- 1Department of Plant Pathology, Guyana Rice Development Board, Rice Research Station, Georgetown, Guyana
- 2Faculty of Food and Agriculture, Department of Food Production, The University of the West Indies, St. Augustine, Trinidad and Tobago
- 3Department of Agricultural Economics and Extension, Faculty of Food and Agriculture, The University of the West Indies, St. Augustine, Trinidad and Tobago
Background: Blast is a devastating disease in rice production. The current research tested the efficacy of biologicals and fungicides against blast. Aqueous extracts of Azadirachta indica, Ocimum basalicum, Cymbopogan flexousus, Thymus vulgaris, Aloe vera, Tagetes patula, Cordia curassavica, Aegle marmelos, Allium fistulosum, Syzygium aromaticum, and Calotropis gigantea were tested in vitro at 5, 10, and 15% concentrations against Magnaporthe oryzae. Also, two isolates, Bacillus cereus OG2L and B. subtilis OG2A, and one isolate, Azotobacter SAG19, and, similarly, five new generation fungicides were evaluated at three different doses against blast pathogen M. oryzae in vitro. The treatments that exhibited the best performance in vitro were further evaluated against blast disease under field conditions.
Results: Extracts of T. patula (5%), C. gigantea (5%), C. curassavica (10%), A. fistulosum (10%), and A. marmelos (15%) showed greater than 81% inhibition to M. oryzae in vitro. Likewise, all three biocontrol agents, viz. B. cereus OG2L, B. subtilis OG2A, and Azotobacter SAG19, demonstrated more than 50% inhibition of mycelial growth of pathogen in vitro, and the commercial formulations of fungicides Propineb, Trifloxystrobin+Tebuconazole, Tebuconazol+Triadimenol, Bacillus subtilis Strain QST 713, and Cinnamon Oil 8%+Clove Oil 2% also significantly inhibited M. oryzae. Under field conditions, C. curassavica (10%), A. marmelos (15%), C. gigantea (5%); B.cereus OG2L; B.subtilis OG2A; Proineb and Trifloxystrobin+Tebuconazole were effective in reducing disease in addition to yield enhancement.
Conclusion: Overall, the plant extracts (C. curassavica at 10%, A. marmelos at 15%, C. gigantea at 5%); bio-agents (B. cereus OG2L, B. subtilis OG2A both at 2g/l), and new generation fungicides Antracol 70WP (Proineb) at 2.5 g/l and Nativo 75 WG (Trifloxystrobin+Tebuconazole) at 0.5 g/l provided effective control against blast disease and superior plant growth and yield compared with other treatments and untreated control.
Background
Blast disease is a serious constraint in rice production at the global level (Swodesh and Yuvraj, 2020; Simkhada and Thapa, 2021). It has been reported from more than 80 countries worldwide (Ou, 1985; CABI, 2018). The disease is caused by the fungal pathogen Magnaporthe oryzae B. C. Couch, and it affects the seedling, tillering, panicle initiation, and grain formation stages of a plant. Roy-Barman and Chattoo (2005) state that blast disease is capable of inflicting loss in rice production equivalent to feeding more than “60 million people” annually. The use of resistance and agrochemicals have been employed as strategies for the management of blast disease over 50 years. The experience in the use of resistant varieties revealed that blast fungus has evolved new mechanisms to overcome the resistance after a few years of cultivation (Vasudevan et al., 2014). The frequent breakdown of resistance resulting in the loss of millions of tons of rice has been reported in India and Japan (Khush and Jena, 2009; Sharma et al., 2012). Therefore, farmers certainly need alternative management strategies to combat blast disease in the event of the breakdown of resistance.
On the other hand, the use of agrochemicals in the management of rice blast disease has been highly dependent on old, highly toxic, and hazardous fungicides (Aktar et al., 2009). The continuous use of fungicide molecules with same mode of action led to the development of resistance in pathogens (Kunova et al., 2014). Furthermore, the overuse of highly hazardous chemicals resulted in pesticide residues and posed negative impacts to the environment (Gaihre and Nose, 2013; Rini and Dipankar, 2021). Therefore, it is needed to search for new generation fungicides with different mode of action to delay the development of resistance in fungi and to effectively control the disease.
Apart from the use of resistant cultivars and new generation fungicides, many researchers have reported sustainable and low-cost alternative environmentally friendly strategies viz. the use of plant extracts and biological control agents for the management of blast disease (Venkateswarlu et al., 2013; Liu et al., 2018; Hashim et al., 2019). Many researchers studied the use of plant extracts for the management of diseases in rice (Harish et al., 2008; Kagale et al., 2011; Devi and Chhetry, 2013; Rani and Singh, 2014; Nazifa et al., 2021; Nur et al., 2021). Similarly, several researchers have explored the potential use of biocontrol agents in the management of rice diseases (Saravanakumar et al., 2009; Nagendran et al., 2013; Liu et al., 2018; Mathurot et al., 2020). However, in Guyana, blast disease posed serious challenges with only a little research done to identify and develop suitable alternative strategies for the control of major diseases of rice (GRDB, 2013; Persaud and Saravanakumar, 2018a,b; Persaud et al., 2019). Therefore, it is essential to develop specific strategies for effective management of blast disease in rice. In this context, the current research was carried out to evaluate different plant extracts, bioagents, and new generation fungicides against blast disease under Guyanese agriculture conditions.
Methods
Isolation of Rice Blast Pathogen
Leaves expressing typical symptoms of blast disease were collected from the rice fields of the Rice Research Station (RRS) at Burma, Mahaicony, East Coast Demarara, Guyana, for isolation of the pathogen. The isolation was done as described in Persaud and Saravanakumar (2018b). The pathogen was identified by studying the colony characteristics of the isolates on PDA by following the method described in a technical bulletin on seed-borne disease and seed health testing of rice (Agarwal et al., 1989). The pathogenicity of isolates was confirmed by following Koch's postulates. The pure culture of the virulent isolate was prepared and stored at 4°C for further use.
