- 1Departamento de Ciencias de la Salud, Universidad Iberoamericana Puebla, Puebla, Mexico
- 2Laboratorio de Micología, Centro de Investigaciones en Ciencias Microbiológicas, Instituto de Ciencias, Benemérita Universidad Autònoma de Puebla, Puebla, Mexico
- 3Facultad de Ciencias Químicas, Benemérita Universidad Autónoma de Puebla, Puebla, Mexico
Biofilms are difficult to eliminate with standard antimicrobial treatments due to their high antibiotic resistance. Therefore, some plants contain natural compounds with antimicrobial activity that can control biofilm formation. The aim of this work was to evaluate the effect of Mexican oregano (Lippia berlandieri Schauer) essential oil (EO) on Pseudomonas aeruginosa and Salmonella Thyphimurium biofilm formation on stainless steel surfaces. EO was added to achieve final concentrations of 0, 200, 250, 300, 400, 600, 800, and 1,600 mg/L. Growth data were modeled using the modified Gompertz model. EO antimicrobial activity against biofilm can be observed for both microorganisms. Moreover, at an EO concentration below inhibition, there is a delay in biofilm formation, thus decreasing the number of microorganisms that are part of the biofilm. The maximum growth rate in biofilm formation showed an inversely proportional behavior in relation to EO concentration. P. aeruginosa requires nearly 1 h to start forming biofilms. There is a delay in biofilm formation, thus decreasing the number of microorganisms that are part of the biofilm (24 h for 400 mg/L and 18 h for 600 mg/L). Salmonella Thyphimurium biofilm formation is affected at 200 mg/L of Mexican oregano EO, causing film detachment from the stainless steel surface after 18 h, while at 250 mg/L biofilm formation was inhibited. Mexican oregano EO could inhibit the development of P. aeruginosa and Salmonella Thyphimurium biofilm formation on stainless steel surfaces. Salmonella was more susceptible than Pseudomonas to Mexican oregano EO according to the concentrations required to inhibit biofilm growth.
Introduction
Biofilms are defined as adherent, matrix-enclosed bacterial populations attached with physical appendages to a surface, followed by coating with extracellular polysaccharides (Furukawa et al., 2010; Laird et al., 2012). In nature, microorganisms usually attach to solid surfaces, especially at liquid–solid interfaces. Biofilms mediate bacteria persistence and shield from hostile environments. The initial microbial adhesion to surfaces is a complex process dependent on non-specific interactions between bacteria and the surface to form slimy layers. Once bacteria firmly established a biofilm, it is very difficult to eradicate because it exhibits an increase of resistance to chemical compounds (Nostro et al., 2010; Ibusquiza et al., 2012; Roy et al., 2012). Many foodborne pathogens are able to survive at low temperatures and attach to, form resistant biofilms, and colonize different surfaces. This resistance is related on the three-dimensional film structure which has enhanced tolerance to physical and chemical treatments and considered a potential hazard, a source of food contamination, and a cause of economic losses by technical failure in water systems, heat exchangers, product quality deterioration, and biocorrosion (Dheilly et al., 2008; Bae et al., 2012; Bodur and Cagri-Mehmetoglu, 2012; Ibusquiza et al., 2012).
Besides that, biofilms are difficult to eliminate with standard antimicrobial treatments due to their high antibiotic resistance relative to free-living cells (Kavanaugh and Ribbeck, 2012). Some plants contain natural compounds with antimicrobial activity; in their natural state, these compounds can play the role of prolonging the shelf-life of food. Many of them have been studied for their potential as food antimicrobials (Rivera-Calo et al., 2015; Swamy et al., 2016; Ambrosio et al., 2017; Rakmai et al., 2018). The use of natural compounds as food additives involves isolation, purification, stabilization, and incorporation of these compounds into food for antimicrobial purposes while maintaining the food's organoleptic and nutritive properties (Pandey et al., 2017; Rakmai et al., 2017a; Navarro-Cruz et al., 2018). The antimicrobial activity of these natural additives is due to the presence of their chemical compounds which can alter and penetrate the microorganism's cell wall lipid structure, disturbing cellular structures and leading to protein denaturation and cell membrane destruction, increasing their permeability and the likelihood of cytoplasmic ruptures, leaks, or cell lysis and eventually microbial death (Rakmai et al., 2017b; Reyes-Jurado et al., 2019). Therefore, natural compounds as essential oils (EOs) are an alternative to be used as antimicrobial in the bacterial biofilm formation.
