- 1Food Technology Department, University of Costa Rica, San Jose, Costa Rica
- 2National Center of Food Science and Technology (CITA), University of Costa Rica, San Jose, Costa Rica
Pollution resulting from the persistence of plastics in the environment has driven the development of substitutes for these materials through fermentation processes using agro-industrial wastes. Polyhydroxybutyrate (PHB) is a rapidly biodegradable material with chemical and mechanical properties comparable to those of some petroleum-derived plastics. PHB accumulates intracellularly as an energy reserve in a wide variety of microorganisms exposed to nutritionally imbalanced media. The objective of this study was to evaluate the use of a banana waste product as a carbon source for PHB production. PHB was extracted by acid methanolysis and detected by gas chromatography-mass spectrometry. Eleven bacterial strains with potential for PHB production were evaluated by in vitro fermentation in a culture broth containing fructose as the carbon source and limited nitrogen. A 22 central composite rotational design was applied to optimize the concentrations of banana juice and ammonium chloride needed to maximize the PHB-producing biomass concentration. The process was then carried out in a 3 L fed-batch fermentation system that included an initial stage of biomass growth. Banana juice was used as the carbon source and fructose pulses were added to maintain the test sugar concentrations of 30, 40, and 50 g/L. The control strain, Cupriavidus necator (ATCC 17699), produced 2.816 g/L of PHB, while productivity of the most promising isolate, C. necator (CR-12), was 0.495 g/L. Maximum biomass production was obtained using 5% banana juice and 2 g/L ammonium chloride. PHB production was not detected in fed-batch fermentations supplemented with 30 or 40 g/L of fructose, while the mean PHB production in fermentations with 50 g/L of fructose was 1.3 g/L.
Introduction
Biotechnological alternatives to conventional plastics are increasingly important in industry worldwide (Sirohi et al., 2020) as they promote sustainability in the agriculture industry and strengthen rural economies (Li and Wilkins, 2021). Some types of biopolymers have valuable characteristics; they are biocompatible and biodegradable by enzymes, microorganisms and fungi present in the environment (Saratale et al., 2019; Sirohi et al., 2020) and can show thermoplastic characteristics with mechanical properties similar to materials derived from petroleum (Naranjo et al., 2014). Unlike biopolymers, petroleum-based products degrade slowly and take 20–100 years to decompose, which results in serious damage to the environment (Mozejko-Ciesielska and Kiewisz, 2016; Amaro et al., 2019; Sirohi et al., 2020). Therefore, there are strong incentives to develop alternative biodegradable materials that decompose rapidly to produce carbon dioxide, water and biomass (Kumar and Kim, 2018; Sirohi et al., 2020).
Polyhydroxybutyrate (PHB), the most common biodegradable homopolymer of polyhydroxyalcanoates (PHA) (Maity et al., 2020), has numerous applications in food, agriculture, medicine and other industries (Li et al., 2017; Sirohi et al., 2020). It naturally accumulates intracellulary in a large number of microorganisms (Oliveira et al., 2004; Mohapatra et al., 2020; Mostafa et al., 2020; Pati et al., 2020; Penkhrue et al., 2020; Nygaard et al., 2021). Some bacteria synthesize PHB as part of the primary metabolism; others, such as Cupriavidus necator, require a nutritional imbalance in the culture media to activate the synthesis metabolism (Grousseau et al., 2014; Getachew and Woldesenbet, 2016; Amini et al., 2020).
The major limitation for commercial use of biopolymers is the high cost of production (Li et al., 2017; Li and Wilkins, 2021). For this reason, several studies have focused on the use of low-cost raw materials from agricultural residues (Sirohi et al., 2020) and fermentation methods that favor competitive production (Shivakumar, 2012; Sallau et al., 2018; Sukruansuwan and Napathorn, 2018; Dalsasso et al., 2019), such as fed batch fermentation processes, which increase the productivity of PHB (Xia et al., 2017; Li and Wilkins, 2020, 2021; Nygaard et al., 2021).
In 2019, Costa Rica exported 120 million boxes of bananas (18.14 kg/box), which reached markets such as the European Union and the United States. The banana industry estimated 328,303 tons of fruit are rejected annually in packing plants (CORBANA, 2019). Rejected fruit has a high content of sugars such as glucose, sucrose and fructose, as well as a variety of minerals, vitamins and amino acids (Chan-Blanco et al., 2003; Guylène et al., 2009) that can be used in fermentative processes.
The objective of this research was to use banana pulp juice, an agroindustrial by-product as the carbon source for PHB production in a submerged fed-batch fermentation.
Materials and Methods
Banana By-Product
The banana by-product, obtained from a pulp production process, was supplied by Compañía Mundimar (Limón, Costa Rica) and consisted of a puree with a high seed content. The material was subjected to enzymatic maceration with Crystalzyme® PMLX (White Labs) to increase the content of fermentable sugars. A pneumatic press was then used to obtain a juice from the banana purée (JBP) with a final concentration of 20° Brix and pH 3.6. Sugar content in JBP was measured by HPLC (Agilent 1260 Infinity). The instrument was equipped with a refractive index detector (RID) (Sullivan and Carpenter, 1993). Mineral content of the substrate was determined by atomic absorption spectrophotometry (AOAC International, 2012b,c,d,e,f). Nitrogen concentration was measured according to AOAC International (2012a).
Bacterial Strains
Eleven native isolates were provided by the Center for Research in Cellular and Molecular Biology of the Universidad de Costa Rica (CIBCM-UCR). These strains are part of a collection generated from samplings in different places in Costa Rica and have been obtained for various research projects of the CIBCM. The strains used in this study were selected from the positive reaction to Nile blue staining, a lipophilic fluorescent dye used to visualize hydrophobic cell structures such as membranes or lipid-like inclusions, like PHB (Juengert et al., 2018). A strain of Cupriavidus necator (ATCC 17699) was used as a control microorganism. The isolates were grown on Trypticase Soy Agar (TSA) (OXOID, CM0131, USA) at 30°C for 24 h. Cultures grown on TSA were used to prepare suspensions in Trypticase Soy Broth (TSB) (OXOID, CM0876, USA) to a concentration equivalent to standard 4 on the McFarland turbidity scale. The suspensions were frozen in 800 μL portions in cryovials with 200 μL 30% sterile glycerol until later use (Tedeschi and De Paoli, 2011).
