- 1Latin American Fund for Irrigated Rice (FLAR), International Center for Tropical Agriculture (CIAT), Palmira, Colombia
- 2Latin American Fund for Irrigated Rice (FLAR), Palmira, Colombia
Globally, rainfed crop yields are about 50% lower than yields obtained under irrigated conditions. The low productivity of rainfed agricultural systems is the main factor that accentuates hunger, poverty, unemployment, and illegal migration in Central America and other regions in Latin America. Because of rainfall dependence, farmers can cultivate their crops during only one growing season per year, with high vulnerability to periodic droughts and flooding. To face the challenge of a sustainable food supply in the context of increasing food demand, since the mid-2000s, FLAR (Latin American Fund for Irrigated Rice), a public-private alliance of organizations from 17 Latin American countries CFC (Common Fund for Commodities), and CIAT (International Center for Tropical Agriculture) have been investing resources and efforts to sustainably intensify and diversify high-risk rainfed production systems through capturing runoff rainwater in reservoirs to transform those systems into highly profitable irrigated systems. This article summarizes concepts, results, and experiences from pilot farms using water harvesting in Nicaragua and Mexico, where irrigation combined with smart crop management practices allowed small-/medium-scale farmers to increase their yields of rice, maize, beans, and high-value crops by two to four times in comparison with historical yields obtained under rainfed conditions.
Introduction
Central America has seven countries and four of them are located across an eco-region denominated Central American Dry Corridor (Guatemala, El Salvador, Honduras, and Nicaragua). Climatic conditions in this region are difficult for agriculture because of the unimodal pattern of precipitation that permits cultivating crops only during a 6-month rainy season. From November to April, rainfall decreases and only those farmers that have irrigation can grow crops.
Natural threats in Central America involve drought, floods, volcanic eruptions, fire, and earthquakes. However, drought is the phenomenon that most affects the socioeconomic stability of small farmers because it not only decreases availability and access to food but also diminishes rural income from marginal agricultural production. FAO reports that ~1.6 million people suffer from food insecurity in the Central American Dry Corridor and 3.5 million require immediate humanitarian assistance. The German-watch agency has cataloged the region as the most vulnerable to risks associated with climate variability, due not only to biophysical factors but also to the institutional and socioeconomic weaknesses of the countries.
In Central America, the socioeconomic risk related to drought and more specifically to rainfed agriculture has historically caused problems of poverty, food insecurity, and unemployment, as well as rural migration. Rainfed crop production depends solely on rainfall and farmers cultivate their crops at the beginning of the rainy season with the aim of ensuring establishment by using only the water that the soil is able to retain. However, rainfed agriculture has two major limitations that make it a highly risky system for both food security and employment, as well as for competitive food production. The first is that, when sowing during the 6 rainy months, the lower supply of solar energy prevailing in these months limits photosynthesis and therefore the productive potential of crops to almost half of the genetic potential of varieties and hybrids. The second limitation is related to the seasonality of production and supply to the market. Because farmers obtain their crops at the same time, this causes an oversupply historically followed by a fall in prices, which drastically affects their income.
To offer a technological alternative to risky rainfed systems, the Latin American Fund for Irrigated Rice (FLAR) carried out a CFC (Common Fund for Commodities)-funded project denominated “Transformation of Upland to Irrigated Agriculture through Use of Water Harvesting in Mexico and Nicaragua” (2008–2012). This project, led by the International Center for Tropical Agriculture (CIAT), focused on the introduction and scaling up of water-harvesting technology, which permitted the transformation of 15 rainfed pilot farms to irrigation and validation of improved crop management practices for attaining high yields in Nicaragua and Mexico. The visible impact demonstrated that water harvesting is a sustainable and profitable alternative for small-scale farmers to guarantee an irrigation water supply for the dry season. Those farmers that adopted the technology became prepared to face periodic droughts without suffering losses in their fields and were able to diversify crop production and even include fishery in their system all year long in a competitive way.
Procedure
The transformation to irrigated agriculture intersects the interests and responsibilities of numerous public and private sector stakeholders, including agencies involved in natural resource and water management, along with agricultural production, including crops and fish, as well as groups concerned with local development and poverty alleviation, within the public and private sectors.
Water-harvesting facilities under the FLAR concept contemplate only the construction of civil works on private property where the ecological water flows of micro basins are not affected and never represent a threat to public security (FLAR, 2014). Water harvesting never contemplates damming of rivers and streams or taking water from wetlands or natural reserves, since only runoff waters that descend through pasture lands or crops that were previously affected by erosion or by floods are harvested. The objective of these works of sustainable use of rainwater is always the transformation toward efficient, safe, and productive irrigation systems, whose scale is adjusted to the specific water needs of the crops. We always start from conscious and detailed observations of the dynamics of how and where the water runs, which allows us to install small and simple systems that are adjusted to the water demand of the producers.
The construction costs of a reservoir for the capture of water for irrigation are often a key factor to consider for the transformation to irrigated agriculture. Consequently, it is essential to consider the demand for water when building a reservoir, that is, the size of the reservoir should be in balance with the needs of the system. A reservoir that captures more water than is required would increase irrigation costs. In contrast, a small structure is limited to an irrigated area of smaller size and whose yields would not justify the investment in the reservoir. There are no pre-established rules to determine the size of a reservoir since the water demands of different crops are highly variable and depend on the duration of the cycle, time of year, and type of irrigation to be used. However, some basic considerations can be followed to determine the amount of water required, which will subsequently determine the necessary size of the reservoir.
Identification of Potential Sites
The main concern when selecting a site for the construction of a reservoir is that it has the capacity to fill completely with rainwater. Runoff volume depends on factors such as type of soils, the predominant cover vegetation, the amount and intensity of precipitation, and the topography of the catchment area that corresponds to the zone where the runoff water comes from. A rigorous evaluation of the conditions of the contribution basin is critical to estimate in advance the volumes of runoff that may descend in a given high-rainfall event in a short period.
