- 1Natural Capital Project, Stanford University, Stanford, CA, United States
- 2School of Plant and Environmental Sciences, Virginia Tech, Blacksburg, VA, United States
- 3The Commonwealth Scientific and Industrial Research Organisation (CSIRO), Brisbane, QLD, Australia
- 4Environment and Production Technology Division, International Food Policy Research Institute (IFPRI), Washington, DC, United States
- 5Department of Geography, McGill University, Montreal, QC, Canada
- 6Department of Entomology, University of Wisconsin-Madison, Madison, WI, United States
- 7Department of Entomology and Nematology, University of California, Davis, Davis, CA, United States
- 8Agroecology Group, Department of Crop Sciences, University of Göttingen, Göttingen, Germany
- 9Department of Wildlife, Fish, and Conservation Biology, University of California, Davis, Davis, CA, United States
Despite decades of study, conservation biocontrol via manipulation of landscape elements has not become a mainstream strategy for pest control. Meanwhile, conservation groups and governments rarely consider the impacts of land management on pest control, and growers can even fear that conservation biocontrol strategies may exacerbate pest problems. By finding leverage points among these actors, there may be opportunities to align them to promote more widespread adoption of conservation biological control at the landscape-scale. But are ecologists measuring the right things and presenting the right evidence to enable such alignment? We articulate key concerns of growers, conservation groups, and governments with regards to implementing conservation biological control at the landscape scale and argue that if ecologists want to gain more traction, we need to reconsider what we measure, for what goals, and for which audiences. A wider set of landscape objectives that ecologists should consider in our measurements include risk management for growers and co-benefits of multifunctional landscapes for public actors. Ecologists need to shift our paradigm toward longer-term, dynamic measurements, and build cross-disciplinary understanding with socioeconomic and behavioral sciences, to enable better integration of the objectives of these diverse actors that will be necessary for landscape management for conservation biocontrol to achieve its full potential.
Scientific understanding of the problem of insect pest control is by no means new. We have been aware for decades that, even with pesticides, nearly 20% of our crop production is lost to animal pest damage (Oerke, 2006), with individual insect pest species causing 5–10% losses alone (Esker et al., 2019). The global cost of invasive insects to agriculture is estimated at US$25 billion per year (Bradshaw et al., 2016), and global expenditures on pesticides exceed US$58 billion per year (Chen, 2017). Up to 50% of the control of pest species is thought to be due to natural enemies (Pimentel, 2005), but over-reliance on chemical control has led to pesticide resistance in many pest species (Insecticide Resistance Action Committee, 2017), with consequent outbreaks of previously managed pests [e.g., diamondback moth (Shelton et al., 2000), brown planthoppers (Bottrell and Schoenly, 2012), Colorado potato beetle (Grafius, 1997)]. Meanwhile, insecticides are wreaking havoc in socio-ecological systems. Substantial evidence has demonstrated the severity of threat these chemicals pose to bees (Whitehorn et al., 2012) and other beneficial insects (Geiger et al., 2010), wildlife populations (Köhler and Triebskorn, 2013; Hallmann et al., 2014), the health of farm-workers and rural communities (Guillette et al., 1998; Bouchard et al., 2011), and water supplies (Larson et al., 1997).
An alternative approach is conservation biocontrol: the strategy of managing pests through agricultural practices that enhance the abundance or activity of their natural enemies. While conservation biocontrol is considered the oldest form of pest control, it became a more focused sub-discipline of ecology in the 1960s (Gurr et al., 1998). Traditional approaches to conservation biocontrol focused on enhancing natural enemy populations through local farm management practices such as flower strips and beetle banks (Landis et al., 2000) as well as preferential use of more selective pesticides (Torres and de Bueno, 2018). More recently, scientists have increasingly drawn on principles from landscape ecology to consider biocontrol in the context of habitats surrounding the farm (Tscharntke et al., 2005, 2007). The natural pest control supported by such habitat is a commonly cited example of how nature can benefit people (Millennium Ecosystem Assessment, 2005; Haines-Young and Potschin, 2018). Yet after decades of research, adoption of conservation biocontrol is far from widespread, and incentives for large-scale landscape coordination to support conservation biocontrol efforts are virtually non-existent. Ecologists need to re-examine the ecological information decision-makers would need to know to enable the development of solutions, and whether academic research is delivering it.
Natural pest control is relevant to more actors than ecologists typically acknowledge. Growers are often considered the target stakeholder group, but within this group lies a heterogeneous set of actors whose decisions vary by operation, motivation, and socioeconomic constraints. Furthermore, other key actors like government and conservation groups can help to set policies or incentives that promote or constrain behaviors impacting landscape-level management, on-farm habitat conservation, or development of habitats surrounding farms. While it is important to recognize that natural pest control would only be one of a long list of concerns for all of these actors, in many cases it may not even be on their list, because they are missing important information or information is not being delivered in a way that addresses their core questions and objectives.
Here, we articulate the information needs of key actors that shape agricultural landscapes at two broadly different scales: growers, who are mostly concerned with the local farm scale, and public-facing actors such as conservation organizations and government agencies that operate over the larger landscape or regional scale. We first outline the pest-control objectives and decision-making processes of these different actors, and then consider whether our current measurements address those objectives and what new approaches may be needed to create actionable information about the landscape ecology of pest control.
Grower Objectives for Pest Control
Growers' objectives can be broken down by stage of decisions about pest management. At the adoption stage, growers must decide whether to invest in and rely on conservation biocontrol strategies. Decisions about adoption are most likely determined by objectives related to increasing profitability or reducing risk at reasonable costs, though environmentally conscious farmers may be self-motivated to adopt ecologically-based management practices. Conservation biocontrol is not an all-or-nothing decision, and many growers will continue to use insecticides and other non-biocontrol management tools even if they do adopt conservation strategies. However, the extent to which conservation biocontrol is included in their pest management plans depends on growers' perceptions of how these strategies can enhance the value of their product (e.g., through price premiums), reduce costs, or mitigate risks of future pest outbreaks. Since chemical pesticides are often deemed “cheap,” “quick,” and “easy,” the tradeoffs and relative advantages of conservation biocontrol as compared to alternative options such as pesticide-based methods (and the relative costs of each) must be made clear. This is especially relevant in situations where farmers face multiple challenges in production and marketing and thus only the most important or urgent issues receive attention.
Adoption objectives can be further broken down by type of operation and cropping system. For example, land owners may be more interested in long-term sustainability than renters. Organic farmers need to comply with regulations against synthetic insecticides when controlling outbreaks. Small or direct-market farmers may be most interested in the public-relations and aesthetic values of the conservation biocontrol strategies. Resource-constrained smallholder farmers may be more sensitive to insecticide prices. Often the scale or fragmented nature of the landscape can mean small farms may need shared interest and cooperation of other nearby farmers, whereas large farms may have the privilege of managing entire landscapes and thus may be more self-sufficient and less reliant on cooperation.
