- 1Department of Life and Environmental Sciences, University of California, Merced, Merced, CA, United States
- 2Department of Natural Resources and Environmental Management, University of Hawai'i at Mãnoa, Honolulu, HI, United States
- 3Environmental Systems Graduate Group, University of California, Merced, Merced, CA, United States
- 4Department of Civil and Environmental Engineering and Gund Institute for Environment, University of Vermont, Burlington, VT, United States
- 5Sustainable Organic Integrated Livelihoods, Cap Haïtien, Haiti
- 6Critical Ecology Lab, Oakland, CA, United States
Ecological sanitation (EcoSan) systems capture and sanitize human excreta and generate organic nutrient resources that can support more sustainable nutrient management in agricultural ecosystems. An emerging EcoSan system that is implemented in Haiti and several other contexts globally couples container-based household toilets with aerobic, thermophilic composting. This closed loop sanitation system generates organic nutrient resources that can be used as part of an ecological approach to soil nutrient management and thus has the potential to contribute to Sustainable Development Goals 2 (zero hunger), 6 (clean water and sanitation for all), and 13 (climate change solutions). However, the role of organic nutrient resources derived from human excreta in food production is poorly studied. We conducted a greenhouse experiment comparing the impact of feces-derived compost on crop production, soil nutrient cycling, and nutrient losses with two amendments produced from wastewater treatment (pelletized biosolids and biofertilizer), urea, and an unfertilized control. Excreta-derived amendments increased crop yields 2.5 times more than urea, but had differing carry-over effects. After a one-time application of compost, crop production remained elevated throughout all six crop cycles. In contrast, the carry-over of crop response lasted two and four crop cycles for biosolids and biofertilizer, respectively, and was absent for urea. Soil carbon concentration in the compost amended soils increased linearly through time from 2.0 to 2.5%, an effect not seen with other treatments. Soil nitrous oxide emissions factors ranged from 0.3% (compost) to 4.6% (biosolids), while nitrogen leaching losses were lowest for biosolids and highest for urea. These results indicate that excreta-derived compost provides plant available nutrients, while improving soil health through the addition of soil organic carbon. It also improved biogeochemical functions, indicating the potential of excreta-derived compost to close nutrient loops if implemented at larger scales. If captured and safely treated through EcoSan, human feces produced in Haiti can meet up to 13, 22, and 11% of major crop needs of nitrogen, phosphorus, and potassium, respectively.
Introduction
Nutrient recovery from organic waste streams and recycling in agriculture is a critical component to achieving Sustainable Development Goal (SDG) 2, which aims to eliminate hunger (Springmann et al., 2018; Gerten et al., 2020). Current linear modes of fertilizer provision rely on external inputs of nutrient, energy, and water for agricultural production, and non-harvestable resources are predominately wasted and/or lost to the environment. This linear model has local to global consequences for human and ecosystem health. The production and intensive use of fertilizers have perturbed biogeochemical cycles to the extent that the planetary boundary for global nitrogen (N) and phosphorus (P) cycles has already been exceeded (Steffen et al., 2015). Nitrogen fertilizers are produced from the energy-intensive Haber-Bosch process, which converts unreactive atmospheric N in the form of dintrogen (N2) to a reactive form of N, ammonia (NH3). This synthetic fixation of N has more than doubled the amount of N that cascades through the environment in different forms of reactive N (Fowler et al., 2013). Only 4 to 14% of N applied to crops is consumed by humans, with the rest lost to watersheds and the atmosphere (Pikaar et al., 2017). Excess reactive N in the form of nitrate contributes to the eutrophication of water systems and contamination of groundwater. Gaseous nitrous oxide (N2O) is a global warming pollutant and contributes to stratospheric ozone depletion and nitrogen oxides (NOx) contribute to smog and acid rain (Zhu and Chen, 2002; Erisman et al., 2013). Similarly, rock phosphate, the predominant source of phosphorus (P) fertilizers, is a non-renewable resource mined from spatially heterogenous mineral reserves (Cordell and White, 2014). Phosphate rock is a finite resource that will eventually be depleted, although recent estimates indicate no imminent scarcity of these reserves based on future rates of consumption (USGS, 2021). Excess phosphorus that is not taken up by plants can bind to sediments, produce P-rich runoff, and eutrophy water bodies (Sharpley and Menzel, 1987).
The provision of nutrients is a critical component of sustaining agricultural production (Tully and Ryals, 2017). While the extensive production and use of mineral fertilizers was an intentional strategy of the Green Revolution to alleviate hunger, it came at a heavy cost to the quality of air, water, and soil resources (Pingali, 2012). Additionally, hunger and malnutrition have persisted despite the continued increase in fertilizer use. A shift away from inorganic fertilization with synthetic and mined resources toward organic fertilization with natural byproducts and end products could alleviate some pressure on N and P planetary boundaries through reduced nutrient loading to the environment (Drinkwater and Snapp, 2007; Eden et al., 2017; Springmann et al., 2018). Recycling organic waste streams to agricultural soils can provide plant available nutrients to support crop or forage production as well as organic matter to improve soil health and ecosystem services. Therefore, ecological approaches to nutrient management reduce waste and foster more resilient agroecosystems (Schipanski et al., 2016), one of the targets of SDG 2.
The capture and transformation of human excreta represents an enormous and largely untapped strategy for circular models of nutrient management (Harder et al., 2019, 2020; Theregowda et al., 2019). Global production of human feces is projected to pass 1 Pg of wet matter per year (Berendes et al., 2018). Though excreta recovery has been practiced throughout cultures and history (Angelakis et al., 2018), this practice has been largely discontinued due to the advent of centralized wastewater treatment systems and inexpensive inorganic fertilizers (Bracken et al., 2007). The current low cost of synthetic N fertilizer can be attributed to fossil-fuel derived hydrogen, which is used in the Haber-Bosch reaction, and is likely to increase in the coming decades as society shifts away from fossil fuels. Alternative sources of N, including N recovered from human excreta, have the potential to be economically feasible, especially if externalities are accounted for. Feces and urine are nutrient-rich waste streams, with feces containing the majority of excreted carbon (C) and approximately half of P and potassium (K), and urine the majority of excreted N and the remaining P and K (Harder et al., 2019). Distinct treatment of feces and urine requires source separation, a method that is not currently practiced on a large scale in Western societies (Larsen et al., 2013).
One area of resource recovery research is on biosolids, the organic residue from wastewater treatment that results from mixed streams. The agronomic benefits and tradeoffs of nutrient recycling from biosolids have been extensively studied (e.g., Wang et al., 2008; Lu et al., 2012). Recent reviews of biosolids land application highlight the relationship between biosolids and ecosystem services, especially those linked to soil health, and the socioeconomic value of circular sanitation economies (Trimmer et al., 2019; Toffey and Brown, 2020). Diverting biosolids from landfills also contributes to climate change mitigation by reducing landfill methane emissions (Brown et al., 2008) and, when land applied, increasing stocks of carbon (C) in soil (Torri et al., 2014; Villa and Ryals, 2021). Land application of treated biosolids is a common practice, but there remains considerable potential to further recover and safely recycle biosolids nutrients. For example, the United States already incorporates 55% of all treated sewage sludge back onto agricultural lands but continues to landfill 30% and incinerate 15% of all sludge produced (Peccia and Westerhoff, 2015). Regulations aimed at minimizing public health risks of potential pathogens, persistent pollutants, and contaminants limit the application amount, timing, and frequency of biosolids land application, yet regulatory limits vary among regions and countries (Gianico et al., 2021). Historically, regulatory limits were based on concentrations of heavy metals and pathogen loading. More recently, emerging contaminants, like pharmaceuticals, have also driven regulatory limits or concerns over biosolids land application, and knowledge gaps remain about the impact of contaminants like microplastics (Clarke and Cummins, 2015).
Compared to biosolids, less research is available on effective nutrient recycling from non-sewered sanitation systems. This is an important gap in knowledge that could inform ecological approaches to nutrient management in regions and societies experiencing underdevelopment following colonization. Approximately 4.2 billion people globally lack access to safely managed sanitation. Of this population, ~2 billion people globally lack access to basic sanitation and 673 million people practice open defecation [World Health Organization, and (WHO) and the United Nations Children's Fund (UNICEF), 2020]. Global flows of wasted human excreta N, P, and C are highly uncertain, but estimates clearly indicate the significance of non-sewered systems to nutrient imbalances, particularly in low and middle income countries. Between 85–93 and 77–90% of the N and P, respectively, excreted by humans in low and middle income countries is released into the environment without treatment (Fuhrmeister et al., 2015), wasting valuable nutrient resources and causing severe consequences for human and ecosystem health. Conventional sewage that uses fresh water to move and treat human waste is unlikely to meet many of these sanitation needs for several social, economic, and ecological reasons (Öberg et al., 2020). Likewise, current forms of non-sewered sanitation systems that do not ensure safe and effective treatment and land application do not meet societal and environmental health goals, and are not considered ecological nutrient management (Guo et al., 2021). Managing sanitation in a changing climate is a challenge, particularly when systems rely on large quantities of fresh water and centralized, extensive infrastructure that requires significant capital and trained management (Kohlitz et al., 2017). Global efforts to enhance access to adequate sanitation under SDG 6 are driving demand for innovative non-sewered sanitation services, particularly in regions that are rapidly urbanizing and where water resources are scarce (Russel et al., 2019; McConville et al., 2020).
Ecological sanitation (EcoSan) systems have the potential to transform nutrient management by explicitly coupling sanitation and agriculture (Langergraber and Muellegger, 2005; Haq and Cambridge, 2012). EcoSan refers to a suite of practices that aim for closed-loop management of human excreta. EcoSan designs are often non-sewered and feature separate collection and treatment of urine and feces. These systems have been implemented throughout the world, but with tremendous potential for growth (GIZ, 2012; Hu et al., 2016). EcoSan may reduce public health risks while creating a locally accessible source of nutrients, thus increasing food security and agroecosystem sustainability, and bolstering local circular economies (Langergraber and Muellegger, 2005). There is particularly high potential for the implementation of EcoSan in emerging urban environments with dense populations located near cropland, which may help offset mineral fertilizer imports (Trimmer and Guest, 2018). Recommendations for optimal designs of EcoSan systems based on local socio-economic and cultural contexts and safety guidelines have been developed to encourage the adoption of EcoSan services (Reed and Shaw, 2003; WHO, 2006; Simha and Ganesapillai, 2017). However, several interrelated economic, institutional, and political barriers need to be overcome in order to realize large-scale adoption of EcoSan (Sinharoy et al., 2019). In the context of nutrient management, the lack of data on the use of novel organic amendments from EcoSan limits understanding of effects on crop production, nutrient cycling, and water quality effects, which impairs decision makers and inhibits widespread adoption (Smith et al., 2016).
An emerging EcoSan system that has been modeled in Haiti and other low resource settings couples container-based toilets with aerobic, thermophilic composting. This model of EcoSan has been demonstrated at medium-scales (~9,000 toilet users per day in 2020) by the non-profit organization, Sustainable Organic Integrated Livelihoods (SOIL), in Haiti since 2006. SOIL's container-based sanitation (CBS) technology separates urine and feces on-site. Feces are combined with a sugarcane residue and transported to a centralized compost facility for sanitization and processing. Aerobic, thermophilic composting of human feces is effective at reducing pathogenic loads to safe levels, which can help achieve public health goals (Berendes et al., 2015; Piceno et al., 2017). Coupled sanitation-agriculture systems that return both nutrients and organic matter to soil can be particularly beneficial in regions that experience severe soil degradation, food insecurity, and climate vulnerability (Bargout and Raizada, 2013). The most severe impacts of these factors are found in Haiti, where 61.9% of the population cannot afford a nutrient adequate diet, 48.2% of the population is undernourished, and which is not currently on track to meet SDG 2 (FAO, IFAD, UNICEF, WFP and WHO, 2020). Progress on SDG6 is also falling short, as only 37.1% of people have access to basic sanitation services, which means having access to a sanitation facility that is not shared with other households but does not include the safe treatment or disposal of waste (United Nations, 2021). In the Cap Haitïen, the second most populous city in Haiti, only 1% of human excreta is safely managed, primarily by SOIL's EcoSan service (Biscan, 2018). The cause of these vulnerabilities are deeply rooted in the country's institutional and environmental colonial legacy (Dubois, 2013). After the Haitian Revolution succeeded in ending slavery and winning independence from France, the country was forced to pay reparations to their enslavers over the next 100 years, draining financial capital and investment. Further, the birth of Haiti as an independent nation had little support from other nations. The US did not recognize it until 1862, and occupied the country from 1915 through 1934. High rates of deforestation, soil infertility, and natural resource depletion are strongly tied to Haiti's political ecology (Baro, 2002). Thus, the restoration of Haiti's soils through circular systems that support ecological nutrient management is an important component of achieving environmental justice and agroecological resilience, and sustainable production systems.
