Biodisinfestation With Agricultural By-Products Developed Long-Term Suppressive Soils Against Meloidogyne incognita in Lettuce Crop
- 1Department of Plant Production and Protection, NEIKER, Basque Institute for Agricultural Research and Development, Derio, Spain
- 2Consejeria Agr Junta Comunidades Castilla-La Mancha, Inst Reg Invest Desarrollo Agroalimentario & Forest, Ctr Invest Apicola y Agroambiental IRIAF, CIAPA, Lab Suelos, Marchamalo, Spain
Soil biodisinfestation is the process generated after the incorporation of organic amendments followed by a plastic cover to control soilborne diseases. Among organic amendments, the use of agricultural by-products could be an interesting alternative as it promotes circular economy. In this study, beer bagasse and defatted rapeseed cake together with fresh cow manure were incorporated into the soil (1.5, 0.5, and 20 kg/m2, fresh weight, respectively) to assess their capacity to reduce disease incidence caused by the root-knot nematode Meloidogyne incognita in protected lettuce crops and develop suppressive soils. The trial was conducted in a commercial greenhouse for 7 weeks during which temperature was continuously recorded at three different soil depths (15, 30, and 45 cm). Short- and long-term effects were assessed: before treatment, after treatment, after first crop post-treatment and one year post-treatment. Disease incidence and changes in nematode community structure were analyzed along with microbiological properties and general physicochemical parameters. After biodisinfestation, microbiological activity significantly increased in the treated soils and changes in the nematode community structure were detected in detriment of M. incognita and other plant-parasitic nematodes. These effects were more apparent after the first crop post-treatment than right after biodisinfestation. In the first crop after biodisinfestation, lettuce yield increased in the treated plots and root galling indices were significantly lower. One year after treatment, differences between treatments could be observed in the incidence of the damage caused by M. incognita that remained lower in the treated plots. In this trial, the addition of beer bagasse and rapeseed cake along with fresh manure in biodisinfestation treatment demonstrated nematicidal effects against M. incognita. Moreover, we suggest that the compounds released during the degradation of these by-products and the sub-lethal temperatures achieved in this trial during biodisinfestation (<42°C) were the key to develop suppressive soils in the long-term.
Introduction
Conventional crop management includes pathogen control and the use of chemical fumigants is the most common practice, but many of them are harmful and cause environmental damages. After the Montreal Protocol (1989), methyl bromide and other fumigants were banned or subjected to restriction in use (Gareau, 2010). The negative attributes of soil chemical fumigants are of increasing concern, and therefore more attention is given to non-chemical approaches for soilborne pest control (Klein et al., 2012; Mocali et al., 2015). Among them, soil biodisinfestation, which includes the use of organic amendments, is widespread in pest management as an effective and healthy alternative for soil treatments (Rosskopf et al., 2020). Soil biodisinfestation is a general term for the different approaches developed to control soilborne diseases using organic matter as main driver, but different terms can be found to define each approach. Biosolarization is based on the incorporation of organic amendment followed by mulching with transparent polyethylene plastic film during hot seasons in order to achieve high soil temperatures. These conditions promote certain physical, microbiological and biochemical processes that contribute to disease reduction and even a decrease in soil pathogen populations (Katan, 2014; Katan and Gamliel, 2014). In climate regions with low solar radiation, labile C-sources are used to boost anaerobic conditions and this strategy is commonly called anaerobic soil disinfestation (ASD) (Shennan et al., 2018; Rosskopf et al., 2020). Another practice is the incorporation of plants of the Brassicaceae family, which is known as biofumigation (Kirkegaard et al., 1993). This approach is based on the release of isothiocyanates (ITCs) produced by the hydrolysis of the glucosinolates (GLs) in Brassicaceous amendments (Matthiessen and Kirkegaard, 2006). These ITCs along with other biocidal compounds produced during decomposition of plant tissue, like volatile fatty acids (VFA) and NH3, have demonstrated biocidal activity (Oka et al., 2007; Dutta et al., 2019).
These biodisinfestation practices have shown positive results against different soilborne pathogens (Ntalli et al., 2020; Rosskopf et al., 2020), plant-parasitic nematodes (PPN) among them, which are important pests of a wide range of crops (Avato et al., 2013; Fourie et al., 2016; Dutta et al., 2019; Talavera et al., 2019). PPNs are very diverse and can cause different damage to host plants. Regarding yield loss, the most common are root-knot (Meloidogyne spp.), cyst (Heterodera spp. and Globodera spp.) and root lesion (Pratylenchus spp.) nematodes (Jones et al., 2013). Root-knot nematodes (RKN) of the genus Meloidogyne are the major plant pathogen with a wide geographical and climatic distribution affecting growth and yield of different agricultural crops (Sasser, 1980; Ijoyah and Koutatouka, 2009; Klein et al., 2012; Jones et al., 2013). Mature females induce gall formation on the root tissue when laying their eggs. This root galling is the most important symptom for RKN affecting root system growth and nutrient uptake capacity that leads to important yield loss (Jones et al., 2013). In particular, Meloidogyne incognita (Kofoid and White, 1919; Chitwood, 1949) is recognized as one of the most destructive species with wide host ranges (Avato et al., 2013; Jones et al., 2013). Despite the scarce related literature, lettuce (Lactuca sativa) is one of the host species with important yield losses recorded in different regions (Koenning et al., 1999; Correia et al., 2019).
Soil nematodes are the most abundant of the Metazoa, most are free-living species with different food sources, and occupy key positions in soil food web (Bongers, 1990; Bongers and Ferris, 1999; Ferris et al., 2001). Thus, the interpretation of the nematode community structure as a whole could provide relevant information to assess disturbances and stress, and could be useful for monitoring changes in the structure and functioning of the soil food web (Bongers and Ferris, 1999; Cesarz et al., 2015). Considering that, several authors developed different indices based on the presence and abundance of the different nematode families (Bongers, 1990; Yeates et al., 1993; Bongers and Bongers, 1998; Bongers and Ferris, 1999; Ferris et al., 2001; Ferris, 2010). However, it is important to consider that intensive agricultural soils are subjected to disturbance and stress conditions due to crop management practices (e.g., tillage, fertilization, and fumigation) in order to choose the proper indices. Despite the scarce information about the effect of biodisinfestation treatments on nematode assemblage, in this trial, together with M. incognita, the effects on presence of the different guilds was assessed. Moreover, analysis of soil nematode community structure could also contribute to the assessment of soil suppressiveness along with other soil parameters (Carrascosa et al., 2014).
The incorporation of organic amendments into the soil in combination with any of the mentioned practices can develop suppressive soils although there is great variability in results of previous studies (Janvier et al., 2007; Bonanomi et al., 2010). Soil suppressiveness consists in disease control, in spite of the presence of soilborne pathogens, which is attributed to an increase of antagonistic microorganisms (Baker and Cook, 1974). The incorporation of organic matter into the soil boosts microbiological activity due to degradation processes, which are enhance by the conditions generated during biodisinfestation treatments (e.g., high temperatures, moisture). This shift in microbiological community can promote antagonistic relationships in detriment of soilborne pathogens (Alabouvette et al., 1996; Janvier et al., 2007). In this trial, beer bagasse was selected to enhance microbial degradation and anaerobic conditions with the consequent release of VFAs. This is the residue obtained in beer production and becomes a source of labile carbon after the fermentation processes. Besides, it has little commercial interest and few studies have been done for agricultural use or other fields. Considering previous studies, the incorporation of crop residues and animal manures and Brassicacea-based management strategies are most common treatments used against PPN (Brennan et al., 2020; Rosskopf et al., 2020). Rapeseed (Brassica napus “Canola”) cake is the by-product obtained after the oil extraction and is often marketed in livestock feed due to its high protein content and low glucosinolates concentration (<15 μmol/g). Unlike other Brassica spp., the main nematicidal effect of Brassica napus “Canola” is attributed to the high nitrogen content which promotes microbial activity and the release of other nematicidal compounds (i.e., NH3, VFAs) (Mazzola et al., 2001; Dutta et al., 2019).
