- Food Chemistry Division, ICMR-National Institute of Nutrition, Hyderabad, India
Millets are nutritionally superior indigenous staple crops packed with high protein, vitamins, and minerals. However, anti-nutrients such as phytic acid, tannins, and polyphenols present in the millets tend to reduce the bio-accessibility of minerals (iron and zinc), due to which the millet diets are greatly compromised. Although most of the cereals, such as wheat flour, brown rice, and barley, contain phytic acid to a level far more than that of the millets, it is important to develop feasible household methods to reduce the level of phytic acid so as to enhance nutrient absorption. The present study was carried out to investigate the effect of traditional processing on nutrient and anti-nutrient retention of three majorly consumed millets, namely, sorghum, finger millet, and pearl millet. These millets were traditionally cooked and then fermented overnight with water and curd. The results show that this type of simple, traditional household-level process significantly reduced the phytic acid content by 62.9% in sorghum, 34.1% in finger millet, and 29.35% in pearl millet. There is a considerable decrease in phytic acid–zinc molar ratio by 71.38, 61.15, and 33.47% and in phytic acid–iron molar ratio by 73.52, 48.07, and 66.39% in sorghum, finger millet, and pearl millet, respectively. Among the macronutrients, the protein and ash contents were significantly increased. A high retention of water-soluble vitamins was observed in the processed millets. Overall, the traditionally cooked millet, fermented overnight and then added with curd, enhanced many essential macro- and micronutrients and concurrently reduced phytic acid, thus forming a sustainably simple household method for improving dietary nutrients.
Introduction
Millets are small-seeded annual grasses belonging to the Graminae (Poaceae) family. They are generally resistant to pests and diseases and have the ability to grow in less fertile, dry land, with a harvesting time of 70–80 days (Devi et al., 2014). Their long storability under normal conditions made them “famine reserves”. Millets are also called “nutri-cereals” due to their high nutritive value (Bhat et al., 2018). Millets are mainly cultivated in African and Asian countries. In 2019, millet cultivation in Central Africa was at 1,120.7 kg/ha, while in India it was 1,211.4 kg/ha (FAOSTAT, 2019). Due to the adaptation of improved technologies, millet production has increased drastically in recent years. The production of millets increased from 87.7 thousand tons (2009) to 1 lakh tons (2019) (FAOSTAT, 2019) in the last decade. In particular, sorghum and finger millet average productivity has improved by 75 and 41%, respectively, during 2015–2016 (Chapke et al., 2018). However, in the past few decades, the global millet consumption has declined at a rate of 0.9%; the per capita consumption of millets fell marginally from 4.6 in 1982 to 3.6 kg around the world, while in India it was 12 kg in 1982 and subsequently reduced to 8 kg during the year 2009 (Rao and Basavaraj, 2015). Millet consumption was reported to be much higher in rural (58.6%) compared to urban (27.5%) populations in India (NSSO, 2012). Some of the reasons for reduced millet consumption are the availability of rice and wheat through the public distribution system, increased per capita income, growing urbanization, and changing tastes and preferences (Bhagavatula et al., 2013). Hence, consumption has shifted from millets to refined cereals. Nevertheless, in recent times, millet consumption has been increased from 43.2 million metric tons (MMT) in 2018 to 45.4 MMT in 2019 (Wallace and Singh, 2019). Sorghum, pearl millet, and finger millet are the most commonly consumed millets among the different millets. In India, pearl millet appeared to be popular in the northern and eastern parts, while sorghum appeared to be popular in the west and south of India and in Eastern India. India is the largest producer of pearl millet, and it is largely concentrated in the states of Rajasthan, North and Central Maharashtra, Gujrat, and Northern Karnataka (Rao and Basavaraj, 2015), whereas finger millet appeared to be most popular in the western and southern parts of India (Muniappan et al., 2018).
Most commonly, millets are consumed in the form of a thick porridge, rotis (Indian flat bread), and dumplings and cooked with vegetables (Rao et al., 2006). Different traditional methods also used for millet processing include boiling, pounding, soaking, fermenting, malting, popping, flaking, and roasting. In recent times, there are many industries and research institutes that developed different processing methods using modern equipment to prepare ready-to-eat and ready-to-cook products like semolina, flakes, pasta, vermicelli, dehulled millets, millet-rich multigrain, and millet-rich multigrain roti.
Cereals and grains not only provide more than 50% of the caloric intake and protein intake of the world but are also a good source of other micronutrients (BNF, 2004). Whole grains are rich sources of fiber, vitamins, minerals, and phytochemicals, such as phenolics, lignans, b-glucan, inulin, resistant starch, and sterols. Millets contain high level of protein, essential fatty acids, dietary fiber, vitamin B, and minerals, such as calcium, iron, zinc, potassium, and magnesium, and help in rendering health benefits like reduction in blood sugar level (diabetes), blood cholesterol and pressure regulation, preventing thyroid disorders, reducing the risk of developing cardiovascular disease, celiac disease, and many other age-related chronic diseases (Jacobs et al., 1995, 1998; Liu et al., 1999, 2000; Anderson et al., 2000; Meyer et al., 2000; Nicodemus et al., 2001; Liu, 2002; Anitha et al., 2021). However, studies also reported that cereals contain “anti-nutrients”, such as phytic acid, that interfere with nutrient absorption in the human body. Not only millet but also other monocotyledonous seeds like wheat, barley, and rice accumulate phytic acid mostly in the aleurone layer, and the level varies between 0.5 and 2.0%, with brown rice at 0.84–0.94%, milled rice at 0.20%, wheat flour at 0.96%, barley at 1.19%, and whole corn at 1.05% (Reddy and Sathe, 2001; Okazaki and Katayama, 2005; Longvah et al., 2017).
Millets have about 0.61% of tannin, 0.48% of phytic acid, 0.2–3.0% of polyphenol, and trypsin inhibitors (Thompson, 1993), out of which phytic acid is a matter of concern. Although the level of phytic acid in millet is far less than that in wheat flour, brown rice, barley, and whole corn, it is still important to reduce the phytic acid content to enhance the bio-accessibility of major nutrients. Phytic acid is the organic form of phosphorous (myoinositol 1,2,3,4,5,6-hexakis dihydrogen phosphate) occurring in plant constituents as the major portion of total phosphorus (Guttieri et al., 2004), with a highly negative charge and a reactive compound that attracts and binds positively charged mineral ions such as iron, zinc, and calcium. This binding changes nutrient digestibility and bioavailability, as monogastric animals (poultry and humans) cannot metabolize phytic acid due to the absence of phytase enzymes in their digestive tract (Lopez et al., 2002; Vats and Banerjee, 2004). Concurrently, reports also reveal that only the highly phosphorylated inositol phosphates, i.e., IP5 and IP6, interfered with mineral utilization but not the lower inositol phosphates, namely, IP1, IP2, IP3, and IP4 (Lönnerdal et al., 1989).
Traditional processing methods are considered to reduce the phytic acid level in cereals and millets. More reduction has been reported especially in traditional Indian cooking style, like pressure cooking, prolonged boiling, steaming, etc., compared to other countries (Agte et al., 1999). In addition, soaking, fermentation, and germination also reduced the phytic acid content (Liang et al., 2008) in millets. It has been reported that fermentation provides optimum pH to degrade phytic acid and increases the availability of minerals and vitamin B (Haard, 1999). The synthesis of vitamins during germination increased several times and reduced the phytic acid concentration (FAO/WHO, 2001), but roasting, puffing, flaking, and decortication showed relatively lesser outcomes on phytic acid reduction. A combination of different processing methods, such as autoclaving along with fermentation, also showed a promising role in phytic acid reduction by 63% (Binita and Khetarpaul, 1997).
Although many pieces of research have been reported on the determination of phytic acid content in different processing methods to reduce the phytic acid levels and its effect on mineral and vitamin availability, there is no report found on the effect of traditionally cooked millet along with curd/yogurt which contain Lactobacillus sp. on improving the nutrition quality of millets. We hypothesized that traditionally cooked and processed millets along with curd attenuate the phytic acid level and enhance the water-soluble vitamin content. Thus, the present study was carried out to observe the trends of phytic acid reduction and predict mineral bioavailability in three commonly consumed millets (sorghum, pearl millet, and finger millet) after the application of various traditional processing methods.
Materials and Methods
Sample Collection
Three millets, namely, sorghum (Sorghum vulgare), pearl millet (Pennisetum typhoideum), and finger millet (Eleusine coracana), were purchased at 2 kg each from three different markets of Secunderabad and Hyderabad (Telangana) and combined into a single (total of 6 kg) sample for the subsequent studies. Traditionally prepared curd was purchased from a local shop in Secunderabad, Telangana.
Sample Preparation
All the millets were cleaned by removing unwanted foreign particles if there was any (Figure 1). The whole quantity of sorghum and pearl millet were soaked in water for 4 h, and then the water was drained. The soaked millets were spread on a blotting paper at room temperature for 30 min. They were coarsely ground using a domestic mixer grinder. The pearl and powder were separated using a sieve (60 mesh, 0.25 mm). Initially, the pearl was added to boiling water and cooked until it turned soft, and then the powder was added and allowed to cook further. Finger millet was made into fine powder using a commercial mill. The powder was added to boiling water (in a ratio of 1:2 w/v) and stirred continuously to avoid the formation of lumps. The sample was cooked until it turns into a thick paste. Then, the paste was made into small round balls (dumplings). All the three cooked millets were allowed to cool down to room temperature and then divided into four equal parts. The first part was stored at −20°C in air-tight containers until further analysis. The second part was mixed with an equal amount of curd and kept for overnight fermentation. Water was added (up to submersible level) to the third and fourth part of the cooked millet, and this was stored at room temperature overnight for fermentation. After overnight fermentation, the fourth part was mixed with an equal quantity of curd. All the samples were homogenized using a domestic mixer grinder and stored at −20°C in air-tight containers until further analysis. All the cooking procedures and subsequent processing were carried out using stainless steel vessels.