The analysis of factors such as the amount and distribution of rainfall, temperature, relative humidity, and winds determines the feasibility of implementing water-harvesting systems. Theoretically, the hydrological balance of the area must be positive during most of the year; this means that the annual rainfall exceeds evaporation, permitting harvesting of the excess runoff water that naturally flows through the mountains during the rainy months. The precipitation of the area will determine the capture area necessary to fill the reservoir. The winds, on the other hand, determine the swell, while the temperature and solar radiation of the area determine water loss factors in the form of evaporation. In almost all countries, the national institutions in charge have complete climatological information, which greatly facilitates analysis prior to selecting the site where the dam will be built.
The process of preselection of potential sites to harvest water begins with an appropriate evaluation of the site where characteristics such as soil type, geology, topography, water supply source, and public safety are considered. The ability of a site to build a reservoir depends on the physical ability of the soil to retain water in the storage area. Clay or loam-clayey soils are excellent for the conformation of the dams as they have sufficient clay contents to decrease the risk of possible infiltration. The relief and topography of the site are the factors that most influence the construction costs of the works. For simple economic reasons, the reservoirs must be located in places where an adequate volume of rainwater is harvested and where the minimum amount of soil must be moved for the construction of a small dam. The size of the works is closely linked to the characteristics of the soils and the predominant vegetation in the contributive area that is being drained, since this allows us to quantify the maximum volumes of excess surface water that can be drained on the site during the rainy months. Information on water balance, amount of runoff, and infiltration rate is important for estimating the area required to fill the reservoir. In addition, special attention is always paid to the relief and control structures for excess water in order to minimize the risk of erosion of the dams.
Calculations of potential runoff are widely used in urban studies and agriculture, for which erosion control and watershed management are priority areas. The choice or ability of a particular site to harvest water is proportional to the amount of runoff that goes down the land and, in turn, depends on many interrelated factors that determine the suitability for the construction of a reservoir. In order to anticipate runoff volumes, the biophysical characteristics of the contributive area, rainfall patterns of the area, geology, soil types, and predominant vegetation must be studied in detail. These estimates can be made at the macro level or by individual farms, depending on the purpose of the initiative. Development agencies and governments want to identify regions that are appropriate for harvesting water, while producers want to know which is the best place to build a reservoir on their land.
Runoff capture is partially dependent on the frequency and intensity of rainfall. Areas of heavy rainfall for a short period may experience large amounts of runoff water even if the annual rainfall records are low. A uniform distribution of precipitation throughout the year generally does not result in much runoff water, even in areas where annual rainfall averages are high; this case applies to areas with soils with high infiltration rates. Historical rainfall data on a daily basis are useful for identifying the most intense rainfall events, which allows estimating maximum runoff levels and helps to take measures for the safe management of excess water. This is useful information in hurricane areas such as Central America, where 200-mm precipitation rates can be reached in a single day, which can compromise the safety of the works.
The properties of the soil determine the amount of runoff water since they affect the capacity of the reservoir to hold water, which means that, once the reservoir is full, water will not be lost due to infiltration. The vegetation of the predominant cover in the contributive area exerts a great influence on the amount of precipitation that will become runoff. Cultivated lands with little coverage and a lot of area with bare soil normally have high runoff rates, as well as pastures compacted by cattle.
Preparation of Preliminary Designs
A preliminary design is essential to provide information about the amount of soil movement required to build the dam, the reservoir's water storage capacity, and a general estimate of the dam construction cost. The preliminary design is simple to prepare and can be completed in a few hours, requiring only basic topographic equipment (level or transit, scale, and measuring tape). Following the preparation of the preliminary design, the information is analyzed and some adjustments may become necessary. If feasible, the dam can be relocated to decrease soil movement or to increase water storage capacity. The weight of the dam can be altered by changing the capacity of the dam, taking into account the amount of water required for irrigation on a particular farm.
The information from a preliminary design also allows estimating the relative efficiency of the potential reservoir site. Ideally, a minimum of 10 m3 of storage capacity is a desired volume for each cubic meter of soil moved. However, relative efficiency can be used only as a guide. For crops of high commercial value, efficiency rates below two or three remain economical, but, for crops that require large amounts of water such as low-value grain crops, efficiency rates above 10 are required. Frequently, small adjustments in the location and works of the dam result in an improvement in construction efficiency. The relative efficiency of the construction also provides pertinent information for developing crop arrangements that maximize the economic return per unit of water used. In each country, pilot sites were identified and prioritized based essentially upon economic efficiency of the selected site and the leadership qualities of the participating farmers. Economic efficiency is defined as the ratio of captured water (m3) in relation to soil movement required for constructing the dam (m3).
Designs consists of topography maps of the reservoir illustrating depth contours, designs of the dam, and a summary table with calculations of soil movement required for dam construction and volume of water at selected depths. All designed reservoirs are small to medium capacity, ranging from 0.15 to 1.0 ha, but will contain sufficient water for irrigating a range of crops during the dry season, with the exception of rice. Most of the beneficiaries involved planted beans, maize, and vegetable crops with much lower water requirements than rice. Normally, for irrigated rice, the minimum index is ten; that is, for 1,000 m3 of soil movement, 10,000 m3 of water should be obtained. For maize and beans, the index is about one-half or about five. For vegetable crops with drip irrigation, an index as low as two is economical.
A good design depends on the correct registration of the topographic evaluation and the contours, followed by a correct interpretation of the data. As mentioned earlier, measurements can be taken with simple topographic equipment. A GPS (global positioning system) is useful for determining the area without having to establish each contour, but this can also be done with simple equipment. A GPS can speed up the process, but it is not mandatory. Field measurements are used to ensure the following:
• A plane of the dam that illustrates the elevation layers from the base to the crown of the dam, the location of the water intake in case gravity irrigation is to be used, and the location of the dike through which excess water is removed.
• A plane of the transverse profile of the dam that clearly shows the layers of soil and both slopes (upright and back).
• A meter-by-meter map of the contours of the dam's vessel that allows a measurement of the dam's water storage capacity.
• A summary table of the volume of soil required to form each layer (1 meter thick) and the surface area of each of the contour lines.