PHB Extraction
Two mL of cultures were centrifuged at 10,600 rcf for 10 min. The cell pellets were resuspended in 2 mL of distilled water and transferred to 10 mL headspace vials. A volume of 6 mL of an 85:15 mixture of methanol:sulfuric acid (Dalal et al., 2010) was added to each sample. Sealed vials were incubated at 90–95°C for 3 h in a thermostatic bath with agitation for the methanolysis process (Cavalheiro et al., 2009; Dobroth et al., 2011; Wu et al., 2012; Davis et al., 2013). The samples were cooled in an ice bath and 1.5 mL of distilled water and 3 mL of chloroform were added. Samples were shaken vigorously and allowed to settle for phase separation. The organic phase was extracted (the sample was dried over anhydrous sodium sulfate) (Coats et al., 2008), and then filtered through a 0.22 μm filter and transferred to vials for Gas Chromatography (GC).
Quantification of PHB
Methyl (R)-3 hydroxybutyrate (3-MHB) (Sigma®, CAS: 3976-69-0) was used as a standard for PHB quantification (Cavalheiro et al., 2009) while benzoic acid was used as an internal standard (Merk, CAS: 65-85-0) (Coats et al., 2008). The methyl esters of 3-hydroxyalcanoic acid were analyzed by gas chromatography (7820A, Agilent Technologies) coupled to mass detection (5977B MSD Agilent Technologies). Detector was operated on Scan mode, with 70 eV ionization voltage. Two microliters samples were injected into a HP-Innowax column, with a length of 30 m, internal diameter of 0.25 mm and width of 0.25 μm (Çiggin et al., 2009; Davis et al., 2013). The helium flow was 8 mL/min. A 20:1 split relation was used. The temperature of the injector and the detector was 250°C. Temperature was increased from 120 to 220°C at a rate of 3°C/min (Davis et al., 2013).
Evaluation of PHB Production Capacity of Native Strains in Flask Culture
Fermentations were carried out in two stages: a growth stage and a PHB production stage with limited nitrogen (Amirul et al., 2008; El-Sayed et al., 2009; Yang et al., 2010; Garcia-Gonzalez et al., 2015). For the first stage, 1 mL of cryopreserved culture was inoculated to 50 mL of TSB in 250 mL Erlenmyer flasks. Cultures were incubated for 24 h at 30°C and 150 rpm in an orbital shaker (Lab Companion, SI-600, Germany). For the second fermentative phase, cultures were centrifuged at 3,320 rcf for 15 min in order to remove the growth medium, and the resulting cell pellet was resuspended in 50 mL of imbalanced culture media for PHB production described by Khanna and Srivastava (2005) with modifications: Fructose: 40 g/L, NH4Cl: 5 g/L, KH2PO4: 1.5 g/L, NaH2PO4: 4 g/L, MgSO4 * 7H2O: 0.51 g/L, CaCl2: 0.02 g/L, trace solution: 1 mL/L (Trace solution composition [ZnSO4 * 7H2O: 13 mg/L, FeSO4 * 7 H2O: 2 mg/L, (NH4)6Mo7O24 * 4H2O: 6 mg/L, H3BO3: 6 mg/L]. Cultures were incubated at 30°C in an orbital shaker at 150 rpm for 48 h. Each strain was evaluated in triplicate. A Completely Randomized Design (CRD) was used with 12 treatments (11 native isolates and the control). The response variable was PHB concentration determined by GC-MS. Results were analyzed by one factor ANOVA using Microsoft Excel (version 18. 2008. 12711. 0).
Design of Medium Supplemented With JBP for Growth of C. necator
Nitrogen (NH4Cl) and carbon (JBP) concentrations in culture media for C. necator (ATCC 17699) were optimized in order to maximize biomass growth. A 22 Central Composite Rotational Design was used to determine test concentrations of both components, according to Table 1. Four replicates of the central point were executed for statistical parameters calculation. Cell concentration was determined by measuring each culture optical density by spectrophotometry at 425 nm. A Response Surface Analysis was performed using JMP 8 software (SAS Institute Inc.).
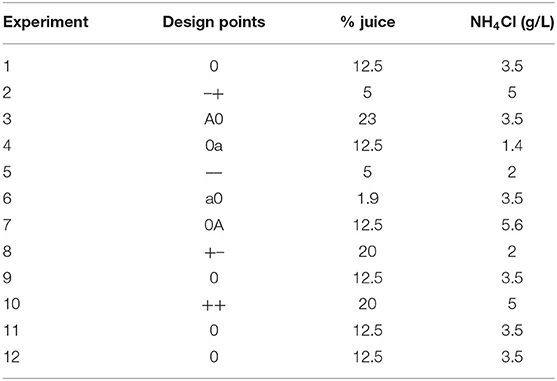
Table 1. JBP percentages and NH4Cl concentrations evaluated by a central composite design for C. necator growth optimization.
For each experiment, 50 mL of culture broth supplemented with the test concentrations of JBP and NH4Cl were prepared in 250 mL erlenmeyer flasks. Besides the test concentrations of NH4Cl and JBP, the media contained 5 g/L NaCl and 2.5 g/L KH2PO4. For each broth formulation, JSB, salts solution and water were combined. pH was adjusted to 7.1 with 1 M NaOH. NH4Cl solution was autoclaved separately and aseptically added to each flask.
Each flask was inoculated with 1 mL of cryopreserved bacterial suspension and incubated at 30°C with 150 rpm agitation for 24 h. The optical density of the culture was measured at the end of the incubation.
PHB Production Process Implementation in Bioreactor
A fed-batch fermentation process was performed in triplicate using the C. necator strain (ATCC 17699). The process was carried out in stirred tank reactors (Applikon® Biotechnology) in a 3 L volume of culture media with the following composition: JBP: 5%; NaCl: 5 g/L; KH2PO4: 4 g/L, NH4Cl: 2 g/L, Na2HPO4 * 2H2O: 4.5 g/L, MgSO4 * 7H2O: 0.51 g/L, CaCl2: 0.02 g/L and 10X trace element solution: 1 mL/L [ZnSO4 * 7H2O: 130 mg/L; FeSO4 * 7H2O: 20 mg/L; (NH4)6Mo7O24 * 4H2O: 60 mg/L; H3BO3: 60 mg/L]. The inoculum was prepared by adding 3 mL cryopreserved culture to 300 mL broth and incubating with shaking at 175 rpm for 24 h at 30°C (Aramvash et al., 2015).