Pest management adoption decisions may also be influenced by real or perceived accompanying risks of conservation biocontrol practices. For example, many California produce growers are reluctant to maintain non-crop vegetation around their farm fields because they fear it will promote wildlife that could spread foodborne diseases (Karp et al., 2015a; Baur et al., 2016). In this case, the actual risk of surrounding habitat to food safety is quite low, but the perception of risk is still pervasive in the minds of many growers (Karp et al., 2015b). Of course, in many cases, real trade-offs accompany conservation biocontrol decisions, and natural habitat may not always be expected to provide adequate pest control (Tscharntke et al., 2016; Karp et al., 2018), or may even be a net source of pests (Blitzer et al., 2012). Even when biocontrol is considered successful, small amounts of pest damage may be unavoidable, which presents a high risk for certain fruits and vegetables that may be culled or sold into less profitable markets if they are aesthetically damaged. Better information about what “success” looks like for conservation biocontrol strategies, and under which conditions they are most likely to succeed, will enable growers to base decisions on the real costs and benefits of these approaches.
Once the decision has been made to adopt a conservation biocontrol strategy, the grower moves into the implementation stages. At the design stage, the objective should be to prevent pest problems before they start. At this stage, growers identify what to do, where, and at what spatial scale (farm vs. landscape) to implement conservation biocontrol strategies. The cost-effectiveness of the approach is also paramount. Here, “cost-effective” may indicate the lowest capital expense or opportunity cost (e.g., use of land for crops or habitat management), or lowest intellectual strain amidst many competing demands on a grower's attention. At the intervention stage, the grower makes a decision about when and how to intervene with a pest problem, as part of a larger integrated pest management strategy, of which conservation biocontrol is only one piece. At this stage of the decision, the objective is to contain a pest problem or keep pest populations below an economically relevant threshold. If the grower decides conservation methods are not providing adequate control in a given growing season, other methods such as natural enemy release or insecticides may need to be employed. Finally, many growers may follow up with an evaluation stage to determine whether their conservation biocontrol strategies were effective, and may judge these against such objectives as reducing crop loss, increasing revenues, reducing labor, and reducing cost of the operation overall.
Public Objectives for Landscape Management
In contrast to individual growers, government and conservation groups both tend to have social objectives in mind that often extend across broad spatial scales. Both groups also answer to various actors, including consumers and the public, and may be less concerned about financial outcomes than farmers.
Conservation objectives for land management may be to enhance or expand habitat for biodiversity and/or ecosystem services such as water regulation, carbon sequestration, flood mitigation, erosion control, pollination, and recreation opportunities or other aesthetic benefits. Indeed, these benefits are often the main objectives for many conservation groups. Pest control may be merely considered a possible co-benefit of landscape management through which to engage the cooperation of growers, or a way of disarming opposition to maintaining natural elements in agricultural landscapes based on the perception that “unmanaged” or natural habitats only harbor pests. Increasingly, conservation organizations are also committed to securing local livelihoods in working landscapes (Kareiva, 2014), so strategies that align with growers' incentives are likely favorable.
Governments' objectives depend on the level (e.g., local or national), the region, and policy and regulatory responsibilities, and could be numerous and wide reaching. For example, government objectives may range from very specific, such as minimizing payouts for US federal crop insurance programs by reducing risk of large-scale outbreaks, to broader aims, such as the U.S. Department of Agriculture (USDA) goals of enhancing or maintaining food sovereignty or sustaining rural communities and a viable and productive agricultural industry. One of the main advantages of habitat management relative to other strategies for pest control is that it promotes multi-functionality in agricultural landscapes that can meet many other government objectives. Such additional objectives may include reconnecting flood plains to mitigate flood risk that threatens many highly-productive rural areas, providing opportunities for agritourism and other revenue sources, and creating sense of place and pride in local production (DeClerck et al., 2016). Indeed, some governments are already investing in habitat management in agricultural landscapes to develop and maintain these benefits, via mechanisms such as agri-environmental schemes in the European Union (Kleijn et al., 2006) and conservation cost-share programs supported by USDA's Natural Resources Conservation Service (NRCS).
Both conservation and government groups may also be interested in conservation biocontrol as a way of reducing risks and associated social costs (such as health care) from pesticides. However, even if growers are encouraged by government policies to adopt some conservation biocontrol strategies, they may continue practicing prophylactic pesticide applications (Pingali et al., 1997; Sogawa, 2015) if their belief systems (Palis et al., 2006), knowledge (Chen et al., 2013), or risk preferences (Liu and Huang, 2013) remain unaddressed. In many cases, this will be a critical barrier for large public actors to address, and they could catalyze the evaluation stage of growers' decision processes by incentivizing on-farm effectiveness measurements (e.g., through programs such as Conservation Innovation Grants funded by USDA NRCS). In other cases, growers may already value reduced exposure to toxic pesticides for health reasons (Tago et al., 2014), or they may have to abide by legislation on pesticide drift (e.g., near water and urban areas as mandated by Cotton Australia CRDC, 2014). Some governments are even discouraging pesticide use directly (such as EU IPM legislation mandating priority be given to non-chemical methods for controlling pests; European Parliament, 2009) and imposing pesticide taxes (Lefebvre et al., 2014; Böcker and Finger, 2016). Such programs may be more effective if implemented at a regional scale and if education in alternative pest management strategies such as biocontrol is offered at the same time.
In general, the landscape-scale decisions of government and conservation groups may interact with the more local decisions of growers. Government and conservation group actors can directly affect habitat conservation in agricultural landscapes through zoning, land retirement, easements, conservation cost-share programs or outright purchasing of land, which will influence the level of conservation biocontrol growers experience locally on their farms. Alternatively, these actors can design incentive programs for individual growers to adopt conservation biocontrol strategies that aggregate up at the landscape scale to create the co-benefits that meet broader social objectives. Incentives for individual growers could be established by conservation groups that help set up compensation schemes to reward growers who provide conservation benefits (such as Water Funds pioneered by the Nature Conservancy; Vogl et al., 2017). They could also be established by government agencies that set policies such as pesticide taxes, farm subsidies, tax credits, payments for ecosystem services or other policies that together shape the enabling (or disabling) conditions for growers to adopt habitat management as a strategy for sustainable pest control. Agglomeration payments (bonus payments for adoption by neighboring farms) may help to encourage both compliance with the program they promote as well as the overall diffusion of the program across rural contexts (Bell et al., 2016). In each case, the habitat management has implications for the success of conservation biocontrol, but each of the three actors will take different actions, at different spatial scales to achieve their objectives, requiring different measures to inform implementation and evaluate impact.
Consumers, buyers, and other stakeholders in the broader food system can influence the goals and objectives of growers, government, and conservation groups. General concerns about food safety, animal welfare, farm worker welfare, clean water, and conservation may either enable or impede conservation biocontrol. For example, as outlined above, it is difficult for California produce growers to maintain natural vegetation in or around their farm fields because buyers often express deep concerns that wildlife may move onto farm fields and spread foodborne diseases (Karp et al., 2015a). Consumers and the general public may also act as constituents to influence legislation and regulation, or to exert pressure on growers through their market demand for certain attributes of agricultural products (e.g., organic products, fair trade-certified products).
