- 1Department of Plant Pathology, The Ohio State University, Wooster, OH, United States
- 2United States Department of Agriculture-Agricultural Research Service Application Technology Research Unit, Wooster, OH, United States
- 3Department of Horticulture and Crop Science, The Ohio State University, Columbus, OH, United States
Anaerobic soil disinfestation (ASD) is a viable option for disease management in tomato production and reduces damage due to a soilborne disease complex consisting of Pyrenochaeta lycopersici, Colletotrichum coccodes, Verticillium dahliae, and Meloidogyne spp. There are plentiful options for ASD carbon sources using byproducts of Midwestern United States agriculture or cover crops, yet these carbon sources have not been evaluated for use in Midwestern settings. Low (10.1 Mg/ha) and high (20.2 Mg/ha) rates of corn gluten meal, distillers dried grains, soybean meal, wheat bran, and dry sweet whey were evaluated as ASD carbon sources in growth chamber and greenhouse bioassays. Cover crops including buckwheat, cowpea, crimson clover, mustard, oilseed radish, sorghum-sudangrass, white clover, and winter rye were evaluated in similar bioassays with one amendment rate (20.2 Mg/ha). Reducing conditions developed in soils regardless of carbon source or rate. Use of high rates of corn gluten meal, distillers dried grains, soybean meal, and wheat bran led to the lowest levels of root rot severity compared to non-treated controls. The higher rate of any byproduct carbon source was always more effective than the lower rate in reducing root rot severity. Use of both rates of soybean meal or corn gluten meal and the high rate of distillers dried grains or dry sweet whey led to significant increases in dry root and shoot biomass compared to controls. For cover crops, ASD with crimson clover, sorghum-sudangrass, white clover, or winter rye amendments reduced root rot severity relative to the aerobic control, but not relative to the anaerobic control. Use of cover crops did not significantly impact plant biomass. A subset of three ASD carbon sources [distillers dried grains, soybean meal, and wheat middlings (midds), all 20.2 Mg/ha] were evaluated in five on-farm ASD trials in high tunnels. Soil temperatures were low during the application period, limiting treatment efficacy. Reducing conditions developed in all soils during ASD treatment, and a moderate but significant reduction in root rot severity was observed following ASD with the soybean meal or wheat midds compared to ASD with distillers dried grains. Tomato yield was not significantly affected by ASD treatment.
Introduction
Protected culture tomato production in Ohio is constrained by a soilborne disease complex consisting of corky root rot (Pyrenochaeta lycopersici), black dot root rot (Colletotrichum coccodes), Verticillium wilt (Verticillium dahliae), and root-knot nematodes (Meloidogyne hapla and Meloidogyne incognita) (Vrisman et al., 2017; Testen and Miller, 2018; Testen et al., 2020). Based on a state-wide survey, members of this complex are prevalent in Ohio high tunnels with P. lycopersici present on 50% of farms, C. coccodes present on 97% of farms, V. dahliae present on 75% of farms and root-knot nematodes present on 56% of farms (Testen et al., 2020). Fusarium, Rhizoctonia, and Pythium spp. likely also damage tomatoes but occur less frequently than the four core pathogens. The recommended approach for managing this soilborne disease complex relies on anaerobic soil disinfestation (ASD), usually applied in autumn, and grafting susceptible cultivars onto disease-resistant rootstocks (Testen and Miller, 2018; Testen et al., 2020).
Anaerobic soil disinfestation is a soilborne disease management strategy mediated by native soil microbial populations (Blok et al., 2000; Momma et al., 2013). The efficacy of ASD against the soilborne disease complex depends on carbon source (Testen and Miller, 2018), as soilborne fungi vary in their sensitivity to ASD with wheat bran, molasses, or ethanol. Root-knot nematodes are highly sensitive to ASD regardless of carbon sources examined to date (Katase et al., 2009; Butler et al., 2012b; Testen and Miller, 2018, 2019). While the efficacy of wheat bran and molasses as ASD carbon sources has been demonstrated in Midwestern production systems (Testen and Miller, 2018, 2019; Testen et al., 2020), there are more potential ASD carbon sources, including cover crops, yet to be examined for efficacy against the tomato soilborne disease complex.
Carbon sources for ASD should be readily available, inexpensive, and easily broken down by soil microbial populations. Agricultural byproducts, usually those sold as animal feed, meet these criteria. The availability and cost of these byproducts varies by region within the United States, depending on local agricultural industries. Agricultural byproducts commonly studied as ASD carbon sources include brans (Yossen et al., 2008; Momma et al., 2010; Shennan et al., 2018; Testen and Miller, 2019), seed meals (Shennan et al., 2018), molasses and molasses products (Butler et al., 2012a; McCarty et al., 2014), ethanol (Momma et al., 2010; Hewavitharana et al., 2014; Testen and Miller, 2018), crop residues (Blok et al., 2000; Messiha et al., 2007), pomaces (Domínguez et al., 2014; Achmon et al., 2016; Serrano-Pérez et al., 2017), poultry litter (Butler et al., 2012a), manures (Núñez-Zofío et al., 2011; López-Robles et al., 2013; Hewavitharana et al., 2014; Khadka et al., 2020), and high protein, fermented products (Ludeking et al., 2011; van Overbeek et al., 2014). Cover crops have potential as ASD carbon sources because they can be produced in situ and can be used to supplement agricultural byproducts as ASD amendments. Various grass, Brassicaceous, and legume cover crops have been used in ASD studies (Blok et al., 2000; Goud et al., 2004; Lamers et al., 2010; Núñez-Zofío et al., 2011; Butler et al., 2012b; Hewavitharana et al., 2014; Korthals et al., 2014; McCarty et al., 2014; Vecchia et al., 2020). As soils, soilborne pathogens, soil microbial communities, and cropping systems differ across regions, it is essential to test the efficacy of various carbon sources when ASD is introduced to a new region (Strauss and Kluepfel, 2015).
The objective of this study was to determine which alternative ASD carbon sources, including cover crops, could effectively reduce damage from the tomato soilborne disease complex, in order to provide Midwestern growers with a range of amendment options. Carbon sources were selected so that they would be appropriate for use by Midwestern growers, meaning they should be readily available at feed mills and relatively inexpensive, or can be produced on-farm. These carbon sources, including cover crops, were assessed in growth chamber and greenhouse bioassays and a subset of these carbon sources were assessed in on-farm trials.
Materials and Methods
Agricultural Byproduct Carbon Source Screening
Corn gluten meal (CG), distillers dried grain (DG), soybean meal (SM), wheat bran (WB), and dry sweet whey (WY) were tested for efficacy as ASD carbon sources at high (H: 20.2 Mg/ha) and low (L: 10.1 Mg/ha) rates. Carbon sources were obtained from The Ohio State University Feedstock Processing Research Facility in Wooster, OH. Wheat bran was included as a standard carbon source known to be effective in ASD against the tomato soilborne disease complex (Testen and Miller, 2018). Soils from tomato high tunnels in three Ohio counties (Wayne, Erie, and Highland) with a known history of soilborne diseases were used in these experiments. Experiments were laid in a randomized complete block design (RCBD) with five replications. One replication consisted of one cup containing one tomato plant. Each experiment was conducted twice.
Soils were placed in 266 mL plastic cups (Hefty, Reynolds Consumer Products, USA), amended with a carbon source, flooded to saturation with sterile distilled water, covered with black polyethylene mulch (1.5 mm super strength embossed mulch, blend of LDPE and LLDPE, PolyExpert, Quebec, Canada), and sealed with rubber bands and electrical tape. Two controls were used in these experiments, a non-amended, flooded, covered control (anaerobic control) and a non-amended, flooded, uncovered control (aerobic control). An iron oxide-painted, 7.62 by 0.635 cm diameter PVC IRIS (Indicator of Reduction in Soils) rod (Rabenhorst and Burch, 2006; Rabenhorst, 2008) (Professional Plastics, Fullerton, CA) was inserted fully into the saturated soil in each cup prior to sealing. Cups were placed in a growth chamber at 25°C in the dark.
