- 1Department of Sciences and Engineering of Biosystems, Instituto Superior de Agronomia, Universidade de Lisboa, Lisboa, Portugal
- 2LEAF, Linking Landscape, Environment, Agriculture and Food, Instituto Superior de Agronomia, Universidade de Lisboa, Lisboa, Portugal
- 3CMAF-CIO, Center of Mathematics, Fundamental Applications and Operations Research, Faculdade de Ciências, Universidade de Lisboa, Lisboa, Portugal
- 4Nestlé Portugal, UNIPESSOAL, LDA–Department of Security, Health and Environmental Sustainability (SHE), Linda-a-Velha, Portugal
In the past years, there has been steady growth in work relating to improve resource efficiency through waste minimization and bioenergy recovery to mitigate climate change. Agro-food industries produce large amounts of bio-waste, challenging innovative energetic valorization strategies in the framework of circular economy principles. Anaerobic digestion (AD) technology is an interesting route to stabilize organic matter and produce biogas as a renewable energy source. This study involves continuous co-digestion of pig slurry (PS), cereal and exhausted coffee wastes (CECW) performed in a continuously stirred tank reactor, with a hydraulic retention time (HRT) of 16 days under at mesophilic conditions (36.9 ± 0.3°C). The experimental trials, were designed to include different cereal and exhausted coffee liquor (CECL) shares in the feeding mixture, corresponding to different PS to CECL ratios (PS:CECL), respectively: 100:0 (T0), 90:10 (T1), 80:20 (T2), and 70:30 (T3), in terms of percentage of inlet feeding rate (v:v). The results obtained for the feeding rate (70:30) yield to the highest specific methane production (SMP = 341 ml.gVS−1) led to a 3.5-fold improvement in comparison with the reference scenario. The synergetic effect between the microbial consortia of PS and the high carbon to nitrogen ratio (C/N) of CECL explain the improvements achieved. The maximum soluble chemical oxygen demand (SCOD) reduction (84.0%) due to the high content and soluble chemical oxygen demand to total chemical oxygen demand ratio (SCOD/TCOD) corroborate the results achieved. The digester stability, evaluated by specific energetic loading rate, was below the limit (0.4 d−1). Results from ANOVA showed a significant effect of CECL on the resulting GPR and SMP values. Additionally, Tukey's “Honest Significant Difference” method, confirmed statistically significant differences between the trials T3-T0, T3-T1, T3-T2, and T2-T0. Thus, co-digestion of PS and of CECL seems to be a promising approach for bioenergy recovery and promoting biowastes circularity.
Introduction
Population explosion and economic growth have led to another problem: a large amount of solid waste generation. According to the World Bank, an increase of 70% from the 2.01 billion tons in 2016 to 3.40 billion tons in 2050 worldwide can be predicted (Kaza et al., 2018). The disposal of organic waste disposal has a significant contribution to global warming due to the greenhouse gases emissions, such as methane (CH4), carbon dioxide (CO2), and nitrous oxide (N2O).
According to Dima et al. (2020), the bioresidues conversion indicates that anaerobic digestion (AD) technology is an interesting route to environmental pollution minimization. It's important to highlight the main advantages, since it is a process eco-friendly, cheaper, and affordable allowing a wide range of uses, such as: the combined production of heat and power, injection into the natural grid or as a fuel in combustion engines (Luz et al., 2017; Rodrìguez-Abalde et al., 2017; Oladejo et al., 2020).
The production of biogas by means of AD of organic wastes hold a lot of promise for a functional green bioeconomy, while reducing organic load and pollutants to the environment (Dima et al., 2020).
Pig is the most consumed animal protein in the world and the global production was approximately 111 thousand tons until June 2020 (FAO, 2020). With the trend of intensification of swine production, swine manure becomes a significant environmental concern in many countries since it contains high concentrations of nitrogen (N), phosphorus (P) in addition to organic matters and creates odor problems. As a result, farm-based swine manure management methods have become essential to protect the immediate environment at the source of production as an alternative to collecting manure from feedlots and storing in basins or pits. Anaerobic digestion is a widely used biotechnology to treat organic wastes. AD technology is considered as one of the most conventional processes in livestock waste management due to the process simplicity and feedstock flexibility. However, using animal manure as a sole feedstock, particularly swine manure, may not be the most efficient way to produce methane-rich biogas because of its inherent low carbon/nitrogen ratio and because it carries certain constrains, as complex materials require longer retention times and larger digestion volumes (Ros et al., 2017; Lopes et al., 2019; Duarte et al., 2021).
The world coffee consumption between 2019 and July 2020 was approximately 10,140,000 tons (ICO—International Coffee Organization, 2020), being after tea the second more consumed beverage (Battista et al., 2020; Rivera et al., 2020). The use of bioresidues from coffee chain is of utmost importance due to the large quantity produced. For each kilogram of coffee beans used are generated 1.88 kg spent coffee grounds (SCG), which are produce through instant coffee industry and consumption in catering outlets and homes (Battista et al., 2020; Rivera et al., 2020). Over 90% of SCG is currently discarded as a valueless waste, although some is used as a boiler fuel after drying or as a mushroom cultivation medium in some countries. This procedure is largely due to the lack of efficiency methods to cope with the enormous amount of SCG produced. Improper management of SCG can cause serious pollution because of the high oxygen consumption during decomposition of the easily degradable organic matter and the potential release of residual caffeine, tannin, and polyphenols. Given its high organic content (i.e., high calorific value), the potential of using SCG as a feedstock for biofuels is an interesting question in the context of waste-to-energy conversion (Kim et al., 2017a,b; Luz et al., 2017; Battista et al., 2020; Rivera et al., 2020).
The livestock co-digestion with other organic waste sources, combined with good manure management practices, have shown an improvement in the economic feasibility in many individual or centralized agro-biogas installations (Rodrìguez-Abalde et al., 2017). The anaerobic co-digestion (AcoD) of two or more substrates can overcome the drawbacks of mono-digestion. This approach is viable for animal manures, which have low carbon to nitrogen ratios (C/N) promoting the Archaea methanogenic. On the other hand, agro-industrial wastes may need nitrogen to improve the economic viability of the AD units to enhance biomethane yield (Kim et al., 2017a). The successful of the co-digestion strategies resides in the selection of co-substrates that must be showing complementary characteristics (Rodrìguez-Abalde et al., 2017; Dima et al., 2020). Anaerobic co-digestion has a role of advantages like process stabilization (balancing the C/N ratio, pH, and solid content) process improvement (buffer capacity, wide the ranges of microorganisms involved, and dilute the inhibitory compounds), and higher quality of methane (Martínez-Ruano et al., 2019; Ahn et al., 2020; Dima et al., 2020).
Anaerobic digestion is a key process in the development of a circular economy, which is a concept that has gained more and more relevance since it covered three dimensions: environmental, economic, and social. This approach aims to promote and implement flows circularity, in order to reduce environmental impacts and at the same time to maximize resource efficiency (zero waste concept) (Ingrao et al., 2018; Barros et al., 2020; Kapoor et al., 2020).
