- 1Department of Food Science, Center for Food Safety, University of Arkansas, Fayetteville, AR, United States
- 2Agricultural Statistics Laboratory, University of Arkansas, Fayetteville, AR, United States
- 3Jones-Hamilton Co., Walbridge, OH, United States
When considering the cost associated with producing potable water and the environmental concerns associated with water scarcity, the reuse of processing water has received increasing interest. While water reuse during poultry processing can be environmentally friendly, it also brings potential food safety and cross-contamination concerns, through the reuse of water which may harbor human-host pathogens. Therefore, in order to effectively utilize reuse water systems, to mitigate environmental and cost concerns with water use, antimicrobials must be investigated to reduce bacterial and pathogen load. Currently, peracetic acid (PAA) is commonly used in processing water up to 2,000 ppm but can be corrosive with documented public health concerns. As such, sodium bisulfate (SBS) may be utilized as it has the potential to be an important anti-microbial in poultry processing facilities as seen in its application in the produce industry. In this study, SBS, PAA, and industrial grade PAA (IG-PAA) were evaluated against microbial populations in water reuse systems inoculated with Salmonella Typhimurium. Fresh, untreated processing plant reuse water was collected at the end of a poultry processing shift. The water was utilized within 1 h of collection, minimum inhibitory concentrations were established, change in pH was investigated, and plate counts after Salmonella addition were performed. When 3 × 107 CFU of Salmonella was added to each microcosm, a 4 to 5 log reduction with 200 ppm PAA was observed compared to a total killing observed by 5 min of treatment with 1, 2, or 3% SBS (P < 0.05). The results of this study indicate that SBS may be an equally effective alternative to PAA for decreasing foodborne pathogen contamination in poultry reuse water.
Introduction
Water scarcity continues to be a global concern (Beekman, 1998; Casani et al., 2005; Meneses et al., 2017; Faour-Klingbeil and Todd, 2018). By 2025 almost half of the world's population will face severe clean water scarcity issues (Alcamo et al., 1997, 2000; Micciche et al., 2018). Factors impacting water scarcity include climate change, shifts in the human diet, and an increase in water use for industrial processing (Meneses et al., 2017). In the food industry, water use increased by 40% throughout a decade from 1998 to 2008 (Klemes et al., 2008; Meneses et al., 2017). To face these environmental, and eventually economic pressures, food processing industries need to develop novel water conservation technologies.
In poultry processing, 21 to 30 L of potable water are used per bird (Northcutt and Jones, 2004; Kiepper et al., 2008; Walsh et al., 2018). There have been studies suggesting water usage could be reduced in poultry processing (Lillard, 1979; Meneses et al., 2017). However, the use of contaminant-free water is pivotal for food safety and the consideration of Hazard Analysis and Critical Control Point (HACCP) plans (Northcutt and Jones, 2004; United States Department of Agriculture, 2016). As a consequence, to reduce the amount of water utilized in poultry processing without compromising on efficiency or product safety, reuse water systems can be implemented (Micciche et al., 2018).
Reuse of water or “the recovery and reconditioning of water from a processing step followed by its subsequent use” is covered in 9 CFR 416.2 and is enforced by the United States Department of Agriculture (USDA) (United States Department of Agriculture, 2016; Meneses et al., 2017). According to the law, the reuse of processing water from upstream steps should not be utilized for downstream processing steps (United States Department of Agriculture, 2016). Also, reuse processing water should be equivalent to potable, or drinkable, water (United States Department of Agriculture, 2016; Meneses et al., 2017). To ensure chemical equivalency, filtration systems are often utilized. For microbial and pathogen elimination, sanitizers are employed (Northcutt and Jones, 2004). Chlorine has long been the sanitizer of choice for the U.S. poultry industry, but it can be hazardous to workers due to its potential to form dangerous by-products, and bacterial resistance has been documented (Casani and Knøchel, 2002; Dunlop et al., 2002; Casani et al., 2005; Ryu and Beuchat, 2005). Furthermore, the European Union has banned the use of chlorine since 1997 and USDA regulations prevent the amount of free chlorine in reuse water to exceed 5 ppm (United States Department of Agriculture, 2003; Johnson, 2015). As a consequence, alternative acid sanitizers such as peracetic acid (PAA) and sodium bisulfate (SBS) are of interest.
Peracetic acid has been utilized as a conventional sanitizer for poultry processing due to its effectiveness against Campylobacter and Salmonella, which are common poultry processing pathogens (Oyarzabal, 2005; Bauermeister et al., 2008a; Kim et al., 2017). However, while its legally allowed maximum concentration has recently increased to 2,000 ppm (21 CFR 173.370), PAA is corrosive to equipment and hazardous to workers (Casani et al., 2005; Kim et al., 2018). Furthermore, there is evidence that peracetic acid can decay into acetic acid within 30 min on poultry carcasses (Walsh et al., 2018). Sodium bisulfate may be an alternative to peracetic acid as it has shown to be an effective antimicrobial against Salmonella on chicken parts (Dittoe et al., 2018a). SBS is an inorganic acidifier with a pKa of 1.96 (Knueven, 1999). This low dissociation constant allows for highly acidic conditions, which Salmonella's acid tolerance mechanism and acid-shock proteins may not be able to compensate for, which would result in cell death (Foster and Hall, 1991; Knueven, 1999; Ryan et al., 2016). It has also been shown to be effective against Campylobacter when utilized pre-harvest (Line, 2002), and a 10% SBS spray decreased Salmonella counts by 2 logs on poultry carcasses (Li et al., 1997). As such, SBS may be an ideal candidate for poultry processing reuse water systems. In current study, we report on the antibacterial effectiveness of three acid sanitizers (peracetic acid, sodium bisulfate, and industrial-grade peracetic acid) added to reuse poultry processing water. More specifically, we hypothesized that SBS, compared to PAA based acidifiers, would achieve a higher rate of reduction of Salmonella Typhimurium populations when reuse water microcosms were inoculated with the bacteria. In the current study, we utilized concentrations of SBS and PAA that have been reported not to affect the sensory characteristics of poultry carcasses (Dittoe et al., 2018a).
