- 1Institute for Environmental Economics and World Trade (IUW), Leibniz University Hannover, Hanover, Germany
- 2Institute of Environmental Policy and Resource Economics, Hochschule Weihenstephan-Triesdorf and Technical University Munich (TUM) Campus Straubing for Biotechnology and Sustainability, Straubing, Germany
- 3International Maize and Wheat Improvement Centre (CIMMYT), Texcoco, Mexico
There is an ongoing debate about how best to feed the growing world population in the long run and associated implications for research and development. Some call for a new Green Revolution to secure the supply of staple foods, whereas others emphasize the importance of diversifying and improving people's diets. We aim to contribute to this debate by reviewing the case of wheat and maize value chains and their contribution to food security in Africa and Asia. We first identify drivers transforming food systems. We then apply these to the cereal value chains and disentangle their effects on food security. We thereby add to the three strands in the literature around production, consumption, and food system transformation and point to different research needs and recommendations for the future. The review highlights: (1) Wheat and maize production will be increasingly impaired by ecological drivers such as land degradation, water scarcity and climate change. (2) There are promising innovations to increase and maintain productivity, but constraints in adopting these innovations have to be overcome (i.e., access to seeds, finance, and education/training). (3) The drivers affect all four dimensions of food security, but first and foremost they determine the availability and stability of maize and wheat. This indirectly also influences the economic and physical access of people to maize and wheat. (4) Research tends to focus on improving the productivity and sustainability of wheat and maize farming which is largely interlinked with the availability dimension of food security. (5) The stability and utilization dimension of food security merits continued yet increased support. First, to address climate change and implications for biotic and abiotic stresses. Second, to promote healthier diets and enable the equitable transformation of food systems.
Introduction
Some 820 million people (or 11% of the current world population) are undernourished in terms of energy intake, and 1.3 billion people (17%) suffer from micronutrient deficiencies (FAO et al., 2019). Most of them live in Asia and Sub-Saharan Africa (SSA). The COVID-19 pandemic is expected to further increase the numbers of vulnerable and food insecure people, especially in SSA. The worldwide recession disturbs global supply chains and will make it more difficult to finance the large food imports some countries especially in SSA depend on (FSIN, 2020a,b; OECD, 2020). Improved food security will depend on the sustainable use and management of resources, including land and water (esp. in Asia) and water and nutrients (esp. in SSA) while staying within planetary boundaries. Finding the right balance between food and nutritional security, protecting the environment and addressing climate change remain major challenges for sustainable food systems and the use and management of land and water (IPCC, 2019; Willett et al., 2019).
There are a number of review papers which have dealt with the general question of how to feed the growing world population in the future (e.g., Conway, 2012; Grote, 2014; Le Mouël and Forslund, 2017). However, only a few papers have been devoted to the specific role of selected staple food crops, including wheat (Shiferaw et al., 2011; Tadesse et al., 2018) and maize (Shiferaw et al., 2013). Here we set out to review the case of wheat and maize value chains and their contribution to food security in Africa and Asia.
The global wheat production amounts to an annual average of around 750 million tons (t) (2016–2018, OECD/FAO, 2019). Major producers in the developed world are Europe and North America and Asia in the developing world (Figure 1), particularly China and India. At the same time, consumption largely outpaces production in Asia but also in Africa, making these two regions major net importers of wheat.
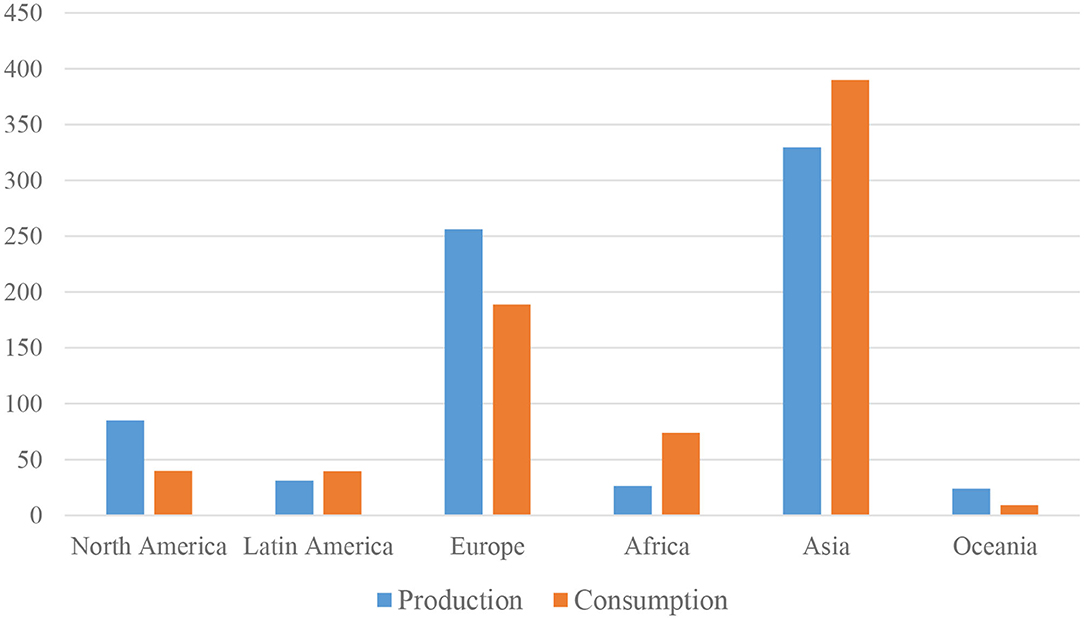
Figure 1. Regional wheat production and consumption [average of 2016–2018 (est.), in million t] (Source: OECD/FAO, 2019).
The technological intensity of wheat production varies among continents and within regions. Wheat yield averages some 3.5 t/ha, but there are large regional differences. Average yields are higher in East Asia and the European Union (4.3–5.3 t/ha). In South Asia yields are 3 t/ha and in Africa 2.6 t/ha. These differences can be partly explained by differences in crop management (e.g., the use of fertilizer, irrigation) and agro-ecology (e.g., temperature, rainfall, soil quality). For predominantly irrigated wheat production in Egypt average yields are 6.5 t/ha (Dixon et al., 2009). Particularly in Asia, high yields, profitability, and institutional support led farmers to increase their wheat production area at the expense of other crops (Hazell, 2009).
Wheat is the staple crop for an estimated 35% of the world population (IDRC, 2010). More than two-thirds of global wheat is used for food and one fifth is used for livestock feed (Figure 2). But the annual per capita consumption of wheat varies widely—from 170 kg in Central Asia to 27 kg in Eastern and Southern Africa (Shiferaw et al., 2013). China and India each consume 17–18% of global wheat (RaboResearch, 2017). However, their large and dietary diverse population imply that wheat provides a national average of around 500 kcal of food energy per capita per day (Dixon et al., 2009). Feed use is prevalent in developed economies but not in Africa (RaboResearch, 2017).
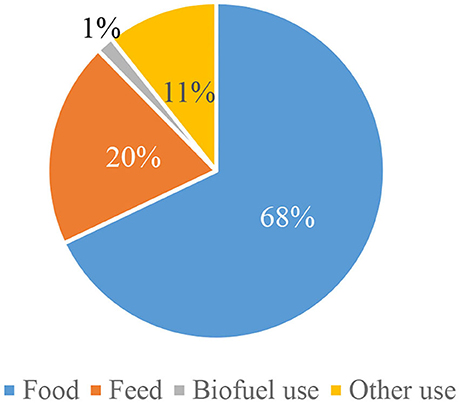
Figure 2. Global use of wheat [average 2016–2018 (est.), in %] (Source: OECD/FAO, 2019).
The global maize production amounts to an annual average of 1,127 million t (2016–18, OECD/FAO, 2019). It can be grown in a wide array of agro-ecologies, including diverse temperatures, altitudes and latitudes, land and soil types—though with quite different yields per ha. The major maize producer is North America, followed by Asia, especially East Asia. Its high yields (relative to other cereals) make it particularly attractive to farmers in areas with land scarcity and high population pressure (Shiferaw et al., 2011). Overall, 61% of global maize production is used as livestock feed and only 13% for human consumption (Figure 3). It plays a particularly important role as staple food in the diets of millions of people in Africa and South Asia (and Latin America), whereas in East Asia most maize is used as livestock feed (Erenstein, 2010b). Like with wheat, there are still substantial maize deficits in Asia and Africa, making both continents major net importers and the central focus of our review.
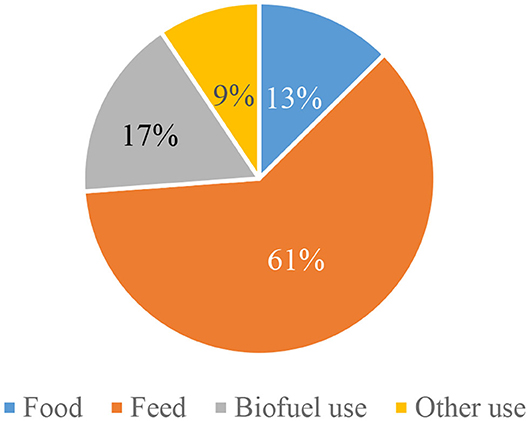
Figure 3. Global use of maize [average 2016–2018 (est.), in %] (Source: OECD/FAO, 2019).
Recommendations on future investments in cereal research vary in the face of limited financial resources. Shiferaw et al. (2013) stress the importance of further increases of maize productivity by breeding new high yielding, stress-tolerant and widely adapted maize varieties accompanied by new farming practices. With respect to wheat, a new “Green Revolution” and sustainable intensification are suggested as strategies to improve global food security (Shiferaw et al., 2011). But also investments in new wheat varieties resistant to diseases and pests, adapted to warmer temperatures, and in need of less water, fertilizer, labor, and fuel are called for. By contrast, Pingali (2015) perceives a disconnect between staple crop demand and supply trends along with a lower need for further investment in staple crop production. He suggests a crop-neutral agricultural policy which is not biased toward staple crops and which opens new ways of investing into horticulture crops. Furthermore, he emphasizes the importance of improving people's diets toward more diversified and better quality food to avoid malnutrition of the poor and overweight and obesity of the middle class. At the same time, his paper shows the continued and stable role for staple cereals in global diets over the last two decades—despite overall per capita increases in calorie intake and the diversity of food groups over the same period.
Furthermore, the FAO (2017: 83–84) projects staple cereals to continue to play a critical role for food security till 2050, contributing nearly half of both daily calories and protein intake in low- and middle-income countries. Together with population growth, this suggests the need for further productivity growth in cereals in the decades to come to stay within planetary boundaries. Staple cereals thereby will continue to play a critical role to ensure an adequate and affordable intake of calories and proteins in diets. What is more, wholegrain cereals like wheat and maize provide multiple dietary components that can help address the triple burden of undernutrition, micronutrient malnutrition and overnutrition (overweight/obesity and non-communicable diseases—Poole et al., 2020). Still, staples are not a panacea for food security and diverse diets call for complementary investments in the other food groups, food system transformation, and overall development. For example, there are climate-resilient and nutrient dense cereals such as sorghum and millets (Rodríguez et al., 2020). Nelson et al. (2018) emphasize the need to provide nutritious diets by promoting the production of micronutrients rather than increasing the availability of macronutrients (calories). Willett et al. (2019) go a step further by calling for a global transformation of the food system and suggesting global targets for healthy diets and sustainable food production.
This paper aims to contribute to the debate by (i) identifying the major drivers of food system changes and applying these to the maize and wheat value chains with a focus on Africa and Asia, (ii) disentangling the effects of the drivers at different levels of the cereal value chains (production, processing and distribution, and consumption) on the four dimensions of food security, and (iii) deriving policy implications for research and development.
The added value of the paper is 3-fold. First, few papers focus on the dynamics of the wheat and maize value chains which allows to draw a much more detailed picture of the research needs and policy recommendations. Second, the focus on Africa and Asia is interesting because the two continents follow very different pathways with respect to cereal production and they are both net cereal importing regions. And third, taking a closer look at food security is pivotal, given the substantial number of food insecure people on these two continents.
The outline of the paper is as follows: We first present the conceptual framework with the cereal value chains and their drivers and their implications for food security in the following section. We then focus on the different dimensions of food security—availability, access, utilization and stability—related to maize and wheat. The results are then used to identify areas of future research and investments in the wheat and maize sector vis-à-vis other crops in the fourth section. The final section concludes.
Conceptual Framework: Cereal Value Chains and Their Drivers of Change
This review paper draws on recent secondary literature sourced from peer-reviewed journals and reports of international organizations such as the UN (FAO, etc.), the World Bank, international agricultural research centers (CIMMYT, IFPRI) and the OECD. It provides a balanced comprehensive review—but is not intended to be a systematic review or meta-analysis, which have been variously criticized (Borenstein et al., 2009) for comparing and combining very different types and quality of studies. We also include both qualitative and quantitative studies to avoid bias (Denyer and Tranfield, 2011).