After 4 weeks, cups were removed from the growth chamber, plastic mulch removed, IRIS rods were removed, five holes were punched into the bottom of the cups using a nail, and cups were returned to the growth chamber for 6 days to dry. Iron oxide paint loss was visually assessed after rinsing the IRIS rods in tap water. After drying, soils were placed in a plastic bag and homogenized with a rubber mallet. Homogenized soils were returned to cups and one 2-week-old tomato “Moneymaker” seedling (seed source: Everwilde Farms, Bloomer, WI) was placed in each pot exactly 1 week after the end of ASD treatments. Plants were fertilized once weekly with a 20–20–20 N–P–K fertilizer solution. Tomatoes were grown in the greenhouse in the same RCBD arrangement for 9 weeks, at which time plants were harvested and roots were washed in tap water.
Roots were evaluated for both root rot severity (percent of roots rotted or discolored) and taproot rot severity using a 1 to 5 scale [1: no taproot rot, 2: 1 to 2 small lesions on the taproot, 3: multiple lesions covering <50% of the taproot, 4: multiple lesions covering more than 50% of the taproot, 5: taproot completely rotten or missing (Testen and Miller, 2018)]. Following root rating, a random subsample of roots was taken from each plant and surface disinfested in 0.6% sodium hypochlorite for 15 s, followed by a sterile water rinse. Five, 2–3 mm long root pieces from each random subsample were plated onto each of three plates of half strength potato dextrose agar (½APDA, IBI Scientific, Dubuque, IA) for a total of 15 root pieces plated per plant. After 2 weeks, fungi growing on the medium were identified morphologically. Following plating, all aboveground tissue (harvested at the soil line) and roots were dried in a 65°C oven for 48 h to obtain dry shoot and root biomass.
Cover Crops Carbon Source Trial
Eight cover crops were assessed for efficacy as ASD carbon sources: two grasses (Sorghum-sudangrass Sorghum × drummondii “Piper” and winter rye Secale cereale), three legumes (cowpea Vigna unguiculata “Iron and Clay,” crimson clover Trifolium incarnatum, and white clover Trifolium repens), two Brassicas (mustard Brassica juncea “Mighty Mustard Pacific Gold” and oilseed radish Raphanus sativus), and buckwheat (Fagopyrum esculentum). All seeds were obtained from Johnny's Selected Seeds (Fairfield, ME). Cover crops were direct seeded (5–7 seeds per pot) in a topsoil blend in Deepots (D40H, Steuwe and Sons, Tangent, Oregon) and were fertilized weekly as described above. After seven weeks, the aboveground portion of the cover crop was harvested and cut by hand into 0.25–0.75 cm pieces. Portions of the taproot were included in the radish cut pieces. Cover crops were mixed at a rate of 20.2 Mg/ha fresh biomass with soil obtained from a high tunnel in Highland County, OH and placed into 266 mL cups. Experiments were laid as a randomized complete block design with five replications. One replication consisted of one cup containing one tomato plant. Each experiment was conducted twice. Wheat middlings (midds) were included as a separate treatment, as a known effective ASD carbon source. Wheat midds are nutritionally similar to but less costly than wheat bran. Wheat bran is the outer seed covering, while midds are the wheat remnants following flour production. IRIS rods were placed into cups, soils were irrigated to saturation with sterile distilled water, and cups were sealed and placed into a growth chamber as described above. The ASD treatment, planting, root rot assessment, and root plating were conducted as described above for the agricultural byproduct carbon source screening. Only 10 root pieces per plant (two plates with five root pieces each) were plated for the cover crops experiments. Following plating, all aboveground tissue (harvested at the soil line) and roots were dried in a 65°C oven for 48 h to obtain shoot and root biomass.
On-Farm ASD Trials
Anaerobic soil disinfestation trials were established in high tunnels on five farms in Wayne (one trial), Holmes (one), Morrow (one), and Knox (two) Ohio counties and a randomized complete block design with four replications was established in each high tunnel. Plots were one m wide and ranged in length from 3 to 9.1 m, depending on the size of the high tunnel. Plots were amended with either wheat midds (ASD-WM), soybean meal (ASD-SM), or distillers dried grains (ASD-DG) at a rate of 20.2 Mg/ha. Carbon sources were obtained from Gerber Feed Services (Dalton, OH, USA). Carbon sources were spread over the treated area and incorporated to a depth of 10–15 cm using a walk behind rototiller. Beds were formed by hand and two lines of drip tape were laid on top of each bed. Three, 30-cm-long IRIS tubes (1.27 cm diameter PVC pipes) were placed in the center of each plot. A HOBO temperature pendant data logger (Onset Computer Corporation, Bourne, MA, USA) was placed in one plot of each treatment per trial. Black plastic mulch (1.5 mm embossed, 1.2 m wide, PolyExpert Inc., Laval, QC, Canada) was laid over each bed, and the sides of the mulch were covered with soil to prevent air exchange. Non-amended, covered plots served as anaerobic controls. Drip irrigation was applied to all plots until soils were saturated to a depth of 20 cm. Plots remained covered for 6 weeks. Trials were initiated in October 2018. Iron oxide paint loss was assessed on IRIS tubes using the visual grid method (Rabenhorst, 2012).
Soils were collected from each on-farm trial immediately following the end of ASD to determine the impacts of the treatments on root rot severity in a post-ASD bioassay. Post-ASD bioassays were laid in a randomized complete block design with four subsamples per sampled plot. One subsample consisted of one cup containing one tomato plant. Soils were placed in Deepots (D16H) and tomato “Moneymaker” seeds were directly sown into the pots and thinned to one plant per pot. Plants were grown for 9 weeks in the greenhouse (12 h day/night cycle, temperature range: 24–30°C) and then assessed for root rot severity, taproot rot severity, root-knot nematode galling (number of galls per root system) as described above, and dry root and shoot biomasses. Following root rating, a random subsample of roots was surface disinfested and plated onto ½APDA as described above. Ten root pieces were plated per plant (two plates of five root pieces each), and plates were observed 2 weeks later to identify fungi morphologically.
Tomatoes were planted in on-farm trials in March to April 2019. Farmers grew their preferred tomato varieties (Knox 1 and Knox 2: “Mountain Fresh,” Morrow: “Red Deuce,” Holmes: “Bigdena,” Wayne: none: high tunnel structural failure), and trials were managed according to farmers' normal production practices. Participating farmers recorded yield data from three plants in the center of each plot during the growing season (Wayne and Knox 1 trials excepted). In August 2019, roots were collected from these three plants and assessed for root rot severity (Wayne and Knox 2 trials excepted).
Statistics
Bioassay and field trial data were combined for analysis to assess overall impacts of carbon sources across environments and soil or location (environments) were used as random blocking factors. Data were analyzed using mixed effects general linear models in Minitab 17 (Minitab Inc. State College, PA) with treatment as a fixed factor and soil (or location), experimental run, and block as random factors. Pairwise comparisons were made using Tukey's HSD with a 5% familywise error rate. Percentages were subjected to the arcsine transformation prior to data analysis. A proportional ordinal logistic regression model using the LOGISTIC procedure of SAS statistical software (version 9.4, SAS Institute Inc., Cary, NC (Allison, 1999; Derr, 2013) (Allison, 1999; Derr, 2013) was used to calculate odds ratios (OR) for taproot rot ratings from post-ASD bioassays. Odds ratios of <1 indicated that a treatment was associated with lower taproot rot ratings compared to a control, while odds ratios of >1 indicate that a treatment was associated with higher taproot rot ratings compared to a control.