The plans graphically indicate both the volume of material movement for each of the layers of the dam and the width and length of the surface layer. According to the topographic measurements taken in the field, the ground floor of the reservoir is drawn digitally or manually. This plan indicates the reference points (meter by meter) of each floor of the dam where the selected soil material is scattered and compacted. The designs also illustrate the contours meter by meter, which is useful in determining the water volume capacity of the reservoir.
Selection of Best Sites
Water harvesting is a most suitable technology for small farmers with limitations in land area to cultivate. With irrigation, these farmers can obtain high income planting small areas compared to planting larger areas without irrigation. The size of the reservoir must be proportional to the size of the surface to be irrigated. Many families have only 1 to 2 ha of arable land, which does not require large reservoirs and for whom designs can be adapted.
During the development of water-harvesting projects, site selection for reservoir construction is a fundamental first step. This requires hard preliminary work to ensure adequate runoff to fill the reservoir, and to avoid large infiltration losses once it is full. Specifications concerning safety are also considered at the design stage. The next step is to prepare a preliminary design to estimate the cost of construction and relate it to storage capacity. This relationship translates into an efficiency index, expressed as the volume of water gained per volume of soil removed for the construction of the reservoir (m3 water/m3 soil removed). For most crops, an efficiency index >10 is desired, although the limit can be decreased for horticultural crops or basic grains of high value. Once a decision has been made, construction proceeds, identifying a source of land for the construction of cutting strips and the ditch. Inadequate attention to these criteria increases the risks of failure.
Studies Required Prior to Construction
Land topography is the single most important factor influencing the costs for constructing catchments. For simple economic reasons, the catchments should be located where the largest storage volume can be obtained with the least amount of soil movement for constructing a small dam. A dam built between two ridges crossing a narrow section of a valley that is immediately below a broad section of valley permits a relatively large area for the catchments with minimal soil movement. It is seldom feasible to develop excavated catchments for irrigation purposes because of the expenses associated with excessive soil movement. The topography of a particular site strongly influences superficial runoff. Steep slopes have high quantities of runoff although this can also happen in small depressions in relatively flat terrain.
A reservoir located in places where a bigger storage volume can be gained with the least soil movement during construction is more efficient. Generally, the best places to harvest water are those with undulating topography from where runoff water converges during the rainy event, and that have a narrow gorge or two ridges that can be joined by constructing a trapezoidal embankment dam (dam). Reservoirs constructed by excavation are in most cases not viable to supply sufficient irrigation water for crops because of their high cost and low capacity.
Water harvesting is dependent upon capturing surface runoff. The quantity of runoff anticipated from a particular watershed depends upon numerous interrelated factors, thus precluding set rules for determining the feasibility of constructing a catchment at a particular site. However, a preliminary evaluation of the most salient features that contribute to runoff permits a rapid evaluation of the suitability of a particular site for harvesting runoff water and maintaining the stored water in a reservoir. The available information to predict runoff should be used before the design phase, which often leads to eliminating potential sites in the initial phase.
Estimating runoff water is of concern to many aspects of development, including urban planning, infrastructure development, as well as agriculture, particularly erosion control and watershed management. Consequently, guidelines have been developed to predict the propensity of an area for runoff. These guidelines are useful in water harvesting and provide a good estimate of the suitability of a particular site. The guidelines include the classification of soils according to runoff potential (referred to as Hydrologic Soil Groups), characterization of vegetative cover based on propensity to runoff, and a combination of the two. Research over the past several decades has permitted the development of runoff curve numbers, a useful tool for estimating the amount of runoff anticipated from a particular site or watershed. The curve numbers combined with precipitation data also permit an estimation of the maximum flow rate, a term useful in predicting the frequency of excess runoff that can result in damage to dam structures and flooding.
After visual identification of a preferred site based on physical characteristics and location, the next step commences with an evaluation of the potential site considering precipitation patterns, soil type/geology, and topography, size, and vegetative cover of the area contributing to surface runoff in order to anticipate the volume of runoff water. In regions of high rainfall or with the possibility of large amounts of runoff during short periods, such as regions exposed to hurricanes, emphasis must be given to controlling large amounts of runoff water. Failures in the dam structure are often a result of poor site selection and/or inadequate attention to the design of structures to dispose of excess runoff. Areas undergoing intense rains from hurricanes require special attention to the disposal of excess water once the reservoir is full. In these cases, the design should include a natural outlet for redirecting the excess water: an extended spillway or means of redirecting runoff water before it reaches the catchment site. Correct site selection and adequate attention to the design phase can significantly decrease the possibility of dam failure. In the initial phase, special attention is given to public safety. For security reasons, a reservoir should never be located at sites where rupture of the dam can cause damage to roads, houses, or other civil structures.
Site selection can commence at a macro level or individual farm level, depending upon the purpose of the initiative. Development agencies and national governments may wish to identify regions that are most appropriate for water harvesting, whereas an individual farmer would want to identify a site most suitable on a particular farm. In either case, some basic information is required, particularly long-term precipitation and geology/soil physical characteristics, since these factors strongly influence the amount of surface runoff and the ability of the reservoir to retain water.
Capturing runoff water is partially dependent upon the frequency and intensity of rainfall. Areas that have intense rains during a short period can experience large amounts of runoff even if the total annual rainfall is low, provided soil types are conducive to runoff. In contrast, uniform rainfall may not result in much runoff water, even though the total annual rainfall may be high, especially under conditions with high soil infiltration rates. Long-term precipitation data, preferably on a daily basis, are useful for estimating the amount of runoff and for identifying maximum rainfall intensity that will permit the design of a suitable means of managing excess water. This is particularly important in areas exposed to hurricanes, where extreme precipitation events can overflow the dam structure. Under these conditions, special attention should be directed to devising means of disposing of excess runoff water.