The goals of circular economy are minimization or elimination of input materials from fossil or non-renewable sources, reduction of greenhouse gases emissions through the circulation of raw materials, agricultural waste and manure, production of clean energy, and increase energy efficiency reducing costs (Barros et al., 2020; Ubando et al., 2020). Nowadays, the global circular economy only comprises 9%, but there is a possibility of reaching 100% (Barros et al., 2020). However, recently, concepts and perceptions such as circular economy, green economy, steady-state economy, and bio-economy are emerging from industry and academia to take care of sustainability alterations and to resolve environmental and socio-economic goals (D'Amato et al., 2019).
In the present research work, a suitable management process for both cereal and exhausted coffee wastes (CECW) and pig slurry (PS) from a closed cycle farm unit is proposed. To this end, a series of bench-scale experiments are conducted to compare the system response in terms of biogas and biomethane production yield upon co-digestion of the PS with different cereal and exhausted coffee liquor (CECL) shares, in contrast with mono-digestion of PS. Cereal and exhausted coffee waste was formerly mechanical pre-treated by a filtration process for extraction of high added-value soluble organic compounds, which would help revalorize this by-product from a material point of view and subsequently facilitate its energetic valorization through the proposed AcoD process. Statistical analysis of the results suggested that the integration of this by-product as co-substrate in AD of PS helps to enhance the circular bioeconomy target in both activities' sectors. To the authors' knowledge, no previous research work focused the role of PS co-digestion of with CECL as a promising approach for bioenergy recovery and promoting biowastes circularity.
Materials and Methods
Origin and Collection of Cereal and Exhausted Coffee Wastes
Cereal and exhausted coffee wastes (CECW) were obtained from the manufacturing process of soluble coffee products (simplified scheme in Figure 1) from the Avanca factory (40°80′51″N 8°57′52″W), one of three Nestlé Portugal's factories. Cereal and exhausted coffee wastes are made of bulk barley (54%), roasted dehydrated chicory (25%), malted barley (10%), roasted coffee (8%), and rye (3%). The 10 fresh samples, collected along the experimental runs, were conditioned in 5 kg plastic containers. After this procedure each lot was transported and delivered to the research unit for the experimental work development. The expedition of each lot occurs according to the assays running period (T1, T2, and T3). For the trial T1, the lot includes three samples; for the trial T2, four samples; and for the trial T3, three samples (all the samples are representative of the CECW generated in the industrial process). At the Lab facilities, the CECW samples were stored at 4°C for further characterization.
Origin and Collection of Pig Slurry
Pig slurry (PS) was from a swine livestock facility located in the Santarém District, Portugal (38°97′65″N 8°68′13″W), with a total area of 212.72 ha and capacity for: 500 breeding sows, 1,620 weaned piglets, and 4,000 fattening piglets. This pig farm works in a closed-cycle operation and the production is divided into four stages: gestation, farrowing, weaning, and fattening/finishing. The slurry management system of the farm includes a storage tank, solid-liquid separation, and a lagoon system. Multiple grab samples, collected from the storage tank, after mixing with a stirring device over a period, were selected according to flow rate. In situ, the temperature measurement of the different composite sample was registered. All samples were conditioned in thermal containers and delivered to Lab facilities for storage at 4°C for further analytical characterization.
Mechanical Pre-treatment
As to facilitate the degradation process (by increasing the surface area of the feeding stock) and to avoid clogging problems and floating layers inside the digester, a mechanical pre-treatment process was carefully delineated, as shown in Figures 2A–C.
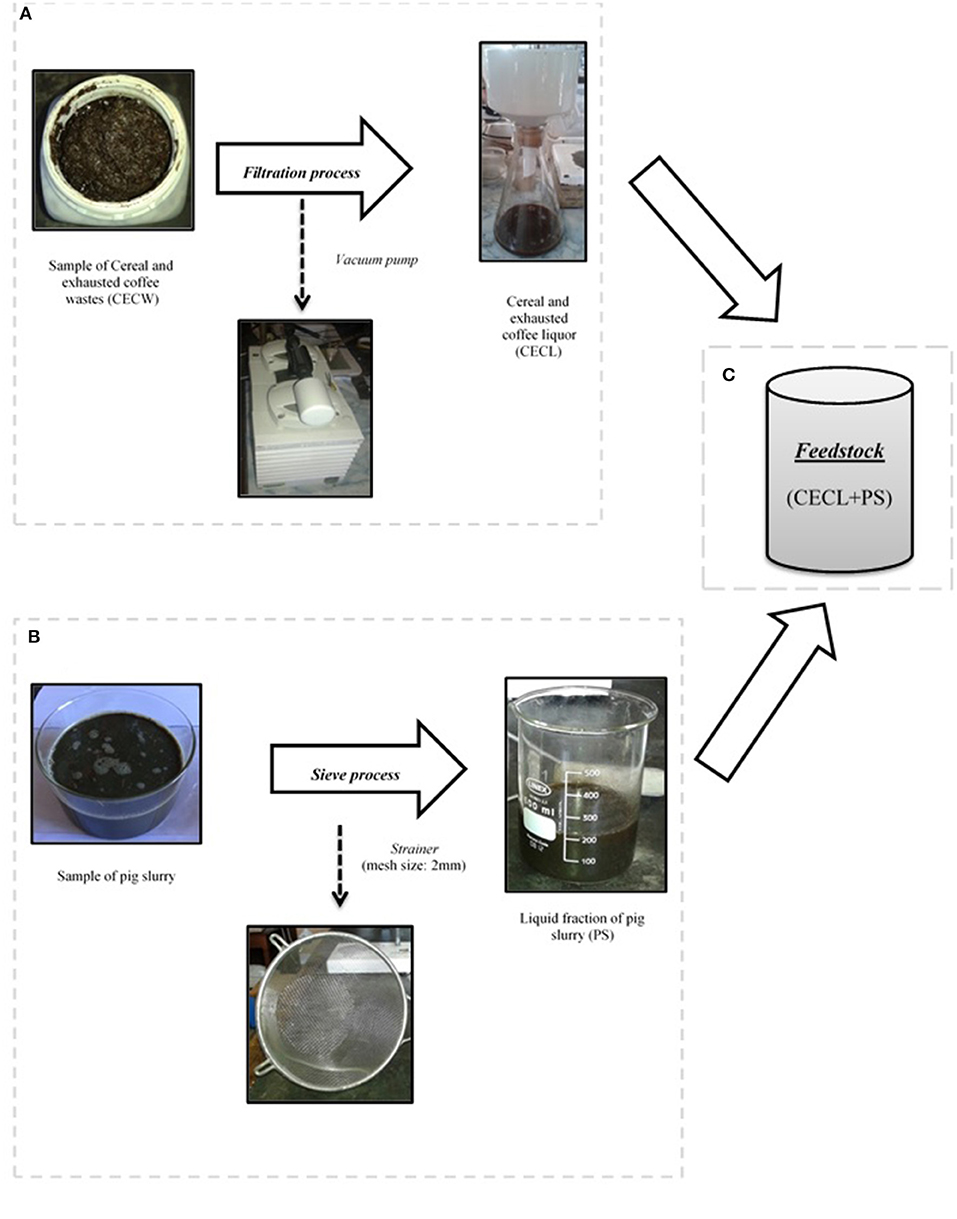
Figure 2. Scheme of the mechanical pre-treatment process of CECW (A) and pig slurry (B) and preparation of feedstock samples (C).