Proximate Analysis
Proximate composition was determined using Association of Official Agricultural Chemists (AOAC) official methods. Moisture was analyzed as per AOAC 934.01 methods. Using MRC (DFO 36-240 SERIES) hot-air oven, ash was quantified using AOAC 942.05 methods in a Thermo Fischer, Heraeus muffle furnace. The total fat content was determined by mixed solvent extraction method (chloroform and methanol, 2:1 ratio) using AOAC 922.06, and dietary fiber was done by enzymatic–gravimetric method using AOAC 991.43. Protein was estimated using AOAC 954.01 protocol by Kjeldahl method (gravimetric and titration), using an automated FOSS Kjeltec™ 8400 Kjeldahl analyzer, with a conversion factor of 5.95. The total carbohydrate was estimated by differential method using the following formula: total carbohydrate = [100 – (moisture + ash + fat + total protein + fiber)] (g/100 g).
Determination of Phytic Acid, Minerals, and Phytic Acid–Mineral Molar Ratio
Total phytic acid (IP6) was analyzed by the anion exchange method (AOAC 986.11). Phytic acid was extracted with 2.4% HCl. First, columns were prepared by adding 0.5 g AG 1-X4 resin; then, 5 ml of distilled water was added to form a resin bed. Furthermore, 15 ml of 0.1 M NaCl was added to remove any contaminating phosphate ions, and 15 ml of water was added again to wash the columns. The samples were prepared with EDTA-NaOH reagent and poured into columns. The columns were allowed to stand for 20 min and then washed with 15 ml water and 0.1 M NaCl to remove unbound foreign materials and lower inositol phosphates, respectively. The resin was eluted with 15 ml 0.7 M NaCl to release the bound inositol hexaphosphate (phytic acid) and collected into 100-ml Kjeldahl flasks. Glass beads (no. 3), 3.0 ml HNO3, and 0.5 ml H2SO4 were added to the Kjeldahl flasks and digested under the hood over medium heat until a cloud of thick yellow vapor fills the neck of the flasks. The, the flasks were cooled, and the salts formed were dissolved in water and then transferred into 50-ml volumetric flasks. Ammonium molybdate solution (2.0 ml) and sulfonic acid reagent (1.0 ml) were added and mixed well and made up the final volume with distilled water. The mixture was incubated for 15 min at room temperature, and the absorbance was measured at 640 nm using a UV–visible spectrophotometer.
The phytic acid concentration in food samples was calculated (phytic acid contains 28.2% phosphorus) as follows:
where mean K is the mean of the concentrations of standard divided by the absorbance of standard.
Mineral content in millet samples was determined by AOAC (2016) using flame atomic absorption spectrophotometry. The finely ground sample (~1 g) was digested using Supra-pure nitric acid (67%) and hydrogen peroxide (30%) at the ratio of 2:1 (v/v) in a microwave Mars Xpress CEM. Then, the samples were allowed to cool to room temperature and made up to 25 ml using volumetric flasks. Elements such as Fe and Zn were determined using a flame-atomic absorption spectrophotometer (Analytikjena ContrAA 700) operated with Aspect CS 2.2.1.0 tech software. Absorbance for Fe was taken at 248.32 nm and for Zn at 213.86 nm.
The molar ratios between phytic acid and minerals were calculated by dividing the mole of phytic acid with a mole of mineral content using the following formula (Dahdouh et al., 2019):
where PA = phytic acid analyzed, MW(PA) = phytic acid molecular weight (660.06 Da), Min = mineral content (Zn/Fe), and MW(Min) = mineral molecular weight (Fe = 55.845 Da; Zn = 65.38 Da).
Determination of Water-Soluble Vitamins
Water soluble vitamins, namely, B2, B3, B5, B6, B9, and C, were quantified by HPLC techniques using ultra-high-performance liquid chromatography (U-HPLC; Dionex Ultimate 3000 RSLC, USA, with Chromeleon software). For the determination of vitamins B2 and B3, a sample (1 g) was extracted with 0.1 M HCl, and the tubes were centrifuged at 4,000 rpm at 10°C for 10 min. The supernatant was collected into amber-colored HPLC auto-sampler vials after filtering through a 0.45-μm polyvinylidene fluoride (PVDF) syringe filter. The vitamins were separated on a reverse-phase chromatographic column (Thermo Scientific BDS Hypersil C18 column 250 × 4.6 mm; particle size, 5 μm). The column temperature was maintained at 40°C. Phosphate buffer (0.05 M, pH 3.2) and acetonitrile were used as mobile phase at the ratio of 70:30 (v/v) and flow rate of 1 ml/min. Fluorescence detector (FLD) was used with excitation λ at 445 nm and emission λ at 522 nm for the quantification of vitamin B2, whereas for vitamin B3, phosphate buffer (25 mM, pH 3.02) and acetonitrile at the ratio of 95:5 (v/v) were used as mobile phase at 1-ml/min flow rate and detected at 260 nm by photodiode array detector (PDA). Vitamin B5 was determined by the method suggested by Woollard et al. (2000). Briefly, 1 g of sample was extracted with 3% acetic acid and centrifuged at 4,000 rpm for 10 min (10°C). The supernatant was collected into HPLC auto-sampler vials after filtering through a 0.45-μm PVDF syringe filter. A Thermo Scientific BDS Hypersil C18 column 250 × 4.6 mm, a 5-μm-particle-sized column (maintained at 40°C), was used with phosphate buffer (0.1 M, pH 2.25) and acetonitrile (95:5) as mobile phase adjusted to a flow rate of 1 ml/min and detected at 205 nm using PDA. The quantification of vitamin B6 was carried out with reference to Valls et al. (2001). Briefly, the sample was extracted with 5% metaphosphoric acid and centrifuged at 4,000 rpm for 10 min at 10°C. The supernatant was filtered using a 0.45-μm PVDF syringe filter and injected. The isocratic mobile phase consisting of 25 mM phosphate buffer (pH 3.2) and acetonitrile (70:30 v/v) was passed through a Thermo Scientific BDS Hypersil C18 column (250 × 4.6 mm; particle size, 5 μm). The column was maintained at 35°C with a mobile phase flow at 0.8 ml/min. FLD was used to determine B6 vitamers fixing the excitation λ at 290 nm and emission λ at 395 nm (Valls et al., 2001). Vitamin B9 was quantified by U-HPLC after extracting with the tri-enzyme technique. Briefly, the sample was extracted with phosphate (K2HPO4) buffer containing ascorbic acid, sodium azide, and 2-mercapto ethanol. The sample was subsequently treated with enzymes (α-amylase, protease, and deconjugase) one by one at an appropriate temperature and finally centrifuged at 4,000 rpm (10°C). The supernatant was purified by passing through a strong anion exchange cartridge (SEP-PAK cartridge), filtered using 0.22-μm PVDF syringe filter, and injected into U-HPLC. Phosphate buffer (pH 2.2) and acetonitrile at the ratio of 95:5 (v/v) were passed through a Phenomenex Luna C18 (150 × 2 mm; 3 μm) column at a flow rate of 0.5 ml/min, and the folate vitamers were detected using FLD with excitation and emission λ at 220 and 440 nm, respectively (Rader et al., 1998; Brouwer et al., 2008). Vitamin C (total ascorbic acid) content in the raw and processed millets was analyzed by extracting the sample (1 g) with 3% metaphosphoric acid (w/v) and 8% glacial acetic acid (v/v). The samples were centrifuged, and the supernatant was filtered through a 0.45-μm PVDF syringe filter into HPLC auto-sampler vials and injected in U-HPLC equipped with PDA. Phosphate buffer (0.05 M, pH 3.2) and acetonitrile at the ratio of 90:10 was used as isocratic mobile phase with 1 ml/min flow rate. A Thermo Scientific BDS Hypersil C18 column (250 × 4.6 mm; particle size, 5 μm) was used as stationary phase, and ascorbic acid was detected at 244 nm using PDA (Ekinci and Kadakal, 2005; Hernandez et al., 2006; Phillips et al., 2010).
Nutrient Retention Factor
Nutrient retention factor is the amount of nutrients retained in foods after preparation, processing, or other treatments. It depends on several factors, such as temperature, time, pressure, and many other cooking practices (Vásquez-Caicedo et al., 2008).
The retention factor for water-soluble vitamins and phytic acid was calculated by using the given formula below and expressed as values between 0 and 1 or as a percentage of retention (0–100%). Nutrient content could be expressed in grams, milligrams, or micrograms, depending on the nutrient.
Statistical Analysis
Descriptive statistical analysis was done using the SPSS package (SPSS for Windows, version 16.0. Chicago, USA). The experiment for phytic acid was carried out in quadruplicate, while all the other experiments were carried out in triplicate analyses, and the results were expressed as mean ± standard deviation (SD). One-way ANOVA was performed to evaluate the significance of differences within the treatments at p < 0.05 level of significance.
Results
Proximate Principles
The proximate principles (moisture, protein, ash, fat, and dietary fiber) were analyzed in the raw pearl millet (PMR), finger millet (FMR), and sorghum millet (SMR), the representative samples of which were traditionally processed, and the results are given in Table 1. All proximate values are expressed as grams in 100 g of the edible portion on dry weight basis. The protein content of raw millets, such as PMR, FMR, and SMR, was 9.45 ± 0.37, 7.18 ± 0.42, and 10.60 ± 0.26 g/100 g, respectively. Among the traditionally processed millet foods, the significantly highest (p < 0.05) amount of protein was found in SMFWC (20.57 ± 0.37 g/100 g) and PMFWC (20.27 ± 0.27 g/100 g), followed by FMFWC (16.92 ± 0.07 g/100 g). The lowest amount of protein was found in SMC (9.91 ± 0.18 g/100 g), PMC (9.30 ± 0.06 g/100 g), and FMFW (7.88 ± 0.12 g/100 g).
