- 1AgResearch, Hamilton, New Zealand
- 2GBDScience, Palmerston North, New Zealand
- 3AgResearch, Palmerston North, New Zealand
- 4Plant & Food Research, Hamilton, New Zealand
Steep, uncultivable hill country below 1,000 m comprises about 40% of New Zealand's land surface area. Hill country farmers require options to increase the resilience of their farms to climatic and economic extremes while addressing soil conservation and water quality issues. We profile and discuss two options that can assist in transforming hill country. The first comprises a simple approach to grazing management in hill country pastures to increase pasture resilience and the second approach focuses on including selected forage shrubs (and trees) to create grazed pasture-shrublands. Deferred grazing, the cessation of grazing from flowering until seed dispersal of the desirable species in a pasture, is an old practice which has novel applications to improve resilience of hill country farming systems. We draw on current research and practitioner experience to demonstrate the impact of deferred grazing on the resilience of the deferred pasture and the farm system. We propose that deferred grazing will: (i) increase resilience of a pasture by enabling it to better recover from biotic and abiotic stresses and (ii) reduce the risk of nutrient and sediment losses in hill country by increasing ground cover, rooting depth and soil structural stability. Introducing woody forage shrubs into hill country pastures is another option that can improve farm profitability and resilience to current and future economic and climatic variabilities. The extensive root networks of shrubs can increase soil structural stability and reduce the risk of soil erosion. In addition, shrubs can supply many other ecosystem services, such as forage and shelter for livestock. In this paper, we discuss: (i) the potential benefits of a grazed pasture-shrubland at farm, landscape and national scales; (ii) candidate woody exotic and indigenous forage species; and (iii) priorities for research.
Introduction
Steep, uncultivable hill country below 1,000 m, which is generally > 20° slope, comprises about 40% of New Zealand's land surface area (Mackay, 2008). In the farmed areas of these landscapes, the livestock grazing systems that dominate rely on perennial pastures because of: (a) an inability to cultivate widely due to moderate to steep slopes; (b) the need to avoid soil disturbance on highly erodible “soft-rock” soils; and (c) the generally mesic Mediterranean-type climates with even rainfall distribution throughout the year (Kriticos, 2012). These systems use year-round animal grazing in situ, as this is the most labour- and energy-efficient means of harvesting forage on hills. Pastures are based on grass-clover mixes, in order to combine the high photosynthetic assimilation capacity and grazing tolerance of grass with the nitrogen (N)-fixing capacity of legumes. However, species diversity within these functional groups is high (Dodd et al., 2004), with variable contributions from adventive grasses, clovers and forbs which colonize the edaphic mosaic.
Lolium perenne L. has been the most widely sown pasture species due to its relative ease of management, high productivity given suitable conditions, palatability, and ease of access to commercial cultivars (Lee et al., 2012). In the late 1960s, L. perenne was included in all sown mixtures (Lancashire et al., 1979) and by the mid-1970s, L. perenne comprised over 70% of the pasture grasses certified in New Zealand (Hunt and Easton, 1989). There remains a strong focus on genetic improvement of L. perenne for New Zealand's pastoral sector (Lee et al., 2012), but in hill country L. perenne is not the dominant grass. Other perennial species, such as Agrostis capillaris L. and Anthoxanthum odoratum L., are generally more abundant and better adapted to low fertility soils and close grazing by sheep and cattle. However, these species are of lower feed quality and their seasonal growth pattern is less aligned with animal demand. Other cultivated grass species, such as Dactylis glomerata L. and Festuca arundinacea Schreb. have received some attention in breeding programs, as they are more drought tolerant than L. perenne (Turner et al., 2012) and better suited to future climate scenarios in which drought is more prevalent (Sheffield and Wood, 2008).
In this context, we consider that the most useful way to think about resilience relates to the pressures on these desirable sown species, and whether they are persistent and maintain their persistence and the resultant productivity of high-quality herbage. In terms of the engineering model of resilience (Holling, 1996), such pressures act to reduce the abundance of desirable plant species, and shift pasture community composition toward low cover and domination by less desirable species [e.g., unpalatable plants, short-lived annuals (Sheath et al., 1984)]. Resilience is indicated by the ability of the sown community to recover after stress or disturbance, rather than revert to naturalized species (Dodd et al., 2004). Several pressures are relevant, including climate and weather patterns, prevalence of erodible soils, soil fertility, over-grazing and pest outbreaks (Tozer et al., 2011).
Decades of research on New Zealand hill country productivity has tended to focus on ensuring the productivity of desirable “improved” pasture species, through: (a) soil fertility—i.e., improving soil nutrient status through fertilizer application to enable the persistence of highly productive species of the genera Lolium and Trifolium (Kemp and Lopez, 2016); (b) grazing management—i.e., how to balance the need to maximize dry matter (DM) intake with the need to maintain growing points and persistence of desirable species (King et al., 2016); and (c) to a lesser extent, increasing the diversity of high feed quality pasture species suited to hill country—i.e., those better adapted to lower soil fertility, variable soil moisture content and poorer grazing control (e.g., Barker et al., 1993). More recent work has attempted to establish high quality forages though aerial herbicide and seed applications as a means of rapidly increasing productivity (Lane et al., 2016), but this carries high costs and risks associated with weed ingress and erosion.
Further productivity gains on sloping land are challenging for several reasons, including: (a) slope- and aspect-induced variation in soil temperature and moisture (Radcliffe, 1982); (b) fertility transfer by grazing animals in situ creates microsite variation that is difficult to counter (Hoogendoorn et al., 2011); (c) there are limitations to leaf area index achievable with herbaceous species under grazing, and therefore there is a photosynthetic capacity limit (Parsons et al., 2011); (d) there is limited ability to increase N input (the major limiting nutrient) without negative environmental impacts on water resources (Dodd et al., 2016); and (e) there are limitations to augmenting rainfall via irrigation in hills because of infrastructure costs and risks of nutrient and sediment losses into waterways. Usually reversion from sown species to more diverse mixes of naturalized grasses and forbs occurs, largely because of high spatial variability in the “habitat” for species (localized soil, climate and defoliation regimes). In the absence of grazing animals this reversion continues to woody species (Bergin et al., 1995). Note that in New Zealand, fire is not a major driver of vegetation structure (that might prevent encroachment of woody species).
We need step-changes in forage management to overcome these limitations and focus more on improving the resilience of the herbage resource, and here we discuss two possibilities. The first option—deferred grazing—retains a focus on herbaceous species but changes grazing management to maximize the resilience and productivity of desirable species. Deferred grazing, the cessation of grazing from flowering until seed dispersal of the desirable species in a pasture, is an old practice (e.g., Suckling, 1959) which has novel applications to improve resilience of hill country pasture systems by enabling pastures to better recover from the inevitable biotic and abiotic disturbances (Holling, 1996) and lower their environmental footprint. The second option changes the forage base by integrating woody species, thereby increasing photosynthetic capacity in the vertical plane, and improving resilience to current and future pressures through diversity in functional plant types (Mori et al., 2012).
Our two alternative strategies also offer opportunities to improve the environmental outcomes of farming. Increasing pasture production has compromised other important ecosystem services, such as clean water and native fauna habitat (Van den Belt and Blake, 2014). Public awareness of these issues is increasing (Hughey et al., 2010) and pressure on farming communities to reduce these impacts has focussed on three broad policy and regulatory areas: water quality, biodiversity and greenhouse gas emissions (Anon, 2015, 2016, 2019a). The two approaches introduced here, deferred grazing management and the integration of woody vegetation into hill country pastures, can harness opportunities to provide a much wider range of ecosystem services than providing feed, including, for example, enhanced soil carbon sequestration, increased soil integrity and intactness protecting waterways from runoff of sediments and nutrients, and a more diverse habitat that will support a greater biodiversity contributing to pest control and pollination services of hill country ecosystems.
Deferred Grazing
Ecophysiological Processes
Harnessing the Reproductive Cycle
Pasture management in New Zealand has focused on maximizing the quantity of high quality herbage produced throughout the year to better match feed supply and livestock demand. To achieve this, research has generally focused on maintaining pastures in a green leafy state and preventing L. perenne from flowering and producing seed as reproductive tillers rapidly lose nutritive value (e.g., Hodgson, 1990). With an increasingly volatile climate, pest pressures and the population collapse of desirable species, ensuring that pastures are resilient and can maintain high proportions of productive species is of concern to farmers (Daly et al., 1999; Tozer et al., 2014; Dodd et al., 2018). This has led to questioning the current paradigm of pasture management and a search for more resilient and sustainable management strategies that enable a reliable supply of high quality forage despite these pressures.
The ability of a pasture to recover from stresses such as drought is determined by the net effects of tiller birth and tiller death during the seasons and the ability of a plant to replace dying tillers (Colvill and Marshall, 1984; Matthew et al., 2015). Increases in tiller size and leaf area can offset a decline in tiller populations that occurs as herbage mass increases (Matthew et al., 2015). However, tiller population decline can also occur through the impact of stresses; thus in the longer term the number of tillers is an important proxy for persistence, as plants with fewer tillers are more vulnerable to abiotic and biotic stresses (e.g., Korte and Chu, 1983). Therefore, strategies that increase tillering of perennial pasture species are likely to increase resilience.
To increase tiller densities in the longer-term and to improve pasture resilience, we propose that management should be more closely aligned to the reproductive development of these species and that flowering and seed production of desirable species should be allowed to occur in some paddocks in some years. This strategy will result in:
(i) An initial decline in vegetative tiller densities in late spring/summer;
(ii) Prioritization of carbohydrate for developing reproductive stems;
(iii) Accumulation of storage carbohydrate in plant bases over the reproductive period;
(iv) Accumulation of root biomass over the reproductive period;
(v) An enforced dormancy of tiller buds over summer in hot and dry environments;
(vi) Release of tiller buds and a flush of tillering in autumn;
(vii) Seedling recruitment in autumn of desirable species from the seedbank, depending on the length of the rest period and the species being rested;
(viii) An increase in tillering and herbage accumulation following the deferred period.
We further propose that with increasing levels of abiotic and biotic stresses, an increasing period of rest is required, with full reproductive development being required in the most stressful environments, on a proportion of the farm in an annual rotation.
In the following section these ecophysiological processes are discussed in the context of developing more resilient hill country pastures. We have focused on the ecophysiology of L. perenne, given the importance of this species in New Zealand farming systems and the extensive body of scientific literature on its ecophysiology. Impacts on legumes are also briefly discussed.
Family Feud for Carbohydrate
Korte (1986) described L. perenne-based swards as consisting “of a dynamic population of short-lived tillers of different ages,” with the rate of tiller birth varying during the year (Duchini et al., 2018). L'Huillier (1987) documented a flush of tillering in spring and early summer, with the highest rates of tillering occurring between November and January, and low and variable rates occurring between February (end of summer) and October (mid-spring) in northern North Island pastures grazed by dairy cattle.
Vegetative Growth
During vegetative growth, water soluble carbohydrate (WSC) is transported to expanding roots, tillers and leaves at the shoot apex (e.g., Ryle, 1970). A small, but biologically significant, proportion of carbon is incorporated into long-term storage carbohydrate, which is typically stored in stem bases or stolons of grasses and legumes (White, 1973; Danckwerts and Gordon, 1987). Over an extended period, the amount of carbohydrate builds up, typically in the base of the stem, providing considerable reserves to recover from defoliation (Alberda, 1957; White, 1973; Danckwerts and Gordon, 1987).
Daughter tillers compete with parent tillers for WSC, which can lead to reduced tillering in times of stress (Donaghy and Fulkerson, 1998). Defoliating low and continuously into the crown of the plant can deplete WSC reserves and lead to tiller and plant death. In contrast, defoliation coinciding with the 3-leaf stage of L. perenne to 5 cm is optimal for the regrowth, productivity and persistence of the L. perenne plant (Donaghy and Fulkerson, 1998). Defoliation at the 3-leaf stage also enables WSC to be allocated to root growth and daughter tiller initiation, which occur after stubble WSC reserves are replenished (Donaghy and Fulkerson, 1998).
Reproductive Growth
During mid-spring to early summer, L. perenne and Trifolium repens L. are particularly vulnerable to environmental stresses due to the plant population re-establishing itself through new tillers (in the case of perennial ryegrass), fragmentation of parent plants and production of new smaller plants [in the case of Trifolium repens, and production of seed (Edwards and Chapman, 2011; Macdonald et al., 2011)]. While this reproductive development period is a major focus of crops, it has often been neglected when investigating pasture persistence (Kemp and Culvenor, 1994). Yet this period provides opportunities that can be harnessed to improve pasture resilience as shall be discussed in this paper.
Perennial ryegrass tillers that become reproductive are generally formed before winter (Korte, 1986). Reproductive development and flowering of perennial ryegrass are triggered after a vernalisation period and in response to a change in daylength and temperature (Halligan et al., 1993). When reproductive stems begin to elongate in mid- to late spring, leaf initiation and tiller bud development are suppressed (Jewiss, 1972). During this period, there is a continual feud for carbohydrate between parent and daughter tillers. Small, shaded tillers are unlikely to obtain the resources they need to survive. In contrast, larger tillers are not dependent on other tillers for WSC and being taller, are better positioned to capture light and photosynthesise (Ong et al., 1978; Colvill and Marshall, 1984). Given this feud, few vegetative tillers survive the period of reproductive growth in undefoliated swards.
During reproductive development, WSC is prioritized for inflorescence and seed development, although a small but biologically significant amount of WSC moves downwards from the reproductive tiller. It is stored in the base of the plant and remobilised for seed production and growth of new tillers (Hampton et al., 1987; Matthew et al., 1991).
The new tillers produced after flowering provide the bulk of flowering tillers in the following year, and are critical for the longevity of a ryegrass plant and for persistence (Korte, 1986; Matthew et al., 2015). Tillers produced after flowering are subjected to less competition for carbohydrate (due to the removal of competition with reproductive tillers) and their chance of survival can be much greater.
One of the most dramatic impacts of post-anthesis tillering on tiller generation and survival of L. perenne is documented by Waller et al. (1999) in summer-dry south western Victoria, Australia. New daughter tiller buds were produced at the base of reproductive tillers which died after setting seed. These buds remained dormant over summer but new tillers emerged in autumn when soil temperatures were lower and the soil moisture content was adequate for growth. Over 50% of reproductive tillers survived over summer and were present in autumn, while in contrast, only 12% of tillers that were vegetative in late spring survived until the autumn rains, when new tiller production occurred (Waller et al., 1999). This corresponded to a 15-fold greater production of new tillers from reproductive tillers than from vegetative tillers during the autumn (Waller et al., 1999). Tillers produced from reproductive tillers were also 25 times more likely to survive than those produced from vegetative tillers (Waller, 2002). In addition, reproductive tillers were able to produce seed, which culminated in many seedlings in the following autumn (Waller et al., 1999).
Based on a knowledge of tiller birth and death as described above, a range of approaches have been proposed to increase tillering. Their impacts on phenological development, ecophysiology, benefits, risks and constraints are summarized in Table 1, Figure 1 and are discussed henceforth.