Our objectives were to determine the responses of plants and soil to organic matter amendments derived from human excreta and to estimate the potential for the recycling of human excreta to meet country-level crop nutrient demands. Specifically, we compared the effects of composted human feces on soil and plant processes to two other human waste products (biosolids and biofertilizer), inorganic fertilization, and an un-amended control. We hypothesized that the application of composted feces to soil would increase crop production, due to the presence of a suite of macro- and micronutrients. We predicted that the boost in crop production would carry-over in time due to slow mineralization of nutrients and improvements in soil health. We further hypothesized these improvements in soil health would lead to lower aqueous and gaseous nutrient losses. We also provide an estimate of the potential for circular sanitation to meet Haiti's crop N, P, and K demands, along with an assessment of hurdles to widespread adoption of circular sanitation-agriculture systems.
Materials and Methods
Experimental Design
We conducted the experiment in a climate-controlled greenhouse at Pope Greenhouse facilities at the University of Hawaii at Mānoa from August 2017 through April 2018. Daily mean temperature was 27.6°C and mean relative humidity was 69.6% over the course of the experiment. Soil used for the experiment was weathered from basic igneous rock with silty clay texture and classified as very-fine, kaolinitic, isohyperthermic Rhodic Eutrustox in the Lahaina series. Soil was collected from the 0 to 30 cm depth of an uncultivated field directly adjacent to an organic agricultural system on Oahu, Hawaii (21.555°N, 158.117°W). The field was previously used for irrigated sugarcane production and had been fallowed for at least 10 years prior to this study. Soil was sieved to 2 mm and mixed well prior to distributing it into growth pots. Each growth pot consisted of a 7.5 L bucket (diameter = 25 cm) perforated for drainage. The base of the pot was layered with a 20 μm Whatman #4 filter followed by 3.5 mm of HCl-rinsed silica sand and 3.5 kg of sieved soil. Each pot was nested inside a second 7.5 L bucket so that leachate could be collected and analyzed for nutrient content.
Potted soils were amended with compost derived from human feces and compared with four other treatments, including biosolids, liquid biofertilizer, urea (46-0-0 N-P-K), and an unfertilized control (n = 3). The compost amendment was generated by SOIL (Kramer et al., 2011), a non-governmental organization that provides ecological-based sanitation services to households and communities in Cap Haïtien, Haiti. SOIL's EcoSan system deploys 20 L container-based, urine-diverting toilets to separate solid (feces) and liquid (urine) waste. Urine is captured in a 4 L plastic container and is disposed of on-site, ideally on a pervious surface, by toilet users. Sugarcane bagasse is used as cover on solid waste after each use. Solid material is collected on a weekly basis and transported to a centralized composting facility where sugarcane bagasse is again used as lining (30 cm on the base of the pile) and cover material (10 cm on top of the pile), resulting in a bagasse-to-feces ratio of 2:1. An aerobic, thermophilic composting process is used to sanitize feces and produce a nutrient-rich soil amendment (Kramer et al., 2011; Ryals et al., 2019). Compost pile temperatures and E. coli concentration are regularly monitoring during the composting process to ensure that thermophilic conditions are achieved. The biosolids amendment was a Class A commercial-grade fertilizer derived from municipal solid waste that has undergone anaerobic digestion, centrifuge dewatering, heat drying and pelletization and was collected from the Honolulu Sand Island Wastewater Treatment Plant (R.M. Towill Corporation, 2017). The liquid biofertilizer amendment was produced from a wastewater treatment facility that uses anaerobic digestion and thermal hydrolysis to produce a pathogen-free liquid biofertilizer (Lystegro, Lystek, Canada). Urea was used as a comparison in this study because it is a widely used synthetic N-based fertilizer. Global production of urea has increased by 25% in the past decade, which outpaces the growth of other N-based fertilizers (e.g., ammonium nitrate; IFASTAT, 2021). All pots were arranged in a randomized block configuration to minimize the effects of microclimate variability within the greenhouse.
All treatments were applied one-time only and immediately prior to the first planting. The application rate for all treatments except for the control was equivalent to 100 kg potentially available N ha−1, which is an intermediate N application rate for the radish crop used in this study, Raphanus raphanistrum sativus (Fox and Valenzuela, 1996; Jawad et al., 2015; Yuan et al., 2015). Plant available nitrogen (PAN) was calculated for each amendment using the following equation:
where Kmin equals mineralizable N. The N mineralization rate of EcoSan compost was estimated at 7% based on the average value of 16 studies measuring N mineralization in composted biosolids between 1977 and 2011 (Rigby et al., 2016). The N mineralization rate of 20% was estimated for the biosolids treatment based on the EPA's recommended rate for anaerobically digested biosolids (United States Environmental Protection Agency, 1995). The N mineralization rate of 40% was provided by the manufacturer for the liquid biofertilizer treatment (Lystek Inc, 2017). All N was considered available for urea, which consists of 46% N. The treatments were added into the pots and incorporated into the soil manually prior to seed planting. Initial soil organic C and total N concentrations and contents and Treatment C, PAN, and total N application amounts are presented in Table 1.
Crop Biomass and Nutrient Content
Nine radish (Raphanus raphanistrum sativus) seeds were sown in each pot, thinned to the three strongest seedlings after 7 days, and harvested after 36 days. Radish was selected for this experiment because of its fast growth rate and time to maturation. Six consecutive crops of radishes were planted in each pot without reapplication of organic amendments or fertilizer. At the end of each crop cycle, radishes were carefully uprooted, washed with deionized water, and divided at the crown to separate above- and belowground plant components. Plant samples were dried at 65°C until a stable dry weight was achieved. Fresh and dry biomass for the above- and belowground components were recorded for all six crop cycles. A subsample of aboveground biomass from each pot after the first two crop cycles was composited and analyzed for macro and micronutrient concentrations at the University of Hawaii Hilo Analytical Laboratory. Calcium (Ca), Potassium (K), Magnesium (Mg), Sodium (Na), Phosphorus (P), Arsenic (As), Copper (Cu), Iron (Fe), Manganese (Mn), Lead (Pb), and Zinc (Zn) concentrations were measured on a Varian Vista-MPX CCD ICP-OES at the University of Hawaii (UH) at Hilo Analytical Laboratory according to the methods described by Zimmermann (2000). Brielfy, samples were dried at 55°C, ground finely, and dry-ashed in a muffle furnace at 500°C. Ash residue is dissolved in 1 M hydrochloric acid prior to analysis on the ICP-OES. Chloride (Cl) concentrations were measured on a Lachat Quickchem 8,500 Series 2 according to the methods described by Jones (2001). Plant nutrient concentrations were multiplied by biomass to calculate nutrient content.
Soil Carbon and Nutrients
Soil organic C and total N concentrations were analyzed immediately after treatment application, and at the end of crop cycles 1, 2, and 6. A subsample of soil was air-dried, ground to a fine powder using a mortar and pestle and analyzed for soil total C and N concentrations on Costech 4,100 Elemental Analyzer at the University of Hawaii at Hilo Analytical Laboratory. Concentrations of exchangeable cations, including calcium (Ca2+), magnesium (Mg2+), potassium (K+) and sodium (Na+), were measured initially and at the end of crop cycle 6 using the ammonium acetate method buffered at pH 7.0 (Lavkulich, 1971) and analyzed on a Thermo iCAP DUO 7,400 ICP-OES at the UH Hilo Analytical Laboratory. Cation exchange capacity was calculated as the sum of base cations. Soils were also analyzed for pH using a slurry method with a 1 to 2 ratio of soil:deionized water.
Soil extractable N [nitrate () + nitrite () and ammonium ()] was measured at five timepoints: immediately after treatment application, and at weeks 3, 6, 9, and 12 of the study. These timepoints are related to the beginning, middle, and end of the first two crop cycles. Three soil cores (1 cm diameter × 10 cm deep) were collected from each pot about 2.5 cm from each radish taproot. To determine amounts of extractable N, 6 g of soil was mixed with 30 mL of 2M KCl, shaken for 60 min on an orbital shaker, and filtered with a Whatman #1 filter. The filtrate was collected, stored in a −20°C freezer, and shipped on dry ice to the University of Hawaii at Hilo Analytical Laboratory for colorimetric analysis on a Lachat Quickchem 8,500 Series 2 (Zimmermann, 1997).
Water and Nutrient Leaching
All pots were watered to field capacity (3,000 mL deionized water to 3.5 kg soil) immediately prior to treatment application. Each pot was watered daily with the same amount of deionized water as needed, typically 100 mL, to maintain approximate field capacity. Excess water equivalent to a 1.85 cm stimulated rainfall event was added approximately weekly to induce leaching events and create variable soil water conditions present in field settings. Leachate was collected 24 h after excess watering events on days 2, 3, 9, 22, 39, 47, 51, 71, 74, 116, 152, and 192, which include four events during the first two crop cycles and events during the fallow period after subsequent crop cycles. The total volume of leachate was recorded for each pot and a 50 mL subsample was collected and stored in at −20 °C. Leachate samples from the first two crop cycles were also analyzed for , NH4+, and phosphate () concentrations. Inorganic N and P in leachate was not measured after the second crop cycle, when concentrations remained below the detection limit of the analyzer.
Concentrations of and NH4+ in each leachate sample were measured by reduction to nitrite and reaction with Griess reagent and the indophenol blue method of reflectometric determination, respectively, using a Reflectoquant (EMD Millipore Corporation; Billerica, MA USA). Lower limits for nutrient detection were < 3 mg for and < 0.2 mg for NH4+. The total mass of N leached in each form was then calculated by multiplying the concentration of NO3-N and NH4-N in each leachate subsample by the total volume of leachate collected during the leaching event. The total mass of N leached from each pot was then calculated by summing the mass of N lost during each leaching event. We then calculated the mean percent N lost via leaching for each treatment group as a function of the amount of total N added to each pot, which varied by treatment since PAN was kept constant.
The concentration of in leachate samples was measured using the phosphomolybdenum blue method of reflectometric determination using a Reflectoquant (EMD Millipore Corporation; Billerica, MA USA). Lower limits for nutrient detection were <5 ppb. The total mass of P leached as was then calculated by multiplying the concentration of PO4-P in each leachate subsample by the total volume of leachate collected during the leaching event. The total mass of phosphorus leached as from each pot was calculated by summing the mass of phosphorus lost during each leaching event.
Greenhouse Gas Emissions
Soil greenhouse gas fluxes were measured prior to and immediately following treatment application and twice per week during the first two cropping cycles. When moisture is held relatively constant, soil greenhouse gas fluxes tend to be highest for the first few days to weeks following application of amendments or fertilizer (Ryals and Silver, 2013). Greenhouse gas fluxes were no longer measured after the second crop cycle, when there were no longer treatment differences observed. Vented static flux chambers (7.5 L) were fitted on top of the growth pots, and 30 mL gas samples were collected from the chamber headspace at 0, 5, 10, 20, and 30-min time points. Gas samples were immediately transferred to 20 mL evacuated glass vials (Wheaton) with a butyl rubber stopper (GeoMicrobial Technologies) and sealed with an aluminum crimp. Samples were analyzed for concentrations of carbon dioxide (CO2), nitrous oxide (N2O), and methane (CH4) on a gas chromatograph outfitted with a thermal conductivity detector, electron capture detector, and flame ionization detector (Shimanzu Analyzer 5,000-A, University of California, Merced). Fluxes were calculated using an iterative exponential curve-fitting approach (Matthias et al., 1978). We summed daily values using linear interpolation between sampling time points to estimate cumulative soil greenhouse emissions over each of the two crop cycles.