Soil properties exert large effects on the amount of runoff water and affect the ability of a reservoir to hold water; that is, after the reservoir is full, the stored water should not be lost due to excessive infiltration. Table 1 provides general information on soil properties (commonly referred to as Hydrologic Soil Groups), infiltration rates, and their compatibility for water harvesting. Soil Group A is sandy or gravelly, having soils with a deep and permeable profile. These soils have high infiltration rates (>10 mm/h) with little runoff and are unsuitable for water harvesting. Soils with a limestone base also fall into this class. Water filtration and soil permeability studies were conducted at all sites with designs having infiltration rates ranging from 2.3 to 6.5 cm/day, considered a low value and suitable for water retention.
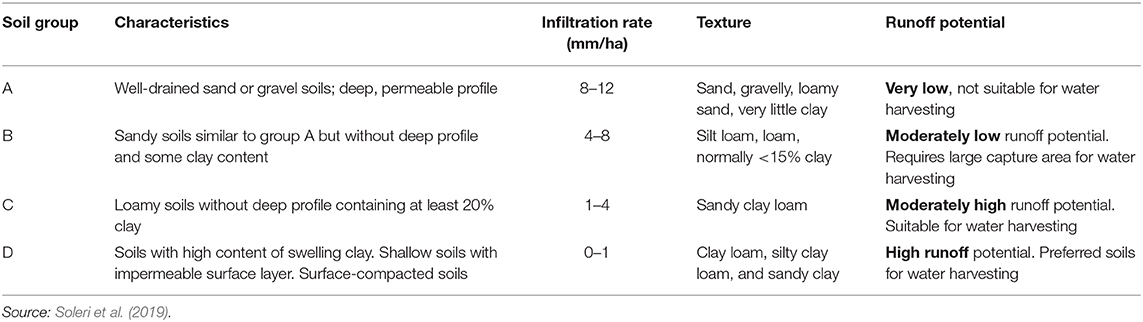
Table 1. Classification of soils according to type, texture, rate of infiltration, resulting runoff potential, and overall suitability for water harvesting.
The vegetation covering the area contributing to surface runoff exerts a large influence on the amount of precipitation that will be subject to runoff. Cultivated land with little ground cover normally has a high rate of runoff; similarly, pasturelands exposed to cattle grazing have rather high runoff rates. In contrast, land covered with native grasses, brush, or forest has decreased runoff. The combination of soil types and vegetation cover permits the determination of runoff curve numbers (CN). Runoff curve numbers are empirical numbers based on research where runoff water has been measured under various soil types and land-use practices. The values range from 30 to 100: the lower the CN, the lesser the runoff potential, and vice versa. Essentially, the curve numbers provide an indication of the permeability of the soil since runoff cannot occur until the soil surface is completely saturated following a rain event. Soils that have high infiltration rates will have low curve numbers. Over-grazing pastures with compacted soils have less water infiltration and higher curve numbers. Cultivated fields left bare result in less water retention, resulting in higher curve numbers. Table 2 provides the curve numbers for various combinations of land use and soil types. Hydrologic Group A is omitted since it has already been determined that soils within this group are not suitable for water harvesting because of high infiltration rates.
Since a great number of variables influence runoff indices, no established norms exist for estimating the catchment area required to fill a specific reservoir. However, there are ways to obtain a rough estimate that is precise enough to prevent the selection of a site that will not have a sufficient amount of runoff. For example, in southern Brazil, ~1,200 mm of precipitation is distributed uniformly throughout the year. The runoff amount is 200 mm. Therefore, a dam with a water mirror of 1 hectare and a depth of 2.5 m requires a catchment area of 12 ha to fill the reservoir. In Rio Grande do Sul, technicians have developed a set of criteria that show that a 10-ha minimum catchment area is required for each water mirror hectare of the reservoir. Studies in Mexico have divided the country into 12 hydrological regions and runoff rates have been estimated for each one of them. For example, in the state of Veracruz, the total annual precipitation is ~1,500 mm, with rains concentrated in a 6-month period, resulting in a runoff rate of 500 mm/year. In consequence, a reservoir with a water mirror of 1 hectare and an average depth of 2.5 m will require 5 ha of catchment area to fill the dam. However, as the Veracruz area is affected by hurricanes and has intense rainfall, it is possible to fill a dam in 1 or 2 days because of this. In northern Nicaragua, average precipitation is ~1,800 mm, but this value is concentrated in 6-months of intense rainfall during the hurricane season, especially in October. During the rainy season from June to October, 800 mm of runoff water has been observed resulting in ~3 ha of catchment area per hectare of dam surface. However, intense rainfall resulting from hurricane influence in October can fill a dam in 24 h. In the driest regions of Nicaragua where rainfall is <1,000 mm/year and runoff is from 100 to 200 mm (diverse soil conditions), a catchment area of nearly 20 ha is needed to fill a dam with a water mirror area of 1 ha and an average depth of 2.5 m. In the south Pacific coast of Costa Rica and in northern Panama, rates of annual precipitation above 4,000 mm are common. In these regions, more attention must be paid to the elimination of excess water using appropriate spillways.
The above-mentioned information gives examples of the variation that can be expected during the establishment of a catchment area required to fill a dam using only runoff. However, some assumptions can be discarded by using a simple observation exercise and talking to farmers. A good practice is to walk in the opposite direction of the runoff flow and try to estimate the total area that is contributing to it. These practices are easily and quickly carried out with geo-referencing tools (GPS). Talking to farmers gives additional information that, combined with observations and some calculations, can generate an approximate estimation of the catchment area required to fill the dam. Finally, the size of the dam can be adjusted during the design phase by taking into account the estimated catchment area.
Analysis of factors such as rain quantity and distribution, temperature, relative humidity, and winds establishes the feasibility to implement water-harvesting systems. Theoretically, the region's hydrological balance must be positive during most of the year, that is, annual precipitation must be higher than evapotranspiration in order to be able to harvest excess runoff rain that drains naturally from the mountains during the rainy months.
The precipitation in the evaluated region will establish the catchment surface needed to fill the dam. On the other hand, winds determine surges, whereas temperature and solar radiation in the region determine water loss factors such as evaporation. In nearly all countries, national institutions in charge of climate have complete and available climatic information, which will ease the analysis prior to site selection where the dam will be constructed.