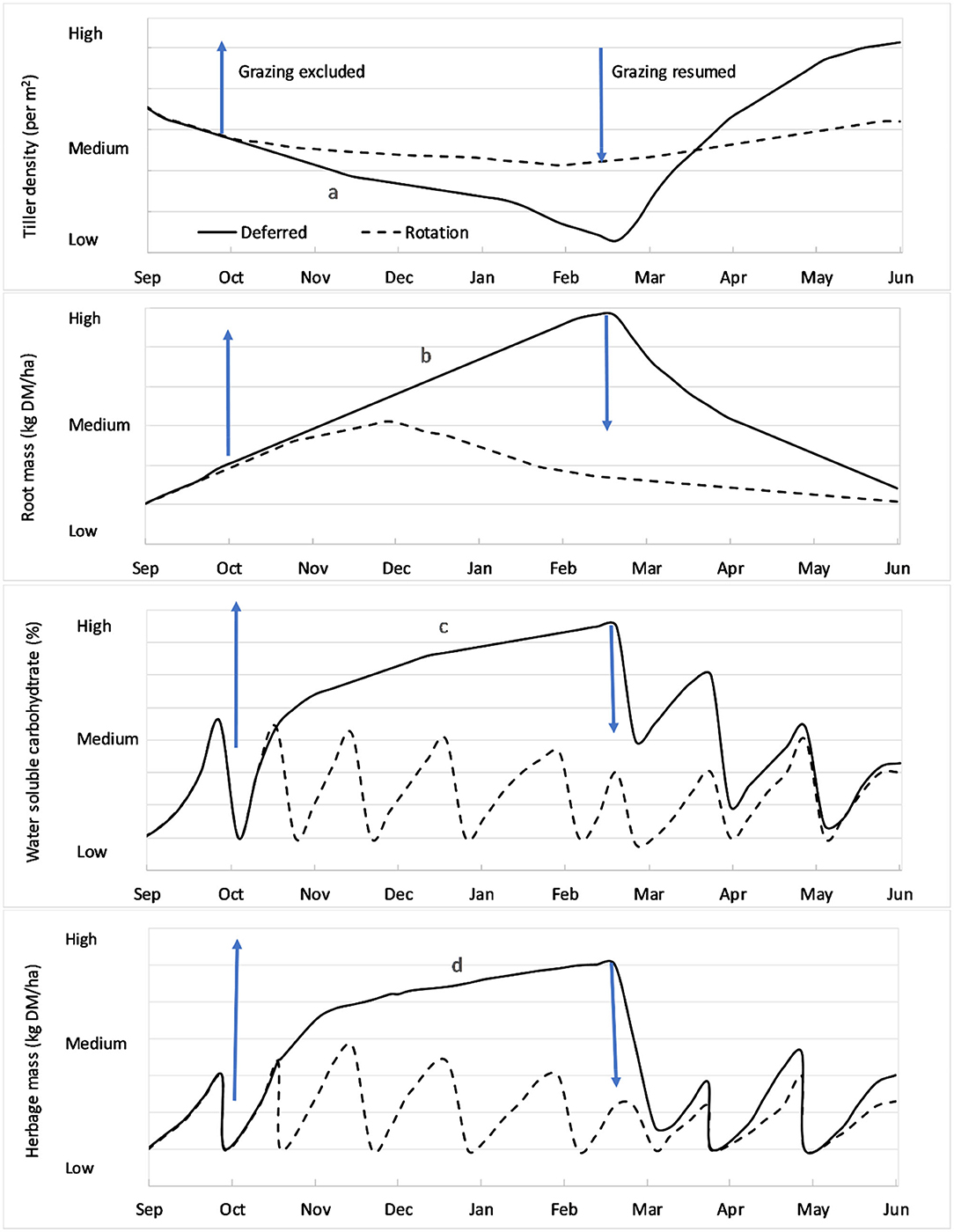
Figure 1. Changes in L. perenne tiller densities, root mass, water soluble carbohydrate content and available herbage in a L. perenne-based pasture in response to rotational grazing ‘- - - -‘; or deferred grazing (i.e., rotational grazing with a rest period between mid-spring and the end of summer) “______” in a high abiotic (e.g., drought) or biotic (e.g., invertebrate pest) stress environment (based on Lee et al., 2009; Dodd and Mackay, 2011). Arrows denote when grazing is excluded and resumed for the deferred grazing treatment. Grazing intervals will depend on biophysical, climatic and enterprise considerations, but typically range from 3 weeks (during spring) to 10 weeks (during summer/winter). In the deferred pasture there is: (a) a decline in tiller density during the deferred period followed by a rapid increase after grazing is resumed; (b) an increase in root mass during the deferred period followed by a decline after grazing is resumed; and an increase in (c) water soluble carbohydrate and (d) herbage mass during the deferred period followed by fluctuating levels in response to subsequent grazing events.
Negligible Reproductive Development
Hard grazing in spring to prevent reproductive development can promote tillering (e.g., Brock and Hay, 1993). In situations where soil temperatures, moisture deficits and nutrients are unlikely to be limiting, an intensive grazing regime can reduce shading and accumulation of dead material. In this situation, a large proportion of the leaves contribute to photosynthesis and lead to high rates of assimilate and biomass production. Hard grazing during spring, to maintain the tiller populations, given adequate soil moisture over summer, can therefore be used as a strategy to increase tiller density in the following autumn. However, the increased tiller density with lower herbage biomass may not be sufficient to compensate for the associated loss in leaf area and light capture (Matthew et al., 1996). This can lead to a decline in leaf area index, tiller populations and reduced pasture persistence. The risk is heightened in a dry summer when intensive defoliation can result in mortality of L. perenne tillers and plants (Brougham, 1960). Root growth at depth may also be compromised. This may restrict access to soil moisture during drought and compromise recovery after a drought (e.g., Korte and Chu, 1983).
Moderate Reproductive Development
Tiller bud development is inhibited during flowering, but buds are released if the inflorescence is removed (Matthew et al., 1991). Matthew et al. (2015) proposed harnessing the post-anthesis tillering perennation strategy of modern New Zealand L. perenne cultivars by decapitating the inflorescence to stimulate tillering (Xia et al., 1990; Garay et al., 1997a,b). Root growth can also be enhanced by allowing moderate reproductive development (Matthew et al., 1986). However, stimulation of daughter tiller production in late spring after decapitation may leave daughter tillers vulnerable to drought stress over summer. “Provided daughter tillers are formed before drought stress occurs, they may simply delay the development of secondary and tertiary tillers until conditions become favorable for growth” (Xia et al., 1990). This assumes that flowering is sufficiently late in the season for further tiller development to be delayed over summer and that available soil moisture enables survival of daughter tillers over summer The strategy has been applied successfully from plot to farmlet scale with increases in tillering and dry matter production (e.g., Matthew et al., 2000, 2016; Da Silva et al., 2004). However, from a management perspective, targeting grazing to a narrow range in phenological development can be operationally challenging and the benefits have not always been realized (Bishop-Hurley et al., 1997; Bishop-Hurley, 1999). For example, one attempt to implement late control in a self-contained farm system failed to generate the anticipated differences between treatments in herbage mass, and consequently effects on sward behavior were negligible (Bishop-Hurley et al., 1997).
Full Reproductive Development
A third strategy involves preventing buds at the base of the reproductive stems from forming tillers post-anthesis so that tiller buds enter enforced dormancy with the onset of hot dry summers (Waller and Sale, 2001). Tillering is then delayed until autumn when conditions are conducive for tiller buds to be released and tiller growth to occur (Korte and Chu, 1983). This can be achieved by resting a pasture from grazing in late spring until late summer (deferred grazing). This strategy also enables seedling recruitment to occur, which can assist in population growth of desirable species. “Autumn clean-up hard grazings” are important to reduce the presence of dead and sheath material that accumulate over summer. This increases the penetration of light into the base of the sward to promote the development of new tillers and will increase their survival (Hunt and Brougham, 1967).
Thus, deferred grazing can enable a dual pathway for enhancing resilience—through the production of daughter tillers from existing plants given sufficient resources, and through the production of seed and seedling recruitment from the seedbank. The beauty of this insurance policy is that if the environmental conditions are not conducive for L. perenne survival over summer, the population can be maintained through seedling recruitment, as occurs in pasture renewal.
Impacts of Deferred Grazing
Sward Characteristics
Our understanding of the ecophysiology and tillering patterns is consistent with results from agronomic field studies. In years when rainfall does not limit growth, resting pastures from late spring until the end of summer or early autumn can lead to an increase in the size, density and vigor/competitive ability of the perennial species, with seedling recruitment contributing to population growth. In drought years, a decline in the perennial species populations may occur—but to a lesser extent when pastures have been rested from grazing over summer than when they have been grazed continuously or grazed using a rigid rotation (L'Huillier and Aislabie, 1987; Hume and Barker, 1991; Kemp et al., 1997, 2000; Nie et al., 1999).
The response of a pasture to a deferred period will depend on the botanical composition at the time of stock exclusion, the seasonal conditions, soil fertility, and the opportunities for recruitment (Garden et al., 2000). In New South Wales, Australia, it was shown pasture rest could increase the content of perennial species and reverse pasture decline, with the rate of response depending on the proportion of perennial species initially present in the pasture (Dowling et al., 2006). Generally, “pastures were responsive to rest tactics when the total perennial grass composition was between 10 and 70% irrespective of the perennial grass species, time of year or site. Above 70%, little benefit is likely to be obtained from resting the pastures and if the desirable perennial content is under 10%, it is unlikely to make use of the additional resources made available through a rest period” (Kemp et al., 2000). In New Zealand, there is a lack of data on these thresholds for perennial ryegrass and how less desirable species, such as Holcus lanatus L. and Agrostis capillaris, may respond to resting pastures over summer (Garden et al., 2000).
The timing of grazing exclusion and resumption of grazing can have a significant impact on the botanical composition (e.g., Sheath and Boom, 1985). If the grazing is timed for when annual grass weeds are undergoing stem elongation, grazing can remove the developing reproductive stems and prevent flowering and seed production of annual weedy species. If pastures are then immediately rested from grazing until late summer/early autumn, later flowering perennial species can still undergo reproductive development, flower and set seed. This strategy can significantly reduce the content of weedy annual grasses while increasing the perennial content (Nie and Zollinger, 2012). Conversely, when a deferred period is extended into mid- or late-autumn, the accumulated pasture biomass present at the end of summer/early autumn can prevent adequate light from reaching the tiller bases. This can suppress grass tillering and increase mortality of new tillers and seedlings that have emerged from the seedbank. This approach has been suggested to reduce competition from resident species in preparation for oversowing new germplasm in hill country pastures (Nie et al., 1998).
Deferred grazing can have a positive impact on legumes. Increases in the contribution of clover to the sward and greater clover growth rates have been documented in response to deferring pastures from late spring until mid-autumn, with positive effects lasting for several years after the deferred period (Nie et al., 1996). This most likely occurred because deferred grazing reduced the grass tiller density [which was inversely associated with clover growing point density (Nie et al., 1996)]. Changes in the soil microclimate, such as a reduction in the soil surface temperature and increase in soil moisture content (e.g., Tozer et al., 2020), also made conditions more conducive for legume growth (e.g., Suckling, 1959). Conversely, if deferring encourages grass populations, the clover content can be suppressed. This occurred on East Coast New Zealand hill country when pastures were deferred between late spring and early autumn (November—March). The clover content (% of total DM) was reduced, although the mass of legume in the swards was greater in deferred than grazed pastures (Korte and Quilter, 1990). The impact of deferred grazing on clover is also likely to depend on clover morphology, as longer grazing intervals favor larger leaved cultivars to a greater extent than smaller leaved cultivars (Edwards and Chapman, 2011).
Impacts of deferred grazing on pasture quality have varied. For example, the proportion of dead matter at the base of the sward and residual herbage mass can increase in response to deferred grazing, which can lead to a reduction in pasture quality (e.g., McCallum et al., 1991). Conversely, improvements in botanical composition through reducing the sward content of annual species and increasing that of perennial species has led to improvements in the nutritive value of pastures and an increase in the livestock carrying capacity (Nie and Zollinger, 2012).
Deferred grazing has improved herbage growth rates after the deferred period which can compensate for the loss of production which occurs when pasture growth rates slow and swards senesce during the deferred period. This has been demonstrated for summer wet and summer dry hill country in New Zealand (e.g., Tozer et al., 2020) and in a Western Australia Mediterranean climate (Proffitt et al., 1993). In Western Australian pastures, where the average annual rainfall was 307 mm and a typical growing season was between May and October, deferred grazing yielded the same quantity of biomass as the grazed control over one growing season. This was despite the deferred pastures having a reduced period available for grazing (Proffitt et al., 1993).
Plant and Soil Interactions
Deferred grazing can increase deep root biomass when compared with intensive grazing (Mackay et al., 1991; Nie, 2011). This can increase plant access to water and nutrients, enhance drought resilience, and reduce nitrate leaching (Bowman et al., 1998; Dunbabin et al., 2003; Durand et al., 2007). The large amount of litter and plant residue material that is trampled into the soil by livestock at the end of the deferred period adds a carbon pulse to the system, which may immobilize soil nitrogen and reduce nitrate leaching losses (Mackay et al., 1991). Consequently, deferred grazing may provide an opportunity for nitrogen conservation in the soil that could be used for pasture regrowth following the deferred period. However, no published literature is available on the effects of deferred grazing on nitrate leaching.
Further, deferred grazing can increase ground cover during and after the deferred period (Nie and Zollinger, 2008), which is an important driver for reducing sediment concentrations in runoff from pastures (Sanjari et al., 2009). Together with the above-mentioned factors this contributes to the regeneration of soil architecture, pore structure and connectivity under deferred grazing (Proffitt et al., 1993). This was observed in a field experiment comparing physical soil properties under deferred and intensive grazing [e.g., decreased bulk density, and increased water infiltration, unsaturated hydraulic conductivity and air permeability (Nie et al., 1997)].
Anecdotally, deferred grazing has been used to increase the soil moisture content of topsoils. The accumulation of dead plant material in combination with reduced transpiration rates of dead rank vegetation are generally thought to reduce water losses. For example, moisture contents were higher under deferred than rotational grazed pastures at a depth of 0–15 cm in the middle of autumn in summer-wet New Zealand hill country (Nie et al., 1997) and 15–30 cm in autumn and winter in summer-dry southern Australian hill country (Nie and Zollinger, 2012). Responses will differ depending on soil type and climate. Increased soil water contents will lower soil temperatures (Watson et al., 1996). This may be exacerbated by shading through taller dead rank plants under deferred grazing. Higher water contents may prevent the occurrence of soil water repellency, a transient soil property correlated with soil water contents that prevents water from infiltrating into the soil (Doerr and Thomas, 2003; Hermansen et al., 2019). This may further reduce runoff and sediment losses (Gillingham and Gray, 2006; Müller et al., 2018). To our knowledge no studies have analyzed changes in runoff response to summer and autumn storms under deferred vs. conventional grazing.
Macro- and Micro-Fauna
Soil temperature and water content are the most important environmental factors affecting microbial growth and activity in soils (Kirschbaum, 1995; Katterer et al., 1998). For example, Baldrian et al. (2010) found that moisture contents were positively correlated with microbial biomass, enzyme activity, and carbon mineralization. Through altering the topsoil microclimate as described above, deferred grazing can also affect microbial activities in the soils, which affects carbon and nitrogen mineralization. Stimulation of soil organic matter mineralization has been observed under deferred grazing (Mackay et al., 1991; Nie et al., 1996).
The accumulation of biomass and changes in microclimate (e.g., increased soil surface moisture and moderation of temperature) that occur when pastures are deferred may change the suitability of habitat for invertebrate pests such as Costelytra zealandica (White) (grass grub) (e.g., Watson et al., 1996). This does not appear to be an issue with pastures opened in early autumn, but may become an issue if pastures are opened in late autumn (McCallum et al., 1991; Anon, 2006).
Facial eczema has been flagged as a concern as it often proliferates when litter levels are high. However, Pseudopithomyces chartarum (Berk. & M.A. Curtis) Jun F. Li, Ariyaw. & K.D. Hyde spore counts have been lower after deferring than in grazed pastures (Suckling, 1959; McCallum et al., 1991). The reasons for the potentially lower spore counts are not known and require research.
