- 1International Center for Tropical Agriculture, Cali, Colombia
- 2French Agricultural Research Centre for International Development (CIRAD), Montpellier, France
- 3Univ Montpellier, Montpellier, France
- 4CGIAR Research Program on Climate Change, Agriculture, and Food Security (CCAFS), Copenhagen, Denmark
- 5French National Agronomic Research Centre (INRA), Montpellier, France
The literature is increasing on how to prioritize climate-smart options with stakeholders but relatively few examples exist on how to co-design climate-smart farming systems with them, in particular with smallholder farmers. This article presents a methodological framework to co-design climate-smart farming systems with local stakeholders (farmers, scientists, NGOs) so that large-scale change can be achieved. This framework is based on the lessons learned during a research project conducted in Honduras and Colombia from 2015 to 2017. Seven phases are suggested to engage a process of co-conception of climate-smart farming systems that might enable implementation at scale: (1) “exploration of the initial situation,” which identifies local stakeholders potentially interested in being involved in the process, existing farming systems, and specific constraints to the implementation of climate-smart agriculture (CSA); (2) “co-definition of an innovation platform,” which defines the structure and the rules of functioning for a platform favoring the involvement of local stakeholders in the process; (3) “shared diagnosis,” which defines the main challenges to be solved by the innovation platform; (4) “identification and ex ante assessment of new farming systems,” which assess the potential performances of solutions prioritized by the members of the innovation platform under CSA pillars; (5) “experimentation,” which tests the prioritized solutions on-farm; (6) “assessment of the co-design process of climate-smart farming systems,” which validates the ability of the process to reach its initial objectives, particularly in terms of new farming systems but also in terms of capacity building; and (7) “definition of strategies for scaling up/out,” which addresses the scaling of the co-design process. For each phase, specific tools or methodologies are used: focus groups, social network analysis, theory of change, life-cycle assessment, and on-farm experiments. Each phase is illustrated with results obtained in Colombia or Honduras.
Introduction
In 2014, the Global Alliance for Climate-Smart Agriculture (GACSA) was launched with the goal of helping 500 million farmers practice climate-smart agriculture (CSA) defined as “agriculture that sustainably increases productivity, enhances resilience (adaptation), reduces/removes GHGs (mitigation) where possible, and enhances achievement of national food security and development goals” (FAO, 2013). Despite the controversies around the meaning of the concept and its lack of theoretical background (Torquebiau et al., 2018), CSA provides the framework within which synergies among adaptation, mitigation, and improved food security for small-scale farmers can be identified, developed, and disseminated. Innovative agricultural systems are needed to find synergies among those three pillars.
Existing studies on the co-design of innovative agricultural systems focus on the development of methods for the design and assessment of farming systems at several levels (Le Gal et al., 2011; Meynard et al., 2012; Prost et al., 2016), from the plot or the herd to the farm or the territory. In such studies, new cropping and livestock systems, combining scientific knowledge with the empirical knowledge of local actors (e.g., men and women farmers, extension services) directly involved, are analyzed and tested. Such studies have shown that the design of innovative agricultural systems has to involve technical, social, and organizational changes and to be analyzed and implemented with stakeholders at multiple levels (Delmotte et al., 2016; Moraine et al., 2016). Participatory mechanisms such as multi-actor innovation platforms associated with exploration tools, such as modeling tools or on-farm experiments, are key in such processes to facilitate mediation and the development of a common language among partners (Dabire et al., 2016). Such multi-actor innovation platforms can be defined as networks intended to strengthen interactions between actors in order to facilitate change that enables innovation (Kilelu et al., 2013). These platforms are virtual, physical, or physico-virtual spaces to learn, jointly conceive, and transform different situations; they are generated by individuals with different origins, different backgrounds, and different interests (Pali and Swaans, 2013).
Thus, the ability of local actors to tackle climate change challenges and mitigate their effects will depend on their ability to innovate and to articulate links among stakeholders while undertaking actions at the local level.
Today, the literature is growing on participatory processes aiming to support climate change policy planning (Rannow et al., 2010; Vervoort et al., 2014; Schroth et al., 2015), with some processes explicitly aiming to promote CSA nationally or locally (Mwongera et al., 2016; Andrieu et al., 2017). However, not many methodological guides exist to co-design climate-smart farming systems with stakeholders.
The purpose of this article is to present a seven-phase methodology to allow family farmers to co-design and adopt CSA options to tackle climate change effects in an open innovation platform. This article is based on the lessons learned during a participatory research conducted in Honduras and Colombia and that was articulated to ongoing research projects in both sites. In these ongoing research projects, groups of farmers, NGOs and research scientists were already working together to sustainably improve their agricultural systems. The article synthetizes and highlight the complementarity between different individual studies conducted from 2015 to 2017 (Acosta-Alba et al., 2019; Howland et al., under review; Osorio-García et al., under review).
After a presentation of the broad methodology, we will show how for each phase, the main results obtained in Colombia and Honduras. We will then discuss the specificity of this process compared with other processes used to design innovative agricultural systems and methodological challenges we found when applying the methodology.
Materials and Methods
Study Site
The research has been conducted in Colombia and Honduras.
Colombia is highly vulnerable to climate change and variability, according to IDEAM (2015), and future climate scenarios suggest an increase in extreme events in frequency and intensity. Such changes in climate might result in a reduction in the yields of subsistence crops (maize, beans, cassava, and plantain) and cash crops, such as coffee and cocoa (CIAT, 2014). Livestock can also be affected by climate change; changes in temperature and rainfall may affect the total production and quality of pastures and forages, thus affecting meat and milk production (BID-CEPAL-DNP., 2014). Particularly in Cauca, climate variability is relatively high according to CDKN, DNP, and DGIS (2013), arguing that the Cauca River watershed is highly deteriorated due to land use management, deforestation, and hydric contamination, among other causes. Considering the latter, efficient use of water is one of the main areas to address in the face of climate change and variability.
Honduras was identified as one of the most vulnerable and affected countries due to climate change between 1996 and 2015 in the global climate risk index (Germanwatch, 2017). Climate change will create further adverse conditions for agricultural production and make maize, bean, and coffee cultivation impossible in many areas. Coffee, which is an important cash crop in the area, will not be able to be produced in 86% of the currently cultivated area (Bouroncle et al., 2015). Anticipated climate change effects by 2050 in Central America will make matters worse, with 5–10% less rainfall and warmer temperatures on the order of 2.0–2.5°C. Further projections predict that rainfall will continue to be erratic and will take more extreme forms (Läderach et al., 2010).
At the time when the research has been conducted, an ethics approval was not required as per our Institution's guidelines and national regulations. However all the steps are based on stakeholders' participation and volunteerism. Consequently, all participants gave oral informed consent.
Description of the Methodology
The methodology comprised seven phases (Figure 1):
1. phase 1: “exploring the status quo and engagement”
2. phase 2: “co-defining the innovation platform”
3. phase 3: “shared diagnosis”
4. phase 4: “identifying solutions”
5. phase 5: “experimenting solutions on the farm.”
6. phase 6: “assessment of the co-design process and disengagement.”
7. phase 7: “strategies for scaling out/up”.
Phase 1
It allowed the identification of an area where the community and/or local stakeholders had an interest in developing practices to tackle climate change effects. Identifying the area was achieved through informal discussions with local actors or stakeholders with links to the area, open meetings, and individual surveys. The first step entailed the identification of an area where existing participatory dynamics were ongoing, including small family farmers (whether individuals or partnerships) and other stakeholders, such as NGOs, public extension services, academia, input suppliers.
This phase also intended identifying the degree of diversity of farmers in the area of intervention to better adapt measures and proposals to the different farmer types (Howland et al., under review). It also aimed to identify the CSA options already known and used by farmers in order to define an appropriate strategy based on their interests. Identifying such practices could also help to define the niche for the operation of the platform that may correspond to new CSA options not seen in the area, existing CSA practices with little adoption, existing practices with efficiency issues, or complementary practices (creating synergies).