Collection and Preparation of Plant Extracts
Fresh and healthy leaves of 11 plants, Azadirachta indica (neem), Ocimum basalicum (tulsi), Cymbopogan flexousus (lemongrass), Thymus vulgaris (thick leaf thyme), Aloe vera (aloe), Tagetes patula (marigold), Cordia curassavica (black sage), Aegle marmelos (bael), Allium fistulosum (chive), Syzygium aromaticum (clove), and Calotropis gigantea (madar) were collected from surrounding areas of the Rice Research Station, Burma, in region number 5 (Mahaica-Abary), Guyana, and carefully transferred to the Laboratory of Plant Pathology Department, RRS, Burma. The plant samples collected were washed with running tap water and then rinsed two times with sterile distilled water and left to air dry for 2 to 3 h. The selected plant parts were cut into small pieces (1–2 cm), and 100 g plant tissue was then ground with 100 ml sterile distilled water (1:1 W/V) with mortar and pestle. The ground samples were filtered through a double layered white muslin cloth into a beaker. The filtrate that constituted 100% concentration was collected and stored in a sterile conical flask at 25–28°C for further study (Devi and Chhetry, 2013).
In vitro Evaluation of Plant Extracts Against M. oryzae
The poisoned food technique was employed to assess the efficacy of plant extracts against mycelial growth of M. oryzae. Eleven plant extracts were prepared at 5, 10, and 15% concentrations in PDA. Varying amounts of each plant extract were dispensed and mixed in slightly warm PDA in requisite quantities to give 5, 10, and 15% concentration. PDA (15 ml) amended with plant extract was poured in a Petri plate for each treatment and replicated three times. Then, a mycelium disc (5 mm) of 10-day-old culture of M. oryzae was placed at the center of the plate containing PDA. The control was prepared by inoculating the pathogen on PDA without the addition of plant extracts. The plates were incubated at 28 ± 2°C, and radial growth of mycelium was measured 9 days after inoculation. Percentage inhibition of mycelial growth in treatment over control was calculated using the following formula: I = (C-T/C) × 100, where I: Inhibition over control; C: Mycelial growth in control; T: Mycelial growth in treatment.
In vitro Screening of Bioagents Against M. oryzae
Three biocontrol strains, Bacillus cereus OG2L, Bacillus subtilis OG2A, and Azotobacter sp. SAG19 were obtained from the culture collection of the Plant Pathology Lab, Department of Food Production, The University of the West Indies, St. Augustine, to study their antagonistic potential against M. oryzae (Saravanakumar et al., 2019). The biocontrol strains were screened by a dual culture test as described in Saravanakumar et al. (2007). The biocontrol isolate was streaked perpendicular to the M. oryzae at a distance of 4 cm and incubated at 28 ± 2°C for 9 days. Plates inoculated only with M. oryzae served as control. Three replicates were maintained for each treatment. The percentage inhibition of mycelial growth by the biocontrol strain was calculated in comparison to control.
In vitro Efficacy of New Generation Fungicides Against M. oryzae
Fungicides, such as Antracol 70WP (2.5, 5.0, 7.5 g/l), Nativo 75 WG (0.5, 0.75, 1.0 g/l), Silvacur Combi 30 EC (1.0, 2.5, 4.0 ml/l), Serenade 1.34 SC (2.0, 4.0, 6.0 ml/l), and Cyclops (0.75, 1.0, 1.25 ml/l) at three concentrations along with the recommended fungicide, Fungione (1.5 ml/l), were evaluated for their efficacy against M. oryzae in vitro using the poisoned food technique (Grover and Moore, 1962). PDA amended with various concentrations of fungicides were inoculated with M. oryzae and incubated at 28 ± 2°C for 9 days. PDA without addition of fungicide served as control, and each treatment was replicated thrice in a completely randomized design (CRD).
Field Evaluation of Plant Extracts, Bioagents, and New Generation Fungicides
Two experimental trials were conducted using the lowland irrigated rice cultivation system during the spring and autumn cropping seasons of 2016 at Onverwagt Back, Guyana. The experiment was carried out using a randomized block design (RBD) with three replicates per treatment. Each plot had a 15 m2 (3 × 5 m) size with 1 m plot-to-plot spacing. The soil is a front-land rice group and classified as Litchfield clay (humic gley, very poorly drained, surface soil strongly acidic to neutral, thick, and very dark gray; low in P, Ca and K). A susceptible cultivar Rustic was established using direct seed planting at a seed rate of 200 kg ha−1. The fertilizer was applied at 21, 42, and 58 DAS (N, P, K at the rate of 120:30:10 kg/ha). Likewise, weed and pest control throughout the crop followed the standard GRDB crop production practices. The plant extracts, biocontrol agents, and fungicides that showed high inhibition to M. oryzae in vitro were selected for evaluation in these trials.
The experiment had an individual application of plant extracts T. patula (5%), C. curassavica (10%), A. marmelos (15%), A. fistulosum (10%), and C. gigantea (5%); biocontrol agents B. cereus OG2L (2g l−1), B. subtilis OG2A (2g l−1), and Azotobacter SAG19 (2g l−1); new generation fungicides Antracol 70WP (2.5 g l−1), Nativo 75 WG (0.5 g l−1), Silvacur Combi 30 EC (1.0 ml l−1), Serenade 1.34 SC (2 ml l−1), Cyclops (0.75 ml l−1), and Fugione (1.5 ml l−1). The treatments were applied as foliar spray using a Cooper Pegler (CP3) manual operated knapsack sprayer at 35 and 45 days after sowing (DAS). The plots that did not receive biologicals and fungicides served as control.
Assessment of Lesion Size, Disease Severity, and Yield
The blast lesion length and disease intensity were recorded on 32 and 52 DAS by randomly tagging five plants per plot. The lesion length was measured by selecting the third, fourth, and fifth leaves from the top of a randomly selected plant. Five lesions per leaf were selected for measuring the length. Percentage disease severity was calculated based on the 0–9 scale of INGER, IRRI (2002) using the following formula: (Mean disease score / 9) × 100.
The effect of treatments on growth parameters was assessed by recording plant height and number of tillers per square meter at harvesting. The panicle length, number of filled and unfilled grains, and 1,000-grain weight were recorded from 20 panicles harvested from individual plots. The grain yield per ha was calculated after harvest by threshing and recording the weight and moisture from each plot.
Statistical Analysis
The data obtained from in vitro experiments assessing the efficacy of biologicals against mycelial growth of M. oryzae and field trials evaluating the efficacy of biologicals and fungicides on blast disease and yield were analyzed using analysis of variance (ANOVA) of the completed RBD and RBD, respectively. The ANOVA and statistical significance were analyzed using the analytical software, Statistix 8.0.
