- 1Laboratorio de Biotecnología Industrial, Centro de Biotecnología Genómica, Instituto Politécnico Nacional, Reynosa, Mexico
- 2Lab. de Biología Molecular, Innovak Global, Investigación y Desarrollo, Chihuahua, Mexico
- 3Centro de Investigación en Ciencia Aplicada y Tecnología Avanzada del IPN, Instituto Politécnico Nacional, Unidad Querétaro, Querétaro, Mexico
- 4Department of Biology, Texas State University, San Marcos, TX, United States
Tequila and mezcal are both traditional Mexican liquors that are produced from cooked Agave spp. must fermentation and usually rely on spontaneous or pure Saccharomyces cerevisiae strain inoculation. In order to contribute to the rational selection of yeast starters for tequila and mezcal productions, we tested a collection of 25 yeasts originally isolated from mezcal musts, spanning 10 different yeast species. These strains were first characterized in a semi synthetic medium (labeled as M2, having 90 g/L fructose and 10 g/L glucose of initial hexoses) at 48 h of culture, observing a differential pattern in the consumption of sugars and productivity. Selected Saccharomyces strains left around 10 g/L of fructose and showed higher fermentation performance. However, some non-Saccharomyces strains, specifically from Torulospora (Td), Kluyveromyces (Km), and Zygosaccharomyces (Zb) genera, consumed almost all the sugar (i.e., Km1Y9 with <5 g/L) and had a high productivity of ethanol. In general, all Saccharomyces strains presented a high production of ethyl-butyrate, ethyl-decanoate, and ethyl-hexanoate with peaks of 10, 38, and 3 μg/L, respectively. In addition, some Kluyveromyces and Torulospora strains showed a high production of phenyl ethyl acetate (i.e., Km1D5 with up to 1400 μg/L); isoamyl acetate (i.e., Km1D5 and Td1AN2 with more than 300 μg/L), and hexyl acetate (i.e., Td1AN2 with 0.3 μg/L). Representative strains of the most productive genera (Saccharomyces, Torulospora, and Kluyveromyces) were selected to evaluate their fermentative performance and survival in a mixed culture on a medium based on Agave tequilana must, and their population kinetics was characterized using specific fluorescent in situ hybridization (FISH) probes in a qualitative and semi-quantitative analysis during fermentation. We observed that the mixture ratios of 0.1:1:1 or 1:1:1 (Saccharomyces:Kluyveromyces:Torulospora), maintained good fermentation productivities, with alcohol yields above 0.45 g/g, and allowed a high survival rate of the non-Saccharomyces strains during the fermentation process. Finally, mixed inoculum fermentations on A. tequilana must medium, including different Saccharomyces strains and the finally selected Torulospora and Kluyveromyces strains, showed the best production parameters in terms of ethanol, carbon dioxide, glycerol, and acetic acid values, as well as improved volatile metabolite profiles as compared to the pure cultures. All these data were used to propose a methodology of selection of strains to be used as a pure or mixed starter for tequila and mezcal fermentations, with high primary metabolite productivity and desired aromatic profile.
Introduction
The aroma and flavor of alcoholic beverages obtained from Agave spp. plants (commonly known as Agave or maguey) are probably the most complex in the liquor world, due to the fact that they are the result of many volatile compounds that may be contained in the raw material and that vary among Agave species (Vera Guzmán et al., 2009). They are further increased and transformed during the cooking of the Agave fructan-rich tissues, previous to carrying out the fermentation. A comprehensive review of all the aromatic compounds found in different liquors, as well as all the different yeast species detected in these fermented products, including tequila and mezcal, can be found in De la Torre-González et al. (2017). This complex mixture defines the sensory attributes and consumer acceptance of these Agave alcoholic beverages. It has been reported in general that the end of the Agave must alcoholic fermentation process is usually carried out almost exclusively by S. cerevisiae (Cedeño, 1995). However, there is an initial presence of other yeasts (non-Saccharomyces), which are usually naturally co-cultured with S. cerevisiae, which give unique organoleptic characteristics to the products produced during fermentation (Lachance, 1995; De la Torre-González et al., 2017). These non-Saccharomyces yeasts have become increasingly important, since they contribute to the production of compounds that provide the aromatic characteristic to Agave beverages (Amaya-Delgado et al., 2013; González-Robles et al., 2015) and wine (Tufariello et al., 2021). The co-existence of different species or strains in the mezcal fermentative process can have positive effects such as the increase of the hexose consumption and alcohol yields (Nolasco-Cancino et al., 2018). The non-Saccharomyces yeasts have shown potential as starters for the Agave alcoholic beverage industry (Arrizon et al., 2006; Amaya-Delgado et al., 2013; Segura-García et al., 2015; De la Torre-González et al., 2017; Nolasco-Cancino et al., 2018). However, most of the reported kinetics of these consortia of Saccharomyces and non-Saccharomyces yeasts have been analyzed for wine (grape juice), where both yeast populations maintain similar growth rates during the first phases (2–3 days) of fermentation, and then present a reduction in the non-Saccharomyces yeast number as the fermentation time progresses (Pina et al., 2005). Fortunately, an increasing number of reports are slowly unraveling the microbial consortia complexities of Agave fermented beverages (Lachance, 1995; Narváez-Zapata et al., 2010; Verdugo Valdez et al., 2011; Páez-Lerma et al., 2013, Kirchmayr et al., 2017; Nolasco-Cancino et al., 2018). A very specific group of yeasts were obtained from Tamaulipas (Mexico) mezcal fermenting musts (De la Torre-González et al., 2017), which have been characterized in terms of their stress tolerance, particularly, when fermenting on high fructose contents (Oliva Hernández et al., 2013; De la Torre-González et al., 2016; Vergara-Álvarez et al., 2019). This is important due to the fact that cooked musts of Agave spp. plants contain, as fermentable sugar, a high fructose content (90%), fructose oligosaccharides (FOS), and also toxic Maillard compounds, furfural, methanol and saponins, among others (Díaz-Montaño et al., 2008; Arroyo-López et al., 2009; Amaya-Delgado et al., 2013; Nava-Cruz et al., 2014), explaining in part why some of our yeasts have been recently characterized as genomically belonging to a separate, specific domestication event, as compared to other S. cerevisiae strains worldwide (Peter et al., 2018). Also, pure and dual mixes of these mezcal strains have been evaluated during a wine-type fermentation, observing that S. cerevisiae/T. delbrueckii inoculum presented a fruitier aroma profile, showing the feasibility of using these mezcal yeasts as inoculum for wine fermentation (De la Torre-González et al., 2017, 2020). However, the specific behavior of the yeast populations and their viability during fermentation of an actual Agave must, and the application of these mezcal yeasts as pure or mixed inoculum on Agave spp. must fermentations, remained to be conducted. Therefore, in this study we evaluated the fermentative performance of 25 Saccharomyces and non-Saccharomyces mezcal strains on a semi synthetic (M2) medium, to assess and screen the strains, and then on an Agave tequilana must-based medium for the selected yeasts, by using pure and mixed cultures to gain information to propose a minimum characterization methodology to select a starter for Agave must (mainly, tequila and mezcal) fermentative applications.