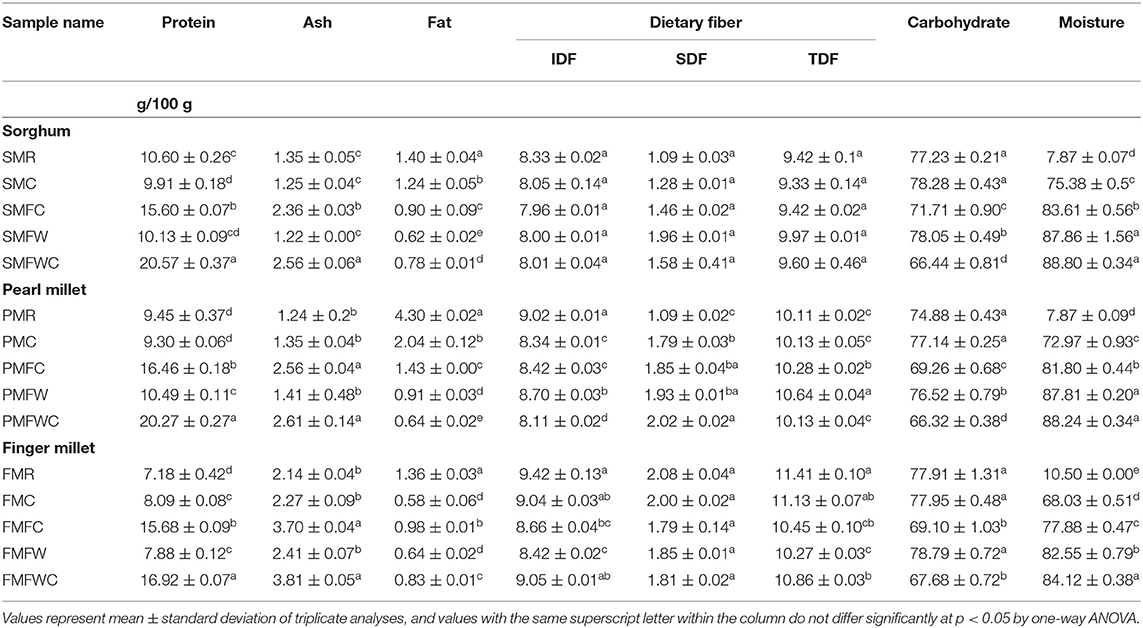
Table 1. Proximate principles of raw and traditionally processed sorghum, pearl millet, and finger millet (g/100 g) in dry weight except for moisture.
FMR contains the highest amount of ash (2.14 ± 0.04 g/100 g) compared to PMR (1.24 g/100 g) and SMR (1.35 g/100 g). Among the different processed foods, the millet cooked, fermented overnight, and added with curd had the highest ash content in all the three millets studied (SMFWC-2.56 ± 0.06, PMFWC-2.61 ± 0.14, and FMFWC-3.81 ± 0.05 g/100 g). The fat content of raw pearl millet was the highest (4.3 g/100 g) among all raw and processed millets. However, SMR and FMR had higher values than their cooked forms (1.40 and 1.36/100 g, respectively). Among the different processing techniques, there was a significant reduction observed in the cooked pearl millet fermented overnight and then added with curd (0.64/100 g). In contrast, the lowest fat content among the differently processed finger millet samples was observed in the cooked finger millet (0.58/100 g). Data on dietary fiber (both insoluble and soluble) analyzed in the raw and cooked millets are presented in Table 1. The total dietary fiber content of sorghum was between 9.33 and 9.97/100 g, while it was 10.13 and 11.4 g/100 g in pearl millet and finger millet, respectively. Among the two dietary fiber fractions, more than 80% are from insoluble dietary fiber. Among three millets, carbohydrate content was observed to be higher in both finger millet and sorghum (around 77%) than in pearl millet (75%). The cooked millets added with curd either before or after fermentation were found to have lesser carbohydrate than the other processed foods.
Total Phytic Acid Content and Its Retention
Data on the total phytic acid content and its retention in traditionally processed sorghum, pearl millet, and finger millet are summarized in Figure 2. The total phytic acid content in raw millets was found to be 8.6 ± 0.15 mg/g (SMR), 5.69 ± 0.19 mg/g (FMR), and 4.77 ± 0.07 mg/g (PMR). Reduction of phytic acid was observed in all the millets after cooking (minimum of 16.14% and maximum of 49.18%) and in subsequent processing where the samples were fermented with or without curd overnight (minimum of 20.96% and maximum of 54.53%). Among the different processes, the maximum reduction in total phytic acid was found in the millets fermented overnight and then added with curd (FMFWC: 3.75 ± 0.06; PMFWC: 3.37 ± 0.17; SMFWC: 3.28 ± 0.09 mg/g).
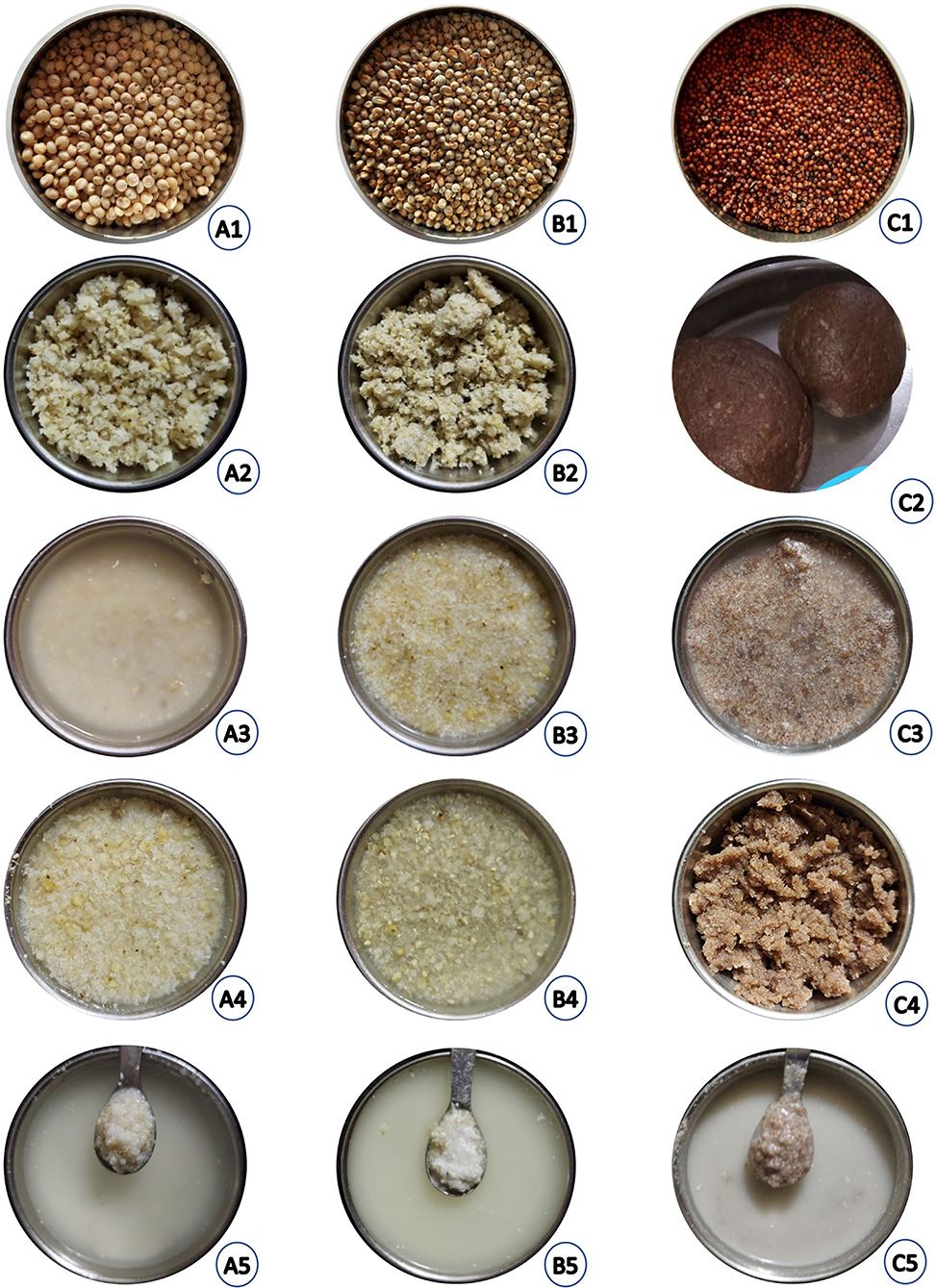
Figure 2. Raw, cooked, and fermented millet foods. (A1–A5): Sorghum (A1—raw, A2—cooked, A3—cooked and fermented with curd overnight, A4—cooked and fermented with water overnight, A5—cooked and fermented with water overnight and added with curd). (B1–B5): Pearl millet (B1—raw, B2—cooked, B3—cooked and fermented with curd overnight, B4—cooked and fermented with water overnight, B5—cooked and fermented with water overnight and added with curd). (C1–C5): Finger millet (C1—raw, C2—cooked, C3—cooked and fermented with curd overnight, C4—cooked and fermented with water overnight, C5—cooked and fermented with water overnight and added with curd).
