- 1Tecnologico de Monterrey, Escuela de Ingeniería y Ciencias, Zapopan, Jalisco, Mexico
- 2Tecnologico de Monterrey, The Institute for Obesity Research, Zapopan, Jalisco, Mexico
Overweight and obesity are two of the world's biggest health problems. They are associated with excessive fat accumulation resulting from an imbalance between energy consumed and energy expended. Conventional therapies for obesity commonly include synthetic drugs and surgical procedures that can lead to serious side effects. Therefore, developing effective, safe, and readily available new treatments to prevent and treat obesity is highly relevant. Many plant extracts have shown anti-obesogenic potential. These plant extracts are composed of different agriceuticals such as fibers, phenolic acids, flavonoids, anthocyanins, alkaloids, lignans, and proteins that can manage obesity by suppressing appetite, inhibiting digestive enzymes, reducing adipogenesis and lipogenesis, promoting lipolysis and thermogenesis, modulating gut microbiota and suppressing obesity-induced inflammation. These anti-obesogenic agriceuticals can be enhanced in plants during their cultivation by applying sustainable fertilization strategies, improving their capacity to fight the obesity pandemic. Biofertilization and nanofertilization are considered efficient, eco-friendly, and cost-effective strategies to enhance plant growth and development and increase the content of nutrients and bioactive compounds, representing an alternative to overproducing the anti-obesogenic agriceuticals of interest. However, further research is required to study the impact of anti-obesogenic plant species grown using these agricultural practices. This review presents the current scenario of overweight and obesity; recent research work describing different plant species with significant effects against obesity; and several reports exhibiting the potential of the biofertilization and nanofertilization practices to enhance the concentrations of bioactive molecules of anti-obesogenic plant species.
Introduction
Overweight and obesity are among the most challenging global health problems, with economic and social costs increasing at alarming rates worldwide (El-Shiekh et al., 2019; Karri et al., 2019; Sun et al., 2021; World Obesity, 2022). It is a multifactorial problem resulting from the excessive energy storage in the form of lipids in the adipocytes, which can lead to numerous life-threatening diseases associated with abnormal body fat accumulation, including type 2 diabetes, dyslipidemia, cardiovascular diseases, osteoarthritis, psychiatric pathologies such as depression, anxiety and mental disorders, obstructive sleep apnea, and certain types of cancer (Bautista et al., 2019; El-Shiekh et al., 2019; Islam et al., 2020). These nutritional pathologies enormously impact people's mortality, morbidity, and quality of life (Sun et al., 2021).
Conventional therapies for the management of obesity generally involve the use of synthetic drugs and surgical procedures. However, their availability, chances of recurrence, and harmful side effects limit their utilization. Hence, it is important to discover and develop effective, safe, affordable, and readily available alternatives to treat obesity (El-Shiekh et al., 2019; Karri et al., 2019). Researchers have proven many plant extracts with potential preventive and therapeutic effects against obesity and negligible or null side effects (Bautista et al., 2019; Karri et al., 2019; Islam et al., 2020). Plant-derived medicines have gained much attention due to their beneficial effects on maintaining health and combating diseases; particularly, plants contain many agriceuticals with anti-obesogenic effects, including fibers, phenolic acids (e.g., coumaric, caffeic acid, and chlorogenic acids), flavonols (e.g., quercetin and kaempferol), flavones (e.g., tricetin, apigenin, and luteolin), isoflavonoids (e.g., daidzein, genistein, and glycitein), flavans-3-ol (e.g., catechin), phytosterols, anthocyanins, alkaloids (e.g., caffeine and capsaicin), curcuminoids, lignans, proteins, and fibers (El-Shiekh et al., 2019; Karri et al., 2019; Islam et al., 2020; Sun et al., 2021). Agriceuticals participate in obesity management by different mechanisms, including inhibition of appetite, lipid and carbohydrate digestion, adipogenesis and lipogenesis, promotion of lipolysis and thermogenesis, and modulation of gut microbiota, which are among the most widely studied mechanisms used to evaluate and establish the anti-obesity potential of different agents (El-Shiekh et al., 2019; Karri et al., 2019; Shang et al., 2021). For example, carrot (Daucus carota L.) extract has shown anti-obesity potential by inhibiting pancreatic lipase (IC50 = 1.63 ± 0.07 mg/mL), a key enzyme for the absorption of dietary fats (Marrelli et al., 2020).
Furthermore, proper management of agronomic practices such as biofertilization and nanofertilization during plant cultivation can significantly stimulate plants' metabolism to overproduce the anti-obesogenic agriceuticals of interest. Additionally, these fertilization methods improve plant growth and development and enhance the efficiency of agricultural inputs, contributing to the development of sustainable agroecosystems (Singh et al., 2021). The latter is of vital importance since the current agricultural system demands urgent actions to improve crop productivity and restore the environmental degradation of agroecosystems. Therefore, implementing new sustainable technologies such as bio- and nanofertilization practices is of significant relevance (Prasad et al., 2019; Akhtar et al., 2022). Biofertilizers involve the application of plant growth-promoting microorganisms (PGPM) with multiple functions, including mobilization of soil nutrients, production of plant growth regulators, and plant protection from phytopathogens. While nanofertilizers consist mainly of nanosized minerals produced through mechano-physical, chemical, and biological methods, nanofertilizers improve plant nutrient uptake and assimilation, and enhance plant nutrition. Several research studies have been conducted to increase the understanding of the diversity, mechanisms of action, and dynamics of soil microbial communities, as well as different nanomaterials, focusing on their beneficial roles in improving the agricultural productivity and the medicinal and bioactive potential of plants (Prasad et al., 2019; Akhtar et al., 2022; Mahapatra et al., 2022).
Dietary intake of enriched anti-obesogenic plants could be a viable strategy for preventing and treating obesity (Islam et al., 2020). Therefore, this review presents an overview of recent research reporting different plant species exhibiting significant effects against obesity. Also, we propose the implementation of sustainable agricultural practices to enhance the agriceutical content of anti-obesogenic plant species. These strategies could help to combat the obesity pandemic and prevent its consequences.
Current scene of overweight and obesity in the world
Overweight and obesity are defined as abnormal or excessive fat accumulation that results from an imbalance between energy consumed and energy expended and represent a health risk; people are now increasingly consuming energy-dense foods with high sugar and fat content while decreasing physical activity due to the growingly sedentary lifestyle and urbanization, and changing modes of transportation (Shang et al., 2021; WHO, 2021a,b). Overweight and obesity are most regularly measured using the body mass index (BMI) scale, a simple weight-for-height index, which is used to determine whether a person is considered underweight, healthy, overweight, or obese. It is calculated by dividing the weight (in kilograms) by the square of the height (in meters) (Kg/m2). An adult with a BMI equal to or >25 is determined as overweight, while a BMI of 30 or greater is considered obese, and the healthy weight goes from 18.50 to 24.99. BMI is the most useful measure for adult overweight and obesity at the population level as it is the same standard for both sexes and all ages (WHO, 2021b). In the case of children and adolescents, the BMI scale is also used to measure body weight. However, BMI scores are interpreted and treated differently since factors such as age, gender, and sexual maturation significantly influence the body composition of younger individuals. The World Health Organization (WHO) developed Child Growth Standards that describe the physiological growth of children under 5 years of age; standards include specific percentiles and standard deviations that are analyzed according to age and sex to determine obesity or overweight. Child growth is universally used as an important reference for the population's nutritional status and health. Childhood obesity is linked to a higher chance of obesity, premature death, and disability in adulthood (Ritchie and Roser, 2019; WHO, 2019, 2021b).
Overweight and obesity affect people in many countries. Global obesity rates are growing because of the high availability of high-calorie foods and increasingly sedentary lifestyles; it has changed from being a problem in high-income countries to extending across all income levels (Ritchie and Roser, 2019; OECD, 2021). Numerous families are unable to access or afford sufficient nutritious foods like vegetables, fruits, legumes, meat, and eggs, while foods with high fat, sugar, and salt contents are more readily available and cheaper, leading to a significant rise in the numbers of children and adults with obesity and overweight in high-, middle-, and low-income countries, especially in urban settings (WHO, 2021a). It is calculated that around 2 billion adults worldwide are overweight, of which 650 million are listed as obese (BMI ≥ 30 kg/m2), meaning that 39% of adults aged 18 or over are overweight, with 13% being obese (Figure 1) (World Obesity, 2022). In the case of children and adolescents, around 18% or 340 million people aged 5–19 and an estimated 5.6% or 38.3 million children under 5 years are affected by overweight or obesity (WHO, 2019, 2021a; World Obesity, 2022). The global prevalence of obesity in adults has almost tripled between 1975 and 2016; among children and adolescents (aged 5–19), it has grown substantially from 4 to 18% in the same time lapse (World Obesity, 2022). If this trend continues, it is expected to reach 2.7 billion overweight and over 1 billion obese adults by 2025, counting 177 million people severely affected by obesity (World Obesity, 2022).
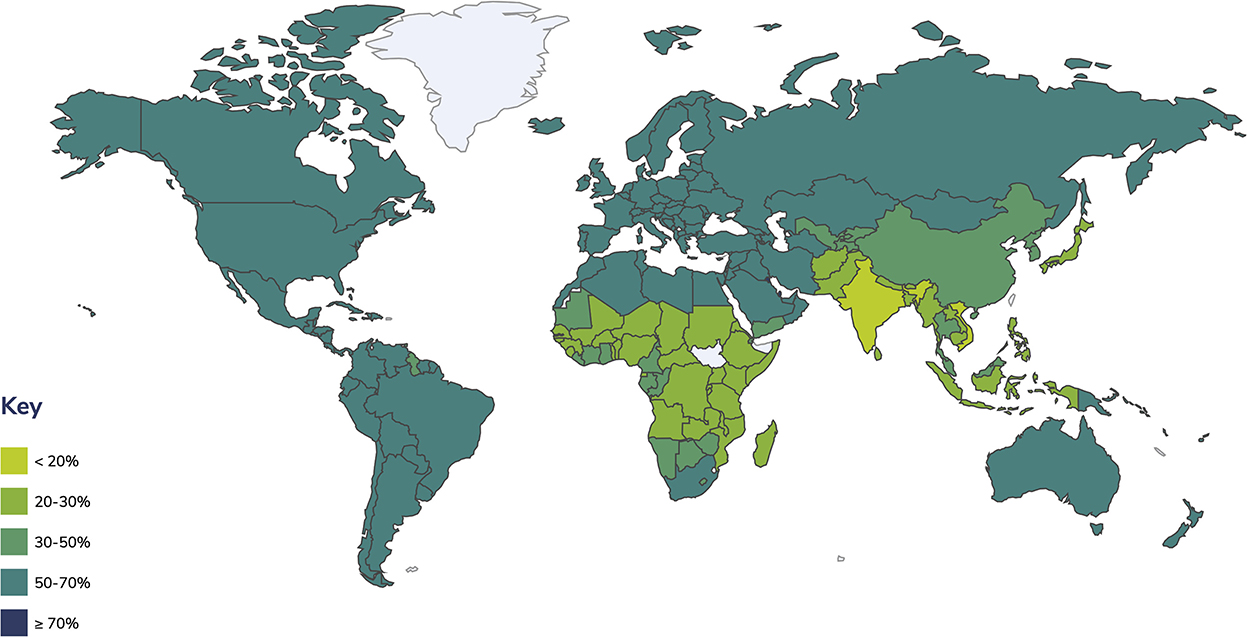
Figure 1. Prevalence of overweight and obesity in adults. Estimates include adults with a BMI ≥ 25 kg/m2 (WHO, 2020).
High body fat is a significant risk factor for several non-communicable diseases (NCDs), including diabetes, cardiovascular diseases, musculoskeletal disorders, and certain cancers (OECD, 2021; Shang et al., 2021). The risks for most obesity-related NCDs depend in part on the age of onset and duration of obesity (WHO, 2019). Obese children and adolescents are at high risk of both short- and long-term health consequences, primarily diabetes, hypertension and cardiovascular disease (mainly heart disease and stroke), musculoskeletal disorders (particularly osteoarthritis and increased risk of fractures), and cancers of the endometrium, breast, and colon (WHO, 2019). In the next 30 years, overweight-related diseases are expected to reduce life expectancy by 2.7 years on average. Obesity is considered one of the major risk factors for early death. According to the Global Burden of Disease study, around 8.15% of worldwide deaths were attributed to obesity in 2019, representing 5.02 million premature deaths due to obesity. Death rates are high (more than 15%) across middle-income countries, particularly Eastern Europe, North Africa, Central Asia, and Latin America, mainly caused by a high prevalence of obesity and poorer healthcare systems compared to high-income countries with also high levels of obesity but about half the deaths of middle-income countries (Ritchie and Roser, 2019). Obesity ranks fifth on the causes and risk factors for death; in addition, obesity is linked to several of the world's leading causes of death, including the first and fourth death causes that correspond to high blood pressure and high blood sugar, respectively (Ritchie and Roser, 2019; OECD, 2021).
The most common weight-loss treatments for obesity include pharmacotherapy, diet modification, surgery, and exercise. However, conventional therapies frequently result in unsatisfactory outcomes or side effects that can impair health (Cao et al., 2019). Therefore, research on new natural alternatives to prevent and manage obesity is particularly interesting.