Materials and Methods
Yeast Strains and Inoculum Growth Conditions
The 25 yeast strains used belong to the mezcal LBI-CBG yeast collection and are conserved in 60% glycerol at −70°C, and the commercial wine strain Saccharomyces cerevisiae Fermichamp (DSM Food Specialties B.V., The Netherlands) was used as a control for its fructophilic character, which is used to reactivate stuck wine fermentations. The strains used are representative of the yeast diversity found in the fermentation of mezcal from Tamaulipas (Mexico), which comprises almost 100 different isolated yeasts, and the studied strains were identified by their 26S rDNA sequences, amplified by using the primers NL1 5′ GCATATCAATAAGCGGGAGGAAAAG 3′ and NL4 5′ GGTCCGTGTTTCAAGACGG3′, reported as adequate for most of the ascomycetous yeasts, which can be reliably identified with their D1/D2 LSU regions (Kurtzman and Robnett, 1998). The D1/D2 LSU gene marker was chosen as it exhibits a lower heterogeneity than the widely used ITS1-ITS2 region, which renders a better species identification (De Filippis et al., 2017) as also suggested by Libkind et al. (2020) in the specific case of yeasts, when comparing the use of these two ribosomal markers with whole-genome analyses. The conditions used for amplification and sequencing of the D1/D2 were reported by Campos-Rivero et al. (2019), as follows: PCR was conducted on a thermocycler (2720 Thermal Cycler; Applied Biosystems, Carlsbad, CA, USA), with a final volume of 25 μl, which consisted of 1.5 mM of MgCl2, 1× of PCR Buffer, 0.8 mM of dNTP, 0.4 μM of each primer, 1.5 U of Taq DNA polymerase, and 10 ng of DNA template. The D1/D2 LSU region (26S rDNA) was amplified with an initial denaturation at 95°C for 10 min, 35 cycles of repeated reactions (denaturation at 94°C for 30 s, annealing at 61°C for 30 s and elongation at 72°C for 1 min) and final elongation of 10 min at 72°C. Amplification was visualized on 1% (w/v) agarose gels stained with SYBR Green (Invitrogen; Thermo Fisher Scientific, Carlsbad, CA, USA). PCR products were purified using Wizard® SV gel and PCR clean-up Kit (Promega Corp., Madison, WI, USA) according to the manufacturer's protocol. D1/D2 LSU amplification products were directly sequenced by using both original primers. Sequencing was performed using an ABI Prism 3130 sequencer (Applied Biosystems). Sequences are deposited on the Genbank and belong to the species S. cerevisiae LCBG- Sc3Y2 (JQ824877), -Sc3Y3 (JQ824872), -Sc3Y4 (JQ824875),- Sc3Y5 (JQ824869), -Sc3Y8 (JQ824874), -ScMosca3 (KT945088), -Sc4Y3 (KT945087), -Sc3D2 (JQ824871), -Sc3D4 (KT945086), -Sc3D5 (KT945085) and -Sc3D6 (JQ824876), Kluyveromyces marxianus strains -Km1D5 (KT945093), -Km4D3 (KT945094) and -Km1Y9 (KT945092), Torulaspora delbrueckii strains -Td1AN1(KT945091), -Td1AN2 (KT945089) and -Td1AN9 (KT945090), Pichia kluyveri strain -Pk4D6 (KT945083), Meyerozyma guilliermondii strain -Pg1Y12 (KT945082), Yamadazyma mexicana Pm1AN3 (KT945081), Candida parapsilosis strains -Cp1Y7 (KT945079), Clavispora lusitaniae strain -Cl4Y4 (KT945080), Rhodotorula mucilaginosa strain -RmP12 (KT945095), and Zygosaccharomyces bailii strain -Zb3Y1 (KT945084) (De la Torre-González et al., 2020). An initial preculture of the tested yeast was grown on YPD agar plates containing 1% yeast extract, 2% peptone, 2% w/w D-glucose, plus 2% bacteriological agar (Difco Laboratories, Franklin Lakes, NJ, USA), all on a w/w basis and incubated at 30°C for 48 h. A loop of this preculture was used as inoculum for liquid YPD medium (3 ml) incubated 24 h at 30°C with shaking at 200 rpm. The optical density of the cultures was determined at 600 nm and the initial inoculum concentration was adjusted using sterile Ringer solution if needed. This active culture was used as the inoculum for a second round of growth on YPD incubated for 48 h at 200 rpm and 30°C before using it as inoculum in the fermentation experiments carried out as described below.
Fermentation Media
All fermentation experiments were carried out in minibioreactor tubes of 50 ml with four-hole vent caps (Corning Science de México, Reynosa, Mexico) containing 20 ml of semi-synthetic medium labeled M2 (Oliva Hernández et al., 2013), to simulate basic composition of sugars of diluted Agave must, and containing per liter: 1 g of yeast extract, 2 g of (NH4)2SO4, 0.4 g of MgSO4·7H2O, 5 g of KH2PO4, 10 g of glucose, and 90 g of fructose. Sugars were autoclaved separately from the rest of the components, and pH was adjusted on the mineral solution to 5 using either concentrated solutions of NaOH or HCl prior to autoclaving. For Agave must-based medium, a freshly collected 8-year-old A. tequilana Weber stem (piña) was ground and mixed with an equal amount of distilled water, and then autoclaved for 1 h at 121°C. The resulting caramelized liquid was filtered, hexose concentration quantified by HPLC as described below, and then diluted with sterile water to an initial hexose concentration of 107 g/L, then supplemented with 2 g/L of (NH4)2SO4 and autoclaved again for 15 min at 121°C. Glucose and fructose concentration in this Agave must medium (and denominated from here on as Agave medium) was 11.6 and 94 g/L, respectively, an approximate glucose/fructose ratio of 1:9. The pure Saccharomyces inoculum used was 3 × 106 cells/ml. Inoculum for fermentations used for FISH analysis consisted of one Saccharomyces and two non-Saccharomyces strains in two proportions: 0.1:1:1 and 1:1:1, respectively. Inoculum in mixed cultures for microbiological enumeration was 3 × 104 cells/ml in a 0.1:1 dilution of Saccharomyces and non-Saccharomyces strains, to allow the sampling and processing directly without dilution for the microscopical FISH analysis. Incubation was performed at 30°C using an agitated incubator at 200 rpm. Each experiment was run in triplicate for each experimental point, and the measurements for each minibioreactor were performed at least two times.
Biomass and CO2 Production Quantification
Biomass was quantified as dry weight by centrifuging 2 ml of each sample in pre-weighed 2 ml Eppendorf tubes; the supernatant was recovered and filtered through a 0.45 μm membrane for further HPLC analysis, and the tubes containing the biomass pellet were stove-dried at 60°C overnight, placed in a silica-gel glass dessicator for at least 4 h, and then weighed. Biomass production was calculated as the difference in the weight of the tube divided by the volume of the centrifuged sample. Duplicate samples were taken from each of the three minibioreactor tubes that comprised one experimental sampling point. The production of carbon dioxide per liter was followed by weighing each minibioreactor every 24 h and comparing with the initial weight after considering the loss of weight due to evaporation, which was measured to be 0.0034 g/h (R2 = 0.9998) per hole in the vent cap under the conditions used in this work: 20 ml of M2 or Agave medium, 30°C and 200 rpm.
Sugar Consumption and Metabolite Quantification by HPLC
The residual concentration (consumption) of hexoses (D-glucose and D-fructose) and the production of primary metabolites (ethanol, glycerol, and acetic acid) in the centrifuged and filtered sample supernatants were measured by HPLC as reported by Narváez-Zapata et al. (2010) by triplicate using an Agilent 1100 series HPLC equipment, coupled to an autosampler and using a BioRadTM Aminex HPX (30 × 7.8 ml) column. The mobile phase was H2SO4 0.005 M. The volume of the injection loop was 25 μl with each run lasting around 30 min with a flow rate of 0.5 ml/min at 35°C. The peaks were detected by IRD or UVD at 190 nm. Calibration curves were constructed using ethanol, glycerol, acetic acid, fructose, and glucose standards ranging from 0.125 to 20 g/L, using linear regression with a Pearson coefficient of not less than 0.95 were chosen.
Mixed Yeasts Populations Quantification by Fish
The specific detection of main yeast species was carried out microscopically by using fluorescence in situ hybridization microscopy technique (FISH). This method allows the quantification of complex mixed populations (two or more strains) by using a combination of species-specific probes and universal probes based on D1/D2 region of the 26S rDNA (Xufre et al., 2006). For this study, the hybridization of the species-specific probes was optimized for Sc (5′-TGACTTACGTCGCAGTCC-3′), Td (5′-GCAGTATTTCTACAGGAT-3′), and Km (5′-AGCTACAAAGTCGCCTTC-3′) for S. cerevisiae, T. delbrueckii and K. marxianus, respectively. Universal EUK probe (5′-ACCAGACTTGCCCTCC-3′), designed in general for eucaria, was also used (Xufre et al., 2006). The isolates were aerobically activated in YM medium (20 g/L of glucose, 5 g/L of yeast extract, and 3 g/L of peptone), under stirring conditions of 250 rpm at 25°C. After the fermentation was carried out on M2 or on Agave medium, 1 μl of sample was taken and deposited on the slide, previously covered with gelatin (0.1%) and 0.01% of KCr (SO4)2, and it was left to dry at room temperature for 40 min. Subsequently, dehydration was carried out with EtOH at three different concentrations (50, 70, and 96%) for a period of 3 min in each one. Then, samples were dried on environmental temperature during 20 min. During this time the hybridization solution was prepared, which consisted of 70% of (NaCl 0.9 M, Na2EDTA 5 mM, Tris/HCl 20 mM pH 7, SDS 0.01%) and 70% of formamide; 9 μl was taken from this solution for every 1 μl of probe, then 10 μl was placed on the already dehydrated and dried sample to be placed in a 50-ml falcon tube to be hybridized in the oven at 42°C from 1 to 4 h. Once the hybridization was finished, a careful washing was given with distilled water, and it was subjected to a washing buffer (0.9M NaCl, 5 mM Na2EDTA, 20mM Tris/HCl pH 7, 0.01% SDS) for 15 min. Once the buffer wash was finished, another wash was given with distilled water, and it was left to dry at room temperature. Once the slide was dry, a few drops of Citifluor® oil (Cargille Laboratories, Cedar Grove, NJ, USA) were placed on the slide to avoid oxidation of the sample when in contact with UV light. Finally, the visualization of the fluorescent detection under the 100× objective was carried out using an Olympus Microscope (Olympus BX51; Olympus, Tokyo, Japan) with a BX-URA2 reflector illuminator, the BX-RFA fluorescence illuminator with filter U-RSL6EM (U-RSL6), U-MWIG2 filters (maximum excitation 552 nm and maximum emission 565 nm) for probes with Cy3 labeling, and U-MNUA2 filter (maximum excitation 372 nm and maximum emission 456 nm) for DAPI (4′, 6-diamidino-2-phenyl indole dilactate). The microscope had an RGB infinity camera attached to the trinocular connected to a personal computer with the Image-Pro Plus version 6.3 analysis and image processing program (Media Cybernetics, Rockville, MD, USA). The summatory of the area of the cells present in 30 randomly selected fields of view were measured by duplicate for each slide with the different probes, and expressed as squared micrometers of cells, throughout the duration of the fermentations. It is worth commenting that after 48 h, the samples presented a diminished fluorescence (as also observed by Andorra et al., 2011); nevertheless, it did not interfere with the area measurement.