Drivers which transform food systems have been analyzed (see for example Béné et al., 2019, 2020). We use their framework and apply it to cereal, especially maize and wheat, value chains (Table 1). Based on this conceptual framework, we first identify relevant drivers transforming wheat and maize (i) production, (ii) distribution/trade and governance, and (iii) consumption. Some drivers affect positively the value chain, while others have negative or ambiguous effects. There can be significant interactions between drivers of change, e.g., some technological innovations are being purposively developed in response to the challenges and opportunities provided by other drivers. Furthermore, the drivers vary across continents and regions, as pointed out for Africa and Asia. For example, land is relatively more available in Africa than in most of Asia, whereas for irrigation infrastructure it is the other way around. Finally, the drivers change over time but with different speed. While the degradation of soil, land and water is already existent in many regions, climate change is expected to have longer-term effects. Also technological innovations differ widely in their usage within and across continents and need to be locally adjusted to their environments.
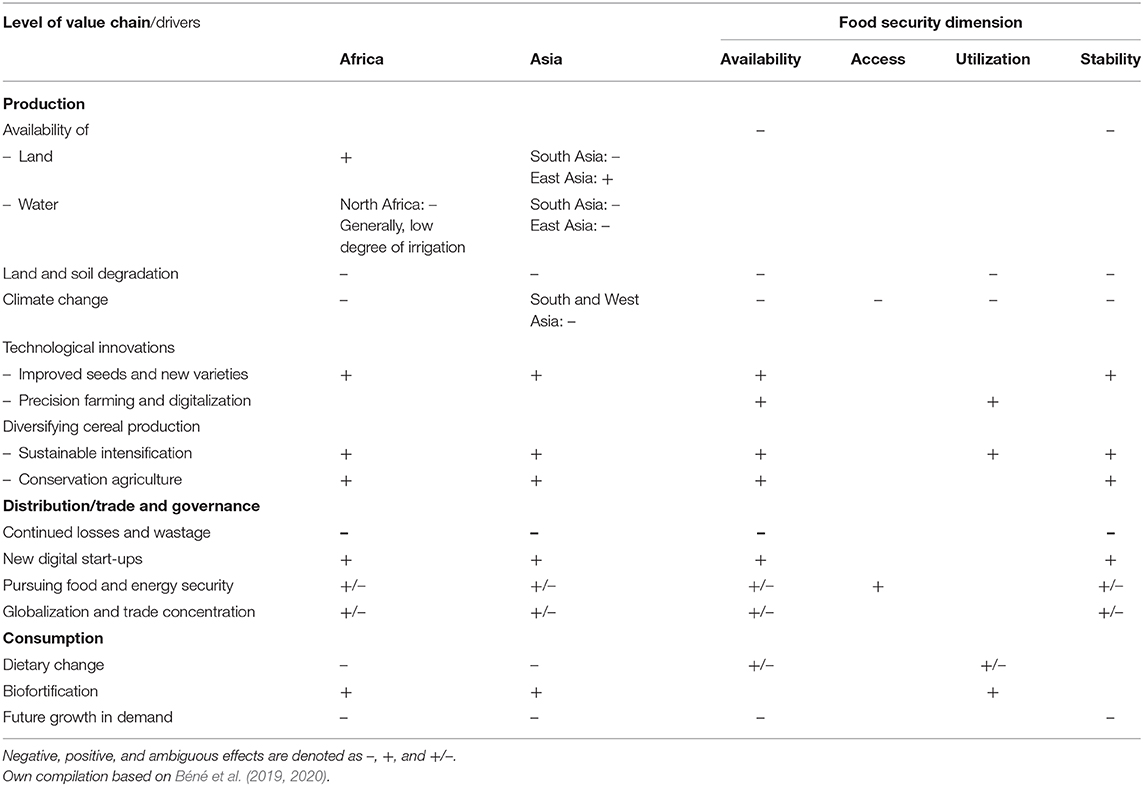
Table 1. Drivers transforming cereal value chains in Africa and Asia and their impacts on food security.
These different drivers have implications for food security which is defined as a situation “when all people at all times have physical and economic access to sufficient, safe and nutritious food that meets their dietary needs and food preferences for an active and healthy life” (FAO, 1996). Food security comprises of four dimensions: (1) availability of food which hinges upon domestic production and/or imports; (2) access to food which depends on individuals having sufficient resources or entitlements to obtain food; (3) utilization of food which is reflected by the quality and diversity of diets, and of clean water; and (4) stability which ensures that food can be accessed by individuals at all times (FAO, 2006). Overall, we anticipate that by disentangling the drivers of change and their effects on the four dimensions of food security, the specific research needs in the maize and wheat value chains become evident.
Production
Declining Land and Water Availability
The declining availability of land and water has a negative effect on cereal value chains. In Africa farming land for future expansion is still perceived to be available (Bruinsma, 2009). In South Asia, the potential for crop expansion is almost negligible, whereas East Asia has some 100 million ha potentially available (Bruinsma, 2009). It needs to be considered that on the one hand, converting land to cropland will generate environmental cost in terms of increased land degradation, CO2 emissions and biodiversity loss (WBGU, 2020). On the other hand, intensification of cereal production can free marginal land and reduce pressure on natural ecosystems from being converted to agriculture (Stevenson et al., 2013).
Irrigation is prevalent in South Asia and in East Asia, followed by North Africa. In SSA, the production of maize and wheat is mostly rainfed and largely depends on the vagaries of the weather. Increasing global water scarcity limits the prospects of developing irrigation systems (Hanjra and Qureshi, 2010; Chartres and Noble, 2015). But not only in Africa, also in South Asia, food production is most likely affected by water scarcity (Falkenmark, 2007). Water for irrigation constitutes generally one of the greatest pressures on freshwater resources. Globally, most freshwater is withdrawn by agriculture—in many countries up to 70%, and even up to 90% in some fast-growing economies (UN Water, 2014; Chartres and Noble, 2015).
Today's food production requires a consumptive water use of 6,800 km3/year (Hanjra and Qureshi, 2010). To feed the growing world population by 2050 on 3,000 kcal per person per day, an additional 5,600 km3/year will be required: a maximum of 800 km3/year will come from blue water resources (i.e., due to irrigation expansion and efficiency improvements) while the remaining 4,800 km3/year will have to come from new green water resources. The water gap will lead to a food gap and affect global food security, if not filled (Rost et al., 2008).
In response to water stress, there is much interest in enhanced irrigation technologies to increase water and energy efficiency. Micro-irrigation systems such as drip and sprinkler methods have the potential to improve both water productivity and energy efficiency in groundwater irrigation. Evidence from Asia suggests that drip and sprinkler methods can result in water savings of 40–80% and increase crop productivity by 100% if properly applied (Sivanappan, 1994). Despite the overall decline in the costs of irrigation equipment, the adoption of irrigation technologies still remains limited due to the lack of financial capital by most smallholders, especially in SSA (Girodano and de Fraiture, 2014).
Land and Soil Degradation
About two-thirds of agricultural land had been degraded in the last 50 years, and 40% seriously (Bindraban et al., 2012). Furthermore, every year soil degradation leads to a loss of an estimated 12 million ha of agricultural land, which causes a potential loss of at least 20 million t of grain per annum (UNCCD, 2021). Nkonya et al. (2016) estimate that about 30% of the global land area is degraded, and three billion people reside on degraded land. Thus, crop production is variously impaired by land degradation, including loss of vegetative cover and soil fertility, desertification, erosion, acidification, and salinization. Land degradation, again, may negatively affect crop productivity, especially if the crop is not well-fertilized (Oelofse et al., 2015). As an example, the yield potential of maize declines by 25% if soil organic matter content drops by 1.7–4.3% (Lucas et al., 1977). A more recent result from Bakker et al. (2004) suggests that crop productivity drops by 4–27% per 10 cm of soil loss. It is thus estimated that land degradation may annually cost the global economy US$ 66 billion from losses in net primary production (Nkonya et al., 2011).
Climate Change
Crop production is also increasingly impaired by weather variability and climate change which is associated with increasing temperatures (e.g., increasing heat stress), especially in the (sub-) tropics, and with more severe and frequent extreme weather events (e.g., droughts, floods) and changing agro-ecological conditions (e.g., crop seasons) (Challinor et al., 2014). Next to declining crop productivity and yields of staple crops (Curtis and Halford, 2014), also some cultivated areas may become unsuitable for cropping. The biggest losses in suitable cropland under climate change are likely to be in Africa (Schmidhuber and Tubiello, 2007), including double cropping area declining by 10–20 million ha. Climate change effects on crop production are often most directly associated with the abiotic stresses of heat and water (Ortiz et al., 2008; Cairns and Prasanna, 2018), but also with biotic stresses such as insects (Deutsch et al., 2018). The past decade has also seen the emergence of new virulent pest and diseases in previously unaffected areas. Thus, there are complex and still poorly understood agro-ecological linkages and systemic effects likely to arise from climate change.
With respect to wheat and maize production, climate change is expected to result in losses, especially in Asia (Shiferaw et al., 2011, 2013) and in SSA (FAO, 2009; Nelson et al., 2009). Average maize production is expected to decline by 9–19% in South Asia and around 10% in SSA from 2000 to 2050. In Tanzania, e.g., it is estimated that due to climate change maize yield will shrink by 33% country-wide and by up to 84% in the central regions (URT, 2007). For wheat production, the estimates are more severe, ranging between 44 and 49% in South Asia and 34% in SSA (Nelson et al., 2009). These ranges of results indicate that there is a great uncertainty with respect to future forecasts under climate change. In addition, it is expected that due to climate change food insecurity will be exacerbated in areas which are already vulnerable and that food access and utilization will be indirectly impaired (Wheeler and von Braun, 2013). In contrast, traditional crops such as sorghum and millets are considered as being more climate resilient and may be more productive in marginal environments (Altieri, 2009), but often lack markets and consumer preference.
Technological Innovations
Improved Seeds and New Varieties
Improved seeds have the potential to transform the maize and wheat value chains. Crop breeding offers particular prospects of addressing emerging challenges associated with climate change and the water crisis, including ongoing efforts to make new varieties drought and heat tolerant and biotic stress tolerant/resistant (Pardey et al., 2013; Asseng et al., 2017; Cairns and Prasanna, 2018). However, part of the challenge is that improved seeds rely on a breeding pipeline—a continuous investment effort to include new traits into the best and adapted products that cannot be switched on and off when needed. Another challenge relates to biotic stresses (such as wheat rust, Pardey et al., 2013) which continue to evolve, circumventing the protective traits put in place. Indeed, much of the needed investment goes to maintenance breeding—i.e., simply maintaining productivity potential. This calls for conserving genetic resources and broadening the genetic base of varieties, making them accessible for breeding, and changing varieties more frequently over time to avoid pests, disease, and climate risks (Smale et al., 2009). Under climate change conditions though, it has been found that a more frequent change in varieties will not be sufficient to deal with increased variability, and may call for increased diversity of cropping systems and wheat varieties (Kahiluoto et al., 2019).
Biotechnology provides new impetus to crop improvement (e.g., molecular markers, gene editing). Prominent examples include Bt crops (Bacillus thuringinesis) which make the crops resistant to certain insects. Opponents of particularly gene editing raise ethical concerns and they emphasize the potential risks for health and the environment (e.g., Zhang et al., 2016). Finally, there is the concern that a few profit-seeking private seed companies which are the main players in research and development of GM crops, will create new dependencies for countries and smallholder farmers. Laxman and Ansari (2011) state that most African countries have not formulated adequate legal and institutional frameworks to regulate, monitor, and ensure safety of GM food produced and/or imported by them. Finally, it is pointed out that food insecurity in Africa is not a technological issue but rather a structural one (Egziabher, 2003).
In contrast, proponents see GM crops as potential sources of increased food supply and environmental sustainability due to the reduced application of pesticides and herbicides. Furthermore, GM crops have the potential of higher nutritional values since they can be enriched with certain nutrients (e.g., Golden rice) or other health benefits. Finally, GM crops can reduce losses and wastage, because the ripening process of products has been reduced or their shelf life extended (Zhang et al., 2016).
Precision Farming and Digitalization
Precision agriculture provides another potential technological revolution already gaining a foothold in much of the developed world though it is still in its infancy in much of Africa and Asia. It includes agricultural mechanization supported by drones, sensors, and/or robotics (WBGU, 2019). It results in an increased precision in applying seeds, water, fertilizers, and pesticides and a better monitoring of land use management based on digital documentation, the use of meteorological data, and satellite positioning systems. However, it has to be ensured that precision farming—once it takes off—does not promote large-scale and specialized production systems but rather results in technical innovations adjusted to small-scale farming systems as prevailing in Africa and Asia (WBGU, 2020).