Potential plant species for the formulation of anti-obesogenic dietary supplements
Recently, the development and consumption of dietary supplements from edible and medicinal plants have become very popular globally. Dietary supplements are products used to supplement nutritional components such as proteins, vitamins, minerals, botanical compounds, and probiotics (Ahmad et al., 2020). Many plants are used as dietary supplements to prevent and treat obesity, including green tea, ginger, aloe vera, and lingonberry (Liu et al., 2019; Shang et al., 2021; Si et al., 2021). Bioactive components derived from plants such as capsaicin, sulforaphane, resveratrol, anthocyanins, kaempferol, and quercetin have shown anti-obesogenic activity (Si et al., 2021; Çakir et al., 2022). Anti-obesogenic mechanisms include appetite suppression, reduction of lipid and carbohydrate absorption, regulation of lipid metabolism, inhibition of adipogenesis and lipogenesis, increase in energy expenditure, improvement of obesity-related inflammation, and modulation of gut microbiota (Figure 2) (Shang et al., 2021).
Suppression of appetite
Suppressed appetite reduces food consumption and prevents overeating and energy intake; it is considered an effective way to improve weight loss (Shang et al., 2021). Thorning et al. (2020) investigated the effect of daily intake of a dietary fiber obtained from a plant cell wall material rich in dietary fibers from potato pulp containing 68% fiber (5.5% soluble and 76.7% insoluble fibers, and 17.8% resistant starch). Results indicated that potato fibers increased the composite satiety score (5% ± 2%), reduced food consumption (6% ± 2%), and desire to eat (6% ± 3%). Also, it produced higher satiety and fullness. Ginseng soluble dietary fiber has also exhibited enhanced glucolipid metabolism levels (mainly on triglycerides), increased satiety, and retarded gastric emptying by modulating the levels of appetite hormones (glucagon-like peptide-1, ghrelin, peptide YY, and cholecystokinin), and improved intestinal structures and the concentrations of fecal short-chain fatty acids (SCFAs) (especially acetic and butyric acids) (Hua et al., 2021). Ma et al. (2021) found that potato dietary fiber modified enzymatically with cellulase and xylanase also improved the production of short-chain fatty acids (SCFAs) of C57BL/6 mice, including the acetic acid (implicated with growth inhibition of tumor cells), n-butyric and iso-butyric acids (energy source important to maintain the stability of intestinal epithelial cells and prevent colorectal cancers), and valeric and iso-valeric acids (effective inhibitor of pathogenic Clostridium), while markedly suppressed the propionic acid production (associated to adverse effects on the immune response). Fernández-Raudales et al. (2018) identified that plant-based protein and soluble corn fiber are important ingredients for formulating high-protein and soluble fiber beverages for weight management. They found significant effects in promoting satiety and controlling appetite; soluble fiber increases gastric distension and reduces the rate of gastric emptying, improving the production of satiety hormones and increasing the satiety sensation, while protein is considered the most satiating macronutrient. Sharafi et al. (2018) obtained similar results using a high-protein, high-fiber beverage on appetite ratings and energy intake.
Yimam et al. (2019a) studied flavonoids (Kuwanon G and Albanin G) isolated from root barks of the medicinal plant Morus alba on appetite suppression and body weight management. The bioflavonoid extract was evaluated in acute food intake in rats and long-term food intake reduction in obese C57BL/6J mice, discovering significant reduction (44.3–58.6%) in both experiments. In the long-term study, the authors observed a 20% decrease in daily calorie intake and 16.5% weight loss compared to baseline. Important reductions in visceral fat deposits and biochemical markers were also identified. In another study, Yimam et al. (2019b) tested a plant-based weight-management product in an in vivo acute food intake study in rats. The product was produced with a mixture of a Magnolia officinalis bark extract, Morus Alba root bark extract, and Ilex paraguariensis leaf extract, resulting in a lignan-, flavonoid-, phenolic-, and caffeine-rich dietary supplement capable of reducing 88.5, 73.8, and 63.1% food intake at 1 h, 2 h, and 4 h after food provision, respectively.
Neurotransmitters and hormones are the central molecules controlling appetite, hunger, and satiety. They act in the hypothalamus regulating food consumption. For instance, leptin, which is a hormone produced and secreted in adipocytes, acts to inhibit caloric intake by signaling that there is sufficient adipose tissue; it increases satiety and energy expenditure. Leptin acts mainly on two neurotransmitters: neuropeptide Y (NPY) (promotes food intake and delays satiety) and melanocortin (catabolic and anorectic activity) (Shawky and Segar, 2018; Shang et al., 2021). Sulforaphane, an isothiocyanate obtained from cruciferous vegetables (e.g., cabbage, broccoli, kale, radish, cauliflower), improves the response to leptin injected intraperitoneally in diet-induced obese mice, representing an important opportunity for the treatments of obesity, involving exogenously administered leptin, sulforaphane reduced the cumulative food intake and weight gain significantly (Shawky and Segar, 2018). Çakir et al. (2022) revealed similar results: sulforaphane reduced obesity by inducing anorectic responses (loss of appetite), reversing leptin resistance, suppressing fatty acid synthesis, and reducing inflammation and ROS accumulation.
People try to treat obesity or lose weight with restrictive diets, but the resultant hunger can lead to overeating; suppression of appetite with anti-obesity compounds from enriched agricultural products represents an alternative to reduce discomfort and facilitate the process of weight loss (Shang et al., 2021).
Inhibition of digestive enzymes to reduce the absorption of carbohydrates and lipids
One of the most critical processes in the development of obesity is the metabolism of carbohydrates and lipids, in which the associated digestive enzymes are essential for their absorption (Shang et al., 2021). Lipases hydrolyze dietary lipids into monoglycerides and fatty acids, which are absorbed in the small intestine and used to resynthesize lipids, increasing fat accumulation in the body. In the same way, carbohydrates are hydrolyzed into monosaccharides and disaccharides by enzymes including α-amylase and α-glucosidase, and a fraction of the monosaccharides are used to produce glycogen, which is then converted into fat (Rajan et al., 2020; Shang et al., 2021). Therefore, using edible and medicinal plants to inhibit digestive enzymes effectively reduces lipid and carbohydrate absorption and prevents obesity (Marrelli et al., 2018; Kamoun et al., 2019; Bajes et al., 2020). Pancreatic and gastric lipases are among the most relevant targets in obesity treatments. Orlistat is a synthetic anti-obesity drug that inhibits gastric and pancreatic lipases, it blocks 30% absorption of dietary fats, but its use has gradually declined due to its side effects, including constipation, vomiting, insomnia, headache, emesis, and stomachache. Thus, there is a growing demand for alternative lipase inhibitors (Marrelli et al., 2018). Kamoun et al. (2019) investigated a new potent gastric lipase inhibitor from an ethanolic extract of star anise (Illicium verum L.); it was identified as the flavonoid myricitrin-5-methyl ether showing 81% lipase inhibitory activity. Marrelli et al. (2018) evaluated a methanolic extract of Moricandia arvensis (L.) on inhibiting pancreatic lipase. Flavonoid glycosides were the major specialized constituents of such extract (mainly quercetin and kaempferol), with kaempferol-3-O-β-(2″-O-glucosyl)-rutinoside and kaempferol-3-O-α-arabinosyl-7-O-rhamnoside being the most abundant flavonols; the crude methanolic extract exhibited a potent inhibitory activity, with an IC50 value of 2.06 ± 0.02 mg/mL. A protein from the Litchi chinensis seed at 100 μg/mL could also inhibit 68.2% of the pancreatic lipase activity, with an IC50 of 73.1 μg/mL (Mhatre et al., 2019). Bajes et al. (2020) described that polysaccharides (e.g., pectin) could reduce fat absorption in the gastrointestinal tract by changing the characteristics of gastrointestinal fluids, restricting pancreatic lipase activity (competitive inhibition), and affecting the fat aggregation state.
Interfering glucose absorption through inhibiting carbohydrate-hydrolyzing enzymes is another effective way to combat obesity and stabilize blood glucose (Khadayat et al., 2020). Ethanolic extracts of Bauhinia forficata Link leaves have shown prominent inhibitory potential against α-amylase (IC50 value 0.1 ± 0.02 μg/mL), α-glucosidase (IC50 value 0.18.2 ± 3.3 μg/mL) and lipase (IC50 value 18.6 ± 1.0 μg/mL), their phytochemical constitution presented high polyphenols concentrations (mainly flavonoids). In addition, extracts showed high antioxidant and antiglycation activities, without cytotoxic and hemolytic effects (Franco et al., 2020). Taviano et al. (2020) reported up to 100% inhibition of α-glucosidase using a hydroalcoholic extract from the aerial parts of Matthiola incana (L.), compared to the antidiabetic drug acarbose. Tuber aqueous and ethanolic extracts of Kedrostis africana (L.) also showed α-amylase (IC50 = 439.45 μg/mL), α-glucosidase (IC50 = 157.99 μg/mL), and lipase (IC50 = 381.86 μg/mL) inhibitory activities (Unuofin et al., 2018).
Thengyai et al. (2020) evaluated several medicinal plants used against diabetes mellitus. They found the highest α-glucosidase and α-amylase inhibitory activity using ethanolic extracts of Vitex glabrata, Terminalia catappa, Salacia chinensis, Senna siamea, and Phyllanthus amarus. The most bioactive plant was the stem bark of V. glabrata, identifying six known compounds: lupeol, β-amyrin, α-amyrin, betulin, betulinic acid, and scopoletin. Lupeol (IC50 7.4 μM) and β-amyrin (IC50 32.33 μM) exhibited potent inhibition of α-glucosidase and α-amylase. Khadayat et al. (2020) also screened 32 medicinal plants for α-amylase inhibitory activity. Methanolic extracts of Acacia catechu, Dioscorea bulbifera, and Swertia chirata showed IC50 values of 49.9, 296.1, and 413.5 μg/mL, respectively. Salvia eriophora leaves were also detected with potent inhibition against α-amylase and α-glucosidase, attributing their effect to the major compounds identified in water (salvigenin, fumaric acid, and quercetagetin-3.6-dimethylether) and ethanolic (fumaric acid, caffeic acid, and epicatechin) extracts (Bursal et al., 2019).
In another study, Jaradat et al. (2019) measured α-amylase and α-glucosidase enzymes' inhibitory activities of Carlina curetum to understand its anti-obesity effects. Results indicated that aqueous and methanolic extracts had significant α-amylase inhibitory effects with IC50 values of 21.37 ± 0.31 and 30.2 ± 0.42 μg/mL, respectively, in comparison with Acarbose. At the same time, the methanolic fraction revealed effective α-glucosidase inhibition with an IC50 value of 27.54 ± 4.28 μg/mL. Finally, Shori (2020) formulated and evaluated four types of yogurt prepared with water extracts of rosemary, dill, oregano, and ginger; herbal-yogurts presented 74.45–77.83% α-amylase inhibition (approximately five times higher than the control). Rosemary and oregano increased the total phenolic content of yogurt significantly, showing significant antioxidant activity after 14 days of storage. Other bioactive compounds described with potential inhibitory effects on digestive enzymes include flavonoids, anthocyanins, saponins, and alkaloids (Elbashir et al., 2018; Jaradat et al., 2019; Shang et al., 2021).
Inhibition of adipogenesis and lipogenesis
Different studies have demonstrated the potential of several plant species in inhibiting the formation of adipocytes from adipose stem cells (ASCs) and suppressing fatty acid synthesis and subsequent triglyceride production in adiposities (Kersten, 2001; Shang et al., 2021). Obesity is associated with the hypertrophy of fat cells and the development of new adipocytes from preadipocytes due to unbalanced and prolonged energy intake (Hussain et al., 2019). Inhibition of adipogenesis and lipogenesis has been a therapeutic target in fighting obesity and obesity-related disorders (Sharma et al., 2018). Inhibition or regulation of adipogenesis is commonly evaluated using the preadipocyte murine 3T3 L1 cell line. C3H10T1/2 is an alternative cell line that can assess anti-adipogenic activity; it corresponds to multipotent embryonic mesenchymal stem cells (MSC) that can be differentiated into adipocytes (Hussain et al., 2019).
The process of adipogenic differentiation is generally under the tight control of several transcription factors, including C/EBPβ/δ (enhancer-binding protein) and PPARγ (peroxisome proliferators-activated receptor gamma), among which the transcription factor PPARγ plays the most crucial role during MSC differentiation to mature fat cells, multiple co-modulators and transcription factors have been involved as regulators of the expression and function of PPARγ (Kawai and Rosen, 2012; Datta et al., 2017). Production of TG in mature adipocytes is induced when there is an expression of adipogenic transcriptional factors such as PPARγ/CCAT/C/EBP, regulating fatty acid synthase (FAS) and acetyl-CoA carboxylase (ACC) (Hussain et al., 2019).