Results
Impacts of Alternative ASD Carbon Sources and Rates on Root Rot Severity in Tomato
Reducing conditions developed in all ASD-treated soils, regardless of carbon source and rate, and the amount of iron oxide paint loss from IRIS tubes was significantly more (Figure 1A, p < 0.0001) for all amended ASD-treated soils than for the aerobic and anaerobic controls. Significantly more iron oxide paint was lost from IRIS tubes in ASD-treated soils amended with DG-H than in soils amended with WB-L or either dry sweet whey amendment rate. The correlation between percentage of iron oxide paint loss and root rot severity was −0.61 (p < 0.0001).
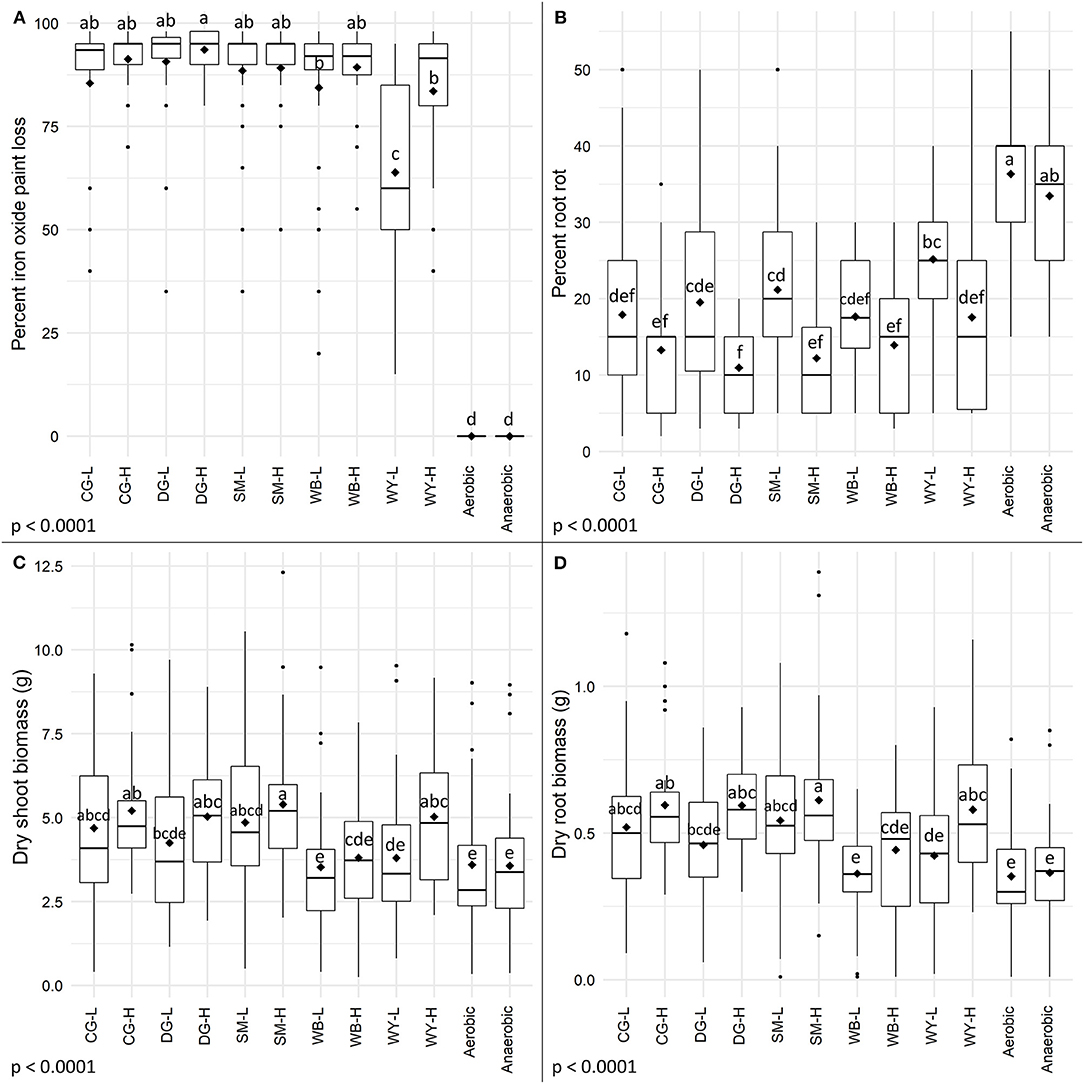
Figure 1. Effects of anaerobic soil disinfestation (ASD) carbon sources and amendment rates on (A) soil reducing conditions as indicated by iron oxide paint loss on IRIS rods, (B) root rot severity, (C) dry shoot biomass, and (D) dry root biomass. Corn gluten meal (CG), distillers dried grains (DG), soybean meal (SM), wheat bran (WB), and dry sweet whey (WY) were evaluated as ASD carbon sources in bioassays at low (L, 10.1 Mg/ha) and high (H, 20.2 Mg/ha) amendment rates and performance was compared to aerobic and anaerobic controls. Treatment means are indicated by diamonds and means that do not share the same letters differ significantly based on Tukey's HSD with a 95% family-wise confidence level.
Anaerobic soil disinfestation with either rate of any carbon source significantly reduced tomato root rot severity compared to the aerobic control (Figure 1B, p < 0.0001), and with the exception of WY-L, compared to the anaerobic control. Root rot severity was significantly lower for tomato plants grown in ASD-treated soil amended with the high rate of DG, SM, and WY than the low rate of each corresponding amendment, but there was no significant effect of rate when CG and WB were used as carbon sources. Both rates of all carbon sources significantly lowered the odds of having a higher taproot rot rating relative to the aerobic and anaerobic controls (Figure 2A, p < 0.0001). The anaerobic and aerobic controls did not differ significantly for proportional odds ratios associated with taproot rot severity. The incidence of C. coccodes recovered from roots was not significantly affected by ASD soil treatment (Table 1, p = 0.17). Fusarium spp. recovery was significantly lower from roots of plants grown in ASD-treated soils regardless of carbon source or rate, with the exception of WY-L, compared to the aerobic control. Only the ASD treatment with CG-H significantly reduced Fusarium spp. recovery compared to the anaerobic control. Significantly less P. lycopersici root colonization (Table 1, p = 0.007) was observed for plants grown in ASD-treated soils amended with DG-H and CG-H compared to the aerobic control.
