- 1Department of Biochemistry, Microbiology and Biotechnology, Kenyatta University, Nairobi, Kenya
- 2Department of Environmental Sciences and Education, Kenyatta University, Nairobi, Kenya
Determination of biologically active components of the soil organic matter, such as soil microbial biomass carbon (C) and nitrogen (N) can be used as indicators for variations in soil productivity due to changes in soil management. Soil agronomic management practices bring about changes in the physical and chemical properties of the soil, resulting in variations in soil microbial biomass and microbial diversity. The effects of tillage, mulch and inorganic fertilizers on soil microbial biomass C and N, microbial populations and bacterial diversity were determined from the treatment combinations which had been applied for 5 years in Central Kenyan Highlands. The test crop used was maize (Zea mays L.). The study involved conventional and minimum tillage systems, mulching and inorganic fertilizers (120 kg N/ha). Tillage (P < 0.001), mulch (P < 0.001), and fertilizer (P = 0.009) significantly affected soil microbial biomass C and N whereby minimum tillage and mulch increased soil microbial biomass C and N. Interestingly, minimum tillage and mulch recorded the highest bacteria and fungi CFUs compared to conventional tillage and inorganic fertilizers. Only fertilizer and mulch (P < 0.001) had significant effect on actinobacteria CFUs. Amplified ribosomal DNA analysis (ARDRA) showed that the highest genetic distance of 0.611 was recorded between treatments conventional tillage + no mulch + no NPK fertilizer and conventional tillage + no mulch + NPK fertilizer. The results demonstrate that minimum tillage and mulching are attractive soil agronomic management practices since they increase soil microbial biomass and bacterial diversity in agricultural soils.
Introduction
Soil agronomic management practices, particulary those which improve soil fertility are widely used by farmers to increase crop production in their farms (Balota et al., 2003; Kara and Bolat, 2008). Changes in levels of soil fertility can be measured by use of active fractions in the soil organic pool such as soil microbial biomass (He et al., 2013). Soil microbial biomass refers to the mass of the living component of the soil organic matter, thus measurement of soil microbial biomass is vital in decomposition of organic materials in the soil and can be used as an early indicator of the variations in soil physical, chemical and biological properties (Baaru et al., 2007). These variations in soil properties may be due to use of soil management practices such as organic and inorganic inputs (Nakhro and Dkhar, 2010). In this study, soil microbial biomass was determined at V4 (maize juvenile stage) which is the stage under control of microRNA156 genes which regulate key maize processes including heteroblasty. As a result, any soil management- induced change at juvenile stage will determine the growth and survival of the plant (Cavaglieri et al., 2009).
Most agricultural soils have limited amounts of nitrogen (N) due to slow rates of N-mineralisation and therefore, there is need of addition of external N by use of inorganic fertilizers (Mucheru-Muna et al., 2007; Mugendi et al., 2007). Application of inorganic fertilizers especially those containing N provides immediate positive impacts in the soil by increasing crop growth and productivity (Mucheru-Muna et al., 2014; Shaheen and Sabir, 2017). Conservation soil management practices such as mulching and reduced tillage have been used by farmers extensively because they enhance stabilization of organic C in the soil (Fuentes et al., 2010). Conventional tillage involves a lot of soil manipulation during land preparation (Kabir, 2004; Alguacil et al., 2008), resulting in degradation of soil aggregates and rapid oxidation of soil organic matter which eventually reduces soil microbial biomass and important microbial communities. On the other hand, reduced tillage systems cause little soil disturbance, maintain soil aggregates and ultimately improve the quality of the soil through increase in soil microbial biomass (Habig and Swanepael, 2015).
The diverse and complex groups of soil microorganisms play a critical role in enhancing soil biological fertility in agricultural soils (Nakhro and Dkhar, 2010). A number of molecular techniques are used to determine the diversity of soil microorganisms based on their genotypic and phenotypic properties (Koeleman et al., 1998). Some of these techniques include ARDRA which involves polymerase chain amplification of 16S rDNA genes using primers targeting the conserved regions of microorganisms, followed by digestion by restriction endonucleases and agarose gel electrophoresis to produce distinguishable DNA fingerprints of microorganisms. Therefore, ARDRA is vital for rapid monitoring of microbial communities in environmental samples as well as diversity of microorganisms in response to changing environmental conditions (Dash and Das, 2018; Panigrahi et al., 2019).
In this study, we hypothesized that tillage, mulching and inorganic fertilization affect soil microbial biomass C and N, bacteria diversity and populations of bacteria, fungi and actinobacteria in the soil. The specific objectives were to; (i) determine the effect of tillage, mulching and NPK fertilizers on soil microbial biomass C and N at maize juvenile stage, (ii) monitor the effect of tillage, mulching and NPK fertilizers on bacteria, fungi and actinobacteria CFUs at different growth stages of maize, and (iii) evaluate the effect of tillage, mulching and NPK fertilizers on bacteria diversity at maize juvenile stage.
Materials and Methods
Experimental Site
The field experiments were conducted at Kirege Primary School (S 00°20'07.0;” E 037°36'46.0” and an altitude of 1,526 meters above sea level), Chuka Division in Tharaka-Nithi County, Kenya and they were part of a field experiment. The annual precipitation of the study site is between 950 and 1,500 mm and the annual mean temperature is 210C. The study site has Humic Nitisol soils with 75 % clay, 18 % silt, 7 % sand, pH (water) 4.65, 0.01 % P, 0.02 % total N and 0.15 % organic carbon (Ngetich et al., 2014).
