- 1Department of Botany, Institute of Science, Banaras Hindu University, Varanasi, India
- 2Department of Mycology and Plant Pathology, Institute of Agricultural Sciences, Banaras Hindu University, Varanasi, India
Environmental stressors such as salinity, drought, high temperature, high rainfall, etc. have already demonstrated the negative impacts on plant growth and development and thereby limiting productivity of the crops. Therefore, in the time to come, more sustainable efforts are required in agricultural practices to ensure food production and security under such adverse environmental conditions. A most promising and eco-friendly way to achieve this goal would be to apply biostimulants to address the environmental concerns. Non-microbial biostimulants such as humic substances (HA), protein hydrolysate, plant-based products and seaweed extracts (SWE), etc. and/or microbial inoculants comprising of plant growth-promoting microbes such as arbuscular mycorrhizal fungi (AMF), fluorescent and non-fluorescent Pseudomonas, Trichoderma spp., Bacillus spp. etc. have tremendous potentiality to enhance plant growth, flowering, crop productivity, nutrient use efficiency (NUE) and translocation, as well as enhancing tolerance to a wide range of abiotic stresses by modifying physiological, biological and biochemical processes of the crop-plants. Similarly, application techniques and timing are also important to achieve the desired results. In this article we discussed the prospects of using seaweed, microbial, and plant-based biostimulants either individually or in combination for managing environmental stresses to achieve food security in a sustainable way. Particular attention was given to the modifications that take place in plant's physiology under adverse environmental conditions and how different biostimulants re-program the host's physiology to withstand such stresses. Additionally, we also discussed how application of biostimulants can overcome the issue of nutrient deficiency in agricultural lands and improve their use efficiency by crop plants.
Introduction
Biostimulants are natural or synthetic products derived from plants and/or microbes, and have the potential to promote the physiological processes of plants benefitting nutrient uptake and translocation, as well as enhanced tolerance to several abiotic stresses. These products can be directly applied to seeds, seedlings and plants or can be introduced into the rhizosphere of crops with the goal to improve plant productivity and growth in a sustainable way under environmental stresses. Plants continuously face stressful events such as drought, salinity, extreme temperature, high rainfall, etc., at every stage of their growth and development starting from seed germination to completion of the entire life cycle. The abiotic stress factors may alter the plant's physiological processes and/or thereby increase their susceptibility to pests and pathogens and serve as a limiting factor for plant's survival, productivity and growth (West et al., 2012; Maharshi et al., 2021). A diverse agro-chemical and traditional approaches are being adopted to mitigate the adverse environmental effects but one of the most promising and eco-friendly ways recognized to achieve this goal is to use biostimulants to address such concerns (Yakhin et al., 2017). It is because of the fact that in most cases it was observed that application of biostimulants can diminish the demand of synthetic fertilizers and pesticides as the biostimulants have the potentiality to enhance the crop's nutrient use efficiency (NUE) for both micro- and macro-nutrients (Calvo et al., 2014; Van Oosten et al., 2017). However, the plant biostimulants (PBs) are not a direct substitute of fertilizers and pesticides. But their application can very well reduce the need for fertilizers and pesticides and thereby reduce their potential impact on environmental hazards in a crop production system. In earlier articles, biostimulants are discussed mostly in the context of plant-based products that have potentiality to stimulate plant growth under various stresses. However, in majority of the articles the microbe-based biostimulants were not discussed or compared with the plant-based biostimulants. Therefore, the current article presents a holistic insight into the various plant- and microbial-biostimulants, their uses under different environmental conditions and a mechanistic view of the biostimulants.
Definition
Many definitions of phyto- and bio-stimulants are available in scientific literatures. According to Zhang and Schmidt (2000) biostimulants are the materials used in trace amounts to promote plant growth. The authors used the words “tracer amount” for biostimulants to distinguish the biostimulants from other nutrients, which also promote plant growth when applied in larger amounts. Later on Kauffman et al. (2007) defined biostimulants with little modifications and according to them “biostimulants” are materials, other than fertilizers, that promote plant growth when applied in low quantities. However, biostimulants are still a “moving target” in the European Union (EU). From the industrial perspective, the definition of biostimulants as given by the European Biostimulants Industry Council (EBIC) in 2012 is “plant biostimulants contain substance and/or micro-organisms whose function when applied to plants or the rhizosphere is to stimulate the natural processes to enhance/benefit nutrient uptake, nutrient efficiency, tolerance to abiotic stresses, and crop quality, with no direct action against the pests” (www.biostimulants.eu). The concept of PBs has continuously been investigated since 1933 but has received considerable focus only in the last two and half decades as a potential mixture to alleviate the negative effects of climate change (Craigie, 2011; Sharma et al., 2014; Ricci et al., 2019; Jindo et al., 2020). Based on the previous considerations the Environmental Protection Agency (EPA) in 2019 defined biostimulants as “naturally-occurring substance or microbe that is used either by itself or in combination with other naturally-occurring substances or microbes for the purpose of stimulating natural processes in plants or in the soil in order to improve nutrient and/ or water use efficiency by plants, help plants tolerate abiotic stress, or improve the physical, chemical, and/ or biological characteristics of the soil as a medium for plant growth.” Since biostimulants are derived from diversity of sources like plants, humic substances, protein hydrolysate, seaweed extracts, macro- and micro-alga, living microbial cultures and plant growth-promoting microbes (PGPM), etc., these compounds are therefore poorly characterized and their mode of actions are also undefined at cellular and molecular levels. Thus, due to the current knowledge gaps the concepts of PBs are still evolving (Calvo et al., 2014; Li et al., 2021), which is somewhat indicative of the diversity of inputs that can be accepted as PBs.
Flurry of researches were conducted in order to evaluate the PBs for improving growth and development of seedlings and subsequently the plants after subjecting to several abiotic stresses like drought, saline environment, extreme temperature, heavy rainfall, etc. (Carillo et al., 2019). Additionally, different kinds of raw materials were also used along with non-microbial biostimulant components such as humic-substances, protein hydrolysate, plant-based products, seaweed extracts, etc. and/or microbial components comprising of PGPMs such as arbuscular mycorrhizal fungi, fluorescent Pseudomonas, Trichoderma spp., Bacillus spp. etc. They all have shown tremendous potentiality to enhance plant growth, flowering, crop productivity, nutrient use efficiency (NUE) and translocation, as well as enhancing tolerance to a wide range of abiotic stresses by modifying physiological, biological and biochemical processes of the crop-plants.
Types of Biostimulants and Their Significance
Abiotic stresses like drought, salinity, extreme temperature, high rainfall, etc. are becoming most important threats to food security resulting from constant changes in climate. To cope with these stresses, plants respond by changing several molecular and physiological processes or initiating a number of defense responses to adapt such stresses. It has been highlighted in several studies that application of biostimulants even at very low quantities can promote plant's performance by re-programming the natural processes of plants under such harmful environments (Huang et al., 2013; de Vasconcelos and Chaves, 2019). Some major categories of biostimulants that are currently recognized by scientists are asserted to stimulate the plant defense responses and provide stress tolerance capabilities to them by both non-microbial and microbial means (Halpern et al., 2015; Gupta et al., 2021a). A graphical representation is presented in Figure 1 for these biostimulants according to their classes.
Non-microbial Biostimulants
The non-microbial biostimulants include beneficial plant botanicals or natural plant biostimulants seaweed extracts and their raw materials like protein hydrolysates, amino acids containing products, humic substances, etc. can improve crop productivity even under environmental stress (Pichyangkura and Chadchawan, 2015; Drobek et al., 2020; Rouphael et al., 2020b; Del Buono, 2021).
Seaweed or Algal Extracts
Seaweeds are diverse group of multicellular macroalgae mostly found in marine water. Most of the algal species belong to the members of Rhodophyta, Phaeophyta, Chlorophyta and Charophyta (Carillo et al., 2020). In ancient agricultural practices, seaweeds were used as sources of organic matter and fertilizer nutrients, but their role as biostimulants was not recognized. The effect of seaweeds extracts as biostimulants has recently been reported by many researchers, which promotes the commercialization of seaweeds and their purified compounds such as different carbohydrates including alginates, fucoidan, carrageenans and some other plant hormones that are significantly associated with plant growth (Battacharyya et al., 2015). Additionally, they also contain plant hormones such as auxins and cytokinins or other hormone-like substances such as sterols and polyamines (Craigie, 2011). Majority of the algal extracts are formulations obtained from brown algae including Ascophyllum nodosum and some other genera such as Fucus, Laminaria spp., etc. (Khan et al., 2009; Sharma et al., 2014). Since microalgae are rich source of metabolites and growth-promoting hormones they can be very well utilized in agriculture in various ways such as soil conditioners and/or plant growth biostimulators (Bulgari et al., 2019; Behera et al., 2021). It was reported that seed germination and seedling growth of lettuce plants were promoted when the seeds were primed with A. nodosum extracts under elevated temperature conditions. These bio-active extracts when sprayed to the plants were also able to enhance the plant's performance under different abiotic stresses, and thereby compensated the possible yield loss and maintained productivity of several crops (Battacharyya et al., 2015).
Humic Substances
Humic substances are the category of naturally occurring constituents of soil organic matter that can be formed by decay and transformation of plants debris, animals, and microbial residues in the environment by microbial activities (Correia et al., 2019). Humic substances are characterized into humins, humic acids (HA) as well as fulvic acids, according to their solubility in water and molecular weights (Bai et al., 2015). These substances can be directly applied onto soil, and can act on fertility of the soil by altering their physical, chemical, biological and physicochemical properties. In several studies, it was observed that application of HA on crop plants can stimulate growth and development of the plants and increase the biomass, but their effect on the host physiology is not fully understood yet (Rose et al., 2014). Humic substances are known to be helpful for uptaking of nutrients and water by forming soluble complexes along with the nutrients. In this way, they help in reducing nutrient leaching and making them more easily available for the plants (Chen et al., 2004). Since humic substances improve the physical properties of soil, they form an enzymatically active component in the rhizosphere that alters the activity of microorganisms and increase the population of rhizosphere microbiome often correlated with enhancing growth of plants (Visser, 1985). Humic substances also help plants to tolerate abiotic stresses. Several earlier studies showed that HA isolated from vermicompost or other similar organic sources, when applied benefited the crop plants by reducing soil sodicity and elevating micro-and macro-nutrients such as N, Fe, Mg, P, S, K, Ca, Cu and Mn in plant parts (Çimrin et al., 2010). Further, HA also helps plants in stress protection by stimulating the biosynthesis of antioxidative and ROS scavenging enzymes (Garcia et al., 2012).
Protein Hydrolysates
Protein hydrolysates are mixtures of proteinaceous and non-proteinaceous compounds including amino acids, peptides and polypeptides. Such mixtures generally arise from the chemical as well as enzymatic hydrolysis of plant and animal raw materials (Colla et al., 2017). The plant-based protein hydrolysates are generally produced from vegetable or fruit wastes, pulses, etc., while animal-based biostimulants are obtained from raw materials of bird feathers, milk casein, skin collagen fibers, animal tissues, fish wastes, etc. (Colla et al., 2015; Scaglia et al., 2017). Several earlier studies confirmed that commercial products of protein hydrolysates from animal origin have negative impacts on plant growth compared to the plant-based protein hydrolysate (Cerdan et al., 2008). For examples, animal-based protein hydrolysates contain high amount of thermostable amino acids like glycine, alanine, and proline including hydroxyproline and hydroxylysine, but their high concentrations may inhibit root growth, nutrient use efficiency and thus negatively affect plant growth and yield (Trovato et al., 2018). Similarly, Rouphael et al. (2021) reported that the application of above 0.05 g N/kg concentration of animal-based protein hydrolysates on basil plants caused a decrease in the number and area of leaves, plant photosynthetic rate, and biomass production. But in some cases application of animal-based protein hydrolysates in higher concentration was found to counter the negative impact of toxic ions as well. However, Botta (2012) examined that use of animal-based hydrolysates increased biomass of shoots in comparison to control plants. Whereas, Trevisan et al. (2019) observed that when a plant-based protein hydrolysates (APR®, ILSA, S.p.A.,Arzigano VI, Itali) was used in the hydroponically grown maize plants, the protein hydrolysates stimulated plant growth and defense responses against several abiotic stresses and the approach was hailed for its eco-friendly manner (Ugolini et al., 2015). Further, Lucini et al. (2015) observed in a metabolomic profiling of lettuce that applications of protein hydrolysate lightened the saline stress by differentially regulating the defense-related genes and metabolites like terpenes, carbohydrates, amino acids and sterols. The hormonal, chelating and antioxidant activities were also observed in several other studies. For example, Colla et al. (2017) published that hormonal activities in complex protein, amino acids and tissue hydrolysates, prolines, etc. with chelating properties protected the plants from several heavy metals and ion toxicities. Some of the nitrogenous compounds like amino acids viz., glycine, betaine and proline also have antioxidant properties, which protect the plants from abiotic stresses by acting as a scavenger of free radicals. Protein hydrolysates are well studied to help in microbial growth and activity in rhizosphere zones and thereby improve overall fertility of soils (Bulgari et al., 2019).