Phytic acid retention factor in the three millets was exhibited in all the four different processing methods employed (Figure 3). In processed sorghum, least retention of phytic acid was observed in SMFWC (0.31), followed by SMFW and SMC (0.35). In comparison, maximum phytic acid retention was found in SMFW (0.47) because the samples treated with curd create a more appropriate environment to activate the phytase enzyme compared to samples without curd fermentation. Among the traditionally processed pearl millet, minimum retention of phytic acid was detected in PMFWC (0.48), and maximum retention was observed in PMFC (0.57), which may be due to the contribution of phytic acid from curd. The other processed samples, PMC (0.56) and PMFW (0.52), retained more phytic acid. The traditionally processed finger millet samples treated with curd retained less phytic acid, i.e., FMFWC had 0.49, and FMFC retained 0.51. The retention factor for other samples, FMFW and FMC, was 0.69 and 0.52, respectively, compared to the raw sample.
Mineral Composition
Data on the iron (Fe) and zinc (Zn) content of raw and domestically processed sorghum, pearl millet, and finger millet are presented in Table 2. Among the three raw millets analyzed, pearl millet had the highest zinc (3.32 ± 0.15 mg/100 g), followed by finger millet (2.09 ± 0.09 mg/100 g) and sorghum (1.95 ± 0.01 mg/100 g). Between the processed millet samples, zinc content was found to increase in the fermented sorghum (with or without curd) samples. The raw sorghum contains 1.95 mg/100 g, which was increased up to 2.72 mg/100 g in SMFW. However, no significant (p < 0.05) change was observed among the raw and processed pearl millet and finger millet samples (Table 2). Determination of iron content in the three different millets revealed that raw finger millet contained the highest level of iron (5.15 ± 0.75 mg/100 g), followed by sorghum (3.32 ± 0.12 mg/100 g) and pearl millet (3.29 ± 0.19 mg/100 g). Among traditionally processed millet foods, elevated Fe content was distinguished in the millets fermented overnight after traditional cooking (SMFW, 7.24 ± 0.52 mg/100 g; PMFW, 8.40 ± 0.334 mg/100 g; FMFW, 8.95 ± 0.63 mg/100 g). The cooked millet fermented along with curd seems to have the second-highest Fe content. However, all the differently processed millet foods appear with an increased iron concentration in all the three millets studied here.
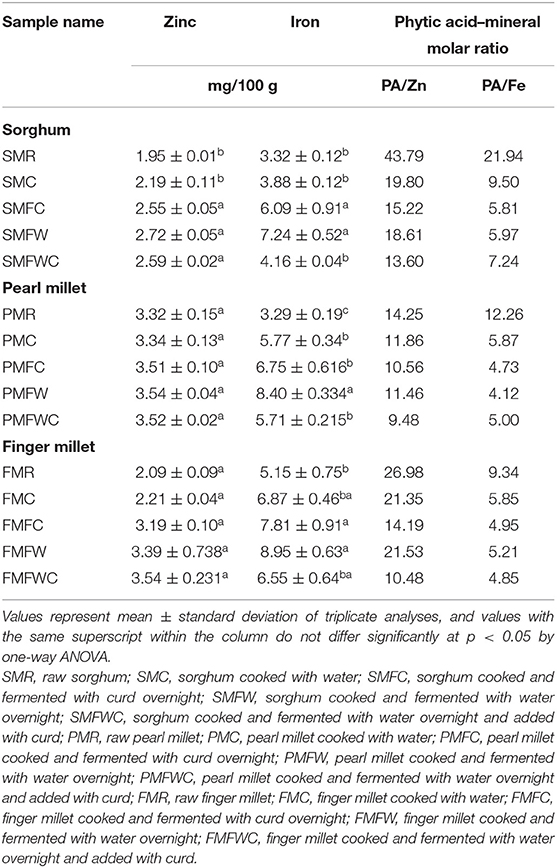
Table 2. Mineral content and phytic acid–mineral molar ratio of traditionally processed sorghum, pearl millet, and finger millet samples.
Estimation of Phytic Acid–Mineral Molar Ratio
Phytic acid–mineral molar ratios (zinc and iron) were determined, and the data are given in Table 2. The raw millets were found to have higher ratio values than the processed samples. The highest molar ratio between phytic acid–Zn and phytic acid–Fe was recorded in raw sorghum (43.79 and 21.94, respectively). The molar ratio between phytic acid and Zn was 14.25 in raw pearl millet and 26.98 in finger millet. The Zn molar ratio was significantly reduced in all the processed millets after fermenting, followed by the addition of curd (SMFWC, 13.60; PMFWC, 9.48; FMFWC, 10.48). The molar ratio between phytic acid and Fe in raw sorghum was 21.94, followed by raw pearl millet (12.26) and finger millet (9.34). Cooking and processing of millet were found to reduce the phytic acid–Fe molar ratios. The lowest Fe molar ratio was found in the cooked millets subsequently fermented overnight in the case of pearl millet and sorghum (4.12 and 5.97, respectively), whereas the cooked finger millet which was then fermented and added with curd seems to be better for the lowest phytic acid–Fe molar ratio (4.85).
Water-Soluble Vitamin Analysis
The effect of traditional processing on water-soluble vitamins in sorghum, pearl millet, and finger millet is presented in Figures 4A–F. Pearl millet was the chief source of vitamin B2 (0.223 ± 0.018 mg/100 g) compared to the other millets. Among traditionally processed millets, vitamin B2 was significantly (p < 0.05) higher in PMFC (0.173 ± 0.002 mg/100 g), followed by SMFW (0.136 ± 0.039 mg/100 g) and FMFWC (0.115 ± 0.012 mg/100 g). However, maximum B2 retention was seen in SMFW (64.4%). Vitamin B3 content was higher in sorghum (2.588 ± 0.112 mg/100 g) and lowest in finger millet (1.723 ± 0.108 mg/100 g). Vitamin B3 content was reduced to 1.806 ± 0.132 mg/100 g in sorghum (SMFWC), with 59% retention, whereas B3 was reduced to 1.349 ± 0.067 mg/100 g in pearl millet (PMFW), with 46% retention, and 0.629 ± 0.021 mg/100 g in finger millet (FMC), with 23% retention.
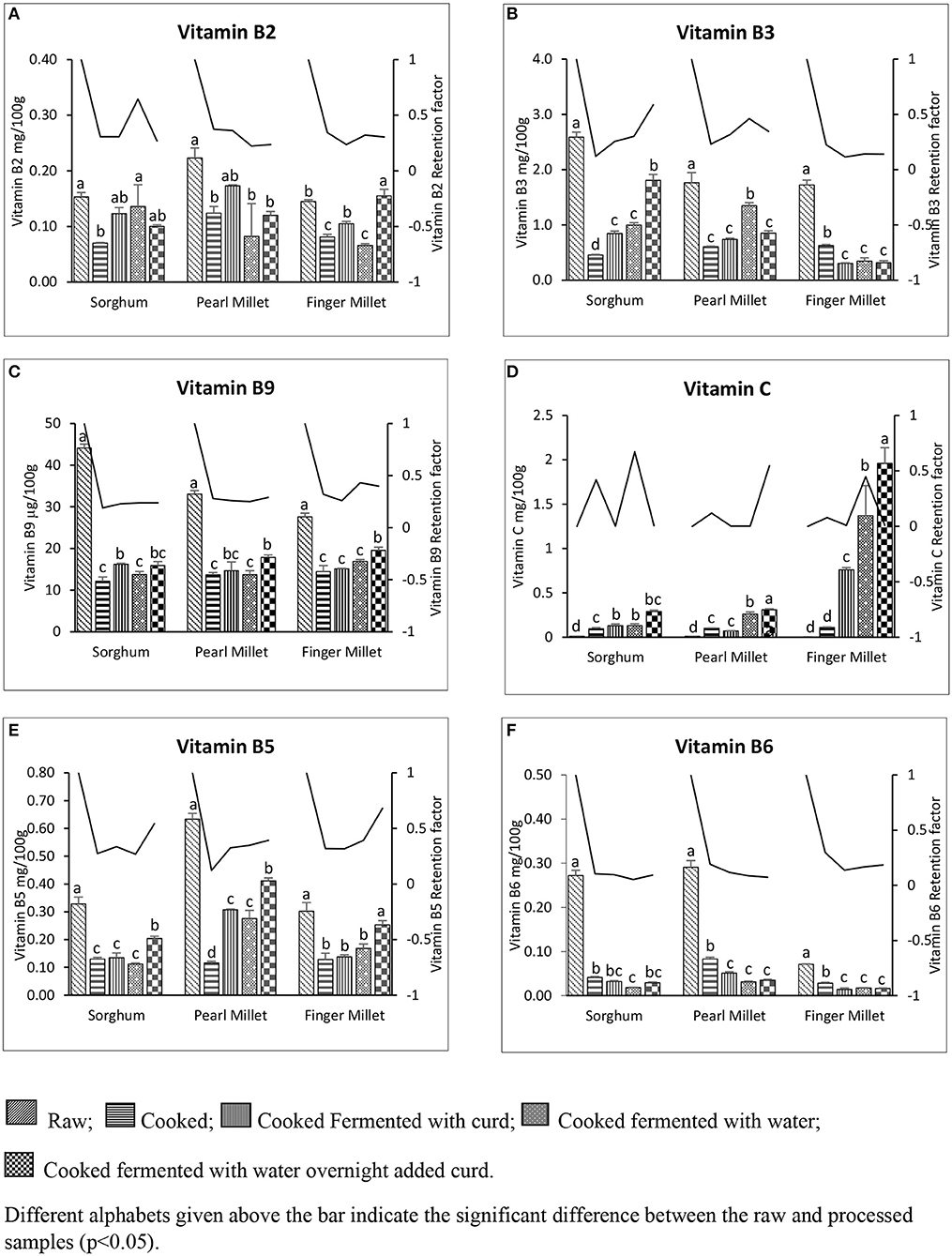
Figure 4. Water-soluble vitamin concentration and its retention in the different raw and cooked millets. (A) Vitamin B2. (B) Vitamin B3. (C) Vitamin B5. (D) Vitamin B6. (E) Vitamin B9. (F) Vitamin C. Different alphabets given above the bar indicate the statistical difference between the raw and processed samples (p < 0.005).
