- 1Department of Food Science, Center for Food Safety, University of Arkansas System Division of Agriculture, Fayetteville, AR, United States
- 2Department of Animal and Dairy Sciences, University of Wisconsin—Madison, Madison, WI, United States
Globally, approximately one-third of food produced for human consumption is lost or discarded, comprising 1. 3 billion tons annually. Factors contributing to food waste from the food manufacturer to the consumer level are numerous. Events that may result in food waste include, but are not limited to, manufacturing food by-products, improper handling within the supply chain (e.g., cold chain deviations), misunderstood food date labels, over-purchasing, and consumer-level temperature abuse. From the manufacturer to consumer, each node in the food supply requires concerted efforts to divert food waste from entering municipal landfills. Depending on the state of the food waste, it is diverted to various outlets, from food donation for consumption to composting for soil amendment. To better understand the opportunities in the United States to divert food waste from landfills, current and emerging federal policies as well as the causes of food waste generation must be understood. Unfortunately, information on both the composition of food waste in the U.S. and how it impacts critical factors in food waste treatment, especially in food waste composting, is limited. Specifically, this review aims to: (1) discuss and compare critical factors that impact the fate of food waste and (2) examine emerging opportunities to advance the processing and products of food waste.
Introduction
Globally, approximately one-third of food produced for human consumption is lost or discarded, which is nearly 1.3 billion tons annually (Gustavsson et al., 2011). Of the food that goes unused, the waste streams can be classified into two primary categories: food loss and food waste. Since there is no legal definition for either terminology, past studies have defined food loss and food waste differently (Chaboud and Daviron, 2017).
However, despite there not being a specific definition of “food waste,” it is important to highlight how groups define this term. For instance, Östergren et al. (2014) and Bellemare et al. (2017) do not define the terms “food waste” and “food loss” separately, but rather refer to them as a single group of “food waste.” However, Parfitt et al. (2010) define food loss at the end of the food supply chain (e.g., retail, consumer) as “food waste” which is also in accordance with Gustavsson et al. (2011). However, Gustavsson et al. (2011) go on to define that all products intended for human consumption—but that do not result in consumption—are considered food waste including products that are diverted for other final uses (e.g., animal feed, industrial by-products). In the current review, the authors follow the definitions of “food loss” and “food waste” set by the Food Agriculture Organization of the United Nations (FAO) (2019b). Specifically, the FAO defines “food loss” as occurring along the food supply chain up to, but not including, the retail level, whereas “food waste” occurs at the consumer and retail level. Therefore, the present review will focus on food waste that occurs after the final product is created, as the majority of food wasted in the U.S. occurs at this final stage of the food supply chain (i.e., retailers, food service providers, and consumers) (Buzby et al., 2014).
According to a database established by the Food Agriculture Organization of the United Nations (FAO) (2019a), based on data from 2016, ~13.8% of food worldwide goes unused due to food loss ranging from 5 to 6% in Australia and New Zealand up to 21% in Central and South Asia across all commodity areas (Table 1A). The FAO database aggregates data from more than 480 publications and reports from various sources and further characterizes food commodity categories. The top three commodities with the most significant amount of loss are (1) roots, tubers, and oil-bearing crops, (2) fruits and vegetables, and (3) meat and animal products, respectively (Table 1B). Notably, at the time of this review, there is not a comprehensive database addressing and characterizing food waste; although, the Food Agriculture Organization of the United Nations (FAO) (2019b, 2021) states that the first estimates of worldwide food waste are in preparation. In a similar effort, Barco et al. (2019) published a recent study suggesting new methodologies for facilitating the quantification of food waste based on European Union data points. However, on a global scale, accruing food waste data is challenging because nearly all studies focus on food waste in developed countries, such as the U.S., United Kingdom, and Japan (Hackes et al., 1997; Harrington et al., 2005; Griffin et al., 2009; Buzby et al., 2011; Lebersorger and Schneider, 2014; Lehmann, 2015; Loke and Leung, 2015; Parry et al., 2015; Liu et al., 2016; Welch et al., 2018; Filimonau et al., 2020).
In the meantime, the Economic Research Service (ERS) in the U.S. Department of Agriculture (USDA) has estimated the amount of food waste annually in the U.S. alone (Buzby et al., 2014). Researchers concluded that ~60.3 million tons of the 195 million tons of food at the retail and consumer levels in 2010 went uneaten. This food waste represents the equivalent of 161.6 billion USD lost where the top three food commodity categories include meat, poultry, and fish (30%; $48 billion), vegetables (19%; $30 billion), and dairy products (17%; $27 billion). With food waste impacting such a large part of the U.S. food supply, researchers are actively looking for opportunities to optimize the uses of food waste. Specifically, this review aims to: (1) discuss and compare critical factors that impact the fate of food waste and (2) examine emerging opportunities to advance the processing and products of food waste.
Histories of U.S. Policies on Food Waste
Over the past decade, initiatives to reduce food waste and to optimize its use have drastically increased (Aboulam et al., 2006; Zhang et al., 2007; Chang and Hsu, 2008; Cuéllar and Webber, 2010). With a piqued interest in the scientific communities and across the general public, lawmakers have been called to action. Federal governmental policy is often motivated by public outcry as well as trade association advocacy over a specific topic, such as the Consumer Brands Association (2017) initiative to address food date labeling. For food waste, the first U.S. federal policy was passed in the mid-1990s (Table 2).
While reducing the amount of food waste generated is the primary goal, donating safe, quality food is the next-best option in reducing the amount of food waste going to landfills (Figure 1). However, food manufacturers and retailers were fearful of liability for any resulting illness or reactions when donating food (143 Cong. Rec. S9532., 1996; Haley, 2013). For this reason, the U.S. Congress passed the Bill Emerson Good Samaritan Food Donation Act (1996); Table 2, which provides criminal and civil liability protection to those bodies that donate food as well as the organizing bodies that receive and distribute the donated food. Of course, if gross negligence and intentional misconduct are found to result in the harm or well-being of a person, the donor or non-profit organization responsible is not protected from civil or criminal liability in the event litigation occurs.
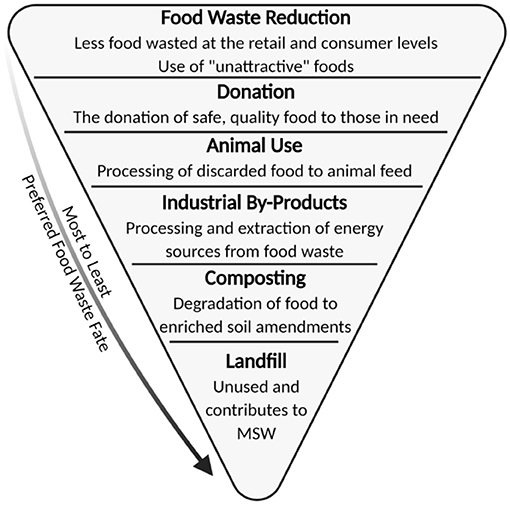
Figure 1. The fate of food waste. Adapted from the U.S. Environmental Protection Agency (EPA) (2017b). The ultimate endpoint of food waste from the best- to worst-case scenario. Created with BioRender.com. MSW, municipal solid waste.
To further encourage food manufacturers to donate food to nonprofits, the U.S. government provides tax incentives to businesses that decide to donate food, according to the Federal Enhanced Tax Deduction for Food Donation: A Legal Guide (Blazek et al., 2016). In 2005, federal tax incentives were temporarily expanded to cover a wider variety of businesses in response to Hurricane Katrina (Katrina Emergency Tax Relief Act, 2005). As a result, food donations to nonprofits in the U.S. sharply increased in the following year (ReFed, 2019a). In 2015, U.S. Congress made the expansion permanent to continue encouraging food donation (Protecting Americans from Tax Hikes Act of, 2015) (Table 2).
If food donation is not an option, the use of food waste in animal feed is highly encouraged. As a result, there are several laws and regulations regarding the use of food waste as animal feed which have been outlined in Table 2. The Swine Health Protection Act (1980); SHPA focuses on ensuring that food waste (e.g., waste derived wholly or in part from meat animal products) used for feed is free of disease-causing pathogens so that the swine, and subsequently, human consumers, are protected. The SHPA requires that food waste containing animal tissue or animal by-products is thermally treated (≥100°C) for at least 30 mins. This waste-to-feed must also be stored under conditions that prevent spoilage microorganisms or the proliferation of pathogenic bacteria. The Ruminant Feed Ban Rule (RFB) (1997) (21 C.F.R. § 589.2000) was also developed in 1997 in response to animal disease outbreaks linked to animal feed and has a shared goal with SHPA—protection of consumer health as well as ruminant animals (Table 2).
In the most extensive food safety reform enacted in the U.S., the Food Safety Modernization Act (2011) was signed into law. While there is a complex set of guidelines in the Preventive Controls for Animal Food (PCAF) Rule, facilities that are in compliance with the Preventive Control for Human Food (PCHF) Rule are not required to follow the additional rules outlined by the PCAF. This includes food processing facilities that produce food under conditions that adhere to the PCHF and the Food, Drug and Cosmetic Act (1938). While this legislation is not solely focused on food waste, it creates another safeguard between food waste and safe animal feed.
In addition to the current rules and regulations, there are several bills regarding food waste that have been introduced to the U.S. Congress (Table 2). In the past few years, several gaps in food waste regulation have been identified, resulting in the introduction of the (1) Food Recovery Act, H.R. 4184. (2015); Food Recovery Act, H.R. 3444; S. 1680. (2017), (2) the Food Date Labeling Act, H.R. 3981; S. 2337 (2019), (3) the Food Donation Act, H.R. 952. (2017); Food Donation Act, S 2787. (2018), and (4) the School Food Recovery Act, H.R. 5607. (2020). All of these policies have been introduced to U.S. Congress. However, none have passed the House of Representatives or Senate.
The FRA has broad, overarching goals to reduce food waste. Unsurprisingly, many of the goals are focused on reducing waste generated at the farm, processing, retail, and consumer levels, respectively (Pingree, 2018). However, some specific goals are targeted at reducing waste generated at U.S. kindergarten through 12th-grade public schools and throughout the federal government. Moreover, the FRA bill aims to reduce food waste by encouraging and supporting USDA and U.S. Environmental Protection Agency (EPA) initiatives to redirect food waste from landfills, such as supporting food anaerobic digestion projects at the local level for energy production. Additionally, the FRA wants to allocate money for research addressing the barriers that prevent the donation of surplus food as well as new technologies to increase the shelf life of highly perishable food. Lastly, if passed, the FRA would mandate the USDA to establish a standard for how much food is wasted at the farm level (Pingree, 2018). However, there are still gaps remaining in food waste regulations.
The FDL bill was written to address the lack of standardization of food date labels in the U.S. (ReFed, 2019b). Partially due to the lack of labeling standardization, consumers discard food based on labels set for quality, not food safety (Gong et al., 2021). Additionally, some retailers in certain states cannot sell food past the date label resulting in more food waste (Kosa et al., 2007; ReFed, 2019b). The FDL has two primary goals: (1) establish a “dual label system” for two label indicators and (2) provide monetary funds for educating consumers about interpreting the aforementioned label system to make better-informed decisions. The dual label system would include one date label, which indicates quality, and the other, a safety-based label. Ultimately, the legislation should result in less food being discarded.
Another bill introduced to the U.S. House of Representatives is the Food Donation Act, H.R. 952. (2017); Food Donation Act, S 2787. (2018). This bill is an expansion of the Bill Emerson Good Samaritan Food Donation Act, allowing for liability protections of a greater scope of donated food products. For example, if this bill is signed into law, foods that are mislabeled in a way that does not impact the safety of the product could be donated.
Recently, the bipartisan SFRA of 2020 was introduced to the House. This bill instructs the USDA to provide grants “to implement food waste measurement and reporting, prevention, education, and reduction projects” at the local level [School Food Recovery Act, H.R. 5607. (2020)]. The School Food Recovery Act, H.R. 5607. (2020) states that when reviewing grant proposals, the USDA must prioritize allocating funds to local agencies that will focus on specific research areas. In the School Food Recovery Act, H.R. 5607. (2020), those areas include the (1) experiential food waste activities that encourage the children involved to participate in food waste measurement and education; and (2) the establishment of local, sustainable food waste measurement, prevention, and reduction projects. Local initiatives to combat food waste have also been proposed and/or passed in at least six states across the U.S. [National Conference of State Legislatures (NCSL), 2020].
As food waste reduction has entered the national dialogue, food waste-based policies have been introduced to the U.S. Congress in efforts to increase the amount of food donated to those in need. However, once the food is no longer safe and cannot be donated, other food waste treatments are needed.
Producers of Food Waste
Food waste results in a reduced availability of consumable food. This waste also impacts the environment by reducing usable land via landfills, creating greenhouse gas emissions, and consuming surface and groundwater resources (Scialabba et al., 2013; Barco et al., 2019). While food waste occurs at every node in the food supply chain, Buzby et al. (2014) found that retail-level establishments waste ~10% of all food. Furthermore, consumers waste ~21% of available food in the U.S. annually (Buzby et al., 2014). Therefore, the present review focuses on these end stages of the food supply chain. However, these data do not account for food waste or loss prior to the retail level. Buzby et al. (2014) did not include any analysis of on-farm up to retail waste due to data limitations. To better understand the contributors of food waste, the authors have split food waste into three categories: (1) industry, (2) retail, and (3) household.
Industry
In this review, the authors are qualifying “industry” as the food processor and/or manufacturer up to the retail level. According to a survey conducted by the Business for Social Responsibility™ (BSR) (2013), U.S. food manufacturers (n = 13) disposed of an estimated 2.4 billion pounds (1.1 billion kg) of food and donated ~700 million pounds (318 million kg) in 2011. However, not all the food that was disposed of by manufacturers went to a landfill—73% of food disposed went into animal feed (Business for Social Responsibility™ (BSR), 2013). These data and analyses were to represent the U.S. food industry as a whole from a survey of 13 respondents, which represents merely 17% of the food manufacturing industry by revenue. Analysis of the food industry and its generated waste is challenging because only one-quarter of the world's major food manufacturing companies have measured food loss and waste within their operations (Flanagan et al., 2019). Furthermore, of those food manufacturers that track their food waste and losses, only 20% had established food waste and loss reduction initiatives and goals (Flanagan et al., 2019). To determine where food waste is occurring in the food industry, efforts are underway to better understand how much food waste is generated in manufacturing facilities.