In this assay, beer bagasse and rapeseed cake together with fresh cow manure were incorporated into the soil as organic amendment in biodisinfestation treatment against RKN M. incognita in a commercial lettuce greenhouse with significant yield losses. The main objectives were (i) to assess the potential ability of these by-products to reduce the damage caused by M. incognita in lettuce crop and (ii) to evaluate suppressive effects, as well as (iii) the impact of biodisinfestation on the soil nematode community in both short- and long-term (before treatment, after treatment, first crop after treatment and one year after treatment). For that, root galling index and crop yield were assessed for disease incidence. The suppressive effects were evaluated through soil microbiological activity (soil respiration rate), the physiological profiles at community-level of heterotrophic bacteria and through the nematode community structure. Nematode community related variables can provide useful information in soil quality assessment, but scarce information can be found in soil biodisinfestation studies. In this case, the relative presence of M. incognita nematode and nematode functional guilds together with plant-parasitic index and the enrichment footprint were calculated. Similar happens with long-term effects after biodisinfestation treatments. Generally, the results right after biodisinfestation treatments are assessed, but few studies evaluated the effects in long-term.
Materials and Methods
Study Site and Soil
The study was conducted in a commercial greenhouse located in the Basque Country, northern Spain (43°17′00′′N; 2°45′00′′W), a humid temperate region. The greenhouse selected for the study was a poly-tunnel consisting of four tunnels (8 × 50 m each tunnel), with lettuce monoculture. The lettuce varietal “Batavia,” commonly grown by farmers in this region, has been continuously cultivated in the same tunnels for the last 20 years. The plants were grown over plastic cover (25 × 30 cm pattern) with a total yield of about 4,500 lettuces per tunnel (12 plants/m2). The soil was loamy (33% sand, 49% silt, 18% clay, with 5 > 6% organic matter) and was naturally infested by Meloidogyne incognita which caused more than 45% yield loss in the last harvest before treatment, even with previous chemical fumigations. In fact, right before biodisinfestation (July), the farmer had to discard the entire lettuce crop grown in the treated tunnels, due to weak growth caused by M. incognita nematode (Figure 1A).
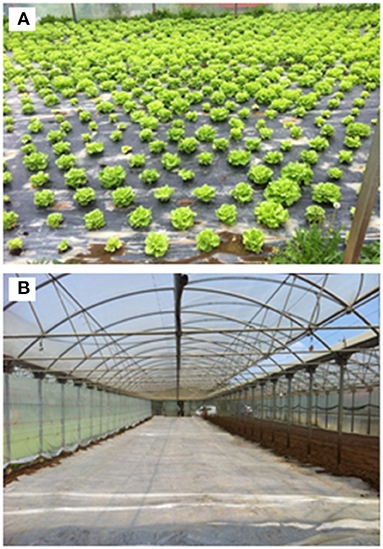
Figure 1. (A) Lettuce crop damaged by M. incognita nematode in the treated greenhouse. (B) View of biodisinfested and controls plots.
Organic Amendments
The organic amendments selected for soil biodisinfestation were fresh cow manure (FCM), beer bagasse (BB) and rapeseed cake (RC). The addition of fresh cow manure was included in farmers' crop management as a traditional practice in this region. It was obtained from a nearby farm, mixed on about 20% with straw, and applied at a dose of 20 kg/m2 (80% moisture). Beer bagasse is the residue obtained in beer production and it was provided by BOGA Basque Craft Beer as residue and incorporated into the soil at a dose of 15 kg/m2 (75% moisture). Finally, pellets of rapeseed (Brassica napus “Canola”) cake were added at a dose of 0.5 kg/m2 (6% moisture) and was supplied by an experimental sheep farm in Alava (Basque Country). These doses were based on previous researches with these byproducts (Gamliel et al., 2000; Mazzola et al., 2001; Guerrero-Díaz et al., 2014; Guerrero et al., 2019). Physicochemical characterizations of the organic amendments were used to adjust the final mixture (Table 1). The doses applied of FCM, BB, and RC represented a ratio of 78:10:12 (dry matter), respectively.
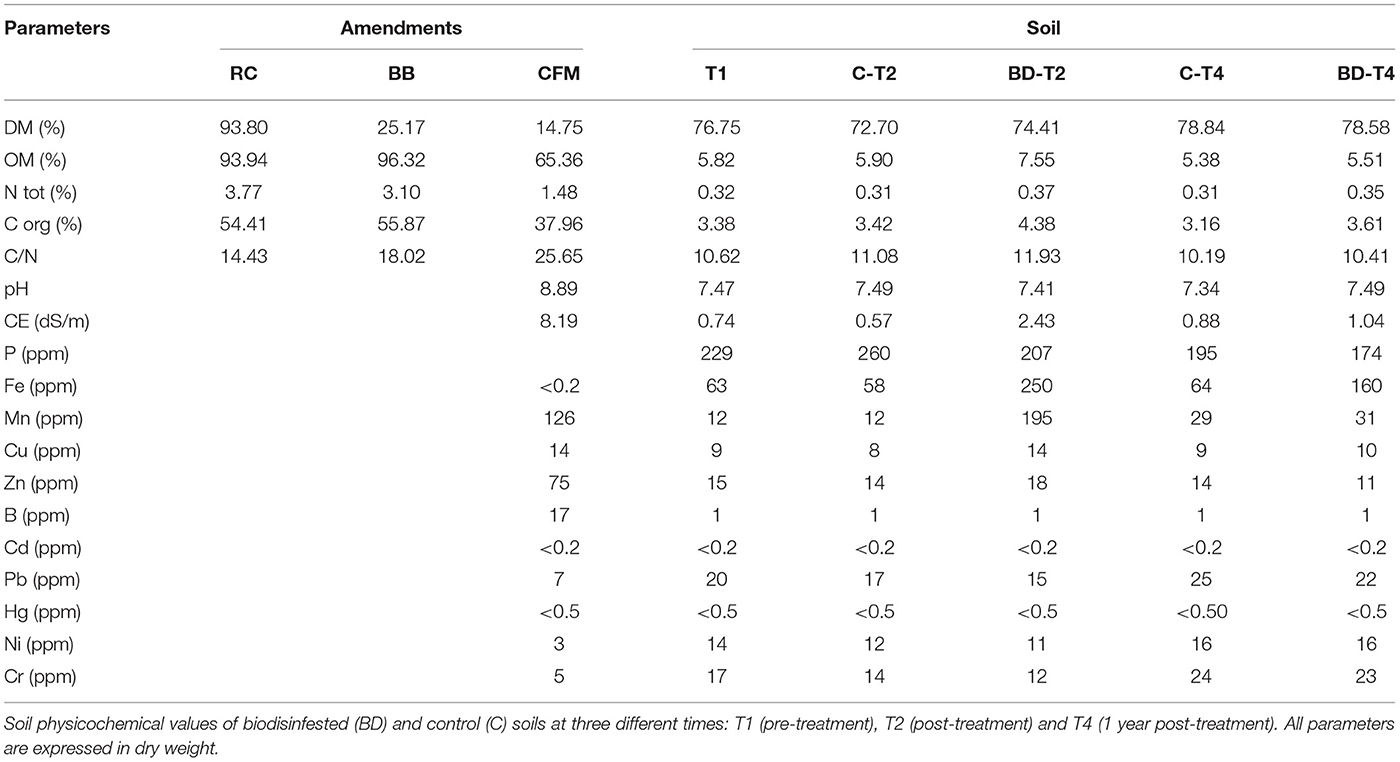
Table 1. Physicochemical values [dry matter (DM), organic matter (OM), total N, organic C, C/N ratio, pH, EC, some available nutrients and presence of heavy metals] of the amendments used for the biodisinfestation treatment: RC (rapeseed cake); BB (beer bagasse); FCM (fresh cow manure).
Experimental Design
The experimental design was structured as a combination of soil treatment and time. For that, two treatments were assessed: (i) non-treated control (C) and (ii) biodisinfested (BD) at different times: before treatment (T1), after treatment (T2), after the first crop post-treatment (T3), and one year after treatment (T4). Depending on the variable, different moments were assessed: soil microbiological properties at T2, T3, and T4; galling index and crop yield at T1, T3, and T4; and the structure of nematode functional guilds and M. incognita relative presence at T1, T2, T3, and T4. Soil treatments were arranged in a complete randomized design (CRD) with four replicate plots per treatment. Four tunnels of a poly-tunnel severely affected by M. incognita were virtually divided lengthwise into two parts in order to obtain four replicates per treatment with a plot size of 4 × 50 m2 each.