Mexican oregano is one of the aromatic plants used as a food additive to enhance food flavor; carvacrol and thymol are the major chemical compounds present in this EO and are the responsible for their antimicrobial action (Avila-Sosa et al., 2010; Anaya-Castro et al., 2017; Cid-Pérez et al., 2019). The aim of this study was to evaluate the inhibitory effect of Mexican oregano (Lippia berlandieri Schauer) EO on Pseudomonas aeruginosa and Salmonella Thyphimurium biofilm formation.
Materials and Methods
Essential Oil, Microorganisms, Culture Preparation, and Stainless Steel Surfaces
Mexican oregano EO was obtained and processed at CIReNa (Natural Resources Research Center, DGTA, Salaices, Chihuahua), where it was characterized based on its carvacrol and thymol contents. The oregano was grown on site, and the EO was obtained by vapor extraction for 4 h using a Clevenger-type apparatus (Silva-Vazquez and Dunford, 2005). Pseudomonas aeruginosa (ATTC 14,210) and Salmonella enterica serovar Typhimurium (ATCC 14028) were obtained from Facultad de Ciencias Químicas Microbiology Laboratory and maintained on tryptone soy agar (TSA, Merk, Mexico, Mexico). At the time of performing the biofilm formation assay, the bacteria were incubated on tryptone soy broth (TSB, Merk, Mexico, Mexico) at 37°C for 18 h until exponential phase was observed. Stainless steel plates (3.0 × 6.0 cm) were prepared according to Speranza et al. (2011) by washing in acetone (Sigma-Adrich, Milwaukee, WI, USA) for 30 min, rinsing in distilled water, then soaking in NaOH (1 N for 1 h), and final rinsing in distilled water prior to use.
Biofilm Formation Assay
Stainless steel plates were placed vertically into jars containing 20 mL of TSB and autoclaved at 121°C for 15 min. EO was added to achieve final concentrations of 0, 200, 250, 300, 400, 600, 800, and 1,600 mg/L and mechanically (using a vortex shaker) incorporated under sterile conditions. Then, 500 μl of bacteria was inoculated to obtain an initial bacterial population of about 103 CFU/ml. The jars were incubated at 37°C during 0, 1, 2, 4, 6, 12, 36, 48, 60, and 72 h. For all the samples, incubation was done without agitation. At these times, the stainless steel plates were aseptically removed and rinsed with sterile distilled water in order to eliminate the unattached cells. The biofilm cells were collected and enumerated according to Xu et al. (2010) by swabbing over the area with sterilized water-moistened cotton swabs and immersing in tubes with 1 ml of 0.1% sterile peptone water. These were vortexed vigorously with 9 ml of 0.1% sterile peptone water to suspend the adhering cells. In order to ensure the viability of the organisms, a growth control was performed with broth cells. Both counts (biofilm and broth) were determined by the pour plating method on TSA and were incubated at 37°C for 48 h. Every test was performed in triplicate.
Data Modeling and Statistical Analysis
The growth data were modeled using the modified Gompertz model (van Boekel, 2008):
where N is the number of microorganisms (UFC/ml) at time t (h), No (UFC/ml) is the number of microorganisms at initial time, A is the asymptotic value of the maximum number of microorganisms, υmax is the maximum growth rate [(log N/No)/h], and λ is the lag phase or the adaptation time period (h) that microorganisms take under new conditions.
Statistical analyses were performed with the general linear model procedure in Minitab 15 (LEAD Technologies Inc., NJ, USA). Significantly (p < 0.05) different means were separated with Tukey's test. Student t-tests (p < 0.05) were performed in order to compare biofilm and broth growth.
Results
Mexican oregano EO was obtained from plants grown in Chihuahua. Several compounds are related to its chemical composition—thymol (1.991 g/ml) and carvacrol (0.353 g/ml) and their precursors (p-cymene, 1,8- cineole, and γ -terpinen)—which were detected in very low concentrations. EO antimicrobial activity against biofilm formation is remarkable; it can be observed that, for both microorganisms (Figures 1, 2), the minimal inhibitory concentration (MIC) values are lower (50%) than the MIC values in broth culture. All bacterial curves presented an adequate data fit to Gompertz model equation ( = 0.906 ± 0.04).