Results
Efficacy of Plant Extracts, Bioagents, and Fungicides Against M. oryzae in vitro
Among 11 plant extracts tested, 5% extracts of T. patula and C. gigantea exhibited significantly high mycelial inhibition (87.04 and 85.93%, respectively) compared with other plant extracts. The testing of 10% plant extracts showed high mycelial inhibition by T. patula (81.11%), C. curassavica (86.30%), A. fistulosum (84.07%), and C. gigantean (85.93%). Similarly, 15% extracts of C. curassavica (86.30%), A. fistulosum (81.48%), C. gigantea (81.48%), and A. marmelos (80.37%) exhibited high inhibition to mycelial growth of M. oryzae. The results also indicate that each concentration of plant extracts tested had varied levels of inhibitory effect against mycelial growth of M. orzyae. The plant extracts that exhibited high inhibition at the lower concentrations were selected for further evaluation under field conditions against blast disease (Table 1).
Similarly, the testing of B. cereus OG2L, B. subtilis OG2A, and Azotobacter SAG19 exhibited more than 50% inhibition to mycelial growth of M. oryzae over control in vitro (Figure 1). All three biocontrol strains were further tested under field conditions against blast disease.
All of the new generation fungicides tested at three different rates in vitro exhibited 100% inhibition to the mycelial growth of M. oryzae. Therefore, the lower dose for each fungicide was used for testing their efficacy under field conditions.
Efficacy of Biologicals and New Generation Fungicides Against Blast Disease in Trials I and II
Trial I recorded significantly lower lesion length and disease severity in A. marmelos (16.60 mm; 46.67%), C. curassavica (18.87 mm; 48.15%), C. gigantea (18.87 mm; 48.89%), B. subtilis OG2A (17.73 mm; 51.85%), B. cereus OG2L (18.73 mm; 48.89%), Antracol 70WP (18.55 mm; 48.89%), Nativo 75 WG (19.00 mm; 48.15%), and Serenade 1.34 SC (25.40 mm; 51.85%) treated plants compared with the control (53.60 mm; 80.00%). The disease reduction in these treatments ranged between 32.75% and 38.54% compared with the control (Figure 2). However, the application of T. patula (42.80 mm; 60.74%), A. fistulosum (46.00 mm; 71.11%), Azotobacter SAG 19 (45.67 mm; 71.85%), and Silvacur Combi 30 EC (44.93 mm; 71.85%) did not show high efficacy in reducing lesion length and disease severity (Table 2).
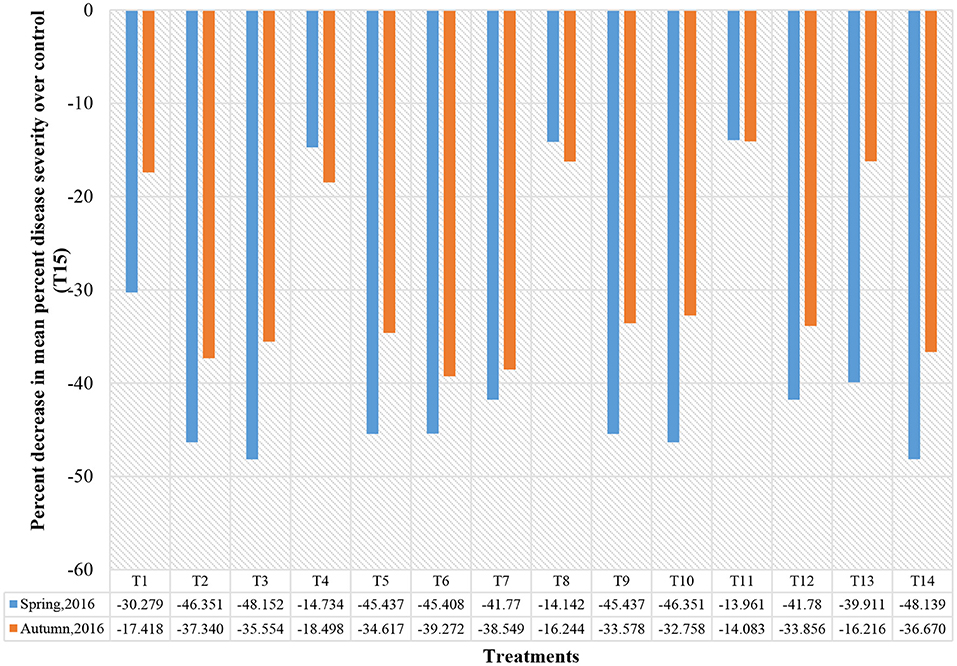
Figure 2. Percentage decrease in mean percent disease severity over the negative control (T15) treatment. T1-Marigold; T2-Black stage; T3-Bael extract; T4-Chives; T5-Madar plant; T6-B. cereus OG2L; T7-B. subtilis OG2A; T8-Azotobacter SAG 19; T9-Antracol 70WP; T10-Nativo 75 WG; T11-Silvacur Combi 30 EC; T12-Serenade 1.34 SC; T13-Cyclops; T14-Fugione (Check); T15-Untreated control.
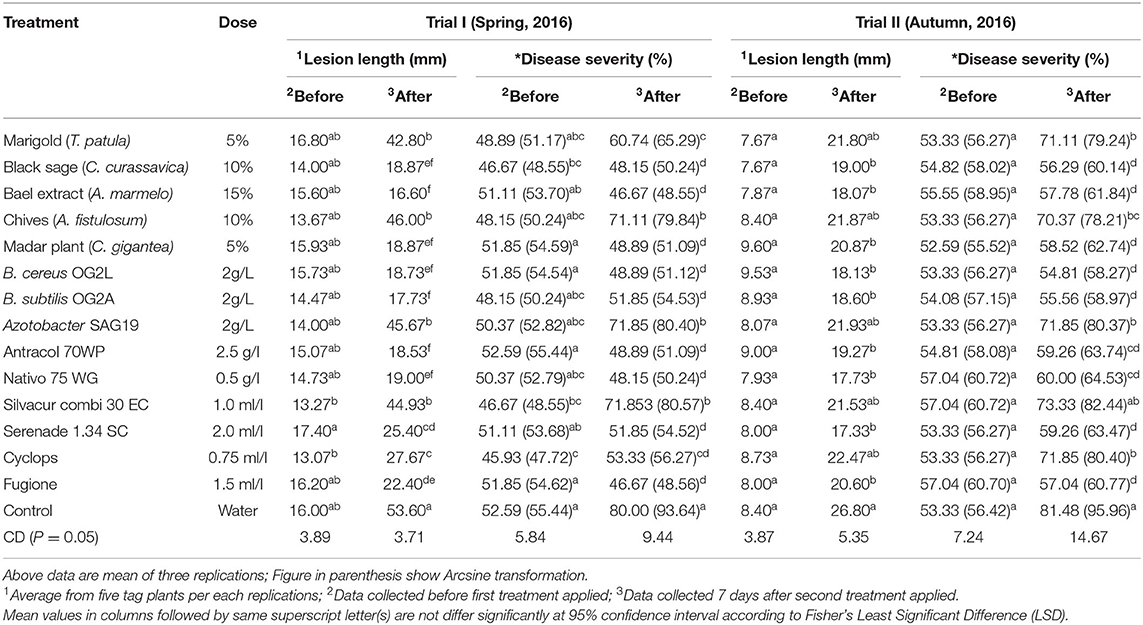
Table 2. Effect of plant extracts, bioagents and new generation fungicides against blast disease under field conditions.