Biodisinfestation was carried out for 7 weeks at the end of summer, from 1st August to 19th September. In the treated plots, BB + RC + FCM were incorporated into the soil and mixed with the rotary tiller. Soil was then spray irrigated for 15 min (30 l/h/m2) and manually covered with a 200 gauges transparent polyethylene plastic film (provided by RAISAFILM). The edges of the plastic mulch were buried 10–15 cm deep in the soil avoiding air pockets formation (Figure 1B). In the control plots, FCM was incorporated at the same dose as in the treated ones but the soil was not covered with plastic film. After 7 weeks of biodisinfestation treatment, plastic cover was removed from the treated tunnels and soil was aerated during 1 week before the transplanting of the next lettuce crop. One year after biodisinfestation, both biodisinfested and control soils supported four crop growing cycles without additional fertilization.
Sampling Procedure and Data Collection
Composed soil samples were randomly collected from each plot with four samples per treatment. For this purpose, topsoil was collected from 0 to 25 cm depth at ten different points in each plot using a core soil sampler (2.5 cm diameter), the margins were excluded to avoid the edge effect. Sample collection was carried out at four moments: in July 2018 before biodisinfestation treatment (T1), in September 2018 after treatment (T2), in November 2018 after harvesting the first crop post-treatment (T3) and in August 2019 one year after biodisinfestation (T4). Soil samples were air-dried at room temperature and sieved at ≤ 2 mm for physicochemical and heavy metals presence analyses. Conversely, measurements of biological parameters were done on fresh soils samples sieved at ≤ 2 mm and stored at 4°C for a maximum of 1 month until analysis. For nematode extraction, fresh non-processed soil samples (600 g) were stored at 4°C and analyzed within 1 week after sampling.
Twenty lettuce roots were randomly collected from each plot in order to assess the GI before biodisinfestation (T1), after the first crop post-treatment (T3) and one year after biodisinfestation (T4). Crop yield (plants/m2) was also assessed at the same sampling times.
Temperature was recorded inside the treated tunnels (ambient temperature) and in the treated soil at three different depths during biodisinfestation treatment as described below.
Analyzed Variables
Organic Amendments and Soil Physicochemical Characterization
A single composed soil sample from each plot was used to determine the following parameters according to standard methods (MAPA, 1994) for all the organic amendments: dry matter (DM), pH, EC, organic matter (OM), N Kjeldhal, P Olsen and organic C. In the case of fresh manure, presence of fecal coliforms Escherichia coli and Salmonella spp. was also assessed following standard methods ISO-7251:2005 and ISO 6579-1:2017, respectively, as well as certain heavy metals and other metals of agronomical importance (USEPA, 2007) determined by ICP analyses.
The same parameters were analyzed for soil characterization, as well as pH and EC (dS/m) (Table 2). These parameters were analyzed only before (T1), after treatment (T2) and one year after biodisinfestation (T4). For that, soil samples were air-dried at room temperature and sieved through a 2 mm mesh before analysis. The physicochemical properties of this soil were also determined according to standard methods (MAPA, 1994), as well as heavy metals (USEPA, 2007).
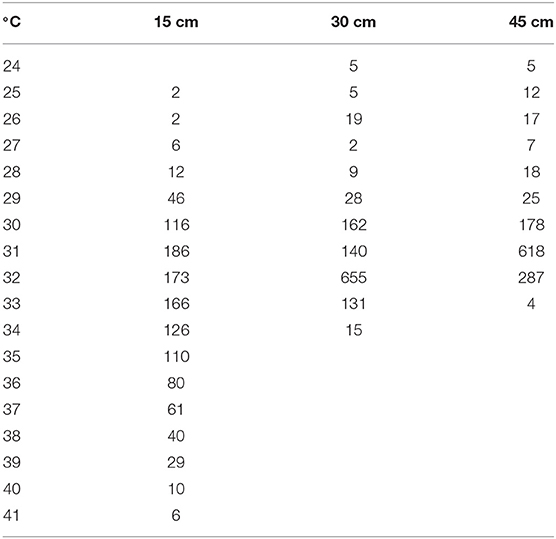
Table 2. Number of hours accumulated during 7 weeks of biodisinfestation treatment at different temperatures and at the different soil depths (15, 30, and 45 cm) in the treated plots.
Temperature
During biodisinfestation, soil temperatures were monitored at 15, 30, and 45 cm depth, as well as the ambient temperature inside the greenhouse. Soil temperatures were recorded in one treated plot every 15 min with specific probes connected to a Hobbo Datalogger (Weather Station, OCC, US, H8-4 32K). Average values per hour at different depths were calculated in order to know the number of hours at the recorded temperatures in each depth along the treatment. The total hours at each temperature were summed to obtain the accumulated hours at each temperature.
Soil Microbiological Properties
Immediately after soil sample collection, fresh samples were stored at 4°C and sieved to <2 mm prior microbiological analyses. Microbial activity was assessed by measuring soil respiration, which indicates total belowground activity. The procedure was an adaptation of ISO standard 16072:2002 by which CO2 emission rate of the soil was measured in a hermetic jar incubated for 3 days at 30°C and quantified by titration with NaOH. In addition, changes in bacterial community structure were determined with Biolog Ecoplates™ through the physiological profiles of the heterotrophic bacteria at community-level. This technique rapidly allows the detection of changes in microbiological structure by measuring the metabolic activity in 31 different C-sources (Garland and Mills, 1991; Insam, 1997). For that, soil water extracts were incubated in Biolog Ecoplates™ (96-well microplates with 31 different substrates) at 30°C and absorbance (590 nm) was measured every 12 h during 10 days. Then, average well color development (AWCD) and the number of utilized substrates (NUS) were calculated at half the incubation time (240 h). Composed samples from each plot were used in duplicate to work with two sub-samples in both microbiological analyses.
Root Galling Index and Yield
The root galling index (GI) of lettuce plants was estimated according to the scale of Bridge and Page (1980) where 0 represents root without galling (healthy root system) and 10 is the maximum degree of galling (root functioning is loose and decaying). For this purpose, 20 plants were randomly collected of each plot and visually evaluated.
In addition, yield data were obtained considering the commercial plants selected by the farmer. These data were adapted in order to obtain the number of commercial plants per square meter that enabled the assessment of disease incidence and yield.
Identification of Root-Knot Nematode
Identification of root-knot nematode species was done by perineal pattern characterization (Hartman and Sasser, 1985). In summary, affected roots were washed under tap water and single galls were selected for the extraction of females. Under magnifying binoculars NIKON SMZ800 (6.3X), selected galls were open and the females were extracted and immersed in lactophenol. The body was then transversally cut to obtain the perineal area. This fragment was dyed and fixed in a glass slide for visualization under optical microscope.
Soil Nematode Community and Presence of M. incognita
Soil nematode extraction was performed following the sieving and Baermann-Funnel technique (Barker, 1985). For that, fresh and non-processed soil samples (600 g) were divided into three sub-replicates of 200 g each. After extraction, nematodes were visually identified at family level with an optical microscope LEIKA DM6000B (200X) and counted on 100 μl of extraction but calculated in 100 g soil (dry weight). The relative presence of the pathogen species M. incognita and the relative presence of the different guilds found in soil samples were calculated to assess the biodisinfestation effects on the pathogen and on the nematode community structure. Bongers and Bongers (1998) proposed the term “guild” as a tool to improve the analysis of soil nematode community that classified the different nematode taxa considering the feeding group (bacterivores: Ba; fungivores: Fu; plant-parasitic: PP; predators: Pr; omnivores: Om) and the life strategy with values between 1 and 5, low values for colonizers and high values for persister (cp value). In addition, plant-parasitic index (PPI) and enrichment footprint (EF) were also calculated. The PPI is an indicator of the global plant-parasitic nematode assemblage and not only a single pathogen specie. The EF considers opportunistic nematode groups and reflects an increase in resources due to an implementation of organic amendments and the resulting increase in microbiological activity (Ferris et al., 2001; Ferris, 2010). Both PPI and EF values were obtained thanks to the free NINJA tool developed by Sieriebriennikov et al. (2014) and can provide information about the effects of soil biodisinfestation on other PPN and opportunistic nematodes which may contribute to develop suppressiveness.