Research by Griffin et al. (2009) analyzed the community food waste stream of a single county (population 97,000) in upstate New York, which featured the entire food system from farm to fork. In this study, researchers interviewed food processing operations (n = 27) regarding their generation of food waste. The authors found that most of the processing facilities were small in scale—but did not define “small”—and served the local marketplace. During the survey, the authors reported that several (n = 4) food processors were unable to estimate how much waste they generated. Meanwhile, other processors could estimate their food waste but used a myriad of measurements (e.g., volume, weight, monetary). Ultimately, the authors estimated that 258,414 pounds (117,215 kg) and 17,660 gallons (66,850 L) of food waste were generated per year from the surveyed operations. It is important to understand how companies track food waste as it has been shown that companies who measure surplus food more effectively manage food waste (Garrone et al., 2016). Based on data gathered by Garrone et al. (2016), the efficiency and effectiveness of surplus food management in the food industry are primarily influenced by measurement, coordination, and organization within the company. Unsurprisingly, the authors found that the companies which regularly measured the quantity of surplus food produced were managed more effectively than those companies that did not monitor the amount of surplus.
Another influential factor in food waste management is coordination within the company regarding a “best” option for the fate of the surplus food, such as rework, donation, or other food waste fates. Similar to coordination, organizational structure within the company can reduce the amount of food waste. For instance, if a company has an established, structured plan for surplus food that can be executed immediately after generation, this will reduce the likelihood that the food will become unsafe for donation or other uses, such as animal feed. Food manufacturers are increasingly aware of the importance of food waste reduction and its influence on food waste generation in the supply chain (Champions 12.3., 2020). In fact, almost 200 food suppliers and retailers have committed to a 50% food waste reduction target within their own operations, to measuring and publishing their food loss and waste, and to take action to reduce their waste (Champions 12.3., 2020). However, food manufacturers are only one aspect of a much more complicated food chain when it comes to food waste.
Retail
At the marketing stage of the supply chain, food waste occurs for a multitude of reasons (Buzby et al., 2011). Retail food waste may occur due to the natural senescence of fruits and vegetables, damage to foods during transportation and stocking (e.g., dented cans), sell-by dates, temperature abuse in cold chain storage, overstocking of product, and improper stock rotations, such as not adhering to the “first in, first out” rule (Buzby et al., 2011; Mena et al., 2011; Goodman-Smith et al., 2020).
Moreover, according to the U.S. Department of Agriculture (USDA)-Economic Research Service (ERS) (2019), 21,435 new food and beverage products were introduced to the marketplace in 2016. As innovative food products hit the shelves, they often have low success rates (Buzby et al., 2011). The failure rate of some product categories exceeds 90%. As a result, unpurchased products are often sent back to the manufacturer, where the product is ultimately discarded, or the product exceeds a safety or quality use-by date and is removed from the shelves (Connor and Schiek, 1997; Buzby et al., 2011). In the diverse food marketplace, there are many factors that contribute to the acute ongoing problem of food waste.
In the BSR report (2013) described in the subsection Industry, U.S. food retailers (n = 10) and wholesalers (n = 3) discarded ~1.3 billion pounds (589 million kg) of food waste that went to landfills or incinerators in 2011. However, the retail and wholesale sector donated an estimated 670 million pounds (303 million kg) of food products during the same year. The survey respondents represented 30% of the industry. Despite donations, ~50% of the food products were wasted, indicating the need for innovative downstream technologies to turn food waste into value-added products, such as agricultural soil amendments (i.e., compost), animal feed, and energy.
Household
Household, or “consumer” food waste, is one of the largest categories of waste that occurs in the food chain (Rohm et al., 2017; Welch et al., 2018; Aschemann-Witzel et al., 2019; Yu and Jaenicke, 2020). On the consumer level, there are many different facets that can contribute to the generation of food waste. Often, these variables are diverse in nature (Chen and Chen, 2018). Table 3 outlines some variables of food waste generation and their potential remedies as summarized by Chen and Chen (2018).
Many contributing factors regarding food wastage in the household are based on consumer behaviors, and thus, could be remedied through education and outreach initiatives. For instance, in the home, a driver of food waste is the misunderstanding of the various date labels, as described in the subsection Policies. The different labeling systems can cause confusion among consumers. An example of this confusion is a consumer throwing away a carton of eggs past the ‘best by’ date and misinterpreting this listed date as an expiration rather than an indicator of quality. Date labeling confusion could be addressed by industry initiatives, consumer education programs, and legislation to create a streamlined date labeling system (Read and Muth, 2021).
A recent trend in food waste reduction is the attempt to change consumer acceptance of “ugly” fruits and vegetables (see Table 3; Pantano, 2015). The concept of ugly produce stems from the rejection of fruits and vegetables at peak ripeness and quality. This produce is being left in the field or sorted out at the processing level due to deformities in shape. The edible but “ugly” produce is now reaching the mainstream through produce delivery boxes and small sections of retailers who advertise the use of imperfect produce to reduce food waste. This acceptance and call-to-action at the consumer level not only brings forth the issue of food waste but allows the consumer to be an active participant in the food waste reduction strategy (de Hooge et al., 2017).
As illustrated, food waste generation occurs at all points in the food chain. Although the remedies for food waste reduction appear different, food waste reduction is possible with planning, education, and action. Nevertheless, food waste generation will continue to occur at some level, so it is valuable to optimize the technologies used to give food waste a new purpose which will be the focus of this review from here forward.
Current Food Waste Uses
The current uses of food waste from best- to the worst-case scenario are illustrated in Figure 1. Unfortunately, an overall estimated 35.6% of food waste generated in the U.S. ends up in a landfill (U.S. Environmental Protection Agency (EPA), 2020. Additionally, when excluding the U.S. food industry manufacturer and supplier sector, more than half (55.9%) of food waste generated by residential, commercial, and institutional sectors is sent to the landfill, according to the U.S. Environmental Protection Agency (EPA) (2020). Overall, the EPA estimates that food waste comprises 24% of all materials sent to landfills in the municipal solid waste stream (MSW) (U.S. Environmental Protection Agency (EPA), 2020).
Of the millions of tons of food waste generated annually in the U.S., an estimated 3.4 to 5.3% of food waste is composted (Buzby et al., 2014; U.S. Environmental Protection Agency (EPA), 2019a). As indicated in Figure 1, other uses of food waste include the creation of animal feed (21.3%), fuel for energy sources (12.5%), donation for human consumption (7.2%), land application (8.9%), controlled combustion (7.5%), and sewer/wastewater treatment (3.6%) [U.S. Environmental Protection Agency (EPA), 2020]. A key to the success of food waste recovery efforts hinges on the composition of the food waste, which varies greatly (Zhang et al., 2007; Chang and Hsu, 2008; Adhikari et al., 2009). While no data analyses are available on the regionality or seasonality of raw food waste composition, it is known that variations in food waste composition directly impact the effectiveness of the composting system as well as animal feed (Chang and Hsu, 2008; Chang and Chen, 2010). This data gap is a problem repeatedly reported in food waste research (Xue et al., 2017). For instance, composted food waste relies on the biological process of food degradation, which, in turn, reduces in volume and creates an enriched soil amendment for further use (Manu et al., 2017). In animal feed, the heterogeneity and composition of food waste can impact the nutrient content making the food-to-feed conversion variable. In this subsection, the optimization of food waste recovery efforts that result in usable secondary products (e.g., energy, soil amendments, animal feed) will be the focus.
Animal Feed
An alternative fate for food waste is animal feed. Over the last decade, the interest in sustainable animal feed production has increased (Angulo et al., 2012a). In 2014, Makkar and Ankers (2014) conducted a survey (n = 1195) that found those involved in the animal feed market (i.e., industry, non-governmental organizations, academe) identified food waste as a priority research area for animal nutrition. Particularly, the use of fruit and vegetable waste (FVW) and crop waste have been studied as a supplement in bovine feed (Angulo et al., 2012b), fish feed (Ulloa et al., 2004; Mo et al., 2014), and inclusion into poultry feed (Khempaka et al., 2009; Abdel-Hafeez et al., 2018; Pandi et al., 2018). In addition, albeit less frequently, studies have investigated the use of food waste products in sheep, goat, and swine feed (Westendorf et al., 1998; Yang et al., 2006; Márquez et al., 2010; Sahoo et al., 2021).
While the redirection of food waste to animal feed is an option, there are some concerns. As described in the subsection Policies, there are parameters (e.g., refraining from feeding mammalian proteins to ruminant animals) that must be adhered to in order to protect the animals and, eventually, human consumers (U.S. Food and Drug Administration (FDA), 2018). With those considerations in mind, many of the studies focus on FVW. However, there are problems that can arise from using horticultural food waste as animal feed. For instance, FVW varies throughout the year due to seasonality. Therefore, those that rely on food waste products for animal feed will have to re-formulate often to address these seasonal changes (Martin et al., 2016). Another issue that can emerge with food waste is the proliferation of pathogen contamination, especially due to the high water activity (≥ 0.9 Aw) (Garcia et al., 2005; Martin et al., 2016). If pathogenic microorganisms are present, many of these organisms can cause disease in the animals if the food waste is not treated, as thoroughly reviewed by Avery et al. (2012). For example, a swine farmer fed unprocessed food waste to pigs, which led to a foot-and-mouth disease outbreak resulting in an 8 billion EUR (~8.8 billion USD) loss (Scudamore and Harris, 2002). In addition to the risk of disease, the high Aw makes processing the food waste to animal feed challenging (Martin et al., 2016). Often, food waste has a moisture content of >80% and would need to be reduced to ~10% to be used as animal feed (Garcia et al., 2005; Martin et al., 2016). These risk factors must be mitigated via processing techniques to utilize food waste as animal feed.
Ruminant
There are many studies that have investigated the use of food waste as livestock feed (Abarghuei et al., 2014; Das et al., 2019; de Evan et al., 2020). For instance, Angulo et al. (2012b) studied the impact of integrating FVW into lactating Holstein cow feed. The authors evaluated the use of FVW and its impact on milk yield and quality. FVW was included in 0, 6, 8, 12, and 18% concentrations of the feedstuff. The top three components of the FVW were cabbage (15%), orange (13%), and refuse (11%). The authors defined “refuse” as small pieces of indiscernible products. The data showed that including FVW did not statistically impact the yield of milk produced or reduce the quality of milk. Although the use of FVW as a dietary ingredient in Holstein cow feed is limited to a proportion of 6–18%, this could still lead to other alternative uses of FVW in the future.
Another study by de Evan et al. (2020) assessed the potential of cauliflower and Romanesco waste (e.g., leaves, stems, and sprouts) in an in vitro ruminant feeding study. Specifically, the authors investigated in vitro ruminal fermentation and intestinal digestibility. de Evan et al. (2020) found that all fractions of cauliflower and Romanesco waste were highly degradable the in vitro rumen study and resulted in high digestibility for a protein source. During these in vitro studies, it was observed that up 24% of dried cauliflower in concentrate can be included in feed for dairy sheep without negative ruminal fermentation effects. However, these studies should be confirmed in vivo and must address the impact of cauliflower and Romanesco waste on overall animal health and end-product quality. While the use of food waste in ruminant nutrition is promising, more research needs to be done.
Fish
Meanwhile, Ulloa et al. (2004) analyzed tropical FVW residues (e.g., green banana, coffee cherry pulp, rice hulls, pineapple, papaya) and their potential uses for fish feedstuff. This study considered many of the factors that would impact developing fish feed from FVW. Based on data gathered analyzing the FVW produced in Costa Rica, the authors found that most FVW products contain high levels of moisture (80–84%) and low levels of protein (5–12.2%), with the exception of protein content levels in papaya (23.2%). In addition to this, the tropical FVW also contained variable amounts of other compounds (e.g., polyphenols, caffeine), high fiber levels (1.6–57.1%), and toxic substances and pesticides. Low protein content and high fiber levels are not beneficial in feed utilization and can hinder fish growth (Ulloa et al., 2004). Additional issues the authors explored include the nutritional density of the waste, the availability throughout the year, and the pollution and toxicity risk of the waste-turned-feed.
In application, Mo et al. (2014) studied the impact of food waste-based diets in polyculture of low trophic level fish (grass carp, bighead, and mud carp). Three diets were fed to the fish–one control and two food waste-based diets–for 6 months. The food waste was acquired from hotel kitchens. Diet A was composed of fruits and vegetables (10%), cereal (53%), bone meal (8%), fish meal (10%), corn starch (15%), and other food waste (4%), whereas Diet B was composed of fruits and vegetables (10%), meat products (25%), cereal (28%), bone meal (8%), fish meal (10%), corn starch (15%) and other food waste (4%). Diet A did not include meat waste and was 53% cereal-based, whereas Diet B was 25% meat and 28% cereal. Over the six-month period, the authors analyzed the presence of plankton, water quality, and fish growth. The water quality did not appear to be significantly impacted by the various feeds. Meanwhile, plankton density was the highest in the Diet A body of water, but not significantly different. However, Diet B feedstuff seemed to be a better formulation in terms of the overall performance on fish growth factors. Thus, the increased diversity in feedstuff generation could allow for more food waste to be utilized for many fishery species.
Poultry
Recently, Abdel-Hafeez et al. (2018) evaluated the use of potato peels and sugar beet pulp with and without enzyme supplementation in broiler chicken diets. The application of enzyme supplementation was investigated due to the significant amounts of non-starch polysaccharides (e.g., cellulose, hemicellulose, xylose, and lignin) in potato peels and sugar beet pulp (Abdel-Hafeez et al., 2018), and poultry do not possess the enzymes required to break down the non-starch polysaccharides (Annison, 1993). Thus, poultry would be unable to access the nutrients without enzyme supplementation (Chesson, 2001). Abdel-Hafeez et al. (2018) fed alternative diets to the broilers from 1 to 42 days of age while observing growth performance, carcass characteristics, and blood parameters. The birds were split into five groups: group 1 was the control; groups 2 and 3 were fed diets containing potato peels and sugar beet pulp at the rate of 15 and 7.5%, respectively; and groups 4 and 5 were fed the same altered diet with an enzyme mixture. The authors found that potato peels can be added to feedstuff at a maximum proportion of 15%, without the inclusion of enzymes, in the grower diet of broilers. Conversely, the inclusion of sugar beet pulp at 7.5% in the diet resulted in poor growth performance in the absence of enzymes. In addition, the authors concluded that poultry producers could include potato peels (≤15%) and sugar beet pulp (≤7.5%) in the diets of broilers after enzyme treatment while potentially reducing costs of conventional feeding methods.