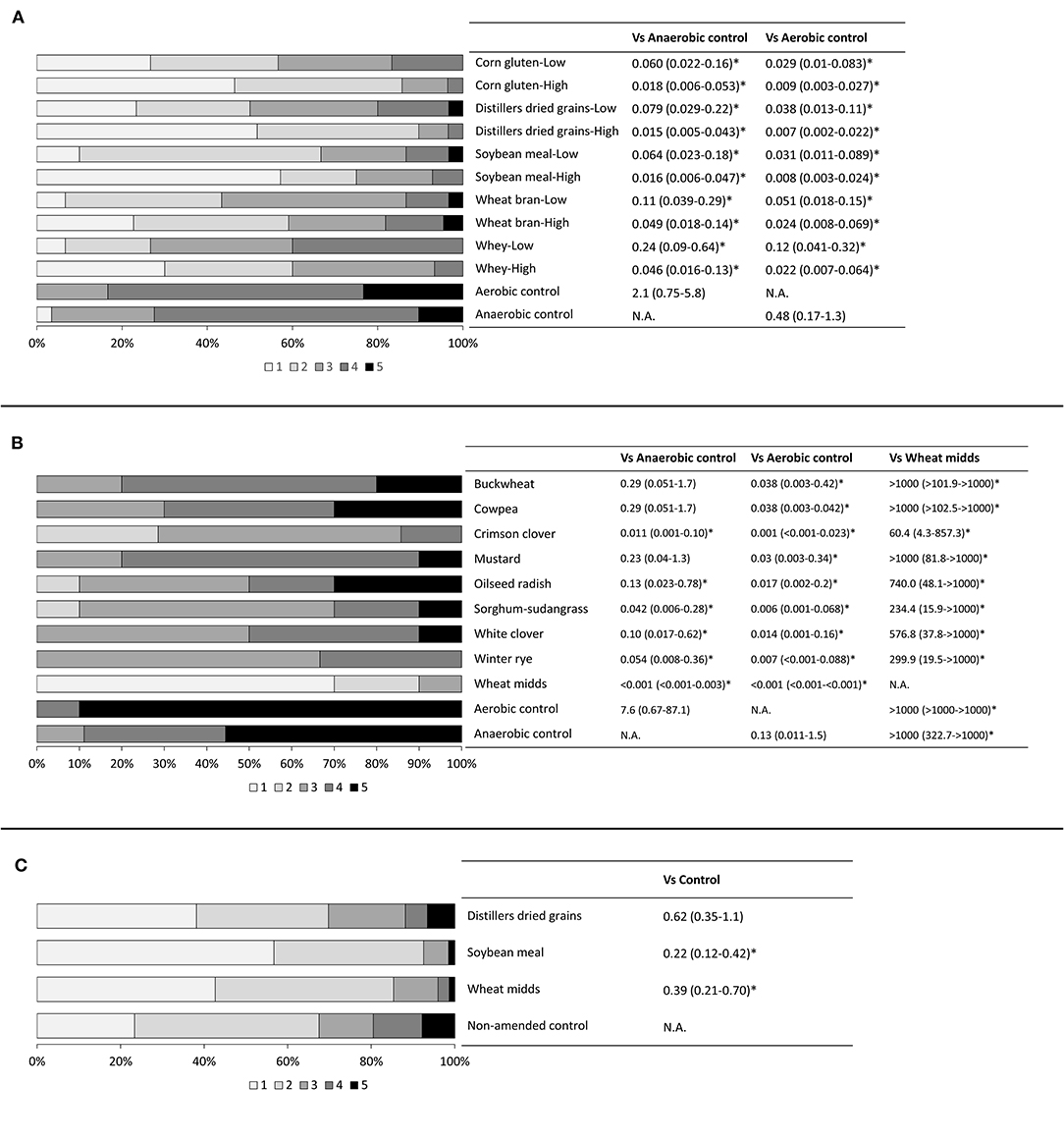
Figure 2. Taproot rot severity of bioassay plants grown in soils subjected to anaerobic soil disinfestation (ASD) using (A) various agricultural byproduct carbon sources, (B) cover crop carbon sources, or (C) a subset of ASD carbon sources evaluated in on-farm trials. Roots were rated on a 1–5 scale with 1: taproot healthy, 2: one to two small lesions or slight discoloration on taproot, 3: multiple lesions covering <50% of the taproot, 4: multiple lesions covering more than 50% of the taproot, 5: taproot completely rotten or missing. Proportional odds ratios are shown, along with confidence intervals, with a star indicating significance at P < 0.05. Odds ratios of <1 indicate that an ASD treatment was associated with lower taproot rot ratings compared to a control, while odds ratios of >1 indicate that an ASD treatment was associated with higher taproot rot ratings compared to a control.
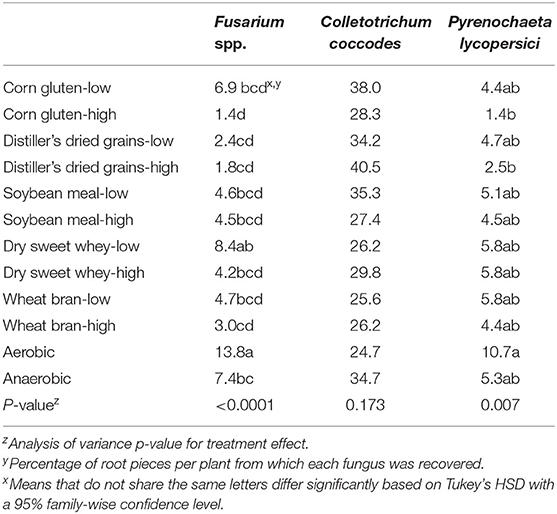
Table 1. Average incidence of root piece infection by various fungi for plants grown in soils treated with anaerobic soil disinfestation with various agricultural byproduct carbon sources at low (10.1 Mg/ha) or high (20.2 Mg/ha) amendment rates.
Root rot severity for plants grown in soils subjected to ASD at low amendment rates trended higher than at high amendment rates, so data were subset by individual carbon sources to examine the impact of amendment rate. General linear models were run on subset data to assess rate effects for individual carbon sources. Root rot severity differed significantly by rate for each individual carbon source (p-value range: 0.001–0.045) indicating that use of a higher amendment rate consistently led to lower levels of root rot severity compared to use of a low amendment rate.
Anaerobic soil disinfestation with agricultural byproducts significantly affected both dry shoot (Figure 1C, p < 0.0001) and dry root biomass (Figure 1D, p < 0.0001). Shoot and root biomass of plants grown in soils amended with either wheat bran rate, DG-L or WY-L did not differ significantly from the shoot and root biomass of control plants. Significantly higher shoot and root biomass was observed in plants grown in soils amended with either rate of soybean or corn gluten meal, DG-H or WY-H and subjected to ASD compared to control plants.
Impacts of Cover Crops as ASD Carbon Sources on Root Rot Severity in Tomato
Reducing conditions developed in soils following ASD with cover crops, and the percentage of iron oxide paint removal with any cover crop amendment was significantly higher (Figure 3A, p < 0.0001) than in either control treatment. The highest amount of iron oxide paint removal was observed in wheat midds-amended soils (98%) and this was significantly higher than the amount of iron oxide paint removal in any cover crop-amended soil. Among the cover crop-amended, ASD-treated soils, the highest amounts of iron oxide paint removal were observed for soils amended with sorghum-sudangrass (63.5%), crimson clover (60%), white clover (45.5%), and winter rye (41.1%). The lowest levels of iron oxide paint removal were observed in soil amended with cowpea (38.5%), buckwheat (15.2%), oilseed radish (13.7%), and mustard (13.2%). The correlation between the percentage of iron oxide paint loss and root rot severity was −0.48 (p < 0.0001).