Microbial Biostimulants
Microbial biostimulants (MBs) include some beneficial bacteria, mainly PGPRs such as Azotobacter, Azospirillum, Rhizobium, fluorescent Pseudomonads, etc., arbuscular mycorrhizal fungi, and some other important fungi like Trichoderma, Piriformospora sp., etc. (Ruzzi and Aroca, 2015; Rouphael et al., 2020b). The list of some categorized MBs and their specific functions are mentioned in Table 1.
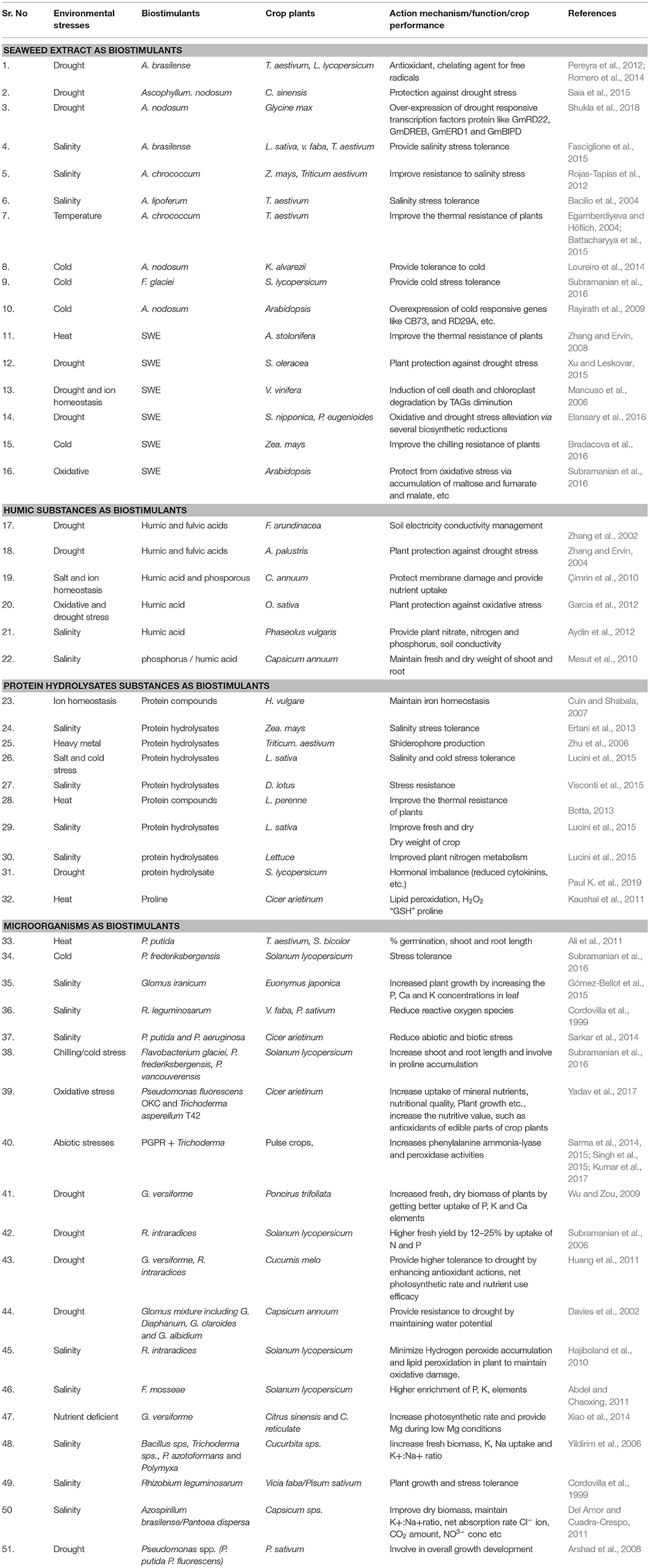
Table 1. The functional and mechanistic roles of several type of biostimulants in crops performances under different abiotic stress condition.
Plant Growth-Promoting Rhizobacteria as Biostimulants
Plant growth-promoting rhizobacteria (PGPR) are root-associated plant-growth promoting bacteria that form symbiotic associations with many crop plants. Phytohormones produced by PGPR effectively participate in the regulation of plant growth. Based on their agricultural output, they are utilized for different purposes such as bio-fertilizers, bio-pesticides and bio-control agents as well as phyto-stimulants (Bhattacharyya and Jha, 2012). PGPR-based biostimulants are considerably used as an effective agroecological technique for stimulating plant growth and enhancing nutrient use efficiency in the crop plants and they also provide resistance to the host plants against a variety of abiotic stresses, from perception to the activation of cellular and molecular events (Le Mire et al., 2016; Lephatsi et al., 2021). PGPR comprises several genera including Arthrobacter, Enterobacter, Azotobacter, Azospirillum, Acinetobacter, Pseudomonas, Streptomyces, Ochrobactrum, Bacillus, Rhodococcus, Rhizobium, Bradyrhizobium, etc. and have the potential to act both as phyto- and bio-stimulants (Zhao et al., 2018). Some of them, mainly Pseudomonas, Rhizophagus, and Bacillus species are commercially exploited in a significant way as soil activators with capability to endorse plant growth (Radhakrishnan et al., 2017). Applications of PGPR as bio-stimulants in agricultural practices have been widely reviewed in several scientific articles as well as in textbooks. Many authors have reported that inoculation of Bacillus, Brevibacillus, and Rhizobium spp. in broccoli roots (Brassica oleracea) increased the photosynthetic rate by reducing chlorophyll degradation or stimulation of its synthesis and thereby increased the overall yield of crops (Khan et al., 2009; Yildirim et al., 2011). Similar results were also observed in tomatoes treated with Azotobacter, Bacillus and Pseudomonas and also in Fragaria ananassa following treatment with different groups of PGPR (Karlidag et al., 2013). PGPR are also able to improve plant's responses against the environmental stresses by stimulating biological and physio-chemical activities of the plants (Ruzzi and Aroca, 2015). Kumar et al. (2019) reported that application of Pseudomonas fluorescens OKC not only protected the pigeonpea plants from salinity stress but also protected from the wilt pathogen Fusarium udum. Similarly, Vaishnav et al. (2020) demonstrated that Sphingobacterium sp. BHU-AV3 can provide tolerance to salinity in tomato plants by inducing the antioxidant activities and metabolism pathways. Other salt-tolerant PGPRs such as Serratia spp., Rhizobium spp., and Azospirillum, etc. are also found to rapidly influence the growth and yield of lettuce and wheat grown in saline soils (Fasciglione et al., 2015; Upadhyay and Singh, 2015). Similar results were also observed in pea (Pisum sativum) crop following treatment with Pseudomonas sp. under drought stress condition by activation of photosynthetic rate and antioxidant properties (Heidari and Golpayegani, 2012).
Arbuscular Mycorrhizal Fungi as Biostimulants
Arbuscular Mycorrhizal Fungi (AMF) are widely spread endo-mycorrhizal fungi having association with the plants established through penetration by the fungal hyphae into the root cortical cells of the host plants (Behie and Bidochka, 2014). This mycorhizal association with plants are beneficial for both the organisms, because the fungi absorbs foods from the host plants and in return helps the plant in increasing their root growth and surface area for absorption of nutrients and water. In addition, they can mediate the hormonal steadiness of the crop plants, and thereby stimulate plant growth and impart tolerance against different stresses. Based on these properties the AMFs are also categorized as biostimulants (Rouphael et al., 2020b). Among the microbial biostimulants, AMFs are crucially involved in supporting the host plant nutrition by increasing the absorption of mineral nutrients beyond the rhizospheric zones of the crop plants. Most predominantly used mycorrhizal biostimulants are Rhizophagus spp., Septoglycus viscosum, Claroideoglomus etunicatum, Claroideoglomus claroideum, etc. in the sustainable agriculture production system (Du Jardin, 2015; Zardak et al., 2018). AMF can improve the crop productivity under nutrient deficient conditions by producing low molecular weight molecules including siderophores, which chelates iron ions, as well as secrete enzymes such as phosphatases that hydrolyse phosphates from organic P compounds (Smith et al., 2011). It has also been observed that application of AMF viz., Scutellospora spp., Acaulospora spp., Gigaspora spp., Glomus aggregatum, Rhizophagus irregulare (syn. G. intraradices), Funneliformis mosseae (syn. G. mosseae), G. etunicatum, G. fasciculatum, and G. deserticola along with some other beneficial microbes in the soil helps in nitrate transport as well (Saia et al., 2015). Sbrana et al. (2014) reviewed that symbiotic association of AMF could lead to changes in the biosynthesis of secondary metabolites in plants including important phyto-chemicals and also improve nutritionally significant compounds.
In addition to the mentioned properties, AMF-based biostimulants also provide tolerance to salinity stress and drought stress by increasing seed germination, growth parameters of shoots and roots, productivity, plant quality and yields (Jayne and Quigley, 2014). Similarly, Feng et al. (2002) showed that the colonization of AMF could increase the content of soluble sugars in salt-treated seedlings of maize, which indicates that these plants have a better osmotic adjustment capacity.
Other Important Beneficial Microbes and Their Consortia as Biostimulants
Bio-priming is one of the most potential tools in the sustainable crop production system without affecting soil health. It is a biological process of seeds/ seedling treatment with beneficial microorganisms to protect seeds and treated crops by stimulating induced systemic resistance to abiotic as well as biotic stresses (Meena et al., 2017; Patel et al., 2017; de Vries et al., 2020). There are important endophytic microbes including some bacteria and fungi found to be involved in stimulating defense responses in plants and at the same time they are also effective directly against phytophagous insects and herbivory damage. For example, Piriformospora indica is capable of forming symbiotic relationship with several important crops and shown to increase their yield and defense against many soil-borne pathogens (Johri et al., 2015). Similarly, Mohd et al. (2017) reported that P. indica effectively mediate protection against arsenic toxicity in rice field following colonization of the paddy roots. In particular, the beneficial microbes comprising of both bacteria and fungi such as Trichoderma spp., Pythium oligandrum, Talaromyces flavus, Glomus spp., Aureobasidium pullulans, Bacillus, Gliocladium, Pseudomonas, and Azospirillum are being used as biopriming agents on seeds that helped in improving seed viability, resisting various kinds of stresses and crop yield. These microbes are defined as “biostimulant microorganisms,” because they can boost plant growth and defense responses from seed germination to the maturity stage (Paul S. et al., 2019). Among different beneficial microorganisms, Trichoderma spp. (including T. harzianum and T. viride) are the most abundantly reported fungi, and has been widely used as a source of different commercial biostimulants and biofungicides (Mahanty et al., 2017). Sureshrao et al. (2016) showed that rice seedlings treated with T. viride had more lateral roots and better seed germination compared to the control plants. Similar outputs were also recorded by Lalitha and Arunalakshmi (2012) in mustard crops. To promote plant growth Trichoderma enhanced phytohormone production in the host plants as well as by secreting hormone-like compounds synthesized in their hyphae (Frac et al., 2018). These phytohormones help the plants to acclimatize with adverse situations by improving growth, and defense responses against a variety of stresses.
