- 1School of Environmental Design and Rural Development, University of Guelph, Guelph, ON, Canada
- 2Department of Plant Agriculture, University of Guelph, Guelph, ON, Canada
There is a general unawareness of food crops indigenous to the African continent that have contributed to Western culture. This under-appreciation is particularly relevant in the current context of societal movements to end historic racism and value the contributions of peoples of African origin and African skin colors. Lack of awareness of the contributions of Africa's crops has negative practical consequences, including inadequate investments in preserving and maximizing the use of crop diversity to facilitate breeding. This paper provides an overview and analysis of African crops that have made significant contributions to the United States and globally, and/or hold potential in the twenty-first century. The paper specifically discusses watermelon, coffee, kola, rooibos, oil palm, shea, cowpea/black eyed pea, leafy greens, okra, yam, sorghum, pearl millet, finger millet, teff, and fonio. The review focuses on the intersection of these crops with racialized peoples, with a particular focus on African-Americans starting with slavery. The analysis includes the sites of domestication of African crops, their historical migration out of Africa, their sociocultural contributions to cuisines and products around the world, their uses today, and the indigenous knowledge associated with traditional cultivation and landrace selection. The untapped potential of local genetic resources and indigenous agronomic strategies are also described. The review demonstrates that African crops played an important role in the development of American cuisine, beverages and household products. Many of these crops are nutritious, high value and stress tolerant. The paper concludes that African crops hold significant promise in improving the resiliency of global food production systems, to mitigate climate change and alleviate food insecurity and rural poverty, especially in dry regions of the world. It is hoped that this review contributes to teaching the next generation of agriculturalists, food scientists and international development professionals about the valuable contributions of Africa's resilient crops and peoples.
Introduction
It can be argued that it is common knowledge that maize (Zea mays L.) originated from Mexico, bread wheat (Triticum aestivum) from the Middle East, and black tea (Camellia sinensis) from East Asia (Kiple and Coneè Ornelas, 2000). These crops, like many others from other centers of origin (domestication), have traversed global boundaries and are widely acknowledged for their contributions to the world's farmers and consumers (Nunn and Qian, 2010). Unfortunately, unlike crops originating from other continents, many plant biologists, agriculturalists, and consumers in the Western world may be unaware of the crops that were domesticated in Africa (National Research Council, 2006; Zuckerman, 2016). These crops include (but are not limited to) watermelon (Citrullus lanatus), coffee (Coffea arabica and C. canephora), kola (Cola acuminate and C. nitida), rooibos (Aspalathus linearis), oil palm (Elaeis guineensis), shea (Vitellaria paradoxa), cowpea/black eyed pea (Vigna unguiculata), okra (Abelmoschus callei), yams (Dioscorea sp.), various cereals, and leafy greens (Carney, 2001a). Such lack of awareness is problematic, as the geographic origin of a crop holds the greatest genetic diversity for breeding. Protecting these genetic resources (e.g., continuously evolving farmer landraces and wild relatives) requires public investments, both financial and scientific (Bargout and Raizada, 2013). Such investments have been challenging for Africa, perhaps because of a lack of public awareness (Nunn and Qian, 2010; Cloete and Idsardi, 2013). While the concept of protecting genetic resources is appreciated by crop scientists, sometimes less appreciated is the value of learning from indigenous oral knowledge despite the fact that it can include thousands of years of accumulated agronomic expertise (Altieri, 2001; Bargout and Raizada, 2013). Without knowing that important crops actually originate in Africa, agriculturalists may have not taken advantage of this experience. As our world is facing climate change, food insecurity, and a need for more sustainable agricultural development, these crops, and their associated resources including genetic diversity and indigenous knowledge may, in turn, provide potential solutions and pathways for resilience and adaptation to these issues (Altieri, 2001; Jarvis et al., 2011; Borelli et al., 2020). Finally, given the current reckoning of racism against peoples with African skin colors, it is important to honor the contributions of farmers of African origin.
The objective of this paper is to provide readers with a comprehensive analysis of the contributions that resilient African food crops have made to the United States and beyond. The paper specifically is intended to provide an understanding of the origins of domestication of these crops, their migration histories out of Africa, their contributions to nutrition, cuisine, culture and household products, their current significance to smallholder farmers and known indigenous cultivation practices. For African crops that influenced Brazil and the Caribbean, the readers are encouraged to consult Vandebroek and Voeks (2018). For a more comprehensive quantification of the interconnections between foreign crops and primary regions of crop diversity in the era of globalization, readers should see Khoury et al. (2016). Lastly, it is important to note that in addition to the Trans Atlantic Slave Trade, millions of African slaves were also forcibly exported throughout the Asian Slave Trade (800 AD to 1900 AD) (Collins, 2006). The contributions of these latter enslaved peoples to the provisioning of indigenous African crops to the rest of the world are not included within the scope of this paper, but perhaps offer a future opportunity for inquiry.
Each crop discussed will begin with tracing and understanding their origins of domestication, migration histories out of Africa, contributions to nutrition, cuisine, culture, and household products, along with available indigenous cultivation practices and their current significance to smallholder farmers. Next, this paper will describe the African-American connections to these crops, where noted in the literature, including the contributions made by slaves, in terms of their establishment beyond Africa. The intersection of racism and traditional African crops will then be analyzed. The paper concludes by discussing the untapped potential of these crops to promote resilient agricultural production and mitigate and adapt to climate change impacts.
Specific Crops of African Origin
While only a partial list of crops, this section provides a detailed analysis of traditional African crops and their contributions to the United States and elsewhere. Starting from their centers of domestication (Figure 1), this section will review the journeys of these crops around the globe and their contributions to, and potential for, agricultural development, food security, culture and cuisine, as well as potential pathways for climate change resilience and adaptation.
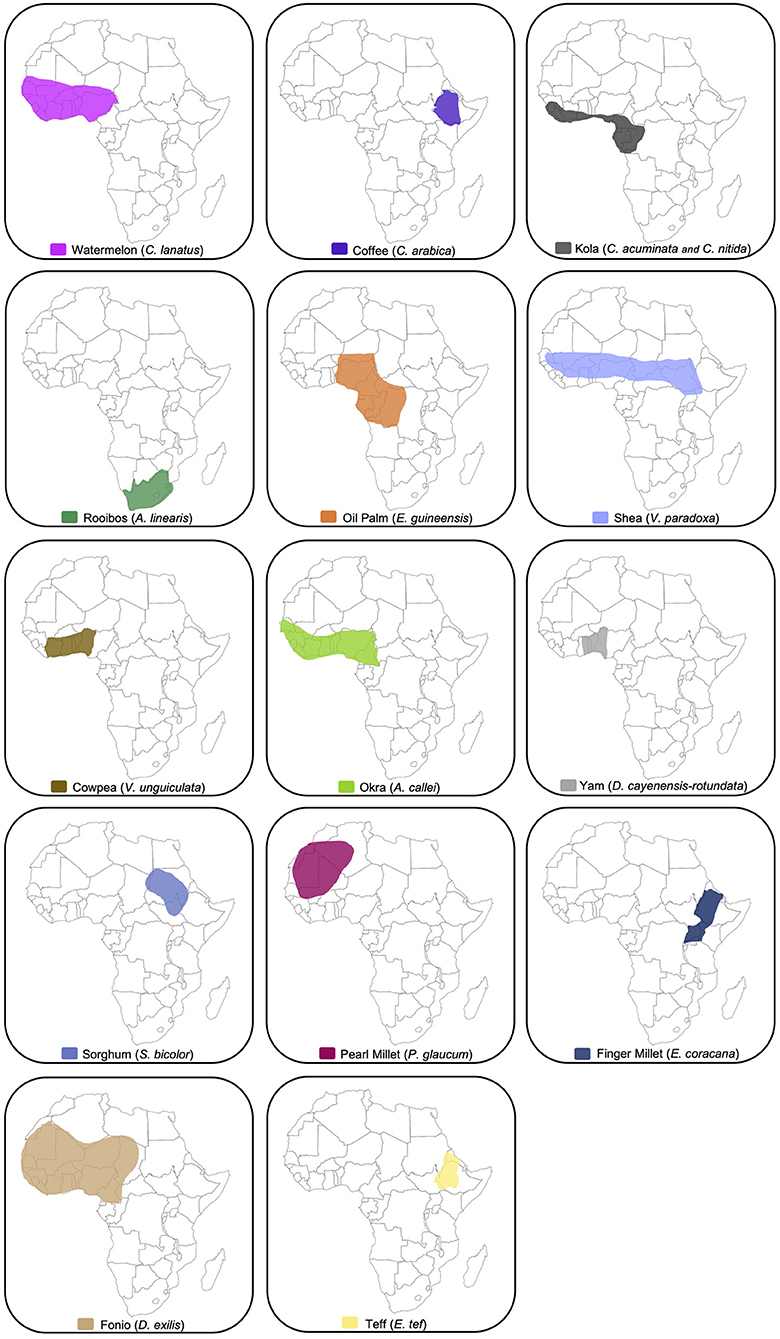
Figure 1. Map showing the hypothesized centers of origin of African food crops. See main text for references.
Watermelon (Citrullus lanatus)
From the nineteenth century to the present day, the watermelon in North America has been a racial stereotype of African-Americans (Figure 2A). The watermelon signified Blacks as “lazy,” “irresponsible,” “criminal,” and as “simple creatures” who could be “satisfied” with a slice of watermelon—despite the deprivation of their civil rights (Lemons, 1977, p. 111; Von Blum, 2012, p. 41). This racial motif emerged during the height of the U.S. Civil War when violence against Blacks was at its worst and the “Negro” caricature was intended to humiliate (Lemons, 1977). Food associated with African-Americans, including the watermelon, eventually became a symbol of autonomy during and after the Civil Rights movement and served as a reminder of the journey captive Africans made through the Trans-Atlantic Slave Trade into America (Mitchell, 2009). Despite this breakthrough, the watermelon today is a reminder of the historical injustices that African Americans continue to face.
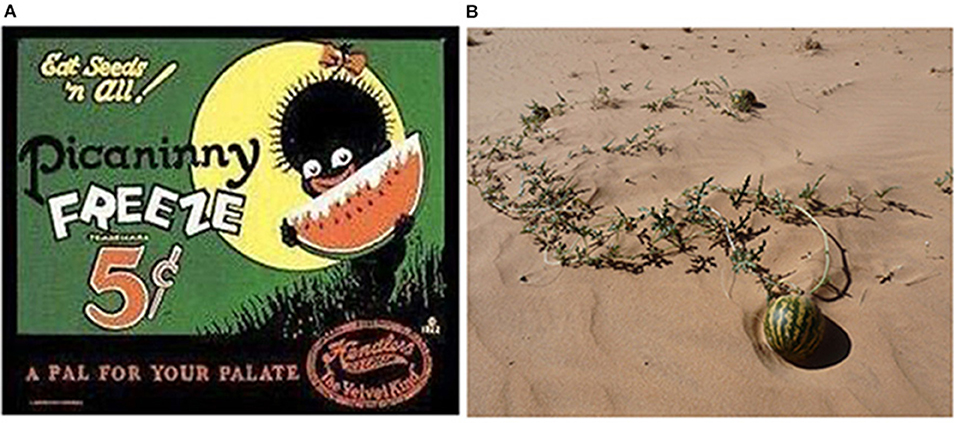
Figure 2. Watermelon (Citrullus sp.). (A) A racist caricature from 1922 depicting the African-American watermelon stereotype. Source: United States Public Domain. (B) Colocynths growing in the Adrar Desert, Mauritania. Source: Ji-Elle. CC BY-SA 3.0.
With the significance of watermelon as a racial trope in the West, watermelons, which belong to the Citrullus genus (Guo et al., 2013), actually originated from Africa. Before the emergence of the watermelon in horticulture, wild watermelons pervaded Pan African landscapes, where dry climates have preserved the seeds, allowing us to learn about their indigenous environments (Zhang et al., 2011; Paris, 2015). The specific origin of sweet, fleshy watermelon (C. lanatus) was widely debated between the northern, southern, and western regions of Africa (Nantoumé et al., 2012; Chomicki and Renner, 2015; Paris, 2015; Chomicki et al., 2019). Recent findings from genomic diversity analysis suggests that C. lanatus was likely domesticated in West Africa (Figure 1) between 4,000-5,000 years ago from C. mucosospermus, which in turn was derived from an earlier melon, C. colocynthis (Guo et al., 2013, 2019; Paris, 2015; Chomicki et al., 2019) (Figure 2B). Both extant ancestors are white, bitter melons, of which C. mucosospermus is widely consumed today in West Africa, not for its fruit, but for its protein- and oil-rich seeds (seeds of egusi melon, formerly C. lanatus ssp mucosospermus) which contribute significantly to food and income security (Achigan-Dako et al., 2008; Jensen et al., 2011b; Paudel et al., 2019).
The earliest archaeological evidence of watermelon (C. lanatus) cultivation was in North Africa, specifically watermelon seeds in Libya more than 5,000 years ago (Wasylikowa and van der Veen, 2004). Thereafter, a painting of an oblong, striped watermelon appears on the walls of an Egyptian pharaoh 4,000 years ago (Chomicki and Renner, 2015). Ancient Egypt has long been recognized as an early site of sweet watermelon cultivation, supported by Ancient Egyptian iconography, Latin, Greek, and Hebrew literature and through archaeological remains of seeds (Andrews, 1956; Paris, 2015).
From ancient Africa, sweet watermelon spread to the Middle East, China, and India, with records dating back to 800 A.D. (Wehner, 2008). Moorish conquerors of Spain introduced watermelon into Europe, but its spread northwards halted as a result of the unfavorable climate for the crop (Wehner, 2008; Paris, 2015). It is reported that Columbus, in his second voyage to the New World, introduced watermelon to indigenous groups and African slaves who then cultivated the crop (Romão, 2000; Dane and Liu, 2007; Pitrat, 2008). There is no archaeological evidence of watermelon in the New World prior to the arrival of Europeans (Blake, 1981). Today, watermelon in the U.S. is primarily produced in the Southern states (Wehner, 2008).
As of 2018, the world harvested more than 3.2 million hectares of watermelon, totalling 103 million tons, making it the second highest fruit crop by production weight in the world [Statistics Division of the Food and Agriculture Organization of the United Nations (FAOSTAT), 2018]. In terms of quantity by tons, watermelon accounts for approximately 12% of total worldwide fruit production [Statistics Division of the Food and Agriculture Organization of the United Nations (FAOSTAT), 2018]. Africa as a continent contributed 6% of this total production globally [Statistics Division of the Food and Agriculture Organization of the United Nations (FAOSTAT), 2018].
Wild Citrullus fruits, as xerophytes, are found in abundance in arid environments, as they need little water to survive, making wild watermelon an essential source of food, fodder, and water during dry seasons (Smith and Cooley, 1973; Yokota et al., 2002; Paris, 2015). Citrulline, derived from Citrullus, is a non-essential amino acid that accumulates in watermelon and is instrumental to oxidative-stress tolerance during drought conditions, including during the seedling stage (Yokota et al., 2002; Zhang et al., 2011; Guo et al., 2013). Other watermelon drought tolerance mechanisms include thick leaves and a deep root system. Due to its drought-resistance, watermelon is noted as a climate change resilient crop (Yokota et al., 2002; Zhang et al., 2011). Nutritionally, watermelon contributes sugars, lycopene (the phytochemical responsible for its reddish pigment), as well as arginine and glutathione, all of which promote cardiovascular health (Guo et al., 2013).
Watermelon additionally provides benefits to smallholder farmers around the world. The crop is usually grown alone or is intercropped with wheat, cassava, maize, pepper, and/or tomato, and improves the profitability of these systems (Adeoye et al., 2011; Nantoumé et al., 2012; Huang et al., 2015). A case study in Nigeria found watermelon grown on smallholder farms provided 100% returns on investment (Adeoye et al., 2011). Studies in China have found that when watermelon is added to a wheat-maize intercropping system, revenues increased by 60% (Huang et al., 2015). An important additional benefit of watermelon is its ability to suppress weeds, as it spreads along the soil surface (Adeoye et al., 2011). The latter trait is especially important in developing nations, where smallholder women farmers can spend up to 50% of their on-farm time removing weeds by hand (Grassi et al., 2015).
Domesticated watermelons have typically been bred for their sweetness or large size (Guo et al., 2019), leading to the disappearance of many varieties over the last century (Parris, 1949; Guo et al., 2013). Unfortunately, many watermelon varieties today are susceptible to different pests and pathogens (Guo et al., 2019). Although there are wilt and anthracnose resistant varieties, further breeding for pest and pathogen resistance is needed (Braun, 1942; Parris, 1949; Adeoye et al., 2011; Guo et al., 2013). Unexploited genetic stocks with potential for further breeding remain in the primitive watermelons in Africa (Guo et al., 2013) including the seven other African species of Citrullus (Guo et al., 2019). In fact, there is genomic evidence of past contributions of these African Citrullus species to disease resistance in modern sweet watermelon (Guo et al., 2019). Therefore, Africa may continue to contribute the benefits of this crop to the rest of the world. Interestingly, indigenous practices as observed in the Tombouctou Region of Mali (West Africa) include the cultivation of multiple types of watermelon side by side in strips within the same plot so as to conserve genetic diversity (Nantoumé et al., 2012).
With the intensification of watermelon production, there is a risk that ancient, indigenous cultivation practices in Africa may be lost (Mujaju and Fatih, 2011; Nantoumé et al., 2012) which might otherwise be informative for farmers elsewhere. Indigenous knowledge is the key to many livelihoods and provides access to genetic resources and conservation strategies (Robinson, 2005; Jensen et al., 2011a; Nantoumé et al., 2012). In North Africa, it appears that watermelon thrives on sandy, well-drained soils (Figure 2B) (Jensen et al., 2011b; Nantoumé et al., 2012). In the Tombouctou Region of Mali, indigenous farmers intercrop watermelon with beans (Jensen et al., 2011b), suggesting that farmers exploit the microbial nitrogen fixation potential associated with beans to help fertilize watermelons. Elsewhere in Mali, watermelon is intercropped with cereal crops (millet, maize or sorghum), interestingly by planting watermelon and cereal seeds in the same hole; one interpretation of this cropping system is that the spreading vines quickly protect cereal seedlings from weed pressure (Nantoumé et al., 2012). The Tombouctou farmers with access to land near a river grow watermelon with the décrue system which uses receding floodwater (Jensen et al., 2011b). This knowledge demonstrates the sustainable exploitation of water sources in the cultivation of watermelon. After sowing, typically no chemical inputs are used, though some farmers apply manure fertilizer (Jensen et al., 2011b).