Given that the objectives and motivations associated with conservation differ across stakeholders, what does that mean for the approaches and contribution of the research community?
Ecologists Are Not Measuring the Right Endpoints to Support Decisions on Conservation Biocontrol
Over the past decade, there has been a significant investment by ecologists in farm and landscape-level studies that focus on testing the broad hypothesis that conserving or restoring habitat within farms or the surrounding landscape can increase biological control. Indeed, a variety of local farm management practices like planting polycultures, intercrops, and flower strips have been shown to bolster enemy abundance and richness, contributing to increased pest control (Letourneau et al., 2011; Iverson et al., 2014; Gurr et al., 2016b; Lichtenberg et al., 2017). The vast majority of landscape-level studies have focused on enemy and pest abundance with some evidence that landscape complexity increases enemy abundance and pest predation rates, but mixed results for the hypothesis that surrounding habitats decrease pest abundance (Bianchi et al., 2006; Chaplin-Kramer et al., 2011; Lichtenberg et al., 2017). The most comprehensive review on this literature to date goes even further to demonstrate that there is no consistent response of pest or enemy abundances to landscape composition alone (Karp et al., 2018). Likewise, when attempting to communicate economic value, studies focus on yields and measure yield in a wide variety of ways such as total yield, marketable yield, or fruit set– with no great evidence for a consistent benefit from landscape complexity. This does not mean that there are no benefits of conserving habitat at a landscape scale—rather, we argue that the benefits may not be consistently expressed in the measurements we have chosen, and more of the same measurements are unlikely to strengthen the evidence base.
Critical questions arise when we consider the appropriateness of measuring insect abundances and yield to assess the effectiveness of conservation biological control techniques. First, are these measures credible, or even fair? Landscape studies of pest control may not demonstrate clear effects on crop yields because so many other factors affecting yields are difficult if not impossible to control for in field-based observation studies. It is easy to imagine that a variety of confounding factors such as soil, climate, inputs, and labor could be masking pest-control-induced effects on crop yields. For example, if natural habitat tends to remain undeveloped only near lands that are more marginal for agriculture, then areas where agriculture is surrounded by natural habitat could be intrinsically less productive (for example, due to poor soils, or steep slopes). We would need massive restoration experiments in highly productive and, consequently, currently highly simplified agricultural systems like the California Central Valley or Midwestern US to fairly test the hypothesis that diverse landscapes can lead to increased crop yields. Likewise, for insect abundances, a greater number of pests in one location than another is not necessarily an indication that natural pest control is functioning less well in one place than the other. Pests might have been even worse without natural habitat for reasons, similar to the yield argument, such as differences in climate, soil or intrinsic productivity between the diverse and simple fields that were sampled. Pests and enemies may also exhibit oscillatory population dynamics, making short-term measurements of population abundances inadequate to detect the longer-term effects of natural habitat on pest population sizes (Levins and Schultz, 1996).
A second question to consider about conservation biocontrol research is whether measures of pest abundance and crop yield are the most relevant. For many crops, quality is more important than quantity (e.g., stone-fruit, grapes, tomatoes; Gunders, 2012), so measuring total yield in terms of weight or number of fruits per plant or per unit area may not be as relevant as marketable yield, even if confounding variables could be controlled. Pest abundance measures likewise may not resonate with growers, because what concerns them most is not the pests themselves but the damage they cause, especially for pests and natural enemies that are cryptic or difficult to identify. Rootworm beetles, for example, live underground and are therefore all but invisible, yet the damage they cause is readily apparent. Moreover, the snapshot abundances or yields measured in a typical ecological study cannot provide insight into the stability of pest control that concerns most growers and many government actors.
In general, many of the growers' objectives—profitability, minimizing risk—are not fully addressed through the two common measures of conservation biocontrol success, yields and insect abundances. The objectives of conservation groups and government—the potential for co-benefits, likelihood of the need for pesticide application, and long-term sustainability—are also not adequately addressed by these snapshot metrics.
A New Research Agenda for Conservation Biocontrol
How can we build on the wealth of information about conservation biocontrol at the farm and landscape scale to sharpen our ecological evidence so that it better links to stakeholder interests for enhanced adoption and implementation? A first step is to consider the alignment of the objectives and endpoints for different actors in agricultural landscapes and the different stages of decisions. This will require building cross-disciplinary understanding for how to integrate this ecological information into socioeconomic and behavioral science to influence decisions on conservation biocontrol and adoption behavior. Once this is identified and agreed, ecologists need to rethink our measurements to meet these needs. The credibility and relevance of information we are providing, called into question above, is critical to ensuring the use of that knowledge (Cash et al., 2003). We propose some possible metrics to meet these criteria here.
For growers (Table 1A), pest abundance may be most relevant to measure at the intervention stage (and Pest Control Advisers hired by many growers already do this), not at the adoption or design stage. Yields may be most important to measure in evaluation stage, but it is important for ecologists to recognize that this covers only part of the objectives—increased revenue could come from enhanced quality as much as or more than quantity (Klatt et al., 2014). Overall operating costs (such as through reduced frequency of insecticide application) to achieve those yields should also be tracked, and traditional economic methods such as cost-benefit analysis can be a helpful tool to elucidate this for growers (Gurr et al., 2016a). Moreover, accounting for the many confounding factors impacting yields is essential whenever attributing any differences to conservation biocontrol, which may require large scale manipulative experiments to adequately address, or the use of potted plants to control for soil, variety, planting dates, and other in situ variables outside of the researcher's control.