It was recently observed that combined plant root colonization by Trichoderma along with mycorrhiza such as Glomus, enhanced plant's growth in terms of increase in the total biomass by about 20 to 30 times compared to the plants where they were applied individually (Sofo et al., 2012). Similarly, Rubin et al. (2017) also suggested that PGPR may also increase root biomass more effectively when applied in combination with other microbes. So, co-inoculation of bacteria and fungi with other beneficial microbes has become the most promising tool for sustainable crop production with few contradictions. A recent microbial biostimulant study have confirmed that the combined application of Bacillus spp., Glomus spp., and Trichoderma spp. not only protected the plants more effectively against a broad range of pathogenic microbes, but also provided protection to the plants under adverse environmental conditions by stimulating the defense mechanisms such as systemic acquired resistance (SAR), and induced systematic resistance (ISR) (Kaewchai, 2009; Colla et al., 2015).
Seed priming and watering of Cucurbita pepo with different organisms including Bacillus pumilis, Paenibacillus azotoformans, Trichoderma harzianum, and Polymyxa were observed helpful in gaining fresh weight, increasing the potassium content and balance the K+:Na+ ratio under salinity stressed condition (Yildirim et al., 2006). It was also reported that the consortia of beneficial microbes including Glomus intraradices, Trichoderma reesei, Trichoderma atroviride, and Heteroconium chaetospira positively affected crop yield and quality (Du Jardin, 2015). Particular attention should be given to plant growth-promoting bacteria (Bacillus spp. and Pseudomonas spp.), endomycorrhizal fungi (Glomus spp.) and rhizospheric colonizer (Trichoderma spp.), that provides long term safety to the cultivated crops against pathogens and help plants for increasing nutrient uptake and use efficiency to gain optimum yields. Nanoparticles and nanomaterials-based biostimulants are considered to be a novel category of biostimulants and recently been reported by Juárez-Maldonado et al. (2019). Similarly, green synthesized silver nanoparticles (AgNPs) by naturally occurring bio-active components is also considered to be a novel category of biostimulants (Tripathi and Pandey-Rai, 2021). These nanomaterial products, when applied as foliar spray on the crop plants even in small quantities, provide tolerance to the abiotic stresses and improve the quantity and quality of the produces. For example, the application of ZnO2 nanoparticles on tomatoes by aerial or soil application increased the number of leaves, plant height, growth, as well as chlorophyll and protein contents (Raliya et al., 2015). Their phyto- or bio-stimulant properties seem to be coupled with particle's structure and nature, and not to their chemical compositions.
Mechanisms of Biostimulants-Mediated Crop Tolerance to Abiotic Stresses
Abiotic stresses, including drought, high rainfall, salinity, extreme cold or heat, etc. are responsible for about 50–70% of yield loss by affecting the plant productivity (Carillo et al., 2019). To overcome the harmful effects from the abiotic stresses, PBs have been used as an efficient agricultural tool for stress tolerance. However, the activities of PBs depend on several factors, such as application techniques, targeted crop as well as timing of inoculation (Bulgari et al., 2019). These abiotic stresses cause several physiological and biological disorders including membrane disfunctioning, reduction of photosynthesis and protein synthesis rate, hormonal imbalance, etc. Most detrimental stress of salinity is ionic imbalances and hyperosmotic condition, which caused by excess production of reactive oxygen species (ROS) and the anti-oxidant defense mechanisms (Di Mola et al., 2020). These ROS like oxygen molecule (O2), superoxide (), hydrogen peroxide (H2O2), hydroxyl radical (HO−) are strong oxidizing and reducible ROS, that generated in plant cells to maintain the homeostasis and stability of the stem cells (Das and Roychoudhury, 2014; Zeng et al., 2017). However, an excess level of ROS is reason for oxidative damage of the cells that cause cell death of the crop plants (Al-Ghamdi and Elansary, 2018). And the drought stress condition may lead to low water potentiality due to high solute concentration, which makes the plant cells to become turgid resulting in wilting and desiccation in plants. Scarcity of water may also cause soil mineral toxicities and can make plants more susceptible to damage from high temperature and high irradiance. Similarly, high temperature affects metabolism, respiration, and other physiological processes of the crops causing retardation in functioning and growth of the plants. This type of heat injury may lead to death of whole plants. Cold temperature stress is also one of the most limiting factors for the crops. Like other abiotic stresses, it can also cause several metabolic and physiological dysfunctioning of the crop such as degradation of chlorophyll, plasmolysis, amylolysis (conversion of starch to sugar), photosynthetic reduction, etc. Waterlogging is another environmental stress that leads to saturation of the soil with water. The major cause of waterlogging is heavy rainfall. Excess of water replace the air from soil pores, causing deficiency of oxygen and accumulation of carbon dioxide that hampers the growth of roots and plants. Therefore, these environmental stresses affect the plants in several ways and ultimately may cause threat to food security throughout the globe (Rouphael et al., 2018a).
To cope up with these problems plants activate several defense mechanisms against different abiotic stresses and PBs can play a significant role in triggering plant's metabolic activities and stimulating performance under such adverse conditions (Rouphael et al., 2018b). There are various microbial (PGPR, AMF and other beneficial microbes) and nonmicrobial (seaweed extracts, protein hydrolysates and humic substances) biostimulants reported that take part in activation and regulation of several types of defense mechanisms by various modes of actions to promote plant growth. They also play a major role s in abiotic stresses by altering the plant's responses via different physiological and biological mechanisms including ROS scavenging activity, osmoprotection, membrane stability, stomatal guiding and xylem conductance, metal chelation, nutrient and water availability and phytohormonal signaling in plants (Van Oosten et al., 2017). The functional and mechanistic roles of different types of biostimulants in crop performance under stress conditions are given in Table 1. Different techniques for inoculating biostimulants are available. Some of those include seed treatment, seedling dip method, and foliar spray or soil application via soil drenching, incorporation of soil marine products and addition of extracts to hydroponics. Mechanisms of actions of these biostimulants vary according to the nature and characteristics of the biostimulants. It has been investigated that the protein-based biostimulants directly penetrate plant tissues of the applied area and enter into the cells, while penetration of the protein hydrolysates in a plant cell takes place through the diffusion process through membrane openings/pores. It is an energy-dependent process (Kolomazník et al., 2012). Other biostimulants like microbial inoculants interact with the plants by penetration of the hyphae into the plant tissues and making the symbiotic or mycorrhizal associations. When these biostimulants enter into the treated leaves and root their translocation starts toward the most distant part of the plants (Pecha et al., 2012). However, for understanding the mode of action, it is necessary to first identify the biologically active compounds (Henda and Bordenave-Juchereau, 2013). Inside the host plants these biostimulants helps in activation of stress-responsive genes by the signal transduction and transcriptional regulations. Schematic representations of physiological effects by different biostimulants under adverse conditions are shown diagrammatically in Figure 2.
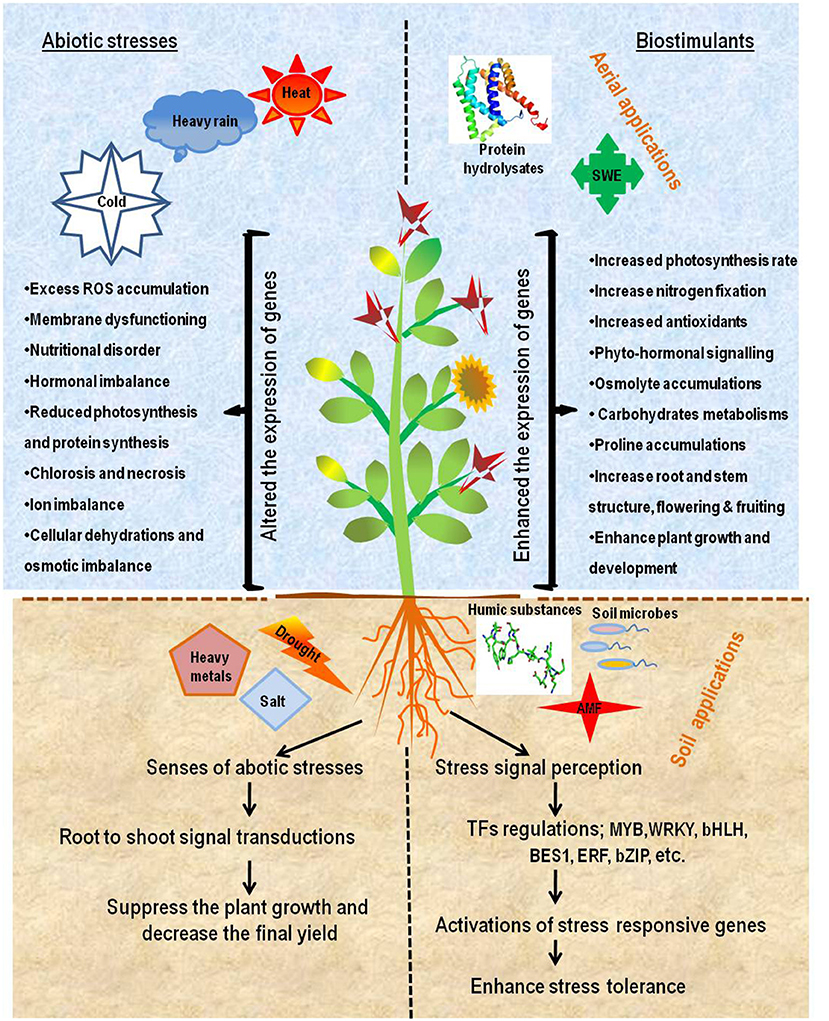
Figure 2. Schematic representation of effects caused by abiotic stresses and biostimulants-mediated abiotic stresses tolerance in plants. The right side of the diagram represents plant damage due to a variety of abiotic stresses including cold, heat, heavy rain, salinity, etc. which eventually leads to yield losses. The left side of diagram represents foliar and soil application of biostimulants including, seaweed extracts (SWE), protein hydrolysates and humic substances, soil microbes, arbuscular mycorrhizal fungi (AMF), respectively, which drives many mechanisms for plant growth and development, regulates multiple transcription factors (TFs) and activates stress responsive genes, leading to enhanced stress tolerance.
Among the seaweed extracts used as biostimulants, Ascophyllum nodosum extract (ANE) has been used extensively to impart tolerance to plants from number of biotic as well as abiotic stresses. It has been previously studied that foliar spray of ANE regulates the genes expression of photosynthesis and ROS scavenging activities under stressed conditions. The mechanism of ROS scavenging involves both enzymatic antioxidants including Superoxide dismutase (SOD), ascorbate peroxidise (APX), glutathione peroxidase (GPX), and catalase (CAT) as well as nonenzymatic antioxidants such as ascorbates, glutathione, proline, glycine betain, tochopherol (Miller et al., 2010; Elansary et al., 2017; Sharma et al., 2019). Further, the accumulation of MDA (malondialdehyde) content in plant cells/tissues is considered as an important indicator for drought-induced peroxidative damage (Shukla et al., 2011). It was also reported by Carvalho et al. (2018), that ANE applications in bean (Phaseolus vulgaris) reduces the ROS-induced MDA production by improving CAT activity and thereby making the plants more tolerant to drought. Similarly, soil drenching with ANE in S. nipponica and P. eugenioides growing soils mitigated drought effects by overproduction of antioxidants and higher lipid peroxidation, thereby reducing the ROS content and particular stresses (Elansary et al., 2016) (Figure 3).