ICT advances have the potential to simply increase access to information and capital and to lower transaction costs. They are already widely visible in Africa and Asia. By 2020, 80% of the adult population globally are expected to own a smartphone (Economist, 2015). Mobile phones and especially smartphones help to share information on market prices for inputs and outputs, the availability of inputs, on customers and their requirements in terms of quantity and quality of outputs or on payment conditions and financial transfers (WBGU, 2019). In addition, they deliver a number of agri-advisory services, e.g., on crop and plant health and diseases or help to overcome literacy and language barriers.
Diversifying Cereal Production
A number of reviews on sustainable cereal production have been compiled in order to promote both cereal yields and their nutritional quality while reducing costs to farmers and the environment (FAO, 2016; WBGU, 2020). They highlight examples from Zambia, where nitrogen-rich trees in maize fields have been identified as being a cost-effective alternative to mineral fertilizer. In East Africa, serious maize pests have been overcome by growing local plants in maize fields. Worldwide, farmers increased their wheat yields by growing legumes which provide nitrogen (Stagnari et al., 2017). Considerable benefits in terms of yields and sustainability have been also found in integrated systems where maize and wheat have been integrated with livestock production and agro-forestry in SSA (e.g., Baudron et al., 2014). However, so far, these approaches have been mostly applied in more difficult farming conditions to increase climate resilience (FAO, 2016). Though they have the potential to increase sustainability and preserve a number of different ecosystem functions (WBGU, 2020).
Sustainable Intensification
Sustainable intensification has been found to increase yields in rainfed and irrigated systems for maize (Roxburgh and Rodriguez, 2016) and wheat, and potentially provide additional ecosystem services (e.g., water and energy savings and greenhouse gas emissions reductions) (Malik et al., 2005; World Bank, 2008; Kassam and Brammer, 2013). In recent years, innovation research has started to address institutional and knowledge barriers to technological adoption in African agriculture especially focusing on knowledge creation among small-scale farmers, extension and advisory services and related stakeholders (Sanyang et al., 2016). Knowledge gaps mainly relate to sustainable intensification and how well it can be effectively out-scaled. Further knowledge gaps relate to greater use of pulses and nitrogen-fixing legumes to improve soil fertility, adoption of more productive and nutritious cereal varieties, water technologies, or pest and disease controls (FAO, 2016). There are several approaches which show promise for sustainable intensification, such as conservation agriculture, agroecology, climate-smart agriculture, and organic farming that all deserve attention in relevant contexts.
Conservation Agriculture
Conservation agriculture (based on minimum tillage, ground cover, and crop rotation) has been variously advocated worldwide as a resource-conserving technology. It has even been labeled one of the “most dramatic technological revolution in crop management” (World Bank, 2008, p. 163). In rice-wheat systems especially in India, farmers increasingly plant wheat directly after the rice harvest (Malik et al., 2005). Conservation agriculture can save inputs and generate substantial economic and environmental benefits, including yield increases for maize and wheat (Erenstein et al., 2012). However, this is not always the case as shown by Pittelkow et al. (2015) in their meta-analysis comparing no-tillage agriculture with conventional tillage. Furthermore, adoption rates of conservation agriculture systems vary regionally, smallholders often being hampered by institutional bottlenecks including low education and training levels (Erenstein et al., 2012). It has been also found to work best in those locations where competition for biomass is low, where livestock is not the major component of the system, rainfall is high, access to herbicides is affordable and mechanization is practiced (Giller et al., 2015). On the one hand, this calls for better targeting and adaptation of conservation agriculture practices, particularly for smallholders (Erenstein et al., 2012). On the other hand, a rigorous, context-sensitive systems agronomy approach is suggested to analyse and explore the potential of conservation agriculture (Giller et al., 2015).
A more general adoption of sustainable farming approaches requires fundamental changes in the governance of food systems. Thus, a concerted action at all levels (international organizations, public and private sectors, civil society) is called for. Investments and provision of public goods (i.e., infrastructure) by the governments is suggested next to promoting technological innovations adapted to smallholder needs, but also supporting farmers' adoption of sustainable production systems by education and training; establishing and protecting farmers' rights to resources; promoting fairer and more efficient markets and value chains; increasing support to long-term agricultural research and development; strengthening formal and informal seed systems; or increasing collaboration with international organizations, instruments, and mechanisms (FAO, 2016).
Distribution/Trade and Governance
Continued Losses and Wastage
The increasingly complex food systems with food transported over longer distances in the context of globalization, urbanization, and dietary change pose continuing challenges. Food losses and wastage occur which differ by type of food, region, and season. Some estimate that 30–50% of all food products globally get wasted (Lundqvist, 2010; Gustavsson et al., 2011; Capone et al., 2014). For cereals, annual global food losses and waste are estimated at around 30%, in comparison to 40–50% for root crops, fruits, and vegetables (FAO, 2013). Cereal wastage has been especially associated with poor storage at the farm and post-harvest level (estimated up to 50–60% by Kumar and Kalita, 2017). With respect to maize, storage insect pests have been reported to cause an estimated 20–30% loss in the tropics; occasionally, the losses are even higher due to grain weevils and the larger grain borer (Shiferaw et al., 2011).
To reduce food losses, a number of initiatives have been successfully developed. For example, a platform on best practices helps to share experiences among stakeholders to reduce losses. On a more practical side, re-usable crates for transportation of fresh food have been found to reduce losses, next to sealed bags which can store cereals for longer periods. In general, it has been found that financial, managerial and technical constraints must be overcome with respect to harvesting and handling techniques as well as storage and cooling facilities (FAO, 2019b).
New Digital Start-Ups
Information as such can be accessed by the farmers by either phoning somebody to call for advice, via SMS or via special internet sites which list respective customers (traders, processors etc.). There are a number of digital start-ups i.e., in Asia but also in Africa. They offer diverse digital solutions for the smallholder farming sector and the food industry (Ekekwe, 2017). In Ghana for example, the start-ups Farmerline and AgroCenta use mobile and web technologies that bring advice on farming, weather forecasts, market information, and financial information in different languages to illiterate farmers in remote areas. The new enterprise Sokopepe offers market information and farm record management services via SMS and web tools to farmers. In Kenya the start-up M-Farm offers pricing data to farmers to remove price information asymmetry (Ekekwe, 2017).
Tadesse and Bahiigwa (2014) find efficiency gains via mobile phone-based information exchange. FAO (2016) reports for example about yield growth based on a smartphone-based “crop manager” which calculates crop and nutrient management recommendations based on local conditions and provides this information to the farmer via SMS. Courtois and Subervie (2015) estimate that Mobile-based Information Systems (MIS) in Ghana reduced asymmetric market information and farmers benefited significantly from higher prices for maize (10%) compared to selling activities without using MIS.
Pursuing Food Security
Food security policies also transform cereal value chains. Many countries in Africa and Asia pursue food security policies which include producer-oriented policy measures to secure domestic food supply and reduce reliance on imports, including input subsidies, producer price support or an increase in storage capacity. For example, an increasing array of countries (including China, Indonesia, Malaysia, the Philippines, Senegal, Ethiopia) have declared food self-sufficiency as their strategic goal to ensure longer-term sustainable domestic food supply. South Asian countries have adopted policy measures to promote food production, especially of staple crops such as wheat and rice (Rasul, 2014). This also has cross-border effects. In the Middle East/North Africa (MENA), wheat has become one of the most protected agricultural commodities. Some MENA countries also started to invest in offshore production as part of their food security policy (Ahmed et al., 2014).
Food security policies can incentivize (un)sustainable production practices. In South Asia, subsidized energy (e.g., electricity) promoted irrigated rice and wheat production with little concern for water use efficiency (Erenstein, 2010a; Rasul, 2016). This has resulted in serious problems such as groundwater depletion, salinity, and waterlogging (Alauddin and Quiggin, 2008). It has also made food production increasingly vulnerable to changes in the availability and price of energy (Rasul, 2014). Food system development may also be enabled by broader policies—e.g., multisectoral policy harmonization, incentives, and diversification of employment sources; next to policies needed to recognize and understand the diversity among smallholder farm households in, e.g., SSA (Frelat et al., 2016).
To secure adequate and affordable staple food to the domestic populace, consumer-oriented policy measures such as food subsidies or price controls are taken; they provide direct support to consumers and vulnerable groups. Malawi, for example, announced that all maize would be sold through the Agricultural Development and Marketing Corporation (ADMARC); they also fixed the price at which ADMARC would buy and sell maize (IRIN, 2008).
Trade-oriented policy measures such as tariffs (e.g., import/export taxes on inputs and outputs) and non-tariff barriers (e.g., import/export restrictions) can further (dis)incentivize cereal value chains. Some such policies again reflect domestic food security policy concerns. For instance, Demeke et al. (2008) count that 25 countries restricted or banned food exports after the food price crisis to reduce transmission of the increase of world prices to their domestic markets. In southern Africa, Zambia has banned the import of maize and wheat for many years to protect local producers. Tanzania has also had an export ban on maize (Keyser, 2015). These interventions did aggravate the volatility of food prices (Demeke et al., 2008). SSA includes areas with good growing conditions that are already producing food surpluses, but international trade is largely impaired by various constraints (Gillson and Fouad, 2015). Many countries in SSA also restrict the use of genetically modified (GM) organisms (Shiferaw et al., 2011). This includes import restrictions on GM maize which hampers interregional (e.g., from South Africa) and international trade (e.g., USA) and thus limits procurement options in case of severe production shortfalls such as during the recurring El Nino across southern Africa (for a discussion on GM crops, see section Production, improved seeds and new varieties).
Globalization and Trade Concentration
Own crop production contributed 60% of calorie availability across diverse smallholder farm households in Africa, with the degree of market access having an influential role on overall food availability (Frelat et al., 2016). Staple cereal production primarily caters to domestic consumption (Gillson and Busch, 2015), with imports making up the shortfall and with the food system increasingly affected by globalization. International trade of wheat is largely (90%) dominated by five large transnational companies (TNCs). These firms can broker deals and reap profits based on their significant market power, even during global food shortages like during the 2007–08 global food crisis (Ahmed et al., 2014). Globalization, financial services and deregulation throughout the food system have transformed TNCs and the role of other actors such as traders and governments, including TNCs diversifying into new industries and expanding their operations into various importing and exporting nations (Ahmed et al., 2014). Regional cross-border food trade in Africa still largely relies on informal channels: estimated at 70% in West Africa and 80% in the East African Community (EAC) and surrounding countries (Keyser, 2015). Furthermore, mainly small traders are involved in such regional trade, facing high transaction costs and time-consuming, repetitive procedures at the borders.
Consumption
Dietary Change
Changing food preferences, diet acceptance and awareness also represent an important driver in cereal value chains (Trethowan and Turner, 2009). For example, wheat and maize are not homogeneous commodities with associated cultural preferences. Wheat comprises bread wheat and durum wheat (e.g., used for pasta), whereas bread wheat includes different qualities catering to leavened or flat bread preferences (Peña-Bautista et al., 2017). With respect to maize, women have a stronger preference for white maize than men. This is also true for higher educated consumers (De Groote and Kimenju, 2008). White maize is generally mostly used as food for human consumption, whereas yellow maize is an important animal feed—especially for monogastrics like poultry and pigs (Mottet et al., 2017).
Due to the strong demand for maize as livestock feed, it is expected that the demand for maize will grow faster than that for wheat (Dixon et al., 2009). Rosegrant et al. (2009) forecast that the demand for maize in the developing world will double between 2010 and 2050. In particular, the maize feed market is growing rapidly in both China and India, where economic growth and increasing per capita incomes are enabling many to buy livestock products (Erenstein, 2010b). Still, consumer preferences vary by geography and income. For instance, cultural and religious reasons result in regional differences: while China has observed a very strong growth in the consumption of high-energy foods such as livestock products, in India, this process has been less pronounced despite comparable income levels (Godfray et al., 2010a) and with a narrower focus on poultry (Hellin et al., 2015).
Cereal dietary transformations took place in Africa before, where tens of millions of people nearly completely changed their diets from traditional sorghum and millet to maize in less than two generations (Byerlee and Heisey, 1997). The transformations still continue nowadays, with ongoing marked shifts between staple cereals (Economist, 2017). Wheat is a case in point, with its consumption showing high increases in non-traditional wheat consuming areas in (urban) Africa (Mason et al., 2015) but also in Asia (Mottaleb et al., 2018a).
Biofortification
Biofortification increases the content of essential micronutrients in the edible parts of cereal crops (Hubert et al., 2010); these can benefit poor households in their ability to combat chronic malnutrition (Hefferon, 2015). While maize provides vitamin A and E, it lacks the lower B vitamins which are relatively more present in sorghum or wheat; maize has also low contents in usable protein, and its leucine blocks the absorption of niacin, a vitamin which prevents protein deficiency in human bodies (McCann, 2005). Maize has been biofortified with β-carotene and wheat with higher levels of micronutrients, such as iron and zinc (Hefferon, 2015). For biofortified provitamin A maize varieties, the color of the maize grain alters from white to yellow or orange which is of lower acceptance compared to non-fortified white maize (De Groote and Kimenju, 2012). However, women have been found to have a stronger preference not only for white but also for fortified maize than men (De Groote and Kimenju, 2008). Furthermore, studies show that African consumers could differentiate between new and conventional maize products based on sensory changes (De Groote et al., 2014). Finally, improved post-harvest practices can reduce potentially toxic compounds in wheat and maize to enhance food quality, safety, and human health (Hubert et al., 2010).