The application of C. curassavica (19.00 mm; 56.29%), A. marmelos (18.07 mm; 57.78%), C. gigantea (20.84 mm; 70.37%), B. cereus OG2L (18.13 mm; 54.81%), B. subtilis OG2A (18.60 mm; 55.56%), Antracol 70WP (19.27 mm; 59.26%), Nativo 75 WG (17.73 mm; 60.00%), Serenade 1.34 (17.33 mm; 59.26%), and Fugione (20.60 mm; 57.04%) had significantly reduced lesion length and disease severity in trial II compared with the untreated control and other treatments (Table 2). The disease reduction in these treatments over control ranged from 41.78% to 48.15% in trial II (Figure 2).
Biologicals and New Generation Fungicides on Growth and Yield Parameters
Plant Height and Number of Tillers
Greater plant height was recorded in B. subtilis OG2A (82.17 cm) treated plants, whereas a high number of tillers per square meter was observed in application of Nativo 75 WG (314.67 per m2). The control plants recorded low plant height (75.77 cm) and number of tillers per square meter (270.67 per m2) in trial I (Table 3). However, no significant differences were observed in plant height and number of tillers among treatments in trial II (Table 4).
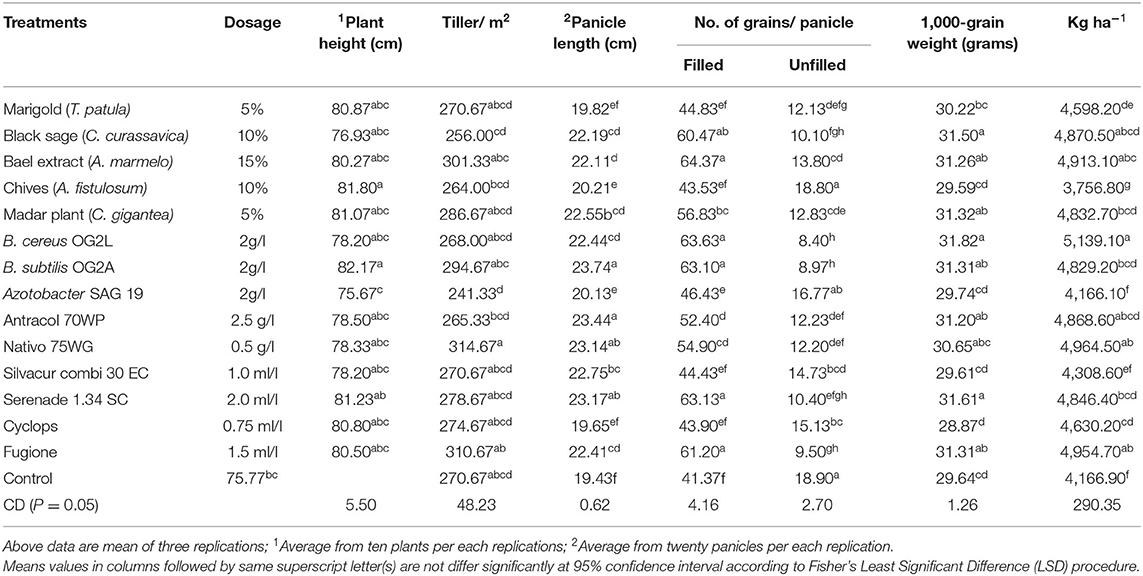
Table 3. Effects of plant extract, bioagents and new generation fungicides against blast disease (P. oryzae) on growth, yield parameters and yield during spring 2016 (Trial I).
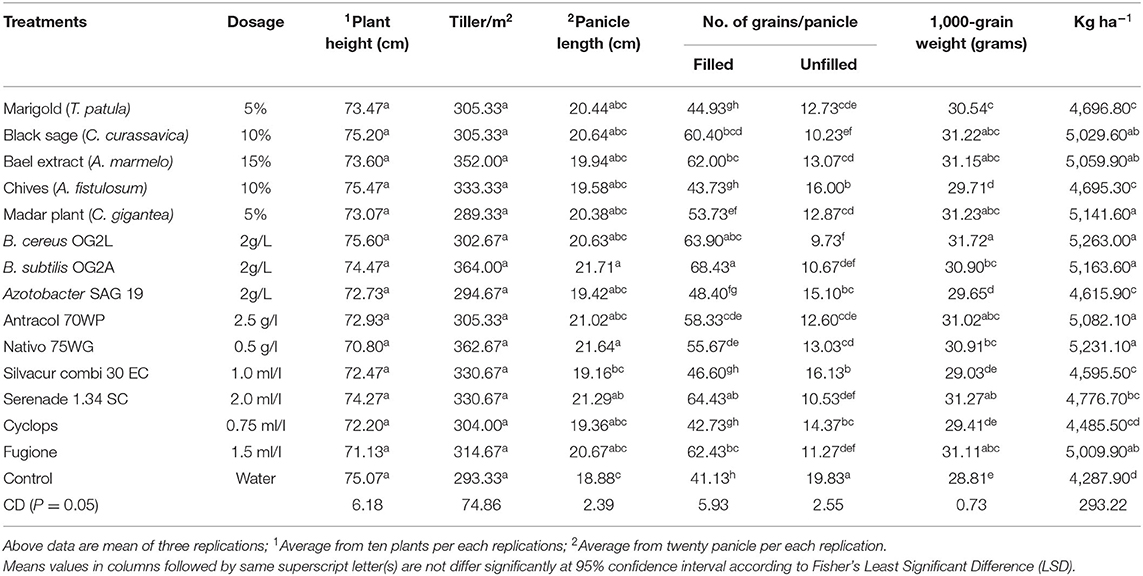
Table 4. Effects of plant extract, bioagents and new generation fungicides against blast disease (P. oryzae) on growth and yield parameters during autumn 2016 (Trial II).