In the first step, the CECW samples from different lots were weighted in a digital balance, with an accuracy of ±0.005 g. The average values, for TS and TVS obtained for the three lots were, respectively; 133.13 ± 2.30 (g kg−1) and 128.33 ± 1.30 (g kg−1) for the lot used in trial T1; 145.21 ± 3.30 (g kg−1) and 140.56 ± 2.36 (g kg−1) for the lot used in trial T2, and 243 ± 4.32 and 237.10 ± 3.38 (g kg−1) for the lot used in trial T3. The variability is justified by the sample collection period (August, 2015 to February, 2016).
The next step was a filtration process using a vacuum pump (Büchi Vac V-500; 230 VAC; 80 Hz; 240 W). The efficiency of the liquid fraction (CECL) recovery used as co-substrate in AcoD trials, based on the mass balance calculated were, respectively: 81.5% (TS) and 82% (TVS) for samples used in trial T1; 71.8% (TS) and 72.1% (TVS) used in trial T2; and 61.9% (TS) and 62.5% (TVS) used in trial T3 (Figure 2A).
For removal of remnants grains and coarse material, the raw 12 PS samples passed through a strainer with a size mesh of 2 mm. The solid fraction removed was also weighted and amounted to 0.39 g (T0); 0.30 g (T1 and T2); and 0.12 g (T3), corresponding to 2.0, 1.6, and 1% of the total samples weight used in the trials T0, T1, T2, and T3, respectively. The efficiency recovery of the PS liquid fraction was 98.0, 98.4, and 99%, which are stored at 4°C to further characterization, and feedstock preparation for the experimental runs (Figure 2B).
The next step includes the preparations of the feedstock samples. According to the experimental design, for each trial they are, respectively: 100(PS):0(CECL) —T0; 90(PS):10(CECL) —T1; 80(PS):20(CECL) —T2; 70(PS):30(CECL) —T3. The blends were prepared on a volume basis (Figure 2C).
Analytical Methods
Three replicate samples of each substrate/co-substrate, feeding mixture and digestate were analyzed: pH, electrical conductivity (EC), total solids (TS), total volatile solids (TVS), total volatile suspended solids (TVSS), total chemical oxygen demand (TCOD), soluble chemical oxygen demand (SCOD), total Kjeldahl nitrogen (TKN), and total ammonium nitrogen (TAN) in accordance with Standard Methods of the American Public Health Association (APHA—American Public Health Association, 2017). Total organic carbon (TOC) was calculated based on the method described by Cuetos et al. (2011). C/N ratio was determined by dividing the TOC by the TKN values. All analytical determinations were performed with analytical grade reagents (≥99% purity).
Lab-Scale Anaerobic Digestion Essays
Substrates, Feeding Mixtures, and Digestates Characterization
The results obtained for the physicochemical characterization of the substrate and co-substrate used along the four trials (each one includes three HRT's) were presented in Table 1. Before the beginning of each cycle, the feeding mixtures were characterized. For comparison purposes, each new PS sample used on the mixture was analyzed for understanding the characteristics variability and the modifications provoked by the addition of CECL along the trials.
In the reference trial (T0), only PS was using as feeding stock. In the following three periods, the feeding mixtures (T1, T2, and T3) were prepared with PS and CECL in different proportions (PS:CECL): 90:10 (T1); 80:20 (T2), and 70:30 (T3), in terms of inlet (v:v).
Table 2 summarizes the results obtained.
Lab-Scale Experiment
The components of the AD lab-scale unit are present in Figure 3, which comprise a cylindrical semi-continuously stirred tank reactor (CSTR) constructed from stainless steel with a total volume of 6.86 L and a working volume of 4.89 L. The working volume is kept constant through hydrostatic pressure: the volume of feed is equal to the volume of digestate that is collected.
The reactor includes a:
• mixer (stirring system—Fisatom Model 712, 50 W, 230 V, with speed range 0–20 × 100 rpm);
• heating system (ERT; power: 220 V; command voltage: 5 V);
• mechanical stirrer (Velp Scientifica, 50 rpm, 60 W);
• feeding pump (Watson Marlow, 120 rpm) and
• gas flowmeter (Milligascounter—Ritter, Germany) with an accuracy of ±3%.
The heating system comprises a heating stirring and feed control interface (inside there are four components: control board, solid state relays, normal relay, and communication interface with computer), and is controlled by a thermostat with an accuracy of ±0.5°C, which served to keep the temperature range at 36.9 ± 0.3°C. This value was based on previous studies developed and published by the authors, to enhance biogas and methane production for PS with other co-substrates (Silva et al., 2020; Azevedo et al., 2021).
The top of the CSTR digester was fitted with a tube to allow the transport of biogas to further quantification in a flowmeter (biogas is collected on a gasholder). Besides, the digester is equipped with one electrode to measure the pH and a thermocouple to register the temperature inside the reactor. The composition of the obtained biogas stream—% v/v of methane (CH4), carbon dioxide (CO2) and nitrogen (N2), as well as hydrogen sulfide (H2S) in ppm—was provided by a portable biogas quality analyzer (LMSxi Multifunction Landfill Gas Analyzer), with an accuracy of ±3% and a detection range for H2S between 200 and 1,500 ppm. A programmable logic controller (PLC) system (ERT–Model K8055) was installed to control mechanical stirrers of feeding tanks and AD reactor, pumps and a heating system, which allows remote monitoring.
The reactor-feeding regime occurs once a day and always at the same time, from Monday to Friday (no feeding during the weekend or bank holidays) along three hydraulic retention times (HRT) of 16 days, under steady state conditions, for each of the four trials considered. The stirring system is composed of a frame agitator and three oblique blades (8–10 rpm) installed on the top of the reactor. The automatic program for the stirring procedure comprises the following steps: (i) activated 2 min before the feeding; (ii) activated along the feeding and the collection of the digestate; (iii) more 3 min; (iv) 3 min four times a day to homogenize the biomass and promote biogas release inside the digester. The program allows the adjustment of set points, namely at the temperature parameter, through a thermal blanket that turns on and off as the minimum and maximum values are reached (36.5–37.5°C) and also activated the stirring inside the reactor. These set-points help to avoid scum/foam formation.
All the relevant parameters were followed for 24 h (during working days), and then a new feed was provided. The feedstock container of the CSTR was stored at 4°C to maintain constant characteristics prior to the anaerobic treatment. Mixed liquor (digestate) samples were drawn and analyzed for monitoring the reactor performance under semi-continuous stirring conditions. Since during the idle period there is no feeding, so the GPR readings results only from consumption by the biomass. This response is due to biomass exposition to variable concentrations, which ultimately induces to contrasting situations: feast conditions, and famine conditions, in the absence of external feeding.
