- 1Department of Post-Harvest Management, College of Agriculture and Veterinary Medicine, Jimma University, Jimma, Ethiopia
- 2Department of Nutrition and Dietetics, College of Public Health and Medical Sciences, Jimma University, Jimma, Ethiopia
- 3Department of Agricultural and Biosystems Engineering, Faculty of Organic Agricultural Sciences, University of Kassel, Witzenhausen, Germany
Background: Development of complementary foods by mixing plant-based (cereals, pulses, oilseeds, and others) ingredients and employing various processing techniques is widely reported. However, information on comparison of anti-nutritional factors and functional properties of extruded and unextruded complementary flours made from a multi-mix is limited. In this regard, this study aims to investigate the influence of extrusion cooking on anti-nutritional and functional properties of newly developed extruded oats, soybean, linseed, and premix composite complementary flours.
Methods: Thirteen different blending ratios of oats, soybean, linseed, and premix were generated using a constrained D-optimal design of the experiment. Each of the 13 blends was divided into two groups: extrusion cooked and unextruded composite flour sample. Anti-nutritional and functional properties were determined using standard methods for both composite flours. ANOVA was used to determine if there was a significant difference for extruded and unextruded composite flours and paired t-tests were used to check variation between extruded and unextruded.
Results: The phytate content of the extruded and unextruded composite flours was 158.93–191.33 mg/100 g and 175.06–203.10 mg/100 g, respectively, whereas the tannin content of the extruded and unextruded composite flours was 8.4–22.89 mg/100 g and 23.67–36.97 mg/100 g, respectively. There was a statistically significant (p < 0.05) difference among the extruded composite flours in terms of phytate and condensed tannin content. Paired t-test has indicated a significant (p < 0.05) difference between extruded and unextruded composite flours for phytate and tannin. Water absorption capacity and bulk density have shown a significant (p < 0.05) difference among extruded and unextruded composite flours. An increase in the proportion of soybean and linseed flour was associated with an increase in phytate, tannin, and water absorption capacity of composite flours. However, bulk density was increased with an increasing proportion of oat in the blend.
Conclusion: The findings revealed that extrusion cooking significantly reduced phytate and condensed tannin content and improved the functional properties of the composite complementary food flour. Further investigation is needed on other anti-nutritional factors that are not included in this report.
Introduction
Child undernutrition remains pervasive and has severe health consequences in low-income and middle-income countries (Black et al., 2008). It remains to be a significant public health problem all over the developing world including Ethiopia (EDHS E, 2016; Hoffman et al., 2017; Tariku et al., 2017). Studies indicate that communities characterized by higher rates of undernutrition are associated with consuming predominantly starchy staples, lower amounts of fruits and vegetables, legumes, and pulses, and little or no animal source foods. Starchy diets such as cereals, roots, and tubers are known to be bulky, with low nutrient density and mineral bioavailability; hence, dependence on such diets leads to impaired growth and development (Kim et al., 2009).
Complementary foods in developing countries are usually made in a form of cereal gruels, which are characterized by low energy density and protein due to the large volume of water relative to its solid matter contents (Inyang and Zakari, 2008; Igyor et al., 2011). More of the solid matter is needed to increase the energy density of the gruel, which in turn makes the gruel too thick and viscous for an infant to consume. Taking into consideration the small intestinal capacity of infants, such diets will not fulfill their energy and other vital nutrient requirements (Ikujenlola and Fashakin, 2005). Cereals, legumes, and other plant-based complementary foods are characterized by high anti-nutritional contents (Samtiya et al., 2020).
Plant foods such as cereals and legumes naturally contain different anti-nutritional compounds. Infants and young children in the ages of complementary feeding have a high nutrient requirement, and the presence of anti-nutrients in their diets can negatively affect their nutritional status. The negative effects of cereal and legume anti-nutritional factors on the bioavailability of essential nutrients and thereby to undernutrition are well-documented (Gilani et al., 2012; Clemens, 2014).
Among the anti-nutritional compounds of most significant importance to be mapped in complementary foods are phytate (or phytic acid1). Phytate [inositol hexaphosphate (IP6)] is widely present in plant-based foods. It is well-documented that even small amounts of phytate in the diet will significantly reduce iron absorption (Davidsson et al., 1997; Hurrell, 2004). The bioavailability of mineral elements for absorption is affected when phytates in grains bind to the minerals and form indigestible complexes (Dahouenon-Ahoussi et al., 2012).
The problem of high dietary bulk density and anti-nutritional factor could be solved using malting and other processes such as extrusion (Hotz and Gibson, 2007). Extrusion is a food processing technique with the characteristics of high temperature and short time applied in the development of food products (Moscicki and van Zuilichem, 2011; Masatcioglu et al., 2014; De Cruz et al., 2015; Tiwari and Jha, 2017). Extrusion is a processing technique that has been shown to decrease anti-nutritional factors, improve the digestibility of starch and protein, and increase Fe bioavailability (Maclean et al., 1983; Saha et al., 1994; Singh et al., 2007; Diaz et al., 2013). Extrusion may further benefit food aid consumers because it can create pre-cooked porridges, which take less energy and time to prepare.
Besides the fact that most of the mothers in developing countries prepare child food from cereals only, complementary food preparation and processing practices can also affect the utilization of the required nutrient from the food they consume. The utilization of locally available ingredients and employing and optimizing the food processing techniques are the key criterion in the development of nutritionally upgraded foods for malnourished populations (Webb et al., 2011). Therefore, this research was initiated to evaluate the effectiveness of extrusion cooking process in reducing the major anti-nutritional factor contents (Phytate and tannin) and improving functional properties of complementary food prepared from oat, soybean, and linseed blended with premix (salt, sugar, moringa, and fenugreek) to improve the minerals, vitamins, and sensory quality of complementary food as indicated in Forsido et al. (2019). This work is the continuation of Forsido et al. (2019).