Vitamin B5 was highest in the pearl millet (0.633 ± 0.021 mg/100 g) among the three raw millets analyzed. However, the processing reduced the B5 content in all the millets. The cooked millet fermented overnight and then added with curd was found to have more vitamin B5 compared to those under the other processes (SMFWC, 0.204 ± 0.008 mg/100 g; PMFWC, 0.411 ± 0.011 mg/100 g; FMFWC, 0.253 ± 0.015 mg/100 g). A maximum of 68% retention was observed in FMFWC, followed by 54% in SMFWC and 39% in PMFWC. Vitamin B6 content in the raw and processed millets is illustrated in Figure 4D. Among the raw millets, highest B6 content was seen in pearl millet (0.291 ± 0.015 mg/100 g) and the lowest in finger millet (0.071 ± 0.001 mg/100 g). The traditionally cooked millets have higher levels of B6 (SMC, 0.041 ± 0.002 mg/100 g; PMC, 0.083 ± 0.004 mg/100 g; FMC, 0.028 ± 0.002 mg/100 g), with retention of 10.4, 19, and 29.7% in SMC, PMC, and FMS, respectively.
Quantification of vitamin B9 by the U-HPLC technique in the raw and traditionally processed millets is shown in Figure 4E. Vitamin B9 (total folate) was more in sorghum (44.135 ± 1.30 μg/100 g), followed by pearl millet (33.309 μg/100 g) and finger millet (27.576 ± 1.23 μg/100 g). The processing was found to reduce the folate content significantly in all the millets studied. Maximum retention of 43% was found in FMFW (19.549 ± 1.08 μg/100 g) among the other processes in finger millets. The cooked millet samples fermented overnight and then added with curd were found to have maximum retention in pearl millet (29%) and sorghum (24%), with folate content of 17.852 ± 0.79 and 16.216 ± 0.28 μg/100 g, respectively. Analyses of vitamin C in the three raw samples and traditionally processed millets are illustrated in Figure 4F. The vitamin C (total ascorbic acid) content in raw millet was found to be below the detectable limit. However, a quantifiable amount of vitamin C was recorded in all the processed millets. The highest vitamin C content was found in the millets cooked and fermented overnight and then added with curd. Finger millet had the highest vitamin C content (FMFWC, 1.96 ± 0.18 mg/100 g), followed by pearl millet (PMFWC, 0.311 mg/100 g) and sorghum (SMFWC, 0.288 mg/100 g).
Discussion
Millets are nutritionally enriched crops which require less maintenance in terms of water, fertilizers, pesticides, etc., compared to other grains and provide reliable harvest. Due to these characteristics, millets are termed next-generation crops (Saleh et al., 2013; Devi et al., 2014). However, it was hypothesized that millets contain phytic acid, which makes them nutritionally inferior in terms of mineral availability. All cereal grains contain phytic acid, which is mainly concentrated in the bran layer, except for maize where 80% was found in the germ. It was greatly emphasized that phytic acid can be reduced by milling, cooking, germination, fermentation, etc.—for example, average phytic acid content in brown rice was estimated to be between 541 and 742 mg/100 g, which can be reduced to 37–64% by milling and a further 20% by cooking (Okazaki and Katayama, 2005; Gupta et al., 2015; Longvah et al., 2017). As high as 742 mg/100 g of phytic acid has been reported in brown rice collected all over India. Reduction of phytic acid content in millets is a great challenge of this hour. Traditional processing/cooking may prove to be a better and simple way in reducing phytic acid content, hence increasing the mineral bioavailability. The present study aimed to investigate the effect of traditional processing on the nutrient and anti-nutrient (phytic acid) components of three major millets, namely, sorghum, pearl millet, and finger millet. The results showed that cooking reduced the protein content in SMC (9.91 ± 0.18) and PMC (9.30 ± 0.06 g/100 g), which may be due to the leaching of soluble nitrogen into the desired solution (water) (Njoki et al., 2014). Protein content was found to be significantly (p < 0.05) higher in cooked millet samples fermented overnight in water and then added with curd (SMFWC, PMFWC, and FMFWC), followed by the samples fermented directly with curd (SMFC, PMFC, and FMFC), as the fermentation process tend to increase the digestibility of protein. Similar findings were reported by Mallasy et al. (2011), where the protein digestibility increased significantly (p < 0.05) from 56.03 to 83.65% in pearl millet supplemented with whey protein and fermented for a period of 14 h. Mariod et al. (2016) and Osman (2011) also demonstrated increased protein content in sorghum and pearl millet due to microbial fermentation.
The moisture content of raw millet was comparable to the reported values of Afify et al. (2012) and Longvah et al. (2017). However, a study conducted by Kulthe et al. (2016) reported a slightly higher moisture content (11.78 g/100 g) in pearl millet. It was observed that the moisture content in all the cooked samples (SMC, FMC, and PMC) increased significantly (p < 0.05) due to the addition of water during cooking (Table 1). The addition of water/curd for overnight fermentation further increases the moisture content of all the samples. Ogodo et al. (2019) described that, with increases in fermentation time, moisture content increases in sorghum, which is attributed to the addition of water to the substrate prior to fermentation. Ojokoh et al. (2015) also revealed a higher moisture content of fermented pearl millet blends compared to unfermented samples. In our study, the highest moisture was found in SMFWC (88.80 ± 0.34 g/100 g), PMFWC (88.24 ± 0.34 g/100 g), and FMFWC (84.12 ± 0.38 g/100 g) because of added curd after overnight fermentation.
Ash content was found to be significantly (p < 0.05) highest in FMFWC (3.81 ± 0.05 g/100 g), PMFWC (2.61 ± 0.14 g/100 g), and SMFWC (2.56 ± 0.06 g/100 g), followed by FMFC (3.70 ± 0.04 g/100 g), PMFC (2.56 ± 0.04 g/100 g), and SMFC (2.36 ± 0.03 g/100 g). This can be attributed to the addition of curd in both treatments, whereas SMFW, PMFW, and FMFW showed no significant (p < 0.05) increase in ash content compared to the cooked and raw samples. Similarly, Pelig-Ba (2009) and Osman (2011) also observed no increase in ash content in fermented millet.
Among all the traditional processing methods, SMFC (0.90 ± 0.09), PMFC (1.43 ± 0.00), and FMFC (0.98 ± 0.01) showed a significantly (p < 0.05) high fat content due to the addition of curd. On the other hand, fat content was reduced in the processed millets since these were fermented only in water (SMFW, 0.62 ± 0.02 g/100 g; PMFW, 0.91 ± 0.03 g/100 g; FMFW, 0.64 ± 0.02 g/100 g). Similar results were reported by Sade (2009) in fermented pearl millet, where fat content was reduced after fermentation from 5.7 to 2.4 g/100 g. Mariod et al. (2016) also reported a reduction in fat content from 3.10 to 2.06 g/100 g in fermented sorghum. Fermentation also reduces the total carbohydrate content of the traditionally processed samples. Cooking did not reduce the carbohydrate content significantly (p > 0.05), whereas samples fermented and added with curd had significantly reduced carbohydrate (p < 0.05). Mariod et al. (2016) stated that microbial fermentation and baking of sorghum decreased the carbohydrate content, a finding which is parallel to that of the present study, where carbohydrate content decreased from 77.23 ± 0.21 (SM) to 66.44 ± 0.81 g/100 g (SMFWC). Nevertheless, an increased amount of IDF, SDF, and TDF was observed among all the fermented samples.
Phytic acid is the principal storage form of phosphorus in seeds which forms insoluble complexes with minerals, such as zinc, calcium, magnesium, and iron, thereby decreasing their bioavailability. The phytic acid content of raw sorghum, pearl millet, and finger millet was 8.6 ± 0.15, 4.77 ± 0.07, and 5.69 ± 0.19 mg/g, respectively. Makokha et al. (2002) and Netravati et al. (2017) also reported similar values in millets. It was reported that cooking the millets reduced the phytic acid content between 11.71 and 16.14%. The major reason for this reduction is the leaching of phytic acid during cooking and thermal degradation (Kataria et al., 1989; Sihag et al., 2015). Our study showed a significant reduction in phytic acid content at each level of treatment, which ranged from 3.16 to 62.9%. Among the traditionally processed sorghum, SMFWC showed the highest reduction of phytic acid at up to 62.9% (3.91 ± 0.05 mg/g). A similar trend in reduction of phytic acid was seen among traditionally processed pearl millets and finger millets, where PMFWC and FMFWC showed the highest values, i.e., 29.35% (3.37 mg/g) and 34.1% (3.75 ± 0.06 mg/g) reduction, respectively. As reported by Haard (1999) and Reale et al. (2007), fermentation provides optimum pH (created by the lactic acid bacteria due to lactic acid production) that activates the phytase enzyme, eventually leading to the degradation of phytic acid and increasing the solubility of minerals. The results obtained were similar to those reported in the literature in such a way that fermentation reduced phytic acid content by 50% (Towo et al., 2006; Kayode et al., 2007; Wedad et al., 2008; Osman, 2011). It was also reported that the reduction of phytic acid increases with an increase in fermentation time (Makokha et al., 2002).