At a landscape scale, the effects of deferred grazing on nitrogen losses via leaching, and phosphorus and sediment losses via runoff and erosion are largely unknown. While, based on first principles, it is assumed that the risk of nutrient and sediment losses will be reduced due to improved ground cover, greater rooting depth, enhanced soil structural stability and nitrogen immobilization, no studies have been conducted to quantify these potential benefits at larger scales. Further, the implications of deferred grazing management for a farm's overall feed supply and its environmental footprint have not been assessed. At a farm-scale, a critical issue is how to guarantee a sufficient supply of high quality feed throughout the year with a low environmental impact. A strategic integration of deferred grazing into a farm system would provide standing forage in late summer/early autumn while enabling feed to accumulate in the paddocks on the rest of the farm. The additional pasture may reduce farmers' reliance on crops to address feed shortages in late autumn and early winter. This could lead to additional environmental benefits through reducing soil cultivation/disturbance, lowering energy consumption and thus greenhouse gas emissions and lowering the risk of nutrient losses to waterways through runoff and erosion.
Woody Species and Their Uses in Farming Systems
The Role of Woody Vegetation
Woody vegetation is almost always a component of farming enterprises throughout New Zealand, on flat to steep land, and at a range of scales. About 11.4 M ha of the country's 26.4 M ha land area are used for pastoralism involving grazing livestock, mostly sheep and beef cattle (8.5 M ha) and dairy cattle (2.6 M ha) (White, 1999; Anon, 2018). The woody component ranges from individual trees and isolated patches or fragments of indigenous (native) species through to introduced (exotic) shrub and tree species, and mixtures of natives and exotics, for a range of purposes (Wilkinson, 1999; Walker et al., 2004; Dodd et al., 2008; Benavides et al., 2009). Native shrub and tree species are also being planted increasingly at strategic locations within farms (Marden et al., 2005; Dodd and Ritchie, 2007; Tane'sTreeTrust, 2011; Norton et al., 2018), and this trend is expected to continue.
The country's forestry estate is 8.1 M ha, 30% of New Zealand's total land area, and comprises about 1.7 M ha of exotic species in production forestry, about 90% of which is Pinus radiata D. Don; native forests cover 6.4 M ha, with 1.2 M ha of these being owned privately (MPI, 2019, 2020). On land > 20° slope, pastoralism and production forestry are frequently competing land uses with the balance between them being influenced by factors including temporal and spatial economics, environmental and political issues and concerns, and rural and community vitality (Douglas et al., 2013b). Ingress and development of woody weed species such as the exotics Ulex europaeus L. and Rubus fruticosus L., and the native Leptospermum scoparium J. R. et G. Forst., can occur on farm land principally because of reduced soil nutrient status/fertilizer inputs and lowered livestock grazing pressures (MacCarter and Gaynor, 1980; Bascand and Jowett, 1982; Bourdôt et al., 2007; Douglas et al., 2015).
Integrating woody exotic and native vegetation into farming systems has numerous potential benefits including provision of shade and shelter for grazing livestock (Gregory, 1995; Hawke and Dodd, 2003; Pollard et al., 2003), enhanced plant and faunal biodiversity (Blackwell et al., 2008; Griffiths et al., 2008; Norton et al., 2018), soil improvement through decomposition of leaf litter and roots, N from legumes and increased soil nutrient levels (Hawke and O'Connor, 1993; Benavides et al., 2009; St. John et al., 2012), and vista enhancement (Swaffield and McWilliam, 2013). Other benefits include increased carbon sequestration (Czerepowicz et al., 2012; Dymond et al., 2013; Mason et al., 2014) although effects on soil carbon mass vary with species (Douglas et al., 2020), fodder for honey bees (Apis mellifera L.) (Butz Huryn, 1995) and birds (MacFarlane et al., 2016), provision of high-value honey (Stephens et al., 2005; Martini, 2016), specialty timbers and other products such as edible nuts (Davies and Macfarlane, 1979; Bull et al., 1985; Davies, 1985b), feedstocks for bioenergy production (Snowdon et al., 2013), and remediation of heavy metal contaminants from soil and groundwater (Robinson et al., 2000; Hahner et al., 2014).
A widespread function of woody vegetation is erosion control, principally of mass movement processes, but also of other types such as surface erosion (wind and mediated by water) and streambank erosion (Pollock, 1986; van Kraayenoord et al., 1986; Bergin et al., 1995; Hicks, 1995; Cairns et al., 2001; Marden et al., 2005; Hughes, 2016). Wide-spaced trees of Populus, Salix, Eucalyptus and other species enable pastoralism to continue on steep, erodible slopes (Thompson and Luckman, 1993; McIvor et al., 2011; Douglas et al., 2013a). Associated with erosion control is the control of stocks and flows of sediments, nutrients and faecal contaminants (Parkyn et al., 2003; Davies-Colley, 2013; Dodd et al., 2016). Regardless of species, woody vegetation has greater vertical and lateral root system development than herbaceous species, conferring increased soil stabilization on slopes and control to greater soil depth (Stokes et al., 2009).
Woody Species for Fodder
The potential of woody species to supply supplementary fodder for grazing livestock in New Zealand's farming systems, particularly those located in areas or regions where pasture production and quality are reduced because of environmental limitations such as soil water deficits, has been recognized for a number of decades (Halliwell, 1979; Davies, 1985a; Sheppard, 1985; Lambert et al., 1989a; Wills et al., 1990; Douglas et al., 1996b; Charlton et al., 2003). Target regions for using woody species for fodder are mainly those on the east coast of the country, extending in the North Island from Northland to Wairarapa, and in the South Island comprising principally Marlborough, Canterbury and Central Otago, the latter of which has a semi-arid climate with annual rainfall of <400 mm (Macara, 2015). Management of individual species has been based mainly on growth form, particularly canopy height, and annual and seasonal growth patterns, and ranged from direct grazing, coppicing and pollarding to grazing-cutting combinations. Canopies of species have been defoliated once or more per year or stock-piled for use in subsequent years depending on livestock feed requirements (Lambert et al., 1989d; Douglas et al., 1996a). The main woody species in New Zealand with potential to supply fodder for livestock (i.e., that can be grown in blocks or grazed directly) are presented in Table 2.
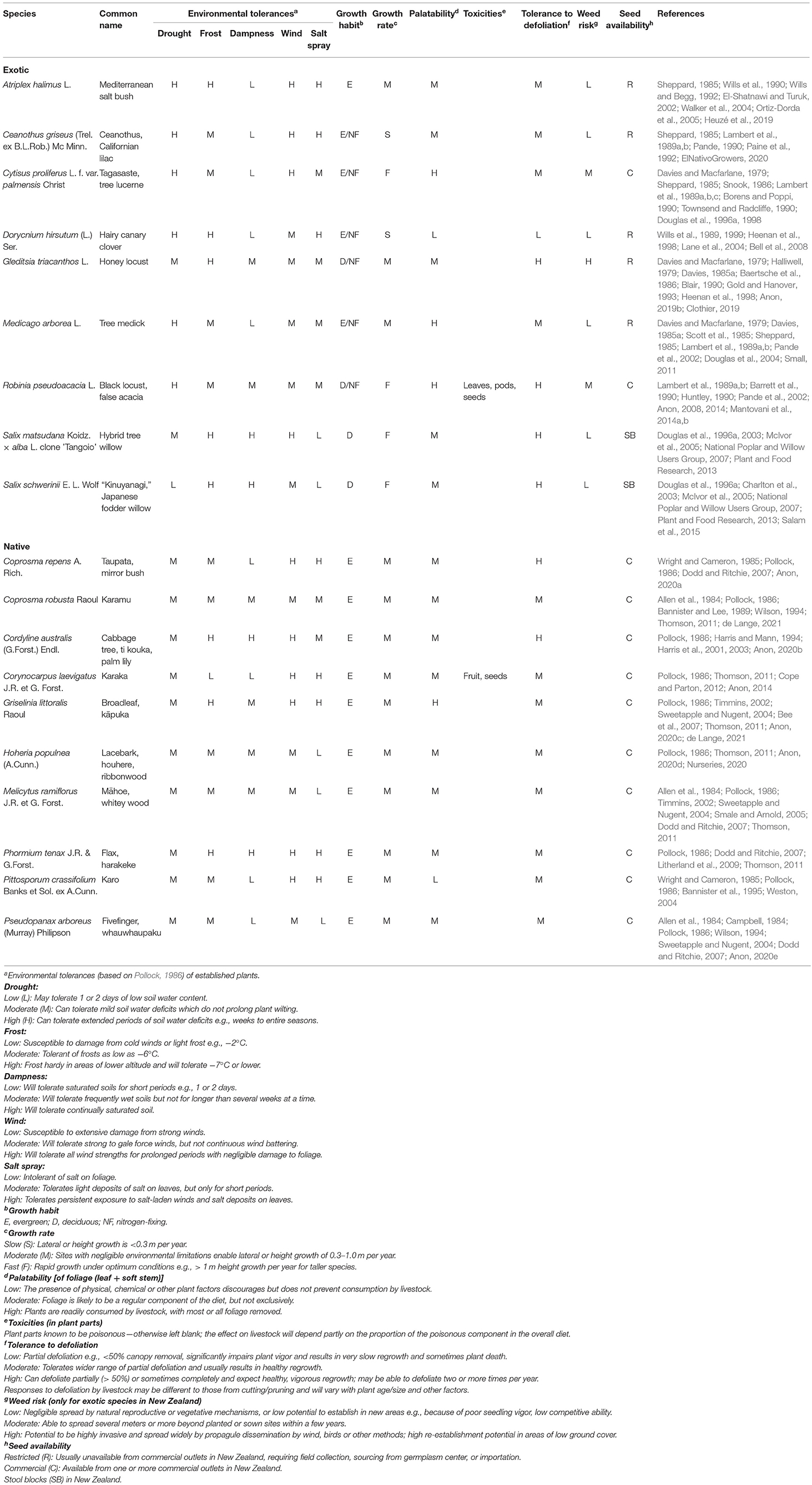
Table 2. Characteristics of exotic and native shrubs/trees potentially useful as forage for sheep and beef cattle in New Zealand.
Exotic Species
Until the early 2000s, most attention was given to Cytisus proliferus var. palmensis (henceforth C. proliferus) (Radcliffe, 1985; Townsend and Radcliffe, 1987, 1990; Lambert et al., 1989a; Borens and Poppi, 1990; Douglas et al., 1998). In Canterbury under grazing by sheep and trimming, or cutting only, edible yield (leaf and stem <6 mm diameter) of plants cut later to 0.5 m, when aged 27 months, averaged 2,130 g DM per plant (Radcliffe et al., 1985). Under a range of cutting and grazing treatments, the highest yields of 330–450 g edible DM (EDM)/plant/year were obtained under cutting (Townsend and Radcliffe, 1990). On two hill sites in Canterbury, mean yield of cut plants was 940 and 1,080 g EDM/plant/year (Radcliffe, 1986). Variation in edible yield between plants at the hill sites and that at the other sites was attributed to numerous factors including differences in incidence and severity of frosts, soil type, and plant layout/spacing. Assuming 7,500 plants/ha, annual yields of 2.5–3.4 t EDM/ha were estimated by Townsend and Radcliffe (1990) for the most productive treatment, which provided additional DM of up to 22% over pasture alone.
The leaves of C. proliferus are very palatable, have crude protein content of 170–260 g/kg DM depending on leaf age and other factors, and in trials with lambs, in vivo digestibility of DM (DMD) was 77% and organic matter digestibility (OMD) was 78% (Borens and Poppi, 1990). In the lower North Island, estimated in vivo DMD was 71–73% (Lambert et al., 1989c) and in vitro OMD attained 77-85% (Douglas et al., 1996a). Over 6 weeks, average growth rate of lambs browsing C. proliferus (81 ± 36 g/day) was less than for those grazing Medicago sativa L. (265 ± 33 g/day) and Bromus catharticus Vahl (151 ± 35 g/day), suggesting that the feeding value of the species was low compared with well-managed pastures (Borens and Poppi, 1990). In Australia, high concentrations of phenolic compounds in summer and autumn (5–6% of DM) reduced palatability and feed intake compared with in winter (<3% of DM) (Wiley, 2006); aspects which have not yet been studied in New Zealand. The foliage contains marginal or low levels of phosphorus and sodium, but in a pasture-tagasaste system it is possible that grazing livestock will meet their requirements through pasture intake. Management of C. proliferus should aim to reduce bark-stripping and keep canopies within stock grazing height and it should be used on drier sites to reduce susceptibility to collar rot (Lambert et al., 1989d).
Species of Dorycnium, principally D. hirsutum and D. pentaphyllum Scop., were first evaluated for revegetation and fodder supply in the 1970s in semi-arid country and rangelands in the South Island and later in the North Island (Wills, 1983; Sheppard and Douglas, 1986; Wills et al., 1989, 1999; Douglas et al., 1996b). They were found to be very drought- and frost-tolerant and tolerant of hard grazing once established. Accessions of D. pentaphyllum were more palatable to sheep than those of D. hirsutum, although there was considerable variation between accessions of D. hirsutum in browsing preference depending on location and browsing time (Wills et al., 1999), and perhaps variation in hairiness and leaf chemical composition. Under very dry conditions, accessions of D. pentaphyllum wilted earlier than those of D. hirsutum. In the early 2000s over two consecutive years, seed multiplication of an elite selection of D. hirsutum was attempted but it failed because of unusually moist conditions. Dorycnium rectum (L.) Ser. in DC. is palatable but its leaves have very high concentrations of condensed tannins (19% of DM), with consequent low DMD (60%) (Waghorn et al., 1998), and it has very low productivity in dry environments (Douglas et al., 1996a).
Early observations of Atriplex halimus found its foliage to be very palatable but plants grew poorly on hills in Marlborough and near Christchurch (Sheppard, 1985). At other locations in the South Island, the species was more adaptable to cold, dry, hill country than other species of Atriplex resulting in >20 ha of A. halimus being established on one farm and thousands of seedlings being produced for planting in the early 1990s (Wills et al., 1990; Wills and Begg, 1992). Atriplex spp., likely including A. halimus, were evaluated in at least two regions in the North Island, but results were not reported. Foliage of A. halimus was found to be highly nutritious with DMD of 78–80%, metabolisable energy content of about 12 MJ/kg DM, and crude protein content of 75–219 g/kg DM (estimated from Table 2 as N concentration ×6.25) (Wills et al., 1990). The most recent report found was the use of A. halimus on a commercial farm in Marlborough (Wills, 2008).
Numerous other woody species were evaluated in the latter half of the twentieth century for fodder potential with the most promising being Ceanothus griseus, Gleditsia triacanthos, Medicago arborea, and Robinia pseudoacacia (Davies and Macfarlane, 1979; Davies, 1985a,b; Sheppard, 1985). Sheppard (1985) described C. griseus and other Ceanothus spp. as palatable. Gleditsia triacanthos, M. arborea, and R. pseudoacacia were all regarded as palatable to livestock, productive, and free from disease (Davies, 1985a,b). Shrubs grown in row plots in summer-moist hill country in the lower North Island had mean annual production (g DM/m row) over 2 years in the order R. pseudoacacia (315) > C. griseus (251) > M. arborea (79) and their foliage was highly digestible (in vitro DMD) being 70% for R. pseudoacacia, 75% for C. griseus and 73% for M. arborea, compared with 69% for pasture (Lambert et al., 1989c). At the same site, these species were the most preferred of a range of species by grazing sheep and goats (Pande et al., 2002). The relatively low yield of M. arborea was because of its inherent canopy size but also possibly low tolerance to the damp conditions at the site, particularly in one of the replicates, or nodulation failure which was suggested as a cause of decreasing plant productivity over 5 years in Canterbury hill country (Radcliffe, 1985). Robinia pseudoacacia is the only species known to have toxic elements in the foliage in New Zealand (Anon, 2014), also reported internationally (Anon, 2008).