Materials and Methods
Experimental Materials
The materials used for this study were oat (Sinana one variety), soybean (Clark 63 variety), linseed (Kulumsa one variety), and premix [Moringa stenopetala, fenugreek (Chala variety), sugar, and iodized salt].
Sample Preparation
The detailed steps followed in sample preparation are described in our publication (Forsido et al., 2019). Different unit operations were followed for the preparation of flours from the different plant-based ingredients. However, all plant-based raw materials were cleaned and milled and all powder samples were stored at refrigeration temperature (4°C) in an airtight container separately until used. The details of the sample preparation procedure were summarized in Figure 1 below.
Experimental Design and Treatment Combinations
The experimental design was carried out with the aid of Minitab software version 16 D-optimal mixture design to generate the treatment combination; accordingly, 13 runs were computer-generated. Upper and lower constraints for each ingredient were decided based on data from food composition tables (Souci et al., 2008) and literatures to fulfill nutritional requirements of children under 2 years. Premix was added to meet recommendations for vitamin and mineral and confer suitable organoleptic characteristics to the flours (Mouquet et al., 2003); this work is the continuation of Forsido et al. (2019). During the experiment, the flour sample from different ingredients was blended according to the proportion generated by Minitab software (13 runs). The blended composite flours were divided into two parts; one part goes for extrusion cooked and the other remains unextruded to compare the effect of extrusion cooking. Laboratory analyses were done separately.
Extrusion Processing
The ingredients were thoroughly mixed for 5 min in a planetary cake mixer (H.LB20/B, Hungary) before extrusion cooking. Independent variables of a co-rotating twin screw food extruder (model Clextral, BC-21 N0 194, Firminy, France) such as material feed rate, water flow rate, barrel temperature of the metering section, moisture content of the raw material, and screw speed were calibrated and adjusted prior to the extrusion cooking process (Forsido et al., 2019). Accordingly, an outlet barrel temperature of 130°C, a screw speed of 150 rpm with the feeder delivering a feed rate of 5.1 g/min, and a feed moisture content of 17% (170 g/kg) were used. The extrudate was allowed to cool to 20°C, and afterward dried in a convective oven at 105°C for 15 min (Semasaka et al., 2010), cooled to room temperature, milled, and sieved (0.5 mm sieve), and the flour was stored in polythene bags at 4°C.
Measurements
Anti-nutrient Factors
Phytate Content Determination
Phytate was determined following the method of Vaintraub and Lapteva (1988). Half gram of composite flour sample was extracted with 10 ml of 2.4% HCl in a mechanical shaker for 1 h at an ambient temperature and centrifuged at 3,000 rpm for 30 min (centrifuge model 800-1). Three milliliters of clear supernatant was collected and mixed with 2 ml of Wade reagent (containing 0.03% solution of FeCl3.6H2O and 0.3% of sulfosalicylic acid in water). The mixture was homogenized and centrifuged at 3,000 rpm for 10 min. A series of phytic acid standard solutions were prepared. The mixtures were centrifuged for 10 min and the absorbance of the solutions (both the sample and standard) was measured at 500 nm using UV-Vis spectrophotometer (T80, China) by using deionized water as a blank. A standard curve was made from absorbance vs. concentration. The amount of phytic acid was calculated using phytic acid standard curve.
Condensed Tannin Content Determination
Condensed tannin content was determined by the method of Maxson and Rooney (1972). Two grams of the sample was added in a screw cap test tube and extracted with 10 ml of 1% HCl in methanol for 24 h at room temperature with mechanical shaking and the solutions were centrifuged at 1,000 rpm for 5 min. One milliliter of supernatant was mixed with 5 ml of vanillin-HCl reagent. D-catechin was used as standard for condensed tannin determination. Forty milligrams of D-catechin was dissolved in 1,000 ml of 1% HCl in methanol and used as stock solution. Stock solutions (0, 0.2, 0.4, 0.6, 0.8, and 1 ml) were added in a test tube, and the volume of each test tube was accustomed to 1 ml with 1% HCl in methanol. Five milliliters of vanillin-HCl reagent was added into each test tube. After 20 min, the absorbance of sample solutions and the standard solutions was measured at 500 nm by using UV-Vis spectrophotometer (T80, China). The calibration curves were prepared using the series of standard solutions. Standard curves were prepared from absorbance vs. concentration and the slopes and intercepts were used for calculation.
where:
A = sample absorbance
B = blank absorbance
d = density of solution (0.791 g/ml).
Functional Properties
Bulk Density (BD)
The bulk density of the composite flour was analyzed according to the method stated by Oladele and Aina (2007) in which a mass of 50 g of the sample was put into a 100-ml measuring cylinder. The cylinder was tapped continuously until a constant volume was obtained. The bulk density was then calculated as weight of the grounded flour (g) divided by its volume (ml).
Water Absorption Capacity (WAC)
Water absorption capacity was determined using the procedure of Adebowale et al. (2005). Ten milliliters of distilled water was added to 1 g of the sample in a beaker and stirred using a magnetic stirrer for 3 min. The suspension obtained was then centrifuged at 3,500 rpm for 30 min, and the supernatant was measured into a 10-ml graduated cylinder. The water absorbed by the flour was calculated as the difference between the initial volume of the sample and the volume of the supernatant.
where:
W1 = weight of flour,
W3 = weight of flour plus tube after centrifuge
W2 = weight of tube.