Fermentation parameters were controlled with the ez-Control console and BioXpert® XP software (Applikon® Biotechnology). Culture conditions were: temperature 30°C, agitation 350 rpm, pH 7 adjusted with 2 M NaOH and 100% air saturation at a flow of 1 vvm. The biomass production stage was carried out over 24 h, and was followed by the PHB production stage, initiated by the addition of a fructose pulse (500 g/L), at an agitation speed of 500 rpm. In this stage, the effect of the initial concentration of fructose (30, 40 or 50 g/L) on PHB production was evaluated. Data were analyzed by one-way ANOVA using the program JMP 8 (SAS Institute Inc.). Samples were collected every 24 h; the concentration of PHB was determined by GC-MS, and sugar content was measured by HPLC (Agilent 1260 Infinity). The instrument was equipped with a refractive index detector (RID). A Zorbax Carbohydrate column with dimensions: 5 μm × 150 mm × 4.6 mm was used for the analysis. The following analytical conditions were applied: flow: 1.2 mL/min; oven and detector temperature; 30°C and injection volume: 5 μL Nitrogen concentration was determined by Rapid N- Exceed® Combustion Method according to AOAC International (2016). The biomass dry weight concentration was determined in duplicate using a thermogravimetric analyzer (TGA 701, Leco).
Results
Characterization of JBP
Enzymatic treatment of banana agroindustrial by-product produced a viscous concentrated juice. Chemical characterization showed values for fermentable sugars and minerals that can be used by the microorganism during fermentation. The ratio of sucrose, glucose and fructose in the JBP was 1:3.7:3.6, respectively. The nitrogen content was 0.056/100 g (Table 2).
PHB Production Capacity of Bacterial Strains
PHB production in native strains was not quantifiable (Measured PHB concentrations stood between detection and quantification limits defined for the method), except for CR-12 which showed a concentration of 0.5 g PHB/L culture broth. The control strain produced 2.82 g/L of culture broth. According to ANOVA, significant differences (p < 0.05) were found for PHB production means between the native strain CR-12 and control strain.
Design of JBP-supplemented Medium for Growth of C. necator
The effects of JBP (p < 0.001) and NH4Cl (p < 0.001) concentrations, as well as both components interaction (p = 0.0464) was significant on C. necator cell growth, with a greater influence of JBP on optical density (Figure 1). The biomass concentration increased as levels of NH4Cl and JBP decreased, as shown in Figure 2. Biomass concentration (OD) presented a linear behavior with an R2 value of 0.99 (Table 3). Based on these results, concentrations of 2 g/L of NH4Cl and 5% JBP were selected for use in fermentation experiments.

Figure 1. Sorted parameter estimates for the response surface analysis: effect of JBP and NH4Cl concentrations in culture medium and their interaction in C. necator cell density. *marks statistically significant factors for p < 0.05.
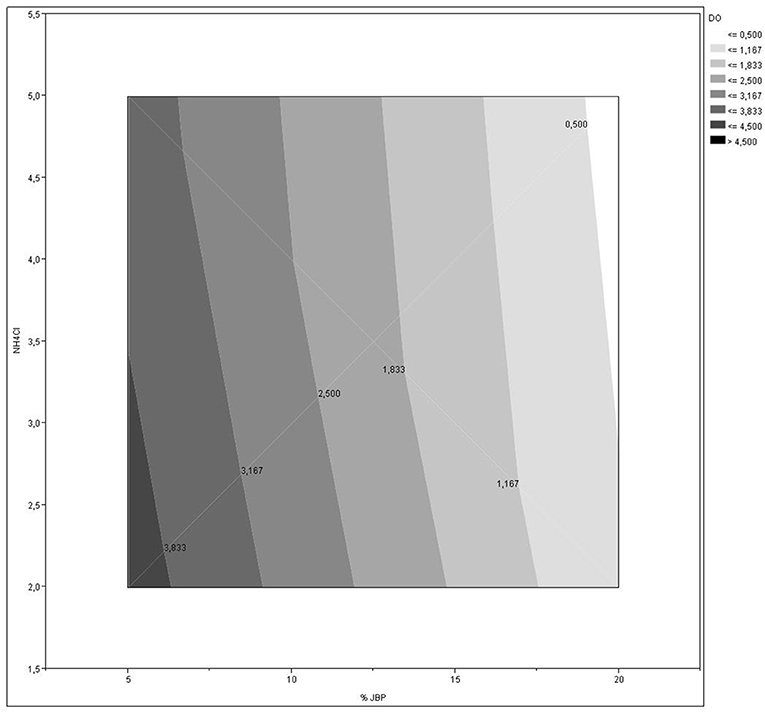
Figure 2. Effect of different JBP and NH4Cl supplementation levels in growth medium on of C. necator cell concentration, measured as optical density at 425 nm.
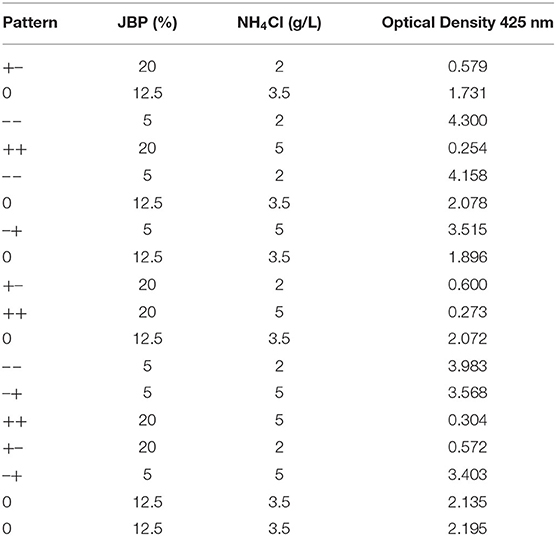
Table 3. Optical Density at 425 nm of C. necator in different formulations of biomass production culture media.
PHB Production Process Implementation in Bioreactor
Dissolved oxygen (DO) saturation was below 15% during the first stage of exponential growth, while levels of 20–40% were observed in the second stage of the process. Oxygen levels remained near zero during the first hours of fermentation. At the beginning of the fermentations, the fructose concentration was 2 g/L. After 25 h of culture, this value reached 50 g/L due to the fructose pulse added to initiate the PHB production stage. The sugar content then decreased and was completely depleted by the time the process reached 72 h. Little change in nitrogen concentration was observed at the end of the growth phase of the microorganism (Figure 3).