The quantification of minerals revealed a significant increase in zinc and iron content in cooked samples, which are in contrast to the results of Borade et al. (1984) and Avola et al. (2012), who explained that pressure cooking leaches out minerals and the anti-nutritional factors from pearl millet grains resulted in a reduction of mineral content. Afify et al. (2012) demonstrated that fermentation decreased the zinc content from 4.43 to 3.29 mg/100 g in sorghum. The present study reveals a slight increase in zinc content after fermentation with curd, but which is not significant at p < 0.05. The iron content of millets also increases after fermentation, which is similar to the finding of Kindiki et al. (2015) that 24 h of fermentation considerably increases iron in pearl millet. Zinc and iron play a significant role in growth and development. Zinc is involved in cellular growth and differentiation. Its deficiency causes impaired growth, immune dysfunction, increased morbidity and mortality, adverse pregnancy outcomes, and abnormal neurobehavioral development. It was estimated that one-third of the population of the world are at a high risk of zinc deficiency and are living in low-income countries (Bagherani and Smoller, 2016). Similarly, iron deficiency also affects cognitive development, pregnancy, resistance to infection, work capacity, productivity, formation of heme proteins, and flavoproteins (Fairweather-Tait and Hurrell, 1996; Swaminathan et al., 2019). Minerals from plant sources have very low bioavailability because they form complexes with non-digestible materials, such as phytic acid (Torre et al., 1991). Fermentation is one of the traditional methods applied to free up these mineral complexes in order to make the minerals readily available (Pranoto et al., 2013).
The phytic acid–mineral molar ratios are used to estimate the negative effect of phytic acid on mineral bioavailability (Dahdouh et al., 2019). These are associated with mineral absorption capacity; the higher the molar ratio, the lower the mineral absorption. Phytic acid–zinc molar ratio <14 and phytic acid–iron molar ratio <1 indicate a positive effect on mineral bioavailability (Table 2). In the present study, the phytic acid–zinc and phytic acid–iron molar ratios for SMR, PMR, and FMR were initially higher than the stated values of 14 and 1. Similar findings were also reported by Netravati et al. (2017) in sorghum and finger millet. This ratio decreased progressively after the application of different processing methods, especially those involving fermentation (SMFWC, PMFC, PMFW, PMFWC, and FMFWC showed a PA–Zn molar ratio that was <14). This reduction in molar ratio was mainly due to the reduction in phytic acid content. A study conducted by Murali and Kapoor (2003) revealed that fermentation of finger millet with individual cultures of Lactobacillus brevis, Lactobacillus fermentum, and Saccharomyces cerevisiae for 24 and 48 h at 37°C resulted in significant reductions in phytic acid content, subsequently resulting in a lower phytic acid–zinc molar ratio. Nair and Iyengar (2009) reported that the low bioavailability of minerals is mainly attributed to the low mineral levels and the presence of high phytic acid content and other anti-nutritional factors. Thus, the bioavailability of minerals (iron and zinc) can be improved significantly by the application of different processing methods like soaking and fermentation (Norhaizan and Nor Faizadatul Ain, 2009; Afify et al., 2011).
Quantification of water-soluble vitamins revealed a significant (p < 0.05) increase in vitamin B2, B3, B5, and B9 among the fermented samples (PMFC, FMFC, SMFC, SMFWC, PMFWC, and FMFWC) compared to the cooked millets. Cooking (SMC, PMC, and FMC) reduced the vitamin B2, B3, B5, and B9 levels significantly (p < 0.05) compared to the raw and fermented samples due to thermal degradation, as vitamins are heat sensitive. Ekinci and Kadakal (2005) and Ochanda et al. (2010) also revealed a significant increase in B-complex vitamins after fermentation. It was reported that the fermentation of cereals with Lactobacillus or yeast strains could increase their vitamin content to a greater extent. These microorganisms could be used as a starter to improve the nutritional quality of food (Nyanzi and Jooste, 2012).
The vitamin C content was below the detectable limit in all the raw millet samples analyzed. These findings were similar to the results reported by Shobana et al. (2013), which showed that the vitamin C content ranged from 0.0 to 0.1 mg/100 g. This content increases significantly at each level of treatment. Nutrient retention factor was calculated for the analyzed water-soluble vitamins to estimate the amount of nutrients retained in foods after traditional processing, which ranged from 0 (no retention) to 1 (complete retention). Calculation of nutrient retention for water-soluble vitamins showed 63% (vitamin C), 64% (vitamin B2), 58% (vitamin B3), and 68% (vitamin B5) retention in the processed millets. Nutrient retention factor depends on several factors, such as temperature, time, pressure, and many other cooking methods (Vásquez-Caicedo et al., 2008). The USDA (2007) reported that the cooking method of white rice in the US retained 90% of riboflavin, 95% of niacin, 90% pyridoxine, 60% of folic acid, and 75% of ascorbic acid. The retention was very high compared to the values for millets in the present study, and this may be due to the long cooking time and method of cooking. Our literature survey found no previous data on nutrient retention in millets during various processing methods.
Conclusion
In the present study, nutrient and anti-nutrient retention of raw and traditionally processed millets was investigated. The study revealed that phytic acid content was significantly reduced in traditionally processed sorghum. The sample that was traditionally cooked, fermented overnight, and then added with curd was found to have a reduced phytic acid content to a greater extent (62.9%), which means that the process may improve the bioavailability of minerals, especially iron and zinc. High retention of water-soluble vitamins, such as B2 (64%), B3 (58%), and B5 (68%), was found in the processed millets. Further investigation on the bioavailability of iron and zinc in these traditionally processed millets is desirable in order to confirm the intake of iron and zinc in millet diets.
Data Availability Statement
The raw data supporting the conclusions of this article will be made available by the authors, without undue reservation.
Author Contributions
RA, TL, and CB contributed to the conception and design of the study and guided the study. HS and KS executed the experiments. HS and CB wrote the first draft of the manuscript and carried out the statistical analysis. All authors contributed to the preparation and revision of the manuscript.
Funding
This project was supported with institutional fund from ICMR-National Institute of Nutrition (17-FC02).
Conflict of Interest
The authors declare that the research was conducted in the absence of any commercial or financial relationships that could be construed as a potential conflict of interest.
Publisher's Note
All claims expressed in this article are solely those of the authors and do not necessarily represent those of their affiliated organizations, or those of the publisher, the editors and the reviewers. Any product that may be evaluated in this article, or claim that may be made by its manufacturer, is not guaranteed or endorsed by the publisher.
Acknowledgments
The authors would like to express their gratitude to the Director, ICMR-Natational Institute of Nutrition, for the financial support and scientific encouragement to conduct this experiment at the said institution.
Abbreviations
AOAC, Association of Official Agricultural Chemists; PA, phytic acid; MW, molecular weight; RF, retention factor; YF, yield factor; SMR, raw sorghum millet; SMC, cooked sorghum millet; SMFC, sorghum millet cooked and fermented with curd overnight; SMFW, sorghum millet cooked and fermented with water overnight; SMFWC, sorghum millet cooked and fermented with water overnight and added with curd; PMR, raw pearl millet; PMC, cooked pearl millet; PMFC, pearl millet cooked and fermented with curd overnight; PMFW, pearl millet cooked and fermented with water overnight; PMFWC, pearl millet cooked and fermented with water overnight and added with curd; FMR, raw finger millet; FMC, cooked finger millet; FMFC, finger millet cooked and fermented with curd overnight; FMFW, finger millet cooked and fermented with water overnight; FMFWC, finger millet cooked fermented with water overnight and added with curd; SDF, soluble dietary fiber; IDF, insoluble dietary fiber; TDF, total dietary fiber; FAO, Food and Agriculture Organization; IP, inositol monophosphate; IP2, inositol bisphosphate; IP3, inositol trisphosphate; IP5, inositol pentakisphosphate; IP6, inositol hexaphosphate; RTE, ready-to-eat; RTC, ready-to -cook; Fe, iron; Zn, zinc; Ca, calcium; Mg, magnesium; Na, sodium; P, phosphorous; K, potassium; DW, dry weight; IZiNCG, International Zinc Nutrition Consultative Group; PDS, public distribution system.
References
Afify, A. E. M. M., El-Beltagi, H. S., Abd El-Salam, S. M., and Omran, A. A. (2011). Bioavailability of iron, zinc, phytate and phytase activity during soaking and germination of white sorghum varieties. PLoS ONE 6, e25512. doi: 10.1371/journal.pone.0025512
Afify, A. E. M. M., El-beltagi, H. S., Abd El-Salam, S. M., and Omran, A. A. (2012). Effect of soaking, cooking, germination and fermentation processing on proximate analysis and mineral content of three white sorghum varieties (Sorghum bicolor L. Moench). Notulae Botanicae Horti Agrobotanici Cluj-Napoca. 40, 92–98. doi: 10.15835/nbha4027930
Agte, V. V., Tarwadi, K. V., and Chiplonkar, S. A. (1999). Phytate degradation during traditional cooking: significance of the phytic acid profile in cereal-based vegetarian meals. J. Food Composition Anal. 12, 161–167. doi: 10.1006/jfca.1999.0826
Anderson, J. W., Hanna, T. J., Peng, X., and Kryscio, R. J. (2000). Whole grain foods and heart disease risk. J. Am. Coll. Nutr. 19, 291–299. doi: 10.1080/07315724.2000.10718963
Anitha, S., Kane-Potaka, J., Tsusaka, T.W., Botha, R., Rajendran, A., Givens, D.I., et al. (2021). A systematic review and meta-analysis of the potential of millets and sorghum for managing and preventing diabetes mellitus. Front. Nutr. 8, 687428. doi: 10.3389/fnut.2021.687428
AOAC (2016). Official Methods of Analysis. 20th Edn. Gaithersburg, MD: The Association of Official Analytical Chemists.