The filling capacity of a reservoir must be the main factor to be considered at the beginning of the site selection process and long before design preparation. As mentioned before, runoff is a function of rainfall quantity and intensity, superficial evaporation, and soil infiltration. Therefore, the required area to capture the runoff water needed to fill the reservoir will also be a function of these variables. The catchment area corresponds to the superficial area from where runoff water drains during the rainy season and that finally will be responsible to fill the reservoir. In regions with intense and high precipitation, with strong slopes and heavy soils, runoff is high and requires a small catchment area to fill the reservoir. On the contrary, in regions with less precipitation, less steep slopes, and light soils, the catchment area required to fill the reservoir must be bigger.
New Technologies for Quick and Accurate Identification of Potential Reservoir Sites
The exact selection and location of the most appropriate sites to build a functional reservoir on a given property are the technical factors that limit the expansion of technology on a commercial scale in Nicaragua, since it takes a lot of time to perform detailed analyses largely based on visual observations supported by topographic surveys and physical analysis of soils on the farms studied. Recently, FLAR, with the University of Tokyo, has been refining a methodology to increase the efficiency of the selection process for potential water-harvesting sites, supported by detailed geospatial analysis of digital elevation maps constructed from geo-referenced topographic information captured with drones on Colombian farms. The main advantage of using fixed-wing drones of the Sensefly E-Bee type equipped with a photogrammetric camera and two GPS for topographic analysis is the great coverage and speed of capture of spatial information that allow up to 250 ha per day.
The process begins with defining the study area by defining the perimeter of the polygon of the area to fly over in Google Earth. Subsequently, the correct location of the control points and RTK ground stations is established on the ground, and they are used to correct the geolocation of the images. Control points must be visible from the air, so their location in clear places is recommended. Once the polygon has been defined, the drone flight plan is programmed, defining with lines the flight route and height according to the desired coverage and accuracy. In addition, the weather conditions are monitored days before to establish the best date for making the flight. Once the drone finishes capturing the images, the information is downloaded to a computer that processes the data.
Using a large number of overlapping images, Pix4D software is capable of building a photogrammetric cloud of points in three dimensions that indicate the different elevations of the terrain studied. Each point indicates an elevation value separated by a few centimeters with a density of points of more than 20 points per square meter. These point clouds are converted to 3D representations of topographic data with which the digital elevation models (DEMs) of the farms with their respective contours were generated. Among the most common applications of topographic data analysis is drainage modeling, since the models allow determining the direction and flow rate of surface water, which is quite useful for determining how and where to control the water that descends a slope. The high densification of topographic data and the analysis of the contours of the land allowed the accurate identification of the elevations of the most appropriate sites for building reservoirs or collection works.
The products generated are orthomosaic or digital elevation models of the farms that are analyzed with AutoCAD 3D software to estimate both the reservoir's water storage capacity and the theoretical volume of soil movement required for its construction. With this information, the efficiency index of each potential reservoir site is estimated, which is obtained through the relationship between the potential volumes of water accumulated for each cubic meter of soil movement. This value is most useful for making a final decision on the location and cost of potential infrastructure. After defining the location and size of the reservoir, the studies focused on analyzing the soils and the coverage of the contributive area and the requirements of the beneficiary area that will be irrigated with water from the reservoir as well as on the digital design of the works at the most appropriate sites through the use of AutoCAD 3D.
The high resolution of the pixels obtained with the drone of 2.5 m × 2.5 m allows identifying clearly in the orthomosaics and in the digital elevation maps the natural depressions of the land most conducive to the construction of reservoirs, a process that speeds up and greatly facilitates the topographic analysis to determine the potential of the property to harvest water. Once several geo-referenced sites have been identified through the analysis of the spatial information collected with drones, the next step is to estimate their efficiency rates to rate their suitability. Subsequently, at the preselected sites, preliminary calculations and designs of the reservoirs must be carried out as well as an estimated construction budget.
Compared to traditional methodologies for selecting potential sites for water harvesting, drones make it possible to obtain spatial information in an easier, faster, and more precise way. The speed of analysis and coverage of the analyzed area increase substantially with a drone vis-à-vis traditional methods of visual observations on land. Digital elevation models constructed from drones are a valuable input for the analysis and planning processes of the farms of the future, given the enormous number of applications, measurements, and calculations that can be performed in a geo-referenced three-dimensional plane.
Construction of Water-Harvesting Reservoirs
As indicated by the plans, the place where the ground floor of the dam will be erected is marked with wooden stakes. Once the ground floor with stakes is demarcated, a bulldozer cuts and removes the organic layer until firm ground is found. This cut (called a “descapote”) in general fluctuates from 20 to 30 centimeters deep. The practice is necessary to prevent or decrease the volume of water infiltration in the lower part of the dam.
Before beginning the lifting of the dam, we must ensure that no infiltration of water occurs below the structure. The trench or tooth is a square channel that is dug along the central line of the dam, which will be subsequently filled with clay soil and well-compacted, so that it serves as a physical barrier that prevents the leakage of water below the base of the dam.
The water intake is generally located at the lowest point of the dam in order to take advantage of the maximum amount of water. In some cases, it may be located on a layer of 1 meter of compacted material. It is important to emphasize that the water intake can be built or not, depending on the height of the dam in relation to the location of the crop. Once the direction of the outlet is demarcated, a channel whose dimensions depend on the diameter of the pipe to be installed is dug. To ensure the durability and resistance of the work, the tube is inserted into a 3/8-inch iron beam and then filled with concrete. Subsequently, the valve is coupled at the water outlet and cement boxes are made, at both the entrance and the exit of the water.
Finally, a metal grid is placed in the entrance box to prevent the entry of sediment, sticks, turtles, or other elements that can obstruct the dam (not clear; such as fish?).
Once the work is done, the lifting of the dam begins, spreading layers of selected material (clay soil) 15 to 25 cm thick with a bulldozer. The material is subsequently compacted with a vibrating compactor. The material should not be too dry because it would not compact, or be very wet because it would open when compacted. If the material is very dry, it will be necessary to obtain a pipe with water to give it moisture at the time of compaction.