Furthermore, Pandi et al. (2018) studied the evaluation of the effects of sweet potato integrated into broiler diets. The authors used broiler diets that contained 0 and 25% sweet potato flour with and without enzyme supplements. While this study was not an analysis of growth performance, the researchers evaluated gastrointestinal tract (GIT) parameters associated with digestion and GIT health and found that these factors were not undermined by the addition of sweet potato flour to broiler feedstuffs. While these studies are not an exhaustive list, they represent a glimpse into food waste-to-feed research that is analyzing and optimizing food waste for animal feedstuffs. However, not all food waste is optimal or available to use as animal feed. In cases such as this, some food waste can be converted into energy.
Energy Production
With food waste that is no longer safe or wholesome for human or animal consumption, it can be directed into further processes that create energy or an energy source by-product (U.S. Environmental Protection Agency (EPA), 2019b). There are three ways to process food waste into alternative energy or by-product: anaerobic digestion (AD), rendering, and biodiesel (U.S. Environmental Protection Agency (EPA), 2019b). Each energy generation pathway has different goals in mind. For instance, the processing of food waste oils and fat to biodiesel creates a renewable energy source and less pollution (Hossain et al., 2008; U.S. Environmental Protection Agency (EPA), 2019b). In contrast, rendering uses food waste, liquid fats, and meat products as raw materials to create processed products such as cosmetics and soaps (Woodgate and Van Der Veen, 2004). Rendering has been a long-standing process to reduce food waste at the manufacturing level and facilitates the use of all parts of the animal in meat production (Shareefdeen et al., 2005). However, AD can create usable energy and a soil amendment from food waste [U.S. Environmental Protection Agency (EPA), 2017a]. Altogether, these processes utilize different parts of food waste and create valuable products.
Biodiesel
Biodiesel is used as an alternative to conventional diesel fuel that does not require significant modifications in current fuel engines (Ma and Hanna, 1999; Sensöz et al., 2000; Demirbas, 2007; Hossain et al., 2008). However, biodiesel can be expensive to produce due to the high cost of the refined oils used (Marchetti et al., 2008). Given this high cost as well as the moral dilemma of using edible materials to create refined feedstocks, researchers have investigated the use of waste products as the raw materials to reduce the cost and limit the number of edible feedstocks used in biodiesel production (Karmee et al., 2006, 2015a,b; Ugarte and He, 2007; Ajanovic, 2010). More recently, studies have focused on the use of food waste as a biodiesel material. For instance, Yang et al. (2014) utilized instant noodle manufacturing waste as a feedstock for conversion to bioethanol and biodiesel. The authors isolated the instant noodle waste into starch residues and palm oil. By treating the food waste, the researchers were able to convert the waste to bioethanol and biodiesel at a conversion of 98.5 and 95.4%, respectively. The authors found that these conversion rates would support the manufacturing-scale use of instant noodle waste as a viable raw material for energy production.
Moreover, Yu et al. (2011) used an oil fraction gathered from food waste leachate. In the process, the authors found that the leachate oil fraction contained various fatty acid methyl esters (FAME), commonly referred to as biodiesel (Bautista et al., 2009; Demirbas, 2009), after pretreatment with sulfuric acid as an acid catalyst. Based on the FAME collected, the authors anticipate that biodiesel production from food waste oil fractions will be feasible as systems become optimized for processing food waste. In addition, Karmee et al. (2015a) found similar results when isolating lipids from café bakery waste. The authors determined the peak biodiesel yield was 100% for the base (KOH) catalyzed transesterification at a 1:10 M ratio of lipid to methanol in 2 h at 60°C. Similarly, Karmee et al. (2015b) found that waste cooking oil could also be a suitable material for biodiesel generation. These studies highlight the future use of food waste as a biodiesel feedstock. However, food waste can be used to generate energy in other ways.
Anaerobic Digestion
Anaerobic digestion, which is the degradation of the product in the absence of oxygen, is emerging as one of the most efficient systems to process food waste (Gujer and Zehnder, 1983; Zhang et al., 2007; Wambugu et al., 2019). In addition to removing food waste from landfills, the energy that is produced via AD is a renewable source (Milán et al., 2001; Bouallagui et al., 2003; Meyer-Kohlstock et al., 2016). The concept of using food waste in AD to produce energy has been thoroughly reviewed by Xu et al. (2018). The biogas produced during AD is comprised of ~65 to 70% methane (CH4) and 35–40% carbon dioxide (CO2), which is ultimately used as compressed natural gas and electric energy. Moreover, the use of food waste in AD to produce energy is not only a concept; there are some active ADs that utilize the food waste stream in the U.S., as described below.
More specifically, The University of Wisconsin (UW) Oshkosh began producing renewable energy via a dry fermentation anaerobic biodigester in 2011 (Potts, 2012). At maximum capacity, the UW Oshkosh's biodigester can generate up to 10% of the campus' electricity and heat and processes 11,000 tons of organic waste annually [Potts, 2012; University of Wisconsin Oshkosh (UWO), 2021]. It utilizes decomposed agricultural plant waste, city of Oshkosh yard clippings, and campus food waste where input materials must balance out to a moisture content of <75%. In a similar fashion, the East Bay Municipal Utility District (EBMUD) in Oakland, California, is the first wastewater treatment plant in the nation to convert food waste to energy via AD (Day, 2012; U.S. Environmental Protection Agency (EPA), 2019b). However, this food waste is not collected from residents' homes; it is obtained from local restaurants and food markets (U.S. Environmental Protection Agency (EPA), 2019b). The utilization of local food waste allows the EBMUD system to produce enough electricity to power the EDMUD facility and generate ~15% more power than it uses annually (Day, 2012). However, AD of food waste and other biological products is sensitive due to the variety of microorganisms that are involved (Fagbohungbe et al., 2016). There are multiple ways to use food waste to create energy or utilize it as animal feed. However, it is necessary to understand the composition and current sources of food waste in order to optimize methods.
Composting
Approaches
As described, food waste originates from various sources ranging from industry to household-scale; thus, food waste composting can occur using a variety of approaches. According to the U.S. Environmental Protection Agency (EPA) (2016), there are four defined types of food waste aerobic composting (Table 4). Turned windrow composting systems can process a wide variety of feedstocks—the raw, green food waste prior to processing—ranging from grease and animal by-products to yard trimmings. However, this system requires mechanized turning based on its size, continuous labor and produces noticeable odor and leachate (Table 4). This outdoor turned windrow system can be contrasted with vermicomposting, which is much smaller in scale and limited in its input/output. As described in Table 4, the vermicomposting utilizes live worms to process the food and yard waste feedstock. Specifically, 800–1,000 worms can consume approximately one-half pound of organic material per day. However, this system is typically on a household scale and is temperature and light-sensitive with little to no odor or leachate production. Additionally, there is aerated static pile and in-vessel composting. Aerated static composting is not turned but is aerated through forced-air below the compost pile. This method of composting requires an investment in equipment and expertise. Whereas in-vessel composting is held in a container, often on a smaller, household scale, but does not necessarily require extensive financial investments. There is a substantial diversity in the feedstock input, the timeframe to process the waste, and equipment and labor requirements depending on the approach used for composting food waste (Table 4). Recently, Awasthi et al. (2020) have highlighted the ever-evolving global food waste composting trends and challenges, including gaseous emissions, nutrient loss mitigation, and assessing mature compost quality.
Laboratory-Based Composting Studies
As food waste has moved to the forefront of research interest and focus, food waste composting studies have rapidly proliferated in the past 20 years. However, to our knowledge, nearly all food waste composting studies utilize in-vessel composters on a pilot- or bench scale. Yet, despite the limited variety in the type of composting system, studies characterizing food waste composting are diverse, as summarized in Table 5. Although all studies in Table 5 utilized a food waste feedstock, the similarities across these composting studies end there. From the bulking agent to the in-vessel design to the measured parameters, the food waste composting studies are limited in comparability.
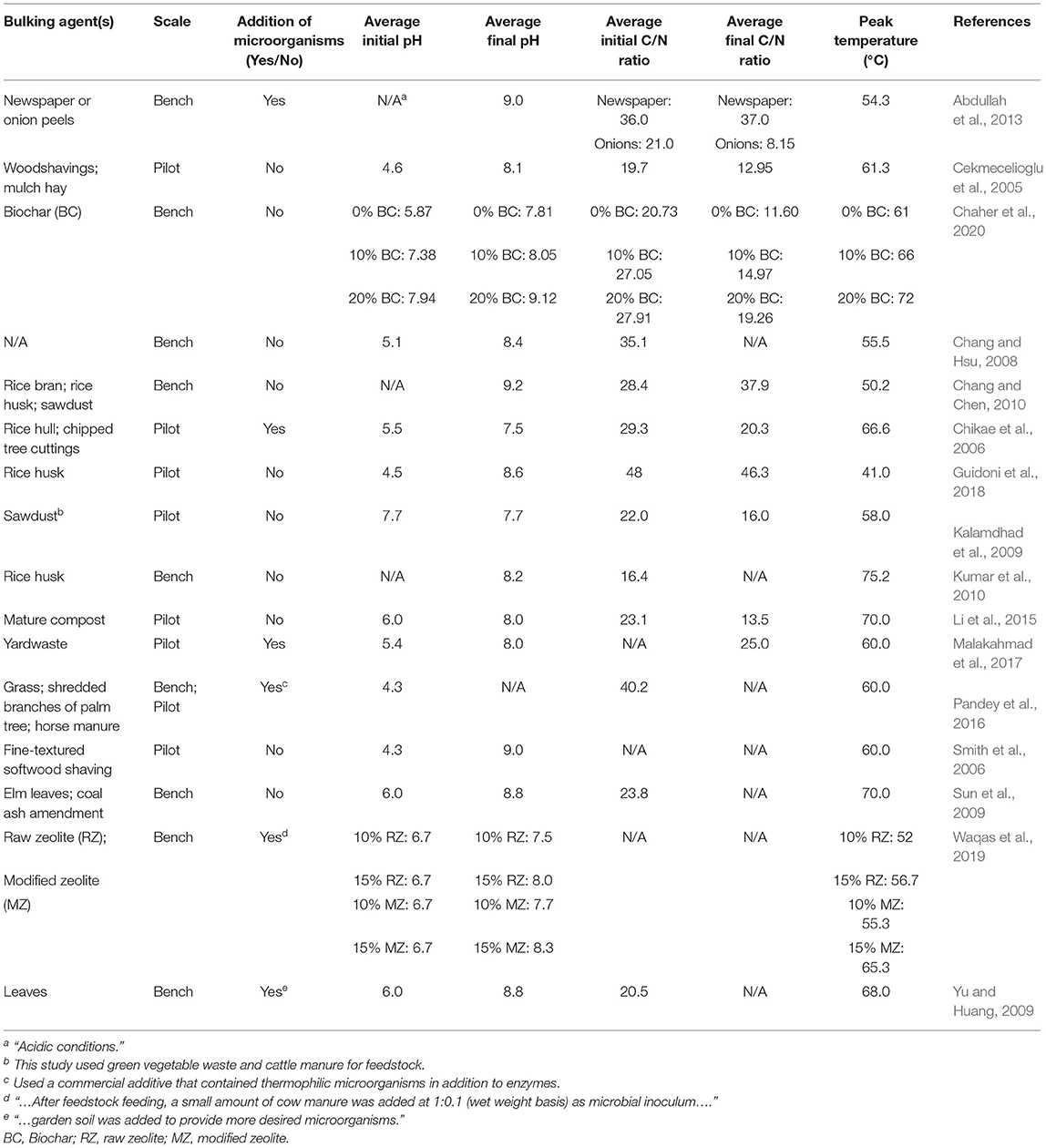
Table 5. Variability in recorded research parameters from in-vessel composting of food waste feedstock.
Bulking agents are the organic additives (e.g., rice husks, grass clippings, sawdust) added to the feedstock used to increase porosity, reduce moisture content, and buffer organic acids produced in composting (Adhikari et al., 2009; Chang and Chen, 2010). Nevertheless, in the available data, several patterns emerged from the published literature on in-vessel food waste composting: (1) the average pH of the compost turned from acidic to basic, (2) the average carbon-to-nitrogen (C/N) ratio changed over time based on bulking agents used, and (3) the peak temperatures recorded ranged from 41 to 75.2°C. These three reported parameters are critical factors in converting raw food waste feedstock to mature compost (Chang and Hsu, 2008; Adhikari et al., 2009; Kumar et al., 2010; Guo et al., 2012; Huet et al., 2012).
Additionally, aeration and moisture content are important factors in the composting of food waste (Chang and Hsu, 2008; Adhikari et al., 2009; Kumar et al., 2010; Huet et al., 2012). Yet, those two factors are irregularly reported in the literature. The importance of aeration and moisture content, in addition to pH, temperature, and C/N ratio, are reviewed extensively by Li et al. (2013). Furthermore, within current research, the reported use of microbial additives varied from study to study (Table 5) and is not well quantified. Effective microorganism (EM) uses in food waste composting is explored further in the subsection Additives in Composting.
Challenges
One of the biggest challenges that arise from food waste composting is environmental odor and leachate production. Community and industry-sized food waste composting methods often generate leachate that must be treated and require an odor control plan [see Table 5; U.S. Environmental Protection Agency (EPA), 2016]. These detectable environmental odors have an established impact on human health (Heida et al., 1995; Bruno et al., 2007; Tsai et al., 2008; Gallego et al., 2012) and are often difficult to address (Jackson et al., 2014) as they are a result of hundreds of identified odorous substances (Smet et al., 1999; Sundberg et al., 2013). Moreover, the diversity and intensity of food waste compost odor can vary significantly based on the composition of food waste (Kim et al., 2009), such as a low starting pH, potentially causing discomfort to those near composting facilities (Müller et al., 2004).
For example, a study by Mohee et al. (2015) found that food waste compost feedstock with a low C/N ratio (17.7 ± 0.7) gave a noticeable noxious odor. A reduction in odor could be achieved by the addition of inorganic additives to raise the C/N ratios (27.2–31.2). Koivula et al. (2004) found similar results in odor reduction after adding inorganic ash to compost. It is important to understand that reducing some of the factors contributing to malodorous compounds may, in turn, reduce the efficiency of other critical factors, such as microbial activity, by altering the pH and composting temperatures (Fang et al., 1997, 1998, 1999; An et al., 2012). However, this was not explored by Mohee et al. in their 2015 study.
