- 1School of Natural Sciences, Bangor University, Bangor, United Kingdom
- 2Leibniz Centre for Agricultural Landscape Research, Müncheberg, Germany
- 3Scotland's Rural College, West Mains Road, Edinburgh, United Kingdom
- 4Department of Botany, School of Natural Sciences, Trinity College Dublin, Dublin, Ireland
- 5School of Engineering, University of Limerick, Limerick, Ireland
Introducing legumes to crop rotations could contribute toward healthy and sustainable diet transitions, but the current evidence base is fragmented across studies that evaluate specific aspects of sustainability and nutrition in isolation. Few previous studies have accounted for interactions among crops, or the aggregate nutritional output of rotations, to benchmark the efficiency of modified cropping sequences. We applied life cycle assessment to compare the environmental efficiency of ten rotations across three European climatic zones in terms of delivery of human and livestock nutrition. The introduction of grain legumes into conventional cereal and oilseed rotations delivered human nutrition at lower environmental cost for most of the 16 impact categories studied. In Scotland, the introduction of a legume crop into the typical rotation reduced external nitrogen requirements by almost half to achieve the same human nutrition potential. In terms of livestock nutrition, legume-modified rotations also delivered more digestible protein at lower environmental cost compared with conventional rotations. However, legume-modified rotations delivered less metabolisable energy for livestock per hectare-year in two out of the three zones, and at intermediate environmental cost for one zone. Our results show that choice of functional unit has an important influence on the apparent efficiency of different crop rotations, and highlight a need for more research to develop functional units representing multiple nutritional attributes of crops for livestock feed. Nonetheless, results point to an important role for increased legume cultivation in Europe to contribute to the farm and diet sustainability goals of the European Union's Farm to Fork strategy.
Highlights
- Life Cycle Assessment was undertaken for 10 cropping sequences across 16 impact categories
- Two functional units were applied to rotation outputs: human and animal nutrition potential
- Legume-modified rotations were compared with conventional baseline rotations
- Legume-modified rotations deliver nutrition at lower environmental cost
- Legume-modified rotations derive maximum benefit when crops go direct to human nutrition.
Introduction
Agricultural practices must evolve to deliver food security whilst reducing environmental impact. On the one hand, modern technologies have been developed and adopted to apply inputs such as fertilizers and water with more precision, producing crops more efficiently within “conventional” intensive systems. On the other hand, there are efforts to break the current state of technological lock-in of intensive mono-cropping by promoting “agro-ecological” intensification in order to reduce high dependence on finite resources such as phosphorus fertilizers and fossil energy whilst reducing greenhouse gas (GHG) emissions, loss of reactive nitrogen and soil degradation (Rockström et al., 2020). Such agro-ecological intensification may include more biological nitrogen fixation by legumes, extended rotations, intercropping and possible introduction of livestock into crop rotations. The European Green Deal Farm to Fork strategy aims to develop a “fair, healthy, and environmentally-friendly food system,” with specific objectives to reduce GHG emissions and chemical pesticide use by 50% and synthetic fertilizer use by 20% by 2030 (European Union, 2020).
Legumes are arable crops from the Leguminosae family, which have the ability to fix nitrogen from the atmosphere and therefore avoid the use of other external sources of nitrogen fertilizers (Peoples et al., 2019). These crops provide a significant quantity of nitrogen to following crops, reducing mineral fertilizer requirements and GHG emissions across entire rotations (Rochette and Janzen, 2005; Watson et al., 2017). Legume cultivation has been associated with other benefits, including diversification of crop rotations (Nemecek et al., 2008; Hufnagel et al., 2020) which can break pest and disease cycles (MacWilliam et al., 2014), improved soil quality and drought resistance through deep root systems, and support for pollinating insects (Peoples et al., 2019). Legumes are mainly grown for food and feed purposes (Nemecek et al., 2008; Watson et al., 2017), but they also supply value chains for, inter alia, alcoholic beverages (Lienhardt et al., 2019), biorefineries (Karlsson et al., 2015) or green manures (Baddeley et al., 2017).
From a human nutritional perspective, legumes are a source of macro- and micro-nutrients providing protein, fiber, folate, iron, potassium, and magnesium and vitamins (Chaudhary et al., 2018b), delivering a richer nutrient profile than cereals or meat alternatives. Substituting meat with protein-rich legume-derived foods has the potential to simultaneously decrease environmental impact whilst improving nutritional profile (Jensen et al., 2012; Peoples et al., 2019; Saget et al., 2020). The EAT-Lancet Commission “planetary healthy” diet recommends a lower daily intake of red meat and an increase of legume intake to deliver a diet which is simultaneously more nutritious and sustainable (Willett et al., 2019). Saget et al. (2021) has shown that replacing just 5% of beef in Germany with pea protein balls could resut in climate mitigation of 8 million tons CO2 eq. annually, 1% of Germany's annual GHG emissions.
Despite these pertinent benefits, legumes are not widely cultivated in Europe, covering only 1.5% of European arable land, compared to 14.5% worldwide (Watson et al., 2017). Meanwhile, large quantities of soybean are imported into Europe as protein-rich animal feed, from countries where its production may drive deforestation (Watson et al., 2017). Therefore, the introduction of legumes to European crop rotations could play a key role in Europe's Farm to Fork strategy, but the current evidence base is fragmented across studies that typically evaluate specific aspects of environmental sustainability and nutrition in isolation. There is an urgent need for more holistic Life Cycle Assessment (LCA) approaches to evaluate the environmental sustainability of increased legume cropping in Europe, using complex functional units (FU) or more sophisticated biophysical allocation across crop products (Brankatschk and Finkbeiner, 2014) in order to represent: (i) the dynamics of particular cropping sequences; (ii) functional output in relation to balanced nutritional requirements.
Cultivating new crops incurs changes to rotation systems (cropping sequences) that have environmental and productivity implications beyond the specific inputs and outputs of the new crop in question. Yet, with few exceptions (Nemecek et al., 2008; MacWilliam et al., 2014), most LCA studies are designed to investigate one isolated crop rather than the whole crop rotation, often missing important nutrient cycling (via crop residues) and crop sequence effects that can strongly influence comparative environmental efficiency (Costa et al., 2020). Numerous authors encourage analysis of entire systems rather than individual crops (Brankatschk and Finkbeiner, 2015; Peter et al., 2017; Brankatschk, 2018). Analyzing whole rotation sequences from cradle-to-gate introduces the challenge of selecting an appropriate functional unit (FU) to represent multiple crop outputs. Previous rotation LCA studies have often related environmental burdens to highly simplified FU such as tons of dry matter or ha.yr (hectare per year) cultivated (e.g., Plaza-Bonilla et al., 2018). Such FU can be misleading, through disregard for the nutritional value of different crops and via the implication that less agricultural activity (and thus potentially productivity) per unit area is always environmentally favorable (Brankatschk, 2018). Brankatschk and Finkbeiner (2014) propose the Cereal Unit (CU), a metric based on the digestible energy content of each crop, to aggregate multiple crop outputs across rotations intended to produce animal feed. An alternative FU is the amount of protein provided for feed (Karlsson et al., 2015). Reflecting the lack of consensus regarding the FU for rotational systems, and the potentially diverse end uses of crops, it may be prudent to apply more than one FU when benchmarking environmental efficiency across different systems (Goglio et al., 2018).
Meanwhile, food (rather than farm) LCA studies have applied FUs defined by single or multiple aspects of human nutrition. Sonesson et al. (2017) propose a quality-adjusted protein metric which considers essential amino acids. Notarnicola et al. (2017) highlight the importance of looking at the wider nutritional composition of products, in terms of fat, protein, and energy content amongst other relevant nutrients. Recently, other authors have combined multiple nutrients within a single functional unit, such as the Nutrient Balance Score (Chaudhary et al., 2018a,b), or the Nutrient Density Unit, a simplified FU that considers the balance of protein, fiber, essential fatty acids, and energy content in a certain product (van Dooren, 2017). These innovations have been applied in recent LCA studies to better represent the nutritional functionality of different foods (McAuliffe et al., 2020). However, with few exceptions (MacWilliam et al., 2014; Li et al., 2018), these more complex metrics of human nutrition have not yet been applied to compare the efficiency of different crop rotations.
In this modeling study, we apply three FUs to benchmark the environmental efficiency of legume-modified crop rotations against counterpart conventional rotations across three climatic regions of Europe, considering potential nutrition delivery to livestock and directly to humans.
Methods
Rotations Across Europe
This study compares the environmental impact of ten crop rotations across three contrasting geo-climatic arable regions in Europe (Table 1). Rotations are categorized into three typologies: cereal-cereal [C-C], cereal-oilseed [C-O], and cereal-oilseed-legume [C-O-L] systems. The first region analyzed was Calabria, southern Italy (IT), representing Mediterranean Europe. The second was Sud-Muntenia in Romania (RO), representing continental Europe, and the last region was eastern Scotland (SC), representing Atlantic Europe. Simulated rotations were adapted from Reckling et al. (2016a), modeled using a rotation generator (Reckling et al., 2016b) in which the following aspects were considered: (i) Crop rotations spanning between 3 and 6 years, (ii) suitable crop sequences (iii) frequency of a crop in rotation (iv) minimum break between the same crops and (iv) maximum frequency of crops of the same crop types. Management of the rotations and further assumptions are available in Supplementary Table 1, whilst details of nutrient cycling and emission factors are summarized in Inventory and Impact Assessment framework.
The Life Cycle Assessment Method
Goal and Scope
An attributional cradle-to-farm-gate LCA was used to benchmark the environmental efficiency of legume-modified rotations against typical rotations that they may replace in different regions of Europe, in relation to provision of feed and food, using novel nutrition-based FUs. The target audience for this study comprises researchers and policymakers with an interest in more sustainable cropping systems for food and feed nutrition. Since our main goal concerns entire rotations and not individual products, we chose FUs to represent potential nutrition for human food and animal feed. Use of crops for bioenergy systems or direct livestock grazing are outside the current scope.