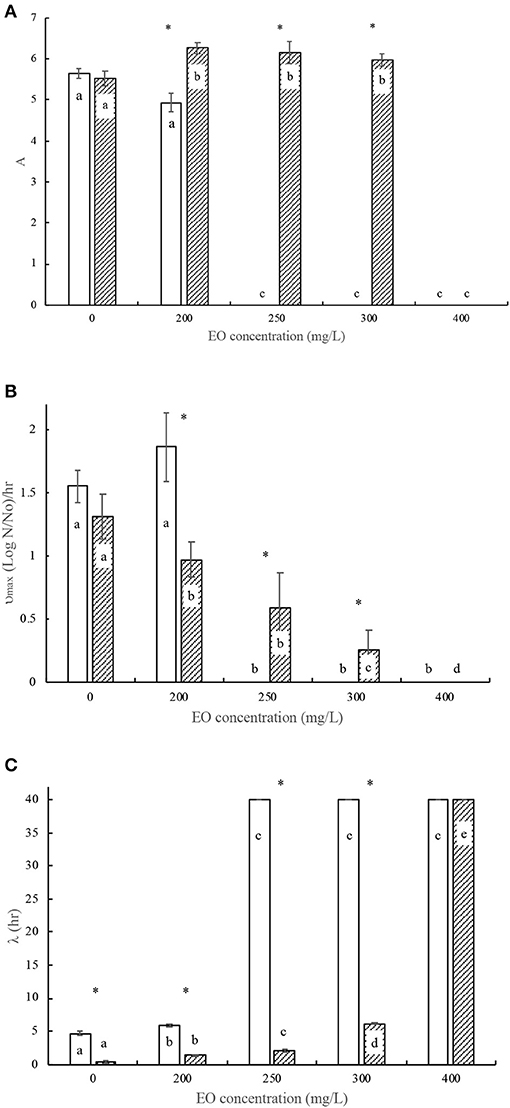
Figure 1. Modified Gompertz model parameters for Pseudomonas aeruginosa biofilm growth (□) and broth growth (■) curves subjected to selected concentrations of Mexican oregano (Lippia berlandieri Schauer) essential oil. (A) Maximum number of microorganisms. (B) Maximum growth rate. (C) Lag phase. Each bar treatment with different letters is significantly different (p < 0.05). Asterisk denotes significant difference between growth conditions (p < 0.05).
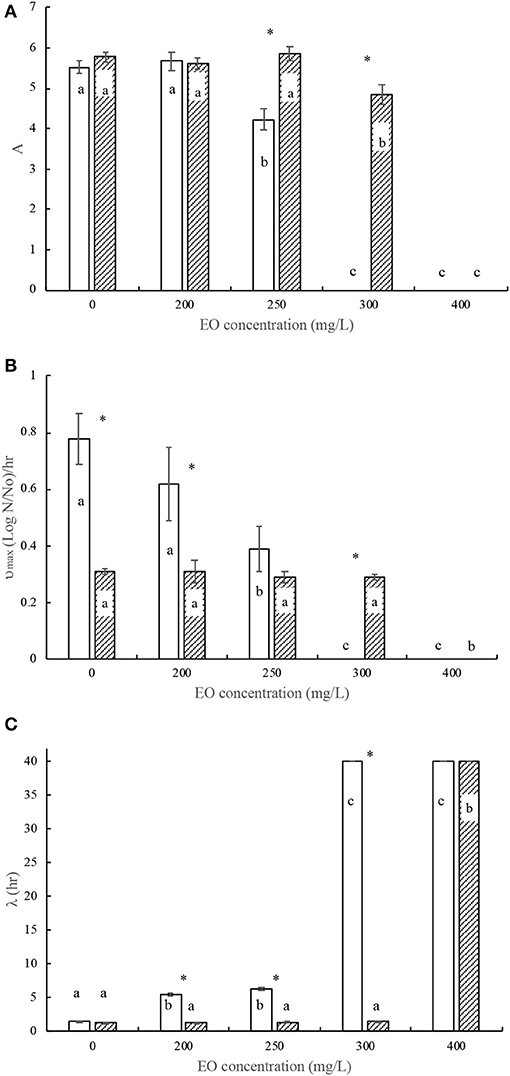
Figure 2. Modified Gompertz model parameters for Salmonella Typhimurium biofilm growth (□) and broth growth (■) curves subjected to selected concentrations of Mexican oregano (Lippia berlandieri Schauer) essential oil. (A) Maximum number of microorganisms. (B) Maximum growth rate. (C) Lag phase. Each bar treatment with different letters is significantly different (p < 0.05). Asterisk denotes significant difference between growth conditions (p < 0.05).