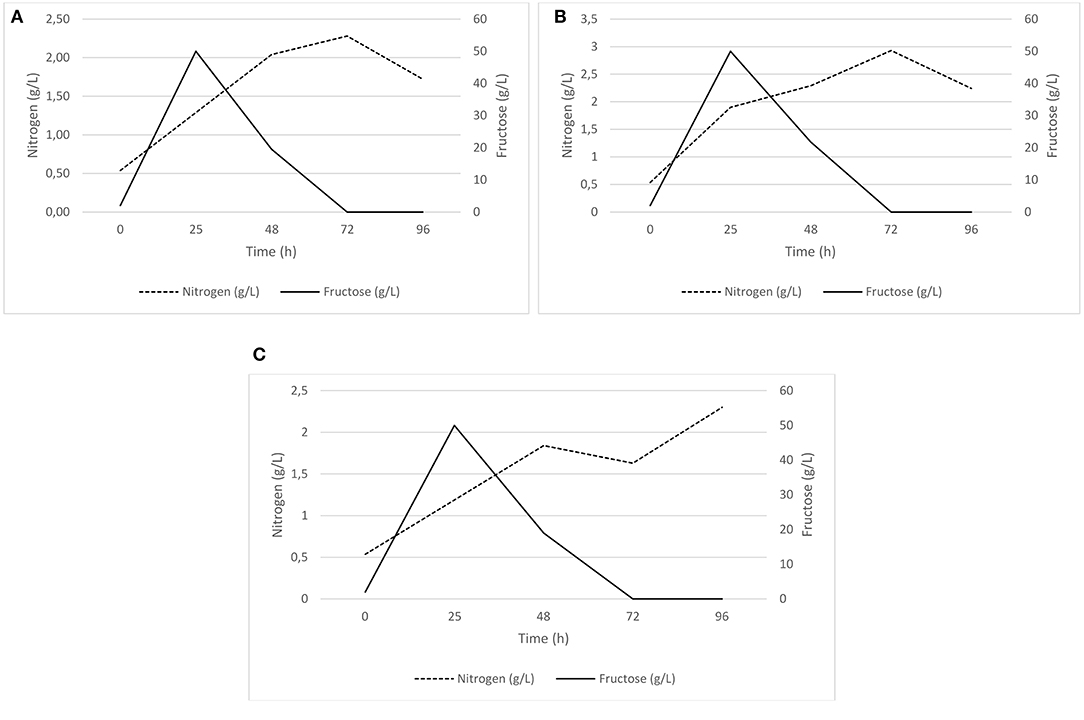
Figure 3. Fructose and nitrogen levels during fermentation supplemented with 50 g/L fructose (A) Repetition 1, (B) Repetition 2, (C) Repetition 3.
A biomass concentration of 24.42 ± 2.25 g/L was obtained at the end of the first fermentation stage. For fermentations where 30 and 40 g/L fructose were tested, PHB production was not detectable at any of the sampling times (24, 48, 72, and 96 h). A maximum PHB production of 1.3 g/L was reached after 96 h of fermentation for the 50 g/L fructose assay. In such experiments, C/N ratio was roughly 10, while C/N ratio obtained in fermentations with 30 and 40 g/L fructose was lower than 10.
In addition to methyl (S)-(+)-3-hydroxybutyrate (PHB) signal, various fatty acids and carboxylic acids were also identified (Table 4).
Discussion
Agro-industrial by-products can contain internal structures such as starches, cellulose and complex sugars that are difficult to transform directly by microorganisms into products of interest (Kulkarni et al., 2015; Sirohi et al., 2020). The enzymatic maceration pretreatment applied to the banana pulp allowed the conversion of the substrate into simple sugars molecules, primarily glucose (Velásquez-Arredondo et al., 2010) and fructose, as well as an increment on proteins and polysaccharides accessibility (Naranjo et al., 2014; Tsang et al., 2019), and facilitated the development of fermentation processes for PHB production. Even though the substrate enzymatic treatment increase the process costs, they are preferred over chemical treatments because the latter generate unwanted secondary compounds (e.g., furfural) that negatively affect the development and metabolism of the microorganisms during fermentation (Lu and Zhong, 2020).
Regarding the native strains PHB production evaluation, the absence of this polymer in the majority such strains may be related to the individual nutritional requirements of the isolates. The optimization of production media is specific for the microorganism used (Pereira et al., 2021), this study used a base medium described for C. necator (suitable for control and CR-12 strains). The other strains may have been inhibited by components in the medium or may require absent nutrients from the utilized base medium for their metabolism proper functioning. PHB production with the native isolate CR-12 and the control strain was lower than that reported by other authors; Nygaard et al. (2019) obtained 4.6 g/L PHB using C. necator strain ATCC 17967 and Zhila et al. (2015) reported values of 7.2–7.7 g/L with strain B-10646 (C. eutrophus) in synthetic media in a two stage fermentation process. However, the PHB concentration of the control strain was higher than the maximum production of 1.62 g/L reported by Sen et al. (2019).
The concentrations of 2 g/L NH4Cl and 5% JBP were optimum within the experimental range. However, results of the study indicate that higher biomass production may be obtained by widening the experimental range to include lower concentrations of these two nutrients. For C. necator, some reports indicate that biomass production is maximized when glucose is used as the fermentation substrate, although other types of sugars such as fructose and xylose have also generated good results (Poomipuk et al., 2014). Given that the sugars in JBP are primarily fructose and glucose, this substrate was expected to maximize cell growth of the microorganism. The C/N ratio in the supplemented culture media was 5.3 based on the concentration and molar mass of glucose, fructose and NH4Cl. This value is below the range reported by Huschner et al. (2015); however, reducing the nitrogen content may result in a higher biomass concentration.