Operational Parameters and Removal Efficiencies
The experimental procedure comprised two main phases, consecutively performed: first, anaerobic mono-digestion (AD) with 100% PS as feedstock, representing the reference scenario (T0); thereafter, AcoD using a feeding mixture of 90:10, 80:20, and 70:30 (v: v) PS:CECL was monitored. The effect driven by the addition of CECL on the feeding stream (in-put) and biogas/biomethane production (out-put) aiming to evaluate the bioreactor performance.
The total monitoring period of the study lasted 192 days, corresponding to 12 cycles, with HRT of 16 days for each cycle. Although the HRT is too short for PS, in the case of the study presented fits well attending to the physico-chemical composition of the pig manure generated in the farm unit, which presents a very low ST and SV concentration and, consequently a very low OLR. Previous studies published by the authors Duarte et al. (2021) support this choice. During all the cycles, the process was controlled to maintain appropriate mesophilic conditions (36.9 ± 0.3°C). The start-up of the experimental study lasted 1 month, which corresponded to the AD phase of PS, comprising two complete operating cycles. Once this stage was completed, AcoD with CECL was initiated and conducted for three consecutive runs (same HRT = 16 days). The AcoD cycles served to observe the effect of the CECL co-substrate addition on the performance of the bioreactor.
The measurements of inlet and outlet flow rates, reactor temperature and biogas production, were registered daily, while biogas quality was analyzed once a week. The feeding mixtures were fully monitored along the trials, in terms of pH, EC, TS, TVS, TCOD, SCOD, TKN, and C/N, to control process performance. The digestates characterizations allow the determination of the removal efficiencies and the digester stability. The biomass production inside the tank was followed during the trials throughout TVSS concentration in the respective digestate collected. The operational parameters—organic loading rate (OLR), biogas production rate (GPR), methane production rate (MPR), specific methane production (SMP), and specific energy loading rate (SELR)—were determined twice per cycle. The specific energy loading rate (SELR, gCOD d−1 gVSS−1) refers to the ratio between the daily average fed organic load (expressed in TCOD) and the active biomass inside the reactor (expressed in TVSS) (Pinto et al., 2016), related to the methanogenic activity. The Equation (1) illustrates this relationship.
Specific energy loading rate:
in which: Q: inlet flow rate (L d−1); [TCOD]: feed total COD concentration (g L−1); [VSS]: digestate volatile suspended solid concentration (g L−1); Vworking: working volume in the reactor (L) and where: Q × [TCOD]inlet: feed organic load (expressed as total COD) and [VSS] × Vworking: mass of the biomass inside the reactor.
Operating and process parameters for each Lab-scale trial are presented in Table 3, which results show are an average of the values presented in Table 2 for the three HRTs considered. It is important to highlight the standard deviations justified by the variability of the samples composition linked with the sample collection period.
Characterization of Statistical Analysis
Statistical analysis was performed using the software (http://www.R-project.org/). Analysis of variance (ANOVA) was used to check if the trials (T0, T1, T2, and T3) were significantly different concerning the GPR (mlbiogas L−1 reactor d−1) and SMP (mlCH4 ) produced. Data sets were balanced with three observations for each trial, being each observation the mean of the operational parameter values in a run with HRT = 16 days.
Additionally, to analyze which trials were significantly different from each other, Tukey's “Honest Significant Difference” method (TukeyHSD) was applied to obtain a set of confidence intervals for all pairwise.
Results and Discussion
Substrates, Feeding Mixtures, and Digestates Characterization
By analyzing Table 1 that shows the physico-chemical characterization of PS and CECL used in trials (which results presented as averages and ranges of triplicate analytical measurements), we can highlight that CECL presents a C/N ratio, approximately, five times higher in comparison with PS performed as mono-substrate. This pin-point that this by-product (CECW), from the manufacturing process of soluble coffee products, has a potential co-substrate to provide more appropriate carbon to nutrient ratio in the feeding blend in order to help improve the conversion process and methane yield (Hagos et al., 2017; Li et al., 2019; Rivera et al., 2020).
Table 2 presents the results concerning the characterization of the feeding mixtures and digestates of the trials performed. The values for TS/TVS ratio had an increment through the three regimes (13, 18, and 34%), respectively with a mean value of 82.0 ± 0.2, which are higher to the values found in literature: 75.7% (Yang et al., 2019).
There is an increase in the SCOD/TCOD ratio over feedings from AD and AcoD essays, being the average values achieved in trial T3, and 29% higher in comparison with T0 values. This increase suggests a higher bioavailability of the substrate for anaerobic microorganisms and, consequently, a higher biogas production.
Moreover, CECL feeding mixture along trial T3 presents a higher content in soluble organic matter (SCOD) than in AD-PS trial that is a three-fold increase. Although the addition of CECL improved TCOD and SCOD contents, when compared with T0, nevertheless, a slightly decrease occurs during trial T1 due to the change of environmental conditions at the livestock facility, when the rain significantly dilutes the slurry in the slurry management system.
As observed in Table 2, the addition of 10, 20, and 30% (v/v) CECL per liter of PS led to an increase in the OLR of the blend. Concretely, the OLR was set at 0.80 ± 0.05 gTVS L−1 reactor d−1 during AD experiments with PS (lasting three complete cycles), whereas it was increased to 1.01 ± 0.08 and 2.03 ± 0.13 gTVS L−1 reactor d−1, during T2 and T3 runs upon the addition of 20 and 30% CECL, respectively. The first phase of T1 with a slightly decrease in the OLR, could be justified by the microbial consortium adaptation to the incorporation of CECL. The analysis of the additional cycles allows the system response under the established conditions, to progressive shock loadings. The bioreactor performance, based on biogas production quality and digestate quality, as control parameters, was monitored along the trials T2 and T3, respectively.
As a result of the anaerobic process performed (AD), the obtained digestates showed a reduction of 52% in TVS if compared with the values of the feed stream (PS), whereas it is important to note that this value was enhanced up to 61% in AcoD runs with CECL (30% v/v) as co-substrate. This implies almost 20% TVS content bioconverted into biogas when occurs the addition of CECL to the feed blend mixture.
Operational Parameters and Removal Efficiencies
Table 3 illustrates the average values of the operational parameters along the trials AD and AcoD. This table summarizes the effect of CECL co-substrate incorporation on the performance and stability of PS AcoD process. In addition to this, the evolution of the process performance in time during the whole set of experiments is reported in Figure 4, in which GPR together with MPR are given vs. the average OLR in the bioreactor. During AD and AcoD trials, the OLR setting in a range of 0.80 ± 0.05 to 2.03 ± 0.13 gTVS L−1reactor d−1, maintaining the same HRT. In any case, the established OLR value complied with the recommendation reported by various researchers of avoiding feeding the anaerobic digester at OLR that can compromise in particular at stability and efficiency (Li et al., 2017; Siddique and Wahid, 2018; Sembera et al., 2019). Furthermore, the average daily GPR during the proposed AcoD regime reached up to 839 ± 6 ml L−1d−1, which implied a significant increase, equal to 2.7 times higher, when compared to the AD operational phase fed with PS as mono-substrate (GPRAD = 225 ± 3 ml L−1d−1). This result indicates that the composition and specific characteristics of each feedstock mixture significantly affect the AD process. It is important to highlight that the GPR value attained in the proposed co-digestion process of PS and CECL is 1.3 times higher than the ones referred by Girotto et al. (2017) and Slorach et al. (2019).