Figure 1 shows significant differences (p < 0.05) between Gompertz growth parameters on P. aeruginosa development in broth culture, with MIC values of 800 and 1,600 mg/L. It can be observed that it has the largest number of microorganisms that adhere on stainless steel surfaces, forming the biofilm. It requires approximately near 8 log(N/No) and near 1 h to start forming biofilms. Moreover, at EO concentration values below MIC (Figure 3A), there is a delay in biofilm formation, thus decreasing the number of microorganisms that are part of the biofilm (24 h for 400 mg/L and 18 h for 600 mg/L). The maximum growth rate in biofilm formation showed an inversely proportional behavior in relation to the EO concentration.
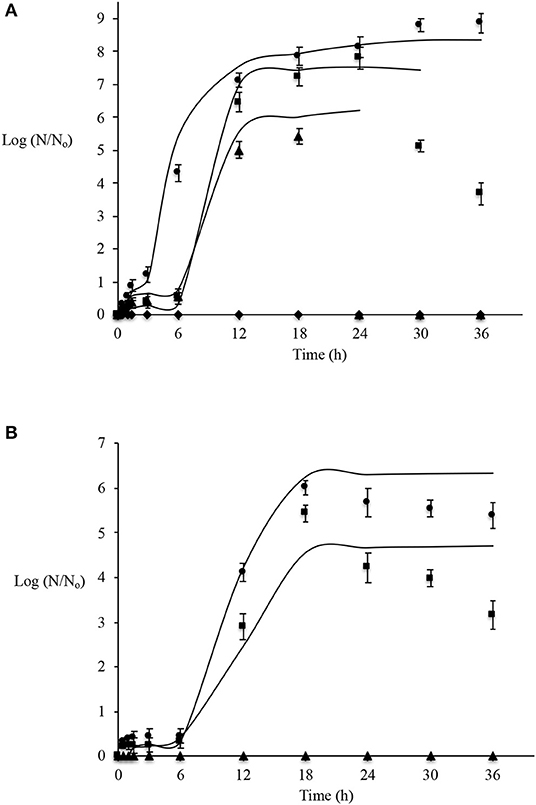
Figure 3. Effect of Mexican oregano (Lippia berlandieri Schauer) essential oil at selected concentrations [0 (•), 400 (■), 600 (▴), and 800 (♦) mg/l] on Pseudomonas aeruginosa (A) and Salmonella Typhimurium (B) [0 (•), 200 (■), and 250 (▴) mg/l] on biofilm formation curves on stainless steel surfaces fitted (-) with the modified Gompertz model.
A comparison between the statistical analyses of the Gompertz parameters on the development of Salmonella Thyphimurium in broth and on stainless steel is shown in Figure 2. In culture broth, Salmonella does not require an adaptation time since they start to grow from the start, while biofilm formation requires ~6 h of lag phase. Significant differences (p < 0.05) are detected in A and υmax parameters, being higher in culture broth. Furthermore, the biofilm-forming bacteria's stationary phase (Figure 3B) is shorter. This may be due to biofilm formation's last phase (phase 5: colonizing cell dispersion). The Salmonella Thyphimurium biofilm formation is affected at 200 mg/L of Mexican oregano EO, causing film detachment from the stainless steel surface after 18 h, while at 250 mg/L biofilm formation was inhibited.
Discussion
The Mexican oregano EO antimicrobial activity is due to the presence of thymol and carvacrol along with other minor components. The main component is thymol, and its concentrations are greater when the extracts are obtained from younger plants; in contrast, in European oregano EO, carvacrol concentration is higher (88%) than thymol (2%) (Chorianopoulos and Kalpoutzakis, 2004). In addition, it is common to find variations on EO chemical composition. It depends on the climate and the soil conditions as well as the phenological plant stage (Avila-Sosa et al., 2014).