During fermentation processes implementation for PHB production, oxygen is an important parameter. C. necator biomass and PHB production have been observed to increase when the concentration of dissolved oxygen is maintained above 15% during the exponential growth phase of the microorganism in a fed-batch system (Cavalheiro et al., 2009). The effect of DO concentration on PHA production by C. necator has also been studied. Both the total concentration of PHAs and the proportion in which the polymers accumulate intracellularly were incremented when DO saturation was increased to 20% in the production phase, compared with the same process using a DO saturation of 2% (Cavalheiro et al., 2012). For all fructose treatments, the DO saturation increased in the second half of the process, and reached 20–40% for most of the consulted studies (Cavalheiro et al., 2009; Atlić et al., 2011; Passanha et al., 2013; Grousseau et al., 2014; Huschner et al., 2015; Dalsasso et al., 2019). In order to obtain PHAs, cultures with a high cell density are required. The biomass concentration in this study was 24.42 ± 2.25 g/L. Biomass values of 27 g/L have been obtained using complex carbon sources from agro-industrial by-products (Atlić et al., 2011; Cavalheiro et al., 2012).
In general, fructose is the most utilized carbon source for C. necator; a better PHB production is usually achieved by using this source than with glucose or other more complex sources such as molasses (Baei et al., 2011). In preliminary experiments, PHB production was higher using this sugar as a carbon source (data not shown). Ienczak et al. (2013) obtained PHA yields of 68% (dry weight) when the biomass concentration was 50 g/L in a fed-batch system using glucose and fructose. For PHB production, the carbon source must be maintained at a high concentration with considerably limited nitrogen. In this research, maximum PHB production was 1.3 g/L at 96 h. This concentration is lower than that reported by other authors under similar conditions of oxygen saturation (Cavalheiro et al., 2009; Atlić et al., 2011; Passanha et al., 2013; Grousseau et al., 2014; Huschner et al., 2015; Dalsasso et al., 2019), however, it is close to the concentration of 3.3 g/L reported by Li and Wilkins (2020) and 1.98 g/L obtained by Amini et al. (2020). A possible explanation for these results is the rapid consumption of fructose in the culture medium, which stimulates consumption of the polymer by the bacterial metabolism (Poomipuk et al., 2014). For fed-batch fermentations, PHB productivity was increased by replacing the single sugar supplement with several pulses of added sugar to maintain a relatively stable sugar concentration throughout the fermentation (Nygaard et al., 2021).
In this research, a roughly constant level of nitrogen was maintained; this may have prevented the C/N imbalance necessary for high PHB yields, or PHB synthesis may have been inhibited by the presence of nitrogen (Wang and Yu, 2007; Nygaard et al., 2021). The C/N ratio may explain the non-detectable levels of PHB in fermentations with fructose concentrations of 30 and 40 g/L. Huschner et al. (2015) found important percentages of PHAs using C/N ratios between 50 and 580, and obtained maximum productivity of C. necator ATCC 17699 at 48 h of culture using a C/N ratio of 90. Nygaard et al. (2019) considered the effect of phosphorous on PHB production and optimized culture media composition for C. necator ATCC 17967, maximum polymer production was 4.6 g/L when a C/N ratio of 10 was used.
Signals of other compounds have been detected during PHB analysis by GC-MS. Werker et al. (2008) suggested that these compounds were products of alcoholysis (or in the case of the current study, butanolysis), or were by-products of the degradation of the cell biomass or microbial metabolites. These authors used the NIST database to tentatively identify the signals for levulinic acid and the succinic acid dibutyl ester, compounds derived from polysaccharides present in the bacterial biomass and from microbial metabolism, respectively. As shown in Table 4, these two compounds were found in the fermentations as methyl ester and dimethyl ester, respectively. It is possible that the levulinic acid detected in various chromatograms was derived from microbial metabolism of the simple sugar used, or from the degradation of the C. necator biomass; levulinic acid can be used as a precursor for the synthesis of biopolymers (Sirohi et al., 2020).
Biosynthesis of PHAs and fatty acids share the same metabolic route with acetyl-CoA and propionyl-CoA as common intermediates. Thus, both routes compete for precursors, especially in nutrient-limited conditions. The mechanisms to explain this competition have not yet been elucidated, and further research is needed to be able to direct the synthesis process toward the component of interest, obtain high PHA productivities and control precisely the composition of these polymers (Magdouli et al., 2015). Consequently, it is possible that the fatty acids detected by GC-MS correspond to products of this metabolic route whose synthesis was favored over that of PHAs.
Data Availability Statement
The raw data supporting the conclusions of this article will be made available by the authors, without undue reservation.
Author Contributions
CV-C contributed to obtaining the funds for the study. CV-C and MA-R did the conception and design of the study. MA-R and VM-V executed all the bacterial culture experiments to produce PHB, both by shaker flask and bioreactor, they extracted and determined the concentration of PHB by GC. All authors contributed to manuscript revision, read, and approved the submitted version.
Funding
This work was funded by the Seventh Framework Program of the European Union and was part of the TRANSBIO Project: Biotransformation of by-products from fruit and vegetable processing industry into valuable bioproducts and had the support of the University of Costa Rica.
Conflict of Interest
The authors declare that the research was conducted in the absence of any commercial or financial relationships that could be construed as a potential conflict of interest.
Acknowledgments
The authors are thankful to the project collaborators (M. Mora, E. Thompsom, N. Guzman), F. Granados (CINA-UCR), the CITA-UCR chemistry laboratory work team and N. Barboza for her contribution in the revision of this document.
References
Amaro, T. M. M. M., Rosa, D., Comi, G., and Iacumin, L. (2019). Prospects for the use of whey for polyhydroxyalkanoate (PHA) production. Front. Microbiol. 10, 1–12. doi: 10.3389/fmicb.2019.00992
Amini, M., Yousefi-Massumabad, H., Younesi, H., Abyar, H., and Bahramifar, N. (2020). Production of the polyhydroxyalkanoate biopolymer by Cupriavidus necator using beer brewery wastewater containing maltose as a primary carbon source. J. Environ. Chem. Eng. 8:103588. doi: 10.1016/j.jece.2019.103588
Amirul, A. A., Yahya, A. R. M., Sudesh, K., Azizan, M. N. M., and Majid, M. I. A. (2008). Biosynthesis of poly(3-hydroxybutyrate-co-4-hydroxybutyrate) copolymer by Cupriavidus sp. USMAA1020 isolated from Lake Kulim, Malaysia. Explor. Horizons Biotechnol. Global Venture 99, 4903–4909. doi: 10.1016/j.biortech.2007.09.040
AOAC International (2012b). “Method 985.35., Minerals in infant formula, enteral products and pet foods,”. in Official Methods of Analysis of AOAC International, 20ath Edn., ed G. W. Latimer Jr (Gaithersburg, MD: AOAC International), 3172
AOAC International (2016). “Method 992.15.06,” in Official Methods of Analysis of AOAC International, 20th Edn., ed G. W. Latimer Jr (Rockville, MD: AOAC International), 3172.