Also called a spillway, the evacuation ditch is a rectangular channel that must have a slope of 5%. The spillway is located from 50 to 100 cm below the maximum height of the dam. Its main function is to evacuate excess water from the reservoir in order to avoid overflows or possible ruptures of the dam. To prevent erosion of the spillway caused by runoff, some mechanisms should be installed to decrease water energy. A good practice is to coat the spillway with plastic and a thin layer of sand and round river stone the size of a hand.
Construction Costs
With information available from FLAR experience in the construction of three reservoirs with a high, medium, and low efficiency index, the costs of a cubic meter of water were estimated under two scenarios of soil removal. With the average cost of soil extraction with the machinery available in Nicaragua of USD 5/m3, the cost of a cubic meter of water is from USD 0.24 to 0.73/m3, with an average cost of USD 0.44. By decreasing the cost of soil removal to USD 3/m3 by obtaining equipment that is more appropriate such as a scrapper, the average cost of irrigation water will decrease to USD 0.27/m3. By amortizing the construction costs of the reservoir over 5 years, the average irrigation costs are USD 0.09/m3 of water with currently available equipment and USD 0.05/m3 with appropriate equipment. Considering a linear depreciation for 20 years results in a cost of only USD 0.013/m3 with appropriate equipment and USD 0.022/m3 with available equipment.
Does the Location of the Reservoir Present Security Risks?
The hydrology of the watershed determines the type of water control structure required. For small storm runoff, an overflow pipe system is normally adequate for disposing of excess water, but, for larger-magnitude storms, an emergency spillway is required to permit excess runoff to be evacuated properly. In areas with large amounts of excess runoff, a bypass ditch is required to divert runoff away from the catchment. The use of appropriate safeguards such as a spillway and bypass channel combined with proper site location greatly decreases the chances of dam failure. All pilot structures constructed by FLAR were located where dam failure would not cause property losses or endanger human welfare.
Environmental assessments were conducted by the national authorities in charge of natural resource management in each county and the licenses for construction and post-project management plans were formulated by the national authorities, who cataloged the project within Category 1 with low to moderate impact over the ecosystem. Inspection visits included activities to verify that there were no affectations for the ecosystem or for the downstream people. Agents proposed reforestation plans for the catchment area, specifying the species of trees to be planted and the planting distances. Likewise, the stability of the dam was verified along with the correct construction of the spillways to evacuate the excess water, thus guaranteeing the continuity of the ecological flow. The maintenance plans of the reservoirs also contemplated the removal every 2 years of sediments accumulated by clogging at the bottom of the reservoir.
How Much Water is Required?
A frequent problem is the underestimation of the volume required to irrigate crops and improve pasture growth, especially in the dry season. Water collected in the first months of the rainy season can be used to provide supplementary irrigation during the rainy season without affecting the amount required for the dry season, since the reservoir will be in a continuous state of recharge in the remainder of the rainy season. The estimation of the amount of water required in the reservoir should consider the potential evapotranspiration (PET) of the crops to be irrigated during the desired time and the losses from evaporation and infiltration in the reservoir and the transport of water to the irrigation system. For example, a reservoir of 1.5 ha and an average depth of 2 m can contain up to 30,000 m3 of water for irrigation, which is enough to supply irrigation for 2 ha of maize, 1 ha of beans, 0.5 ha of vegetables, and 0.5 ha of pastures during the dry season. This considers that evaporation losses can add up to almost 6,000 m3 and another 3,000 m3 because of infiltration.
Water demand for crops is estimated using formulas that consider various climatic variables, including temperature, wind speed, and relative humidity, among others. The classic formula for estimating the water requirement is the Penman equation that derives the PET of the crop. The PET values for various crops in Nicaragua estimated by INTA vary considerably throughout the year. For example, in the northern region of Nicaragua, the PET at the beginning of the dry season is ~110 mm/month, which means that the water demand for crop development is 1,100 m3/ha/month. On the other hand, the PET at the end of the dry season (April-May) increases to almost 168 mm/month, which is equivalent to 1,800 m3/ha/month. In summary, crops require ~70% more water during April and May to maintain sustained growth vis-à-vis December and January. However, PET is also the main engine of production and, generally, the higher the PET, the greater the crop's potential yield (Pulver and Jaramillo, 2015).
To maintain crop development, the PET is the same once the crop has developed. However, variation is great between crops during early development and the duration of the cycle, a factor to consider when building the reservoir. For example, most bean cultivars have a cycle of <90 days, while that of most maize varieties is 120 to 140 days. Bean cultivation at the end of the rainy season requires water for only 2 or 3 months, an estimated 3,000 m3/ha for the entire cycle. On the other hand, the cultivation of maize under irrigation in the dry season requires considerably more water, reaching rates of 5,000 to 7,000 m3/ha/cycle, mainly due to the long duration of the cycle and the high PET during the dry season. Short-cycle vegetables such as eggplant, on the other hand, can be grown with <2,000 m3/ha/cycle since the maturation of the crop takes only 60 days.
There are several forms of irrigation: sprinkler, drip, gravity, and flood. The type of irrigation system to be used will have a direct effect on the calculation of water demand. Drip irrigation systems are highly efficient, but expensive; so, they are normally profitable for the production of crops of high commercial value. Sprinkler irrigation systems are normally used for grain production, but they require pressure pumping and the amount of water lost by evapotranspiration can be high. Gravity irrigation is suitable for row crops, but special attention is required when watering by gravity. Flood irrigation is generally inefficient in the use of water, but commonly practiced in rice and plantation crops.
In summary, the information on the irrigation system to be used is useful for estimating water demand, which determines the size of the reservoir to be built. Drip irrigation is usually the most efficient system, in which 90% of the amount irrigated is used by the crop; whereas, in gravity and sprinkler systems, the crop uses ~60%. Flood irrigation is the most inefficient as the crop uses only 30–40% of the water applied.