Panicle Length
In trial I, greater panicle length was recorded in plots treated with B. subtilis OG2A (23.74 cm), Antracol 70WP (23.44 cm), Serenade 1.34 SC (23.17 cm), and Nativo 75 WG (23.14 cm). The statistical analysis revealed that there was no significant difference in panicle length among these four treatments. Shorter panicle length was recorded in the control (19.43 cm) and Cyclops (19.65 cm), T. patula (19.82 cm), A. fistulosum (20.21 cm), treated plots (Table 3).
In trial II, the plots treated with B. subtilis OG2A (21.71 cm), Nativo 75 WG (21.64 cm), and Serenade 1.34 SC (21.29 cm) recorded the greater panicle length. The shorter panicle length was recorded in control (18.88 cm) and Silvacur Combi 30 EC (19.16 cm), Cyclops (19.36 cm), Azotobacter SAG 19 (19.42 cm) treated plots (Table 4).
Grain-Filling Potential
A high number of filled grains per panicle was recorded in plots treated with A. marmelos (64.37 filled grains/panicle) and B. subtilis OG2A (68.43 filled grains/panicle) compared with the control (19.43; 18.88 filled grains/panicle) in trials I and II, respectively. Also, statistically similar and higher numbers of filled grains per panicle were observed in extracts of C. curassavica, A. marmelos, B. cereus OG2L; B. subtilis OG2A, Serenade 1.34 SC, and Fugione treatments compared to the control (Tables 3, 4).
A significantly low number of unfilled grains per panicle was observed in treatments B. cereus OG2L (8.40 No/panicle) followed by B. subtilis OG2A (8.97 No/panicle), Fugione (9.50 No/panicle), C. curassavica (10.10 No/panicle), and Serenade 1.34 SC (10.40 No/panicle) in trial I (Table 3). Similarly, a low number of unfilled grains per panicle was recorded in plots treated with B. cereus OG2L (9.73 No/panicle) followed by C. curassavica (10.23 No/panicle), Serenade 1.34 SC (10.53 No/panicle), B. subtilis OG2A (10.67 No/panicle), and Fugione (11.27 No/panicle) (Table 4).
Grain Weight and Yield
B. cereus OG2L, Serenade 1.34 SC, and C. curassavica treated plants recorded significantly high 1,000-grain weight compared with the control in trials I and II. In addition to these treatments, Nativo 75 WG and Fugione treated plots also recorded greater 1,000-grain weight compared with the application of Cyclops, Silvacur Combi 30, and A. fistulosum in both trials (Tables 3, 4).
Both field trials recorded significantly higher grain yield from plots treated with B. cereus OG2L (5139.10; 5263.00 kg ha−1), Nativo 75 WG (4964.50; 5231.10 kg ha−1), Fugione (4954.70; 5009.90 kg ha−1), A. marmelos (4913.10; 5059.90 kg ha−1), C. curassavica (4870.50; 5029.60 kg ha−1), and Antracol 70WP (4868.60; 5082.10 kg ha−1) compared with other treatments and control (4166.90; 4287.90 kg ha−1) (Tables 3, 4). It was also noted that the application of A. fistulosum (3756.80 kg ha−1) recorded poorer grain yield than the control in trial I.
The application of B. cereus OG2L and Nativo 75 WG had high percentage increases in grain yield that ranged between 19.14% and 23.33%. Similarly, C. curassavica, A. marmelos, C. gigantea, B. subtilis OG2A, Antracol 70WP, and Fugione consistently showed a 15% increase in grain yield over control. It was observed that application of T. patula, A. fistulosum, Azotobacter SAG 19, Silvacur Combi 30 EC, and Cyclops did not reduce disease severity and enhance grain yield in both trials.
Discussion
Blast disease is a major problem for rice cultivation in every part of the world where rice is grown. Apart from breeding and identification of blast-resistant genotypes, the use of plant extracts and biocontrol agents play an important role in the present-day concept of sustainable agriculture and integrated disease management (IDM). The antimicrobial properties of plant extracts have widened their scope for exploring it as a promising strategy for plant disease control (Saravanakumar et al., 2015). The plant extracts of Andrographis paniculata, Calotropis procera, and Eucalyptus globules were effective in reducing the stem rot of paddy (Venkateswarlu et al., 2013). Similarly, Kagale et al. (2011) report the reduction of sheath blight disease by Ipomoea carnea and Zizyphus jujube extracts under in vitro and field conditions. Antifungal activity of Acorus calamus was demonstrated against rice brown spot pathogen, Drechslera oryzae, by Devi and Chhetry (2013). The use of Aloe vera and Cassia occidentalis seed extracts at 100% concentration significantly reduced Xanthomas oryzae in rice (Rani and Singh, 2014). The review by Garg et al. (2021) elucidates the use of extracts of neem leaf (Azadirachta indica), garlic tuber (Allium sativum), and ginger rhizome (Zingiber officinale) against rice blast pathogen. Recently, Kapil and Rabin (2021) report the antifungal activity of C. arabica extracts against the mycelial growth of blast pathogen. The current study investigated 11 plant extracts, of which five significantly inhibited the blast pathogen, M. oryzae in vitro. The extracts of C. gigantea at 5%, C. curassavica at 10%, and A. marmelos at 15% were found to be highly effective in the control of blast disease in rice under field conditions.