Functional Unit for Human Food (FUFood)
The first sub-goal was to quantify the potential contribution of crude grain rotation outputs to human nutrition. Human food nutritional FUs are commonly applied to processed food rather than farm-level LCA studies (McAuliffe et al., 2020). FUs based on nutrient scores aggregate quantities of different nutrients, ranging from three nutrients as proposed by van Dooren (2017) for the Nutrient Density Unit (NDU) to 27 nutrients as applied by Chaudhary et al. (2018b). The latter metric is particularly relevant to assess food prepared for final consumption, often containing many ingredients. However, the former metric is simpler, especially for crude agricultural grains that have not yet been processed into final products and therefore cannot be assessed at high resolution. Additionally, the three-nutrient and energy score correlates well with the nutrient-rich foods index (NRF 12:3) as shown by Saget et al. (2020). Thus, we adapted the formula proposed by van Dooren (2016), accounting for protein, fiber, and energy content of the crude grains compared to the daily recommended intake values (Equation 1). We omitted the essential fatty acid owing to lack of comparable data.
Equation (1): The Nutrient density Unit, adapted from van Dooren (2016):
Protein is the amount of protein in 100 g of the product, expressed in grams.
Fiber is the amount of fiber in 100 g of the product, expressed in grams.
DVprot is the recommended daily value intake of protein, expressed in grams.
DVfibre is the recommended daily value intake of fiber, expressed in grams.
Si is the kilocalorie energy content in 100 g of the product.
SDRI is the recommended daily intake of energy, expressed in kilocalories.
DVprotand DVfibrewere set at 50 and 25, respectively based on a 2,000 kcal dietary reference intake (SDRI) as proposed by van Dooren (2017). In order to use readily available and consistent data for both food and feed FUs in this “proof-of-concept” study, human-digestible protein, fiber, and energy values were taken from (Heuzé et al., 2017), using values for pigs as proxies. Implications of data availability are discussed later. The composition of nutritional values calculated for each crop can be found in Supplementary Table 3.
Functional Unit for Animal Feed (FUFeed)
For the second sub-goal, to evaluate the efficiency of rotations to deliver animal feed, we analyzed two FU:
(i) Cereal unit (CU) (Brankatschk and Finkbeiner, 2014), representing the sum of metabolisable energy in all macronutrients (crude protein, crude lipids, crude fiber, and nitrogen-free extracts containing hydrocarbons) – calculated as weighted average energy across the German livestock profile (pigs, poultry, cattle, and horses). The final value is converted into 1 kg of barley feed energy equivalent.
(ii) Total digestible protein (DP) content, considering values for ruminants, of each grain crop and straw under the rotations.
The values for crude protein were taken from Heuzé et al. (2017), while values for the cereal unit were adopted from Brankatschk and Finkbeiner (2014). The values for final DP and CU for each crop and straw were calculated firstly per kilo of product, then multiplied by the yields of that product, and finally aggregated by summing all products across each rotation. The final output of each rotation was then divided by the time length (years) of the rotation. To ensure transparency, the calculation of each crop under each rotation is recorded in Supplementary Table 3. No variation of the nutrient content was considered for the crop according to fertilization rates or regional aspects. The final input and output of all rotations per FU analyzed can be observed in Supplementary Table 5.
System Boundaries
The LCA was completed from cradle to farm gate. All processes from the extraction of raw materials, manufacturing, use, and all farm operations up to the harvesting of the grains were considered. Since the main goal was to study crop rotation sequences, downstream processes such as transportation, drying, and storage of grains, were excluded from the analysis. System boundaries are described in Figure 1.
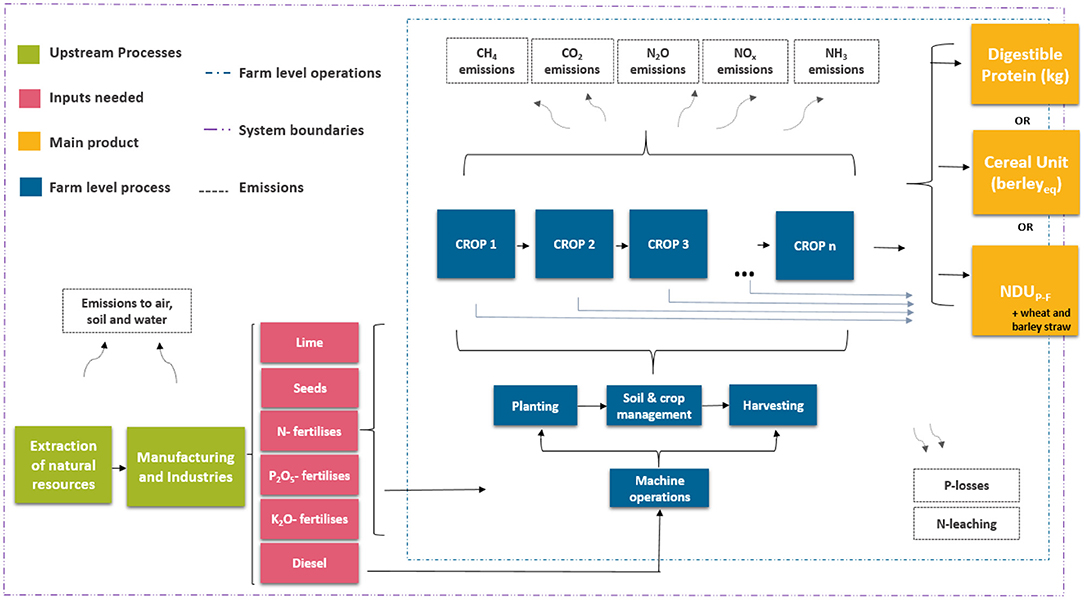
Figure 1. LCA boundaries of the rotation systems to deliver nutrition to animals (DP and CU) and to humans (NDUP−F).
Inventory and Impact Assessment Framework
Modeling was undertaken in Open LCA v1.9 (GreenDelta, 2006), using Ecoinvent v.3.5 database for background data (Moreno-Ruiz et al., 2018). Activity data and crop performance all originate from crop sequence simulations published previously (Reckling et al., 2016a). All field emissions were re-calculated in the present study based on the most recent emission factors, with the exception of nitrate () leaching which was calculated using an N balance approach in Reckling et al. (2016a). In this study, ammonia (NH3), nitrous oxide (N2O), and carbon dioxide (CO2) emissions were calculated according to Intergovernmental Panel on Climate Change (IPCC) (2006, 2019) emission factors (Supplementary Table 2), whilst phosphorus (P) runoff was calculated according to a 1% loss factor applied in a previous crop LCA study (Styles et al., 2015) (Supplementary Table 2). The inventory was generated using assumptions and allocations fully described in section Assumptions in the Supplementary Material.
Life Cycle Impact Assessment (LCIA) was performed using the method recommended by the European Commission - Product Environmental Footprint (PEF) guidelines (European Environmental Bureau, 2018). This method was selected because it is comprehensive and aligns with the aim to harmonize European environmental footprint studies. The method recommends the calculation of 16 environmental impact categories (Supplementary Table 4) and is appropriate to the geographic location of the analyzed rotations (i.e., Europe). PEF guidelines were also followed for normalization. After presentation in their specific units, indicator values for each impact category were divided by average annual EU27 per capita burdens to generate normalized scores. Normalized scores (expressed as person.year−1) for all categories were summed up to calculate total environmental impact with an assumption of equal weighting, an optional step in PEF guidelines (European Environmental Bureau, 2018) that can facilitate simplified communication and reporting. Categories with the largest normalized scores, cumulatively responsible for at least 80% of the total environmental impact, were investigated in more detail in the results section. The human toxicity categories were not reported in detail because (i) there was no primary or secondary data about crop protection application to the rotations, and (ii) of the uncertainty related to these categories, classified as interim categories within the PEF method (European Environmental Bureau, 2018).
Sensitivity Analysis
We decided to test the robustness of the apparent efficiency of legume rotations for the NDUP−F FU by simulating more efficient N-fertilizer use across all non-legume crops in each rotation. The simulation assumed use of nitrification inhibitors (NI) and urease inhibitors (UI). We varied the N2O Emission Factors, yields, and N application based on published meta-analyses (Abalos et al., 2014; Gilsanz et al., 2016; Thapa et al., 2016; Li et al., 2017) to understand how this would affect the results on overall impact categories. The specific factors adopted are elaborated in section Assumptions in the Supplementary Material.
Results
Overall, the legume-modified rotations delivered more DP per ha per year (animal feed) and more NDUP−F (human food) across the regions studied (Table 2). This was not the case for CU (animal feed). These results are explained further in sections Human Nutrition Footprints for human nutrition and Animal Nutrition Footprints for animal nutrition.
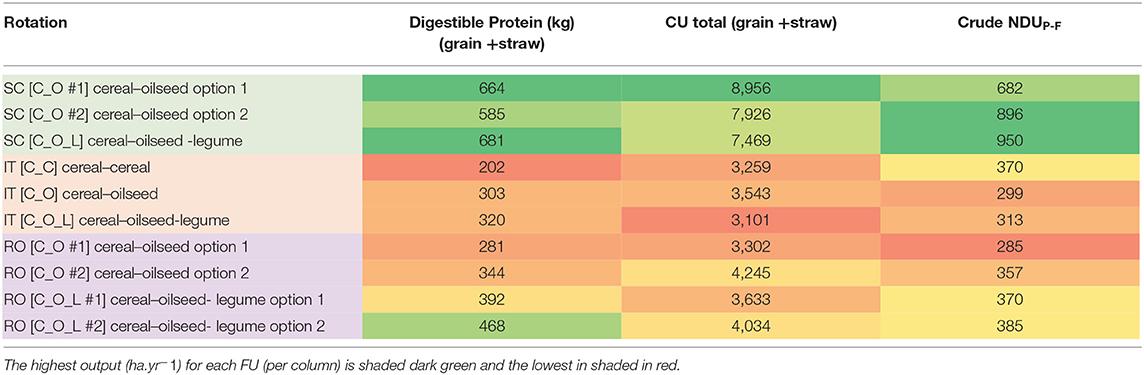
Table 2. Outputs of the rotations in terms of DP, CU, and NDUP−F per hectare per year of each rotation in Scotland (SC), Italy (IT), and Romania (RO).