Potential for Ecological Sanitation to Meet Crop Nutrient Demands in Haiti
We used available data on crop biomass, crop nutrient demand, and the production of human excreta in Haiti to demonstrate the relevance of EcoSan at scale. We estimated the potential contribution of excreta-derived nutrients to meet annual crop N, P, and K demands in Haiti. Crop nutrient demand was calculated by multiplying the average production from 2017 to 2019 of FAOSTAT-reported crops in Haiti by crop-specific nutrient removal (Roy et al., 2006; FAOSTAT Statistical Database, 2021). Sisal, yam, melon, and chicory root were excluded because adequate removal values were not found. Crops that are grown in Haiti but not included in the FAOSTAT database were excluded from this analysis. Urine and fecal nutrients were calculated using Haiti-specific values in Jönsson et al. (2005) and an estimated Haitian population of 11.26 million. The maximum potential percentage demand met by supply assumed 100% of nutrients embedded in excreta could be recovered. We recognize that this accounts for neither the nutrient losses that occur during the composting process or urine recovery, nor the potential increases in nutrient retention or nutrient use efficiency with elevated soil organic matter. We also considered barriers to widespread adoption of EcoSan and recommend pathways to overcome these barriers.
Statistical Analysis
One-way analyses of variance (ANOVA) were used to determine significant differences in each parameter measured between treatment groups for each crop round. Analyses included a blocking effect to account for potential spatial variation in greenhouse conditions. When the results of the ANOVA indicated a significant difference between means (p < 0.05), a Tukey Post-Hoc Test was conducted to determine differences between specific treatment groups. To assess the changes over time, we used repeated measures multivariate analysis of variance (MANOVA) with aboveground biomass, belowground biomass, total biomass, and soil extractable N as response variables and treatment, time, and treatment × time interactions as model effect factors. Statistical tests were performed using JMP Pro 14.2 (SAS Institute Inc, 1989). Variables that were not normally distributed were log transformed to meet assumptions for ANOVA and MANOVA. Data are reported as mean values followed by ± standard error. Statistical significance was determined as p < 0.05.
Results
Crop Biomass and Nutrient Content
There was a significant treatment effect on crop production after the first crop cycle (p < 0.0001; Figure 1). The strongest total plant biomass response was observed with the human waste amendments, which was more than two-fold greater than urea fertilization (p < 0.0001) and ten-fold greater than the control (p < 0.0001). Treatment differences among human waste amendments were not statistically significant and ranged from 2.3 to 3.5 gdry. Urea increased plant biomass compared to control (p < 0.0001). Treatment effects were similar for both above- and belowground plant components, and the block effect was not significant.
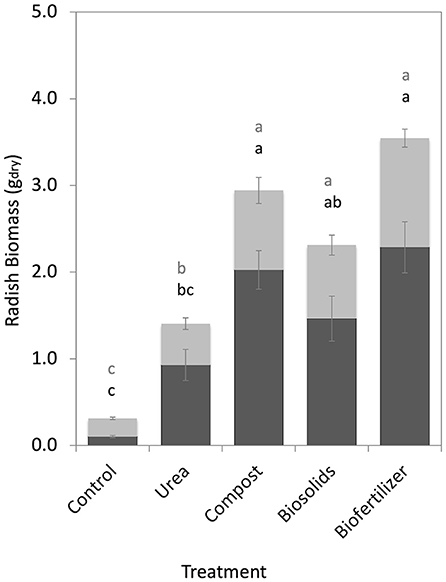
Figure 1. Total plant biomass of the first crop of radishes grown in pots with soils with amendments derived from human excreta, compared to a synthetic N-based fertilizer (urea) and an unamended/unfertilized control. Light bars represent treatment means of dry aboveground biomass, and dark bars represent treatment means of dry belowground biomass. Error bars indicate ± standard error for each biomass component. Differences in lightly shaded letters indicate significant treatment differences for aboveground biomass, whereas darker letter indicate treatment difference of belowground biomass, as determined by an ANOVA and post hoc Tukey means comparison test with significance determined as p < 0.05.
Plant biomass responses to treatment diverged over time following the one-time application (Figure 2). There were significant treatment differences for aboveground, belowground, and total plant biomass through time over the course of all six consecutive crop cycles (p < 0.0001). There was also a significant block effect for aboveground biomass (p = 0.004) and a significant interaction between time and treatment (p < 0.001) for all plant biomass measurements. Urea did not significantly increase plant biomass relative to the control beyond the first crop cycle. The human waste amendments differed in the duration of their carry-over effect on plant biomass. Biosolids increased total plant biomass relative to control for the first two crop cycles, while Biofertilizer had an effect for the first three crop cycles. In contrast, Compost significantly increased plant biomass throughout all six crop cycles, and remained 2–3 times greater than all other treatments at the end of the experiment. Over the course of six crop cycles, cumulative plant biomass from human waste amended soils ranged from 6.2 ± 0.4 gdry (Biosolids) to 11.1 ± 0.5 gdry (Compost), while it was only 3.9 ± 0.3 gdry for Urea and 2.9 ± 0.1 gdry for Control.
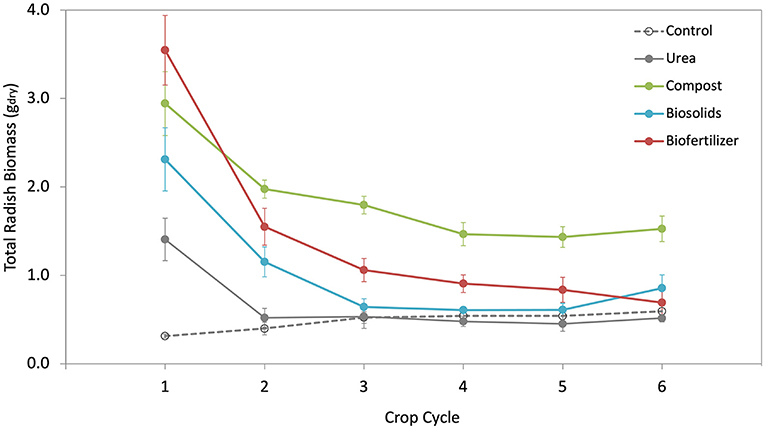
Figure 2. Total plant biomass of six consecutive radish crop cycles. Treatments were added only one-time, prior to planting seeds in the first crop cycle. Symbols represent treatment means at the time of harvest, with ± standard error bars.
Plant tissue nutrient concentrations did not differ greatly among treatments, although some differences were observed (Supplementary Table 1). Compost increased N concentration in leaves by 35% compared to all other treatments in the first crop cycle (p = 0.007), but this effect did not carry-over to the next crop cycle (p = 0.25). Leaf tissue Na concentrations of all amendment treatments were significantly greater than control, but only the Biofertilizer treatment was significantly greater than crop fertilized with urea. In the first crop cycle, leaf Cl tissue was highest for Biosolids (p < 0.001), but no significant difference was detected in the second crop cycle. There were no significant treatment effects in Ca, K, Mg, P, As, Cu, Fe, Mn, Pb, or Zn leaf concentrations.
Soil Organic Carbon and Nutrients
There was no significant difference in total C or N concentrations of the potted soil immediately after treatment application (Day 1; p = 0.43 for %N, p = 0.30 for %C). However, at the end of the first crop cycle (Day 37), we detected a significant treatment effect on soil organic C concentration (p = 0.0011). Compost amended soils had a significantly higher soil organic C concentration (2.48 ± 0.04 %C) than the four other treatments. Soil organic C concentrations of the remaining treatments were not significantly different from the control, which was 2.04 ± 0.05 %C. At the end of six consecutive crop cycles (Day 239), both soil organic C and total N were significantly greater in Compost than all other treatments (Figure 3). Mean soil organic C concentration in Compost was 37% greater and soil total N concentration was 29% greater compared to other treatments. Soil organic C concentration in compost increased linearly through time (R2 = 0.93).
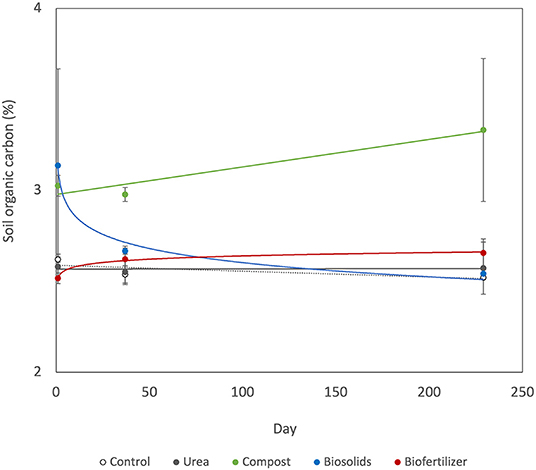
Figure 3. Percent soil organic carbon immediately after adding amendments (Day 1), after the first crop cycle (Day 37), and after the sixth crop cycle (Day 239).
Soil inorganic N pools decreased significantly through time (p < 0.001), with a significant treatment effect (p = 0.019) and treatment and time interaction (p = 0.024). Initial inorganic N pools were approximately two times greater for soils amended with Biosolids and four times greater for soils amended with Biofertilizer, compared to Control. This trend was driven primarily by higher amounts of extractable upon application of these amendments. By the end of the first crop cycle (Day 36), soil inorganic N pools were very low, and not significantly influenced by treatment. Compost and Biosolids amended soils experienced a small pulse in soil extractable N at the mid-point of the second crop cycle, which again subsided by the end of that cycle (Figure 4).
Cation exchange capacity did not differ significant across treatments at the end of the experiment (p = 0.29; Table 2). Base saturation was dominated by calcium (mean 88 ± 0.7% of total CEC) across all treatments. Extractable K+ concentrations were significantly higher than all other treatments (ANOVA p-value = 0.0003; Tukey p-values < 0.005). The urea, biosolids, and biofertilizer treatments had an average of 56% less extractable K+ relative to the control by the end of the experiment. In contrast, the compost treatment had 190% more extractable K+ relative to the control. The compost treatment also had significantly higher concentrations of extractable Mg+ and Na+ compared to the control, urea, and biofertilizer treatments (Table 2). Soil pH averaged 7.83 ± 0.03 across all treatments, and there were no significant differences in soil pH among treatments or through time.
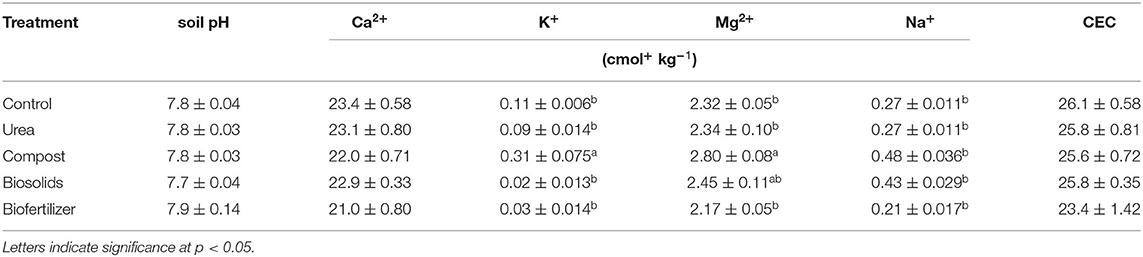
Table 2. Soil pH, extractable nutrients, and cation exchange capacity (CEC) after the sixth crop cycle.
Water and Nutrient Leaching
The amount of water leached from the pots during the first crop cycle varied from 10 to 17% of total amount of water added, and there was a significant treatment effect (p = 0.006). Compost leached significantly less water than Control, Urea, and Biofertilizer (p < 0.05), and marginally significantly less than Biosolids (p = 0.10). The amount of water leached from Biosolids was significantly less than Control. By the end of the sixth crop cycle, the treatment effect on water loss via leaching was considerably stronger compared to the first crop cycle with similar trends (Figure 5). Relative to the amount of water added, 7 and 10% of water was lost via leaching from Compost and Biosolids, respectively. Compost leached 2.4 and 3.2 times less water than the Urea and Control, respectively. There was not a significant difference in water leached between Biofertilizer, Urea, and Control.