Statistical Analysis
Minitab version16 software package was used to analyze the data. Normality and constant variance of the error terms were checked. Analyses of variance (ANOVAs) were done to compare variation within 13 treatments of extrusion cooked and 13 unextruded composite flour. Paired t-tests were used to check if there is a significant difference in anti-nutritional factors and functional properties among extruded and unextruded composite flours. The statistical significance of the terms in the regression equations was examined by ANOVA) for each response, and the significance test level was set at 5%.
Results and Discussion
Anti-nutritional Factors of Composite Flour
Phytate
The phytate contents of extruded and unextruded composite flours were indicated in Table 1. ANOVA for models, the interaction of components, and coefficient of determination (R2 value) are summarized in Table 2. ANOVA indicates that there was a significant (p < 0.05) difference in phytate contents of extruded composite flours at the quadratic model; however, there was no significant difference for unextruded composite flour. The coefficient of determination (R2 values) of phytate contents of both extruded and unextruded composite flour indicated that the models could sufficiently predict the responses.
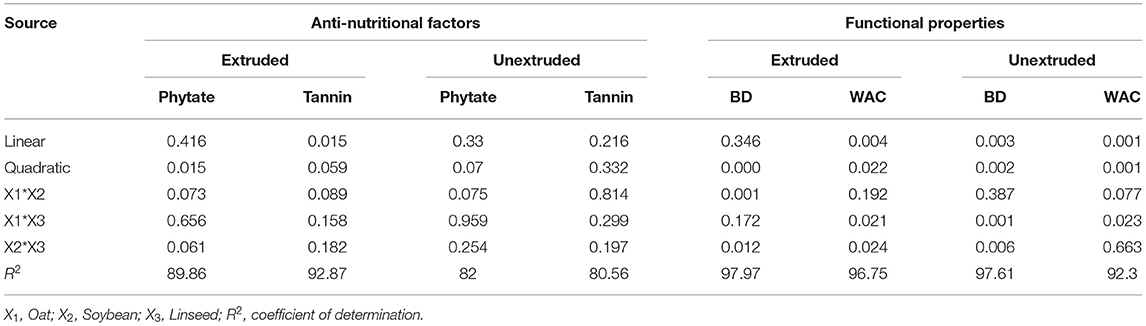
Table 2. Analysis of variance (ANOVA) for anti-nutritional factors content and functional properties.
The phytate content of the extruded and unextruded composite flour ranged from 158.93 to 191.33 mg/100 g and 175.06 to 203.10 mg/100 g, respectively (Table 1). The average daily intake of phytate was estimated to be 2,000–2,600 mg for vegetarian diets as well as diets of inhabitants of rural areas of developing countries and 150–1,400 mg for mixed diets (Reddy, 2002). The value of phytate obtained in the present study is lower compared to the acceptable concentrations. This might be due to processing conditions followed during sample preparation (dehusking, boiling, and mild temperature roasting) and extrusion cooking.
The lowest phytate content was recorded at the lower proportion of soybean and a high proportion of oat and linseed in the oat–soybean–linseed blend for both extruded and unextruded composite flours. The highest phytate content was recorded at the maximum portion of soybean in oat–soybean–linseed blend for the extruded and unextruded composite flours as indicated in Figures 2A,B respectively.
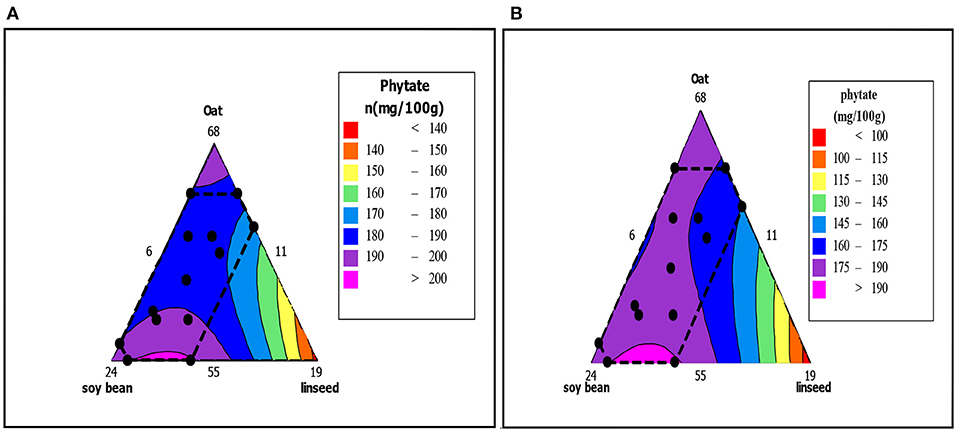
Figure 2. Contour plot of phytate content (mg/100 g) extruded (A) and unextruded (B) complementary flour.
This result was in agreement with previous findings. Liener (2000) reported that phytate content of bread increased as the amount of soybean flour increased in the bread. This increase is because of the high amount of phytate found in soybeans. Tajoddin et al. (2011) also reported that phytate content is high in legumes and it decreases the bioavailability of essential minerals and protein by forming insoluble phytate–mineral and phytate–protein complexes. Phytate present in raw materials and foods of plant origin is suggested to be a significant factor responsible for lowering the availability of minerals and some proteins (Shimelis and Rakshit, 2005).
Paired t-test of phytate has shown a significant difference (p < 0.05) between extruded and unextruded composite flour (Table 3). The phytate contents of extruded composite flour were significantly reduced than unextruded composite flour. This might be due to high-temperature treatment during extrusion cooking that degrades the phytate. Similarly, Omosebi et al. (2018) reported that, extrusion process brought about significant reduction in the phytic acid content of complementary diet from quality protein maize and soybean protein concentrate. Osman et al. (2010) also reported that, the apparent decrease in phytate content during cooking may be because of the formation of insoluble complexes between phytate and other components such as phytate–protein and phytate–mineral complexes; this consequently lowers the amount of free phytate.