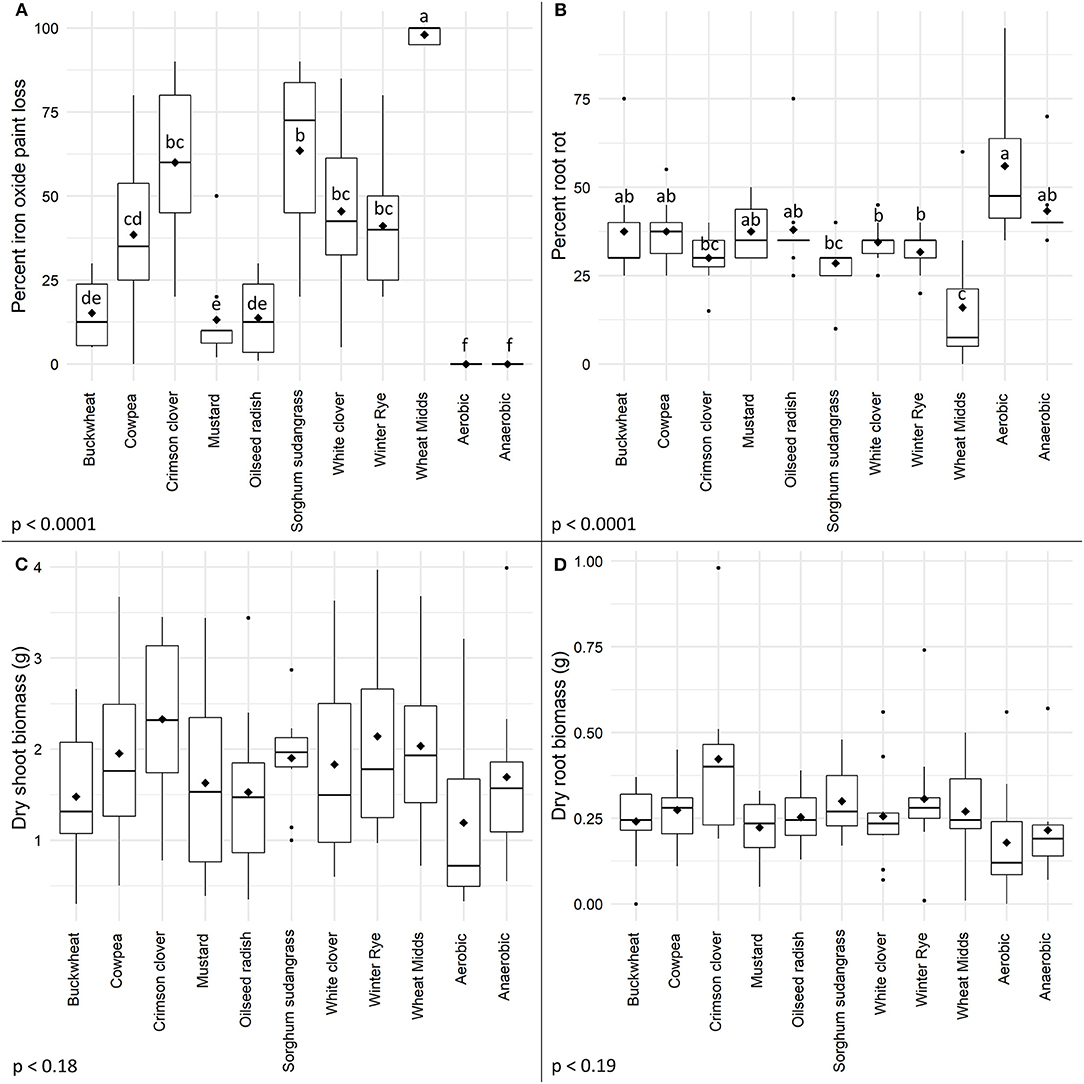
Figure 3. Effects of cover crops as anaerobic soil disinfestation carbon sources on (A) soil reducing conditions as indicated by iron oxide paint loss on IRIS rods, (B) root rot severity, (C) dry shoot biomass and (D) dry root biomass. Cover crop ASD treatment (20.2 Mg/ha) amendment rates were compared to aerobic and anaerobic controls and a standard ASD treatment with wheat midds amendment. Treatments means are indicated by diamonds and means that do not share the same letters differ significantly based on Tukey's HSD with a 95% family-wise confidence level.
Root rot severity was significantly impacted by ASD treatment (Figure 3B, p < 0.0001). A significant reduction in root rot severity was observed in plants grown in soils amended with wheat midds compared to plants grown in either control soil. No cover crop amendment significantly reduced root rot severity relative to the anaerobic control, but ASD with crimson clover, sorghum-sudangrass, white clover, or winter rye significantly reduced root rot severity relative to the aerobic control. All cover crops assessed led to significantly lower odds of increased taproot severity relative to the aerobic control (Figure 2B, p < 0.0001). Use of buckwheat, cowpea, and mustard did not significantly impact odds of higher taproot rot ratings relative to the anaerobic control, while amendment with all other cover crops significantly reduced odds relative to the anaerobic control. Plants grown in cover crop-amended ASD-treated soils or either control soil had significantly higher odds of increased taproot rot ratings relative to those grown in soils amended with wheat midds and subjected to ASD. No ASD treatment significantly reduced the recovery of P. lycopersici (Table 2, p = 0.063) or C. coccodes (p = 0.49) from roots. The incidence of Fusarium spp. recovery from roots was reduced significantly (Table 2, p = 0.003) for plants grown in wheat midds-amended soils compared to plants grown in aerobic control soils or soils amended with oilseed radish. Anaerobic soil disinfestation with cover crops as carbon sources did not significantly affect either dry shoot (Figure 3C, p = 0.18) or root (Figure 3D, p = 0.19) biomass.
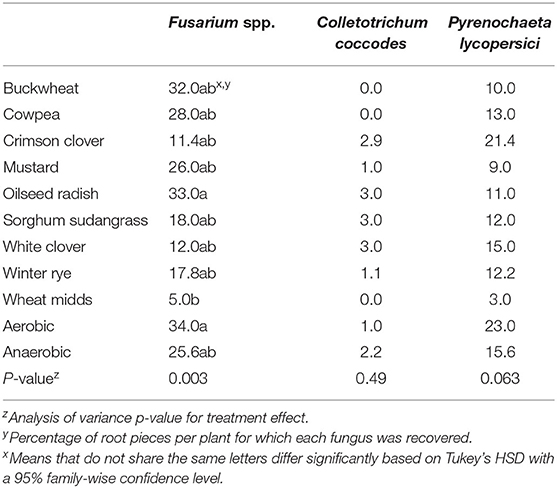
Table 2. Average incidence of root piece infection by various fungi for plants grown in soils treated with anaerobic soil disinfestation with various cover crop carbon sources and a wheat midds standard (20.2 Mg/ha).
Soil Temperatures and Soil Reducing Conditions in On-Farm ASD Trials
Average soil temperatures over the treatment period were low, ranging from 12.0 to 17.3°C (Table 3).

Table 3. Average soil temperatures (°C) during anaerobic soil disinfestation treatment in on-farm trials assessing distiller's dried grains, soybean meal, and wheat midds as carbon sources (20.2 Mg/ha).
Reducing conditions developed in all ASD-treated plots (Table 4, p < 0.0001). The highest levels of iron oxide paint removal were observed in plots amended with soybean meal (37.4%), followed by plots amended with distillers dried grains (32.7%) and wheat midds (28.7%). Iron oxide paint loss was significantly higher in plots amended with soybean meal than in plots amended with wheat midds. All ASD treatments led to significantly higher amounts of iron oxide paint loss compared to control soils.
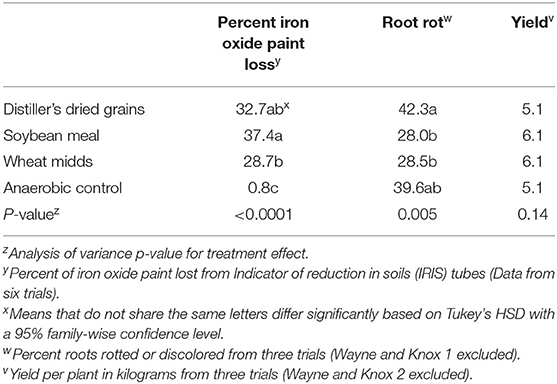
Table 4. Mean soil reducing conditions, root rot severity, and yield from on-farm trials conducted to assess three anaerobic soil disinfestation carbon sources (20.2 Mg/ha).
Impacts of ASD on Yield and Root Rot in On-Farm Trials
In post-ASD bioassays, root rot severity was significantly affected by treatment (Table 5, p = 0.002) as were dry shoot biomass (p < 0.0001) and dry root biomass (p = 0.008). Root rot severity in plants grown in soils collected from ASD-SM-treated plots (13.7%) was significantly lower than root rot severity in plants grown in soils collected from anaerobic control plots (18.4%). The odds of higher taproot rot ratings were significantly reduced (Figure 2C, p < 0.0001) for plants grown in ASD-treated soils amended with either wheat midds or soybean meal relative to plants grown in soils collected from anaerobic control plots (Figure 2C, p < 0.0001). Anaerobic soil disinfestation did not significantly reduce the incidence of Fusarium spp. (Table 6, p = 0.15) or C. coccodes (p = 0.68) recovered from plants grown in treated vs. control soils. The incidence of P. lycopersici recovered from plants grown in soils treated with ASD-WM (10.8% of root pieces, p = 0.004) was significantly lower than incidence in plants grown in soils collected from ASD-DG (19.9%) or anaerobic control (18.8%) plots. Dry shoot biomass was significantly higher in plants grown in ASD-treated soils with any amendment compared to plants grown in soils collected from control plots (Table 5). Dry root biomass was significantly higher for plants grown in soils collected from plots treated with either ASD-WM or ASD-DG compared to plants grown in soils collected from control plots. Root-knot nematodes were present in the Wayne trial post-ASD bioassays. Root-knot nematode galling was significantly reduced (p = 0.003) in plants grown in soils from any ASD treatment (ASD-SM: 0.9 galls per root system, ASD-DG: 1.7, ASD-WM: 1.9) compared to plants grown in soils collected from control plots (8.2 galls per root system).