Since the early 2000s, most research on woody species has focused on the yield and fodder value of Populus and Salix spp., with nearly all being conducted in the lower North Island. Earlier investigations in the same regions and elsewhere found that P. deltoides × nigra “Flevo” and S. matsudana × alba “Tangoio” were palatable to animals, productive and disease-free (Davies, 1985a), and yield of “Tangoio” was greater than for other Salix spp. (Radcliffe, 1985). Foliage of S. matsudana × alba had greater DMD than that of the leafier S. viminalis (64 vs. 57%) and its voluntary intake of DM by sheep and goats was greater, possibly because of differences between the species in concentrations of lignin and condensed tannin (McCabe and Barry, 1988). “Tangoio” had greater nutritive value than S. schwerinii on summer-moist hill country and on drought-prone sands in terms of in vitro OMD (64–81% vs. 40–62%), lower lignin concentration (59 vs. 95 g/kg DM) (Douglas et al., 1996a), and lower condensed tannin concentration (59 vs. 255 g/kg DM) (Oppong et al., 2001), and it was more drought-tolerant (Douglas et al., 1996a).
The promising characteristics of “Tangoio” led to the establishment and management of browse or coppice blocks of the clone (Douglas et al., 2003; National Poplar and Willow Users Group, 2007) and research on their potential for improving livestock performance during summer drought. Consuming foliage of the clone reduced liveweight loss of ewes and beef cattle and maintained or increased ewe reproductive performance during pre-mating and mating periods, in comparison to stock grazing “drought pasture” (Moore et al., 2003; McWilliam et al., 2005; Pitta et al., 2007). “Tangoio” has potential to reduce methane emissions in ruminants (Ramírez-Restrepo et al., 2010) and may benefit hoggets with parasite burdens (Musonda et al., 2009), possibly because of the type and concentration of its foliar condensed tannins. Supplementing pasture with P. deltoides × nigra “Veronese” has also increased ewe reproductive performance (McWilliam et al., 2004).
Annual yield of edible fodder of trees of Salix and Populus spp. has been estimated for wide-spaced trees and for those in browse blocks. Individual trees of “Tangoio” aged 5–10 years [diameter at breast height (DBH) = 0.09–0.20 m; canopy width 2.3–6.3 m] yielded 3–22 kg DM/tree compared with 1.6–18.0 kg DM/tree for “Veronese” (DBH = 0.07–0.21 m; canopy width 2.2–4.2 m) (Kemp et al., 2001) and over a greater range of tree age/size, exponential relationships were developed between edible fodder and diameter at breast height (Kemp et al., 2003). With knowledge of tree stocking rate, these data enabled estimation of yield on a per hectare basis for feed budgeting. In browse blocks involving Salix spp., edible yield has been up to 7.0 t DM/ha/year in established stool blocks (Jones and McIvor, 2013), although this can vary considerably with tree density and planting arrangement.
Native Species
Almost no research has been conducted on the potential of native woody species to supply fodder for livestock in farming enterprises, including on annual and seasonal production, nutritional characteristics (palatability, digestibility, and nutrient contents), livestock performance, and optimum grazing/cutting management for the benefit of the plants and the stock that graze them. A notable exception is the research conducted on L. scoparium and Cassinia leptophylla (Forst.f.) R.Br. on summer-moist hill country in the 1980s (Lambert et al., 1989a), which found that both species had low potential as fodder sources. Cassinia leptophylla was unpalatable to sheep and goats and L. scoparium was intolerant of defoliation and its foliage had low palatability and digestibility (Lambert et al., 1989d).
In an analysis of preferences by deer for shrub and tree species, those such as Aristotelia serrata, Coprosma grandifolia, Cordyline australis, Griselinia littoralis, Melicytus ramiflorus, and Pseudopanax arboreus were preferred, but most species were not selected, or avoided. Preferred species had relatively low (<4 g/kg DM) foliar lignin concentration (Forsyth et al., 2002). In addition to concentrations of cellulose and phenolics, Bee et al. (2011) concluded that season and associated species can also influence diet selection. Exclusion of cattle in forests in Westland resulted in an increase in abundance of woody species (Buxton et al., 2001) and where sheep and cattle were excluded from forest fragments in the Waikato region, abundance of woody species including Coprosma grandiflora, Schefflera digitate, and M. ramiflorus increased (Burns et al., 2011). The preference of species to ruminants was categorized by Sweetapple and Nugent (2004) who found that highly preferred species included C. grandiflora, Coprosma lucida, G. littoralis, M. ramiflorus, and S. digitata. New Zealand's Carmichaelia spp. are regarded as highly palatable to many introduced herbivores including sheep, deer and goats (Dawson, 2016). Smale et al. (2008) reported that the palatability of seedlings of tree species to sheep was unknown.
There are numerous anecdotal reports on the palatability of native species to livestock with one of the most recent describing G. littoralis, M. ramiflorus, Phormium tenax, and P. arboreus as being moderately to highly palatable (Clothier, 2019). Under the heading of “Stock Fodder Use,” species listed as beneficial to stock were A. serrata, C. grandifolia, C. robusta, Hebe stricta, Hoheria populnea, and P. tenax (Dodd and Ritchie, 2007), which implied that they were palatable to varying extents.
The regrowth responses of native woody species to grazing by livestock in terms of morphology and growth rate are unknown or uncertain and often subjective, and no studies have been conducted involving the key potential candidates reviewed here. An indication of potential responses can be gleaned from brief descriptions from nursery growers, for example Anon (2020a,c), on responses likely from coppicing, pollarding or form pruning.
With the (social/political/market) expectation that farm systems and enterprises become more environmentally sustainable, and with increasing recognition that a number of native species have fodder potential, there is an urgent need to progress on research on this largely untapped resource.
Implementation in Farm Systems
The previous sections have explored two approaches to a departure from conventional New Zealand pasture management. Deferred grazing departs from the norm in that, while pasture remains the core forage base, the focus shifts from maximizing animal intake efficiency in the short term, to maintaining pasture botanical composition, feed quality and resilience in the longer-term (Da Silva et al., 2004; Nie and Zollinger, 2012). This is achieved by recognizing the importance of tiller demography and reproductive physiology in our primary perennial pasture species. Little is known about the establishment and management requirements of forage shrubs and trees. Woody forages depart from the norm in that they provide an additional forage resource with quite different plant functional traits. This is achieved by exploiting the vertical dimension to the under-utilized resources available in grassland systems—light and space above-ground and soil moisture and nutrients below-ground (Dodd et al., 1998).
While there is a substantial body of literature exploring the dynamics of these two approaches as components of a farm system, there is less consideration of their impact on the whole system. However, some relevant points have been made. Deferred grazing could be used to maintain pasture quality over the whole farm in sheep, beef and dairy systems throughout New Zealand, by removing a proportion of the paddocks from the grazing round and thus increasing grazing pressure on the remaining paddocks. This generally coincides with the spring surplus when there is feed in excess to requirements, which then becomes a challenge in terms of maintaining feed quality for young livestock (Sheath and Boom, 1985). This was demonstrated in East Coast North Island hill country by Suckling (1959), who found that deferring a proportion of the farm enabled the remainder of the feed to be adequately controlled. The utilization of the deferred pastures at the end of summer also allows the covers to increase on the remainder of the farm. This assists in filling the late autumn / winter feed gap when pasture growth rates are often low and there is insufficient pasture available to meet livestock demand. Livestock generally find it more difficult to remove poor quality herbage from steep than easy country. For this reason Sheath et al. (1984) recommend that priority be given to “control of steep land during late spring-early summer, because of likely longer-term benefits in pasture composition, density and production.” Deferred grazing can assist in controlling pastures on steep hill country farms. By deferring some paddocks and reducing the area available for grazing, the stocking rate is effectively increased on the rest of the farm. This enables the feed supply to better match feed demand and pasture quality to be maintained.
In dairy systems, a short deferred period, in which L. perenne-based pastures were allowed to flower followed by hard grazing by dairy cattle, led to increased DM production in the spring during the deferred period by 24% and the summer-autumn following the deferred period by 22%. This resulted in increased milk solids production of about 11.5% per cow (Da Silva et al., 2004). Deferred grazing has been found to be more profitable than a traditional hay/silage system (McCallum et al., 1991), although these authors suggested that no more than 10% of the dairy farm should be deferred in a year. This method of storing and making available standing feed also lowers the carbon footprint by reducing or eliminating the need for machinery and other energy inputs that are required to make hay or silage.
The benefits of these two approaches have been outlined in sections Deferred Grazing and Woody Species and Their Uses in Farming Systems, so why have they not been adopted more widely by New Zealand pastoral farmers? As highlighted, one of the most remarkable features of the New Zealand landscape is the enormous range in environmental diversity within small spatial extents–from soil type, topography and climatic points of view. Thus, it might seem strange that the management of our grazing systems seems to focus on achieving a limited variety of vegetation communities and livestock enterprises.
Part of the reason has to do with the self-identity of New Zealand grassland farmers, who have strong cultural values tied to pasture management and animal husbandry, in the context of a long-held “productivist orientation” (Rosin, 2013). This has been supported by historically high levels of central government investment in relevant research and development (Jacobsen and Scobie, 1999) and the existence of influential supporting organizations such as DairyNZ and non-government organizations (e.g., NZ Grassland Association, NZ Society of Animal Production). Central government subsidies have historically also been important, in that land development grants and minimum price support aimed at increasing foreign exchange earnings (Le Heron and Roche, 1999) were critical in establishing the dominant pastoral vegetation structure as the natural capital base across all types of topography. Maintaining this vegetation structure is predicated on ongoing low-cost inputs of fossil fuel, phosphatic fertilizer and relatively high stocking rates, in an environment that would naturally revert to woody vegetation, including a range of undesirable plants (Bergin et al., 1995; Williams, 2011).
As noted previously, there has historically been strong emphasis on the efficiency of forage utilization in grazing systems. This has been supported by a wealth of research into grazing management and both animal and plant genetic improvement. The two approaches we have discussed inevitably challenge that focus. Deferred grazing at first glance creates the impression of unutilized feed and the development of low quality pasture, while woody forages at first glance creates the impression of lost grazing opportunity during relatively slow establishment, shading of productive pasture and providing a low quality feed (Dodd et al., 2005). In addition, the combination of two forage resources with differing optimal management creates a problem for free-range animal systems.
Overcoming these first impressions, be they real or imagined, is the role of research. However, there are strong causal loop processes operating within the research community that tend to positively reinforce investment in improvements to existing systems (where the value proposition for research is readily quantifiable) and negatively suppress investments in understanding the dynamics of alternative systems (Turner et al., 2016). Hence, the quantum of research and extension activity in components such as deferred grazing and woody forages is orders of magnitude lower than that in conventional pasture management.
In addition, the generation of new understanding through research is likely insufficient to achieve wider uptake. Extension research has highlighted a range of barriers to the uptake of new technologies in agricultural contexts, including issues such as complexity, compatibility with identity and objectives, flexibility, value add, capital outlay, uncertainty, and physical and social infrastructure requirements (Vanclay, 2004). Local studies confirm the role of cost, perceptions of value add, complexity, learning requirements and compatibility with objectives as barriers to the uptake of environmental management practices (e.g., van Reenen, 2012). Farmer adoption of new technologies and practices, and acceptance of risk are linked to age demographics (Brown et al., 2019), thus responsiveness to wider societal expectations will be a generational process. In New Zealand one of the most powerful pathways to adoption is exemplars—farmers follow closely what other farmers are doing and learn rapidly from their peers (both in terms of what to try and what to avoid).
Acknowledging that, key questions for research to answer in gaining a better understanding of these alternative approaches include effects on pasture botanical composition and feed quality, secondary benefits for soil and water quality, biophysical pasture-tree interactions, optimal browse management, and long-term cost-benefit data. Development of supporting technologies out of the R&D community could include virtual fencing, low-cost tree protectors and drones to assist in the establishment of tree seedlings in difficult to access hill country. These enable much more spatially explicit control of grazing to: (1) define deferred areas without additional fencing; (2) ensure livestock graze deferred areas upon re-opening; (3) protect establishing shrubs while utilizing understory pasture; and (4) separate the timing of shrub browsing and interstitial pasture grazing.
Beyond answering component questions outlined above, how would these fit into existing grazing systems?
Deferred grazing is easy to implement, with no capital outlay, and can be implemented anywhere on-farm. The main considerations will be an assessment of which paddocks have an appropriate threshold of desirable species and will benefit most, in terms of improving sown species content, and how to overcome the short-term feed supply/demand imbalance (McCallum et al., 1991). Also, the timing of the deferred grazing period (i.e., opening and closing the gate) is critical and requires an understanding of the lifecycle of desirable and weedy species. In most New Zealand hill country environments, the late spring/early summer period has been characterized by high pasture growth rates and a burgeoning feed surplus (Radcliffe, 1982), hence removing paddocks from grazing is a sensible tactic for improving the level of grazing control on the rest of the farm. However, many intensive systems are now stocked to levels where demand exceeds supply year-round, with the shortfall made up from what used to be known as supplementary feed (Clark et al., 2007). Hence, the short-term loss of pasture-based supply must be made up from additional bought-in feed, or lower production levels accepted. Therefore, in intensive systems, the decision around what area to defer will be a trade-off of the long-term pasture quality benefit and the short-term cost.
Browse shrubs are somewhat more challenging, both in terms of the new knowledge around species selection, establishment and management at the component level, and at the systems level such issues as the additional equipment potentially needed, an understanding of optimal locations for implementation within the system, animal health and welfare implications. Similarly, the question of how much area to devote to browse shrubs applies, which could be addressed by modeling. While component-based models of the interactions between woody plants and understory forage exist, these are not incorporated into the current suite of mainstream farm systems models in New Zealand. However, such models have been developed overseas (e.g., Monjardino et al., 2010).
Ultimately, greater uptake of these two alternative landscape components in hill country grazing systems will be a function of how well they can be shown to deliver to a range of secondary objectives within farm systems (water quality, soil structural stability, landscape and species diversity) while simultaneously maintaining or enhancing the value from livestock enterprises, via feed quality, pasture persistence, feed supply and animal welfare. In other terms, how they can enhance the quantum and balance of all relevant ecosystem services.
Recommendations
On the basis of the discussion in this paper, we offer the following recommendations for research and practice for achieving a step-change in the management of New Zealand's hill country forage base consistent with the concept of increasing the diversity of landscape structure and function.
1. Additional research into the environmental benefits of deferred grazing practices, specifically the reduction of sediment and nutrient losses to water resources through runoff and leaching.
2. More widespread adoption of deferred grazing as a tool to control pasture quality over the whole farm and to increase pasture resilience—particularly where pastures are subjected to multiple and simultaneous stresses (such as drought, invertebrate pest predation and overgrazing).
3. More widespread adoption of proven exotic woody species as multifunctional plants—providing forage, soil structural stability, shade and shelter (and potentially additional biological N-fixation), and other ecosystem services (e.g., vista enhancement, increased carbon sequestration, pollination, nectar source for birds, habitat for invertebrates and birds).
4. Additional research effort into the benefits of woody shrubs for animal welfare and reduction of sediment delivery to waterways.
5. Additional research effort into the potential use of native woody plants in similar ways to those acknowledged and proposed for exotic species.
6. Development of models to explore the interactions between woody plants and understory forages and their impact on New Zealand hill country farm systems.
Author Contributions
KT, GD, MD, and KM: design, writing of sections, reviewing, editing, and proofing. All authors contributed to the article and approved the submitted version.
Conflict of Interest
The authors declare that the research was conducted in the absence of any commercial or financial relationships that could be construed as a potential conflict of interest.
Acknowledgments
Thanks to Ian McIvor, Cory Matthews, Cara Brosnahan and the reviewers for their helpful comments on the manuscript and to the Ministry of Primary Industries (Sustainable Farming Fund Projects 405641 and 405264) for funding the publication of this paper.
References
Alberda, T. (1957). Water-Soluble Carbohydrate Content of Free Developing Plants of Perennial Ryegrass. Jaarboek: Instituut voor biologisch en scheikundig Onderzoek van Landbouwgewassen.
Allen, R. B., Payton, I. J., and Knowlton, J. E. (1984). Effects of ungulates on structure and species composition in the Urewera forests as shown by exclosures. N. Z. J. Ecol. 7, 119–130.