Phytates bind minerals in the digestive tract, making it difficult for the body to absorb and use these nutrients (Sandberg, 2002; Melaku et al., 2005). Thus, lowering the phytic acid is expected to enhance the bioavailability of such minerals (Anuonye et al., 2010).
Condensed Tannin
The tannin content of extruded and unextruded composite flours ranged from 8.4 to 22.89 mg/100 g and 23.67 to 36.97 mg/100 g, respectively (Table 4). Condensed tannin content of the extruded composite flours showed a significant (p < 0.05) effect at the linear and quadratic model; however, the interaction effect was not significant. The regression models developed to predict the tannin content explained 92.87 and 80.56% of the variations (Table 2) for extruded and unextruded composite flour, respectively.
The highest tannin content was recorded at high proportion of soybean and linseed in the oat–soybean–linseed blend (55:19:11%), and the lowest tannin content was recorded at highest oat proportion in the blend (65:14:6%). The tannin content of the formulas increased with increasing the proportion of linseed and soybean flour in the formulation (Figures 3A,B). This increase might be due to high anti-nutritional (tannin) contents of soybean and linseed. The finding of the present study was in agreement with Samuel et al. (2012) who reported that the amount of tannin in bread increased as the amount of soybean flour increased.
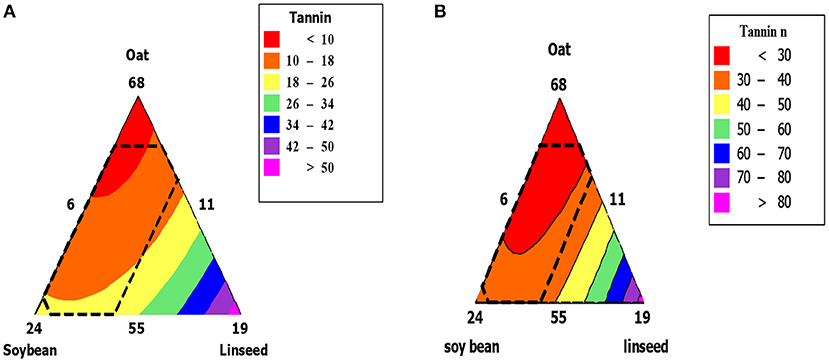
Figure 3. Contour plot of tannin content (mg/100 g) of extruded (A) and unextruded composite flour (B).
Tannins cause decrease iron absorption, alter excretion of cations, increase excretion of proteins and essential amino acids, and consequently damage the intestinal tract, depress growth, and enhance carcinogenesis (Anuonye et al., 2010).
Paired t-test of condensed tannin indicated that there was a highly significant difference (p < 0.01) between extrusion cooked and unextruded composite flour (Table 5). The condensed tannin content of extruded composite flour was lower than unextruded composite flour. The reduction of tannin contents of extruded composite flour might be due to thermal degradation and denaturation of the condensed tannin during extrusion cooking. Similarly, Kataria et al. (1989) reported that the complete reduction in the levels of tannin during extrusion cooking process might be due to thermal degradation and denaturation of the anti-nutrient. About 38.08% tannin reduction recorded in this research is slightly greater than the study of Omosebi et al. (2018) who reported about 20% reduction in tannin content of the extruded complementary diet from quality protein maize and soybean protein concentrate. Anuonye et al. (2010) reported that a 61.22% reduction of tannin contents for extruded pigeon pea-unripe plantain flour blend was greater than the current finding. Similarly, extrusion at high temperature was reported to reduce anti-nutritional factors including phytate and tannins (Kuar et al., 2015; Nikmaram et al., 2017).
The condensed tannin contents for both extrusion cooked and unextruded composite flour can be considered safe since they are below the total acceptable tannin daily intake. The total acceptable tannin daily intake for a man is 560 mg (Ikpeme-Emmanuel et al., 2012). The results showed that the concentrations of condensed tannin in the composite flour were below toxic levels. Generally, besides improving the nutrition properties of complementary food, extrusion cooking can reduce the anti-nutritional factor of complementary foods (Forsido et al., 2019).
Functional Properties of Composite Flour
Bulk Density (BD)
The mean values of BD of unextruded and extruded composite flours are presented in Table 6. The BD ranges from 0.79 to 0.84 g/ml and 0.93 to 0.98 g/ml for unextruded and extruded composite flour, respectively. ANOVA has shown a significant (p < 0.05) difference in BD of extruded and unextruded composite flour in the linear model, quadratic model, and interaction among the ingredients. The regression models developed to predict the bulk density explained 97.61 and 97.97% for unextruded and extruded composite flour, respectively (Table 2). A high coefficient of determination (R2 value) indicates that the model can sufficiently predict the responses. The BD of composite flour was high in the blend at which the proportion of oat was highest and low at the highest percentage of soybean and linseed in the mix, as described in Table 6. Figures 4A,B also showed a similar trend, increasing the proportion of oat increased the BD of composite flour. This could be due to high fiber contents in oat, which contributes to the increase in the bulk density of the flour. The finding of this research was in agreement with the result of Veronica et al. (2006) who reported that as fiber and protein-rich materials are added to starchy materials, the density of the expanded product is increased. Bolarinwa et al. (2015) reported that the bulk density of the composite flour decreased with an increasing level of soybean flour substitution in the mixes. Siddharth (2014) also reported that the higher bulk density might be due to the presence of more crude fiber in the composite flour sample.
Paired t-test has shown no significant difference between extrusion cooked and unextruded composite flour in BD. However, the BD of the extrusion cooked composite flour was higher than the unextruded composite flour. This variation might be due to high temperature during extrusion cooking, which leads to gelatinization of starch that increases bulk densities of the flour. The current result agrees with the report of Meuser and Wiedmann (1989) who reported an increase in bulk density as the temperature increases; this is due to the liquefaction of sugar via melting during the extrusion process. A similar result confirmed that extrusion cooking results in increasing bulk density and water absorption capacity of the extrudate (Filli et al., 2010).