Experimental Design
The experiment was established on 7th April 2013 and was set up in a randomized complete block design and replicated three times. The treatments were; minimum tillage + mulch + NPK fertilizer (M1), minimum tillage + no mulch + no NPK fertilizer (M2), minimum tillage + no mulch + NPK fertilizer (M3), minimum tillage + mulch + no NPK fertilizer (M4), conventional tillage + mulch + NPK fertilizer (C1), conventional tillage + no mulch + no NPK fertilizer (C2), conventional tillage + no mulch + NPK fertilizer (C3) and conventional tillage + mulch + no NPK fertilizer (C4). In conventional tillage, weeding was done using a hand hoe at a depth of 15 cm at the beginning of the season whereas in minimum tillage weed control was achieved by manual uprooting. Inorganic fertilizers which were used are NPK (23:23:0) and triple super phosphate (TSP). For plots receiving NPK fertilizers, nitrogen was applied at a rate of 120 kg ha−1 in split whereby 60 kg ha−1 of NPK (23:23:0) was applied as starter fertilizer while the remaining 60 kg ha−1 was applied by top dressing with urea 30 days after planting. Phosphorous was blanket applied to give a supply of 90 kg ha−1 inclusive of the amount supplied by the starter fertilize. For plots which did not receive NPK fertilizer, TSP was used to supply phosphorus at a rate of 90 kg ha−1. Dried maize stovers from the previous cropping season were broadcasted at the rate of 3 Mg ha−1 7 days after emergence for mulch treatments. The plots were 8.0 m by 8.0 m with 1 m and 3 m wide alleys separating plots within a block and between the blocks, respectively.
Maize Crop Management
Maize crop (Zea mays L.) was planted on 7th April 2013 for the first cropping season and also in the subsequent 4 years. Maize planting was done immediately after soil preparation. A spacing of 0.75 by 0.50 m between and within rows was used in planting three maize seeds per hill which were thinned to two plants per hill 2 weeks after emergence to give the recommended plant density of 53,333 plants ha−1 (Jaetzold et al., 2007).
Soil Sampling
Undisturbed soil was sampled at 0–20 cm depth from seven points in each plot, pooled together to obtain an homogenous soil sample for each plot. Sampling was done at four different times; before maize planting (1st November 2016), when the maize was at vegetative 4 (V4) stage (22nd November 2016), when the maize was at vegetative 6 (V6) stage (3rd January 2017) and at harvesting stage of the maize (28th March 2017) as described by Hajabbasi and Schumacher (1994). The soil was used for determination of soil microbial biomass C and N, bacteria diversity, enumeration of bacteria, fungi and actinobacteria, and also for determination of soil physicochemical properties.
Determination of Soil Microbial Biomass C and N
The soil microbial biomass C and N was determined using chloroform fumigation-extraction technique (Baaru et al., 2007). The microbial biomass C and N were obtained using the following equations according to Bailey et al. (2002) using a correction factor of 0.45.
Enumeration of Microbial Populations
Isolation and enumeration of bacteria, fungi and actinobacteria from the soil was done using spread plate method. Nutrient agar (NA) was used for bacteria isolation, Potato dextrose agar (PDA) for fungi isolation and M1 media (10 g of starch, 4 g of yeast extract, 2 g of peptone and 18 g of agar in 1 liter of distilled water) for actinobacteria isolation and enumeration. Ten grams of the sample soil collected was suspended in 90 ml of sterile water blanks in 250 ml conical flasks and diluted serially to yield three dilutions. Using a sterile pipette, 0.1 ml of the the first dilution (10−1) was plated onto three petri dishes containing Potato Dextrose agar (PDA), 0.1 ml of 10−2 dilution was plated onto three petri dishes containing M1 media while 0.1 ml of 10−3 dilution was plated onto three petri dishes containing nutrient agar (NA).
DNA Extraction, PCR Amplification and Amplified ribosomal DNA Restriction Analysis (ARDRA) of Bacteria 16S rDNA Gene
Genomic DNA from bacteria isolates was extracted using Quick-DNA™ miniprep kit (UK) following the manufacturer's instructions and 16S rDNA gene amplified using primers 27f (5′- AGA GTT TGA TCM TGG CTC AG - 3′) and 1492r (5′- CGG TTA CCT TGT TAC GAC TT - 3′) which are complementary to conserved regions of 16S rDNA gene in bacteria (Monis et al., 2005). The PCR products were then digested separately using EcoRI, HaeIII and MspI restriction enzymes according to the manufacturers' instructions (Odee et al., 2002).
Data Analyses
Data was tested for homogeneity of variance by Bartlett test. Data on soil microbial biomass C and N, populations of bacteria, fungi and actinobacteria were analyzed by three-way ANOVA and wherever feasible means were separated by Tukey's Honest Significance Difference (HSD) at P < 0.05 using SAS (version 9) software. Restriction patterns were analyzed using GenAIEx software (version 6.5) to determine the diversity of bacteria isolates. The bacteria diversity profiles were determined using Shannon's Information Index I. Dendrograms were drawn using Darwin software (version 6.0) based on Nei's unbiased genetic distance. Principal Coordinate Analysis for bacterial diversity were drawn using PAST software version 3.
Results
Effects of Tillage, Mulching and Inorganic Fertilization on Soil Microbial Biomass C and N
Minimum tillage + mulch + no NPK fertilizer treatment produced the highest microbial biomass C and N with an average of 122.27 μg g−1 and 12.73 μg g−1, respectively while conventional tillage + no mulch + NPK fertilizer produced the lowest microbial biomass C and N of 82.07 μg g-1 and 4.50 μg g−1 respectively (Table 1). Moreover, the effects of tillage (P < 0.001), mulch (P < 0.001) and fertilizer (P = 0.009) were significantly different on soil microbial biomass C and N. On average, mulch treatment produced highest microbial biomass C and N of 110.29 μg g-1 and 8.43 μg g−1, respectively whereas NPK fertilizer treatment produced the lowest microbial biomass C and N of 86.48 μg g−1 and 6.76 μg g−1.