Different modalities have been used to identify the diversity of farmers:
- surveys addressed to 40 farms (80 men and women) and
- mobilizing a database of 175 farms (328 men and women) in the case of Colombia (CIAT and IFPRI, 2014).
In both cases, the data used provided details for men and women farmers to be able to consider gender differentiated access to land or perspectives on climate (Table 1). In addition, men and women can have different access to technical information.
Drawing from the collected data, and using XLSTAT, a factor analysis was conducted followed by a cluster analysis applied to the data generated by the factor analysis. In Colombia, for the factor analysis, we considered farmers' perception of climate change, their endogenous adaptation strategy to climate change, their access to technical information and social characteristics (gender, age, level of education) as active variable and adoption of potential CSA practices as supplementary variables. In Honduras, given the agrarian reform that occurred at the study site we used as active variables structural characteristics of the farms (area of the farm, land tenure) and data on adoption of potential CSA practices as supplementary variables.
This first phase also aimed to identify actors already recognized by farmers as support for the adoption of these options and that could be key stakeholders to be included in the platform. It was assumed that not everyone would play the same role in the participatory process and that different potential categories of stakeholders could be identified. Empowered stakeholders are those who take part in defining, implementing, assessing, and promoting the project (i.e., the farmers, but they may include other actors from the study area, such as scientists). Some stakeholders, which we call allies, can be involved in part of the project to work on some aspect(s) of the project (for instance, based on its expertise, an institution in charge of environmental issues). They may come and go throughout the life of the project. Some stakeholders come from within the project environment, who are not those empowered or allies, but can be mobilized at a certain time (local extension services, for instance). The facilitator plays a key role in coordinating the activities of different actors.
The identification of key stakeholders to include in the following phase started by asking to the surveyed farmers who the actors were that provide technical support on the CSA practices already being used. Social network analysis allows visualizing and improving understanding on how individuals of an area are connected by different ties (information on CSA practices, in our case) (Spielman et al., 2011). In our case, it helped us understand what local actor was identified as an important source of information leading to the adoption of CSA practices. In Colombia regarding the number of surveyed farmers, the social network analysis was performed for each type of farmer considering who the information sources were for the adopted practices in order to allow visualizing key actors within networks. In Honduras the social network analysis was conducted for the whole surveyed farmers.
This type of analysis was conducted with Pajek (http://mrvar.fdv.uni-lj.si/pajek/), free software (see manual at http://vlado.fmf.uni-lj.si/pub/networks/pajek/doc/pajekman.pdf).
Phase 2
To co-define the innovation platform, participatory workshops were conducted. In these workshops, members (interested local stakeholders identified in the first phase) agreed on the objectives of the platform (what it means to tackle climate change effects) and how it would operate (who will be the facilitator?, how to work together?, how frequently will members meet?, among other questions).
The main features of the open innovation platform are its ability to mobilize stakeholders and its flexibility to integrate new stakeholders (Nederlof et al., 2011; Kilelu et al., 2013; Pali and Swaans, 2013). For this purpose, these authors have identified different challenges, such as the formalization of a shared “organizational myth” that is a slogan synthesizing the main collective vision, the definition of clear objectives, governance and operating rules.
The definition of objectives took into account the three dimensions of the climate-smart agriculture concept: adaptation, mitigation, and food security, and, on the other hand, the technical, social, and organizational dimensions. The roles of different actor types were identified (Who wants to take part as an empowered stakeholder, ally, or from within the project environment? Who wants to be the facilitator?). One rule of thumb was volunteerism.
General rules were also identified to favor the participation of all, once the objectives were set. They particularly corresponded to the rules of participation in activities and meetings.
Phase 3
To develop a shared diagnosis, platform members characterized the strengths and weaknesses of their farms, the opportunities and barriers, as well as the main challenges they need to overcome in order to define an action plan combining trials, workshops, and exchanges (intra- and extra-area).
Diagnosis was conducted through workshops to lay down more specifically the action plan within the platform and the time frame, according to the objectives set in the previous phase.
One of the aims of this type of research is being able to generate changes in knowledge, attitude, and skills on the part of both stakeholders and the technical team. During this phase, the platform members also defined a monitoring system of expected changes, including knowledge indicators, performance of identified technical solutions, and adoption rate to ensure that the stages of the action plan were achieving the technical and social changes defined (Table 2). It was thus important to include indicators for this purpose, measured before and after the first training activities on key concepts (language standardization).
Phase 4
In the fourth phase platform members defined the technical and organizational options they wanted to test. This phase aimed to prioritize solutions showing themselves to have positive effects on the farm, according to the CSA pillars.
In Colombia, these solutions were prioritized during a workshop based on a vulnerability assessment previously conducted by the NGO under the CCAFS program. In Honduras, as was done to set the objectives, we defined potential technical and organizational solutions related to the three pillars of climate-smart agriculture: adaptation, mitigation, and food security. To define each solution, it was necessary to articulate the propositions of both the technical team and farmers with the results from phase 1, which have identified the CSA practices available in the area under study.
We then conducted an ex ante quantification of the effects they might have on farms in relation to the productivity, adaptation, and mitigation pillars.
Projection tools, such as a CSA performance calculator (including three dimensions: productivity, adaptation, and mitigation) allowed platform members to prioritize relevant solutions they can test under the specific conditions of their farms.
An Excel-based calculator was used in Colombia, which had the purpose of both performing an ex ante evaluation of the effects of innovative practices on CSA pillars at the farm level and serving as a tool for discussion with farmers around changes to be implemented on their farms, under climate change scenarios.
The calculator considers the different farm components (Figure 2), and allows farmers to evaluate the effects of practices prioritized by platform members on the CSA pillars, at the farm level. When entering the data, the calculator conducts some simplified calculations using the parameters summarized in Table 3, which were extracted from the data from the area under study (baseline) and from the literature.
In Honduras, given the lack of modeling skills, the climate-smartness was instead assessed qualitatively, giving a score according to the number of practices addressing one, two, or the three pillars according to the literature on such practices.
Phase 5
The purpose of this phase was testing and adapting the most promising technical solutions to the actual farm conditions in the area. This phase had the following additional objectives:
- Measuring the effects of practices under actual conditions to validate them along with the evaluation methodologies used.
- Understanding the feasibility of the practices.
- Understanding the features of the farms where synergies among the three dimensions of the CSA concept were observed.
Here, the idea was to take into consideration factors not included in previous phases, but that could be key factors in the adoption process, such as the actual time required to apply such a practice and its management.
In this phase, platform members tested new CSA options through participatory mechanisms, such as Farmer Field Schools (FFS).
For comparison purposes, we had at least three or four farmers of the same type experimenting with the same technical solution. To generate knowledge for both the innovation platform members and the technical team and find out whether the practice allowed an effective improvement compared with the conventional practice, it was important to be able to compare the farmers' conventional practice with the new practice (having an experimental plot and a control plot in Honduras or comparing the experimental plot with previous data in Colombia).
Experimental farmers were volunteering platform members representing the diversity of the area (see the classification made in phase 1). The farmers accepted allocating a land plot and their labor to the experimentation, while the technical team participated by purchasing the necessary inputs.
Phase 6
In the sixth phase, the data generated by the monitoring system defined in phase 3 were used to validate the ability of the process to meet the agreed objectives in order to decide whether it was worthwhile to continue with a new cycle of the process (restarting at phase 3). Otherwise, it is assumed that local actors, particularly farmers, have all the tools they need to proceed independently, the technical team can undergo a disengagement process and phase 7 can start making use of the lessons learned to scale out/up the process and reach more farmers
Assessment took place at the end of the action plan defined in phase 3.