Biofilm formation ability has been associated with bacterial virulence colonization, environmental survival, antibiotic resistance, surface characteristics, and growth medium (Ferreira et al., 2013; Di Ciccio et al., 2015). During this process, the bacteria can change their phenotype and morphology, affecting the structural changes necessary to for the biofilm to develop and causing the detachment shortly after starting its formation or avoiding their formation (Chmielewski and Frank, 2003). P. aeruginosa's fast biofilm forming is due to cell surface characteristics such as flagella, pili, adhesion, and capsule proteins that also influence adhesion. Kumar and Anand (1998) mentioned that pili acts as a velcro to anchor bacteria to some surfaces and also acts as chemoreceptors, directing to some specific sites. By possessing these surface characteristics in combination with a nutrient-rich medium, biofilm formation is adapted quickly on stainless steel surfaces. Srey et al. (2013) suggested that Salmonella strains preferably adhere to hydrophobic surface materials that depend on surface interfacial free energies. Moreover, bacterial cell walls (conferred by peptidoglycan anionic teichoic acids) and cell surface hydrophobicity (enhanced by the presence of lactic acid), which affect the electrostatic charge, are the main factors that cause biofilms to attach to stainless steel surfaces (Bridier et al., 2015).
Burt (2004) reported that the oregano EO antimicrobial effect is not attributed to a single mechanism but to several, due to multiple cell targets, since it can act on cytoplasmic membrane proteins and on lipophilic compounds, specifically with the protein's hydrophobic parts. As a consequence, they can modify enzyme activities that regulate energy production or structural compound synthesis. It can be observed that the biofilm formation initial phases would be avoided where the exopolysaccharide matrix is synthesized to establish an irreversible physical contact between them and the surface. Ultee et al. (1998) also reports that carvacrol interacts with bacterial membranes, changing its permeability for cations (H+ and K+), where ion gradient dissipation leads to a deterioration in the essential processes in the cell and finally to cell death. Biological effects may depend on the concentration and the exposure time, modifying the composition of the cell membrane.
Furthermore, Dat et al. (2012) found that biofilm formation might be enhanced by low pH through cell-to-cell signaling. Moreover, pH changes may trigger numerous signaling substances. These substances could contribute to the overall acid tolerance response and promote biofilm formation, which is known as an adaptation to external stressful environments. The biofilms with low pH can allow the higher antimicrobial action of thymol and carvacrol (whose pH dissociation values are acidic). According to Martínez-Graci et al. (2015), optimal pH should be considered at which the antimicrobial agent may have a state of dissociation that allows better antimicrobial activity. Rivera-Calo et al. (2015) mentioned that the EO antimicrobial effect may be attributed to their ability to penetrate through the bacterial membrane to the cell interior and to exhibit an inhibitory activity on the cell's functional properties. Because biofilm formation is an active process that requires energy for various extracellular components, Wang et al. (2015) suggested that the inhibitory effect of biofilm formation may be due to an increase of ATP permeability in the cytoplasmic membrane. Salmonella Thyphimurium growth in broth suggests ATP use to maintain its viability, leaving behind the biofilm production.
The Mexican oregano EO could inhibit the development of P. aeruginosa and Salmonella Thyphimurium biofilm formation on stainless steel surfaces. Salmonella was more susceptible than Pseudomonas to Mexican oregano EO according to the concentrations required to inhibit biofilm growth. The Mexican oregano EO could be an alternative for cleaning stainless steel surfaces and equipment.
Data Availability Statement
The datasets generated for this study are available on request to the corresponding author.
Author Contributions
RA-S, FR-J, and CO-V wrote the manuscript. FR-J, TC-P, and PH-C designed and performed the experiments. RM-P provided and characterized the microorganisms. All the authors approved the final manuscript.
Funding
This study was funded in part by the Facultad de Ciencias Químicas of Benemérita Universidad Autónoma de Puebla and the National Council for Science and Technology of México (CONACYT).
Conflict of Interest
The authors declare that the research was conducted in the absence of any commercial or financial relationships that could be construed as a potential conflict of interest.
Acknowledgments
FR-J gratefully acknowledges the financial support for her Postdoctoral Fellowship from PRODEP No. 511-6/17-11061.