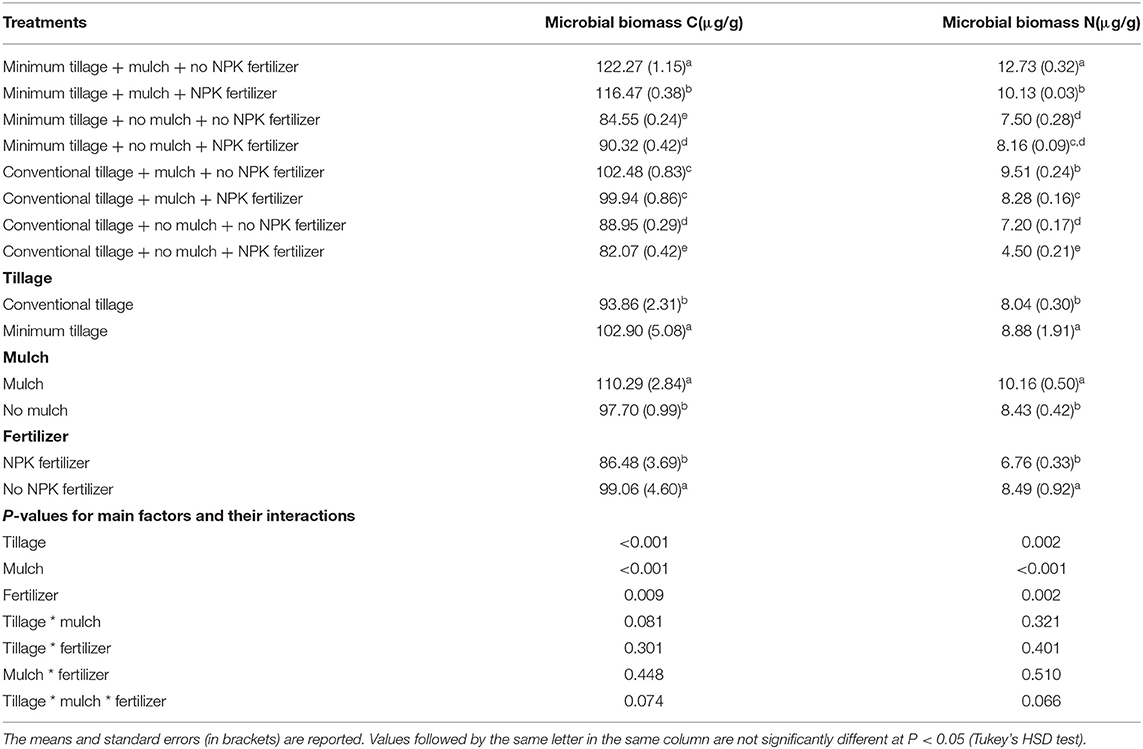
Table 1. Soil microbial biomass C and N as influenced by tillage, mulch and inorganic fertilization, and their interactions.
Effects of Tillage, Mulching and Inorganic Fertilization on Bacteria Populations
In this study, the number of bacteria was significantly affected by tillage (P = 0.009), mulch (P = 0.001) and fertilizer (P = 0.001) at V4, V6 and maize harvest stages. Application of mineral fertilizers reduces bacteria population whereas mulch treatments enhance bacteria populations. At V4 (juvenile) stage, the highest number of bacteria CFUs was obtained in mulch treatment whereas the lowest number of bacteria CFUs was obtained in NPK fertilizer treatment. A similar trend was observed at V6 and maize harvest stages. Before maize planting, the number of bacteria was not significantly affected by tillage, mulch and fertilizer (Figure 1).
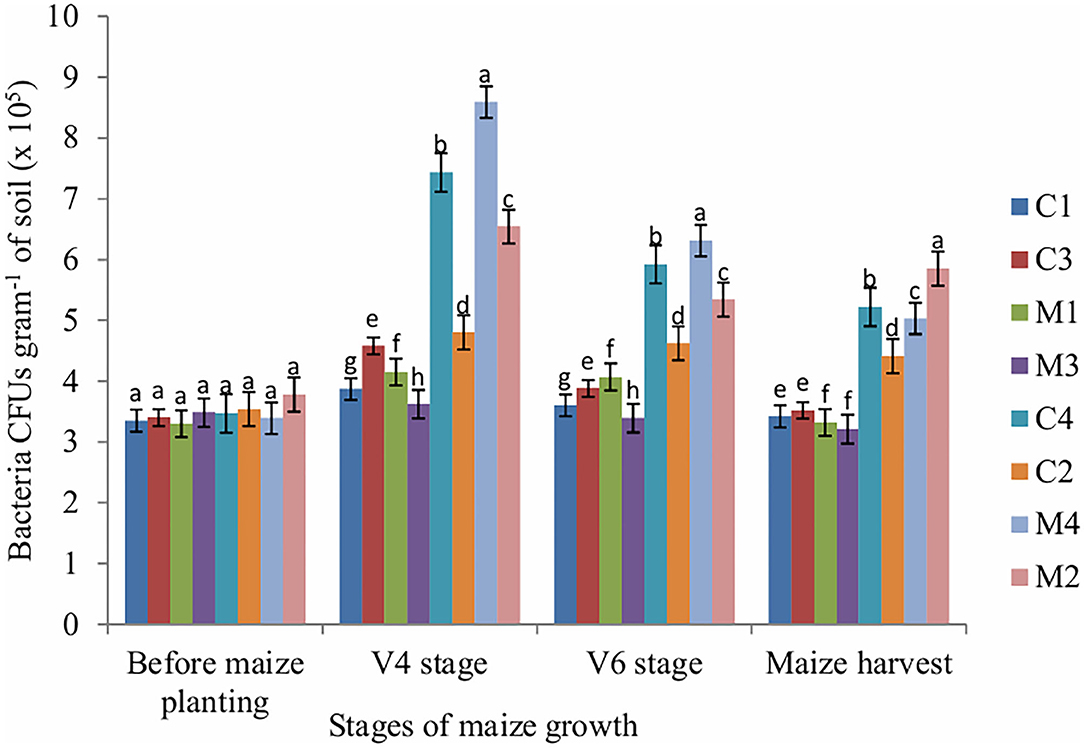
Figure 1. Bacteria CFUs as influenced by tillage, mulching and inorganic fertilization. Stages of maize growth were; before maize planting: 0 days, V4 stage: 22 days, V6 stage: 63 days, and maize harvest stage: 147 days. Vertical bars represent means and whiskers – standard errors. C1, Conventional tillage + mulch + NPK fertilizer treatment; C2, Conventional tillage + no mulch + no NPK fertilizer treatment; C3, Conventional tillage + no mulch + NPK fertilizer treatment; C4, Conventional tillage + mulch + no NPK fertilizer treatment; M4, Minimum tillage + mulch + no NPK fertilizer treatment; M2, Minimum tillage + no mulch + no NPK fertilizer treatment; M3, Minimum tillage + no mulch + NPK fertilizer treatment; M1, Minimum tillage + mulch + NPK fertilizer treatment. Different letters indicate significant differences at P < 0.05 (Tukey's HSD test).