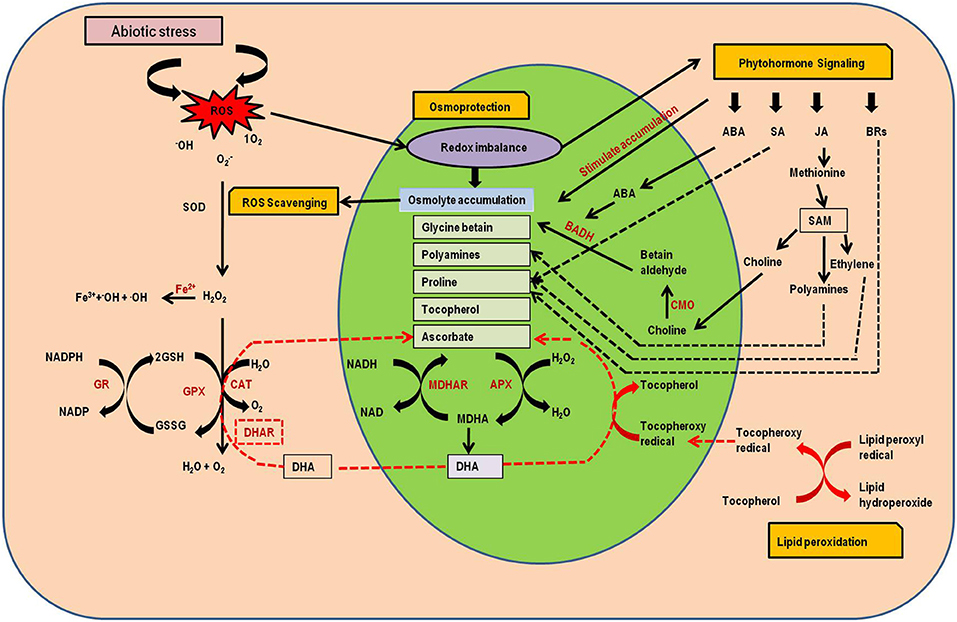
Figure 3. Schematic representation of antioxidant defense mechanisms against several abiotic stresses in plant. A series of redox coupled reactions with some important signaling pathways including osmoprotection by osmolyte accumulation, ROS scavenging, lipid peroxidation and phytohormonal signaling pathways are presented. BADH, betain aldehyde dehydrogenase; CMO, cholin monooxygenase; SAM, S-adenosylmethionine; GSSG, glutathione disulphide; CAT, catalase; GSH, glutathione; GR, glutathione reductase; DHAR, dehydroascorbate reductase; MDHAR, monodehydroascorbate reductase.
The bioactive constituents isolated from A. nodosum extracts were found to regulate various steps of post-transcriptional and post-translational regulation of different TFs family proteins, such as AP2/ERF, NF-YA, WRKY, COR15A, MYB, LHY1 and some others which provide stress tolerance to crops (Goni et al., 2018; Jithesh et al., 2019). Shukla et al. (2018) reported that binding of AtGRF7 to the promoter element of AtDREB2a down-regulates its expression, which leads to salinity tolerance. It was reported in Arabidopsis that, plants treated with ANE lowered the levels of AtGRF7-led overexpression of AtDREB2a and AtRD29 genes and thereby provided better salinity tolerance (Shukla et al., 2018). ANE is also involved in regulation of cold or freezing stress tolerance by inducing the expression of cold-responsive genes such as COR15A, CBF3 and RD29A (Rayirath et al., 2009). Further, transcriptome studies by Nair et al. (2012) revealed that the lipophilic components of ANE regulate the expression of more than thousands of genes in response to freezing stress. They also concluded that seaweed extract components have the tendency to positively regulate P5CS1 and P5CS2 genes involved in proline biosynthesis and negatively regulate the expression of proline catabolic genes. The accumulation of proline inside the plant increases the ability to tolerate different types of environmental stresses.
Protein hydrolysates and humic substances also play crucial roles in stress tolerance. They involve in activation of antioxidant enzymes and free radicals having ROS scavenging properties and chelating ionic compounds and metals (Abd El-Mageed et al., 2017). The exogenous application of proline and betaine to Hordeum vulgare reduces the NaCl-induced efflux of potassium ions by relatively low concentrations of compatible solutes (Cuin and Shabala, 2005). Garcia et al. (2012) reported that extracts isolated from vermicompost when used for treatment of rice (Oryza sativa L.) plants it differentially regulated H+ ATPases located on the plasma membranes and provided tolerance to salinity and drought stresses. Similarly, when tomato (Solanum lycopersicum) plants were treated with vermicompost it has led to more than 40% of proton extrusion by facilitating the potential of acid growth, hormonal regulations and nutrient uptake (Zandonadi et al., 2016). Similarly, microbial biostimulants comprises of various kinds of beneficial endophytic, symbiotic and ectoparasitic microbes. These microbes show positive interactions with the plants or colonize in the rhizosphere and stimulate several growth responsive genes. They also involve significantly in activation of several defense responsive mechanisms including SAR and ISR. PGPR have been found to enhance the growth of several vegetables and crop plants including lettuce, beans, tomato and pepper etc. by production of 1-aminocyclopropane-1-carboxylate (ACC) deaminase and ISR in several plants against drought and other stresses (Mayak et al., 2004). ACC deaminase producing microbes induce longer roots of the host plants that provide better uptake of water and minerals even in drought like situations (Zahir et al., 2008). It was documented that a volatile organic compound (VOC) produced by a Bacillus spp. involved in ISR. Similarly, plants treated with exopolysaccharides (EPS)-producing bacteria also helped in improving the soil structure and provided resistance to water stress. EPS by interacting with the sodium ions in soil also helps in reducing the salt stress (Sandhya et al., 2009). Similarly, co-inoculation of Rhizobium with Pseudomonas in maize plants helped in accumulation of proline and uptake of potassium ions resulted in salt tolerance (Bano and Fatima, 2009). There are some other beneficial microbes that have tendency to regulate the phytohormones, viz., abscisic acid (ABA), ethylene (ET), salicylic acid (SA) and jasmonic acid (JA), Brassinosteroid (BRs) mediated singling pathways which are mostly stimulate the accumulation of antioxidant in the plant cells and engaged with the defense responses of the host plants (Sharma et al., 2019). It was also observed that wheat plants treated with Bacillus amyloliquefaciens and Azospirillum brasilense had the tendency to tolerate heat at their young seedling stage. Additionally, Rhizoglomus irregular, Glomus (AMF) or Bacillus licheniformis inoculation with the diverse communities of beneficial bacteria involved in solubilisation of phosphate and production of siderophores compounds and indole-3 acetic acid (IAA), which normally benefits the crop's performance (Saia et al., 2015; Rai et al., 2021).
In addition to the above-mentioned biostimulants, the use of their natural products or whole organisms can also have dual benefits against both biological and environmental stresses. For example, seed priming of tomato plants with Trichoderma spp. provides resistance to Fusarium spp. by accumulation of antioxidant, defense-related WRKY proteins, and quantifiable lignin in plant cells (Hyder et al., 2017). Similarly, Woo and Pepe (2018) reported that potential biostimulants comprising more than one microbe such as Trichoderma-Azotobacter and Trichoderma-Glomus like microbial formulations had proved better agricultural tools for sustainable crop management in improving harvest quality and quantity of final yield.
Role of Phyto- or Bio-Stimulants in Improving Nutrient Use Efficiency and Management of Post-Harvest Losses
The soil nutrient imbalances or poor quality of soils cause chronic diseases in crop plants surviving in the nutrient-deficient area. In nutrient deficient condition the plants are unable to synthesize important molecules like chlorophyll, nucleic acids, proteins and lipids that are important for normal growth of the crops. Plants become susceptible to several diseases and many enzymes may not function properly under such situations and even complete plant failure may appear at seedling stage in nutrient-deficient conditions. Hence, it is important that plants should be very effective in nutrient use efficiency (NUE). NUE by plant is dependent on the plant's ability for efficient uptake of nutrients such as N from soil followed by its internal transport, storage and remobilization. NUE is different from biological nitrogen fixation (BNF) in the context that in BNF soil bacteria such as Rhizobia can fix atmospheric nitrogen (N2) and convert it to the usable NH3 form which then can be utilized by plants. According to several scientific studies on biostimulants, it was proved that biostimulant components have the ability to increase nutrient uptake with increasing crop productivity (Ebic Welcomes Compromise Reached At Trilogue Meeting on Fertilising Products Regulation, 2019) through different strategies (Garcia-Martínez et al., 2010). In fact its application may be effective in bad soil circumstances as they have the ability to change soil texture as well as increasing macro- and micro-nutrient solubility and modify architecture of the roots system for better transport of nutrients in plants (Halpern et al., 2015). Protein hydrolysates also have the tendency to form complex structures with soil nutrients and therefore they become more available to crops (Farrell et al., 2014). Di Mola et al. (2019) confirmed in their study that plants treated with protein hydrolysates and SWE-based biostimulants enhances the fresh biomass under optimal and sub-optimal nitrogen compositions (0 and 10 kg ha/1) compared to the untreated plants, but the efficiency of PBs was reduced under excesses nitrogen fertilizer (20 and 30 kg ha/1). Similarly, another study showed that synergistic application of non-microbial and microbial (Trichoderma virens) based biostimulants on lettuce plants grown with suboptimal, optimal and supraoptimal nitrogen conditions (0, 70 and 140 kg ha/1) (Rouphael et al., 2020a). The valuable impressions of PBs were less prominent under optimal nitrogen conditions, while absent under excesses N circumstances. There are so many symbiotic and non-symbiotic microbes that are present in the soil including Azospirillum, Azotobacter, Bacillus, Klebsiella, Azorhizobium, Bradyrhizobium, Rhizobium, and Sinorhizobium that widely used as plant growth promoters as they increase the amount of N, P, K and other micro-nutrients in soil (Bhardwaj et al., 2014). Recently, Gupta et al. (2021b) and Ngoroyemoto et al. (2021) reported that treatment of the onion (Allium cepa) and Amaranthus (Amaranthus hybridus) plants individually with two PGPR strains namely Bacillus licheniformis and Pseudomonas fluorescens and a seaweed extract or in combinations improved the mineral nutrition, growth and yield parameters of onion and Amaranthus.
Several experiments were also done to investigate the positive effect of PBs on the productivity of crops. For example, Koleska et al. (2017)'s report showed that foliar spray of biostimulants products on tomato plants grown under NPK nutrient deficient conditions had better yield in comparison to control plants. Similar results have been observed by Anjum et al. (2014) where growth of garlic plants were stimulated by use of PBs under nutrient deficient conditions. Sandepogu et al. (2019) have demonstrated an experiment on combined application of A. nodosum extract and humic substances on lettuce and spinach plants. A combination of 0.25% ANE + 0.2% HA was found suitable for better growth and productivity of crops as compared to the other treatments. Similarly, Fan et al. (2014) where they noticed that pre-harvest root treatment of spinach plants by ANE extract had efficiency to minimize the post-harvest losses (PHL) by reducing ROS and lipid peroxidation activity. Sandepogu et al. (2019) concluded that treatment with PBs before harvesting of crops not only improved the NUE and mineral status but also addressed the issue of reducing post-harvest losses in a sustainable way.
Conclusion and Future Perspectives
The biostimulants including natural substances and microbial inoculants are a novel category of agricultural inputs having great tendency to stimulate better N uptake, plant-growth and tolerance to different abiotic stresses. This review provides knowledge on the current understanding on the progress in the area of identification and utilization of biostimulants in agriculture and further highlights their effects on enhancing crop tolerance to abiotic stresses. The article also focuses on the plant defense mechanisms following application of biostimulants under different environmental stress conditions. Due to high diversity and complexity of biostimulants, characterization of their bioactive components, physiological and molecular stimulation, signaling molecules and mode of actions are still not worked out thoroughly and therefore, are important interests for future researches and commercial applications. The effect of PBs depends on many parameters including raw materials, application techniques, targeted crop and timing of inoculation as well as climatic conditions. From the previous studies and observations it was observed that synergistic application of different biostimulants or consortia of microbial inoculants can play significant roles in plant growth promotion and promote crop's tolerance to survive in challenging environments. Therefore, it would be equally useful to have products combining plant-based extracts mixed with microbial strains for greater efficacy and effectiveness.
Author Contributions
All authors listed have made a substantial, direct, and intellectual contribution to the work and approved it for publication.
Conflict of Interest
The authors declare that the research was conducted in the absence of any commercial or financial relationships that could be construed as a potential conflict of interest.
Publisher's Note
All claims expressed in this article are solely those of the authors and do not necessarily represent those of their affiliated organizations, or those of the publisher, the editors and the reviewers. Any product that may be evaluated in this article, or claim that may be made by its manufacturer, is not guaranteed or endorsed by the publisher.