Baby foods should be easy to disperse and have lower paste thickness, both of which are associated with higher BD (Padmashree et al., 1987). Milling affects BD of a product as it reduces the particle size. BD determines the type of package needed for a certain food as it is an indication of porosity of a product (Iwe and Onadipe, 2001). Lower BD is desirable in complementary feeding as it corresponds to higher nutrient content in a smaller volume of the food and thus advantageous in making infant gruel (Kulkarni et al., 1991; Iwe and Ngoddy, 1998; Imtiaz, 2011; Kinyuru, 2012).
Water Absorption Capacity (WAC)
The chemical composition of food such as the presence of water binding the hydrophilic groups and the gel-forming capacity of the macromolecules influences its WAC (Gomez and Aguilera, 1983). Processing-induced damages to starch granules facilitate absorption of a large quantity of water and consequently leads to swelling and increased viscosity (Colonna, 1989).
The value of WAC of extruded and unextruded composite flour ranged between 3.06–3.41 ml and 2.16–2.37 g/g, respectively. ANOVA for extruded composite flours has shown highly significant (p < 0.01) effects in the quadratic model and interaction between oat with soybean and soybean with linseed. ANOVA of unextruded composite flour has indicated significant effects (p < 0.05) in the linear model, quadratic model, and interaction between oat and linseed and between soybean and linseed but no significant difference for the interaction between oat and soybean. The regression models, developed to predict the WAC of the composite flour, explained 92.3 and 96.75% for unextruded and extruded composite flours, respectively (Table 2).
The minimum WAC was recorded at the highest proportion of oat flour in the formulation, and maximum values of the WAC were recorded at the high proportion of soybean and linseed flour in the formulation as indicated in contour (Figure 5). The WAC of the blend was increased with an increasing proportion of soybean and linseed in the blend for both extruded and unextruded composite flour. This increase may be due to the high WAC of soybean as compared to oat; the protein denatured during extrusion cooking made the soybean flour absorb more water. The finding of this study agrees with the report of Amarjeet et al. (1995), who reported increased water absorption with increased soybean flour fortification. Ojinnaka et al. (2013) also reported that WAC increases with increased inclusion of soybean flour to the samples. Igyor et al. (2011) reported that protein binds water and fat while retaining them. Thus, the availability of soy protein has increased its ability to absorb water. The WAC indicates the amount of water available for gelatinization (Kulkarni et al., 1991).
Paired t-tests indicated that there was no significant difference between extrusion cooked and unextruded composite flour in the WAC. However, WAC of the extrusion cooked composite flour was higher than the unextruded composite. The high WAC recorded in extruded composite flour might be due to starch gelatinization during the extrusion cooking process. High temperature during extrusion cooking results in the extruded product losing its highest amount of water because the extruded product is expected to absorb more water than unextruded products. The increase in water absorption capacity implies high digestibility of the starch. This finding confirms the result of Asare et al. (2012) who concluded that the transformation and modifications could explain the high value of WAC of the extruded product in starches and protein complexes in the products arising from the extrusion process. During extrusion, starch gelatinization will take place, and WAC is an index of gelatinization since native starch does not absorb water at room temperature (Ding et al., 2006). Higher WAC indicates higher protein content in the formulations, which absorbs and binds to more water (Otegbayo et al., 2000). A gruel or porridge prepared for complementary feeding should neither be too thick as it can be difficult for the infant to consume nor too thin that energy and nutrient density is reduced (Michaelsen et al., 2003).
Conclusion
The results revealed that anti-nutritional factor contents of extrusion cooked composite flour have been significantly reduced compared to unextruded composite flour. The extrusion cooking operations (mixing, shearing force, and high-temperature heating) involved could contribute to a reduction of phytate and condensed tannin content of composite flour. The value of phytate and condensed tannin recorded in this study is below the limit of acceptable daily intake. Generally, the findings demonstrated that extrusion cooking conditions, 130°C barrel temperature, 150 rpm screw speed, and 5.1 g/min feed rate, used in this study could be suitable to reduce anti-nutritional factors and improve the functional properties of composite flour. Further investigation is needed to be conducted on different anti-nutritional and functional properties of both extruded and unextruded composite flours, which are not included in this report.
Data Availability Statement
The raw data supporting the conclusions of this article will be made available by the authors, without undue reservation.
Author Contributions
HTD performed the experiments, conceived and designed the experiments, analyzed and interpreted the data, and wrote the paper. SFF conceived and designed the experiments and analyzed and interpreted the data. TB analyzed and interpreted the data. OH conceived and designed the experiments. All authors contributed to the article and approved the submitted version.
Funding
This work was supported by Jimma University College of Agriculture and Veterinary Medicine, and the German Federal Ministry of Education and Research (Grant Number 031A247A).
Conflict of Interest
The authors declare that the research was conducted in the absence of any commercial or financial relationships that could be construed as a potential conflict of interest.
Publisher's Note
All claims expressed in this article are solely those of the authors and do not necessarily represent those of their affiliated organizations, or those of the publisher, the editors and the reviewers. Any product that may be evaluated in this article, or claim that may be made by its manufacturer, is not guaranteed or endorsed by the publisher.
References
Adebowale, Y. A., Adeyemi, A., and Oshodi, A. A. (2005). Variability in the physicochemical, nutritional and antinutritional attributes of six Mucuna species. Food Chem. 89, 37–48. doi: 10.1016/j.foodchem.2004.01.084
Amarjeet, K., Sidhu, J. S., and Singh, B. (1995). Effect of enzyme active Soya flour and sodium streroyl −2-lactylate additions on white bread-making properties. J. Chem. Microbiol. 17, 105–109.