It is hoped that a wider understanding of the African origins of this major global fruit may lead to a greater appreciation of the cultural importance of watermelons to the African diaspora, as well as new research and investment in conservation and breeding efforts to exploit traditional African watermelon landraces, and of the associated agronomic indigenous knowledge. Additional efforts to make use of watermelon as a nutritious, profitable, drought tolerant crop are especially required in a world in need of solutions to combat climate-change and food insecurity.
Coffee (Coffea arabica, Coffea canephora, and Coffea liberica)
There are many species of coffee trees, but approximately two-thirds of commercial coffee production globally comes from the beans of Coffea arabica (Figure 3A), which was domesticated at least 1,500 years ago in the highlands of Ethiopia (Figure 1) (Anthony et al., 2002). There are additional sites of C. arabica diversity in nearby Sudan and Kenya (Thomas, 1942; Sylvain, 1955). Much of the remaining one-third of commercial coffee comes from C. canephora, the more bitter and caffeinated robusta coffee, which is used for instant coffee and espresso; it originates from lowland tropical forests in Africa (Congo, Cameroon, Ivory Coast, Central African Republic, Guinea) (Leroy et al., 2014). A third African species of coffee, Liberica coffee (Coffea liberica), is less popular globally but is also commercially cultivated, mostly in Malaysia (Saw et al., 2015). Coffee is often consumed for its stimulating effects on the central nervous system. According to Ethiopian folklore, the psychoactive nature of the coffee berry was discovered when a goat herder's goats began to dance on their hind legs shortly after consuming the beans (Pendergast, 1999). Now, the world consumes over 2.5 billion cups of coffee per day (Arnot et al., 2006).
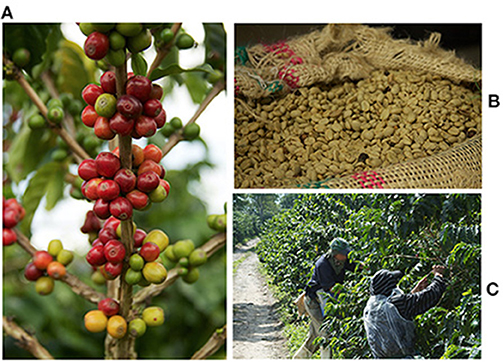
Figure 3. Coffee (Coffea arabica and C. canephora). (A) Coffee (C. arabica) berries. (B) Roasted coffee beans ready to be husked. (C) Plantation workers picking coffee berries in Colombia. Source: M. Savage. CC BY 2.0.
It is thought that the dominant C. arabica coffee spread from Ethiopia to Arab societies through trade, or through the establishment of plantations in Mocha, now current-day Yemen, which was then part of Ethiopia in the sixth century A.D. (Pendergast, 1999; Carney, 2001a; Anthony et al., 2002). From the sixteenth century, the center of power in the Islamic world shifted to the Ottoman Empire, with Constantinople as its capital, and intended to protect their monopoly on coffee (Colburn, 2002). All beans to be traded had to be partially roasted (Figure 3B) to prevent their germination and establishment worldwide (Pendergast, 1999). These efforts were circumvented in the 1600s when a Dutch trader smuggled plants into the East Indies, now present-day Indonesia (Law, 1850; Pendergast, 1999; Carney, 2001a). Records show that coffee was brought to India, a country with a rich and longstanding history with the crop, prior to the establishment of the East India Company by way of Haj pilgrims and traders traveling from Yemen (Wild, 1994). By the end of the seventeenth century, drinking coffee was a habit of many Northern Europeans, leading to the desire to establish plantations in their respective colonies in the West Indies and Latin America in the eighteenth century including in Colombia and Brazil—today famous for their coffee (Aregay, 1988; Pendergast, 1999; Carney, 2001a). Indeed, though Arabica, Robusta, and Liberica coffee originated in Africa, they are now grown on five continents, with most of the world's coffee production concentrating in the Americas [Statistics Division of the Food and Agriculture Organization of the United Nations (FAOSTAT), 2018]. Brazil is the world's leading producer of coffee, producing over 3.5 million tons in 2018 [Statistics Division of the Food and Agriculture Organization of the United Nations (FAOSTAT), 2018]. The establishment of coffee plantations in the Americas (Figure 3C) is a result of the labor from imported African and Indigenous-American slaves, who were forced to work on the plantations of European colonies (Pendergast, 1999; Nunn and Qian, 2010).
While C. liberica is often used as a strong rootstock for grafting other Coffea species, there is an anticipated higher demand for C. liberica due to its lower caffeine levels and clustered fruit maturation, leading to higher quality of coffee and seed weights, which is of growing interest to breeding programs (Amidou et al., 2007; Patay et al., 2016).
The global coffee industry could learn from the traditional agronomic practices associated with African communities' longstanding history with coffee production. The Jimma Highlands of Ethiopia are recognized today to host the greatest genetic diversity of Arabica coffee (Denu et al., 2016; Hwang et al., 2020). Traditional coffee cultivation in the Jimma Highlands is described as “wild coffee,” “semi-forest coffee,” or “community forest” management systems, in which coffee berries are directly harvested from wild plants in natural forests (Gole, 2003; Denu et al., 2016; Wood et al., 2019). Here, as indigenous tree species in the forest are conserved to provide shade for the coffee trees, the ground vegetation between coffee trees is removed, and regenerated coffee seedlings fill in empty space between trees (Hwang et al., 2020). While this practice has occurred since the eighteenth century Ethiopian coffee trade, these systems are reported to be the most sustainable coffee production systems available today as they offer the greatest potential for carbon sequestration and indigenous species conservation (Gole, 2003), especially in comparison to exploitative plantations that require mass land clearing (Denu et al., 2016; Wood et al., 2019; Hwang et al., 2020). However, the remaining forests are increasingly threatened through various modes of degradation. Namely, increasing coffee prices and simultaneous increase in intensity of coffee forest production have led small-scale farmers to remove competing native understory shrubs in natural forests and fallen coffee beans from forest floors, which risks the ability of semi-natural coffee forests to regenerate and ultimately, risks the loss of this diverse genetic hotspot (Gole, 2003; Hwang et al., 2020). Therefore, while there is potential to offset greenhouse gas emissions from intensive agricultural land use by adopting indigenous coffee agroforestry practices (Denu et al., 2016), a question remains as to how global powers, pressures, and policies will affect participatory conservation of natural forests and applicable indigenous knowledge in sustainable production systems, such as wild coffee management systems, and the benefits the world can accrue from these resources in the future (Gole, 2003; Wood et al., 2019; Hwang et al., 2020).
Kola Trees (Cola acuminata and C. nitida)
Coca cola is often considered “as American as apple pie,” despite the fact that its flavor came from kola nuts which originate from Africa (Hall, 2008). Kola nuts are found inside the pods of kola trees (Figures 4A,B) which originate from tropical West-Central Africa, from Sierra Leone to Congo (Figure 1) (Lovejoy, 1980; Carney, 2001a; Angiosperm Phylogeny Group, 2009). Kola made its way to the Americas during the 1700s as a lucrative export crop from Africa (Carney, 2001a). While many cola beverages today no longer contain kola nut extract, the flavor still resembles the taste of chewed kola nuts, which act as a stimulant, much in the same way as coffee or tea (Lovejoy, 1980; Hall, 2008; Durand et al., 2015).
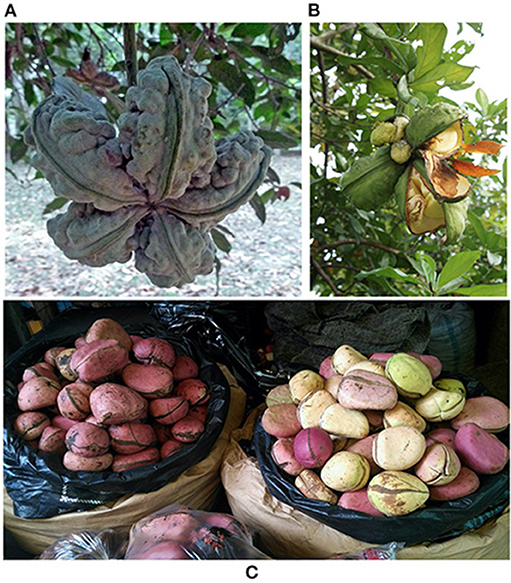
Figure 4. Kola (Cola acuminata and C. nitida). (A) Kola fruit pods. Source: Scamperdale. CC BY-NC. (B) Split kola fruit pods. Source: M. Hermann. CC BY-SA 3.0. (C) Kola nuts for sale at a Nigerian market. Source: N. Chinenye. CC BY-SA 4.0.
In West Africa prior to European arrival, kola was a commercial crop (Hall, 2008) that was used in acts of hospitality for guests in one's home; in cultural or religious rituals including dowries, births, or puberty rituals; to curb hunger, fatigue, thirst, and other ailments; and to signify the wealth and heritage of the owners of the kola trees—even to recognize a new friendship (Adebayo and Oladele, 2012; Durand et al., 2015). For these reasons, kola nuts were desired by African slaves in the New World, leading to their export from Africa (Carney, 2001a). In the creation of soul food, kola nuts were used for flavoring and frying (Mendes, 1971).
Today, there is increased demand for kola, attributed to its use in pharmaceutical industries, leading to its high market value (Figure 4C) (Adebayo and Oladele, 2012; Durand et al., 2015). This demand makes kola an important source of income for many indigenous groups involved in its production; however the demand remains in excess of supply (Adebayo and Oladele, 2012; Durand et al., 2015). As of 2018, all of the world's production of kola came from Africa, with over half of Africa's production situated in Nigeria [Statistics Division of the Food and Agriculture Organization of the United Nations (FAOSTAT), 2018]. Kola production today is at risk of endangerment, due to fast rates of forest degradation, urbanization, and neglect from governments and scientists (Durand et al., 2015). Various West African indigenous groups have different prohibitions with the aim of conserving this crop, including prohibiting the use of productive kola trees as firewood (Durand et al., 2015).
Rooibos (Aspalathus linearis)
Rooibos is an indigenous South African tea crop that grows abundantly in local mountains (Figure 5A) (Van Wyk and Gorelik, 2017). Rooibos represents 10% of the global herbal tea market and is enjoyed in over 37 countries today (Joubert and de Beer, 2011; Wynberg, 2017). The leaves and stems of rooibos, meaning “red bush,” are known for their health-promoting antioxidant-rich phenolic compounds, and as caffeine-free and low-tannin substitutes for coffee and tea (Joubert and de Beer, 2011). There is a huge diversity of cultivated and wild rooibos in South Africa, as well as 52 other species from the Cape region, that are used in the region for traditional herbal teas (Van Wyk and Gorelik, 2017)—most of which are not yet known outside of Africa. The use of rooibos goes beyond tea into cosmetics, novel foods, slimming preparations, extracts, and flavorings (Wynberg, 2017). Sensory aroma profiles of rooibos vary, partly due to differing climatic conditions in production years (Joubert et al., 2016; Jolley et al., 2017). Most samples of rooibos include aroma characteristics of either “rooibos-woody,” “fynbos-floral,” “honey,” and “hay/dried grass,” with varying defining attributes consisting of “fruity-sweet,” “caramel,” and “apricot” (Jolley et al., 2017). These unique sensory aroma profiles open doors for promoting this South African crop to niche markets around the world (Jolley et al., 2017).
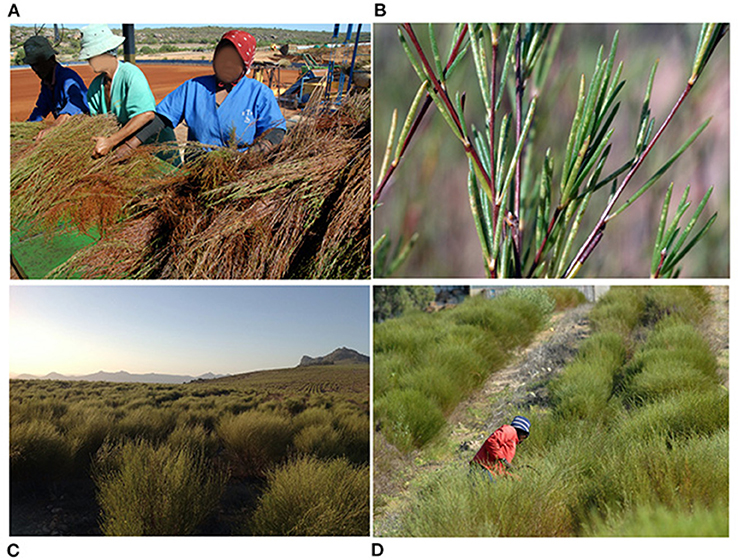
Figure 5. Rooibos (Aspalathus linearis) growing in South Africa. (A) Rooibos bush harvest. Source: R. Bosch. CC BY-SA 2.0. (B) Needle-like leaves of rooibos bush. Source: R. Bosch. CC BY-SA 2.0. (C) Monocultures of rooibos. Source: B. Nel. CC BY-NC-SA 2.0. (D) Woman harvesting rooibos. Source: R. Bosch. CC BY-SA 2.0.
The rooibos industry has not extended beyond that of South Africa (Figure 1), however the industrialization of rooibos production today is built on the knowledge and tradition of the local San and Khoi peoples, after they introduced the species to foreign botanists in 1772 (Wynberg, 2017). The San represent the earliest extant relatives of the first humans (Schlebusch et al., 2012), while the Khoisan culture is known for its click-sound language (Knight et al., 2003). The exploitation of rooibos came about when indigenous groups taught colonial farmers that they could sift through sandy anthills, or “golden nests” around the rooibos bushes to find rooibos seeds accumulated by ants (Ives, 2014; Wynberg, 2017).
Traditionally, the local peoples of South Africa have a special kettle that permanently simmers rooibos to create a dedoction or fermentation, where the heating concentrates it into a non-alcoholic liquor rather than the simple tea infusion method adopted in the West (Van Wyk and Gorelik, 2017). In fact, local tools are used to bruise the leaves against rocks to aid in fermentation. This raises the question as to whether Westerners truly benefit from the health properties of rooibos, if consumed by using ways other than traditional means of preparing this tea.
Rooibos is a legume which associates with symbiotic root bacteria that convert atmospheric gas into usable nitrogen, and thus the crop requires little nitrogen fertilization (Muofhe and Dakora, 1999). Additionally, rooibos's needle-like leaves (Figure 5B) minimize moisture loss, and its vertical root structure is able to absorb water before evaporation, rendering the plant as drought tolerant (Ives, 2014).
While there is an argument that rooibos is a sustainable crop for Southern Africa since it is adapted to that region, increasing monocultures (Figure 5C) of the crop have led to degradation of the Mountain fynbos ecosystem through vast land clearing, habitat destruction, use of chemical inputs, introduction of unfavorable genetic materials, the reliance on a narrow genetic base of rooibos in plantations (Figure 5D), and the overexploitation of wild rooibos to meet global demand (Wynberg, 2017). Fortunately, rooibos is an example of an African crop enjoyed around the world that provides its primary economic benefits to Africa.
African Oil Palm (Elaeis guineensis)
African oil palm is a tree indigenous to the African continent (Singh et al., 2013b), and its reddish fruit (mesocarp) provides palm oil (Figure 6A). The whitish center of the fruit (Figure 6B) is a source of soap, and the origin of the soap brand Palmolive (Gibbs, 1939). Today, palm oil is the most consumed vegetable oil globally (Cramb and Curry, 2012). The center of origin for oil palm is in the tropical rainforest region of West and Central Africa (Figure 1), from Nigeria and Cameroon, southeast to the Congo Basin (Carney, 2001a; Hall, 2008). Fossilized pollen grains of oil palm found in the Niger Delta suggest that the plant evolved in this region millions of years ago (Zeven, 1964). Palm oil was brought by traders to ancient Egypt by 3000 B.C.E. (Kiple and Coneè Ornelas, 2000). It is hypothesized that oil palm trees dispersed from the center of origin across Africa through the migration of floating seeds in interconnected waterways, and through the droppings of seeds consumed by migrating birds (Davies, 1968; Hayati et al., 2004).
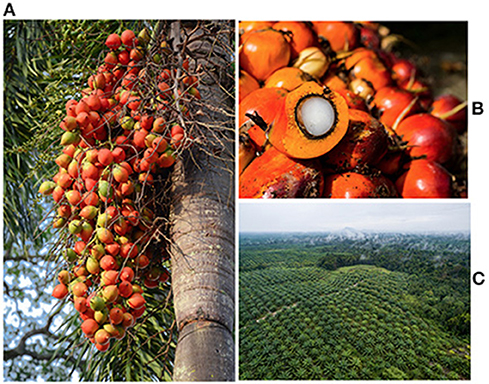
Figure 6. Oil Palm (Elaeis guineesnsis). (A) Oil palm fruits. Source: T. Crazzolara. CC BY 2.0. (B) Whitish center of an oil palm kernel. Source: N. Sujana. CC BY-NC-ND 2.0. (C) Indonesian palm oil plantation. Source: N. Sujana. CC BY-NC-ND 2.0.
Throughout the Middle Passage, oil palm was used in slave ship provisions throughout the 1700s, where many ingredients, both from Africa and Europe, would be mixed in an oil palm sauce to sustain slaves throughout their forced journey—thus contributing to the development of African-American and American cuisine (Hall, 2008). By the eighteenth century, oil palm was being cultivated by slaves in Jamaica and Martinique (Carney, 2001a). The spread of oil palm throughout the Americas eventually reached Brazil, where palm oil is known as dendé and has contributed to Afro-Brazilian cuisine (Carney, 2001a).
Palm oil played a very practical role in the preparation of many African dishes and African-American soul food cuisine. In Africa, palm oil was often used for frying, however as palm oil was not readily available in the U.S. South, slaves were forced to find alternative mediums for frying (Yentsch, 2008). Frying was often the fastest method of cooking (due to the specific high capacity of oil compared to water or air), especially when propane or electricity was/is not available, and many oils, including palm and shea, added extra flavor (Whit, 1999; Yentsch, 2008). Additionally, palm oil played a key role in the trading of slaves—the oil was rubbed onto the freshly shaved skin of enslaved African peoples before selling them in a market to make them look younger (Hall, 2008).
Presently, over 84% of the world's palm oil production (in tons) takes place in Malaysia and Indonesia [Statistics Division of the Food and Agriculture Organization of the United Nations (FAOSTAT), 2018]—another example of non-African nations being the primary economic beneficiaries of African crops. The Asian intensification of palm oil resulted from the Dutch introducing palms from West Africa to the Bogor Botanical Gardens in Indonesia in 1848, and the British introducing oil palm seeds to Malaysia in the 1870s—during the era of pan-continental colonization (Basiron and Weng, 2004; Cramb and Curry, 2012). Though there is another species of oil palm (E. oleifera) which originated in the Americas, African oil palm dominates Southeast Asia (Arias et al., 2015). The Yangambi research station in the Congo facilitated the oil palm Dura and Pisifera (D x P) hybridization, resulting in the tenera fruit form and ultimately a higher accumulation of oil in palm fruit mesocarp, which has led to production increases in Southeast Asia since the mid-twentieth century (Seng et al., 2011; Singh et al., 2013a; Teh et al., 2017; Gan et al., 2018). In addition to these genetic improvements made possible through breeding programs, it is argued that political and economic conditions have made large-scale production of oil palm outside of Africa more feasible than inside the continent (Shevade and Loboda, 2019; Yacob, 2019; Pakiam, 2020).