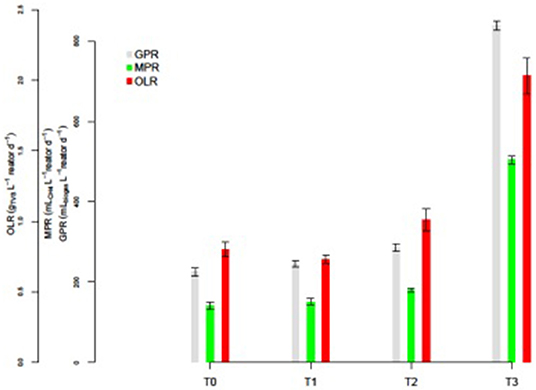
Figure 4. Biogas production rate (GPR), methane production rate (MPR), and average organic loading rate (OLR) during the operational time, where AD and AcoD correspond to the first and second experimental phases: AD of PS (T0) and AcoD of PS after introduction of CECL (T1, T2, and T3).
Furthermore, in terms of SMP (L CH4 ) as well as MPR (LCH4 d−1) the blend of PS with 30% (v/v) CECL provided considerable enhancements, equivalent to 1.6 and 3.6-fold increase, respectively, in comparison with reference scenario. Attending to other studies on AcoD bioconversion technology with biomass substrates the present study achieved higher values of SMP comparing with the application of food waste residues as co-substrates (Zamanzadeh et al., 2016; Girotto et al., 2017; Xie et al., 2017; Slorach et al., 2019; Battista et al., 2020). In addition, Kim et al. (2017b) performed a study with SCG and different waste feedstocks, achieving SMP below 450 ml , results 35% lower than the average values obtained in the present research. These results show that the addition of the CECL originating an increase of TOC, more specially, a 2.6 times the value from the initial trial (T0) to the third trial T3. The improvement of this value generates higher methane yields in the trials following T0, which contributes to balancing the feedstock composition, allowing better performance of the AD biotechnological process in accordance with referred by Ma and Liu (2019). This behavior could be probably, justified by the different feed regimes (feast and famine) applied during the experimental set-up. So and, taking in account the biomass response to this variability, the storage phenomenon can assume a direct conversion of all the readily biodegradable organic substrate into storage material during feast conditions.
As it can be observed in Figure 4, the microbial consortia in the bioreactor not only was able to tolerate the organic shock introduced by the addition of CECL to the feed blend with PS, but also responded positively and was able to adapt to this new co-substrate. This behavior can be explained by the system positive response to the pH values (3.5 ± 0.2) of CECL introduced in the AcoD feeding stream, mainly due to the higher buffer capacity of PS substrate. This is patent by the enhancement of bioconversion observed from the shift to co-digestion process (along the trials T1–T3 illustrated in Figure 4), corroborated by the increase and stabilization in GPR and MPR yields during various subsequent trials (T1, T2, and T3) in comparison with the reference mono-digestion phase T0 (2.7 and 2.6 times higher, respectively). Generally, changes in operational and environmental parameters including temperature, pH, OLR, HRT, ammonia toxicity, C/N, etc., impressively hence a syntrophic relationship between microorganisms and consequently the performance and instability of AD process (Fischer et al., 2019).
The data shown in Figure 5 establish the correlation between the key physico-chemical parameters to evaluate the bioreactor performance and stability along the trial operational time, referred by Fischer et al. (2019). Regarding pH, both digestates present values were slightly alkaline, around 7.4 with an exception for the trial T3 (pH = 7.0), due to the good buffering capacity of PS which, shows a good adaptation to a more acidic feeding in T3, indicating the existence of sufficient alkalinity to neutralize the volatile fatty acids (VFA) produced along the process. At the feeding level of the trial T3, the value obtained (6.2) is the threshold of what is acceptable for the digestion. For this reason, a fourth trial was not considered, as an increase in the proportion of CECL could destabilize the bacterial consortium or could increase of inhibitory compounds. Usually, to avoid the inhibition of methanogenic bacteria, one chooses to keep the pH close to neutrality.
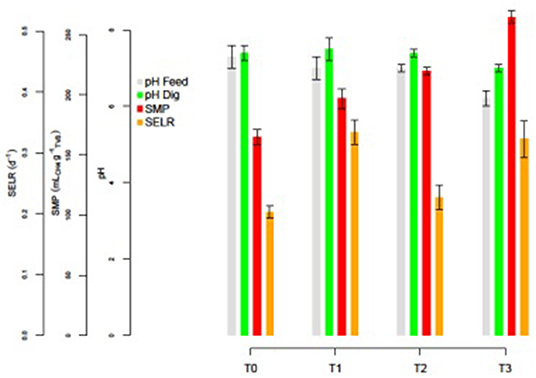
Figure 5. The pH of the feed stream and of the digestate, the specific methane production (SMP), and the specific energy loading rate of the system (SELR).
The VFA have not been measured, but this parameter was followed throughout the determination of crossed-linked parameters, such as pH, total alkalinity, ammonia nitrogen, organic matter removal efficiencies, and SELR to control process stability.
Concerning TAN concentrations, both digestates had values below the recommended limit of 1.7–1.8 g L−1 (Yenigün and Demirel, 2013). Furthermore, the digestate from the AcoD trial shows lower EC and TAN than the one from mono-digestion trial, what is clearly an advantage for further use of digestate. These values also indicate a good possibility of the digestate being used as a biofertilizer in the future, after being submitted to an organic amendment process to prevent nitrification of the soils (Sawada and Toyota, 2015). The results obtained by the authors are in accordance with Silva et al. (2020) and Azevedo et al. (2021), which performed similar AD trials and AcoD with PM with different co-substrates, in same operational conditions, such as HRTs and temperature.
The experimental results (Figure 6) showed that the biogas from trials T1, T2, and T3 AcoD runs contained higher average % of methane, respectively 61.5, 62.8, and 62.0%, than the biogas from experiment T0 (AD mono-substrate), which was on average 60.5%. These results are in accordance with the values obtained for the average % of carbon dioxide content in each of the trials T0, T1, T2, and T3, respectively, 39.4, 37.5, 36.5, and 37.8%. The residual percentage not detected in the biogas composition was probably due to the concentration of H2S, which was not accurately quantified. From a statistical point of view, Figure 6 shows that there are no significant differences in values of CH4, between trial T3 and trial T0, but trial T2 differs significantly from trial T0. Regarding values of CO2, any of the trials T1, T2, and T3, differs significantly from trial T0. The percentages obtained from biogas quality are in accordance with values of removal efficiencies as well as TVSS concentration, which is the biomass indicator. Overall, trial T3 recorded the best bioconversion of organic matter, as expected.