Phytochemicals, particularly polyphenols such as resveratrol, apigenin, quercetin, and myricetin, bind to specific residues of the PPARγ receptor (Phe264, His266, Ile281, Cys285, and Met348) to suppress adipogenesis at early stages of cell differentiation (Aranaz et al., 2019). For instance, the Viburnim opulus fruit extracts demonstrated significant inhibition of adipogenic differentiation in 3T3-L1 cells. Using extracts at 75 μg/mL concentration was sufficient to suppress lipid accumulation at nearly 20% and change the size of lipid droplets. In addition, the Viburnim opulus extract reduced the intracellular ROS production by 20% and the leptin levels by 21–30%. Extracts contained 24 phenolic compounds with chlorogenic acid as the quantitatively dominant compound (Podsedek et al., 2020). Hussain et al. (2019) extracted phenolic compounds from canola (Brassica napus L.) meal using different solvents to assess anti-adipogenic activity on the C3H10T1/2 cell line, 80% acetone extract showed significant adipogenesis inhibitory activity and downregulated the gene expression of the transcription factor PPARγ, its characterization revealed antioxidant-rich composition including kaempferol and sinapic and ferulic acids as the main bioactive phenolic compounds. Clitoria ternatea flower petal extract, commonly used as a natural food colorant, also could inhibit the adipogenesis process and lipid accumulation in the preadipocyte 3T3-L1 cell line; at the early stages, extract inhibited proliferation and delayed cell cycle by downregulating the expression of phospho-ERK1/2 and phospho-Akt signaling pathway, and at later stages, it suppressed differentiation of preadipocytes through reducing expression of transcription factors PPARγ and C/EBPα. Subsequently, FAS and ACC enzyme activity was altered, diminishing TG accumulation (Chayaratanasin et al., 2019). In addition, Allium hookeri aqueous root extract exhibited inhibition of lipid accumulation and adipogenesis by downregulating gene expression of transcription factors CCAAT/EBPα and hormone-sensitive lipase (HSL) and lipoprotein lipase (LPL) enzymes in 3T3-L1 preadipocytes, also oral administration of the extract markedly reduced body weight gain, adipose tissue weight, adipocyte cell size and serum leptin levels in high-fat diet (HFD)-induced obese mice, additionally the extract significantly suppressed lipogenesis by decreasing hepatic mRNA expression levels of 3-hydroxy-3-methylglutaryl CoA (HMG-CoA) reductase, low-density lipoprotein receptor (LDLR) and sterol regulatory element-binding transcription factor (SREBP)-2 which correspond to cholesterol synthesis genes, and SREBP-1c and FAS associated with fatty acid synthesis (Kim H. J. et al., 2019).
Sharma et al. (2018) investigated Garcinia cambogia extract mixed with pear pomace extract in the adipogenesis of 3T3-L1 preadipocytes. The mixture exerted a synergistic effect in reducing the expression of C/EBPα, PPARγ, and FAS. Furthermore, other studies have also demonstrated in vitro and in vivo inhibition of adipogenesis through the downregulation of adipogenic-related factors such as PPARγ, C/EBPα/β, SREBP1c, FAS, and ACC, using various plant extracts, including Opuntia streptacantha and Opuntia ficus-indica (Héliès-Toussaint et al., 2020), Citrullus colocynthis (Jemai et al., 2020), Cucurbita ficifolia (Alshammari and Balakrishnan, 2019), Citrus aurantium (bitter orange) (Park et al., 2019), Punica granatum (pomegranate) (Sorrenti et al., 2019), Zea mays var. indurate (purple maize) (Zhang et al., 2019), Mangifera indica (mango) (Fang et al., 2018), Aronia melanocarpa (chokeberry) (Kim N. H. et al., 2018), Cissus quadrangularis (Lee et al., 2018), and Chrysanthemum indicum (Nepali et al., 2018).
Regarding lipogenesis, sunflower (Helianthus annuus L.) seed extract has shown anti-lipogenesis properties in HFD-induced obese mice by significantly reducing body weight gain, adipose tissue weight and mass, the cell size of adipocytes, and serum levels of triglycerides, total cholesterol (TC), very low-density lipoprotein (VLDL)/LDL-cholesterol, alanine aminotransferase, and aspartate aminotransferase (AST). In addition, extract supplementation decreased the expression of proteins associated with lipogenesis pathways and improved the expression of lipolysis and thermogenesis-related pathways in the adipose tissue (Yun et al., 2022). He et al. (2018) evaluated the inhibitory effect of Camellia euphlebia flower extracts on lipogenesis. Results indicated that treatments significantly reduced triglyceride levels and lipid accumulation and effectively downregulated mRNA expression of FAS, HMG-CoA reductase, and glycerol-3-phosphate acyl transferase (GAPAT). Aged black garlic extract has also suppressed lipogenesis and triggered lipolysis by decreasing protein expression of PPARγ, perilipin, hormone-sensitive lipase (HSL), and Ser563-pHSL in mature 3T3-L1 adipocytes (Nam et al., 2018).
Agriceuticals play an essential role in the cell differentiation and proliferation of adiposities; therefore, consuming anti-adipogenic plants could be a relevant strategy to prevent and manage obesity (Alshammari and Balakrishnan, 2019).
Regulation of lipid metabolism in adipocytes
Lipolysis is a metabolic process that consists of the breakdown of triglycerides into their constituents: glycerol and free fatty acids (FFAs), the main enzymes acting in lipolysis include adipose triglyceride lipase (ATGL), hormone-sensitive lipase (HSL), monoglyceride lipase (MGL) and lipoprotein lipase (LPL). The glycerol is then used as a carbon source for gluconeogenesis, while the FFAs are transported in the blood and oxidized in tissues to generate energy through the β-oxidation process (Grabner et al., 2021). Most TGs are accumulated in adipocytes. Therefore, the promotion of their hydrolysis and the degradation of FFAs in adipocytes are essential to inhibit the growth of white adipose tissue (WAT) and reduce the size of adipocytes (Grabner et al., 2021; Shang et al., 2021).
An extract of unripe Rubus coreanus containing ellagic acid promoted lipolytic effects mediated by the downregulation of adipogenic and lipogenic gene expression in HFD-induced obese C57BL/6 mice (Kim K. J. et al., 2020). Treated mice exhibited significant body weight and adipose tissue mass reductions compared to HFD-fed mice; lipolysis-associated proteins, including ATGL, phosphorylated-HSL, and PLIN1, significantly increased in the mice epididymal white adipose tissue (Kim K. J. et al., 2020). Another study with Moringa oleifera Lam. leaf extract elucidated its capacity to inhibit fat accumulation based on its lipolytic and anti-adipogenic properties. In vitro experiments in 3T3-L1 adipocytes showed upregulation of the expression of the lipolysis-associated protein HSL and downregulation of adipogenesis-related proteins PPARγ, CCAAT/EBPα/β, and FAS at 400 μg/mL extract concentration. They found that the inhibition of fat accumulation is also related to the activation of the AMPK pathway; in vivo studies evaluating the extract at 0.5 g/kg body weight (BW) in HFD mice indicated that treatments significantly reduced body weight, epididymal, perirenal, and mesenteric fat weight, fat tissue size, hepatic fat accumulation, and the serum levels of TC, LDL-cholesterol, and AST, also significantly downregulated the expression of PPARγ and FAS and upregulated the lipolysis-related protein adipose triglyceride lipase (ATGL). The phytochemical characterization revealed the presence of isoquercitrin, chrysin-7-glucoside, and quercitrin as the dominant constituents in the extracts; the three compounds showed synergistic effects to inhibit adipogenesis, but particularly, quercitrin exhibited important inhibitory activity of fat accumulation (Xie et al., 2018).
Kim Y. J. et al. (2019) found that treating HFD-induced obese mice with a root ethanolic extract of Platycodon grandiflorus regularized and controlled their body weight and fat mass by increasing lipolysis [particularly by improving perilipin 1 (PLIN1), ATGL, and HSL expression] and fatty acid oxidation. Treatments also promoted the browning process of epididymal white adipose tissue by modifying the browning-related gene expression and the enzyme activity of carnitine palmitoyltransferase (CPT1A). The root water extract of Allium hookeri also reduced serum triglyceride levels, adipose tissue weight, and adipocyte cell size in HFD-induced obese mice due to upregulating gene of CPT1A, which is involved in fatty acid β-oxidation in the liver (Kim H. J. et al., 2019). Coffee (Coffea arabica L.) fruit containing chlorogenic, caffeoylquinic, caffeic, malic, and quinic acids has also shown inhibitory adipogenesis and lipolytic activities in 3T3-L1 adipocytes; coffee reduced lipid accumulation by nearly 47% (Duangjai et al., 2018).
Wei et al. (2018) found that grape seed procyanidin extract limits porcine preadipocyte differentiation and proliferation, as demonstrated by decreased lipid accumulation, reduced mRNA expressions of PPARγ and fatty acid–binding protein 4 (FABP4), diminished enzymatic activity of glycerol-3-phosphate dehydrogenase (GPDH) (key enzyme for triacylglycerol biosynthesis), increased expression of preadipocyte factor-1, and induced G0/G1 adipocyte cell cycle arrest, extracts also promoted lipolysis of mature adipocytes as seen by attenuated lipid content, increased release of FFAs and glycerol, and improved expression of mRNA of important lipolytic transcription factors including HSL and ATGL. In addition, Oroxylum indicum (L.) Kurz fruit pods extract, mainly composed of flavonoids, glycosides, alkaloids, steroids, and tannins, has demonstrated dose-dependent inhibition of preadipocyte to adipocyte differentiation process, a reduction of lipid accumulation and an inhibitory effect on lipase activity without reducing cell viability in 3T3-L1 preadipocytes (Hengpratom et al., 2018).
Promotion of thermogenesis
Increasing thermogenesis is an effective way to increase energy expenditure and manage obesity (Shang et al., 2021). Thermogenesis is the process that transforms chemical energy to generate heat and mainly occurs in thermogenic adipocytes, which are brown and beige adipocytes (Hussain et al., 2020; Milton-Laskíbar et al., 2020). One of the major contributors to thermogenesis in brown adipose tissue (BAT) is the uncoupling protein 1 (UCP1), which is located in the inner membrane of mitochondria and acts by uncoupling oxidative phosphorylation from the production of ATP. Its enhancement increases respiration and promotes the loss of energy in the form of heat (Hussain et al., 2020; Milton-Laskíbar et al., 2020; Shang et al., 2021). Brown adipocytes have numerous mitochondria and UCPs implicated in heat generation (Shang et al., 2021). White adipose tissue can also produce UCP1 by a process known as browning, which results in the generation of beige adipose tissue (Pan et al., 2019; Milton-Laskíbar et al., 2020).
Many plants have been reported to expend energy by promoting thermogenesis and helping to maintain energy balance without requiring physical activity (Shang et al., 2021). For instance, a co-treatment with a high-temperature processed-green tea (Camellia sinensis L.) extract carrying higher levels of epimerized catechins, combined with an enzymatically modified isoquercetin (hydrolyzed rutin, then transglycosylation reaction), increased the brown adipocyte marker UCP1 and COXIV mitochondrial protein levels by 2.9-fold, also the maximal and basal oxygen consumption improved by 1.57- and 1.39-fold, respectively, in brown adipocytes. The co-treatment administered to HFD-induced obese mice also increased the expression of brown adipocyte markers and mitochondrial proteins in adipose tissue. After 2 weeks, mice lost 3% body weight and 7.09% body fat, while energy expenditure increased by 8.95% (Im et al., 2022).
Bolin et al. (2020) also found that a polyphenol-rich green tea extract induced thermogenesis in mice, decreased body weight, adipocyte size, and insulin resistance, increased energy expenditure, BAT thermogenesis, and stimulated browning in subcutaneous WAT. Cinnamomum cassia extract, which main agriceuticals were identified as coumarin, cinnamic acid, cinnamaldehyde, and 2-methoxy cinnamaldehyde, significantly increased body temperature and energy expenditure by inducing thermogenesis in mice exposed to a cold environment. There was a significant rise in the thermogenic proteins UCP1, peroxisome proliferator-activated receptor-γ coactivator-1α (PGC1-α), and PRDM16 in adipose tissue. Particularly, protein expression of UCP1 increased by 4-fold compared to the control group. In addition, the expression of lipolysis protein pHSL was increased, the lipid droplets were reduced, and the mitochondrial number was also significantly higher in BAT (Li et al., 2021a).
Zhou et al. (2020) investigated the effects of a polyphenol-rich cranberry extract in HFD–fed obese C57BL/6J mice. After 16 weeks, the body weight of treated mice was 22.5% lower than in the HFD group, and the energy expenditure significantly increased, which was associated with the improvement of BAT thermogenesis and the browning process in WAT as revealed by the enhancement of mRNA expression of thermogenic genes (especially, Ucp1, which was 5.78 times higher than the HFD group) and browning-related genes (particularly, Cd137, which was 3.13 times higher than in the HFD group). Phytochemicals extracted from unripe Rubus coreanus increased thermogenesis-associated proteins, including PPARα, CPT1, UCP1, and PGC1α, in inguinal white adipose tissue of treated mice as compared to HFD-fed mice (Kim K. J. et al., 2020). Other in vivo and in vitro studies with similar results on the induction of thermogenesis and lipid metabolism, induce fat browning and increase fatty acid oxidation include the use of a flavonoid-rich Millettia speciosa root extract (Wang et al., 2022), resveratrol, oxyresveratrol and pterostilbene (Andrade et al., 2019; Pan et al., 2019; Milton-Laskíbar et al., 2020), capsaicin from chili pepper (Montanari et al., 2019), and Citrus aurantium (bitter orange) (Park et al., 2019).
Modulation of gut microbiota
Gut microbiota should be viewed as another organ in the human body since it significantly impacts host physiology and metabolism (Cao et al., 2019). The gut microbiota has been proven to play an essential role in obesity because it can influence several processes to control obesity, including inflammation, hormone regulation, energy harvest, and metabolic-related factors (Shang et al., 2021). Obese patients present lower gut microbial diversity than lean people; therefore, the presence and abundance of specific anti-obesogenic gut microbial species are altered in obese persons. Some species of Firmicutes, Lactobacillus, and Bacteroidetes have been positively linked to the development of obesity, while Bifidobacterium, most Lactobacillus species, and certain Bacteroidetes have shown anti-obesity effects (Cao et al., 2019).