Statistical Analysis
Analyses were performed with a one-way factor (soil treatment) analysis of variance (ANOVA) with the linear mixed model procedure (proc MIXED) of the statistical software SAS 9.4, which contained both fixed- and random-effects. Each soil treatment and time combination was considered as a fixed factor, and each replicate plot a random factor nested in each combination of soil treatment and time. Means of significant variables were separated with the multiple range Tukey HSD test with adjustment for the P-values against an overall experiment-wise type I error rate of α = 0.05. Nematode related variables were squared root transformed to stabilize the variances.
Results
Organic Amendments and Soil Physicochemical Characterization
Among the organic amendments, FCM presented the highest amount of organic matter (65.36%) but similar N total content as RC (1.48 and 1.36, respectively). FCM showed also a high content of organic C (37.96%) followed by RC (10.3%) (Table 1). The final mix of the different by-products presented a C/N ratio of 23 and OM content of 53.52% considering the application ratio (FCM:BB:RC 78:10:12).
The soil before treatment (T1) had an initial OM content of 5.82% and after biodisinfestation (T2) the controls (C) remained around this value, whereas in treated soil (BD) this value reached 7.55%. Even though N total content slightly increased in the treated soils, organic C content and EC increased after biodisinfestation compared to the controls. Likewise, Fe and Mn content increased significantly in the treated soils after treatment (249 and 195 ppm, respectively). One year after biodisinfestation (T4), all parameters recovered the initial values before treatment except for Fe, which remained high (159 ppm) regarding to the control (63 ppm). In all cases, no appreciable differences were observed and the heavy metals remained below the toxicity levels (Table 1).
Temperature
During the 7 weeks of biodisinfestation treatment, the ambient temperature range recorded inside the treated tunnels was 13.2–57.2°C. Soil temperature ranges were 25.5–41.6°C at 15 cm depth, 24.5–34.5°C at 30 cm and 24.8–33°C at 45 cm in the soil of the treated tunnels (BD). The highest temperatures were registered in the first week of BD from 2nd to 6th August (Figure 2). The soil reached the highest temperatures at 15 cm depth accumulating 29, 10, and 6 h at 39, 40, and 41°C, respectively. At 30 and 45 cm soil depth the temperature remained nearly constant between 31 and 32°C accumulating 655 h at 32°C at 30 cm and 618 h at 31°C at 45 cm (Table 2).
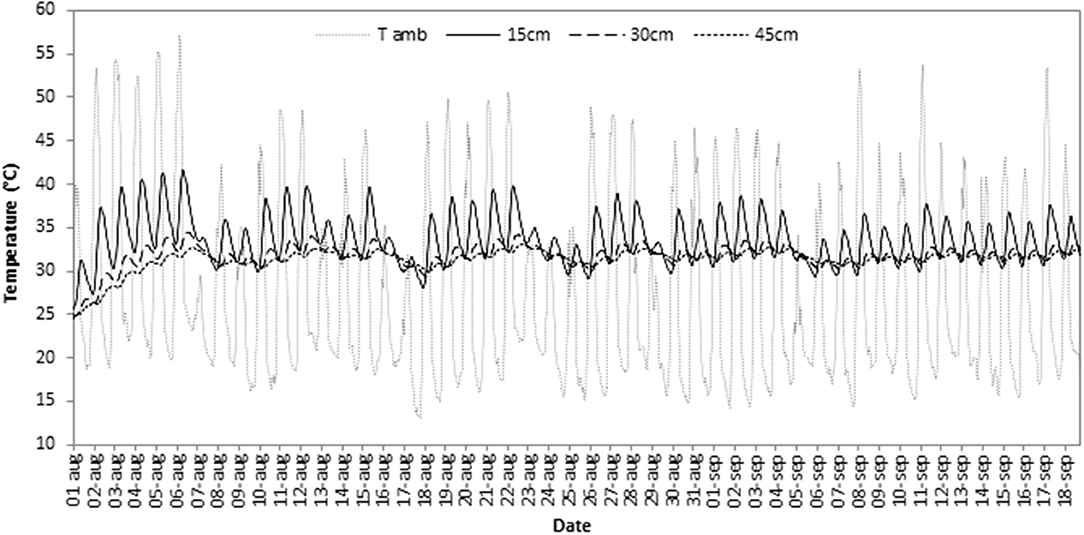
Figure 2. Mean temperatures per hour for each day during seven weeks of biodisinfestation treatment at ambient temperature inside the greenhouse (T amb) and at three different soil depths (15, 30, and 45 cm) in the treated soils.
Microbiological Soil Properties
Soil respiration rate was significantly higher (P <0.0001) in treated soils (4.31 mgC/g/h) than in controls (1.50 mgC/g/) after biodisinfestation and after the first crop (1.95 and 0.53 mgC/g/h, respectively) but it gradually decreased in T4 showing values closer to those of the control (1.34 and 1.02 mgC/g/h, respectively) (Figure 3A). Regarding Biolog EcoPlates™, no significant differences (P = 0.1077 at T2; P = 0.3166 at T3; P = 0.6779 at T4) were observed between treatments in AWCD (Figure 3B) at half of the incubation time. Similar happened with the NUS (Figure 3C) but, in this case, a statistically significant decrease (P = 0.003) occurred in the treated tunnels in T2.
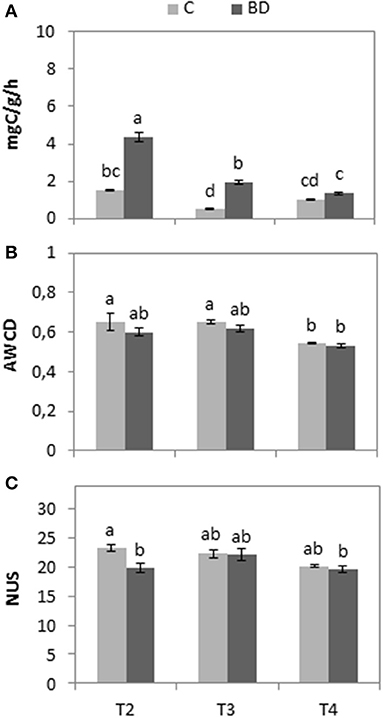
Figure 3. (A) Mean values and standard errors of soil respiration rate (mgC/g/h) in dry matter after 72 h incubation time at 30°C. (B) Mean values and standard errors of average well color development (AWCD). (C) Number of used substrates (NUS) in Biolog Ecoplates™ at half of the incubation time. All values of control (C) and biodisinfested (BD) soils measured at three different times: T2 (post-treatment), T3 (post-crop after treatment) and T4 (1 year after treatment). Values with the same letter are not significantly different among time and treatment combinations according to Tukey HSD test (P < 0.05).
Root Galling Index and Yield Data
Lettuce plants collected before treatment (T1) in the control plots showed a GI between 5 and 6, similar values to those in the treated plots. In the first harvest after treatment (T3), considerable difference (P < 0.0001) was observed between the plants collected in treated and control tunnels. While in the controls the GI remained close to 5, hardly any galls were detected on the roots sampled in the treated soils. One year after BD (T4), the lettuces in the control plots still showed significant root galling and crop damage (P < 0.0001). The samples from the treated soil began to show root galling with index values of 1–3 (Figure 4A) but without crop losses caused by M. incognita.
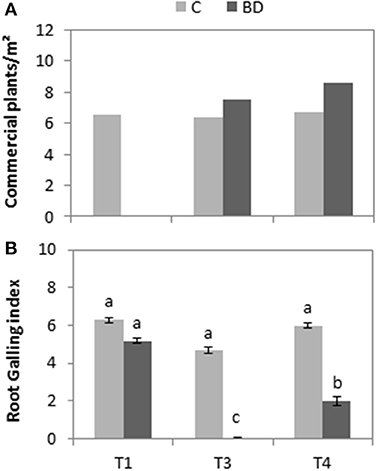
Figure 4. (A) Average and standard errors for root galling index according to root-knot nematode rating chart by Bridge and Page (1980) of lettuce plants. (B) Number of commercial lettuces collected. For both, lettuce were collected from the biodisinfested (BD) and control (C) plots at three different times: T1 (pre-treatment), T3 (post-crop after treatment) and T4 (1 year after treatment). Values with the same letter are not significantly different among time and treatment combinations according to Tukey HSD test (P < 0.05).