Three types of ex post-assessment were conducted:
- Assessment of changes in the knowledge of stakeholders involved in the innovation platform,
- Assessment of the interest of experimental farmers in adopting the changes tried, and
- Assessment of environmental impacts.
The changes in knowledge were measured at the beginning and at the end of the process (Osorio-García et al., under review). The same knowledge surveys conducted at the beginning of the research study were re-run with the same farmers.
Within a multi-year process, it was possible to measure the start of the adoption process. A distinction has been made between the trial phase and the adoption phase. It was considered as adoption at the moment a farmer decided to increase the initial experimental area or to invest his/her own resources to continue implementing the practice. In Honduras, given that the experiments were newer, we instead assessed the opportunities for and barriers to adoption of the improved varieties during two field days on experimental plots with 10 and 22 farmers, respectively, of the communities.
The environmental impact assessment (conducted exclusively in Colombia) of CSA options considered climate change but also other environmental issues (Acosta-Alba et al., 2019). The methodological framework, known as LCA4CSA (life-cycle analysis for climate-smart agriculture), was built upon a life-cycle analysis (LCA) for agricultural systems adapted to the CSA concept.
It was decided to use LCA as it can be applied to link environmental concerns to food security issues (Hayashi et al., 2005). The main advantages of LCA are that (i) it comprises all production steps, from the extraction of raw materials to the farm gate and up to the consumer or even disposal and recycling (cradle to gate or cradle to grave); (ii) it specifies the main role given to the system; (iii) it measures quantitative indicators by impact categories (avoiding the need for grades and facilitating comparison between scenarios or different options to serve the same purpose); (iv) it shows the production steps or processes that contribute the most to each impact category; and (v) it prevents a situation in which one environmental issue is solved while new ones are created (JRC, 2010).
After defining the goal and the system to study, an inventory of all the resources employed and the resulting emissions from manufacturing and using all inputs, feed, and machines as well as all the agricultural and transformation operations was carried out, including transportation, energy, and the extraction of required minerals (Figure 3). Surveys were conducted with 13 farmers to collect specific data on all agricultural operations, as well as the products used on the farms trying the CSA options, describing the amount, origin, and composition. When machinery, buildings, and tools were used, they were also registered, taking into account the hours of use and how many times they were used in a year, as well as the energy consumption (e.g., electric power, gas, oil, heating). Other socioeconomic indicators were also considered, for instance, hours of paid work, costs, and profits. Productivity indicators, such as edible kilocalories, were very useful. Methodological guides, such as the IPCC guidelines 2007 and AGRIBALYSE (Koch and Salou, 2016), were available to undertake the inventory of emissions.
The inventory was then translated into impact indicators associated with the natural environment, human health, and human resources (JRC, 2010). Methods to assess environmental impact are available as software and databases; currently, the most comprehensive are SimaPro and Ecoinvent, respectively (PRE, 2017). The impacts to be assessed, models, and indicators are also described in the ENVIFOOD protocol (Food SCP RT, 2013). Impact categories usually taken into consideration are climate change (greenhouse effect), (stratospheric) ozone depletion, human toxicity, respiratory inorganic compounds, ionizing radiation, photochemical ozone creation (at soil level), acidification (soil and water), eutrophication (soil and water), ecotoxicity, land use, and depletion of resources (mineral, fossil, and renewable energy resources; water).
After a first analysis, the list of impact categories was reduced to focus on the main farmers' environmental concerns. For this purpose, a workshop with 48 platform members allowed the participants to exchange major environmental concerns about the local and regional reality. However, we followed the recommendation to assign at least one impact category for each environmental compartment (air, water, biota, sediments; Fränzle et al., 2012), and maintain those impact categories that are most important to agriculture (global warming, acidification, eutrophication, toxicity, land use, water use, energy use, particles emitted). They are related to the use of pesticides, fertilizers, energy, land, and water resources (Notarnicola et al., 2017). The categories not selected by the stakeholders participating in the platform but having considerable importance were maintained and included in discussions.
The results were used to analyze the different CSA options within the platform and see the changes in the indicators, which show the importance of reducing impacts and/or links among categories (reducing one impact might increase another one). The assessment was carried out at the farm and crop system level to have an overview that implicitly includes possible interactions among components. LCA4CSA also allowed identifying critical points where emissions originated.
Phase 7
The main objective was to link platform outputs with the specific agendas of decision-makers and public administration based on an analysis of public policies and enabling conditions. Such analysis would identify tools (programs, subsidies, incentives, among others) allowing (i) more farmers to adopt CSA options, as defined in the platform (inside and outside the platform's area of intervention), and (ii) to leverage institutional enabling factors and to overcome limiting factors for the adoption of prioritized CSA options in the platform's area of intervention.
This work consequently entailed (1) a local scaling process, which consists of promoting the adoption of CSA options in the territory of the innovation platform, and (2) an expansion process, which consists of the adjustment or creation of an enabling institutional environment at the regional or national level to promote the adoption of CSA options. In this phase, scaling out/up both the open platform and the solutions tested to other territories was considered.
To be able to convince new stakeholders to support scaling out/up the process and solutions, it was necessary to show that phases 1 through 6 achieved relevant technical and social changes (highlighting the importance of the monitoring and evaluation process). Thus, this phase aimed to support an analysis on the trajectory to go from co-designing of CSA farming systems to a large-scale innovation process.
Phase 7 required the involvement of stakeholders, both public and private (producers' organizations, NGOs, companies), that work at a larger scale than the implementers of the innovation platform or researchers/experts with more knowledge and ability regarding institutional and policy analysis (to define the scaling-out/-up strategy). These new stakeholders provided support to the generalization process for the adoption of CSA options in the area of intervention and other areas.
The basic principle of the methods was based on the analysis of stakeholders, as well as an institutional and policy analysis (Grimble and Wellard, 1997; Reed et al., 2009). The basic assumption was that the institutional environment was an important driver of practice adoption by farmers (but not the only one). We focused on formal institutions although some analysis on informal institutions in the climate-smart village in Cauca can be found in Martinez-Baron (2016). The method aimed to identify the institutional potential for and the barriers to a wider adoption of CSA options, from a multi-level approach. To do this, the method consisted of four steps:
- Based on a systematic review of the policy documents at the national level, we mapped the set of policies and instruments affecting the adoption of CSA options. The main difficulty at this point was defining the scope of the policies to be considered. Since CSA covers climate change and productivity issues, the first policy area to take into consideration was climate change policies (nationally determined contribution, national strategy on climate change, adaptation to climate change and/or mitigation plan) with a focus on agricultural practices. The second policy area was agriculture and food security (national strategy for agricultural development, food security plan). However, other policy areas could also have been taken into consideration, such as environmental, water, and social policies. Along with the reviews of policy documents, public stakeholders involved in the implementation of policies and instruments were also identified (ministries, local representations of the ministries), as well as non-government actors (NGOs, unions, donors) who are committed to the adoption of CSA by farmers. We then identified bottlenecks in policy implementation and interventions. This step was based on interviews with stakeholders responsible for policy implementation and providing support to farmers. These interviews aimed at identifying human, financial, and institutional bottlenecks. Special attention has been paid to the assessment of the relationships among actors, with the purpose of identifying synergies and tensions among them and those interventions that could limit the effectiveness and efficiency of policy implementation and support to farmers. A focus was made on the actual implementation of the policy and the intervention at the study sites. The results of these first two steps have been summarized in a policy and intervention matrix that allowed summing up the information and assessing bottlenecks in implementation, as well as synergies and tensions among institutions, which could affect the adoption of potential CSA options (Figure 4). Vertical analysis of this matrix allowed characterizing the implementation of specific instruments. Horizontal analysis of this matrix allowed identifying the synergies and tensions among all the stakeholders and instruments. Then we assessed the lack of services at the local level (for instance, the lack of specific funding options to support the adoption of CSA options). It was based on the information collected in phase 1 regarding key stakeholders providing technical support on CSA practices. This information was complemented during workshops with 30 farmers per site to establish the demand for and supply of services for technical changes tried and validated during phases 5 and 6. This information was useful to establish strategies, especially regarding the development of new local services (to fill gaps) and for better coordination in the provision of services by different stakeholders, as well as the complementarity of the supply of different services enabling the adoption of CSA options.