References
Abd El-Mageed, T. A., Semida, W. M., and Rady, M. M. (2017). Moringa leaf extract as biostimulant improves water use efficiency, physio-biochemical attributes of squash plants under deficit irrigation. Agric. Water Manag. 193, 46–54. doi: 10.1016/j.agwat.2017.08.004
Abdel, A. H. A., and Chaoxing, H. (2011). Effect of arbuscular mycorrhizal fungi on growth, mineral nutrition, antioxidant enzymes activity and fruit yield of tomato grown under salinity stress. Sci. Hortic. 127, 228–233. doi: 10.1016/j.scienta.2010.09.020
Al-Ghamdi, A. A., and Elansary, H. O. (2018). Synergetic effects of 5-aminolevulinic acid and Ascophyllum nodosum seaweed extracts on Asparagus phenolics and stress related genes under saline irrigation. Plant Physiol. Biochem. 129, 273–284. doi: 10.1016/j.plaphy.2018.06.008
Ali, S. Z., Sandhya, V., Grover, M., Linga, V. R., and Bandi, V. (2011). Effect of inoculation with a thermotolerant plant growth promoting Pseudomonas putida strain AKMP7 on growth of wheat (Triticum spp.) under heat stress. J. Plant Interact. 6, 239–246. doi: 10.1080/17429145.2010.545147
Anjum, K., Ahmed, M., Baber, J. K., Alizai, M. A., Ahmed, N., and Tareen, M. H. (2014). Response of garlic bulb yield to bio-stimulant (bio-cozyme) under calcareous soil. Life Sci. Int. J. 8, 3058–3062.
Arshad, M., Shaharoona, B., and Mahmood, T. (2008). Inoculation with Pseudomonas spp. containing ACC-deaminase partially eliminates the effects of drought stress on growth, yield, and ripening of pea (Pisum sativum L.). Pedosphere 18, 611–620. doi: 10.1016/S1002-0160(08)60055-7
Aydin, A., Kant, C., and Turan, M. (2012). Humic acid application alleviates salinity stress of bean (Phaseolus vulgaris L.) plants decreasing membrane leakage. Afr. J. Agric. Res. 7, 1073–1086. doi: 10.5897/AJAR10.274
Bacilio, M., Rodriguez, H., Moreno, M., Hernandez, J. P., and Bashan, Y. (2004). Mitigation of salt stress in wheat seedlings by a gfp-tagged Azospirillum lipoferum. Biol. Fertil. Soils 40, 188–193. doi: 10.1007/s00374-004-0757-z
Bai, Y., Wu, F., Xing, B., Meng, W., Shi, G., Ma, Y., et al. (2015). Isolation and characterization of Chinese standard fulvic acid sub-fractions separated from forest soil by stepwise elution with pyrophosphate buffer. Sci. Rep. 5:8723. doi: 10.1038/srep08723
Bano, A., and Fatima, M. (2009). Salt tolerance in Zea mays (L). following inoculation with Rhizobium and Pseudomonas. Biol. Fertil. Soils 45, 405–413. doi: 10.1007/s00374-008-0344-9
Battacharyya, D., Babgohari, M. Z., Rathor, P., and Prithiviraj, B. (2015). Seaweed extracts as biostimulants in horticulture. Sci. Hortic. 196, 39–48. doi: 10.1016/j.scienta.2015.09.012
Behera, B., Supraja, K. V., and Paramasivan, B. (2021). Integrated microalgal biorefinery for the production and application of biostimulants in circular bioeconomy. Bioresour. Technol. 2021:125588. doi: 10.1016/j.biortech.2021.125588
Behie, S. W., and Bidochka, M. J. (2014). Nutrient transfer in plant–fungal symbioses. Trends Plant Sci. 19, 734–740. doi: 10.1016/j.tplants.2014.06.007
Bhardwaj, D., Ansari, M. W., Sahoo, R. K., and Tuteja, N. (2014). Biofertilizers function as key player in sustainable agriculture by improving soil fertility, plant tolerance and crop productivity. Microb. Cell Fact. 13, 1–10. doi: 10.1186/1475-2859-13-66
Bhattacharyya, P. N., and Jha. (2012). Plant growth-promoting rhizobacteria (PGPR): Emergence in agriculture. World J. Microbiol. Biotechnol. 28, 1327–1350. doi: 10.1007/s11274-011-0979-9
Botta, A. (2012). “Enhancing plant tolerance to temperature stress with amino acids: an approach to their mode of action,” in I World Congress on the Use of Biostimulants in Agriculture, Vol. 1009, 29–35. doi: 10.1007/s00299-016-1980-4
Botta, A. (2013). Enhancing plant tolerance to temperature stress with amino acids: an approach to their mode of action. Acta Hortic. 1009, 29–35.
Bradacova, K., Weber, N. F., Morad-Talab, N., Asim, M., Imran, M., Weinmann, M., et al. (2016). Micronutrients (Zn/Mn), seaweed extracts, and plant growth-promoting bacteria as cold-stress protectants in maize. Chem. Biol. Technol. Agric. 3, 1–10. doi: 10.1186/s40538-016-0069-1
Bulgari, R., Franzoni, G., and Ferrante, A. (2019). Biostimulants application in horticultural crops under abiotic stress conditions. Agronomy 9:306. doi: 10.3390/agronomy9060306
Calvo, P., Nelson, L., and Kloepper, J. W. (2014). Agricultural uses of plant biostimulants. Plant Soil 383, 3–41. doi: 10.1007/s11104-014-2131-8
Carillo, P., Ciarmiello, L. F., Woodrow, P., Corrado, G., Chiaiese, P., and Rouphael, Y. (2020). Enhancing sustainability by improving plant salt tolerance through macro-and micro-algal biostimulants. Biology 9:253. doi: 10.3390/biology9090253
Carillo, P., Colla, G., Fusco, G. M., Dell'Aversana, E., El-Nakhel, C., Giordano, M., et al. (2019). Morphological and physiological responses induced by protein hydrolysate-based biostimulant and nitrogen rates in greenhouse spinach. Agronomy 9:450. doi: 10.3390/agronomy9080450
Carvalho, M. E. A., De Camargo, E., Castro, P. R., Gaziola, S. A., and Azevedo, R. A. (2018). Is seaweed extract an elicitor compound? Changing proline content in drought-stressed bean plants. Comun. Sci. 9, 292–297. doi: 10.14295/CS.v9i2.2134
Cerdan, M., Sánchez-Sánchez, A., Oliver, M., Juárez, M., and Sánchez-Andreu, J. J. (2008). “Effect of foliar and root applications of amino acids on iron uptake by tomato plants,” in IV Balkan Symposium on Vegetables and Potatoes, Vol. 830, 481–488.
Chen, Y., Clapp, C. E., and Magen, H. (2004). Mechanisms of plant growth stimulation by humic substances: the role of organo-iron complexes. Soil Sci. Plant Nutr. 50, 1089–1095. doi: 10.1080/00380768.2004.10408579
Çimrin, K. M., Türkmen, Ö., Turan, M., and Tuncer, B. (2010). Phosphorus and humic acid application alleviate salinity stress of pepper seedling. Afr. J. Biotechnol. 9, 5845–5851.
Colla, G., Hoagland, L., Ruzzi, M., Cardarelli, M., Bonini, P., Canaguier, R., et al. (2017). Biostimulant action of protein hydrolysates: unraveling their effects on plant physiology and microbiome. Front. Plant Sci. 8:2202. doi: 10.3389/fpls.2017.02202
Colla, G., Nardi, S., Cardarelli, M., Ertani, A., Lucini, L., Canaguier, R., et al. (2015). Protein hydrolysates as biostimulants in horticulture. Sci. Hortic. 196, 28–38. doi: 10.1016/j.scienta.2015.08.037
Cordovilla, M. D. P., Berrido, S. I., Ligero, F., and Lluch, C. (1999). Rhizobium strain effects on the growth and nitrogen assimilation in Pisum sativum and Vicia faba plant growth under salt stress. J. Plant Physiol. 154, 127–131. doi: 10.1016/S0176-1617(99)80328-9
Correia, S., Queiros, F., Ribeiro, C., Vilela, A., Aires, A., Barros, A. I., et al. (2019). Effects of calcium and growth regulators on sweet cherry (Prunus avium L.) quality and sensory attributes at harvest. Sci. Hortic. 248, 231–240. doi: 10.1016/j.scienta.2019.01.024
Craigie, J. S. (2011). Seaweed extract stimuli in plant science and agriculture. J. Appl. Phycol. 23, 371–393. doi: 10.1007/s10811-010-9560-4
Cuin, T. A., and Shabala, S. (2005). Exogenously supplied compatible solutes rapidly ameliorate NaCl-induced potassium efflux from barley roots. Plant Cell Physiol. 46, 1924–1933. doi: 10.1093/pcp/pci205
Cuin, T. A., and Shabala, S. (2007). Amino acids regulate salinity-induced potassium efflux in barley root epidermis. Planta 225, 753–761. doi: 10.1007/s00425-006-0386-x
Das, K., and Roychoudhury, A. (2014). Reactive oxygen species (ROS) and response of antioxidants as ROS-scavengers during environmental stress in plants. Front. Environ. Sci. 2, 53. doi: 10.3389/fenvs.2014.00053
Davies, F. T. Jr, Olalde-Portugal, V., Aguilera-Gomez, L., Alvarado, M. J., Ferrera-Cerrato, R. C., and Boutton, T. W. (2002). Alleviation of drought stress of Chile ancho pepper (Capsicum annuum L. cv. San Luis) with arbuscular mycorrhiza indigenous to Mexico. Sci. Hortic. 92, 347–359. doi: 10.1016/S0304-4238(01)00293-X
de Vasconcelos, A. C. F., and Chaves, L. H. G. (2019). Biostimulants and their role in improving plant growth under abiotic stresses. Biostimul. Plant Sci. 2019, 1–14. doi: 10.5772/intechopen.88829
de Vries, F. T., Griffiths, R. I., Knight, C. G., Nicolitch, O., and Williams, A. (2020). Harnessing rhizosphere microbiomes for drought-resilient crop production. Science 368, 270–274. doi: 10.1126/science.aaz5192
Del Amor, F. M., and Cuadra-Crespo, P. (2011). Plant growth-promoting bacteria as a tool to improve salinity tolerance in sweet pepper. Funct. Plant Biol. 39, 82–90. doi: 10.1071/FP11173
Del Buono, D. (2021). Can biostimulants be used to mitigate the effect of anthropogenic climate change on agriculture? It is time to respond. Sci. Total Environ. 751:141763. doi: 10.1016/j.scitotenv.2020.141763
Di Mola, I., Cozzolino, E., Ottaiano, L., Giordano, M., Rouphael, Y., Colla, G., et al. (2019). Effect of vegetal-and seaweed extract-based biostimulants on agronomical and leaf quality traits of plastic tunnel-grown baby lettuce under four regimes of nitrogen fertilization. Agronomy 9:571. doi: 10.3390/agronomy9100571
Di Mola, I., Cozzolino, E., Ottaiano, L., Nocerino, S., Rouphael, Y., Colla, G., et al. (2020). Nitrogen use and uptake efficiency and crop performance of baby spinach (Spinacia oleracea L.) and Lamb's Lettuce (Valerianella locusta L.) grown under variable sub-optimal N regimes combined with plant-based biostimulant application. Agronomy 10:278. doi: 10.3390/agronomy10020278
Drobek, M., Frac, M., and Cybulska, J. (2020). Correction: Plant biostimulants: importance of the quality and yield of horticultural crops—a review. Agronomy 2019, 9, 335. Agronomy 10:433. doi: 10.3390/agronomy10030433
Du Jardin, P. (2015). Plant biostimulants: definition, concept, main categories and regulation. Sci. Hortic. 196, 3–14. doi: 10.1016/j.scienta.2015.09.021
Ebic Welcomes Compromise Reached At Trilogue Meeting on Fertilising Products Regulation (2019). First Step Towards Eu-Wide Market Creation for Biostimulants. Available online: http://www.biostimulants.eu/2018/11/ebicwelcomes-compromise-reached-at-trilogue-meeting-on-fertilising-products-regulation-first-step-towards-euwide-market-creation-for-biostimulants/ (accessed June 5, 2019).