In this context, agriceuticals can modulate the composition and abundance of gut microbiota to combat obesity (Shang et al., 2021). Recent findings revealed that several plants, such as apples, grapes, pomegranate, berries, turmeric, garlic, chili, soy, and sorghum, can inhibit obesity by increasing gut microbiota diversity and abundance, downregulating obesogenic gut microbiota, and upregulating species implicated in suppression of obesity (Cao et al., 2019). Administration of 200 mg/kg capsanthin for 12 weeks significantly increased microbial diversity and modulated the intestinal bacterial environment of HFD-induced obese C57BL/6J mice. rRNA sequencing of cecal microbiota indicated that capsanthin improved the abundance of Bacteroidetes, Bifidobacterium, Clostridium, Akkermansia, and Allobaculum, and reduced the abundance of Ruminococcus and the Firmicutes/Bacteroidetes (F/B) ratio compared to the HFD group. Interesting facts about these bacteria include that the abundance of Bifidobacteria is negatively correlated with BMI; Clostridium bolteae is in higher proportion in lean people (BMI < 20); Akkermansia, a gut commensal bacterium, has been reported to prevent obesity and improve intestinal inflammation and obesity-related insulin resistance; Ruminococcus bromii and Ruminococcus obeum are present in high proportion in obese people; and an increased F/B ratio is closely associated with obesity and diabetes (Wu et al., 2020).
Diez-Echave et al. (2020) investigated the effects of a polyphenolic extract of Hibiscus sabdariffa in HFD-fed obese mice. Its major components belonged to the family of organic acids (mainly hibiscus acid), phenolic acids (especially chlorogenic acid and its derivatives), and flavonoids (mainly derivates of quercetin, myricetin and kaempferol, and anthocyanins). The extract strengthened gut integrity by increasing the production of mucins and proteins implicated with mucosal barrier maintenance, exerted prebiotic function by counteracting the alterations and dysbiosis in gut microbiota made by the HFD (HFD increased Firmicutes from 38.3 to 62.9%, and reduced Bacteroidetes from 38.3 to 3.9%), significantly reduced the Firmicutes to Bacteroidetes (F/B) ratio (this ratio is an indicator of health status, a high rate reflects obesity-related intestinal dysbiosis), and positively modulated the gut microbiota composition. In addition, extracts improved weight loss, glucose tolerance, insulin sensitivity, regularized LDL/HDL cholesterol ratio, and reduced inflammation state in the liver by decreasing the expression of proinflammatory mediators and adipokines (Diez-Echave et al., 2020).
Moreover, ginseng soluble dietary fiber balanced the F/B ratio, promoted colon health, and significantly improved the proliferation of probiotics, including Bifidobacterium pseudolongum and Lactobacillus helveticus, which were correlated with glucolipid metabolism (Hua et al., 2021). Ma et al. (2021) used enzymatic-modified potato dietary fiber to regulate intestinal microbiota; the enzymatic treatment with cellulases and xylanases significantly enhanced the bioactivity of dietary fiber to treat dysbiosis of gut microbiota, treatments increased the abundance and diversity of gut microbiota of mice, and significantly reduced the F/B ratio.
Supplementation with a chlorophyll-rich spinach extract in HFD-fed mice also showed modulatory effects on intestinal microbiota, reversing the HFD-induced gut dysbiosis and increasing Bacteroidetes and Akkermansia (Li et al., 2019). In another study, an ellagitannin- and anthocyanin-rich extract of pomegranate was applied in combination with the probiotic strain Lactobacillus rhamnosus, substantially diminished triglyceride content and intracellular lipid accumulation in the 3T3-L1 cell line. Treatments also suppress mRNA expression of adipogenic transcriptional factors, and there was a synergistic effect between probiotics and polyphenols of the pomegranate extract. These findings provide insights for developing new nutraceutical/probiotic-based alternatives to manage obesity (Sorrenti et al., 2019). These findings are in agreement with other studies that reported important alternatives to improve the intestinal barrier integrity, exert prebiotic effects, or remedy obesity-associated gut microbiota dysbiosis and lipid accumulation by using blueberry polyphenol extract (Guo et al., 2019; Jiao et al., 2019; Si et al., 2021), ripened Pu-erh tea extract (Lu et al., 2019), 1% water extracts of green tea (catechin-rich), black tea (carrying brown/black pigments theaflavins and thearubigins), and oolong tea (containing green and black tea components), which mainly exerted their modulatory effect by inhibiting the production of lipopolysaccharides and increasing the formation of SCFAs (Liu et al., 2019), and citrus peel extract rich in polymethoxyflavones and hydroxylpolymethoxyflavones (Tung et al., 2018).
Suppression of obesity-induced inflammation
Obesity is associated with the development of chronic low-grade inflammation. As adipocytes increase in size and number to store excess triglycerides, they produce and release inflammatory mediators, including proinflammatory cytokines and adipokines, which promote the recruitment and infiltration of immune cells, mainly macrophages, T cells [αβ, γδ, natural killer T (NKT) cells, and regulatory T cells (Tregs), NK cells, B cells, and leukocytes] into adipose tissue, resulting in inflammation, insulin resistance, alterations of glucolipid metabolism, and dysfunction of innate and adaptive immune response (Xu C. et al., 2018; Terzo et al., 2020; Shang et al., 2021). Macrophages in adipose tissues are recognized as the primary source of proinflammatory cytokines, such as interleukin-6 (IL-6), interleukin-1β (IL-1β), and tumor necrosis factor α (TNF-α). Therefore, they are essential to inflammation development during obesity (Terzo et al., 2020).
Oxidative stress can be a precursor or a result of inflammation. It triggers the activation of signaling molecules such as the nuclear factor kappa-light-chain-enhancer of activated B cells (NF-κB), which promote the release of inflammatory cytokines (Xu C. et al., 2018). Some agriceuticals can attenuate obesity-induced inflammation (Shang et al., 2021). A Millettia speciosa root extract rich in flavonoids significantly decreased inflammatory-associated adipokines (IL-6 and TNF-α) levels in high-fat diet-induced obese C57BL/6 mice (Wang et al., 2022). Terzo et al. (2020) investigated the effect of chronic intake of pistachio to suppress obesity-associated inflammation in HFD-fed mice, they found significant reductions in the serum TNF-α and IL-1β levels, the number and area of adipocytes, and the mRNA expression of IL-1β, TNF-α, F4-80, and CCL-2 in subcutaneous and visceral adipose tissues, pistachio intake also reduced the F/B ratio and gut bacteria related with the development of inflammation including Oscillospira, Coprobacillus, Desulfovibrio, and Bilophila. In addition, Anthocyanin-rich tart cherry extract significantly reduced IL-6 levels and enhanced redox status by increasing antioxidant capacity and superoxide dismutase (SOD) activity in HFD-fed C57BL/6J mice (Nemes et al., 2019).
Noratto et al. (2019) evaluated the supplementation of quinoa (Chenopodium quinoa Willd.) in obese-diabetic mice. Quinoa consumption diminished plasma and liver cholesterol and lessened obesity-associated inflammation and oxidative stress by reducing protein carbonyls and interleukin (IL)-6. Olive (Olea europaea L.) leaf extract containing phenolic compounds, like oleuropeoside, has also exhibited anti-inflammatory effects in HFD-fed obese mice; the leaf extract lessened TNF-α, IL-1β, and IL-6 RNA expression in liver and adipose tissues (Vezza et al., 2019). Daily supplementation with aged garlic extracts also alleviated inflammation associated with obesity in 51 healthy obese adults; treated participants had significantly lower levels of serum IL-6 and TNF-α than placebo capsules. Extracts also modulated immune cell distribution (Xu L. et al., 2018). High-hydrostatic pressure extract of ginger roots reduced mRNA levels of proinflammatory cytokines, body weight, WAT mass, and serum and hepatic lipid levels, downregulated the expression of obesity-linked microRNA (miR)-21 and miR-132, and enhanced the AMPK activity in HFD-induced Sprague-Dawley rats (Kim S. et al., 2018). Kwon et al. (2018) elucidated the effects of long-term consumption of luteolin-enriched artichoke leaf in HFD-induced obese C57BL/6N mice. After 16 weeks, treated animals showed lower inflammation, as recognized by the decreased plasma IL-6, IL-1β, and plasminogen activator inhibitor-1 (PAI-1) levels.
Fertilization strategies to enrich anti-obesogenic agriceuticals
Interestingly, plants can be biofortified with anti-obesogenic agrichemicals during plant cultivation by applying different fertilization strategies, improving their capacity to fight obesity (Gastélum-Estrada et al., 2021; Jacobo-Velázquez, 2022). However, modern agriculture is responsible for severe environmental and health problems associated with overfertilization, soil degradation, reduction of agricultural lands, low plant nutrient-use-efficiency, water availability, and food security (Acharya and Pal, 2020; Khatoon et al., 2020; Hesham et al., 2021; Singh et al., 2021). Therefore, in this case, implementing sustainable practices to enrich anti-obesity phytochemicals is of major relevance to not contribute to the current agriculture-related problems. Among these technologies, the development of biofertilizers and nanofertilizers has gained attention in recent years due to their reduced environmental impact, low cost, sustainability, and their ability to increase the content of nutrients and bioactive compounds (Prasad et al., 2019; Guardiola-Márquez et al., 2022; Mahapatra et al., 2022).
The importance of these technologies for obesity lies in the fact that soil microorganisms and nanoparticles can significantly increase the phytochemical content and nutritional value of plants and their fruits, improving antioxidant activities, macro-, and micronutrient levels, bioactive compounds, and photosynthetic pigments (Figure 3) (Khatoon et al., 2020).
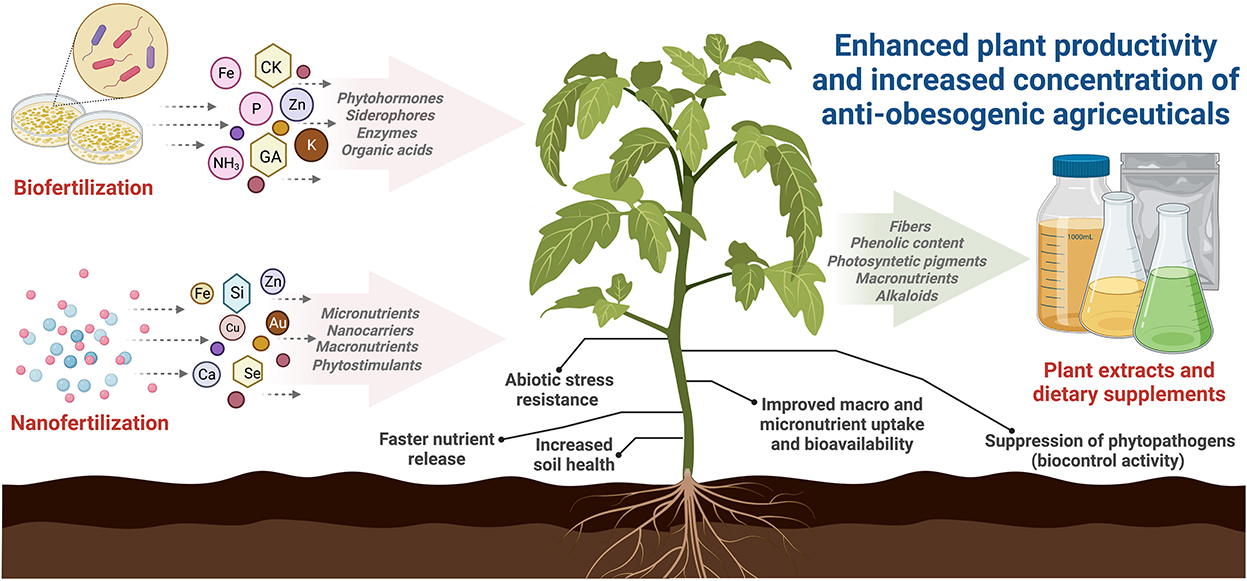
Figure 3. Proposed sustainable strategies to enhance plant productivity and increase the concentration of anti-obesogenic agriceuticals.
Biofertilizers based on plant growth-promoting soil microorganisms
Biofertilizers are formulations of beneficial microorganisms that promote plant health and growth by stimulating plant metabolism or increasing nutrient uptake from soil reserves, abiotic stress resistance, and suppression of plant pathogens (Kour et al., 2020; Hesham et al., 2021; Guardiola-Márquez et al., 2022). Soil microorganisms interact with the root system of plants by trading nutrients and signaling metabolites. Plant root secretions represent a source of carbon and nutrients for the metabolism of microbial populations, as well as serving as signals either to trigger specific metabolic responses or to repel plant pathogens and as modulators of rhizosphere communities to attract beneficial microbes (Ojuederie et al., 2019; Khatoon et al., 2020). Plant root exudates include sugars, amino acids, proteins, flavonoids, organic acids, fatty acids, etc., which offer an exclusive environment and are essential growth substrates for soil microbe establishment (Khatoon et al., 2020). In return, plant growth-promoting microorganisms (PGPM) colonize the plant's rhizosphere and root system and promote growth through different mechanisms, including atmospheric nitrogen fixation, mineral solubilization (phosphorus, potassium, and zinc), siderophores production, heavy metal sequestering, mineralization of soil organic matter, suppression of phytopathogens and production of phytohormones (auxins, cytokinins, and gibberellins) and enzymes (phosphatases, and catalases) (Prasad et al., 2019; Khatoon et al., 2020; Sammauria et al., 2020; Hesham et al., 2021; Guardiola-Márquez et al., 2022; Mahapatra et al., 2022).