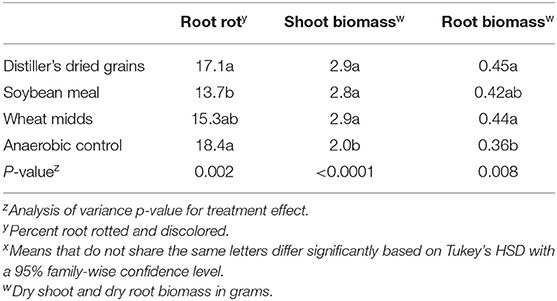
Table 5. Impacts of anaerobic soil disinfestation (ASD) on root rot severity, root biomass, and shoot biomass in post-ASD bioassays using soils collected from plots treated in on-farm trials.
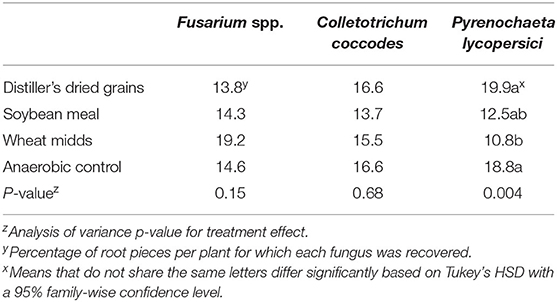
Table 6. Average incidence of root piece infection by various fungi for plants grown in post-ASD bioassays using soils treated on-farm with anaerobic soil disinfestation with various carbon sources (20.2 Mg/ha).
The Wayne trial was lost due to a high tunnel collapse in the winter of 2019, but post-ASD bioassays were completed for this trial. Due to extenuating circumstances, yield data were not obtained from the Knox 2 trial and root rot severity data were not obtained from the Knox 1 trial.
Anaerobic soil disinfestation did not significantly impact average yield per plant in on-farm trials (Table 4, p = 0.14). Root rot severity in high tunnel-grown plants was significantly impacted by ASD treatment in on-farm trials (p = 0.005). Root rot severity was significantly lower for plants grown in plots treated with ASD-SM or ASD-WM compared to plants grown in ASD-DG soils. No treatment differed significantly from the anaerobic control, but ASD-SM and ASD-WM root rot severity trended lower.
Discussion
Anaerobic soil disinfestation is effective for management of the tomato soilborne disease complex (Testen and Miller, 2018; Testen et al., 2020). To improve this management strategy for Midwestern vegetable growers, we assessed alternative ASD carbon sources, including cover crops, to determine their impact on root rot severity due caused by P. lycopersici and C. coccodes. Carbon sources were evaluated in growth chamber and greenhouse bioassays, and several amendments resulted in reduced root rot severity following ASD treatment. While a subset of carbon sources was evaluated in on-farm trials, their full efficacy was likely not realized due to unusually low soil temperatures during the treatment period.
Agricultural byproducts are easy to procure and relatively inexpensive as ASD carbon sources. Based on 2020 pricing at a feed mill in Northeastern Ohio, the least expensive carbon sources were wheat midds, distillers dried grains and corn gluten feed [all $0.30 (USD) per kilogram] while soybean meal and dry sweet whey cost $0.43 and $1.31 per kilogram, respectively. Wheat midds are nutritionally equivalent to but less costly than wheat bran, while corn gluten feed is a corn processing byproduct with a lower protein (22% protein) content than corn gluten meal (Feedipedia, 2020). No carbon source outperformed wheat bran, a proven ASD standard, for reducing root rot severity. Two carbon sources are not realistic options for Midwestern growers. Whey is not realistic due to high cost and a lack of efficacy at low amendment rates. Corn gluten meal was not evaluated in field trials due to potential phytotoxicity as corn gluten meal is a known bioherbicide (Bingaman and Christians, 1995). The bioherbicide effects of corn gluten meal survived the ASD process. When tomato seeds were directly sown into soils treated after ASD with corn gluten meal, reduced germination and phytotoxicity were observed (data not shown), in line with the pre-emergent herbicide characteristics of corn gluten meal (McDade and Christians, 2000). This led to the need to transplant 2-week-old tomato seedlings in bioassays. Corn gluten meal may be a viable option as transplants are used for tomato production, but field trials are needed to confirm this, and corn gluten feed may be a less phytotoxic and less expensive alternative to corn gluten meal.
Lowering ASD carbon source amendment rates while maintaining treatment efficacy would greatly reduce input costs. While low amendment rates for soybean meal, distiller's grains and wheat midds led to significant reductions in root rot severity after ASD compared to controls in this study, the reductions were less than those observed when high amendment rates were used. Therefore, high amendment rates are needed for effective control of the tomato soilborne disease complex. High carbon source rates are especially needed for autumn applications of ASD when pathogen populations are at their highest following a cropping cycle. Further research is needed to determine the lowest amendment rate that is as effective as the 20.2 Mg/ha amendment rate. One key limitation to amendment rate studies is a lack of pathogen population thresholds associated with yield losses. This information would help to determine whether an ASD carbon source and rate is sufficient to manage root rot. In a meta-analysis of ASD studies, higher carbon source amendment rates tended to lead to greater reductions in soilborne disease damage (Shrestha et al., 2016), so it is likely that most amendment recommendations will trend toward use of higher rates.
When working with disease complexes, it can be difficult to determine which pathogens are most affected by the imposed treatment as the impacts of ASD occur in a carbon source dependent manner (Hewavitharana et al., 2014; Testen and Miller, 2018). Assessment of root rot severity is a general measure of the impacts of ASD on root rotting fungi, while assessment of taproot severity allows us to infer effects on P. lycopersici. While all agricultural byproducts, with the exception of WY-L, reduced recovery of Fusarium spp. compared to the aerobic control, only high rates of corn gluten and distillers dried grains similarly reduced P. lycopersici recovery. No cover crop significantly reduced recovery of any soilborne fungus from roots after ASD, but use of wheat midds in that trial reduced incidence of Fusarium spp. compared to the aerobic control. Use of wheat midds in on-farm trials significantly reduced incidence of P. lycopersici in roots. We did not observe a carbon source that reduced the incidence of C. coccodes recovery in any study presented here. Testen and Miller (2018) demonstrated that use of wheat bran reduced the recovery frequency of P. lycopersici but not C. coccodes following ASD. Most carbon sources examined led to lower taproot rot severity compared to controls, which indicates that P. lycopersici damage is reduced during ASD. Root-knot nematode damage was reduced significantly following ASD with any carbon source in the Wayne trial. This suggests that root-knot nematodes are highly sensitive to ASD and pathogens vary in their sensitivity to ASD applications at low temperatures. Further studies are needed to clarify the efficacy of carbon sources against specific members of the soilborne disease complex and the differential sensitivity of pathogens to ASD carbon sources suggests the need for carbon source mixtures.