Impact Category Results
The results for all 16 impact categories across each impact category and for each nutritional FU are displayed in radar charts (normalized scores) and tables (indicator values) below, and in (Supplementary Figure 1). Six impact categories were responsible for at least 80% of the total environmental impact: climate change, terrestrial eutrophication, marine eutrophication, land use, terrestrial and freshwater acidification, and respiratory inorganics (Supplementary Table 8). The results for these impact categories are described below.
Process Contributions
For each of the six priority impact categories, we investigated process contributions >1% of the impact for each rotation. Five of the six priority impact categories relate to synthetic nitrogen fertilizers (SNF) and associated field emissions. We found that NH3 emission into the air due to volatilization from N-based fertilizers was the main driver for terrestrial and freshwater acidification and respiratory inorganics. Climate change potential is driven by N2O emissions after SNF application, followed by CO2 emitted by urea and lime application and by nitric acid production (Figure 2). The latter emission is derived from a market dataset for this fertilizer formulation from the Ecoinvent database (Moreno-Ruiz et al., 2018). In this study, at least 95% of overall land use relates to direct land occupation by each rotation sequence (Supplementary Table 7). The land use category is therefore inversely related to land efficiency, i.e., how many hectares are needed to deliver the FU (Table 2). Marine eutrophication potential is mostly linked with leaching (Figure 2) and this data was calculated for each crop under each rotation from the model of Reckling et al. (2016a).
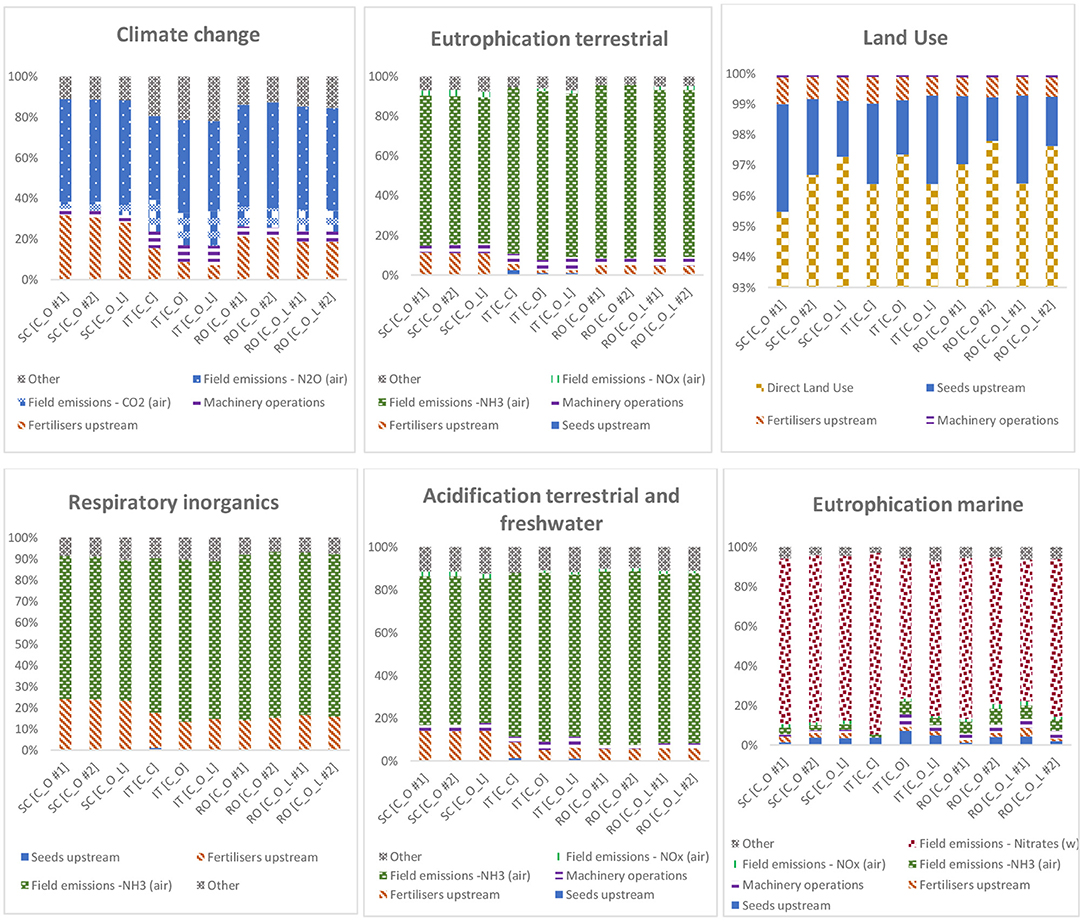
Figure 2. Process contributions for the six priority impact categories across the ten rotations of cereal (C_), oilseed (_O_), and legume (_L) in Scotland (SC), Italy (IT), and Romania (RO) for the NDUP−F FU.
Human Nutrition Footprints
The greatest amount of (potential) human nutrition per hectare year was delivered by Scottish rotations (NDUP−F 682–950), followed by Romanian (285–385) and Italian (299–370) rotations, respectively. The highest NDU P−F values are associated with higher-yielding legume-modified rotations in Scotland (Table 2). The SNF applications per one NDUP−F for each rotation can be observed in Supplementary Table 6, as a useful metric of nutrient use efficiency from a nutrition perspective and as a proxy for wider resource and environmental efficiency. Italian rotations had the lowest SNF requirement per NDUP−F followed by Scotland and Romania. However, the introduction of a legume crop into the Scottish rotation was highly beneficial, reducing the SNF requirements per NDUP−F by almost half, from 0.28 kg N per NDUP−F for cereal-oilseed rotation (SC [C_O #1]) to 0.14 kg N per NDUP−F for the legume-modified option (SC [C_O_L]). The Romania legume-modified rotation incurred a reduction of 0.15 kg N per NDUP−F, from 0.36 kg N/NDUP−F for the cereal-oilseed rotation (RO [C_O #1]), to 0.21 kg N/NDUP−F for the legume-modified option (RO [C_O_L #2]) (Supplementary Table 6). Italian rotations presented both the smallest requirement of SNF per NDUP−F (0.04 kg N and 0.11 kg N for C_O and C_O_L rotations, respectively) and the smallest reduction of SNF attributable to the legume-modified rotation (0.04 kg N per NDUP−F).
For the FUFood, all the legume rotations across all regions incurred lower environmental impacts across the majority of the 16 environmental impact categories assessed (Table 3). Scottish legume-modified rotations performed better across all impact categories. A few trade-offs were found for Romania, where the legume-modified rotation scored better in 14 impact categories but worse in two categories (Ecotoxicity freshwater and marine eutrophication) relative to non-legume rotations (Table 3). For Italy, more trade-offs were observed, where the legume-modified rotation scored better across 10 out of 16 impact categories compared with both the rapeseed and cereal rotations. The Romanian legume-modified rotations showed a slightly better performance for soybean than the common bean. Despite the yields of soybean being slightly lower, the NDUP−F of the grain is higher. The radar chart for Romanian rotations is available below (Figure 3), while the equivalent figures for Scottish and Italian rotations can be found in Supplementary Figure 1.
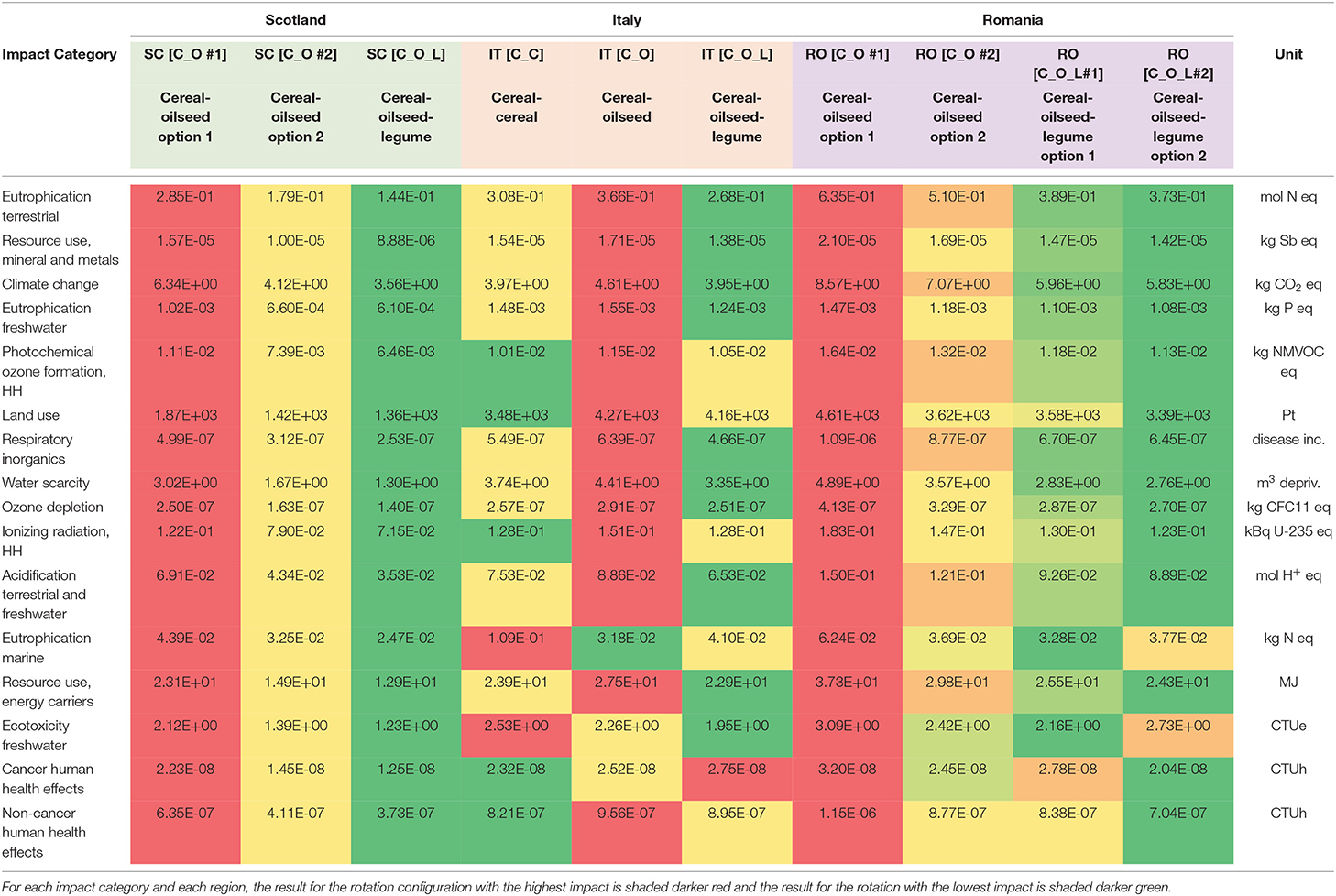
Table 3. Heat map of impact scores across all impact categories for all rotations analyzed in Scotland (SC), Italy (IT), and Romania (RO), expressed per unit of protein, fiber, and energy nutritional output (NDUP−F).