Mixed Yeasts Populations Quantification by Differential Plate Counts
A second population analysis, considering standard microbiological enumerations on these mixed cultures, was also conducted, by enumeration of the culturable yeasts in mixed cultures by using WL Differential and Nutrient Agars (Sigma-Aldrich, St. Louis, MO, USA). The count plating technique allows the differentiation of Saccharomyces and non-Saccharomyces colony populations and their quantification, which in turn allows the whole culturable yeast population determination, as established in De la Torre-González et al. (2016), based on the work of Hedges (2002). Limitations of the differential WL media include that it was only reliably useful to determine unequivocally dual mixed cultures of Saccharomyces and non-Saccharomyces strains (De la Torre-González et al., 2020) due to the indistinguishable appearance of Torulaspora delbrueckii and Kluyveromyces marxianus colonies used in this work. In parallel, total and viable cell counts were also determined microscopically by counting on a Neubauer chamber, using methylene blue staining as an indicator of viability. All the samples were analyzed in triplicate.
Aromatic Profile Quantification by GC-MS
Analysis of volatile compounds was performed according to the methodology of Márquez-Lemus et al. (2019) with some modifications. Fermentation samples were centrifuged at 7000 rpm (5697×g) for 15 min at 10°C in a Sigma 6K15 centrifuge. One gram of the centrifuged sample was deposited in a 20-ml vial with a magnetic cap and a teflon-silicon septum, and volatiles were extracted by a 2-cm PDMS/DVB/CAR solid-phase micro extraction fiber (Supelco; Sigma-Aldrich) while being heated at 45°C and agitated at 200 rpm for 1 h. The fiber was desorbed at 250°C, splitless mode, to the injection port of a 7890A gas chromatograph (Agilent Technologies, Santa Clara, CA, USA) equipped with a capilar column DB-5MS (60 m × 250 μm diameter, 0.25 μm film thickness, Agilent Technologies Inc.) and coupled to a 5975C single quadrupole mass spectrometer (Agilent Technologies, Inc.). The carrier gas was helium (99.99 % purity) at a flow rate of 1.0 ml/min, and the following temperature program was used: 3 min at 40°C, first ramp of 5°C/min up to 180°C, second ramp of 20°C/min up to 260°C, and held for 5 min. The transfer line was set at 280°C. A solution of 20 mg/kg of 3-octanol was used as the internal standard. Electronic impact mode at 70 eV was used, with a gain factor of 1, with ionization source and quadrupole temperatures of 230 and 150°C, respectively, and a mass range between 33 and 500 μma. Identification of volatile compounds was obtained by comparing their mass spectra with those of the NIST/EPA/NIH Mass Spectra Library database version 1.7.
Statistical Analysis
Production differences of all the metabolites during fermentations were assessed by statistical analysis performed using the Analyze-it software for Microsoft Excel (version 2.20) and JMP routine of the SAS software for ANOVA analysis.
Results
Pure Inoculum Fermentation Performance on Semi-synthetic (M2) Medium
Yeasts were firstly characterized in semi synthetic M2 medium. Results obtained showed a differential profile at 48 h (early stationary phase) in the consumption of sugars and fermentative metabolites (Table 1). All tested yeast species were able to produce ethanol, with S. cerevisiae strains being the most productive, ranging from 25 to 30 g/L as expected, but some of the non-Saccharomyces strains were also able to produce around 30 g/L such as the K. marxianus (Km1Y9), T. delbrueckii (Td1AN9) as well as Z. bailii (Zb3Y1) and C. lusitaniae (Cl4Y4) during this fermentation time. Regarding residual sugars, all the strains consumed first glucose present on the medium and still had residual fructose at this sampling time, being around 10 g/L in the case of the fructophilic strain control Fermichamp. In general, the non-Saccharomyces strains consumed at a lower rate the two hexoses than the S. cerevisiae strains. Some non-Saccharomyces strains such as Kluyveromyces (Km1Y9) and the Torulaspora (Td1AN91) also left <10 g/L of fructose at this time. All yeasts were able to produce glycerol at this time, the Torulaspora strains being the ones that exhibited a higher average (>2 g/L), followed by the Saccharomyces strains. The M. guilliermondii strain (Pg1Y12) exhibits the lowest glycerol accumulation with 0.22 g/L. On the other hand, all the strains of non-Saccharomyces showed a lower concentration of acetic acid than the strains of S. cerevisiae, except K. marxianus (Km4D3) which showed an increased in the concentration (0.4 g/L) of acetic acid, a parameter that is important for the alcoholic beverage industry. Regarding volatile metabolite production on M2 (Table 2), all tested strains presented a similar production of some volatiles, such as 2-methyl-1-butanol (between 200 and 500 μg/L), a similar production of ethyl-butyrate (around 2 mg/L). In contrast, Saccharomyces strains produced higher ethyl-hexanoate concentrations and lower concentrations of isoamyl acetate and phenyl ethyl acetate, when compared with the non-Saccharomyces strains. Hexyl acetate was only detected for a Torulaspora (Td1AN2) strain. From all these data, plus previous works on tolerance to osmotic, oxidative, and temperature stresses (Oliva Hernández et al., 2013; De la Torre-González et al., 2016; Vergara-Álvarez et al., 2019), we selected a group of three S. cerevisiae mezcal strains (plus Fermichamp as fructophilic control) and the best performing strains of the Kluyveromyces (Km1Y9), Torulaspora (Td1AN9) and Zygosaccharomyces (Zb3Y1) genera, although it is worth mentioning that some other promising strains are still being investigated.
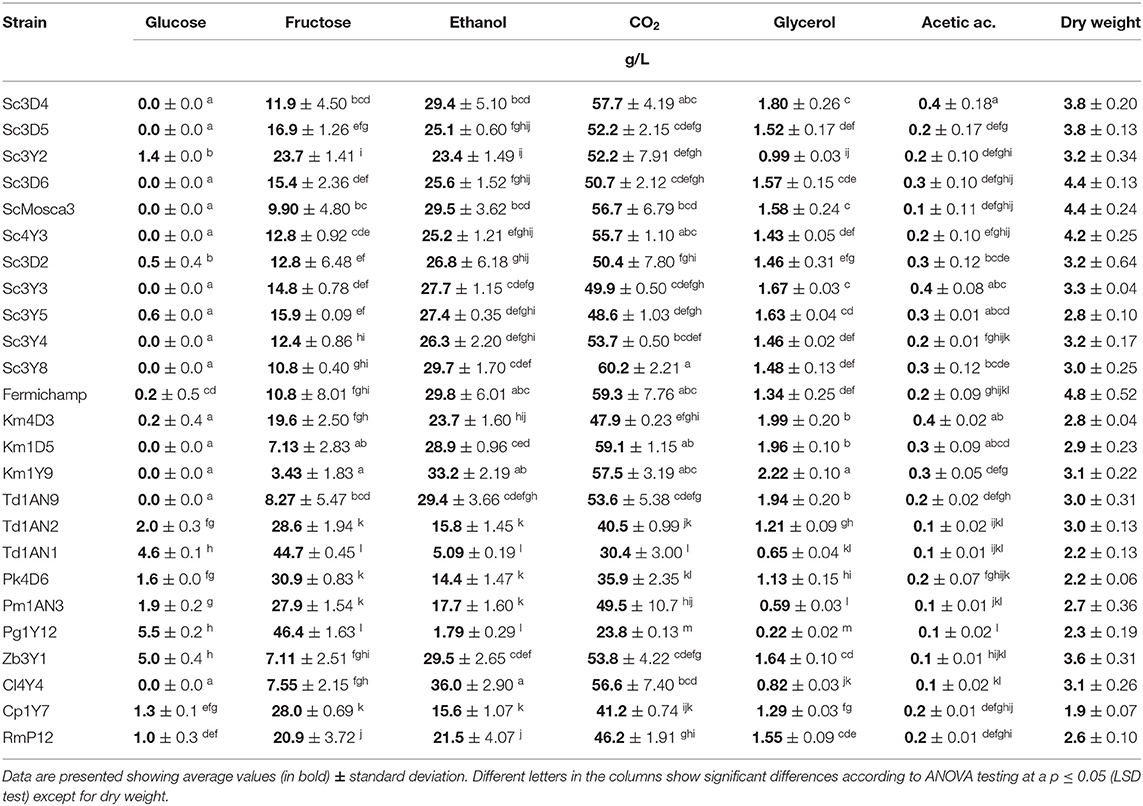
Table 1. Primary metabolite production and residual hexoses for all yeasts strains tested at 48 h of fermentation in semi-synthetic medium M2.