Future Growth in Demand
Demographic drivers transforming cereal value chains include population growth and urbanization. This growth is expected to mainly happen in Africa and Asia. While in Africa, a doubling of its population up to 2.5 billion is expected till 2050 due to its high fertility rates, in Asia with its already high population density, an increase by another billion up to 5.3 billion is likely (UN, 2019; Worldometer, 2020). The growing population is expected to increase the demand for wheat from 621 million t during 2004–2006 to more than 900 million t in 2050 (Hellin et al., 2012). Staple cereals are projected to continue to supply half of the daily calories and protein intake in low- and middle-income countries till 2050 (FAO, 2017). Thus, population growth alone will thereby continue to be a major driver for staple food demand such as wheat and maize in Africa and Asia.
Urbanization will also increase further over the coming decades. In Africa, 43% of the population is currently urban, whereas in Asia, it is almost 51% (Worldometer, 2020). It is estimated that more than 70% of the world population will live in cities by 2050−20% more than now (Ritchie and Roser, 2019). At the same time per capita incomes are expected to increase in many developing countries. Taken together, urbanization and income growth change consumption habits, which is variously observed in highly populated regions across Asia, the Middle East and Latin America (Delgado, 2003; Gulati and Dixon, 2008). This includes a rise in demand for diet diversity (Pingali, 2015) but also for more livestock products with major implications for greenhouse gas emissions and land-clearing (FAO, 2017). This “nutritional or dietary transition” however also coincides with people eating high-energy diets including sugars, fats and oils, next to meat (Popkin, 2010; Tilman and Clark, 2014).
Implications of Cereal Value Chain Transformation for Food Security
Food security is affected by diverse drivers to different extents in Africa and Asia. While most environmental aspects have negative impacts on especially the availability and stability of food security in most of Africa and Asia, the adoption of technological innovations and some policy approaches are expected to result in positive outcomes. There are also implications for utilization and access but these remain somewhat masked by discussions of aggregated trends rather than on individual aspects. We change perspectives now and highlight some of the constraints but also opportunities departing from the four food security dimensions.
Food Availability
Availability of food is one of the key food security dimensions and hinges upon domestic production and/or imports on the one hand and population growth and demand patterns on the other. Wheat and maize are the most widely cultivated and traded cereals in the world. Still, the growth rate of yields for major cereals steadily slowed from 3.2% in 1960 to 1.5% in 2000 (Lumpkin, 2011), raising concerns about the ability to meet global food demand growth (Mueller et al., 2012; Ray et al., 2012). Existing cropland areas are threatened by steady land degradation (FAO, 2011), increasing water scarcity (UNDP, 2007) and competing land uses (including urbanization and non-agricultural economic development). Yet according to Bruinsma (2009), in developing countries, 80% of the needed production increases have to come from higher yields and cropping intensity up to 2050 and only 20% from the expansion of farmland. Further competition for land may increase in the future from climate policy and the need to halt the loss of biodiversity. Thus, availability of wheat and maize also hinges on ecological and political drivers of change, while technological factors via e.g., precision agriculture have the potential to ease some of the pressure.
Asia is a case in point. Its cereal production virtually doubled between 1970 and 1995 [Asian Development Bank (ADB), 2000] associated with the uptake of modern varieties complemented by intensification practices. Modern varieties were widely adopted since the 1960s on about 90% of the area in Asia and were central to the Asian “Green Revolution” (Hazell, 2009). The spread of semi-dwarf crop varieties were complemented by irrigation and fertilizer and policy support, contributing to significant yield increases from 1965 to 1985 (Erenstein, 2010a). Maize production doubled in the second half of the twentieth century linked to the use of improved crop varieties and more inputs including fertilizer, water, and pesticides (Evenson and Gollin, 2003). Yet the “Green Revolution” that ensured food sufficiency in much of Asia has stalled, most notably in India (Evenson et al., 1999; Hazell, 2009; Pingali, 2012). Since 2000 yields stagnated in major wheat growing regions which is explained by many interlinked factors, such as slowing rates of genetic enhancement, loss of soil fertility, declining input use-efficiency, and a number of biotic and abiotic stresses, associated inter alia with the prevailing cropping systems practices and the associated institutional environment (FAO, 2016).
Africa's food system has taken a different pathway. The green revolution did not take off and cereal yields and production remain at very low levels. van Ittersum et al. (2016) project Africa's population to increase 2.5-fold and demand for cereals approximately to triple by 2050, whereas current levels of cereal consumption already depend on substantial imports. This calls for sustainable intensification paired with cropland expansion since land is still relatively available.
On aggregate Africa and South Asia are net food importers in terms of calories (FAO, 2017: 29). Availability of staples such as wheat and maize thereby remains a concern for the decades to come and calls for continued investment in domestic production capacity where countries have a comparative advantage to reduce reliance on global markets. In developing economies, agricultural development normally precedes the development of other sectors—but the reliance on agricultural imports presents new unchartered territory as well as the need to produce and trade non-agricultural products. At the same time, reliance on imports has its limits—as maize and wheat are heterogeneous commodities. A case in point is the preference for white maize—which is much less produced and traded compared to yellow maize and so can pose procurement challenges. Similarly, an anti-GM stance in southern Africa limits the region's ability to import maize, with non-GM maize being a much smaller market. Trade in wheat and maize is also dominated by just a few countries (Gillson and Busch, 2015)—bringing geopolitical dependence and associated uncertainty. Indeed, there are potential food security and resilience risks for many importing regions if maize availability in the USA for example would decrease due to factors such as diversion to non-food uses, climatic factors, or plant diseases (Wu and Guclu, 2013; Seekell et al., 2018).
Food Access
Access to food is another key food security dimension and is largely influenced by economic factors such as individuals having sufficient resources or entitlements to obtain food. Food prices and per capita incomes mainly determine the economic access of people to food. The poor are particularly at risk as they spend a large share of their household income on food (von Braun, 2008)—particularly the urban poor and rural food net buyers often spend 50–90% of their income on food. Even rural net sellers who first benefit from increased food prices might be negatively affected if food inflation pushes up overall inflation (Ruel et al., 2010; von Braun and Tadesse, 2012).
Changing demand- and supply-side factors impact on cereal prices. Nelson et al. (2009) forecast that maize prices will rise by 34% up to 100% between 2010 and 2050. Increasing prices of maize grain make it less affordable for poor consumers in several regions of the world (Shiferaw et al., 2011). Changing diets toward consumption of more livestock products add demand of maize for livestock production. Rosegrant (2008) estimates that the increased biofuel demand during the period 2000–07 accounted for 30% of the increase in weighted average grain prices; the biggest impact of close to 40% was found for maize prices and 22% for wheat prices. Higher prices for maize have also affected the supply and demand of other crops. On the demand side, consumers shifted from maize to rice and wheat consumption. On the supply side, maize production became more profitable, so that farmers shifted from rice, wheat or other crops to the production of maize. Overall, these demand- and supply-side effects have tended to increase the price of rice and wheat and other crops.
Trade restrictions also impact prices since they decrease the availability of staple crops. The poor lose since they have to pay higher prices to secure their food. If governments then try to keep domestic prices lower than world prices, this can additionally hurt the rural poor since low food prices result in less production so that less casual and seasonal labor will be required in rural areas (Economist, 2012).
Future food price trends and short-term variability in food prices are also expected to be influenced by weather variability and climate change via changes in food supply and food demand (Wheeler and von Braun, 2013). Various agro-ecologic and economic models have been used to examine the effects. Fischer et al. (2002) and Schmidhuber and Tubiello (2007) find that SSA is very likely to be hit hardest by climate change in terms of the economic output of agriculture. Thus, the poorest and already most food-insecure regions are expected to suffer the largest contraction of agricultural incomes. However, welfare improvements may derive from the improved distribution of benefits among different stakeholders along both input and output value chains, including processors, traders, and consumers (Dixon et al., 2007; Erenstein et al., 2012).
Food Utilization
Utilization is another key food security dimension and is reflected by the quality and diversity of diets. Many poor people across the developing world still face challenges in terms of securing caloric intake and/or securing a diverse quality diet. In countries such as Lesotho, Malawi, Zambia, or Zimbabwe, people cover large shares of total calories only with maize. Micronutrient malnutrition thereby may persist, even while overall caloric consumption may have increased, and dietary diversity decreased or stayed stable (Gómez et al., 2011; Pingali, 2015). Urbanization, dietary changes, and the nutritional transition also have major implications for food utilization. Excess caloric intake, poor-quality diets, and low physical activity have resulted in obesity and chronic diseases, even among the poor, in developing countries (Wheeler and von Braun, 2013). Whole grain consumption is an important source of dietary fiber, and typically at lower than recommended levels. Much of the challenge thereby is the form in which foods are processed and consumed, rather than the original staples per se (Poole et al., 2020).
There are two pathways through which climate change affects food utilization: diet and health. The former refers to the nutritional content while the latter refers to food safety, including diseases and infections affecting health. For the first pathway, climate change is likely to alter the types of crops that are grown and decrease the nutrient content of the crops (Lobell and Burke, 2010). Poor households have been found to substitute high-value food for low nutrient food and to reduce the diversity of their diets (Aberman and Cohen, 2012). Taub (2010) shows that elevated CO2 levels cause a decrease in protein concentrations of wheat and rice by 5–14%. However, it is also possible that due to climate change, the diversity and choice of food will shift again to more drought-tolerant sorghum or millet. The reverse had been observed in the past (Santpoort, 2020) driven by consumer preferences and markets. Regarding health effects, various forms of diseases such as malaria are likely to spread or recede with climate variability and change. Changing climatic conditions can initiate a vicious circle where infectious diseases cause hunger, which, in turn, make the affected populations more susceptible to infectious diseases. The result can be a substantial decline in labor productivity and an increase in poverty and even mortality. Extreme weather events such as floods can also lead to outbreaks of, for example, water-borne diseases in places where basic public infrastructure such as sanitation and hygiene is lacking. As a consequence, they lower the capacity of exposed people to effectively use food (Schmidhuber and Tubiello, 2007).
Agricultural policies have contributed in some countries to increased food production, but not necessarily to more nutritious diets and better health. A case in point is India's minimum support prices which have helped shape the rice-wheat systems and secured their availability, but reduced incentives to diversify and grow other crops (Erenstein, 2010b). The promotion of staple crop production can displace traditional food crops with important micronutrients which have had a negative impact on nutrition (Webb, 2009). Pingali and Roger (1995) report about the displacement of indigenous leafy vegetables and fish due to rice monoculture cultivation in the Philippines. A decline in vegetable crops and even in the nutritional content of cereals has been associated with the massive use of mineral fertilizer to increase cereal yields (Fan et al., 2008). Negative environmental impacts can arise from intensive agriculture, including an increased incidence of waterborne and water-related diseases (Pingali, 2007).
Food Stability
Stability is the final key food security dimension which ensures that food can be accessed by individuals at all times. It is very much driven by economic, environmental and political factors.
Rapid increases in food prices during the food price crisis greatly affected the livelihoods of millions of people (FAO, 2009), driving an estimated 110 million people into poverty and an additional 44 million into undernourishment (Nellemann et al., 2009). Ivanic et al. (2011) find from their simulations that the food price hike in 2011 pushed 68 million people into poverty while pulling 24 million out of poverty, leading to a net increase of 44 million poor. Following three decades of declining world food prices, the world food crisis set off a series of price spikes in the subsequent years (Gillson and Busch, 2015). And the trade policy responses in the aftermath of the food crisis often aggravated the global situation (Battat and Lampietti, 2015). In addition, most staple cereal production is consumed domestically and not traded (Gillson and Busch, 2015). If farmers cannot sell the extra produce on the market, then they also may not have the incentives to use more costly inputs (fertilizers and seeds) thereby adjusting their production to their own household needs.