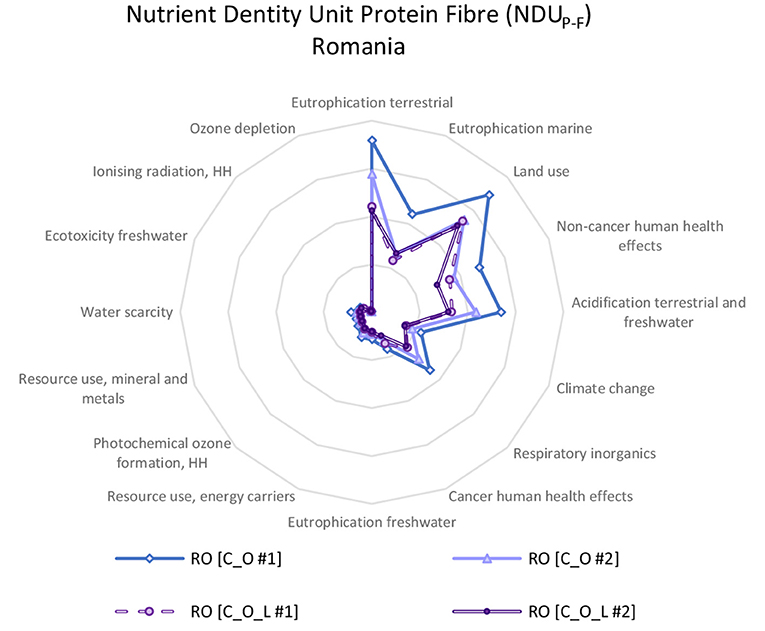
Figure 3. Normalized environmental scores per unit of protein and fiber nutritional output (NDUP−F) across Romanian crop rotations. RO [C_O #1] refers to cereal-oilseed rotation option 1, RO [C_O #2] refers to cereal-oilseed rotation option 2, RO [C_O_L #1] refers to cereal-oilseed-legume rotation option 1 (with common bean) and [C_O_L #2] refers to cereal-oilseed-legume rotation option 2 (with soybean).
The introduction of fava beans with a high NDUP−F into the Italian cereal-rapeseed rotation increased the DM yield of the following rapeseed crop by 20%, from 2,275 kg ha−1 to 2,730 kg ha−1 (Supplementary Table 5). However, in the Italian cereal-cereal rotations, the oat crop (twice in the rotation) contributed to a higher NDUP−F ha.yr−1 for this rotation compared to the cereal–oilseed and to the cereal–oilseed-legume options in the region, owing to the yield and nutritional composition (high fiber) of oats. In Italy, the amount of fertilizer required per NDUP−F was highest for the cereal-oilseed rotation, making it the worst performing of the Italian rotations across all impact categories except marine eutrophication. The Italian cereal-cereal rotation incurred high burdens in this category due to the high leaching values for winter oats.
The introduction of peas in the Scottish cereal-rapeseed rotation decreased the requirement for SNF whilst increasing the final output of NDUP−F. This happens because peas have a higher NDUP−F per kilo of grain (Supplementary Table 3), and even whilst yielding 860 kg ha−1 less than spring barley, they deliver almost 180 more NDUP−F ha−1 (Supplementary Table 5). Additionally, peas need no SNF. Peas are also responsible for an increase of 94 NDUP−F ha−1 from the following barley crop due to a yield boost compared with the cereal-oilseed rotation in Scotland (Supplementary Table 5). Therefore, the Scottish legume modified rotation achieves the highest overall environmental efficiency per NDUP−F (Table 2). The Scottish cereal-oilseed rotation 2 with nutrient-dense oats scores better than the cereal-oilseed rotation 1 with a less-nutritionally-dense second wheat crop (SC [C_O #1]) (Supplementary Table 5).
Environmental burdens per NDUP−F are lower for Scottish rotations than Italian rotations, except for climate change. Despite the higher SNF requirements in Scotland than Italy (Supplementary Table 6), this is because of the N source used. According to the International Fertilization Association information from 2015 to 2018 (IFASTAT, 2020), Italy consumes at least 72% of N in the form of urea. Urea fertilizer not only releases carbon when applied but also has a higher ammonia volatilization rate of 15% against 5% for ammonium nitrate used in Scotland (Supplementary Table 2). The climate change potential per NDUP−F is higher overall for the Scottish cereal-rapeseed rotation because of direct N2O emissions derived from a large amount of total N applied and because of the upstream emissions from ammonium nitrate production (Moreno-Ruiz et al., 2018).
The Romanian sunflower-cereal rotation (RO [C_O #1]) requires more fertilizer to produce one NDUP−F than any other rotation. This rotation comprises three crops, two of which, wheat and maize, delivered low NDUP−F (Supplementary Table 5). In the first legume-modified Romanian rotation (RO [C_O_L#1]), common beans contributed to a slightly higher NDUP−F and also increased the yield of the following crop (maize). The second legume-modified rotation (RO [C_O_L#2]) introduced soybean, which has one of the highest protein contents of all crops, contributing to a slightly higher rotation level NDUP−F than for the common bean rotation and therefore scoring better across impact categories.
Animal Nutrition Footprints
Cereal Unit
Using the CU as a FU, legume-modified rotations scored better in most regions compared with cereal-cereal and cereal-oilseed rotations. The exception occurred in Italy, where the cereal-oilseed rotation was more environmentally efficient across 9 of the 16 impact categories and where the cereal-cereal rotations incurred the largest environmental burdens (Table 4). Scottish rotations produced the most CU per hectare year (Table 2), delivering 2–3 times more than Italian and Romanian rotations. Legume-modified rotations delivered 17% lower CU scores in Scotland compared with the cereal-oilseed rotation SC [C_O #1]. In Italy, IT [C_O_L] delivered 12% lower than IT [C_O] (Table 2). However, the soybean-modified rotation in Romania (RO [C_O_L #2]) had a 23% higher CU than the cereal-oilseed rotation 1 (RO [C_O #1) as can be seen in Table 2.
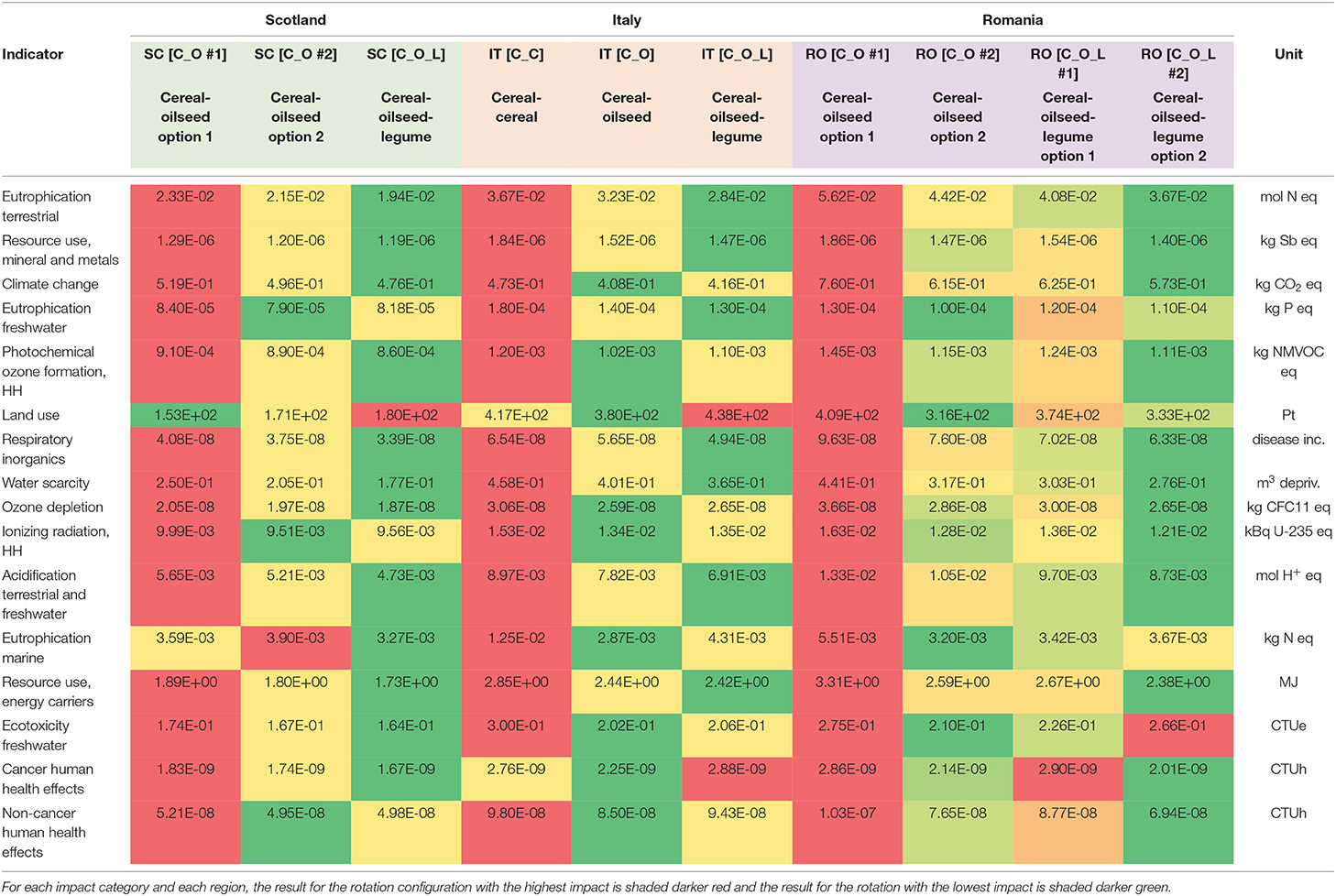
Table 4. Heat map of impact scores across all impact categories for all rotations analyzed in Scotland (SC), Italy (IT), and Romania (RO), expressed per cereal unit.