Rather than attempting to reduce the odors from food waste composting, Mao et al. (2006) identified which compounds were exceeding the human olfactory threshold in a food waste composting facility in Taiwan. The study authors identified six types of compounds that crossed the olfactory threshold—ammonia, amines, dimethyl sulfide, acetic acid, ethyl benzene, and p-Cymene. These compounds contribute to the odor nuisance problem and require intervention to reduce the odors released in the community. As food waste composting grows in the U.S., an infrastructure to combat food waste composting odors will become necessary (D'Imporzano et al., 2008; Tsai et al., 2008; Gallego et al., 2012; Qamaruz-Zaman and Milke, 2012; Sundberg et al., 2013; Maulini-Duran et al., 2014; Ermolaev et al., 2015).
Moreover, food waste composting produces more than mature compost and odors. During the composting process, some liquid percolates through the compost and begins to pool (Sall et al., 2019). This liquid is referred to as “leachate” and contains much more than water (Yu et al., 2011). Food waste compost leachate includes water, oils, minerals, heavy metals, suspended solids, and various other by-products (Le Man et al., 2010; Heo et al., 2011; Yu et al., 2011). To protect the soil integrity and water supply, this leachate should be collected and treated for toxic organic pollutants (Behera et al., 2010; Roy et al., 2018; Sall et al., 2019). Considering the regulatory framework of some countries requiring further treatment, research efforts on the utilization of the leachate have begun to arise (Behera et al., 2010; Le Man et al., 2010; Heo et al., 2011; Li et al., 2013; Sall et al., 2019). For example, Sall et al. (2019) highlighted the opportunity for the use of FVW leachate to be used as a crop fertilizer. In addition to the work being done to mitigate food waste environmental challenges, other research is focused on the use of additives in composting for process optimization.
Advancing Food Waste Utilization
Additives in Composting
Additives are diverse materials added to the feedstock or other compostable organic materials. These additives are often multifaceted in nature. Additives normally serve the purpose of (1) accelerating the composting process, (2) enhancing the finished product for its intended use (e.g., nutrient availability), (3) or both.
Biological
To decrease the time between the feedstock and mature, finished compost, biological additives can be used to accelerate the process (Wakase et al., 2008; Fan et al., 2018). Effective microorganisms, sometimes referred to as bio-inoculants, are the microorganisms that can be added to food waste composting systems and assist in the conversion of food waste into mature compost (Bolta et al., 2003; Vargas-García et al., 2005; Jurado et al., 2015). While most EM are sold as proprietary commercial solutions, an additive EM mixture often contains some combination of the following microorganisms: fungi, yeasts, lactic acid bacteria, and phototrophic bacteria (Wakase et al., 2008; Pan et al., 2012; EM Research Organization, 2016). Those microorganisms are frequently utilized for ammonia absorption (Wakase et al., 2008) or in the breakdown of lignocellulose (Jurado et al., 2015).
In 2016, Muttalib et al. (2016) published a review of the application of EM in food waste composting. Overall, the authors found that EM is a promising additive in the acceleration of food waste composting. However, the uncertainty of microbial reactions and variability in food waste compost composition was also highlighted, noting that more research is needed in the area of EM additives in diverse food waste streams. Since Muttalib et al. (2016) published this review, additional studies have been published on EM and food waste compost.
In 2017, Siddiquee et al. (2017) published a study with empty fruit branches from the oil palm industry utilizing two Trichoderma strains as additives. However, the Trichoderma strains (SICCI and 11B) were not mixed and were used as individual inocula. The laboratory-scale composting process occurred for 30 days under anaerobic conditions, which varied from most types of food waste composting as these are typically aerobic processes (Table 4). The authors concluded that this process, for both Trichoderma strains, was an effective method to dispose of empty fruit branches from the oil palm industry. However, further work is needed to determine the effectiveness of mixed green waste, such as food waste.
Manu et al. (2017) used modified composting drums to allow natural air circulation within the food waste compost and inoculated the waste with EM. The feedstock was a combination of food waste sourced from a university dining hall, and grass trimmings, leaves, and small plants were used as bulking agents. The authors used a control (drum 1), a modified drum with additional air ventilation and turning (drum 2), and a modified drum (same modifications as drum 2) with the addition of EM (drum 3). This study utilized a commercially available liquid EM solution (EM•1®) that contained Lactobacillus plantarum, L. casei, L. fermentum, L. delbrueckii, Bacillus subtilis, Saccharomyces cerevisiae, and Rhodopseudomonas palustris.
Manu et al. (2017) noted that both drum 1 and 2 resulted in active decomposition to be complete in 54 days, whereas drum 3 was completed by day 36. The study determined that active decomposition concluded when the temperature profile of the compost dipped down and remained constant at 30°C, which was the ambient temperature at the time of the study. Drum 3, with added EM, reached thermophilic (≥55°C) temperatures after 9 days of composting and peaked at ~60°C during the fifth week of decomposition, then rapidly decreased to 30°C. Importantly, extended periods at thermophilic temperatures are an effective time-temperature combination considered to be lethal for many human and plant pathogens as well as noxious weed seeds (Avery et al., 2012; Pandey et al., 2016; Manu et al., 2017). Moreover, the lignocellulosic components decomposed more efficiently in the presence of the EM inoculum, and the mature compost was composed of more fine particles (≤15 mm) in drum 3 (21%) compared to the 2 and 18% from drums 1 and 2, respectively. The fine particles are the most desirable product of composting to use as a homogenous, humus-like soil amendment (Pan et al., 2012). Manu and collaborators studied the performance of multiple composting vessels, and future research should focus on the optimization and scaling-up of food waste compost systems with the use of EM in mind.
A study by Nakasaki and Hirai (2017) utilized a yeast, Pichia kudriavzevii RB1, as a biological additive to assist in food waste degradation. This specific yeast type assists in the control of organic acids produced during the composting process (Nakasaki et al., 2013). The authors used a synthetic food waste composed of commercial rabbit food (pelleted, timothy grass meal blend) and rice with a sawdust as the bulking agent and EM. This study found that utilizing a yeast inoculum and controlling the temperature (≤40°C) for 2 days (days three and four) of composting accelerated the composting process of a “high concentration of easily degradable carbonaceous compounds” (Nakasaki and Hirai, 2017). Under these circumstances, composting is accelerated; however, the composting process often reaches temperatures that are lethal to yeast (≥55°C) and would require additional energy to cool and maintain the cooled temperature of the compost for those 2 days (Nakasaki et al., 2013; Nakasaki and Hirai, 2017). Moreover, this process was tested with a synthetic feedstock; thus further research is needed to determine its effectiveness in variable compost, such as in the presence of high fat or animal proteins.
Overall, EM is a promising avenue to accelerate the food waste composting process, but there are still many unknowns. More research utilizing authentic food waste is needed; in addition, further optimization of EM and the characterization of food waste and microbial interactions are needed. However, biological additives are not the only additives that can be optimized for food waste composting.
Organic Matter
Bulking agents such as yard trimmings or other by-products U.S. Environmental Protection Agency (EPA) (2019c) often must be added to the feedstock to enable composting via optimization of the critical factors described in the Composting subsection. The addition of organic matter to food waste as a bulking agent can help modify pH, increase porosity, decrease moisture content, and balance C/N ratios (Adhikari et al., 2009; Chang and Chen, 2010). Organic matter is often the by-product of other waste streams, as seen in Table 5.
Abdullah et al. (2013) studied the impact of different bulking agents, specifically onion peels and newspapers, on food waste composting. In their study, the authors found that the onion peels were a more suitable bulking agent when compared to the newspaper for composting household kitchen waste. This is particularly interesting because the authors also studied the use of EM and, in contrast with other studies, found that there were no significant differences in compost with EM additives when compared to compost without. The use of EM and organic additives and their interactions together in the decomposition of food waste is an area that needs further exploration.
In another study, Adhikari et al. (2009) investigated the influence of organic bulking agents on raw and cooked produce food waste compost maturation. The researchers used (1) chopped wheat (Triticum) straw, (2) chopped mature hay composed of 80% timothy (milium), and 20% clover (triphullum), and (3) pine (pinus) wood shavings as their bulking agents. For the bulking agent to be considered the most successful, the authors determined that the final compost had to meet the following criteria: (1) reach thermophilic temperatures (≥ 50°C) during its active composting stage, (2) lose the most dry and wet mass during its maturation phase, (3) degrade so that no individual particles would be recognizable, (4) have a final moisture content under 50%, and (5) hold an acceptable level of total nitrogen (≥ 2.5%), total phosphorus (≥ 0.25%), and total potassium (≥3.0%) once matured. The food waste with a 20% dry matter content of chopped wheat straw and the chopped mature hay both were successful in meeting the outlined criteria. However, the pinewood shavings did not meet multiple criteria factors such as thermophilic temperatures, particle decomposition, and nutrient levels. The authors determined that further research is needed when utilizing wood shavings in combination with other organic materials.
Other studies have used rice husk, leaves, wood shavings, sawdust, and sometimes a combination of multiple organic additives as their bulking agent (Table 4). Bulking agent selection can be directly influenced by availability; thus, if there is a specific bulking agent readily available, the composting process would need to be optimized with that in mind specifically. However, it is challenging to compare the bulking agent studies due to the differences in composter design, waste source, waste-to-organic matter ratio, environmental conditions, and other factors. With this in mind, there are many opportunities to study the influence of organic and inorganic additives in food waste composting.
Inorganic Additives
Aside from the addition of organic bulking agents and biological inoculants into the feedstock, inorganic additives are also utilized to encourage more rapid degradation into stable, mature compost or to enrich the end-product of the composting process (Barthod et al., 2018). However, those two goals are not frequently achieved together in the same compost batch. For instance, in food waste compost, the pH increases over time and becomes stable in an alkaline environment. As indicated previously, the initial low pH has been linked to malodourous compounds; therefore, to quickly increase the pH of the compost, studies have added alkaline additives, such as lime, red mud, fly ash, and calcium bentonite (Wong et al., 1997; Fang and Wong, 1999; Fang et al., 1999; An et al., 2012; Gabhane et al., 2012). However, the addition of basic additives can initially decrease the thermophilic microbial activity (Chen and Li, 2006). This initial impact on microbial activity is essential to consider due to the role microorganisms play in composting (Additives in Composting section).
For instance, a study by Gabhane et al. (2012) utilized the feedstock that was composed of sundried grass cuttings and fallen leaves and vegetable waste (1:1 ratio) and analyzed the impact of several organic and inorganic additives. The additives used include polyethylene glycol, jaggary, phosphogypsum, fly ash, and lime. The authors declared the inorganic additives were the phosphogypsum, fly ash, and lime, based on their negligible amounts of organic matter and organic carbon (Gabhane et al., 2012). However, the authors ultimately concluded that none of the inorganic additives positively influenced the composting efficiency or the quality of finished compost. Of note, Gabhane et al. (2012) did find that jaggery and polyethylene glycol improved the composting process by increasing microbial biomass and encouraging enzymatic activity. Jaggery and polyethylene glycol increased the rate of organic matter degradation and improved the quality of mature compost. Unfortunately, the authors found that the use of this additive in composting was cost-prohibitive.
An et al. (2012) also considered inorganic additives in the food waste composting process. The feedstock was composed of steamed rice, meat, potatoes, carrots, leaves, and starter culture with no inorganic additive, one additive, or two additives. The additives used in this study were coal ash and uric acid, both of which are often found in agricultural and industrial waste (An et al., 2012). The authors found that the inclusion of additives increased oxygen uptake, lowered the final C/N value, increased active composting temperatures, increased the pH, and reduced a greater amount of organic matter over time. These results were consistent with both the coal ash-only treatment and the coal ash/uric acid treatment. Interestingly, the authors also found increased thermophilic and mesophilic microbial activity in the treatments that included the coal ash-only and coal ash and uric acid amendment throughout the experiment when compared to the control. This contrasts with studies by Wong et al. (1997) and Fang et al. (1998), who found the presence of alkaline additives decreased microbial activity in the composting process. This could be the result of varying food waste feedstock composition and experimental design differences across studies, making it an imperfect comparison.
The study of additives has a far-reaching scope due to the various biological, organic, and inorganic additives that can potentially be used in the composting process, especially since many additives can be derived from under-utilized waste streams. The appropriateness of additives used is often based on the composition of the feedstock; wherein, food waste is very diverse. Considering any negative environmental impacts, such as run-off or odor, more research is needed on the application of additives in food waste composting.
Application of Alternative Technologies to Food Waste
Conventional Ensiling
Silage is the anaerobic fermentation and preservation of moist forages, such as legumes, grasses, or cereals (Adesogan and Salawu, 2004). This high moisture, fermented product is often used for livestock feed (Derbyshire et al., 1976; Eriksson and Murphy, 2004; Jaakkola et al., 2006). Ensiling these harvested crops for animal feed is not a new methodology but one that is still being improved and researched today.
Ensiling is often conducted on-site where the forage is harvested and does not necessarily require any established infrastructure aside from land, moving and packing equipment, and something to cover the silage pile (e.g., tarp). This is advantageous for producers due to its limited maintenance during the ensiling process. This process can be broken into four general phases, as adapted from Weinberg and Muck (1996) and is described in Figure 2.
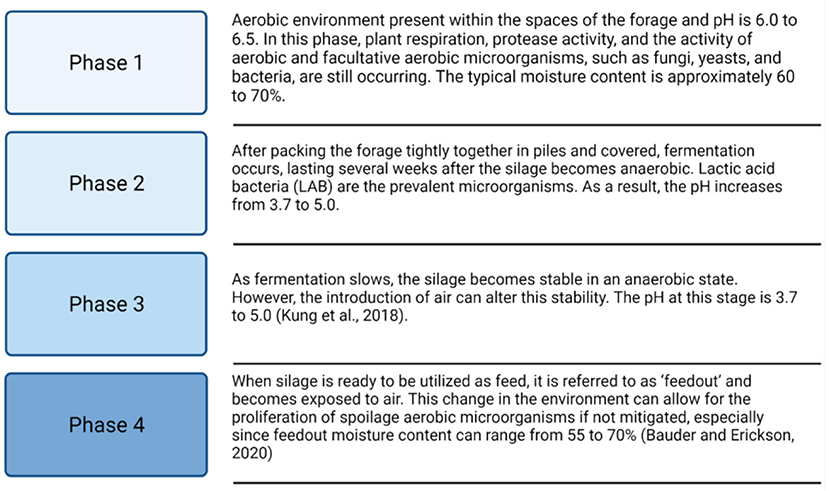
Figure 2. The phases of ensiling. Adapted from Weinberg and Muck (1996). The general four phases in the standard ensiling process of forages to silage. Created with Biorender.com.