Anaerobic soil disinfestation efficacy depends not just on carbon source but also soil temperatures (Shennan et al., 2018), and pathogen populations are more greatly reduced at warmer soil temperatures (Shrestha et al., 2016). In Ohio, autumn applications of ASD fit into tomato cropping cycles used by most farmers, particularly in high tunnels. These autumn ASD applications are made when soilborne pathogen populations are at their highest. Another difficulty of autumn ASD application is that soil temperatures may become too low for effective treatments, especially if applications are made too late in the season or temperatures drop earlier than anticipated. The average soil temperatures in all on-farm trials were lower (average temperatures ranged from 12.0 to 17.3°C) than in other ASD studies conducted in Ohio in which average soil temperatures ranged from 16.3 to 27.8°C for September and October high tunnel applications (Testen et al., 2020) or 23.6 to 30.8°C for summer applications on muck soils (Testen and Miller, 2019). Despite these lower soil temperatures, soil reducing conditions as indicated by iron oxide paint loss were only slightly lower in most trials compared to ASD trials conducted with warmer soil temperatures (Testen and Miller, 2019; Testen et al., 2020). Soil reducing conditions still develop at cool soil temperatures, even if ASD does not effectively reduce soilborne pathogens (Shennan et al., 2018). Average soil temperatures for effective management of the tomato soilborne disease complex likely range from 20 to 25°C, but further growth chamber assays are needed to identify minimum average temperatures at which ASD is effective.
Cover crops had not been examined for control of the tomato soilborne disease complex prior to this study, but they did not prove to be as effective as carbon sources derived from agricultural byproducts. Cover crop amendments led to soil reducing conditions during ASD that were lower than those obtained from use of agricultural byproducts. The grass and clover amendments used in this study reduced root rot relative to the aerobic control after ASD; crimson clover and sorghum sudangrass reduced root rot severity equivalent to wheat midds. No cover crop reduced taproot rot severity in a manner equivalent to wheat midds, but taproot rot severity was, in general, reduced compared to controls. Cover crop amendments did not increase biomass, unlike biomass increases observed with some agricultural byproducts. This may be due to less overall nitrogen provided by cover crops compared to high protein agricultural byproducts. Nutritional content of the cover crops used in this study was not assessed but cover crops can have protein contents in the same range as some effective agricultural byproduct carbon sources, such as wheat midds (15.5–17.3% protein). Crude protein content of cover crops can range from 10 to 24% (Heins and Paulson, 2018), but this is very dependent on growing conditions. Additionally, the carbon within cover crops may be less available to soil microbes than the readily liable carbon in ag byproducts, but this would need to be assessed in future studies. Cover crops may be an option for more frequent ASD applications or as a supplement to agricultural byproduct carbon sources. Cover crops vary in their efficacy as ASD carbon sources. They have been shown to be slightly more effective in reducing Rhizoctonia populations than molasses as ASD carbon sources (McCarty et al., 2014) or as effective as molasses for reducing Fusarium oxysporum but not Sclerotium rolfsii (Butler et al., 2012b).
This study demonstrated the efficacy of multiple carbon sources used in ASD against the tomato soilborne disease complex. While no carbon source significantly reduced root rot significantly compared to controls in on-farm trials due to low treatment soil temperatures, wheat bran and midds, soybean meal, distillers dried grains, and corn gluten meal consistently reduced disease in bioassays for which soil temperatures were higher. Future studies to assess the efficacy of carbon sources against specific pathogens at a range of temperatures and timings would allow farmers to fine-tune their carbon source selection for soil pathogens present on their farms and current weather conditions. Future studies should also examine combinations of carbon sources or use of cover crops to supplement carbon sources to design ASD amendment mixtures to target a wider range of soilborne pathogens.
Data Availability Statement
The raw data supporting the conclusions of this article will be made available by the authors, without undue reservation.
Author Contributions
AT and SM designed the study. AT, FR, MH, and MM conducted field, greenhouse, and lab work. AT conducted statistical analyses and wrote the first draft of the manuscript. All authors edited the manuscript and approved submission.
Funding
This research was funded by a Research and Education grant (LNC17-393) from the North Central Regional SARE program and state and federal funds appropriated to The Ohio State University College of Food, Agricultural, and Environmental Sciences.
Conflict of Interest
The authors declare that the research was conducted in the absence of any commercial or financial relationships that could be construed as a potential conflict of interest.
Acknowledgments
We thank A. Nanes, R. Khadka, M. Bosques-Martinez, J. Anderson, J. Pollok, J. Garcia, H. Kanyagha, and T. Klass for assistance in setting up field trials. We thank The Ohio State University Feedstock Processing Research Facility for carbon sources for the carbon source screening and our farmer collaborators for providing their assistance in this project.
References
Achmon, Y., Harrold, D. R., Claypool, J. T., Stapleton, J. J., VanderGheynst, J. S., and Simmons, C. W. (2016). Assessment of tomato and wine processing solid wastes as soil amendments for biosolarization. Waste Manag. 48, 156–164. doi: 10.1016/j.wasman.2015.10.022
Allison, P. D. (1999). Logistic Regression Using the SAS System: Theory and Application. Cary, NC: SAS Institute Inc.
Bingaman, B. R., and Christians, N. E. (1995). Greenhouse screening of corn gluten meal as a natural control product for broadleaf and grass weeds. HortScience 30, 1256–1259. doi: 10.21273/HORTSCI.30.6.1256
Blok, W. J., Lamers, J. G., Termorshuizen, A. J., and Bollen, G. J. (2000). Control of soilborne plant pathogens by incorporating fresh organic amendments followed by tarping. Phytopathology 90, 253–259. doi: 10.1094/PHYTO.2000.90.3.253
Butler, D. M., Kokalis-Burelle, N., Muramoto, J., Shennan, C., McCollum, T. G., and Rosskopf, E. N. (2012a). Impact of anaerobic soil disinfestation combined with soil solarization on plant–parasitic nematodes and introduced inoculum of soilborne plant pathogens in raised-bed vegetable production. Crop Prot. 39, 33–40. doi: 10.1016/j.cropro.2012.03.019
Butler, D. M., Rosskopf, E. N., Kokalis-Burelle, N., Albano, J. P., Muramoto, J., and Shennan, C. (2012b). Exploring warm-season cover crops as carbon sources for anaerobic soil disinfestation (ASD). Plant Soil 355, 149–165. doi: 10.1007/s11104-011-1088-0
Derr, R. E. (2013). “Ordinal response modeling with the LOGISTIC procedure,” in Proceedings of the SAS Global Forum 2013 Conference (Cary, NC: SAS Institute Inc).
Domínguez, P., Miranda, L., Soria, C., Santos, B., de los Chamorro, M., Romero, F., et al. (2014). Soil biosolarization for sustainable strawberry production. Agron. Sustain. Dev. 34, 821–829. doi: 10.1007/s13593-014-0211-z
Feedipedia. (2020). An On-Line Encyclopedia of Animal Feeds. Feedipedia. Available online at: https://www.feedipedia.org/ (accessed April 27, 2020).