Aramvash, A., Akbari Shahabi, Z., Dashti Aghjeh, S., and Ghafari, M. D. (2015). Statistical physical and nutrient optimization of bioplastic polyhydroxybutyrate production by Cupriavidus necator. Int. J. Environ. Sci. Technol. 12, 2307–2316. doi: 10.1007/s13762-015-0768-3
Atlić, A., Koller, M., Scherzer, D., Kutschera, C., Grillo-Fernandes, E., Horvat, P., et al. (2011). Continuous production of poly([R]-3-hydroxybutyrate) by Cupriavidus necator in a multistage bioreactor cascade. Appl. Microbiol. Biotechnol. 91, 295–304. doi: 10.1007/s00253-011-3260-0
Baei, S., Najafpour, G. D., Younesi, H., Tabandeh, F., Issazadeh, H., and Khodabandeh, M. (2011). Growth kinetic parameters and biosynthesis of polyhydroxybutyrate in Cupriavidus necator DSMZ 545 on selected substrates. Chem. Indus. Chem. Eng. Q. 17, 1–8. doi: 10.2298/CICEQ100216043B
Cavalheiro, J. M. B. T., de Almeida, M. C. M. D., Grandfils, C., and da Fonseca, M. M. R. (2009). Poly(3-hydroxybutyrate) production by Cupriavidus necator using waste glycerol. Process Biochem. 44, 509–515. doi: 10.1016/j.procbio.2009.01.008
Cavalheiro, J. M. B. T., Raposo, R. S., de Almeida, M. C. M. D., Teresa Cesário, M., Sevrin, C., Grandfils, C., et al. (2012). Effect of cultivation parameters on the production of poly(3-hydroxybutyrate-co-4-hydroxybutyrate) and poly(3-hydroxybutyrate-4-hydroxybutyrate-3-hydroxyvalerate) by Cupriavidus necator using waste glycerol. Bioresour. Technol. 111, 391–397. doi: 10.1016/j.biortech.2012.01.176
Chan-Blanco, Y., Bonilla-Leiva, A. R., and Velázquez, A. C. (2003). Using banana to generate lactic acid through batch process fermentation. Appl. Microbiol. Biotechnol. 63, 147–152. doi: 10.1007/s00253-003-1374-8
Çiggin, A. S., Karahan, Ö., and Orhon, D. (2009). Effect of high nitrate concentration on PHB storage in sequencing batch reactor under anoxic conditions. Bioresour. Technol. 100, 1376–1382. doi: 10.1016/j.biortech.2008.08.036
Coats, E. R., Loge, F. J., Wolcott, M. P., Englund, K., and McDonald, A. G. (2008). Production of natural fiber reinforced thermoplastic composites through the use of polyhydroxybutyrate-rich biomass. Bioresour. Technol. 99, 2680–2686. doi: 10.1016/j.biortech.2007.03.065
Dalal, J., Sarma, P. M., Lavania, M., Mandal, A. K., and Lal, B. (2010). Evaluation of bacterial strains isolated from oil-contaminated soil for production of polyhydroxyalkanoic acids (PHA). Pedobiologia 54, 25–30. doi: 10.1016/j.pedobi.2010.08.004
Dalsasso, R. R., Pavan, F. A., Bordignon, S. E., Aragão, G. M. F., and de Poletto, P. (2019). Polyhydroxybutyrate (PHB) production by Cupriavidus necator from sugarcane vinasse and molasses as mixed substrate. Process Biochem. 85, 12–18. doi: 10.1016/j.procbio.2019.07.007
Davis, R., Kataria, R., Cerrone, F., Woods, T., Kenny, S., O'Donovan, A., et al. (2013). Conversion of grass biomass into fermentable sugars and its utilization for medium chain length polyhydroxyalkanoate (mcl-PHA) production by Pseudomonas strains. Bioresour. Technol. 150, 202–209. doi: 10.1016/j.biortech.2013.10.001
Dobroth, Z. T., Hu, S., Coats, E. R., and McDonald, A. G. (2011). Polyhydroxybutyrate synthesis on biodiesel wastewater using mixed microbial consortia. Bioresour. Technol. 102, 3352–3359. doi: 10.1016/j.biortech.2010.11.053
El-Sayed, A., Azhar, H. M., Abdelhady, A., Abdel, H., and Khodair, T. (2009). Batch production of polyhydroxybutyrate (PHB) by Ralstonia eutropha and alcaligenes latus using bioreactor different culture strategies. J. Appl. Sci. Res. 5, 556–564.