The finished silage is ready to be utilized as livestock feed. During the feedout stage, the proliferation of aerobic microorganisms can cause spoilage in the finished silage (Inglis et al., 1999; Saarisalo et al., 2006). In order to eliminate spoilage while still fulfilling the role as feed, additives are used in the ensiling process to help fermentation as well as during preservation (Derbyshire et al., 1976; Lindgren et al., 1983; Adesogan and Salawu, 2004; Saarisalo et al., 2006; Knický and Spörndly, 2009). More recently, Muck et al. (2018) have researched and reviewed the advancements of silage additives in-depth, including microbial inoculants, chemical additives, and enzymes. While there is extensive research on the optimization of silage technologies for forage, there is limited work being done utilizing alternative feedstocks, such as food waste.
Ensiling Food Waste
As discussed in the current review, food waste is very diverse and is present in significant excess worldwide. To divert this waste from entering landfills, emerging technologies are being optimized to turn food waste into a usable by-product. There are several different ways to compost food waste into a usable soil amendment, as outlined in Table 4. However, many of these methods require extensive infrastructure, personnel, and management to turn food waste into mature compost. These required investments could possibly discourage food waste composting.
Interestingly, green silage shares some characteristics featured in food waste. With comparable initial pH (4–6), high moisture content (≥60%), starting ambient temperature, and reliance on microbial activity, the nature of composting and ensiling tend to overlap. Specifically, turned windrow composting and aerated static piles share commonalities with ensiling. Turned windrow composting relies on large piles of food waste to degrade over time but requires the mechanized turning of the piles, whereas the aerated static piles do not require mechanized turning but do necessitate some type of installed forced-air infrastructure. These aeration requirements are in stark contrast to the anaerobic ensiling process, where the proliferation of anaerobic bacteria is the driving force of fermentation and remains in covered, outdoor static piles. To reduce the costs of turned windrow and aerated static pile composting, there is value in investigating the use of ensiling science and technologies in food waste composting.
To the best of the authors' knowledge, there has not been any published research regarding the application of silage technologies to food waste composting. There are some studies specifically utilizing silage technologies in the conversion of food waste to animal feed. Nearly 40 years ago, Moon et al. (1981) observed the potential for ensiling vegetable food waste. A more recent study by Yang et al. (2006) sought to determine the effects of a lactic acid bacterium (Lactobacillus salivarius) inoculant on pulverized restaurant and bakery food waste and broiler poultry litter mixture in the conversion to swine feed. The authors found that under anaerobic conditions, 0.2% LAB on a wet basis was the optimal additive amount to convert and store fermented food waste swine feed. However, the authors did note that further studies would be needed to prove the impact with an in vivo animal feeding study. A similar study by Froetschel et al. (2014) found that ensiled food waste was a successful energy source for cattle feed.
While gaps still exist in conventional silage technology, even more gaps are present in research regarding the ensiling potential of food waste. Food waste is an issue that is pervasive in every community, and applying existing science and technology, such as ensiling techniques, is an option to divert food waste from landfills. This helps improve both conventional and non-traditional ensiling techniques while generating economically valuable food waste by-products.
Discussion
Future Research
Society and the food industry continue to evolve. In-depth, accelerated data collection, streamlined food recovery plans, and food waste-preventive laws are desirable for regulatory agencies, food processors, and the public. The benefits of accurate food waste data help identify weaknesses in the food chain, from the food manufacturer to the consumer environment. Presently, data indicate that much of the food waste occurring in developed countries is at the retail and consumer levels (Rohm et al., 2017; Welch et al., 2018; Aschemann-Witzel et al., 2019; Yu and Jaenicke, 2020). Besides future sweeping rules and regulations, much of the food waste initiatives are implemented by local governments, nonprofit organizations, and the food industry as there are limited federal regulations. In order for these entities to handle food waste efficiently, more food waste research is needed.
On the food industry and retail scales, the manner in which the public interacts with food is constantly evolving. With more retailers now offering online shopping for convenience, curbside grocery pick-up and grocery delivery services (e.g., Instacart; San Francisco, CA) are becoming more prevalent. With the increase in popularity of online grocery shopping, questions regarding the impact of online shopping on consumer choices have arisen. For instance, absent from meandering the aisles of a retailer, does a consumer make less impulsive grocery choices when shopping online? Or do consumers over-purchase items that are based on the scale, such as meat or some produce? In addition to behavioral research regarding online grocery shopping, research is needed based on what occurs after the groceries are brought home. Does purposeful grocery shopping in an online purchasing model reduce food waste in the home? As the food retail environments change, more research is needed to understand the motivation and impact of consumer decisions and how it impacts food waste generation.
Food waste generation will continue to occur at some level, especially in consumer environments. Efforts to divert food waste from landfills are of the utmost importance. This diversion of food waste likely will result in valuable by-products, such as animal feed and soil amendments. Another option is by diverting wasted food by-products and utilizing them as functional ingredients in human food (Lehmann, 2021). One of the challenging factors in diverting food waste, particularly retail and consumer waste, is that it comes from a variety of sources. There is a gap in the literature on the characterization of food waste. As discussed in the present review, moisture, pH, C/N ratio, and bulking agents are all critical factors in food waste processing, whether for animal feed or otherwise. To better optimize how diverted food waste is processed, studies are needed to further characterize what types of food products are being wasted. From this information, other questions arise, such as is there a seasonality aspect to food waste? Do seasonal changes impact what comprises food waste, and how do the changes impact the aforementioned critical factors? Moreover, in the U.S., research is needed to investigate if there are quantifiable differences based on the region. Although considerable strides have been made to quantify how much food waste is being generated, more work needs to be done.
In addition, as described in this review, the transformation from feedstocks to mature compost is a challenge. The compost system, the feedstock, bulking agents, and additives utilized all impact the efficiency and quality of the mature compost. It is challenging to compare the composting systems since the methodologies rely on varying metabolic activities (e.g., vermi-composting compared to turned windrow). There will likely never be a one-size-fits-all system when it comes to food waste composting, but more research is needed. One area in the literature that is frequently overlooked is mature compost quality. Frequently, as reviewed, many studies are attempting to optimize the food waste composting process and less so the mature end-product. Moreover, in doing so, the success of some composting additives is the absence of cost analysis. Further research must consider the cost of infrastructure and additives when conducting food waste composting research and future, practical applications. For food waste research to become applicable outside of the laboratory, efficiency, quality, and cost must all be considered. This area of research would highly benefit from a systematic literature review; however, researchers should proceed with caution given the variety of bulking agents and feedstock compositions utilized.
As researchers look to optimize the food waste composting process, a mostly unexplored avenue is the application of alternative, existing technologies, such as ensiling. The anaerobic fermentation of forages is a process that can occur in large quantities and uses additives similar to composting to create an economically viable product. Ensiling and additives have been used for decades, and as such, the available research is extensive (Muck et al., 2018). Even so, there is a lack of research using this technology to process food waste into mature compost. As described in the present review, ensiling requires less labor and infrastructure than many food waste composting methods and is an area of great potential for food waste composting that warrants further investigation.
As food waste concerns mount with a growing global population, innovative methods to cope with food waste generation become a necessity. Food waste studies with the inclusion of characterized food waste data, consumer behaviors, optimization of by-product methods, attention to by-product quality, or application of existing technologies are crucial data for the advancement of food waste reduction programs. As these data are released by non-governmental organizations, governmental, and peer-reviewed publications, federal, state, and local agencies can create informed legislation regarding the reduction of food waste.
Conclusions and Recommendations
Studies of quantifying and utilizing food waste vary significantly throughout the literature. The variability in results likely exists due to the many factors outlined in this review, including, but not limited to, food waste producer, the volume of waste, location, size of the study, and treatment of waste. For instance, many food waste studies focus on composting efficiency using homogenous, synthetic food waste inputs in a laboratory-scale environment with minimal focus on compost quality or cost. For this reason, researchers may have difficulty choosing the most applicable variables for a study due to the range of food waste characteristics, additives, and methods used across the literature. Moreover, those in a community setting who are looking to reduce food waste may have even greater difficulty identifying the most applicable method for their community or household.
The following recommendations are based in the current review to aid researchers in moving forward on the management and utilization of food waste:
• Systematic evaluation of consumer behaviors should be investigated in the online grocery purchasing model.
• Food waste needs further compositional characterization on a seasonal and regional level.
• Food waste composting studies must utilize community food waste rather than synthetic, designed food waste.
• Food waste composting research must consider the cost and efficiency of additives.
• Application of existing technologies needs to be investigated in the transformation from feedstock (e.g., green food waste) to mature by-products.
Author Contributions
SJ: conceptualization, writing—original draft, writing—review, editing, and visualization. KG: resources, writing—review, editing, supervision, and funding acquisition. SR: resources, writing—review, editing, supervision, project administration, and funding acquisition. All authors contributed to the article and approved the submitted version.
Funding
This work was supported in part by the National Institute of Food and Agriculture (NIFA), U.S. Department of Agriculture (USDA) Hatch Act.
Conflict of Interest
The authors declare that the research was conducted in the absence of any commercial or financial relationships that could be construed as a potential conflict of interest.
Publisher's Note
All claims expressed in this article are solely those of the authors and do not necessarily represent those of their affiliated organizations, or those of the publisher, the editors and the reviewers. Any product that may be evaluated in this article, or claim that may be made by its manufacturer, is not guaranteed or endorsed by the publisher.
References
143 Cong. Rec. S9532. (1996). Statement of Sen. Santorum. Daily Ed. Aug. 2, 1996. Available online at: https://www.congress.gov/104/crec/1996/08/02/CREC-1996-08-02-pt1-PgS9532.pdf (accessed August 5, 2020).
Abarghuei, M. J., Rouzbehan, Y., Salem, A. Z. M., and Zamiri, M. J. (2014). Nitrogen balance, blood metabolites and milk fatty acid composition of dairy cows fed pomegranate-peel extract. Livest. Sci. 164, 72–80. doi: 10.1016/j.livsci.2014.03.021
Abdel-Hafeez, H., Saleh, E., Tawfeek, S., Youssef, I., and Abdel-Daim, A. (2018). Utilization of potato peels and sugar beet pulp with and without enzyme supplementation in broiler chicken diets: effects on performance, serum biochemical indices and carcass traits. J. Anim. Physiol. Anim. Nutr. 102, 56–66. doi: 10.1111/jpn.12656
Abdullah, N., Chin, N. L., Mokhtar, M. N., and Taip, F. S. (2013). Effects of bulking agents, load size or starter cultures in kitchen-waste composting. Int. J. Recycl. Org. Waste Agric. 2:3. doi: 10.1186/2251-7715-2-3
Aboulam, S., Morvan, B., and Revel, J. C. (2006). Use of a rotating-drum pilot plant to model the composting of household waste on an industrial scale. Compost. Sci. Util. 14, 184–190. doi: 10.1080/1065657X.2006.10702282
Adesogan, A. T., and Salawu, M. B. (2004). Effect of applying formic acid, heterolactic bacteria or homolactic and heterolactic bacteria on the fermentation of bi-crops of peas and wheat. J. Sci. Food Agric. 84, 983–992. doi: 10.1002/jsfa.1745
Adhikari, B. K., Barrington, S., Martinez, J., and King, S. (2009). Effectiveness of three bulking agents for food waste composting. Waste Manag. 29, 197–203. doi: 10.1016/j.wasman.2008.04.001
Ajanovic, A. (2010). Biofuels versus food production: Does biofuels production increase food prices? Energy 36, 2070–2076. doi: 10.1016/j.energy.2010.05.019
An, C.-J., Huang, G.-H., Yao, Y., Sun, W., and An, K. (2012). Performance of in-vessel composting of food waste in the presence of coal ash and uric acid. J. Hazard. Mater. 203–204, 38–45. doi: 10.1016/j.jhazmat.2011.11.066
Angulo, J., Mahecha, L., Yepes, S. A., Yepes, A. M., Bustamante, G., Jaramillo, H., et al. (2012a). Quantitative and nutritional characterization of fruit and vegetable waste from marketplace: a potential use as bovine feedstuff? J. Environ. Manage. 95 Suppl, S203–209. doi: 10.1016/j.jenvman.2010.09.022
Angulo, J., Mahecha, L., Yepes, S. A., Yepes, A. M., Bustamante, G., Jaramillo, H., et al. (2012b). Nutritional evaluation of fruit and vegetable waste as feedstuff for diets of lactating Holstein cows. J. Environ. Manage. 95, S210–S214. doi: 10.1016/j.jenvman.2011.06.050
Annison, G. (1993). The role of wheat non-starch polysaccharides in broiler nutrition. Aust. J. Agric. Res. 44, 405–422. doi: 10.1071/AR9930405
Aschemann-Witzel, J., Giménez, A., and Ares, G. (2019). Household food waste in an emerging country and the reasons why: consumer's own accounts and how it differs for target groups. Resour. Conserv. Recycl. 145, 332–338. doi: 10.1016/j.resconrec.2019.03.001
Avery, L. M., Booth, P., Campbell, C., Tompkins, D., and Hough, R. L. (2012). Prevalence and survival of potential pathogens in source-segregated green waste compost. Sci. Total. Environ. 431, 128–138. doi: 10.1016/j.scitotenv.2012.05.02
Awasthi, S. K., Sarsaiya, S., Awasthi, M. K., Liu, T., Zhao, J., Kumar, S., et al. (2020). Changes in global trends in food waste composting: Research challenges and opportunities. Bioresour. Technol. 299:122555. doi: 10.1016/j.biortech.2019.122555
Barco, H., Oribe-Garcia, I., Vargas-Viedma, M. V., Borges, C. E., Martín, C., and Alonso-Vicario, A. (2019). New methodology for facilitating food wastage quantification. Identifying gaps and data inconsistencies. J. Environ. Manage.234, 512–524. doi: 10.1016/j.jenvman.2018.11.037
Barthod, J., Rumpel, C., and Dignac, M.-F. (2018). Composting with additives to improve organic amendments. A review. Agron. Sustain. Dev. 38, 17. doi: 10.1007/s13593-018-0491-9
Bautista, L. F., Vicente, G., Rodríguez, R., and Pacheco, M. (2009). Optimisation of FAME production from waste cooking oil for biodiesel use. Biomass Bioenergy 33, 862–872. doi: 10.1016/j.biombioe.2009.01.009
Behera, S. K., Park, J. M., Kim, K. H., and Park, H.-S. (2010). Methane production from food waste leachate in laboratory-scale simulated landfill. Waste Manag. 30, 1502–1508. doi: 10.1016/j.wasman.2010.02.028
Bellemare, M. F., Çakir, M., Peterson, H. H., Novak, L., and Rudi, J. (2017). On the measurement of food waste. Am. J. Agric. Econ. 99, 1148–1158. doi: 10.1093/cce/aax034
Blazek, K., Balkus, O., Leib, E. B., Civita, N., Maley, M., Nuckolls, K., et al. (2016). Federal enhanced tax deduction for food donation—a legal guide. Available online at: https://www.chlpi.org/wp-content/uploads/2013/12/Food-Donation-Fed-Tax-Guide-for-Pub-2.pdf (accessed February 7, 2020).