Materials and Methods
Bacterial Strain
The Salmonella Typhimurium UK-1 (ATCC 68169) used in this study was originally isolated from a chicken spleen inoculated 3 days prior with a virulent S. Typhimurium strain from an infected horse and the complete genome was sequenced by Luo et al. (2011) and Curtiss and Hassan (1991). It was maintained in Luria Bertani (LB) broth (Difco Laboratories, Detroit, MI, USA) at 37°C and at a concentration of 3 × 109 CFU (Colony Forming Units)/mL. Concentrations were verified through plating onto Xylose Lysine Desoxycholate (XLD) agar Difco Laboratories, Detroit, MI, USA).
Water Collection
Processing water samples were collected from a commercial poultry processing facility at the intake of the reuse water sanitization system. Intake waters were chlorinated (20 to 50 ppm total chlorine) and used in an inside-outside bird washer before water reuse collection. Water was transported to the laboratory at room temperature and utilized within 1 h of sample collection.
Salmonella Killing Assay of Reuse Water Microcosms
Upon arrival, 20 mL of reuse water was individually added to sterile Erlenmeyer flask alone or with acid sanitizers to generate the following concentrations: 3% SBS (w/v) (Jones-Hamilton Co, Walbridge, Ohio), 2% SBS (w/v), 1% SBS (w/v), 0.02% PAA (v/v) (Sigma-Aldrich, St. Louis, MO, USA), or 0.02% Industrial Grade PAA (v/v) (IG-PAA). The composition of the PAA stock solution was Acetic Acid at ~45%, Peracetic acid at ~39%, Hydrogen Peroxide at ~6%, and water at ~10% (Sigma-Aldrich, St. Louis, MO, USA). The composition of the IG-PAA stock solution was Acetic Acid 33 to 39%, Hydrogen Peroxide 8.5 to 10.5%, Peracetic Acid 20 to 23%, Water 28 to 35%. Three technical replicates were performed per trial, with a total of 6 independent trials in all. Each trial was repeated on a separate day using freshly collected poultry processing water from the same source. At every time point, the pH was also measured using a Symphony B10P (VWR, Radnor, PA, USA).
The Salmonella killing assay was performed by adding 100 μL 3 × 109 CFU/mL Salmonella Typhimurium UK-1 to the reuse water microcosms with the acid sanitizers (1, 2, and 3% SBS, PAA, or IG-PAA). A no treatment control was also included. A 100 μL aliquot of the microcosms were plated onto XLD immediately and samples were collected every 5 min for 30 min. Incubation of plates occurred at 37°C for 24 h. The limit of detection (LOD) was determined to be 10 CFU/mL (Sutton, 2011).
Minimum Inhibitory Concentration (MIC) and Minimum Bactericidal Concentration (MBC)
One milliliter of a 1% triphenyl tetrazolium chloride solution (TTC) was added to 100 mL of reuse water filtered with a sterile cheesecloth. To each well of a 96-well plate, 50 μL of reuse water with TTC was added. Stock solutions of each acid sanitizer were prepared in deionized water in the following concentrations: 12% SBS, 0.8% PAA, 0.8% IG-PAA. Further serial dilutions (1:1) were made ranging from 6 to 0.09% for the SBS treatment and 0.4 to 0.00625% for the PAA and IG-PAA treatments. This was performed by thoroughly mixing 50 μL of stock acidifier to the first well with 50 μL of reuse water and transferring 50 μL of acidifiers water to the next well for subsequent mixing and transfer. For positive control wells, 50 μL of phosphate buffered saline (PBS) was added in place of acid sanitizers. To each well, 50 μL of LB inoculated with 3 × 108 CFU/mL of Salmonella UK-1 was added and mixed. For negative control wells, 50 μL of sterile LB was added. This further diluted each sanitizer concentration in the well by a factor of 2. The plate was placed in an Infinite M200 microtiter plate reader (Tecan Männedorf, Switzerland), set to 37°C, shaken every 15 min, and reads were taken at 24 h at 480 nm. Changes in absorbance were indicative of bacterial growth and analyzed. For each well that had no change in absorbance an MBC test was performed by plating 50 μL onto XLD which was incubated at 37°C for 24 h. The concentration of sanitizer was considered to be bactericidal if no growth was detected.
Statistics
A one-way analysis of variance (ANOVA) was performed using non-parametric test (Kruskal-Wallis, Siegel and Castellan, 1988) for the Salmonella killing assay and a parametric test for the change in pH. When the ANOVA indicated a significant result, differences between the mean values were determined using pairwise comparisons test. Statistical significance was determined at 0.05 level. All data analyses were conducted using R (Version 3.5.1; R Core Team., Vienna, Austria).