References
Ambrosio, C. M. S., de Alencar, S. M., de Sousa, R. L. M., Moreno, A. M., and Da Gloria, E. M. (2017). Antimicrobial activity of several essential oils on pathogenic and beneficial bacteria. Ind. Crops Prod. 97, 128–136. doi: 10.1016/j.indcrop.2016.11.045
Anaya-Castro, M. A., Ayala-Zavala, J. F., Muñoz-Castellanos, L., Hernández-Ochoa, L., Peydecastaing, J., and Durrieu, V. (2017). β-Cyclodextrin inclusion complexes containing clove (Eugenia caryophyllata) and Mexican oregano (Lippia berlandieri) essential oils: preparation, physicochemical and antimicrobial characterization. Food Packag. Shelf Life 14, 96–101. doi: 10.1016/j.fpsl.2017.09.002
Avila-Sosa, R., Gastélum-Franco, M. G., Camacho-Dávila, A., Torres-Muñoz, J. V., and Nevárez-Moorillón, G. V. (2010). Extracts of Mexican oregano (Lippia berlandieri Schauer) with antioxidant and antimicrobial activity. Food Bioproc. Technol. 3, 434–440. doi: 10.1007/s11947-008-0085-7
Avila-Sosa, R., Portillo-Ruiz, M. C., Viramontes-Ramos, S., Muñoz-Castellanos, L. N., and Nevárez-Moorillón, G. V. (2014). Effect of Mexican oregano (Lippia berlandieri Schauer) essential oil fractions on the growth of Aspergillus spp. in a bread model system. J. Food Process. Preserv. 39, 776–783. doi: 10.1111/jfpp.12287
Bae, Y. M., Baek, S. Y., and Lee, S. Y. (2012). Resistance of pathogenic bacteria on the surface of stainless steel depending on attachment form and efficacy of chemical sanitizers. Int. J. Food Microbiol. 153, 465–473. doi: 10.1016/j.ijfoodmicro.2011.12.017
Bodur, T., and Cagri-Mehmetoglu, A. (2012). Removal of Listeria monocytogenes, Staphylococcus aureus and Escherichia coli O157:H7 biofilms on stainless steel using scallop shell powder. Food Control. 25, 1–9. doi: 10.1016/j.foodcont.2011.09.032
Bridier, A., Sanchez-Vizuete, P., Guilbaud, M., Piard, J. C., Naitali, M., and Briandet, R. (2015). Biofilm-associated persistence of food-borne pathogens. Food Microbiol. 45, 167–178. doi: 10.1016/j.fm.2014.04.015
Burt, S. (2004). Essential oils: their antibacterial properties and potential applications in foods-a review. Int. J. Food Microbiol. 94, 223–253. doi: 10.1016/j.ijfoodmicro.2004.03.022
Chmielewski, R. A. N., and Frank, J. F. (2003). Biofilm formation and control in food processing facilities. Compr. Rev. Food Sci. Food Saf. 2, 22–32. doi: 10.1111/j.1541-4337.2003.tb00012.x
Chorianopoulos, N., and Kalpoutzakis, E. (2004). Essential oils of Satureja, Origanum, and Thymus species: chemical composition and antibacterial activities against foodborne pathogens. J. Agric. Food Chem. 52, 8261–8267. doi: 10.1021/jf049113i
Cid-Pérez, T. S., Avila-Sosa, R., Ochoa-Velasco, C. E., Rivera-Chavira, B. E., and Nevárez-Moorillón, G. V. (2019). Antioxidant and antimicrobial activity of Mexican oregano (Poliomintha longiflora) essential oil, hydrosol and extracts from waste solid residues. Plants 1, 1–13. doi: 10.3390/plants8010022
Dat, N. M., Hamanaka, D., Tanaka, F., and Uchino, T. (2012). Control of milk pH reduces biofilm formation of Bacillus licheniformis and Lactobacillus paracasei on stainless steel. Food Control 23, 215–220. doi: 10.1016/j.foodcont.2011.07.013
Dheilly, A., Linossier, I., Darchen, A., Hadjiev, D., Corbel, C., and Alonso, V. (2008). Monitoring of microbial adhesion and biofilm growth using electrochemical impedancemetry. Appl. Microbiol. Biotechnol. 79, 157–164. doi: 10.1007/s00253-008-1404-7
Di Ciccio, P., Vergara, A., Festino, A. R., Paludi, D., Zanardi, E., Ghidini, S., et al. (2015). Biofilm formation by Staphylococcus aureus on food contact surfaces: relationship with temperature and cell surface hydrophobicity. Food Control 50, 930–936. doi: 10.1016/j.foodcont.2014.10.048