Bolta, S. V., Mihelic, R., Lobnik, F., and Lestan, D. (2003). Microbial community structure during composting with and without mass inocula. Compost. Sci. Util. 11, 6–15. doi: 10.1080/1065657X.2003.10702104
Bouallagui, H., Cheikh, R. B., Marouani, L., and Hamdi, M. (2003). Mesophilic biogas production from fruit and vegetable waste in a tubular digester. Bioresour. Technol. 86, 85–89. doi: 10.1016/S0960-8524(02)00097-4
Bruno, P., Caselli, M., de Gennaro, G., Solito, M., and Tutino, M. (2007). Monitoring of odor compounds produced by solid waste treatment plants with diffusive samplers. Waste Manag. 27, 539–544. doi: 10.1016/j.wasman.2006.03.006
Business for Social Responsibility™ (BSR) (2013). Analysis of U.S. Food Waste Among Food Manufacturers, Retailers, and Wholesalers—Prepared for the Food Waste Reduction Alliance. Available online at: https://www.foodwastealliance.org/wp-content/uploads/2013/06/FWRA_BSR_Tier2_FINAL.pdf (accessed May 12, 2020).
Buzby, J. C., Farah-Wells, H., and Hyman, J. (2014). The estimated amount, value, and calories of postharvest food losses at the retail and consumer levels in the United States. USDA-ERS Econ. Inf. Bull. 121:39. doi: 10.2139/ssrn.2501659
Buzby, J. C., Hyman, J., Stewart, H., and Wells, H. F. (2011). The value of retail- and consumer-level fruit and vegetable losses in the United States. J. Consumer Aff. 45, 492–515. doi: 10.1111/j.1745-6606.2011.01214.x
Cekmecelioglu, D., Demirci, A., and Graves, R. E. (2005). Feedstock optimization of in-vessel food waste composting systems for inactivation of pathogenic microorganisms. J. Food Prot. 68, 589–596.
Chaboud, G., and Daviron, B. (2017). Food losses and waste: Navigating the inconsistencies. Glob. Food Sec. 12, 1–7. doi: 10.1016/j.gfs.2016.11.004
Chaher, N. E. H., Chakchouk, M., Engler, N., Nassour, A., Nelles, M., and Hamdi, M. (2020). Optimization of food waste and biochar in-vessel co-composting. Sustainability 12:1356. doi: 10.3390/su12041356
Champions 12.3. (2020). World's Leading Food Retailers and Providers Engage Nearly 200 Suppliers in Cutting Food Loss and Waste in Half (Press release). Available online at: www.Champions123.org (accessed September 10, 2021).
Chang, J. I., and Chen, Y. J. (2010). Effects of bulking agents on food waste composting. Bioresour. Technol. 101, 5917–5924. doi: 10.1016/j.biortech.2010.02.042
Chang, J. I., and Hsu, T.-E. (2008). Effects of compositions on food waste composting. Bioresour. Technol. 99, 8068–8074. doi: 10.1016/j.biortech.2008.03.043
Chen, C. R., and Chen, R. J. C. (2018). Using two government food waste recognition programs to understand current reducing food loss and waste activities in the U.S. Sustainability 10:2760. doi: 10.3390/su10082760
Chen, J., and Li, Y. (2006). Coal fly ash as an amendment to container substrate for Spathiphyllum production. Bioresour. Technol. 97, 1920–1926. doi: 10.1016/j.biortech.2005.08.009
Chesson, A. (2001). Non-starch polysaccharide degrading enzymes in poultry diets: influence of ingredients on the selection of activities. World Poultry Sci. J. 57, 251–263. doi: 10.1079/WPS20010018
Chikae, M., Ikeda, R., Kerman, K., Morita, Y., and Tamiya, E. (2006). Estimation of maturity of compost from food wastes and agro-residues by multiple regression analysis. Bioresour. Technol. 97, 1979–1985. doi: 10.1016/j.biortech.2005.09.026
Connor, J. M., and Schiek, W. A. (1997). Food Processing: An Industrial Powerhouse in Transition. Hoboken, NJ: John Wiley and Sons, Inc.
Consumer Brands Association (2017). Best if Clearly Labeled: How the Consumer Packaged Goods Industry is Reducing Confusion and Food Waste. Available online at: https://consumerbrandsassociation.org/wpcontent/uploads/2019/11/ConsumerBrands_ClearlyLabeled.pdf (accessed July 27, 2020).
Cuéllar, A. D., and Webber, M. E. (2010). Wasted food, wasted energy: the embedded energy in food waste in the United States. Environ. Sci. Technol. 44, 6464–6469. doi: 10.1021/es100310d
Das, N. G., Huque, K. S., Amanullah, S. M., and Makkar, H. P. S. (2019). Feeding of processed vegetable wastes to bulls and its potential environmental benefit. Anim. Nutr. 5, 87–94. doi: 10.1016/j.aninu.2018.04.002
Day, D. (2012). Beyond Net Zero. Treatment Plant Operator. https://www.tpomag.com/editorial/2012/12/beyond_net_zero (accessed July 1, 2020).
de Evan, T., Vintimilla, A., Molina-Alcaide, E., Ranilla, M. J., and Carro, M. D. (2020). Potential of recycling cauliflower and romanesco wastes in ruminant feeding: In vitro studies. Animals 10:1247. doi: 10.3390/ani10081247
de Hooge, I. E., Oostindjer, M., Aschemann-Witzel, J., Normann, A., Loose, S. M., and Almli, V. L. (2017). This apple is too ugly for me!: Consumer preferences for suboptimal food products in the supermarket and at home. Food. Qual. Prefer. 56, 80–92. doi: 10.1016/j.foodqual.2016.09.012
Demirbas, A. (2007). Importance of biodiesel as transportation fuel. Energy Policy 35, 4661–4670. doi: 10.1016/j.enpol.2007.04.003
Demirbas, A. (2009). Progress and recent trends in biodiesel fuels. Energy Convers. Manag. 50, 14–34. doi: 10.1016/j.enconman.2008.09.001
Derbyshire, J. C., Gordon, C. H., and Waldo, D. R. (1976). Formic acid as a silage preservative for milking cows. J. Dairy Sci. 59, 278–287. doi: 10.3168/jds.S0022-0302(76)84195-1
D'Imporzano, G., Crivelli, F., and Adani, F. (2008). Biological compost stability influences odor molecules production measured by electronic nose during food-waste high-rate composting. Sci. Total Environ. 402, 278–284. doi: 10.1016/j.scitotenv.2008.04.053
EM Research Organization (2016). How EM Works. Available online at: https://www.emrojapan.com/how/ (accessed June 23 2020).
Eriksson, T., and Murphy, M. (2004). Ruminal digestion of leguminous forage, potatoes and fodder beets in batch culture: I. Fermentation pattern. Anim. Feed Sci. Technol. 111, 73–88. doi: 10.1016/j.anifeedsci.2003.05.001
Ermolaev, E., Jarvis, Å., Sundberg, C., Smårs, S., Pell, M., and Jönsson, H. (2015). Nitrous oxide and methane emissions from food waste composting at different temperatures. Waste Manag. 46, 113–119. doi: 10.1016/j.wasman.2015.08.021
Fagbohungbe, M. O., Herbert, B. M. J., Hurst, L., Li, H., Usmani, S. Q., and Semple, K. T. (2016). Impact of biochar on the anaerobic digestion of citrus peel waste. Bioresour. Technol. 216, 142–149. doi: 10.1016/j.biortech.2016.04.106
Fan, Y. V., Klemeš, J. J., Lee, C. T., and Ho, C. S. (2018). Efficiency of microbial inoculation for a cleaner composting technology. Clean Technol. Environ. Policy 20, 517–527. doi: 10.1007/s10098-017-1439-5
Fang, M., and Wong, J. W. C. (1999). Effects of lime amendment on availability of heavy metals and maturation in sewage sludge composting. Environ. Pollut. 106, 83–89. doi: 10.1016/S0269-7491(99)00056-1
Fang, M., Wong, J. W. C., Li, G. X., and Wong, M. H. (1997). Effect of coal ash residues on the microbiology of sewage sludge composting. Stud. Environ. Sci. 66, 511–523. doi: 10.1016/S0166-1116(97)80067-2
Fang, M., Wong, J. W. C., Li, G. X., and Wong, M. H. (1998). Changes in biological parameters during co-composting of sewage sludge and coal ash residues. Bioresour. Technol. 64, 55–61. doi: 10.1016/S0960-8524(97)00156-9
Fang, M., Wong, J. W. C., Ma, K. K., and Wong, M. H. (1999). Co-composting of sewage sludge and coal fly ash: nutrient transformations. Bioresour. Technol. 67, 19–24. doi: 10.1016/S0960-8524(99)00095-4
Filimonau, V., Todorova, E., Mzembe, A., Sauer, L., and Yankholmes, A. (2020). A comparative study of food waste management in full service restaurants of the United Kingdom and the Netherlands. J. Clean Prod. 258:120775. doi: 10.1016/j.jclepro.2020.120775
Flanagan, K., Lipinski, B., and Goodwin, L. (2019). SDG Target 12.3 on Food Loss and Waste: 2019 Progress Report. Available online at: https://champions123.org/wp-content/uploads/2019/09/champions-12-3-2019-progress-report.pdf (accessed March 7, 2020).
Food Agriculture Organization of the United Nations (FAO). (2019a). Food Loss Index. Online statistical working system for loss calculations. http://www.fao.org/food-loss-and-food-waste/flw-data (accessed February 4, 2020).
Food Agriculture Organization of the United Nations (FAO) (2019b). The State of Food and Agriculture 2019. Moving Forward on Food Loss and Waste Reduction. http://www.fao.org/3/ca6030en/ca6030en.pdf (accessed January 20, 2020).
Food Agriculture Organization of the United Nations (FAO). (2021). Food Loss and Food Waste. http://www.fao.org/food-loss-and-food-waste/flw-data (accessed August 18 2021).
Froetschel, M. A., Ross, C. L., Stewart, R. L. Jr., Azain, M. J., Michot, P., and Rekaya, R. (2014). Nutritional value of ensiled grocery food waste for cattle. J. Anim. Sci. 92, 5124–5133. doi: 10.2527/jas.2014-8126
Gabhane, J., William, S. P. M. P., Bidyadhar, R., Bhilawe, P., Anand, D., Vaidya, A. N., et al. (2012). Additives aided composting of green waste: Effects on organic matter degradation, compost maturity, and quality of the finished compost. Bioresour. Technol. 114, 382–388. doi: 10.1016/j.biortech.2012.02.040
Gallego, E., Roca, F. J., Perales, J. F., Sánchez, G., and Esplugas, P. (2012). Characterization and determination of the odorous charge in the indoor air of a waste treatment facility through the evaluation of volatile organic compounds (VOCs) using TD–GC/MS. Waste Manag. 32, 2469–2481. doi: 10.1016/j.wasman.2012.07.010
Garcia, A. J., Esteban, M. B., Márquez, M. C., and Ramos, P. (2005). Biodegradable municipal solid waste: characterization and potential use as animal feedstuffs. Waste Manag. 25, 780–787. doi: 10.1016/j.wasman.2005.01.006
Garrone, P., Melacini, M., Perego, A., and Sert, S. (2016). Reducing food waste in food manufacturing companies. J. Clean Prod. 137, 1076–1085. doi: 10.1016/j.jclepro.2016.07.145
Gong, Z., Yi-Fan Su, L., Shiyue Zhang, J., Chen, T., and Wang, Y.-C. (2021). Understanding the association between date labels and consumer-level food waste. Food Qual. Prefer. 2021:104373. doi: 10.1016/j.foodqual.2021.104373
Goodman-Smith, F., Mirosa, M., and Skeaff, S. (2020). A mixed-methods study of retail food waste in New Zealand. Food Policy 92:101845. doi: 10.1016/j.foodpol.2020.101845
Griffin, M., Sobal, J., and Lyson, T. A. (2009). An analysis of a community food waste stream. Agr. Hum. Val. 26, 67–81. doi: 10.1007/s10460-008-9178-1
Guidoni, L. L. C., Marques, R. V., Moncks, R. B., Botelho, F. T., da Paz, M. F., Corrêa, L. B., et al. (2018). Home composting using different ratios of bulking agent to food waste. J. Environ. Manage 207, 141–150. doi: 10.1016/j.jenvman.2017.11.031
Gujer, W., and Zehnder, A. J. (1983). Conversion processes in anaerobic digestion. Water Sci. Technol. 15, 127–167. doi: 10.2166/wst.1983.0164
Guo, R., Li, G., Jiang, T., Schuchardt, F., Chen, T., Zhao, Y., et al. (2012). Effect of aeration rate, C/N ratio and moisture content on the stability and maturity of compost. Bioresour. Technol. 112, 171–178.
Gustavsson, J., Cederberg, C., Sonesson, U., van Otterdijk, R., and Meybeck, A. (2011). Global Food Losses and Food Waste: Extent, Causes and Prevention. Available online at: http://www.fao.org/3/a-i2697e.pdf (accessed January 14, 2020).
Hackes, B. L., Shanklin, C. W., Kim, T., and Su, A. Y. (1997). Tray service generates more food waste in dining areas of a continuing-care retirement community. J. Am. Diet Assoc. 97, 879–882. doi: 10.1016/S0002-8223(97)00213-7
Haley, J. (2013). The Legal Guide to the Bill Emerson Good Samaritan Food Donation Act. University of Arkansas School of Law. http://media.law.uark.edu/arklawnotes/2013/08/08/the-legal-guide-to-the-bill-emerson-good-samaritan-food-donation-act/ (accessed March 14, 2020).