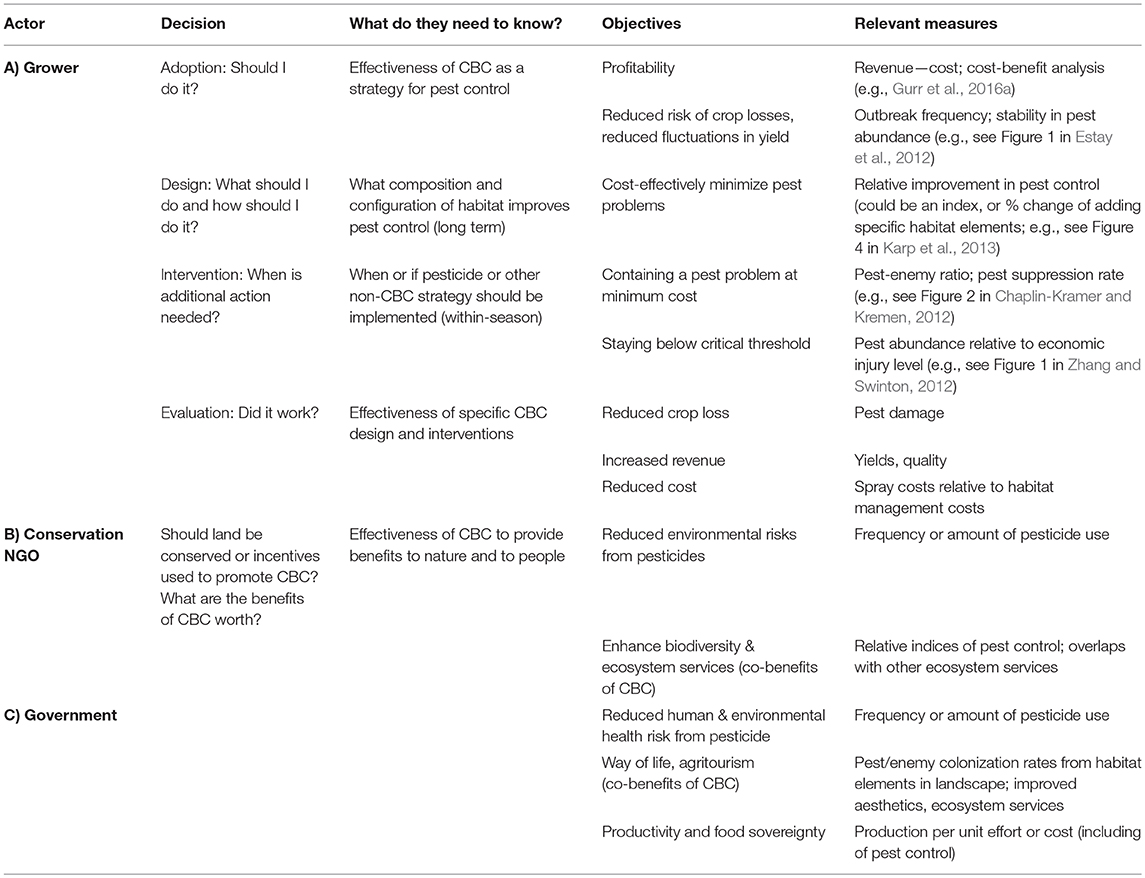
Table 1. Description of conservation biocontrol (CBC) measures to address the objectives of decision-makers in agricultural landscapes.
For the design stage of decisions about conservation biocontrol, information should be more focused on pest suppression rather than mere abundances, and encouragingly, evidence is mounting for the role of natural habitat in suppressing pest population growth rates, for example in cereal aphids (Thies et al., 2011; Rusch et al., 2016). Scientists hoping to inform conservation biocontrol design should increasingly prioritize such measures of the functional attributes of pest control, across more taxonomic groups (beyond aphids that currently dominate such experimental approaches) and longer time-frames (capturing more of the growing season than the week or two that are typical of field-based predator-exclusion studies). This is not to say that highly complex information is the only type that is useful. In fact, adoption is more likely if the information or rule of thumb is simple. Designing conservation biocontrol interventions at the farm or landscape scale may not require absolute measures of pest control, but instead could benefit even from relative indices of where habitat management could make the most difference (Karp et al., 2013).
To the extent that risk reduction is a major objective of growers, we need much longer-term studies, likely over much broader scales, to detect and model rare events like major pest outbreaks and to identify conditions under which conservation biocontrol may fail. The importance of habitat in this case is long-term strategic prevention, as opposed to tactical within-season management. Evidence across longer time scales is needed to demonstrate effects on the stability of pest control, a critical consideration for decision-makers to adequately address the potential tradeoff between short-term losses and long-term gains.
We also need more cross-scale work to help identify where interventions that can be managed by individual farmers (beetle banks, flower strips, etc.) can provide benefit alone and where they first require investments in creating adequate habitat at a landscape scale (Schellhorn et al., 2015). The vast majority of studies consider habitat at either a local or a landscape scale, but the few that integrate the two suggest a certain amount of substitutability may exist (with local complexity compensating for landscape homogenization or vice versa; Tscharntke et al., 2007; Chaplin-Kramer and Kremen, 2012). However, how much habitat is needed at one scale to support the function of the habitat at another scale is not well-understood. Furthermore, pesticide application at the landscape level should be taken into account in such cross-scale studies, to test the extent to which chemical suppression of natural enemy densities may be interfering with potential local benefits of pest control provision from natural habitat (Gagic et al., 2019). In fact, land-use intensity (including higher insecticide use) may be an even more important driver of landscape effects than resource availability (Jonsson et al., 2012).
For conservation groups and governments (Tables 1B,C), mapping out win-wins between pest control, other ecosystem services, and biodiversity resulting from habitat management may also only require relative indices for pest control to show where the value is likely to be highest. For governments in particular (Table 1C), quality of life or agritourism potential could also be addressed in pest control studies through qualitative measures of countryside aesthetic (voter satisfaction, farmer surveys). Understanding the consequences of those measures for pest control may need to be more quantitative, at least in terms of addressing the perception that pests come from habitat through documentation of colonization rates. As discussed for growers' risk-reduction objectives, large-scale and long-term measures of frequency of outbreaks would be needed to address impacts of a strategy on the stability of production, which would impact government concerns like crop insurance pay-outs or food sovereignty.
Measurements on associated reductions in chemical pesticides and resulting increases in ecosystem services should also be considered. Taking an example from successful local-scale conservation biocontrol programs, a multi-country field experiment quantified impacts of floral strips not just on rice pest abundances and crop yields but also on pesticide spray frequencies (Gurr et al., 2016a). As a result, the study's findings were highly relevant to growers (as floral strips were shown to be cost-effective), as well as to governments and conservationists (as floral strips reduced pesticide sprays). The co-benefits of such interventions can be substantial. Participatory programs and mass media campaigns in Vietnam, showcasing how floral strips support biocontrol, pollination and landscape aesthetics alike, have been very successful at garnering public support (Westphal et al., 2015). Such campaigns could be even more important at the landscape scale, to generate the type of collective action needed.
For both conservation groups and government, understanding the potential for reductions in pesticide use resulting from conservation biocontrol requires more than just ecology. To tackle this important aspect of pest control, ecologists will need to work with behavioral scientists to better understand determinants of grower decisions to spray. Large-scale correlative studies between landscape complexity and pesticide use may be informative, but also potentially misleading because grower behavior (and response to the same pest problem) may differ across landscape complexity gradients as well (Meehan et al., 2011; Larsen, 2013; Larsen et al., 2015).
Operationalization Challenges and a Path Forward
Even with the right measures, it can be challenging to integrate ecological information for such complex systems as crop-pest-enemy food webs into any decision. There are considerable limitations to our current understanding of the ecology of pest-control approaches, highlighted by the fact that generalities are so hard to find (Karp et al., 2018). Many studies show conflicting answers to the same questions about the pest-control responses to local and landscape habitat dynamics, and there are many conditions under which natural habitat may fail to provide pest control (e.g., if habitat is more favorable for pests than enemies, crops provide better resources than habitat, enemies are not effective control agents; Tscharntke et al., 2016). We need to communicate these limitations, as well as the risks and challenges posed by adoption of conservation biocontrol, in addition to its benefits. We need to be clear about when we expect it to work, and when we do not, or ecologists will lose credibility with decision-makers who find a mismatch between scientific reports and their own observations or perceptions. It is unrealistic to expect that we can offer one-size-fits-all recommendations, but rather, the evidence will likely have to be tailored to specific systems. While it can be frustrating when successes in one context cannot easily be transferred to another, there are systems that are already well studied (e.g., aphids; Rusch et al., 2016) and serve as an excellent starting place to reframe ecological study to better meet decision needs.