Egamberdiyeva, D., and Höflich, G. (2004). Effect of plant growth-promoting bacteria on growth and nutrient uptake of cotton and pea in a semi-arid region of Uzbekistan. J. Arid Environ. 56, 293–301. doi: 10.1016/S0140-1963(03)00050-8
Elansary, H. O., Skalicka-Wozniak, K., and King, I. W. (2016). Enhancing stress growth traits as well as phytochemical and antioxidant contents of Spiraea and Pittosporum under seaweed extract treatments. Plant Physiol. Biochem. 105, 310–320. doi: 10.1016/j.plaphy.2016.05.024
Elansary, H. O., Yessoufou, K., Abdel-Hamid, A. M., El-Esawi, M. A., Ali, H. M., and Elshikh, M. S. (2017). Seaweed extracts enhance Salam turfgrass performance during prolonged irrigation intervals and saline shock. Front. Plant Sci 8:830. doi: 10.3389/fpls.2017.00830
Ertani, A., Schiavon, M., Muscolo, A., and Nardi, S. (2013). Alfalfa plant-derived biostimulant stimulate short-term growth of salt stressed Zea mays L. plants. Plant Soil 364, 145–158. doi: 10.1007/s11104-012-1335-z
European Biostimulants Industry Council (2012). EBIC and Biostimulants in Brief . Available online at: http://www.biostimulants.eu/
Fan, D., Kandasamy, S., Hodges, D. M., Critchley, A. T., and Prithiviraj, B. (2014). Pre-harvest treatment of spinach with Ascophyllum nodosum extract improves post-harvest storage and quality. Sci. Hortic. 170, 70–74. doi: 10.1016/j.scienta.2014.02.038
Farrell, M., Prendergast-Miller, M., Jones, D. L., Hill, P. W., and Condron, L. M. (2014). Soil microbial organic nitrogen uptake is regulated by carbon availability. Soil Biol. Biochem. 77, 261–267. doi: 10.1016/j.soilbio.2014.07.003
Fasciglione, G., Casanovas, E. M., Quillehauquy, V., Yommi, A. K., Goñi, M. G., Roura, S. I., et al. (2015). Azospirillum inoculation effects on growth, product quality and storage life of lettuce plants grown under salt stress. Sci. Hortic. 195, 154–162. doi: 10.1016/j.scienta.2015.09.015
Feng, G., Zhang, F., Li, X., Tian, C., Tang, C., and Rengel, Z. (2002). Improved tolerance of maize plants to salt stress by arbuscular mycorrhiza is related to higher accumulation of soluble sugars in roots. Mycorrhiza 12, 185–190. doi: 10.1007/s00572-002-0170-0
Frac, M., Hannula, S. E., Bełka, M., and Jedryczka, M. (2018). Fungal biodiversity and their role in soil health. Front. Microbiol. 9:707. doi: 10.3389/fmicb.2018.00707
Garcia, A. C., Santos, L. A., Izquierdo, F. G., Sperandio, M. V. L., Castro, R. N., and Berbara, R. L. L. (2012). Vermicompost humic acids as an ecological pathway to protect rice plant against oxidative stress. Ecol. Eng. 47, 203–208. doi: 10.1016/j.ecoleng.2012.06.011
Garcia-Martínez, A. M., Díaz, A., Tejada, M., Bautista, J., Rodríguez, B., Santa María, C., et al. (2010). Enzymatic production of an organic soil biostimulant from wheat-condensed distiller solubles: effects on soil biochemistry and biodiversity. Process Biochem. 45, 1127–1133. doi: 10.1016/j.procbio.2010.04.005
Gómez-Bellot, M. J., Ortuño, M. F., Nortes, P. A., Vicente-Sánchez, J., Bañón, S., and Sánchez-Blanco, M. J. (2015). Mycorrhizal euonymus plants and reclaimed water: biomass, water status and nutritional responses. Sci. Hortic. 186, 61–69. doi: 10.1016/j.scienta.2015.02.022
Goni, O., Quille, P., and O'Connell, S. (2018). Ascophyllum nodosum extract biostimulants and their role in enhancing tolerance to drought stress in tomato plants. Plant Physiol. Biochem. 126, 63–73. doi: 10.1016/j.plaphy.2018.02.024
Gupta, S., Kulkarni, M. G., White, J. F., Stirk, W. A., Papenfus, H. B., DoleŽal, K., et al. (2021a). “Categories of various plant biostimulants- mode of application and shelf-life,” in Biostimulants for Crops From Seed Germination to Plant Development–A Practical Approach, eds. S. Gupta, J. Van Staden (Amsterdam: Elsevier Inc), 1–60. doi: 10.1016/B978-0-12-823048-0.00018-6
Gupta, S., Stirk, W. A., Plačková, L., Kulkarni, M. G., DoleŽal, K., and Van Staden, J. (2021b). Interactive effects of plant growth-promoting rhizobacteria and a seaweed extract on the growth and physiology of Allium cepa L. (onion). J. Plant Physiol. 262:153437. doi: 10.1016/j.jplph.2021.153437
Hajiboland, R., Aliasgharzadeh, N., Laiegh, S. F., and Poschenrieder, C. (2010). Colonization with arbuscular mycorrhizal fungi improves salinity tolerance of tomato (Solanum lycopersicum L.) plants. Plant Soil 331, 313–327. doi: 10.1007/s11104-009-0255-z
Halpern, M., Bar-Tal, A., Ofek, M., Minz, D., Muller, T., and Yermiyahu, U. (2015). The use of biostimulants for enhancing nutrient uptake. Adv. Agron. 130, 141–174. doi: 10.1016/bs.agron.2014.10.001
Heidari, M., and Golpayegani, A. (2012). Effects of water stress and inoculation with plant growth-promoting rhizobacteria (PGPR) on antioxidant status and photosynthetic pigments in basil (Ocimum basilicum L.). J. Saudi Soc. Agric. Sci. 11, 57–61. doi: 10.1016/j.jssas.2011.09.001
Henda, Y. B., and Bordenave-Juchereau, S. (2013). “Using marine cryptides against metabolic syndrome,” in Bioactive Compounds from Marine Foods: Plant and Animal Sources (Amsterdam: Wiley), 95–112. doi: 10.1002/9781118412893.ch5
Huang, J., Levine, A., and Wang, Z. (2013). Plant abiotic stress. Sci. World J. 2013:432836. doi: 10.1155/2013/432836
Huang, Z., Zou, Z., He, C., He, Z., Zhang, Z., and Li, J. (2011). Physiological and photosynthetic responses of melon (Cucumis melo L.) seedlings to three Glomus species under water deficit. Plant Soil 339, 391–399. doi: 10.1007/s11104-010-0591-z
Hyder, S., Inam-ul-Haq, M., Bibi, S., Humayun, A., Ghuffar, S., and Iqbal, S. (2017). Novel potential of Trichoderma spp. as biocontrol agent. J. Entomol. Zool. Stud. 5, 214–222.
Jayne, B., and Quigley, M. (2014). Influence of arbuscular mycorrhiza on growth and reproductive response of plants under water deficit: a meta-analysis. Mycorrhiza 24, 109–119. doi: 10.1007/s00572-013-0515-x
Jindo, K., Canellas, L. P., Albacete, A., Figueiredo dos Santos, L., Frinhani Rocha, R. L., Carvalho Baia, D., et al. (2020). Interaction between humic substances and plant hormones for phosphorous acquisition. Agronomy 10:640. doi: 10.3390/agronomy10050640
Jithesh, M. N., Shukla, P. S., Kant, P., Joshi, J., Critchley, A. T., and Prithiviraj, B. (2019). Physiological and transcriptomics analyses reveal that Ascophyllum nodosum extracts induce salinity tolerance in Arabidopsis by regulating the expression of stress responsive genes. J. Plant Growth Regul. 38, 463–478. doi: 10.1007/s00344-018-9861-4
Johri, A. K., Oelmüller, R., Dua, M., Yadav, V., Kumar, M., Tuteja, N., et al. (2015). Fungal association and utilization of phosphate by plants: success, limitations, and future prospects. Front. Microbiol. 6:984. doi: 10.3389/fmicb.2015.00984
Juárez-Maldonado, A., Ortega-Ortíz, H., Morales-Díaz, A. B., González-Morales, S., Morelos-Moreno, Á., Sandoval-Rangel, A., et al. (2019). Nanoparticles and nanomaterials as plant biostimulants. Int. J. Mol. Sci. 20:162. doi: 10.3390/ijms20010162
Karlidag, H., Yildirim, E., Turan, M., Pehluvan, M., and Donmez, F. (2013). Plant growth-promoting rhizobacteria mitigate deleterious effects of salt stress on strawberry plants (Fragaria × ananassa). Hortscience 48, 563–567. doi: 10.21273/HORTSCI.48.5.563
Kauffman, G. L., Kneivel, D. P., and Watschke, T. L. (2007). Effects of a biostimulant on the heat tolerance associated with photosynthetic capacity, membrane thermostability, and polyphenol production of perennial ryegrass. Crop Sci. 47, 261–267. doi: 10.2135/cropsci2006.03.0171
Kaushal, N., Gupta, K., Bhandhari, K., Kumar, S., Thakur, P., and Nayyar, H. (2011). Proline induces heat tolerance in chickpea (Cicer arietinum L.) plants by protecting vital enzymes of carbon and antioxidative metabolism. Physiol. Mol. Biol. Plants 17, 203–213. doi: 10.1007/s12298-011-0078-2
Khan, W., Menon, U., Subramanian, S., Jithesh, M., Rayorath, P., Hodges, D., et al. (2009). 0.05 de Aluminio Esmaltado 1uew/180 17. Seaweed extracts as biostimulants of plant growth and development. J. Plant Growth Regul. 28, 386–99. doi: 10.1007/s00344-009-9103-x
Koleska, I., Hasanagić, D., Todorović, V., Murtić, S., Klokić, I., Paradiković, N., et al. (2017). Biostimulant prevents yield loss and reduces oxidative damage in tomato plants grown on reduced NPK nutrition. J. Plant Interact. 12, 209–218. doi: 10.1080/17429145.2017.1319503
Kolomazník, K., Pecha, J., Friebrová, V., Janáčová, D., and Vašek, V. (2012). Diffusion of biostimulators into plant tissues. Heat Mass Transfer. 48, 1505–1512. doi: 10.1007/s00231-012-0998-6
Kumar, G., Bajpai, R., Sarkar, A., Mishra, R. K., Gupta, V. K., Singh, H. B., et al. (2019). Identification, characterization and expression profiles of Fusarium udum stress-responsive WRKY transcription factors in Cajanus cajan under the influence of NaCl stress and Pseudomonas fluorescens OKC. Sci. Rep. 9:14344. doi: 10.1038/s41598-019-50696-x
Kumar, G., Maharshi, A., Patel, J., Mukherjee, A., Singh, H. B., and Sarma, B. K. (2017). Trichoderma: A Potential Fungal Antagonist to Control Plant Diseases. SATSA Mukhapatra-Annual Technical Issue, Vol. 21, 206–218.
Lalitha, P., and Arunalakshmi, K. (2012). Effect of Trichoderma viride on germination of mustard and survival of mustard seedlings. Int. J. Life Sci. Biotechnol. Pharma Res. 1, 137–140.
Le Mire, G., Nguyen, M., Fassotte, B., du Jardin, P., Verheggen, F., Delaplace, P., et al. (2016). Implementing biostimulants and biocontrol strategies in the agroecological management of cultivated ecosystems. Biotechnol. Agron. Soc. Environ. 20, 299–313.