Regarding yield data, the maximum number of lettuce that could be harvested were 12 plants/m2. Before the trial, any lettuce was harvested for in the plots that were treated later due to severe damage caused by M. incognita. After biodisinfestation, the first crop grown faster in the treated soils and an increase of yield was observed (7.5 plants/m2). Moreover, lettuce yield improved one year after biodisinfestation (8.6 plants/m2) when 70% of total yield was harvested (Figure 4B). In contrast, in the control plots yield remained constant during the assessment period with around 55% (6.5 plants/m2) in the three sampling moments (T1, T3, and T4).
M. incognita Presence and Nematode Community Analyses
The relative presence detected of M. incognita was around 50% in all plots before treatment and it decreased below 30% in all of them at T2 with no significant differences between treatments (P = 0.4757). Although no significant difference (P = 0.1368) could be observed at T3, M. incognita was not detected in the biodisinfested soils. One year after treatment, no statistically significant differences (P = 0.1368) were observed between treatments with low presence of M. incognita in the control (<10%) but the absence of the pathogen continued in the biodisinfested soils (Figure 5A).
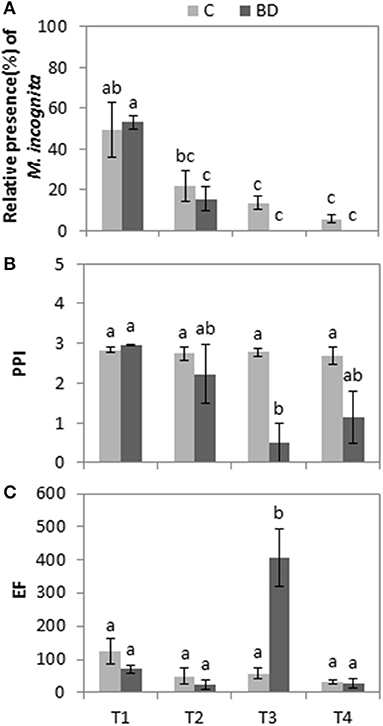
Figure 5. (A) Relative presence (%) of Meloidogyne incognita. (B) Plan parasitic index (PPI). (C) Enrichment footprint (EF). Values at T1 (pre-treatment), T2 (post-treatment), T3 (post-crop after treatment) and T4 (1 year after treatment) in the biodisinfested (BD) and control (C) soils. Values with the same letter are not significantly different among time and treatment combinations according to Tukey HSD test (P < 0.05).
Regarding the nematode community indexes, in the control soils PPI remained stable throughout the study, while in the biodisinfested soil this index decreased after the treatment (T2), especially after first crop post-treatment (T3) (P = 0.006), and remained at low values one year later with significant difference (P = 0.0136) regarding to the control (Figure 5B). The EF stayed stable over time with low values in both biodisinfested and control soils. Only at T3 this index increased significantly (P <0.0001) in the treated tunnels but shown initial levels one year later (Figure 5C).
According to the relative presence of the different guilds (Figure 6), PP3 dominated at T1 in all soils (about 50%) due to the presence of M. incognita, which is classified in this guild. After biodisinfestation, bacterivores became the dominant group in both soils (>48%) but at T3 this feeding group displaced the other groups in the biodisinfested soils (98.10% of presence) and stayed one year later (87.94%). While the presence of PPN in the control soils barely changed at T2, in the treated soils no presence was detected at T3 and only 2.38% one year later without detection of M. incognita.
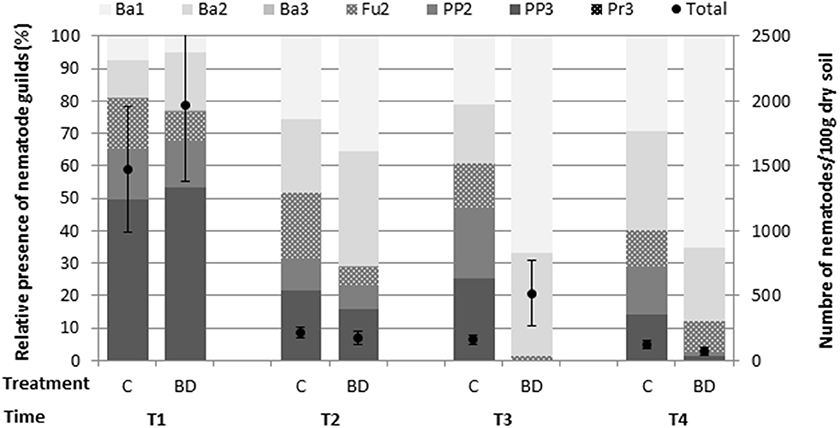
Figure 6. Percentage of relative presence of different nematode guilds are represented by bar chart: Ba1 (bacterivores cp-1), Ba2 (bacterivores cp-2), Ba3 (bacterivores cp-3), Fu2 (fungivores cp-2), PP2 (plant-parasitic cp-2), PP3 (plant-parasitic cp-3) and Pr3 (predators cp-3). Total number of nematodes presented in 100 g of soil (dry matter) is represented by dot chart with the standard errors. Values of soil samples collected in the biodisinfested (BD) and control (C) soils at four different times: T1 (pre-treatment), T2 (post-treatment), T3 (post-crop after treatment) and T4 (1 year after treatment).
Discussion
The application of agricultural by-products followed by solarization has demonstrated to be effective against RKN M. incognita in lettuce crop. In this study, FCM + BB + RB resulted to be effective against M. incognita after biosolarization in a humid temperate area. The low solar radiation in these regions limits biodisinfestation processes related with high temperatures. However, positive results against M. incognita were obtained in similar studies carried out in northern areas (Núñez-Zofío et al., 2011; Ojinaga et al., 2020). During the assay, the maximum temperature achieved in the upper layer, at 15 cm (41.6°C), was below the maximum average temperature (45–55°C) registered in biosolarization performed in Mediterranean regions where this technique is included in crop management (Katan, 2017). However, comparable temperature values were obtained in similar trials carried out in summer in warmer geographical areas (Kaşkavalci, 2007; Núñez-Zofío et al., 2013) where maximum soil temperature averages registered were <42°C. The solar radiation is critical to achieve high temperatures in the soil but this effect is primarily achieved in the upper soil layers (Katan, 2017). Temperature data registered during this trial (Figure 2) showed how the temperatures in the upper soil layers increased as ambient temperature increased whereas the lower layers remained more stable at lower temperatures. Sub-lethal temperatures (30–35°C) were achieved in the lower layers during the most part of the assay which might render nematodes more vulnerable to biocidal compounds or to antagonistic microorganisms (Oka, 2010). In the upper layer (15 cm), 16 h accumulated between 40 and 41°C (Table 2) were enough to reduce population of M. incognita and GI, in agreement with the conclusion obtained by other authors in similar studies (Wang and McSorley, 2008; Guerrero-Díaz et al., 2014).
In this study, the effects of biodisinfestation were expected just after treatment, but only some physicochemical variables (Table 1) and soil respiration (Figure 3) showed differences between treatments at this time. On the contrary, nematode related variables showed significant differences after harvesting the first lettuce crop after biodisinfestation, at T3 (Figures 5, 6). More complex soil microorganisms like nematodes required more time to establish after any disturbance such as tillage and organic amendment incorporation than bacteria and fungi, which reacted faster after changes in environmental conditions (Bongers, 1999; Treonis et al., 2010). This shift in microbial population was reflected in the respiration rate after biodisinfestation in the treated soils, primarily due to the incorporation of organic amendments. The incorporation of FCM + BB + RB into the soil incremented the organic matter content more than FCM alone (Table 1) which contributed to increase the microbiological activity boosted by the high temperatures achieved under plastic film (Katan, 2017; Waisen et al., 2020). However, while significant differences were observed in soil respiration rate (Figure 3A), the physiological profile of heterotrophic bacterial community barely varied between treatments (Figures 3B,C). This might be due to changes in the soil physicochemical properties generated by biodisinfestation that favored certain indigenous microbiological communities. Previous studies have demonstrated that populations of antagonistic microorganisms like Trichoderma, Bacillus, and Pseudomonas spp. were enhanced after biosolarization resulting, in many cases, in suppressive soils (Stapleton, 2000; Moosavi, 2020; Rosskopf et al., 2020).