Results
Reuse Water Microcosms-Change in Salmonella Concentrations
Because time point 0 was measured immediately after the addition of Salmonella and acid sanitizers, there were initial killings observed in all treatment groups when compared to the no treatment control. The average detected log CFU/mL at time point zero was 6.84, 4.66, 4.38, 4.34, 5.47, 6.73 for the negative control, PAA, Ig-PAA, and 1, 2, and 3% SBS groups, respectively. The standard deviations were 0.03, 0.218, 0.33, 0.59, 0.15, and 0.13, respectively. Both the 2 and 3% SBS treatments were statistically not different compared to the control at time 0 (P > 0.05), whereas the PAA, IG-PAA, and 1% SBS were statistically significant (P < 0.05). By the 5-min time point, no Salmonella counts were detected for the SBS treatments (Figure 1). Replicates within the PAA treatment varied with the time required to reduce Salmonella below the limit of detection (Figure 1). It also took 25 min for one replicate of IG-PAA to be reduced below the limit of detection. One replicate for standard PAA persisted after 30 min. No significant change over time was detected for the no treatment control.
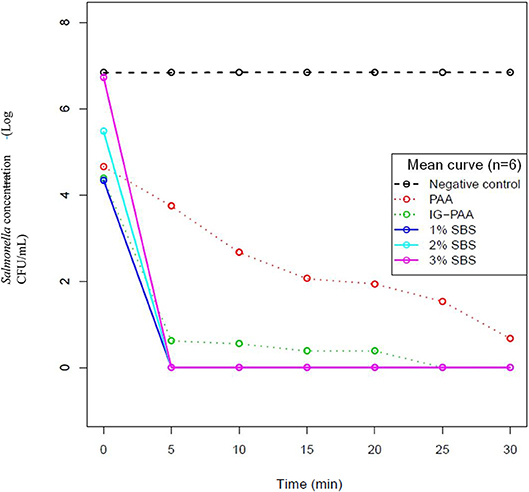
Figure 1. Salmonella Typhimurium killing assay over time in each treatment. S. Typhimurium inoculated microcosms with five acidifier treatments were immediately plated onto XLD (n = 6). Every 5 min after that for 30 min, plating occurred, and log-transformed counts were graphed. A value of 0.1 log CFU/mL was applied to visualize overlapping data points. Data points at zero were below the limit of detection (10 CFU/mL). The solid blue, cyan, and magenta line represents 1, 2, and 3% SBS treatments. The dashed red and green lines represent 200 ppm PAA and 200 ppm IG-PAA, respectively. The black dashed line represents the no treatment negative control.
Reuse Water Microcosms-Change in pH
There were no significant changes in pH over time across treatments (Figure 2). There were similarities between the pH of reuse with or without the addition of S. Typhimurium for each treatment. There was a significant treatment effect on pH. At time point 0 and 30 min, when Salmonella was inoculated, all treatments were significantly different from each other except for the 3 and 2% SBS treatment and the 2 and 1% SBS treatment (P < 0.05) (Table 1). At 30 min the average pH for 1, 2, and 3% SBS were 1.58, 1.36, and 1.23. For PAA, the average was 4.02, and IG-PAA average pH was at 5.13. The average for the negative control was 7.52.
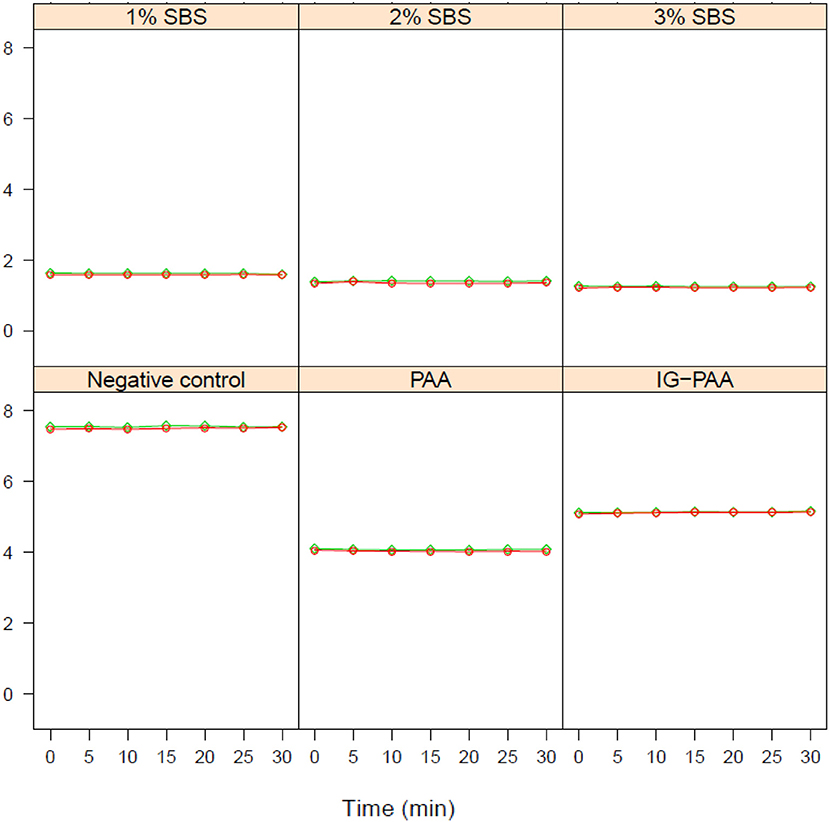
Figure 2. Impact on reuse water pH over time. The pH of reuse water with or without Salmonella Typhimurium inoculation after treatment with acidifiers. The average pH over three replicates at each time point is reported. S. Typhimurium was inoculated at time point zero at a concentration of 3 × 107CFU/mL. The green diamonds represent pH of reuse water with S. Typhimurium. The red circles represent pH of reuse water without Salmonella addition. These data points are similar enough that overlaps occur.