Anon (2008). Toxicity of Black Locust. Available Online at : http://www.woodweb.com/knowledge_base/Toxicity_of_Black_Locust.html (accessed December 16, 2019).
Anon (2014). Info 30 - Poisonous Plants. Available online at: https://studyres.com/doc/7778237/info-30—poisonous-plants# (accessed January 20, 2021).
Anon (2015). Water Quality in New Zealand: Land Use and Nutrient Pollution. New Zealand: Wellington.
Anon (2016). Climate Change and Agriculture: Understanding the Biological Greenhouse Gases. New Zealand: Wellington.
Anon (2018). Agricultural and Horticultural Land Use. Stats NZ, New Zealand Government. Available online at: http://archive.stats.govt.nz/browse_for_stats/environment/environmental-reporting-series/environmental-indicators/Home/Land/land-use.aspx (accessed February 10, 2020).
Anon (2019b). Gleditsia triacanthos (honey locust). CAB International. Available online at: https://www.cabi.org/isc/datasheet/25272 (accessed January 30, 2020).
Anon (2020a). Coprosma repens: taupata. Available online ar: https://www.southernwoods.co.nz/shop/coprosma-taupatu-coprosma/ (accessed January 17, 2020).
Anon (2020b). Cordyline australis: Cabbage Tree - Ti Kouka. Available online at: https://www.southernwoods.co.nz/shop/cordyline-australis/ (accessed February 20, 2020).
Anon (2020c). Griselinia littoralis: Broadleaf-kapuka. Available online at: https://www.southernwoods.co.nz/shop/griselinia-littoralis/ (accessed January 13, 2020).
Anon (2020d). Hoheria populnea: houhere - lacebark. Available online at: https://www.southernwoods.co.nz/shop/hoheria-populnea/ (accessed January 14, 2020).
Anon (2020e). Pseudopanax arboreus: NZ Five Finger. Available online at: https://www.southernwoods.co.nz/shop/pseudopanax-arboreus/ (accessed January 14, 2020).
Baertsche, S. R., Yokoyama, M. T., and Hanover, J. W. (1986). Short rotation, hardwood tree biomass as potential ruminant feed-chemical composition, nylon bag ruminal degradation and ensilement of selected species. J. Anim. Sci. 63, 2028–2043. doi: 10.2527/jas1986.6362028x
Baldrian, P., Merhautová, V., Petránková, M., Cajthaml, T., and Šnajdr, J. (2010). Distribution of microbial biomass and activity of extracellular enzymes in a hardwood forest soil reflect soil moisture content. Appl. Soil Ecol. 46, 177–182. doi: 10.1016/j.apsoil.2010.08.013
Bannister, P., Colhoun, C. M., and Jameson, P. E. (1995). The winter hardening and foliar frost resistance of some New Zealand species of Pittosporum. NZ. J. Bot. 33, 409–414. doi: 10.1080/0028825X.1995.10412967
Bannister, P., and Lee, W. G. (1989). The frost resistance of fruits and leaves of some Coprosma species in relation to altitude and habitat. NZ. J. Bot. 27, 477–479. doi: 10.1080/0028825X.1989.10414128
Barker, D. J., Lancashire, J. A., Moloney, S. C., Dymock, N., Stevens, D. R., and Turner, J. D. (1993). Introduction, production, and persistence of five grass species in dry hill country 8. Summary and conclusions. NZ. J. Agric. Res. 36, 61–66. doi: 10.1080/00288233.1993.10427486
Barrett, R. P., Mebrahtu, T., and Hanover, J. W. (1990). “Black locust: a multi-purpose tree species for temperate climates,” in Advances in New Crops, eds. J. Janick and J. E. Simon. (Portland, OR: Timber Press), 278–283.
Bascand, L. D., and Jowett, G. H. (1982). Scrubweed cover of South Island agricultural and pastoral land 2. Plant distribution and managerial problem status. NZ. J. Exp. Agric. 10, 455–492. doi: 10.1080/03015521.1982.10427919
Bee, J. N., Kunstler, G., and Coomes, D. A. (2007). Resistance and resilience of New Zealand tree species to browsing. J. Ecol. 95, 1014–1026. doi: 10.1111/j.1365-2745.2007.01261.x
Bee, J. N., Tanentzap, A. J., Lee, W. G., Lavers, R. B., Mark, A. F., Mills, J. A., et al. (2011). Influence of foliar traits on forage selection by introduced red deer in New Zealand. Basic Appl. Ecol. 12, 56–63. doi: 10.1016/j.baae.2010.09.010
Bell, L., Ryan, M., Ewing, M., and Moore, G. (2008). Prospects for three Dorycnium species as forage plants in agricultural systems: a review of their agronomic characteristics. Aust. J. Exp. Agric. 48, 467–479. doi: 10.1071/EA07109
Benavides, R., Douglas, G. B., and Osoro, K. (2009). Silvopastoralism in New Zealand: review of effects of evergreen and deciduous trees on pasture dynamics. Agroforestry Syst. 76, 327–350. doi: 10.1007/s10457-008-9186-6
Bergin, D. O., Kimberley, M. O., and Marden, M. (1995). Protective value of regenerating tea tree stands on erosion-prone hill country, East Coast, North Island, New Zealand. NZ. J. Forestry Sci. 25, 3–19.
Bishop-Hurley, G. J. (1999). An evaluation of a dairy systems study of the effects of contrasting spring grazing managements on pasture and animal performance: a thesis submitted in partial fulfilment of the requirements for the degree of Doctor of Philosophy (Ph.D.), Pastures and Crops Group, Institute of Natural Resources, College of Sciences, Massey University, Palmerston North, New Zealand.
Bishop-Hurley, G. J., Matthews, P. N. P., Hodgson, J., Dake, C., and Matthew, C. (1997). Dairy systems study of the effects of contrasting spring grazing managements on pasture and animal production. Proc. NZ. Grassland Association 59, 209–214. doi: 10.33584/jnzg.1997.59.2243
Blackwell, G., Fukuda, Y., Maegli, T., and MacLeod, C. J. (2008). Room for everyone? Refugia and native biodiversity in New Zealand's agricultural landscapes. NZ. J. Agric. Res. 51, 473–476. doi: 10.1080/00288230809510478
Blair, R. M. (1990). “Gleditsia triacanthos L. Honeylocust,” in Silvics of North America Volume 2. Hardwoods, eds. R.M. Burns and B.H. Honkala (Washington, DC: United States Department of Agriculture Forest Service), 358–364.
Borens, F. M. P., and Poppi, D. P. (1990). The nutritive value for ruminants of tagasaste (Chamaecytisus palmensis), a leguminous tree. Anim. Feed Sci. Technol. 28, 275–292. doi: 10.1016/0377-8401(90)90159-6
Bourdôt, G. W., Fowler, S. V., Edwards, G. R., Kriticos, D. J., Kean, J. M., Rahman, A., et al. (2007). Pastoral weeds in New Zealand: status and potential solutions. NZ. J. Agric. Res. 50, 139–161. doi: 10.1080/00288230709510288
Bowman, D. C., Devitt, D. A., Engelke, M. C., and Rufty, T. W. (1998). Root architecture affects nitrate leaching from bentgrass turf. Crop Sci. 38, 1633–1639. doi: 10.2135/cropsci1998.0011183X003800060036x
Brock, J. L., and Hay, R. J. M. (1993). “An ecological approach to forage management”, in Proceedings of the XVII International Grassland Congress. ed. M.J. Baker (Palmerston North, New Zealand: New Zealand Grassland Association et al.).
Brougham, R. W. (1960). The effects of frequent hard grazings at different times of the year on the productivity and species yields of a grass-clover pasture. NZ. J. Agric. Res. 3, 125–136. doi: 10.1080/00288233.1960.10419866
Brown, P., Daigneault, A., and Dawson, J. (2019). Age, values, farming objectives, past management decisions, and future intentions in New Zealand agriculture. J. Environ. Manage. 231, 110–120. doi: 10.1016/j.jenvman.2018.10.018
Bull, P., Jackson, D. R., and Bedford, T. (1985). Edible Tree Nuts in New Zealand. Wellington: Government Publishing New Zealand.
Burns, B. R., Floyd, C. G., Smale, M. C., and Arnold, G. C. (2011). Effects of forest fragment management on vegetation condition and maintenance of canopy composition in a New Zealand pastoral landscape. Austral Ecol. 36, 153–166. doi: 10.1111/j.1442-9993.2010.02130.x
Butz Huryn, V. M. (1995). Use of native New Zealand plants by honey bees (Apis mellifera L.): a review. NZ. J. Bot. 33, 497–512. doi: 10.1080/0028825X.1995.10410621
Buxton, R., Timmins, S., Burrows, L., and Wardle, P. (2001). Impact of cattle on Department of Conservation grazing leases in South Westland: results from monitoring 1989-99, and recommendations. Sci. Conserv. 179, 5–31.
Cairns, I., Handyside, B., Harris, M., Lambrechtsen, N., and Ngapo, N. (2001). Soil Conservation Technical Handbook. Wellington: New Zealand Association of Resource Management.
Campbell, D. J. (1984). The vascular flora of the DSIR study area lower Orongorongo Valley, Wellington, New Zealand. NZ. J. Bot. 22, 223–270. doi: 10.1080/0028825X.1984.10425254
Charlton, J. F. L., Douglas, G. B., Wills, B. J., and Prebble, J. E. (2003). “Farmer experience with tree fodder”, in Tree Fodder Workshop: Using Trees on Farms as Supplementary Fodder and for Other Purposes. ed. J. F. L. Charlton. (Palmerston North: Grassland Research and Practice Series).
Clark, D. A., Caradus, J. R., Monaghan, R. M., Sharp, P., and Thorrold, B. S. (2007). Issues and options for future dairy farming in New Zealand. NZ. J. Agric. Res. 50, 203–211. doi: 10.1080/00288230709510291
Clothier, S. (2019). 4 Native Trees to Use for Fodder in New Zealand. Lifestyle Magazine Group. Available online at: https://thisnzlife.co.nz/4-native-trees-to-use-for-fodder-in-new-zealand/ (accessed December 16, 2019).
Colvill, K. E., and Marshall, C. (1984). Tiller dynamics and assimilate partitioning in Lolium perenne with particular reference to flowering. Annals Appl. Biol. 104, 543–557. doi: 10.1111/j.1744-7348.1984.tb03038.x
Cope, R., and Parton, K. (2012). “Poisonous plants of Australia and New Zealand,” in Veterinary Toxicology: Basic and Clinical Principles, ed. C.G. Gupta. 2nd ed (London: Academic Press, Elsevier), 1095–1112. doi: 10.1016/B978-0-12-385926-6.00101-0
Czerepowicz, L., Case, B., and Doscher, C. (2012). Using satellite image data to estimate aboveground shelterbelt carbon stocks across an agricultural landscape. Agric. Ecosyst. Environ. 156, 142–150. doi: 10.1016/j.agee.2012.05.014
Da Silva, S. C., Hodgson, J., Matthew, C., Matthews, P. N. P., and Holmes, C. W. (2004). Herbage production and animal performance on perennial ryegrass/white clover dairy pastures under alternative spring grazing managements. J. Agric. Sci. 142, 97–108. doi: 10.1017/S0021859604003946
Daly, M. J., Fraser, T., Perkins, A., and Moffat, C. M. (1999). Farmer perceptions of reasons for perennial pasture persistence and the relationship of these with management practice, species composition, and soil fertility. Proc. NZ. Grassland Association 61, 9–15. doi: 10.33584/jnzg.1999.61.2348
Danckwerts, J., and Gordon, A. (1987). Long-term partitioning, storage and re-mobilization of 14C assimilated by Lolium perenne (cv. Melle). Annals Bot. 59, 55–66. doi: 10.1093/oxfordjournals.aob.a087285
Davies, D. J. G. (1985a). “Overview: the role of fodder trees,” in Fodder Trees: A Summary of Current Research in New Zealand, eds. L.A. Logan and J.E. Radcliffe (Christchurch: Crop Research Division, DSIR), 7–8.
Davies, D. J. G. (1985b). “Results of tagasaste trials and plantings of fruit, nut and pod-bearing trees from 1977-85 at Lincoln,” in Fodder Trees: A Summary of Current Research in New Zealand, eds. L.A. Logan and J.E. Radcliffe (Christchurch: Crop Research Division, DSIR), 26–33.
Davies, D. J. G., and Macfarlane, R. P. (1979). Multiple-purpose trees for pastoral farming in New Zealand: with emphasis on tree legumes. NZ. Agric. Sci. 13, 177–186.
Davies-Colley, R. J. (2013). “River water quality in New Zealand: an introduction and overview,” in Ecosystem Services in New Zealand - Conditions and Trends, ed. J.R. Dymond. (Lincoln: Manaaki Whenua Press), 432–447.
de Lange, P.J. (2021). Coprosma robusta Fact Sheet (content continuously updated). New Zealand Plant Conservation Network. Available online at: https://www.nzpcn.org.nz/flora/species/coprosma-robusta/ (accessed January 20, 2021).
Dodd, M., Chapman, D., Ludemann, C., Griffiths, W., Tozer, K., and Donnelly, L. (2018). The measurement of perennial ryegrass persistence. J. NZ. Grasslands 80, 161–169. doi: 10.33584/jnzg.2018.80.339
Dodd, M. B., Barker, D. J., and Wedderburn, M. E. (2004). Plant diversity effects on herbage production and compositional changes in New Zealand hill country pastures. Grass Forage Sci. 59, 29–40. doi: 10.1111/j.1365-2494.2004.00402.x
Dodd, M. B., Lauenroth, W. K., and Welker, J. M. (1998). Differential water resource use by herbaceous and woody plant life-forms in a shortgrass steppe community. Oecol. Aquatica 117, 504–512. doi: 10.1007/s004420050686
Dodd, M. B., and Mackay, A. D. (2011). Effects of contrasting soil fertility on root mass, root growth, root decomposition and soil carbon under a New Zealand perennial ryegrass/white clover pasture. Plant Soil 349, 291–302. doi: 10.1007/s11104-011-0873-0
Dodd, M. B., McDowell, R. W., and Quinn, J. M. (2016). A review of contaminant losses to water from pastoral hill lands and mitigation options. Hill Country Grassland Res. Pract. Series 16, 137–148.
Dodd, M. B., McGowan, A. W., Power, I. L., and Thorrold, B. S. (2005). Effects of variation in shade level, shade duration and light quality on perennial pastures. NZ. J. Agric. Res. 48, 531–543. doi: 10.1080/00288233.2005.9513686
Dodd, M. B., Quinn, J. M., Thorrold, B. S., Parminter, T. G., and Wedderburn, M. E. (2008). Improving the economic and environmental performance of a New Zealand hill country farm catchment: 3. Short-term outcomes of land-use change. NZ. J. Agric. Res. 51, 155–169. doi: 10.1080/00288230809510444
Dodd, M. B., and Ritchie, H. (2007). Farming With Native Trees: A Guide for Farmers From Northland to Waikato. Rotorua: Ensis.
Doerr, S. H., and Thomas, A. D. (2003). “Soil moisture: a controlling factor in water repellency,” in Soil Water Repellency - Occurrence, Consequences and Amelioration, eds. C.J. Ritsema & L.W. Dekker (Amsterdam: Elsevier), 137–151. doi: 10.1016/B978-0-444-51269-7.50016-3
Donaghy, D. J., and Fulkerson, W. J. (1998). Priority for allocation of water-soluble carbohydrate reserves during regrowth of Lolium perenne. Grass Forage Sci. 53, 211–218. doi: 10.1046/j.1365-2494.1998.00129.x
Douglas, G., Mackay, A., Vibart, R., Dodd, M., McIvor, I., and McKenzie, C. (2020). Soil carbon stocks under grazed pasture and pasture-tree systems. Sci. Total Environ. 715:136910. doi: 10.1016/j.scitotenv.2020.136910
Douglas, G., McIvor, I., Manderson, A., Koolaard, J., Todd, M., and Braaksma, S. (2013a). Reducing shallow landslide occurrence in pastoral hill country using wide-spaced trees. Land Degradation Dev. 24, 103–114. doi: 10.1002/ldr.1106
Douglas, G. B., Barry, T. N., Faulknor, N. A., Kemp, P. D., Foote, A. G., Cameron, P. N., et al. (2003). Willow coppice and browse blocks: establishment and management. Grassland Res. Pract. Series 10, 41–51.