Cereal stability can be impaired by abiotic (e.g., weather) and biotic shocks (e.g., diseases, pests). Since staples such as wheat and maize are planted on large areas, concerns exist that pests, diseases, and climate change may pose risks of catastrophic yield losses (Hazell, 2009). Agriculture nowadays indeed depends heavily on only a few crops, so a single plant disease has the potential to cause a food crisis (FAO, 2019a). In fact, most of the produced wheat is susceptible to a highly virulent strain of wind-born stem rust (Pardey et al., 2013). It already spread across eastern and southern Africa and the Middle East, and worryingly, rust continues to recombine and produce new virulent strains and can be carried long distances through the atmosphere (Meyer et al., 2017). Yellow rust epidemics have caused yield losses of up to 40% in Azerbaijan, Ethiopia, Iraq, Morocco, Syria, Tajikistan, and Uzbekistan (Cornell Chronicle, 2012). In 2017, it emerged in Argentina affecting 3 million ha and causing huge damages (Carmona et al., 2019). In 2016, the presence of the devastating wheat blast disease was confirmed in Bangladesh posing a new threat for South Asia's food security (Mottaleb et al., 2018b). In the case of maize, biotic stresses can reduce yields by more than 30%. While some diseases and pests are of global importance, others are more regional. Their occurrence has been associated with climate change, but also changing cropping practices or susceptible cultivars. Particularly challenging are the recent instances of new virulent pest and diseases cropping up in previously unaffected areas—cases in point being the emergence of Maize Lethal Necrosis (MLN) in eastern Africa (Mahuku et al., 2015) and Fall Army Worm across Africa (Economist, 2018) and Asia. Worrying too is that biotic stresses are set to increase under climate change (Deutsch et al., 2018).
The stability of food supplies is also increasingly impacted by weather variability through changes in food supply, food price, and policy. These fluctuations in crop yields and local food production can adversely affect the stability of food supplies and thus food security (Schmidhuber and Tubiello, 2007). The effects have been more severe in some important agricultural regions such as the Midwest of the USA, the Northeast of Argentina, Southern Africa, or Southeast Australia (Fischer et al., 2002) and these areas are likely to expand. Again, SSA and parts of South Asia—the poorest regions with the highest levels of chronic undernourishment—are most exposed to the highest degree of instability in food production (IFPRI et al., 2020).
Stocks are often kept to ensure the stability of food supplies. It is estimated that in 2015 global cereal stocks amount to around 627 million t—the highest level in 15 years. This is equal to about 57% of worldwide food consumption requirements, but with an unequal global distribution, including increased stockpiling in China. India also has high levels of cereal reserves, and it increased them further after the food price crises. Also, Zambia, and Malawi piled up their stocks as a response to the price increases to avoid market price distortions. But stockholding costs and losses are often immense (FAO, 2014; Pinstrup-Andersen, 2015).
Research Needs
In a dynamic world, diverse trends (demographic, cultural, ecological, technological, economic, and political) proceed to transform wheat and maize value chains and call for continued research and development efforts to enhance current and future global food security. In the case of wheat and maize, this implies that continued research investments are needed into developing and facilitating the uptake of more productive, stress-tolerant, and nutritious wheat and maize varieties affordable to the poor. However, diversified farming methods and good farming practices can already result in more sustainable production and enhance productivity and production (WBGU, 2020). At the same time, continued research efforts are needed to develop and facilitate the uptake of sustainable intensification to increase wheat and maize productivity (land, water, energy) and reducing the environmental footprint in the face of climate change, increasing water scarcity and land degradation. This also calls for new methodologies and modeling approaches (e.g., Kubitza et al., 2020).
There are three strands in the literature pointing out different research needs and recommendations for the future. These research needs which differ regionally can also be categorized according to the four dimensions of food security.
The first strand stresses the production side and the need to increase the availability of cereals, especially in SSA and parts of Asia. Hazell (2009) for example acknowledges the success of the Green Revolution, but he points at the continuing need to increase yields to meet growing food needs, especially in Asia and SSA. Crop breeding toward high yielding, stress-tolerant and widely-adapted varieties to overcome biotic and abiotic stresses do play a key role in meeting future maize and wheat demand. But it depends on the development of institutional innovations to enhance farmers' access to information, seeds, other inputs, finance but also output markets. In the long run, large public and private sector investment and sustained political commitment and policy support are needed (Shiferaw et al., 2011, 2013). Other researchers also alert that climate resilience needs much more attention by breeders, seed and grain traders and farmers (Kahiluoto et al., 2019). Climate policy, sustainable intensification, conservation agriculture and mixed cropping systems are needed to sustain output without compromising the environment and its services. The application of ICT-based approaches such as precision farming techniques or mobile phones, may also increase sustainability of wheat and maize production but more research is needed to explore its full potential (WBGU, 2019).
The second strand of literature highlights the need to focus on the consumption side and thus is more concerned with food utilization. Hefferon (2015) suggests further research on biofortification of wheat and maize to enhance the micronutrients and hence improve the nutritional status of consumers especially in developing countries. Similarly, McDermott et al. (2015) call for refocusing agricultural research from the current emphasis on supply-driven production and productivity goals toward consumption and improvement of diet quality incorporating the role of maize and wheat. In order to achieve improvements in nutrition outcomes, they suggest researchers to engage in new partnerships from various disciplines including agriculture, food science, social sciences to business and delivery science (McDermott et al., 2015). Pingali (2015) also highlights the need to diversify diets of the middle class and poor as a way to tackle malnutrition and obesity challenges at the same time. For the middle class this is of importance due to health issues concerning overweight and obesity, and for the poor in order to avoid malnutrition by improving access to protein, micronutrients, and vitamins. Hefferon (2015) suggests increased research and development of traditional crops such as sorghum, millet, and pigeon pea, whereas Krause et al. (2019) suggest to increase the consumption of indigenous vegetables, e.g., in SSA. On the consumption side, the move away from livestock products is most essential to combat climate change and preserve the environment as it eases the growing competition for land and reduces greenhouse gas emissions (Willett et al., 2019).
The third strand suggests more research on the food system transformation and their associated value chains in order to improve food security in its four dimensions in developing countries. This is of particular importance in the context of shocks such as the COVID-19 pandemic to ensure that food systems become more resilient (Seekell et al., 2018). Godfray et al. (2010b) depart from the idea of maximizing productivity and rather suggest optimization across the food and marketing system. They stress selected aspects such as reducing waste or changing diets and eventually call for a global strategy to ensure sustainable and equitable food security. Gómez et al. (2010) suggest to improve the performance of food value chains in order to serve the poor. They stress the importance of market efficiency, the extent of post-harvest losses and the role of on-farm conservation and also suggest developing an integrative, multidimensional framework to study evolving food value chains in developing countries. Finally, the connection between food security and food system sustainability has been emphasized at the global level (Capone et al., 2014). Wheeler and von Braun (2013) promote investments in adaptation and mitigation actions toward a “climate-smart food system” that is more resilient to climate change influences on food security. Furthermore, since climate change is expected to further increase variability of global wheat and maize supply, as underlined by the IPCC (2019), there is the need to develop strategies, in both research and policy, to deal with this variability and the constant growth in demand.
Conclusion
Looking at the wheat and maize value chains and African and Asian food security, we find important differences. While production and demand for wheat and maize will continue to increase due to population growth and increasing per capita incomes, the growth rates will differ regionally:
• Africa expects the highest population growth and already has the largest food insecurity problems in the world. At the same time, it has the lowest maize and wheat yields per ha, and is likely to be the region most negatively impaired by climate change. While there are many places with good growing conditions already producing food surpluses, many regions in Africa nevertheless depend on cereal imports, especially wheat.
• Asia faces challenges in meeting the growing demand for food, water, and energy for a growing population. It benefitted from the Green revolution but is now struggling with nutritional deficiencies. Although rice is the central staple in many Asian countries, Asia is also a major producer of wheat with medium (South Asia) to high yields (East Asia). Maize is increasingly produced, but largely for livestock production. Food insecurity remains a problem, especially in South Asia and in relation to food access. But also overweight and obesity can be observed (esp. India and China) which is mainly an issue of utilization. Food security policies have been found to result in unsustainable agriculture.
The decline of natural resources (soils, water, land, biodiversity, and many other ecosystem services) will continue in Africa if not more nutrients are provided to the soils. In contrast, in many parts of Asia, the intensity of production has to be reduced by using less fertilizers. Farming has to become more sustainable and more emphasis should be put on increasing the sustainability of production by diversifying farming. For Africa, this may additionally mean sustainable intensification paired with the expansion of land which is still relatively available. Only this way, food self-sufficiency can be increased in the longer run so that net food importing countries may become less dependent on imports (WBGU, 2020).
Apart from the regional perspective, there are also product-specific differences which need to be highlighted. For wheat the per capita global human consumption levels have been relatively stable over time and space. This is also true for maize with respect to human consumption, especially in Africa; however, in Asia, maize has an important feed dimension (esp. East Asia) which has substantively increased the demand for maize. In the future, maize production is therefore expected to grow faster as compared to wheat.
The research agenda, in general, so far focused largely on the availability and stability dimensions of staple cereal food security. This includes improving the productivity and sustainability of wheat and maize farming and ability to cope with biotic and abiotic stresses, which are set to worsen with climate change and thereby merit continued and increased investment. To add to the access and utilization dimensions of food security, more has to be done to promote healthier diets and further the equitable transformation of the cereal value chains. Biofortification alone will not solve the problem of unbalanced diets especially since consumers' awareness and willingness to pay bears much insecurity. Echoing the Royal Society (2009), due to the scale of the challenge, no option should be ruled out, and different approaches and strategies may be needed in different regions and circumstances. Indeed, the scale of the food security challenge requires additional investments across complementary research areas, and increased attention for one should thereby not be in lieu of the other (Poole et al., 2020). Staple cereals such as wheat and maize will continue to play a critical role to ensure an adequate and affordable intake of calories and proteins in diets in Africa and Asia, but clearly are not a panacea and diverse diets call for complementary investments across food groups (including fruits and vegetables) and food system transformation.
Author Contributions
UG, AF, and TN wrote the first draft of the manuscript. OE critically revised the text and made substantial contributions to the manuscript. All authors approved the final version of the manuscript.
Funding
The authors acknowledge financial support by the CGIAR Research Programs on Maize (CRP MAIZE) and Wheat (CRP WHEAT).
Disclaimer
The contents and opinions expressed herein are those of the authors and do not necessarily reflect the views of the associated and/or supporting institutions.
Conflict of Interest
The authors declare that the research was conducted in the absence of any commercial or financial relationships that could be construed as a potential conflict of interest.
References
Aberman, N. L., and Cohen, M. J. (2012). “Nutrition and bioenergy,” in The Impact of Climate Change and Bioenergy on Nutrition, eds B. Thomson and M. J. Cohen (Dordrecht: Springer). Available online at: https://www.springer.com/gp/book/9789400701090
Ahmed, G., Hamrick, D., and Gereffi, G. (2014). Shifting Governance Structures in the Wheat Value Chain: Implications for Food Security in the Middle East and North Africa. Duke University, Center on Globalization, Governance and Competitiveness (Duke CGGC). Available online at: http://www.cggc.duke.edu/pdfs/2013-08-28_CGGC_Report_Wheat_GVC_and_food_security_in_MENA.pdf
Alauddin, M., and Quiggin, J. (2008). Agricultural intensification, irrigation and the environment in South Asia: issues and policy options. Ecol. Econ. 65, 111–124. doi: 10.1016/j.ecolecon.2007.06.004
Altieri, M. A. (2009). Agroecology, Small Farms, and Food Sovereignty, 61 Monthly Review. Available online at: https://monthlyreview.org/2009/07/01/agroecology-small-farms-and-food-sovereignty/
Asian Development Bank (ADB) (2000). Rural Asia: Beyond the Green Revolution. Manila: Asian Development Bank.
Asseng, S., Cammarano, D., Basso, B., Chung, U., Alderman, P. D., Sonder, K., et al. (2017). Hot spots of wheat yield decline with rising temperatures. Global Change Biol. 23, 2464–2472. doi: 10.1111/gcb.13530
Bakker, M. M., Govers, G., and Rounsevell, M. D. A. (2004). The crop productivity-erosion relationship: an analysis based on experimental work. Catena 57, 55–76. doi: 10.1016/j.catena.2003.07.002
Battat, M., and Lampietti, J. (2015). “The grain chain: trade and food security in Arab countries,” in Trade Policy and Food Security: Improving Access to Food in Developing Countries in the Wake of High World Prices, eds I. Gillson and A. Fouad (Washington, DC: The World Bank Group), 189–214.
Baudron, F., Jaleta, M., Okitoi, O., and Tegegn, A. (2014). Conservation agriculture in African mixed crop-livestock systems: expanding the niche. Agric. Ecosyst. Environ. 187, 171–182. doi: 10.1016/j.agee.2013.08.020
Béné, C., Fanzo, J., Prager, S. D., Achicanoy, H. A., Mapes, B. R., Alvarez Toro, P., et al. (2020). Global drivers of food system (un)sustainability: a multi-country correlation analysis. PLoS ONE 15:e0231071. doi: 10.1371/journal.pone.0231071
Béné, C., Prager, S. D., Achicanoy, H. A. E., Alvarez Toro, P., Lamotte, L., Bonilla Cedrez, C., et al. (2019). Understanding food systems drivers: a critical review of the literature. Glob. Food Sec. 23, 149–159. doi: 10.1016/j.gfs.2019.04.009
Bindraban, P. S., van der Velde, M., Ye, L., van den Berg, M., Materechera, S., Kiba, D. I., et al. (2012). Assessing the impact of soil degradation on food production. Curr. Opin. Environ. Sustain. 4, 478–488. doi: 10.1016/j.cosust.2012.09.015
Borenstein, M., Hedges, L. V., Higgins, J. P. T., and Rothstein, H. R. (2009). Introduction Meta-Analysis. Chichester: Wiley.