When pH was compared to S. Typhimurium population levels for one of the blocks (n = 3), the lower the pH, the greater the population of viable S. Typhimurium at time point zero, except the negative control (Figure 3A). However, at 5 and 10-min time points, this relationship was not present as all replicates exhibited no growth in this block except for PAA (Figures 3B,C).
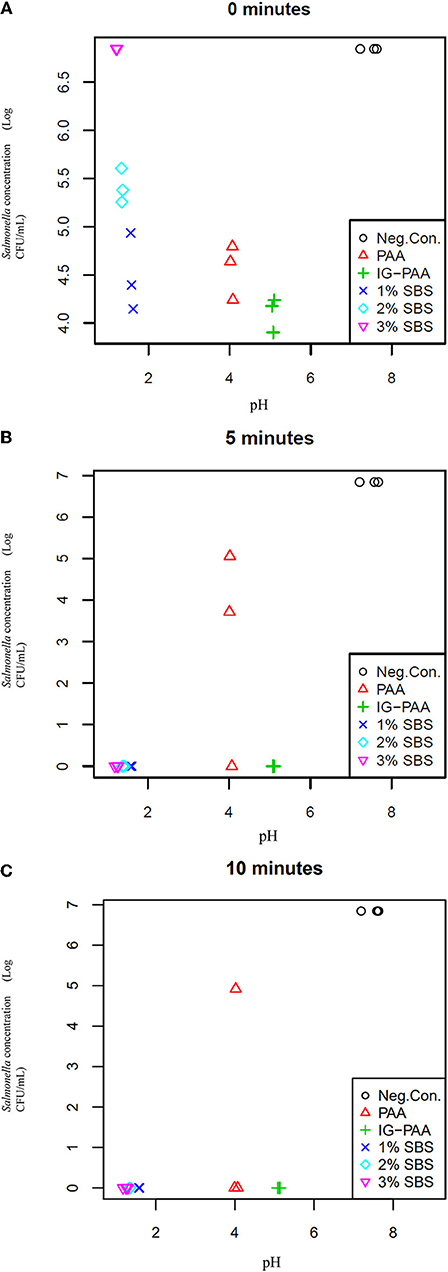
Figure 3. Comparison of pH and Salmonella Typhimurium concentration at 0, 5, and 10 min.(A) Represents comparisons of pH and S. Typhimurium concentration at time point 0 min. The pH was adjusted using the acidifier treatments. The inoculum added initially was 3 × 107 CFU/mL S. Typhimurium UK-1 to each microcosm (n = 3). Plots (B,C) represent the comparison of pH and Salmonella concentration at 5 and 10 min and were generated in the same manner as (A).
Minimum Inhibitory and Bactericidal Concentration Assays
The MIC for SBS, PAA, and IG-PAA were 0.375, 0.05, and 0.10%. The MBC for SBS, PAA, and IG-PAA were 0.75, 0.10, and 0.10%, respectively. These concentrations were validated using the microtiter plate reader and are illustrated in Figures 4A–C. An increase in OD at 480 nm was indicative of dehydrogenation of TTC, and therefore metabolic activity and bacterial presence (O'Bryan et al., 2008). The minimum inhibitory concentration was the lowest concentration that did not exhibit a change in absorbance compared to the negative control. The MIC values were higher for SBS and PAA compared to their MIC responses.
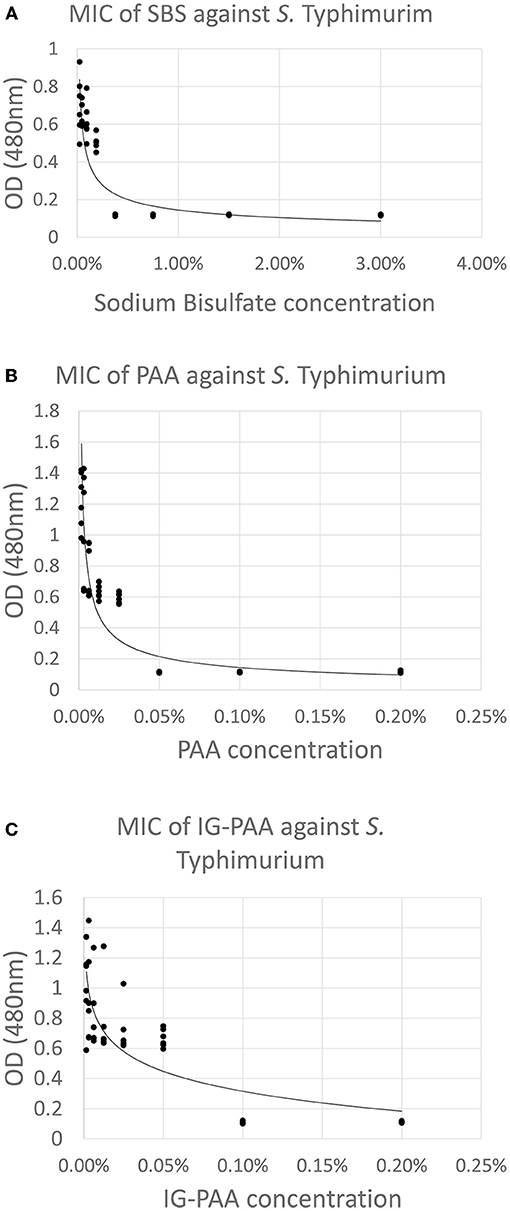
Figure 4. (A) MIC of SBS against S. Typhimurium in reuse water with 0.01% TTC. One milliliter of 1% TTC was added to filtered reuse water which was inoculated with 5 × 107 CFU/mL S. Typhimurium UK-1. After a 24 h incubation at 37°C with shaking, the absorbance at 480 nm was taken and plotted against concentration (n = 6). The MIC was determined to be 0.375% (3,750 ppm) Plots (B,C) represent MIC against PAA and IG-PAA, respectively, and were generated in the same manner as (A), but using their respective acid sanitizers. The MIC was 0.05% (500 ppm) and 0.10% (1,000 ppm), respectively.