We also recognize the challenge in obtaining the long-term and large-scale datasets we have called for here, as well as the consistency in funding to support such research. Ecologists must form new alliances to deliver information tailored to the scale of the decision and to the context of other enabling conditions for successful implementation (Cease et al., 2015). Advancing the science at these larger scales will require partnerships to extend measures beyond the spatial and temporal scales typical of academic research projects. Academics could partner with governments already collecting long-term datasets to be able to ask questions over the right scales. These include the USDA's own Risk Management Agency's “Cause of Loss” dataset that identifies when pest outbreaks were severe enough to trigger crop insurance claims (USDA RMA, 2018), as well as several massive datasets on pest abundance and damage gathered by the Ministries of Agriculture in China (Lu et al., 2010, 2012; Zhang et al., 2018) and Spain (Paredes et al., 2015). New ecoinformatic approaches are being developed to analyze such datasets (Rosenheim and Gratton, 2017). Long-term spatial informatics will be a key research frontier to secure more reliable pest control services and more sustainable pest management for agriculture.
Still, information is never the only limiting factor in changing perceptions and behavior, and often not even the most important one. Even if credible and relevant evidence is assembled across the large spatial and temporal scales described above, success for conservation biocontrol at the landscape scale will depend on whether an institution exists that can help coordinate growers and incentivize behaviors that change the entire landscape. There are many other social and institutional aspects influencing decisions and equally important research questions to investigate change on those fronts—how do we align interests for collective action and community-level institutions to remove barriers to cooperation? What behavioral factors (like risk aversion, cultural evolution) drive decisions, sometimes in a more fundamental way than economic factors? How can we move from seeing food as a commodity to something more encompassing, capable of securing cultural values, livelihood, equity, justice, health? Mainstream adoption of more sustainable means of pest control demands nothing short of a paradigm shift in research and practice, requiring different communities (development, conservation, health) to work together more to seek solutions for shared challenges. While we respect that the magnitude of this problem extends well beyond ecology, ecologists can take a first step toward engaging in this way, by making more deliberate measures that better reflect what people care about.
Essentially, while ecologists, economists, and other scientists need to improve and re-focus on what we measure, improved measurements within disciplines is not enough. Breaking down disciplinary silos is necessary to ensure that ecological evidence is expressed in forms that allow linkage or integration with other fields. For example, the majority of economic threshold decision rules do not explicitly take into account the potential of biological control or the probability distribution of the biocontrol level (Hamilton et al., 2004; Zhang and Swinton, 2009); more practical decision support would integrate ecological and economic information. While departmental divisions at universities may hinder such integration, these are increasingly being broken down by interdisciplinary institutes at leading universities, which cut across many programs and departments. And while supporting interdisciplinary work has traditionally been challenging, new funding mechanisms like NSF Coupled Human-Natural Systems program and conveners like SESYNC are changing this. The study of pest control should benefit enormously from multi-disciplinary teams taking advantage of such opportunities. When ecological information is well-integrated with social and behavioral understanding, obstacles to incorporating conservation biocontrol approaches can be overcome (Salliou et al., 2019). However, scientists also need to step out of the academy altogether to engage deeply with the end users experiencing the problem and with whom we ultimately want to craft an ecological solution. This could be facilitated by shifts in academic systems to align career incentives with real-world problem-solving outcomes rather than merely publications for the scientific community. The emergence of boundary organizations that sit at the interface of research innovation and practical decision-support, often within universities, is another promising avenue for enhancing actionability of academic science (Keeler et al., 2017).
We have shown here how much evidence for conservation biocontrol is currently lacking, especially for adoption and design stages of grower decisions, and for better engaging the organizations that create incentives in agricultural landscapes. Proponents of conservation biocontrol are missing key opportunities for alignment by not directly addressing conservation and government audiences and their objectives. Despite these complexities and the lack of progress toward adoption after several decades of conservation biocontrol research, we believe ecologists are up to the challenge of reforming what and how we measure pest control to better inform decisions in agricultural landscapes. Many of our proposed measures require thinking beyond ecology—pest control is an interdisciplinary problem, and we need to integrate more social science like economics and behavior into our understanding of these systems. Stronger partnerships between academic and government scientists could create opportunities for novel uses of existing data and also ensure higher relevance of information to government actors. Closer connections between ecologists and farmers, which can be facilitated by re-investing in agricultural extension organizations, can also begin to bridge understanding between these stakeholders. The field of conservation biocontrol has grown considerably over the past decade, with many bright young scholars dedicating themselves to the promise of more sustainable agriculture through habitat management. It's time now to pursue a new research agenda for conservation biocontrol to deliver on that promise.
Author Contributions
All authors listed have made a substantial, direct and intellectual contribution to the work, and approved it for publication.
Funding
This work was supported by the National Socio-Environmental Synthesis Center (SESYNC) under funding received from the National Science Foundation DBI-1052875.
Conflict of Interest Statement
The authors declare that the research was conducted in the absence of any commercial or financial relationships that could be construed as a potential conflict of interest.
Acknowledgments
We thank the whole Pest Control Tools venture team, whose rich discussions led to the formulation of this paper: Fabrice DeClerck, Heather Grab, Lauren Hunt, Mattias Jonsson, Ashley Larsen, Emily Martin, Alejandra Martínez-Salinas, Tim Meehan, Katja Poveda, Adrien Rusch, and Steve Wratten.