Lephatsi, M. M., Meyer, V., Piater, L. A., Dubery, I. A., and Tugizimana, F. (2021). Plant responses to abiotic stresses and rhizobacterial biostimulants: metabolomics and epigenetics perspectives. Metabolites 11:457. doi: 10.3390/metabo11070457
Li, H., Yue, H., Li, L., Liu, Y., Zhang, H., Wang, J., et al. (2021). Seed biostimulant Bacillus sp. MGW9 improves the salt tolerance of maize during seed germination. AMB Express 11, 1–15. doi: 10.1186/s13568-021-01237-1
Loureiro, R. R., Reis, R. P., and Marroig, R. G. (2014). Effect of the commercial extract of the brown alga Ascophyllum nodosum Mont. on Kappaphycus alvarezii (Doty) Doty ex PC Silva in situ submitted to lethal temperatures. J. Appl. Phycol. 26, 629–634. doi: 10.1007/s10811-013-0085-5
Lucini, L., Rouphael, Y., Cardarelli, M., Canaguier, R., Kumar, P., and Colla, G. (2015). The effect of a plant-derived biostimulant on metabolic profiling and crop performance of lettuce grown under saline conditions. Sci. Hortic. 182, 124–133. doi: 10.1016/j.scienta.2014.11.022
Mahanty, T., Bhattacharjee, S., Goswami, M., Bhattacharyya, P., Das, B., Ghosh, A., et al. (2017). Biofertilizers: a potential approach for sustainable agriculture development. Environ. Sci. Pollut. Res. 24, 3315–3335. doi: 10.1007/s11356-016-8104-0
Maharshi, A., Rashid, M. M., Teli, B., Yadav, S. K., Singh, D. P., and Sarma, B. K. (2021). Salt stress alters pathogenic behaviour of Fusarium oxysporum f. sp. ciceris and contributes to severity in chickpea wilt incidence. Physiol. Mol. Plant Pathol. 113:101602. doi: 10.1016/j.pmpp.2021.101602
Mancuso, S., Briand, X., Mugnai, S., and Azzarello, E. (2006). Marine bioactive substances (IPA Extract) improve foliar ion uptake and water stress tolerance in potted Vitis vinifera plants. Adv. Hortic. Sci. 20, 156–161.
Mayak, S., Tirosh, T., and Glick, B. R. (2004). Plant growth-promoting bacteria that confer resistance to water stress in tomatoes and peppers. Plant Sci. 166, 525–530. doi: 10.1016/j.plantsci.2003.10.025
Meena, V. S., Meena, S. K., Verma, J. P., Kumar, A., Aeron, A., Mishra, P. K., et al. (2017). Plant beneficial rhizospheric microorganism (PBRM) strategies to improve nutrients use efficiency: a review. Ecol. Eng. 107, 8–32. doi: 10.1016/j.ecoleng.2017.06.058
Mesut, Ç. K., and T. Önder, T. Metin Burcu, T. (2010). Phosphorus and humic acid application alleviate salinity stress of pepper seedling. Afr. J. Biotechnol. 9, 5845–5851.
Miller, G. A. D., Suzuki, N., Ciftci-Yilmaz, S. U. L. T. A. N., and Mittler, R. O. N. (2010). Reactive oxygen species homeostasis and signalling during drought and salinity stresses. Plant Cell Environ. 33, 453–467. doi: 10.1111/j.1365-3040.2009.02041.x
Mohd, S., Shukla, J., Kushwaha, A. S., Mandrah, K., Shankar, J., Arjaria, N., et al. (2017). Endophytic fungi Piriformospora indica mediated protection of host from arsenic toxicity. Front. Microbiol. 8:754. doi: 10.3389/fmicb.2017.00754
Nair, P., Kandasamy, S., Zhang, J., Ji, X., Kirby, C., Benkel, B., et al. (2012). Transcriptional and metabolomic analysis of Ascophyllum nodosum mediated freezing tolerance in Arabidopsis thaliana. BMC Genomics, 13, 1–23. doi: 10.1186/1471-2164-13-643
Ngoroyemoto, N., Kulkarni, M. G., Stirk, W. A., Gupta, S., Finnie, J. F., and Van Staden, J. (2021). Interactions between microorganisms and a seaweed-derived biostimulant on the growth and biochemical composition of Amaranthus hybridus L. Nat. Prod. Commun. 15, 1–11. doi: 10.1177/1934578X20934228
Patel, J. S., Kharwar, R. N., Singh, H. B., Upadhyay, R. S., and Sarma, B. K. (2017). Trichoderma asperellum (T42) and Pseudomonas fluorescens (OKC)-enhances resistance of pea against Erysiphe pisi through enhanced ROS generation and lignifications. Front. Microbiol. 8:306. doi: 10.3389/fmicb.2017.00306
Paul, K., Sorrentino, M., Lucini, L., Rouphael, Y., Cardarelli, M., Bonini, P., et al. (2019). A combined phenotypic and metabolomic approach for elucidating the biostimulant action of a plant-derived protein hydrolysate on tomato grown under limited water availability. Front. Plant Sci. 10:493. doi: 10.3389/fpls.2019.00493
Paul, S., Sarkar, D., Rajput, R. S., Singh, S., Parihar, M., Parewa, H. P., et al. (2019). Trichoderma: a part of possible answer towards crop residue disposal. J. Appl. Nat. Sci. 11, 516–523. doi: 10.31018/jans.v11i2.2090
Pecha, J., Fürst, T., Kolomazník, K., Friebrová, V., and Svoboda, P. (2012). Protein biostimulant foliar uptake modeling: the impact of climatic conditions. AIChE J. 58, 2010–2019. doi: 10.1002/aic.12739
Pereyra, M. A., Garcia, P., Colabelli, M. N., Barassi, C. A., and Creus, C. M. (2012). A better water status in wheat seedlings induced by Azospirillum under osmotic stress is related to morphological changes in xylem vessels of the coleoptile. Appl. Soil Ecol. 53, 94–97. doi: 10.1016/j.apsoil.2011.11.007
Pichyangkura, R., and Chadchawan, S. (2015). Biostimulant activity of chitosan in horticulture. Sci. Hortic. 196, 49–65. doi: 10.1016/j.scienta.2015.09.031
Radhakrishnan, R., Hashem, A., and Abd_Allah, E. F. (2017). Bacillus: a biological tool for crop improvement through bio-molecular changes in adverse environments. Front. Physiol. 8:667. doi: 10.3389/fphys.2017.00667
Rai, N., Kumar, V., Sharma, M., and Akhter, Y. (2021). Auxin transport mechanism of membrane transporter encoded by AEC gene of Bacillus licheniformis isolated from metagenome of Tapta Kund Hotspring of Uttrakhand, India. Int. J. Biol. Macromol. 185, 277–286. doi: 10.1016/j.ijbiomac.2021.06.097
Raliya, R., Nair, R., Chavalmane, S., Wang, W. N., and Biswas, P. (2015). Mechanistic evaluation of translocation and physiological impact of titanium dioxide and zinc oxide nanoparticles on the tomato (Solanum lycopersicum L.) plant. Metallomics 7, 1584–1594. doi: 10.1039/C5MT00168D
Rayirath, P., Benkel, B., Hodges, D. M., Allan-Wojtas, P., MacKinnon, S., Critchley, A. T., et al. (2009). Lipophilic components of the brown seaweed, Ascophyllum nodosum, enhance freezing tolerance in Arabidopsis thaliana. Planta. 230, 135–147. doi: 10.1007/s00425-009-0920-8
Ricci, M., Tilbury, L., Daridon, B., and Sukalac, K. (2019). General principles to justify plant biostimulant claims. Front. Plant Sci. 10:494. doi: 10.3389/fpls.2019.00494
Rojas-Tapias, D., Moreno-Galván, A., Pardo-Díaz, S., Obando, M., Rivera, D., and Bonilla, R. (2012). Effect of inoculation with plant growth-promoting bacteria (PGPB) on amelioration of saline stress in maize (Zea mays). Applied Soil Ecology, 61, 264–272. doi: 10.1016/j.apsoil.2012.01.006
Romero, A. M., Vega, D., and Correa, O. S. (2014). Azospirillum brasilense mitigates water stress imposed by a vascular disease by increasing xylem vessel area and stem hydraulic conductivity in tomato. Appl. Soil Ecol. 82, 38–43. doi: 10.1016/j.apsoil.2014.05.010
Rose, M. T., Patti, A. F., Little, K. R., Brown, A. L., Jackson, W. R., and Cavagnaro, T. R. (2014). A meta-analysis and review of plant-growth response to humic substances: practical implications for agriculture. Adv. Agron. 124, 37–89. doi: 10.1016/B978-0-12-800138-7.00002-4
Rouphael, Y., Carillo, P., Colla, G., Fiorentino, N., Sabatino, L., El-Nakhel, C., et al. (2020a). Appraisal of combined applications of Trichoderma virens and a biopolymer-based biostimulant on lettuce agronomical, physiological, and qualitative properties under variable N regimes. Agronomy 10:196. doi: 10.3390/agronomy10020196
Rouphael, Y., Carillo, P., Cristofano, F., Cardarelli, M., and Colla, G. (2021). Effects of vegetal-versus animal-derived protein hydrolysate on sweet basil morpho-physiological and metabolic traits. Sci. Hortic. 284, 110123. doi: 10.1016/j.scienta.2021.110123
Rouphael, Y., Kyriacou, M. C., and Colla, G. (2018a). Vegetable grafting: a toolbox for securing yield stability under multiple stress conditions. Front. Plant Sci. 8:2255. doi: 10.3389/fpls.2017.02255
Rouphael, Y., Kyriacou, M. C., Petropoulos, S. A., De Pascale, S., and Colla, G. (2018b). Improving vegetable quality in controlled environments. Sci. Hortic. 234, 275–289. doi: 10.1016/j.scienta.2018.02.033
Rouphael, Y., Lucini, L., Miras-Moreno, B., Colla, G., Bonini, P., and Cardarelli, M. (2020b). Metabolomic responses of maize shoots and roots elicited by combinatorial seed treatments with microbial and non-microbial biostimulants. Front. Microbiol. 11:664. doi: 10.3389/fmicb.2020.00664
Rubin, R. L., van Groenigen, K. J., and Hungate, B. A. (2017). Plant growth promoting rhizobacteria are more effective under drought: a meta-analysis. Plant Soil 416, 309–323. doi: 10.1007/s11104-017-3199-8
Ruzzi, M., and Aroca, R. (2015). Plant growth-promoting rhizobacteria act as biostimulants in horticulture. Sci. Hortic. 196, 124–134. doi: 10.1016/j.scienta.2015.08.042
Saia, S., Rappa, V., Ruisi, P., Abenavoli, M. R., Sunseri, F., Giambalvo, D., et al. (2015). Soil inoculation with symbiotic microorganisms promotes plant growth and nutrient transporter genes expression in durum wheat. Front. Plant Sci. 6:815. doi: 10.3389/fpls.2015.00815
Sandepogu, M., Shukla, P. S., Asiedu, S., Yurgel, S., and Prithiviraj, B. (2019). Combination of Ascophyllum nodosum extract and humic acid improve early growth and reduces post-harvest loss of lettuce and spinach. Agriculture 9, 240. doi: 10.3390/agriculture9110240
Sandhya, V. Z. A. S., Grover, M., Reddy, G., and Venkateswarlu, B. (2009). Alleviation of drought stress effects in sunflower seedlings by the exopolysaccharides producing Pseudomonas putida strain GAP-P45. Biol. Fertil. Soils 46, 17–26. doi: 10.1007/s00374-009-0401-z
Sarkar, A., Patel, J. S., Yadav, S., Sarma, B. K., Srivastava, J. S., and Singh, H. B. (2014). Studies on rhizosphere-bacteria mediated biotic and abiotic stress tolerance in chickpea (Cicer arietinum L.). Vegetos 27, 158–169. doi: 10.5958/j.2229-4473.27.1.025
Sarma, B. K., Yadav, S. K., Patel, J. S., and Singh, H. B. (2014). Molecular mechanisms of interactions of Trichoderma with other fungal species. Open Mycol J. 8, 140–147. doi: 10.2174/1874437001408010140
Sarma, B. K., Yadav, S. K., Singh, S., and Singh, H. B. (2015). Microbial consortium-mediated plant defense against phytopathogens: readdressing for enhancing efficacy. Soil Biol. Biochem. 87, 25–33. doi: 10.1016/j.soilbio.2015.04.001
Sbrana, C., Avio, L., and Giovannetti, M. (2014). Beneficial mycorrhizal symbionts affecting the production of health-promoting phytochemicals. Electrophoresis 35, 1535–1546. doi: 10.1002/elps.201300568
Scaglia, B., Pognani, M., and Adani, F. (2017). The anaerobic digestion process capability to produce biostimulant: the case study of the dissolved organic matter (DOM) vs. auxin-like property. Sci. Total Environ. 589, 36–45. doi: 10.1016/j.scitotenv.2017.02.223
Sharma, A., Shahzad, B., Kumar, V., Kohli, S. K., Sidhu, G. P. S., Bali, A. S., et al. (2019). Phytohormones regulate accumulation of osmolytes under abiotic stress. Biomolecules 9:285. doi: 10.3390/biom9070285
Sharma, H. S., Fleming, C., Selby, C., Rao, J. R., and Martin, T. (2014). Plant biostimulants: a review on the processing of macroalgae and use of extracts for crop management to reduce abiotic and biotic stresses. J. Appl. Phycol. 26, 465–490. doi: 10.1007/s10811-013-0101-9
Shukla, P. S., Agarwal, P. K., and Jha, B. (2011). Improved salinity tolerance of Arachis hypogaea (L). by the interaction of halotolerant plant-growth-promoting rhizobacteria. J. Plant Growth Regul. 31, 195–206. doi: 10.1007/s00344-011-9231-y
Shukla, P. S., Borza, T., Critchley, A. T., Hiltz, D., Norrie, J., and Prithiviraj, B. (2018). Ascophyllum nodosum extract mitigates salinity stress in Arabidopsis thaliana by modulating the expression of miRNA involved in stress tolerance and nutrient acquisition. PLoS ONE 13:e0206221. doi: 10.1371/journal.pone.0206221
Singh, B. N., Singh, A., Singh, G. S., and Dwivedi, P. (2015). Potential role of Trichoderma asperellum T42 strain in growth of pea plant for sustainable agriculture. J. Pure Appl. Microbiol. 9, 1069–1074.