Douglas, G. B., Bulloch, B. T., and Foote, A. G. (1996a). Cutting management of willows (Salix spp.) and leguminous shrubs for forage during summer. NZ. J. Agric. Res. 39, 175–184. doi: 10.1080/00288233.1996.9513176
Douglas, G. B., Gadgil, R. L., Ede, F. J., Kimberley, M. O., Sandberg, A. M., Lowe, A. T., et al. (2004). Relative performance of 18 nitrogen-fixing plant species at three unstable coastal sand dune sites in New Zealand. NZ. J. Forestry Sci. 34, 219–237.
Douglas, G. B., Mackay, A. D., Dodd, M. B., Lloyd-West, C. M., and Gray, R. A. J. (2015). Factors determining shrub abundance on uncultivable hill country. J. NZ. Grasslands 77, 239–244. doi: 10.33584/jnzg.2015.77.454
Douglas, G. B., Wall, A. J., Dodd, M. B., Hawke, M. F., and McIvor, I. R. (2013b). “Balancing pastoral and plantation forestry options in New Zealand and the role of agroforestry”, in XXII International Grassland Congress (Sydney: New South Wales Department of Primary Industry).
Douglas, G. B., Wills, B. J., Pryor, H. N., Foote, A. G., and Trainor, K. D. (1996b). Establishment of perennial legume species in drought-prone North and South Island sites. Proc. NZ. Grassland Assoc. 58, 253–257. doi: 10.33584/jnzg.1996.58.2199
Douglas, G. B., Woodfield, D. R., and Foote, A. G. (1998). Elite selection of tagasaste (Chamaecytisus palmensis) for drought-prone sites. Proc. NZ. Grassland Association 60, 181–186. doi: 10.33584/jnzg.1998.60.2299
Dowling, P. M., Michalk, D. L., Kemp, D. R., Millar, G. D., Priest, S. M., King, W. M., et al. (2006). Sustainable grazing systems for the Central Tablelands of New South Wales. 2. Effect of pasture type and grazing management on pasture productivity and composition. Aust. J. Exp. Agric. 46, 457–469. doi: 10.1071/EA04040
Duchini, P. G., Guzatti, G. C., Echeverria, J. R., Américo, L. F., and Sbrissia, A. F. (2018). Experimental evidence that the perennial grass persistence pathway is linked to plant growth strategy. PLoS ONE 13:e0207360. doi: 10.1371/journal.pone.0207360
Dunbabin, V., Diggle, A., and Rengel, Z. (2003). Is there an optimal root architecture for nitrate capture in leaching environments? Plant Cell Environ. 26, 835–844. doi: 10.1046/j.1365-3040.2003.01015.x
Durand, J. L., Bariac, T., Ghesquière, M., Biron, P., Richard, P., Humphreys, M., et al. (2007). Ranking of the depth of water extraction by individual grass plants, using natural 18O isotope abundance. Environ. Exp. Bot. 60, 137–144. doi: 10.1016/j.envexpbot.2006.09.004
Dymond, J. R., Ausseil, A. G., Kirschbaum, M. U. F., Carswell, F. E., and Mason, N. W. H. (2013). Opportunities for restoring indigenous forest in New Zealand. J. R. Soc. N. Z. 43, 141–153. doi: 10.1080/03036758.2012.736393
Edwards, G. R., and Chapman, D. F. (2011). Plant responses to defoliation and relationships with pasture persistence. Pasture Persistence 15, 39–46.
ElNativoGrowers (2020). A Guide to Ceanothus (Wild Lilac). Available online at: https://elnativogrowers.com/a-guide-to-ceanothus-wild-lilac/ (accessed January 30, 2020).
El-Shatnawi, M. D. K. J., and Turuk, M. (2002). Dry matter accumulation and chemical content of saltbush (Atriplex halimus) grown in Mediterranean desert shrublands. NZ. J. Agric. Res. 45, 139–144. doi: 10.1080/00288233.2002.9513503
Forsyth, D. M., Coomes, D. A., Nugent, G., and Hall, G. M. J. (2002). Diet and diet preferences of introduced ungulates (Order: Artiodactyla) in New Zealand. NZ. J. Zool. 29, 323–343. doi: 10.1080/03014223.2002.9518316
Garay, A. H., Hodgson, J., and Matthew, C. (1997a). Effect of spring grazing management on perennial ryegrass and ryegrass-white clover pastures. 1. Tissue turnover and herbage accumulation. NZ. J. Agric. Res. 40, 25–35. doi: 10.1080/00288233.1997.9513227
Garay, A. H., Matthew, C., and Hodgson, J. (1997b). Effect of spring grazing management on perennial ryegrass and ryegrass-white clover pastures. 2. Tiller and growing point densities and population dynamics. NZ. J. Agric. Res. 40, 37–50. doi: 10.1080/00288233.1997.9513228
Garden, D. L., Lodge, G. M., Friend, D. A., Dowling, P. M., and Orchard, B. A. (2000). Effects of grazing management on botanical composition of native grass-based pastures in temperate south-east Australia. Aust. J. Exp. Agric. 40, 225–245. doi: 10.1071/EA98010
Gillingham, A., and Gray, M. (2006). Measurement and modelling of runoff and phosphate movement from seasonally dry hill-country pastures. NZ. J. Agric. Res. 49:233–245. doi: 10.1080/00288233.2006.9513714
Gold, M. A., and Hanover, J. W. (1993). Honeylocust (Gleditsia triacanthos), a multi-purpose tree for the temperate zone. Int. Tree Crops J. 7, 189–207. doi: 10.1080/01435698.1993.9752919
Gregory, N. G. (1995). The role of shelterbelts in protecting livestock: a review. NZ. J. Agric. Res. 38, 423–450. doi: 10.1080/00288233.1995.9513146
Griffiths, G. J. K., Holland, J. M., Bailey, A., and Thomas, M. B. (2008). Efficacy and economics of shelter habitats for conservation biological control. Biol. Control 45, 200–209. doi: 10.1016/j.biocontrol.2007.09.002
Hahner, J. L., Robinson, B. H., Hong-Tao, Z., and Dickinson, N. M. (2014). The phytoremediation potential of native plants on New Zealand dairy farms. Int. J. Phytoremediation 16, 719–734. doi: 10.1080/15226514.2013.856845
Halligan, E., Forde, M., and Warrington, I. (1993). Discrimination of ryegrasses by heading date under various combinations of vernalization and daylength: perennial ryegrass varieties. Plant Varieties Seeds. 61, 51–160.
Halliwell, G. (1979). A guide to tree forage crops. New Zealand Tree Crops Association. Available online at: https://treecrops.org.nz/crops/fodder-and-forage/forage/ (accessed January 14, 2020).
Hampton, J. G., Clemence, T. G. A., and Hebblethwaite, P. D. (1987). The effect of lodging on 14C-assimilate distribution after anthesis in Lolium perenne, CV. S24, grown for seed. Grass Forage Sci. 42, 121–127. doi: 10.1111/j.1365-2494.1987.tb02098.x
Harris, W., Beever, R. E., Parkes, S., Webster, R., and Scheele, S. (2003). Genotypic variation of height growth and trunk diameter of Cordyline australis (Lomandraceae) grown at three locations in New Zealand. NZ. J. Bot. 41, 637–653. doi: 10.1080/0028825X.2003.9512875
Harris, W., Beever, R. E., and Smallfield, B. (2001). Variation in response to cold damage by populations of Cordyline australis and of some other species of Cordyline (Lomandraceae). NZ. J. Bot. 39, 147–159. doi: 10.1080/0028825X.2001.9512723
Harris, W., and Mann, J. D. (1994). Preliminary investigation of the suitability of Cordyline australis (Asphodelaceae) as a crop for fructose production. NZ. J. Crop Hortic. Sci. 22, 439–451. doi: 10.1080/01140671.1994.9513856
Hawke, M. F., and Dodd, M. B. (2003). Livestock shelter from trees – a review. Grassland Res. Pract. Series 10, 65−72.
Hawke, M. F., and O'Connor, M. B. (1993). Soil pH and nutrient levels at Tiketere agroforestry research area. NZ. J. Forestry Sci. 23, 40–48.
Heenan, P. B., Breitwieser, I., Glenny, D., De Lange, P. J., and Brownsey, P. J. (1998). Checklist of dicotyledons and pteridophytes naturalised or casual in New Zealand: additional records 1994–1996. NZ. J. Bot. 36, 155–162. doi: 10.1080/0028825X.1998.9512556
Hermansen, C., Moldrup, P., Müller, K., Jensen, P. W., van den Dijssel, C., Jeyakumar, P., et al. (2019). Organic carbon content controls the severity of water repellency and the critical moisture level across New Zealand pasture soils. Geoderma 338, 281–290. doi: 10.1016/j.geoderma.2018.12.007
Heuzé, V., Tran, G., Hassoun, P., and Lebas, F. (2019). 'Saltbush (Atriplex halimus)' [Online]. Feedipedia, a programme by INRA, CIRAD, AFZ and FAO. Available online at: https://www.feedipedia.org/node/24708 (accessed 31 January 31, 2020).
Hicks, D. L. (1995). Control of Soil Erosion on Farmland: A Summary of Erosion's Impact on New Zealand Agriculture, and Farm Management Practices Which Counteract it. Wellington: MAF Policy, Ministry of Agriculture.
Holling, C. S. (1996). “Engineering vs ecological resilience,” in Engineering within Ecological constraints, ed. P.C. Schulze (Washington, DC: National Academy of Science), 31–44.
Hoogendoorn, C. J., Bowatte, S., and Tillman, R. W. (2011). Simple models of carbon and nitrogen cycling in New Zealand hill country pastures: exploring impacts of intensification on soil C and N pools. NZ. J. Agric. Res. 51, 221–249. doi: 10.1080/00288233.2011.599395
Hughes, A. O. (2016). Riparian management and stream bank erosion in New Zealand. N. Z. J. Mar. Freshwater Res. 50, 277–290. doi: 10.1080/00288330.2015.1116449
Hughey, K. F. D., Cullen, R., and Kerr, G. N. (2010). “A decade of public perceptions of the New Zealand Environment: a focus on water and its management”, in 61st New Zealand Association of Economists Conference. (Auckland: University of Auckland Business School: New Zealand Association of Economists and Law and Economics Association of New Zealand).
Hume, D. E., and Barker, D. J. (1991). Natural reseeding of five grass species in summer dry hill country. Proc. NZ. Grassland Association 53, 97–104. doi: 10.33584/jnzg.1991.53.1988
Hunt, L. A., and Brougham, R. W. (1967). Some changes in the structure of a perennial ryegrass sward frequently but leniently defoliated during the summer. NZ. J. Agric. Res. 10, 397–404. doi: 10.1080/00288233.1967.10426368
Hunt, W. F., and Easton, H. S. (1989). Fifty years of ryegrass research in New Zealand. Proc. NZ. Grassland Association 50, 1–23. doi: 10.33584/jnzg.1989.50.1876
Huntley, J. C. (1990). “Robinia pseudoacacia L. Black Locust,” in Silvics of North America Volume 2. Hardwoods, eds. R.M. Burns and B.H. Honkala (Washington, DC: United States Department of Agriculture Forest Service), 755–761.
Jacobsen, V., and Scobie, G. M. (1999). “Agricultural R&D policy in New Zealand,” in Paying for Agricultural Productivity, eds. J. Alston, P. Pardey, and V. Smith (Washington, DC: International Food Policy Research Institute), 247–275
Jewiss, O. R. (1972). Tillering in grasses—its significance and control. Grass Forage Sci. 27, 65–82. doi: 10.1111/j.1365-2494.1972.tb00689.x
Jones, T. H., and McIvor, I. (2013). Willows and poplars for drought mitigation. NZ. Tree Grower 12–14.
Katterer, T., Reichstein, M., Andren, O., and Lomander, A. (1998). Temperature dependence of organic matter decomposition: a critical review using literature data analyzed with different models. Biol. Fertil. Soils 27, 258–262. doi: 10.1007/s003740050430
Kemp, D. R., and Culvenor, R. A. (1994). Improving the grazing and drought tolerance of temperate perennial grasses. NZ. J. Agric. Res. 37, 365–378. doi: 10.1080/00288233.1994.9513074
Kemp, D. R., Michalk, D. L., Dowling, P. M., and Klein, T. A. (1997). “Analysis of pasture management practices within a pasture composition matrix model,” in: Proceedings of the 18th International Grasslands Congress. Section 22 (Winnipeg: International Grassland Congress).
Kemp, D. R., Michalk, D. L., and Virgona, J. M. (2000). Towards more sustainable pastures: lessons learnt. Aust. J. Exp. Agric. 40, 343–356. doi: 10.1071/EA99001
Kemp, P., and Lopez, I. (2016). Hill country pastures in the southern North Island of New Zealand: an overview. Grassland Res. Pract. Series 16, 289–297.
Kemp, P. D., Barry, T. N., and Douglas, G. B. (2003). Edible forage yield and nutritive value of poplar and willow. Grassland Res. Pract. Series 10, 53–63.
Kemp, P. D., Mackay, A. D., Matheson, L. A., and Timmins, M. E. (2001). The forage value of poplars and willow. Proc. NZ. Grassland Association 63, 115–119. doi: 10.33584/jnzg.2001.63.2444
King, W., Rennie, G., Devantier, B., and Hoogendoorn, C. (2016). Impacts of grazing management on hill country pastures: principles and practices. Grassland Res. Pract. Series 16, 203–212.
Kirschbaum, M. U. F. (1995). The temperature-dependence of soil organic matter decomposition, and the effect of global warming on soil organic-C storage. Soil Biol. Biochem. 27, 753–760. doi: 10.1016/0038-0717(94)00242-S
Korte, C. J. (1986). Tillering in ‘Grasslands Nui' perennial ryegrass swards 2. Seasonal pattern of tillering and age of flowering tillers with two mowing frequencies. NZ. J. Agric. Res. 29, 629–638. doi: 10.1080/00288233.1986.10430456
Korte, C. J., and Chu, A. C. P. (1983). Some effects of drought on perennial ryegrass swards. Proc. NZ. Grassland Association 44, 211–216. doi: 10.33584/jnzg.1983.44.1625
Korte, C. J., and Quilter, S. J. (1990). Effects of summer grazing and fertiliser on the clover content of hill country swards in the Gisborne region. Proc. NZ. Grassland Association 51, 113–117. doi: 10.33584/jnzg.1990.51.1927
Kriticos, D. J. (2012). Regional climate-matching to estimate current and future sources of biosecurity threats. Biol. Invasions 14, 1533–1544. doi: 10.1007/s10530-011-0033-8
Lambert, M. G., Jung, G. A., and Costall, D. A. (1989a). Forage shrubs in North Island hill country. 1. Forage production. NZ. J. Agric. Res. 32, 477–483. doi: 10.1080/00288233.1989.10417920
Lambert, M. G., Jung, G. A., Fletcher, R. H., Budding, P. J., and Costall, D. A. (1989b). Forage shrubs in North Island hill country. 2. Sheep and goat preferences. NZ. J. Agric. Res. 32, 485–490. doi: 10.1080/00288233.1989.10417921
Lambert, M. G., Jung, G. A., Harpster, H. W., Budding, P. J., and Wewala, G. S. (1989c). Forage shrubs in North Island hill country. 3. Forage digestibility. NZ. J. Agric. Res. 32, 491–497. doi: 10.1080/00288233.1989.10417922
Lambert, M. G., Jung, G. A., Harpster, H. W., and Lee, J. (1989d). Forage shrubs in North Island hill country. 4. Chemical composition and conclusions. NZ. J. Agric. Res. 32, 499–506. doi: 10.1080/00288233.1989.10417923
Lancashire, J., Harris, A., Armstrong, C., and Ryan, D. (1979). Perennial ryegrass cultivars. Proc. NZ. Grassland Association 40, 114–124. doi: 10.33584/jnzg.1978.40.1519
Lane, P., Davies, S., Hall, E., and Moore, G. (2004). Dorycnium species as alternatives to forages. RIRDC Publication No 04/159 RIRDC Project No UT-25A. Kingston, ACT: Rural Industries Research and Development Corporation.