Oil palm has many edible and non-edible uses, including food industry products (salad dressings, margarine, processed foods, infant and health foods), medicinal uses, medium chain triglycerides (cosmetics and other personal care products), a substitute for polyethylene in food packaging, lubricants, and agrochemicals (used as an inert ingredient in pesticide formulations), renewable energy resources, and waste from its empty-fruit bunches as mulch and fertilizer (Basiron and Weng, 2004; Hall, 2008). Crude palm oil is one of the world's richest sources of carotenoids, containing 15 times the retinol content of carrots—this boosts the immune system and prevents night blindness (Nagendran et al., 2000). Additionally, palm oil possesses significant antioxidant properties and vitamin E (Nagendran et al., 2000; Basiron and Weng, 2004).
Initially, oil palm was promoted by the Malaysian government in a diversification program meant to reduce the dependency of large-scale commercial plantations on Hevea brasiliensis, the rubber tree, in the 1960s (Basiron, 2007; Shevade and Loboda, 2019). Oil palm is a high-yielding crop with a long productive lifespan. The fruit is produced starting at 3 years after planting and continues for another 25–30 years (Cramb and Curry, 2012). Unfortunately, most palm-production takes place on commercial plantations (Figure 6C), leading to vast environmental degradation and the undermining of smallholder farmer and indigenous peoples' livelihoods through global “land-grabs” (Tan et al., 2009; Cramb and Curry, 2012; Obidzinski et al., 2012). The genetic diversity of oil palm has deteriorated due to the clearing of natural oil-palm forests for intensified cultivation (Hayati et al., 2004). Consequently, the intensification of oil palm worldwide has come at the expense of tropical forest biodiversity loss (Koh and Wilcove, 2008). High genetic diversity has been observed in Nigeria, Cameroon, Sierra Leone, and Guinea, thus these regions hold promise for future oil palm improvement (Hayati et al., 2004) as do interspecific hybrids from African oil palm and E. oleifera from the Americas (Arias et al., 2015). Greater investment into Africa to preserve this biodiversity will ensure a brighter future for one of the world's most valuable crops (Sunilkumar et al., 2020).
Shea Tree (Vitellaria paradoxa, Formerly Butyrospermum paradoxa and B. parkii)
Shea butter is found in soaps and cosmetics around the world, but few may realize that it comes from Africa, specifically from the shea tree (Figure 7A) which originates in the African savannah, from the Atlantic Coast, inland to Lake Chad (Figure 1) (Carney, 2001a; Hall, 2008). The crop remains wild or semi-domesticated, and yet it is argued to be the most socio-economically important crop of the dry/semi-arid region of Africa today (Tom-Dery et al., 2018). Presently, two subspecies of shea trees exist, subspecies paradoxa ranging from Senegal to Central African Republic, and subspecies nilotica in Sudan and Uganda (Allal et al., 2011). Evidence suggests that the phylogeographic split of these two subspecies of V. paradoxa between West and East Africa are a result of past climate shifts (i.e., last glacial maximum) which fragmented the distribution of the species across the sub-Saharan part of the continent (Allal et al., 2011). Together, these subspecies are found stretching across a 6,000 km East-West belt covering a total of 21 countries; the trees can represent 70–80% of the woody vegetation in the dry regions of Benin, Togo and Ghana (Tom-Dery et al., 2018). It is hypothesized that the seed dispersal of both shea species across the shea belt occurred because of birds, ungulates, and primates consuming the fruit, as well as humans consuming and anthropogenically shaping savannah woodlands (Tom-Dery et al., 2018).
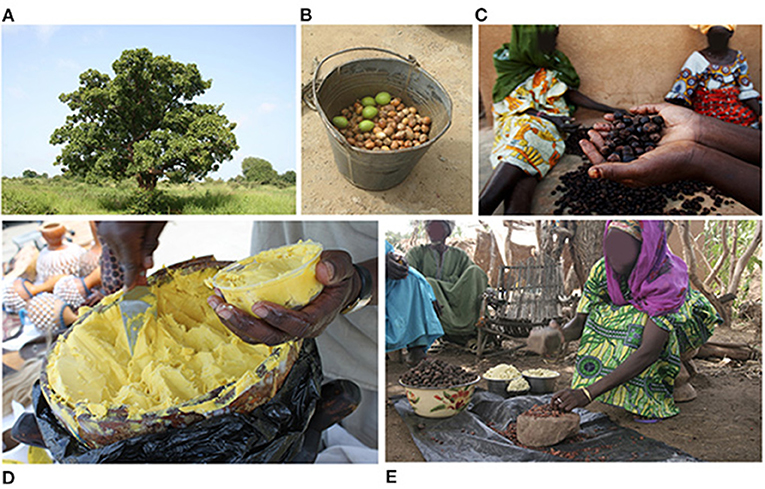
Figure 7. Images of shea (Vitellaria paradoxa) growth and processing from Africa. (A) Vitellaria sp. tree in Burkina Faso. Source: M. Schmidt. CC BY-SA 3.0. (B) Shea nuts and some unripe shea fruits collected in Ghana. Source: F. Khron. CC BY-NC-SA 2.0. (C) Shea nuts. Source: U.S. Government Works. (D) Processed shea butter. Source: Daveynin. CC BY 2.0. (E) Women processing shea nuts into shea butter in Burkina Faso. Source: TREEAID. CC BY 2.0.
Vitellaria trees produce shea nuts (Figures 7B,C) (also known as karité nuts in French) from which a fat is extracted from the seed kernel that is known as “shea butter” (Figure 7D) (Maranz and Wiesman, 2003). The value of shea was widely recognized by British and French colonial administrations of West Africa in the nineteenth century, leading to the start of shea exports worldwide (Carney and Elias, 2006; Elias and Carney, 2007). Similar to the use of palm oil during the Transatlantic Slave Trade, shea was used to “bathe” slaves in West African markets during the Columbian Exchange to make them appear younger to potential buyers. Historical records show how in the Ouamkam Bayou market, in what is now Northern Ghana, shea and other oils were used cosmetically on enslaved peoples just before they were sold and forcibly shipped across the Atlantic (Block-Steele, 2011).
Sub-Saharan Africa continues to provide this desired product to the world, as currently all of the world's production of shea nuts is concentrated in West Africa, with Nigeria (53%) and Mali (24%) contributing the most to this production [Statistics Division of the Food and Agriculture Organization of the United Nations (FAOSTAT), 2018].
Shea has many uses around the world, including as a human food source: the pulp of shea can be consumed as it has a sweet taste due to its sugar content; it is also rich in protein, potassium, calcium, ascorbic acid, iron, and vitamin C; provides an important fat for human diets; and has anti-inflammatory and antioxidant properties (Maranz et al., 2004; Honfo et al., 2014). The nutritional profile of shea has led to its worldwide demand by consumers for cosmetic and pharmaceutical purposes (Honfo et al., 2014). It is also used as a cooking oil and for fueling lighting sources, most commonly in rural West Africa (Maranz et al., 2004; Carney and Elias, 2006). The leaves, roots, and bark of the shea tree are also used in making tools, for medicinal treatments, the litter as fertilizer, and the overall tree as firewood, all of which contribute to the livelihoods of the agriculturalists maintaining these trees (Maranz et al., 2004; Honfo et al., 2014; Elias, 2015).
Shea plays a key role in annual cycles of food security and income generation, especially in communities across the African shea belt: shea ripens at the start of monsoon rains, when food reserves are typically at their lowest (Carney, 2001a; Maranz et al., 2004; Honfo et al., 2014). At this time of vulnerability, various ethnovarieties of shea, as identified by local farmers and resource users through indigenous knowledge systems, provide food and income (Karambiri et al., 2017). For example, shea is part of the filière feminine, a female commodity chain, whereby the crop connects African women producers, whose livelihoods often depend on the harvest and processing of this crop, to Western women consumers, who are the main consumers of shea cosmetic products (Elias and Carney, 2007). Much like the gendered production of yam by males, the production, collection, processing, and trading of shea are performed by women (Figure 7E), often in social settings (Maranz et al., 2004; Elias and Carney, 2007). Processing of butter from shea nuts is derived from ancient knowledge that is passed on matrilineally (Elias and Carney, 2007). Additionally, phenotypic preferences for ethnovarieties of shea have been identified by various gendered and ethnic groups, based on shared geography, knowledge, and experiences in shaping parklands containing shea forest (Gwali et al., 2012; Karambiri et al., 2017). These ethnovarieties include those most resistant to changes in climate, as well as those with precocious shea fruit which bear nuts earlier in the season than other ethnovarieties. By lengthening the fruit bearing period, this trait reduces farmer competition for this valuable crop, providing more equitable access to harvests (Karambiri et al., 2017).
In recognizing the gendered dynamics of shea forest management, harvest, and processing, many women in Sub-Saharan communities act as stewards of shea tree conservation (Gwali et al., 2012). For example, many rural communities in Burkina Faso enforce a 2 week ban on the harvesting of early fruit, or prohibit the felling of healthy productive trees, especially as shea trees show peak production after 45 years but can live for hundreds of years (Carney and Elias, 2006; Elias and Carney, 2007). The shea trees of Africa thus provide inter-generational benefits, not only to local women in West Africa, but also to the world's consumers. Thus, many development initiatives have centered around shea in order to preserve local knowledge around shea ethnovarieties to benefit women, their local livelihoods, and in turn, species conservation of shea in the sub-Saharan context (Karambiri et al., 2017).
Remarkably, shea trees can thrive in as little as 400 mm of annual rainfall, and can survive 8 months of drought (Tom-Dery et al., 2018). The trees can protect the soil from erosion from cycles of drought-rainfall and provide shade to protect annual crop plants. Therefore, this crop may represent a strategy for mitigating and adapting to climate change impacts to agriculture, while providing an important food source to local communities in Africa and beyond. Unfortunately, shea tree numbers are declining, with overexploitation (i.e., overharvesting shea for food, timber, fuelwood, and animal fodder), shifts in eco-climatic zones, and intensive cotton production posing the most significant threats to parklands housing shea tree species (Gaisberger et al., 2017). Both in situ conservation (i.e., preserving local knowledge and understanding local priorities and conflicts over conserving existing genetic resources) and ex situ conservation measures, including the collection of valuable seed sources for planting in alternative climates, are needed (Gaisberger et al., 2017; Karambiri et al., 2017). Moreover, there are concerns about the loss of shea pollinators, as well as the need for domestication and grafting measures to maximize crop productivity and profitability (Karambiri et al., 2017; Tom-Dery et al., 2018). Therefore, more investment research is needed to protect this wild but valuable sub-Saharan African crop for both present and future generations with respect to genetic conservation and cultural agroforestry practices in order to enhance local food security and income generation opportunities during future droughts.
Cowpea/Black Eyed Pea (Vigna unguiculata)
Cowpea (Figure 8A), otherwise known as black eyed pea, crowder pea, and southern pea in the West, originates from tropical west-central Africa (Figure 1), despite the popular belief that its center of origin is within the Americas (Carney, 2001a; Akpalu et al., 2014; Gilmer, 2015). Archaeological evidence has been found of human use of cowpea in Ghana in West Africa dating back to 3600–3200 B.P. with a later finding (1700–1500 B.C.) in India, demonstrating the long-distance migration of this crop by ancient peoples (D'Andrea et al., 2007). Interestingly, it is hypothesized that wild cowpeas dispersed throughout the African continent by birds (Timko and Singh, 2008). Beyond Africa, cowpea is primarily consumed in both the Americas and South Asia. Although it was originally believed that Spanish colonists of the Southwest introduced cowpea to the Americas, it is now thought that African slaves were responsible for its establishment in what is now the United States (Gremillion, 1993). Cowpea today plays an integral role in African-American culture, specifically in traditional soul food dishes such as “hopping John” (boiled black-eyed peas and rice), which have been synthesized from the experiences, history, and journeys of resilience of enslaved peoples (Whit, 2008).
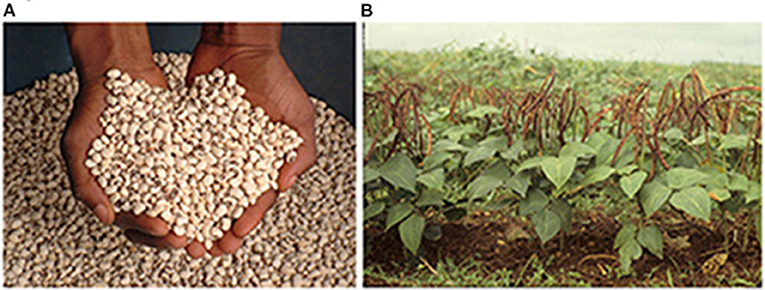
Figure 8. Cowpea (Vigna unguiculata). (A) Cowpea dry grain. Source: Global Crop Diversity Trust. CC BY-NC-SA 2.0. (B) Cowpea plants at the podding stage. Source: IITA. CC BY-NC 2.0.
As of 2018, the world harvested more than 12.4 million hectares of cowpea, with Africa contributing 98% of global production [Statistics Division of the Food and Agriculture Organization of the United Nations (FAOSTAT), 2018]. In Sub-Saharan Africa, cowpea is primarily consumed as a staple food source by over 200 million people (Nono-Womdim et al., 2012). This legume can be consumed in a variety of ways: leaves and immature pods (Figure 8B) can be consumed as leafy vegetables, either boiled, blanched, dried, or fermented, but mainly cowpea is consumed as a dry grain that is boiled or milled (Akpalu et al., 2014; Owade et al., 2020). The way that cowpea is consumed contributes to African sociocultural identities. For example, in many West African countries such as Nigeria, Benin, and Ghana, cowpea is prepared as a purée, made into a dough, milled into a flour, boiled, dehulled and steamed with banana leaf, or even fried—all of which typically are prepared as snacks and/or side dishes with cereals, roots, and tubers (Phillips et al., 2003; Madodé et al., 2011).
In rural areas of many developing nations, cowpea is a critical source of protein and nutrients which is particularly important since animal protein may be inaccessible, and many diets primarily consist of starchy root crops and cereals, which are amino-acid deficient or incomplete, respectively (Akpalu et al., 2014; Ravelombola et al., 2017). In terms of protein, cowpea grain provides the much needed amino acids lysine and tryptophan, along with minerals and vitamins such as folate, thiamin, and riboflavin (Phillips et al., 2003; Singh et al., 2003; Timko and Singh, 2008). Cowpea leaves added to grain cowpea dishes additionally improves their nutritional content (Madodé et al., 2011; Gonçalves et al., 2016; Owade et al., 2020). Overall, cowpeas consumed regularly in diversified diets can assist children and pregnant and/or breastfeeding mothers in attaining nutritional security, or fighting micronutrient deficiencies such as vitamin A deficiency (Akpalu et al., 2014; Owade et al., 2020). The high protein content of cowpea is derived from its association with symbiotic root nitrogen-fixing microbes which ultimately also contributes to sustainable agriculture and restoration of soil health (Akpalu et al., 2014; Martins et al., 2014; Horn et al., 2016). Reports of post-harvest losses of cowpea grain and leaves, however, are high, namely due to challenges in handling, distribution, and nutritional quality deterioration in storage (Owade et al., 2020). Solutions that mitigate these challenges are needed to benefit the smallholder farmers who rely on this crop the most, especially during the dry season when other vegetable crops may be scarce (Seidu et al., 2012; Owade et al., 2020). A promising example is the Purdue Improved Crop Storage (PICS) bag, which reduces post-harvest losses due to fungal pathogens and insect pests at a low cost for smallholders (Baoua et al., 2012).
In addition to its nutritional properties, the other hallmark of cowpea is its adaptability to drier regions, where many legumes normally do not perform well; in fact, cowpea is one of the most drought-resistant crops in regions without irrigation and irregular rainfall in Sub-Saharan Africa and parts of Asia (Singh et al., 1997; Akpalu et al., 2014). The crop is reported to be tolerant to drought even at the germination stage—a critical stage for successful plant establishment (Ravelombola et al., 2017; Carvalho et al., 2019). As a result, cowpea will become increasingly important and desirable in diets around the world, with anthropocentric demands and needs for protein sources concurrently increasing with climate change, and the stresses associated with it such as drought (Carvalho et al., 2019).
Unfortunately, cowpea is susceptible to numerous pests, and viral, bacterial and fungal diseases, as well as root-knot nematodes and parasitic weeds such as Striga (Timko and Singh, 2008), though recent efforts show promise in breeding resistance to Striga (Omoigui et al., 2017b). This means that the diversity of native cowpea landraces in East and Central Africa may serve as useful resources for resistance breeding (Omoigui et al., 2017a; Owade et al., 2020). Furthermore, the knowledge possessed by African cowpea farmers may be informative and beneficial for integrated pest management (IPM) practices, both for Africa and elsewhere. For example, smallholder farmers in Nigeria are reported to burn newly cleared lands to reduce pest populations, practice multi-cropping and mixed cropping to act as barriers to respective pests, and directly apply herbal mixtures as seed dressings, herbal smoke, and pesticides (Kitch et al., 1997). There is an unfortunate decline in the use of traditional pest management strategies, in part due to the perception of traditional methods as “backward” and “primitive” by smallholder farmers themselves (Kitch et al., 1997)—perhaps a legacy of colonialism. Nonetheless, the preservation of this knowledge may prove beneficial to IPM in cowpea production around the globe.
Further understanding of the origins of the cowpea legume may shine light on its significance to the African identity, and potential for future improvement especially in a time of needing to adapt to and mitigate the impacts of climate change. Indeed, cowpea is growing in popularity worldwide, particularly in developed countries, where it is experiencing a growth rate of 2.9% per annum in terms of the area under cultivation (Nedumaran et al., 2015).