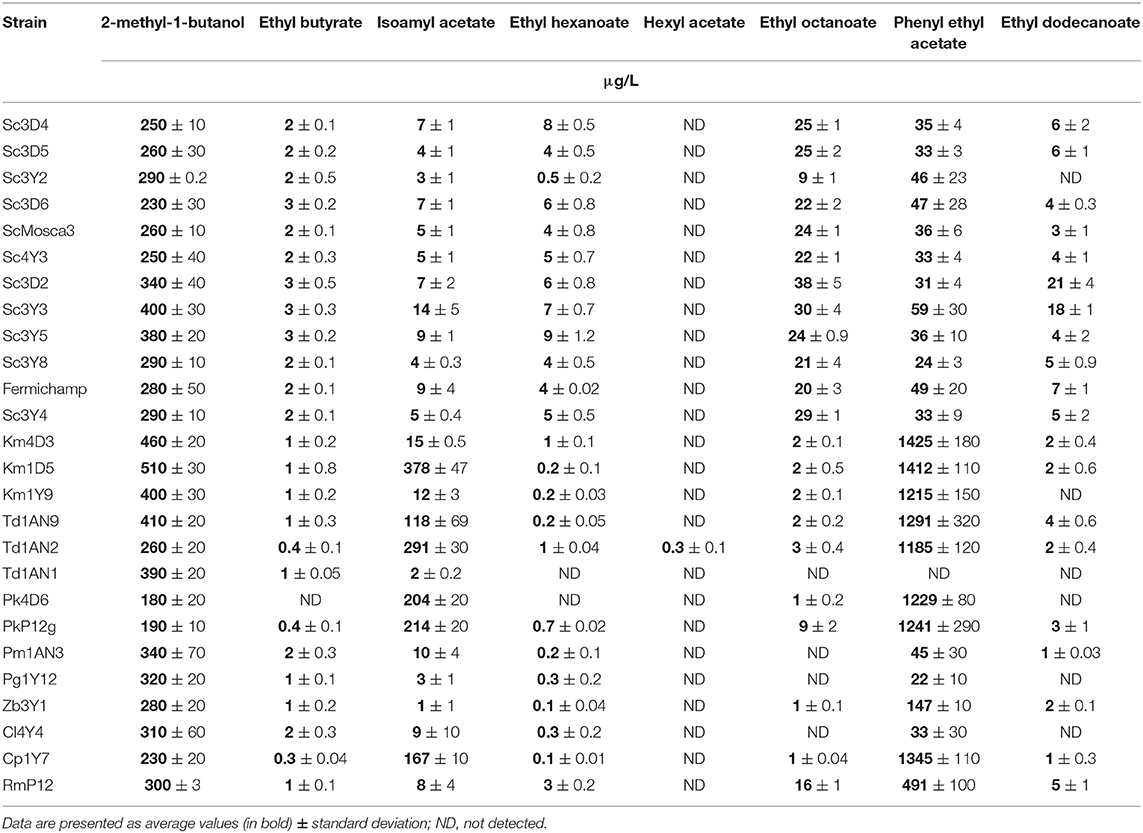
Table 2. Volatile metabolite production for all yeast strains tested at 48 h of fermentation in semi synthetic medium M2.
Pure Inoculum Fermentation Performance on Agave Medium
Fermentative profile of these selected yeasts was summarized at 96 h on Agave medium (Table 3) choosing the final stage of the fermentation. Mezcal Saccharomyces and non-Saccharomyces strains exhibited high fructose consumption (left around 3 g/L) and a high ethanol productivity (around 50 g/L). Notably, the control strain Fermichamp had a very poor growth and was unable to consume the hexoses, and consequently did not ferment the Agave medium, probably due to the presence of a high concentration of inhibitory Maillard compounds, saponins, and furfural (Díaz-Montaño et al., 2008). In general, Zb3Y1 produced the higher concentrations of glycerol and the lower concentration of acetic acid. In terms of dry weight, strain Km1Y9 is very promising, as it produced the lowest amount of biomass. Regarding the production of volatile metabolites for the individually inoculated strains on Agave medium, we can observe (Table 5 upper panel) that, for the Fermichamp strain, the detected 2-methyl-1-butanol is part of the composition of the Agave medium, as this strain is unable to ferment it. The non-Saccharomyces strains Td1AN9 and Km1Y9 were characterized by their high production of phenyl ethyl acetate and no production of ethyl octanoate. From these results, we decide not to use the commercial strain Fermichamp for the mixed inoculum; hence, three mezcal S. cerevisiae strains in combination with the three previously selected non-Saccharomyces strains were further characterized.
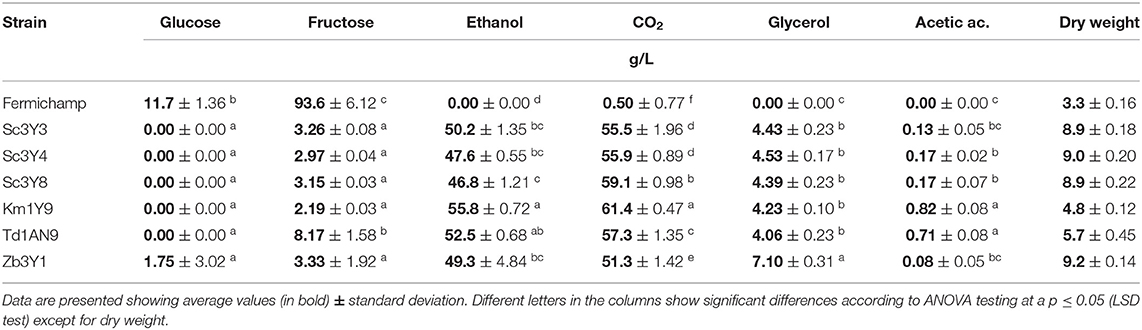
Table 3. Residual hexoses and primary metabolite production for the selected yeast strains at 96 h fermenting as individual inoculum on Agave medium.
Mixed Yeast Fermentation Performance on Agave Medium
For testing the performance of mixed inocula on Agave medium, three non-Saccharomyces strains (K. marxianus 1Y9, T. delbrueckii 1AN9, and Z. bailli 3Y1) were selected to be assayed in combination with each of the S. cerevisiae strains (3Y3, 3Y4, and 3Y8) inoculated at a ratio of 1:1 for mixed inoculum fermentations on Agave medium. The mixed cultures of the three S. cerevisiae with K. marxianus, T. delbrueckii, and Z. bailli were performed under semi-anaerobic growth conditions (1-hole vent caps) which allowed a longer carbon dioxide production and a high reproducibility of the fermentations. Hexose consumption, CO2, dry weight, and ethanol production were analyzed at 96 h (Table 4). In general, the mixed cultures showed a moderate increase on ethanol, glycerol, and acetic acid concentrations as compared with their pure inoculum fermentations (Table 3). Accordingly, residual fructose in these mixed cultures was also lower than the pure cultures and having high yields of ethanol (YEtOH/S) ranging from 0.46 to 0.49. According to the statistical analysis on these data, fermentation performance for primary metabolites of the mixed inoculum of Sc3Y8 combined with either Km1Y9 or Td1AN9 was the best, followed by the mixed inoculum of Sc3Y3 with Km1Y9. Regarding volatile metabolite production for the selected strains fermenting in the Agave medium as mixed inoculum (Table 5, lower panel), we observed that, as expected from the individually inoculated experiments (Table 5, upper panel), the use of Td1AN9 and Km1Y9 produced a fermented product with a higher phenyl ethyl acetate concentration, being the highest for the combination with any of the Saccharomyces mezcal strains.
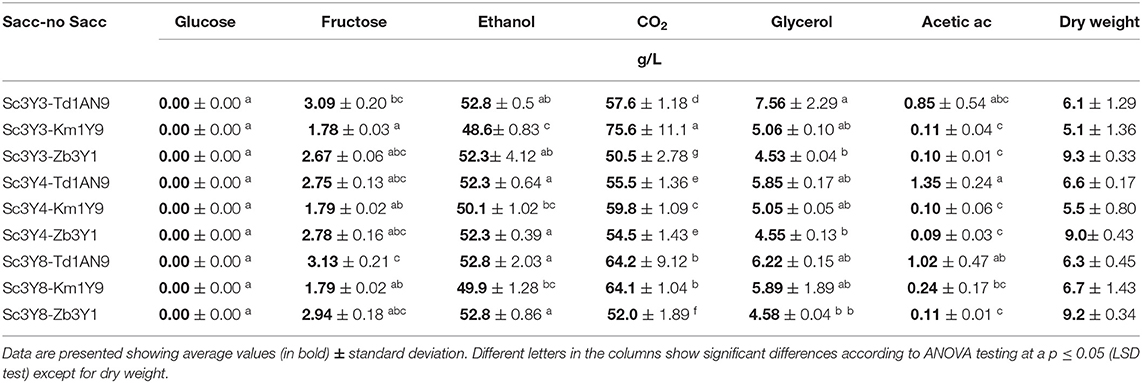
Table 4. Primary metabolite profiles and hexose consumption for the mixed cultures at 96 h in Agave medium.