Discussion
Acidifiers are often used to control biological contamination within poultry processing facilities and have been used in poultry feed, in the form of organic acids, to control bacteria (Smyser and Snoeyenbos, 1979; Paster et al., 1987; Kurschner and Diken, 1997; Dittoe et al., 2018b). The acidifying properties of compounds such as PAA and SBS allow for the decrease of pH which can interfere with cellular metabolism, particularly through disruption of the proton gradient (Kim et al., 2005). Treatment of poultry processing chiller water with peracetic acid has been shown to reduce bacterial loads, as well as Salmonella and Campylobacter concentrations (Bauermeister et al., 2008a,b). Peracetic acid at 200 ppm in the chiller tank did not significantly impact sensory characteristics of the finished product (Bauermeister et al., 2008a,b). Other foodborne pathogens have been shown to be significantly reduced within 10 min by the application of 0.1% PAA (Briñez et al., 2006). In Bauermeister et al. (2008a), PAA reduced S. Typhimurium concentrations between 1.5 and 2 log CFU/carcass when inoculated with 106 CFU/carcass compared to 0.03% sodium hypochlorite which only produced a 3 log reduction.
Our data are in congruence with the results reported in Bauermeister et al. (2008a), a 3 to 4 log CFU/mL reduction with PAA (Bauermeister et al., 2008b). However, our results indicate that within the microcosms containing reuse water, SBS appeared to be more consistently effective at producing reductions in Salmonella populations than PAA or IG-PAA after 5 min. At timepoint zero there were apparent initial reduction in S. Typhimurium populations. Other studies which have investigated SBS or PAA in different matrices such as apples have not observed initial reductions of pathogens (Kim et al., 2018). The 3% SBS treatment showed no significant change at timepoint zero compared to the negative control despite containing the most acidifier and lowest pH. This may be due to experimental error, but the reproducibility between replicates suggests there may be other possibilities that will require future studies to determine the specific mechanism(s) associated with the response of S. Typhimurium to SBS. Non-parametric tests were used for timepoint zero comparisons of the Salmonella concentrations because they do not require any distributional assumption, which is advantageous for data acquired from the small sample size. On the other hand, ANOVA F- or t-tests require the assumption of normality and equal variance, which is not always possible to assume for small sample sizes (<30), especially when it is impossible to accurately test the assumption with our sample size (n = 6).
Furthermore, the concentration of residual chlorine was not determined in this experiment. However, based on the negative control data, the impact of any previously added antimicrobials was not a significant source of pathogen reduction. Additionally, tetrathionate enrichment after acid neutralization with neutralizing buffered peptone water was performed and indicated no recovery of S. Typhimurium in the SBS treated water (data not shown). The decay of peracetic acid into acetic acid, was not tested in this experiment. However, this may help explain the survival and recovery of S. Typhimurium populations in peracetic acid compared to the total reduction observed with SBS. Walsh et al. (2018) demonstrated that within 15 min 15.8 mg PAA/kg chicken decreased to 0.4 mg PAA/kg chicken resulting in a near equivalent increase in acetic acid concentrations. There is potential for microorganisms, such as Salmonella, to utilize acetic acid as a food source and recover from sanitization (Stampi et al., 2001; Kitis, 2004).
The MIC and MBC data in this experiment indicate that over a 24-h period, lower concentrations of PAA and IG-PAA are needed for inhibitory and bactericidal activity. To ensure chemical consistency, filtration was applied for the MIC assay, which impacts the organic loads present within the water. The presence of highly concentrated organic matter can slow the rate of disinfection with PAA (Lillard, 1979; Gehr and Cochrane, 2002; Gehr et al., 2003; Casani et al., 2005; McKee, 2011). This effect may influence the MIC and MBC results.