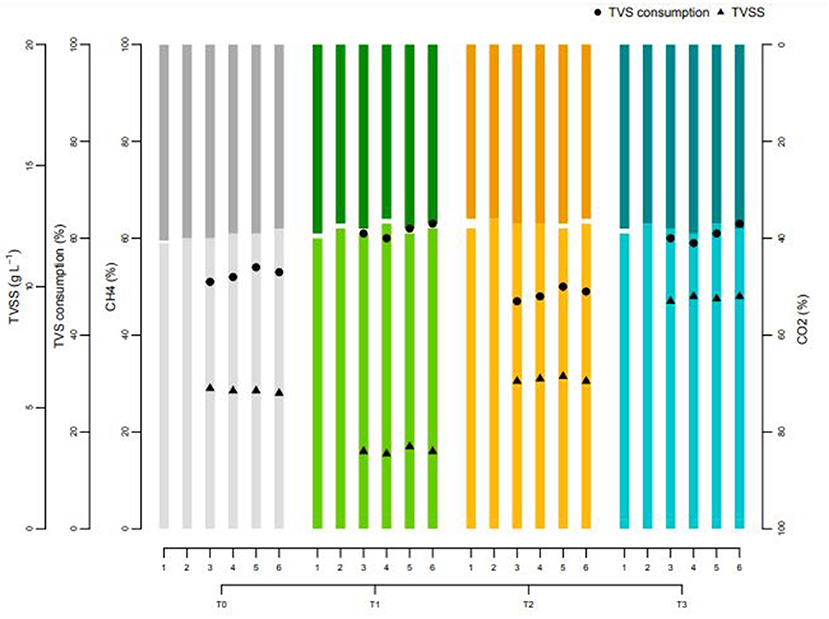
Figure 6. Concentration of CH4 and CO2, evolution of organic matter consumption and suspended solids during the different trials.
The specific energy-loading rate profile along AD vs. AcoD experiments is a good indicator for comparison the system stability. According to Evans et al. (2012), the limit value for SELR as an indicator of the process stability is 0.4 d−1. This metric based on the energy-loading rate per unit of methanogenic biomass, indirectly serves to evaluate the methanogenic activity. If this capacity is higher than the recommended value, the digester might become unstable, due to the rate of acidogenesis outpacing the rate of methanogenesis. Values higher than 0.4 d−1 indicate instability among the microbial consortia biomass and feeding mixture loading. The analysis of results shown in Table 3, the SELR indicator maintain the values 0.20 d−1 during T0 (using PS as mono-substrate), whereas achieved 0.32 d−1 during AcoD phase of PS with 30% (v/v) CECL. This behavior point out the buffer capacity and stability in the bioreactor, proved by OLR enhance, two and half times higher in comparison with AD scenario.
Co-digestion of CECL improved continuous stirred tank reactor performance and enabled it to operate at higher OLR. Co-digestion regime of PS with CECL ensured improved nutrient balance and process stabilization, thus increasing biomethane yield (Hagos et al., 2017; Shrestha et al., 2017).
Characterization of Statistical Analysis
Results from ANOVA suggested that there are significant differences (p < 0.05) between the trials concerning the GPR and SMP produced. Moreover, one applies Tukey's method to determine confidence intervals for all pairwise. If 0 does not belong to an interval, then we have evidence of different operational parameter value means, at the specified family-wise significance level.
In Figure 7, that shows Tukey's “Honest Significant Difference” confidence intervals for the mean pairwise differences concerning the GPR (on the left) and the SMP (on the right) produced, we can visualize that the 95% confidence intervals corresponding to the pairs T3–T0, T3–T1, T3–T2, and T2–T0 do not contain the zero. Consequently, there is evidence that the trials of these pairs produced different GPR and SMP means which reinforces the role of CECW as a co-substrate to enhance AcoD.
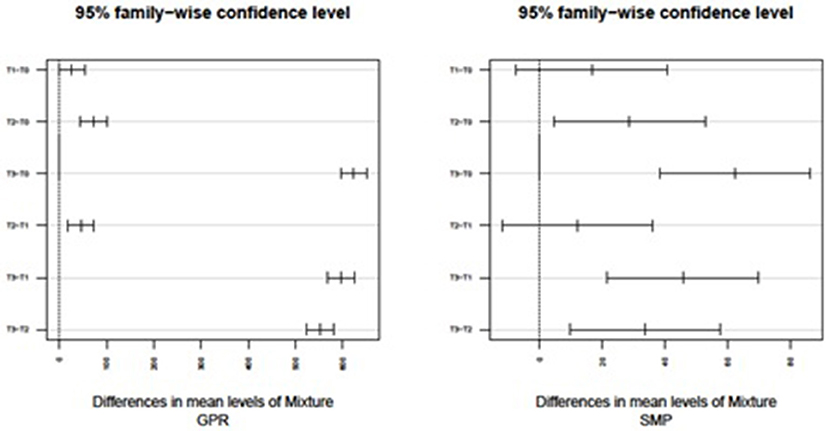
Figure 7. Tukey's “Honest Significant Difference” confidence intervals for the mean pairwise differences concerning the GPR (on the left) and the SMP (on the right) produced.
Conclusions
In this research work, the impact of the incorporation of CECL on AD performance of PS is addressed. Pig slurry AD process faces two main challenges: biodegradability improvement and methane yield enhancement. However, finding suitable co-substrates and optimum operating conditions are among the major challenges in biogas plants. The type and structure of substrates together with their biodegradability are the key factors for methane production. The goal was to face the challenge of sustainability improvement in terms energy intensification in the framework of circular economy for both pig facilities and cereal and exhausted coffee industry. Upon the blends of CECL (30%) as co-substrate with the PS, 2.5-fold increase of the OLR as well as 2.8-fold of C/N ratio was provided to the system. Results obtained confirm not only significant improvement in terms of biomethane production yield, but also enhanced system stability upon co-digestion of PS with cereal exhausted coffee liquor, if compared to mono-digestion of PS. The shift from mono-digestion regime into co-digestion regime (AcoD), by the addition of 30% (v/v) of CECL, led to an increase of 1.2 times in TVS biodegradation upon the same HRT = 16 days. Furthermore, the average daily GPR and, SMP of the system successfully increased by 3.7 and 1.6 times, ensured during three consecutive HRTs.
Overall, co-AD could represent a technically and economically viable solution for the CECW treatment. Additional revenues related to the possibility to convert already existing digesters that treat animal waste or, generally, nitrogen-rich waste could help the widespread of AD of CECW. This study can contribute to open a route for most commercial anaerobic configurations, which use several different substrates with various compositions to incorporate CECW for industrial scale biogas production.
Through the lens of Circular Bio-Economy (CBE), it is important to highlight the innate net positive energy of CECL that can be recovered and recycled, by which biogas technology can become an “Engine” for future bioenergy solutions.