African Leafy Greens
Consumption of leafy greens representing a diversity of plant species is common amongst sub-Saharan African diaspora and African-Americans, but culinary use of many African vegetables is gradually being lost in tropical America (Vandebroek and Voeks, 2018). In sub-Saharan Africa, many consumed leafy greens are indigenous to the continent and include (but are not limited to) African nightshade (Solanum scabrum), baobab leaves (Adansonia digitate), jute mallow (Corchorus olitorius, origin debated), leaves of Cucurbitaceae plants, amaranth leaves (Figure 9A) (Amaranthus sp. which have both African and New World origins), spider plant (Figure 9B) (Cleome gynandra), slenderleaf (Crotolaria sp.), celosia (Figure 9C) (origin debated), and other leguminous leafy greens, such as cowpea (V. unguiculata, see above) (Jansen Van Rensburg et al., 2004; National Research Council, 2006; Cernansky, 2015; Moyo et al., 2020). West Africa (Figure 1), from which many enslaved peoples originated, has arguably the world's richest tradition of eating leafy greens (Vandebroek and Voeks, 2018). However, in African-American diets, the leafy greens typically consumed are surprisingly not indigenous to Africa but were introduced to the Americas from Europe via the Slave Trade (Whit, 2008). Using ingredients and techniques from European, American, and African cultures, and even plants introduced to slaves by other indigenous American slaves, slaves synthesized these elements to create African-American cuisine (Whit, 2008). Unlike many white Southerners in the United States, greens were seen as reserved for slaves, whom were resourceful in growing and consuming leafy greens on plantations and subsistence gardens, and even in foraging wild plants (Whit, 2008; Mitchell, 2009). In time, incorporating greens into African-American cuisine, or soul food, became tradition. Types of greens such kale, collard greens, mustard, spinach, poke, cress, turnips, beet tops, dandelion, milkweed, marsh marigold, and chard began to be prepared as boiled greens cooked with meat, usually served with corn bread and “pot likker” (Whit, 2008).
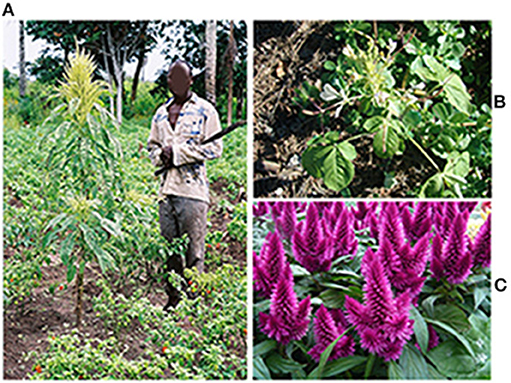
Figure 9. African ‘Leafy Greens'. (A) Amaranth (Amaranthus cruentu). Source: Scamperdale. CC BY-NC-SA 2.0. (B) Spider plant (Cleome gynandra). Source: F. Starr and K. Starr. CC BY 2.0. (C) Celosia (Celosia sp.). Source: B. Pettinger. CC BY-NC-SA 2.0.
Leafy greens, both indigenous to Africa and those used in African-American cuisine, are highly nutritious, rich in calcium, copper, magnesium, manganese, potassium, zinc, iron, folic acid and vitamins A, C, B1, B2, B5, B6, and E, and are useful in promoting brain function, immune system health, and hormone production (National Research Council, 2006; Mitchell, 2009; Moyo et al., 2020). Folkloric reporting on the consumption and use of traditional African leafy vegetables suggests they have other valuable medicinal properties, including the ability to heal peptic ulcers, toothaches, jaundice, and intestinal helminthiasis (Moyo et al., 2020). Moreover, in combination with the above nutritional properties, many African leafy greens are reported to have antioxidant, anti-inflammatory, and anticancer properties (Moyo et al., 2020). Combined, these reports show the potential of African leafy vegetables to improve local food and nutritional security and in turn, prevent and mitigate many non-communicable diseases. However, the typical ways of cooking greens associated with soul food (simmering vegetables for long periods of time and cooking with high fat meats for flavor) compromise the nutritional value of greens (Mitchell, 2009). Soaking up the “likker” from the pot with cornbread, a common soul food practice, provided a way to consume some of the leached vitamins from cooking (Whit, 2008). It is interesting to note the value of teaching such traditional African-American food eating practices.
Today, however, leafy greens indigenous to the African continent are relatively underutilized and undervalued in diets and food systems, particularly among urban consumers across Africa and around the world (Faber et al., 2010; Cloete and Idsardi, 2013; Gido et al., 2017). This is often attributed to a lack of awareness surrounding the benefits of consuming these nutritious vegetables (Kansiime et al., 2018); the belief that consumption of these traditional vegetables is inferior or for the poor (Cloete and Idsardi, 2013; Ronoh et al., 2018); poor post-harvest storage, handling, distribution, marketing practices, along with a lack of access to seeds of leafy vegetables (Muhanji et al., 2011; Croft et al., 2016).
In sub-Saharan Africa, the value of leafy greens goes beyond health benefits and contributions to combatting malnutrition. Agronomically, many indigenous vegetables mature quicker than non-native plants, such as amaranth which can be harvested continuously in 3 week intervals, thus providing large yields within a small plot of land; or celosia which grows like a weed and is tolerant to pests, disease, poor soil, and drought (National Research Council, 2006). Many leafy greens can act as an in situ conservation mechanism, such that the planting of greens preserves ancient genetic heritage of these plants, protects native pollinator species by providing forages, and provides efficient and effective ground covers to protect and restore soil health while suppressing weeds (National Research Council, 2006; Aleni, 2018). Additionally, leafy greens require almost no capital investment, and are almost entirely produced, sold, and prepared by women—offering women a link to markets, income and empowerment (National Research Council, 2006).
A greater appreciation of the value of Africa's leafy greens is needed. These plants are increasingly being displaced, as preference for non-indigenous vegetables dominate markets, and land-clearing threatens the pervasiveness and genetic stocks of these wild greens (Jansen Van Rensburg et al., 2004; Cernansky, 2015; Vandebroek and Voeks, 2018).
Okra (West African Abelmoschus callei, and Indo-African A. esculentus)
African American historian Jessica B. Harris once wrote, “Everywhere that okra points its green tip, Africa has been” (Harris, 2003). Today, okra (Figure 10A) is integral to the food sovereignty, history and identity of many African-American cultural groups, such as the Creole peoples (Marks, 2015). Related to hibiscus (Hibiscus spp.) and cotton (Gossypium spp.), okra was cultivated in ancient Egypt and Abyssinia, including in present-day Ethiopia, Eritrea, and Sudan (Ahiakpa et al., 2017). Two okra species are important commercially: A. esculentus (“Indian okra,” responsible for 95% of global production) and A. callei (“African okra,” responsible for 5% of global production) (Kumar et al., 2010). An interesting feature of both species is their high chromosome number (2n = 130–200) associated with polyploidy (Ahiakpa et al., 2017). The genetic diversity of A. callei is located exclusively in West Africa (Figure 1), pointing to that region as the site of domestication (Figure 1), whereas A. esculentus landraces are located in Northern India (Uttar Pradesh), Africa and globally (Aladele et al., 2008; Kumar et al., 2010; Benchasri, 2012; Werner et al., 2016; Ahiakpa et al., 2017). Therefore, the authentic site of A. esculentus domestication is disputed and requires further combined archaeological and genetic diversity evidence.
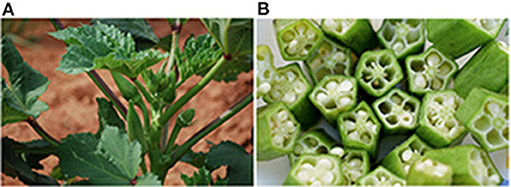
Figure 10. African okra (Abelmoschus callei). (A) Okra plant at the podding stage. Source: M. Shelton. CC BY-NC 2.0. (B) Sliced okra pods prepared for cooking. Source: S. Handa.
Regardless of origin, it is clear that both species, as well as other okra species, have been widely grown throughout Africa for centuries, thus rendering the continent as a launching point for okra into the Americas (Werner et al., 2016; Vandebroek and Voeks, 2018). Okra was in the New World by 1658, specifically in Brazil, and was brought and planted by slaves in Barbados by 1750, leading to its establishment in the Southern U.S. soon after (Carney, 2001a). Okra is also noteworthy for the preservation of slave history and food culture and is commonly remembered through oral tradition (Yentsch, 2008). A general consensus within the scholarship is that okra crops were either shipped to the Southern U.S. as cheap food for slaves, or that slaves brought okra harvests with them on the Middle Passage (Carney, 2001a; Hall, 2008; Whit, 2008; Marks, 2015). Either way, it is regarded that slaves planted okra in plantation gardens for sustenance (Yentsch, 2008). In Southern African-American food history, it is known that slaves often cooked okra as fried fritters or used its mucilage as a thickener in gumbo stews (Yentsch, 2008; Marks, 2015). Creole gumbo (gumbo meaning “okra”) is described as the “heart of Southern Creole food,” which synthesizes the African, Native American, Spanish and/or French cultural elements of Creole cultural heritage (Marks, 2015). Creole gumbo was traditionally accompanied by the left-over parts of a pig including the feet, tails, and chitterlings (small intestines) (Bower, 2008).
Often referred to as “the perfect villager's vegetable,” okra pods are rich in vitamins A, C, K, thiamin, soluble fiber, folate (essential for pregnant women), minerals such as magnesium and calcium, and balanced protein including the amino acids lysine and tryptophan which are deficient in cereal grains (Mitchell, 2009; Kumar et al., 2010; United States Department of Agriculture (USDA), 2020). An twentieth century observer in the Americas noted that okra soup provided “extraordinary health benefits to convalescents and underweight seniors (and could) revitalize those exhausted by sex” (Van Andel et al., 2012). Okra mucilage is useful as a topical medicine; dried and crushed okra seeds are used as a stimulant substitute for caffeine (Figure 10B); while the okra pith (stem fiber) is used in paper and biosorbents (Carney, 2001a; National Research Council, 2006; Benchasri, 2012; Vandebroek and Voeks, 2018). In eighteenth century Guyana, in South America, there are even reports of okra being used to induce abortions (Carney, 2001a).
Today, okra is a global crop. For example, it is widely consumed in the Americas, India, and in the global tropics and subtropics (Benchasri, 2012; Werner et al., 2016), with the majority of global production occurring in India (62%) and Nigeria (21%) [Statistics Division of the Food and Agriculture Organization of the United Nations (FAOSTAT), 2018]. Okra is yet another example of an indigenous African crop, nutritious and stress tolerant, which deserves more attention for its contribution to global food systems and societies.
African Yam (Dioscorea sp.)
Cultivated yams comprise a diversity of global species of which guinea yam (Dioscorea cayenensis-rotundata complex) is the most cultivated and has a history rooted both in Africa and across the Atlantic. Western grocery stores frequently mislabel the small, orange-colored, dicot sweet potato (Ipomoea batatas) from the New World as yams, though true yams are monocots, evolutionary separated from sweet potato by more than 100 million years. Guinea yams are large tubers (5–10 kg each) that originated from a tree species in the Niger River basin between Eastern Ghana and Western Nigeria (Figure 1) (Carney, 2001a; Hall, 2008; Girma et al., 2014b; Scarcelli et al., 2019).
From West Africa, guinea yams (Figure 11A), along with water yams (D. alata from the Asia-Pacific that were later cultivated in West Africa) (Arnau et al., 2017), were introduced to the Americas via large slave ship provisions, where Portuguese colonizers expected slaves to subsist on the crop for the duration of their forced migration to the New World (Carney, 2001a; Hall, 2008; Mitchell, 2009). Once reaching the New World, the use of the introduced yams began to spread by Haitian slaves and black peasants all over the Americas (Hall, 2008), though there are indigenous yam species in the Americas which were, and continue to be, cultivated (Nascimento et al., 2013). According to the FAO, the world produced over 72 million tons of yams in 2018, where Africa's contribution alone makes up 92% of global production [Statistics Division of the Food and Agriculture Organization of the United Nations (FAOSTAT), 2018].
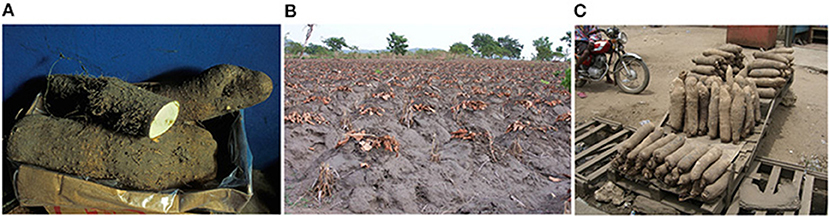
Figure 11. African Yam (Dioscorea cayenensis-rotundata complex). (A) Sliced yam (Dioscorea sp.) Source: A. Denney. CC BY-NC-SA 2.0. (B) Traditional yam mound cultivation technique where mounds are covered with mulch. Source: IITA. CC BY-NC 2.0. (C) Harvested yams brought to a market (see motorbike for scale). Source: L. Kreusel. CC BY-NC 2.0.
Since the beginnings of slavery, yam has been adopted in the diets of the U.S. South, as the crop contributed to the nourishment of slaves in the face of oppression and dehumanization (Mitchell, 2009). Yams, along with other root crops introduced to the U.S. South, were eaten as a mash known as fufu—the same name used in West Africa (Holloway, 2005; Mitchell, 2009). By using blades of their hoes heated under the sun as frying pans, African-American slaves made hoecakes (later evolving into American pancakes) out of fufu (Whit, 1999; Holloway, 2005). Fufu today is a favored dish amongst traditional West and Central African cultures as well as Creole culture in the U.S. South (Holloway, 2005; Mitchell, 2009).
This African crop has a number of advantages. Farmers can easily regenerate yams asexually from pieces of tuber (referred to as seed-tubers), but this convenience can come at the cost of disease susceptibility, since the progeny are genetic clones (Washaya et al., 2016). Conveniently, yams can be left in the ground year-round until they are needed, which is particularly useful in poor countries lacking post-harvest cold storage (Coursey, 1961). This year-round access to a nutritional crop is useful during periods of food scarcity, as it is a valuable source of protein (7% content, greater than white rice), iron, dietary fiber, vitamin C, and carbohydrates (>75% starch) (Muzac-Tucker et al., 1993; Washaya et al., 2016). Yams are utilized mainly as food for human consumption, and can be processed by pounding, boiling, mashing, frying, drying or chipping (Mahalakshmi et al., 2007).
When yams are cultivated in traditional Nigerian farming systems, they are “gendered,” meaning men hold the primary responsibility for their production (Lagemann, 1977). This tradition excludes the role of women in agriculture, thus inhibiting their income generation through limits to access and control over on-farm resources.
Understanding the agronomic practices associated with African yam may provide lessons for the rest of the world. Traditionally, yams were grown in yam-mounds in African slave-plantations, perhaps to facilitate harvesting of the large-sized tubers—preventing having to dig underground (Figure 11B). The nineteenth century military ruler Oluyole in Ibadan, Nigeria, commanded local slaves (not to be confused with African-American slaves) to plant yam seedlings in large mounds of soil, weeds, and plantain stalks, and to have seedling roots grow throughout the mound (Hall, 2008). Farmers in Nigeria are known to fill mounds with palm oil leaves and residues to fertilize the yams (Lagemann, 1977). There are reports that the mound technique significantly increases yam yields by weight, with yields of up to 40 kg each (Figure 11C) (Hall, 2008).
There is historical and cultural significance behind the African yam. Devaluing the uses of yams has led to neglect of their production, and thus their potential roles in food security, income generation, and culture for the rural poor (Washaya et al., 2016). A greater appreciation of yams, including African yams, and their contributions to the New World, may lead to wider cultivation and consumption of this hardy and caloric crop.
African Cereals
Important drought tolerant cereal crops originated in Africa including sorghum (Sorghum bicolor), pearl millet (Pennisetum glaucum), finger millet (Eleusine coracana), fonio (Digitaria exilis), and teff (Eragrostis tef ). Of these, finger millet, teff, and sorghum were domesticated in Eastern Africa (Carney, 2001a), while pearl millet and fonio originated from western Africa (Burgarella et al., 2018) (Figure 1). All of these crops are millets—defined as small grain cereals. As significant portions of the mineral content and dietary fiber of seeds are in the seed coat, and given that these grains are eaten whole, millet grains are excellent sources of these nutrients and make people feel full (National Research Council, 1996). The captains and surgeons on slave ships commented that surplus sorghum and pearl millet from Africa were crucial to feeding human captives throughout the Middle Passage (Carney, 2001a). Slaves adopted cooking techniques associated with African cereals and applied them to Native American crops upon arrival. For example, knowledge of cooking millet porridge was transferred to Native American chickahominy corn grits (Mitchell, 2009). Relative to cereals such as maize (Zea mays), Asian rice (Oryza sativa), and bread wheat (Triticum aestivum), African cereals are not widely grown and/or known in most Western countries today, but they are all important to Africa, and many are critical to the survival of dryland farmers around the world.
Sorghum (Figure 12A) is the world's fifth most important cereal crop, and grows in dry regions of every continent, but was domesticated in Central Eastern Sudan more than 5,000 years ago with thousands of years of continuous archaeological evidence discovered in Egyptian Nubia at Qasr Ibrim (Winchell et al., 2017; Smith et al., 2019) (Figure 1). After sorghum arrived to the United States via the slave trade, the crop was heavily cultivated to be processed into sugar and molasses in the mid 1850s (Winberry, 1980; Rao et al., 2013). Sweet sorghum, in contrast to grain sorghum, refers to cultivated varieties with juicy and sweet stalks useful for syrup production (Rao et al., 2013); it was indirectly adopted into the cuisine of slaves. Slaves used molasses to sweeten meals such as candied yams or porridge, or to make pulled candy; however access to molasses was dependent on the amenities of the slave owner's kitchen (Yentsch, 2008; Mitchell, 2009).
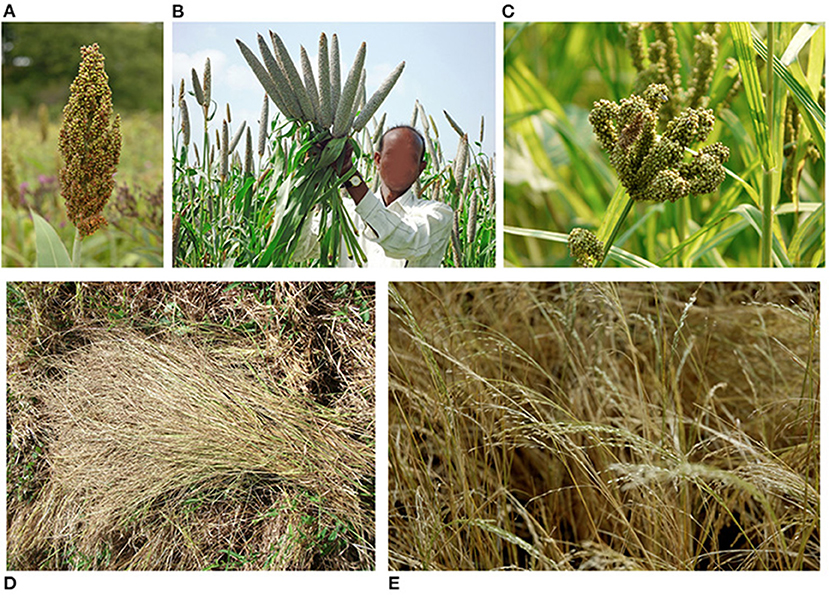
Figure 12. African Cereals. (A) Sorghum (Sorghum bicolor). Source: USDA. Public Domain Mark 1.0. (B) Pearl Millet (Pennisetum glaucum). Source: CGIAR. CC BY-NC-SA 2.0. (C) Finger millet (Eleusine coracana). Source: D. Valke. CC BY-SA 2.0. (D) Fonio (Digitaria exilis). Source: T. Passages. CC BY 2.0. (E) Tef (Eragrostis tef). Source: ILRI. CC BY-NC-SA 2.0.