Biofertilization has been applied to many plant species with anti-obesogenic activity (Table 1). The inoculation of plant growth-promoting bacteria (PGPB) (Pseudomonas poae and Bacillus amyloliquefaciens subsp. plantarum) on collard (Brassica oleracea var. acephala) cv. Georgia significantly improved its nutritional value, growth, and yield. Particularly, B. amyloliquefaciens markedly elevated the total phenolics (32.37%), flavonoids (62.31%), ascorbic acid (21.92%), rosmarinic acid (35.97%), carotenoids (21.92%), chlorophyll (31.93%) and magnesium (23.77%), iron (79.71%), copper (211.90%), zinc (44.26%) contents (Helaly et al., 2020). B. oleracea species have been reported to possess lipase-inhibitory properties and strong anti-adipogenic and anti-lipogenic effects (Le et al., 2020; Nallamuthu et al., 2022). Nallamuthu et al. (2022) evidenced that B. oleracea extracts significantly suppressed the intracellular accumulation of triglycerides, down-regulated the key adipogenic transcription factors (PPARγ, C/EPB-α, p-Akt, PI3k, and FAS enzyme), and modulated the levels of leptin and adiponectins in 3T3-L1 pre-adipocytes. Similarly, lettuce (Consentino et al., 2022), maize (Vishnupradeep et al., 2022), tomato (Katsenios et al., 2021), onion (Pellegrini et al., 2021), strawberry (Rahman et al., 2018), and many other plants have been treated with different formulations of biofertilizers resulting in significant beneficial effects on their agriceutical composition associated with anti-obesogenic activity (Table 1).
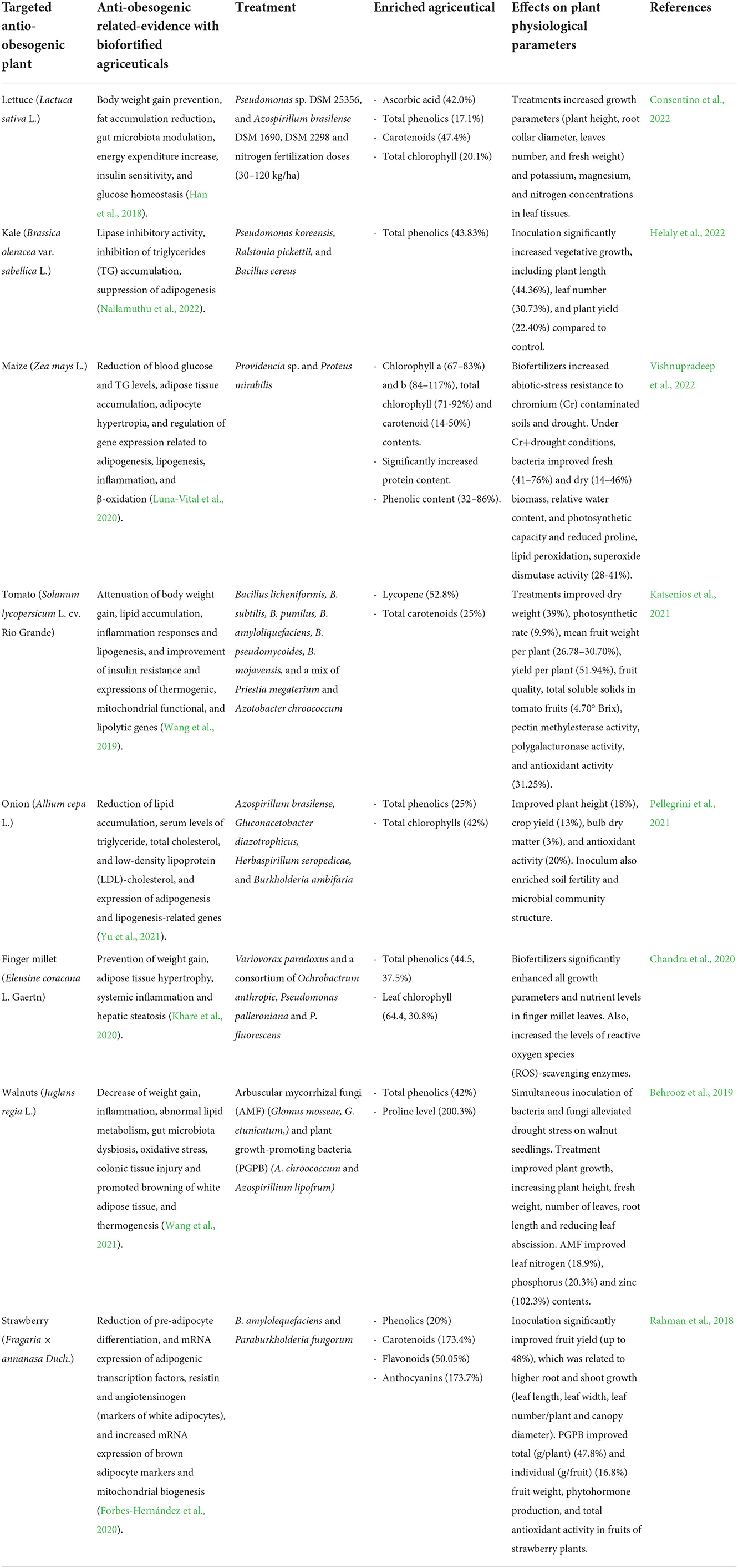
Table 1. Studies of biofortification with anti-obesogenic agriceuticals using beneficial soil microorganisms during plant cultivation.
Soil and foliar-applied nanoparticles used as nanofertilizers
Nanofertilization involves the application of nanoparticles (NPs) of < 100 nm in size (Yusefi-Tanha et al., 2020; Kumar et al., 2021). NPs have unique physicochemical properties that provide many advantages compared to their bulk macrostructure analogs, including a high surface area to volume ratio, enhanced reactivity, unique functionalization properties, electrical conductivity, and mechanical strength, improving plant nutrient transport and use (De La Torre-Roche et al., 2020; Yusefi-Tanha et al., 2020; El-Ghamry et al., 2021; Kumar et al., 2021). The small size of nanomaterials allows their penetration through biological barriers, easily diffusing into the plant vascular system after root or foliar application (De La Torre-Roche et al., 2020). Various publications have reported that nanofertilizers have shown enhanced absorption rates, faster nutrient release, increased nutrient availability, and nutrient use efficiency, resulting in improved plant nutrition and increased plant resistance against biotic and abiotic stress; nanofertilizers present greater efficacy compared with conventional chemical fertilizers (De La Torre-Roche et al., 2020; Kumar et al., 2021; Umar et al., 2021).
In the same way as biofertilizers, nanofertilizers can enhance the agriceutical content in plants (Table 2), Azeez et al. (2017) investigated the influence of biologically synthesized silver nanoparticles (AgNPs) on the phenolic and flavonoid content and antioxidant activity of Amaranthus caudatus L., significant improvements of 21.9 and 68.19% in phenolic contents were obtained using 25 and 50 ppm AgNPs, respectively, while flavonoids were significantly enhanced by 32.58 and 35.80% with the same treatments. The 2,2-diphenyl-1-picrylhydrazyl (DPPH) antioxidant activity was improved by 43.3 and 38.7% with 25 and 50 ppm of AgNPs. A. caudatus grown with 50 ppm of AgNPs led to the highest flavonoid composition, being kaempferol and quercetin the most abundant flavonoids in A. caudatus. Various authors have associated the phenolic and flavonoid content of Amaranthus species with anti-obesogenic activity (Kanikowska et al., 2019; Yuniarto et al., 2020; Prince et al., 2021). Prince et al. (2021) observed significant reductions in weight gain, organ weight, abdominal fat deposition, liver fat infiltration and steatosis, and improvements in glucose tolerance, hepatic antioxidant levels, and lipid parameters in high-carbohydrate-high-fat diet-fed obese rats administered with Amaranthus powder. Table 2 includes other studies where nanofertilization improves the agriceutical composition of anti-obesogenic plants.
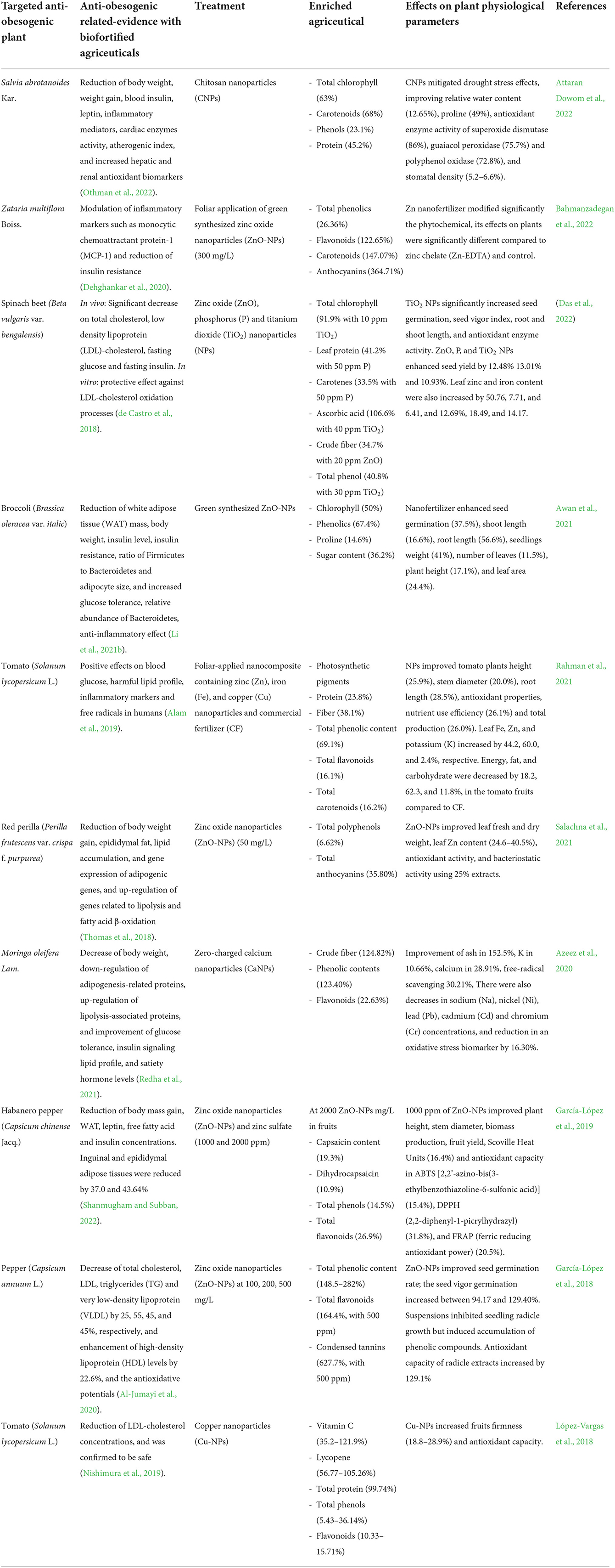
Table 2. Studies of biofortification with anti-obesogenic agriceuticals using nanoparticles during plant cultivation.
Biofertilization and nanofertilization techniques have been applied to many plant species with anti-obesity activity; however, there are no reports where these practices are used mainly to enhance the anti-obesogenic effect; further research is required to study the impact of these agricultural practices on the effects against obesity of edible and medicinal plants.
In addition, the plant species shown in Tables 1, 2 are rich in compounds widely used by the food, pharmaceutical, or cosmetic industries due to their proven bioactivity. Particularly they have demonstrated therapeutic effects against obesity. However, producing, extracting, and obtaining therapeutic levels of such compounds is costly, mainly because the yield per plant is low, which demands different techniques to increase their production (Araujo et al., 2020). The application of plant growth-promoting bacteria and nanoparticles have been studied for this purpose. Most of the agriceuticals responsible for the reduction of lipid accumulation, appetite, absorption of carbohydrates and lipids, adipogenesis, lipogenesis, lipid metabolism, obesity-associated inflammation, and the promotion of thermogenesis and modulation of gut microbiota, correspond to fibers, polyphenols (flavonoids, phenolic acids, lignans, tannins), alkaloids, lipids, protein, and photosynthetic pigments, which are the same phytochemical groups that can be enriched using the bio- and nanofertilizers, indicating that it could be possible to enhance anti-obesity mechanisms and reduce obesity rates if these techniques are applied strategically to plants with anti-obesogenic activity.
Conclusions
In this review, we presented the current situation of overweight and obesity, including relevant statistics regarding their prevalence and importance. Several reports have demonstrated the therapeutic potential of plant extracts to treat and prevent obesity, showing different mechanisms by which agriceuticals contribute to counteracting obesity. However, more plant species need to be evaluated to establish safe and effective protocols for producing and extracting the key compounds responsible for the therapeutic effects. Also, it is important to determine the effective doses and possible toxicity or long-term effects of these compounds in humans since most of the presented works have been performed in in vitro and in vivo studies. Pharmacokinetic studies involving human subjects are recommended before these extracts could be distributed or massively administered as anti-obesogenic treatments. Further research is needed to assess probable synergistic interactions between extracts of different plant species, which could lead to the formulation of polyherbal preparations to enhance the anti-obesogenic effect resultant from acting on several obesity targets simultaneously. Moreover, we established that biofertilization and nanofertilization are sustainable practices capable of increasing the concentrations of the plant bioactive molecules, but it is also necessary to explore which agriceuticals and at what level they are enhanced with these strategies. Proper investigation of anti-obesity plant species and implementing sustainable agronomic practices for their production could be a promising way to fight obesity.
Author contributions
CG-M and DJ-V wrote the manuscript. All authors approved the final manuscript.
Funding
This study was based upon research supported by Tecnologico de Monterrey—The Institute for Obesity Research – Challenge-Based Research Funding Program 2022 (I018 - IOR001 - C5-T1 – E). CG-M received a scholarship (CVU No. 875797) from Consejo Nacional de Ciencia y Tecnología (CONACYT) from Mexico.