- Lastly, a strategy to scale out/up was defined and discuss with local actors (to scale out at the local level) and/or national stakeholders (to scale up) proposing possible changes or adjustments of policies that could foster the adoption process. This discussion was held during a workshop to present the results of the analyses.
Implementation of the Methodology
Phase 1: Exploring the Initial Situation and Engagement
The rural area of Cauca, northwest of Popayán in Colombia, and the watershed of Puca in Honduras were selected for the existence of ongoing dynamics. In the first case, farmers supported by an NGO called Fundacion Ecohabitats and scientists were developing the “climate-smart village (CSV)” approach as part of the CGIAR Research Program on Climate Change, Agriculture, and Food Security (CCAFS). In Honduras, the site was selected because most of the farmers were part of local research committees (Classen et al., 2008) supported by an NGO called FIPAH (Fundación para la Investigación Participativa con Agricultores de Honduras).
In the selected study site of Colombia, the factor analysis (the two first factors explaining 53 % of the inertia) followed by the cluster analysis allowed to identify three main types of farmers according to their social characteristics, perceptions, endogenous strategies in relation to climate change, and access to information (Figure 5):
- The adaptive
- The passive
- The skeptics
The first type of farmers (adaptive) included younger men and women in consensual unions who had higher levels of education. They belonged to the departmental federation of coffee growers. In the past, they took note of droughts and they thought that climate change was highly likely to occur and that it would have a strong impact on their farms. To address this concern, half of them reported having implemented changes. The farmers of the second type (passive) have observed drought; they reported that climate change was highly likely to happen and have a strong impact on their farms but few of them declared having implemented any changes. This group was composed mainly of young and old men. Finally, the third type (the skeptics) included mainly young women and old men with a high proportion of them having no education or a primary level of education. They generally did not belong to any farmer group. In general, they have not reported any climate events in the past (although a few reported having been affected by hailstorms) and most of them do not believe in climate change (i.e., they think that it is not very likely to happen) or they think that changes in the climate will not affect their farms.
These classifications suggested developing a differentiated strategy within the platform in the following phases to ensure the adoption of CSA options, according to the farmer profiles.
In the Colombian case, the skeptics used more CSA practices than the other two types, but the frequency of implementation of a given practice was generally higher for the adaptive farmers (Figure 6). The most-implemented practices were mulching (the adaptives and passives) and no burning (the skeptics).
The three types of farmers mentioned an important diversity of actors and sources of information, including producer organizations, NGOs, radio, television, and agrochemical sellers, but the analysis focused on the most frequently mentioned sources, which were UMATA (Unidad Municipal de Asistencia Técnica Agropecuaria), the departmental federation of coffee growers, the family, and neighbors. Comparing the results of the centrality analysis for the three types, the departmental federation of coffee growers was the first actor mentioned in terms of information delivery (Table 4) and thus the most central actor for the three networks.
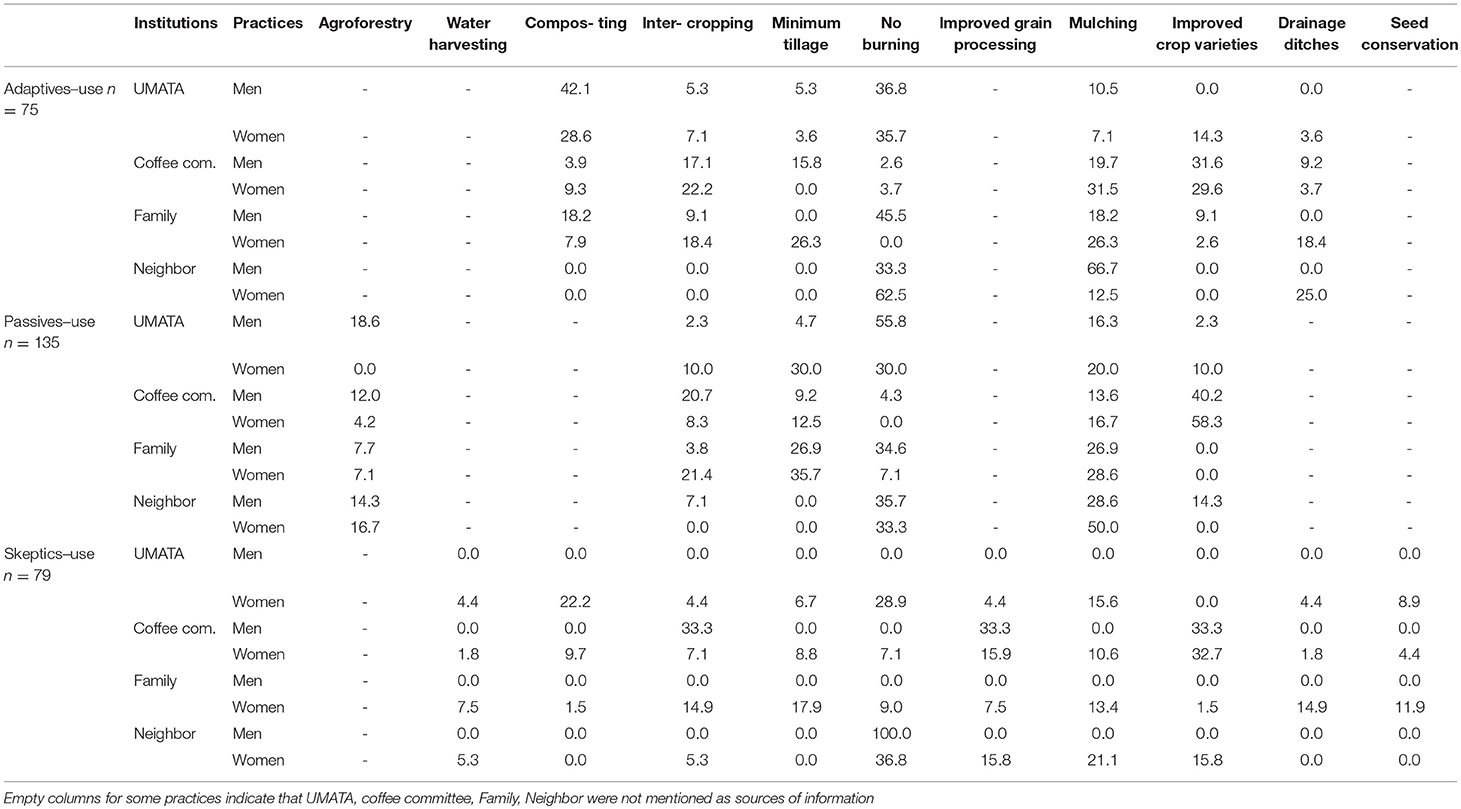
Table 4. Sources of information on potential adaptive practices for the three types of farmers considering the four main actors mentioned by farmers in Colombia (%).
In Honduras, the factor analysis (the two first factors explaining 57% of the inertia) followed by the cluster analysis also allowed to identify three main types of farmers (Figure 5). Farmers of the first type were small farmers, their land belonged to farmer associate enterprises and the agronomic practices they implemented on coffee or maize cropping systems were conventional (use of mineral fertilizers and pesticides). Farmers of the second type presented higher cropping area and were owners of part of their land (the rest belonging to associate enterprises). They used practices with higher mitigation potential (such as soil conservation practices or hedgerows and lower amounts of mineral fertilizers) than the other types. They also had more diversified livelihoods. The land of farmers of type 3 also belonged to associative enterprises but the areas were higher than areas of farmers of type 1 and they had relatively high level of crop diversification.