Harrington, J. M., Myers, R. A., and Rosenberg, A. A. (2005). Wasted fishery resources: discarded by-catch in the USA. Fish. Fish. 6, 350–361. doi: 10.1111/j.1467-2979.2005.00201.x
Heida, H., Bartman, F., and van der Zee, S. C. (1995). Occupational exposure and indoor air quality monitoring in a composting facility. Am. Ind. Hyg. Assoc. J. 56, 39–43. doi: 10.1080/15428119591017295
Heo, H. S., Kim, S. G., Jeong, K.-E., Jeon, J.-K., Park, S. H., Kim, J. M., et al. (2011). Catalytic upgrading of oil fractions separated from food waste leachate. Bioresour. Technol. 102, 3952–3957. doi: 10.1016/j.biortech.2010.11.099
Hossain, A. S., Salleh, A., Boyce, A. N., Chowdhury, P., and Naqiuddin, M. (2008). Biodiesel fuel production from algae as renewable energy. Am. J. Biochem. Biotechnol. 4, 250–254. doi: 10.3844/ajbbsp.2008.250.254
Huet, J., Druilhe, C., Trémier, A., Benoist, J. C., and Debenest, G. (2012). The impact of compaction, moisture content, particle size and type of bulking agent on initial physical properties of sludge-bulking agent mixtures before composting. Bioresour. Technol. 114, 428–436. doi: 10.1016/j.biortech.2012.03.031
Inglis, G. D., Yanke, L. J., Kawchuk, L. M., and McAllister, T. A. (1999). The influence of bacterial inoculants on the microbial ecology of aerobic spoilage of barley silage. Can. J. Microbiol. 45, 77–87. doi: 10.1139/w98-207
Jaakkola, S., Rinne, M., and Heikkilä, T. (2006). Effects of restriction of silage fermentation with formic acid on milk production. Agric. Food Sci. 15, 200–218.
Jackson, D., Rosales-Guevara, L., and Blake, R. (2014). Environmental odors web site: Providing communities and health officials with the tools to address odor issues. J. Environ. Health 77, 38–39. Available online at: https://www.ncbi.nlm.nih.gov/pmc/articles/PMC4561993/ (accessed May 14, 2020).
Janssen, A. M., Nijenhuis-de Vries, M. A., Boer, E. P. J., and Kremer, S. (2017). Fresh, frozen, or ambient food equivalents and their impact on food waste generation in Dutch households. Waste Manag. 67, 298–307. doi: 10.1016/j.wasman.2017.05.010
Jurado, M. M., Suárez-Estrella, F., López, M. J., Vargas-García, M. C., López-González, J. A., and Moreno, J. (2015). Enhanced turnover of organic matter fractions by microbial stimulation during lignocellulosic waste composting. Bioresour. Technol. 186, 15–24. doi: 10.1016/j.biortech.2015.03.059
Kalamdhad, A. S., Singh, Y. K., Ali, M., Khwairakpam, M., and Kazmi, A. A. (2009). Rotary drum composting of vegetable waste and tree leaves. Bioresour. Technol. 100, 6442–6450. doi: 10.1016/j.biortech.2009.07.030
Karmee, S., Chandna, D., Ravi, R., and Chadha, A. (2006). Kinetics of base-catalyzed transesterification of triglycerides from Pongamia oil. J. Am. Oil. Chem. Soc. 83, 873–877. doi: 10.1007/s11746-006-5040-z
Karmee, S., Lindardi, D., Lee, J., and Lin, C. S. K. (2015a). Conversion of lipid from food waste to biodiesel. Waste Manag. 41,169–173. doi: 10.1016/j.wasman.2015.03.025
Karmee, S., Patria, R., and Lin, C. (2015b). Techno-economic evaluation of biodiesel production from waste cooking oil—a case study of Hong Kong. Int. J. Mol. Sci. 16, 4362–4371. doi: 10.3390/ijms16034362
Khempaka, S., Molee, W., and Guillaume, M. (2009). Dried cassava pulp as an alternative feedstuff for broilers: effect on growth performance, carcass traits, digestive organs, and nutrient digestibility. J. Appl. Poult. Res. 18, 487–493. doi: 10.3382/japr.2008-00124
Kim, K.-H., Pal, R., Ahn, J.-W., and Kim, Y. H. (2009). Food decay and offensive odorants: a comparative analysis among three types of food. Waste Manag. 29, 1265–1273. doi: 10.1016/j.wasman.2008.08.029
Knický, M., and Spörndly, R. (2009). Sodium benzoate, potassium sorbate and sodium nitrite as silage additives. J. Sci. Food. Agric. 89, 2659–2667. doi: 10.1002/jsfa.3771
Koivula, N., Räikkönen, T., Urpilainen, S., Ranta, J., and Hänninen, K. (2004). Ash in composting of source-separated catering waste. Bioresour. Technol. 93, 291–299. doi: 10.1016/j.biortech.2003.10.025
Koivupuro, H.-K., Hartikainen, H., Silvennoinen, K., Katajajuuri, J.-M., Heikintalo, N., Reinikainen, A., et al. (2012). Influence of socio-demographical, behavioural and attitudinal factors on the amount of avoidable food waste generated in Finnish households. Int. J. Consum. Stud. 36, 183–191. doi: 10.1111/j.1470-6431.2011.01080.x
Kosa, K. M., Cates, S. C., Karns, S., Godwin, S. L., and Chambers, D. (2007). Consumer knowledge and use of open dates: results of a web-based survey. J. Food Prot. 70, 1213–1219. doi: 10.4315/0362-028X-70.5.1213
Kumar, M., Ou, Y.-L., and Lin, J.-G. (2010). Co-composting of green waste and food waste at low C/N ratio. Waste Manag. 30, 602–609. doi: 10.1016/j.wasman.2009.11.023
Le Man, H., Behera, S. K., and Park, H. S. (2010). Optimization of operational parameters for ethanol production from Korean food waste leachate. Int. J. Environ. Sci. Te. 7, 157–164. doi: 10.1007/BF03326127
Lebersorger, S., and Schneider, F. (2014). Food loss rates at the food retail, influencing factors and reasons as a basis for waste prevention measures. Waste Manag. 34:1911. doi: 10.1016/j.wasman.2014.06.013
Lehmann, E. (2021). Future Protein Mission: Growing Protein to Feed a Growing World. Available online at: https://blog.csiro.au/future-protein-mission/?utm_medium=emailandutm_source=rasa_ioandPostID=37868324andMessageRunDetailID=6455394536 (accessed September 10, 2021).
Lehmann, L. (2015). The Garbage Project Revisited: from a 20th century archaeology of food waste to a contemporary study of food packaging waste. Sustainability 7, 6994–7010. doi: 10.3390/su7066994
Li, Z., Huang, G., Yu, H., Zhou, Y., and Huang, W. (2015). Critical factors and their effects on product maturity in food waste composting. Environ. Monit. Assess. 187:217. doi: 10.1007/s10661-015-4430-9
Li, Z., Lu, H., Ren, L., and He, L. (2013). Experimental and modeling approaches for food waste composting: a review. Chemosphere 93, 1247–1257. doi: 10.1016/j.chemosphere.2013.06.064
Lindgren, S., Kaspersson, A., Rydberg, E., Lingvall, P., and Kartzow, A. D. (1983). Effect of inoculants, grain and formic acid on silage fermentation. Swed. J. Agric. Res. 13, 91–100.
Liu, C., Hotta, Y., Santo, A., Hengesbaugh, M., Watabe, A., Totoki, Y., et al. (2016). Food waste in Japan: trends, current practices and key challenges. J. Clean Prod. 133, 557–564. doi: 10.1016/j.jclepro.2016.06.026
Loke, M. K., and Leung, P. (2015). Quantifying food waste in Hawaii's food supply chain. Waste Manag. Res. 33:1076. doi: 10.1177/0734242x15607427
Ma, F., and Hanna, M. A. (1999). Biodiesel production: a review. Bioresour. Technol. 70, 1–15. doi: 10.1016/S0960-8524(99)00025-5
Makkar, H. P. S., and Ankers, P. (2014). Towards sustainable animal diets: a survey-based study. Anim. Feed Sci. Technol. 198, 309–322. doi: 10.1016/j.anifeedsci.2014.09.018
Malakahmad, A., Idrus, N. B., Abualqumboz, M. S., Yavari, S., and Kutty, S. R. M. (2017). In-vessel co-composting of yard waste and food waste: an approach for sustainable waste management in Cameron Highlands, Malaysia. Int. J. Recycl. Org. Waste Agric. 6, 149–157. doi: 10.1007/s40093-017-0163-9
Manu, M. K., Kumar, R., and Garg, A. (2017). Performance assessment of improved composting system for food waste with varying aeration and use of microbial inoculum. Bioresour. Technol. 234, 167–177. doi: 10.1016/j.biortech.2017.03.023
Mao, I. F., Tsai, C.-J., Shen, S.-H., Lin, T.-F., Chen, W.-K., and Chen, M.-L. (2006). Critical components of odors in evaluating the performance of food waste composting plants. Sci. Total Environ. 370, 323–329. doi: 10.1016/j.scitotenv.2006.06.016
Marchetti, J. M., Miguel, V. U., and Errazu, A. F. (2008). Techno-economic study of different alternatives for biodiesel production. Fuel Process Technol. 89, 740–748. doi: 10.1016/j.fuproc.2008.01.007
Márquez, M. A., Diánez, F., and Camacho, F. (2010). The use of vegetable subproducts from greenhouses (VSG) for animal feed in the Poniente region of Almería. Renew. Agr. Food Syst. 26, 4–12. doi: 10.1017/S1742170510000013
Martin, D. S., Ramos, S., and Zufía, J. (2016). Valorisation of food waste to produce new raw materials for animal feed. Food Chem. 198, 68–74. doi: 10.1016/j.foodchem.2015.11.035
Maulini-Duran, C., Artola, A., Font, X., and Sánchez, A. (2014). Gaseous emissions in municipal wastes composting: effect of the bulking agent. Bioresour. Technol. 172, 260–268. doi: 10.1016/j.biortech.2014.09.041
Mena, C., Adenso-Diaz, B., and Yurt, O. (2011). The causes of food waste in the supplier–retailer interface: evidences from the UK and Spain. Resour. Conserv. Recycl. 55, 648–658. doi: 10.1016/j.resconrec.2010.09.006
Meyer-Kohlstock, D., Haupt, T., Heldt, E., Heldt, N., and Kraft, E. (2016). Biochar as additive in biogas-production from bio-waste. Energies 9:247. doi: 10.3390/en9040247
Milán, Z., Sánchez, E., Weiland, P., Borja, R., Martin, A., and Ilangovan, K. (2001). Influence of different natural zeolite concentrations on the anaerobic digestion of piggery waste. Bioresour. Technol. 80, 37–43. doi: 10.1016/S0960-8524(01)00064-5
Mo, W. Y., Cheng, Z., Choi, W. M., Man, Y. B., Liu, Y., and Wong, M. H. (2014). Application of food waste based diets in polyculture of low trophic level fish: Effects on fish growth, water quality and plankton density. Mar. Pollut. Bull. 85, 803–809. doi: 10.1016/j.marpolbul.2014.01.020
Mohee, R., Boojhawon, A., Sewhoo, B., Rungasamy, S., Somaroo, G. D., and Mudhoo, A. (2015). Assessing the potential of coal ash and bagasse ash as inorganic amendments during composting of municipal solid wastes. J. Environ. Manage. 159, 209–217. doi: 10.1016/j.jenvman.2015.05.008
Moon, N. J., Ely, L. O., and Sudweeks, E. M. (1981). Effect of inoculation of vegetable processing wastes with Lactobacillus plantarum on silage fermentation. J. Sci. Food Agric. 32, 675–683. doi: 10.1002/jsfa.2740320708
Muck, R. E., Nadeau, E. M. G., McAllister, T. A., Contreras-Govea, F. E., Santos, M. C., and Kung, L. Jr. (2018). Silage review: recent advances and future uses of silage additives. J. Dairy Sci. 101, 3980–4000. doi: 10.3168/jds.2017-13839
Müller, T., Thißen, R., Braun, S., Dott, W., and Fischer, G. (2004). (M)VoC and composting facilities part 1: (M)VoC emissions from municipal biowaste and plant refuse. Environ. Sci. Pollut. Res. 11, 91–97. doi: 10.1007/BF02979708
Muttalib, S. A. A., Ismail, S. N. S., and Praveena, S. M. (2016). Application of effective microorganism (EM) in food waste composting: a review. Asia Pac. Env. Occup. Health J. 2:1.
Nakasaki, K., Araya, S., and Mimoto, H. (2013). Inoculation of Pichia kudriavzevii RB1 degrades the organic acids present in raw compost material and accelerates composting. Bioresour. Technol. 144, 521–528. doi: 10.1016/j.biortech.2013.07.005
Nakasaki, K., and Hirai, H. (2017). Temperature control strategy to enhance the activity of yeast inoculated into compost raw material for accelerated composting. Waste Manag. 65, 29–36. doi: 10.1016/j.wasman.2017.04.019
National Conference of State Legislatures (NCSL) (2020). Fighting Food Waste. Available online at: https://www.ncsl.org/research/environment-and-natural-resources/fighting-food-waste.aspx (accessed August 10, 2021).
Neff, R. A., Spiker, M., Rice, C., Schklair, A., Greenberg, S., and Leib, E. B. (2019). Misunderstood food date labels and reported food discards: A survey of U.S. consumer attitudes and behaviors. Waste Manag. 86, 123–132. doi: 10.1016/j.wasman.2019.01.023
Östergren, K., Gustavsson, J., Bos-Brouwers, H., Timmermans, T., Hansen, O.-J., Møller, H., et al. (2014). FUSIONS Definitional Framework for Food Waste. Available online at: https://www.eufusions.org/phocadownload/Publications/FUSIONS%20Definitional%20Framework%20for%20Food%20Waste%202014.pdf (accessed January 20, 2020).
Pan, I., Dam, B., and Sen, S. K. (2012). Composting of common organic wastes using microbial inoculants. 3 Biotech. 2, 127–134. doi: 10.1007/s13205-011-0033-5
Pandey, P. K., Vaddella, V., Cao, W., Biswas, S., Chiu, C., and Hunter, S. (2016). In-vessel composting system for converting food and green wastes into pathogen free soil amendment for sustainable agriculture. J. Clean Prod. 139, 407–415. doi: 10.1016/j.jclepro.2016.08.034
Pandi, J., Glatz, P., Forder, R., Komolong, B., and Chousalkar, K. (2018). Evaluation of the effects of sweet potato (Ipomoea batatas (L.) Lam) in broiler diets. J. Anim. Physiol. Anim. Nutr. 102, e216–e224. doi: 10.1111/jpn.12730
Pantano, K. (2015). Rise of ‘Ugly’ Produce in Europe, Its Future in the U.S. Available online at: https://www.foodqualityandsafety.com/article/rise-of-ugly-produce-in-europe-its-future-in-the-u-s/ (accessed April 7, 2020).