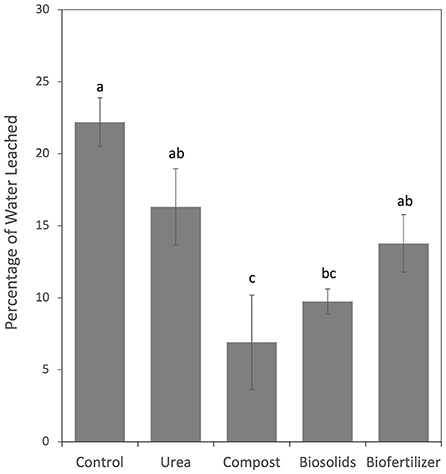
Figure 5. Cumulative water lost via leaching as a percentage of cumulative water applied. Bars represent treatment means. Error bars indicate ± standard error. Letters indicate significant treatment differences as determined by an ANOVA and post hoc Tukey means comparison test with significance determined as p < 0.05.
Inorganic N ( + ) leaching rates were highest at the beginning of the experiment following treatment application, with contributing between 80 and 99% of total inorganic N leached across all treatments. During the first crop cycle, the amount of leached was highest for Urea, whereas the amount of leached was highest for Compost. Approximately 93% of leached from compost occurred within the first three weeks, leading to a total of 709 ± 169 mg N leached from Compost. This amount was an order of magnitude higher than Urea, which had the second highest leaching. There were no significant treatment differences after Day 21. By the second crop cycle (beginning Day 39), dynamics of N leaching changed dramatically, reducing from an average across all treatments of 148 mg -N in crop cycle 1 to 1.9 mg -N in crop cycle 2. Similarly, the amount of -N that leached was reduced from an average of 5.6 to 0.09 mg -N in crop cycles 1 and 2, respectively. There was a significant treatment difference in cumulative N leaching (p = 0.0069). The Urea treatment lost significantly more N via leaching (20% of total N added) than the Biosolids treatment (0.5% of total N added; p = 0.0044). Cumulative N leaching from the Compost treatment (11% of total N added) and the Biofertilizer treatment (8% of total N added) was not significantly different from Urea. We did not detect leaching from any of the treatments.
Soil Greenhouse Gas Emissions
There were no significant treatment differences in soil CO2 fluxes through time or in cumulative CO2 efflux from potted soils (Figure 6A). Fluxes of CH4 were negligible or low throughout the experiment. There were no significant treatment differences in cumulative soil CH4 flux (Figure 6B). Fluxes of N2O were greatest within the first two weeks of the experiment. There was a significant treatment difference in cumulative soil N2O flux (p < 0.0001). Biosolids cumulative soil N2O flux was significantly greater than all other treatments (Figure 6C). Soil N2O emissions factors ranged from 0.03 ± 0.01 (Compost) to 4.5 ± 0.6 (Biosolids). There was a significant treatment difference in soil N2O emissions factors (p = 0.0005). The emissions factor for Biosolids was significantly higher than all other treatments (p < 0.008), except Biofertilizer (p = 0.25). Compost soil N2O emissions factor was significantly less than Biosolids (p = 0.0004) and Biofertilizer (p = 0.004) and marginally significant compared to Urea (p = 0.10).
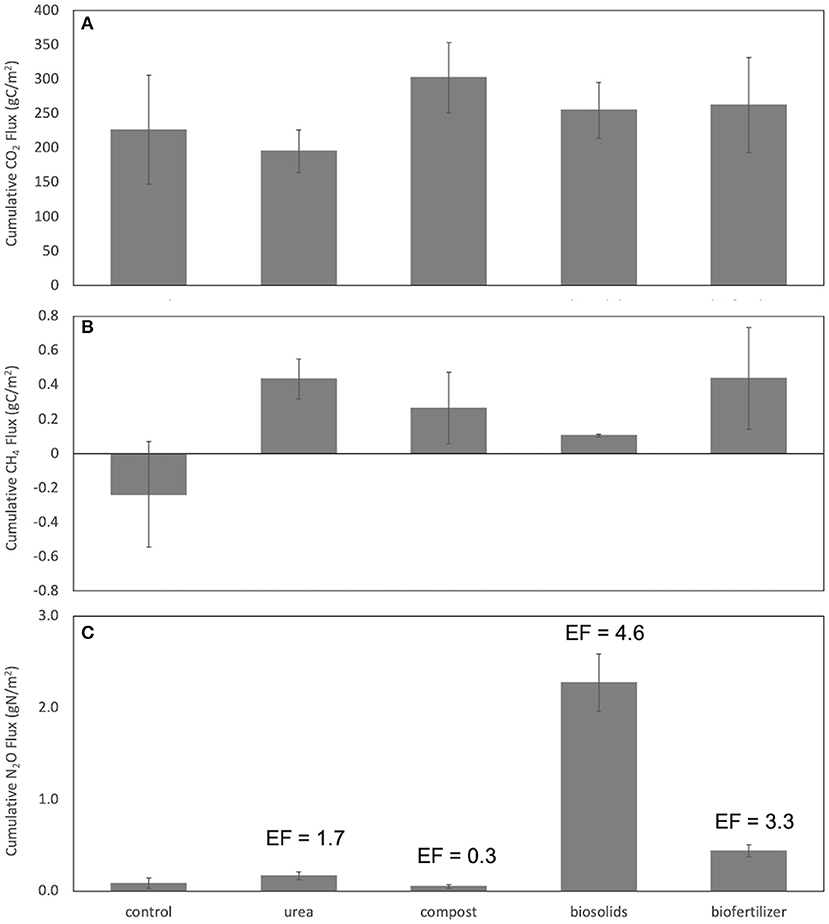
Figure 6. Cumulative soil emissions of (A) CO2, (B) CH4, and (C) N2O during the first two crop cycles from days 1 through 73. Bars represent treatment means. Error bars indicate ± standard error. EF indicates treatment N2O emissions factors, calculated as the percent of total N added lost as gaseous N2O.
The fate of total added N was summarized in a partial N mass balance (Figure 7). We calculated a partial N mass balance based on the amount of measured N losses from treatments relative to the unfertilized control, including inorganic N leaching, N2O–N, and plant N uptake. Nitrogen losses accounted for from the Compost treatment exceeded the amount of N added in units of PAN, largely due to initial NO3 leaching losses. The highest proportion of unaccounted N losses (76% of PAN added) was from the Urea treatment, while 20 and 56% of PAN added in Biosolids and Biofertilizer treatments were unaccounted for. We also observed increases in soil total N in the Compost and Biofertilizer treatments which were greater than cumulative N losses. In contrast, soil total N from the Urea treatment declined slightly over time relative to the control.
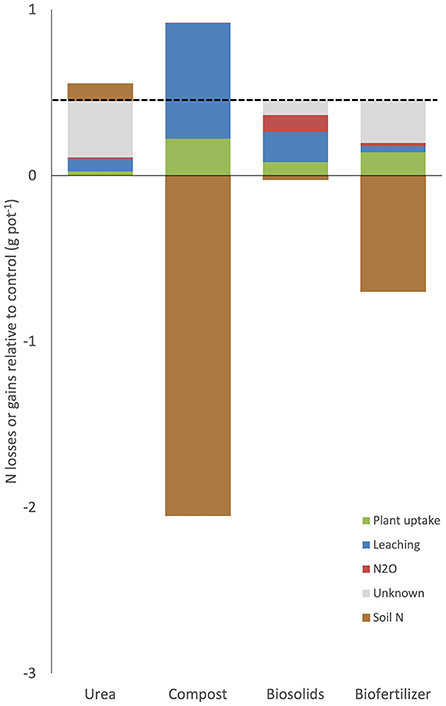
Figure 7. A partial N mass balance illustrating the losses of added PAN from pots in the forms of plant N uptake (green), N2O–N emissions (red), and inorganic N leached (blue). Nitrogen mass loss from each of these pathways was determined as treatment minus control to account for plant and microbial use of N without amendments or fertilizer. Gray bars indicated unknown losses of N, which may include N2 or other forms of reactive N. The black dotted line indicates the amount of PAN added to each pot. Brown bars represent. Brown bars represent treatment differences (treatment—control) in soil total N. Positive values indicate fluxes to the environment, whereas negative values indicate N sinks in the pots.
Potential for Ecological Sanitation to Meet Crop Nutrient Demands in Haiti
Average annual crop production (2017–2019) of 24 FAOSTAT crop types in Haiti was 3.4 MMt yr−1 (Supplementary Table 2). Five crops—sugar cane, mangoes, bananas, avocados, and rice—contributed nearly 85% of total annual crop production. Total N, P, and K demand from annual crop production is presented in Table 3. The capture and transformation of human excreta via composting recovery could potentially supply 13, 22, and 11% of crop N, P, and K (Table 3), assuming 100% nutrient recovery. Urine, which is currently disposed of on-site in SOIL's sanitation system but also has the potential for nutrient recovery, could meet an even greater amount of crop nutrient demand, potentially up to 83, 44, and 32% of N, P, and K. Several ecological, technical, and sociocultural barriers impede the widespread adoption of coupled sanitation-agriculture systems (Table 4).
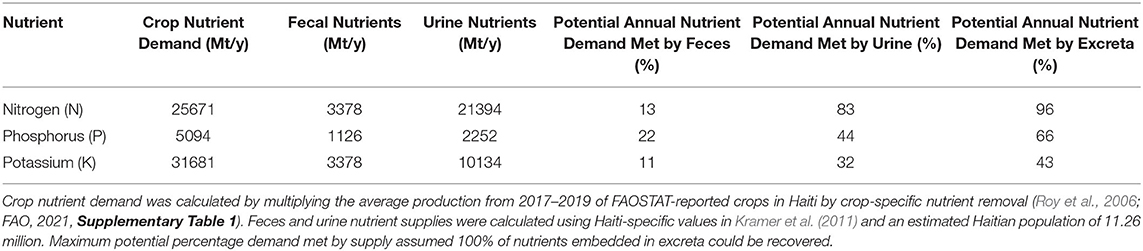
Table 3. Estimated potential contribution of excreta-derived nutrients to meet crop N, P, and K demand in Haiti.
Discussion
Crop and Soil Responses to Human Waste Amendments
We measured the response of plant production to a one-time application of three human excreta-derived soil amendments: compost from container-based EcoSan, pelletized biosolids from wastewater treatment, and biofertilizer from thermal hydrolysis after wastewater treatment. In the first crop cycle, all three excreta-derived amendments increased plant production significantly relative to urea-fertilized and unfertilized control soils. These results align with short-term greenhouse and field studies of excreta-derived amendments (e.g., Sumner, 2000; Elliott and O'Connor, 2007; Moya et al., 2019a; Brown et al., 2020).
The agronomic effects of biosolids have been well studied in the context of urban waste management (Brown et al., 2020). Sustained increases in crop yields, reduction in N fertilizer requirements, and increases in soil organic matter have been observed with the application of biosolids to vegetable production systems and urban gardens across a range of soil types and climates (e.g., Ozores-Hampton and Peach, 2002; Alvarez-Campos and Evanylo, 2019). Residues from wastewater treatment can also be treated further with thermal hydrolysis to produce liquid biofertilizers that are rich in mineral N and beneficial microorganisms. Liquid biofertilizers have been less extensively studied relative to biosolids, and little information is available on the effects of soil nutrient cycling and loss associated with their application. Recent studies indicate similar positive benefits to plant production (Badewa and Oelbermann, 2020; de Matos Nascimento et al., 2020). Our results corroborate existing studies on the agronomic benefits of biosolids and biofertilizer, and provide new information on the longevity of these responses across crop cycles.
There are few experiments testing the effect of EcoSan compost on plant production and soil nutrient cycling processes. EcoSan compost has been shown to increase yields of banana (Jothimani et al., 2013), maize (Krause et al., 2016), marigold (Jothimani and Sangeetha, 2012), cauliflower (Sharda and Shinjo, 2020), and lettuce (Schröder et al., 2021) relative to standard fertilization practices in the given contexts. There is a paucity of data on the effects of EcoSan compost on soil physiochemical properties and nutrient losses. We found that, while EcoSan compost did not significantly alter soil CEC, it did increase the concentrations of exchangeable K+ and Mg2+ in a soil with deficient levels of these macronutrients. While tissue K+ and Mg2+concentrations of radishes grown in soils blended with EcoSan compost were not significantly higher than those of other treatments, alleviation of these macronutrient deficiencies likely contributed to greater biomass. A field experiment comparing EcoSan manure to a mineral fertilizer in cauliflower production systems also observed increases in crop production and plant uptake of N, P, and K (Sharda and Shinjo, 2020).