Conflict of interest
The authors declare that the research was conducted in the absence of any commercial or financial relationships that could be construed as a potential conflict of interest.
Publisher's note
All claims expressed in this article are solely those of the authors and do not necessarily represent those of their affiliated organizations, or those of the publisher, the editors and the reviewers. Any product that may be evaluated in this article, or claim that may be made by its manufacturer, is not guaranteed or endorsed by the publisher.
References
Acharya, A., and Pal, P. K. (2020). Agriculture nanotechnology: translating research outcome to field applications by influencing environmental sustainability. NanoImpact 19, 100232. doi: 10.1016/j.impact.2020.100232
Ahmad, R., Ahmad, N., AlHudaithi, N., AlHebshi, A., and Bukhari, A. (2020). Extraction and UHPLC–DAD detection of undeclared substances in market-available dietary supplements and slimming products in Eastern region, Saudi Arabia: an application of principal component analysis. Biomed. Chromatogr. 34, e4698. doi: 10.1002/bmc.4698
Akhtar, N., Ilyas, N., Meraj, T. A., Pour-Aboughadareh, A., Sayyed, R. Z., Mashwani, Z. U. R., et al. (2022). Improvement of plant responses by nanobiofertilizer: a step towards sustainable agriculture. Nanomaterials 12, 965. doi: 10.3390/nano12060965
Alam, P., Raka, M. A., Khan, S., Sarker, J., Ahmed, N., Nath, P. D., et al. (2019). A clinical review of the effectiveness of tomato (Solanum lycopersicum) against cardiovascular dysfunction and related metabolic syndrome. J. Herb. Med. 16, 100235. doi: 10.1016/j.hermed.2018.09.006
Al-Jumayi, H. A., Elhendy, H. A., and Darwish, A. M. (2020). Biological effects of red chili pepper (Capsicum annuum) consumption on high fat diet female albino rats. Pak. J. Biol. Sci. 23, 150–158. doi: 10.3923/pjbs.2020.150.158
Alshammari, G. M., and Balakrishnan, A. (2019). Pumpkin (Cucurbita ficifolia Bouché) extract attenuate the adipogenesis in human mesenchymal stem cells by controlling adipogenic gene expression. Saudi J. Biol. Sci. 26, 744–751. doi: 10.1016/j.sjbs.2018.10.002
Andrade, J. M. O., Barcala-Jorge, A. S., Batista-Jorge, G. C., Paraíso, A. F., de Freitas, K. M., de Farias Lelis, D., et al. (2019). Effect of resveratrol on expression of genes involved thermogenesis in mice and humans. Biomed. Pharmacother 112, 108634. doi: 10.1016/j.biopha.2019.108634
Aranaz, P., Navarro-Herrera, D., Zabala, M., Miguéliz, I., Romo-Hualde, A., López-Yoldi, M., et al. (2019). Phenolic compounds inhibit 3T3-L1 adipogenesis depending on the stage of differentiation and their binding affinity to PPARγ. Molecules 24, 1045. doi: 10.3390/molecules24061045
Araujo, N. A. F., Brandao, R. M., Barguil, B. M., Cardoso, M. D. G., Pasqual, M., Rezende, R. A. S., et al. (2020). Plant growth-promoting bacteria improve growth and modify essential oil in rose (Rosa hybrida L.) cv. black prince. Front. Sustain. Food Syst. 4, 606827. doi: 10.3389/fsufs.2020.606827
Attaran Dowom, S., Karimian, Z., Mostafaei Dehnavi, M., and Samiei, L. (2022). Chitosan nanoparticles improve physiological and biochemical responses of Salvia abrotanoides (Kar.) under drought stress. BMC Plant Biol. 22, 364. doi: 10.1186/s12870-022-03689-4
Awan, S., Shahzadi, K., Javad, S., Tariq, A., Ahmad, A., Ilyas, S. A., et al. (2021). preliminary study of influence of zinc oxide nanoparticles on growth parameters of Brassica oleracea var italic. J. Saudi Soc. Agric. 20, 18–24. doi: 10.1016/j.jssas.2020.10.003
Azeez, L., Adejumo, A. L., Simiat, O. M., and Lateef, A. (2020). Influence of calcium nanoparticles (CaNPs) on nutritional qualities, radical scavenging attributes of Moringa oleifera and risk assessments on human health. J. Food Meas. Charact. 14, 2185–2195. doi: 10.1007/s11694-020-00465-6
Azeez, L., Lateef, A., and Adebisi, S. A. (2017). Silver nanoparticles (AgNPs) biosynthesized using pod extract of Cola nitida enhances antioxidant activity and phytochemical composition of Amaranthus caudatus Linn. Appl. Nanosci. 7, 59–66. doi: 10.1007/s13204-017-0546-2
Bahmanzadegan, A., Tavallali, H., Tavallali, V., and Karimi, M. A. (2022). Variations in biochemical characteristics of Zataria multiflora in response to foliar application of zinc nano complex formed on pomace extract of Punica granatum. Ind. Crops Prod. 187, 115369. doi: 10.1016/j.indcrop.2022.115369
Bajes, H. R., Almasri, I., and Bustanji, Y. (2020). Plant products and their inhibitory activity against pancreatic lipase. Rev. Bras. Farmacogn. 30, 321–330. doi: 10.1007/s43450-020-00055-z
Bautista, R. J. H., Mahmoud, A. M., Königsberg, M., and Guerrero, N. E. L. D. (2019). Obesity: pathophysiology, monosodium glutamate-induced model and anti-obesity medicinal plants. Biomed. Pharmacother. 111, 503–516. doi: 10.1016/j.biopha.2018.12.108
Behrooz, A., Vahdati, K., Rejali, F., Lotfi, M., Sarikhani, S., Leslie, C., et al. (2019). Arbuscular mycorrhiza and plant growth-promoting bacteria alleviate drought stress in walnut. HortScience 54, 1087–1092. doi: 10.21273/HORTSCI13961-19
Bolin, A. P., Sousa-Filho, C. P. B., Marinovic, M. P., Rodrigues, A. C., and Otton, R. (2020). Polyphenol-rich green tea extract induces thermogenesis in mice by a mechanism dependent on adiponectin signaling. J. Nutr. Biochem. 78, 108322. doi: 10.1016/j.jnutbio.2019.108322
Bursal, E., Aras, A., Kil,iç, Ö., Taslimi, P., Gören, A. C., and Gülçin, I. (2019). Phytochemical content, antioxidant activity, and enzyme inhibition effect of Salvia eriophora Boiss. & Kotschy against acetylcholinesterase, α-amylase, butyrylcholinesterase, and α-glycosidase enzymes. J. Food Biochem. 43, e12776. doi: 10.1111/jfbc.12776
Çakir, I., Pan, P. L., Hadley, C. K., El-Gamal, A., Fadel, A., Elsayegh, D., et al. (2022). Sulforaphane reduces obesity by reversing leptin resistance. Elife 11, e67368. doi: 10.7554/eLife.67368
Cao, S. Y., Zhao, C. N., Xu, X. Y., Tang, G. Y., Corke, H., Gan, R. Y., et al. (2019). Dietary plants, gut microbiota, and obesity: effects and mechanisms. Trends Food Sci. Technol. 92, 194–204. doi: 10.1016/j.tifs.2019.08.004
Chandra, D., Srivastava, R., Glick, B. R., and Sharma, A. K. (2020). Rhizobacteria producing ACC deaminase mitigate water-stress response in finger millet (Eleusine coracana (L.) Gaertn.). 3 Biotech 10, 1–15. doi: 10.1007/s13205-019-2046-4
Chayaratanasin, P., Caobi, A., Suparpprom, C., Saenset, S., Pasukamonset, P., Suanpairintr, N., et al. (2019). Clitoria ternatea flower petal extract inhibits adipogenesis and lipid accumulation in 3T3-L1 preadipocytes by downregulating adipogenic gene expression. Molecules 24, 1894. doi: 10.3390/molecules24101894
Consentino, B. B., Aprile, S., Rouphael, Y., Ntatsi, G., De Pasquale, C., Iapichino, G., et al. (2022). Application of PGPB combined with variable N doses affects growth, yield-related traits, N-fertilizer efficiency and nutritional status of lettuce grown under controlled condition. Agronomy 12, 236. doi: 10.3390/agronomy12020236
Das, B., Yonzone, R., Saha, S., Murmu, D. K., and Kundu, S. (2022). Comprehensive assessment of ZnO, P and TiO2 nanoparticles sustaining environment in response to seed germination, antioxidants activity, nutritional quality and yield of Spinach Beet (Beta vulgaris var. bengalensis). Res. Sq. doi: 10.21203/rs.3.rs-1315549/v1
Datta, P., Sharma, A., Pal, B., and Mohit, K. (2017). “The role of adipokines and adipogenesis in the pathogenesis of osteoarthritis,” in Cytokine Effector Functions in Tissues, eds M. Foti and M. Locati (Amsterdam: Academic Press), 99–107.
de Castro, A. P. R. B., de Moraes, A. E. A., Simabuco, F. M., Massa, G. O., Corona, L. P., Bezerra, R. M. N., et al. (2018). Beetroot leaves (Beta vulgaris L.) assist in cholesterol reduction in dyslipidemic obese adults. Free Radic. Biol. Med. 120, S123. doi: 10.1016/j.freeradbiomed.2018.04.406
De La Torre-Roche, R., Cantu, J., Tamez, C., Zuverza-Mena, N., Hamdi, H., Adisa, I. O., et al. (2020). Seed biofortification by engineered nanomaterials: a pathway to alleviate malnutrition? J. Agric. Food Chem. 68, 12189–12202. doi: 10.1021/acs.jafc.0c04881
Dehghankar, L., Gholami, M., and Ghazalian, F. (2020). Effects of 8 weeks combined training along with Zataria multiflora supplement ingestion on serum levels of MCP-1 and insulin resistance in overweight men. J. Pract. Stud. Biosci. Sport 8, 34–46. doi: 10.22077/JPSBS.2019.1956.1450
Diez-Echave, P., Vezza, T., Rodríguez-Nogales, A., Ruiz-Malagón, A. J., Hidalgo-García, L., Garrido-Mesa, J., et al. (2020). The prebiotic properties of Hibiscus sabdariffa extract contribute to the beneficial effects in diet-induced obesity in mice. Food Res. Int. 127, 108722. doi: 10.1016/j.foodres.2019.108722
Duangjai, A., Nuengchamnong, N., Suphrom, N., Trisat, K., Limpeanchob, N., Saokaew, S., et al. (2018). Potential of coffee fruit extract and quinic acid on adipogenesis and lipolysis in 3T3-L1 adipocytes. Kobe J. Med. Sci. 64, E84.