The main objective of this study was to evaluate the use of acidifiers for biological sanitation of poultry processing water in an effort to reuse and conserve water within the processing facility. Our results indicate that, in microcosms, total reduction can be observed with SBS, while no recovery of S. Typhimurium was found for SBS treatments via tetrathionate enrichment or direct plating after 5 min. This may be due to the difference in concentration and the pH of these treatments. However, the concentrations of SBS were utilized due to previous results indicating no impact on sensory characteristics of skin on chicken carcasses (Dittoe et al., 2018a). The limit of peracetic acid concentration has recently been increased from 200 to 2,000 ppm in poultry processing, and studies have detailed how PAA can be corrosive to equipment and caustic to the skin of personnel (Casani et al., 2005; Peracetic acid, 2013; Walsh et al., 2018). While SBS is generally recognized as safe (GRAS) (Food Drug Administration., 2018) it should be noted that low pH can also be dangerous to personnel and further studies may be needed to validate the efficacy of SBS at a pH that is more typically seen within the poultry processing facility (Bauermeister et al., 2008a). The concentrations of SBS tested in this study were shown to be more effective in reducing S. Typhimurium in poultry processing reuse water microcosms than PAA or IG-PAA.
Conclusion
Environmental pressures will soon be felt throughout all food industries as fresh water becomes increasingly scarce. To combat this, the poultry industry may utilize reuse water systems but will face the risk of incomplete sanitation or an inability to reduce organic load. Our results showed a significant reduction of S. Typhimurium in microcosms containing acid sanitizers. In this study it was demonstrated that sodium bisulfate could be utilized in preventing S. Typhimurium contamination of processing reuse water. These results provide initial insights into the use of alternative antimicrobial treatments in alternative poultry production systems. A next logical step will be to determine if treated reuse water will reduce microbial cross contamination on chicken carcasses and not impact shelf life when substituted for fresh water. Lianou and Koutsoumanis (2013) concluded that phenotypic responses and genetic variation among strains of the same foodborne pathogen are important to understand inactivation and growth characteristics. In Salmonella specifically, Andino and Hanning (2015) detail the importance of genetic variation within serovars in regard to virulence and control at the pre- and post-harvest level. As such, a multiple strain (cocktail) of Salmonella should be considered to determine if genetic variation plays a factor. In addition, testing at a pilot scale level will determine if SBS can practically be utilized on a much greater scale to reduce microbial contamination in poultry processing reuse systems. Finally, elucidating the mechanism(s) of the response by Salmonella and other microorganism to SBS should provide additional information for optimizing concentrations and more efficient means for application to reduce bacterial loads in reuse water.
Author Contributions
All authors listed have made a substantial, direct and intellectual contribution to the work, and approved it for publication.
Conflict of Interest Statement
CK is employed by the company Jones-Hamilton.
The remaining authors declare that the research was conducted in the absence of any commercial or financial relationships that could be construed as a potential conflict of interest.
Acknowledgments
AM is supported by a Distinguished Doctoral Fellowship and support from the Department of Food Science at the University of Arkansas. Jones-Hamilton is acknowledged for its support and assistance. The Foundation for Meat & Poultry Research & Education is acknowledged for its support and assistance.
References
Alcamo, J., Döll, P., Kaspar, F., and Siebert, S. (1997). Global Change and Global Scenarios of Water Use and Availability: An Application of Water Gap 1.0. Center for Environmental Systems Research (CESR), University of Kassel, 1720.
Alcamo, J., Henrichs, T., and Rösch, T. (2000). World Water in 2025: Global Modeling and Scenario Analysis for the World Commission on Water for the 21st Century. Center for Environmental Systems Research (CESR), University of Kassel.
Andino, A., and Hanning, I. (2015). Salmonella enterica: survival, colonization, and virulence differences among serovars. Sci. World J. 2015, 1–16. doi: 10.1155/2015/520179
Bauermeister, L. J., Bowers, J. W., Townsend, J. C., and McKee, S. R. (2008b). Validating the efficacy of peracetic acid mixture as an antimicrobial in poultry chillers. J. Food Prot. 71, 1119–1122. doi: 10.4315/0362-028X-71.6.1119
Bauermeister, L. J., Bowers, J. W. J., Townsend, J. C., and McKee, S. R. (2008a). The microbial and quality properties of poultry carcasses treated with peracetic acid as an antimicrobial treatment. Poult. Sci. 87, 2390–2398. doi: 10.3382/ps.2008-00087
Beekman, G. B. (1998). Water conservation, recycling and reuse. Water Res. Dev. 14, 353–364. doi: 10.1080/07900629849268
Briñez, W. J., Roig-Sagués, A. X., Herrero, M. M. H., Lopez-Pedemonte, T., and Guamis, B. (2006). Bactericidal efficacy of peracetic acid in combination with hydrogen peroxide against pathogenic and non pathogenic strains of Staphylococcus spp., Listeria spp. and Escherichia coli. Food Control 17, 516–521. doi: 10.1016/j.foodcont.2005.02.014
Casani, S., and Knøchel, S. (2002). Application of HACCP to water reuse in the food industry. Food Control 13, 315–327. doi: 10.1016/S0956-7135(02)00037-3
Casani, S., Rouhany, M., and Knøchel, S. (2005). A discussion paper on challenges and limitations to water reuse and hygiene in the food industry. Water Res. 39, 1134–1146. doi: 10.1016/j.watres.2004.12.015
Curtiss, R., and Hassan, J. O. (1991). “Nonrecombinant and recombinant avirulent Salmonella live vaccines for poultry,” in Colonization Control of Human Bacterial Enteropathologens in Poultry, eds L. C. Blankenship, J. S. Bailey, N. A. Cox, S. E. Craven, R. J. Reinersmann, and N. S. Stern (New York, NY: Academic Press, Inc.), 169–198.