Only a handful of studies exist that conduct a nutrient mass balance in the agricultural use of EcoSan compost (e.g., Krause and Rotter, 2018; Schröder et al., 2021). The effects of EcoSan compost on water infiltration, nutrient leaching, or greenhouse gas emissions are similarly poorly studied. Our study provides some of the first observations of these parameters in soils treated with EcoSan and can be used to inform future research. Extrapolation of observations from pot studies can be useful for building conceptual models and predicting treatment effects, but field experiments are necessary for furthering understanding of plant-soil feedbacks (Forero et al., 2019). Additional studies are needed in field contexts, across multiple climate and edaphic conditions, and through time to refine our understanding of the fate and transport of recycled nutrients in EcoSan systems. In our greenhouse experiment, we detected treatment differences in N loss pathways as a function of N applied and compared to an unfertilized control. This approach assumes that measured N losses were derived from the fertilizer or organic amendments. However, the addition of fertilizers or organic matter amendments to a soil can induce positive or negative priming effects, thereby changing rates of C and N mineralization of soil organic matter that are non-additive (Jenkinson et al., 1985; Kuzyakov et al., 2000). Field studies using stable isotopes of 15N and 13C can be used to quantify the direction and magnitude of potential priming effects, improve our understanding of the fate of added nutrients, and clarify the nutrient use efficiency of these novel organic amendments (Gardner and Drinkwater, 2009; Lerch et al., 2019).
Our results, combined with a handful of other available studies, suggest that nutrients in human excreta-derived amendments provide a viable substitute for mineral fertilizers by building soil organic C and promoting internal nutrient cycling. Maintaining or increasing soil organic C is a central principle of ecological nutrient management because of its role in the biological, physical, and chemical functions of soil (Reeves, 1997; Tully and Ryals, 2017). We found the EcoSan compost has the potential to increase soil organic C content, and numerous studies have reported the carbon sequestration potential of compost derived from other feedstocks (e.g., (Ryals et al., 2014; Mar Montiel-Rozas et al., 2016; Tautges et al., 2019) even when considering life cycle greenhouse gas emissions (DeLonge et al., 2013; Martínez-Blanco et al., 2013). Our observed carry-over impact on plant production with EcoSan compost suggests improved internal nutrient cycling. The timeframe for which EcoSan compost can continue to supply nutrients after application remains an important question that could inform best management practices for application amount and frequency through time. Higher rates or frequency of EcoSan compost may be needed early on while soil organic matter is accruing, however application rates could potential decrease as internal nutrient cycling processes are enhanced through time. This could extend the agricultural areas that could receive and benefit from ecological nutrient management using EcoSan compost. Long-term field trials across multiple soil types, crop types, and climates are needed to better quantify agronomic and ecological benefits and potential consequences of EcoSan compost use in agriculture.
We found distinct longevities of the carry-over effect of the soil amendments. The interannual boost in plant production after a one-time application of an organic matter amendment can be attributed to the slow-release of nutrients initially present in organic forms, as well as changes in soil properties that continue to promote plant production (Habteselassie et al., 2006; Ryals and Silver, 2013). We detected no carry-over effect for urea, indicating that the N that was not taken up by the crop within the first season is lost to the environment, rather than conserved in the soil (Peoples et al., 2004). In contrast, crop production was significantly greater than the control for two and four crop cycles for the biosolids and biofertilizer treatments, respectively. This result was surprising since biosolids contained more organic C and total N than the biofertilizer. A possible explanation is that there were greater N gaseous and leaching losses from the biosolids-amended soils, whereas unintended losses from the biofertilizer-amended soils were minimal. The carry-over effect lasting the longest was in the compost treatment. Crop production declined slightly in the second crop cycle, but was elevated by about two-times more than the control throughout all six crop cycles. The longer carry-over effect in the compost amended soils can be attributed, in part, to a greater amount of total N added in the compost treatment (6.46 gN/pot in Compost compared to 2.26 gN/pot for Biosolids and 0.61 gN/pot for Biofertilizer; Table 1) since treatments were applied based on equivalent PAN. However, differences in total N do not fully explain treatment differences in carry-over effect as evidenced by the longer carry-over effect of biofertilizer relative to biosolids. Compost may have also had a stronger improvement on soil structure and aggregate stability, which could have also contributed to the trends in water leaching.
Potential for Ecological Sanitation to Meet Sustainable Development Goal 2
Direct and indirect crop benefits from the application of organic matter amendments are widely documented across agricultural production systems (Diacono and Montemurro, 2011). By providing a source of essential macro- and micronutrients for plants, organic matter amendments can relieve nutrient limitations to productivity that are inherent in a soil or created by extraction through biomass harvesting or soil degradation. Nutrients that are complexed with organic matter become available through a microbially-mediated mineralization process, thus providing a slow-release fertilizer that benefits crop growth beyond a single growing season. Indirectly, organic amendments benefit crops by increasing the soil organic matter pool. Soil organic matter is associated with a multitude of biological, physical, and chemical soil functions and is a key component of soil health (Kibblewhite et al., 2008). This increase in soil organic matter stocks can foster more resilient and productive agroecosystems through improved soil health and soil structure. The nutrients and organic matter embodied in human excreta are a vastly underutilized resource to produce organic amendments. In our experiment, we found that EcoSan compost was an effective source of plant available nutrients. The organic matter embodied in the compost improved soil functions, which has been shown widely in land application studies that use compost from different feedstocks (e.g., Goswami et al., 2017; Bekchanov and Mirzabaev, 2018; Lehmann et al., 2020).
We considered the potential for nutrient recovery from human excreta to meet nutrient demand in Haiti. EcoSan is currently deployed at small- to medium-scales in Haiti, primarily through container-based sanitation coupled with centralized aerobic, thermophilic composting. Human feces, if collected and safely treated, could supply 13, 22, and 11% of the country's N, P, and K crop demand, as well as provide a source of organic matter. For perspective of the considerable potential for circular sanitation economies to supply nutrients for agriculture, human urine and feces has the potential to meet 22% of P demand on a global-scale (Mihelcic et al., 2011). Emerging technologies for nutrient recovery from urine, including alkaline dehydration (Simha et al., 2020), suggest higher potentials, with urine alone able to meet 35% of N and 25% of P demands (Simha, 2021). If nutrients were also recovered from urine in Haiti, an additional 83, 44, and 32% of the country's crop demand of N, P, and K could be met. In SOIL's current toilet design, urine is disposed of on-site due to difficulties with transporting large volumes of liquid. Therefore, future research and investment in urine nutrient recovery is needed to make significant advancements in achieving SDG 2 through circular sanitation.
Interactions With Other Sustainable Development Goals
Transitioning to ecological nutrient management using compost from closed-loop sanitation systems not only contributes to eliminating hunger (SDG 2), but also has reinforcing and indivisible interactions with multiple other SDGs, particularly the goals of clean water and sanitation for all (SDG 6) and climate change action (SDG 13; Nilsson et al., 2016). EcoSan technologies are designed with the explicit aim of returning nutrients to agricultural soil (Hu et al., 2016). In these closed-looped nutrient systems, the goals of providing safely managed sanitation and ending hunger worldwide are inextricably linked. We found that these goals are complementary. The sanitation process used in this study consumed little water compared to flush toilets (Haq and Cambridge, 2012), provided a safe and dignified sanitation option (Russel et al., 2015), and produced a nutrient-rich, pathogen-free compost (Berendes et al., 2015; Piceno et al., 2017). Compost yielded sustained increases in plant production for multiple crop cycles and was most adept at retaining water. These findings suggest that the land application of feces-derived compost increases the resiliency of agroecosystems.
Circular nutrient management through EcoSan can also be an important, and overlooked, climate change solution. EcoSan can support climate change solutions in five ways. First, greenhouse gas reductions can be achieved by converting from alternative waste management fates (Ryals et al., 2019; McNicol et al., 2020). Second, soil greenhouse gas emissions can be avoided from the displacement of mineral fertilizers by compost. In Haiti, mineral fertilizer use is very low (Bargout and Raizada, 2013), but this offset may be large in regions where fertilizers are commonly used. In our study, soil nitrous oxide emissions were least from composted soils and most from biosolids amended soils. Third, EcoSan can promote soil carbon sequestration. Compost has high potential to increase soil organic C pools in agricultural settings (Ryals et al., 2014; Paustian et al., 2016), but the extent to which compost derived from human feces impacts soil carbon has not been well documented. We found a significant increase in soil C from a one-time application of compost, an effect that was not observed in any other treatment. Fourth, increases in soil organic matter from EcoSan compost can help agroecosystems adapt to climate change by increasing resiliency to drought and flooding conditions. In this study, we also found that compost leached the least amount of water, suggesting increased soil water retention and greater water use by plants. Finally, EcoSan services themselves can be climate resilient in design by, for example, using container-based toilets that can be easily sealed with a watertight lid or elevated in the event of a flood.
Food insecurity (SDG 2) and poverty (SDG 1) are partially driven by low soil fertility, which is in turn influenced by farming and forestry practices. However, focusing on the roles of soil fertility and the farming decisions of smallholder farmers oversimplifies the drivers of these chronic problems and impedes our ability to meaningfully address the SDGs. In countries like Haiti, where colonial subjugation and later neoliberal economic subordination have shaped food systems, circular nutrient management may also offer an alternative natural resource that increases independence (Steckley and Shamsie, 2015; Trimmer et al., 2020). In addition to providing climate change solutions, EcoSan amplifies the positive effects of domestic food security and sanitation through increased domestic community self-sufficiency and reduced dependence on foreign food aid and agronomic intervention (Wanner, 2015). The establishment of a circular nutrient economy may offer an alternative to historical international efforts to reduce poverty by prioritizing a dependent, export-driven agro-economy (Otero et al., 2013). We show that the improvements to soil fertility and water retention in the closed-loop sanitation system are poised to increase crop production and potentiate economic growth at the local community scale without necessitating economically and ecologically unsustainable resource inputs (e.g., synthetic fertilizers, large scale irrigation). Current efforts by foreign countries and non-governmental organizations to “open” Haiti's food markets to be export-driven require an increase in synthetic fertilizer application from the low rate of nitrogenous fertilizer application of 7670 tons km-2 year-1 as of 2001 (Bargout and Raizada, 2013). The required inputs for increased export crop production would contradict the objectives of climate action (SDG 13) and making cities and human settlements inclusive, safe, resilient, and sustainable (SDG 11) by first increasing leaching and gaseous efflux of nutrients from soils, and then by making local communities vulnerable to foreign policies and the global economy, which have historically subordinated the Haitian (Otero et al., 2013). Finally, by increasing farmers' physical and financial access to locally derived nutrients for farming and therefore their potential income, implementation of EcoSan systems may serve to promote economic equality within strongly class-stratified societies like Haiti.
Barriers and Drivers of EcoSan Compost
Current ecological, institutional, and cultural barriers hinder the widespread implementation of coupled sanitation-agriculture systems (Table 4). EcoSan toilet user attitudes toward source separation and nutrient recovery may be agreeable (Lamichhane and Babcock, 2013; Russel et al., 2015; Simha, 2021), yet other barriers likely impede the large-scale adoption of these practices. For example, a recent multinational survey by Simha (2021) demonstrates that among university community members, there was greater acceptance for human urine recycling than acceptance of disposal, and widespread belief that human urine can be safely used as a crop fertilizer. However, of all options to manage human urine, sending it to a wastewater treatment plant (WWTP) was the most popular among respondents. They argue that for widespread acceptance, urine-diverting sanitation systems must be connected to treatment systems that function as well as or better than WWTPs. 17 Simha et al. (2017) identified and analyzed factors affecting farmers' attitudes on human waste recycling in Vellore district, India. A lower willingness to recycle feces they attribute in part to “faecophobia” and assert that while there may be cultural underpinnings to this view, more work should be done to understand the origins of faecophobia rather than assume that feces recycling should not be pursued due to persistent disapproval. Recent work by Fischer et al. (2021) emphasizes the need for recognition of the social and political context in which a sanitation technology is situated, in reference to the business failure of the Peepoo toilet bag in Nairobi, Kenya.