Scottish rotations incurred smaller environmental impacts per CU than Italian rotations, except for climate change (Table 4). Scottish rotations deliver the most CU ha.yr−1. However, the SNF requirement per CU produced was higher than Italian rotations (Supplementary Table 6). Therefore, the N2O emissions were higher for Scottish than for Italian rotations. In Italy SNF was mainly applied as urea, with high volatilization rates (Supplementary Table 2), leading to high NH3 emissions for Italian rotations and higher burdens for terrestrial and freshwater acidification, respiratory inorganics, and terrestrial eutrophication compared with Scottish rotations.
Romanian rotations delivered slightly more CU ha.yr−1 than Italian rotations (Table 2). The Romanian cereal-oilseed rotation (RO [C_O #2]) delivers more CU than the common bean-modified rotation (RO [C_O_L #1]), and even though the cereal-oilseed rotations needed more SNF than legume-modified rotations, the impact of RO [C_O #2] per CU is lower for climate change (Figure 4). Marine eutrophication potential was mostly linked to nitrate leaching to water (Figure 2). For Romanian rotations, the highest leaching per FU occurred in maize and soybean followed by winter wheat and sunflower crops. The RO [C_O_L #2] included both soybean and maize, scoring higher for marine eutrophication than the cereal-oilseed rotation RO [C_O #2] and the common bean-modified rotation RO [C_O_L #1] (Supplementary Table 5). However, RO [C_O #1] scored higher worst among them for the same impact (marine eutrophication), combining sunflower, wheat, and maize in the rotation. The Italian cereal-cereal (IT [C_C]) rotation also scored high on marine eutrophication due to the high nitrate leaching associated with winter oats.
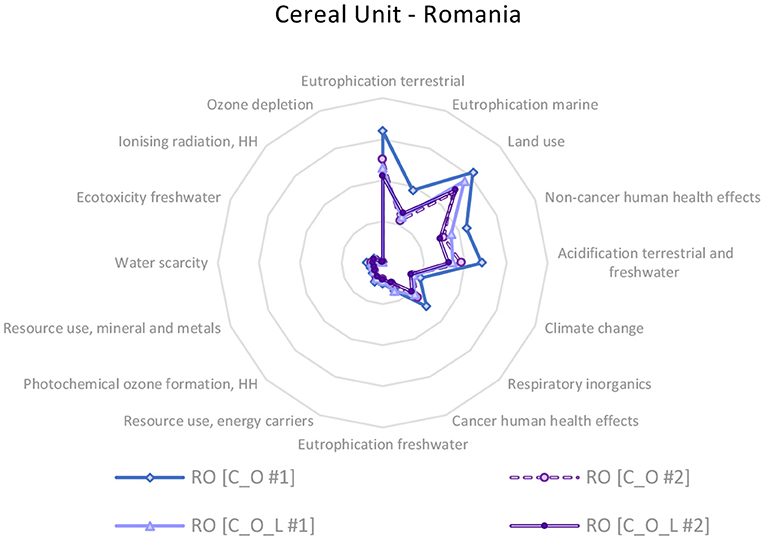
Figure 4. Normalized environmental scores per cereal unit (CU) of animal feed energy output across Romanian crop rotations. RO [C_O #1] refers to cereal-oilseed rotation option 1, RO [C_O #2] refers to cereal-oilseed rotation option 2, RO [C_O_L #1] refers to cereal-oilseed-legume rotation option 1 (with common beans) and [C_O_L #2] refers to cereal-oilseed-legume rotation option 2 (with soybeans).
Digestible Protein
When FUFeed is measured in terms of DP (protein) rather than CU (energy) delivered, introducing legumes into typical rotations appears more beneficial. This can be observed for Romania (Figure 5). Per kg DP, all legume-modified rotations scored lower environmental impacts across the majority of 16 impact categories compared with the cereal-cereal and cereal-oilseed rotations within their regions (Table 5). Despite sometimes having lower yields than other cereal crops, legumes have two main advantages: a high protein content (Supplementary Table 3) and no requirement for SNF. All legume-modified rotations produced more DP per hectare-year of rotation than the other options in their regions (Table 2). Scottish rotations produced the most DP per hectare, followed by Romanian then Italian rotations. In Scotland, the legume-modified rotation (SC [C_O_L]) was only slightly better than the first cereal-oilseed rotation (SC [C_O #1]) in terms of DP production per ha owing to the very high yields of wheat and barley (Supplementary Table 5).
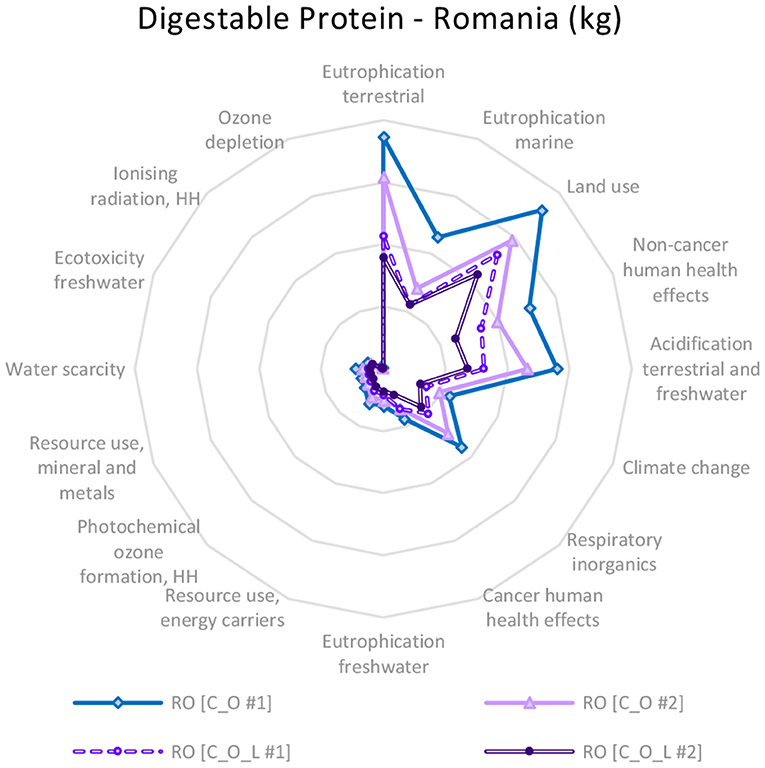
Figure 5. Normalized environmental scores per kg digestible protein (DP) animal feed nutritional output across Romanian crop rotations. RO [C_O #1] refers to cereal-oilseed rotation option 1, RO [C_O #2] refers to cereal-oilseed rotation option 2, RO [C_O_L #1] refers to cereal-oilseed-legume rotation option 1 (with common beans) and [C_O_L #2] refers to cereal-oilseed-legume rotation option 2 (with soybeans).
