strategy for a targeted delivery of bioactive compounds during gastrointestinal conditions. The encapsulated hydrophilic compound in the inner aqueous phase will be released from the inner W1 droplets when the lipid phase is digested by lipases during intestinal conditions. Therefore, depending on the characteristics of the double emulsions, such as lipid or surfactant type, the release of the encapsulated compounds may be accelerated or delayed (Frank et al., 2012). In fact, it has been recently reported a delayed release of bioactive peptides during gastrointestinal conditions of protein-stabilized double emulsions (Giroux et al., 2016). Moreover, double emulsions with gelled oil-water inner phases showed higher release of free fatty acids under simulated gastrointestinal conditions and consequently higher bioaccessibility of lipophilic bioactive compounds in comparison with double emulsions with liquid oil-water inner phases.
Concluding Remarks
In recent years, the potential of emulsion-based nanostructured systems for the encapsulation, protection and release of active ingredients is being intensively explored. In this short review, the distinguishing characteristics and potential uses of nanoemulsions, solid lipid nanoparticles and double emulsions are presented with special focus on the key aspects for their formation, stabilization and fate during digestion. Nanoemulsions are the most described system, and their nanometer droplet size has shown to effectively enhance the functionality of active ingredients. Solid lipid nanoparticles may potentially protect encapsulated active ingredients against oxidation processes. Nonetheless, the crystallization degree of solid lipid nanoparticles should be carefully selected since too compact lipid crystalline structures may result in the expulsion of the active compound of the inner lipid core and promote its degradation. Finally, double emulsions are a specific type of system that is emerging as a strategy to deliver both hydrophilic and lipophilic active ingredients yet their stabilization remains as a scientific challenge. Overall, the design of such emulsion-based nanostructures opens new opportunities for the protection of active ingredients to be incorporated in food products providing optimal functionality during the product shelf life and during their digestion.
Author Contributions
OM-B is the head of the research unit and contributed in the promotion of manuscript and the overall writing and revision of the text. LS-T is a post-doctoral researcher and was the main author of the manuscript. MA-A, AM-R, and AT-I are Ph.D. students and contributed in the manuscript writing, providing assistance to the main author.
Conflict of Interest Statement
The authors declare that the research was conducted in the absence of any commercial or financial relationships that could be construed as a potential conflict of interest.
Acknowledgments
LS-T thanks the Secretaria d'Universitats i Recerca del Departament d'Empresa i Coneixement de la Generalitat de Catalunya for the Beatriu de Pinós post-doctoral grant (BdP2016 00336). MA-A, AM-R, and AT-I thank the Univerity of Lleida for the predoctoral Research Funds.
References
Abbas, S. K., Karangwa, E., Bashari, M., and Zhang, X. (2013). An overview of ultrasound-assisted food-grade nanoemulsions. Food Eng. Rev. 5, 139–157. doi: 10.1007/s12393-013-9066-3
Aditya, N. P., Aditya, S., Yang, H. J., Kim, H. W., Park, S. O., Lee, J., et al. (2015). Curcumin and catechin co-loaded water-in-oil-in-water emulsion and its beverage application. J. Funct. Foods 15, 35–43. doi: 10.1016/j.jff.2015.03.013
Altuntas, O. Y., Sumnu, G., and Sahin, S. (2017). Preparation and characterization of W/O/W type double emulsion containing PGPR–lecithin mixture as lipophilic surfactant. J. Disper. Sci. Technol. 38, 486–493. doi: 10.1080/01932691.2016.1179121