In the specific context of SOIL, a 2015 study following SOIL's service pilot found that 71% of participating households were willing to pay to continue receiving the container-based EcoSan service (Russel et al., 2015). However, in 2019, Russel et al., identified challenges related to large-scale implementation container-based sanitation EcoSan, including the higher operational cost due to providers addressing the full sanitation value chain. However, they suggest that container-based sanitation may ultimately be more cost-effective by using novel treatment technologies that include resource recovery. Here, we provide a summary of barriers to widespread implementation of EcoSan systems and recommendations to overcome barriers based on literature and practical experience (Table 4), with a specific focus on ecological barriers. Our research provides new data on the agronomic and ecological drivers of EcoSan compost.
Ecological barriers to widespread adoption of EcoSan compost can be addressed through knowledge sharing, assessment of local resources, and new scientific research. Chemical fertilizers are often promoted through agricultural extension and subsidized by government programs (Moya et al., 2019b). Education about the importance of soil health promoting practices such as organic amendment application, and studies on the long-term effects of feces-derived compost could shift culture away from exclusive reliance on chemical fertilizers. Composting requires addition of a C-rich bulking agent (e.g., sugarcane bagasse) to maintain an optimal C:N ratio for aerobic decomposition (Moya et al., 2019b). Adequate bulking agent must be supplied and maintained at the user level for application after toilet use and be available to the composting facility for large scale thermophilic composting (Russel et al., 2019). Regional agroecological and economic analyses could supply valuable data on the availability and feasibility of various organic waste streams to serve as compost bulking agents.
Limitations and Future Research Directions
We found that soil amendments derived from human excreta promoted plant production and improved soil nutrient cycling compared to urea, a nitrogen-based fertilizer, in a greenhouse study. Further, we found significant carry-over effects for potted soils amended with EcoSan compost. While these results point to the promising role of human excreta in contributing to the SDG 2 goal, there are some limitations to our study that require further research. The fertilizer comparison used in this study was urea, a N-based fertilizer that did not contain other macro- or micronutrients that can also limit plant growth. We were unable to quantify gaseous fluxes of ammonia, which is often the major N loss pathway from urea fertilization of soil (Rawluk et al., 2001). Our results are also constrained by methodological limitations of pot studies, including controlled environmental conditions that are different from field conditions and disturbance of soil physical and biological properties (Dalling et al., 2013).
Multiple knowledge gaps on the ecological benefits and risk of composted human feces must be addressed to fully realize the potential of EcoSan as a solution to hunger. Detailed land application studies on the effect of various application rates of composted feces on crop yield, greenhouse gas emissions, soil carbon sequestration, and soil health could supply site-specific data. This would enhance the capacity of local agricultural extension and technical assistance agencies to promote composted feces. Studies should include the net climate change mitigation potential of compost application to proximate cropland, accounting for transport costs of bulky compost material. Evaluations of the sustainability of EcoSan must also include risk assessments of potential inadvertent consequences to human health. Risks of the fate and transport of pharmaceuticals, persistent pollutants, and other emerging contaminants in EcoSan products should be better understood in order to develop strategies to minimize risks to people and the environment (Krause et al., 2021).
Conclusion
The capture of human excreta and its transformation into a resource for agricultural is an underutilized solution toward ending hunger. We show that soil amendments derived from human waste had multiple benefits to crop production and soil nutrient cycling. EcoSan compost boosted plant production, which remained elevated relative to control after six consecutive crop cycles. This finding indicates there are both short-term benefits from plant-available nutrients and long-term benefits to soil health and nutrient mineralization. Transformation of human feces and recycling as a soil amendment could potentially provide 13, 22, and 11% of annual crop N, P, and K demand within Haiti, a country with an urgent need for both improved sanitation and soil restoration. Urine, which is currently not included in local EcoSan nutrient recovery systems, could provide an additional 83, 44, 32% of annual crop N, P, and K demand in Haiti. Thus, EcoSan compost can contribute to SDG 2 by creating resilient and productive agroecosystems, particularly those farmed by small-scale producers, and also intersects with multiple sustainable development goals.
Data Availability Statement
The raw data supporting the conclusions of this article will be made available by the authors, without undue reservation.
Author Contributions
RR and KP contributed to conception and design of the study and performed the statistical analysis. RR and SH collected and analyzed soil, plant, water, and gas samples. RR wrote the first draft of the manuscript. EB and SP wrote sections of the manuscript. All authors contributed to manuscript revision, read, and approved the submitted version.
Funding
This work was supported with research funding to RR from the Department of Life and Environmental Sciences at University of California, Merced and the Department of Natural Resources and Environmental Management at University of Hawai'i at Mānoa.
Conflict of Interest
The authors declare that the research was conducted in the absence of any commercial or financial relationships that could be construed as a potential conflict of interest.
Publisher's Note
All claims expressed in this article are solely those of the authors and do not necessarily represent those of their affiliated organizations, or those of the publisher, the editors and the reviewers. Any product that may be evaluated in this article, or claim that may be made by its manufacturer, is not guaranteed or endorsed by the publisher.
Acknowledgments
We thank Naomi Jun for assistance with greenhouse gas sampling and Dr. Steve Hart for use of his gas-chromatograph. We also thank the Honolulu Sand Island Wastewater Treatment Plant, Lystek International, and Sustainable Organic Integrated Livelihoods for contributing the organic matter amendments used in this study. Finally, we thank two reviewers whose insightful comments improved the quality of our manuscript.
Supplementary Material
The Supplementary Material for this article can be found online at: https://www.frontiersin.org/articles/10.3389/fsufs.2021.716140/full#supplementary-material
References
Alvarez-Campos, O., and Evanylo, G. K. (2019). biosolids improve urban soil properties and vegetable production in urban agriculture. Urban Agric. Reg. Food Syst. 4, 1–11. doi: 10.2134/urbanag2019.04.0002
Angelakis, A. N., Asano, T., Bahri, A., Jimenez, B. E., and Tchobanoglous, G. (2018). Water reuse: from ancient to modern times and the future. Front. Environ. Sci. 6:26. doi: 10.3389/fenvs.2018.00026
Badewa, E., and Oelbermann, M. (2020). Achieving soil security through biobased residues. Soil Sci. 5:7.
Bargout, R. N., and Raizada, M. N. (2013). Soil nutrient management in Haiti, pre-Columbus to the present day: lessons for future agricultural interventions. Agric. Food Secur. 2:11. doi: 10.1186/2048-7010-2-11
Baro, M. (2002). Food insecurity and liveelihood systems in Northwest Haiti. J. Polit. Ecol. 9, 1–34. doi: 10.2458/v9i1.21633
Bekchanov, M., and Mirzabaev, A. (2018). Circular economy of composting in Sri Lanka: Opportunities and challenges for reducing waste related pollution and improving soil health. J. Clean. Prod. 202, 1107–1119. doi: 10.1016/j.jclepro.2018.08.186
Berendes, D., Levy, K., Knee, J., Handzel, T., and Hill, V. R. (2015). Ascaris and Escherichia coli inactivation in an ecological sanitation system in Port-au-Prince, Haiti. PLoS ONE 10:e0125336. doi: 10.1371/journal.pone.0125336
Berendes, D. M., Yang, P. J., Lai, A., Hu, D., and Brown, J. (2018). Estimation of global recoverable human and animal faecal biomass. Nat. Sustain. 1, 679–685. doi: 10.1038/s41893-018-0167-0
Bracken, P., Wachtler, A., Panesar, A. R., and Lange, J. (2007). The road not taken: how traditional excreta and greywater management may point the way to a sustainable future. Water Supply 7, 219–227. doi: 10.2166/ws.2007.025
Brown, S., Ippolito, J. A., Hundal, L. S., and Basta, N. T. (2020). Municipal biosolids—a resource for sustainable communities. Curr. Opin. Environ. Sci. Health 14, 56–62. doi: 10.1016/j.coesh.2020.02.007
Brown, S., Kruger, C., and Subler, S. (2008). Greenhouse gas balance for composting operations. J. Environ. Qual. 37, 1396–1410. doi: 10.2134/jeq2007.0453
Clarke, R. M., and Cummins, E. (2015). Evaluation of “Classic” and emerging contaminants resulting from the application of biosolids to agricultural lands: a review. Hum. Ecol. Risk Assess. Int. J. 21, 492–513. doi: 10.1080/10807039.2014.930295
Cordell, D., and White, S. (2014). Life's Bottleneck: sustaining the world's phosphorus for a food secure future. Annu. Rev. Environ. Resour. 39, 161–188. doi: 10.1146/annurev-environ-010213-113300
Dalling, J. W., Winter, K., Andersen, K. M., and Turner, B. L. (2013). Artefacts of the pot environment on soil nutrient availability: implications for the interpretation of ecological studies. Plant Ecol. 214, 329–338. doi: 10.1007/s11258-013-0172-3
de Matos Nascimento, A., Maciel, A. M., Silva, J. B. G., Mendon?a, H. V., de Paula, V. R., and Otenio, M. H. (2020). Biofertilizer application on corn (Zea mays) increases the productivity and quality of the crop without causing environmental damage. Water Air Soil Pollut. 231:414. doi: 10.1007/s11270-020-04778-6
DeLonge, M. S., Ryals, R., and Silver, W. L. (2013). A Lifecycle model to evaluate carbon sequestration potential and greenhouse gas dynamics of managed grasslands. Ecosystems 16, 962–979. doi: 10.1007/s10021-013-9660-5
Diacono, M., and Montemurro, F. (2011). “Long-Term effects of organic amendments on soil fertility,” in Sustainable Agriculture. eds E. Lichtfouse, M. Hamelin, M. Navarrete, and P. Debaeke (Dordrecht: Springer Netherlands), 761–786.
Drinkwater, L. E., and Snapp, S. S. (2007). “Nutrients in agroecosystems: rethinking the management paradigm,” in Advances in Agronomy (New York, NY: Elsevier), 163–186.
Eden, M., Gerke, H. H., and Houot, S. (2017). Organic waste recycling in agriculture and related effects on soil water retention and plant available water: a review. Agron. Sustain. Dev. 37:11. doi: 10.1007/s13593-017-0419-9
Elliott, H. A., and O'Connor, G. A. (2007). Phosphorus management for sustainable biosolids recycling in the United States. Soil Biol. Biochem. 39, 1318–1327. doi: 10.1016/j.soilbio.2006.12.007
Erisman, J. W., Galloway, J. N., Seitzinger, S., Bleeker, A., Dise, N. B., Petrescu, A. M. R., et al. (2013). Consequences of human modification of the global nitrogen cycle. Philos. Trans. R. Soc. B Biol. Sci. 368:20130116. doi: 10.1098/rstb.2013.0116
FAO IFAD, UNICEF, WFP and WHO. (2020). The State of Food Security and Nutrition in the World 2020. Transforming Food Systems for Affordable Healthy Diets. Rome: FAO. doi: 10.4060/ca9692en
FAOSTAT Statistical Database (2021). Food and Agriculture Organization of the United Nations. Rome: FAOSTAT Statistical Database. Available online at: http://www.fao.org/faostat/en/#data
Fischer, K., Kokko, S., and McConville, J. (2021). No legitimacy: a study of private sector sanitation development in the Global South. Environ. Innov. Soc. Transit. 38, 68–78. doi: 10.1016/j.eist.2020.11.006
Forero, L. E., Grenzer, J., Heinze, J., Schittko, C., and Kulmatiski, A. (2019). Greenhouse- and field-measured plant-soil feedbacks are not correlated. Front. Environ. Sci. 7:184. doi: 10.3389/fenvs.2019.00184
Fowler, D., Coyle, M., Skiba, U., Sutton, M. A., Cape, J. N., Reis, S., et al. (2013). The global nitrogen cycle in the twenty-first century. Philos. Trans. R. Soc. B Biol. Sci. 368:20130164. doi: 10.1098/rstb.2013.0164
Fox, R. L., and Valenzuela, H. (1996). Vegetables grown under tropical/subtropical conditions. Hawaii Inst. Trop. Agric. Hum. Resour. 5, 324–326.