Dittoe, D. K., Atchley, J. A., Feye, K. M., Knueven, C. J., and Ricke, S. C. (2018a). “Abstract #163: Effect of sodium bisulfate salt on mitigating the presence of an antibiotic resistant strain of Salmonella Enteritidis on chicken drum sticks,” in Poultry Science Association Annual Meeting (San Antonio, TX).
Dittoe, D. K., Ricke, S. C., and Kiess, A. S. (2018b). Organic acids and potential for modifying the avian gastrointestinal tract and reducing pathogens and disease. Front. Vet. Sci. 5:216. doi: 10.3389/fvets.2018.00216
Dunlop, P. S. M., Byrne, J. A., Manga, N., and Eggins, B. R. (2002). The photocatalytic removal of bacterial pollutants from drinking water. J. Photochem. Photobiol. A Chem. 148, 355–363. doi: 10.1016/S1010-6030(02)00063-1
Faour-Klingbeil, D., and Todd, E. C. (2018). The impact of climate change on raw and untreated wastewater use for agriculture, especially in arid regions: a review. Foodborne Pathog. Dis. 15, 61–72. doi: 10.1089/fpd.2017.2389
Food Drug Administration. (2018). GRAS Substances (SCOGS) Database. Available online at: https://www.fda.gov/food/ingredientspackaginglabeling/gras/scogs/default.htm
Foster, J. W., and Hall, H. K. (1991). Inducible pH homeostasis and the acid tolerance response of Salmonella typhimurium. J. Bacteriol. 173, 5129–5135. doi: 10.1128/jb.173.16.5129-5135.1991
Gehr, R., and Cochrane, D. (2002). Peracetic acid (PAA) as a disinfectant for municipal wastewaters: encouraging performance results from physicochemical as well as biological effluents. Proc. Water Environ. Fed. 2002, 182–198. doi: 10.2175/193864702785033527
Gehr, R., Wagner, M., Veerasubramanian, P., and Payment, P. (2003). Disinfection efficiency of peracetic acid, UV and ozone after enhanced primary treatment of municipal wastewater. Water Res. 37, 4573–4586. doi: 10.1016/S0043-1354(03)00394-4
Johnson, R. (2015). US-EU poultry dispute on the use of pathogen reduction treatments (PRTs). Congr. Res. Ser. 7–5700.
Kiepper, B. H., Merka, W. C., and Fletcher, D. L. (2008). Proximate composition of poultry processing wastewater particulate matter from broiler slaughter plants. Poult. Sci. 87, 1633–1636. doi: 10.3382/ps.2007-00331
Kim, S. A., Hong Park, S., In Lee, S., Owens, C. M., and Ricke, S. C. (2017). Assessment of chicken carcass microbiome responses during processing in the presence of commercial antimicrobials using a next generation sequencing approach. Sci. Rep. 7:43354. doi: 10.1038/srep43354
Kim, S. A., Park, S. H., Knueven, C., Basel, R., and Ricke, S. C. (2018). A decontamination approach using a combination of bisulfate of soda and peracetic acid against listeria innocua inoculated on whole apples. Food Control 84, 106–110. doi: 10.1016/j.foodcont.2017.07.036
Kim, Y. Y., Kil, D. Y., Oh, H. K., and Han, I. K. (2005). Acidifier as an alternative material to antibiotics in animal feed. Asian Aust. J. Anim. sci. 18:1048. doi: 10.5713/ajas.2005.1048
Kitis, M. (2004). Disinfection of wastewater with peracetic acid: a review. Environ. Int. 30, 47–55. doi: 10.1016/S0160-4120(03)00147-8
Klemes, J., Smith, R., and Kim, J. K. (Eds.). (2008). Handbook of Water and Energy Management in Food Processing. Swatson: Woodhead Publishing, 48. doi: 10.1533/9781845694678
Knueven, C. J. (1999). Sodium Bisulfate as Acidulant in Foods. U.S. Patent No 5,958,491. Washington, DC: U.S. Patent and Trademark Office.
Kurschner, L. M., and Diken, G. M. (1997). Use of Peracetic Acid to Sanitize Processed Fowl. U.S. Patent No. 5,632,676. Washington, DC: U.S. Patent and Trademark Office.
Li, Y., Slavik, M. F., Walker, J. T., and Xiong, H. (1997). Pre-chill spray of chicken carcasses to reduce Salmonella typhimurium. J. Food Sci. 62, 605–607. doi: 10.1111/j.1365-2621.1997.tb04441.x
Lianou, A., and Koutsoumanis, K. P. (2013). Strain variability of the behavior of foodborne bacterial pathogens: a review. Int. J. Food Microbiol. 167, 310–321. doi: 10.1016/j.ijfoodmicro.2013.09.016
Lillard, H. S. (1979). Levels of chlorine and chlorine dioxide of equivalent bactericidal effect in poultry processing water. J. Food Sci. 44, 1594–1597. doi: 10.1111/j.1365-2621.1979.tb09097.x
Line, J. E. (2002). Campylobacter and Salmonella populations associated with chickens raised on acidified litter. Poult. Sci. 81, 1473–1477. doi: 10.1093/ps/81.10.1473
Luo, Y., Kong, Q., Yang, J., Golden, G., Wanda, S. Y., Jensen, R. V., et al. (2011). Complete genome sequence of the universal killer Salmonella enterica serovar Typhimurium UK-1 (ATCC 68169). J. Bacteriol. 193, 4035–4036. doi: 10.1128/JB.05224-11
McKee, S. R. (2011). “Salmonella and Campylobacter control during poultry processing,” in International Poultry Scientific Forum (Atlanta, GA).