References
Baur, P., Driscoll, L., Gennet, S., and Karp, D. (2016). Inconsistent food safety pressures complicate environmental conservation for California produce growers. California Agricult. 70, 142–151. doi: 10.3733/ca.2016a0006
Bell, A., Parkhurst, G., Droppelmann, K., and Benton, T. G. (2016). Scaling up pro-environmental agricultural practice using agglomeration payments: proof of concept from an agent-based model. Ecol. Econ. 126, 32–41. doi: 10.1016/j.ecolecon.2016.03.002
Bianchi, F. J. J. A., Booij, C. J. H., and Tscharntke, T. (2006). Sustainable pest regulation in agricultural landscapes: a review on landscape composition, biodiversity and natural pest control. Proc. R. Soc. B-Biol. Sci. 273, 1715–1727. doi: 10.1098/rspb.2006.3530
Blitzer, E. J., Dormann, C. F., Holzschuh, A., Klein, A.-M., Rand, T. A., Tscharntke, T., et al. (2012). Spillover of functionally important organisms between managed and natural habitats. Agric. Ecosyst. Environment. 146, 34–43. doi: 10.1016/j.agee.2011.09.005
Böcker, T., and Finger, R. (2016). European pesticide tax schemes in comparison: an analysis of experiences and developments. Sustainability 8, 1–22. doi: 10.3390/su8040378
Bottrell, D. G., and Schoenly, K. G. (2012). Resurrecting the ghost of green revolutions past: the brown planthopper as a recurring threat to high-yielding rice production in tropical Asia. J. Asia Pac. Entomol. 15, 122–140. doi: 10.1016/j.aspen.2011.09.004
Bouchard, M. F., Chevrier, J., Harley, K. G., Kogut, K., Vedar, M., Calderon, N., et al. (2011). Prenatal exposure to organophosphate pesticides and IQ in 7-year-old children. Environ. Health Perspect. 119, 1189–1195. doi: 10.1289/ehp.1003185
Bradshaw, C. J. A., Leroy, B., Bellard, C., Roiz, D., Albert, C., Fournier, A., et al. (2016). Massive yet grossly underestimated global costs of invasive insects. Nat. Commun. 7:12986. doi: 10.1038/ncomms12986
Cash, D. W., Clark, W. C., Alcock, F., Dickson, N. M., Eckley, N., Guston, D. H., et al. (2003). Knowledge systems for sustainable development. Proc. Nat. Acad. Sci. U.S.A. 100, 8086–8091. doi: 10.1073/pnas.1231332100
Cease, A. J., Elser, J. J., Fenichel, E. P., Hadrich, J. C., Harrison, J. F., Robinson, B., et al. (2015). Living with locusts: Connecting soil nitrogen, locust outbreaks, livelihoods, and livestock markets. Bioscience 65, 551–558. doi: 10.1093/biosci/biv048
Chaplin-Kramer, R., and Kremen, C. (2012). Pest control experiments show benefits of complexity at landscape and local scales. Ecol. Appl. 22, 1936–1948. doi: 10.1890/11-1844.1
Chaplin-Kramer, R., O'Rourke, M. E., Blitzer, E. J., and Kremen, C. (2011). A meta-analysis of crop pest and natural enemy response to landscape complexity. Ecol. Lett. 14, 922–932. doi: 10.1111/j.1461-0248.2011.01642.x
Chen, J. (2017). Global Markets for Biopesticides. Report Code CHM029F. BCC Research. Available online at: https://www.bccresearch.com/market-research/chemicals/biopesticides-global-markets-report-chm029f.html (accessed 19 July, 2019).
Chen, R., Huang, J., and Qiao, F. (2013). Farmers' knowledge on pest management and pesticide use in Bt cotton production in china. China Econ. Rev. 27, 15–24. doi: 10.1016/j.chieco.2013.07.004
Cotton Australia CRDC (2014). Australian Grown Cotton Sustainability Report. Available online at: http://crdc.com.au/publications/australian-grown-cotton-sustainability-report (accessed 19 July, 2019).
DeClerck, F., Jones, S., Attwood, S., Bossio, D., Girvetz, E., Chaplin-Kramer, B., et al. (2016). Agricultural ecosystems and their services: the vanguard of sustainability? Curr. Opin. Environ. Sustainabil. 23, 92–99. doi: 10.1016/j.cosust.2016.11.016
Esker, P., Nelson, A., Pethybridge, S. J., McRoberts, N., Willocquet, L., and Savary, S. (2019). The global burden of pathogens and pests on major food crops. Nat. Ecol. Evol. 3, 430–439. doi: 10.1038/s41559-018-0793-y
Estay, S. A., Lima, M., Labra, F. A., and Harrington, R. (2012). Increased outbreak frequency associated with changes in the dynamic behaviour of populations of two aphid species. Oikos 121, 614–622. doi: 10.1111/j.1600-0706.2011.19525.x
European Parliament (2009). Directive 2009/128/EC of the European Parliament and of the Council of 21 October 2009 establishing a framework for Community action to achieve the sustainable use of pesticides. J. Eur. Union 309, 71–86.
Gagic, V., Hulthen, A. D., Marcora, A., Wang, X., Jones, L., and Schellhorn, N. A. (2019). Biocontrol in insecticide sprayed crops does not benefit from semi-natural habitats and recovers slowly after spraying. J. Appl. Ecol. doi: 10.1111/1365-2664.13452 [Epub ahead of print].
Geiger, F., Bengtsson, J., Berendse, F., Weisser, W. W., Emmerson, M., Morales, M. B., et al. (2010). Persistent negative effects of pesticides on biodiversity and biological control potential on European farmland. Basic Appl. Ecol. 11, 97–105. doi: 10.1016/j.baae.2009.12.001
Grafius, E. (1997). Economic impact of insecticide resistance in the Colorado potato beetle (Coleoptera: Chrysomelidae) on the Michigan potato industry. J. Econ. Entomol. 90, 1144–1151. doi: 10.1093/jee/90.5.1144
Guillette, E. A., Meza, M. M., Aquilar, M. G., Soto, A. D., and Garcia, I. E. (1998). An anthropological approach to the evaluation of preschool children exposed to pesticides in Mexico. Environ. Health Perspect. 106, 347–353. doi: 10.1289/ehp.98106347
Gunders, D. (2012). Wasted: How America Is Losing up to 40 Percent of Its Food from Farm to Fork to Landfill. NRDC Issue Paper: 12-06-B.
Gurr, G. M., Lu, Z., Zheng, X., Xu, H., Zhu, P., Chen, G., et al. (2016a). Multi-country evidence that crop diversification promotes ecological intensification of agriculture. Nat. Plants 2:16014. doi: 10.1038/nplants.2016.14
Gurr, G. M., van Emden, H. F., and Wratten, S. (1998). “Habitat manipulation and natural enemy efficiency: implications for control of pests,” in Conservation Biological Control, eds P. Barbosa (Academic Press, San Diego), 155–183 doi: 10.1016/B978-012078147-8/50055-4
Gurr, G. M., Wratten, S. D., Landis, D. A., and You, M. (2016b). Habitat management to suppress pest populations: progress and prospects. Ann. Rev. Entomol. 62, 91–109. doi: 10.1146/annurev-ento-031616-035050
Haines-Young, R., and Potschin, M. B. (2018). Common International Classification of Ecosystem Services (CICES) V5.1 and Guidance on the Application of the Revised Structure. Available online at: www.cices.eu (accessed 19 July, 2019).
Hallmann, C. A., Foppen, R. P. B., van Turnhout, C. A. M., de Kroon, H., and Jongejans, E. (2014). Declines in insectivorous birds are associated with high neonicotinoid concentrations. Nature 511, 341–343. doi: 10.1038/nature13531
Hamilton, A. J., Schellhorn, N. A., Endersby, N. M., Ridland, P. M., Ward, S. A., et al. (2004). A dynamic binomial sequential sampling plan for Plutella xylostella (Lepidoptera: Plutellidae) on broccoli and cauliflower in Australia. J. Econ. Entomol. 97, 127–135. doi: 10.1093/jee/97.1.127
Insecticide Resistance Action Committee (2017). Pest Database. IRAC. Available online at: http://www.irac-online.org/pests (accessed 19 July, 2019).