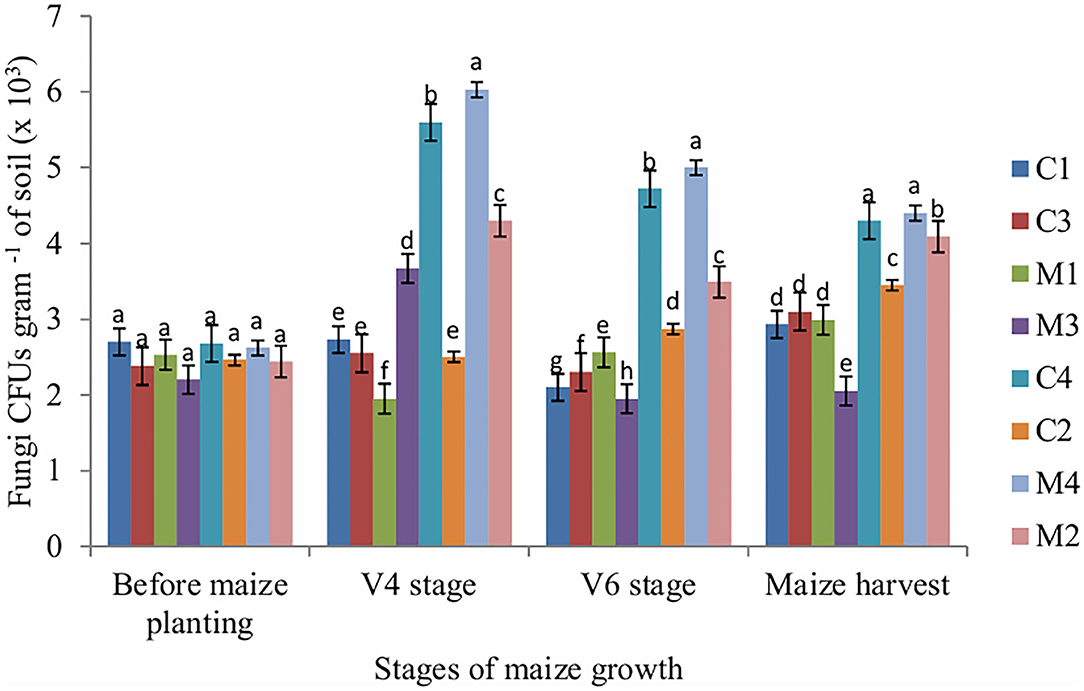
Figure 2. Fungi CFUs as influenced by tillage, mulching and inorganic fertilization. Stages of maize growth were; before maize planting: 0 days, V4 stage: 22 days, V6 stage: 63 days, and maize harvest stage: 147 days. Vertical bars represent means and whiskers – standard errors.
Effects of Tillage, Mulching and Inorganic Fertilization on Fungi and Actinobacteria Populations
The number of fungi CFUs in soil sampled at V4, V6 and maize harvest stages was significantly affected by tillage (P = 0.001), mulch (P = 0.002) and fertilizer (P = 0.001). Mulch treatment enhanced fungi populations across all stages of maize growth whereas NPK fertilizer application reduced fungi populations. The number of actinobacteria CFUs was significantly (P = 0.011) affected by fertilizer only at V4 stage whereas at V6 stage the number of actinobacteria CFUs was significantly (P = 0.002) affected by mulch only. However, the number of actinobacteria CFUs in the soil sampled before maize planting and maize harvest stages was not significantly affected by tillage, mulch and fertilizer (Figure 3).
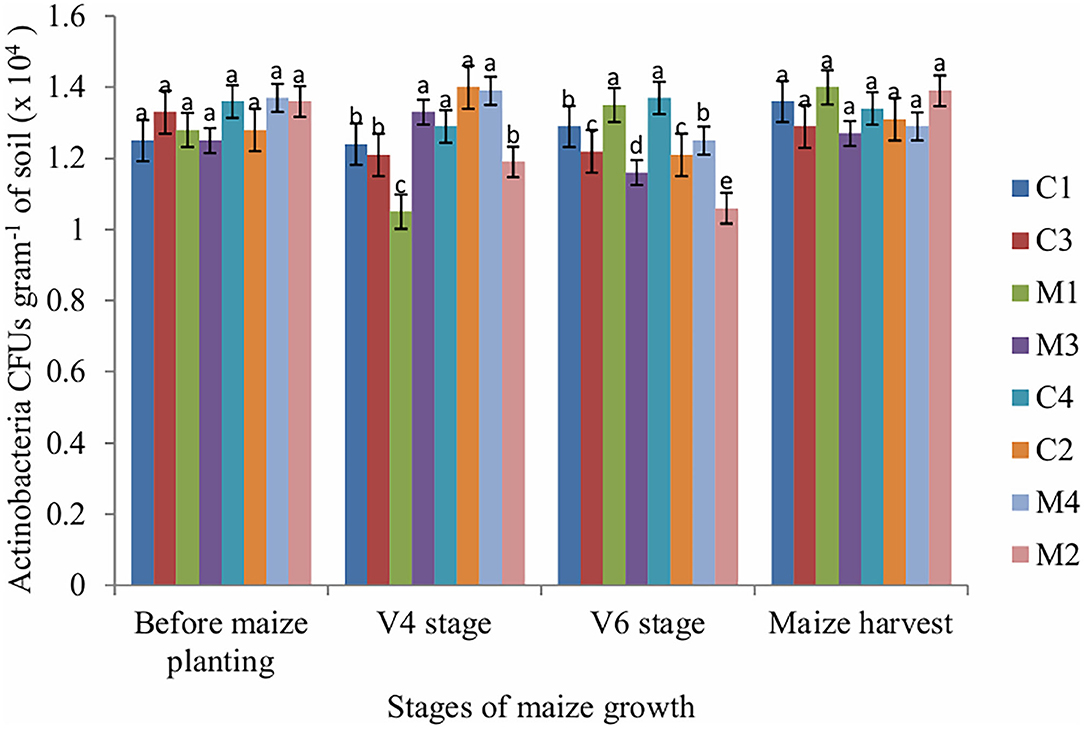
Figure 3. Actinobacteria CFUs as influenced by tillage, mulching and inorganic fertilization. Stages of maize growth were; before maize planting: 0 days, V4 stage: 22 days, V6 stage: 63 days, and maize harvest stage: 147 days. Vertical bars represent means and whiskers – standard errors.