Anuonye, J. C., John, O., Evans, E., and Shemelohim, A. (2010). Nutrient and antinutrient composition of extruded acha/soybean blends. J. Food Proc. Preserv. 4, 45–50. doi: 10.1111/j.1745-4549.2009.00425.x
Asare, E. K., Sefa Dedeh, S., Afoakwa, E. O., Sakyi Dawson, E., and Budu, A. S. (2012). Extrusion cooking of rice groundnut cowpea mixtures–effects of extruder characteristics on nutritive value and physic functional properties of extrudates using response surface methodology. J. Food Proc. Preserv. 36, 465–476. doi: 10.1111/j.1745-4549.2011.00605.x
Black, R. E. L. H., Allen, Z. A., Bhutta, L. E., Caulfield, M., De Onis, M., et al. (2008). Maternal and child undernutrition: global and regional exposures and health consequences. Lancet 371, 243–260. doi: 10.1016/S0140-6736(07)61690-0
Bolarinwa, I. F., Olaniyan, S. A., Adebayo, L. O., and Ademola, A. A. (2015). Malted sorghum-soy composite flour: preparation, chemical and physico- chemical properties. J. Food Proc. Technol. 6, 467–478. doi: 10.4172/2157-7110.1000467
Clemens, S. (2014). Zn and Fe biofortification: the right chemical environment for human bioavailability. Plant Sci. 225, 52–57. doi: 10.1016/j.plantsci.2014.05.014
Colonna, P. (1989). “Extrusion cooking of starch and starchy products,” in Extrusion Cooking, eds C. Mercier, P. Linko, and J. M. Harper (Minnesota: American Association of Cereal Chemists), 247–319.
Dahouenon-Ahoussi, E., Adjou, E. S., Lozes, E., Yehouenou, L., Hounye, R., Famy, N., et al. (2012). Nutritional and microbiological characterization of pulp powder of locust bean (Parkia biglobosaBenth.) used as a supplement in infant feeding in Northern Benin. Afr. J. Food Sci. 6, 232–238. doi: 10.5897/AJFS12.016
Davidsson, L., Galan, P., Cherouvrier, F., Kastenmayer, P., Juillerat, M. A., Hercberg, S., et al. (1997). Bioavailability in infants of iron from infant cereals: effect of dephytinization. Am. J. Clin. Nutr. 65, 916–920. doi: 10.1093/ajcn/65.4.916
De Cruz, C., Kamarudin, M., Saad, C., and Ramezani-Fard, E. (2015). Effects of extruder die temperature on the physical properties of extruded fish pellets containing taro and broken rice starch. Anim. Feed Sci. Technol. 199, 137–145. doi: 10.1016/j.anifeedsci.2014.11.010
Diaz, J. M. R., Kirjoranta, S., Tenitz, S., Penttil,ä, P. A., Serimaa, R., Lampi, A., et al. (2013). Use of amaranth, quinoa and kañiwa in extruded corn-based snacks. J. Cereal Sci. 58, 59–67. doi: 10.1016/j.jcs.2013.04.003
Ding, Q., Ainsworth, P., Tucker, G., and Marson, H. (2006). The effect of extrusion conditions on the functional and physical properties of wheat based expanded snacks. J. Food Eng. 73, 142–148. doi: 10.1016/j.jfoodeng.2005.01.013
EDHS E (2016). Demographic and Health Survey 2016: Key Indicators Report. The DHS Program ICF 363–364.
Filli, K. B., Nkama, I., Jideani, V. A., and Abubakar, U. M. (2010). Influence of extrusion variables on some functional properties of extruded millet-soybean for the manufacture of ‘fura': a Nigerian traditional food. Afr. J. Food Sci. 4, 342–352.
Forsido, S. F., Duguma, H. T., Lema, T. B., Sturm, B., and Hensel, O. (2019). Nutritional and sensory quality of composite extruded complementary food. Food Sci. Nutr. 7, 882–889. doi: 10.1002/fsn3.940
Gilani, G. S., Sarwar Gilani, G., and Wu Xiao, C. (2012). Impact of antinutritional factors in food proteins on the digestibility of protein and the bioavailability of amino acids and on protein quality. Br. J. Nutr. 108(Suppl. 2), S315–S332. doi: 10.1017/S0007114512002371
Gomez, M. H., and Aguilera, J. M. (1983). Changes in the starch fraction during extrusion cooking of corn. J. Food Sci. 48, 40–43 doi: 10.1111/j.1365-2621.1983.tb10747.x
Hoffman, D., Cacciola, T., Barrios, P., and Simon, J. (2017). Temporal changes and determinants of childhood nutritional status in Kenya and Zambia. J. Health Popul. Nutr. 36, 1–13. doi: 10.1186/s41043-017-0095-z
Hotz, C., and Gibson, R. S. (2007). Traditional food processing and preparation practices to enhance the bioavailability of micronutrients in plants-based diets. J. Nutr. 137, 1097–1100. doi: 10.1093/jn/137.4.1097
Hurrell, R. F. (2004). Phytic acid degradation as a means of improving iron absorption. Int. J. Vitamin Nutr. Res. 74, 445–452. doi: 10.1024/0300-9831.74.6.445
Igyor, M. A., Yusufu, P. A., and Sengev, I. A. (2011). Evaluation of physicochemical, functional and sensory properties of fermented fura powder supplemented with soy. Nig. Food J. 28, 113–121. doi: 10.4314/nifoj.v28i2.62679
Ikpeme-Emmanuel, C. A., Ekpeyoung, I. O., and Igile, G. O. (2012). Chemical and protein quality of soybean (Glycine max) and tigernut (Cyperus esculentus) based weaning food. J. Food Res. 1, 1–9. doi: 10.5539/jfr.v1n2p246
Ikujenlola, A. V., and Fashakin, J. B. (2005). The Physico-chemical properties of a complementary diet from vegetable proteins. J. Food Agric. Environ. 3, 23–26.