Iverson, A. L., Marin, L. E., Ennis, K. K., Gonthier, D. J., Connor-Barrie, B. T., Remfert, J. L., et al. (2014). Do polycultures promote win-wins or trade-offs in agricultural ecosystem services? a meta-analysis. J. Appl. Ecol. 51, 1593–1602. doi: 10.1111/1365-2664.12334
Jonsson, M., Buckley, H. L., Case, B. S., Wratten, S. D., Hale, R. J., and Didham, R. (2012). Agricultural intensification drives landscape-context effects on host-parasitoid interactions in agroecosystems. J. Appl. Ecol. 49, 706–714. doi: 10.1111/j.1365-2664.2012.02130.x
Kareiva, P. (2014). New conservation: setting the record straight and finding common ground. Conserv. Biol. 28, 634–636. doi: 10.1111/cobi.12295
Karp, D. S., Baur, P., Atwill, E. R., De Master, K., Gennet, S., Iles, A., et al (2015a). The unintended ecological and social impacts of food safety regulations in California's Central Coast Region. BioScience 65, 1173–1183. doi: 10.1093/biosci/biv152
Karp, D. S., Chaplin-Kramer, R., Meehan, T. D., Martin, E. A., DeClerck, F., Grab, H., et al. (2018). Crop pests and predators exhibit inconsistent responses to surrounding landscape composition. Proc. Nat. Acad. Sci. U.S.A.115, E7863–E7870. doi: 10.1073/pnas.1800042115
Karp, D. S., Gennet, S., Kilonzo, C., Partyka, M., Chaumont, N., Atwill, E. R., and Kremen, C. (2015b). Comanaging fresh produce for nature conservation and food safety. Proc. Nat. Acad. Sci. U.S.A.112, 11126–11131. doi: 10.1073/pnas.1508435112
Karp, D. S., Mendenhall, C. D., Sandí, R. F., Chaumont, N., Ehrlich, P. R., Hadly, E. A., et al. (2013). Forest bolsters bird abundance, pest control and coffee yield. Ecol. Lett. 16, 1339–1347. doi: 10.1111/ele.12173
Keeler, B. L., Chaplin-Kramer, R., Guerry, A. D., Addison Prue, F. E., Bettigole, C., Burke, I. C., et al. (2017). Society is ready for a new kind of science-Is academia? BioScience 67, 591–592. doi: 10.1093/biosci/bix051
Klatt, B. K., Holzschuh, A., Westphal, C., Clough, Y., Smit, I., Pawelziket, E., et al. (2014). Bee pollination improves crop quality, shelf life and commercial value. Proc. R. Soc. B Biol. Sci. 281:20132440. doi: 10.1098/rspb.2013.2440
Kleijn, D., Baquero, R. A., Clough, Y., Diaz, M., De Esteban, J., Fernández, F., et al. (2006). Mixed biodiversity benefits of agri-environment schemes in five European countries. Ecol. Lett. 9, 243–254. doi: 10.1111/j.1461-0248.2005.00869.x
Köhler, H.-R., and Triebskorn, R. (2013). Wildlife ecotoxicology of pesticides: can we track effects to the population level and beyond? Science 341, 759–765. doi: 10.1126/science.1237591
Landis, D. A., Wratten, S. D., and Gurr, G.M., (2000). Habitat management to conserve natural enemies of arthropod pests in agriculture. Ann. Rev. Entomol. 45, 175–201. doi: 10.1146/annurev.ento.45.1.175
Larsen, A. E. (2013). Agricultural landscape simplification does not consistently drive insecticide use. Proc. Nat. Acad. Sci. 110, 15330–15335. doi: 10.1073/pnas.1301900110
Larsen, A. E., Gaines, S. D., and Deschênes, O. (2015). Spatiotemporal variation in the relationship between landscape simplification and insecticide use. Ecol. Appl. 25, 1976–1983. doi: 10.1890/14-1283.1
Larson, S. J., Capel, P. D., and Majewski, M. (1997). Pesticides in Surface Waters: Distribution, Trends, and Governing Factors. Boca Raton, FL: CRC Press.
Lefebvre, M., Langrell, S. R. H., and Gomez-y-Paloma, S. (2014). Incentives and policies for integrated pest management in Europe: a review. Agronomy Sustainable Dev. 35, 27–45. doi: 10.1007/s13593-014-0237-2
Letourneau, D. K., Armbrecht, I., Rivera, B. S., Lerma, J. M., Carmona, E. J., Daza, M. C., et al. (2011). Does plant diversity benefit agroecosystems? a synthetic review. Ecol. Appl. 21, 9–21. doi: 10.1890/09-2026.1
Levins, R., and Schultz, B. B. (1996). Effects of density dependence, feedback, and environmental sensitivity on correlations among predators, prey, and plant resources: models and practical implications. J. Anim. Ecol. 65, 802–812. doi: 10.2307/5678
Lichtenberg, E. M., Kennedy, C. M., Kremen, C., Batáry, P., Berendse, F., Bommarco, R., et al. (2017). A global synthesis of the effects of diversified farming systems on arthropod diversity within fields and across agricultural landscapes. Global. Change Biol. 23, 4946–4957. doi: 10.1111/gcb.13714
Liu, E. M., and Huang, J. (2013). Risk preferences and pesticide use by cotton farmers in China. J. Dev. Econ. 103, 202–215. doi: 10.1016/j.jdeveco.2012.12.005
Lu, Y., Wu, K., Jiang, Y., Guo, Y., and Desneux, N. (2012). Widespread adoption of Bt cotton and insecticide decrease promotes biocontrol services. Nature 487, 362–365. doi: 10.1038/nature11153
Lu, Y., Wu, K., Jiang, Y., Xia, B., and Li, P. (2010). Mirid bug outbreaks in multiple crops correlated with wide-scale adoption of Bt cotton in China. Science 328, 1151–1154. doi: 10.1126/science.1187881
Meehan, T. D., Werling, B. P., Landis, D. A., and Gratton, C. (2011). Agricultural landscape simplification and insecticide use in the Midwestern United States. Proc. Nat. Acad. Sci. U.S.A. 108, 11500–11505. doi: 10.1073/pnas.1100751108
Millennium Ecosystem Assessment (2005). Ecosystems and Human Well-being: Synthesis. Washington, DC: Island Press.
Oerke, E.-C. C. (2006). Crop losses to pests. J. Agricult. Sci. 144, 31–43. doi: 10.1017/S0021859605005708
Palis, F. G., Flor, R. J., Warburton, H., and Hossain, M. (2006). Our farmers at risk: behaviour and belief system in pesticide safety. J. Publ. Health 28, 43–48. doi: 10.1093/pubmed/fdi066
Paredes, D., Cayuela, L., Gurr, G. M., and Campos, M. (2015). Is ground cover vegetation an effective biological control enhancement strategy against olive pests? PLoS ONE. 10, 1–13. doi: 10.1371/journal.pone.0117265
Pimentel, D. (2005). Environmental and economic costs of the application of pesticides primarily in the United States. Environ. Dev. Sustainabil. 7, 229–252. doi: 10.1007/s10668-005-7314-2
Pingali, P. L., Hossain, M., and Gerpacio, R.V. (1997). “Fertilizers and pesticides: higher levels versus improved efficiencies,” in Asian Rice Bowls: The Returning Crisis? (Wallingford; New York, NY: CAB International and IRRI).