Bruinsma, J. (2009). The resource outlook to 2050: by how much do land, water use and crop yields need to increase by 2050? Paper presented at the Export Meeting on How to Feed the World in 2050 (Rome: FAO). Available online at: http://www.fao.org/3/a-ak971e.pdf
Byerlee, D., and Heisey, P. W. (1997). “Evolution of the African maize economy,” in Africa's Emerging Maize Revolution, Maize Revolutions in Sub-Saharan Africa, eds D. Byerlee and C. K. Eicher (Boulder, CO: Lynne Rienner Publishers). Available online at: https://www.researchgate.net/publication/228275899_Maize_Revolutions_in_Sub-Saharan_Africa (accessed December 06, 2020).
Cairns, J. E., and Prasanna, B. M. (2018). Developing and deploying climate-resilient maize varieties in the developing world. Curr. Opin. Plant Biol. 45, 226–230. doi: 10.1016/j.pbi.2018.05.004
Capone, R., El Bilali, H., Debs, P., Cardone, G., and Driouech, N. (2014). Food system sustainability and food security: connecting the dots. J. Food Security 2, 13–22. doi: 10.12691/jfs-2-1-2
Carmona, M. A., Sautua, F. J., Pérez-Hernández, O., Grosso, C., Vettorello, L., Milanesio, B., et al. (2019). Rapid emergency response to yellow rust epidemics caused by newly introduced lineages of Puccinia striiformis f. sp. tritici in Argentina. Trop. Plant Pathol. 44, 385–391. doi: 10.1007/s40858-019-00295-y
Challinor, A. J., Watson, J., Lobell, D. B., Howden, S. M., Smith, D. R., and Chhetri, N. (2014). A meta-analysis of crop yield under climate change and adaptation. Nat. Clim. Change 4, 287–291. doi: 10.1038/nclimate2153
Chartres, C. J., and Noble, A. (2015). Sustainable intensification: overcoming land and water constraints on food production. Food Security 7, 235–245. doi: 10.1007/s12571-015-0425-1
Conway, G. (2012). One Billion Hungry: Can We Feed the World? Ithaca; London: Cornell University Press.
Cornell Chronicle (2012). Rust-Tracker' to Monitor Deadly Wind-Borne Wheat Fungus. Available online at: http://www.news.cornell.edu/stories/2012/09/rust-tracker-launched-identify-wheat-risk (accessed December 06, 2020).
Courtois, P., and Subervie, J. (2015). Farmer bargaining power and market information systems. Am. J. Agr. Econ. 97, 953–977. doi: 10.1093/ajae/aau051
Curtis, T., and Halford, N. G. (2014). Food security: the challenge of increasing wheat yield and the importance of not compromising food safety. Ann. Appl. Biol. 164, 354–372. doi: 10.1111/aab.12108
De Groote, H., Gunaratna, N. S., Okuro, J. O., Wondimu, A., Chege, C. K., and Tomlins, K. (2014). Consumer acceptance of quality protein maize (QPM) in East Africa. J. Sci. Food Agric. 94, 3201–3212. doi: 10.1002/jsfa.6672
De Groote, H., and Kimenju, S. C. (2008). Comparing consumer preferences for color and nutritional quality in maize: application of a semi-double-bound logistic model on urban consumers in Kenya. Food Policy 33, 362–370. doi: 10.1016/j.foodpol.2008.02.005
De Groote, H., and Kimenju, S. C. (2012). Consumer preferences for maize products in urban Kenya. Food Nutr. Bull. 33, 99–110. doi: 10.1177/156482651203300203
Delgado, C. J. (2003). Rising consumption of meat and milk in developing countries has created a new food revolution. J. Nutr. 133, 3907–3910. doi: 10.1093/jn/133.11.3907S
Demeke, M., Pangrazio, G., and Maetz, M. (2008). Country Responses to the Food Security Crisis: Nature and Preliminary Implications of the Policies Pursued. Rome: FAO. Available online at: http://www.fao.org/3/a-au717e.pdf (accessed December 06, 2020).
Denyer, D., and Tranfield, D. (2011). “Producing a systematic review”, in The SAGE Handbook of Organizational Research Methods, eds D. Buchanan and A. Bryman (Los Angeles; London; New Delhi: SAGE), 671–689.
Deutsch, C. A., Tewksbury, J. J., Tigchelaar, M., Battisti, D. S., Merrill, S. C., Huey, R. B., et al. (2018). Increase in crop losses to insect pests in a warming climate. Science 361, 916–919. doi: 10.1126/science.aat3466
Dixon, J., Braun, H. J., Kosina, P., and Crouch, J., (eds.). (2009). Wheat Facts and Futures 2009. Mexico, D.F.: CIMMYT. Available online at: https://repository.cimmyt.org/handle/10883/1265 (accessed December 06, 2020).
Dixon, J., Hellin, J., Erenstein, O., and Kosina, P. (2007). U-impact pathway for diagnosis and impact assessment of crop improvement. J. Agric. Sci. 145, 195–206. doi: 10.1017/S0021859607007046
Economist (2012). Corn Laws, Revisited. Available online at: https://www.economist.com/feast-and-famine/2012/09/14/corn-laws-revisited (accessed December 06, 2020).
Economist (2015). Planet of the Phones: The Smartphone is Ubiquitous, Addictive and Transformative. Available online at: https://www.economist.com/leaders/2015/02/26/planet-of-the-phones (accessed December 06, 2020).
Economist (2017). A Circular Tale of Changing Food Preferences: Of Rice and Men. Available online at: https://www.economist.com/international/2017/03/11/a-circular-tale-of-changing-food-preferences (accessed December 06, 2020).
Economist (2018). Hungry Caterpillars and Hungry People - An Army of Worms is Invading Africa: Stopping the Spread of Spodoptera frugiperda. Available online at: https://www.economist.com/middle-east-and-africa/2018/01/18/an-army-of-worms-is-invading-africa (accessed December 06, 2020).
Egziabher, T. B. G. (2003). The use of genetically modified crops in agriculture and food production, and their impacts on the environment – a developing world perspective. Acta Agric. Scand. Sect. B Soil Plant Sci. 53(sup1), 9–13.
Ekekwe, N. (2017). How Digital Technology is Changing Farming in Africa. Watertown, MA: Harvard Businesss Review. Available online at: https://hbr.org/2017/05/how-digital-technology-is-changing-farming-in-africa (accessed December 06, 2020).
Erenstein, O. (2010a). A comparative analysis of rice-wheat systems in Indian Haryana and Pakistan Punjab. Land Use Policy 27, 869–879. doi: 10.1016/j.landusepol.2009.12.001
Erenstein, O. (2010b). The evolving maize sector in Asia: challenges and opportunities. J. New Seeds 11, 1–15. doi: 10.1080/15228860903517770
Erenstein, O., Sayre, K., Wall, P., Hellin, J., and Dixon, J. (2012). Conservation agriculture in maize- and wheat-based systems in the (Sub)tropics: lessons from adaptation initiatives in South Asia, Mexico, and Southern Africa. J. Sustain. Agric. 36, 180–206. doi: 10.1080/10440046.2011.620230
Evenson, R. E., and Gollin, D. (2003). Assessing the impact of the green revolution, 1960 – 2000. Science 300, 758–762. doi: 10.1126/science.1078710
Evenson, R. E., Pray, C. E., and Rosegrant, M. W. (1999). Agricultural Research and Productivity Growth in India. IFPRI Research Report 109. International Food Policy Research Institute, Washington, DC. Available online at: https://www.ifpri.org/publication/agricultural-research-and-productivity-growth-india (accessed December 06, 2020).
Falkenmark, M. (2007). Shift in thinking to address the 21st century hunger gap: moving focus from blue to green water management. Water Resour. Manage. 21, 3–18. doi: 10.1007/s11269-006-9037-z
Fan, M. S., Zhao, F. J., and Fairweather-Tait, S. J. (2008). Evidence of decreasing mineral density in wheat grain over the last 160 years. J. Trace Elements Med. Biol. 22, 315–324. doi: 10.1016/j.jtemb.2008.07.002
FAO (1996). Declaration on World Food Security. World Food Summit. Rome: FAO. Available online at: http://www.fao.org/3/w3613e/w3613e00.htm
FAO (2006). Food Security. Policy Brief. Rome: Food and Agriculture Organization. Available online at: https://reliefweb.int/report/world/policy-brief-food-security-issue-2-june-2006
FAO (2009). How to Feed the World in 2050. High-Level Experts Forum. Rome: FAO. Available online at: https://www.jstor.org/stable/25593700
FAO (2011). The State of the World's Land and Water Resources for Food and Agriculture (SOLAW) —Managing Systems at Risk. Earthscan; FAO: Rome; London. Available online at: http://www.fao.org/3/a-i1688e.pdf
FAO (2013). SAVE FOOD: Global Initiative on Food Losses and Waste Reduction. Key Findings. Available online at: http://www.fao.org/save-food/key-findings/en (accessed December 06, 2020).
FAO (2016). Save and Grow in Practice: Maize, Rice, Wheat. A Guide to Sustainable Cereal Production. Rome: FAO. Available online at: http://www.fao.org/3/a-i4009e.pdf
FAO (2017). The Future of Food and Agriculture: Trends and Challenges. Rome: FAO. Available online at: http://www.fao.org/3/a-i6583e.pdf (accessed December 06, 2020).
FAO (2019a). The State of the World's Biodiversity for Food and Agriculture. Rome: FAO. Available online at: http://www.fao.org/3/CA3129EN/ca3129en.pdf (accessed December 06, 2020).
FAO (2019b). Reducing Post-Harvest Losses. FAO Partnerships Working for the Sustainable Development Goals. Rome: FAO. Available online at: http://www.fao.org/3/ca6344en/ca6344en.pdf (accessed December 06, 2020).
FAO, IFAD, UNICEF, WFP, and WHO. (2019). The State of Food Security and Nutrition in the World 2019. Safeguarding Against Economic Slowdowns and Downturns. Rome: FAO. Available online at: http://www.fao.org/3/ca5162en/ca5162en.pdf
Fischer, G., Shah, M., and van Velthuizen, H. (2002). Climate Change and Agricultural Vulnerability: A Special Report Prepared as a Contribution to the World Summit on Sustainable Development (International Institute for Applied Systems Analysis, Austria). Available online at: https://www.accc.gv.at/pdf/JB-Report.pdf
Frelat, R., Lopez-Ridaura, S., Giller, K. E., Herrero, M., Douxchamps, S., Djurfeldt, A. A., et al. (2016). Drivers of household food availability in sub-Saharan Africa based on big data from small farms. Proc. Natl. Acad. Sci. U.S.A. 113, 458–463. doi: 10.1073/pnas.1518384112
FSIN (2020a). 2020 Global Report on Food Crises. Food Security Information Network (FSIN). Available online at: https://reliefweb.int/sites/reliefweb.int/files/resources/WFP-0000114546.pdf
FSIN (2020b). Regional Focus on the Intergovernmental Authority on Development (IGAD) Member States. Rome: Food Security Information Network.
Giller, K. E., Andersson, J. A., Corbeels, M., Kirkegaard, J., Mortensen, D., Erenstein, O., et al. (2015). Beyond conservation agriculture. Front. Plant Sci. 6:870. doi: 10.3389/fpls.2015.00870
Gillson, I., and Busch, C. (2015). “Trade policy responses to high and volatile food prices,” in Trade Policy and Food Security: Improving Access to Food in Developing Countries in the Wake of High World Prices, eds I. Gillson and A. Fouad (Washington, DC: World Bank Publications), 87–118.
Gillson, I., and Fouad, A. (2015). Trade Policy and Food Security: Improving Access to Food in Developing Countries in the Wake of High World Prices. Washington, DC: World Bank Publications. Available online at: http://hdl.handle.net/10986/20537
Girodano, M., and de Fraiture, C. (2014). Small private irrigation: Enhancing benefits and managing trade-offs. Agric. Water Manag. 131, 175–182. doi: 10.1016/j.agwat.2013.07.003
Godfray, H. C., Beddington, J. R., Crute, I. R., Haddad, L., Lawrence, D., Muir, J. F., et al. (2010b). Food security: The challenge of feeding 9 billion people. Science 327, 812–818. doi: 10.1126/science.1185383
Godfray, H. C., Crute, I. R., Haddad, L., Lawrence, D., Muir, J. F., Nisbett, N., et al. (2010a). The future of the global food system. Philos. Trans. R. Soc. Lond. B Biol. Sci. 365, 2769–2777. doi: 10.1098/rstb.2010.0180
Gómez, M. I., Barrett, C. B., Buck, L. E., De Groote, H., Ferris, S., Gao, H. O., et al. (2011). Research principles for developing country food value chains. Science 332, 1154–1155. doi: 10.1126/science.1202543
Gómez, M. I., Barrett, C. B., Buck, L. E., De Groote, H., Ferris, S., Gao, O., et al. (2010). Principles for developing country food value chains. Science 332, 1154–1155.