In Honduras, 15 local organizations were identified (Figure 7). The main local organization was the associated farmer enterprise because families whose land was acquired through the agrarian reform were obliged to be part of it for its use. Other key actors were the water committee giving access to water provided families paid an annual fee, the church, and NGOs and foundations that have been working in the area for several years: ANAFAE (Asociación Nacional para el Fomento de la Agricultura Ecológica), red COMAL (Red de Comercialización Comunitaria Alternativa), CIAL (Comité de Investigación Agrícola Local)1, and PUCA. There also exist some community-consulting structures that have been established by the government, with a lesser number of memberships, such as the forestry consulting group and the patronato (the official local decision-making body).
Phase 2: Co-defining the Innovation Platform
At both study sites, farmers from the different types were the empowered actors, whereas the NGOs were identified as facilitators and the research scientists as key allies. In Colombia, additional allies were the families or the UMATA, whereas in Honduras allies were the directorate of agriculture (DICTA) that promoted technical options at the local level or red Comal, a community network of commercialization of agroecological products. Key actors previously identified such as the departmental federation of coffee growers in Colombia were not directly involved due to the constraints and lack of flexibility to test new practices that have not been previously studied and analyzed by the national federation of coffee growers; they were nevertheless identified as actors of the project environment.
The degree of participation depended on the type of actor (ally, empowered stakeholder, or from within the project environment). Participation required that everyone engaged in activities and meetings was able to provide his/her point of view, testimony, and experience (particularly those marginalized and silent masses), and that everyone could express himself or herself.
These rules also included the frequency of meetings (weekly in Colombia except in the coffee harvesting period), according to the type of actor, how stakeholders would be mobilized for activities and meetings, the type of activities allowed or not allowed within the platforms, how decisions would be made (voting, survey…), how conflicts would be managed, and how stakeholders would be replaced.
The project was summarized in a slogan or what we can define as an “organizational myth” For instance, in Honduras, the myth has been: “The territory of Puca in Gracias innovates to turn climate change into an opportunity.”
The intersection of the dimensions of CSA allowed us to build a goal matrix (Table 5). This matrix was also used to prioritize and make objectives evolve.
Phase 3: Shared Diagnosis
The action plan contained the activities to be carried out in order to achieve the objectives set in phase 2. The same matrix (Table 5) was used, but this time indicating the activities associated with the objectives. It was a multi-year action plan given the type of changes that needed to be tried. This action plan usually included training activities on the issues that need to be developed and more exploratory exercises to prioritize solutions (phase 4), individual trials, field visits (phase 5), mid-term evaluations, and a final assessment (phase 6).
Phase 4: Identifying Solutions
In the case of Colombia solutions such as vegetable home gardens with drip irrigation, compost, or water harvesting tanks were selected with the active role of the NGO and with the assumption that they would help to diversify the production system and strengthen food security of farms dedicated to cash crops.
The calculator was used individually and during a workshop with 60 farmers, choosing volunteers to share the information on their farms and then discussing the results. The calculator was used with support from the scientists. The farmers were able to observe and compare their farms “with” and “without” the solution.
The calculator automatically generated graphs such as the one shown in Figure 8, and these were analyzed with the farmer, referring to the disaggregated data calculated at the crop or animal species level, usually more specific, which can help the farmer to determine the adjustments needed to improve the farm results with respect to the three CSA pillars.
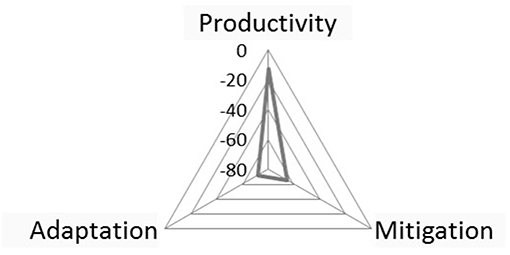
Figure 8. Potential change in climate-smartness of the farm of one volunteer comparing a before and after situation using the practice (%).
Such exercises allowed identifying the potential of combining practices at the farm level. It showed that the practices were mainly addressing adaptation and food security and that addressing the three CSA pillars relied on the substitution of chemical fertilizers with organic fertilizers.
In Honduras, solutions such as improved varieties of bean, sorghum and maize tolerant to drought, biopesticides, and compost to decrease the use of chemical agricultural inputs, and solar dryer for banana sub-products increasingly used in the food were selected by farmers. Here men were mainly interested in improved varieties whereas women were much more interested in the solar dyer to help them support a change in their food pattern.
The climate-smartness was instead assessed qualitatively according to the number of practices addressing the different pillars used on the farm (Table A1). The results discussed in focus groups showed that many farmers already apply an array of CSA practices on their farms such as live barriers, minimal tillage, composting and organic pest management. It was found that the farmers depending on the type of cultivation (for cash or subsistence) had different levels of mitigation adaptation and productivity due to different prioritizations between achieving high levels of agricultural production and ecological sustainability and resilience. The proposed additional practices had the potential to link agricultural production and ecological sustainability.
Phase 5: Experimenting Solutions on the Farm
In Colombia, the prioritized solutions were grouped in “portfolios” to be able to benefit from the aggregated effect of the set of solutions. The diversification of the farm with the introduction of homegardens had implications on labor organization at the farm level and task repartition between men and women given the time dedicated to the management of coffee and sugar cane cropping systems.
In the case of Honduras, the bean Lenca Precoz and maize DICTA Lempira were of special interest for farmers considering criteria such a size of the grain or resistance to fungal infection. For the solar dryer, its introduction implied the creation of collective rules of management by a group of women.
Phase 6: Assessment of the Co-design Process and Disengagement
The assessments of the process highlighted its positive results but also the need to explore new avenues before envisioning a disengagement of the technical team at the study sites.
In Colombia, the survey showed that the proportion of farmers with a low understanding of what climate change (formulated in their own words) decreased significantly (Figure 9).
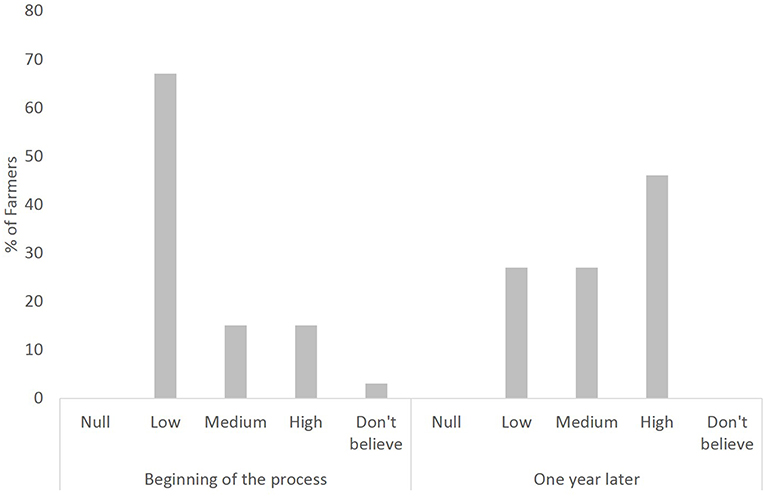
Figure 9. Comparative analysis of farmers' Ability to describe what climate change means for their farm functioning, before and after the participatory process.
We also assessed the adoption by experimental farmers of the tested practices. Not all of the tested practices were new in the study area (see Figure 6) but they were new for the experimental farmers. For these experimental farmers, our assessment showed a positive process of adoption of the practices, particularly compost, and vegetable home gardens since farmers increased the initial experimental area or invested their own resources to continue implementing them (Figure 10).