Meneses, Y. E., Stratton, J., and Flores, R. A. (2017). Water reconditioning and reuse in the food processing industry: current situation and challenges. Trends in Food Sci. Technol. 61, 72–79. doi: 10.1016/j.tifs.2016.12.008
Micciche, A. C., Feye, K. M., Rubinelli, P. M., Wages, J. A., Knueven, C., and Ricke, S. C. (2018). The implementation and food safety issues associated with poultry processing reuse water for conventional poultry production systems in the United States. Front. Sustain. Food Syst. 2:70. doi: 10.3389/fsufs.2018.00070
Northcutt, J. K., and Jones, D. R. (2004). A survey of water use and common industry practices in commercial broiler processing facilities. J. Appl. Poult. Res. 13, 48–54. doi: 10.1093/japr/13.1.48
O'Bryan, C. A., Crandall, P. G., Chalova, V. I., and Ricke, S. C. (2008). Orange essential oils antimicrobial activities against Salmonella spp. J. Food Sci. 73, M264–M267. doi: 10.1111/j.1750-3841.2008.00790.x
Oyarzabal, O. A. (2005). Reduction of Campylobacter spp. by commercial antimicrobials applied during the processing of broiler chickens: a review from the United States perspective. J. Food Prot. 68, 1752–1760. doi: 10.4315/0362-028X-68.8.1752
Paster, N., Pinthus, E., and Reichman, D. (1987). A comparative study of the efficacy of calcium propionate, agrosil and adofeed as mold inhibitors in poultry feed. Poult. Sci. 66, 858–860. doi: 10.3382/ps.0660858
Peracetic acid (2013). Peracetic acid. MSDS No. SLP5503. Sciencelab.com, Inc. 14025 Smith Rd. Houston, TX. Available online at https://www.sciencelab.com/msds.php?msdsId=9926439 (Accessed May 15, 2018).
Ryan, D., Ojha, U. K., Jaiswal, S., Padhi, C., and Suar, M. (2016). The Small RNA DsrA influences the acid tolerance response and virulence of Salmonella enterica serovar Typhimurium. Front. Microbiol. 7:599. doi: 10.3389/fmicb.2016.00599
Ryu, J. H., and Beuchat, L. R. (2005). Biofilm formation by Escherichia coli O157: H7 on stainless steel: effect of exopolysaccharide and curli production on its resistance to chlorine. Appl. Environ. Microbiol. 71, 247–254. doi: 10.1128/AEM.71.1.247-254.2005
Siegel, S., and Castellan, N. J. (1988). Non Parametric Statistics for the Behavioural Sciences. New York, NY: MacGraw Hill Int.
Smyser, C. F., and Snoeyenbos, G. H. (1979). Evaluation of organic acids and other compounds as Salmonella antagonists in meat and bone meal. Poult. Sci. 58, 50–54. doi: 10.3382/ps.0580050
Stampi, S., De Luca, G., and Zanetti, F. (2001). Evaluation of the efficiency of peracetic acid in the disinfection of sewage effluents. J. Appl. Microbiol. 91, 833–838. doi: 10.1046/j.1365-2672.2001.01451.x
United States Department of Agriculture, Food Safety Inspection Service. (2003). Use of Chlorine to Treat Poultry Chiller Water. FSIS Notice 45-03. Available online at: http:// www.fsis.usda.gov/OPPDE/rdad/FSISNotices/45-03.htm (Accessed October 25, 2018).
United States Department of Agriculture, Food Safety Inspection Service. (2016). Sanitation Performance Standards Compliance Guide. Available online at: https://www.fsis.usda.gov/wps/portal/fsis/topics/regulatory-compliance/compliance-guides-index/sanitation-performance-standards/sanitation-compliance-guide (Accessed October 30, 2018).
Keywords: sodium bisulfate, Salmonella, reuse water, peracetic acid, poultry processing
Citation: Micciche AC, Feye KM, Rubinelli PM, Lee JA, Knueven CJ and Ricke SC (2019) Comparison of Acid Sanitizers on Salmonella Typhimurium Inoculated Commercial Poultry Processing Reuse Water. Front. Sustain. Food Syst. 2:90. doi: 10.3389/fsufs.2018.00090
Received: 26 October 2018; Accepted: 11 December 2018;
Published: 08 January 2019.
Edited by:
Joshua B. Gurtler, Agricultural Research Service, United States Department of Agriculture, United StatesReviewed by:
Zhinong Yan, Walmart, United KingdomLei Zhang, Neogen, United States
Thomas Matthew Taylor, Texas A&M University, United States
Copyright © 2019 Micciche, Feye, Rubinelli, Lee, Knueven and Ricke. This is an open-access article distributed under the terms of the Creative Commons Attribution License (CC BY). The use, distribution or reproduction in other forums is permitted, provided the original author(s) and the copyright owner(s) are credited and that the original publication in this journal is cited, in accordance with accepted academic practice. No use, distribution or reproduction is permitted which does not comply with these terms.
*Correspondence: Steven C. Ricke, c3JpY2tlQHVhcmsuZWR1