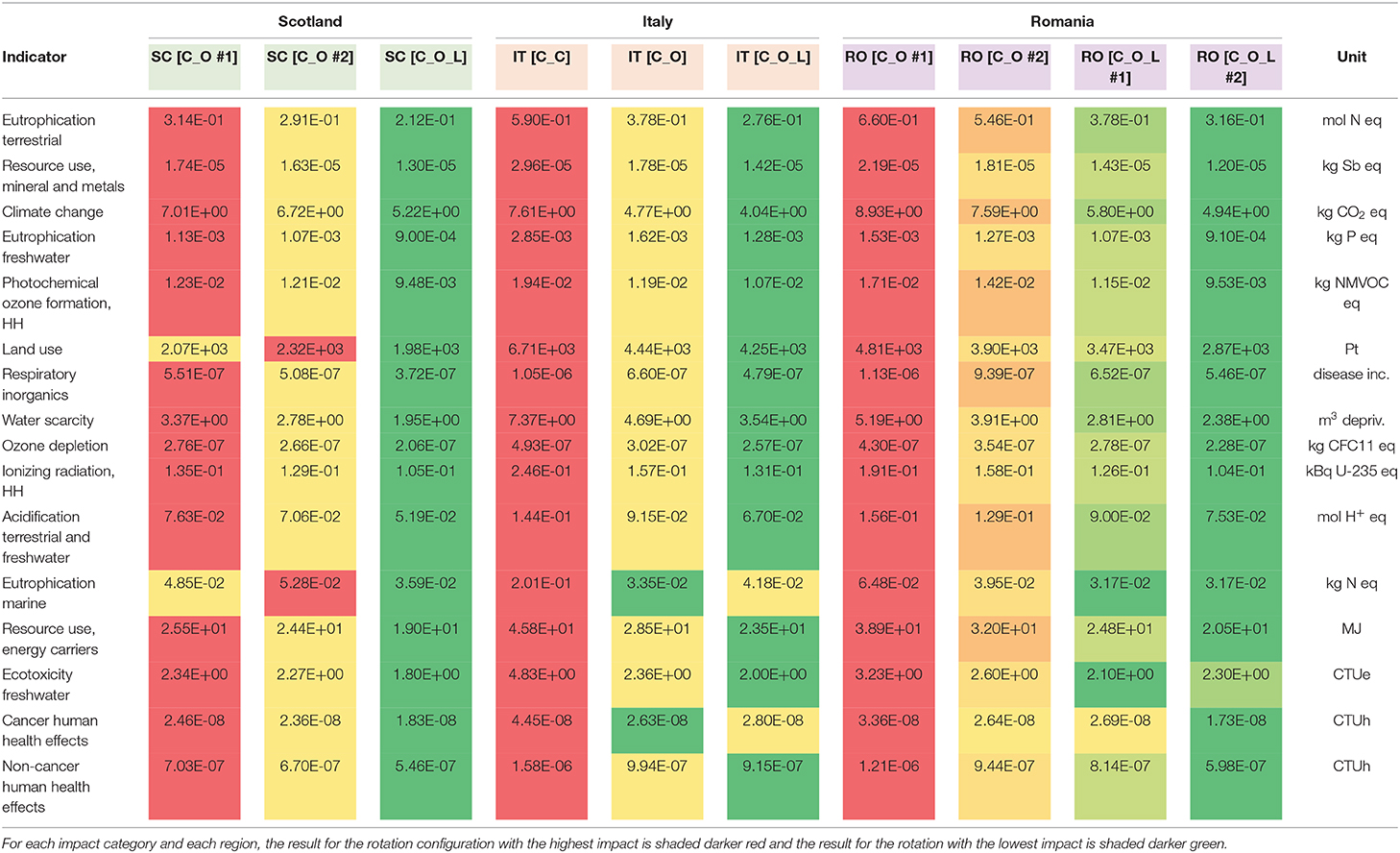
Table 5. Heat map of impact scores across all impact categories for all rotations analyzed in Scotland (SC), Italy (IT), and Romania (RO), expressed per DP.
The SNF required to produce 1 kg of DP was considerably higher for cereal-cereal and cereal-oilseed rotations than for legume-modified rotations across all regions (Supplementary Table 7). Consequently, cereal-cereal and cereal-oilseed rotations incurred larger burdens per kg DP for terrestrial and freshwater acidification, respiratory inorganics, and terrestrial eutrophication. Marine eutrophication burden was greatest overall for the Italian cereal-cereal rotation (IT [C_C]) because of the high nitrate leaching from the oat crop. Additionally, marine eutrophication burdens were greater for legume-modified rotations in Italy than cereal-oilseed (IT [C_O_L]) rotations because of leaching from fava bean residues (Reckling et al., 2016b).
Sensitivity Analysis
Values for SNF application, yields (DM), Nitrogen Use Efficiency (NUE), leaching, and emission factors before and after the sensitivity analysis can be observed in Supplementary Table 9, for each crop under each of the ten rotations across all regions studied. The results of the sensitivity analysis for NDUP−F (Supplementary Table 10) did not show significant changes to the major conclusions on the comparative environmental efficiency of legume and non-legume rotations across different regions in Europe. However, the simulation of the use of nitrification inhibitors resulted in a reduction in climate change impacts from entire rotations of 20% on average for non-legume rotations and 18% on average for legume-modified rotations, as can be observed in Supplementary Figure 2.
Discussion
Assessing Sustainable Human Nutrition
The NDUP−F FU applied here provides a unique perspective on the comparative efficiency of legume-modified rotations to deliver key components of human nutrition (protein, fiber, and energy) – factors rarely considered in farm- or rotation-level LCA studies. MacWilliam et al. (2014) and Li et al. (2018) evaluated rotations in terms of protein and essential nutrient outputs, but did not apply the NDU considered here nor evaluate the full PEF suite of impact categories. According to McAuliffe et al. (2020), nutritional footprint studies are not yet taken to their full potential, and commodity-level LCA results are sometimes confused with diet-level results. Critical details about farm management and rotations often get overlooked in diet-level LCA, compromising results, and limiting their value in informing food system transitions that necessitate changes in practices at the farm level – e.g., changes to cropping sequences. Results in this study show that choice of FU can change the comparative performance of rotations for some impact categories, and that nutritional FUs have an important role to play in farm level LCA – bridging the gap between state-of-the-art studies in food LCA and crop rotation LCA (Costa et al., 2020) to provide a more robust evidence base for integrated solutions to food chain sustainability. However, NDUP−F remains a relatively crude proxy for human nutrition because (i) the nutritional content of grains changes according to farm practices, choice of varieties, and fertilization management (AHDB, 2019), (ii) grain processing and preparation influences the final nutritional value (Saget et al., 2020), and (iii) the NDU focus only on few elements of human nutrition – protein, fiber, and energy. More refined estimates of human nutrition consider different aspects, such protein quality via, for example, the amino acid profile (Leinonen et al., 2019), or other bioavailable micronutrients (which may be enhanced by cultivation and biofortification strategies). Biofortification can be achieved through different methods, such as conventional plant breeding, genetic engineering, agronomics tactics, and more recently plant growth-promoting bacteria (PGPB) strategies (Roriz et al., 2020). The latter, for instance, improves crop yields and also iron availability for human diets (an aspect not assessed in this study).
Here, pig digestibility values were used as a proxy for human digestibility, owing to a lack of alternative, universally applicable data. Another limitation of the NDUP−F is the residual need for some allocation or system expansion because not all outputs are used for human food. Here, economic allocation was used to partition the main grain products and straw. Despite these limitations, we propose the application of a nutritional FU to assess the environmental efficiency of rotations whose outputs are primarily destined for human food, in order to generate more coherent evidence for sustainable food system transitions. Our results also highlight the importance of looking at impact categories other than climate change, such as respiratory inorganics, marine eutrophication, and terrestrial and freshwater acidification (European Commission, 2017) to fully reflect impacts from e.g., fertilizer use and to provide a fuller picture of environmental sustainability.
Assessing Sustainable Animal Nutrition
From an animal feed perspective, energy intake is often adopted as a FU because it represents the primary component of ruminant diets (Huws et al., 2018; AHDB, 2020). Nevertheless, digestible protein is a critical aspect of animal nutrition, not least because Europe currently imports soybean from other countries where its production may drive deforestation (Watson et al., 2017). To reflect current limitations of LCA methods for rotational systems, the adoption of more than one FU has been recommended (Nemecek et al., 2011; Brankatschk, 2018; Goglio et al., 2018). Results here show that the choice of energy or protein as the primary functional unit leads to different conclusions on the environmental efficiency of different crop rotations. Integration of multiple components of nutrition into a single NDU as for human nutrition is complicated in the case of livestock owing to different requirements and consequences across species. For example, lipid contents are important and can reduce methane emissions from cattle (Newbold et al., 2004; Belanche et al., 2012). Further research is needed to develop a more integrated FU for animal nutrition, analogous to the NDU for human nutrition. In the meantime, applying multiple functional units provides a useful sensitivity analysis and may avoid the inference of false precision that can arise when using a single metric oriented toward a particular aspect of nutrition. This study did not evaluate agroforestry systems, livestock grazing on temporary leys nor non-food-or-feed uses of crops (bioenergy, textiles, cosmetics, etc.). Functional units may need to broadened out further to consider more complex integrated systems.
Implications for European Cropping Systems
Results from this study highlight that legume-modified rotations generally deliver nutrition to humans and livestock more environmentally sustainably than typical cereal rotations across different European regions. The main benefits of legume incorporation were reduced SNF requirements, enhanced yields in following crops, and improved nutritional profile of outputs. Legume-modified rotations also exhibit a greater degree of autarchy (reduced need for external inputs) – an important characteristic of food system sustainability (Pretty, 2008). Whilst previous studies indicated that legumes could increase N leaching (Nemecek et al., 2008), this was not a significant trade-off in our study when considered across the higher nutritional output of legume-modified rotations. For example, in some rotations, winter cereals cultivated after legumes “mopped up” much of the N in legume residues, reducing fertilizer requirements (Reckling et al., 2016a). Furthermore, technical options to improve the efficiency of synthetic fertilizer use cannot match the environmental advantage conferred by incorporation of legumes into rotations, and previous studies have shown the feasibility of replacing imported soy-based feeds with local legumes (Hörtenhuber et al., 2011; Smith et al., 2013; White et al., 2015). Thus, legumes could play a crucial role in improving the sustainability of cropping systems at farm level. However, high availability of inexpensive external resources (e.g., synthetic fertilizers and imported protein-rich feeds, alongside marginally competitive annual gross margins (excluding multi-annual rotation effects) for legumes (Preissel et al., 2015; Zander et al., 2016) deter widespread farmer uptake. There is also a lack of incentive through public policies which tend to favor alternative crops for bioenergy and biodiesel production (Zander et al., 2016,Watson et al., 2017).
Nonetheless, legumes also have an important role to play in the more radical food system change required to avoid critical exceedance of planetary boundaries (Springmann et al., 2018; Willett et al., 2019; Lynch et al., 2020). Such dietary change may involve legume substitution of not just cereal and oilseed crops within rotations, but the livestock that feed off those crops (Tilman and Clark, 2014; Goldstein et al., 2017; Chaudhary et al., 2018a; Willett et al., 2019; Saget et al., 2020). Indeed, this study highlights the value of legumes in delivering protein and fiber for human nutrition directly from cropping systems. Proper accounting for the nutritional outputs of cropping systems could strengthen the evidence base for a demand (diet) driven shift in food system configuration to improve overall sustainability.