Elbashir, S. M. I., Devkot, H. P., Wada, M., Kishimoto, N., Moriuchi, M., Shuto, T., et al. (2018). Free radical scavenging, α-glucosidase inhibitory and lipase inhibitory activities of eighteen Sudanese medicinal plants. BMC Complement. Altern. Med. 18, 1–12. doi: 10.1186/s12906-018-2346-y
El-Ghamry, A., El-Khateeb, A., Mosa, A. A., and El-Ramady, H. (2021). Bio-nano fertilizers preparation using a fully-automated apparatus: a case study of nano-selenium. Env. Biodivers. Soil Secur. 5, 171–183. doi: 10.21608/jenvbs.2021.88095.1139
El-Shiekh, R. A., Al-Mahdy, D. A., Hifnawy, M. S., and Abdel-Sattar, E. A. (2019). In-vitro screening of selected traditional medicinal plants for their anti-obesity and anti-oxidant activities. S. Afr. J. Bot. 123, 43–50. doi: 10.1016/j.sajb.2019.01.022
Fang, C., Kim, H., Noratto, G., Sun, Y., Talcott, S. T., Mertens-Talcott, S. U., et al. (2018). Gallotannin derivatives from mango (Mangifera indica L.) suppress adipogenesis and increase thermogenesis in 3T3-L1 adipocytes in part through the AMPK pathway. J. Funct. Foods (2018) 46, 101–109. doi: 10.1016/j.jff.2018.04.043
Fernández-Raudales, D., Yor-Aguilar, M., Andino-Segura, J., Hernández, A., Egbert, R., López-Cintrón, J. R., et al. (2018). Effects of high plant protein and high soluble fiber beverages on satiety, appetite control and subsequent food intake in healthy men. Food Nutr. Sci. 9, 751. doi: 10.4236/fns.2018.96057
Forbes-Hernández, T. Y., Cianciosi, D., Ansary, J., Mezzetti, B., Bompadre, S., Quiles, J. L., et al. (2020). Strawberry (Fragaria× ananassa cv. Romina) methanolic extract promotes browning in 3T3-L1 cells. Food Funct. 11, 297–304. doi: 10.1039/C9FO02285F
Franco, R. R., Alves, V. H. M., Zabisky, L. F. R., Justino, A. B., Martins, M. M., Saraiva, A. L., et al. (2020). Antidiabetic potential of Bauhinia forficata Link leaves: a non-cytotoxic source of lipase and glycoside hydrolases inhibitors and molecules with antioxidant and antiglycation properties. Biomed. Pharmacother. 123, 109798. doi: 10.1016/j.biopha.2019.109798
García-López, J. I., Niño-Medina, G., Olivares-Sáenz, E., Lira-Saldivar, R. H., Barriga-Castro, E. D., Vázquez-Alvarado, R., et al. (2019). Foliar application of zinc oxide nanoparticles and zinc sulfate boosts the content of bioactive compounds in habanero peppers. Plants 8, 254. doi: 10.3390/plants8080254
García-López, J. I., Zavala-García, F., Olivares-Sáenz, E., Lira-Saldívar, R. H., Díaz Barriga-Castro, E., Ruiz-Torres, N. A., et al. (2018). Zinc oxide nanoparticles boosts phenolic compounds and antioxidant activity of Capsicum annuum L. during germination. Agronomy 8, 215. doi: 10.3390/agronomy8100215
Gastélum-Estrada, A., Serna-Saldívar, S. O., and Jacobo-Velázquez, D. A. (2021). Fighting the COVID-19 pandemic through biofortification: innovative approaches to improve the immunomodulating capacity of foods. ACS Food Sci. Technol. 1, 480–486. doi: 10.1021/acsfoodscitech.1c00019
Grabner, G. F., Xie, H., Schweiger, M., and Zechner, R. (2021). Lipolysis: cellular mechanisms for lipid mobilization from fat stores. Natute 3, 1445–1465. doi: 10.1038/s42255-021-00493-6
Guardiola-Márquez, C. E., Pacheco, A., Mora-Godínez, S., Schüßler, A., Gradilla-Hernández, M. S., Senés-Guerrero, C., et al. (2022). Septoglomus species dominate the arbuscular mycorrhiza of five crop plants in an arid region of northern Mexico. Symbiosis 87, 93–106. doi: 10.1007/s13199-022-00851-2
Guo, J., Han, X., Tan, H., Huang, W., You, Y., Zhan, J., et al. (2019). Blueberry extract improves obesity through regulation of the gut microbiota and bile acids via pathways involving FXR and TGR5. IScience 19, 676–690. doi: 10.1016/j.isci.2019.08.020
Han, Y., Zhao, C., He, X., Sheng, Y., Ma, T., Sun, Z., et al. (2018). Purple lettuce (Lactuca sativa L.) attenuates metabolic disorders in diet induced obesity. J. Funct. Foods 45, 462–470. doi: 10.1016/j.jff.2018.04.027
He, D., Zhang, P., Sai, X., Li, X., Wang, L., Xu, Y., et al. (2018). Camellia euphlebia flower extract inhibits oleic acid-induced lipid accumulation via reduction of lipogenesis in HepG2 cells. Eur. J. Integr. Med. 17, 1–8. doi: 10.1016/j.eujim.2017.11.002
Helaly, A. A., Hassan, S. M., Craker, L. E., and Mady, E. (2020). Effects of growth-promoting bacteria on growth, yield and nutritional value of collard plants. Ann. Agric. Sci. 65, 77–82. doi: 10.1016/j.aoas.2020.01.001
Helaly, A. A., Mady, E., Salem, E. A., and Randhir, T. O. (2022). Stimulatory effects of growth-promoting bacteria on growth, nutritional composition, and yield of kale plants. J. Plant Nutr. 45, 2465–2477. doi: 10.1080/01904167.2022.2046084
Héliès-Toussaint, C., Fouché, E., Naud, N., del Socorro Santos-Diaz, M., Nègre-Salvayre, A., Barba de la Rosa, A. P., et al. (2020). Opuntia cladode powders inhibit adipogenesis in 3 T3-F442A adipocytes and a high-fat-diet rat model by modifying metabolic parameters and favouring faecal fat excretion. BMC Complement. Med. Ther. 20, 1–12. doi: 10.1186/s12906-020-2824-x
Hengpratom, T., Lowe, G. M., Thumanu, K., Suknasang, S., Tiamyom, K., Eumkeb, G., et al. (2018). Oroxylum indicum (L.) Kurz extract inhibits adipogenesis and lipase activity in vitro. BMC Complement. Altern. Med. 18, 1–14. doi: 10.1186/s12906-018-2244-3
Hesham, A. E. L., Kaur, T., Devi, R., Kour, D., Prasad, S., Yadav, N., et al. (2021). “Current trends in microbial biotechnology for agricultural sustainability: conclusion and future challenges,” in Current Trends in Microbial Biotechnology for Sustainable Agriculture (Singapore: Springer), 555–572.
Hua, M., Fan, M., Li, Z., Sha, J., Li, S., Sun, Y., et al. (2021). Ginseng soluble dietary fiber can regulate the intestinal flora structure, promote colon health, affect appetite and glucolipid metabolism in rats. J. Funct. Foods 83, 104534. doi: 10.1016/j.jff.2021.104534
Hussain, M. F., Roesler, A., and Kazak, L. (2020). Regulation of adipocyte thermogenesis: mechanisms controlling obesity. FEBS J. 287, 3370–3385. doi: 10.1111/febs.15331
Hussain, S., Rehman, A. U., Luckett, D. J., Blanchard, C. L., Obied, H. K., Strappe, P., et al. (2019). Phenolic compounds with antioxidant properties from canola meal extracts inhibit adipogenesis. Int. J. Mol. Sci. 21, 1. doi: 10.3390/ijms21010001
Im, H., Lee, J., Kim, K., Son, Y., and Lee, Y. H. (2022). Anti-obesity effects of heat-transformed green tea extract through the activation of adipose tissue thermogenesis. Nutr. Metab. 19, 1–14. doi: 10.1186/s12986-022-00648-6
Islam, M. T., Ali, E. S., and Mubarak, M. S. (2020). Anti-obesity effect of plant diterpenes and their derivatives: a review. Phytother. Res. 34, 1216–1225. doi: 10.1002/ptr.6602
Jacobo-Velázquez, D. A. (2022). Definition of biofortification revisited. ACS Food Sci. Technol. 2, 782–783. doi: 10.1021/acsfoodscitech.2c00110
Jaradat, N. A., Al-lahham, S., Zaid, A. N., Hussein, F., Issa, L., Abualhasan, M. N., et al. (2019). Carlina curetum plant phytoconstituents, enzymes inhibitory and cytotoxic activity on cervical epithelial carcinoma and colon cancer cell lines. Eur. J. Integr. Med. 30, 100933. doi: 10.1016/j.eujim.2019.100933
Jemai, R., Drira, R., Makni, M., Fetoui, H., and Sakamoto, K. (2020). Colocynth (Citrullus colocynthis) seed extracts attenuate adipogenesis by down-regulating PPARγ/SREBP-1c and C/EBPα in 3T3-L1 cells. Food Biosci. 33, 100491. doi: 10.1016/j.fbio.2019.100491
Jiao, X., Wang, Y., Lin, Y., Lang, Y., Li, E., Zhang, X., et al. (2019). Blueberry polyphenols extract as a potential prebiotic with anti-obesity effects on C57BL/6 J mice by modulating the gut microbiota. J. Nutr. Biochem. 64, 88–100. doi: 10.1016/j.jnutbio.2018.07.008
Kamoun, J., Rahier, R., Sellami, M., Koubaa, I., Mansuelle, P., Lebrun, R., et al. (2019). Identification of a new natural gastric lipase inhibitor from star anise. Food Funct. 10, 469–478. doi: 10.1039/C8FO02009D
Kanikowska, D., Kanikowska, A., Rutkowski, R., Włochal, M., Orzechowska, Z., Juchacz, A., et al. (2019). Amaranth (Amaranthus cruentus L.) and canola (Brassica napus L.) oil impact on the oxidative metabolism of neutrophils in the obese patients. Pharm. Biol. 57, 140–144. doi: 10.1080/13880209.2019.1569696
Karri, S., Sharma, S., Hatware, K., and Patil, K. (2019). Natural anti-obesity agents and their therapeutic role in management of obesity: a future trend perspective. Biomed. Pharmacother. 110, 224–238. doi: 10.1016/j.biopha.2018.11.076
Katsenios, N., Andreou, V., Sparangis, P., Djordjevic, N., Giannoglou, M., Chanioti, S., et al. (2021). Evaluation of plant growth promoting bacteria strains on growth, yield and quality of industrial tomato. Microorganisms 9, 2099. doi: 10.3390/microorganisms9102099
Kawai, M., and Rosen, C. J. (2012). “Bone marrow fat and bone mass,” in Translational Endocrinology of Bone: Reproduction, Metabolism, and the Central Nervous System, eds A. K. Singh, A. Kumar, and P. K. Singh (Cambridge: Woodhead Publishing), 129–157. doi: 10.1016/B978-0-12-815879-1.00007-0
Kersten, S. (2001). Mechanisms of nutritional and hormonal regulation of lipogenesis. EMBO Rep. 2, 282–286. doi: 10.1093/embo-reports/kve071
Khadayat, K., Marasini, B. P., Gautam, H., Ghaju, S., and Parajuli, N. (2020). Evaluation of the alpha-amylase inhibitory activity of Nepalese medicinal plants used in the treatment of diabetes mellitus. Clin. Phytoscience 6, 1–8. doi: 10.1186/s40816-020-00179-8
Khare, P., Maurya, R., Bhatia, R., Mangal, P., Singh, J., Podili, K., et al. (2020). Polyphenol rich extracts of finger millet and kodo millet ameliorate high fat diet-induced metabolic alterations. Food Funct. 11, 9833–9847. doi: 10.1039/D0FO01643H
Khatoon, Z., Huang, S., Rafique, M., Fakhar, A., and Kamran, M. A. (2020). Unlocking the potential of plant growth-promoting rhizobacteria on soil health and the sustainability of agricultural systems. J. Environ. Manage. 273, 111118. doi: 10.1016/j.jenvman.2020.111118
Kim, H. J., Lee, M. J., Jang, J. Y., and Lee, S. H. (2019). Allium hookeri root extract inhibits adipogenesis by promoting lipolysis in high fat diet-induced obese mice. Nutrients 11, 2262. doi: 10.3390/nu11102262
Kim, K. J., Jeong, E. S., Lee, K. H., Na, J. R., Park, S., Kim, J. S., et al. (2020). Unripe Rubus coreanus miquel extract containing ellagic acid promotes lipolysis and thermogenesis in vitro and in vivo. Molecules 25, 5954. doi: 10.3390/molecules25245954
Kim, N. H., Jegal, J., Kim, Y. N., Heo, J. D., Rho, J. R., Yang, M. H., et al. (2018). Chokeberry extract and its active polyphenols suppress adipogenesis in 3T3-L1 adipocytes and modulates fat accumulation and insulin resistance in diet-induced obese mice. Nutrients 10, 1734. doi: 10.3390/nu10111734
Kim, S., Lee, M. S., Jung, S., Son, H. Y., Park, S., Kang, B., et al. (2018). Ginger extract ameliorates obesity and inflammation via regulating microRNA-21/132 expression and AMPK activation in white adipose tissue. Nutrients 10, 1567. doi: 10.3390/nu10111567
Kim, Y. J., Ryu, R., Choi, J. Y., and Choi, M. S. (2019). Platycodon grandiflorus root ethanol extract induces lipid excretion, lipolysis, and thermogenesis in diet-induced obese mice. J. Med. Food 22, 1100–1109. doi: 10.1089/jmf.2019.4443
Kour, D., Rana, K. L., Yadav, A. N., Yadav, N., Kumar, M., Kumar, V., et al. (2020). Microbial biofertilizers: Bioresources and eco-friendly technologies for agricultural and environmental sustainability. Biocatal. Agric. Biotechnol. 23, 101487. doi: 10.1016/j.bcab.2019.101487
Kumar, Y., Singh, K. T. T., and Raliya, R. (2021). Nanofertilizers and their role in sustainable agriculture. Ann. Plant Soil Res. 23, 238–255. doi: 10.47815/apsr.2021.10067
Kwon, E. Y., Kim, S. Y., and Choi, M. S. (2018). Luteolin-enriched artichoke leaf extract alleviates the metabolic syndrome in mice with high-fat diet-induced obesity. Nutrients 10, 979. doi: 10.3390/nu10080979
Le, T. N., Chiu, C. H., and Hsieh, P. C. (2020). Bioactive compounds and bioactivities of Brassica oleracea L. var. italica sprouts and microgreens: an updated overview from a nutraceutical perspective. Plants 9, 946. doi: 10.3390/plants9080946
Lee, H. J., Le, B., Lee, D. R., Choi, B. K., and Yang, S. H. (2018). Cissus quadrangularis extract (CQR-300) inhibits lipid accumulation by downregulating adipogenesis and lipogenesis in 3T3-L1 cells. Toxicol. Rep. 5, 608–614. doi: 10.1016/j.toxrep.2018.02.008