Smith, S. E., Jakobsen, I., Grønlund, M., and Smith, F. A. (2011). Roles of arbuscular mycorrhizas in plant phosphorus nutrition: interactions between pathways of phosphorus uptake in arbuscular mycorrhizal roots have important implications for understanding and manipulating plant phosphorus acquisition. Plant Physiol. 156, 1050–1057. doi: 10.1104/pp.111.174581
Sofo, A., Tataranni, G., Xiloyannis, C., Dichio, B., and Scopa, A. (2012). Direct effects of Trichoderma harzianum strain T-22 on micropropagated shoots of GiSeLa6®(Prunus cerasus × Prunus canescens) rootstock. Environ. Exp. Bot. 76, 33–38. doi: 10.1016/j.envexpbot.2011.10.006
Subramanian, K. S., Santhanakrishnan, P., and Balasubramanian, P. (2006). Responses of field grown tomato plants to arbuscular mycorrhizal fungal colonization under varying intensities of drought stress. Sci. Hortic. 107, 245–253. doi: 10.1016/j.scienta.2005.07.006
Subramanian, P., Kim, K., Krishnamoorthy, R., Mageswari, A., Selvakumar, G., and Sa, T. (2016). Cold stress tolerance in psychrotolerant soil bacteria and their conferred chilling resistance in tomato (Solanum lycopersicum Mill.) under low temperatures. PLoS ONE 11:e0161592. doi: 10.1371/journal.pone.0161592
Sureshrao, K. S., Pradeeprao, K. T., Dnyanobarao, G. S., Agrawal, T., and Kotasthane, A. S. (2016). Root growth stimulation in rice (Oryza sativa L.) by seed biopriming with Trichoderma sp. Appl. Biol. Res. 18, 30–38. doi: 10.5958/0974-4517.2016.00005.7
Trevisan, S., Manoli, A., and Quaggiotti, S. (2019). A novel biostimulant, belonging to protein hydrolysates, mitigates abiotic stress effects on maize seedlings grown in hydroponics. Agronomy 9:28. doi: 10.3390/agronomy9010028
Tripathi, D., and Pandey-Rai, S. (2021). “Impacts of green synthesized silver nanoparticles with natural bioactive compounds on plant's developmental behavior,” in Natural Bioactive Compounds (New York, NY: Academic Press), 435–452.
Trovato, M., Mattioli, R., and Costantino, P. (2018). From A. rhizogenes RolD to plant P5CS: exploiting proline to control plant development. Plants 7:108. doi: 10.3390/plants7040108
Ugolini, L., Cinti, S., Righetti, L., Stefan, A., Matteo, R., D'Avino, L., et al. (2015). Production of an enzymatic protein hydrolyzate from defatted sunflower seed meal for potential application as a plant biostimulant. Ind. Crops Prod. 75, 15–23. doi: 10.1016/j.indcrop.2014.11.026
Upadhyay, S. K., and Singh, D. P. (2015). Effect of salt-tolerant plant growth-promoting rhizobacteria on wheat plants and soil health in a saline environment. Plant Biol. 17, 288–293. doi: 10.1111/plb.12173
Vaishnav, A., Singh, J., Singh, P., Rajput, R. S., Singh, H. B., and Sarma, B. K. (2020). Sphingobacterium sp. BHU-AV3 induces salt tolerance in tomato by enhancing antioxidant activities and energy metabolism. Front. Microbiol. 11:443. doi: 10.3389/fmicb.2020.00443
Van Oosten, M. J., Pepe, O., De Pascale, S., Silletti, S., and Maggio, A. (2017). The role of biostimulants and bioeffectors as alleviators of abiotic stress in crop plants. https://chembioagro.springeropen.com/ Chem. Biol. Technol. Agric. 4:5. doi: 10.1186/s40538-017-0089-5
Visconti, F., de Paz, J. M., Bonet, L., Jordà, M., Quinones, A., and Intrigliolo, D. S. (2015). Effects of a commercial calcium protein hydrolysate on the salt tolerance of Diospyros kaki L. cv. “Rojo Brillante” grafted on Diospyros lotus L. Sci. Hortic. 185, 129–138. doi: 10.1016/j.scienta.2015.01.028
Visser, S. A. (1985). Physiological action of humic substances on microbial cells. Soil Biol. Biochem. 17, 452–462. doi: 10.1016/0038-0717(85)90009-4
West, J. S., Townsend, J. A., Stevens, M., and Fitt, B. D. (2012). Comparative biology of different plant pathogens to estimate effects of climate change on crop diseases in Europe. Eur. J. Plant Pathol. 133, 315–331. doi: 10.1007/s10658-011-9932-x
Woo, S. L., and Pepe, O. (2018). Microbial consortia: promising probiotics as plant biostimulants for sustainable agriculture. Front. Plant Sci. 9:1801. doi: 10.3389/fpls.2018.01801
Wu, Q., and Zou, Y. (2009). Mycorrhizal influence on nutrient uptake of citrus exposed to drought stress. Philip. Agric. Sci. 92, 33–38.
Xiao, J. X., Hu, C. Y., Chen, Y. Y., Yang, B., and Hua, J. (2014). Effects of low magnesium and an arbuscular mycorrhizal fungus on the growth, magnesium distribution and photosynthesis of two citrus cultivars. Sci. Hortic. 177, 14–20. doi: 10.1016/j.scienta.2014.07.016
Xu, C., and Leskovar, D. I. (2015). Effects of A. nodosum seaweed extracts on spinach growth, physiology and nutrition value under drought stress. Sci. Hortic. 183, 39–47. doi: 10.1016/j.scienta.2014.12.004
Yadav, S. K., Singh, S., Singh, H. B., and Sarma, B. K. (2017). Compatible rhizosphere-competent microbial consortium adds value to the nutritional quality in edible parts of chickpea. J. Agric. Food Chem. 65, 6122–6130. doi: 10.1021/acs.jafc.7b01326
Yakhin, O. I., Lubyanov, A. A., Yakhin, I. A., and Brown, P. H. (2017). Biostimulants in plant science: a global perspective. Front. Plant Sci. 7:2049. doi: 10.3389/fpls.2016.02049
Yildirim, E., Karlidag, H., Turan, M., Dursun, A., and Goktepe, F. (2011). Growth, nutrient uptake, and yield promotion of broccoli by plant growth promoting rhizobacteria with manure. HortScience 46, 932–936. doi: 10.21273/HORTSCI.46.6.932
Yildirim, E., Taylor, A. G., and Spittler, T. D. (2006). Ameliorative effects of biological treatments on growth of squash plants under salt stress. Sci. Hortic. 111, 1–6. doi: 10.1016/j.scienta.2006.08.003
Zahir, Z. A., Munir, A., Asghar, H. N., Shaharoona, B., and Arshad, M. (2008). Effectiveness of rhizobacteria containing ACC deaminase for growth promotion of peas (Pisum sativum) under drought conditions. J. Microbiol. Biotechnol. 18, 958–963.
Zandonadi, D. B., Santos, M. P., Caixeta, L. S., Marinho, E. B., Peres, L. E. P., and Façanha, A. R. (2016). Plant proton pumps as markers of biostimulant action. Sci. Agric. 73, 24–28. doi: 10.1590/0103-9016-2015-0076
Zardak, S., Movahhedi Dehnavi, M., Salehi, A., and Gholamhoseini, M. (2018). Effects of using arbuscular mycorrhizal fungi to alleviate drought stress on the physiological traits and essential oil yield of fennel. Rhizosphere 6, 31–38. doi: 10.1016/j.rhisph.2018.02.001
Zeng, J., Dong, Z., Wu, H., Tian, Z., and Zhao, Z. (2017). Redox regulation of plant stem cell fate. EMBO J. 36, 2844–2855. doi: 10.15252/embj.201695955
Zhang, X., and Ervin, E. H. (2004). Cytokinin-containing seaweed and humic acid extracts associated with creeping bentgrass leaf cytokinins and drought resistance. Crop Sci. 44, 1737–1745. doi: 10.2135/cropsci2004.1737
Zhang, X., and Ervin, E. H. (2008). Impact of seaweed extract-based cytokinins and zeatin riboside on creeping bentgrass heat tolerance. Crop Sci. 48, 364–370. doi: 10.2135/cropsci2007.05.0262
Zhang, X., and Schmidt, R. E. (2000). Hormone-containing products' impact on antioxidant status of tall fescue and creeping bentgrass subjected to drought. Crop Sci. 40, 1344–1349. doi: 10.2135/cropsci2000.4051344x
Zhang, X., Schmidt, R. E., Ervin, E. H., and Doak, S. (2002). Creeping bentgrass physiological responses to natural plant growth regulators and iron under two regimes. HortScience 37, 898–902. doi: 10.21273/HORTSCI.37.6.898
Zhao, D., Zhao, H., Zhao, D., Zhu, X., Wang, Y., Duan, Y., et al. (2018). Isolation and identification of bacteria from rhizosphere soil and their effect on plant growth promotion and root-knot nematode disease. Biol. Control 119, 12–19. doi: 10.1016/j.biocontrol.2018.01.004
Keywords: biostimulants, abiotic stress tolerance, microbial inoculants, sea weed extract, plant-based biostimulants, crop improvement
Citation: Rai N, Rai SP and Sarma BK (2021) Prospects for Abiotic Stress Tolerance in Crops Utilizing Phyto- and Bio-Stimulants. Front. Sustain. Food Syst. 5:754853. doi: 10.3389/fsufs.2021.754853
Received: 07 August 2021; Accepted: 26 October 2021;
Published: 22 November 2021.
Edited by:
Pushp Sheel Shukla, Dalhousie University, CanadaReviewed by:
Shubhpriya Gupta, University of KwaZulu-Natal, South AfricaSikander Pal, University of Jammu, India
Luigi Lucini, Catholic University of the Sacred Heart, Italy
Copyright © 2021 Rai, Rai and Sarma. This is an open-access article distributed under the terms of the Creative Commons Attribution License (CC BY). The use, distribution or reproduction in other forums is permitted, provided the original author(s) and the copyright owner(s) are credited and that the original publication in this journal is cited, in accordance with accepted academic practice. No use, distribution or reproduction is permitted which does not comply with these terms.
*Correspondence: Birinchi Kumar Sarma, YmlyaW5jaGlfa3NAeWFob28uY29t