Fuhrmeister, E. R., Schwab, K. J., and Julian, T. R. (2015). Estimates of nitrogen, phosphorus, biochemical oxygen demand, and fecal coliforms entering the environment due to inadequate sanitation treatment technologies in 108 low and middle income countries. Environ. Sci. Technol. 49, 11604–11611. doi: 10.1021/acs.est.5b02919
Gardner, J. B., and Drinkwater, L. E. (2009). The fate of nitrogen in grain cropping systems: a meta-analysis of 15 N field experiments. Ecol. Appl. 19, 2167–2184. doi: 10.1890/08-1122.1
Gerten, D., Heck, V., Jägermeyr, J., Bodirsky, B. L., Fetzer, I., Jalava, M., et al. (2020). Feeding ten billion people is possible within four terrestrial planetary boundaries. Nat. Sustain. 3, 200–208. doi: 10.1038/s41893-019-0465-1
Gianico, A., Braguglia, C., Gallipoli, A., Montecchio, D., and Mininni, G. (2021). Land application of biosolids in europe: possibilities, con-straints and future perspectives. Water 13:103. doi: 10.3390/w13010103
GIZ (2012). Worldwide List of 324 Documented EcoSan Projects by Various Organisations. Gesellschaft fur Internationale Zusammenarbeit (GIZ) GmbH.
Goswami, L., Nath, A., Sutradhar, S., Bhattacharya, S. S., Kalamdhad, A., Vellingiri, K., et al. (2017). Application of drum compost and vermicompost to improve soil health, growth, and yield parameters for tomato and cabbage plants. J. Environ. Manage. 200, 243–252. doi: 10.1016/j.jenvman.2017.05.073
Guo, S., Zhou, X., Simha, P., Mercado, L. F. P., Lv, Y., and Li, Z. (2021). Poor awareness and attitudes to sanitation servicing can impede China's Rural Toilet Revolution: Evidence from Western China. Sci. Total Environ. 794:148660. doi: 10.1016/j.scitotenv.2021.148660
Habteselassie, M. Y., Miller, B. E., Thacker, S. G., Stark, J. M., and Norton, J. M. (2006). Soil nitrogen and nutrient dynamics after repeated application of treated dairy-waste. Soil Sci. Soc. Am. J. 70, 1328–1337. doi: 10.2136/sssaj2005.0189
Haq, G., and Cambridge, H. (2012). Exploiting the co-benefits of ecological sanitation. Curr. Opin. Environ. Sustain. 4, 431–435. doi: 10.1016/j.cosust.2012.09.002
Harder, R., Wielemaker, R., Larsen, T. A., Zeeman, G., and Öberg, G. (2019). Recycling nutrients contained in human excreta to agriculture: pathways, processes, and products. Crit. Rev. Environ. Sci. Technol. 49, 695–743. doi: 10.1080/10643389.2018.1558889
Harder, R., Wielemaker, R., Molander, S., and Öberg, G. (2020). Reframing human excreta management as part of food and farming systems. Water Res. 175:115601. doi: 10.1016/j.watres.2020.115601
Hu, M., Fan, B., Wang, H., Qu, B., and Zhu, S. (2016). Constructing the ecological sanitation: a review on technology and methods. J. Clean. Prod. 125, 1–21. doi: 10.1016/j.jclepro.2016.03.012
IFASTAT (2021). Urea Production and Trade Stats 2008-2019. Paris: International Fertilizer Association. Available online at:https://www.ifastat.org (accessed July 15, 2021).
Jawad, R., Nawaz, S., Hammad, H. M., Salik, M. R., and Farhad, W. (2015). Nitrogen and sowing method affect radish growth and yield under arid environments of Pakistan. Sci. Int. 27, 2245–2250.
Jenkinson, D. S., Fox, R. H., and Rayner, J. H. (1985). Interactions between fertilizer nitrogen and soil nitrogen-the so-called ‘priming’ effect. J. Soil Sci. 36, 425–444. doi: 10.1111/j.1365-2389.1985.tb00348.x
Jones, J. B. (2001). Laboratory Guide for Conducting Soil Tests and Plant Analysis. Boca Raton, FL: CRC Press LLC.
Jönsson, H., Stintzing, A. R., Vinnerȧs, B., and Salomon, E. (2005). Guidelines on the Use of Urine and Faeces in Crop Production, Vol. 37. Stockholm Environment Institute.
Jothimani, P., Sangeeetha, R., Kavitha, B., and Senthilraja, K. (2013). Effect of ecosan compost on growth and yield of Banana. Int. J. Adv. Life Sci. 6, 131–138.
Jothimani, P., and Sangeetha, R. (2012). Impact of ecosanitation programme and human waste compost on growth and yield of Marigold. Int. J. Adv. Life Sci. 2, 45–53.
Kibblewhite, M. G., Ritz, K., and Swift, M. J. (2008). Soil health in agricultural systems. Philos. Trans. R. Soc. B Biol. Sci. 363, 685–701. doi: 10.1098/rstb.2007.2178
Kohlitz, J. P., Chong, J., and Willetts, J. (2017). Climate change vulnerability and resilience of water, sanitation, and hygiene services: a theoretical perspective. J. Water Sanit. Hyg. Dev. 7, 181–195. doi: 10.2166/washdev.2017.134
Kramer, S., Preneta, N., Kilbride, A., Page, L. N., Coe, C. M., and Dahlberg, A. (2011). The SOIL Guide to Ecological Sanitation. Sustainable Organic Integrated Livelihoods, p. 144. Available online at:http://www.oursoil.org/resources
Krause, A., Häfner, F., Augustin, F., and Udert, K. M. (2021). Qualitative risk analysis for contents of dry toilets used to produce novel recycling fertilizers. Circ. Econ. Sustain. 3, 1–40. doi: 10.1007/s43615-021-00068-3
Krause, A., Nehls, T., George, E., and Kaupenjohann, M. (2016). Organic wastes from bioenergy and ecological sanitation as a soil fertility improver: a field experiment in a tropical Andosol. SOIL 2, 147–162. doi: 10.5194/soil-2-147-2016
Krause, A., and Rotter, V. (2018). Recycling improves soil fertility management in smallholdings in tanzania. Agriculture 8:31. doi: 10.3390/agriculture8030031
Kuzyakov, Y., Friedel, J. K., and Stahr, K. (2000). Review of mechanisms and quantification of priming effects. Soil Biol. Biochem. 1485–1498. doi: 10.1016/S0038-0717(00)00084-5
Lamichhane, K. M., and Babcock, R. W. (2013). Survey of attitudes and perceptions of urine-diverting toilets and human waste recycling in Hawaii. Sci. Tot. Environ. 443, 749–756. doi: 10.1016/j.scitotenv.2012.11.039
Langergraber, G., and Muellegger, E. (2005). Ecological sanitation—a way to solve global sanitation problems? Environ. Int. 31, 433–444. doi: 10.1016/j.envint.2004.08.006
Larsen, T. A., Udert, K. M., and Lienert, J. (2013). Source Separation and Decentralization for Wastewater Management. London: IWA Publishing.
Lavkulich, L. (1971). Method 18.4 Exchangeable Cations and Total Exchange Capacity by the Ammonium Acetate Method at pH 7.0. Boca Raton, FL: CRC Press.
Lehmann, J., Bossio, D. A., Kögel-Knabner, I., and Rillig, M. C. (2020). The concept and future prospects of soil health. Nat. Rev. Earth Environ. 1, 544–553. doi: 10.1038/s43017-020-0080-8
Lerch, T. Z., Dignac, M. F., Thevenot, M., Mchergui, C., and Houot, S. (2019). Chemical changes during composting of plant residues reduce their mineralisation in soil and cancel the priming effect. Soil Biol. Biochem. 136:107525. doi: 10.1016/j.soilbio.2019.107525
Lu, Q., He, Z. L., and Stoffella, P. J. (2012). Land application of biosolids in the USA: A Review. Appl. Environ. Soil Sci. 2012, 1–11. doi: 10.1155/2012/201462
Lystek Inc (2017). LysteGro Fertilizer Composition. Lystek Organic Materials Recovery Center, 1014 Chadbourne Road Fairfield, California, 94534–9700.
Mar Montiel-Rozas, M., Panettieri, M., Madejón, P., and Madejón, E. (2016). Carbon sequestration in restored soils by applying organic amendments. Land Degrad. Dev. 27, 620–629. doi: 10.1002/ldr.2466
Martínez-Blanco, J., Lazcano, C., Christensen, T. H., Muñoz, P., Rieradevall, J., Møller, J., et al. (2013). Compost benefits for agriculture evaluated by life cycle assessment. A review. Agron. Sustain. Dev. 33, 721–732. doi: 10.1007/s13593-013-0148-7
Matthias, A. D., Yarger, D. N., and Weinbeck, R. S. (1978). A numerical evaluation of chamber methods for determining gas fluxes. Geophys. Res. Lett. 5, 765–768. doi: 10.1029/GL005i009p00765
McConville, J. R., Kvarnström, E., Nordin, A. C., Jönsson, H., and Niwagaba, C. B. (2020). Structured approach for comparison of treatment options for nutrient-recovery from fecal sludge. Front. Environ. Sci. 8:36. doi: 10.3389/fenvs.2020.00036
McNicol, G., Jeliazovski, J., François, J. J., Kramer, S., and Ryals, R. (2020). Climate change mitigation potential in sanitation via off-site composting of human waste. Nat. Clim. Change 10, 545–549. doi: 10.1038/s41558-020-0782-4
Mihelcic, J. R., Fry, L. M., and Shaw, R. (2011). Global potential of phosphorus recovery from human urine and feces. Chemosphere 84, 832–839. doi: 10.1016/j.chemosphere.2011.02.046
Moya, B., Parker, A., Sakrabani, R., and Mesa, B. (2019a). Evaluating the efficacy of fertilisers derived from human excreta in agriculture and their perception in antananarivo, Madagascar. Waste Biomass Valoriz. 10, 941–952. doi: 10.1007/s12649-017-0113-9
Moya, B., Sakrabani, R., and Parker, A. (2019b). Realizing the circular economy for sanitation: assessing enabling conditions and barriers to the commercialization of human excreta derived fertilizer in Haiti and Kenya. Sustainability 11:3154. doi: 10.3390/su11113154
Nilsson, M., Griggs, D., and Visbeck, M. (2016). Map the interactions between sustainable development goals. Nat. News 534:320.
Öberg, G., Metson, G. S., Kuwayama, Y., and Conrad, S. A. (2020). Conventional sewer systems are too time-consuming, costly and inflexible to meet the challenges of the 21st century. Sustainability 12:6518. doi: 10.3390/su12166518
Otero, G., Pechlaner, G., and Gürcan, E. C. (2013). The Political economy of “food security” and trade: uneven and combined dependency: the political economy of “food security” and trade. Rural Sociol. 78, 263–289. doi: 10.1111/ruso.12011
Ozores-Hampton, M., and Peach, D. R. A. (2002). Biosolids in vegetable production systems. Horttechnology 12, 336–340. doi: 10.21273/H.O.R.T.T.E.C.H.12.3.336
Paustian, K., Lehmann, J., Ogle, S., Reay, D., Robertson, G. P., and Smith, P. (2016). Climate-smart soils. Nature 532, 49–57. doi: 10.1038/nature17174
Peccia, J., and Westerhoff, P. (2015). We should expect more out of our sewage sludge. Environ. Sci. Technol. 49, 8271–8276. doi: 10.1021/acs.est.5b01931
Peoples, M. B., Boyer, E. W., Goulding, K. W. T., Heffer, P., Ochwoh, V. A., Vanlauwe, B., et al. (2004). Agriculture and the Nitrogen Cycle: Assessing the Impacts of Fertilizer use on Food Production and the Environment. Washington, D.C: Island Press.