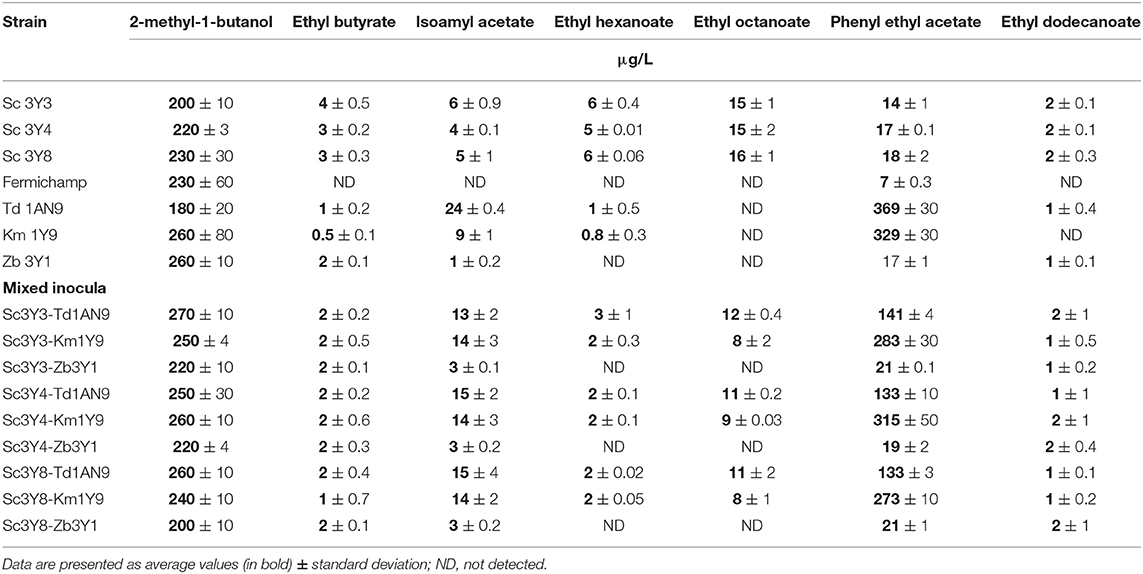
Table 5. Volatile metabolite production for selected yeasts, fermenting as individual (upper panel) or mixed (lower panel) inocula fermentations, tested at 96 h of fermentation in Agave medium.
Population Quantification by Fish Analysis
To assess the population dynamics of the selected yeast species, a FISH analysis was performed, since it allows the reliable identification of two or more simultaneous strains in mixed cultures. From the previous results for the mixed yeast fermentations on Agave media, strains selected for this analysis were Sc3Y3 and the non-Saccharomyces strains Km1Y9 and Td1AN9, to be evaluated simultaneously in mixed cultures, to assess the influence of the presence of the three yeast populations. For this aim, ScX, KmX, and TdX probes allowed us to identify genera Saccharomyces, Kluyveromyces, and Torulospora, respectively. Formamide concentration was adjusted to 30% since this concentration allowed a good fluorescence signal and hybridization for all the probes selected (data not shown). Populations for each genus were inferred from the measured fluorescent area obtained by the different probes on these mixed fermentations on Agave medium. Two inoculation ratios were assayed for this FISH approach. The 0.1:1:1 (Figure 1A) and the 1:1:1 (Figure 1B) for Sc3Y3, Td1AN9, and Km1Y9 strains, respectively, visually show the population dynamics for each yeast in mixed fermentations. Quantitatively, Figures 2A, B present the fluorescent areas for each yeast species and the total area for both inoculation ratios (left axis) and compares them with the total cell concentration by using a Neubauer chamber (black dots, right axis). We can observe that DAPI (blue diamonds, all yeasts present) fluorescent areas had similar profiles to total cell count, as it was expected. Main changes were observed at the beginning of the fermentative process, probably linked to the yeast ratio differences. All yeasts showed a rapid increase on their areas during the first 24 h of culture, and in both inoculation ratios; Saccharomyces strain (red squares) had a higher final fluorescent area, whilst both K. marxianus (purple dots) and T. delbrueckii (green triangles) had the same profiles, being lower in area than the one for S. cerevisiae, nonetheless remaining present in the culture. To assess in more detail the growth and compatibility of the mixed inoculum, and to link it with the metabolites' profiles, we chose the combination of the three S. cerevisiae mezcal strains with T. delbrueckii to specifically follow by differential plate counts the evolution of their viable and culturable populations.
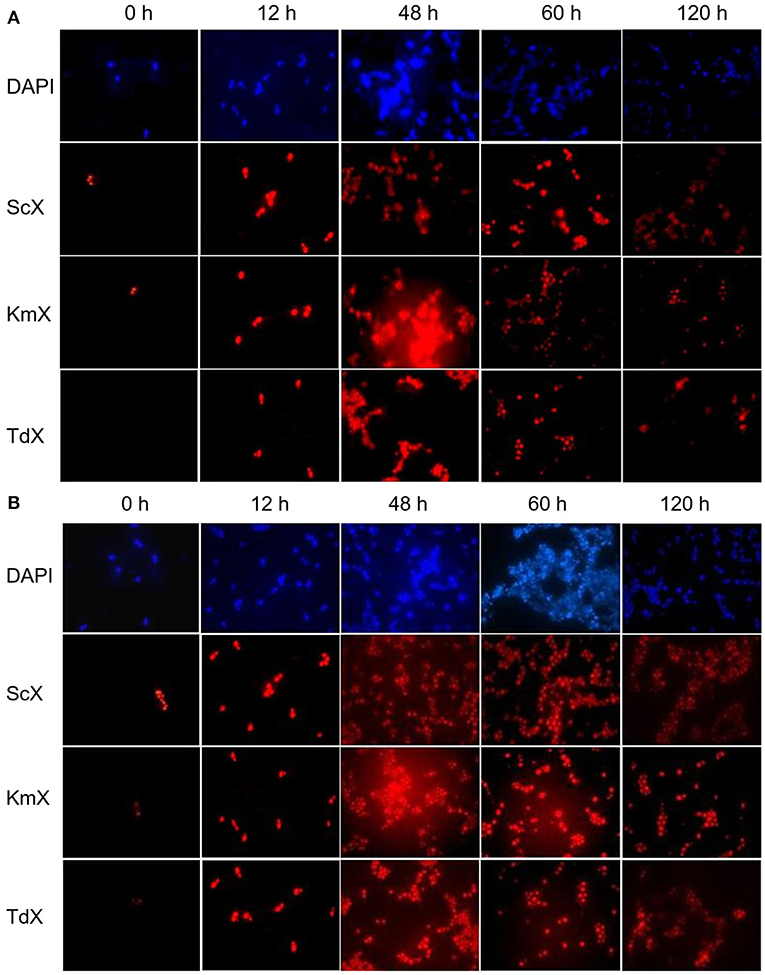
Figure 1. Qualitative examples of FISH images obtained during fermentation of Agave medium using specific probes for Saccharomyces cerevisiae (Sc3Y3), Torulaspora delbrueckii (Td1AN9), and Kluyveromyces marxianus (Km1Y9) strains at inoculation ratios of (A) 0.1:1:1, and (B) 1.1:1, respectively. Fluorescent detection was carried out using an Olympus Microscope (Olympus BX51) at 100×.
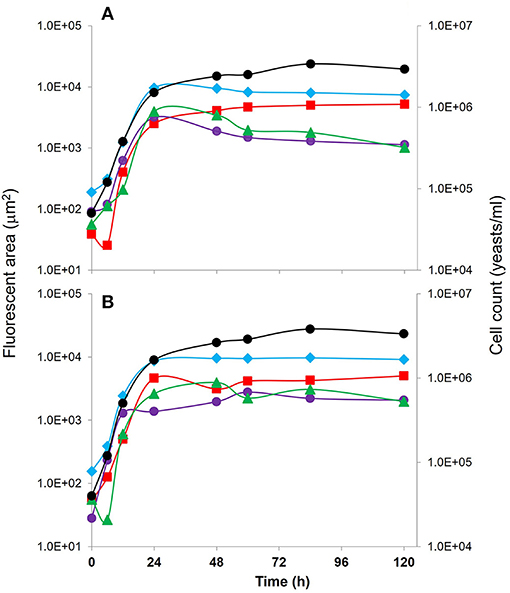
Figure 2. Quantitative growth kinetics measured as total fluorescent areas (from FISH analysis) and as colony plate counts of mixed cultures (Sc:Td:Km) of Sc3Y3, Td1AN9, and Km1Y9 strains for two mixed inocula ratios: (A) 0.1:1:1, and (B) and 1:1:1, fermenting on Agave medium. Symbols: (all yeasts),
,
,
,
cell count (yeasts/ml). Standard deviation was below 10% for all data.