Lane, P. M. S., Lee, S. A., and Willoughby, B. E. (2016). Hill country cropping with no land-based equipment. Hill Country Grassland Res. Pract. Series 16, 251–256.
Le Heron, R., and Roche, M. (1999). Rapid reregulation, agricultural restructuring and the reimaging of agriculture in New Zealand. Rural Sociol 64, 203–218. doi: 10.1111/j.1549-0831.1999.tb00014.x
Lee, J. M., Donaghy, D. J., Sathish, P., and Roche, J. R. (2009). Interaction between water-soluble carbohydrate reserves and defoliation severity on the regrowth of perennial ryegrass (Lolium perenne L.)-dominant swards. Grass Forage Sci. 64, 266–275. doi: 10.1111/j.1365-2494.2009.00692.x
Lee, J. M., Matthew, C., Thom, E. R., and Chapman, D. F. (2012). Perennial ryegrass breeding in New Zealand: a dairy industry perspective. Crop Pasture Sci. 63, 107–127. doi: 10.1071/CP11282
L'Huillier, P. J. (1987). Tiller appearance and death of Lolium perenne in mixed swards grazed by dairy cattle at two stocking rates. NZ. J. Agric. Res. 30, 15–22. doi: 10.1080/00288233.1987.10430472
L'Huillier, P. J., and Aislabie, D. W. (1987). Natural reseeding in perennial ryegrass/white clover dairy pastures. Proc. NZ. Grassland Association 49, 111–115. doi: 10.33584/jnzg.1988.49.1812
Litherland, A., Deighton, D., and Leathwick, D. (2009). An evaluation of anthelmintic properties, assessed using faecal nematode egg counts, of New Zealand native flax (Phormium tenax). N. Z. Vet. J. 56, 339–342. doi: 10.1080/00480169.2008.36857
MacCarter, L. E., and Gaynor, D. L. (1980). Gorse: a subject for biological control in New Zealand. NZ. J. Exp. Agri. 8, 321–330. doi: 10.1080/03015521.1980.10426282
Macdonald, K. A., Matthew, C., Glassey, C. B., and McLean, N. (2011). Dairy farm systems to aid persistence. Pasture Persistence 15, 199–210.
MacFarlane, A. E. T., Kelly, D., and Briskie, J. V. (2016). Introduced blackbirds and song thrushes: useful substitutes for lost mid-sized native frugivores, or weed vectors? N. Z. J. Ecol. 40, 80–87. doi: 10.20417/nzjecol.40.9
Mackay, A. D. (2008). Impacts of intensification of pastoral agriculture on soils: current and emerging challenges and implications for future land uses. N. Z. Vet. J. 56, 281–288. doi: 10.1080/00480169.2008.36848
Mackay, A. D., Budding, P. J., Tatez, K. R., Orchard, V. A., Hart, P. B. S., and Kettles, H. A. (1991). Pastoral fallow for improving low fertility hill country pastures. Proc. N. Z. Grassland Association 53, 209–213. doi: 10.33584/jnzg.1991.53.1979
Mantovani, D., Veste, M., and Freese, D. (2014a). Black locust (Robinia pseudoacacia L.) ecophysiological and morphological adaptations to drought and their consequence on biomass production and water-use efficiency. N. Z. J. Forestry Sci. 44:29. doi: 10.1186/s40490-014-0029-0
Mantovani, D., Veste, M., and Freese, D. (2014b). Effects of drought frequency on growth performance and transpiration of young black locust (Robinia pseudoacacia L.). Int. J. Forestry Res. 11. doi: 10.1155/2014/821891
Marden, M., Rowan, D., and Phillips, C. (2005). Stabilising characteristics of New Zealand indigenous riparian colonising plants. Plant Soil. 278, 95–105. doi: 10.1007/s11104-004-7598-2
Mason, N. W. H., Beets, P. N., Payton, I., Burrows, L., Holdaway, R. J., and Carswell, F. E. (2014). Individual-based allometric equations accurately measure carbon storage and sequestration in shrublands. Forests 5, 309–324. doi: 10.3390/f5020309
Matthew, C., Assuero, S. G., Black, C. K., and Sackville Hamilton, N. R. (2015). “Tiller dynamics of grazed swards,” in Grassland Ecophysiology and Grazing Ecology, eds. G. Lemaire, J. Hodgson, A. de Moraes, C. Carvalho, and C. Nabinger. (Wallingford: CABI International).
Matthew, C., Chu, A. C. P., Hodgson, J., and Mackay, A. D. (1991). Early summer pasture control: what suits the plant? Proc. N. Z. Grassland Association 53, 73–77. doi: 10.33584/jnzg.1991.53.2008
Matthew, C., Hernández Garay, A., and Hodgson, J. (1996). Making sense of the link between tiller density and pasture production. Proc. N. Z. Grassland Association 57, 83–87. doi: 10.33584/jnzg.1995.57.2190
Matthew, C., Hernandez-Garay, A., and Hodgson, J. (2000). “Late control” spring grazing management of perennial ryegrass swards: effect on sward structure and botanical composition. Agronomy N. Z. 30, 121–127.
Matthew, C., Mackay, A., and Chu, A. (1986). Techniques for measuring the root growth of a perennial ryegrass (Lolium perenne L.) dominant pasture under contrasting spring managements. Proc. Agronomy Soc. N. Z. 16, 59–64.
Matthew, C., Mackay, A., and Robin, A. (2016). Do phytomer turnover models of plant morphology describe perennial ryegrass root data from field swards? Agriculture 6:28. doi: 10.3390/agriculture6030028
McCabe, S. M., and Barry, T. N. (1988). Nutritive value of willow (Salix sp.) for sheep, goats and deer. J. Agric. Sci. 111, 1–9. doi: 10.1017/S0021859600082745
McCallum, D. A., Thomson, N. A., and Judd, T. G. (1991). Experiences with deferred grazing at the Taranaki Agricultural Research Station. Proc. NZ. Grassland Association 53, 79–83. doi: 10.33584/jnzg.1991.53.1996
McIvor, I., Cumming, H., and Hurst, S. (2005). “Response of four Salix species to soil water deficit,” in Proceedings of 35th NZ Agronomy Society (Agronomy Society of New Zealand), 35, 74–80.
McIvor, I., Douglas, G., Dymond, J., Eyles, G., and Marden, M. (2011). “Pastoral hill slope erosion in New Zealand and the role of poplar and willow trees in its reduction,” in Soil Erosion Issues in Agriculture, eds. D. Godone and S. Silvia. (InTech - Open Access Publisher), 257–278.
McWilliam, E. L., Barry, T. N., Lopez-Villalobos, N., Cameron, P. N., and Kemp, P. D. (2004). The effect of different levels of poplar (Populus) supplementation on the reproductive performance of ewes grazing low quality drought pasture during mating. Anim. Feed Sci. Technol. 115, 1–18. doi: 10.1016/j.anifeedsci.2004.03.006
McWilliam, E. L., Barry, T. N., López-Villalobos, N., Cameron, P. N., and Kemp, P. D. (2005). Effects of willow (Salix) supplementation for 31 and 63d on the reproductive performance of ewes grazing low quality drought pasture during mating. Anim. Feed Sci. Technol. 119, 87–106. doi: 10.1016/j.anifeedsci.2004.11.013
Monjardino, M., Revell, D., and Pannell, D. J. (2010). The potential contribution of forage shrubs to economic returns and environmental management in Australian dryland agricultural systems. Agric. Syst. 103, 187–197. doi: 10.1016/j.agsy.2009.12.007
Moore, K. M., Barry, T. N., Cameron, P. N., Lopez-Villalobos, N., and Cameron, D. J. (2003). Willow (Salix sp.) as a supplement for grazing cattle under drought conditions. Anim. Feed Sci. Technol. 104, 1–11. doi: 10.1016/S0377-8401(02)00326-7
Mori, A. S., Furukawa, T., and Sasaki, T. (2012). Response diversity determines the resilience of ecosystems to environmental change. Biol. Rev. 88, 349–364. doi: 10.1111/brv.12004
MPI (2019). New Zealand's forests [Online]. Ministry for Primary Industries. Available online at: https://www.mpi.govt.nz/news-and-resources/open-data-and-forecasting/forestry/new-zealands-forests/ (accessed February 10, 2020).
MPI (2020). Indigenous (native) forestry [Online]. New Zealand: Ministry for Primary Industries. Available online at: https://www.mpi.govt.nz/growing-and-harvesting/forestry/indigenous-forestry/ (accessed February 10, 2020).
Müller, K., Mason, K., Strozzi, A. G., Simpson, R., Komatsu, T., Kawamoto, K., et al. (2018). Runoff and nutrient loss from a water-repellent soil. Geoderma 322, 28–37. doi: 10.1016/j.geoderma.2018.02.019
Musonda, K., Barry, T. N., McWilliam, E. L., Lopez-Villalobos, N., and Pomroy, W. E. (2009). Grazing willow (Salix spp.) fodder blocks for increased reproductive rates and internal parasite control in mated hoggets. Anim. Feed Sci. Technol. 150, 46–61. doi: 10.1016/j.anifeedsci.2008.08.003
National Poplar and Willow Users Group (2007). Growing poplar and willow trees on farms. Guidelines for establishing and managing poplar and willow trees on farms: National Poplar and Willow Users Group. 72p.
Nie, Z. (2011). “Use of perennial grass in grazing systems of Southern Australia to adapt to a changing climate,” in Climate Change - Research and Technology for Adaptation and Mitigation, eds. J.A.A. Blanco and H. Kheradmand (London: IntechOpen), 431–452. doi: 10.5772/23949
Nie, Z., and Zollinger, R. (2008). “Impacts of strategic grazing on herbage accumulation and nutritive value of naturalised hill pasture,” in Proceedings of the XXI International Grassland Congress. (Hohhot: Guangdong People's Publishing House).
Nie, Z. N., Barker, D. J., Mackay, A. D., Valentine, I., and Hodgson, J. (1998). Influence of the timing and duration of pastoral fallowing and nitrogen fertiliser on pasture and white clover (Trifolium repens) growth in hill country. N. Z. J. Agric. Res. 41, 19–29. doi: 10.1080/00288233.1998.9513284
Nie, Z. N., Barker, D. J., Valentine, I., Mackay, A. D., and Hodgson, J. (1999). Changes in the population of germinated seed in a hill pasture during and after a pastoral fallow. Grass Forage Sci. 54, 127–136. doi: 10.1046/j.1365-2494.1999.00160.x
Nie, Z. N., Mackay, A. D., Valentine, I., Barker, D. J., and Hodgson, J. (1997). Influence of pastoral fallow on plant root growth and soil physical and chemical characteristics in a hill pasture. Plant Soil 197, 201–208. doi: 10.1023/A:1004249613045
Nie, Z. N., Valentine, I., Mackay, A. D., Barker, D. J., and Hodgson, J. (1996). Long-term effects of pastoral fallowing on the distribution and performance of white clover (Trifolium repens L.) in a hill country pasture. Grassland Res. Pract. Series 6, 75–78.
Nie, Z. N., and Zollinger, R. P. (2012). Impact of deferred grazing and fertilizer on plant population density, ground cover and soil moisture of native pastures in steep hill country of southern Australia. Grass Forage Sci. 67, 231–242. doi: 10.1111/j.1365-2494.2011.00838.x
Norton, D. A., Butt, J., and Bergin, D. O. (2018). Upscaling restoration of native biodiversity: a New Zealand perspective. Ecol. Manag. Restoration 19, 26–35. doi: 10.1111/emr.12316
Nurseries, B. (2020). Hoheria growing guide [Online]. Available online at: https://www.burncoose.co.uk/site/content.cfm?ref=Hoheria+Growing+Guide (accessed January 23, 2020).
Ong, C. K., Marshall, C., and Saoar, G. R. (1978). The physiology of tiller death in grasses. 2. Causes of tiller death in a grass sward. Grass Forage Sci. 33, 205–211. doi: 10.1111/j.1365-2494.1978.tb00821.x
Oppong, S. K., Kemp, P. D., Douglas, G. B., and Foote, A. G. (2001). Browse yield and nutritive value of two Salix species and Dorycnium rectum in New Zealand. Agroforestry Systems 51, 11–21. doi: 10.1023/A:1006412021394
Ortiz-Dorda, J., Martinez-Mora, C., Correal, E., Simon, B., and Cenis, J. L. (2005). Genetic structure of Atriplex halimus populations in the Mediterranean basin Annals Bot. 95, 827–834. doi: 10.1093/aob/mci086
Paine, T. D., Hanlon, C. C., Pittenger, D. R., Ferrin, D. M., and Malinoski, M. K. (1992). Consequences of water and nitrogen management on growth and aesthetic quality of drought-tolerant woody landscape plants. J. Environ. Hortic. 10, 94–99. doi: 10.24266/0738-2898-10.2.94
Pande, R. S. (1990). Feed value of tagasaste (Chamaecytisus palmernsis) for goats and preferential browsing activities by goats and sheep in multi-species shrub/pasture conditions. M.Ag.Sc. thesis, Massey University.
Pande, R. S., Kemp, P. D., and Hodgson, J. (2002). Preference of goats and sheep for browse species under field conditions. N. Z. J. Agric. Res. 45, 97–102. doi: 10.1080/00288233.2002.9513498
Parkyn, S. M., Davies-Colley, R. J., Halliday, N. J., Costley, K. J., and Croker, G. F. (2003). Planted riparian buffer zones in New Zealand: do they live up to expectations? Restoration Ecol. 11, 436–447. doi: 10.1046/j.1526-100X.2003.rec0260.x
Parsons, A., Rowarth, J., Thornley, J., and Newton, P. (2011). “Primary production of grasslands, herbage accumulation and use, and impacts of climate change,” in Grassland Productivity and Ecosystem Services, ed. G. Lemaire (Wallingford: CABI), 3–18. doi: 10.1079/9781845938093.0003
Pitta, D. W., Barry, T. N., Lopez-Villalobos, N., and Kemp, P. D. (2007). Willow fodder blocks—An alternate forage to low quality pasture for mating ewes during drought? Anim. Feed Sci. Technol. 133, 240–258. doi: 10.1016/j.anifeedsci.2006.04.006
Plant Food Research (2013). Willows for the farm: specially selected for New Zealand conditions. Available online at: http://www.poplarandwillow.org.nz/documents/brochure-1-willows-for-the-farm.pdf (accessed December 5, 2019).
Pollard, J. C., Littlejohn, R. P., and Pearse, A. J. T. (2003). Shade and shelter for farmed deer in New Zealand: results from a survey of farmers. N. Z. J. Agric. Res. 46, 287–294. doi: 10.1080/00288233.2003.9513556
Pollock, K. M. (1986). Plant materials handbook for soil conservation. Volume 3, Native plants. Water and Soil Miscellaneous Publication No. 95. Wellington, New Zealand: Water And Soil Directorate, Ministry Of Works And Development.