Avola, G., Patanè, C., and Barbagallo, R. N. (2012). Effect of water cooking on proximate composition of grain in three Sicilian chickpeas (Cicer arietinum L.). LWT Food Sci. Technol. 49, 217–220. doi: 10.1016/j.lwt.2012.07.004
Bagherani, N., and Smoller, B. R. (2016). An overview of zinc and its importance in dermatology-Part I: importance and function of zinc in human beings. Glob. Dermatol. 3, 330–336. doi: 10.15761/GOD.1000185
Bhagavatula, S., Rao, P. P., Basavaraj, G., and Nagaraj, N. (2013). Sorghum and Millet Economies in Asia – Facts, Trends and Outlook. Patancheruvu, Andhra Pradesh: International Crops Research Institute for the Semi-Arid Tropics.
Bhat, S., Nandini, C., and Tippeswamy, V. (2018). Significance of small millets in nutrition and health -A review. Asian J. Diary Food Res. 37, 35–40. doi: 10.18805/ajdfr.DR-1306
Binita, R., and Khetarpaul, N. (1997). Probiotic fermentation: effect on antinutrients and digestibility of starch and protein of indigenously developed food mixture. Nutr. Health. 11, 139–147. doi: 10.1177/026010609701100301
Borade, V. P., Kadam, S. S., and Salunkhe, D. K. (1984). Changes in phytate phosphorus and minerals during germination and cooking of horse gram and moth bean. Plant Foods Hum Nutr. 34, 151–157. doi: 10.1007/BF01094843
Brouwer, V. D., Storozhenko, S., Steene, J. C. V. D., Wille, S. M. R., Stove, C. P., Straeten, D. V. D., et al. (2008). Optimisation and validation of a liquid chromatography – tandem mass spectrometry method for folates in rice. J Chromatogr A. 1215, 125–132. doi: 10.1016/j.chroma.2008.11.004
Chapke, R. R., Shyamprasad, G., and Das, I. K. (2018). Improved Millets Production Technologies and Their Impact. Technology Bulletin. Hyderabad: ICAR-Indian Institute of Millets Research.
Dahdouh, S., Grande, F., Espinosa, S. N., Vincent, A., Gibson, R., Bailey, K., et al. (2019). Development of the FAO/INFOODS/IZINCG global food composition database for phytate. J Food Composition Anal. 78, 42–48. doi: 10.1016/j.jfca.2019.01.023
Devi, P. B., Vijayabharathi, R., Sathyabama, S., Malleshi, N. G., and Priyadarisini, V. B. (2014). Health benefits of finger millet (Eleusine coracana L.) polyphenols and dietary fiber: a review. J. Food Sci. Technol. 51, 1021–1040. doi: 10.1007/s13197-011-0584-9
Ekinci, R., and Kadakal, C. (2005). Determination of seven water-soluble vitamins in tarhana, a traditional Turkish cereal food, by high-performance liquid chromatography. Acta Chromatogr. 15, 289.
Fairweather-Tait, S., and Hurrell, R. F. (1996). Bioavailability of minerals and trace elements: members of EC flair concerted action no. 10: Measurements of micronutrient absorption and status. Nutr. Res. Rev. 9, 295–324. doi: 10.1079/NRR19960016
FAO/WHO (2001). Human Vitamin and Mineral Requirements. Report of a joint FAO/WHO expert consultation Bangkok. Thailand: FAO/WHO.
FAOSTAT (2019). Millet Production. Available online at: http://www.fao.org/faostat/en/#data/QC (accessed November 5, 2021).
Gupta, R. K., Gangoliya, S. S., and Singh, N. K. (2015). Reduction of phytic acid and enhancement of bioavailable micronutrients in food grains. J. Food Sci. Technol. 52, 676–684. doi: 10.1007/s13197-013-0978-y
Guttieri, M., Bowen, D., Dorsch, J. A., Raboy, V., and Souza, E. (2004). Identification and characterization of a low phytic acid wheat. Crop Sci. 44, 418–424. doi: 10.2135/cropsci2004.4180
Haard, N. F. (1999). Fermented Cereals: A Global Perspective. FAO Agricultural Services Bulletin 138. Rome: Food and Agriculture Organisation.
Hernandez, Y., Lobo, G. M., and Gonzalez, M. (2006). Determination of vitamin C in tropical fruits: a comparative evaluation of methods. Food Chem. 96, 654–664. doi: 10.1016/j.foodchem.2005.04.012
Jacobs, D. R., Meyer, K. A., Kushi, L. H., and Folsom, A. R. (1998). Whole grain intake may reduce risk of coronary heart disease death in postmenopausal women: the Iowa Women's Health Study. Am. J. Clin. Nutr. 68, 248–257. doi: 10.1093/ajcn/68.2.248
Jacobs, D. R., Slavin, J., and Marquart, L. (1995).Whole grain intake and cancer: a review of literature. Nutr. Cancer 22, 221–229. doi: 10.1080/01635589509514411
Kataria, A., Chauhan, B. M., and Punia, D. (1989). Antinutrients and protein digestibility (in vitro) of mung bean as affected by domestic processing and cooking. Food Chem. 32, 9–17. doi: 10.1016/0308-8146(89)90003-4
Kayode, A. P. P., Hounhouigan, J. D., and Nout, M. J. R. (2007). Impact of brewing process operations on phytate, phenolic compounds and in vitro solubility of iron and zinc in opaque sorghum beer. LWT Food Sci Technol. 40, 834–841. doi: 10.1016/j.lwt.2006.04.001
Kindiki, M. M., Onyango, A., and Kyalo, F. (2015). Effects of processing on nutritional and sensory quality of pearl millet flour. Food Sci. Qual. Manage. 42, 13–19.
Kulthe, A. A., Thorat, S. S., and Lande, S. B. (2016). Characterization of pearl millet cultivars for proximate composition, minerals and anti-nutritional contents. Adv. Life Sci. 5, 4672–4675.
Liang, J., Han, B. Z., Nout, M. R., and Hamer, R. J. (2008). Effects of soaking, germination and fermentation on phytic acid, total and in vitro soluble zinc in brown rice. Food Chem. 110, 821–828. doi: 10.1016/j.foodchem.2008.02.064
Liu, S. (2002). A prospective study of dietary fiber intake and risk of cardiovascular disease among women. J. Am. Coll. Cardiol. 39, 49–56. doi: 10.1016/S0735-1097(01)01695-3
Liu, S., Manson, J. E., Stampfer, M. J., Hu, F. B., Gio vanucci, E., Colditz, G.A., et al. (2000). A prospective study of whole grain intake and risk of type 2 diabetes mellitus in US women. Am. J. Public Health. 90, 1409–1415. doi: 10.2105/AJPH.90.9.1409
Liu, S., Stampfer, M. J., Hu, F. B., Giovanucci, E., Rimm, E., Manson, J. E., et al. (1999). Whole grain consumption and risk of coronary heart disease: results from the nurses' health study. Am. J. Clin. Nutr. 70, 412–419. doi: 10.1093/ajcn/70.3.412
Longvah, T., Ananthan, R., Bhaskarachary, K., and Venkaiah, K. (2017). Indian Food Composition Tables. Hyderabad: National Institute of Nutrition, Indian Council of Medical Research, 2–58.
Lönnerdal, B. O., Sandberg, A. S., Sandström, B., and Kunz, C. (1989). Inhibitory effects of phytic acid and other inositol phosphates on zinc and calcium absorption in suckling rats. J. Nutr. 119, 211–214. doi: 10.1093/jn/119.2.211
Lopez, H. W., Leenhardt, F., Coudray, C., and Remesy, C. (2002). Minerals and phytic acid interactions: is it a real problem for human nutrition? Int. J. Food Sci. Technol. 37, 727–739. doi: 10.1046/j.1365-2621.2002.00618.x
Makokha, A. O., Oniang'o, R. K., Njoroge, S. M., and Kamar, O. K. (2002). Effect of traditional fermentation and malting on phytic acid and mineral availability from sorghum (Sorghum bicolor) and finger millet (Eleusine coracana) grain varieties grown in Kenya. Food Nutr. Bull. 23, 241–245. doi: 10.1177/15648265020233S147
Mallasy, L. O., El Tinay, A. H., Elkhalifa, A. O., Mohiedeen, I. E., Ali, M. A. M., and Yousif, N. E. (2011). Effect of fermentation and cooking on protein characteristics of pearl millet flour supplemented with whey protein. Electron. J. Environ. Agric. Food Chem. 10, 2057–2064.
Mariod, A. A., Idris, Y. M., Osman, N. M., Mohamed, M. A., Sukrab, A. M., Farag, M. Y., et al. (2016). Three Sudanese sorghum-based fermented foods (kisra, hulu-mur and abreh): comparison of proximate, nutritional value, microbiological load and acrylamide content. Ukrainian J. Food Sci. 4, 216–228. doi: 10.24263/2310-1008-2016-4-2-4
Meyer, K. A., Kushi, L. H., Jacobs, D. R. J., Slavin, J., Sellers, T. A., and Folsom, A. R. (2000). Carbohydrates, dietary fiber, incident type 2 diabetes mellitus in older women. Am. J. Clin. Nutr. 71, 921–930. doi: 10.1093/ajcn/71.4.921
Muniappan, K., Raghavan, V., Nachimuthu, V., Raveendran, M., Panaiyuran, S., Vediyappan, V., et al (2018). Scaling Up Small Millet Post-harvest and Nutritious Food Products Project. Technical report. CIFSRF, Canada. Available online at: http://hdl.handle.net/10625/57029 (accessed February 15, 2021).
Murali, A., and Kapoor, R. (2003). Effect of natural and pure culture fermentation of finger millet on zinc availability as predicted from HCl extractability and molar ratios. J. Food Sci. Technol. 40, 112–114.
Nair, K. M., and Iyengar, V. (2009). Iron content, bioavailability & factors affecting iron status of Indians. Indian J. Med. Res. 130, 634–645.