Ferreira, S., Fraqueza, M. J., Queiroz, J. A., Domingues, F. C., and Oleastro, M. (2013). Genetic diversity, antibiotic resistance and biofilm-forming ability of Arcobacter butzleri isolated from poultry and environment from a Portuguese slaughterhouse. Int. J. Food Microbiol. 162, 82–88. doi: 10.1016/j.ijfoodmicro.2013.01.003
Furukawa, S., Akiyoshi, Y., Komoriya, M., Ogihara, H., and Morinaga, Y. (2010). Removing Staphylococcus aureus and Escherichia coli biofilms on stainless steel by cleaning-in-place (CIP) cleaning agents. Food Control 21, 669–672. doi: 10.1016/j.foodcont.2009.10.005
Ibusquiza, P. A., Herrera, J. J. R., Vázquez-Sánchez, D., and Cabo, M. L. (2012). Adherence kinetics, resistance to benzalkonium chloride and microscopic analysis of mixed biofilms formed by Listeria monocytogenes and Pseudomonas putida. Food Control 25, 202–2010. doi: 10.1016/j.foodcont.2011.10.002
Kavanaugh, N. L., and Ribbeck, K. (2012). Selected antimicrobial essential oils eradicate Pseudomonas spp. and Staphylococcus aureus biofilms. Appl. Environ. Microbiol. 78, 4057–4061. doi: 10.1128/AEM.07499-11
Kumar, C. G., and Anand, S. K. (1998). Significance of microbial biofilms in food industry: a review. Int. J. Food Microbiol. 42, 9–27. doi: 10.1016/S0168-1605(98)00060-9
Laird, K., Armitage, D., and Phillips, C. (2012). Reduction of surface contamination and biofilms of Enterococcus sp. and Staphylococcus aureus using a citrus-based vapour. J. Hosp. Infect. 80, 61–66. doi: 10.1016/j.jhin.2011.04.008
Martínez-Graciá, C., González-Bermúdez, C. A., Cabellero-Valcárcel, A. M., Santaella-Pascual, M., and Frontela-Saseta, C. (2015). Use of herbs and spices for food preservation: advantages and limitations. Curr. Opin. Food Sci. 6, 38–43. doi: 10.1016/j.cofs.2015.11.011
Navarro-Cruz, A. R., Ochoa-Velasco, C. E., Caballero-Alvarez, F. J., Lazcano-Hernández, M. A., Vera-López, O., López-Malo, A., et al. (2018). Effect of pH and Mexican oregano (Lippia berlandieri Schauer) essential oil added to carboxymethyl cellulose and starch edible films on Listeria monocytogenes and Staphylococcus aureus. J. Food Qual. 1, 1–6. doi: 10.1155/2018/1659394
Nostro, A., Scaffaro, R., Ginestra, G., D'Arrigo, M., Botta, L., Marino, A., et al. (2010). Control of biofilm formation by poly-ethylene-co-vinyl acetate films incorporating nisin. Appl. Microbiol. Biotechnol. 87, 729–737. doi: 10.1007/s00253-010-2598-z
Pandey, A. K., Kumar, P., Singh, P., Tripathi, N. N., and Bajpai, V. K. (2017). Essential oils: sources of antimicrobials and food preservatives. Front. Microbiol. 7:2161. doi: 10.3389/fmicb.2016.02161
Rakmai, J., Cheirsilp, B., Mejuto, J. C., Simal-Gándara, J., and Torrado-Agrasar, A. (2018). Antioxidant and antimicrobial properties of encapsulated guava leaf oil in hydroxypropyl-beta-cyclodextrin. Ind. Crop. Prod. 111, 219–225. doi: 10.1016/j.indcrop.2017.10.027
Rakmai, J., Cheirsilp, B., Mejuto, J. C., Torrado-Agrasar, A., and Simal-Gándara, J. (2017b). Physico-chemical characterization and evaluation of bio-efficacies of black pepper essential oil encapsulated in hydroxypropyl-beta- cyclodextrin. Food Hydrocolloid. 65, 157–164. doi: 10.1016/j.foodhyd.2016.11.014
Rakmai, J., Cheirsilp, B., Torrado-Agrasar, A., Simal-Gándara, J., and Mejuto, J. C. (2017a). Encapsulation of yarrow essential oil in hydroxypropyl-beta-cyclodextrin: physiochemical characterization and evaluation of bio-efficacies. CYTA-J. Food 15, 409–417. doi: 10.1080/19476337.2017.1286523