Andrade, J., and Corredig, M. (2016). Vitamin D3 and phytosterols affect the properties of Polyglycerol Polyricinoleate (PGPR) and protein interfaces. Food Hydrocoll. 54, 278–283. doi: 10.1016/j.foodhyd.2015.10.001
Andrade, J., Wright, A. J., and Corredig, M. (2018). In vitro digestion behavior of water-in-oil-in-water emulsions with gelled oil-water inner phases. Food Res. Int. 105, 41–51. doi: 10.1016/j.foodres.2017.10.070
Awad, T. S., Helgason, T., Kristbergsson, K., Decker, E. A., Weiss, J., and McClements, D. J. (2008). Effect of cooling and heating rates on polymorphic transformations and gelation of tripalmitin Solid Lipid Nanoparticle (SLN) suspensions. Food Biophys. 3, 155–162. doi: 10.1007/s11483-008-9057-8
Bonnaire, L., Sandra, S., Helgason, T., Decker, E. A., Weiss, J., and McClements, D. J. (2008). Influence of lipid physical state on the in vitro digestibility of emulsified lipids. J. Agric. Food Chem. 56, 3791–3797. doi: 10.1021/jf800159e
Chee, C. P., Gallaher, J. J., Djordjevic, D., Faraji, H., McClements, D. J., Decker, E. A., et al. (2005). Chemical and sensory analysis of strawberry flavoured yogurt supplemented with an algae oil emulsion. J. Dairy Res. 72, 311–316. doi: 10.1017/S0022029905001068
Dickinson, E. (2009). Hydrocolloids as emulsifiers and emulsion stabilizers. Food Hydrocoll. 23, 1473–1482. doi: 10.1016/j.foodhyd.2008.08.005
Dickinson, E. (2011). Double emulsions stabilized by food biopolymers. Food Biophys. 6, 1–11. doi: 10.1007/s11483-010-9188-6
Frank, K., Walz, E., Gräf, V., Greiner, R., Köhler, K., and Schuchmann, H. P. (2012). Stability of anthocyanin-rich W/O/W-emulsions designed for intestinal release in gastrointestinal environment. J. Food Sci. 77, N50–N57. doi: 10.1111/j.1750-3841.2012.02982.x
Giroux, H. J., Robitaille, G., and Britten, M. (2016). Controlled release of casein-derived peptides in the gastrointestinal environment by encapsulation in water-in-oil-in-water double emulsions. LWT Food Sci. Technol. 69, 225–232. doi: 10.1016/j.lwt.2016.01.050
Guerra-Rosas, M. I., Morales-Castro, J., Ochoa-Martínez, L. A., Salvia-Trujillo, L., and Martín-Belloso, O. (2016). Long-term stability of food-grade nanoemulsions from high Methoxyl pectin containing essential oils. Food Hydrocoll. 52, 438–446. doi: 10.1016/j.foodhyd.2015.07.017
Guo, Q., Bellissimo, N., and Rousseau, D. (2017). The physical state of emulsified edible oil modulates its in vitro digestion. J. Agric. Food Chem. 65, 9120–9127. doi: 10.1021/acs.jafc.7b03368
Helgason, T., Awad, T. S., Kristbergsson, K., McClements, D. J., and Weiss, J. (2009). Effect of surfactant surface coverage on formation of Solid Lipid Nanoparticles (SLN). J. Coll. Inter. Sci. 334, 75–81. doi: 10.1016/j.jcis.2009.03.012
Helgason, T., Salminen, H., Kristbergsson, K., McClements, D. J., and Weiss, J. (2015). Formation of transparent solid lipid nanoparticles by microfluidization: influence of lipid physical state on appearance. J. Coll. Inter. Sci. 448, 114–122. doi: 10.1016/j.jcis.2015.02.010
Kentish, S., Wooster, T. J, Ashokkumar, M., Balachandran, S., Mawson, R., and Simons, L. (2008). The use of ultrasonics for nanoemulsion preparation. Innovat. Food Sci. Emerg. Technol. 9, 170–175. doi: 10.1016/j.ifset.2007.07.005
Liang, R., Shoemaker, C. F., Yang, X., Zhong, F., and Huang, Q. (2013). Stability and bioaccessibility of β-carotene in nanoemulsions stabilized by modified starches. J. Agric. Food Chem. 61, 1249–1257. doi: 10.1021/jf303967f
Márquez, A. L., Palazolo, G. G., and Wagner, J. R. (2007). Water in Oil (w/o) and Double (w/o/w) emulsions prepared with spans: microstructure, stability, and rheology. Coll. Polym. Sci. 285, 1119–1128. doi: 10.1007/s00396-007-1663-3