Effects of Tillage, Mulching and Inorganic Fertilization on Bacteria Diversity
In this study, sixty (60) bacteria isolates were obtained which were placed in 15 groups based on their differences and similarities on morphological and biochemical characteristics. The colony size of the isolates ranged from 0.4 to 2.3 mm while the margin ranged from entire to wavy. The color of the bacteria isolates varied from cream to white with flat or raised elevation in nutrient agar plates. The bacteria isolates showed varied response to catalase, oxidase, urease, citrate, triple sugar iron (TSI) and motility tests. The Shannon's Information Index I showed that the 15 bacteria groups from the eight (8) treatments were genetically diverse with M4 treatment having the highest genetic diversity estimate of H= 0.30 while C3 treatment had the lowest genetic diversity estimate of H = 0.10 (Table 2).

Table 2. Shannon's Information Index I and percentage of Polymorphic Loci (% P) of bacteria isolates from the eight treatments based on ARDRA analysis.
Assessment of pairwise Nei unbiased genetic distance showed that the highest distance of 0.611 was between C2 and C3 treatments. M1 and M2 treatments had the lowest genetic distance between them of 0.002 (Table 3). Principal coordinate analysis of the bacteria isolates from the 8 treatments showed that there was genetic variation. Isolates from M1, M2, M3, M4, and C4 treatments were most distributed and they were found in two quadrants while isolates from C3 treatment were the least distributed and they were found in only one quadrant (Figure 4).
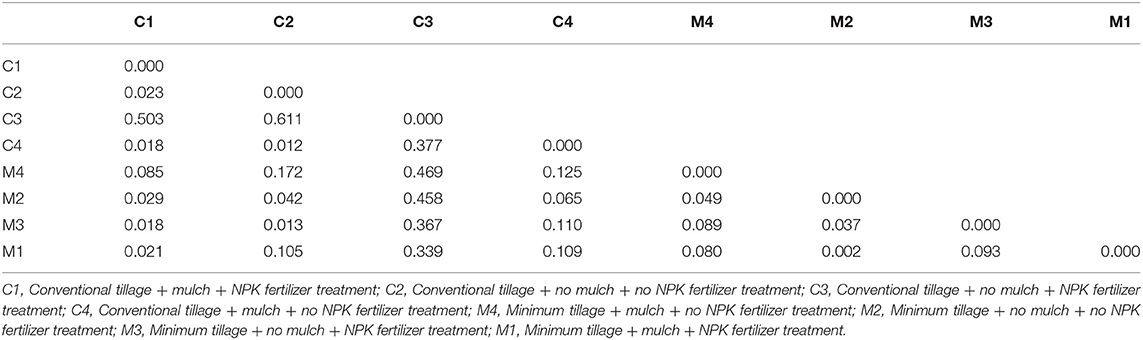
Table 3. Pairwise Population Matrix of Nei Unbiased Genetic Distance of eight treatments based on ARDRA analysis.
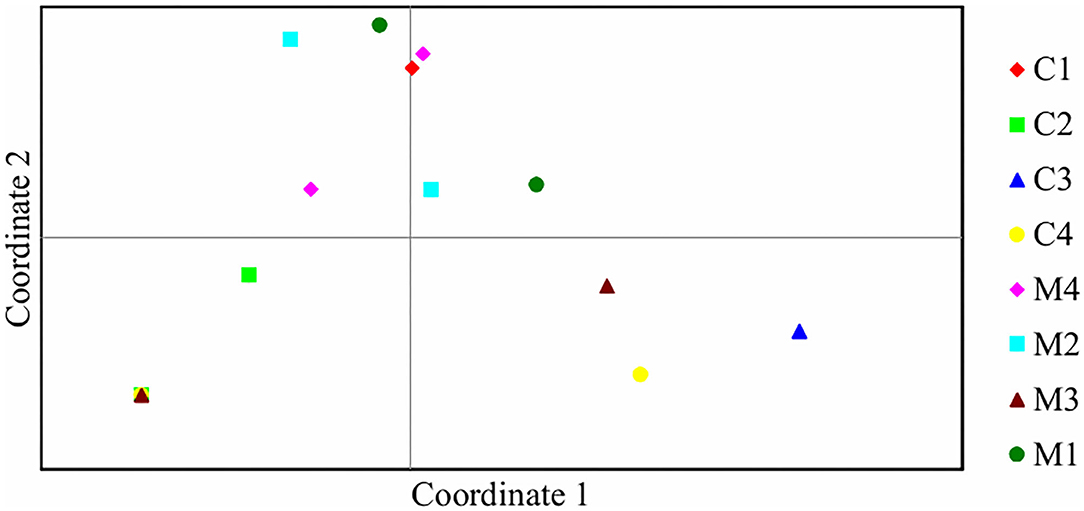
Figure 4. Principal coordinate analysis of 15 bacteria isolates based on ARDRA restriction patterns. Percentage of variation explained by the first two coordinates; 1, 32.71%; 2, 20.39%.
Discussion
Soil Microbial Biomass C and N at Juvenile Stage
In this work, we demonstrated significant effect of typical smallholder soil agronomic management practices on soil microbial biomass. Minimum tillage produced higher soil microbial biomass C and N than conventional tillage using hand hoes. Minimum tillage favors availability of nutrients to soil microorganisms and also protect soil biological components such as bacteria and fungi which constitute the greater portion of soil microbial biomass (Yeboah et al., 2016). In conventional tillage brings about loss of substantial amounts of C in form of CO2 (Balota et al., 2003), therefore contributing to low microbial biomass C and N. Hand hoes resulted in disruption of microhabitats of soil bacteria and fungi as well as physical breakage of filamentous microorganisms, resulting to low microbial biomass C and N.