This suppressive effect was observed in the nematode related variables, which showed clearer differences after first harvest post-treatment and one year later than right after biodisinfestation. The reduction of M. incognita population was significant after first crop post-treatment with regard to the control (Figure 5A) and the same happened with PPN, both represented by PPI (Figure 5B). Although no statistical difference was observed in PPI one year after biodisinfestation, PPN represented <5% of total population identified in the treated soils (Figure 6). In concordance with previous studies, biodisinfestation effects on PPN remained longer periods by favoring antagonist microorganisms on detrimental of PPN (Ntalli et al., 2020). Conversely, bacterivorous nematodes were favored by the new conditions via enhancing the food source. In fact, after first crop post-treatment this group represented >95% of the whole community with higher number of individuals per 100 g/dry soil, manly represented by Rhabditidae taxa. Nematodes of this family have a short life-cycle and high colonization ability under high microbial activity (Bongers, 1990; Bongers and Bongers, 1998). This fact can be observed on EF (Figure 5C), which showed very high values after the first harvest after biodisinfestation, in the treated soils regarding to the other samplings. This result might also explain the increase of crop yield and even the early harvest of the first crop after biodisinfestation in the treated soils as a consequence of nutrients availability by higher microbiological activity. Thus, the incorporation of organic amendments in biodisinfestation practices might enhance the degradation rate increasing crop yield. One year after treatment, bacterivorous nematodes remained at high proportion (>85%) due to the prevalence of opportunistic bacterivores families (Ba1), nevertheless, nematode community tended toward equilibrium with the presence of fungivorous and an emerging population of PPN. The population of PPN remained lower in the treated soils than in the controls (2.38 and 29.04%, respectively) one year after treatment, after supporting four susceptible crop cycles (Figures 5B, 6). Among PPN families identified in the soil samples one year post-treatment, presence of Meloidogyne was not detected in the samples but root galling was observed. According to Tzortzakakis (2010), GI and presence of M. incognita might not be necessarily correlated. Furthermore, some eggs might detach from the root and survived in the lower soil layers waiting for hutching under favorable conditions (Curtis et al., 2009). That might explain the beginning of galling one year after biodisinfestation in plants grown in the treated soils, but with low GI (1.99) that enables plant growth without disease development (Figure 4).
Among the soil processes generated by biodisinfestation practices, there is still no general consensus on the main cause of soil pest reduction. According to these results, we suggested that first effects were generated by the biocidal compounds, mainly VFAs and ammonia, released by the degradation of RC and BB together with FCM amendments followed by shift in antagonist microbial community, as it was suggested by several authors (Mazzola et al., 2001; Cohen et al., 2005; Mocali et al., 2015). This increase in the resident antagonist microorganisms generated beneficial effects in the following crops, even one year after biodisinfestation, after four susceptible crop cycles. This shift in the microbiological community might have an impact on PPN reproduction rate as well. This change promoted colonization by opportunistic bacterivores nematodes at the expense of other groups. Although nematode community tended to equilibrium one year after treatment, the significant decrease of PPN after the first harvest post-treatment and one year later, along with yield increase, suggested that the proliferation of antagonistic organisms via biodisinfestation might be the main factor in M. incognita population decrease in long-term, resulting in suppressive soils (Moosavi, 2020). Moreover, M. incognita suppression might also be induced by fungivores or other nematodes when competing for same ecological niches (Oka, 2010).
The combination of organic amendments might have an important role and further research is needed on proper mixtures to be incorporated in these practices. In this study, positive results were obtained with BB + RC + FCM. The effects of fresh manures have been widely demonstrated (Rosskopf et al., 2020) but, in this case, the aim was to enhance effectiveness through implementation of certain by-products. According to previous studies, rapeseed cake (Brassica napus “canola”) has demonstrated to be effective against soilborne pathogens despite the low glucosinolates content (Mazzola et al., 2001). In this case, the main effect was attributed to the high nitrogen concentration that led to release of NH3 via microbial degradation (Oka, 2010). On the other hand, the incorporation of BB as labile C-source could enhance the microbial degradation and the consequent release of VFAs (e.g., acetic, butyric, formic, and propionic acids) which have nematicidal effect (Oka, 2010). Besides, the increase in Fe and Mn in the treated plots (Table 1), which have also demonstrated biocidal effects, indicated that reductive conditions were enhanced by these amendments (Fernandez-Bayo et al., 2018). Regarding the properties of these by-products, could be interesting to consider them when choosing organic amendments in biodisinfestation treatments against Meloidogyne species.
In this case, the combination of these agro-industrial by-products with fresh manure demonstrated to be effective in short- and long-term against M. incognita and the whole PPN assemblage. The assessment of nematode functional guilds and EF provided information about the impact of biodisinfestation treatments on nematodes and their role in soil suppressiveness. The evaluation of nematode community offers useful information about the status of the soil food web that can help to better understand the development of suppressive soils due to biodisinfestation. Regarding the microbiological analysis, soil respiration rate has proven to be effective to measure microbial activity. While other analysis should be done, or combined with Biolog EcoPlates™, to understand the changes in the bacterial community structure. Metagenomics or other “-omics” techniques could be interesting alternatives to better understand these processes.
Our assay proved the effectiveness of biodisinfestation treatments in geographical areas with low solar radiation. This suggests, that high temperatures (>42°) are not necessarily required to achieve positive effects in soil biodisinfestation and soil supressiveness. Moreover, we considered that the sub-lethal temperatures were one of the main effects of soil suppressiveness. In some cases, biosolarization in Mediterranean areas can achieve high temperatures (>50°C) that lead to pasteurization with the consequent damage of possible antagonists. Sub-lethal temperatures can be enough to promote biodisinfestation processes, as well as long-term suppressiveness. The study of sub-lethal temperatures in indigenous microflora and nematode community would be interesting to understand the processes in soil suppressiveness after biodisinfestation.
Data Availability Statement
The original contributions presented in the study are included in the article/supplementary materials, further inquiries can be directed to the corresponding author/s.
Author Contributions
MG, JL-P, and SL contributed to conception and design of the study. MG, BJ, and LA conducted the assay and samplings. MG and JL-P performed the laboratory analyses. SL and MG organized the database and performed the statistical analysis. MG wrote the manuscript. All authors contributed to manuscript revision, read, and approved the submitted version.
Funding
This research has been financially supported by FEDER funds (project INIA RTA 2015-00060-C04-04 Comprehensive revaluation of by-products based on their potential uses: obtainment of biofumigants and fertilizers and project MICINN RTA PID2019-106148RR-C44 Integration of revalorized agro-food by-products into an enhanced circular economy model: new applications for horticultural and extensive crops in commercial facilities) and by the Department of Environment, Territorial Planning, Agriculture and Fisheries of the Basque Government (projects REVABIO and REVAL2). MG was the recipient of a predoctoral contract (project reference INIA-2017-0043) of the State Training Subprogram of the Spanish Ministry of Economy, Industry and Competitiveness (MICINN).
Conflict of Interest
The authors declare that the research was conducted in the absence of any commercial or financial relationships that could be construed as a potential conflict of interest.
Acknowledgments
The authors want to thanks the family of farmers who collaborated and allowed us to carry out this assay.
References
Alabouvette, C., Hoeper, H., Lemanceau, P., and Steinberg, C. (1996). “Soil supressiveness to diseases induced by soilborne plant pathogens,” in Soil Biochemistry, eds G. Stotzky, J.-M. Bollag (New York, NY: Marcel Dekker), 371–413.
Avato, P., D'Addabbo, T., Leonetti, P., and Argentieri, M. P. (2013). Nematicidal potential of Brassicaceae. Phytochem. Rev. 12, 791–802. doi: 10.1007/s11101-013-9303-7
Baker, K. F., and Cook, R. J. (1974). Biological Control of Plant Pathogens. San Fransisco: American Phytopathology Society, 433pp.
Barker, K. R. (1985). “Nematode extraction and bioassays,” in An Advanced Treatise on Meloidogyne: Methodology, Vol. 2, eds K. R. Barker, C. C. Carter, J. N. Sasser (Raleigh, NC: North Carolina State University Graphics, Raleigh), 19–35.