Reyes-Jurado, F., Navarro-Cruz, A. R., Ochoa-Velasco, C. E., Palou, E., López-Malo, A., and Avila-Sosa, R. (2019). Essential oils in vapor phase as alternative antimicrobials: a review. Crit. Rev. Food Sci. Nutr. 1, 1–10. doi: 10.1080/10408398.2019.1586641
Rivera-Calo, J. R., Crandall, P. G., O'Bryan, C. A., and Ricker, S. C. (2015). Essential oils as antimicrobials in food systems-A review. Food Control. 54, 111–119. doi: 10.1016/j.foodcont.2014.12.040
Roy, V., Meyer, M. T., Smith, J. A. I., Gamby, S., Sintim, H. O., Ghodsii, R., et al. (2012). AI-2 analogs and antibiotics: a synergistic approach to reduce bacterial biofilms. Appl. Microbiol. Biotechnol. 97, 2627–2638. doi: 10.1007/s00253-012-4404-6
Silva-Vazquez, R., and Dunford, N. T. (2005). Bioactive components of Mexican oregano as affected by moisture and plant maturity. J Essent Oil Res. 17, 668–671. doi: 10.1080/10412905.2005.9699028
Speranza, B., Corbo, M. R., and Sinigaglia, M. (2011). Effects of nutritional and environmental conditions on Salmonella sp. biofilm formation. J. Food Sci. 76, 12–16. doi: 10.1111/j.1750-3841.2010.01936.x
Srey, S., Jahid, I. K., and Ha, S. D. (2013). Biofilm formation in food industries: a food safety concern. Food Control. 31, 572–585. doi: 10.1016/j.foodcont.2012.12.001
Swamy, M. K., Akhtar, M. S., and Sinniah, U. R. (2016). Antimicrobial properties of plant essential oils against human pathogens and their mode of action: an updated review. Evid Based Complement. Altern. Med. 1, 1–21. doi: 10.1155/2016/3012462
Ultee, A., Gorris, L. G. M., and Smid, E. J. (1998). Bactericidal activity of carvacrol towards the food-borne pathogen Bacillus cereus. J. Appl. Microbiol. 85, 211–218. doi: 10.1046/j.1365-2672.1998.00467.x
van Boekel, M. A. J. S. (2008). Kinetic modeling of food quality: a critical review. Compr. Rev. Food Sci. Food Saf. 7, 144–158. doi: 10.1111/j.1541-4337.2007.00036.x
Wang, H., Zhang, X., Zhang, Q., Ye, K., Xu, X., and Zhou, G. (2015). Comparison of microbial transfer rates from Salmonella spp. biofilm growth on stainless steel to selected processed and raw meat. Food Control 50, 574–580. doi: 10.1016/j.foodcont.2014.09.049
Keywords: biofilms, foodborne pathogens, inactivation, Mexican oregano, essential oil
Citation: Reyes-Jurado F, Munguía-Pérez R, Cid-Pérez TS, Hernández-Carranza P, Ochoa-Velasco CE and Avila-Sosa R (2020) Inhibitory Effect of Mexican Oregano (Lippia berlandieri Schauer) Essential Oil on Pseudomonas aeruginosa and Salmonella Thyphimurium Biofilm Formation. Front. Sustain. Food Syst. 4:36. doi: 10.3389/fsufs.2020.00036
Received: 02 December 2019; Accepted: 17 March 2020;
Published: 24 April 2020.
Edited by:
Paula Teixeira, Catholic University of Portugal, PortugalReviewed by:
Jesus Simal-Gandara, University of Vigo, SpainGuillermo Alfredo Picó, Institute of Biotechnological and Chemical Processes Rosario (IPROBYQ), Argentina
Copyright © 2020 Reyes-Jurado, Munguía-Pérez, Cid-Pérez, Hernández-Carranza, Ochoa-Velasco and Avila-Sosa. This is an open-access article distributed under the terms of the Creative Commons Attribution License (CC BY). The use, distribution or reproduction in other forums is permitted, provided the original author(s) and the copyright owner(s) are credited and that the original publication in this journal is cited, in accordance with accepted academic practice. No use, distribution or reproduction is permitted which does not comply with these terms.
*Correspondence: Raúl Avila-Sosa, cmF1bC5hdmlsYUBjb3JyZW8uYnVhcC5teA==