Population Quantification by Differential Agar Plate Counts
Solid WL Differential and Nutrient Agar (Sigma-Aldrich) were used for viable population differentiation during fermentation kinetics of the three mixed cultures tested, which comprised each selected Saccharomyces cerevisiae strain in combination with T. delbrueckii (Td1AN9), at the inoculation ratio of 0.1:1 (Sc:Td), to mimic naturally occurring conditions of mezcal fermentations. We observed (Figure 3) a rapid increase of S. cerevisiae populations during the first 24 h, but contrary to the behavior reported on other fermentations, for example, on grape juice (De la Torre-González et al., 2020), a drop of viability/culturability for the Saccharomyces strains was assessed at around 48 h after inoculation, while the T. delbrueckii strain remained highly viable up to the end of the fermentation. Depending on the specific S. cerevisiae strain tested, they were able to further increase their culturable population, but always remaining below of that of T. delbrueckii.
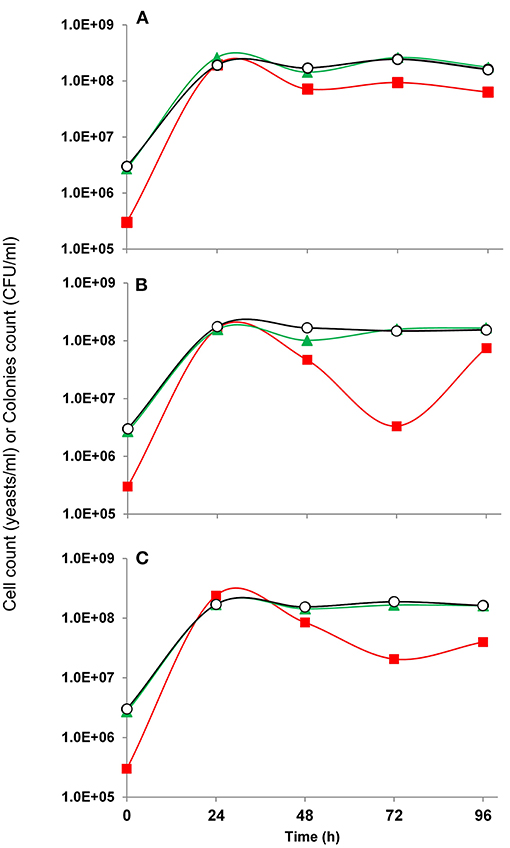
Figure 3. Population growth kinetics of mixed cultures of mezcal S. cerevisiae and non-Saccharomyces (Torulaspora delbrueckii) strains fermenting Agave medium. (A) Sc3Y3-Td1AN9, (B) Sc3Y4-Td1AN9, (C) Sc3Y8-Td1AN9. The data shown were obtained from triplicates of two independent experiments. Markers are average values of: total cell count (yeasts/ml),
(CFU/ml),
(CFU/ml), –○– viable cell count (viable yeasts/ml). Standard deviation was below 10% for all data. Notice that total and viable cell count markers overlap.
Discussion
The use of non-conventional yeasts as inoculum for the traditional fermentative process has increased in the literature, since some strains can increase the aroma complexity of the fermented products (Pina et al., 2005; De la Torre-González et al., 2017; Tufariello et al., 2021). Tequila and mezcal liquors are produced from cooked Agave must fermentation, which usually depends on spontaneous or pure S. cerevisiae strain inoculation (Cedeño, 1995; Díaz-Montaño et al., 2008). The yeasts used in this work were obtained from mezcal musts and have been selected for having a higher tolerance to stress, particularly for osmotic, oxidative, and temperature unfavorable conditions (De la Torre-González et al., 2016; Vergara-Álvarez et al., 2019). In this study, we aimed to characterize the application potential of some of the yeasts found in mezcal from Tamaulipas (De la Torre-González et al., 2017), first by pure strain inoculation on a semi synthetic medium resembling Agave must hexose initial concentrations, and we found that all the strains, but mainly Saccharomyces, Torulospora, Kluyveromyces, Clavispora, and Zygosaccharomyces were the ones with the best fermentative performances, and also were good producers of volatile compounds, as previously observed for some of these genera (Arellano et al., 2008; Lopez-Alvarez et al., 2012; Páez-Lerma et al., 2013; Nolasco-Cancino et al., 2018). Particularly, T. delbrueckii had unique oenological characteristics such as production of specific aromas, as observed for Tondini et al. (2019) and Tufariello et al. (2021) for wine. Indeed, based on our results, these non-Saccharomyces strains could be used as pure inoculum to carry out to completion the Agave fermentation. Some authors have reported the use of few non-Saccharomyces strains as pure inoculum for Agave tequilana must fermentations, such as K. marxianus, Pichia spp., Hanseniaspora spp., and Kloeckera spp. to produce tequila (Arrizon et al., 2006; Díaz-Montaño et al., 2008; Amaya-Delgado et al., 2013; González-Robles et al., 2015; Segura-García et al., 2015) and mezcal from Oaxaca (Nolasco-Cancino et al., 2018).
Concerning S. cerevisiae, the used strains in this work were highly productive and readily adapted to Agave must conditions, whereas control Fermichamp was not able to ferment the Agave medium, as has been also observed for other wine S. cerevisiae strains by Arrizon et al. (2006). Another important factor that could have driven the selection of these yeasts, besides the toxic compounds found in the Agave musts, concerning the carbon source, is the presence of cellulose and the fructose polysaccharides (usually reported as ATF, Agave tequilana fructans, or as agavin), which is related to inulin but has a more branched structure, which implies that, besides the β-glucosidases reported for this genus, a battery of (endo and exo) fructanases and fructosyltransferases are probably active in these strains, as evidenced by Arrizon et al. (2012) and Vicente-Magueyal et al. (2020) for non-Saccharomyces yeasts, by Corona-González et al. (2015) for S. cerevisiae, and also observed by us for some of these strains (data not shown). Among the mezcal Saccharomyces strains of this study, the main differences were found regarding their ability to ferment fructose, the accumulation/degradation of 2-methyl-1-butanol, and the levels of ethyl-decanoate and ethyl-hexanoate. In general, the volatile compounds produced by these pure mezcal strains on the Agave medium are of great aromatic value, particularly isoamyl acetate and phenyl ethyl acetate, compounds which could render (in the appropriate amounts) good organoleptic characteristics (fruity, sweet, pineapple) to a fermentative process (De la Torre-González et al., 2017). For other S. cerevisiae strains from Agave fermentations, there are also reports of the differential production of methanol, 2-phenyl ethanol, n-propanol, acetoin, and amyl alcohols (Arrizon et al., 2006; Díaz-Montaño et al., 2008). In other fermented products, like wine, Romano et al. (2003) have reported that, for 30 S. cerevisiae wine strains fermenting Aglianico grape must, that a significant variability was observed amongst the strains in terms of the level of production of isoamyl alcohol, isobutanol, and acetic acid, whereas they all were very similar in terms of acetaldehyde, n-propanol, and ethyl acetate productions, which indicates that, even considering that S. cerevisiae is by far the most studied yeast, there is still a high variability in aromatic profiles that can be obtained with pure cultures of this species, and that is worth continuing the analysis of isolates from many different technological and natural environments.
Regarding the K. marxianus and T. delbrueckii, both strains used in the mixed cultures induced a higher phenyl ethyl acetate and isoamyl acetate in the fermented must, as evidenced by a fruiter aroma in the products. The yeast T. delbrueckii has been frequently reported to be important in wine production, as it has a high ethanol tolerance and a low acetic acid production when combined with S. cerevisiae, for the production of different types of wines, and where signature compounds were identified, such as linalool, 3-methyl-1-butanol, 2-phenylethanol, ethyl butanoate, ethyl decanoate, phenylacetaldehyde, ethyl propanoate, ethyl isobutanoate, and ethyl dihydrocinnamate, as well as benzyl alcohol (Tufariello et al., 2021). All this was linked to a higher β-glucosidase activity in the T. delbrueckii strains used than in the S. cerevisiae yeasts. Yeast K. marxianus (GRO6) has been proposed as a good candidate to be used as sole inoculum for tequila production, as it is also able to complete the fermentation with an adequate organoleptic profile (Amaya-Delgado et al., 2013).
Mixed cultures of non-Saccharomyces (Km1Y9, Td1AN9, and Zb3Y1) and S. cerevisiae strains (Sc3Y3, Sc3Y4 and Sc3Y8) showed an improved fermentation capacity as compared to pure cultures, having a higher ethanol, carbon dioxide, and glycerol production, and a lower residual fructose. Pure and mixed yeast populations on Agave medium, inoculated at two different radii, had similar profiles when assessed by FISH analysis (as demonstrated in Figure 2) where S. cerevisiae remained as the most abundant yeast, and the non-Saccharomyces strain (either K. marxianus or T. delbrueckii) had a drop in its population at the end of the fermentation. However, when viable and culturable populations were quantified by differential plating, it was evident that, contrary to what has been reported in other fermentation systems, the S. cerevisiae strain culturable concentrations decreased with time, while T. delbrueckii strain remained highly viable and culturable during the whole process (Figure 3), which is favorable in terms of a more extended period of production of volatile compounds on this medium. A similar behavior was also observed by Andorra et al. (2011) for mixed fermentations of S. cerevisiae and Hanseniaspora guilliermondii in a synthetic grape must, when comparing populations obtained by culture-independent (FISH probes and qPCR) and plating (using cycloheximide as differential factor in the media) techniques, observing that while the plating technique indicated that S. cerevisiae remained highly culturable throughout the fermentation, the H. guilliermondii strain sharply decreased its culturability with time, but when assessed by FISH probes and flow cytometry, they observed a species-specific intensity of hybridization, as H. guilliermondii signal (rRNA content) remained the same at a high, stable value during the whole fermentation, and even after boiling a sample of this yeast for 10 mins, which emphasize the importance to clearly assess the metabolic state of the strains and the specific stability/resistance of their rRNA, if culture-independent techniques are used to monitor yeast populations in alcoholic fermentations.