Netravati, H., Geetha, K., Vikram, S.R., and Reddy, Y.A.N. (2017), Effect of household germination on phytic acid iron in finger millet [Eleusine coracana (L.) Gaertn]. Indian J. Pure Appl. Biosci. 5, 958–963. doi: 10.18782/2320-7051.5740
Nicodemus, K. K., Jacobs, D. R. J., and Folsom, A. R. (2001). Whole and refined grain intake and risk of incident postmenopausal breast cancer. Cancer Causes Control 12, 917–925. doi: 10.1023/A:1013746719385
Njoki, J. W., Sila, D. N., and Onyango, A. N. (2014). Impact of processing techniques on nutrient and anti-nutrient content of grain amaranth (Amaranth albus). Food Sci. Qual. Manage. 25, 10–17.
Norhaizan, M. E., and Nor Faizadatul Ain, A. W. (2009). Determination of phytate, iron, zinc, calcium contents and their molar ratios in commonly consumed raw and prepared food in Malaysia. Malays. J. Nutr. 15, 213–222.
NSSO (2012). Consumption expenditure survey (68th round), International Household Survey Network Schedule 1.0 (Type 1). India.
Nyanzi, R., and Jooste, P. J. (2012). “Cereal-based functional foods”, in Probiotics, ed E. Rigobelo (Rijeka: InTech Open).
Ochanda, S. O., Akoth, O. C., Mwasaru, A. M., Kagwiria, O. J., and Mathooko, F. M. (2010). Effects of malting and fermentation treatments on group B-vitamins of red sorghum, white sorghum and pearl millets in Kenya. J. Appl. Biosci. 34, 2128–2134.
Ogodo, A. C., Ugbogu, O. C., Onyeagba, R. A., and Okereke, H. C. (2019). Microbiological quality, proximate composition and in vitro starch/protein digestibility of Sorghum bicolor flour fermented with lactic acid bacteria consortia. Chem. Biol. Technol. Agric. 6, 1–9. doi: 10.1186/s40538-019-0145-4
Ojokoh, A. O., Fayemi, O. E., Ocloo, F. C. K., and Nwokolo, F. I. (2015). Effect of fermentation on proximate composition, physicochemical and microbial characteristics of pearl millet (Pennisetum glaucum (L.) R. Br.) and Acha (Digitaria exilis (Kippist) Stapf) flour blends. J. Agric. Biotechnol. Sustain. Dev. 7, 1–8. doi: 10.5897/JABSD2014.0236
Okazaki, Y., and Katayama, T. (2005). Reassessment of the nutritional function of phytic acid, with special reference to myo-inositol function. J. Jpn. Soc. Nutr. Food Sci. 58, 151–156. doi: 10.4327/jsnfs.58.151
Osman, M. A. (2011). Effect of traditional fermentation process on the nutrient and antinutrient contents of pearl millet during preparation of Lohoh. J. Saudi Soc. Agric. Sci. 10, 1–6. doi: 10.1016/j.jssas.2010.06.001
Pelig-Ba, K. B. (2009). Effect of ash, KOH and millet on the fermentation of Parkia biglobosa seeds to form a condiment. Pakistan J. Nutr. 8, 1548–1554. doi: 10.3923/pjn.2009.1548.1554
Phillips, K. M., Tarrag'o-Trani, M. T., Gebhardt, S. E., Exler, J., Patterson, K. Y., Haytowitz, D. B., et al. (2010). Stability of vitamin C in frozen raw fruit and vegetable homogenates. J. Food Composition Anal. 23, 253–259. doi: 10.1016/j.jfca.2009.08.018
Pranoto, Y., Anggrahini, S., and Efendi, Z. (2013). Effect of natural and Lactobacillus plantarum fermentation on in-vitro protein and starch digestibilities of sorghum flour. Food Biosci. 2, 46–52. doi: 10.1016/j.fbio.2013.04.001
Rader, J. I., Weaver, C. M., and Angyal, G. (1998). Use of a microbial assay with tri-enzyme extraction for measurement of pre-fortification levels of folates in enriched cereal grain products. Food Chem. 62, 451–465. doi: 10.1016/S0308-8146(98)00089-2
Rao, P. P., and Basavaraj, G. (2015). Status and Prospects of Millet Utilization in India and Global Scenario (pp-197-209). Hyderabad: ICAR-Indian Institute of Millets Research.
Rao, P. P., Birthal, P. S., Reddy, B. V., Rai, K. N., and Ramesh, S. (2006). Diagnostics of sorghum and pearl millet grains-based nutrition in India. Int. Sorghum Millets Newslett. 47, 93–96.
Reale, A., Konietzny, U., Coppola, R., Sorrentino, E., and Greiner, R. (2007). The importance of lactic acid bacteria for phytate degradation during cereal dough fermentation. J. Agric. Food Chem. 55, 2993–2997. doi: 10.1021/jf063507n
Sade, F. O. (2009). Proximate, antinutritional factors and functional properties of processed pearl millet (Pennisetum glaucum). J. Food Technol. 7, 92–97.
Saleh, A. S. M., Zhang, Q., Chen, J., and Shen, Q. (2013). Millet grains: nutritional quality, processing, and potential health benefits. Compreh. Rev. Food Sci. Food Saf. 12, 281–295. doi: 10.1111/1541-4337.12012
Shobana, S., Krishnaswamy, K., Sudha, V., Malleshi, N. G., Anjana, R. M., Palaniappan, L., et al. (2013). Finger millet (Ragi, Eleusine coracana L.): a review of its nutritional properties, processing, and plausible health benefits. Adv. Food Nutr. Res. 69, 1–39. doi: 10.1016/B978-0-12-410540-9.00001-6
Sihag, M. K., Sharma, V., Goyal, A., Arora, S., and Singh, A. K. (2015). Effect of domestic processing treatments on iron, β-carotene, phytic acid and polyphenols of pearl millet. Cogent Food Agric. 1, 1–12. doi: 10.1080/23311932.2015.1109171
Swaminathan, S., Hemalatha, R., Pandey, A., Kassebaum, N. J., Laxmaiah, A., Longvah, T., et al. (2019). The burden of child and maternal malnutrition and trends in its indicators in the states of India: the Global Burden of Disease Study 1990-2017. Lancet Child Adolesc. Health 3, 855–870. doi: 10.1016/S2352-4642(19)30273-1
Thompson, L. U. (1993). Potential health benefits and problems associated with antinutrients in foods. Food Res. Int. 26, 131–149. doi: 10.1016/0963-9969(93)90069-U
Torre, M., Rodriguez, A. R., and Saura-Calixto, F. (1991). Effects of dietary fiber and phytic acid on mineral availability. Crit. Rev. Food Sci. Nutr. 30, 1–22. doi: 10.1080/10408399109527539
Towo, E., Matuschek, E., and Svanberg, U. (2006). Fermentation and enzyme treatment of tannin sorghum gruels: effects on phenolic compounds, phytate and in vitro accessible iron. Food Chem. 94, 369–376. doi: 10.1016/j.foodchem.2004.11.027
USDA (2007). Table of Nutrient Retention Factors, Release 6, Beltsville Human Nutrition Research Center (BHNRC), Agricultural Research Service (ARS), U.S. Department of Agriculture.
Valls, F., Sancho, M. T., Fernandez-Muino, M. A., and Checa, M. A. (2001). Determination of vitamin B6 in cooked sausages. J. Agric. Food Chem. 49, 38–41. doi: 10.1021/jf0003202
Vásquez-Caicedo, A. L., Bell, S., and Hartmann, B. (2008). Report on Collection of Rules on Use of Recipe Calculation Procedures Including the Use of Yield and Retention Factors for Imputing Nutrient Values for Composite Foods. Brussels: European Food Information Resource Network.
Vats, P., and Banerjee, U. C. (2004). Production studies and catalytic properties of phytases (myo-inositolhexakis phosphate phosphohydrolases): an overview. Enzyme Microb. Technol. 35, 3–14. doi: 10.1016/j.enzmictec.2004.03.010
Wallace, M., and Singh, S. K. (2019). Assessments of Commodity and Trade Issues by USDA. Washington, DC: USDA Foreign Agricultural Service. 11p.
Wedad, H. A., El Tinay, A. H., Mustafa, A. I., and Babiker, E. E. (2008). Effect of fermentation, malt-pretreatment and cooking on antinutritional factors and protein digestibility of sorghum cultivars. Pakistan J. Nutr. 7, 335–341. doi: 10.3923/pjn.2008.335.341
Keywords: millets, traditional cooking, fermentation, antinutrients, nutrition retention
Citation: Sheethal HV, Chandrama Baruah, Kalpuri Subhash, Rajendran Ananthan and Longvah T (2022) Insights of Nutritional and Anti-nutritional Retention in Traditionally Processed Millets. Front. Sustain. Food Syst. 5:735356. doi: 10.3389/fsufs.2021.735356
Received: 02 July 2021; Accepted: 16 December 2021;
Published: 01 February 2022.
Edited by:
Ramadjita Tabo, International Crops Research Institute for the Semi-Arid Tropics, MaliReviewed by:
Alexandru Rusu, Biozoon Food Innovations GmbH, GermanyC. K. Sunil, Indian Institute of Food Processing Technology, India
Copyright © 2022 Sheethal, Baruah, Subhash, Ananthan and Longvah. This is an open-access article distributed under the terms of the Creative Commons Attribution License (CC BY). The use, distribution or reproduction in other forums is permitted, provided the original author(s) and the copyright owner(s) are credited and that the original publication in this journal is cited, in accordance with accepted academic practice. No use, distribution or reproduction is permitted which does not comply with these terms.
*Correspondence: Rajendran Ananthan, YW5hbnRoYW4ubmluQGdtYWlsLmNvbQ==