Application of mulch increase amount of organic C and release readily available N in the soil (Mugwe et al., 2009; He et al., 2013), resulting in high microbial biomass C and N in mulch-treated soils. Use of dead mulch in agriculture provides many benefits to crops such as retaining moisture in the soil, reducing fluctuations in temperature, controlling soil erosion as well as suppressing of weeds (Diallo-Diagne et al., 2016). The findings of this study are consistent with those reported by Diallo-Diagne et al. (2016) who reported elevated levels of microbial biomass C and N in soils treated with organic materials. Inorganic fertilizers produce toxic compounds in the soil which result in low organic C content which in turn affect growth of soil microorganisms (Nakhro and Dkhar, 2010). As a result, there is decrease in soil microbial biomass C and N following application of inorganic fertilizers like NPK. On the other hand, organic materials increase amount of organic C and retain soil moisture, hence increase soil microbial biomass C and N (Kiboi et al., 2018; Bolo et al., 2021).
Bacteria Populations Before Maize Planting, V4, V6 and Harvest Stages
The numbers of bacteria obtained from soil sampled before maize planting were lowest compared to those obtained at V4, V6 and maize harvest stages. This could be ascribed to absence of organic acids and chelating compounds in root exudates before maize planting, which increase the availability of nutrients present in soil required for bacteria growth (Cavaglieri et al., 2009). In the present study, V4 stage produced highest number of bacteria CFUs compared to V6 stage, harvest stage and before maize planting. As the plant grows it produces exudates which influence the number of microorganisms in the rhizosphere (Johnston-Monje et al., 2016). The quantity of the exudates produced by a particular plant varies with the age of the plant (Cavaglieri et al., 2009). Therefore, the population of bacteria at V6 and maize harvest stages is less compared to the population of bacteria at V4 stage. These findings are similar to those obtained by Di Cello et al. (1997) who reported that the populations of Burkholderia cepacia associated with roots of maize plant decreased as the plant approached maturity.
Mineral fertilizers and organic inputs can have an influence on the total number of bacteria found in a particular soil (Zhen et al., 2014). Mulch increases amount of organic C in the soil (Nakhro and Dkhar, 2010) which favors establishment of more bacteria because C is one of the major nutrients required by microorganisms to carry out metabolism. Application of soluble inorganic fertilizers increases the toxic levels of ammonia and alters the soil pH which is detrimental to growth of bacteria (He et al., 2013), resulting in decrease of bacteria populations in NPK fertilizer treated soils.
Fungi and Actinobacteria Populations Before Maize Planting, V4, V6 and Harvest Stages
The populations of fungi and actinobacteria obtained from soils sampled before maize planting were less than those obtained from soils sampled at V4, V6 and maize harvest stages. Growth of plants produces beneficial compounds such as energy and C sources which act as nutrients for sporulation of soil fungi (Tahat and Sijam, 2012). Minimum tillage produced a higher number of fungi compared to conventional tillage because reduced tillage systems do not create imbalance in soil properties such as aeration, temperature, pH, moisture and mixing of residues in the soil (Reji et al., 2012). Changes in these soil properties negatively affect soil microorganisms (Klikocka et al., 2012), especially fungi and as a result the populations of soil fungi was less in conventional tillage soils.
Organic materials such as mulch enhance abundant quantities of organic C which are vital for establishment of more fungi in the soil (Mader et al., 2000). As a result, application of mulch enhances growth of fungi compared to no application. Organic materials also provides readily available C (Mader et al., 2000) which is vital for metabolism of soil microorganisms such as actinobacteria, resulting in more actinobacteria CFUs in mulch treated soils.
Bacteria Diversity at Juvenile Stage
The Shannon's Information Index showed that the bacteria isolates obtained in this study were genetically diverse with bacteria isolates from M4 treatment recording the highest genetic diversity estimate while isolates from C3 treatment recorded the lowest genetic diversity estimate. This could be ascribed to land use practices employed by farmers as well as the genotype of the host plant (Lei et al., 2017; Bolo et al., 2021). Soil management practices such as organic inputs favor growth of more bacteria while other practices like mineral fertilization limit growth and survival of soil bacteria (Cerny et al., 2003; Mwenda et al., 2011), resulting in variation of bacteria isolates obtained from different soil agronomic practices.
A relatively wide Nei unbiased genetic distance of 0.611 was observed among isolates obtained from C2 and C3 treatments. This could be possibly due to different genetic origins of bacteria isolates in these two treatments. However, isolates from M1 and M2 treatments had the lowest genetic distance between them of 0.002. Principal coordinate analysis further showed genetic differentiation among the bacteria isolates from different treatments (Rohwer et al., 2001; Silva et al., 2013). This shows that there was high diversity in the bacteria isolates obtained at juvenile stage of maize growth.
Conclusions
In this study, minimum tillage and mulching using dried maize stovers increased soil microbial biomass C and N, population of bacteria, fungi and actinobacteria while the same parameters were decreased by conventional tillage and inorganic fertilization. However, combinations of different treatments did not significantly affect soil microbial biomass and bacterial diversity in this study. Our experimental findings further shows that there was considerable genetic variation within the bacteria populations obtained from different treatments. This study points out that minimum tillage and mulching are attractive soil agronomic management practices since they increase soil microbial biomass and bacterial diversity, which are key indicators for soil fertility.
Data Availability Statement
The original contributions presented in the study are included in the article/supplementary material, further inquiries can be directed to the corresponding author/s.
Author Contributions
MN performed the laboratory work, field experiments, data collection and analyses. EN and MM-M provided technical guidance on setting up of the research experiments. All authors contributed to the article and approved the submitted version.
Conflict of Interest
The authors declare that the research was conducted in the absence of any commercial or financial relationships that could be construed as a potential conflict of interest.
Acknowledgments
The authors wish to express their appreciation to International Atomic Energy Agency (IAEA, grant 16916) for the financial support in this study. We are also grateful to Mr Antony Njagi for his technical support in management of the field experiments.