Today, grain sorghum is produced for human consumption whereas sweet sorghum is consumed for processing into syrup and/or biofuel (Sami et al., 2013). In general, sorghum is on its way to losing its reputation as an underutilized crop. Research over the last two decades has supported the development of this crop in order to maximize its agronomic potential, both in feeding the world and in counteracting climate change. For example, the diversity in sorghum genetics has led to the development of resistance to striga (a parasitic weed), pests and disease (including bird pests), biofortification (particularly of vitamin A), tannin-free genotypes and improved protein quality, along with tolerance to cold, heat shock, and drought in both commercial and traditional sorghum varieties (Rao et al., 2013; Akinseye et al., 2017; Reddy, 2017). Currently, sorghum is a staple food source for more than 500 million humans, and a critical source of feed and fodder for animals, in more than 30 countries around the world (Akinseye et al., 2017). Despite these genetic improvements which are of value to agricultural systems and diets around the world, 70% of global acreage dedicated to sorghum production remains situated within the African continent [Statistics Division of the Food and Agriculture Organization of the United Nations (FAOSTAT), 2018].
Pearl millet (P. glaucum) (Figure 12B) was domesticated in the Western Sahara more than 4,900 years ago (Figure 1) at the intersection of Southwestern Algeria, Northeastern Mauritania and Northwestern Mali, and reached South Asia in ancient times (Oumar et al., 2008; Clotault et al., 2011; Manning et al., 2011; Burgarella et al., 2018). Interestingly, in the last 1,000 years, pearl millet has had a history of being cultivated in agroforestry systems, particularly under the canopies of shea trees (Gwali et al., 2012). It is one of the most drought tolerant of all major cereals and today remains a major food crop throughout Africa and India (Jukanti et al., 2016; Serba and Yadav, 2016).
Finger millet (E. coracana), its name derived from its grain head shaped like fingers (Figure 12C), was domesticated in East Africa (Western Kenya to the Ethiopian highlands) 7,000 years ago (Figure 1) and reached India by 3000 B.C. (Hilu and de Wet, 1976; Hatakeyama et al., 2017). Finger millet is one of the richest grain sources of minerals including calcium and the amino acid lysine which is deficient in many other cereals (Chandra et al., 2016). Today, the crop is cultivated throughout Africa, India, Nepal, China, and Japan (Goron and Raizada, 2015).
In comparison to the millets and sorghum, fonio and teff are lesser known crops outside of Africa. The major benefit of white fonio (Digitaria exilis, Figure 12D), native to West Africa (Figure 1) (Olodo et al., 2018; Abrouk et al., 2020), is that certain varieties mature within 6–8 weeks of being planted (National Research Council, 1996), the fasting known maturing cereal. The short duration of white fonio may allow farmers to grow a nutritious cereal at the onset of the dry season using residual moisture from the rainy season or during shortened rainy seasons, expected to become more prevalent due to climate change. Given that the vast majority of chronic malnutrition globally occurs in the sub-tropics during extended dry seasons (e.g., Sahel, India), this crop could thus contribute to the alleviation of hunger and poverty. Indeed, fonio can provide food in the critical mid-season hunger period, before longer duration crops can be harvested (Vall et al., 2011). Nutritionally, white fonio has a high mineral content and possesses a balance of amino acids including tryptophan, cysteine, and methionine, which are deficient in many other cereals (Adoukonou-Sagbadja et al., 2006). Despite the benefits of fonio, it remains an incompletely domesticated crop, with seed shattering and lower yields—luckily, many landraces in West Africa exist based on varying maturity classes, which could prove useful for the future development of this crop (Ayenan et al., 2018). Currently, all global fonio production occurs in Africa, with over 70% taking place in Guinea [Statistics Division of the Food and Agriculture Organization of the United Nations (FAOSTAT), 2018]. Fonio has significant untapped potential and could be more widely shared beyond its current borders, particularly to mitigate climate change.
Teff (Figure 12), a staple crop of Ethiopia and Eritrea, is commonly used to make enjera bread. Evidence shows teff was domesticated in Ethiopia at least 2,000 years ago (Figure 1), but is possibly much more ancient (D'Andrea, 2008; Chanyalew et al., 2019). Teff has many underutilized benefits: it is resistant to heat, many diseases, pests, heavy and poor nutrient soils, and can be used as a ground cover for suppressing weeds, while the stover is useful as a building material (National Research Council, 1996; Chanyalew et al., 2019). Teff is also an excellent source of balanced amino acids unlike the world's major cereals, and similar to the other millets, it is consumed whole with its mineral-rich seed coats; it is accordingly valued by indigenous cultures to nourish pregnant women and children (Girma et al., 2014a; Chanyalew et al., 2019). As a “lazy-man's crop,” teff can be grown merely by broadcasting seeds onto the soils of recessional flood plains (National Research Council, 1996). Perhaps most critical, teff grain maturation can occur even during post-flowering drought (Girma et al., 2014a), which may become more prevalent; this tolerance is an unusual trait compared to most cereals. However, like the other millets from Africa, teff suffers from low yields (including from lodging) compared to the world's major cereal crops (at least under ideal conditions) (Girma et al., 2014a) and contributes to significant post-harvest labor for women (Chanyalew et al., 2019). Compared to the other lesser explored African cereals discussed in this paper, teff is relatively more well-known in Western countries, as it has a history of being promoted as a superfood throughout Europe in recent decades (Gebremariam et al., 2014; Koubová et al., 2018).
It is important that the development of sorghum, African millets, fonio and teff continues to provide solutions to the challenges posed by climate change worldwide. All are gluten free, thus providing opportunity to enhance their use and value as grains for people living with celiac disease (Girma et al., 2014a; Chanyalew et al., 2019). It may be that one day, pearl millet, finger millet, fonio, and teff will be as widely cultivated outside of Africa as sorghum is today.
Discussion
The Intersection of Racism, Human Resiliency, and Africa's Crops
African crops hold a hidden truth behind how and why certain cultural and food histories are appreciated, and others are ignored. It should not be surprising that a large proportion of African crops grown and/or used throughout the world today originated from West Africa (Figure 1), since this region's ports were critical for the trans-Atlantic slave trade (Carney, 2001a). Furthermore, the West African region is gradually being identified as a major cradle of ancient crop domestication (yam, cowpea, sorghum, pearl millet, African rice, numerous leafy greens) to rival the more famous Mesopotamia and Mexico, to help redefine human food history (Scarcelli et al., 2019). While beyond the scope of this paper, a very useful area of future study would be to explore the impact of the slave trade from Africa to Asia and elsewhere on modern cuisine and agricultural resources. This appears to be less well-studied, as studies of the Trans-Atlantic Slave Trade has often overshadowed the institutionalization of enslaved Africans elsewhere (Collins, 2006; Lovejoy, 2012).
Twelve million slaves were stolen from Africa and shipped to the Americas between 1501 and 1866 (Nunn, 2008) including 4 million to the Caribbean islands and 400,000 to the United States (Voyages: The Trans-Atlantic Slave Trade Database, 2020). The persecution associated with the trans-Atlantic slave trade was backed by negative and racist ideologies that viewed indigenous and traditional food systems as inferior (Carney, 2001b; Turner and Turner, 2007; Coté, 2016). This view of inferiority is perhaps a side effect of why there are few preserved histories told from the perspectives of enslaved peoples and an accurate or fair representation of their contributions to agronomy and cuisine today (Carney, 2001b; Slocum, 2010; Chatelain, 2015; Gilmer, 2015). And yet, settlers extracted and exploited these same crops for their very own gain, as they had done with crops of indigenous communities in settler-colonial North America (Wolfe, 2006). It was, after all, African slave labor and knowledge of growing these crops that migrated out of Africa, which fed colonists, slaves, and slave owners, and partially led to the development of European colonies, food capitalism, and the current foodways in America (Carney, 2001a; Wolfe, 2006; Slocum, 2010; Esch and Roediger, 2014). The private property system imposed through colonialism played a large role in continuous land theft and the assimilation of indigenous groups around the globe (Wolfe, 2006), thus contributing to the negative intergenerational impacts on these communities, knowledge systems, and traditional uses of crops in our food system today (Borras and Franco, 2010; Peluso and Lund, 2011; Coté, 2016). Indeed, a common theme that emerges from examining the global crops that originate in Africa is racism. By acknowledging and appreciating these crops, and the injustices (i.e., slavery) associated with a subset of them, there is an opportunity for reconciliation. Additionally, in reconciling, celebrating, and conserving the histories and benefits these crops, there is a further opportunity for fostering equity and enhancing resilience in African communities, and learning from their knowledge systems to inform our shared food systems (Holt-Giménez, 2015).
In addition to forced slavery, Africa was colonized. While many of the crops native to Africa have delivered many benefits around the world, Africa has not had its fair share, impeded by colonization and historical exploitation including the persecution of indigenous groups (Maclean, 2002; Lange, 2004; Bjornlund et al., 2020). While Africa has, in some ways, benefitted from crops with origins outside of the continent (e.g., maize, cassava, peanut, Asian rice, etc.), these introduced crops have frequently displaced local crops (Bjornlund et al., 2020). For example, peanut, a native Brazilian crop widely grown in Africa, has largely overshadowed the nutritional, agricultural, economic, and culinary value of the bambara groundnut (Vigna subterranea), an African counterpart possessing equally important benefits in terms of nutrition and livelihoods (National Research Council, 2006). Colonization did not focus solely on nations, but on undermining people and their foods, histories, cultures, and local development (Turner and Turner, 2007; Coté, 2016). Similar to slavery, institutionalized colonial policies fed the racist view that African peoples and their crops, were inferior, and not advantageous in terms of economic or agricultural development (Demi, 2014). Even today, Africa is mainly studied through a Western-centric lens which focuses more often on crises related to food than Africa's indigenous plant domesticates, culture, histories, and practices (Akena, 2012; Bjornlund et al., 2020). Furthermore, the histories and potential of Africa's crops have been overshadowed by the focus of knowledge-transfer from the West (Carney, 2001a; Akena, 2012). Perhaps, these are the reasons that many Western consumers rarely know of the African origins of the foods and household products they consume (Zuckerman, 2016). Although, to be fair, do most Africans know that crops such as maize and cassava, which are central to many African food traditions today, originate from the New World?
Today, there are global movements to end anti-Black racism against African-Americans and other peoples of color (Burch et al., 2020). More than ever before, understanding Africa's food contributions has particular implications for African-American cultures and the wider society, particularly to deconstruct the racialized stereotypes of certain foods, like that of watermelon and the racist and derogatory “coon” caricature noted earlier (Lemons, 1977). For African-Americans, many of the crops discussed here, including leafy greens, yam, cowpea/black eyed pea, and okra, were key ingredients that comprised many soul food dishes accompanied by animal parts that were despised by slave owners and thus given to their slaves to eat (Vester, 2015). Thus, as a cuisine, soul food today is a mode of resistance, and a signal of resilience and racial pride, as African Americans used creativity to incorporate these ingredients, historically used to devalue their worth as humans, into their cultural cuisine (Slocum, 2010; Vester, 2015). By recognizing the history of soul food as well as other foods not popularly associated with Africa [e.g., coffee, cowpea (black eyed peas)], African-Americans have the capacity to connect with their ancestors or ancestral traditions from whom they may have been torn from due to slavery. With connections to food comes the opportunity to fill in the gaps of one's own personal story of resilience through adversity—and for others to recognize such resilience, including the world's current and future agricultural researchers, food scientists and international development professionals.
Future Potential of Africa's Resilient Crops
It is apparent from this review that Africa's crops, including watermelon, cowpea, leafy greens, okra, African yam, cereals, kola, coffee, rooibos, oil palm, and shea, have contributed to the world's farmers and consumers (Carney, 2001a). These crops have a wealth of untapped potential, given their benefits to nutritional diversity and security, plant breeding, biodiversity conservation, as well as resilience to environmental stress posed by the increasing need to mitigate and adapt to climate change impacts, and other global demographic and socioeconomic trends impacting agricultural production.
While climate change impacts will disproportionately impact African agriculture (Niang et al., 2014), the potential solutions may also be situated on the continent. The availability of these solutions, however, depends on the ability to conserve and sustainably utilize these crop resources. For instance, many of the African crops described in this review exhibit and possess traits for heat and drought tolerance, likely because they originated in the dry subtropics of Africa or regions with extended dry seasons. These crops include cowpea, sorghum, fonio, pearl millet, teff, watermelon (Fahad et al., 2017), and also other crops that were not the focus of this review (e.g., bambara groundnut) (Mabhaudhi et al., 2018). Furthermore, Africa's agroforestry crops (including palm, kola, coffee, and shea) have the potential to sequester carbon to mitigate and adapt to climate change impacts in agriculture (Mbow et al., 2014; Tschora and Cherubini, 2020). The low-input requirement of many of these crops is another principal reason why they should be used for sustainable agricultural development and climate change mitigation and adaptation purposes (Mabhaudhi et al., 2019). Climate change calls for the need to decrease the reliance on natural gas consumption associated with nitrogen fertilizer production (Mancus, 2007). In agronomic terms, this means shifting a more significant proportion of production to crops such as cowpea and rooibos, because of their association with nitrogen-fixing bacteria, as well as low-nitrogen tolerant crops like teff (Muofhe and Dakora, 1999; Akpalu et al., 2014; Assefa et al., 2015).
Despite these benefits, the fact is that many of Africa's indigenous crops have limited yields (e.g., teff, fonio), compared to crops such as maize, Asian rice, wheat, and soybeans, at least under ideal conditions and with chemical inputs (Girma et al., 2014a). Limited yields partially explain their underutilization in agriculture, even within Africa (Bjornlund et al., 2020; Borelli et al., 2020). Once again, Africa holds the solution: the traditional cultivation practices and landraces established by indigenous peoples in Africa, as well as the high genetic diversity at the centers of origin of these crops, hold the knowledge and stress-tolerance alleles needed to enhance agronomic practices and plant breeding, respectively (Ajani et al., 2013). African genetic resources may be used to restore the biodiversity lost from modern crop production systems, including coffee, kola, and oil palm, which today have a narrow genetic base following generations of intensive selection (Hayati et al., 2004; Durand et al., 2015). By investing in the preservation of these genetic stocks and landraces, these resources will be available for future breeding and development efforts. To enable such advancements, we recommend a greater global and philanthropic investment for neglected African crops, such as fonio, to establish seedbanks and breeding programs within the Collaborative Group of International Agricultural Research (CGIAR) system. For seedbanks of African crops that exist outside of Africa, some thought should be given to relocate or duplicate certain seedbanks in Africa (e.g., millets from ICRISAT in India). The goal should be to better integrate these seedbanks with domestic breeding programs and into communities, with the appropriate funding to address local African needs.
Fortunately, there have been investments by the Gates Foundation to fund the breeding of certain crops discussed, such as cowpea, which build upon highly-effective, long-term efforts by the CGIAR centers (Fatokun et al., 2020). Despite these investments, these institutions are typically cash-strapped given their broad global mandate to address a diversity of crops and challenges, as well as their inclination to further advancements in dominant global crops oriented for export (Bjornlund et al., 2020). Pressure should be placed on multi-national companies that profit from African crops (e.g., the coffee industry) to help fund such local African crop initiatives.
With respect to cultivation practices, it is interesting (and perhaps unsurprising) to note that whereas people migrate crops, the associated indigenous knowledge is often left behind (Mabhaudhi et al., 2019; Borelli et al., 2020). As one example, in pre-Columbian Haiti, cassava, beans and maize, indigenous to the Americas, were intercropped in conuco mounds by the Taino people, to prevent erosion and retain water and nutrients on hillsides (Whitmore and Turner, 2001; Bargout and Raizada, 2013). Yet, while these individual crops are now widely grown on vulnerable hillsides around the world, they are not produced within their original sustainable agronomic system (Bargout and Raizada, 2013). In this review, examples of indigenous cultivation practices in Africa were noted that may hold lessons in sustainability for the rest of global agriculture. Indigenous farmers and their experience should be better integrated into breeding programs, partnerships, and collaborative networks working to address contemporary issues in crop production. This integration may keep indigenous peoples connected to their culture and resources, for the long-term benefit of their livelihoods. A specific program should be established to collect and publish indigenous agronomic knowledge from Africa, either within the FAO or CGIAR system (e.g., Bioversity International). Additionally, funding is needed to support social scientists to gather and collect indigenous knowledge associated with specific African landraces, so that this information may be added to seed passport information for breeders.
Further improvements in Africa's crops have the potential to not only assist consumers globally, but also alleviate food and nutritional insecurity and poverty for many smallholder farmers and rural communities around the world. Diversifying crop production to include a wider availability of underutilized crops could enhance nutritional and income resiliency. This need is particularly relevant in Africa, where the population is projected to increase from its current 1.3 billion to 4.3 billion by 2100 (United Nations Population Division, 2019)—not surprisingly similar to the demographic histories of other post-colonial nations such as India (United Nations Population Division, 2019). The World Bank and regional organizations should allocate funds that promote local value addition (e.g., food processing, packaging, and storage) to create jobs and keep profits within Africa, mainstream these crops, and reorient the history of Africa being solely a source of cheap, raw commodities. In applying a gendered lens, we also see a greater need for research agendas focused on reducing the burden of labor for women farmers, which include subsidizing appropriate mechanization along the value chain (e.g., post-harvest processing such as milling) which will also increase interest and production in these crops.
The efforts recommended above are not possible, however, without first recognizing crop histories and the undervaluing and underutilization of indigenous knowledge in the conventional food system today. We encourage the development and implementation of learning resources for African and American educators to include food origins and histories in science, history, and geography curricula in schools, as well as in museums. We should do so with the intent to raise awareness, appreciation, and understanding of the histories of the foods today, and to harmonize further connections between people, our food, culture, and environments, to better support the opportunity for reconciliation and a more sustainable food system.
Author Contributions
ES conducted the research and wrote the manuscript. MNR conceptualized the review, assisted with research, and edited the manuscript. All authors contributed to the article and approved the submitted version.