McClements, D. J. (2011). Edible nanoemulsions: fabrication, properties, and functional performance. Soft Matter 7, 2297–2316. doi: 10.1039/C0SM00549E
McClements, D. J. (2018). The biophysics of digestion: lipids. Curr. Opin. Food Sci. 21, 1–6. doi: 10.1016/j.cofs.2018.03.009
McClements, D. J., and Rao, J. (2011). Food-grade nanoemulsions: formulation, fabrication, properties, performance, biological fate, and potential toxicity. Critic. Rev. Food Sci. Nutr. 51, 285–330. doi: 10.1080/10408398.2011.559558
Mehnert, W., and Mäder, K. (2001). Solid lipid nanoparticles: production, characterization and applications. Adv. Drug Deliv. Rev. 47, 165–196. doi: 10.1016/S0169-409X(01)00105-3
Mezzenga, R. (2007). Equilibrium and non-equilibrium structures in complex food systems. Food Hydrocoll. 21, 674–682. doi: 10.1016/j.foodhyd.2006.08.019
Muller, R. H., Mader, K., and Gohla, S. (2000). Solid Lipid Nanoparticles (SLN) for controlled drug delivery - a review of the state of the art. Eur. J. Pharmac. Biopharm. 50, 161–177. doi: 10.1016/S0939-6411(00)00087-4
Muschiolik, G., and Dickinson, E. (2017). Double emulsions relevant to food systems: preparation, stability, and applications. Comprehens. Rev. Food Sci. Food Safety 16, 532–555. doi: 10.1111/1541-4337.12261
Nik, A. M., Langmaid, S., and Wright, A. J. (2012a). Digestibility and β-carotene release from lipid nanodispersions depend on dispersed phase crystallinity and interfacial properties. Food Funct. 3, 234–245. doi: 10.1039/C1FO10201J
Nik, A. M., Langmaid, S., and Wright, A. J. (2012b). Nonionic surfactant and interfacial structure impact crystallinity and stability of ??-Carotene loaded lipid nanodispersions. J. Agric. Food Chem. 60, 4126–4135. doi: 10.1021/jf204810m
Ozturk, B., Sanem, A., Ozilgen, M., and McClements, D. J. (2014). Formation and stabilization of nanoemulsion-based vitamin e delivery systems using natural surfactants: quillaja saponin and lecithin. J. Food Eng. 142, 57–63. doi: 10.1016/j.jfoodeng.2014.06.015
Pan, Y., Tikekar, R. V., and Nitin, N. (2016). Distribution of a model bioactive within solid lipid nanoparticles and nanostructured lipid carriers influences its loading efficiency and oxidative stability. Int. J. Pharmac. 511, 322–330. doi: 10.1016/j.ijpharm.2016.07.019
Qian, C., Decker, E. A., Xiao, H., and McClements, D. J. (2013). Impact of lipid nanoparticle physical state on particle aggregation and β-carotene degradation: potential limitations of solid lipid nanoparticles. Food Res. Int. 52, 342–349. doi: 10.1016/j.foodres.2013.03.035
Qian, C., and McClements, D. J. (2011). Formation of nanoemulsions stabilized by model food-grade emulsifiers using high-pressure homogenization: factors affecting particle size. Food Hydrocoll. 25, 1000–1008. doi: 10.1016/j.foodhyd.2010.09.017
Reis, P., Holmberg, K., Watzke, H., Leser, M. E., and Miller, R. (2009). Lipases at interfaces: a review. Adv. Coll. Inter. Sci. 147–148, 237–250. doi: 10.1016/j.cis.2008.06.001
Sagalowicz, L., and Leser, M. E. (2010). Delivery systems for liquid food products. Curr. Opin. Coll. Inter. Sci. 15, 61–72. doi: 10.1016/j.cocis.2009.12.003
Salvia-Trujillo, L., Qian, C., Martín-Belloso, O., and McClements, D. J. (2013). Influence of particle size on lipid digestion and β-carotene bioaccessibility in emulsions and nanoemulsions. Food Chem. 141. doi: 10.1016/j.foodchem.2013.03.050
Salvia-Trujillo, L., Soliva-Fortuny, R., Rojas-Grau, M. A., McClements, D. J., and Martin-Belloso, O. (2017a). Edible nanoemulsions as carriers of active ingredients: a review. Ann. Rev. Food Sci. Technol. 8, 439–466. doi: 10.1146/annurev-food-030216-025908