Goud, J.-K. C., Termorshuizen, A. J., Blok, W. J., and van Bruggen, A. H. C. (2004). Long-term effect of biological soil disinfestation on verticillium wilt. Plant Dis. 88, 688–694. doi: 10.1094/PDIS.2004.88.7.688
Heins, B., and Paulson, J. (2018). Research: Cover Crops for Grazing Systems. University Minneapolis Extension Publication. Available online at: https://extension.umn.edu/soil-management-and-health/research-cover-crops-grazing-systems
Hewavitharana, S. S., Ruddell, D., and Mazzola, M. (2014). Carbon source-dependent antifungal and nematicidal volatiles derived during anaerobic soil disinfestation. Eur. J. Plant Pathol. 140, 39–52. doi: 10.1007/s10658-014-0442-5
Katase, M., Kubo, C., Ushio, S., Ootsuka, E., Takeuchi, T., and Mizukubo, T. (2009). Nematicidal activity of volatile fatty acids generated from wheat bran in reductive soil disinfestation. Nematol,. Res. 39, 53–62. doi: 10.3725/jjn.39.53
Khadka, R. B., Marasini, M., Rawal, R., Testen, A. L., and Miller, S. A. (2020). Effects of anaerobic soil disinfestation carbon sources on soilborne diseases and weeds of okra and eggplant in Nepal. Crop Prot. 135:104846. doi: 10.1016/j.cropro.2019.104846
Korthals, G. W., Thoden, T. C., van den Berg, W., and Visser, J. H. M. (2014). Long-term effects of eight soil health treatments to control plant-parasitic nematodes and Verticillium dahliae in agro-ecosystems. Appl. Soil Ecol. 76, 112–123. doi: 10.1016/j.apsoil.2013.12.016
Lamers, J. G., Runia, W. T., Molendijk, L. P. G., and Bleeker, P. O. (2010). Perspectives of anaerobic soil disinfestation. Acta Hortic. 883, 277–283. doi: 10.17660/ActaHortic.2010.883.34
López-Robles, J., Olalla, C., Rad, C., Díez-Rojo, M. A., López-Pérez, J. A., Bello, A., et al. (2013). The use of liquid swine manure for the control of potato cyst nematode through soil disinfestation in laboratory conditions. Crop Prot. 49, 1–7. doi: 10.1016/j.cropro.2013.03.004
Ludeking, D. J. W., Paternotte, S. J., Runia, W. T., and Molendijk, L. P. G. (2011). Biological soil disinfestation with organic fermentation products. Acta Hortic. 915, 133–139. doi: 10.17660/ActaHortic.2011.915.16
McCarty, D. G., Inwood, S. E. E., Ownley, B. H., Sams, C. E., Wszelaki, A. L., and Butler, D. M. (2014). Field evaluation of carbon sources for anaerobic soil disinfestation in tomato and bell pepper production in Tennessee. HortScience 49, 272–280. doi: 10.21273/HORTSCI.49.3.272
McDade, M. C., and Christians, N. E. (2000). Corn gluten meal—a natural preemergence herbicide: effect on vegetable seedling survival and weed cover. Am. J. Altern. Agric. 15, 189–191. doi: 10.1017/S0889189300008778
Messiha, N. A. S., Diepeningen, A. D., van Wenneker, M., Beuningen, A. R., van Janse, J. D., Coenen, T. G. C., et al. (2007). Biological soil disinfestation (BSD), a new control method for potato brown rot, caused by Ralstonia solanacearum race 3 biovar 2. Eur. J. Plant Pathol. 117, 403–415. doi: 10.1007/s10658-007-9109-9
Momma, N., Kobara, Y., Uematsu, S., Kita, N., and Shinmura, A. (2013). Development of biological soil disinfestations in Japan. Appl. Microbiol. Biotechnol. 97, 3801–3809. doi: 10.1007/s00253-013-4826-9
Momma, N., Momma, M., and Kobara, Y. (2010). Biological soil disinfestation using ethanol: effect on Fusarium oxysporum f. sp. lycopersici and soil microorganisms. J. Gen. Plant Pathol. 76, 336–344. doi: 10.1007/s10327-010-0252-3
Núñez-Zofío, M., Larregla, S., and Garbisu, C. (2011). Application of organic amendments followed by soil plastic mulching reduces the incidence of Phytophthora capsici in pepper crops under temperate climate. Crop Prot. 30, 1563–1572. doi: 10.1016/j.cropro.2011.08.020
Rabenhorst, M. C. (2008). Protocol for using and interpreting IRIS tubes. Soil Surv. Horiz. 49, 74–77. doi: 10.2136/sh2008.3.0074
Rabenhorst, M. C. (2012). Simple and reliable approach for quantifying IRIS tube data. Soil Sci. Soc. Am. J. 76, 307–308. doi: 10.2136/sssaj2011.0267n
Rabenhorst, M. C., and Burch, S. N. (2006). Synthetic iron oxides as an indicator of reduction in soils (IRIS). Soil Sci. Soc. Am. J. 70, 1227–1236. doi: 10.2136/sssaj2005.0354
Serrano-Pérez, P., Rosskopf, E., De Santiago, A., and Rodríguez-Molina, M. C. (2017). Anaerobic soil disinfestation reduces survival and infectivity of Phytophthora nicotianae chlamydospores in pepper. Sci. Hortic. 215, 38–48. doi: 10.1016/j.scienta.2016.12.003
Shennan, C., Muramoto, J., Koike, S., Baird, G., Fennimore, S., Samtani, J., et al. (2018). Anaerobic soil disinfestation is an alternative to soil fumigation for control of some soilborne pathogens in strawberry production. Plant Pathol. 67, 51–66. doi: 10.1111/ppa.12721
Shrestha, U., Aug,é, R. M., and Butler, D. M. (2016). A meta-analysis of the impact of anaerobic soil disinfestation on pest suppression and yield of horticultural crops. Front. Plant Sci. 7:1254. doi: 10.3389/fpls.2016.01254
Strauss, S. L., and Kluepfel, D. A. (2015). Anaerobic soil disinfestation: a chemical-independent approach to pre-plant control of plant pathogens. J. Integr. Agric. 14, 2309–2318. doi: 10.1016/S2095-3119(15)61118-2
Testen, A. L., Bosques Martinez, M., Jimenez Madrid, A., Deblais, L., Taylor, C., Paul, P. A., et al. (2020). On-farm evaluations of anaerobic soil disinfestation and grafting for management of a widespread soilborne disease complex in protected culture tomato production. Phytopathology. doi: 10.1094/PHYTO-07-20-0288-R
Testen, A. L., and Miller, S. A. (2018). Carbon source and soil origin shape soil microbiomes and tomato soilborne pathogen populations during anaerobic soil disinfestation. Phytobiomes 2, 138–150. doi: 10.1094/PBIOMES-02-18-0007-R
Testen, A. L., and Miller, S. A. (2019). Anaerobic soil disinfestation to manage soilborne diseases in muck soil vegetable production systems. Plant Dis. 103, 1757–1762. doi: 10.1094/PDIS-09-18-1578-RE
van Overbeek, L., Runia, W., Kastelein, P., and Molendijk, L. (2014). Anaerobic disinfestation of tare soils contaminated with Ralstonia solanacearum biovar 2 and Globodera pallida. Eur. J. Plant Pathol. 138, 323–330. doi: 10.1007/s10658-013-0331-3
Vecchia, L., Di Gioia, F., Ferrante, A., Hong, J. C., White, C., and Rosskopf, E. N. (2020). Integrating cover crops as a source of carbon for anaerobic soil disinfestation. Agronomy 10:1614. doi: 10.3390/agronomy10101614
Vrisman, C. M., Testen, A. L., Elahi, F., and Miller, S. A. (2017). First report of tomato brown root rot complex caused by Colletotrichum coccodes and Pyrenochaeta lycopersici in Ohio. Plant Dis. 101:247. doi: 10.1094/PDIS-05-16-0623-PDN
Keywords: Pyrenochaeta lycopersici, Colletotrichum coccodes, Meloidogyne, soil amendment, disease management, Verticillium dahliae
Citation: Testen AL, Rotondo F, Mills MP, Horvat MM and Miller SA (2021) Evaluation of Agricultural Byproducts and Cover Crops as Anaerobic Soil Disinfestation Carbon Sources for Managing a Soilborne Disease Complex in High Tunnel Tomatoes. Front. Sustain. Food Syst. 5:645197. doi: 10.3389/fsufs.2021.645197
Received: 22 December 2020; Accepted: 08 April 2021;
Published: 13 May 2021.
Edited by:
Francesco Di Gioia, Pennsylvania State University (PSU), United StatesReviewed by:
David Michael Butler, The University of Tennessee, Knoxville, United StatesJason Hong, Horticultural Research Laboratory (USDA-ARS), United States
Copyright © 2021 Testen, Rotondo, Mills, Horvat and Miller. This is an open-access article distributed under the terms of the Creative Commons Attribution License (CC BY). The use, distribution or reproduction in other forums is permitted, provided the original author(s) and the copyright owner(s) are credited and that the original publication in this journal is cited, in accordance with accepted academic practice. No use, distribution or reproduction is permitted which does not comply with these terms.
*Correspondence: Anna L. Testen, YW5uYS50ZXN0ZW5AdXNkYS5nb3Y=