In terms of resistance to stress, data suggest that it is not only the presence of fructose as the main fermentable sugar but also that of toxic compounds present on the Agave must, as furfural and vanillin (data not shown), that affects the viability and metabolic performance of the strains, as has been also observed by Arrizon et al. (2006) for other Agave yeast strains. This clearly requires further investigation, but from the data obtained here and our previous work (Oliva Hernández et al., 2013; De la Torre-González et al., 2016, 2020; Vergara-Álvarez et al., 2019) with these mezcal strains, the selection of Agave must isolated strains (Saccharomyces and non-Saccharomyces) to be used as inoculum for tequila and mezcal (as the main liquors produced from Agave spp. plants, but there are others) would need to be tested at 30°C to assess that are be able to grow on agar plates containing 500 g/L of fructose, to be able to grow on yeast extract and peptone agar plates containing 8% ethanol and fructose as a carbon source. Finally, they should present a high viability/culturability on Agave must diluted at 10°Bx for 5 days. This could result in a robust selection of yeasts that have high fermentative performances that can be then tested in terms of their survival rates when cultured together, and then their aromatic profiles when fermented as pure inoculum or in co-culture, to select those with the desired, more complex bouquet. It is worth noting that there was not a formal organoleptic evaluation of the fermented musts obtained in this work, as there is still a step of distillation that needs to be performed to obtain the final products. As the focus of our work was the screening of a high number of strains, small scale fermentations were carried out, hence distillation was not carried-out. Certainly, the next stage would require us to produce pilot scale fermentations to be able to distill and obtain enough product to carry out the sensory evaluation.
Conclusion
Rational selection of yeast starters for tequila and mezcal productions must consider high fructose selective media (as the ones used in this work), since pure o mixed yeast starters may greatly influence the fermentative performance of the selected yeast. In this study, we selected three Saccharomyces cerevisiae and three non-Saccharomyces mezcal strains to be tested in a mixed starter. Specifically, T. delbrueckii (Td1AN9) and K. marxianus (Km1Y9) were the best candidates to be considered, either as pure inoculum, or even better, as part of a mixed inoculum with an Agave-isolated Saccharomyces cerevisiae strain, due to the high productivity of ethanol and glycerol; low acetic acid production; and increased levels of phenyl ethyl acetate, isoamyl acetate, and hexyl acetate in the Agave medium. In addition, the high survival rates of these non-Saccharomyces strains during fermentation increase their technological feasibility as inoculum for Agave musts, with an extended metabolic activity during fermentation.
Data Availability Statement
The raw data supporting the conclusions of this article will be made available by the authors, without undue reservation.
Author Contributions
FJD-G helped in planning and performed all the experiments, CPL-C financed and planned the whole thesis and supervised the metabolic and fermentation experiments, JAN-Z financed and planned the molecular biology experiments and helped analyze the data, DH planned and financed the FISH experiments, and PAV-L planned and supervised the GC-MS experiments. The manuscript was written, proofread, and approved by all authors. All authors contributed to the article and approved the submitted version.
Funding
This research was funded by Instituto Politécnico Nacional—Secretaría de Investigación y Posgrado projects SIP 2021-0289 and 2021-0975.
Conflict of Interest
Author FJD-G is employed by Innovak Global.
The remaining authors declare that the research was conducted in the absence of any commercial or financial relationships that could be construed as a potential conflict of interest.
Publisher's Note
All claims expressed in this article are solely those of the authors and do not necessarily represent those of their affiliated organizations, or those of the publisher, the editors and the reviewers. Any product that may be evaluated in this article, or claim that may be made by its manufacturer, is not guaranteed or endorsed by the publisher.
Acknowledgments
We acknowledge the doctoral scholarship granted to FJD-G (CONACyT, México) and the technical support of E. A. De la Cruz-Arguijo and M. S. Ramírez-González for preserving the LBI-CBG microorganism's collection.
References
Amaya-Delgado, L., Herrera-López, E. J., Arrizon, J., Arellano-Plaza, M., and Gschaedler, A. (2013). Performance evaluation of Pichia kluyveri, Kluyveromyces marxianus and Saccharomyces cerevisiae in industrial tequila fermentation. World J. Microbiol. Biotechnol. 29, 875–881. doi: 10.1007/s11274-012-1242-8
Andorra, I., Monteiro, M., Esteve-Zarzoso, B., Albergaria, H., and Mas, A. (2011). Analysis and direct quantification of Saccharomyces cerevisiae and Hanseniaspora guilliermondii populations during alcoholic fermentation by fluorescence in situ hybridization, flow cytometry and quantitative PCR. Food Microbiol. 28, 1483–1491. doi: 10.1016/j.fm.2011.08.009
Arellano, M., Pelayo, C., Ramírez, J., and Rodriguez, I. (2008). Characterization of kinetic parameters and formation of volatile compounds during the tequila fermentation by wild yeasts isolated from agave juice. J. Ind. Microbiol. Biotechnol. 35, 835–841. doi: 10.1007/s10295-008-0355-4
Arrizon, J., Fiore, C., Acosta, G., Romano, P., and Gschaedler, A. (2006). Fermentation behaviour and volatile compound production by agave and grape must yeasts in high sugar Agave tequilana and grape must fermentations. Antonie van Leeuwenhoek 89, 181–189. doi: 10.1007/s10482-005-9022-1
Arrizon, J., Morel, S., Gschaedler, A., and Monsan, P. (2012). Fructanase and fructosyltransferase activity of non-Saccharomyces yeasts isolated from fermenting musts of Mezcal. Bioresource Technology 110, 560–565. doi: 10.1016/j.biortech.2012.01.112
Arroyo-López, F. N., Querol, A., and Barrio, E. (2009). Application of a substrate inhibition model to estimate the effect of fructose concentration on the growth of diverse Saccharomyces cerevisiae strains. J. Ind. Microbiol. Biotechnol. 36, 663–669. doi: 10.1007/s10295-009-0535-x
Campos-Rivero, G., Sánchez-Teyer, L. F., De la Cruz-Arguijo, E. A., Ramírez-González, M. S., Larralde-Corona, C. P., and Narváez-Zapata, J. A. (2019). Bioprospecting for fungi with potential pathogenic activity on leaves of Agave tequilana Weber var Azul. J. Phytopathol. 167, 283–297. doi: 10.1111/jph.12797
Cedeño, M. (1995). Tequila production. Crit. Rev. Biotechnol. 15, 1–11. doi: 10.3109/07388559509150529
Corona-González, R. I., Pelayo-Ortiz, C., Jacques, G., Guatemala, G., Arriola, E., Arias, J. A., et al. (2015). Production of fructanase by a wild strain of Saccharomyces cerevisiae on tequila agave fructan. Antonie Leeuwenhoek 107, 251–261. doi: 10.1007/s10482-014-0323-0
De Filippis, F., Laiola, M., Blaiotta, G., and Ercolini, D. (2017). Different amplicon targets for sequencing-based studies of fungal diversity. App. Environ. Microbiol. 83:e00905–17. doi: 10.1128/AEM.00905-17
De la Torre-González, F. J., Narváez-Zapata, J. A., and Larralde-Corona, C. P. (2017). “Microbial diversity and flavor quality of fermented beverages,” in Microbial Production of Food Ingredients and Additives (New York, NY: Academic Press), 125–154. doi: 10.1016/B978-0-12-811520-6.00005-2
De la Torre-González, F. J., Narváez-Zapata, J. A., López-y-López, V. E., and Larralde-Corona, C. P. (2016). Ethanol tolerance is decreased by fructose in Saccharomyces and non-Saccharomyces yeasts. LWT Food Sci. Technol. 67, 1–7. doi: 10.1016/j.lwt.2015.11.024
De la Torre-González, F. J., Narváez-Zapata, J. A., Taillandier, P., and Larralde-Corona, C. P. (2020). Mezcal as a novel source of mixed yeasts inocula for wine fermentation. Processes 8:1296. doi: 10.3390/pr8101296