Funding
No funding was used to conduct the research, however, publication fees were paid by NSERC grant 400924 to MNR. NSERC played no role in this manuscript.
Conflict of Interest
The authors declare that the research was conducted in the absence of any commercial or financial relationships that could be construed as a potential conflict of interest.
References
Abrouk, M., Ahmed, H. I., Cubry, P., Šimoníková, D., Cauet, S., Pailles, Y., et al. (2020). Fonio millet genome unlocks African orphan crop diversity for agriculture in a changing climate. Nat. Commun. 11, 1–13. doi: 10.1038/s41467-020-18329-4
Achigan-Dako, E. G., Fagbemissi, R., Avohou, H. T., Vodouhe, R. S., Coulibaly, O., and Ahanchede, A. (2008). Importance and practices of Egusi cops (Citrullus lanatus (Thunb.) Matsum. and Nakai, Cucumeropsis mannii Naudin and Lagenaria siceraria (Molina) Standl. cv. ‘Aklamkpa') in sociolinguistic areas in Benin. Biotech. Agron. Soc. Environ. 12, 393–403.
Adebayo, S. A., and Oladele, O. I. (2012). Medicinal values of kolanut in Nigeria: implication for extension service delivery. Life Sci. J. 9, 887–891.
Adeoye, I. B., Olajide-Taiwo, F. B., Adebisi-Adelani, O., Usman, J. M., and Badmus, M. A. (2011). Economic analysis of watermelon based production system in Oyo State, Nigeria. ARPN J. Agric. Biol. Sci. 6, 53–59.
Adoukonou-Sagbadja, H., Dansi, A., Vodouhè, R., and Akpagana, K. (2006). Indigenous knowledge and traditional conservation of fonio millet (Digitaria exilis, Digitaria iburua) in Togo. Biodiv. Conserv. 15, 2379–2395 doi: 10.1007/978-1-4020-5283-5_4
Ahiakpa, J. K., Madgy, M., Werner, O., Amoatey, H. M., Yeboah, M. A., Appiah, et al. (2017). Intra-specific variation in West African and Asian germplasm of okra (Abelmoschus spp. L.) Ann. Agric. Sci. 62, 131–138. doi: 10.1016/j.aoas.2017.08.002
Ajani, E. N., Mgbenka, R. N., and Okeke, M. N. (2013). Use of indigenous knowledge as a strategy for climate change adaptation among farmers in sub-Saharan Africa: implications for policy. Asian J. Agr. Ext. 2, 23–40. doi: 10.9734/AJAEES/2013/1856
Akena, F. A. (2012). Critical analysis of the production of Western knowledge and its implications for indigenous knowledge and decolonization. J. Black Stud. 43, 599–619. doi: 10.1177/0021934712440448
Akinseye, F. M., Adam, M., Agele, S. O., Hoffmann, M. P., Traore, P. C. S., and Whitbread, A. M. (2017). Assessing crop model improvements through comparison of sorghum (Sorghum bicolor L. moench) simulation models: a case study of West African varieties. Field Crops Res. 201, 19–31. doi: 10.1016/j.fcr.2016.10.015
Akpalu, M. M., Salaam, M., Oppong-Sekyere, D., and Akpalu, S. E. (2014). Farmers' knowledge and cultivation of Cowpea (Vigna unguiculata (L.) Verdc.) in three communities of Bolgatanga Municipality, Upper East Region, Ghana. Br. J. Appl. Sci. Tech. 4, 775–792. doi: 10.9734/BJAST/2014/6969
Aladele, S. E., Aryo, O. J., and de Lapena, R. (2008). Genetic relationships among West African okra (Abelmoschus caillei) and Asian genotypes (Abelmoschus esculentus) using RAPD. Afr. J. Biotech. 7, 1426–1431.
Aleni, C. (2018). From conservation to commercialisation: African indigenous vegetables in Arua, District of Uganda (masters' thesis). Humboldt-Universität zu Berlin, Berlin, Germany.
Allal, F., Sanou, H., Millet, L., Vaillant, A., Camus-Kulandaivelu, L., Logossa, Z. A., et al. (2011). Past climate changes explain the phylogeography of Vitellaria paradoxa over Africa. Hereditary 107, 174–186. doi: 10.1038/hdy.2011.5
Altieri, M. A. (2001). Agriculture, traditional. Encyc. Biodiv. 1, 109–118. doi: 10.1016/B0-12-226865-2/00009-2
Amidou, A., Michel, N., Serge, H., and Valérie, P. (2007). Genetic basis of species differentiation between Coffea liberica Hiern and C. canephora Pierre: analysis of an interspecific cross. Genet. Resour Crop. Evol. 54, 1011–1021. doi: 10.1007/s10722-006-9195-0
Andrews, A. C. (1956). Melons and watermelons in the classical era. Osiris 12, 368–375. doi: 10.1086/368603
Angiosperm Phylogeny Group (2009). An update of the Angiosperm Phylogeny Group classification for the orders and families of flowering plants: APG III. Bot. J. Linn. Soc. 161, 105–121. doi: 10.1111/j.1095-8339.2009.00996.x
Anthony, F., Combes, M. C., Astorga, C., Bertrand, B., Graziosi, G., and Lashermes, P. (2002). The origin of cultivated Coffea arabica L. varieties revealed by AFLP and SSR markers. Theor. Appl. Genet. 104, 894–900. doi: 10.1007/s00122-001-0798-8
Aregay, M. W. (1988). The early history of Ethiopia's coffee trade and the rise of Shawa. J. Afric. Hist. 29, 19–25. doi: 10.1017/S0021853700035969
Arias, D., González, M., Prada, C, F., Ayala-Diaz, I., Montoya, C., Daza, E., et al. (2015). Genetic and phenotypic diversity of natural American oil palm (Elaeis oleifera (H.B.K.) Cortés) accessions. Tree Genet. Genom. 11, 1–13. doi: 10.1007/s11295-015-0946-y
Arnau, G., Bhattacharjee, R., Sheela, M. N., Chair, H., Malapa, R., Lebot, V., et al. (2017). Understanding the genetic diversity and population structure of yam (Dioscorea alata L.) using microsatellite markers. PLoS ONE 12:e0174150. doi: 10.1371/journal.pone.0174150
Arnot, C., Boxall, P. C., and Cash, S. B. (2006). Do ethical consumers care about price? A revealed preference analysis of fair trade coffee purchases. Can. J. Agric. Econ. 54, 555–565. doi: 10.1111/j.1744-7976.2006.00066.x
Assefa, K., Cannarozzi, G., Girma, D., Kamies, R., Chanyalew, S., Plaza-Wüthrich, S., et al. (2015). Genetic diversity in tef [Eragrostis tef (Zucc.) Trotter]. Front. Plant Sci. 6:177. doi: 10.3389/fpls.2015.00177
Ayenan, M. A. T., Sodedji, K. A. F., Nwanko, C. I., Olodo, K. F., and Alladassi, M. E. B. (2018). Harnessing genetic resources and progress in plant genomcis for fonio (Digitaria spp.) improvement. Genet. Resour. Crop Evol. 65, 373–386. doi: 10.1007/s10722-017-0565-6
Baoua, I. B., Margam, V., Amadou, L., and Murdock, L. L. (2012). Performance of triple bagging hermetic technology for postharvest storage of cowpea grain in Niger. J. Stor. Prod. R. 51, 81–85. doi: 10.1016/j.jspr.2012.07.003
Bargout, R. N., and Raizada, M. N. (2013). Soil nutrient management in Haiti, pre-Columbus to the present day: lessons for future agricultural interventions. Agric. Food Secur. 2:11. doi: 10.1186/2048-7010-2-11
Basiron, Y. (2007). Palm oil production through sustainable plantations. Eur. J. Lipid Sci. Technol. 109, 289–295. doi: 10.1002/ejlt.200600223
Benchasri, S. (2012). Okra (Abelmoschus esculentus (L.) Moench) as a valuable vegetable of the world. Ratarstvo i Povrtarstvo 49, 105–122. doi: 10.5937/ratpov49-1172
Bjornlund, V., Bjornlund, H., and Van Rooyen, A. F. (2020). Why agricultural production in sub-Saharan Africa remains low compared to the rest of the world – a historical perspective. Int. J. Water Res. Dev. 36, S20–S53. doi: 10.1080/07900627.2020.1739512
Blake, L. W. (1981). Early acceptance of watermelon by Indians of the United States. J. Ethnobiol. 1, 193–199.
Block-Steele, K. (2011). The language of action: a creative study of resistance to slavery from West Africa to the days across the sea. Independent Study Project (ISP) Collection 991, 1–48.
Borelli, T., Hunter, D., Padulosi, S., Amaya, N., Meldrum, G., Beltrame, D. M. O., et al. (2020). Local solutions for sustainable food systems: the contributions of orphan crops and wild edible species. Agronomy 10, 1–25. doi: 10.3390/agronomy10020231
Borras, S. M., and Franco, J. (2010). Towards a broader view of the politics of global land grab: rethinking land issues, reframing resistance. [working paper series]. Initiative in Critical Agrarian Studies, Land Deal Politics Initiative and Transnational Institute, 1–39.
Bower, A. L. (2008). “Recipes for history: The National Council of Negro Women's five historical cookbooks,” in African American Foodways: Explorations of History and Culture, ed A. L. Bower (Urbana, IL: University of Illinois Press), 153–174.
Braun, A. E. (1942). Resistance of watermleon to the wilt disease. Am. J. Bot. 29, 683–684. doi: 10.1002/j.1537-2197.1942.tb10267.x
Burch, A. D. S., Cai, W., Gianordoli, G., McCarthy, M., and Patel, J. K. (2020). How black lives matter reached every corner of America. New York Times. Available online at: https://www.nytimes.com/interactive/2020/06/13/us/george-floyd-protests-cities-photos.html (accessed June 27, 2020).
Burgarella, C., Cubry, P., Kane, N. A., Varshney, R. K., Mariac, C., Liu, X., et al. (2018). A Western Sahara centre of domestication inferred from pearl millet genomes. Nat. Ecol. Evol. 2, 1377–1380. doi: 10.1038/s41559-018-0643-y
Carney, J., and Elias, M. (2006). Revealing gendered landscapes: indigenous female knowledge and agroforestry of African shea. Can. J. Afric. Stud. 40, 235–267. doi: 10.1080/00083968.2006.10751344
Carney, J. A. (2001a). African rice in the Columbian exchange. J. Afr. Hist. 42, 377–396. doi: 10.1017/S0021853701007940
Carney, J. A. (2001b). Black Rice: The African Origins of Rice Cultivation in the Americas. Cambridge, MA: Harvard University Press.
Carvalho, M., Matos, M., Castro, I., Monterio, E., Rosa, E., Lino-Neto, T., et al. (2019). Screening of worldwide cowpea collection to drought tolerant at a germination stage. Sci. Hort. 247, 107–115. doi: 10.1016/j.scienta.2018.11.082
Chandra, D., Chandra, S., Arora, P., and Sharma, A. K. (2016). Review of finger millet (Eleusine coracana (L.) Gaertn): a power house of health benefiting nutrients. Food Sci. Human Wellness 5, 149–155. doi: 10.1016/j.fshw.2016.05.004
Chanyalew, S., Ferede, S., Damte, T., Fikre, T., Genet, Y., Kebede, W., et al. (2019). Significance and prospects of an orphan crop tef. Planta 250, 753–676. doi: 10.1007/s00425-019-03209-z
Chatelain, M. (2015). “Black women's food writing and the archive of black women's history,” in Dethroning the Deceiftful Pork Chop: Rethinking African American Foodways From Slavery to Obama, ed J. J. Wallach (Fayetteville, AR: University of Arkansas Press), 31–45. doi: 10.2307/j.ctt1ffjdh9.9
Chomicki, G., and Renner, S. S. (2015). Watermelon origin solved with molecular phylogenetics including Linnean material: another example of museomics. New Phytol. 205, 526–532. doi: 10.1111/nph.13163
Chomicki, G., Schaefer, H., and Renner, S. S. (2019). Origin and domestication of cucurbitaceae crops: insights from phylogenies, genomics, and archaeology. New Phytol. 226, 1240–1255. doi: 10.1111/nph.16015
Cloete, P. C., and Idsardi, E. F. (2013). Consumption of indigenous and traditional food crops: perceptions and realities from South Africa. Agroecol. Sustain. Food Syst. 37, 902–914. doi: 10.1080/21683565.2013.805179
Clotault, J., Thuillet, A.-C., Buiron, M., De Mita, S., Couderc, M., Haussmann, B. I. G., et al. (2011). Evolutionary history of pearl millet (Pennisetum glaucum [L.] R. Br.) and selection on flowering genes since its domestication. Mol. Biol. Evol. 29, 1199–1212. doi: 10.1093/molbev/msr287
Colburn, M. (2002). The Republic of Yemen: Development Challenges in the 21st Century. London: Catholic Institute for International Relations.
Collins, R. O. (2006). The African slave trade to Asia and the Indian Ocean islands. Afr. Asian Stud. 5, 3–4. doi: 10.1163/156920906779134821
Coté, C. (2016). “Indigenizing” food sovereignty. Revitalizing Indigenous food practices and ecological knowledges in Canada and the United States. Humanities 5, 1–14. doi: 10.3390/h5030057
Coursey, D. G. (1961). The magnitude and origins of storage losses in Nigerian yams. J. Sci. Food Agric. 12, 574–580. doi: 10.1002/jsfa.2740120807
Cramb, R., and Curry, G. N. (2012). Oil palm and rural livelihoods in the Asia-Pacific region: an overview. Asia Pac. View 53, 223–239. doi: 10.1111/j.1467-8373.2012.01495.x
Croft, M. M., Marshall, M. I., and Hallett, S. G. (2016). Market barriers faced by formal and informal vendors of African leafy vegetables in Western Kenya. J. Food Distr. Res. 47, 49–60. doi: 10.22004/ag.econ.249999
D'Andrea, A. C. (2008). T'ef (Eragrostis tef) in ancient agricultural systems of highland Ethiopia. Econ. Bot. 62, 547–566. doi: 10.1007/s12231-008-9053-4
D'Andrea, A. C., Kahlheber, S., Logan, A. L., and Watson, D. J. (2007). Early domesticated cowpea (Vinga unguiculata) from Central Ghana. Antiquity 81, 686–698. doi: 10.1017/S0003598X00095661
Dane, F., and Liu, J. (2007). Diversity and origin of cultivated and citron type watermelon (Citrullus lanatus). Genet. Resour. Crop Evol. 54, 1255–1265. doi: 10.1007/s10722-006-9107-3
Davies, O. (1968). The origins of agriculture in West Africa. Curr. Anthropol. 9, 479–482. doi: 10.1086/200945
Demi, S. M. (2014). African indigenous food crops: their roles in combatting chronic diseases in Ghana (Masters' thesis). University of Toronto, Toronto, ON, Canada.
Denu, D., Platts, P. J., Kelbessa, E., Gole, T. W., and Marchant, R. (2016). The role of traditional coffee management in the forest conservation and carbon storage in the Jimma highlands, Ethiopia. Forests, Trees Livelihoods 25, 226–238. doi: 10.1080/14728028.2016.1192004
Durand, D., Hubert, A., Nafan, D., Adolphe, A., Farid, B., Alphonse, S., et al. (2015). Indigenous knowledge and socio-economic values of three kola species (Cola nitidia, Cola acuminata, and Garcinia kola) used in Southern Benin. Eur. Sci. J. 11, 206–227.
Elias, M. (2015). Gender, knowledge-sharing and management of shea (Vitellaria paradoxa) parklands in central-west Burkina Faso. J. Rural Stud. 38, 27–38. doi: 10.1016/j.jrurstud.2015.01.006
Elias, M., and Carney, J. (2007). African shea butter: a feminized subsidy from nature. Africa 77, 37–62. doi: 10.3366/afr.2007.77.1.37
Esch, E., and Roediger, D. (2014). “Race and the management of labour in United States history,” in Theorizing Anti-racism: linkages in Marxism and Critical Race Theories, eds A. B. Bakan, and E. Dua (Toronto, ON: University of Toronto Press), 340–376.
Faber, M., Oelofse, A., Van Jaaesveld, P. J., Wenhold, F. A. M., and Jansen Van Rensburg, W. S. (2010). African leafy vegetables consumed by households in the Limpopo and KwaZulu-Natal provinces in South Africa. S. Afr. J. Clin. Nutr. 23, 30–38. doi: 10.1080/16070658.2010.11734255
Fahad, S., Bajwa, A. A., Nazir, U., Anjum, S. A., Farooq, A., Zohaib, A., et al. (2017). Crop production under drought and heat stress: plant responses and management options. Front. Plant Sci. 8:1147. doi: 10.3389/fpls.2017.01147
Fatokun, C. A., Abou, T., Ongom, P., Lopez, K., and Ousmane, B. (2020). Cowpea receives more research support. CGIAR Research Program on Grain and Dryland Cereals. Available online at: https://www.cgiar.org/news-events/news/cowpea-receives-more-research-support/ (accessed June 27, 2020).
Gaisberger, H., Kindt, R., Loo, J., Schmidt, M., Bognounou, F., Da, S. S., et al. (2017). Spatially explicit multi-threat assessment of food tree species in Burkina Faso: a fine-scale approach. PLoS ONE 12:e0184457. doi: 10.1371/journal.pone.0184457
Gan, S. T., Wong, W. C., Wong, C. K., Soh, A. C., Kilian, A., Low, E.-T. L., et al. (2018). High density SNP and DArT-based genetic linkage maps of two closely related oil palm populations. J. Appl. Genet. 59, 23–34. doi: 10.1007/s13353-017-0420-7
Gebremariam, M. M., Zarnkow, M., and Becker, T. (2014). Teff (Eragrostis tef ) as a raw material for malting, brewing and manufacturing of gluten-free foods and beverages: a review. J. Food Sci Technol. 51, 2881–2895. doi: 10.1007/s13197-012-0745-5
Gibbs, F. W. (1939). The history of the manufacture of soap. Ann. Sci. 4, 169–190. doi: 10.1080/00033793900201191
Gido, E. O., Ayuya, O. I., Owuor, G., and Bokelmann, W. (2017). Consumption intensity of leafy African indigenous vegetables: towards enhancing nutritional security in rural and urban dwellers in Kenya. Agr. Food Econ. 5, 1–16. doi: 10.1186/s40100-017-0082-0
Gilmer, R. A. (2015). “Native American contributions to African American foodways: slavery, colonialism, and cuisine,” in Dethroning the Deceiftful Pork Chop: Rethinking African American Foodways from Slavery to Obama, ed J. J. Wallach (Fayetteville, AR: University of Arkansas Press), 17–29. doi: 10.2307/j.ctt1ffjdh9.8
Girma, D., Assefa, K., Chanyalew, S., Cannarozzi, G., Kuhlemeier, C., and Tadele, Z. (2014a). The origins and progress of genomics research on Tef (Eragrostis tef). Plant Biotech. J. 12, 534–540. doi: 10.1111/pbi.12199
Girma, G., Hyma, K. E., Asiedu, R., Mitchell, S. E., Gedil, M., and Spillane, C. (2014b). Next-generation sequencing based genotyping, cytometry and phenotyping for understanding diversity and evolution of guinea yams. Theor. Appl. Genet. 127, 1783–1794. doi: 10.1007/s00122-014-2339-2
Gole, T. W. (2003). Vegetation of the Yayu Forest in SW Ethiopia: Impacts of Human Use and Implications for in situ Conservation of Wild Coffea arabica L. Populations. Göttingen: Cuvillier Verlag.