In Colombia the environmental assessment discussed in the innovation platform allowed to distinguish the impacts of emissions produced on the farm (e.g., technical operations for weeding, fertilizing), as well as the contributions of fertilizers (manufacturing and transportation of those inputs), fuel, and energy used mainly in coffee processing (it includes the production and transportation of those fuels) (Figure 11). It particularly showed that the compost had positive effects on reducing GHG emissions and using water or mineral resources but could have negative effects on other environmental problems such as acidification.
The results obtained by using LCA4CSA highlighted new technical options such as better consideration of animal feeding as a means to decrease greenhouse gas emissions or a better management of the produced organic fertilizer to decrease acidification.
In Honduras, the new knowledge brought by the process was also assessed by the staff of DICTA that mentioned having improved their knowledge on how to conduct a participatory and systemic research even when recognizing their lack of resources (technical skills in some dimensions of the project) for a proper follow-up for some of the activities.
Barriers such as access to some seeds were mentioned, along with opportunities such as the development of an avocado nursery during the project allowing other farmers to have access to the planting material.
Phase 7: Definition of Scaling-Out/-Up Strategies
In Colombia and Honduras, policy and actor analysis showed that many local organizations (farmers' organizations) that were able to provide support to farmers regarding technical advice for CSA practices were not supported by public policies; hence, more support to these organizations could be an efficient leverage strategy to increase farmers' adoption of CSA practices. In both situations, the complementarity of services provided to farmers for the adoption of CSA practices was still a bottleneck. Although technical advice and climate information were under development through public programs, other key services such as credit access or land titling were lagging, thus impeding further and larger adoption of CSA practices. A strategy for upscaling should thus consider filling those gaps, through discussion with the specific institutions in charge of those services. In Colombia specifically, a strategy for upscaling consisted of promoting the innovation platform (embedded in the climate-smart village concept) within the departmental and national policy regarding climate change. At the department scale, the Environmental Authority of Cauca was able to reconcile the implementation of both education and environmental policies through the climate-smart village approach, achieving its goals and implementing policies in other municipalities. At the national scale, the Ministry of Agriculture of Colombia was envisioning the CSV approach as an implementation mechanism of the Climate Change Adaptation Plan and promoting the approach across Latin American countries as the way to implement on the ground adaptation processes in the agricultural sector. For outscaling, the strategy consisted of promoting the innovation platform and CSA approach in surrounding areas with similar agroecological and meteorological conditions. In Honduras, the suggested upscaling strategy was two-fold. First, it consisted of better coordinating the innovation platform dynamic with the existing policies and programs supporting food security, as they were effectively implemented and relied on significant human and financial resources. Second, it consisted of reorienting the existing agricultural program that was actually providing seeds and inputs to farmers without climate considerations toward a CSA-specific input delivery.
Discussion
The Features of the Design Process of Climate-Smart Farming Systems
Many typologies have been made of the processes used to design innovative agricultural systems, according to the availability of the knowledge on the systems to be designed (Hatchuel et al., 2006), the iterative or disruptive characteristics of the process (Meynard et al., 2012), and the farmers' participation and use of modeling tools in these processes (Le Gal et al., 2011). The seven-phase methodology presented here tries to address the specificity of a process intended to design climate-smart farming systems. Such specificity lies mainly in the need to reduce trade-offs among the three pillars of climate-smart agriculture. Such trade-offs may arise at the farm level when prioritizing practices address one pillar and not the others (Torquebiau et al., 2018). They may also arise at different steps of the production and transformation process when good CSA practices are applied but without considering emissions that may occur when transforming such products. These trade-offs may also occur at the agroecosystem level when, for example, the decrease in GHG emissions is made at the expense of other environmental impacts. Particularly in our study, we showed that the compost could have a negative effect on acidification. Colombian soils are rather acid and highly sensitive to acid deposition (Kuylenstierna et al., 2001) leading to soil depletion. Considering such trade-offs implies developing specific analytical tools such as the CSA calculator or the LCS4CSA. In our research, most of the practices identified had adaptation or food security as an entry point, rather than mitigation; however, the identification of co-benefits and synergies between pillars gives smallholder farmers a role to play in emission reduction actions when considering their aggregated contributions (Martinez Baron et al., 2018).
As was shown for agroecosystem conversion (Altieri, 2002), implementing redesigned climate-smart farming systems is a transitional process going from weak to strong changes (Duru et al., 2014). For these authors, supporting such changes relies on a common assessment of the farming system and the socio-ecological system—that considers the ecological system and the actors involved in its management, and the socio-technical systems—at the intersection between niches, regimes, and institutional landscape (Geel, 2002). Our methodology tries to address some of these dimensions considering not only the technical options that can be implemented at the farm scale to improve its climate smartness, but also their impact on the ecological system, the farmers' knowledge changes that need to occur, and the institutional and policy landscape that may support such changes. The platforms and the experiments (on-farm or via the modeling tools) conducted from phases 1–6 are virtual space supporting existing innovation niches. The definition of the scaling-out/-up strategies proposed in phase 7 allows the identification of lock-in situations and of a window of opportunity for these innovation niches.
Strengths and Limitation of the Methodology
Our research tries to fill the knowledge gap on how to make operational the co-design of changes at the local level that address the challenges caused by climate change. It relies on the articulated use of a large array of methodological tools (factor analysis, social network analysis, on-farm experiment, life-cycle assessment, policy mapping) that were necessary given the complexity of co-design processes. It involved a pluri-disciplinary team, particularly in Colombia, where the technical team was made-up of an anthropologist, an economist, an agronomist, and policy and environmental scientists, in this last case with modeling skills. In Honduras, because of the lack of modeling skills, the assessment of the climate-smartness of the farm with the CSA calculator was replaced by an expert assessment based on the literature. In other terms, the level of detail used to implement each phase of the method can be adjusted according to the skills available in a given institution.
In the process, knowledge sharing between farmers and scientists was key, as shown in other local participatory processes. The specific benefit of our process was the involvement of other stakeholders such as NGOs or policymakers, thus allowing a plurality of views. However, it is also its main methodological challenge given that game of power may arise between stakeholders and some of them may use the process to legitimize other personal agenda even after various years of collaboration (e.g., political agenda, access to funds).
One key aspect of the method was the implementation of a monitoring system that helped to identify whether the process was on a good track and whether adjustments in the activities were needed. Such a monitoring system also helps to identify when the disengagement process needs to occur. However, such monitoring system can be heavy regarding the amount and diversity of data collected (here agronomic data were collected but also environmental and social data) in contexts where farmers and even local stakeholders are not used to monitor and document what they are doing. In this study, the disengagement had not yet occurred. Despite the improvement of farmers' knowledge, attitude, and skills, the threshold used to decide on disengagement was not clearly specified. Such a threshold needs to be clarified with the members of the platforms form the beginning in order to not generate frustration, as suggested by Vall et al. (2016).
Conclusions
Co-designing climate-smart farming systems is a multi-dimensional and complex process, in which changes in knowledge, technologies, and in the institutional environment must take place. For these reasons, it is necessary to use a participatory and systems approach such as the one proposed in this guide. The innovation platform is the core of the process. It requires a clear identification of the stakeholders making up the platform, a clarification of their roles, and commonly agreed objectives (i.e., the general operating rules). Generating local and scientific knowledge is a key factor to identify appropriate solutions to tackle climate change, ensure that the process is on the right track, and convince new stakeholders of scaling out/up their results. For these reasons, we proposed to articulate a variety of methodologies for the analysis of key stakeholders, knowledge changes regarding climate change, as well as the results obtained with the practices (in terms of food security, resilience, and greenhouse gas emissions) within an enabling policy environment. The different methodologies were used in the seven complementary phases presented here. This proposal was tested in Honduras and Colombia, but it is not intended as a rigid scheme. It can be adapted to the capacities of the supporters of new platforms and to different contexts.