Imtiaz, H. (2011). Evaluation of weaning foods formulated from germinated wheat and mungbean from Bangladesh. Afr. J. Food Sci. 5, 897–903. doi: 10.5897/AJFS11.180
Inyang, C. U., and Zakari, U. M. (2008). Effect of germination and fermentation of pearl millet on proximate, chemical and sensory properties of instant “fura”- A Nigerian cereal food. Pakistan J. Nutr. 7, 9–12. doi: 10.3923/pjn.2008.9.12
Iwe, M. O., and Ngoddy, P. O. (1998). Proximate composition and some functional properties of extrusion cooked soybean and sweet potato blends. J. Plant Food Human Nutr. 53, 121–132. doi: 10.1023/A:1008056019865
Iwe, M. O., and Onadipe, O. O. (2001). Effect of extruded fall fat soy flour into sweet potato. Flour on functional properties of the mixture. J. Sustain. Agric. Environ. 2, 109–117.
Kataria, A., Chauhan, B. M., and Punia, D. (1989). Antinutrients and protein digestibility (in vitro) of mungbean as affected by domestic processing and cooking. Food Chem. 3, 9–17. doi: 10.1016/0308-8146(89)90003-4
Kim, F. M., Camilla, H., Nanna, R., Pernille, K., Maria, S., Lotte, L., et al. (2009). Choice of foods and ingredients for moderately malnourished children six months to5 years of age. Food Nutr. Bull. 30, 344–345. doi: 10.1177/15648265090303S303
Kinyuru, J. N. (2012). Development and evaluation of complementary foods based on traditional foodstuffs in Western Kenya (Ph.D. Dissertation), Jomo Kenyatta University of Agriculture and Technology, Nairobi.
Kuar, S., Sharma, S., Singh, B., and Dar, B. N. (2015). Effect of extrusion variables (temperature, moisture) on the antinutritional components of cereal brans. J. Food Sci. Technol. 52, 1670–1676. doi: 10.1007/s13197-013-1118-4
Kulkarni, K. D., Kulkarni, D. N., and Ingle, U. M. (1991). Sorghum malt-based weaning food formulation: preparation, functional properties and nutritive value. Food Nutr. Bull. J. 13, 322–328. doi: 10.1177/156482659101300401
Liener, I. E. (2000). Non-Nutritive Factors and Bioactive Compounds in Soy -Soy in Animal Nutrition, ed J. K. Drackley, Savoy, IL: Federation of Animal Science Societies.
Maclean, W. C., López, D. R., and Gastañaduy, A. (1983). The effect of decortication and extrusion on the digestibility of sorghum by preschool children. J. Nutr. 113, 2071–2077. doi: 10.1093/jn/113.10.2071
Masatcioglu, T. M., Ng, P. K., and Koksel, H. (2014). Effects of extrusion cooking conditions and chemical leavening agents on lysine loss as determined by furosine content in corn-based extrudates. J. Cereal Sci. 60, 276–81. doi: 10.1016/j.jcs.2014.06.008
Maxson, E., and Rooney, L. (1972). Evaluation of methods for tannin analysis in sorghum grain. Cereal Chem. 49:719.
Melaku, U., West, C. E., and Habtamu, F. (2005). Content of zinc, iron, calcium and their absorption inhibitors in foods commonly consumed in Ethiopia. J. Food Compos. Anal. 18, 803–817. doi: 10.1016/j.jfca.2004.09.008
Meuser, F., and Wiedmann, W. (1989). “Extrusion plant design,” in Extrusion Cooking, eds C. Mercier, P. Linko, and J. M. Harper (St. Paul, MN: American Association of Cereal Chemists), 91–155.
Michaelsen, K. F., Weaver, L., Branca, F., and Robertson, A. (2003). Feeding and Nutrition of Infants and Young Children. Guidelines for the WHO European Region With Emphasis on the Former Soviet Union. WHO Regional Publication European series 87, 1–296.
Moscicki, L., and van Zuilichem, D. J. (2011). “Extrusion-cooking and related technique,” in Extrusion-Cooking Techniques: Applications, Theory and Sustainability, ed L. Moscicki (Weinheim: Wiley), 1–24. doi: 10.1002/9783527634088
Mouquet, C., Salvignol, B., Van Hoan, N., and Monvois Tre‘Che, J. S. (2003). Ability of a “very low-cost extruder” to produce instant infant flours at a small scale in Vietnam. Food Chem. 82, 249–255. doi: 10.1016/S0308-8146(02)00545-9
Nikmaram, N., Leong, S. Y., Kouba, M., Zhu, Z., Barba, F. J., Greiner, R., and Roohinejad, S. (2017). Effect of extrusion on the anti-nutritional factors of food products: an overview. Food Control 79, 62–73. doi: 10.1016/j.foodcont.2017.03.027
Ojinnaka, M. C., Ebinyasi, C. S., Ihemeje, A., and Okorie, S. U. (2013). Nutritional evaluation of complementary food gruels formulated from blends of soybean flour and ginger modified cocoyam starch. Adv. J. Food Sci. Technol. 5, 1325–1330. doi: 10.19026/ajfst.5.3105
Oladele, A. K., and Aina, J. O. (2007). Chemical composition and functional properties of flour from two varieties of tigernut (Cyperus esculentus). Afr. J. Biotechnol. 6, 2473–2476. doi: 10.5897/AJB2007.000-2391
Omosebi, M. O., Osundahunsi, O. F., and Fagbemi, T. N. (2018). Effect of extrusion on protein quality, antinutritional factors, and digestibility of complementary diet from quality protein maize and soybean protein concentrate. J. Food Biochem. 42:e12508. doi: 10.1111/jfbc.12508
Osman, N. M., Mohamed Ahmed, I. A., and Babiker, E. E. (2010). Fermentation and cooking of sicklepod (Cassia obtusifolia) leaves: changes in chemical and amino acid composition, antinutrients and protein fractions and digestibility. Int. J. Food Sci. Technol. 45, 124–132. doi: 10.1111/j.1365-2621.2009.02111.x
Otegbayo, B. O., Sobande, F. O., and Aina, J. O. (2000). Effects of Soy-substitution on physicochemical qualities of extruded plantain Snack. Trop. Oil Seeds J. 5, 69–78.