Garcia-Gonzalez, L., Mozumder, M. d. S. I., Dubreuil, M., Volcke, E. I. P., and De Wever, H. (2015). Sustainable autotrophic production of polyhydroxybutyrate (PHB) from CO2 using a two-stage cultivation system. Biomass Valorization Fuels Energy Mater. Chem. 257, 237–245. doi: 10.1016/j.cattod.2014.05.025
Getachew, A., and Woldesenbet, F. (2016). Production of biodegradable plastic by polyhydroxybutyrate (PHB) accumulating bacteria using low cost agricultural waste material. BMC Res Notes 9, 1–9. doi: 10.1186/s13104-016-2321-y
Grousseau, E., Blanchet, E., Déléris, S., Albuquerque, M. G. E., Paul, E., and Uribelarrea, J.-L. (2014). Impact of sustaining a controlled residual growth on polyhydroxybutyrate yield and production kinetics in Cupriavidus necator. Bioresour. Technol. 148, 30–38. doi: 10.1016/j.biortech.2013.08.120
Guylène, A., Parfait, B., and Fahrasmane, L. (2009). Bananas, raw materials for making processed food products. Trends Food Sci. Technol. 20, 78–91. doi: 10.1016/j.tifs.2008.10.003
Huschner, F., Grousseau, E., Brigham, C. J., Plassmeier, J., Popovic, M., Rha, C., et al. (2015). Development of a feeding strategy for high cell and PHA density fed-batch fermentation of Ralstonia eutropha H16 from organic acids and their salts. Process Biochem. 50, 165–172. doi: 10.1016/j.procbio.2014.12.004
Ienczak, J. L., Schmidell, W., and de Aragão, G. M. F. (2013). High-cell-density culture strategies for polyhydroxyalkanoate production: a review. J. Indus. Microbiol. Biotechnol. 40, 275–286. doi: 10.1007/s10295-013-1236-z
Juengert, J. R., Bresan, S., and Jendrossek, D. (2018). Determination of polyhydroxybutyrate (PHB) content in Ralstonia eutropha using gas chromatography and nile red staining. Bioprotocol 8:e2748. doi: 10.21769/BioProtoc.2748
Khanna, S., and Srivastava, A. K. (2005). Recent advances in microbial polyhydroxyalkanoates. Process Biochem. 40, 607–619. doi: 10.1016/j.procbio.2004.01.053
Kulkarni, S. O., Kanekar, P. P., Jog, J. P., Sarnaik, S. S., and Nilegaonkar, S. S. (2015). Production of copolymer, poly (hydroxybutyrate-co-hydroxyvalerate) by Halomonas campisalis MCM B-1027 using agro-wastes. Int. J. Biol. Macromol. 72, 784–789. doi: 10.1016/j.ijbiomac.2014.09.028
Kumar, P., and Kim, B. S. (2018). Valorization of polyhydroxyalkanoates production process by co-synthesis of value-added products. Bioresour. Technol. 269, 544–556. doi: 10.1016/j.biortech.2018.08.120
Li, M., and Wilkins, M. (2020). Fed-batch cultivation and adding supplements to increase yields of polyhydroxybutyrate production by Cupriavidus necator from corn stover alkaline pretreatment liquor. Bioresour. Technol. 299:122676. doi: 10.1016/j.biortech.2019.122676
Li, M., and Wilkins, M. R. (2021). Fed-batch polyhydroxybutyrate production by Paraburkholderia sacchari from a ternary mixture of glucose, xylose and arabinose. Bioprocess Biosyst. Eng. 44, 185–193. doi: 10.1007/s00449-020-02434-1
Li, T., Elhadi, D., and Chen, G. Q. (2017). Co-production of microbial polyhydroxyalkanoates with other chemicals. Metab. Eng. 43, 29–36. doi: 10.1016/j.ymben.2017.07.007
Lu, Z.-Y., and Zhong, J.-J. (2020). Effect of furfural addition on validamycin-A production in fermentation of Streptomyces hygroscopicus 5008. Process Biochem. 92, 43–48. doi: 10.1016/j.procbio.2020.03.002
Magdouli, S., Brar, S. K., Blais, J. F., and Tyagi, R. D. (2015). How to direct the fatty acid biosynthesis towards polyhydroxyalkanoates production? Biomass Bioenergy 74, 268–279. doi: 10.1016/j.biombioe.2014.12.017
Maity, S., Das, S., Mohapatra, S., Tripathi, A. D., Akthar, J., Pati, S., et al. (2020). Growth associated polyhydroxybutyrate production by the novel Zobellellae tiwanensis strain DD5 from banana peels under submerged fermentation. Int. J. Biol. Macromol. 153, 461–469. doi: 10.1016/j.ijbiomac.2020.03.004
Mohapatra, S., Pattnaik, S., Maity, S., Sharma, S., Akhtar, J., Pati, S., et al. (2020). Comparative analysis of PHAs production by Bacillus megaterium OUAT 016 under submerged and solid-state fermentation. Saudi J. Biol. Sci. 27, 1242–1250. doi: 10.1016/j.sjbs.2020.02.001
Mostafa, Y. S., Alrumman, S. A., Otaif, K. A., Alamri, S. A., Mostafa, M. S., and Sahlabji, T. (2020). Production and characterization of bioplastic by polyhydroxybutyrate accumulating Erythrobacter aquimaris isolated from mangrove rhizosphere. Molecules 25:179. doi: 10.3390/molecules25010179
Mozejko-Ciesielska, J., and Kiewisz, R. (2016). Bacterial polyhydroxyalkanoates: still fabulous? Microbiol. Res. 192, 271–282. doi: 10.1016/j.micres.2016.07.010
Naranjo, J. M., Cardona, C. A., and Higuita, J. C. (2014). Use of residual banana for polyhydroxybutyrate (PHB) production: case of study in an integrated biorefinery. Waste Manage. 34, 2634–2640. doi: 10.1016/j.wasman.2014.09.007
Nygaard, D., Yashchuk, O., and Hermida, É. B. (2019). Evaluation of culture medium on poly(3-hydroxybutyrate) production by Cupriavidus necator ATCC 17697: application of the response surface methodology. Heliyon 5:e01374. doi: 10.1016/j.heliyon.2019.e01374
Nygaard, D., Yashchuk, O., Noseda, D. G., Araoz, B., and Hermida, É. B. (2021). Improved fermentation strategies in a bioreactor for enhancing poly(3-hydroxybutyrate) (PHB) production by wild type Cupriavidus necator from fructose. Heliyon 7:e05979. doi: 10.1016/j.heliyon.2021.e05979
Oliveira, F. C., Freire, D. M. G., and Castilho, L. R. (2004). Production of poly(3-hydroxybutyrate) by solid-state fermentation with Ralstonia eutropha. Biotechnol. Lett. 26, 1851–1855. doi: 10.1007/s10529-004-5315-0
Passanha, P., Esteves, S. R., Kedia, G., Dinsdale, R. M., and Guwy, A. J. (2013). Increasing polyhydroxyalkanoate (PHA) yields from Cupriavidus necator by using filtered digestate liquors. Bioresour. Technol. 147, 345–352. doi: 10.1016/j.biortech.2013.08.050
Pati, S., Maity, S., Dash, A., Jema, S., Mohapatra, S., Das, S., et al. (2020). Biocompatible PHB production from Bacillus species under submerged and solid-state fermentation and extraction through different downstream processing. Curr. Microbiol. 77, 1203–1209. doi: 10.1007/s00284-020-01922-7
Penkhrue, W., Jendrossek, D., Khanongnuch, C., Pathomareeid, W., Aizawa, T., Behrens, R. L., et al. (2020). Response surface method for polyhydroxybutyrate (PHB) bioplastic accumulation in Bacillus drentensis BP17 using pineapple peel. PLoS ONE 15:e0230443. doi: 10.1371/journal.pone.0230443
Pereira, J. R., Araújo, D., Freitas, P., Marques, A. C., Alves, V. D., Sevrin, C., et al. (2021). Production of medium-chain-length polyhydroxyalkanoates by Pseudomonas chlororaphis subsp. Aurantiaca: cultivation on fruit pulp waste and polymer characterization. Int. J. Biol. Macromol. 167, 85–92. doi: 10.1016/j.ijbiomac.2020.11.162
Poomipuk, N., Reungsang, A., and Plangklang, P. (2014). Poly-β-hydroxyalkanoates production from cassava starch hydrolysate by Cupriavidus sp. KKU38. Int. J. Biol. Macromol. 65, 51–64. doi: 10.1016/j.ijbiomac.2014.01.002
Sallau, A. B., Salim, B., and Salihu, A. (2018). Assessment of bioplastic producing potential of Bacillus subtilis using some agricultural residues as carbon source. Sci. J. Univ. Zakho 6, 42–45. doi: 10.25271/2018.6.2.427
Saratale, R. G., Saratale, G. D., Cho, S. K., Kim, D. S., Ghodake, G. S., Kadam, A., et al. (2019). Pretreatment of kenaf (Hibiscus cannabinus L.) biomass feedstock for polyhydroxybutyrate (PHB) production and characterization. Bioresour. Technol. 282, 75–80. doi: 10.1016/j.biortech.2019.02.083
Sen, K. Y., Hussin, M. H., and Baidurah, S. (2019). Biosynthesis of poly(3-hydroxybutyrate) (PHB) by Cupriavidus necator from various pretreated molasses as carbon source. Biocatal. Agricul. Biotechnol. 17, 51–59. doi: 10.1016/j.bcab.2018.11.006
Shivakumar, S. (2012). Polyhydroxybutyrate (PHB) production using agro-industrial residue as substrate by Bacillus thuringiensis IAM 12077. Int. J. ChemTech Res. 4, 1158–1162.