Piceno, Y. M., Pecora-Black, G., Kramer, S., Roy, M., Reid, F. C., Dubinsky, E. A., et al. (2017). Bacterial community structure transformed after thermophilically composting human waste in Haiti. PLoS ONE 12:e0177626. doi: 10.1371/journal.pone.0177626
Pikaar, I., Matassa, S., Rabaey, K., Bodirsky, B. L., Popp, A., Herrero, M., et al. (2017). Microbes and the next nitrogen revolution. Environ. Sci. Technol. 51, 7297–7303. doi: 10.1021/acs.est.7b00916
Pingali, P. L. (2012). Green revolution: impacts, limits, and the path ahead. Proc. Natl. Acad. Sci. 109, 12302–12308. doi: 10.1073/pnas.0912953109
R.M. Towill Corporation (2017). Sand Island Wastewater Treatment Plant Facility Plan. Honolulu, Hawai‘i: Department of Environmental Services City and County of Honolulu.
Rawluk, C. D. L., Grant, C. A., and Racz, G. J. (2001). Ammonia volatilization from soils fertilized with urea and varying rates of urease inhibitor NBPT. Can. J. Soil Sci. 81, 239–246. doi: 10.4141/S00-052
Reed, B., and Shaw, R. (2003). Using human waste. Waterlines 22, 15–18. doi: 10.3362/0262-8104.2003.053
Reeves, D. W. (1997). The role of soil organic matter in maintaining soil quality in continuous cropping systems. Soil Tillage Res. 43, 131–167. doi: 10.1016/S0167-1987(97)00038-X
Rigby, H., Clarke, B. O., Pritchard, D. L., Meehan, B., Beshah, F., Smith, S. R., et al. (2016). A critical review of nitrogen mineralization in biosolids-amended soil, the associated fertilizer value for crop production and potential for emissions to the environment. Sci. Total Environ. 541, 1310–1338. doi: 10.1016/j.scitotenv.2015.08.089
Roy, R. N., Finck, A., Blair, G. J., and Tandon, H. L. S. (2006). FAO Plant Nutrition for Food Security: A Guide for Integrated Nutrient Management. Rome: Food and Agriculture Organization of the United Nations, 366.
Russel, K., Tilmans, S., Kramer, S., Sklar, R., Tillias, D., and Davis, J. (2015). User perceptions of and willingness to pay for household container-based sanitation services: experience from Cap Haitien, Haiti. Environ. Urban. 27, 525–540. doi: 10.1177/0956247815596522
Russel, K. C., Hughes, K., Roach, M., Auerbach, D., Foote, A., Kramer, S., et al. (2019). Taking container-based sanitation to scale: opportunities and challenges. Front. Environ. Sci. 7:190. doi: 10.3389/fenvs.2019.00190
Ryals, R., Kaiser, M., Torn, M. S., Berhe, A. A., and Silver, W. L. (2014). Impacts of organic matter amendments on carbon and nitrogen dynamics in grassland soils. Soil Biol. Biochem. 68, 52–61. doi: 10.1016/j.soilbio.2013.09.011
Ryals, R., McNicol, G., Porder, S., and Kramer, S. (2019). Greenhouse gas fluxes from human waste management pathways in Haiti. J. Clean. Prod. 226, 106–113. doi: 10.1016/j.jclepro.2019.04.079
Ryals, R., and Silver, W. L. (2013). Effects of organic matter amendments on net primary productivity and greenhouse gas emissions in annual grasslands. Ecol. Appl. 23, 46–59. doi: 10.1890/12-0620.1
Schipanski, M. E., MacDonald, G. K., Rosenzweig, S., Chappell, M. J., Bennett, E. M., Kerr, R. B., et al. (2016). Realizing resilient food systems. Bioscience 66, 600–610. doi: 10.1093/biosci/biw052
Schröder, C., Häfner, F., Larsen, O. C., and Krause, A. (2021). Urban organic waste for urban farming: growing lettuce using vermicompost and thermophilic compost. Agronomy 11:1175. doi: 10.3390/agronomy11061175
Sharda, K. C., and Shinjo, H. (2020). Effects of human urine and ecosan manure on plant growth and soil properties in Central Nepal. Sanit. Value Chain 4, 19–37.
Sharpley, A. N., and Menzel, R. G. (1987). The impact of soil and fertilizer phosphorus on the environment. In Adv. Agron. 41, 297–324. doi: 10.1016/S0065-2113(08)60807-X
Simha, P. (2021). Alkaline Urine Dehydration: How to dry source-separated human urine and recover nutrients? (doctoral thesis). Swedish University of Agricultural Sciences.
Simha, P., and Ganesapillai, M. (2017). Ecological Sanitation and nutrient recovery from human urine: How far have we come? a review. Sustain. Environ. Res. 27, 107–116. doi: 10.1016/j.serj.2016.12.001
Simha, P., Lalander, C., Nordin, A., and Vinnerås, B. (2020). Alkaline dehydration of source-separated fresh human urine: preliminary insights into using different dehydration temperature and media. Sci. Total Environ. 733:139313. doi: 10.1016/j.scitotenv.2020.139313
Simha, P., Lalander, C., Vinner?s, B., and Ganesapillai, M. (2017). Farmer attitudes and perceptions to the re-use of fertiliser products from resource-oriented sanitation systems - The case of Vellore, South India. Sci. Tot. Environ. 581–582, 885–896. doi: 10.1016/j.scitotenv.2017.01.044
Sinharoy, S. S., Pittluck, R., and Clasen, T. (2019). Review of drivers and barriers of water and sanitation policies for urban informal settlements in low-income and middle-income countries. Util. Policy 60:100957. doi: 10.1016/j.jup.2019.100957
Smith, P., House, J. I., Bustamante, M., Sobocká, J., Harper, R., Pan, G., et al. (2016). Global change pressures on soils from land use and management. Glob. Change Biol. 22, 1008–1028. doi: 10.1111/gcb.13068
Springmann, M., Clark, M., Mason-D'Croz, D., Wiebe, K., Bodirsky, B. L., Lassaletta, L., et al. (2018). Options for keeping the food system within environmental limits. Nature 562, 519–525. doi: 10.1038/s41586-018-0594-0
Steckley, M., and Shamsie, Y. (2015). Manufacturing corporate landscapes: the case of agrarian displacement and food (in)security in Haiti. Third World Q. 36, 179–197. doi: 10.1080/01436597.2015.976042
Steffen, W., Richardson, K., Rockstrom, J., Cornell, S. E., Fetzer, I., Bennett, E. M., et al. (2015). Planetary boundaries: guiding human development on a changing planet. Science 347, 1259855–1259855. doi: 10.1126/science.1259855
Sumner, M. E. (2000). Beneficial use of effluents, wastes, and biosolids. Commun. Soil Sci. Plant Anal. 31, 1701–1715. doi: 10.1080/00103620009370532
Tautges, N. E., Chiartas, J. L., Gaudin, A. C. M., O'Geen, A. T., Herrera, I., and Scow, K. M. (2019). Deep soil inventories reveal that impacts of cover crops and compost on soil carbon sequestration differ in surface and subsurface soils. Glob. Change Biol. 25, 3753–3766. doi: 10.1111/gcb.14762
Theregowda, R.B., González-Mejía, A.M., Ma, X., and Garland, J. (2019). Nutrient recovery from municipal wastewater for sustainable food production systems: an alternative to traditional fertilizers. Environ. Eng. Sci. 36, 833–842. doi: 10.1089/ees.2019.0053
Toffey, W., and Brown, S. (2020). Biosolids and ecosystem services: making the connection explicit. Curr. Opin. Environ. Sci. Health 14, 51–55. doi: 10.1016/j.coesh.2020.02.002
Torri, S. I., Corrêa, R. S., and Renella, G. (2014). Soil carbon sequestration resulting from biosolids application. Appl. Environ. Soil Sci. 2014, 1–9. doi: 10.1155/2014/821768
Trimmer, J. T., and Guest, J. S. (2018). Recirculation of human-derived nutrients from cities to agriculture across six continents. Nat. Sustain. 1, 427–435. doi: 10.1038/s41893-018-0118-9
Trimmer, J. T., Miller, D. C., Byrne, D. M., Lohman, H. A. C., Banadda, N., Baylis, K., et al. (2020). Re-envisioning sanitation as a human-derived resource system. Environ. Sci. Technol. 54, 10446–10459. doi: 10.1021/acs.est.0c03318
Trimmer, J. T., Miller, D. C., and Guest, J. S. (2019). Resource recovery from sanitation to enhance ecosystem services. Nat. Sustain. 2, 681–690. doi: 10.1038/s41893-019-0313-3
Tully, K., and Ryals, R. (2017). Nutrient cycling in agroecosystems: balancing food and environmental objectives. Agroecol. Sustain. Food Syst. 41, 761–798. doi: 10.1080/21683565.2017.1336149
United Nations (2021). SDG 6 Snapshot in Haiti. Available online at: https://www.sdg6data.org/country-or-area/Haiti
United States Environmental Protection Agency (1995). Process Design Manual: Land Application of Sewage Sludge and Domestic Septage. Cincinnati: Office of Research and Development.
Villa, Y. B., and Ryals, R. (2021). Soil carbon response to long-term biosolids application. J. Environ. Qual. doi: 10.1002/jeq2.20270
Wang, H., Brown, S. L., Magesan, G. N., Slade, A. H., Quintern, M., Clinton, P. W., et al. (2008). Technological options for the management of biosolids. Environ. Sci. Pollut. Res. Int. 15, 308–317. doi: 10.1007/s11356-008-0012-5
Wanner, T. (2015). The new ‘passive revolution’ of the green economy and growth discourse: maintaining the ‘sustainable development’ of neoliberal capitalism. New Polit. Econ. 20, 21–41. doi: 10.1080/13563467.2013.866081
WHO (2006). Guidelines for the Safe Use of Wastewater, Excreta and Greywater. Paris, France: World Health Organization.
World Health Organization and (WHO) and the United Nations Children's Fund (UNICEF). (2020). Progress on Drinking Water, Sanitation and Hygiene Update and SDG Baselines 2017 Launch version July 12 Main report.
Yuan, W.-L., Yuan, S., Deng, X., Gan, C., Cui, L., and Wang, Q. (2015). Effect of N management on root yield and N uptake of radishes in Southern China. HortScience 50, 750–753. doi: 10.21273/HORTSCI.50.5.750
Zhu, Z. L., and Chen, D. L. (2002). Nitrogen fertilizer use in China—contributions to food production, impacts on the environment and best management strategies. Nutr. Cycl. Agroecosystems 63, 117–127. doi: 10.1023/A:1021107026067
Zimmermann, C. F. (2000). “Chapter 2. sampling and analysis of soils and plant tissue chapter,” in Plant Nutrient Management In Hawaii's Soils: Approaches for Tropical and Subtropical Agriculture, eds J.A. Silva, and R. Uchida (Hawaii: University of Hawaii), 25–26.
Zimmermann, C. F. (1997). Method 440.0 : determination of carbon and nitrogen in sediments and particulates of estuarine/coastal waters using elemental analysis (Revision 1.4. [Cincinnati, OH]: U.S. Environmental Protection Agency, Office of Research and Development, National Exposure Research Laboratory, [1997]).
Keywords: ecological sanitation, greeenhouse-gas (GHG) emission, soil carbon, excreta, compost, sustainable develop goals
Citation: Ryals R, Bischak E, Porterfield KK, Heisey S, Jeliazovski J, Kramer S and Pierre S (2021) Toward Zero Hunger Through Coupled Ecological Sanitation-Agriculture Systems. Front. Sustain. Food Syst. 5:716140. doi: 10.3389/fsufs.2021.716140
Received: 28 May 2021; Accepted: 31 August 2021;
Published: 01 October 2021.
Edited by:
Marney E. Isaac, University of Toronto, CanadaReviewed by:
Ariane Krause, Leibniz Institute of Vegetable and Ornamental Crops, GermanyPrithvi Simha, Swedish University of Agricultural Sciences, Sweden
Copyright © 2021 Ryals, Bischak, Porterfield, Heisey, Jeliazovski, Kramer and Pierre. This is an open-access article distributed under the terms of the Creative Commons Attribution License (CC BY). The use, distribution or reproduction in other forums is permitted, provided the original author(s) and the copyright owner(s) are credited and that the original publication in this journal is cited, in accordance with accepted academic practice. No use, distribution or reproduction is permitted which does not comply with these terms.
*Correspondence: Rebecca Ryals, cnJ5YWxzQHVjbWVyY2VkLmVkdQ==