Rosenheim, J. A., and Gratton, C. (2017). Ecoinformatics (Big Data) for agricultural entomology: pitfalls, progress, and promise. Annu. Rev. Entomol. 62, 399–417. doi: 10.1146/annurev-ento-031616-035444
Rusch, A., Chaplin-Kramer, R., Gardiner, M. M., Hawro, V., Holland, J., Landis, D., et al. (2016). Agricultural landscape simplification reduces natural pest control: a quantitative synthesis. Agricult. Ecosyst. Environ. 221, 198–204. doi: 10.1016/j.agee.2016.01.039
Salliou, N., Muradian, R., and Barnaud, C. (2019). Governance of ecosystem services in agroecology: when coordination is needed but difficult to achieve. Sustainability 11:1158. doi: 10.3390/su11041158
Schellhorn, N. A., Parry, H. R., Macfadyen, S., Wang, Y., and Zalucki, M. P. (2015). Connecting scales: Achieving in-field pest control from areawide and landscape ecology studies. Insect Sci. 22, 35–51. doi: 10.1111/1744-7917.12161
Shelton, A. M. V., Sances, F., Hawley, J., Tang, J. D., Boune, M., Jungers, D., et al. (2000). Assessment of insecticide resistance after the outbreak of diamondback moth (Lepidoptera: Plutellidae) in California in 1997. J. Econ. Entomol. 93, 931–936. doi: 10.1603/0022-0493-93.3.931
Sogawa, K. (2015). “Planthopper Outbreaks in Different Paddy Ecosystems in Asia: Man-Made Hopper Plagues that Threatened the Green Revolution in Rice,” in Rice Planthoppers-Ecology, Management, Socio Economics and Policy, eds K. L. Heong, J. Cheng, and M. M. Escalada. (Hangzhou: Zhejiang University Press, Springer Science+Business Media Dordrecht.
Tago, D., Andersson, H., and Treich, N. (2014). “Pesticides and health: a review of evidence on health effects, valuation of risks, and benefit-cost analysis,” in Preference Measurement in Health (in the series‘Advances in Health Economics and Health Services Research’), eds G. C. Blomquist and K. Bolin (Bingley: Emerald Group Publishing, 203–295.
Thies, C., Haenke, S., Scherber, C., Bengtsson, J., Bommarco, R., Clement, L. W., et al (2011). The relationship between agricultural intensification and biological control: experimental tests across Europe. Ecol. Appl. 21, 2187–2196. doi: 10.1890/10-0929.1
Torres, J. B., and de Bueno, A. F. (2018). Conservation biological control using selective insecticides-a valuable tool for IPM. Biol. Cont. 126, 53–64. doi: 10.1016/j.biocontrol.2018.07.012
Tscharntke, T., Bommarco, R., Clough, Y., Crist, T. O., Kleijn, D., Rand, T. A., et al. (2007). Conservation biological control and enemy diversity on a landscape scale. Biol. Control 43, 294–309. doi: 10.1016/j.biocontrol.2007.08.006
Tscharntke, T., Karp, D. S., Chaplin-Kramer, R., Batáry, P., Declerck, F., Gratton, C., et al. (2016). When natural habitat fails to enhance biological pest control – five hypotheses. Biol. Conserv. 204, 449–458. doi: 10.1016/j.biocon.2016.10.001
Tscharntke, T., Klein, A. M., Kruess, A., Steffan-Dewenter, I., and Thies, C. (2005). Landscape perspectives on agricultural intensification and biodiversity-ecosystem service management. Ecol. Lett. 8, 857–874. doi: 10.1111/j.1461-0248.2005.00782.x
USDA RMA (2018). Risk Management Agency. Cause of Loss Data Historical Files. Available online at: https://www.rma.usda.gov/SummaryOfBusiness/CauseOfLoss (accessed 19 July, 2019).
Vogl, A. L., Goldstein, J. H., Daily, G. C., Vira, B., Bremer, L. L., McDonald, R., et al. (2017) Mainstreaming investments in watershed services to enhance water security: barriers opportunities. Environ. Sci. Policy 75, 19–27. doi: 10.1016/j.envsci.2017.05.007
Westphal, C., Stefan, V., Horgan, F. G., Gurr, G. M., Escalada, M, Chien, H., et al. (2015). Promoting multiple ecosystem services with flower strips and participatory approaches in rice production landscapes. Basic Appl. Ecol. 16, 681–689. doi: 10.1016/j.baae.2015.10.004
Whitehorn, P. R., O'Conner, S., Wackers, F. L., and Goulson, D. (2012). Neonicotinoid pesticide reduces bumble bee colony growth and queen production. Science 336, 351–352. doi: 10.1126/science.1215025
Zhang, W., Lu, Y., van der Werf, W., Huang, J., Wu, F., Zhou, K., et al. (2018). Multidecadal, county-level analysis of the effects of land use, Bt cotton, and weather on cotton pests in China. Proc. Nat. Acad. Sci. U.S.A. 115:201721436. doi: 10.1073/pnas.1721436115
Zhang, W., and Swinton, S. M. (2009). Incorporating natural enemies in an economic threshold for dynamically optimal pest management. Ecol. Model. 220, 1315–1324. doi: 10.1016/j.ecolmodel.2009.01.027
Keywords: pest control, natural enemies, ecosystem services, stakeholders, decision-support
Citation: Chaplin-Kramer R, O'Rourke M, Schellhorn N, Zhang W, Robinson BE, Gratton C, Rosenheim JA, Tscharntke T and Karp DS (2019) Measuring What Matters: Actionable Information for Conservation Biocontrol in Multifunctional Landscapes. Front. Sustain. Food Syst. 3:60. doi: 10.3389/fsufs.2019.00060
Received: 02 April 2019; Accepted: 11 July 2019;
Published: 06 August 2019.
Edited by:
Matthew Heard, Centre for Ecology and Hydrology (CEH), United KingdomReviewed by:
Tom H. Oliver, University of Reading, United KingdomHouston Wilson, University of California, Riverside, United States
Copyright © 2019 Chaplin-Kramer, O'Rourke, Schellhorn, Zhang, Robinson, Gratton, Rosenheim, Tscharntke and Karp. This is an open-access article distributed under the terms of the Creative Commons Attribution License (CC BY). The use, distribution or reproduction in other forums is permitted, provided the original author(s) and the copyright owner(s) are credited and that the original publication in this journal is cited, in accordance with accepted academic practice. No use, distribution or reproduction is permitted which does not comply with these terms.
*Correspondence: Rebecca Chaplin-Kramer, YmNoYXBsaW5Ac3RhbmZvcmQuZWR1