Gonçalves, A., Goufo, P., Barros, A., Domínguez-Perles, R., Trindade, H., Rosa, E. A. S., et al. (2016). Cowpea (Vigna unguiculata L. Walp), a renewed multipurpose crop for a more sustainable agri-food system: nutritional advantages and constraints. J. Sci. Food Agric. 96, 2941–2951. doi: 10.1002/jsfa.7644
Goron, T. L., and Raizada, M. N. (2015). Genetic diversity and genomic resources available for the small millet crops to accelerate a new green revolution. Front. Plant Sci. 6:157. doi: 10.3389/fpls.2015.00157
Grassi, F., Landberg, J., and Huyer, S. (2015). Running out of time: the reduction of women's work burden in agricultural production. Food and Agriculture Organization of the United Nations, 1–37.
Gremillion, K. T. (1993). Adoption of old world crops and processes of cultural heritage in the historic Southeast. Southeast Archaeol. 12, 15–20.
Guo, S., Zhang, J., Sun, H., Salse, J., Lucas, W. J., Haiying, Z., et al. (2013). The draft genome of watermelon (Citrullus lanatus) and resequencing of 20 diverse accessions. Nat. Genet. 45, 51–58. doi: 10.1038/ng.2470
Guo, S., Zhao, S., Sun, H., Wang, X., Wu, S., Lin, T., et al. (2019). Resequencing of 414 cultivated and wild watermelon accessions identifies selection for fruit quality traits. Nat. Genet. 51, 1616–1623. doi: 10.1038/s41588-019-0518-4
Gwali, S., Okullo, J. B. L., Eilu, G., Nakabonge, G., Nyeko, P., and Vuzi, P. (2012). Traditional management and conservation of shea trees (Vitellaria paradoxa subspecies nilotica) in Uganda. Environ. Dev. Sustain. 14, 347–363. doi: 10.1007/s10668-011-9329-1
Hall, R. L. (2008). “Food crops, medicinal plants, and the atlantic slave trade,” in African American Foodways: Explorations of History and Culture, ed A. L. Bower (Urbana, IL: University of Illinois Press), 17–44.
Harris, J. B. (2003). Beyond Gumbo: Creole Fusion Food From the Atlantic Rim. New York, NY: Simon and Schuster.
Hatakeyama, M., Aluri, S., Balachadran, M. T., Sivarajan, S. R., Patrignani, A., Grüter, S., et al. (2017). Multiple hybrid de novo genome assembly of finger millet, an orphan allotetraploid crop. DNA Res. 25, 39–47. doi: 10.1093/dnares/dsx036
Hayati, A., Wickneswari, R., Maizura, I., and Rajanaidu, N. (2004). Genetic diversity of oil palm (Elaeis guineensis Jacq.) germplasm collections from Africa: implications for improvement and conservation of genetic resources. Theor. Appl. Genet. 108, 1274–1284. doi: 10.1007/s00122-003-1545-0
Hilu, K. W., and de Wet, J. M. J. (1976). Domestication of Eleusine coracana. Econ. Bot. 30, 199–208. doi: 10.1007/BF02909728
Holt-Giménez, E. (2015). Racism and capitalism: dual challenges for the food movement. J. Agr. Food Syst. Comm. Dev. 5, 23–25. doi: 10.5304/jafscd.2015.052.014
Honfo, F. G., Akissoe, N., Linnemann, A. R., Soumanou, M., and Van Boekel, M. A. J. S. (2014). Nutritional composition of shea products and chemical properties of shea butter: a review. Crit. Rev. Food Sci. Nutri. 54, 673–686. doi: 10.1080/10408398.2011.604142
Horn, L. N., Ghebrehiwot, H. M., and Shimelis, H. A. (2016). Selection of novel cowpea genotypes derived through gamma irradiation. Front. Plant Sci. 7:262. doi: 10.3389/fpls.2016.00262
Huang, C., Liu, Q., Heerink, N., Stomph, T., Li, B., Liu, R., et al. (2015). Economic performance and sustainability of a novel intercropping system on the North China Plain. PLoS ONE 10:e0135518. doi: 10.1371/journal.pone.0135518
Hwang, B., Hundera, K., Mekuria, B., Wood, A., and Asfaw, A. (2020). Intensified management of coffee forest in Southwest Ethiopia detected by landsat imagery. Forests 11, 1–21. doi: 10.3390/f11040422
Ives, S. (2014). Farming the South African “bush”: ecologies of belonging and exclusion in rooibos tea. Am. Ethnol. 41, 698–713. doi: 10.1111/amet.12106
Jansen Van Rensburg, W. S., Venter, S. L., Netshiluvhi, T. R., Van Den Heever, E., Vorster, H. J., and de Ronde, J. A. (2004). Role of indigenous leafy vegetables in combating hunger and malnutrition. S. Afr. J. Bot. 70, 52–59. doi: 10.1016/S0254-6299(15)30268-4
Jarvis, D. I., Hodgkin, T., Sthapit, B. R., Fadda, C., and Lopez-Noriega, I. (2011). A heuristic framework for identifying multiple ways of supporting the conservation and use of traditional crop varieties within the agricultural production system. Crit. Rev. Plan Sci. 30, 125–176. doi: 10.1080/07352689.2011.554358
Jensen, B. D., Munisse, P., Andersen, S. B., and Christiansen, J. L. (2011a). Diversity of landraces, agricultural practices and traditional uses of watermelon (Citrullus lanatus) in Mozambique. Afr. J. Plant Sci. 5, 75–86.
Jensen, B. D., Touré, F. M., Hamattal, M. A., Touré, F. A., and Nantoumé, A. D. (2011b). Watermelons in the sand of Sahara: cultivation and use of indigenous landraces in the Tombouctou region of Mali. Ethnobot. Res. Appl. 9, 152–162. doi: 10.17348/era.9.0.151-162
Jolley, B., van der Rijst, M., Joubert, B., and Muller, M. (2017). Sensory profile of rooibos originating from the western and northern cape governed by production year development of rooibos aroma wheel. S. Afr. J. Bot. 110, 161–166. doi: 10.1016/j.sajb.2016.08.005
Joubert, E., and de Beer, D. (2011). Rooibos (Aspalathus linearis) beyond the farm gate: from herbal tea to potential phytopharmaceutical. South Afr. J. Bot. 77, 869–886. doi: 10.1016/j.sajb.2011.07.004
Joubert, E., Jolley, B., Koch, I. S., Muller, M., Van der Rijst, M., and De Beer, D. (2016). Major production areas of rooibos (Aspalathus linearis) deliver herbal tea of similar phenolic and phenylpropenoic acid glucoside content. S. Afr. J. Bot. 103, 162–169. doi: 10.1016/j.sajb.2015.08.015
Jukanti, A. K., Laxmipathi Gowda, C. L., Rai, K. N., Manga, V. K., and Bhatt, R. K. (2016). Crops that feed the world 11. Pearl millet (Pennisetum glaucum L.): an important source of food security, nutrition and health in the arid and semi-arid tropics. Food Secur. 8, 307–329. doi: 10.1007/s12571-016-0557-y
Kansiime, M. K., Ochieng, J., Kessy, R., Karanja, D., Romney, D., and Afari-Sefa, V. (2018). Changing knowledge and perceptions of African indigenous vegetables: the role of community-based nutritional outreach. Dev. Pract. 28, 480–493. doi: 10.1080/09614524.2018.1449814
Karambiri, M., Elias, M., Vinceti, B., and Grosse, A. (2017). Exploring local knowledge and preferences for shea (Vitellaria paradoxa) ethnovarieties in Southwest Burkina Faso through a gender and ethnic lens. Forests, Trees Livelihoods 26, 13–28. doi: 10.1080/14728028.2016.1236708
Khoury, C. K., Achicanoy, H. A., Bjorkman, A. D., Navarro-Racines, C., Guarino, L., Flores-Palacios, X., et al. (2016). Origins of food crops connec countries worldwide. Proc. R. Soc. B. 283, 1–9. doi: 10.1098/rspb.2016.0792
Kiple, K. F., and Coneè Ornelas, K. (2000). The Cambridge World History of Food. Cambridge: Cambridge University Press. doi: 10.1017/CHOL9780521402156
Kitch, L. W., Bottenberg, H., and Wolfson, J. L. (1997). “Indigenous knowledge and cowpea pest management in sub-Saharan Africa,” in Advances in Cowpea Research, eds B. B. Singh, D. R., Mohan Raj, K. E. Dashiell, and L. E. N. Jackai (Ibadan: International Institute of Tropical Agriculture), 292–301.
Knight, A., Underhill, P. A., Moretensen, H. M., Zhivotovsky, L. A., Lin, A. A., Henn, B. M., et al. (2003). African Y chromosome and mtDNA divergence provides insight into the history of click languages. Curr. Biol. 13, 464–473. doi: 10.1016/S0960-9822(03)00130-1
Koh, L. P., and Wilcove, D. S. (2008). Is oil palm agriculture really destroying tropical biodiversity? Conserv. Lett. 1, 60–64 doi: 10.1111/j.1755-263X.2008.00011.x
Koubová, E., Sumczynski, D., Šenkárová, L., Orsavová, J., and Fišera, M. (2018). Dietary intakes of minerals, essential and toxic trace elements for adults from Eragrostis tef L.: a nutritional assessment. Nutrients 10, 1–11. doi: 10.3390/nu10040479
Kumar, S., Dagnoko, S., Haougui, A., Ratnadass, A., Pasternak, D., and Kouame, C. (2010). Okra (Abelmoschus spp.) in West and Central Africa: potential and progress on its improvement. Afr. J. Agric. Res. 5, 3590–3598.
Lagemann, J. (1977). Traditional African Farming Systems in Eastern Nigeria an Analysis of Reaction to Increasing Population Pressure. München: Weltforum Verlag.
Lange, M. K. (2004). British colonial legacies and political development. World Dev. 32, 905–922. doi: 10.1016/j.worlddev.2003.12.001
Lemons, J. S. (1977). Black stereotypes as reflected in popular culture, 1880-1920. Am. Q. 29, 102–116. doi: 10.2307/2712263
Leroy, T., De Bellis, F., Legnate, H., Musoli, P., Kalonji, A., Solórzano, R. G. L., et al. (2014). Developing core collections to optimize the management and the exploitation of diversity of the coffee Coffea canephora. Genetica 142, 185–199. doi: 10.1007/s10709-014-9766-5
Lovejoy, P. E. (1980). Kola in the history of West Africa (La kola dans l'histoire de l'Afrique occidentale). Cahiers d'Études Afr. 20, 97–134. doi: 10.3406/cea.1980.2353
Lovejoy, P. E. (2012). Transformations in slavery: a history of slavery in Africa. New York, NY: Cambridge University Press. doi: 10.1017/CBO9781139014946
Mabhaudhi, T., Chibarabada, T. P., Chimonyo, V. G. P., and Modi, A. T. (2018). Modelling climate change impact: a case of bambara groundnut (Vigna subterranea). Phys. Chem. Earth 105, 25–31. doi: 10.1016/j.pce.2018.01.003
Mabhaudhi, T., Chibarabada, T. P., Chimonyo, V. G. P., Murugani, V. G., Pereira, L. M., Sobratee, N., et al. (2019). Mainstreaming underutilized indigenous and traditional crops into food systems: a South African perspective. Sustainability 11, 1–22. doi: 10.3390/su11010172
Maclean, L. M. (2002). Constructing a social safety net in Africa: an institutionalist analysis of colonial rule and state social policies in Ghana and Côte d'Ivoire. Stud. Comp. Int. Dev. 37, 64–90. doi: 10.1007/BF02686231
Madodé, Y. E., Houssou, P. A., Linnemann, A. R., Hounhouigan, D. J., Nout, M. J. R., and van Boekel, M. A. J. S. (2011). Preparation, consumption, and nutritional composition of West African cowpea dishes. Ecol. Food Nutr. 50, 115–136. doi: 10.1080/03670244.2011.552371
Mahalakshmi, V., Ng, Q., Atalobhor, J., Ogunsola, D., Lawson, M., and Ortiz, R. (2007). Development of a West African yam Dioscorea spp. core collection. Genet. Resour. Crop Evol. 54, 1817–1825. doi: 10.1007/s10722-006-9203-4
Mancus, P. (2007). Nitrogen fertilizer dependency and its contradictions: a theoretical exploration of social-ecological metabolism. Rural Soc. 72, 269–288. doi: 10.1526/003601107781170008
Manning, K., Pelling, R., Higham, T., Schwenniger, J.-L., and Fuller, D. Q. (2011). 4500-Year old domesticated pearl millet (Pennisetum glaucum) from the Tilemsi Valley, Mali: new insights into an alternative cereal domestication pathway. J. Archaeol. Sci. 38, 312–322. doi: 10.1016/j.jas.2010.09.007
Maranz, S., Kpikpi, W., Wiesman, Z., Sauveur, A. D.-S., and Chapagain, B. (2004). Nutritional values and Indigenous preferences for shea fruits (Vitellaria paradoxa C.F. Gaertn. F.) in African agroforestry parklands. Econ. Bot. 58, 588–600. doi: 10.1663/0013-0001(2004)058[0588:NVAIPF]2.0.CO;2
Maranz, S., and Wiesman, Z. (2003). Evidence for indigenous selection and distribution of the shea tree, Vitellaria paradoxa, and its potential significance to prevailing parkland savanna tree patterns in sub-Saharan Africa north of the equator. J. Biogeogr. 30, 1505–1516. doi: 10.1046/j.1365-2699.2003.00892.x
Marks, C. (2015). “Creole cuisine as culinary border culture: reading recipes as testimonies of hybrid identity and cultural heritage,” in Dethroning the Deceiftful Pork Chop: Rethinking African American Foodways FSlavery to Obama, ed J. J. Wallach (Fayetteville, AR: University of Arkansas Press), 79–92. doi: 10.2307/j.ctt1ffjdh9.12
Martins, C. M., Lawlor, D. W., Quilambo, O. A., and Kunert, K. J. (2014). Evaluation of four Mozambican cowpea landraces for drought tolerance. S. Afr. J. Plant Soil 31, 87–91. doi: 10.1080/02571862.2014.907453
Mbow, C., Van Noordwijk, M., Luedeling, E., Neufeldt, H., Minang, P. A., and Kowero, G. (2014). Agroforestry solutions to address food security and climate change challenges in Africa. Curr. Opin. Env. Sust. 6, 61–67. doi: 10.1016/j.cosust.2013.10.014
Moyo, S. M., Serem, J. C., Bester, M. J., Mavumengwana, V., and Kayitesi, E. (2020). African green leafy vegetables health benefits beyond nutrition. Food Rev. Int. 1–18, doi: 10.1080/87559129.2020.1717519
Muhanji, G., Roothaert, R. L., Webo, C., and Stanley, M. (2011). African indigenous vegetable enterprises and market access for small-scale farmers in East Africa. Int. J. Agric. Sust. 9, 194–202. doi: 10.3763/ijas.2010.0561
Mujaju, C., and Fatih, M. (2011). Distribution patterns of cultivated watermelon forms in Zimbabwe using DIVA-GIS. Int. J. Biodivers. Conserv. 3, 474–481.
Muofhe, M. L., and Dakora, F. D. (1999). Nitrogen nutrition in nodulated field plants of the shrub tea legume Aspalathus linearis assessed using 15N natural abundance. Plant Soil 209, 181–186. doi: 10.1023/A:1004514303787
Muzac-Tucker, I., Asemota, H. N., and Ahmad, M. H. (1993). Biochemical composition and storage of Jamaican yams (Dioscorea sp). J. Sci. Food Agric. 62, 219–224. doi: 10.1002/jsfa.2740620303
Nagendran, B., Unnithan, U., Choo, Y., and Sundram, K. (2000). Characteristics of red palm oil, a carotene- and vitamin E–rich refined oil for food uses. Food Nutri. Bull. 21, 189–194. doi: 10.1177/156482650002100213
Nantoumé, A. D., Traoré, S., Christiansen, J. L., Andersen, S. B., and Jensen, B. D. (2012). Traditional uses and cultivation of indigenous watermelons (Citrullus lanatus) in Mali. Int. J. Biodivers. Conserv. 4, 461–471. doi: 10.5897/IJBC11.217
Nascimento, W. F., Rodrigues, J. F., Koehler, S., Gepts, P., and Veasey, E. A. (2013). Spatially structured genetic diversity of the Amerindian yam (Dioscorea trifida L.) assessed by SSR and ISSR markers in Southern Brazil. Genet. Res. Crop Evol. 60, 2405–2420. doi: 10.1007/s10722-013-0008-y
National Research Council (1996). Lost Crops of Africa: Volume I: Grains. Washington, DC: The National Academies Press.
National Research Council (2006). Lost Crops of Africa: Vol II: Vegetables. Washington, DC: The National Academies Press.
Nedumaran, S., Abinaya, P., Jyosthnaa, P., Shraavya, B., Rao, P., and Bantilan, C. (2015). Grain legumes production, consumption and trade trends in developing countries. International Crops Research Institute for the Semi-Arid Tropics (ICRISAT). Working Paper Series No. 60, 1–57.
Niang, I., Ruppel, O. C., Adrabo, M. A., Essel, A., Lennard, C., Padgham, J., et al. (2014). “Africa,” in Climate Change 2014: Impacts, Adaptation, and Vulnerability. Part B: Regional Aspects. Contribution of Working Group II to the Fifth Assessment Report of the Intergovernmental Panel on Climate Change, eds V. R. Barros, C. B. Field, D. J. Dokken, M. D. Mastrandrea, K. J. Mach, T. E. Bilir, et al. (Cambridge: Cambridge University Press), 1199–1265.
Nono-Womdim, R., Ojiewo, C., Abang, M., and Olouch, M. O. (2012). Good agricultural practices for African indigenous vegetables. Scr. Hort. 15, 83–89.