Data Availability Statement
The raw data supporting the conclusions of this article will be made available by the authors, without undue reservation.
Author Contributions
SSo: contributed to the conceptualization, methodology, investigation, data curation, and writing the original draft. ED: contributed to the conceptualization, methodology, data curation, reviewing and editing, and supervision. MM: contributed to the conceptualization, reviewing and editing, and supervision. SSa: reviewing and editing. All authors contributed to the article and approved the submitted version.
Funding
This work was supported by the Linking Landscape, Environment, Agriculture and Food (LEAF) research unit (UID/AGR/04129/2020) and Center of Mathematics, Fundamental Applications and Operations Research (CMAF-CIO) research unit (UID/MAT/04561/2019).
Conflict of Interest
The authors declare that the research was conducted in the absence of any commercial or financial relationships that could be construed as a potential conflict of interest.
Acknowledgments
The authors would like to thank Nestlé Group, specifically, the collaborators from Avanca Factory, Portugal, for exhausted coffee wastes samples. The authors are thankful for Domingos Figueiredo's support during analytical procedures.
Abbreviations
AcoD, anaerobic co-digestion; AD, anaerobic digestion; CECW, cereal and exhausted coffee wastes; CECL, cereal and exhausted coffee liquor; CSTR, continuous stirred tank reactor; C/N, carbon nitrogen ratio; EC, electrical conductivity; HRT, hydraulic retention time; GPR, gas production rate; MPR, methane production rate; OLR, organic loading rate; PS, pig slurry; SCOD, soluble chemical oxygen demand; SELR, specific energy loading rate; SGP, specific gas production; SMP, specific methane production; TAN, total ammoniacal nitrogen; TCOD, total carbon oxygen carbon; TOC, total organic carbon; TKN, total Kjeldahl nitrogen; TS, total solids; TVS, total volatile solids.
References
Ahn, Y., Lee, W., Kang, S., and Kim, S. (2020). Enhancement of sewage sludge digestion by co-digestion with food waste and swine waste. Waste Biomass Valor. 11, 2421–2430. doi: 10.1007/s12649-018-00558-w
APHA—American Public Health Association (2017). Standard Methods for the Examination of Water and Wastewater, 23rd Edn. Washington, DC: American Public Health Association.
Azevedo, A., Gominho, J., and Duarte, E. (2021). Performance of anaerobic co-digestion of pig slurry with pineapple (ananas comosus) bio-waste residues. Waste Biomass Valor. 12, 303–311. doi: 10.1007/s12649-020-00959-w
Barros, M., Salvador, R., Carlos de Francisco, A., and Piekarski, C. (2020). Mapping of research lines on circular economy practices in agriculture: from waste to energy. Renew. Sustain. Energy Rev. 131:109958. doi: 10.1016/j.rser.2020.109958
Battista, F., Barampouti, E., Mai, S., Bolzonella, D., Malamis, D., Moustakas, K., et al. (2020). Added-value molecules recovery and biofuels production from spent coffee grounds. Renew. Sustain. Energy Rev. 131:110007. doi: 10.1016/j.rser.2020.110007
Cuetos, M. J., Fernández, C., Gómez, X., and Morán, A. (2011). Anaerobic co-digestion of swine manure with energy crop residues. Biotechnol. Bioprocess Eng. 16, 1044–1052. doi: 10.1007/s12257-011-0117-4
D'Amato, D., Droste, N., Winkler, K., and Toppinen, A. (2019). Thinking green, circular or bio: eliciting researchers' perspectives on a sustainable economy with Q method. J. Cleaner Product. 230, 460–476. doi: 10.1016/j.jclepro.2019.05.099
Dima, A., Pârvulescu, O., Mateescu, C., and Dobre, T. (2020). Optimization of substrate composition in anaerobic co-digestion of agricultural waste using central composite design. Biomass Bioenergy 138:105602. doi: 10.1016/j.biombioe.2020.105602
Duarte, E., Fragoso, R., Smozinski, N., and Tavares, J. (2021). Enhancing bioenergy recovery from agro-food biowastes as a strategy to promote circular economy. J. Sustain. Dev. Energy Water Environ. Syst. 9:1080320. doi: 10.13044/j.sdewes.d8.0320
Evans, P., Amador, J., Nelsen, D., Parry, D., and Stensel, H. (2012). Factors controlling stable anaerobic digestion of food waste and FOG. Proc. Water Environ. Feder. 14, 2630–2647. doi: 10.2175/193864712811726004
Fischer, M., Güllert, S., Refai, S., Künzel, S., Deppenmeier, U., Streit, W., et al. (2019). Long-term investigation of microbial community composition and transcription patterns in a biogas plant undergoing ammonia crisis. Microb. Biotechnol. 12, 305–323. doi: 10.1111/1751-7915.13313
Girotto, F., Lavagnolo, M., Pivato, A., and Cossu, R. (2017). Acidogenic fermentation of the organic fraction of municipal solid waste and cheese whey for bio-plastic precursors recovery – effects of process conditions during batch tests. Waste Manage. 70, 71–80. doi: 10.1016/j.wasman.2017.09.015
Hagos, K., Zong, J., Li, D., Liu, C., and Lu, X. (2017). Anaerobic co-digestion process for biogas production: progress, challenges and perspectives. Renew. Sustain. Energy Rev. 76, 1485–1496. doi: 10.1016/j.rser.2016.11.184
ICO—International Coffee Organization (2020). World Coffee Consumption. Available online at: http://www.ico.org/prices/new-consumption-table.pdf (accessed October 26, 2020).