Conclusions
It is increasingly recognized that evaluation of food system sustainability should account for interactions among crops in rotation cycles, not just the inputs and outputs of single crops cultivated within such systems. In this study, we applied three functional units to aggregate multiple crop outputs and compare the environmental efficiency of ten crop rotations in terms of delivery of human and livestock nutrition. Across three European climatic zones, the introduction of legumes into conventional cereal and oilseed rotations increased protein production and overall nutritional output whilst reducing synthetic fertilizer inputs. Thus, for most of the 16 impact categories studied, legume-modified rotations delivered animal, and especially human, nutrition at a lower environmental cost than conventional rotations. Our results show that choice of functional unit has an important influence on the apparent efficiency of different crop rotations. Application of a nutrient density unit representing energy, protein and fiber highlighted the value of introducing legumes into rotations for the purpose of direct human nutrition. This study also points to the need to develop functional units capable of representing multiple (species specific) nutritional attributes of livestock feed. In the meantime, applying multiple functional units (e.g., based on metabolisable energy and digestible protein) can provide a more balanced picture of crop system efficiency with respect to animal nutrition. Evaluating entire crop rotations using nutritional indices as functional units highlights the important role for more legume cultivation in Europe to improve the sustainability of cropping systems. Legumes have high potential to underpin the transition to healthy and sustainable diets targeted by, inter alia, the European Green New Deal Farm to Fork strategy.
Data Availability Statement
The original contributions presented in the study are included in the article/Supplementary Material, further inquiries can be directed to the corresponding author/s.
Author Contributions
MC: conceptualization, methodology, LCA analysis and modeling, and wrote-original draft. MR: rotations data modeling and reviewing. DC: data and support for sensitivity analysis, reviewing and editing, supervision and advice. RR: reviewing and editing, supervision and advice. SS: reviewing and editing. MW: reviewing. DS: conceptualization, wrote the manuscript, reviewing and editing, supervision, and advice. All authors contributed to the article and approved the submitted version.
Funding
This research was supported by the TRUE project, funded by the EU Framework Programme for Research and Innovation H2020, Grant Agreement number 727973. MR was funded by the Deutsche Forschungsgemeinschaft (DFG, German Research Foundation) – 420661662 and supported by the EU H2020 project Legumes Translated, Grant Agreement number 817634.
Conflict of Interest
The authors declare that the research was conducted in the absence of any commercial or financial relationships that could be construed as a potential conflict of interest.
Acknowledgments
Authors are grateful for the constructive comments of peer reviewers.
Supplementary Material
The Supplementary Material for this article can be found online at: https://www.frontiersin.org/articles/10.3389/fsufs.2021.656005/full#supplementary-material
References
Abalos, D., Jeffery, S., Sanz-Cobena, A., Guardia, G., and Vallejo, A. (2014). Meta-analysis of the effect of urease and nitrification inhibitors on crop productivity and nitrogen use efficiency. Agric. Ecosyst. Environ. 189, 136–144. doi: 10.1016/j.agee.2014.03.036
AHDB (2020). A Guide to Assessing Daily Cow Feed Requirements, Formulating Diets and Monitoring Performance. Chapter 7: Managing Your Feeding [WWW Document]. Available online at: https://ahdb.org.uk/knowledge-library/feeding-managing-your-feeding (accessed August, 2020).
Baddeley, J. A., Pappa, V. A., Pristeri, A., Bergkvist, G., Monti, M., Reckling, M., et al. (2017). “Legume-based green manure crops,” in Legumes in Cropping Systems, eds D. Murphy-Bokern, F. L. Stoddard, and C. A. Watson (Wallingford, CT: CABI International), 125–138. doi: 10.1079/9781780644981.0125
Belanche, A., Doreau, M., Edwards, J. E., Moorby, J. M., Pinloche, E., and Newbold, C. J. (2012). Shifts in the rumen microbiota due to the type of carbohydrate and level of protein ingested by dairy cattle are associated with changes in rumen fermentation. J. Nutr. 142, 1684–1692. doi: 10.3945/jn.112.159574
Brankatschk, G. (2018). Modeling Crop Rotations and Co-products in Agricultural Life Cycle Assessments. Wiesbaden: Springer. doi: 10.1007/978-3-658-23588-8
Brankatschk, G., and Finkbeiner, M. (2014). Application of the cereal unit in a new allocation procedure for agricultural life cycle assessments. J. Clean. Prod. 73, 72–79. doi: 10.1016/j.jclepro.2014.02.005
Brankatschk, G., and Finkbeiner, M. (2015). Modeling crop rotation in agricultural LCAs - challenges and potential solutions. Agric. Syst. 138, 66–76. doi: 10.1016/j.agsy.2015.05.008
Chaudhary, A., Gustafson, D., and Mathys, A. (2018a). Multi-indicator sustainability assessment of global food systems. Nat. Commun. 9, 1–13. doi: 10.1038/s41467-018-03308-7
Chaudhary, A., Marinangeli, C. P. F., Tremorin, D., and Mathys, A. (2018b). Nutritional combined greenhouse gas life cycle analysis for incorporating canadian yellow pea into cereal-based food products. Nutrients 10:490. doi: 10.3390/nu10040490
Costa, M. P., Chadwick, D., Saget, S., Rees, R. M., Williams, M., and Styles, D. (2020). Representing crop rotations in life cycle assessment: a review of legume LCA studies. Int. J. Life Cycle Assess. 25, 1942–1956. doi: 10.1007/s11367-020-01812-x
European Commission (2017). PEFCR Guidance Document - Guidance for the Development of Product Environmental Footprint Category Rules (PEFCRs), Version 6.3. European Commission.
European Environmental Bureau (2018). The EU Product Environmental Footprint (PEF) Methodology What Can it Deliver and What Not? An NGO Viewpoint, 1–10. European Environmental Bureau.
Gilsanz, C., Báez, D., Misselbrook, T. H., Dhanoa, M. S., and Cárdenas, L. M. (2016). Development of emission factors and efficiency of two nitrification inhibitors, DCD and DMPP. Agric. Ecosyst. Environ. 216, 1–8. doi: 10.1016/j.agee.2015.09.030
Goglio, P., Brankatschk, G., Knudsen, M. T., Williams, A. G., and Nemecek, T. (2018). Addressing crop interactions within cropping systems in LCA. Int. J. Life Cycle Assess. 23, 1735–1743. doi: 10.1007/s11367-017-1393-9
Goldstein, B., Moses, R., Sammons, N., and Birkved, M. (2017). Potential to curb the environmental burdens of American beef consumption using a novel plant-based beef substitute. PLoS ONE 12:e0189029. doi: 10.1371/journal.pone.0189029
GreenDelta (2006). OpenLCA, Professional Life Cycle Assessment (LCA) and Footprint Software [WWW Document]. GreenDelta.
Heuzé, V., Tran, G., and Kaushik, S. (2017). Feedpedia, A Programme by INRA, CIRAD, AFZ and FAO (2017) [WWW Document]. Available online at: https://feedipedia.org/
Hörtenhuber, S. J., Lindenthal, T., and Zollitsch, W. (2011). Reduction of greenhouse gas emissions from feed supply chains by utilizing regionally produced protein sources: the case of Austrian dairy production. J. Sci. Food Agric. 91, 1118–1127. doi: 10.1002/jsfa.4293
Hufnagel, J., Reckling, M., and Ewert, F. (2020). Diverse approaches to crop diversification in agricultural research. A review. Agron. Sustain. Dev. 40:14. doi: 10.1007/s13593-020-00617-4
Huws, S. A., Creevey, C. J., Oyama, L. B., Mizrahi, I., Denman, S. E., Popova, M., et al. (2018). Addressing global ruminant agricultural challenges through understanding the rumen microbiome: past, present, and future. Front. Microbiol. 9:2161. doi: 10.3389/fmicb.2018.02161
Jensen, E. S., Peoples, M. B., Boddey, R. M., Gresshoff, P. M., Hauggaard-Nielsen, H., Alves, B. J., et al. (2012). Legumes for mitigation of climate change and the provision of feedstock for biofuels and biorefineries. A review. Agron. Sustain. Dev. 32, 329–364. doi: 10.1007/s13593-011-0056-7
Karlsson, H., Ahlgren, S., Strid, I., and Hansson, P. A. (2015). Faba beans for biorefinery feedstock or feed? Greenhouse gas and energy balances of different applications. Agric. Syst. 141, 138–148. doi: 10.1016/j.agsy.2015.10.004
Leinonen, I., Iannetta, P. P. M., Rees, R. M., Russell, W., Watson, C., and Barnes, A. P. (2019). Lysine supply is a critical factor in achieving sustainable global protein economy. Front. Sustain. Food Syst. 3:27. doi: 10.3389/fsufs.2019.00027
Li, L., Wu, W., Giller, P., O'Halloran, J., Liang, L., Peng, P., et al. (2018). Life cycle assessment of a highly diverse vegetable multi-cropping system in Fengqiu County, China. Sustain. 10, 1–17. doi: 10.3390/su10040983
Li, T., Zhang, W., Yin, J., Chadwick, D., Norse, D., Lu, Y., et al. (2017). Enhanced-efficiency fertilizers are not a panacea for resolving the nitrogen problem. Glob. Chang. Biol. 24, e511–e521. doi: 10.1111/gcb.13918
Lienhardt, T., Black, K. S., Saget, S., Costa, M. P., Chadwick, D., et al. (2019). Just the tonic! Legume biorefining for alcohol has the potential to reduce Europe's protein deficit and mitigate climate change. Environ. Int. 130:104870. doi: 10.1016/j.envint.2019.05.064
Lynch, J., Domingo, N., Colgan, K., Thakrar, S., Hill, J., Tilman, D., et al. (2020). Global Food System Emissions Could Preclude Achieving the 1.5° and 2°C Climate Change Targets - ORA - Oxford University Research Archive [WWW Document]. Available online at: https://ora.ox.ac.uk/objects/uuid:7a43bb76-594d-49fc-9005-5546f9f1d9d8 (accessed November 14, 2020).