Nunn, N. (2008). The long-term effects of Africa's slave trades. Q. J. Econ. 123, 139–176. doi: 10.1162/qjec.2008.123.1.139
Nunn, N., and Qian, N. (2010). The Columbian exchange: a history of disease, food, and ideas. J. Econ. Perspect. 24, 163–188. doi: 10.1257/jep.24.2.163
Obidzinski, K., Andriani, R., Komarudin, H., and Andrianto, A. (2012). Environmental and social impacts of oil palm plantations and their implications for biofuel production in Indonesia. Ecology and Society. 17:25. doi: 10.5751/ES-04775-170125
Olodo, K. F., Gueye, M. C., Calatayud, C., Diop, B. M., Kane, N. A., Ngom, A., et al. (2018). EST-SSR development for Digitaria exilis and its relatives D. iburua and D. longiflora from transcriptome sequences. Plant Genet. Resour. 17, 280–284. doi: 10.1017/S1479262118000400
Omoigui, L. O., Kamara, A. Y., Alunyo, G. I., Bello, L. L., Oluoch, M., Timko, M. P., et al. (2017a). Identification of new sources of resistance to Striga gesnerioides in cowpea Vigna unguiculata accessions. Genet. Resour. Crop Evol. 64, 901–911. doi: 10.1007/s10722-016-0410-3
Omoigui, L. O., Kamara, A. Y., Moukoumbi, Y. D., Ogunkanmi, L. A., and Timko, M. P. (2017b). Breeding cowpea for resistance to Striga gesnerioides in the Nigerian dry savannas using marker-assisted selection. Plant Breedi. 136, 393–399. doi: 10.1111/pbr.12475
Oumar, I., Mariac, C., Pham, J.-L., and Vigouroux, Y. (2008). Phylogeny and origin of pearl millet (Pennisetum glaucum [L.] R. Br) as revealed by microsatellite loci. Theor. Appl. Genet. 117, 489–497. doi: 10.1007/s00122-008-0793-4
Owade, J. O., Abong, G., Okoth, M., and Mwang'ombe, A. W. (2020). A review of the contribution of cowpea leaves to food and nutrition security in East Africa. Food Sci. Nutr. 8, 36–47. doi: 10.1002/fsn3.1337
Pakiam, G. K. (2020). Smallerholder involvement in tree crops in Malaya, with special reference to oil and coconut palms in Johor, 1862-1963. Aust. Econ. Hist. Rev. 60, 268–275. doi: 10.1111/aehr.12198
Paris, H. S. (2015). Origin and emergence of the sweet dessert watermelon, Citrullus lanatus. Ann. Bot. 116, 133–148. doi: 10.1093/aob/mcv077
Patay, É. B., Bencsik, T., and Papp, N. (2016). Phytochemical overview and medicinal importance of Coffea species from the past until now. Asian Pac. J. Trop. Med. 9, 1127–1135. doi: 10.1016/j.apjtm.2016.11.008
Paudel, L., Clevenger, J., and McGregor, C. (2019). Refining the egusi locus in watermleon using KASP assays. Sci. Hort. 257, 1–7. doi: 10.1016/j.scienta.2019.108665
Peluso, N. L., and Lund, C. (2011). New frontiers of land control: introduction. J. Peas. Stud. 38, 667–681. doi: 10.1080/03066150.2011.607692
Pendergast, M. (1999). Uncommon Grounds: The History of Coffee and How It Transformed Our World. New York, NY: Basic Books.
Phillips, R. D., McWatters, K. H., Chinnan, M. S., Hung, Y.-C., Beuchat, L. R., Sefa-Dedeh, S., et al. (2003). Utilization of cowpeas for human food. Field Crops Res. 82, 193–213. doi: 10.1016/S0378-4290(03)00038-8
Pitrat, M. (2008). “Melon,” in Handbook of Plant Breeding, Vegetables I, eds J. Prohens, and F. Nuez (New York, NY: Springer), 283–315. doi: 10.1007/978-0-387-30443-4_9
Rao, P. S., Kumar, C. G., and Reddy, B. V. S. (2013). “Sweet sorghum: from theory to practice,” in Characterization of Improved Sweet Sorghum Cultivars, Springer Briefs in Agriculture, eds P. S. Rao, and C. G. Kumar (Berlin: Springer). doi: 10.1007/978-81-322-0783-2
Ravelombola, W. S., Shi, A., Weng, Y., and Clark, J. (2017). Evaluation of salt tolerance at germination stage in cowpea [Vigna unguiculata (L.) Walp]. Hort. Sci. 52, 1168–1176. doi: 10.21273/HORTSCI12195-17
Reddy, P. S. (2017). “Sorghum, Sorghum bicolor (L.) Moench,” in Millets and Sorghum: Biology and Genetic Improvement, ed J. V. Patil (West Sussex: John Wiley and Sons), 1–48. doi: 10.1002/9781119130765.ch1
Robinson, J. (2005). Desertification and disarray: the threats to plant genetic resources of Southern Darfur, Western Sudan. Plant Genet. Resour. 3, 3–11. doi: 10.1079/PGR200451
Romão, R. L. (2000). Northeast Brazil: a secondary center of diversity for watermlon (Citrullus lanatus). Genet. Resour. Crop Evol. 47, 207–213. doi: 10.1023/A:1008723706557
Ronoh, R., Ekhuya, N. A., Linde, M., Winkelmann, T., Abukutsa-Onyango, M., Dinssa, F. F., et al. (2018). African nightshades: genetic, biochemical and metabolite diversity of an undertutilised indigenous leafy vegetable and its potential for plant breeding. J. Hort. Sci. Biotech. 93, 113–121. doi: 10.1080/14620316.2017.1358112
Sami, R. A., Yeye, M. Y., Ishiyaku, M. F., and Usman, I. S. (2013). Heritability studies in some sweet sorghum (Sorghum Bicolor. L. Moench) genotypes. J. Biol. Agric. Health 3, 49–51.
Saw, A. K.-C., Yam, W.-S., Wong, K.-C., and Lai, C.-S. (2015). A comparative study of the volatile constituents of Southeast Asian Coffea arabica, Coffea liberica, and Coffea robusta green beans and their antioxidant activities. J. Essent. Oil Bear Plant. 18, 64–73. doi: 10.1080/0972060X.2014.977580
Scarcelli, N., Cubry, P., Akakpo, R., Thuillet, A.-C., Obidiegwu, J., Baco, M. N., et al. (2019). Yam genomics supports West Africa as a major cradle of crop domestication. Sci. Adv. 5, 1–7. doi: 10.1126/sciadv.aaw1947
Schlebusch, C. M., Skoglund, P., Sjödin, P., Gattepaille, L. M., Hernandez, D., Jay, F., et al. (2012). Genomic variation in seven Khoe-San groups reveals adaptation and complex African history. Science 338, 374–379. doi: 10.1126/science.1227721
Seidu, J. M., Bobobee, E. Y. H., Kwenin, W. K. J., Frimpong, R., Kugbe, S. D., Tevor, W. J., et al. (2012). Preservation of indigenous vegetables by solar drying. J. Agric. Bio. Sci. 7, 407–415.
Seng, T.-Y., Saad, S. H. M., Chin, C.-W., Ting, N.-C., Singh, R. S. H., Zaman, F. Q., et al. (2011). Genetic linkage map of a high yielding FELDA Deli X Yangambi oil palm cross. PLoS ONE 6:e26593. doi: 10.1371/journal.pone.0026593
Serba, D. D., and Yadav, R. S. (2016). Genomic tools in pearl millet breeding for drought tolerance: status and prospects. Front. Plant Sci. 7:1724. doi: 10.3389/fpls.2016.01724
Shevade, V. S., and Loboda, T. V. (2019). Oil palm plantations in Peninsular Malaysia: determinants and contraints on expansion. PLoS ONE 14:e210628. doi: 10.1371/journal.pone.0210628
Singh, B. B., Ajeigbe, H. A., Tarawali, S. A., Fernandez-Rivera, S., and Abubakar, M. (2003). Improving the production and utilization of cowpea as food and fodder. Field Crops Res. 84, 169–177. doi: 10.1016/S0378-4290(03)00148-5
Singh, B. B., Chambliss, O. L., and Sharma, B. (1997). “Recent advances in cowpea breeding,” in Advances in Cowpea Research, eds B. B. Singh, D. R., Mohan Raj, K. E. Dashiell, and L. E. N. Jackai (Ibadan: International Institute of Tropical Agriculture), 30–49.
Singh, R., Low, E.-T. L., Ooi, L. C.-L., Ong-Abdullah, M., Ting, N.-C., and Nagappan, J. (2013a). The oil palm SHELL gene controls oil yield and encodes a homologue of SEEDSTICK. Nature 500, 340–346. doi: 10.1038/nature12356
Singh, R., Meilina, O.-A., Low, E.-T. L., Manaf, M. A. A., Rosli, R., Nookiah, R., et al. (2013b). Oil palm genome sequence reveals divergence of interfertile species in old and new worlds. Nature 500, 335–341. doi: 10.1038/nature12309
Slocum, R. (2010). Race in the study of food. Prog. Hum. Geogr. 35, 303–327. doi: 10.1177/0309132510378335
Smith, D. T., and Cooley, A. W. (1973). Wild watermelon emergence and control. Weed Sci. 21, 570–573. doi: 10.1017/S0043174500032501
Smith, O., Nicolson, W. V., Kistler, L., Mace, E., Clapham, A., Rose, P., et al. (2019). A domestication history of dynamic adaptation and genomic deterioration in Sorghum. Nat. Plant. 5, 369–379. doi: 10.1038/s41477-019-0397-9
Statistics Division of the Food Agriculture Organization of the United Nations (FAOSTAT) (2018). Food and Agriculture Data. Available online at: http://www.fao.org/faostat/en/#data (accessed June 27, 2020).
Sunilkumar, K., Murugesan, P., Mathur, R. K., and Rajesh, M. K. (2020). Genetic diversity in oil palm (Elaeis guineensis and Elaeis oleifera) germplasm as revealed by microsatellite (SSR) markers. Ind. J. Agric. Sci. 90, 741–745.
Tan, K. T., Lee, K. T., Mohamed, A. R., and Bhatia, S. (2009). Palm oil: addressing issues and towards sustainable development. Renew. Sust. Enery Rev. 13, 420–427. doi: 10.1016/j.rser.2007.10.001
Teh, C.-K., Muaz, S. D., Tangaya, P., Fong, P.-Y., Oong, A.-L., Mayes, S., et al. (2017). Characterizing haplosufficiency of SHELL gene to improve fruit form prediction in introgressive hybrids of oil palm. Sci. Rep. 7:3118. doi: 10.1038/s41598-017-03225-7
Thomas, A. S. (1942). The wild arabica coffee on the Boma Plateau, Anglo-Egyptian Sudan. Emp. Expt. Agric. 10, 2017–2212.
Timko, M. P., and Singh, B. B. (2008). “Cowpea, a multifunctional legume,” in Genomics of Tropical Crop Plants, eds P. H. Moore and R. Ming (New York, NY: Springer), 227–258. doi: 10.1007/978-0-387-71219-2_10
Tom-Dery, D., Eller, F., Reisdorff, C., and Jensen, K. (2018). Shea (Vitellaria paradoxa C. F. Gaertn.) at the crossroads: current knowledge and research gaps. Agroforest. Syst. 92, 1353–1371. doi: 10.1007/s10457-017-0080-y
Tschora, H., and Cherubini, F. (2020). Co-benefits and trade-offs of agroforestry for climate change mitigation and other sustainability goals in West Africa. Global Ecol. Conserv. 22:e00919. doi: 10.1016/j.gecco.2020.e00919
Turner, N. J., and Turner, K. L. (2007). Traditional food systems, erosion and renewal in Northwestern North America. Ind. J. Tradit. Knowl. 6, 57–68.
United Nations Population Division (2019). World Population Prospects 2019. Available online at: https://population.un.org/wpp/Graphs/Probabilistic/POP/TOT/903 (accessed July 17, 2020).
United States Department of Agriculture (USDA) (2020). Food Data Central. Available online at: https://fdc.nal.usda.gov/ (accessed June 29, 2020).
Vall, E., Andrieu, N., Beavogui, F., and Sogodogo, D. (2011). Les cultures de soudure comme stratégie de lutte contre l'insécurité alimentaire saisonnière en Afrique de l'Ouest: le cas du fonio (Digitaria exilis Stapf). Cahiers. Agric. 20, 294–300. doi: 10.1684/agr.2011.0499
Van Andel, T., Maas, P., and Dobreff, J. (2012). Ethnobotanical notes from Daniel Rolander's Diarium Surinamicum (1754-1756): are these plants still used in Suriname today? Taxon 61, 852–863. doi: 10.1002/tax.614010
Van Wyk, B.-E., and Gorelik, B. (2017). The history and ethnobotany of Cape herbal teas. S. Afr. J. Bot. 110, 18–38. doi: 10.1016/j.sajb.2016.11.011
Vandebroek, I., and Voeks, R. (2018). The gradual loss of African indigenous vegetables in tropical America: a review. Econ. Bot. 722, 543–571. doi: 10.1007/s12231-019-09446-3
Vester, K. (2015). “A Date with a Dish,” in Dethroning the Deceiftful Pork Chop: Rethinking African American Foodways from Slavery to Obama, ed J. J. Wallach (Fayetteville, AR: University of Arkansas Press), 47–60. doi: 10.2307/j.ctt1ffjdh9.10
Von Blum, P. (2012). African American visual representation: from repression to resistance. J. Pan Afr. Stud. 5, 41–51.
Voyages: The Trans-Atlantic Slave Trade Database (2020). Trans-Atlantic Slave Trade - Essays. Available online at: http://www.slavevoyages.org/voyage/essays (accessed July 1, 2020).
Washaya, S., Mupangwa, J. F., and Muranda, E. (2016). Nutritional value and utilization of yams (Dioscorea steriscus) by residents of Bindura Town high density suburbs, Zimbabwe. Adv. Agric. 2016:5934738. doi: 10.1155/2016/5934738
Wasylikowa, K., and van der Veen, M. (2004). An archaeobotanical contribution to the history of watermelon, Citrullus lanatus (Thunb.) Mastum. and Nakai (syn. C. vulgaris Schrad.). Veg. Hist. Archaeobot. 13, 213–217. doi: 10.1007/s00334-004-0039-6
Wehner, T. C. (2008). “Watermelon,” in Handbook of Plant Breeding, Vegetables I, eds J. Prohens and F. Nuez (New York, NY: Springer), 381–418. doi: 10.1007/978-0-387-30443-4_12
Werner, O., Magdy, M., and Ros, R. M. (2016). Molecular systematics of Abelmoschus (Malvaceae) and genetic diversity within the cultivated species of this genus based on nuclear ITS and chloroplast rpL16 sequence data. Genet. Resour. Crop Evol. 63, 429–445. doi: 10.1007/s10722-015-0259-x
Whit, W. C. (1999). Soul food as cultural creation. J. Study Food Soc. 3, 37–47. doi: 10.2752/152897999786690717
Whit, W. C. (2008). “Soul food as cultural creation,” in African American Foodways: Explorations of History and Culture, ed A. L. Bower (Urbana, IL: University of Illinois Press), 45–58.
Whitmore, T. M., and Turner, B. L. (2001). Cultivated Landscapes of Middle America on the Eve of Conquest. New York, NY: Oxford University Press.
Winchell, F., Stevens, C. J., Murphy, C., Champion, L., and Fuller, D. Q. (2017). Evidence for sorghum domestication in fourth millenium BC Eastern Sudan: spikelet morphology from cermaic impressions of the Butana group. Curr. Anthropol. 58, 673–683. doi: 10.1086/693898
Wolfe, P. (2006). Settler colonialism and the elimination of the native. J. Genoc. Res. 8, 387–409. doi: 10.1080/14623520601056240
Wood, A., Tolera, M., Snell, M., O'Hara, P., and Hailu, A. (2019). Community forest management (CFM) in south-west Ethiopia: maintaining forests, biodiversity and carbon stocks to support wild coffee conservation. Global Env. Change 59, 1–11. doi: 10.1016/j.gloenvcha.2019.101980
Wynberg, R. (2017). Making sense of access and benefit sharing in the rooibos industry: towards a holistic, just and sustainable framing. S. Afr. J. Bot. 110, 39–51. doi: 10.1016/j.sajb.2016.09.015
Yacob, S. (2019). Government, business and lobbyists: the politics of palm oil in US-Malaysia relations. Int. Hist. Rev. 41, 909–930. doi: 10.1080/07075332.2018.1457556
Yentsch, A. (2008). “Excavating the South's African American food history,” in African American Foodways: Explorations of History and Culture, ed A. L. Bower (Urbana, IL: University of Illinois Press), 59–100.
Yokota, A., Kawasaki, S., Iwano, M., Nakamura, C., Miyake, C., and Akashi, K. (2002). Citrulline and DRIP-1 protein (ArgE homologue) in drought tolerance of wild watermelon. Ann. Bot. 89, 825–832. doi: 10.1093/aob/mcf074
Zeven, A. C. (1964). On the origin of the oil palm (Elaeis guineensis Jacq.). Grana Palynol. 5, 121–123. doi: 10.1080/00173136409429132
Zhang, H., Gong, G., Guo, S., Ren, Y., Xu, Y., and Ling, K. S. (2011). Screening the USDA watermelon germplasm collection for drought tolerance at the seedling stage. Hort. Sci. 46, 1245–1248. doi: 10.21273/HORTSCI.46.9.1245
Zuckerman, C. (2016). 5 African foods you thought were American. National Geographic Magazine, Washington, DC. Available online at: https://www.nationalgeographic.com/culture/food/the-plate/2016/09/5-foods-from-africa/#close (accessed October 5, 2020).
Keywords: Africa, crop, domestication, African-American, cuisine, genetic resources, agronomy, resilience
Citation: Sousa EC and Raizada MN (2020) Contributions of African Crops to American Culture and Beyond: The Slave Trade and Other Journeys of Resilient Peoples and Crops. Front. Sustain. Food Syst. 4:586340. doi: 10.3389/fsufs.2020.586340
Received: 23 July 2020; Accepted: 25 November 2020;
Published: 15 December 2020.
Edited by:
Ademola Braimoh, World Bank Group, United StatesReviewed by:
Meine van Noordwijk, World Agroforestry Centre (ICRAF), IndonesiaCarlo Fadda, Bioversity International, Italy
Copyright © 2020 Sousa and Raizada. This is an open-access article distributed under the terms of the Creative Commons Attribution License (CC BY). The use, distribution or reproduction in other forums is permitted, provided the original author(s) and the copyright owner(s) are credited and that the original publication in this journal is cited, in accordance with accepted academic practice. No use, distribution or reproduction is permitted which does not comply with these terms.
*Correspondence: Manish N. Raizada, cmFpemFkYUB1b2d1ZWxwaC5jYQ==