Many researchers also report that the plant extracts possess several secondary metabolites and phytochemical compounds exhibiting antifungal, antimicrobial, and insecticidal properties (Swodesh and Yuvraj, 2020; Moutoshi et al., 2021). The GC-MS analysis by Mujeeb et al. (2014) revealed that A. marmelos contains many bioactive compounds, such as alcohols, aldehydes, fatty acid methyl esters, flavonoids, terpenoids, phenolics, and steroids. The presence of terpenoid in A. marmelos could cause disruption in microbial cell membranes (Gurjar et al., 2012). Likewise, four unique chemical compounds, Cordiaquinones A, B, J and K, reported in C. curassavica show antifungal activities against Candida albicans, Cladosporium cucumerinum, and larvicidal properties against Aedes aegypti (Ioset et al., 2000). Parihar and Balekar (2016) report the acaricidal, antimicrobial, and insecticidal activities of Calotropis procera. The same authors report that the different plant parts of madar possess various bioactive compounds, such as calotoxin, calotropin, calotropagenin, flavonoids, stigmasterol, polyphenolic compounds, and β-sitosterol. The leaf extracts of the madar plant are reported for high inhibition against fungal pathogens, Microsporum boulardii, Aspergillus flavus, and A. niger (Parihar and Balekar, 2016). Therefore, the efficacy of C. gigantean (madar), C. curassavica (black sage), and A. marmelos (bael) against blast disease in rice in the current investigation could be attributed to the existence of antimicrobial compounds in those plant extracts. The use of biocontrol agents has currently received greater attention in the management of plant diseases. Several consortia of bioagents have been evaluated against the M. oryzae pathogen (Moutoshi et al., 2021). Suryadi et al. (2013) reports that a consortium of Bacillus firmus E65, Bacillus cereus, and Pseudomonas aeruginosa C32b significantly inhibited the growth of M. oryzae, and Wen-Ching et al. (2021) found three strains of bioagents, viz. Bacillus subtilis 5, B.cereus 3S5, and Pseudomonas fluorecens 10S2, to be highly effective in vitro and greater than 31% reduction in disease severity than the control under a field experiment. This finding is in agreement with the results of the present investigation in which two treatments with different strains of the biocontrol agents, B. cereus OG2L and B. subtilis OG2A showed significant control of the blast disease. In addition, these biocontrol strains positively influenced the growth and yield in the current study. The growth promotion, antagonism, and regulation of defense enzymes are reported as key mechanisms in the control of rice diseases by biocontrol agents, such as Pseudomonas fluorescens and B. subtilis (Harish et al., 2008; Kandasamy et al., 2009; Saravanakumar et al., 2009; Karthiba et al., 2010; Yasmin et al., 2017). Therefore, it is proposed in the current study that the antagonism, induction of defense enzymes, and growth promotion would play a key role in the reduction of disease severity and increase of yield in rice.
The current study also demonstrates the effective control of blast disease by an application of two of the new generation fungicides, Antracol 70WP (Propineb) at 2.5 g/l and Nativo 75 WG (Trifloxystrobin, Tebuconazole) at 0.5 g/l. The current findings are in agreement with the results of Dutta et al. (2012), who report an application of fungicides Gain (Tricycloazole), Score (Difenoconazole 25%), and Nativo (Tebuconazole+Trifloxystobin) to be effective in reducing blast disease by 12.85% 11.46%, and 10.15%, respectively. The same authors also report that application of the Nativo fungicide increased the grain yield compared with control. Similarly, Gupta et al. (2013) evaluate seven fungicides on three rice varieties (Jaya, Basmati-370 and PC-19) at 0.1% concentration against brown spot disease in rice. The application of propiconazole reduced brown spot disease significantly by 73%, 69%, and 70% for the three varieties tested and increased the yield by 1.9, 1.2, and 2.1 t ha−1 compared with control. An effective control of blast disease and increase in rice yield is reported by the application of a new generation fungicide, Metaminostrobin 20 SC (Jagadeeshwar et al., 2014). Similarly, a combination of fungicides, RIL-068/F1 48 WG (Kresoxim methyl 40% + Hexaconazole 8% WG), Dhanucop Team (Tricyclazole 75% WP) and Conika 50% WP (Kasugamycin 5% + Copper Oxychloride 45% WP) were found to be effective against rice blast (Kumar and Veerabhadraswamy, 2014). The combination of two fungicides was found to be more effective in disease control due to their different modes of action on the target organism as demonstrated in the current investigation.
Conclusion
Management of Blast Disease in Rice
The bio-efficacy of 11 botanicals, three biocontrol agents, and five new generation fungicides for the management of rice blast disease was carried out under Guyana agricultural conditions.
The application of plant extracts, viz. black sage at 10%, bael extract at 15%, and madar plant at 5%, demonstrated superior blast disease control, higher plant growth, and grain yield compared with untreated control in two field trials.
Similarly, two of the three isolates, viz. B. cereus OG2L and B. subtilis OG2A each at 2 g/L, also showed higher level of control of the blast disease and positively influenced the growth and yield parameters and grain yield compared with untreated control.
Of the five new generation fungicides evaluated, two new generation fungicides (Antracol 70WP at 2.5 g/l and Nativo 75 WG at 0.5 g/l) along with the positive check, Fugione at 1.5 ml/l, also showed positive influence in plant growth, yield parameters, and increase in grain yield compared with untreated control.
Therefore, in the case of a blast disease outbreak or epidemic disease situation, the following management strategies could be recommended for the control of the blast disease in rice:
(i) Use of plant extracts (black sage at 10%, bael at 15%, and madar plant 5%) and two bioagents (B. cereus OG2L and B. subtilis OG2A at 2 g/l) identified in this study.
(ii) Use of two new generation fungicides (Antracol 70WP at 2.5 g/l andNativo 75 WG at 0.5 g/l), which are also identified in this research for superior control of blast disease.
Data Availability Statement
The original contributions presented in the study are included in the article/supplementary material, further inquiries can be directed to the corresponding author/s.
Author Contributions
RP: designed and executed the experiments, analyzed the data, and drafted the manuscript. DS: supervised, provided technical advice, guidance, and edited the manuscript. All other authors provided support, technical advice, read, and agreed with the content of the manuscript.
Conflict of Interest
The authors declare that the research was conducted in the absence of any commercial or financial relationships that could be construed as a potential conflict of interest.
Publisher's Note
All claims expressed in this article are solely those of the authors and do not necessarily represent those of their affiliated organizations, or those of the publisher, the editors and the reviewers. Any product that may be evaluated in this article, or claim that may be made by its manufacturer, is not guaranteed or endorsed by the publisher.
Acknowledgments
RP is thankful to Guyana Rice Development Board (GRDB) for providing him with the necessary funding and laboratory facility to conduct this research work at the GRDB, Rice Research Station, Burma in Guyana. Also for The University of West Indies to allow the use of their laboratory facility for isolation of the biocontrol agents.
References
Agarwal, P. C., Mortensen, C. N., and Mathur, S. B. (1989). “Seed-borne diseases and seed health testing of rice,” in Phytopathological Papers 30, Kew, Surrey: Commonwealth Mycological Institute, 106.
Aktar, M. W., Sengupta, D., and Chowdhury, A. (2009). Impact of pesticides use in agriculture: their benefits and hazards. Interdiscip. Toxicol. 2, 1–12. doi: 10.2478/v10102-009-0001-7
CABI (2018). Invasive Species Compendium: Magnaporthe oryzae (rice blast disease). Wallingford, UK: CAB International. Available online at: www.cabi.org/isc
Devi, O. J., and Chhetry, G. K. N. (2013). Evaluation of antifungal properties of certain plants against Drechslera oryzae causing brown leaf spot of rice in manipur valley. Int. J. Scientific Res. Publicat. 3, 1–3.