Padmashree, T. S., Vijayalakshmi, L., and Puttaraj, S. (1987). Effect of traditional processing on the functional properties of cowpea (Vigna Catjang) flour. J. Food Sci. Technol. 24, 221–224.
Reddy, N. R. (2002). “Occurrence, distribution, content, and dietary intake of phytate,” in Food Phytates, eds N. R. Reddy, S. K. Sathe (Boca Raton, FL: CRC Press), 25–51.
Saha, P. R., Weaver, C. M., and Mason, A. C. (1994). Mineral bioavailability in rats from intrinsically labeled whole wheat flour of various phytate levels. J. Agric. Food Chem. 42, 2531–2535. doi: 10.1021/jf00047a029
Samtiya, M., Aluko, R. E., and Dhewa, T. (2020). Plant food anti-nutritional factors and their reduction strategies: an overview. Food Prod. Proc. Nutr. 2, 1–14. doi: 10.1186/s43014-020-0020-5
Samuel, F. O., Otegbayo, B. O., and Alalade, T. (2012). Nutrient and anti-nutrient content of soy-enriched tapioca. Food Nutr. Sci. 3, 784–789. doi: 10.4236/fns.2012.36105
Sandberg, S. A. (2002). Bioavailability of minerals in legumes. Br. J. Nutr. 88, 281–285. doi: 10.1079/BJN/2002718
Semasaka, C., Kong, X. Z., and Hua, Y. (2010). Optimization of extrusion on blend flour composed of corn, millet and soybean. Pakistan J. Nutr. 9, 291–297. doi: 10.3923/pjn.2010.291.297
Shimelis, A. E., and Rakshit, S. K. (2005). Proximate composition and physicochemical properties of improved dry bean (Phaseolus vulgaris L.) varieties grown in Ethiopia. Food Eng. Bioprocess Technol. Progr. 38, 331–338. doi: 10.1016/j.lwt.2004.07.002
Siddharth, M. (2014). Determination of physical characteristics of extruded snack food prepared using little millet (Panicum sumatranc) based composite flours. 2, 213–218.
Singh, S., Gamlath, S., and Wakeling, L. (2007). Nutritional aspects of food extrusion: a review. Intern. J. Food Sci. Technol. 42, 916–929. doi: 10.1111/j.1365-2621.2006.01309.x
Souci, S. W., Fachmann, W., and Kraut, H. (2008). Food Composition and Nutrition Tables. Die Zusammensetzung der Lebensmittel, Nährwert-Tabellen. La composition des aliments Tableaux des valeurs nutritives. Stuttgart: Medpharm Scientific Publishers.
Tajoddin, M. D., Shinde, M., and Lalitha, J. (2011). In vivo reduction the phytic acid content of mungbean (Phaseolus aureus L.) cultivars during germination. Am. Eurasian J. Agr. Environ. Sci. 10, 127–132.
Tariku, A., Bikis, G. A., Woldie, H., Wassie, M. M., and Worku, A. G. (2017). Child wasting is a severe public health problem in the predominantly rural population of Ethiopia: a community based cross–sectional study. Arch. Public Health 75, 1–9. doi: 10.1186/s13690-017-0194-8
Tiwari, A., and Jha, S. (2017). Extrusion cooking technology: principal mechanism and effect on direct expanded snacks - an overview. Int. J. Food Stud. 6, 113–128. doi: 10.7455/ijfs/6.1.2017.a10
Vaintraub, I. A., and Lapteva, N. A. (1988). Colourimetric determination of phytate in unpurified extracts of seeds, and the products of their processing. Anal. Biochem. 175, 227–230 doi: 10.1016/0003-2697(88)90382-X
Keywords: anti-nutritional factors, complementary food, composite flour, extrusion cooking, functional properties
Citation: Duguma HT, Forsido SF, Belachew T and Hensel O (2021) Changes in Anti-nutritional Factors and Functional Properties of Extruded Composite Flour. Front. Sustain. Food Syst. 5:713701. doi: 10.3389/fsufs.2021.713701
Received: 23 May 2021; Accepted: 07 September 2021;
Published: 11 November 2021.
Edited by:
Veronica Calado, Federal University of Rio de Janeiro, BrazilReviewed by:
Abiodun Olapade, University of Ibadan, NigeriaPankaj B. Pathare, Sultan Qaboos University, Oman
Copyright © 2021 Duguma, Forsido, Belachew and Hensel. This is an open-access article distributed under the terms of the Creative Commons Attribution License (CC BY). The use, distribution or reproduction in other forums is permitted, provided the original author(s) and the copyright owner(s) are credited and that the original publication in this journal is cited, in accordance with accepted academic practice. No use, distribution or reproduction is permitted which does not comply with these terms.
*Correspondence: Haile Tesfaye Duguma, aGFpbGV0ZXNmYXllODQwQGdtYWlsLmNvbQ==