Grote, U. (2014) Can we improve global food security? A socio-economic and political perspective. Food Sec. 6, 187–200. doi: 10.1007/s12571-013-0321-5.
Gulati, A., and Dixon, J. (2008). Maize in Asia: Changing Markets and Incentives. New Delhi: Academic Foundation.
Gustavsson, J., Cederberg, C., Sonesson, U., van Otterdijk, R., and Meybeck, A. (2011). Global Food Losses and Food Waste: Extent, Causes and Prevention. Rome: FAO.
Hanjra, M. A., and Qureshi, M. E. (2010). Global water crisis and future food security in an era of climate change. Food Policy 35, 365–377. doi: 10.1016/j.foodpol.2010.05.006
Hazell, P. B. R. (2009). The Asian green revolution. IFPRI Discussion Paper 00911 (Washington, DC: International Food Policy Research Institute).
Hefferon, K. L. (2015). Nutritionally enhanced food crops; progress and perspectives. Int. J. Mol. Sci. 16, 3895–3914. doi: 10.3390/ijms16023895
Hellin, J., Krishna, V. V., Erenstein, O., and Boeber, C. (2015). India's poultry revolution: the rapid growth of the poultry industry and implications for its sustenance and the global poultry trade. Int. Food Agribusiness Manag. Rev. 18, 151–164. doi: 10.22004/ag.econ.207008
Hellin, J., Shiferaw, B., Cairns, J.E., Reynolds, M., Ortiz-Monasterio, I., Banziger, M., et al. (2012). Climate change and food security in the developing world: Potential of maize and wheat research to expand options for adaptation and mitigation. J. Dev. Agric. Econ. 4, 311–321. doi: 10.5897/JDAE11.112
Hubert, B., Rosegrant, M. W., van Boekel, M. A. J. S., and Ortiz, R. (2010). The future of food: scenarios for 2050. Crop Sci. 50, 33–50. doi: 10.2135/cropsci2009.09.0530
IDRC (2010). Facts and Figures on Food and Biodiversity. Canada: IDRC Communications, International Development Research Centre. Availble online at: https://www.idrc.ca/en/research-in-action/facts-figures-food-and-biodiversity
IFPRI, Concern Worldwide, and Welthungerhilfe (2020). 2020 Global Hunger Index. Bonn, Washington, DC, Dublin. Available online at: https://www.globalhungerindex.org/results.html
IPCC (2019). Climate Change and Land: An IPCC Special Report on Climate Change, Desertification, Land Degradation, Sustainable Land Management, Food Security, and Greenhouse Gas Fluxes in Terrestrial Ecosystems. IPCC, Geneva. Available online at: https://www.ipcc.ch/report/srccl/
IRIN (2008). Cheer and Concern Over Ban on Private Sale of Maize. IRIN. Available online at: http://www.irinnews.org/Report.aspx?ReportId=80052
Ivanic, M., Martin, W., and Zaman, H. (2011). Estimating the short-run poverty impacts of the 2010-11 surge in food prices. Policy Research Working Paper 5633. Washington, DC: World Bank, Development Research Group. Available online at: https://openknowledge.worldbank.org/bitstream/handle/10986/3399/WPS5633.pdf?sequence=1
Kahiluoto, H., Kaseva, J., Balek, J., Olesen, J. E., Ruiz-Ramos, M., Gobin, A., et al. (2019). Decline in climate resilience of European wheat. PNAS 116, 123–128. doi: 10.1073/pnas.1804387115
Kassam, A., and Brammer, H. (2013). Combining sustainable agricultural production with economic and environmental benefits. Geogr. J. 179, 11–18. doi: 10.1111/j.1475-4959.2012.00465.x
Keyser, J. C. (2015). “Regional trade of food staples and crop inputs in Africa,” in Trade Policy and Food Security: Improving Access to Food in Developing Countries in the Wake of High World Prices, eds I. Gillson and A. Fouad (Washington, DC: The World Bank Group), 153–188.
Krause, H., Faße, A., and Grote, U. (2019). Welfare and food security-effects of commercializing African indigenous vegetables in Kenya. Cogent. Food Agric. 5:1700031. doi: 10.1080/23311932.2019.1700031
Kubitza, C., Krishna, V. V., Schulthess, U., and Jain, M. (2020). Estimating adoption and impacts of agricultural management practices in developing countries using satellite data. A scoping review. Agron. Sustain. Dev. 40:16. doi: 10.1007/s13593-020-0610-2
Kumar, D., and Kalita, P. (2017). Reducing postharvest losses during storage of grain crops to strengthen food secuirty in developing countries. Foods 6:8. doi: 10.3390/foods6010008
Laxman, L., and Ansari, A. H. (2011). GMOs, safety concerns and international trade: developing countries' perspective. J. Int. Trade Law Policy 10, 281–307. doi: 10.1108/14770021111165535
Le Mouël, C., and Forslund, A. (2017). How can we feed the world in 2050? A review of the responses from global scenario studies. Eur. Rev. Agric. Econ. 44, 541–591. doi: 10.1093/erae/jbx006
Lobell, D., and Burke, M. (2010). Climate Change and Food Security: Adapting Agriculture to a Warmer World. Dordrecht; New York, NY: Springer. Available online at: https://www.springer.com/gp/book/9789048129515
Lucas, R. E., Holtman, J. B., and Connor, L. J. (1977). “Soil carbon dynamics and cropping practices,” in Agriculture and Energy, ed W. Lockeretz (New York, NY: Academic Press), 333–351.
Lumpkin, T. A. (2011). Help for developing countries in a world of rising grain prices. Acta Hortic 916, 31–46. doi: 10.17660/ActaHortic.2011.916.4
Lundqvist, J. (2010). “Producing more or wasting less. Bracing the food security challenge of unpredictable rainfall,” in Re-Thinking Water and Food Security, Fourth Marcelino Botín Foundation Water Workshop, eds L. Martínez-Cortina, G. Garrido, and L. López-Gunn (London: Taylor & Francis Group). Available online at: http://citeseerx.ist.psu.edu/viewdoc/download?doi=10.1.1.463.4927&rep=rep1&type=pdf
Mahuku, G., Lockhart, B. E., Wanjala, B., Jones, M. W., Kimunye, J. N., Stewart, L. R., et al. (2015). Maize Lethal necrosis (MLN), an emerging threat to maize-based food security in Sub-Saharan Africa. Phytopathology 105, 956–965. doi: 10.1094/PHYTO-12-14-0367-FI
Malik, R. K., Yadav, A., and Singh, S. (2005). “Resource conservation technologies in rice-wheat cropping systems Indo-Gangetic Plains,” in Conservation Agriculture: Status and Prospects, eds I. P. Abrol, R. K. Gupta, and R. K. Malik (New Delhi: Centre for Advancement of Sustainable Agriculture). Available online at: https://www.yumpu.com/en/document/read/26300889/conservation-agriculture
Mason, N. M., Jayne, T. S., and Shiferaw, B. (2015). Africa's rising demand for wheat: trends, drivers, and policy implications. Dev. Policy Rev. 33, 581–613. doi: 10.1111/dpr.12129
McCann, J. C. (2005). Maize and Grace: Africa's encounter with a New World Crop 1500-2000. Cambridge: Harvard University Press. Available online at: http://www.hup.harvard.edu/catalog/MCCMAI.html
McDermott, J., Johnson, N., Kadiyala, S., Kennedy, G., and Wyatt, A. (2015). Agricultural research for nutrition outcomes – re-thinking the agenda. Food Secur. 7, 593–607. doi: 10.1007/s12571-015-0462-9
Meyer, M., Burgin, L., Hort, M. C., Hodson, D. P., and Gilligan, C. A. (2017). Large-scale atmospheric dispersal simulations identify likely airborne incursion routes of wheat stem rust into Ethiopia. Phytopathology 107, 1175–1186. doi: 10.1094/PHYTO-01-17-0035-FI
Mottaleb, K. A., Rahut, D. B., Kruseman, G., and Erenstein, O. (2018a). Changing food consumption of households in developing countries: a Bangladesh case. J. Int. Food Agribus. Mark. 30, 156–174. doi: 10.1080/08974438.2017.1402727
Mottaleb, K. A., Singh, P. K., Sonder, K., Kruseman, G., Tiwari, T. P., Barma, N. C. D., et al. (2018b). Threat of wheat blast to South Asia's food security: an ex-ante analysis. PLoS ONE 13:e0197555. doi: 10.1371/journal.pone.0197555
Mottet, A., de Haan, C., Falcucci, A., Tempio, G., Opio, C., and Gerber, P. (2017). Livestock: on our plates or eating at our table? A new analysis of the feed/food debate. Glob. Food Sec. 14, 1–8. doi: 10.1016/j.gfs.2017.01.001
Mueller, N. D., Gerber, J. S., Johnston, M., Ray, D. K., Ramankutty, N., and Foley, J. A. (2012). Closing yield gaps through nutrient and water management. Nature 490, 254–257. doi: 10.1038/nature11420
Nellemann, C., MacDevette, M., Manders, T., Eickhout, B., Svihus, B., Prins, A. G., and Kaltenborn, B. P., (eds.). (2009). The Environmental Food Crisis – The Environment's Role in Averting Future Food Crises. A UNEP Rapid Response Assessment. Nairobi: United Nations Environment Programme (UNEP). Available online at: https://www.pbl.nl/en/publications/The_Environmental_Food_Crisis_the_environments_role_in_averting_future_food_crises
Nelson, G., Bogard, J., Lividini, K., Arsenault, J., Riley, M., Sulser, T. B., et al. (2018). Income growth and climate change effects on global nutrition security to mid-century. Nat. Sustain. 1, 773–781. doi: 10.1038/s41893-018-0192-z
Nelson, G. C., Rosegrant, M. W., Koo, J., Robertson, R., Sulser, T., Zhu, T., et al. (2009). Climate Change Impact on Agriculture and Costs of Adaptation. Washington, DC: International Food Policy Research Institute. Available online at: https://www.ifpri.org/cdmref/p15738coll2/id/130648/filename/130821.pdf
Nkonya, E., Gerber, N. J., von Braun, J., and De Pinto, A. (2011). Economics of Land Degradation: The Costs of Action Versus Inaction. IFPRI Brief 68. IFPRI, Washington, DC.
Nkonya, E., Mirzabaev, A., and von Braun, J., (eds.). (2016). “Economics of land degradation and improvement: an introduction and overview,” in Economics of Land Degradation and Improvement: A Global Assessment for Sustainable Development (Cham: Springer Nature Switzerland AG), 1–14. doi: 10.1007/978-3-319-19168-3
OECD (2020). OECD Policy Responses to Coronavirus (COVID-19) – COVID-19 and Africa: Socio-Economic Implications and Policy Responses. Paris: Organisation for Economic Co-operation and Development (OECD). Available online at: https://www.oecd.org/coronavirus/policy-responses/covid-19-and-africa-socio-economic-implications-and-policy-responses-96e1b282/
Oelofse, M., Markussen, B., Knudsen, L., Schelde, K., Olesen, J. E., Jensen, L. S., et al. (2015). Do soil organic carbon levels affect potential yields and nitrogen use efficiency? An analysis of winter wheat and spring barley field trials. Eur. J. Agron. 66, 62–73. doi: 10.1016/j.eja.2015.02.009
Ortiz, R., Sayre, K. D., Govaerts, B., Gupta, R., Subbarao, G. V., Ban, T., et al. (2008). Climate change: can wheat beat the heat? Agric. Ecosyst. Environ. 126, 46–58. doi: 10.1016/j.agee.2008.01.019
Pardey, P. G., Beddow, J. M., Kriticos, D. J., Hurley, T. M., Park, R. F., Duveiller, E., et al. (2013). Right-sizing stem-rust research. Science 340, 147–148. doi: 10.1126/science.122970
Peña-Bautista, R. J., Hernandez-Espinosa, N., Jones, J. M., Guzmán, C., and Braun, H. J. (2017). Wheat-based foods: their global and regional importance in the food supply, nutrition, and health. Cereal Foods World 62, 231–249. doi: 10.1094/CFW-62-5-0231
Pingali, P. (2007). Agricultural growth and economic development: a view through the globalization lens. Agric. Econ. 37, 1–12. doi: 10.1111/j.1574-0862.2007.00231.x
Pingali, P. (2012). Green revolution: impacts, limits, and the path ahead. PNAS 109, 12302–12308. doi: 10.1073/pnas.0912953109
Pingali, P. (2015). Agricultural policy and nutrition outcomes – getting beyond the preoccupation with staple grains. Food Secur. 7, 583–591. doi: 10.1007/s12571-015-0461-x
Pingali, P., and Roger, P. (1995). Impact of Pesticides on Farmer Health and the Rice Eco-System. Boston, MA: Kluwer Academic Publishers. Available online at: https://www.springer.com/gp/book/9780792395218
Pinstrup-Andersen, P., (ed.). (2015) Food Price Policy in an Era of Market Instability - A Political Economy Analysis. London: Oxford University Press. Available online at: https://global.oup.com/academic/product/food-price-policy-in-an-era-of-market-instability-9780198718574?cc=deandlang=enand.