References
Alguacil, M. M., Lumini, E., Roldan, A., Salinas-Garcia, J. R., Bonfante, P., and Bianciotto, V. (2008). The impact of tillage practices on arbuscular mycorrhizal fungal diversity in subtropical crops. Ecol. Applications 18, 527–536. doi: 10.1890/07-0521.1
Baaru, M. W., Mungendi, D. N., Bationo, A., Verchot, L., and Waceke, W. (2007). “Soil microbial biomass carbon and nitrogen as influenced by organic and inorganic inputs at Kabete, Kenya,” in Advances in Integrated Soil Fertility Management in Sub-Saharan Africa: Challenges and Opportunities (Dordrecht: Springer), 827-832. doi: 10.1007/978-1-4020-5760-1_78
Bailey, V., Peacock, A., Smith, J., and Bolton, H. (2002). Relationship between soil microbial biomass determined by chloroform fumigation-extraction, substrate-induced respiration and phospholipid fatty acid analysis. Soil Biol. Biochem. 34, 1385–1389. doi: 10.1016/S0038-0717(02)00070-6
Balota, E. L., Colozzi-Filho, A., Andrade, D. S., and Dick, R. P. (2003). Microbial biomass in soils under different tillage and crop rotation systems. Biol. Fertil. Soils 38, 15–20. doi: 10.1007/s00374-003-0590-9
Bolo, P., Kihara, J., Mucheru-Muna, M., Njeru, E. M., Kinyua, M., and Sommer, R. (2021). Application of residue, inorganic fertilizer and lime affect phosphorus solubilizing microorganisms and microbial biomass under different tillage and cropping systems in a Ferralsol. Geoderma 390:114962. doi: 10.1016/j.geoderma.2021.114962
Cavaglieri, L., Orlando, J., and Etcheverry, M. (2009). Rhizosphere microbial community structure at different maize plant growth stages and root locations. Microbiol. Res. 164, 391–399. doi: 10.1016/j.micres.2007.03.006
Cerny, J., Balik, J., Pavlíkova, D., Zitkova, M., and Sykora, K. (2003). The influence of organic and mineral nitrogen fertilizers on microbial biomass nitrogen and extractable organic nitrogen in long-term experiments with maize. Plant Soil Environments 49, 560–564. doi: 10.17221/4194-PSE
Dash, H. R., and Das, S. (2018). “Molecular methods for studying microorganisms from atypical environments,” in Methods in Microbiology (Madhya Pradesh: Academic Press), 45, 89–122. doi: 10.1016/bs.mim.2018.07.005
Di Cello, F., Bevivino, A., Chiarini, L., Fani, R., Paffetti, D., Tabacchioni, S., et al. (1997). Biodiversity of a Burkholderia cepacia population isolated from the maize rhizosphere at different plant growth stages. Appl. Environ. Microbiol. 63, 4485–4493. doi: 10.1128/AEM.63.11.4485-4493.1997
Diallo-Diagne, N. H., Assigbetse, K., Sall, S., Masse, D., Bonzi, M., Ndoye, I., et al. (2016). Response of soil microbial properties to long-term application of organic and inorganic amendments in a tropical soil (Saria, Burkina Faso). Open J. Soil Sci. 6:21. doi: 10.4236/ojss.2016.62003
Fuentes, M., Govaerts, B., Hidalgo, C., Etchevers, J., González-Martín, I., Hernández-Hierro, J. M., et al. (2010). Organic carbon and stable 13C isotope in conservation agriculture and conventional systems. Soil Biol. Biochem. 42, 551–557. doi: 10.1016/j.soilbio.2009.11.020
Habig, J., and Swanepael, C. (2015). Effects of conservation agriculture and fertilization on soil microbial diversity and activity. Environments 2, 358–384. doi: 10.3390/environments2030358
Hajabbasi, M. A., and Schumacher, T. E. (1994). Phosphorus effects on root growth and development in two maize genotypes. Plant Soil 158, 39–46. doi: 10.1007/BF00007915
He, Y., Qi, Y., Dong, Y., Xiao, S., Peng, Q., Liu, X., et al. (2013). Effects of nitrogen fertilization on soil microbial biomass and community functional diversity in temperate grassland in Inner Mongolia, China. Clean–Soil Air Water 41, 1216–1221. doi: 10.1002/clen.201200021
Jaetzold, R., Schmidt, H., Homet, Z. B., and Shisanya, C. A. (2007). Farm Management Handbook of Kenya: Natural Conditions and Farm Information (2 nd ed., Vol. 11/C). Nairobi: Eastern Province, Ministry of Agriculture/ GTZ.
Johnston-Monje, D., Lundberg, D. S., Lazarovits, G., Reis, V. M., and Raizada, M. N. (2016). Bacterial populations in juvenile maize rhizospheres originate from both seed and soil. Plant Soil 405, 337–355. doi: 10.1007/s11104-016-2826-0
Kabir, Z. (2004). Tillage or no-tillage: Impact on mycorrhizae. Canadian J. Plant Sci. 85, 23–29. doi: 10.4141/P03-160
Kara, O., and Bolat, L. (2008). The effect of different land uses on soil microbial biomass carbon and nitrogen in bartin province. Turkey J. Agric. 32, 281–288. doi: 10.1002/ldr.850
Kiboi, M. N., Ngetich, K. F., Mugendi, D. N., Muriuki, A., Adamtey, N., and Fliessbach, A. (2018). Microbial biomass and acid phosphomonoesterase activity in soils of the Central Highlands of Kenya. Geoderma Regional 15, 1–10. doi: 10.1016/j.geodrs.2018.e00193
Klikocka, H., Narolski, B., Klikocka, O., Glowacka, A., Juszezka, D., Onuch, J., et al. (2012). The effect of soil tillage and nitrogen fertilization on microbiological parameters of soil on which Spring Triticale is grown. Poland J. Environmental Stud. 21, 1675–1685. doi: 10.17221/14/2014-pse
Koeleman, J. G. M., Stoof, J., Biesmans, D. J., Savelkoul, P. H. M., and Vandenbroucke-Grauls, C. M. J. E. (1998). Comparison of amplified ribosomal DNA restriction analysis, Random amplified polymorphic DNA analysis and amplified fragment length polymorphism fingerprinting for identification of Acinetobacter Genomic species and Typing of Acinetobacter baumannii. J. Clin. Microbiol. 36, 2522–2529. doi: 10.1128/JCM.36.9.2522-2529.1998
Lei, Y., Xiao, Y., Li, L., Jiang, C., Zu, C., Li, T., et al. (2017). Impact of tillage practices on soil bacterial diversity and composition under the tobacco-rice rotation in China. J. Microbiol. 55, 349–356. doi: 10.1007/s12275-017-6242-9