Bonanomi, G., Antignani, V., Capodilupo, M., and Scala, F. (2010). Identifying the characteristics of organic soil amendments that suppress soil-borne plant diseases. Soil Biol. Biochem. 42, 136–144. doi: 10.1016/j.soilbio.2009.10.012
Bongers, T. (1990). The maturity index: an ecological measure of environmental disturbance based on nematode species composition. Oecologia 83, 14–19. doi: 10.1007/BF00324627
Bongers, T. (1999). The maturity index, the evolution of nematode life history traits, adaptive radiation and cp-scaling. Plant Soil 212, 13–22. doi: 10.1023/A:1004571900425
Bongers, T., and Bongers, M. (1998). Functional diversity of nematodes. Appl. Soil Ecol. 10, 239–251. doi: 10.1016/S0929-1393(98)00123-1
Bongers, T., and Ferris, H. (1999). Nematode community structure as a bioindicator in environmental monitoring. Trends Ecol. Evol. 14, 224–228. doi: 10.1016/S0169-5347(98)01583-3
Brennan, R. J. B., Glaze-Corcoran, S., Wick, R., and Hashemi, M. (2020). Biofumigation: an alternative strategy for the control of plant parasitic nematodes. J. Integr. Agric. 19, 1680–1690. doi: 10.1016/S2095-3119(19)62817-0
Bridge, J., and Page, S. L. J. (1980). Estimation of root-knot nematode infestation levels on roots using a rating chart. Trop. Pest Manag. 26, 296–298. doi: 10.1080/09670878009414416
Carrascosa, M., Sánchez-Moreno, S., and Alonso-Prados, J. L. (2014). Relationships between nematode diversity, plant biomass, nutrient cycling and soil suppressiveness in fumigated soils. Eur. J. Soil Biol. 62, 49–59. doi: 10.1016/j.ejsobi.2014.02.009
Cesarz, S., Reich, P. B., Scheu, S., Ruess, L., Schaefer, M., and Eisenhauer, N. (2015). Nematode functional guilds, not trophic groups, reflect shifts in soil food webs and processes in response to interacting global change factors. Pedobiologia 58, 23–32. doi: 10.1016/j.pedobi.2015.01.001
Chitwood, B. G. (1949). Root-knot nematodes – Part 1. A revision of the genus Meloidogyne Goeldi, 1887. Proc. Helminthol. Soc. Washington 16, 90–104.
Cohen, M. F., Yamasaki, H., and Mazzola, M. (2005). Brassica napus seed meal soil amendment modifies microbial community structure, nitric oxide production and incidence of Rhizoctonia root rot. Soil Biol. Biochem. 37, 1215–1227. doi: 10.1016/j.soilbio.2004.11.027
Correia, É. C. S., da, S., da Silva, N., Costa, M. G. S., and Wilcken, S.R.S. (2019). Response of lettuce cultivars to Meloidogyne javanica and Meloidogyne incognita race 1 and 2. Rev. Cienc. Agron. 50, 100–106. doi: 10.5935/1806-6690.20190012
Curtis, R. H. C., Robinson, A. F., and Perry, R. N. (2009). “Hatch and host location,” in Root-Knot Nematodes, eds R. N. Perry, M. Moens, J. L. Starr (Wallingford, Oxfordshire: CAB International), 139–162.
Dutta, T. K., Khan, M. R., and Phani, V. (2019). Plant-parasitic nematode management via biofumigation using brassica and non-brassica plants: current status and future prospects. Curr. Plant Biol. 17, 17–32. doi: 10.1016/j.cpb.2019.02.001
Fernandez-Bayo, J. D., Randall, T. E., Harrold, D. R., Achmon, Y., Hestmark, K. V., and Su, J. (2018). Effect of management of organic wastes on inactivation of Brassica nigra and Fusarium oxysporum f.sp lactucae using soil biosolarization. Pest Manag. Sci. 74, 1892–1902. doi: 10.1002/ps.4891
Ferris, H. (2010). Form and function: metabolic footprints of nematodes in the soil food web. Eur. J. Soil Biol. 46, 97–104. doi: 10.1016/j.ejsobi.2010.01.003
Ferris, H., Bongers, T., and De Goede, R. G. M. (2001). A framework for soil food web diagnostics: extension of the nematode faunal analysis concept. Appl. Soil Ecol. 18, 13–29. doi: 10.1016/S0929-1393(01)00152-4
Fourie, H., Ahuja, P., Lammers, J., and Daneel, M. (2016). Brassicacea-based management strategies as an alternative to combat nematode pests: a synopsis. Crop Prot. 80, 21–41. doi: 10.1016/j.cropro.2015.10.026
Gamliel, A., Austeraweil, M., and Kritzman, M. (2000). Non-chemical approach to soilborne pest management - organic amendments. Crop Prot. 19, 847–853. doi: 10.1016/S0261-2194(00)00112-5
Gareau, B. J. (2010). A critical review of the successful CFC phase-out versus the delayed methyl bromide phase-out in the montreal protocol. Int. Environ. Agreements 10, 209–231. doi: 10.1007/s10784-010-9120-z
Garland, J. L., and Mills, A. L. (1991). Classification and characterisation of heterotrophic microbial communities on the basis of pattern of community-level sole-carbon-source utilization. Appl. Environ. Microbiol. 57, 2351–2359. doi: 10.1128/AEM.57.8.2351-2359.1991
Guerrero, M. M., Lacasa, C. M., Martínez, V., Martínez-Lluch, M. C., Larregla, S., and Lacasa, A. (2019). Soil biosolarization for Verticillium dahliae and Rhizoctonia solani control in artichoke crops in southeastern Spain. Spanish J. Agric. Res. 17, 1–11. doi: 10.5424/sjar/2019171-13666
Guerrero-Díaz, M. M., Lacasa-Martínez, C. M., Hernández, A., Martínez, V., Martínez, M. C., Fernández-Molina, P., et al. (2014). Biosolarization with agroindustrial by-product for the control of soilborne pathogens in protected pepper crops in southeast Spain. Acta Hortic. 1044, 157–161. doi: 10.17660/ActaHortic.2014.1044.19
Hartman, K. M., and Sasser, J. N. (1985). “Identification of Meloidogyne species on the basis of differential host test and perineal pattern morphology,” in An Advanced Treatise on Meloidogyne. Volume II: Methodology. A Cooperative Publication of the Department of Plant Pathology and the United States Agency for International Development, eds K. R. Barker, C. C. Carter, J. N. Sasser (Raleigh, NC: North Carolina State University Graphics), 69–77.
Ijoyah, M. O., and Koutatouka, M. (2009). Effect of soil solarization using plastic mulch in controlling root-knot nematode (Meloidogyne spp.) infestation and yield of lettuce at Anse Boileau, Seychelles. Afr. J. Biotechnol. 8, 6787–6790.
Insam, H. (1997). “A new set of substrates proposed for community characterization in environmental samples,” in Microbial Communities. Functional Versus Structural Approaches, eds H. Insam and A. Rangger (Springer), 260–261. doi: 10.1007/978-3-642-60694-6_25
Janvier, C., Villeneuve, F., Alabouvette, C., Edel-Hermann, V., Mateille, T., and Steinberg, C. (2007). Soil health through soil disease suppression: which strategy from descriptors to indicators? Soil Biol. Biochem. 39, 1–23. doi: 10.1016/j.soilbio.2006.07.001
Jones, J. T., Haegeman, A., Danchin, E. G. J., Gaur, H. S., Helder, J., Jones, M. G. K., et al. (2013). Top 10 plant-parasitic nematodes in molecular plant pathology. Mol. Plant Pathol. 14, 946–961. doi: 10.1111/mpp.12057
Kaşkavalci, G. (2007). Effect of soil solarization and organic amendment treatments for controlling Meloidogyne incognita in tomato cultivars in Western Anatolia. Turk. J. Agric. For. 31, 159–167. Available online at: https://journals.tubitak.gov.tr/agriculture/abstract.htm?id=8891
Katan, J. (2014). Soil solarization: the idea, the research and its development. Phytoparasitica 43, 1–4. doi: 10.1007/s12600-014-0419-0
Katan, J. (2017). Diseases caused by soilborne pathogens: biology, management and challenges. J. Plant Pathol. 99, 305–315. doi: 10.4454/jpp.v99i2.3862
Katan, J., and Gamliel, A. (2014). Plant health management: soil solarization. Encycl. Agric. Food Syst. 4, 460–471. doi: 10.1016/B978-0-444-52512-3.00256-4
Kirkegaard, J. A., Gardner, P. A., Desmarchelier, J. M., and Angus, J. F. (1993). “Biofumigation: using Brassica species to control pests and diseases in horticulture and agriculture,” in 9th Australian Research Assembly on Brassicas, eds N. Wratten and R. J. Mailer (Agricultural Research Institute, Wagga Wagga), 77–82.