Salvia-Trujillo, L., Verkempinck, S. H. E., Sun, L., Van Loey, A. M., Grauwet, T., and Hendrickx, M. E. (2017b). Lipid digestion, micelle formation and carotenoid bioaccessibility kinetics: influence of emulsion droplet size. Food Chem. 229, 653–662. doi: 10.1016/j.foodchem.2017.02.146
Shen, Z., Apriani, C., Weerakkody, R., Sanguansri, L., and Augustin, M. A. (2011). Food matrix effects on in vitro digestion of microencapsulated tuna oil powder. J. Agric. Food Chem. 59, 8442–8449. doi: 10.1021/jf201494b
Solans, C., Izquierdo, P., Nolla, J., Azemar, N., and Celma, M. G. (2005). Nano-emulsions. Curr. Opin. Coll. Inter. Sci. 10, 102–110. doi: 10.1016/j.cocis.2005.06.004
Stratulat, I., Britten, M., Salmieri, S., Fustier, P., St-gelais, D., Champagne, C. P., et al. (2013). Enrichment of cheese with bioactive lipophilic compounds. J. Funct. Foods 6, 48–59. doi: 10.1016/j.jff.2013.11.023
Tikekar, R. V., and Nitin, N. (2011). Effect of physical state (solid vs. liquid) of lipid core on the rate of transport of oxygen and free radicals in solid lipid nanoparticles and emulsion. Soft. Matter. 7, 8149–8157. doi: 10.1039/c1sm05031a
Troncoso, E., Aguilera, J. M., and McClements, D. J. (2012). Influence of particle size on the in vitro digestibility of protein-coated lipid nanoparticles. J. Coll. Inter. Sci. 382, 110–116. doi: 10.1016/j.jcis.2012.05.054
Walstra, P. (1993). Principles of emulsion formation. Chem. Eng. Sci. 48, 333–349. doi: 10.1016/0009-2509(93)80021-H
Weiss, J., Decker, A. E., McClements, D. J., Kristbergsson, K., Helgason, T., and Awad, T. (2008). Solid lipid nanoparticles as delivery systems for bioactive food components. Food Biophys. 3, 146–154. doi: 10.1007/s11483-008-9065-8
Weiss, J., Takhistov, P., and McClements, D. J. (2006). Functional materials in food nanotechnology. J. Food Sci. 71, R107–R116. doi: 10.1111/j.1750-3841.2006.00195.x
Wissing, S. A., Kayser, O., and Müller, R. H. (2004). Solid lipid nanoparticles for parenteral drug delivery. Adv. Drug Deliv. Rev. 56, 1257–1272. doi: 10.1016/j.addr.2003.12.002
Wooster, T. J., Golding, M., and Sanguansri, P. (2008). Impact of oil type on nanoemulsion formation and ostwald ripening stability. Langmuir 24, 12758–12765. doi: 10.1021/la801685v
Yamanaka, Y., Kobayashi, I., Neves, M. A., Ichikawa, S., Uemura, K., and Nakajima, M. (2017). Formulation of W/O/W emulsions loaded with short-chain fatty acid and their stability improvement by layer-by-layer deposition using dietary fibers. LWT Food Sci. Technol. 76, 344–350. doi: 10.1016/j.lwt.2016.07.063
Keywords: nanoemulsions, solid lipid nanoparticles, double emulsions, bioactives, foods
Citation: Salvia-Trujillo L, Artiga-Artigas M, Molet-Rodríguez A, Turmo-Ibarz A and Martín-Belloso O (2018) Emulsion-Based Nanostructures for the Delivery of Active Ingredients in Foods. Front. Sustain. Food Syst. 2:79. doi: 10.3389/fsufs.2018.00079
Received: 10 July 2018; Accepted: 07 November 2018;
Published: 26 November 2018.
Edited by:
Antonio Augusto Vicente, University of Minho, PortugalReviewed by:
Guadalupe Virginia Nevárez-Moorillón, Autonomous University of Chihuahua, MexicoPablo Fuciños, Laboratório Ibérico Internacional de Nanotecnologia (INL), Portugal
Copyright © 2018 Salvia-Trujillo, Artiga-Artigas, Molet-Rodríguez, Turmo-Ibarz and Martín-Belloso. This is an open-access article distributed under the terms of the Creative Commons Attribution License (CC BY). The use, distribution or reproduction in other forums is permitted, provided the original author(s) and the copyright owner(s) are credited and that the original publication in this journal is cited, in accordance with accepted academic practice. No use, distribution or reproduction is permitted which does not comply with these terms.
*Correspondence: Olga Martín-Belloso, b21hcnRpbkB0ZWNhbC51ZGwuY2F0