Pittelkow, C. M., Linquist, B. A., Lundy, M. E., Liang, X., van Groeningen, K. J., Lee, J., et al. (2015). When does no-till yield more? A global meta-analysis. Field Crops Res. 183, 156–168. doi: 10.1016/j.fcr.2015.07.020
Poole, N., Donovan, J., and Erenstein, O. (2020). Agri-nutrition research: revisiting the contribution of maize and wheat to human nutrition and health. Food Policy 101976. doi: 10.1016/j.foodpol.2020.101976. [Epub ahead of print].
Popkin, B. M. (2010). Will China's nutrition transition overwhelm its health care system and slow economic growth? Health Aff. 27, 1064–1076. doi: 10.1377/hlthaff.27.4.1064
RaboResearch (2017). Global Wheat Consumption. Utrecht: Rabobank. Available online at: https://research.rabobank.com/far/en/sectors/grains-oilseeds/global_wheat_demand_article_1.html
Rasul, G. (2014). Food, water, and energy security in South Asia: a nexus perspective from the Hindu Kush Himalayan region. Environ. Sci. Policy 39, 35–48. doi: 10.1016/j.envsci.2014.01.010
Rasul, G. (2016). Managing the food, water, and energy nexus for achieving the Sustainable Development Goals in South Asia. Environ. Dev. 18, 14–25. doi: 10.1016/j.envdev.2015.12.001
Ray, D. K., Ramankutty, N., Mueller, N. D., West, P. C., and Foley, J. A. (2012). Recent patterns of crop yield growth and stagnation. Nat. Commun. 3:1293. doi: 10.1038/ncomms2296
Ritchie, H., and Roser, M. (2019). Urbanization. Available online at: https://ourworldindata.org/urbanization
Rodríguez, J. P., Rahman, H., Thushar, S., and Singh, R. K. (2020). Healthy and resilient cereals and pseudo-cereals for marginal agriculture: molecular advances for improving nutrient bioavailability. Front. Genet. 11:49. doi: 10.3389/fgene.2020.00049
Rosegrant, M. R., Ringler, C., Sulser, T. B., Ewing, M., Palazzo, A., and Zhu, T. (2009). Agriculture and Food Security Under Global Change: Prospects for 2025/2050. Washington, DC: IFPRI. Available online at: http://alliance.cgxchange.org/documentation-for-the-development-of-the-cgiar-strategy-and-mega-programs/SRF_IMPACT10-10-09c.pdf
Rosegrant, M. W. (2008). Biofuels and Grain Prices: Impacts and Policy Responses. Testimony for the U.S. Senate Committee on Homeland Security and Governmental Affairs. Washington, DC: International Food Policy Research Institute. Available online at: http://large.stanford.edu/courses/2011/ph240/chan1/docs/rosegrant20080507.pdf
Rost, S., Gerten, D., Bondeau, A., Lucht, W., Rohwer, J., and Schaphoff, S. (2008). Agricultural green and blue water consumption and its influence on the global water system. Water Resour. Res. 44:1–7. doi: 10.1029/2007WR006331
Roxburgh, C. W., and Rodriguez, D. (2016). Ex-ante analysis of opportunities for the sustainable intensification of maize production in Mozambique. Agric. Syst. 142, 9–22. doi: 10.1016/j.agsy.2015.10.010
Royal Society (2009). Reaping the Benefits: Science and the Sustainable Intensification of Global Agriculture. London: Royal Society. Available online at: https://royalsociety.org/~/media/Royal_Society_Content/policy/publications/2009/4294967719.pdf
Ruel, M. T., Garrett, J. L., Hawkes, C., and Cohen, M. J. (2010). The food, fuel, and financial crises affect the urban and rural poor disproportionately: a review of the evidence. J. Nutr. 140, 170–176. doi: 10.3945/jn.109.110791
Santpoort, R. (2020). The drivers of maize area expansion in Sub-Saharan Africa. How policies to boost maize production overlook the interests of smallholder farmers. Land 9:68. doi: 10.3390/land9030068s
Sanyang, S., Taonda, S. J. B., Kuiseu, J., Coulibaly, N., and Konate, L. (2016). A paradigm shift in African agricultural research for development: the role of innovation platforms. Int. J. Agric. Sustain. 14, 187–213. doi: 10.1080/14735903.2015.1070065
Schmidhuber, J., and Tubiello, F. N. (2007). Global food security under climate change. PNAS 104, 19703–19708. doi: 10.1073/pnas.0701976104
Seekell, D., D'Odorico, P., and MacDonald, G. K. (2018). Food, trade, and the environment. Environ. Res. Lett. 13:100201. doi: 10.1088/1748-9326/aae065
Shiferaw, B., Prasanna, B., Hellin, J., and Banziger, M. (2011). Crops that feed the world 6. Past successes and future challenges to the role played by maize in global food security. Food Secur. 3, 307–327. doi: 10.1007/s12571-011-0140-5
Shiferaw, B., Smale, M., Braun, H. J., Duveiller, E., Reynolds, M., and Muricho, G. (2013). Crops that feed the world 10. Past successes and future challenges to the role played by wheat in global food security. Food Secur. 5, 291–317. doi: 10.1007/s12571-013-0263-y
Sivanappan, R. K. (1994). Prospects of micro-irrigation in India. Irrigat. Drain. Syst. 8, 49–58. doi: 10.1007/BF00880798
Smale, M., Hazell, P., Hodgkin, T., and Fowler, C. (2009). “Do we have an adequate global strategy for securing the biodiversity of major food crops?” in Agrobiodiversity and Economic Development, eds A. Kontoleon, U. Pascual, and M. Smale (Oxon: Routledge). Available online at: https://books.google.de/books?hl=deandlr=andid=ZXR8AgAAQBAJandoi=fndandpg=PP1andots=j9KbCIb4eRandsig=B9XgHHGBW0XJ0DavbJwiJ9QhZiUandredir_esc=y#v=onepageandqandf=false
Stagnari, F., Maggio, A., Galieni, A., and Pisante, M. (2017). Multiple benefits of legumes for agriculture sustainability: an overview. Chem. Biol. Technol. Agric. 4:2. doi: 10.1186/s40538-016-0085-1
Stevenson, J. R., Villoria, N., Byerlee, D., Kelley, T., and Maredia, M. (2013). Green Revolution research saved an estimated 18 to 27 million hectares from being brought into agricultural production. Proc. Natl. Acad. Sci. U.S.A. 110, 8363–8368. doi: 10.1073/pnas.1208065110
Tadesse, G., and Bahiigwa, G. (2014). Mobile phones and farmers' marketing decisions in ethiopia. World Dev. 68, 296–307. doi: 10.1016/j.worlddev.2014.12.010
Tadesse, W., Bishaw, Z., and Assefa, S. (2018). Wheat production and breeding in Sub-Saharan Africa. Challenges and opportunities in the face of climate change. Int. J. Climate Change Strategies Manage. 11, 696–715. doi: 10.1108/IJCCSM-02-2018-0015
Taub, D. (2010). Effects of rising atmospheric concentrations of carbon dioxide on plants. Nature Educ. Knowl. 1:21. Available online at: https://www.nature.com/scitable/knowledge/library/effects-of-rising-atmospheric-concentrations-of-carbon-13254108/
Tilman, D., and Clark, M. (2014). Global diets link environmental sustainability and human health. Nature 515, 518–522. doi: 10.1038/nature13959
Trethowan, R., and Turner, M. (2009). New targets in wheat improvement. CAB Rev. Perspect. Agric. Vet. Sci. Nutr. Nat. Resour. 4, 1–14. doi: 10.1079/PAVSNNR20094009
UN (2019). World Population Prospects 2019. New York, NY: United Nations, Department of Economic and Social Affairs, Population Division. Available online at: https://population.un.org/wpp/
UN Water (2014). Water statistics. Available online at: http://www.unwater.org/statistics/en/?page=11andipp=10andtx_dynalist_~pi1%5Bpar%5D=YToxOntzOjE6IkwiO3M6MDoiIjt9
UNCCD (2021). The United Nations Decade for Deserts (2010-2020) and the Fight Against Desertification. Bonn: United Nations Convention to Combat Desertification. Available online at: https://www.unccd.int/actions/united-nations-decade-deserts-2010-2020-and-fight-against-desertification
UNDP (2007). Human Development Report 2006 – Beyond Scarcity: Power, Poverty and the Global Water Crisis. New York, NY: United Nations Development Programme. Available online at: https://www.undp.org/content/dam/undp/library/corporate/HDR/2006%20Global%20HDR/HDR-2006-Beyond%20scarcity-Power-poverty-and-the-global-water-crisis.pdf
URT (2007). National Adaptation Programme of Action (NAPA). United Republic of Tanzania, Vice President's Office, Division of Environment. Available online at: https://unfccc.int/resource/docs/napa/tza01.pdf
van Ittersum, M. K., van Bussel, L. G. J., Wolf, J., Grassini, P., van Wart, J., Guilpart, N., et al. (2016). Can sub-Saharan Africa feed itself? Proc. Natl. Acad. Sci. U.S.A. 113, 14964–14969. doi: 10.1073/pnas.1610359113
von Braun, J. (2008). Food and Financial Crisis. Implications for Agriculture and the Poor. Food Policy Report. International Food Policy Research Institute, Washington, DC. Available online at: https://core.ac.uk/download/pdf/6289061.pdf
von Braun, J., and Tadesse, G. (2012). Global food price volatility and spikes: an overview of costs, causes, and solutions. ZEF-Discussion Papers on Development Policy No. 161 (Bonn: Center for Development Research). Available online at: http://ssrn.com/abstract=1992470
WBGU (2019). Our Common Digital Future. Advisory Council on Global Change (WBGU), Berlin. Available online at: https://www.wbgu.de/fileadmin/user_upload/wbgu/publikationen/hauptgutachten/hg2019/pdf/wbgu_hg2019_en.pdf
WBGU (2020). Rethinking Land in the Anthropocene: From Separation to Integration. Advisory Council on Global Change (WBGU), Berlin. Available online at: https://www.wbgu.de/en/publications/publication/landshift
Webb, P. J. R. (2009). “More food, but not yet enough: 20th century successes in agricultural growth and 21st century challenges,” in Fiat Panis: For a World Without Hunger, ed H. Eiselen (Stuttgart: Hampp Media/Balance Publications). 410–434.
Wheeler, T., and von Braun, J. (2013). Climate change impacts on global food security. Science 341, 508–513. doi: 10.1126/science.1239402
Willett, W., Rockström, J., Loken, B., Springmann, M., Lang, T., Vermeulen, S., et al. (2019). Food in the anthropocene: the EAT–Lancet Commission on healthy diets from sustainable food systems. Lancet 393, 447–492. doi: 10.1016/S0140-6736(18)31788-4
World Bank (2008). World Development Report 2008: Agriculture for Development. Washington, DC: World Bank. Available online at: http://siteresources.worldbank.org/INTWDR2008/Resources/WDR_00_book.pdf
Worldometer (2020). World Population. Available online at: https://www.worldometers.info
Wu, F., and Guclu, H. (2013). Global maize trade and food security: implications from a social network model. Risk Anal. 33, 2168–2178. doi: 10.1111/risa.12064
Keywords: cereal, utilization, stability, global food production, drivers, transformation
Citation: Grote U, Fasse A, Nguyen TT and Erenstein O (2021) Food Security and the Dynamics of Wheat and Maize Value Chains in Africa and Asia. Front. Sustain. Food Syst. 4:617009. doi: 10.3389/fsufs.2020.617009
Received: 13 October 2020; Accepted: 31 December 2020;
Published: 03 February 2021.
Edited by:
Ademola Braimoh, World Bank Group, United StatesReviewed by:
Tilahun Amede, International Crops Research Institute for the Semi-Arid Tropics (ICRISAT), EthiopiaFabio Bartolini, University of Pisa, Italy
Copyright © 2021 Grote, Fasse, Nguyen and Erenstein. This is an open-access article distributed under the terms of the Creative Commons Attribution License (CC BY). The use, distribution or reproduction in other forums is permitted, provided the original author(s) and the copyright owner(s) are credited and that the original publication in this journal is cited, in accordance with accepted academic practice. No use, distribution or reproduction is permitted which does not comply with these terms.
*Correspondence: Ulrike Grote, grote@iuw.uni-hannover.de