MacWilliam, S., Wismer, M., and Kulshreshtha, S. (2014). Life cycle and economic assessment of Western Canadian pulse systems: the inclusion of pulses in crop rotations. Agric. Syst. 123, 43–53. doi: 10.1016/j.agsy.2013.08.009
McAuliffe, G. A., Takahashi, T., and Lee, M. R. F. (2020). Applications of nutritional functional units in commodity-level life cycle assessment (LCA) of agri-food systems. Int. J. Life Cycle Assess. 25, 208–221. doi: 10.1007/s11367-019-01679-7
Moreno-Ruiz, E., Valsasina, L., Brunner, F., Symeonidis, A., FitzGerald, D., Treyer, K., et al. (2018). Documentation of Changes Implemented in Ecoinvent Data 3.5. Zürich: Ecoinvent.
Nemecek, T., Huguenin-Elie, O., Dubois, D., Gaillard, G., Schaller, B., and Chervet, A. (2011). Life cycle assessment of Swiss farming systems: II. Extensive and intensive production. Agric. Syst. 104, 233–245. doi: 10.1016/j.agsy.2010.07.007
Nemecek, T., von Richthofen, J. S., Dubois, G., Casta, P., Charles, R., and Pahl, H. (2008). Environmental impacts of introducing grain legumes into European crop rotations. Eur. J. Agron. 28, 380–393. doi: 10.1016/j.eja.2007.11.004
Newbold, C. J., McIntosh, F. M., Williams, P., Losa, R., and Wallace, R. J. (2004). Effects of a specific blend of essential oil compounds on rumen fermentation. Anim. Feed Sci. Technol. 114, 105–112. doi: 10.1016/j.anifeedsci.2003.12.006
Notarnicola, B., Tassielli, G., Renzulli, P. A., Castellani, V., and Sala, S. (2017). Environmental impacts of food consumption in Europe. J. Clean. Prod. 140, 753–765. doi: 10.1016/j.jclepro.2016.06.080
Peoples, M. B., Hauggaard-Nielsen, H., Huguenin-Elie, O., Jensen, E. S., Justes, E., and Williams, M. (2019). “The contributions of legumes to reducing the environmental risk of agricultural production,” in Agroecosystem Diversity, eds G. Lemaire, P. C. De Faccio Carvalho, S. Kronberg, and S. Recous, (Academic Press), 123–143. doi: 10.1016/B978-0-12-811050-8.00008-X
Peter, C., Specka, X., Aurbacher, J., Kornatz, P., Herrmann, C., Heiermann, M., et al. (2017). The MiLA tool: modeling greenhouse gas emissions and cumulative energy demand of energy crop cultivation in rotation. Agric. Syst. 152, 67–79. doi: 10.1016/j.agsy.2016.12.008
Plaza-Bonilla, D., Nogué-Serra, I., Raffaillac, D., Cantero-Martínez, C., and Justes, É. (2018). Carbon footprint of cropping systems with grain legumes and cover crops: a case-study in SW France. Agric. Syst. 167, 92–102. doi: 10.1016/j.agsy.2018.09.004
Preissel, S., Reckling, M., Schläfke, N., and Zander, P. (2015). Magnitude and farm-economic value of grain legume pre-crop benefits in Europe: a review. F. Crop. Res. 175, 64–79. doi: 10.1016/j.fcr.2015.01.012
Pretty, J. (2008). Agricultural sustainability: concepts, principles and evidence. Philos. Trans. R. Soc. B Biol. Sci. 363, 447–465. doi: 10.1098/rstb.2007.2163
Reckling, M., Bergkvist, G., Watson, C. A., Stoddard, F. L., Zander, P. M., Walker, R. L., et al. (2016a). Trade-offs between economic and environmental impacts of introducing legumes into cropping systems. Front. Plant Sci. 7:669. doi: 10.3389/fpls.2016.00669
Reckling, M., Hecker, J. M., Bergkvist, G., Watson, C. A., Zander, P., Schläfke, N., et al. (2016b). A cropping system assessment framework—evaluating effects of introducing legumes into crop rotations. Eur. J. Agron. 76, 186–197. doi: 10.1016/j.eja.2015.11.005
Rochette, P., and Janzen, H. H. (2005). Towards a revised coefficient for estimating N2O emissions from legumes. Nutr. Cycl. Agroecosyst. 73, 171–179. doi: 10.1007/s10705-005-0357-9
Rockström, J., Edenhofer, O., Gaertner, J., and DeClerck, F. (2020). Planet-proofing the global food system. Nat. Food 1, 3–5. doi: 10.1038/s43016-019-0010-4
Roriz, M., Carvalho, S. M. P., Castro, P. M. L., and Vasconcelos, M. W. (2020). Legume biofortification and the role of plant growth-promoting bacteria in a sustainable agricultural era. J. Agronomy. 10:435. doi: 10.3390/agronomy10030435
Saget, S., Costa, M., Barilli, E., Wilton de Vasconcelos, M., Santos, C. S., Styles, D., et al. (2020). Substituting wheat with chickpea flour in pasta production delivers more nutrition at a lower environmental cost. Sustain. Prod. Consum. 24, 26–38. doi: 10.1016/j.spc.2020.06.012
Saget, S., Costa, M., Santos, C. S., Vasconcelos, M. W., Gibbons, J., Styles, D., et al. (2021). Substitution of beef with pea protein reduces the environmental footprint of meat balls whilst supporting health and climate stabilisation goals. J. Clean. Prod. 297:126447. doi: 10.1016/j.jclepro.2021.126447
Smith, L. A., Houdijk, J. G. M., Homer, D., and Kyriazakis, I. (2013). Effects of dietary inclusion of pea and faba bean as a replacement for soybean meal on grower and finisher pig performance and carcass quality. J. Anim. Sci. 91, 3733–3741. doi: 10.2527/jas.2012-6157
Sonesson, U., Davis, J., Flysjö, A., Gustavsson, J., and Witthöft, C. (2017). Protein quality as functional unit – a methodological framework for inclusion in life cycle assessment of food. J. Clean. Prod. 140, 470–478. doi: 10.1016/j.jclepro.2016.06.115
Springmann, M., Clark, M., Mason-D'Croz, D., Wiebe, K., Bodirsky, B. L., Lassaletta, L., et al. (2018). Options for keeping the food system within environmental limits. Nature 562, 519–525. doi: 10.1038/s41586-018-0594-0
Styles, D., Gibbons, J., Williams, A. P., Dauber, J., Stichnothe, H., Urban, B., et al. (2015). Consequential life cycle assessment of biogas, biofuel and biomass energy options within an arable crop rotation. GCB Bioenergy 7, 1305–1320. doi: 10.1111/gcbb.12246
Thapa, R., Chatterjee, A., Awale, R., McGranahan, D. A., and Daigh, A. (2016). Effect of enhanced efficiency fertilizers on nitrous oxide emissions and crop yields: a meta-analysis. Soil Sci. Soc. Am. J. 80, 1121–1134. doi: 10.2136/sssaj2016.06.0179
Tilman, D., and Clark, M. (2014). Global diets link environmental sustainability and human health. Nature 515, 518–522. doi: 10.1038/nature13959
van Dooren, C. (2016). “Proposing the nutrient density unit as the functional unit in LCAs of foods,” in International Conference on Life Cycle Assessment of Food (Dublin).
van Dooren, C. (2017). “Proposing the nutrient density unit as the functional unit in LCAs of foods proposing the nutrient density unit as the functional unit in LCAs of foods,” in International Conference on Life Cycle Assessment of Food 2016 (Dublin), 1–10.
Watson, C. A., Reckling, M., Preissel, S., Bachinger, J., Bergkvist, G., Kuhlman, T., et al. (2017). Grain legume production and use in european agricultural systems. Adv. Agron. 144, 235–303. doi: 10.1016/bs.agron.2017.03.003
White, G. A., Smith, L. A., Houdijk, J. G. M., Homer, D., Kyriazakis, I., and Wiseman, J. (2015). Replacement of soya bean meal with peas and faba beans in growing/finishing pig diets: effect on performance, carcass composition and nutrient excretion. Anim. Feed Sci. Technol. 209, 202–210. doi: 10.1016/j.anifeedsci.2015.08.005
Willett, W., Rockström, J., Loken, B., Springmann, M., Lang, T., Vermeulen, S., et al. (2019). Food in the anthropocene: the EAT–lancet commission on healthy diets from sustainable food systems. Lancet. 393:447–492. doi: 10.1016/S0140-6736(18)31788-4
Keywords: legumes, nutritional functional unit, rotation systems, animal feed, human food chain
Citation: Costa MP, Reckling M, Chadwick D, Rees RM, Saget S, Williams M and Styles D (2021) Legume-Modified Rotations Deliver Nutrition With Lower Environmental Impact. Front. Sustain. Food Syst. 5:656005. doi: 10.3389/fsufs.2021.656005
Received: 19 January 2021; Accepted: 18 March 2021;
Published: 13 April 2021.
Edited by:
Rakesh Bhardwaj, National Bureau of Plant Genetic Resources (ICAR), IndiaReviewed by:
Narendra Kumar, Indian Institute of Pulses Research (ICAR), IndiaUday Chand Jha, Indian Institute of Pulses Research (ICAR), India
Copyright © 2021 Costa, Reckling, Chadwick, Rees, Saget, Williams and Styles. This is an open-access article distributed under the terms of the Creative Commons Attribution License (CC BY). The use, distribution or reproduction in other forums is permitted, provided the original author(s) and the copyright owner(s) are credited and that the original publication in this journal is cited, in accordance with accepted academic practice. No use, distribution or reproduction is permitted which does not comply with these terms.
*Correspondence: Marcela P. Costa, Y21hcmNlbGFwb3J0b0BnbWFpbC5jb20=; David Styles, RGF2aWQuU3R5bGVzQHVsLmll