Dutta, D., Saha, S., Ray, D. P., and Bag, M. K. (2012). Effect of different active fungicides molecules on the management of rice blast disease. Intl. J. Agric. Env. Biotech. 5, 247–251.
Gaihre, Y. R., and Nose, A. (2013). High yielding capabilities and genetic variation in crossing of sheath blight disease resistant rice line. Field Crops Res. 149, 133–140. doi: 10.1016/j.fcr.2013.04.009
Garg, P., Moirangthem, I., Sharma, A., and Kuri, A. (2021). Applictaion of different bio-agents and plant extracts in blast of paddy disease: a review. Plant Cell Biotechnol. Mole. Biol. 22, 64–72. Available online at: https://www.ikppress.org/index.php/PCBMB/article/view/6487
GRDB (2013). Technical Annual Report, GRDB. Available online at: http://www.grdb.gy/
Grover, R. K., and Moore, J. D. (1962). Toximetric Studies of Fungicides against the Brown Rot Organism, Sclerotium fructicola and Sclerotium laxa. Phytopathology 52, 876–880.
Gupta, V., Shamas, N., Razdan, V. K., Sharma, B. C., Sharma, R., Kaur, K., Singh, I., John, D., and Kumar, A. (2013). Foliar Application of Fungicides for the Management of Brown Spot Disease in Rice (Oryza sativa L.) caused by Bipolaris oryzae. Afr. J. Agric. Res. 8, 3303–3309. doi: 10.5897/AJAR2013.7282
Gurjar, M., Singh, A.li S., Akhtar, M., and Singh, K. S. (2012). Efficacy of plant extracts in plant disease management. Agricult. Sci. 3, 425–433. doi: 10.4236/as.2012.33050
Harish, S., Saravanakumar, D., Radjacommare, R., Ebenezar, E. G., and Seetharaman, K. (2008). Use of plant extracts and biocontrol agents for the management of brown spot disease in rice. Biocontrol 53, 555–557. doi: 10.1007/s10526-007-9098-9
Hashim, I., Mamiro, D., Mabagala, R. B., and Tefera, T. (2019). Reduction of initial occurrence of rice blast (Pyricularia oryzae) inocula on seeds by microbial and hot water seed treatments. Aust. J. Crop Sci. 13, 309–314. doi: 10.21475/ajcs.19.13.02.p1474
Ioset, J. R., Marston, A., Gupta, M. P., and Hostettmann, K. (2000). Antifungal and Larvicidal Cordiaquinones from the Roots of Cordia curassavica. Phytochemistry 53, 613–617. doi: 10.1016/S0031-9422(99)00604-4
IRRI (2002). Standard Evaluation System (SES) for Rice. IRRI, Manila, Philippine: INGER Genetic Resources Center.
Jagadeeshwar, R., Varma, N. R. G., Reddy, P. R. R., Raju, C. S., Vanisree, S., Reddy, B. G., and Dayakar, S. (2014). Screening of new fungicides against location specific diseases of rice occurring in Southern Telangana zone of Andhra Pradesh. The J.Res. Angrau. 42, 18–21.
Kagale, S., Marimuthu, T., Kagale, J., Thayumanavan, B., and Samiyappan, R. (2011). Induction of systemic resistance in rice by leaf extracts of Zizyphus jujuba and Ipomoea carnea against Rhizoctonia solani. Plant Signal Behav. 6, 919–923. doi: 10.4161/psb.6.7.15304
Kandasamy, S., Loganathan, K., Muthuraj, R., Duraisamy, S., Seetharaman, S., Thiruvengadam, R., Ponnusamy, B., and Ramasamy, S. (2009). Understanding the molecular basis of plant growth promotional effect of Pseudomonas fluorescens on rice through protein profiling. Proteome Sci. 7:47. doi: 10.1186/1477-5956-7-47
Karthiba, L., Saveetha, K., Suresh, S., Raguchander, T., Saravanakumar, D., and Samiyappan, R. (2010). PGPR and entomopathogenic fungus bioformulation for the synchronous management of leaffolder pest and sheath blight disease of rice. Pest Manag. Sci. 66, 555–564. doi: 10.1002/ps.1907
Khush, G. S., and Jena, K. K. (2009). “Current status and future prospects for research on blast resistance in rice (Oryza sativa L.),” in: Advances in Genetics, Genomics and Control of Rice Blast Disease, eds G. L. Wangand and B. Valent (Dordrecht: Springer). 1–10.
Kumar, M. K. P., and Veerabhadraswamy, A. L. (2014). Appraise a combination of fungicides against blast and sheath blight diseases of paddy (Oryza sativa L.). J. Experim. Biol. Agricult. Sci. 2, 49–57.
Kunova, A., Pizzatti, C., Bonaldi, M., and Cortesi, P. (2014). Sensitivity of nonexposed and exposed populations of Magnaporthe oryzae from rice to tricyclazole and azoxystrobin. Plant Dis. 98, 512–518. doi: 10.1094/PDIS-04-13-0432-RE
Liu, L., Liang, M., Li, L., Sun, L., Xu, Y, Gao, J, Wang, L., Hou, Y., and Huang, S. (2018). Synergistic effects of the combined application of Bacillus subtilis H158 and strobilurins for rice sheath blight control. Biol. Control 117, 182–187. doi: 10.1016/j.biocontrol.2017.11.011
Mathurot, C., Teerayut, T., and Wasu, P. (2020). Evaluation of biocontrol activities of streptomyces spp. against rice blast disease fungi. Pathogens. 9, 1–16. doi: 10.3390/pathogens9020126
Moutoshi, C., Nur, U. M., Chhana, U., Mahfuzur, R., and Tofazzal, I. (2021). Biological and biorational management of blast diseases in cereals caused by Magnaporthe oryzae. Critic. Rev. Biotechnol. 41, 7994–1022. doi: 10.1080/07388551.2021.1898325
Mujeeb, F., Bajpai, P., and Pathak, N. (2014). Phytochemical evaluation, antimicrobial activity and determination of bioactive components from leaves of Aegle marmelos. BioMed Res. Int. 497606:11. doi: 10.1155/2014/497606
Nagendran, K., Karthikeyan, G., Peeran, M. F., Raveendran, M., Prabakar, K., and Raguchander, T. (2013). Management of bacterial leaf blight disease in rice with endophytic bacteria. World Appl. Sci. J. 28, 2229–2241. doi: 10.5829/idosi.wasj.2013.28.12.2009
Nazifa, Z., Aminuzzaman, F. M., Laila, L., and Rehena, M. K. (2021). In vitro Efficacy of Botanicals against Rice Blast Pathogen Magnaporthe oryzae oryzae. Am. J. Plant Sci. 12:662–678. doi: 10.4236/ajps.2021.124045
Nur, H. N., Neni, K. C. M. R., Nor, Y. Y., and Siti, N. H. M. L. (2021). IOP Conference series. Earth Environ. Sci. 685, 01–26.