To establish the hydrological requirements per cycle for different crops in a given region, various mathematical models can be used. One is Penman-Monteith that uses variables such as the latitude of a specific region, the crop cycle, the PET (potential evapotranspiration), and the Kc (consumptive use constant/crop/stage). In Nicaragua, it was possible to find available and detailed information on the PET for different regions of the country (Salinas and Rodríguez, 1990, INTA). This facilitated the analysis of the hydrological requirements of the crops considered by the project in specific regions to be cultivated during the dry season (Table 3).
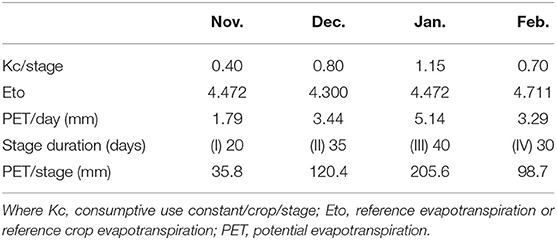
Table 3. Model for estimating the hydrological requirements for 1 ha of maize used for grain of 135 days, sown in November in the Jalapa region (Nicaragua), North latitude 13°55'30”.
Adding the accumulated PET (mm) during the months in which the crop will be cultivated and multiplying this value by 10, we obtain the water consumption in m3 by cycle. For the above-mentioned example, the water consumption for 1 ha of maize cultivated during November in the Jalapa region (Nicaragua) was 4,605 m3/ha/cycle.
Role of Agronomy
Reservoir construction is only the first activity in the transformation of rainfed farming systems into competitive irrigated agriculture. However, based on FLAR's experience, the most challenging task is to convince farmers to apply the recommended smart crop management practices because of their traditional farming culture, the perception of risk of investing in rented land, limited access to credit, and unstable commodity prices, among many other factors. For this reason, the project staff organized multiple practical courses and field activities with extension agents of the national institutions that are in charge of technology transfer, private extension personnel, environmental authorities, and farmers to illustrate the economic benefits of improved crop management under irrigated conditions.
One of the most important and influencing factors on crop yields is taking advantage of the solar radiation that varies throughout the year. Adjusting the planting date to these variations allows the crop to receive maximum solar radiation and express its yield potential. In some countries where solar radiation data are limited, it is possible to estimate this value using historical data of hours of sunshine or calories per unit of area. For example, for rice, the crop's phase that demands higher solar radiation is the one that goes from the start of the floral primordium to the complete flowering phase (45–100 days after sowing). As the highest solar radiation peak in Nicaragua occurs during February and March (~>500 calories/cm2/day), the optimum sowing period for the dry season occurs from 15 November to 30 December. The optimum sowing period for the second cycle is from 15 June to 30 July.
To guarantee optimal plant growth, the crop's PET does not vary once the plants have been established. However, the initial growth rate and its duration are different for each crop. For example, most bean varieties have a cycle of <90 days, whereas maize has a cycle that lasts from 120 to 140 days. A bean plot planted at the end of the rainy season requires water for 2 or 3 months and its water need will be ~3,000 m3/ha/cycle.
For maize with irrigation during the dry season, the crop requires much more water, reaching rates from 5,000 to 7,000 m3/ha/cycle. This is mostly due to its longer growth cycle and higher PET during the dry season. Short-cycle vegetables such as eggplant can be cultivated with <2,000 m3/crop cycle as they reach maturity after ~60 days. Irrigated rice cultivated during the dry season consumes ~7,000 m3/ha of water just to maintain the crop's growth. If water loss through infiltration is considered, water demand might exceed 10,000 m3/ha/crop.
The use of supplementary irrigation during the rainy season avoids yield losses as a consequence of periodic droughts that can be more frequent due to climatic variability. Supplementary irrigation is a practice that does not require a large quantity of water and it is carried out during the rainy season when the reservoir is constantly recharged with rainwater. In this case, a small reservoir is able to supply water to large areas. For example, a reservoir of 15,000 m3 provides additional irrigation to more than 10 ha of maize during the rainy season, but it provides sufficient water for only 3 ha of maize in the dry season.
Full irrigation refers to the practice when a crop's hydrological demand is supplied mostly through an irrigation source with little contribution from rains. This type of irrigation is limited to the dry season and must be adjusted according to the climatic conditions of the region. For example, in drier regions, planting should start immediately after rains have stopped to use residual humidity in the terrain and avoid the quick drying of the reservoir due to evaporation.
The following five agronomic management practices were the basis to increase yields in transformed systems and were applied to improved certified germplasm of rice, maize, and beans:
Plant Population
Rainfed farmers are accustomed to using a large amount of seeds without a technical basis. The negative consequences of high plant populations are weak plants with a limited response to fertilizer and high susceptibility to fungal diseases. The project adjusted optimal numbers of plants in order to obtain healthy plants and avoid interspecific competence.
Seed Treatment
In traditional rainfed systems, farmers spray their crops with toxic and repetitive insecticide applications. Treating seed with specific products protected plants against damage caused by insects during crop establishment. Early application of wide-spectrum non-selective insecticides causes the appearance of secondary pests and is destructive to biological controllers. Treated seeds represent an attractive alternative to substitute for foliar spray applications. Seed treatment with selective insecticides provided effective control of pests during crop establishment without affecting biologically controllers.
Integrated Weed Management
Decreasing crop competition with weeds is essential to obtaining high yield. An effective weed control practice implies crop rotation, adequate soil preparation, use of high-quality certified seeds, balanced nutrition, and optimal water management. Chemical control is only one component in the program. The application of pre-emergent herbicides guarantees effective weed control and the residual control period will be determined by soil moisture during crop establishment. In the case of resurgence, the use of a mixture of post-emergent herbicides when weeds have two to three leaves represented the most effective control.
Balanced Nutrition
In traditional rainfed systems, farmers do not adjust fertilizer quantities based on soil analysis, yield potential, and environmental offer. In some cases, they use recipes of fertilizers that are not adjusted to soil type, solar radiation offer, or crop demand. For cereals such as rice and maize, the project found out that efficient use of nitrogen is a determining factor for attaining high yield. To obtain high nitrogen-use efficiency, farmers need to avoid applying urea on saturated soil due to high losses of the element via volatilization/denitrification.