Mader, P., Edenhofer, S., Boller, T., Wiemken, A., and Niggli, U. (2000). Arbuscular mycorrhizae in a long-term field trial comparing low-input (organic, biological) and high-input (conventional) farming systems in a crop rotation. Biol. Fertility Soils 31, 150–156. doi: 10.1007/s003740050638
Monis, P. T., Giglio, S., and Saint, C. P. (2005). Comparison of SYTO9 and SYBR Green I for real-time polymerase chain reaction and investigation of the effect of dye concentration on amplification and DNA melting curve analysis. Anal. Biochem. 340, 24–34. doi: 10.1016/j.ab.2005.01.046
Mucheru-Muna, M., Mugendi, D., Kung'u, J., Mugwe, J., and Bationo, A. (2007). Effects of organic and mineral fertilizer inputs on maize yield and soil chemical properties in a maize cropping system in Meru South District, Kenya. Agroforestry Systems 69, 189–197. doi: 10.1007/s10457-006-9027-4
Mucheru-Muna, M., Mugendi, D., Pypers, P., Mugwe, J., Kung'u, J., Vanlauwe, B., et al. (2014). Enhancing maize productivity and profitability using organic inputs and mineral fertilizer in central Kenya small-holder farms. Exp. Agric. 50, 250–269. doi: 10.1017/S0014479713000525
Mugendi, D. N., Mucheru-Muna, M., Mugwe, J., Kung'u, J., and Bationo, A. (2007). Improving food production using “best bet” soil fertility technologies in the Central Highlands of Kenya. Advances in Integrated Soil Fertility Management in Sub-Saharan Africa: Challenges Opportunities 1996, 345–351. doi: 10.1007/978-1-4020-5760-1_31
Mugwe, J., Mugendi, D., Mucheru-Muna, M., Merckx, R., Chianu, J., and Vanlauwe, B. (2009). Determinants of the decision to adopt intergrated soil fertility management practices by smallholder farmers in Central highlanda of Kenya. Exp. Agric. 45, 61–75. doi: 10.1017/S0014479708007072
Mwenda, G. M., Karanja, N. K., Boga, H., Kahindi, J. H. P., Muigai, A., and Odee, D. (2011). Abundance and diversity of legume nodulating Rhizobia in soils of Embu district, Kenya. Trop. Subtropical Agroecosystems 13, 1–10. doi: 10.1016/j.syapm.2018.02.001
Nakhro, N., and Dkhar, M. S. (2010). Populations and biomass carbon in paddy field soil. Agron. J. 9, 102–110. doi: 10.3923/ja.2010.102.110
Ngetich, K. F., Mucheru-Muna, M., Mugwe, J. N., Shisanya, C. A., Diels, J., and Mugendi, D. N. (2014). Length of growing season, rainfall temporal distribution, onset and cessation dates in the Kenyan highlands. Agric. Forest Meteorol. 188, 24–32. doi: 10.1016/j.agrformet.2013.12.011
Odee, D. W., Haukka, K., Mclnroy, S. G., Sprent, J. I., Sutherland, J. M., and Young, J. P. W. (2002). Genetic and symbiotic characterization of rhizobia isolated from tree and herbaceous legumes grown in soils from ecologically diverse sites in Kenya. Soil Biol. Biochem. 34, 801–811. doi: 10.1016/S0038-0717(02)00009-3
Panigrahi, S., Velraj, P., and Rao, T. S. (2019). “Functional microbial diversity in contaminated environment and application in bioremediation,” in Microbial Diversity in the Genomic Era (Tamil Nadu: Academic Press), 359–385. doi: 10.1016/B978-0-12-814849-5.00021-6
Reji, P. M., Feng, Y., Githinji, L., Ankumah, R., and Balkcom, K. S. (2012). Impact of no-tillage and Conventional tillage systems on soil microbial communities. Appl. Environmental Soil Sci. 2012:548620. doi: 10.1155/2012/548620
Rohwer, F., Breitbart, M., Jara, J., Azam, F., and Knowlton, N. (2001). Diversity of bacteria associated with the caribbean coral montastrae franksi. Coral Reefs 20, 85–91. doi: 10.1007/s003380100138
Shaheen, A., and Sabir, N. (2017). Effect of tillage, residue and fertilizer on yields within a wheat-maize cropping system. Sarhad J. Agric. 33, 127–138. doi: 10.17582/journal.sja/2017.33.1.127.138
Silva, A. P., Babujia, L. C., Matsumoto, M. F., and Guimaraes Hungria, M. (2013). Bacteria Diversity under different tillage and crop rotation systems in an Oxisol of Southern Brazil. Open Agric. J. 7, 40–47. doi: 10.2174/1874331501307010040
Tahat, M. M., and Sijam, K. (2012). Mycorrhizal fungi and abiotic environmental conditions relationship. Res. J. Environmental Sci. 6:125. doi: 10.3923/rjes.2012.125.133
Yeboah, S., Zhang, R., Cai, L., Li, L., Xie, J., Luo, Z., et al. (2016). Tillage effect on soil organic carbon, microbial biomass carbon and crop yield in spring wheat-field pea rotation. Plant Soil Environment 62, 279–228. doi: 10.17221/66/2016-PSE
Keywords: soil microbial biomass C and N, bacteria, fungi, actinobacteria, amplified ribosomal DNA restriction analysis
Citation: Nyamwange MM, Njeru EM and Mucheru-Muna M (2021) Tillage, Mulching and Nitrogen Fertilization Differentially Affects Soil Microbial Biomass, Microbial Populations and Bacterial Diversity in a Maize Cropping System. Front. Sustain. Food Syst. 5:614527. doi: 10.3389/fsufs.2021.614527
Received: 13 October 2020; Accepted: 15 March 2021;
Published: 16 April 2021.
Edited by:
Adam C.N. Wong, University of Florida, United StatesReviewed by:
Andrey S. Zaitsev, University of Giessen, GermanyChunjie Tian, Chinese Academy of Science, China
Copyright © 2021 Nyamwange, Njeru and Mucheru-Muna. This is an open-access article distributed under the terms of the Creative Commons Attribution License (CC BY). The use, distribution or reproduction in other forums is permitted, provided the original author(s) and the copyright owner(s) are credited and that the original publication in this journal is cited, in accordance with accepted academic practice. No use, distribution or reproduction is permitted which does not comply with these terms.
*Correspondence: Methuselah Mang'erere Nyamwange, bm1ldGh1MTk5MkBnbWFpbC5jb20=