Author Contributions
Each of the authors has been responsible of the design, implementation, and/or discussion of at least one specific phase of the methodology. NA was responsible of the coordination of the whole methodology and specifically of phases 3 and 4. FH was responsible for the design and implementation of the methodology used in phase 1. IA-A was in charge of the design and implementation of the environmental assessment conducted in phase 6 and J-FL, of the one used in phase 7. AO-G conducted the knowledge and adoption assessment in phase 6 and CG-T of the baseline study conducted in Honduras in phase 1. DM-B and AL supported the institutional analysis in phases 1 and 7. EC developed the method for the phase 2 and gave overall methodological support in both countries.
Conflict of Interest Statement
The authors declare that the research was conducted in the absence of any commercial or financial relationships that could be construed as a potential conflict of interest.
Acknowledgments
This work was funded by the FONTAGRO program (FTG/RF-14837-RG; Contract #80), Agropolis Foundation (Contract #1502-006), and as part of the CGIAR Research Program on Climate Change, Agriculture, and Food Security (CCAFS), which is carried out with support from the CGIAR Trust Fund and through bilateral funding agreements. For details, please visit https://ccafs.cgiar.org/donors. The views expressed in this document cannot be taken to reflect the official opinions of these organizations. CCAFS is led by the International Center for Tropical Agriculture (CIAT).
Footnotes
1. ^Comité Departamental de Cafeteros del Cauca. https://www.federaciondecafeteros.org/static/files/Cauca09.pdf. Accessed on September 10th
References
Acosta-Alba, I., Chia, E., and Andrieu, N. (2019). The LCA4CSA framework: using life cycle assessment to strengthen environmental sustainability analysis of climate smart agriculture options at farm and crop system levels. Agric. Syst. 171, 155–170. doi: 10.1016/j.agsy.2019.02.001
Altieri, M. A. (2002). Agroecology: the science of natural resource management for poor farmers in marginal environment. Agric. Ecosyst. Environ. 93, 1–24. doi: 10.1016/S0167-8809(02)00085-3
Andrieu, N., Sogoba, B., Zougmoré, R. B., Howland, F., Samake, O., Bonilla-Findji, O., et al. (2017). Prioritizing investments for climate-smart agriculture: lessons learned from mali. Agric. Syst. 154, 13–24. doi: 10.1016/j.agsy.2017.02.008
BID-CEPAL-DNP. (2014). “Impactos Económicos del Cambio Climático en Colombia – Síntesis,” in Banco Interamericano de Desarrollo, Monografía No. 221 y Naciones Unidas, LC/L.3851, eds S. Calderón, G. Romero, A. Ordóñez, A. Álvarez, C. Ludeña, L. Sánchez, C. de Miguel, K. Martínezy and M. Pereira, (Washington, DC). Available online at: https://publications.iadb.org/handle/11319/6900
Bouroncle, C., Imbach, P., Läderach, P., Beatriz, R., Medellín, C., Fung, E., et al. (2015). La Agricultura de Honduras y el Cambio Climático: Dónde Están las Prioridades Para la Adaptación? Santiago de Cali: CGIAR.
CDKN, DNP, DGIS. (2013). Agricultura, Vulnerabilidad y Adaptación (AVA): Desarrollo Compatible con el Clima en el Sector Agrícola del Alto Cauca Colombiano. Available online at: http://www.minambiente.gov.co/images/BosquesBiodiversidadyServiciosEcosistemicos/pdf/nodo_pacifico/VISION-AVA-FINAL.pdf
CIAT (2014). Evaluación de la Vulnerabilidad al Cambio Climático de la Agricultura y del Recurso Hídrico en los Andes de Colombia, Ecuador y Perú. Available online at: https://ccafs.cgiar.org/publications/evaluación-de-la-vulnerabilidad-al-cambio-climático-de-la-agricultura-y-del-recurso#.XNLDJ6bgo0o (accessed April 16, 2018).
CIAT and IFPRI (2014). CCAFS Gender Survey – Colombia Climate Smart Village. doi: 10.7910/DVN/28324, Harvard Dataverse, V5, UNF:6:oyz94Y7onUlf1BuIpJQvoQ = = [fileUNF] (accessed April 16, 2018).
Classen, L., Humphries, S., FitzSimons, J., Kaaria, S., Jiménez, J., Sierra, F., et al. (2008). Opening participatory spaces for the most marginal: learning from collective action in the Honduran hillsides. World Dev. 36, 2402–2420. doi: 10.1016/j.worlddev.2008.04.007
Dabire, D., Andrieu, N., Djmen, P., Coulibaly, K., Posthumus, H., Diallo, A. M., et al. (2016). Operationalizing an innovation platform approach for community-based participatory research on conservation agriculture in Burkina Faso. Exp. Agric. 53, 460–479. doi: 10.1017/S0014479716000636
Delmotte, S., Barbier, J.-M., Mouret, J.-C., Le Page, C., Wery, J., Chauvelon, P., et al. (2016). Participatory integrated assessment of scenarios for organic farming at different scales in Camargue, France. Agric. Syst. 143, 147–158. doi: 10.1016/j.agsy.2015.12.009
Duru, M., Fares, M., and Therond, O. (2014). Un cadre conceptuel pour penser maintenant (et organiser demain) la transition agroécologique de l'agriculture dans les territoires. Cah Agric 23, 84–95. doi: 10.1684/agr.2014.0691
FAO (2013). Climate-smart agriculture sourcebook|CCAFS: CGIAR Research Program on Climate Change, Agriculture and Food Security. Availabe online at: https://ccafs.cgiar.org/publications/climate-smart-agriculture-sourcebook#.WaQfGT5JbIU
Food SCP RT (2013). ENVIFOOD Protocol, Environmental Assessment of Food and Drink Protocol. Brussels: European Food Sustainable Consumption and Production Round Table (SCP RT), Working Group 1.
Fränzle, S., Markert, B., and Wünschmann, S. (2012). “The compartments of the environment–structure, function and chemistry,” in Introduction to Environmental Engineering (Weinheim: Wiley-VCH Verlag GmbH & Co. KGaA), 125–196.
Geel, F. W. (2002). Technological transitions as evolutionary reconfiguration processes: a multi-level perspective and a case-study. Res Pol. 31, 1257–1274. doi: 10.1016/S0048-7333(02)00062-8
Germanwatch (2017). Global Climate Risk Index 2017. 32. Available online at: https://germanwatch.org/sites/germanwatch.org/files/publication/16411.pdf (accessed January 12, 2018).
Grimble, R., and Wellard, K. (1997). Stakeholder methodologies in natural resource management: a review of principles, contexts, experiences and opportunities. Agric. Syst. 55,173–193. doi: 10.1016/S0308-521X(97)00006-1
Hatchuel, A., Le Masson, P., and Weil, B. (2006). Les Processus D'innovation. Conception Innovante et Croissance des Entreprises. Paris: Hermès.
Hayashi, K., Gaillard, G., and Nemecek, T. (2005). “Life cycle assessment of agricultural production systems: current issues and future perspectives,” in Proceedings of the International Seminar on Technology Development for Good Agricultural Practice in Asia and Oceania (Tsukuba).
IDEAM, PNUD, MADS, DNP, and CANCILLERÍA. (2015). Nuevos Escenarios de Cambio Climático Para Colombia 2011- 2100 Herramientas Científicas Para La Toma de Decisiones – Enfoque Nacional – Departamental: Tercera Comunicación Nacional de Cambio Climático. Bogota.
IPCC (2007). “Climate change 2007: the physical science basis,” Contribution of Working Group I to the Fourth Assessment Report of the Intergovernmental Panel on Climate Change, eds S, Solomon, D. Qin, M. Manning, Z. Chen, M. Marquis, K. B. Averyt, M. Tignor and H. L., Miller (Cambridge; New York, NY).