Water Management
Irrigation is strongly linked with soil preparation and leveling. To guarantee an even water distribution, FLAR recommended the use of a Landplane, which is an implement for soil preparation that levels the soil surface at low cost (less than USD 46 per ha). After using a Landplane, the farmers focused on bund construction, drainage, and equipment installation.
FLAR has validated many crop irrigation systems: drip, sprinkler, canal, and flood. The type of system used by farmers is directly related to crop water needs. Drip irrigation is efficient for water management but is also expensive and economically feasible only for high-value crops such as vegetables or any other high-value market crop. Sprinkler irrigation is commonly used for cereal crops but it requires pumping to obtain adequate pressure. This system implies great water losses through evaporation and drift caused by wind. However, in fields with topography that cuts off water movement through canals, this is the only method that can be used. Irrigation through canals can be used to water crops that are planted in rows, thus facilitating irrigation by gravity. This irrigation system can be inefficient according to soil texture, terrain slope, and evaporation. Gravity irrigation is quite inefficient; however, this is the most common practice in rice and other plantation crops.
Lessons Learned
On pilot farms, competitive production of irrigated crops such as rice and maize represented higher income for growers, reaching rates of up to USD 2,000/ha. Forage production for cattle feed was another alternative for internal use and for sale. Biomass yield of fresh maize was ~15 t/ha, representing additional income of USD 800/ha. Milk production increased almost 4 liters per animal, representing an income increase of USD 75 per animal per year. Tilapia production not only actively empowered women in processing and trade but also improved family income and nutrition. In addition to the economic benefits, the initiative had positive impacts on the environment and natural resource management. The promotion of irrigated agriculture decreases dependency upon rainfed production in Central American countries. Additionally, the adoption of disease-resistant varieties, integrated pest management practices and irrigation, significantly decreased pesticide use. Improved fertilizer management diminished nutrient contamination of water sources and decreased emissions of ammonia, a potent greenhouse gas, from urea fertilizer. Crop diversification opened an opportunity to produce what the market demanded, especially high-value crops that represent higher revenue.
The development of local capacity was the basis to replicate and extend the use of this type of rainwater harvesting system in other countries. To achieve this objective, the project permanently trained farmers and local agricultural technicians in aspects such as selection and characterization of potential sites, topographic surveys, design and construction of reservoirs, installation of different irrigation systems, and agronomic practices for high productivity.
Data Availability Statement
The datasets generated for this study are available on request to the corresponding author.
Author Contributions
SJ: technical implementer of the CFC-funded FLAR project Transformation of upland to irrigated agriculture through use of water harvesting in Costa Rica, Mexico, and Nicaragua and main writer of this paper. EG: research director at FLAR and co-writer of this paper. EP: scientific leader of water harvesting and rice agronomy programs around the world, including the CFC-funded FLAR project Transformation of upland to irrigated agriculture through use of water harvesting in Costa Rica, Mexico, and Nicaragua as P.I. All authors contributed to the article and approved the submitted version.
Funding
All funds were provided by CFC (Common fund for Commodities) project: Transforming of Upland to Irrigated Rice through Use of Water Harvesting in Costa Rica, Mexico, and Nicaragua (CFC/FIGR/15).
Conflict of Interest
The authors declare that the research was conducted in the absence of any commercial or financial relationships that could be construed as a potential conflict of interest.
Acknowledgments
The authors thank Common Fund for Commodities (CFC), International Center for Tropical Agriculture (CIAT), ANAR (National Rice Growers Association of Nicaragua), Proarroz (National Rice Millers Association of Nicaragua), Conarroz (National Rice Board of Costa Rica), Senumisa (Semillas del Nuevo Milenio S.A.), Mexican Rice Council (CMA), Global Communities: Partners for Good, United States Agency for International Development (USAID), and FEDEARROZ (National Federation of Colombian Rice Growers).
References
FLAR (Fondo Latinoamericano de Arroz de Riego) (2014). Plan Estratégico Operativo del FLAR 2014-2018. Palmira: Centro Internacional de Agricultura Tropical (CIAT).
Pulver, E., and Jaramillo, S. (2015). Manual de Cosecha de Agua Lluvia con Fines Productivos. Palmira: FLAR.
Salinas, M., and Rodríguez, R. (1990). La evapotranspiración Potencial en Nicaragua. Instituto Nacional de Tecnología Agropecuaria (INTA).
Soleri, D., Cleveland, D. A., and Smith, S. E. (2019). Food Gardens for a Changing World. CABI. Available online at: http://www.ext.colostate.edu/mg/gardennotes/214.html
Keywords: rainfed and irrigated, water-harvesting, rice, crop management, technology transfer
Citation: Jaramillo S, Graterol E and Pulver E (2020) Sustainable Transformation of Rainfed to Irrigated Agriculture Through Water Harvesting and Smart Crop Management Practices. Front. Sustain. Food Syst. 4:437086. doi: 10.3389/fsufs.2020.437086
Received: 23 January 2019; Accepted: 22 September 2020;
Published: 06 November 2020.
Edited by:
Alberto Sanz Cobeña, Centro de Estudios e Investigación para la Gestión de Riesgos Agrarios y Medioambientales (CEIGRAM), SpainReviewed by:
Guillermo Guardia, Polytechnic University of Madrid, SpainEduardo Aguilera, Centro de Estudios e Investigación para la Gestión de Riesgos Agrarios y Medioambientales (CEIGRAM), Spain
Copyright © 2020 Jaramillo, Graterol and Pulver. This is an open-access article distributed under the terms of the Creative Commons Attribution License (CC BY). The use, distribution or reproduction in other forums is permitted, provided the original author(s) and the copyright owner(s) are credited and that the original publication in this journal is cited, in accordance with accepted academic practice. No use, distribution or reproduction is permitted which does not comply with these terms.
*Correspondence: Santiago Jaramillo, sjara77@gmail.com