Proffitt, A., Bendotti, S., Howell, M., and Eastham, J. (1993). The effect of sheep trampling and grazing on soil physical properties and pasture growth for a red-brown earth. Aust. J. Agric. Res. 44, 317–331. doi: 10.1071/AR9930317
Radcliffe, J. E. (1982). Effects of aspect and topography on pasture production in hill country. NZ. J. Agric. Res. 25, 485–496. doi: 10.1080/00288233.1982.10425211
Radcliffe, J. E. (1985). “Fodder tree production under cutting, for 5 years in Canterbury hill country,” in Fodder Trees - A Summary of Current Research in New Zealand. ed. L.A. Logan, Radcliffe, J. E.(Christchurch: Crop Research Division, DSIR), 19–23.
Radcliffe, J. E., Alexander, R., and Townsend, R. (1985). “Effect on tagasaste of cutting and sheep grazing at different times of the year,” in Fodder Trees - A Summary of Current Research in New Zealand. eds. L.A. Logan and J.E. Radcliffe (Lincoln: Crop Research Division, DSIR), 24–25.
Ramírez-Restrepo, C. A., Barry, T. N., Marriner, A., López-Villalobos, N., McWilliam, E. L., Lassey, K. R., et al. (2010). Effects of grazing willow fodder blocks upon methane production and blood composition in young sheep. Anim. Feed Sci. Technol. 155, 33–43. doi: 10.1016/j.anifeedsci.2009.10.003
Robinson, B. H., Mills, T. M., Petit, D., Fung, L. E., Green, S. R., and Clothier, B. E. (2000). Natural and induced cadmium-accumulation in poplar and willow: implications for phytoremediation. Plant Soil 227, 301–306. doi: 10.1023/A:1026515007319
Rosin, C. (2013). Food security and the justification of productivism in New Zealand. J. Rural Stud. 29, 50–58. doi: 10.1016/j.jrurstud.2012.01.015
Ryle, G. J. A. (1970). Distribution patterns of assimilated 14C in vegetative and reproductive shoots of Lolium perenne and L. temulentum. Annals Appl. Biol. 66, 155–167. doi: 10.1111/j.1744-7348.1970.tb04613.x
Salam, M. M. A., Pelkonen, P., Mehtätalo, L., and Gong, J. (2015). Using stem analysis data for modeling the volume of Kinuyanagi willow (Salix schwerinii E. L. Wolf). Baltic Forestry 21, 259–271.
Sanjari, G., Yu, B., Ghadiri, H., Ciesiolka, C. A. A., and Rose, C. W. (2009). Effects of time-controlled grazing on runoff and sediment loss. Soil Res. 47, 796–808. doi: 10.1071/SR09032
Scott, D., Keoghan, J. M., Cossens, G. G., Maunsell, L. A., Floate, M. J. S., Wills, B. J., et al. (1985). Limitations to pasture production and choice of species. Grassland Res. Pract. Series 3, 9–15.
Sheath, G., Webby, R., and Pengelly, W. (1984). Management of late spring-early summer pasture surpluses in hill country. Proc. N. Z. Grassland Association 45, 199–206. doi: 10.33584/jnzg.1984.45.1657
Sheath, G. W., and Boom, R. C. (1985). Effects of November-April grazing pressure on hill country pastures 1. Pasture structure and net accumulation rates. N. Z. J. Exp. Agric. 13, 317–328. doi: 10.1080/03015521.1985.10426100
Sheffield, J., and Wood, E. F. (2008). Projected changes in drought occurrence under future global warming from multi-model, multi-scenario, IPCC AR4 simulations. Climate Scenarios 31, 79–105. doi: 10.1007/s00382-007-0340-z
Sheppard, J. S. (1985). “Observations on shrubs with fodder potential in Canterbury and Marlborough,” in Fodder Trees: A Summary of Current Research in New Zealand, eds. L.A. Logan and J.E. Radcliffe (Christchurch: Crop Research Division, DSIR), 14–15.
Sheppard, J. S., and Douglas, G. B. (1986). “Management and uses of Dorycnium spp,” in Water and Soil Miscellaneous Publication No. 94, eds. C.W.S. Van Kraayenoord and R.L. Hathaway (Wellington: Water and Soil Directorate, Ministry of Works and Development), 260–262.
Smale, M. C., Dodd, M. B., Burns, B. R., and Power, I. L. (2008). Long-term impacts of grazing on indigenous forest remnants on North Island hill country, New Zealand. N. Z. J. Ecol. 32, 57–66.
Smale, M. C. W. R. C., and Arnold, G.C. (2005). Vegetation recovery in rural kahikatea (Dacrycarpus dacrydioides) forest fragments in the Waikato region, New Zealand, following retirement from grazing. N. Z. J. Ecol. 29, 261–269.
Small, E. (2011). Alfalfa and Relatives: Evolution and Classification of Medicago. Ottawa, ON: NRC Research Press
Snook, L. C. (1986). Tagasaste (tree lucerne), High Production Fodder Crop. Shepparton: Night Owl Publishers.
Snowdon, K., McIvor, I., and Nicholas, I. (2013). IEA Bioenergy Task 43, Report 2013:PRO1. Promising resources and systems for producing bioenergy feedstocks: short rotation coppice with willow in New Zealand. New Zealand: IEA Bioenergy.
Stephens, J. M. C., Molan, P. C., and Clarkson, B. D. (2005). A review of Leptospermum scoparium (Myrtaceae) in New Zealand. N. Z. J. Bot. 43, 431–449. doi: 10.1080/0028825X.2005.9512966
St. John, M. G., Bellingham, P. J., Walker, L. R., Orwin, K. H., Bonner, K. I., Dickie, I. A., et al. (2012). Loss of a dominant nitrogen-fixing shrub in primary succession: consequences for plant and below-ground communities. J. Ecol.100, 1074–1084. doi: 10.1111/j.1365-2745.2012.02000.x
Stokes, A., Atger, C., Bengough, A., Fourcaud, T., and Sidle, R. (2009). Desirable plant root traits for protecting natural and engineered slopes against landslides. Plant Soil 324, 1–30. doi: 10.1007/s11104-009-0159-y
Suckling, F. E. T. (1959). Pasture management trials on unploughable hill country at te Awa. N. Z. J. Agric. Res. 2:488–543. doi: 10.1080/00288233.1959.10418033
Swaffield, S., and McWilliam, W. (2013). “Landscape aesthetic experience and ecosystem services,” in Ecosystem Services in New Zealand - Conditions and Trends, ed. J.R. Dymond. (Lincoln: Manaaki Whenua Press), 349–362.
Sweetapple, P. J., and Nugent, G. (2004). Seedling ratios: a simple method for assessing ungulate impacts on forest understories. Wildlife Soc. Bull. 32, 137–147, 111. doi: 10.2193/0091-7648(2004)32[137:SRASMF]2.0.CO;2
Tane'sTreeTrust (2011). Planting and Managing Native Trees. New Zealand: Tane's Tree Trust. Available online at: www.tanestrees.org.nz.
Thompson, R. C., and Luckman, P. G. (1993). Performance of biological erosion control in New Zealand soft rock hill terrain. Agroforestry Systems 21, 191–211. doi: 10.1007/BF00705230
Thomson, G. M. (2011). The Naturalisation of Animals and Plants in New Zealand. New York, NY: Cambridge University Press. doi: 10.1017/CBO9780511841750
Timmins, S. M. (2002). Impact of cattle on conservation land licensed for grazing in South Westland, New Zealand. N. Z. J. Ecol. 26, 107–120.
Townsend, R. J., and Radcliffe, J. E. (1987). Establishment and management of tagasaste. Proc. N. Z. Grassland Association. 48, 109–113. doi: 10.33584/jnzg.1987.48.1794
Townsend, R. J., and Radcliffe, J. E. (1990). Tagasaste forage production systems. N. Z. J. Agric. Res. 33, 627–634. doi: 10.1080/00288233.1990.10428467
Tozer, K. N., Cameron, C. A., and Thom, E. R. (2011). Pasture persistence: farmer observations and field measurements. Pasture Persistence Grassland Res. Pract. Series 15, 25–30.
Tozer, K. N., Chapman, D. F., Bell, N. L., Crush, J. R., King, W. M., Rennie, G. M., et al. (2014). Botanical survey of perennial ryegrass-based dairy pastures in three regions of New Zealand: implications for ryegrass persistence. N. Z. J. Agric. Res. 57, 14–29. doi: 10.1080/00288233.2013.863785
Tozer, K. N., Müller, K., Craven, C., Tarbotton, I., Coster, A., Burke, R., et al. (2020). Effect of deferred grazing during late spring and summer on pasture productivity in Waikato and Bay of Plenty hill country. Journal of the New Zealand Grasslands 82, 111–119. doi: 10.33584/jnzg.2020.82.448
Turner, J. A., Klerkx, L., Rijswijk, K., Williams, T., and Barnard, T. (2016). Systemic problems affecting co-innovation in the New Zealand Agricultural Innovation System: identification of blocking mechanisms and underlying institutional logics. NJAS - Wageningen J. Life Sci. nces 76, 99–112. doi: 10.1016/j.njas.2015.12.001
Turner, L. R., Holloway-Phillips, M. M., Rawnsley, R. P., Donaghy, D. J., and Pembleton, K. G. (2012). The morphological and physiological responses of perennial ryegrass (Lolium perenne L.), cocksfoot (Dactylis glomerata L.) and tall fescue (Festuca arundinacea Schreb.; syn. Schedonorus phoenix Scop.) to variable water availability. Grass Forage Sci. 67, 507–518. doi: 10.1111/j.1365-2494.2012.00866.x
Van den Belt, M., and Blake, D. (2014). Ecosystem services in New Zealand agro-ecosystems: a literature review. Ecosystem Services 9, 115–132. doi: 10.1016/j.ecoser.2014.05.005
van Kraayenoord, C. W. S., Pollock, K. M., and Hathaway, R. L. (1986). Plant Materials Handbook for Soil Conservation: Principles and Practices. Water and Soil Miscellaneous Publication No. 93. Wellington: Water and Soil Directorate, Ministry of Works and Development.
van Reenen, E. (2012). Increasing uptake of environmental practices on sheep and beef farms. Report for Kellogg rural leaders programme. (New Zealand: Beef + Lamb New Zealand and the Agricultural Marketing and Research Development Trust (AGMARDT), New Zealand).
Vanclay, F. (2004). Social principles for agricultural extension to assist in the promotion of natural resource management. Aust. J. Exp. Agric. 44, 213–222. doi: 10.1071/EA02139
Waghorn, G. C., Douglas, G. B., Niezen, J. H., McNabb, W. C., and Foote, A. G. (1998). Forages with condensed tannins - their management and nutritive value for ruminants. Proc. NZ. Grassland Association 60, 89–98. doi: 10.33584/jnzg.1998.60.2315
Walker, S., Lee, W. G., and Rogers, G. M. (2004). The woody vegetation of Central Otago, New Zealand. N. Z. J. Bot. 42, 589–612. doi: 10.1080/0028825X.2004.9512914
Waller, R. (2002). Summer-autumn grazing management for perennial ryegrass. Australian Farm J. 44–45.
Waller, R. A., Quigley, P. E., Saul, G. R., Kearney, G. A., and Sale, P. W. G. (1999). Tactical versus continuous stocking for persistence of perennial ryegrass (Lolium perenne L.) in pastures grazed by sheep in south-western Victoria. Aust. J. Exp. Agric. 39, 265–274. doi: 10.1071/EA98154
Waller, R. A., and Sale, P. W. G. (2001). Persistence and productivity of perennial ryegrass in sheep pastures in south-western Victoria: a review. Aust. J. Exp. Agric. 42, 117–144. doi: 10.1071/EA00049
Watson, R., Harris, S., Bell, N., and Neville, F. (1996). Deferred grazing to enhance white clover content in pastures. Grassland Res. Pract. Series 6, 154–154.
Weston, R. J. (2004). Composition of essential oils from the leaves of seven New Zealand species of Pittosporum (Pittosporaceae). J. Essential Oil Res. 16, 453–458. doi: 10.1080/10412905.2004.9698770
White, J. G. H. (1999). “The farmlands of New Zealand,” in New Zealand Pasture and Crop Science, eds. J. White and J. Hodgson (Auckland: Oxford University Press), 1–10.
White, L. M. (1973). Carbohydrate reserves of grasses: a review. J. Range Manag. 26, 13–18. doi: 10.2307/3896873
Wiley, T. (2006). “Fodder shrubs,” in Perennial Pastures for Western Australia (Perth, WA: South Perth, Western Australia: Department of Agriculture and Food Western Australia), 191–202.
Wilkinson, A. G. (1999). Poplars and willows for soil erosion control in New Zealand. Biomass Bioenergy 16, 263–274. doi: 10.1016/S0961-9534(99)00007-0
Williams, P. A. (2011). Secondary succession through non-native dicotyledonous woody plants in New Zealand. N. Z. Nat. Sci. 36, 73–91.
Wills, B. (2008). Plant Solutions for Drought-Prone Saline Soils at Bonavaree. Marlborough: Starborough Flaxbourne Soil Conservation Group, 1–2.
Wills, B. J. (1983). Forage Plants for the Semi-Arid High Country and Rangelands of New Zealand. Canterbury: Special Publication; Centre of Resource Management; Lincoln College, 26, 59–65.
Wills, B. J., and Begg, J. S. C. (1992). Forage shrubs for the South Island dry hill country: 2. Salt/lime amendments have potential to improve the versatility of saltbush (Atriplex halimus L.). Proc. N. Z. Grassland Association 54, 121–125. doi: 10.33584/jnzg.1992.54.2053
Wills, B. J., Begg, J. S. C., and Brosnan, M. (1990). Forage shrubs for the South Island dry hill country: 1. Atriplex halimus L. (Mediterranean saltbush). Proc. N. Z. Grassland Association 52, 161–165. doi: 10.33584/jnzg.1990.52.1975
Wills, B. J., Begg, J. S. C., and Foote, A. G. (1989). Dorycnium species - two new legumes with potential for dryland pasture rejuvenation and resource conservation in New Zealand. Proc. NZ. Grassland Association 50, 169–174. doi: 10.33584/jnzg.1989.50.1878
Wills, B. J., Douglas, G. B., Foote, A. G., and Trainor, K. D. (1999). Germplasm characterization and palatability of Dorycnium species under New Zealand dryland conditions. FAO/International Plant Genetic Resources Newsletter 120, 8–14.
Wilson, H. D. (1994). Regeneration of native forest on Hinewai Reserve, Banks Peninsula. N. Z. J. Bot 32, 373–383. doi: 10.1080/0028825X.1994.10410480
Wright, A. E., and Cameron, E. K. (1985). Botanical features of northeastern Great Barrier Island, Hauraki Gulf, New Zealand. J. R. Soc. N. Z. 15, 251–278. doi: 10.1080/03036758.1985.10416830
Keywords: grazing management, woody forages, hill country, pasture management, deferred grazing
Citation: Tozer K, Douglas G, Dodd M and Müller K (2021) Vegetation Options for Increasing Resilience in Pastoral Hill Country. Front. Sustain. Food Syst. 5:550334. doi: 10.3389/fsufs.2021.550334
Received: 09 April 2020; Accepted: 07 January 2021;
Published: 23 February 2021.
Edited by:
Pablo Gregorini, Lincoln University, New ZealandReviewed by:
Thomas M. R. Maxwell, Lincoln University, New ZealandStacey Alan Gunter, United States Department of Agriculture, United States
Copyright © 2021 Tozer, Douglas, Dodd and Müller. This is an open-access article distributed under the terms of the Creative Commons Attribution License (CC BY). The use, distribution or reproduction in other forums is permitted, provided the original author(s) and the copyright owner(s) are credited and that the original publication in this journal is cited, in accordance with accepted academic practice. No use, distribution or reproduction is permitted which does not comply with these terms.
*Correspondence: Katherine Tozer, S2F0aGVyaW5lLnRvemVyQGFncmVzZWFyY2guY28ubno=