Ingrao, C., Faccilongo, N., Di Gioia, L., and Messineo, A. (2018). Food waste recovery into energy in a circular economy perspective: a comprehensive review of aspects related to plant operation and environmental assessment. J. Clean. Prod. 184, 869–892. doi: 10.1016/j.jclepro.2018.02.267
Kapoor, R., Ghosh, P., Kumar, M., Sengupta, S., Gupta, A., Kumar, S., et al. (2020). Valorization of agricultural waste for biogas based circular economy in India: a research outlook. Bioresour. Technol. 304:123036. doi: 10.1016/j.biortech.2020.123036
Kaza, S., Yao, L., Bhada-Tata, P., and Woerden, F. (2018). What a Waste 2.0: A Global Snapshot of Solid Waste Management to 2050. Urban Development Series. Washington, DC: World Bank. doi: 10.1596/978-1-4648-1329-0
Kim, J., Kim, H., Baek, G., and Lee, C. (2017b). Anaerobic co-digestion of spent coffee grounds with different waste feedstocks for biogas production. Waste Manage. 60, 322–328. doi: 10.1016/j.wasman.2016.10.015
Kim, J., Kim, H., and Lee, C. (2017a). Ulva biomass as a co-substrate for stable anaerobic digestion of spent coffee grounds in continuous mode. Bioresour. Technol. 241, 1182–1190. doi: 10.1016/j.biortech.2017.06.012
Li, Q., Li, H., Wang, G., and Wang, X. (2017). Effects of loading rate and temperature on anaerobic co-digestion of food waste and waste activated sludge in a high frequency feeding system, looking in particular at stability and efficiency. Bioresour. Technol. 237, 231–239. doi: 10.1016/j.biortech.2017.02.045
Li, Y., and Chen, Y, Wu, J. (2019). Enhancement of methane production in anaerobic digestion process: a review. Appl. Energy 240, 120–137. doi: 10.1016/j.apenergy.2019.01.243
Lopes, M., Baptista, P., Duarte, E., and Moreira, A. (2019). Enhanced biogas production from anaerobic co-digestion of pig slurry and horse manure with mechanical pre-treatment. Environ. Technol. 40, 1289–1297. doi: 10.1080/09593330.2017.1420698
Luz, F., Cordiner, S., Manni, A., Mulone, V., and Rocco, V. (2017). Anaerobic digestion of coffee grounds soluble fraction at laboratory scale: evaluation of the biomethane potencial. Appl. Energy 207, 166–175. doi: 10.1016/j.apenergy.2017.06.042
Ma, Y., and Liu, Y. (2019). Turning food waste to energy and resources towards a great environmental and economic sustainability: an innovative integrated biological approach. Biotechnol. Adv. 37:107414. doi: 10.1016/j.biotechadv.2019.06.013
Martínez-Ruano, J., Restrepo-Serna, D., Carmona-Garcia, E., Giraldo, J., Aroca, G., and Cardona, C. (2019). Effect of co-digestion of milk-whey and potato stem on heat and power generation using biogas as an energy vector: techno-economic assessment. Appl. Energy 241, 504–518. doi: 10.1016/j.apenergy.2019.03.005
Oladejo, O., Dahunsi, S., Adesulu-Dahunsi, A., Ojo, S., Lawal, A., Idowu, E., et al. (2020). Energy generation from anaerobic co-digestion of food waste, cow dung and piggery dung. Bioresour. Technol. 313:123694. doi: 10.1016/j.biortech.2020.123694
Pinto, N., Carvalho, A., Pacheco, J., and Duarte, E. (2016). Study of different ratios of primary and waste activated sludge to enhance the methane yield. Water Environ. J. 30, 1747–6585. doi: 10.1111/wej.12188
Rivera, X., Gallego-Schmid, A., Najdanovic-Visak, V., and Azapagic, A. (2020). Life cycle environmental sustainability of valorisation routes for spent coffee grounds: from waste to resources. Resour. Conserv. Recycl. 157:104751. doi: 10.1016/j.resconrec.2020.104751
Rodrìguez-Abalde, Á., Flotats, X., and Fernández, B. (2017). Optimization of the anaerobic co-digestion of pasteurized slaughterhouse waste, pig slurry and glycerine. Waste Manage. 61, 521–528. doi: 10.1016/j.wasman.2016.12.022
Ros, M., Filho, J., Murcia, M., Bustamante, M., Moral, R., Coll, M., et al. (2017). Mesophilic anaerobic digestion of pig slurry and fruit and vegetable waste: dissection of the microbial community structure. J. Clean. Prod. 156, 757–765. doi: 10.1016/j.clepro.2017.04.110
Sawada, K., and Toyota, K. (2015). Effects of the application of digestates from wet and dry anaerobic fermentation to Japanese paddy and upland soils on short-term nitrification. Microbes Environ. 30, 37–43. doi: 10.1264/jsme2.ME14080
Sembera, C., Macintosh, C., Astals, S., and Koch, K. (2019). Benefits and drawbacks of food and dairy waste co-digestion at a high organic loading rate: a Moosburg WWTP case study. Waste Manage. 95, 217–226. doi: 10.1016/j.wasman.2019.06.008
Shrestha, S., Fonoll, X., Khanal, S., and Raskin, L. (2017). Biological strategies for enhanced hydrolysis of lignocellulosic biomass during anaerobic digestion: current status and future perspectives. Bioresour. Technol. 245, 1245–1257. doi: 10.1016/j.biortech.2017.08.089
Siddique, M. D., and Wahid, Z. (2018). Achievements and perspectives of anaerobic co-digestion: a review. J. Clean. Prod. 194, 359–371. doi: 10.1016/j.jclepro.2018.05.155
Silva, I., Jorge, C., Brito, L., and Duarte, E. (2020). A pig slurry feast/famine feeding regime strategy to improve mesophilic anaerobic digestion efficiency and digestate hygienisation. Waste Manage. Res. doi: 10.1177/0734242X20972794
Slorach, P., Jeswani, H., Cuéllar-Franca, R., and Azapagic, A. (2019). Environmental sustainability of anaerobic digestion of household food waste. J. Environ. Manage. 236, 798–814. doi: 10.1016/j.jenvman.2019.02.001
Ubando, A., Felix, C., and Chen, W. (2020). Biorefineries in circular economy: a comprehensive review. Bioresour. Technol. 299:122585. doi: 10.1016/j.biortech.2019.122585
Xie, S., Wickham, R., and Nghiem, L. (2017). Synergistic effect from anaerobic co-digestion of sewage sludge and organic wastes. Int. Biodeterior. Biodegrad. 116, 191–197. doi: 10.1016/j.ibiod.2016.10.037
Yang, J., Wang, D., Luo, Z., and Zeng, W. (2019). Anaerobic mono-digestion of pig manure in a leach bed coupled with a methanogenic reactor: effects of the filter media. J. Clean. Prod. 234, 1094–1011. doi: 10.1016/j.jclepro.2019.0654
Yenigün, O., and Demirel, B. (2013). Ammonia inhibition in anaerobic digestion: a review. Process Biochem. 48, 901–911. doi: 10.1016/j.procbio.2013.04.012
Keywords: anaerobic co-digestion, cereal and exhausted coffee wastes, pig slurry, biogas production, waste management, biowastes circularity
Citation: Sousa S, Duarte E, Mesquita M and Saraiva S (2021) Energetic Valorization of Cereal and Exhausted Coffee Wastes Through Anaerobic Co-digestion With Pig Slurry. Front. Sustain. Food Syst. 5:642244. doi: 10.3389/fsufs.2021.642244
Received: 15 December 2020; Accepted: 05 March 2021;
Published: 31 March 2021.
Edited by:
Francisco Jesus Fernandez Morales, University of Castilla-La Mancha, SpainReviewed by:
Antonio Moran, Universidad de León, SpainSara Mateo, Autonomous University of Madrid, Spain
Copyright © 2021 Sousa, Duarte, Mesquita and Saraiva. This is an open-access article distributed under the terms of the Creative Commons Attribution License (CC BY). The use, distribution or reproduction in other forums is permitted, provided the original author(s) and the copyright owner(s) are credited and that the original publication in this journal is cited, in accordance with accepted academic practice. No use, distribution or reproduction is permitted which does not comply with these terms.
*Correspondence: Elizabeth Duarte, ZWR1YXJ0ZUBpc2EudWxpc2JvYS5wdA==