Díaz-Montaño, D. M., Délia, M. L., Estarrón-Espinosa, M., and Strehaiano, P. (2008). Fermentative capability and aroma compound production by yeast strains isolated from Agave tequilana Weber juice. Enzyme Microb. Technol. 42, 608–616. doi: 10.1016/j.enzmictec.2007.12.007
González-Robles, I. W., Estarrón-Espinosa, M., and Díaz-Montaño, D. M. (2015). Fermentative capabilities and volatile compounds produced by Kloeckera/Hanseniaspora and Saccharomyces yeast strains in pure and mixed cultures during Agave tequilana juice fermentation. Antonie Leeuwenhoek 108, 525–536. doi: 10.1007/s10482-015-0506-3
Hedges, A. J. (2002). Estimating the precision of serial dilutions and viable bacterial counts. Int. J. Food Microbiol. 76, 207–214. doi: 10.1016/S0168-1605(02)00022-3
Kirchmayr, M. R., Segura-García, L. E., Lappe-Oliveras, P., Moreno-Terrazas, R., de la Rosa, M., and Gschaedler-Mathis, A. (2017). Impact of environmental conditions and process modifications on microbial diversity, fermentation efficiency and chemical profile during the fermentation of mezcal in Oaxaca. LWT Food Sci. Technol. 79, 160–169. doi: 10.1016/j.lwt.2016.12.052
Kurtzman, C. P., and Robnett, C. J. (1998). Identification and phylogeny of ascomycetous yeasts from analysis of nuclear large subunit (26S) ribosomal DNA partial sequences. Antonie Leeuwenhoek 73, 331–371. doi: 10.1023/A:1001761008817
Lachance, M. A. (1995). Yeast communities in a natural tequila fermentation. Antonie Leeuwenhoek 68, 151–160. doi: 10.1007/BF00873100
Libkind, D., Cade,Ž, N., Opulente, D. A., Langdon, Q. K., Rosa, C. A., Sampaio, J. P., et al. (2020). Towards yeast taxogenomics: lessons from novel species descriptions based on complete genome sequences. FEMS Yeast Res. 20:foaa042. doi: 10.1093/femsyr/foaa042
Lopez-Alvarez, A., Diaz-Perez, A. L., Sosa-Aguirre, C., Macias-Rodriguez, L., and Campos-Garcia, J. (2012). Ethanol yield and volatile compound content in fermentation of agave must by Kluyveromyces marxianus UMPe-1 comparing with Saccharomyces cerevisiae baker's yeast used in tequila production. J. Biosci. Bioeng. 113, 614–618. doi: 10.1016/j.jbiosc.2011.12.015
Márquez-Lemus, M., Valadez-Carmona, L., García-Zebadúa, J. C., Ortiz-Moreno, A., Vázquez-Landaverde, P. A., Alamilla-Beltrán, L., et al. (2019). Assessment of the variation of the volatile compound composition and antioxidant activity in Opuntia fruits liquors during the maceration process. CYTA J Food 17, 501–508. doi: 10.1080/19476337.2019.1595158
Narváez-Zapata, J. A., Rojas-Herrera, R. A., Rodríguez-Luna, I. C., and Larralde-Corona, C. P. (2010). Culture-independent analysis of lactic acid bacteria diversity associated with mezcal fermentation. Curr. Microbiol. 61, 444–450. doi: 10.1007/s00284-010-9636-z
Nava-Cruz, N. Y., Medina-Morales, M. A., Martinez, J. L., Rodriguez, R., and Aguilar, C. N. (2014). Agave biotechnology: an overview. Crit. Rev. Biotechnol. 35, 1–14. doi: 10.3109/07388551.2014.923813
Nolasco-Cancino, H., Santiago-Urbina, J. A., Wacher, C., and Ruíz-Terán, F. (2018). Predominant yeasts during artisanal mezcal fermentation and their capacity to ferment maguey juice. Front. Microbiol. 9:2900. doi: 10.3389/fmicb.2018.02900
Oliva Hernández, A. A., Taillandier, P., Reséndez Pérez, D., Narváez Zapata, J. A., and Larralde Corona, C. P. (2013). The effect of hexose ratios on metabolite production in Saccharomyces cerevisiae strains obtained from the spontaneous fermentation of mezcal. Antonie Leeuwenhoek Int. J. Gen. Mol. Microbiol. 103, 833–843. doi: 10.1007/s10482-012-9865-1
Páez-Lerma, J. B., Arias-García, A., Rutiaga-Quiñones, O. M., Barrio, E., and Soto-Cruz, N. O. (2013). Yeasts isolated from the alcoholic fermentation of Agave duranguensis during Mezcal production. Food Biotechnol. 27, 342–356. doi: 10.1080/08905436.2013.840788
Peter, J., De Chiara, M., Friedrich, A., Yue, J.-X., Pflieger, D., Bergström, A., et al. (2018). Genome evolution across 1,011 Saccharomyces cerevisiae isolates. Nature 556, 339–344. doi: 10.1038/s41586-018-0030-5
Pina, C., Antonio, J., and Hogg, T. (2005). Inferring ethanol tolerance of Saccharomyces and non-Saccharomyces yeasts by progressive inactivation. Biotechnol. Lett. 26, 1521–1527. doi: 10.1007/s10529-005-1787-9
Romano, P., Fiore, C., Paraggio, M., Caruso, M., and Capece, A. (2003). Function of yeast species and strains in wine flavour. Int. J. Food Microbiol. 86, 169–180. doi: 10.1016/S0168-1605(03)00290-3
Segura-García, L. E., Taillandier, P., Brandam, C., and Gschaedler, A. (2015). Fermentative capacity of Saccharomyces and non-Saccharomyces in agave juice and semi-synthetic medium. LWT Food Sci. Technol. 60, 284–291. doi: 10.1016/j.lwt.2014.08.005
Tondini, F., Lang, T., Chen, L., Herderich, M., and Jiranek, V. (2019). Linking gene expression and oenological traits: comparison between Torulaspora delbrueckii and Saccharomyces cerevisiae strains. Int. J. Food Microbiol. 294, 42–49. doi: 10.1016/j.ijfoodmicro.2019.01.014
Tufariello, M., Fragasso, M., Pico, J., Panighel, A., Castellarin, S. D., Flamini, R., et al. (2021). Influence of non-Saccharomyces on wine chemistry: a focus on aroma-related compounds. Molecules 26:644. doi: 10.3390/molecules26030644
Vera Guzmán, A. M., Santiago García, P. A., and López, M. G. (2009). Compuestos volátiles aromáticos generados durante la elaboración de mezcal de Agave angustifolia y Agave potatorum. Rev. Fitotec. Mex. 32:273. doi: 10.35196/rfm.2009.4.273-279
Verdugo Valdez, A., Segura Garcia, L., Kirchmayr, M., Ramírez Rodríguez, P., González Esquinca, A., Coria, R., et al. (2011). Yeast communities associated with artisanal mezcal fermentations from Agave salmiana. Antonie Leeuwenhoek 100, 497–506. doi: 10.1007/s10482-011-9605-y
Vergara-Álvarez, I., Quiroz-Figueroa, F., Tamayo-Ordóñez, M. C., Oliva-Hernández, A. A., Larralde-Corona, C. P., and Narváez-Zapata, J. A. (2019). Flocculation and expression of FLO genes of a Saccharomyces cerevisiae mezcal strain with high stress tolerance. Food Technol. Biotechnol. 57, 544–553. doi: 10.17113/ftb.57.04.19.6063
Vicente-Magueyal, F. J., Bautista-Méndez, A., Villanueva-Tierrablanca, H. D., García-Ruíz, J. L., Jiménez-Islas, H., and Navarrete-Bolaños, J. L. (2020). Novel process to obtain agave sap (aguamiel) by directed enzymatic hydrolysis of agave juice fructans. LWT-Food Sci. Technol. 127:109387. doi: 10.1016/j.lwt.2020.109387
Xufre, A., Albergaria, H., Inácio, J., Spencer-Martins, I., and Gírio, F. (2006). Application of fluorescence in situ hybridisation (FISH) to the analysis of yeast population dynamics in winery and laboratory grape must fermentations. Int. J. Food Microbiol. 108, 376–384. doi: 10.1016/j.ijfoodmicro.2006.01.025
Keywords: Saccharomyces, Kluyveromyces, Torulaspora, mezcal, tequila, mixed starter, aroma profile, Agave
Citation: Larralde-Corona CP, De la Torre-González FJ, Vázquez-Landaverde PA, Hahn D and Narváez-Zapata JA (2021) Rational Selection of Mixed Yeasts Starters for Agave Must Fermentation. Front. Sustain. Food Syst. 5:684228. doi: 10.3389/fsufs.2021.684228
Received: 23 March 2021; Accepted: 09 August 2021;
Published: 08 September 2021.
Edited by:
Avinash Sharma, National Centre for Cell Science, IndiaReviewed by:
Guadalupe Virginia Nevárez-Moorillón, Autonomous University of Chihuahua, MexicoShankar Prasad Sha, Kurseong College, India
Copyright © 2021 Larralde-Corona, De la Torre-González, Vázquez-Landaverde, Hahn and Narváez-Zapata. This is an open-access article distributed under the terms of the Creative Commons Attribution License (CC BY). The use, distribution or reproduction in other forums is permitted, provided the original author(s) and the copyright owner(s) are credited and that the original publication in this journal is cited, in accordance with accepted academic practice. No use, distribution or reproduction is permitted which does not comply with these terms.
*Correspondence: Claudia Patricia Larralde-Corona, cGxhcnJhbGRlQGlwbi5teA==