Klein, E., Katan, J., and Gamliel, A. (2012). Soil suppressiveness to Meloidogyne javanica as induced by organic amendments and solarization in greenhouse crops. Crop Prot. 39, 26–32. doi: 10.1016/j.cropro.2012.02.013
Koenning, S. R., Overstreet, C., Noling, J. W., Donald, P. A., Becker, J. O., and Fortnum, B. A. (1999). Survey of crop losses in response to phytoparasitic nematodes in the United States for 1994. J. Nematol. 31, 587–618.
Kofoid, C. A., and White, W. A. (1919). A new nematode infection of man. J. Am. Med. Assoc. 72, 567–569. doi: 10.1001/jama.1919.02610080033010
MAPA (1994). Métodos Oficiales de Análisis, Tomo III. Ed. Secretaría General Técnica. Madrid: Ministerio de Agricultura, Pesca y Alimentación. p. 662.
Matthiessen, J., and Kirkegaard, J. (2006). Biofumigation and enhanced biodegradation: opportunity and challenge in soilborne pest and disease management. CRC. Crit. Rev. Plant Sci. 25, 235–265. doi: 10.1080/07352680600611543
Mazzola, M., Granatstein, D. M., Elfving, D. C., and Mullinix, K. (2001). The suppression of specific apple root pathogens by Brassica napus seed meal amendment regardless of glucosinolate content. Phytopathology 91, 673–679. doi: 10.1094/PHYTO.2001.91.7.673
Mocali, S., Landi, S., Curto, G., Dallavalle, E., Infantino, A., Colzi, C., et al. (2015). Resilience of soil microbial and nematode communities after biofumigant treatment with defatted seed meals. Ind. Crops Prod. 75, 79–90. doi: 10.1016/j.indcrop.2015.04.031
Moosavi, M. R. (2020). “Efficacy of microbial biocontrol agents in integration with other managing methods against phytoparasitic nematodes,” in Management of Phytonematodes: Recent Advances and Future Challenges, eds R. Ansari, R. Rizvi, and I. Mahmood (Singapore: Springer), 229–258.
Ntalli, N., Adamski, Z., Doula, M., and Monokrousos, N. (2020). Nematicidal amendments and soil remediation. Plants 9, 1–20. doi: 10.3390/plants9040429
Núñez-Zofío, M., Larregla, S., and Garbisu, C. (2011). Application of organic amendments followed by soil plastic mulching reduces the incidence of Phytophthora capsici in pepper crops under temperate climate. Crop Prot. 30, 1563–1572. doi: 10.1016/j.cropro.2011.08.020
Núñez-Zofío, M., Larregla, S., Garbisu, C., Guerrero, M. M., Lacasa, C. M., and Lacasa, A. (2013). Application of sugar beet vinasse followed by solarization reduces the incidence of Meloidogyne incognita in pepper crops while improving soil quality. Phytoparasitica 41, 181–191. doi: 10.1007/s12600-012-0277-6
Ojinaga, M., Gandariasbeitia, M., Orbegozo, E., Ortíz, A., Guerrero, M. M., Lacasa, C. M., et al. (2020). Biodisinfestation for Meloidogyne and Verticillium control in commercial protected crops in the Basque Country Atlantic area (Northern Spain). Acta Hortic. 1270, 327–336. doi: 10.17660/ActaHortic.2020.1270.40
Oka, Y. (2010). Mechanisms of nematode suppression by organic soil amendments-a review. Appl. Soil Ecol. 44, 101–115. doi: 10.1016/j.apsoil.2009.11.003
Oka, Y., Shapira, N., and Fine, P. (2007). Control of root-knot nematodes in organic farming systems by organic amendments and soil solarization. Crop. Prot. 26, 1556–1565. doi: 10.1016/j.cropro.2007.01.003
Rosskopf, E., Di Gioia, F., Hong, J. C., Pisani, C., and Kokalis-Burelle, N. (2020). Organic amendments for pathogen and nematode control. Annu. Rev. Phytopathol. 58, 277–311. doi: 10.1146/annurev-phyto-080516-035608
Sasser, J. N. (1980). Root-knot nematodes: a global menace to crop production. Plant Dis. 64, 36–41. doi: 10.1094/PD-64-36
Shennan, C., Muramoto, J., Koike, S., Baird, G., Fennimore, S., Samtani, J., et al. (2018). Anaerobic soil disinfestation is an alternative to soil fumigation for control of some soilborne pathogens in strawberry production. Plant Pathol. 67, 51–66. doi: 10.1111/ppa.12721
Sieriebriennikov, B., Ferris, H., and de Goede, R. G. M. (2014). NINJA: an automated calculation system for nematode-based biological monitoring. Eur. J. Soil Biol. 61, 90–93. doi: 10.1016/j.ejsobi.2014.02.004
Stapleton, J. J. (2000). Soil solarization in various agricultural production systems. Crop Prot. 19, 837–841. doi: 10.1016/S0261-2194(00)00111-3
Talavera, M., Miranda, L., Gómez-Mora, J. A., Vela, M. D., and Verdejo-Lucas, S. (2019). Nematode management in the strawberry fields of Southern Spain. Agronomy 9:252. doi: 10.3390/agronomy9050252
Treonis, M., Austin, E. E., Buyer, J. S., Maul, J. E., Spicera, L., and Zasada, I. A. (2010). Effects of organic amendment and tillage on soil microorganisms and microfauna. Appl. Soil Ecol. 46, 103–110. doi: 10.1016/j.apsoil.2010.06.017
Tzortzakakis, E. A. (2010). The correlation of root galling with eggs in root and juveniles in soil of root knot nematodes (Meloidogyne spp.) infecting greenhouse vegetables. Nematol. Mediterr. 38, 99–101. Available online at: http://www.inaav.ba.cnr.it/vol38-1,%202010/vol38-1n.pdf
USEPA (2007). United States Environmental Protection Agency. Framework for Metals Risk Assessment. Washington, DC: Office of the Science Advisor. EPA 120/R-07/001.
Waisen, P., Cheng, Z., Sipes, B. S., DeFrank, J., Marahatta, S. P., and Wang, K. H. (2020). Effects of biofumigant crop termination methods on suppression of plant-parasitic nematodes. Appl. Soil Ecol. 154:103595. doi: 10.1016/j.apsoil.2020.103595
Wang, K. H., and McSorley, R. (2008). Exposure time to lethal temperatures for Meloidogyne incognita suppression and its implication for soil solarisation. J. Nematol. 40, 7–12.
Keywords: rapeseed cake, beer bagasse, organic amendment, solarization, root-knot nematode, nematode guilds, sub-lethal temperatures
Citation: Gandariasbeitia M, López-Pérez JA, Juaristi B, Abaunza L and Larregla S (2021) Biodisinfestation With Agricultural By-Products Developed Long-Term Suppressive Soils Against Meloidogyne incognita in Lettuce Crop. Front. Sustain. Food Syst. 5:663248. doi: 10.3389/fsufs.2021.663248
Received: 02 February 2021; Accepted: 26 April 2021;
Published: 31 May 2021.
Edited by:
Jesus Fernandez Bayo, University of California, Davis, United StatesReviewed by:
Emily Shea, University of California, Davis, United StatesYigal Achmon, Guangdong Technion-Israel Institute of Technology (GTIIT), China
Copyright © 2021 Gandariasbeitia, López-Pérez, Juaristi, Abaunza and Larregla. This is an open-access article distributed under the terms of the Creative Commons Attribution License (CC BY). The use, distribution or reproduction in other forums is permitted, provided the original author(s) and the copyright owner(s) are credited and that the original publication in this journal is cited, in accordance with accepted academic practice. No use, distribution or reproduction is permitted which does not comply with these terms.
*Correspondence: Maite Gandariasbeitia, mgandariasbeitia@neiker.eus