Parfitt, J., Barthel, M., and Macnaughton, S. (2010). Food waste within food supply chains: quantification and potential for change to 2050. Philos. Trans. R. Soc. Lond. B. Biol. Sci. 365, 3065–3081. doi: 10.1098/rstb.2010.0126
Parry, A., Bleazard, P., and Okawa, K. (2015). Preventing food waste: case studies of Japan and the United Kingdom. OECD Food Agric. Fish. Pap. 76. doi: 10.1787/5js4w29cf0f7-en
Pingree, C. (2018). Summary: H.R. 3444, the Food Recovery Act. Available online at: https://pingree.house.gov/legislation/summary-hr-3444-the-food-recovery-act.htm (accessed February 5, 2020).
Potts, M. (2012). ‘Feed the Beast’ aims at collecting waste to fuel UWO's biodigester. UW Oshkosh Today. Available online at: https://uwosh.edu/today/17028/feed-the-beast-aims-at-collecting-waste-to-fuel-uwos-biodigester/ (accessed August 12, 2021).
Qamaruz-Zaman, N., and Milke, M. W. (2012). VFA and ammonia from residential food waste as indicators of odor potential. Waste Manag. 32, 2426–2430. doi: 10.1016/j.wasman.2012.06.023
Read, Q. D., and Muth, M. K. (2021). Cost-effectiveness of four food waste interventions: is food waste reduction a “win–win?”. Resour. Conserv. Recycl. 168:105448. doi: 10.1016/j.resconrec.2021.105448
ReFed (2019a). Federal Tax Incentives. Available online at: https://www.refed.com/tools/food-waste-policy-finder/federal-policy/federal-tax-incentives (accessed March 4, 2020).
ReFed (2019b). Standardized Date Labeling. Available online at: https://www.refed.com/solutions/standardized-date-labeling (accessed March 4, 2020).
Rohm, H., Oostindjer, M., Aschemann-Witzel, J., Symmank, C. L, Almli, V., et al. (2017). Consumers in a sustainable food supply chain (COSUS): Understanding consumer behavior to encourage food waste reduction. Foods 6, 104. doi: 10.3390/foods6120104
Roy, D., Azaïs, A., Benkaraache, S., Drogui, P., and Tyagi, R. D. (2018). Composting leachate: characterization, treatment, and future perspectives. Rev. Environ. Sci. Biotechnol. 17, 323–349. doi: 10.1007/s11157-018-9462-5
Ruminant Feed Ban Rule (RFB) (1997). Animal proteins prohibited in ruminant feed, 21 C.F.R. 589.2000 (1997; amended 2008).
Saarisalo, E., Jalava, T., and Skyttä, E. (2006). Effect of lactic acid bacteria inoculants, formic acid, potassium sorbate and sodium benzoate on fermentation quality and aerobic stability of wilted grass silage. Agric. Food Sci. 15, 185–199. doi: 10.2137/145960606779216263
Sahoo, A., Sarkar, S., Lal, B., Kumawat, P., Sharma, S., and De, K. (2021). Utilization of fruit and vegetable waste as an alternative feed resource for sustainable and eco-friendly sheep farming. Waste Manag. 128, 232–242. doi: 10.1016/j.wasman.2021.04.050
Sall, P. M., Antoun, H., Chalifour, F.-P., and Beauchamp, C. J. (2019). Potential use of leachate from composted fruit and vegetable waste as fertilizer for corn. Cogent. Food Agric. 5:1580180. doi: 10.1080/23311932.2019.1580180
Scialabba, N., Jan, O., Tostivint, C., Turbé, A., O'Connor, C., Lavelle, P., et al. (2013). Food Wastage Footprint: Impacts on Natural Resources. (Rome: FAO).
Scudamore, J., and Harris, D. (2002). Control of foot and mouth disease: lessons from the experience of the outbreak in Great Britain in 2001. Sci. Tech. Rev. 21, 699–707. doi: 10.20506/rst.21.3.1351
Sensöz, S., Angin, D., and Yorgun, S. (2000). Influence of particle size on the pyrolysis of rapeseed (Brassica napus L.): fuel properties of bio-oil. Biomass Bioenergy 19, 271–279. doi: 10.1016/S0961-9534(00)00041-6
Shareefdeen, Z., Herner, B., Webb, D., Verhaeghe, L., and Wilson, S. (2005). An odor predictive model for rendering applications. Chem. Eng. J. 113, 215–220. doi: 10.1016/j.cej.2005.03.006
Siddiquee, S., Shafawati, S. N., and Naher, L. (2017). Effective composting of empty fruit bunches using potential Trichoderma strains. Biotechnol. Rep. 13, 1–7. doi: 10.1016/j.btre.2016.11.001
Smet, E., Van Langenhove, H., and De Bo, I. (1999). The emission of volatile compounds during the aerobic and the combined anaerobic/aerobic composting of biowaste. Atmos. Environ. 33, 1295–1303. doi: 10.1016/S1352-2310(98)00260-X
Smith, D. R., Cawthon, D. L., Sloan, J. J., and Freeman, T. M. (2006). In-vessel, mechanical rotating drum composting of institutional food residuals. Compost. Sci. Util. 14, 155–161. doi: 10.1080/1065657X.2006.10702277
Sun, W., Huang, G. H., Zeng, G., Qin, X., and Sun, X. (2009). A stepwise-cluster microbial biomass inference model in food waste composting. Waste Manag. 29, 2956–2968. doi: 10.1016/j.wasman.2009.06.023
Sundberg, C., Yu, D., Franke-Whittle, I., Kauppi, S., Smårs, S., Insam, H., Romantschuk, M., and Jönsson, H. (2013). Effects of pH and microbial composition on odour in food waste composting. Waste Manag. 33, 204–211. doi: 10.1016/j.wasman.2012.09.017
Tsai, C.-J., Chen, M.-L., Ye, A.-D., Chou, M.-S., Shen, S.-H., and Mao, I. F. (2008). The relationship of odor concentration and the critical components emitted from food waste composting plants. Atmos. Environ. 42, 8246–8251. doi: 10.1016/j.atmosenv.2008.07.055
U.S. Department of Agriculture (USDA)-Economic Research Service (ERS) (2019). New Products. Available online at: https://www.ers.usda.gov/topics/food-markets-prices/processing-marketing/new-products/ (accessed February 4, 2020).
U.S. Environmental Protection Agency (EPA) (2016). Types of Composting and Understanding the Process. Available online at: https://www.epa.gov/sustainable-management-food/types-composting-and-understanding-process (accessed May 12 2020).
U.S. Environmental Protection Agency (EPA) (2017a). Environmental Benefits of Anaerobic Digestion (AD). Available online at: https://www.epa.gov/anaerobic-digestion/environmental-benefits-anaerobic-digestion-ad (accessed February 4, 2020).
U.S. Environmental Protection Agency (EPA) (2017b). Food Recovery Hierarchy. Available online at: https://www.epa.gov/sustainable-management-food/food-recovery-hierarchy (accessed January 29, 2020).
U.S. Environmental Protection Agency (EPA) (2019a). Facts and Figures About Materials, Waste and Recycling. Available online at: https://www.epa.gov/facts-and-figures-about-materials-waste-and-recycling/food-material-specific-data#FoodTableandGraph (accessed May 12, 2020).
U.S. Environmental Protection Agency (EPA) (2019b). Industrial Uses for Wasted Food. Available online at: https://www.epa.gov/sustainable-management-food/industrial-uses-wasted-food (accessed May 12, 2020).
U.S. Environmental Protection Agency (EPA) (2019c). Reducing the Impact of Wasted Food by Feeding the Soil and Composting. Available online at: https://www.epa.gov/sustainable-management-food/reducing-impact-wasted-food-feeding-soil-and-composting (accessed May 12, 2020).
U.S. Environmental Protection Agency (EPA) (2020). 2018 Wasted Food Report. Available online at: https://www.epa.gov/sites/default/files/2020-11/documents/2018_wasted_food_report-11-9-20_final.pdf (accessed August 10, 2021).
U.S. Food Drug Administration (FDA) (2018). Bovine Spongiform Encephalopathy. Available online at: https://www.fda.gov/animal-veterinary/compliance-enforcement/bovine-spongiform-encephalopathy (accessed March 15, 2020).
U.S. Food Drug Administration (FDA). (2019). How to Cut Food Waste and Maintain Food Safety. Available online at: https://www.fda.gov/media/101389/download (accessed July 12, 2020).
Ugarte, D. D. L. T., and He, L. (2007). Is the expansion of biofuels at odds with the food security of developing countries? Biofuel Bioprod. Biorefin. 1, 92–102. doi: 10.1002/bbb.16
Ulloa, J., Van Weerd, J., Huisman, E., and Verreth, J. (2004). Tropical agricultural residues and their potential uses in fish feeds: the Costa Rican situation. Waste Manag. 24, 87–97. doi: 10.1016/j.wasman.2003.09.003
University of Wisconsin Oshkosh (UWO). (2021). Energy. https://uwosh.edu/cso/about-us/energy/ (accessed September 10, 2021).
Vargas-García, M. C., López, M. J., Suárez, F., and Moreno, J. (2005). Laboratory study of inocula production for composting processes. Bioresour. Technol. 96, 797–803. doi: 10.1016/j.biortech.2004.07.012
Wakase, S., Sasaki, H., Itoh, K., Otawa, K., Kitazume, O., Nonaka, J., et al. (2008). Investigation of the microbial community in a microbiological additive used in a manure composting process. Bioresour. Technol. 99, 2687–2693. doi: 10.1016/j.biortech.2007.04.040
Wambugu, C. W., Rene, E. R., Van de Vossenberg, J., Dupont, C., and Van Hullebusch, E. D. (2019). Role of biochar in anaerobic digestion based biorefinery for food waste. Front. Energy. Res. 7:14. doi: 10.3389/fenrg.2019.00014
Waqas, M., Nizami, A. S., Aburiazaiza, A. S., Barakat, M. A., Asam, Z. Z., Khattak, B., et al. (2019). Untapped potential of zeolites in optimization of food waste composting. J. Environ. Manage. 241, 99–112. doi: 10.1016/j.jenvman.2019.04.014
Weinberg, Z. G., and Muck, R. E. (1996). New trends and opportunities in the development and use of inoculants for silage. FEMS Microbiol. Rev. 19, 53–68. doi: 10.1111/j.1574-6976.1996.tb00253.x
Welch, D., Swaffield, J., and Evans, D. (2018). Who's responsible for food waste? Consumers, retailers and the food waste discourse coalition in the United Kingdom. J. Consum. Cult. 2018:1–21. doi: 10.1177/1469540518773801
Westendorf, M. L., Dong, Z. C., and Schoknecht, P. A. (1998). Recycled cafeteria food waste as a feed for swine: nutrient content digestibility, growth, and meat quality. J. Anim. Sci. 76:2976. doi: 10.2527/1998.76122976x
Wong, J. W. C., Fang, M., Li, G. X., and Wong, M. H. (1997). Feasibility of using coal ash residues as co-composting materials for sewage sludge. Environ. Technol. 18, 563–568. doi: 10.1080/09593331808616574
Woodgate, S., and Van Der Veen, J. (2004). The role of fat processing and rendering in the European Union animal production industry. Biotechnol. Agron. Soc. Environ. 8, 283–294.
Xu, F., Li, Y., Ge, X., Yang, L., and Li, Y. (2018). Anaerobic digestion of food waste—challenges and opportunities. Bioresour. Technol. 247, 1047–1058. doi: 10.1016/j.biortech.2017.09.020
Xue, L., Liu, G., Parfitt, J., Liu, X., Herpen, V. E., Stenmarck, Å., et al. (2017). Missing food, missing data? A critical review of global food losses and food waste data. Environ. Sci. Technol. 51, 6618–6633. doi: 10.1021/acs.est.7b00401
Yang, S. Y., Ji, K. S., Baik, Y. H., Kwak, W. S., and McCaskey, T. A. (2006). Lactic acid fermentation of food waste for swine feed. Bioresour. Technol. 97, 1858–1864. doi: 10.1016/j.biortech.2005.08.020
Yang, X., Lee, J. H., Yoo, H. Y., Shin, H. Y., Thapa, L. P., Park, C., et al. (2014). Production of bioethanol and biodiesel using instant noodle waste. Bioprocess Biosyst. Eng 37, 1627–1635. doi: 10.1007/s00449-014-1135-3
Yu, H., and Huang, G. H. (2009). Effects of sodium acetate as a pH control amendment on the composting of food waste. Bioresour. Technol. 100, 2005–2011. doi: 10.1016/j.biortech.2008.10.007
Yu, M. J., Jo, Y.-B., Kim, S.-G., Lim, Y.-K., Jeon, J.-K., Park, S. H., et al. (2011). Synthesis of biodiesel from an oil fraction separated from food waste leachate. Korean J. Chem. Eng. 28, 2287–2292. doi: 10.1007/s11814-011-0134-1
Yu, Y., and Jaenicke, E. C. (2020). Estimating food waste as household production inefficiency. Am. J. Agr. Econ. 102, 525–547. doi: 10.1002/ajae.12036
Keywords: food waste, animal feed, compost, energy production, additives
Citation: Jones SL, Gibson KE and Ricke SC (2021) Critical Factors and Emerging Opportunities in Food Waste Utilization and Treatment Technologies. Front. Sustain. Food Syst. 5:781537. doi: 10.3389/fsufs.2021.781537
Received: 22 September 2021; Accepted: 02 December 2021;
Published: 24 December 2021.
Edited by:
Carlos Martin-Rios, University of Applied Sciences and Arts of Western Switzerland, SwitzerlandReviewed by:
José L. S. Pereira, Instituto Politecnico de Viseu, PortugalAeslina Abdul Kadir, Universiti Tun Hussein Onn Malaysia, Malaysia
Copyright © 2021 Jones, Gibson and Ricke. This is an open-access article distributed under the terms of the Creative Commons Attribution License (CC BY). The use, distribution or reproduction in other forums is permitted, provided the original author(s) and the copyright owner(s) are credited and that the original publication in this journal is cited, in accordance with accepted academic practice. No use, distribution or reproduction is permitted which does not comply with these terms.
*Correspondence: Steven C. Ricke, c3JpY2tlQHdpc2MuZWR1