Sirohi, R., Prakash, P. J., Kumar, G. V., Gnansounou, E., and Sindhu, R. (2020). Critical overview of biomass feedstocks as sustainable substrates for the production of polyhydroxybutyrate (PHB). Bioresour. Technol. 311:123536. doi: 10.1016/j.biortech.2020.123536
Sukruansuwan, V., and Napathorn, S. C. (2018). Use of agro-industrial residue from the canned pineapple industry for polyhydroxybutyrate production by Cupriavidus necator strain A-04. Biotechnol. Biofuels 11, 1–15. doi: 10.1186/s13068-018-1207-8
Sullivan, D., and Carpenter, D. (1993). AOAC International, Methods of Analysis for Nutrition Labeling Arlington, VA: AOAC International.
Tedeschi, R., and De Paoli, P. (2011). “Collection and Preservation of Frozen Microorganisms,” in Methods in Biobanking. Methods in Molecular Biology (Methods and Protocols). Vol. 675 (Totowa, NJ: Humana Press), 313–326.
Tsang, Y. F., Kumar, V., Samadar, P., Yang, Y., Lee, J., Ok, Y. S., et al. (2019). Production of bioplastic through food waste valorization. Environ. Int. 127, 625–644. doi: 10.1016/j.envint.2019.03.076
Velásquez-Arredondo, H. I., Ruiz-Colorado, A. A., and De Oliveira, S. (2010). Ethanol production process from banana fruit and its lignocellulosic residues: energy analysis. Energy 35, 3081–3087. doi: 10.1016/j.energy.2010.03.052
Wang, J., and Yu, H.-Q. (2007). Biosynthesis of polyhydroxybutyrate (PHB) and extracellular polymeric substances (EPS) by Ralstonia eutropha ATCC 17699 in batch cultures. Appl. Microbiol. Biotechnol. 75, 871–878. doi: 10.1007/s00253-007-0870-7
Werker, A., Lind, P., Bengtsson, S., and Nordström, F. (2008). Chlorinated-solvent-free gas chromatographic analysis of biomass containing polyhydroxyalkanoates. Water Res. 42, 2517–2526. doi: 10.1016/j.watres.2008.02.011
Wu, S. C., Liou, S. Z., and Lee, C. M. (2012). Correlation between bio-hydrogen production and polyhydroxybutyrate (PHB) synthesis by Rhodopseudomonas palustris WP3-5. Challenges Environ. Sci. Eng. 113, 44–50. doi: 10.1016/j.biortech.2012.01.090
Xia, J., Li, R., He, A., Xu, J., Liu, X., Li, X., et al. (2017). Production of poly(B-L-malic acid) by Aureobasidium pullulans HA-4D under solid-state fermentation. Bioresour. Technol. 244, 289–295. doi: 10.1016/j.biortech.2017.07.148
Yang, Y. H., Brigham, C. J., Budde, C., Boccazzi, P., Willis, L. B., Hassan, M. A., et al. (2010). Optimization of growth media components for polyhydroxyalkanoate (PHA) production from organic acids by Ralstonia eutropha. Appl. Microbiol. Biotechnol. 87, 2037–2045. doi: 10.1007/s00253-010-2699-8
Keywords: polyhydroxybutyrate, Cupriavidus necator, banana by-product, agro-industrial wastes, fed-batch fermentation, methanolysis
Citation: Arias-Roblero M, Mora-Villalobos V and Velazquez-Carrillo C (2021) Evaluation of Fed-Batch Fermentation for Production of Polyhydroxybutyrate With a Banana Pulp Juice Substrate From an Agro Industrial By-Product. Front. Sustain. Food Syst. 5:681596. doi: 10.3389/fsufs.2021.681596
Received: 16 March 2021; Accepted: 04 May 2021;
Published: 02 June 2021.
Edited by:
Guadalupe Virginia Nevárez-Moorillón, Autonomous University of Chihuahua, MexicoReviewed by:
Nicolas Oscar Soto-Cruz, Durango Institute of Technology, MexicoYejun Han, University of Chinese Academy of Sciences, China
Copyright © 2021 Arias-Roblero, Mora-Villalobos and Velazquez-Carrillo. This is an open-access article distributed under the terms of the Creative Commons Attribution License (CC BY). The use, distribution or reproduction in other forums is permitted, provided the original author(s) and the copyright owner(s) are credited and that the original publication in this journal is cited, in accordance with accepted academic practice. No use, distribution or reproduction is permitted which does not comply with these terms.
*Correspondence: Carmela Velazquez-Carrillo, Y2FybWVsYS52ZWxhenF1ZXpAdWNyLmFjLmNy