JRC (2010). ILCD Handbook. International Reference Life Cycle Data System. Analysis of Existing Environmental Impact Assessment Methodologies for Use in Life Cycle Assessment, 1st Edn. JRC EC.
Kilelu, C. W., Klerkx, L., and Leeuwis, C. (2013). Unravelling the role of innovation platforms in supporting co-evolution of innovation: contributions and tensions in a smallholder dairy development programme. Agric. Syst. 118, 65–77. doi: 10.1016/j.agsy.2013.03.003
Koch, P., and Salou, T. (2016). AGRIBALYSE: Rapport Méthodologique - Version 1.3. ADEME, Angers. Available online at: http://www.ademe.fr/sites/default/files/assets/documents/agribalyse_methodologie_v1_3.pdf
Kuylenstierna, J. C. I., Rodhe, H., Cinderby, S., and Hicks, K. (2001). Acidification in developing countries: ecosystem sensitivity and the critical load approach on a global scale. AMBIO. A. J. Hum. Environ. 30, 20–28. doi: 10.1579/0044-7447-30.1.20
Läderach, P., Haggar, J., Lau, C., Eitzinger, A., Ovalle, O., Baca, M., et al. (2010). Mesoamerican Coffee: Building a Climate Change Adaptation Strategy. CIAT Policy Brief No. 2. Cali: Centro Internacional de Agricultura Tropical (CIAT), 4.
Le Gal, P.-Y., Dugu,é, P., Faure, G., and Novak, S. (2011). How does research address the design of innovative agricultural production systems at the farm level? A Rev. Agric. Syst. 104, 714–728. doi: 10.1016/j.agsy.2011.07.007
Martinez Baron, D., Orjuela, G., Renzoni, G., Loboguerrero Rodríguez, A. M., and Prager, S. (2018). Small-scale farmers in a 1.5oC future: the importance of local social dynamics as an enabling factor for implementation and scaling of climate-smart agriculture. Curr Opin Env Sust. 31, 112–119. doi: 10.1016/j.cosust.2018.02.013
Martinez-Baron, D. (2016). Análisis del cambio institucional en la zona rural noroccidental de Popayán en un contexto de variabilidad climática. Master thesis on Rural Development, Pontificia Javeriana University. Bogotá, Colombia.
Meynard, J.-M., Dedieu, B., and Bos, A.-P. (2012). “Re-design and co-design of farming systems: an overview of methods and practices,” in Farming Systems Research into the 21st Century: The New Dynamic, eds I. Darnhofer, D. Gibbon, and B. Dedieu (Springer), 407–432.
Moraine, M., Grimaldi, J., Murgue, C., Duru, M., and Therond, O. (2016). Co-design and assessment of cropping systems for developing crop-livestock integration at the territory level. Agric. Syst. 147, 87–97. doi: 10.1016/j.agsy.2016.06.002
Mwongera, C., Shikuku, K. M., Winowiecki, L., Twyman, J., Läderach, P., Ampaire, E., et al. (2016). Climate-smart agriculture rapid appraisal (CSA-RA): a tool for prioritizing context-specific climate-smart agriculture technologies. Agric. Syst. 151, 192–203. doi: 10.1016/j.agsy.2016.05.009
Nederlof, S., Wongtschowski, M., and van der Lee, F. (2011). “Putting heads together,” in Agricultural Innovation Platforms in Practice Notes and Queries. Mundie Sal Vol. Bulletin 3, eds F. van der Lee, S, Nederlof, and M, Wongtschowski (Amsterdam: KIT Publishers), 192.
Notarnicola, B., Sala, S., Anton, A., McLaren, S. J., Saouter, E., and Sonesson, U. (2017). The role of life cycle assessment in supporting sustainable agri-food systems: a review of the challenges. J. Cleaner Product. 140, 399–409. doi: 10.1016/j.jclepro.2016.06.071
Pali, P., and Swaans, K. (2013). Guidelines for Innovation Platforms: Facilitation, Monitoring and Evaluation. Nairobi: ILRI Manual 8.
PRE (2017). SimaPro Life Cycle Analysis (software) by PRé Sustainability. Available online at: https://simapro.com/ (accessed May 14, 2018).
Prost, L., Berthet, E. T. A., Cerf, M., Jeuffroy, M.-H., Labatut, J., and Meynard, J.-M. (2016). Innovative design for agriculture in the move towards sustainability: scientific challenges. Res. Eng. Design 28, 119–129. doi: 10.1007/s00163-016-0233-4
Rannow, S., Loibl, W., Greiving, S., Gruehn, D., and Meyer, B. C. (2010). Potential impacts of climate change in Germany—identifying regional priorities for adaptation activities in spatial planning. Landsc. Urban. 98, 160–171. doi: 10.1016/j.landurbplan.2010.08.017
Reed, M. S., Graves, A., Dandy, N., Posthumus, H., Hubacek, K., Morris, J., et al. (2009). Who's in and why? a typology of stakeholder analysis methods for natural resource management. J. Environ. Manage. 90, 1933–1949. doi: 10.1016/j.jenvman.2009.01.001
Schroth, O., Pond, E., and Sheppard, S. (2015). Evaluating presentation formats of local climate change in community planning with regard to process and outcomes. Landsc. Urban Plan. 142:147–158. doi: 10.1016/j.landurbplan.2015.03.011
Spielman, D. J., Davis, K., Negash, M., and Ayele, G. (2011). Rural innovation systems and networks: findings from a study of Ethiopian smallholders. Agri. Hum. Values 28, 195–212. doi: 10.1007/s10460-010-9273-y
Torquebiau, E., Rosenzweig, C., Chatrchyan, A. M., Andrieu, N., and Khosla, R. (2018). Identifying climate-smart agriculture research needs. Cah Agric. 27:26001. doi: 10.1051/cagri/2018010
Vall, E., Chia, E., Blanchard, M., Koutou, M., Coulibaly, K., and Andrieu, N. (2016). La co-conception en partenariat de systèmes agricoles innovants. Cah. Agric. 25:15001. doi: 10.1051/cagri/2016001
Vervoort, J. M., Thornton, P. K., Kristjanson, P., Förch, W, Ericksen, P. J., Kok, K., et al. (2014). Challenges to scenario-guided adaptive action on food security under climate change. Glob. Environ. Chang. 28, 383–394. doi: 10.1016/j.gloenvcha.2014.03.001
Appendix
Keywords: climate change, innovation platform, Latin America, smallholder farmers, participatory research
Citation: Andrieu N, Howland F, Acosta-Alba I, Le Coq J-F, Osorio-Garcia AM, Martinez-Baron D, Gamba-Trimiño C, Loboguerrero AM and Chia E (2019) Co-designing Climate-Smart Farming Systems With Local Stakeholders: A Methodological Framework for Achieving Large-Scale Change. Front. Sustain. Food Syst. 3:37. doi: 10.3389/fsufs.2019.00037
Received: 17 September 2018; Accepted: 30 April 2019;
Published: 24 May 2019.
Edited by:
Mario Herrero, Commonwealth Scientific and Industrial Research Organisation, Brisbane, AustraliaReviewed by:
Eduardo Aguilera, Centro de Estudios e Investigación para la Gestión de Riesgos Agrarios y Medioambientales (CEIGRAM), SpainUday Nidumolu, Commonwealth Scientific and Industrial Research Organisation, Australia
Copyright © 2019 Andrieu, Howland, Acosta-Alba, Le Coq, Osorio-Garcia, Martinez-Baron, Gamba-Trimiño, Loboguerrero and Chia. This is an open-access article distributed under the terms of the Creative Commons Attribution License (CC BY). The use, distribution or reproduction in other forums is permitted, provided the original author(s) and the copyright owner(s) are credited and that the original publication in this journal is cited, in accordance with accepted academic practice. No use, distribution or reproduction is permitted which does not comply with these terms.
*Correspondence: Nadine Andrieu, nadine.andrieu@cirad.fr