Ou, S. H. (1985). “Rice diseases, 2nd ed,” in Commonwealth Mycological Institute. Kew, Surrey, England: C.A.B. International, Farnham Royal, Slough.
Parihar, G., and Balekar, N. (2016). Calotropis procera: A Phytochemical and Pharmacological Review. TJPS. 40, 115–131.
Persaud, R., Khan, A., Isaac, W. A., Ganpat, W., and Saravanakumar, D. (2019). Plant extracts, bioagents and new generation fungicides in the control of rice sheath blight in Guyana. Crop Prot. 119, 30–37. doi: 10.1016/j.cropro.2019.01.008
Persaud, R., and Saravanakumar, D. (2018a). Screening for blast resistance in rice using AMMI models to understand G x E interaction in Guyana. Phytoparasitica 46, 551–568. doi: 10.1007/s12600-018-0681-7
Persaud, R., and Saravanakumar, D. (2018b). Differential expression of proteins in resistant and susceptible rice genotypes against blast infection. Physiol. Mol. Plant Pathol. 103, 62–70. doi: 10.1016/j.pmpp.2018.05.001
Rani, M., and Singh, N. K. (2014). Control of Rice Blight Pathogen Xanthomonas oryzae through Herbal Plant Extract. Int. J. Pharmaceut. Sci. Res. 5, 5469–5473.
Rini, P., and Dipankar, M. (2021). Fungicidal management of blast disease (Pyricularia grisea) of rice. Pharma Innov. J. 10, 786–790.
Saravanakumar, D., Harish, S., Loganathan, M., Vivekananthan, R., Rajendran, L., Raguchander, T., et al. (2007). Rhizobacterial bioformulation for the effective management of Macrophomina root rot in mungbean. Archiv. Phytopathol. Plant Protect. 40, 323–337. doi: 10.1080/03235400600587326
Saravanakumar, D., Karthiba, L., Ramjegathesh, R., Prabakar, K., and Raguchander, T. (2015). “Characterization of bioactive compounds from botanicals for the management of plant diseases,” in Sustainable Crop Disease Management using Natural Products, eds S. Ganesan, K. Vadivel, and J. Jayaraman, (Wallingford, Oxfordshire: CABI publication), 1–18.
Saravanakumar, D., Lavanya, N., Muthumeena, K., Raguchander, T., and Samiyappan, R. (2009). Fluorescent pseudomonad mixtures mediate disease resistance in rice plants against sheath rot (Sarocladium oryzae) disease. Biocontrol. 54:273–286. doi: 10.1007/s10526-008-9166-9
Saravanakumar, D., Thomas, A., and Banwarie, N. (2019). Antagonistic potential of lipopeptide producing Bacillus amyloliquefaciens against major vegetable pathogens. Euro. J. Plant Pathol. 154, 319–335. doi: 10.1007/s10658-018-01658-y
Sharma, T. R., Rai, A. K., Gupta, S. K., Vijayan, J., Devanna, B. N., and Ray, S. (2012). Rice blast management through host-plant resistance: retrospect and prospects. Agric.Res.1, 37–52. doi: 10.1007/s40003-011-0003-5
Simkhada, K., and Thapa, R. (2021). Rice blast, a major threat to the rice production and its various management techniques. Book Chapt. 21:53924. doi: 10.13140/RG.2.2.3(4303)0.53924
Suryadi, Y., Susilowati, D. N., Riana, E., and Mubarik, N. R. (2013). Management of rice blast disease (Pyricularia oryzae) using formulated bacterial consortium. Emir. J. Food Agri. 25, 349–357. doi: 10.9755/ejfa.v25i5.12564
Swodesh, R., and Yuvraj, D. (2020). A Review On Various Management Method Of Rice Blast Disease. Malaysian J. Sustain. Agric. 4(1): 29–33. doi: 10.26480/mjsa.01.2020.29.33
Vasudevan, K., Cruz, C. M. V., Gruissem, W., and Bhullar, N. K. (2014). Large scale germplasm screening for identification of novel rice blast resistance sources. Front. Plant Sci. 505, 1–9. doi: 10.3389/fpls.2014.00505
Venkateswarlu, N., Vijaya, T., Bhargav, D. S., Chandramouli, K., Pragathi, D., Anitha, D., Reddy, V. N., and Sreeramulu, A. (2013). In vitro inhibitory effects of medicinal plants extracts on Sclerotium oryzae- a fungi causing stem rot disease in paddy. Int J. Pharm. Bio. Sci. 3, 147–151.
Wen-Ching, C., Tai-Ying, C., Aileen, L. D., and Chien-Sen, L. (2021). The control of rice blast disease by the novel biofungicide formulations. Sustainability 11, 1–9. doi: 10.3390/su11123449
Keywords: bioagents, rice blast disease, plant extracts, fungicides, management
Citation: Persaud R, Saravanakumar D, Persaud M and Seepersad G (2021) Biologicals and New Generation Fungicides in the Management of Blast Disease in Rice. Front. Sustain. Food Syst. 5:797441. doi: 10.3389/fsufs.2021.797441
Received: 18 October 2021; Accepted: 12 November 2021;
Published: 14 December 2021.
Edited by:
Sandeep Kumar, Indian Institute of Pulses Research (ICAR), IndiaReviewed by:
Chetan Kumar Jangir, National Research Centre on Seed Spices (ICAR), IndiaRam Swaroop Bana, Indian Agricultural Research Institute (ICAR), India
Copyright © 2021 Persaud, Saravanakumar, Persaud and Seepersad. This is an open-access article distributed under the terms of the Creative Commons Attribution License (CC BY). The use, distribution or reproduction in other forums is permitted, provided the original author(s) and the copyright owner(s) are credited and that the original publication in this journal is cited, in accordance with accepted academic practice. No use, distribution or reproduction is permitted which does not comply with these terms.
*Correspondence: Rajendra Persaud, cmFqcGVyc2F1ZDI1QHlhaG9vLmNvbQ==; cnBlcnNhdWRAZ3JkYi5neQ==