Li, X., Lu, H. Y., Jiang, X. W., Yang, Y., Xing, B., Yao, D., et al. (2021a). Cinnamomum cassia extract promotes thermogenesis during exposure to cold via activation of brown adipose tissue. J. Ethnopharmacol. 266, 113413. doi: 10.1016/j.jep.2020.113413
Li, X., Tian, S., Wang, Y., Liu, J., Wang, J., Lu, Y., et al. (2021b). Broccoli microgreens juice reduces body weight by enhancing insulin sensitivity and modulating gut microbiota in high-fat diet-induced C57BL/6J obese mice. Eur. J. Nutr. 60, 3829–3839. doi: 10.1007/s00394-021-02553-9
Li, Y., Cui, Y., Lu, F., Wang, X., Liao, X., Hu, X., et al. (2019). Beneficial effects of a chlorophyll-rich spinach extract supplementation on prevention of obesity and modulation of gut microbiota in high-fat diet-fed mice. J. Funct. Foods 60, 103436. doi: 10.1016/j.jff.2019.103436
Liu, J., Hao, W., He, Z., Kwek, E., Zhao, Y., Zhu, H., et al. (2019). Beneficial effects of tea water extracts on the body weight and gut microbiota in C57BL/6J mice fed with a high-fat diet. Food Funct. 10, 2847–2860. doi: 10.1039/C8FO02051E
López-Vargas, E. R., Ortega-Ortíz, H., Cadenas-Pliego, G., de Alba Romenus, K., Cabrera de la Fuente, M., Benavides-Mendoza, A., et al. (2018). Foliar application of copper nanoparticles increases the fruit quality and the content of bioactive compounds in tomatoes. Appl. Sci. 8, 1020. doi: 10.3390/app8071020
Lu, X., Liu, J., Zhang, N., Fu, Y., Zhang, Z., Li, Y., et al. (2019). Ripened Pu-erh tea extract protects mice from obesity by modulating gut microbiota composition. J. Agric. Food Chem. 67, 6978–6994. doi: 10.1021/acs.jafc.8b04909
Luna-Vital, D., Luzardo, I., Cuellar, L., Loarca, G., and Mejia, E. G. D. (2020). Ferulic acid and anthocyanin from colored maize correlated with prevention of high-fat induced obesity in mice by modulating lipid, glucose and inflammatory pathways. Curr. Dev. Nutr. 4, 433–433. doi: 10.1093/cdn/nzaa045_066
Ma, Q., Wang, W., Ma, Z., Liu, Y., Mu, J., Wang, J., et al. (2021). Enzymatic-modified dietary fibre fraction extracted from potato residue regulates the gut microbiotas and production of short-chain fatty acids of C57BL/6 mice. J. Funct. Foods 84, 104606. doi: 10.1016/j.jff.2021.104606
Mahapatra, D. M., Satapathy, K. C., and Panda, B. (2022). Biofertilizers and nanofertilizers for sustainable agriculture: phycoprospects and challenges. Sci. Total Environ. 803, 149990. doi: 10.1016/j.scitotenv.2021.149990
Marrelli, M., Morrone, F., Argentieri, M. P., Gambacorta, L., Conforti, F., Avato, P., et al. (2018). Phytochemical and biological profile of Moricandia arvensis (L.) DC.: an inhibitor of pancreatic lipase. Molecules 23, 2829. doi: 10.3390/molecules23112829
Marrelli, M., Russo, N., Chiocchio, I., Statti, G., Poli, F., Conforti, F., et al. (2020). Potential use in the treatment of inflammatory disorders and obesity of selected wild edible plants from Calabria region (Southern Italy). S. Afr. J. Bot. 128, 304–311. doi: 10.1016/j.sajb.2019.11.029
Mhatre, S. V., Bhagit, A. A., and Yadav, R. P. (2019). Proteinaceous pancreatic lipase inhibitor from the seed of Litchi chinensis. Food Technol. Biotechnol. 57, 113. doi: 10.17113/ftb.57.01.19.5909
Milton-Laskíbar, I., Gómez-Zorita, S., Arias, N., Romo-Miguel, N., González, M., Fernández-Quintela, A., et al. (2020). Effects of resveratrol and its derivative pterostilbene on brown adipose tissue thermogenic activation and on white adipose tissue browning process. J. Physiol. Biochem. 76, 269–278. doi: 10.1007/s13105-020-00735-3
Montanari, T., Boschi, F., and Colitti, M. (2019). Comparison of the effects of browning-inducing capsaicin on two murine adipocyte models. Front. Physiol. 10, 1380. doi: 10.3389/fphys.2019.01380
Nallamuthu, I., Jain, A., and Anand, T. (2022). Comparative evaluation of Brassica oleracea, Ocimum basilicum, and Moringa oleifera leaf extracts on lipase inhibition and adipogenesis in 3T3-L1 adipocytes. J. Food Biochem. 46, e14158. doi: 10.1111/jfbc.14158
Nam, H., Jung, H., Kim, Y., Kim, B., Kim, K. H., Park, S. J., et al. (2018). Aged black garlic extract regulates lipid metabolism by inhibiting lipogenesis and promoting lipolysis in mature 3T3-L1 adipocytes. Food Sci. Biotechnol. 27, 575–579. doi: 10.1007/s10068-017-0268-y
Nemes, A., Homoki, J. R., Kiss, R., Hegedus, C., Kovács, D., Peitl, B., et al. (2019). Effect of anthocyanin-rich tart cherry extract on inflammatory mediators and adipokines involved in type 2 diabetes in a high fat diet induced obesity mouse model. Nutrients 11, 1966. doi: 10.3390/nu11091966
Nepali, S., Cha, J. Y., Ki, H. H., Lee, H. Y., Kim, Y. H., Kim, D. K., et al. (2018). Chrysanthemum indicum inhibits adipogenesis and activates the AMPK pathway in high-fat-diet-induced obese mice. Am. J. Chin. Med. 46, 119–136. doi: 10.1142/S0192415X18500076
Nishimura, M., Tominaga, N., Ishikawa-Takano, Y., Maeda-Yamamoto, M., and Nishihira, J. (2019). Effect of 12-week daily intake of the High-Lycopene Tomato (Solanum Lycopersicum), A variety named “PR-7”, on lipid metabolism: a randomized, double-blind, Placebo-Controlled, parallel-group study. Nutrients 11, 1177. doi: 10.3390/nu11051177
Noratto, G. D., Murphy, K., and Chew, B. P. (2019). Quinoa intake reduces plasma and liver cholesterol, lessens obesity-associated inflammation, and helps to prevent hepatic steatosis in obese db/db mouse. Food Chem. 287, 107–114. doi: 10.1016/j.foodchem.2019.02.061
OECD (2021). Overweight and Obesity Among Adults. Available online at: https://www.oecd-ilibrary.org/sites/0f705cf8-en/index.html?itemId=/content/component/0f705cf8-en (accessed July 25, 2022).
Ojuederie, O. B., Olanrewaju, O. S., and Babalola, O. O. (2019). Plant growth promoting rhizobacterial mitigation of drought stress in crop plants: implications for sustainable agriculture. Agronomy 9, 712. doi: 10.3390/agronomy9110712
Othman, M. S., Khaled, A. M., Aleid, G. M., Fareid, M. A., Hameed, R. A., Abdelfattah, M. S., et al. (2022). Evaluation of antiobesity and hepatorenal protective activities of Salvia officinalis extracts pre-treatment in high-fat diet-induced obese rats. Environ. Sci. Pollut. 29, 75043–75056. doi: 10.1007/s11356-022-21092-2
Pan, M. H., Koh, Y. C., Lee, T. L., Wang, B., Chen, W. K., Nagabhushanam, K., et al. (2019). Resveratrol and oxyresveratrol activate thermogenesis via different transcriptional coactivators in high-fat diet-induced obese mice. J. Agric. Food Chem. 67, 13605–13616. doi: 10.1021/acs.jafc.9b05963
Park, J., Kim, H. L., Jung, Y., Ahn, K. S., Kwak, H. J., Um, J. Y., et al. (2019). Bitter orange (Citrus aurantium Linné) improves obesity by regulating adipogenesis and thermogenesis through AMPK activation. Nutrients 11, 1988. doi: 10.3390/nu11091988
Pellegrini, M., Spera, D. M., Ercole, C., and Del Gallo, M. (2021). Allium cepa L. inoculation with a consortium of plant growth-promoting bacteria: Effects on plants, soil, and the autochthonous microbial community. Microorganisms 9, 639. doi: 10.3390/microorganisms9030639
Podsedek, A., Zakłos-Szyda, M., Polka, D., and Sosnowska, D. (2020). Effects of Viburnum opulus fruit extracts on adipogenesis of 3T3-L1 cells and lipase activity. J. Funct. Foods 73, 104111. doi: 10.1016/j.jff.2020.104111
Prasad, M., Srinivasan, R., Chaudhary, M., Choudhary, M., and Jat, L. K. (2019). “Plant growth promoting rhizobacteria (PGPR) for sustainable agriculture: perspectives and challenges,” in PGPR Amelioration in Sustainable Agriculture, ed G. Karsenty (New York, NY: Academic Press), 167–179.
Prince, M. R. U., Zihad, S. N. K., Ghosh, P., Sifat, N., Rouf, R., Al Shajib, G. M., et al. (2021). Amaranthus spinosus attenuated obesity-induced metabolic disorders in high-carbohydrate-high-fat diet-fed obese rats. Front. Nutr. 8, 653918. doi: 10.3389/fnut.2021.653918
Rahman, M., Sabir, A. A., Mukta, J. A., Khan, M., Alam, M., Mohi-Ud-Din, M., et al. (2018). Plant probiotic bacteria Bacillus and Paraburkholderia improve growth, yield and content of antioxidants in strawberry fruit. Sci. Rep. 8, 1–11. doi: 10.1038/s41598-018-20235-1
Rahman, M. H., Hasan, M. N., Nigar, S., Ma, F., Aly Saad Aly, M., Khan, M. Z. H., et al. (2021). Synthesis and characterization of a mixed nanofertilizer influencing the nutrient use efficiency, productivity, and nutritive value of tomato fruits. ACS Omega 6, 27112–27120. doi: 10.1021/acsomega.1c03727
Rajan, L., Palaniswamy, D., and Mohankumar, S. K. (2020). Targeting obesity with plant-derived pancreatic lipase inhibitors: a comprehensive review. Pharm. Res. 155, 104681. doi: 10.1016/j.phrs.2020.104681
Redha, A. A., Perna, S., Riva, A., Petrangolini, G., Peroni, G., Nichetti, M., et al. (2021). Novel insights on anti-obesity potential of the miracle tree, Moringa oleifera: a systematic review. J. Funct. Foods 84, 104600. doi: 10.1016/j.jff.2021.104600
Ritchie, H., and Roser, M. (2019). Obesity. Available online at: https://ourworldindata.org/obesity (accessed July 25, 2022).
Salachna, P., Mizielińska, M., Płoszaj-Witkowska, B., and Jaszczak, A. (2021). Zinc oxide nanoparticles enhanced biomass and zinc content and induced changes in biological properties of red Perilla frutescens. Materials 14, 6182. doi: 10.3390/ma14206182
Sammauria, R., Kumawat, S., Kumawat, P., Singh, J., and Jatwa, T. K. (2020). Microbial inoculants: potential tool for sustainability of agricultural production systems. Arch. Microbiol. 202, 677–693. doi: 10.1007/s00203-019-01795-w
Shang, A., Gan, R. Y., Xu, X. Y., Mao, Q. Q., Zhang, P. Z., and Li, H. B. (2021). Effects and mechanisms of edible and medicinal plants on obesity: an updated review. Crit. Rev. Food Sci. Nutr. 61, 2061–2077. doi: 10.1080/10408398.2020.1769548
Shanmugham, V., and Subban, R. (2022). Comparison of the anti-obesity effect of enriched capsanthin and capsaicin from Capsicum annuum L. fruit in obesity-induced C57BL/6J mouse model. Food Technol. Biotechnol. 60, 202–212. doi: 10.17113/ftb.60.02.22.7376
Sharafi, M., Alamdari, N., Wilson, M., Leidy, H. J., and Glynn, E. L. (2018). Effect of a high-protein, high-fiber beverage preload on subjective appetite ratings and subsequent ad libitum energy intake in overweight men and women: a randomized, double-blind placebo-controlled, crossover study. Curr. Dev. Nutr. 2, nzy022. doi: 10.1093/cdn/nzy022
Sharma, K., Kang, S., Gong, D., Oh, S. H., Park, E. Y., Oak, M. H., et al. (2018). of Garcinia cambogia extract and pear pomace extract additively suppresses adipogenesis and enhances lipolysis in 3T3-L1 cells. Pharmacogn. Mag. 14, 220. doi: 10.4103/pm.pm_388_17
Shawky, N. M., and Segar, L. (2018). Sulforaphane improves leptin responsiveness in high-fat high-sucrose diet-fed obese mice. Eur. J. Pharmacol. 835, 108–114. doi: 10.1016/j.ejphar.2018.07.050
Shori, A. B. (2020). Inclusion of phenolic compounds from different medicinal plants to increase α-amylase inhibition activity and antioxidants in yogurt. J. Taibah Univ. Sci. 14, 1000–1008. doi: 10.1080/16583655.2020.1798072
Si, X., Bi, J., Chen, Q., Cui, H., Bao, Y., Tian, J., et al. (2021). Effect of blueberry anthocyanin-rich extracts on peripheral and hippocampal antioxidant defensiveness: the analysis of the serum fatty acid species and gut microbiota profile. J. Agric. Food Chem. 69, 3658–3666. doi: 10.1021/acs.jafc.0c07637
Singh, R. P., Handa, R., and Manchanda, G. (2021). Nanoparticles in sustainable agriculture: an emerging opportunity. J. Control Release 329, 1234–1248. doi: 10.1016/j.jconrel.2020.10.051
Sorrenti, V., Randazzo, C. L., Caggia, C., Ballistreri, G., Romeo, F. V., Fabroni, S., et al. (2019). Beneficial effects of pomegranate peel extract and probiotics on pre-adipocyte differentiation. Front. Microbiol 10, 660. doi: 10.3389/fmicb.2019.00660
Sun, W., Shahrajabian, M. H., and Cheng, Q. (2021). Natural dietary and medicinal plants with anti-obesity therapeutics activities for treatment and prevention of obesity during lock down and in post-Covid-19 era. Appl. Sci. 11, 7889. doi: 10.3390/app11177889
Taviano, M. F., Miceli, N., Acquaviva, R., Malfa, G. A., Ragusa, S., Giordano, D., et al. (2020). Cytotoxic, antioxidant, and enzyme inhibitory properties of the traditional medicinal plant Matthiola incana (L.) R. Br. Biol. 9, 163. doi: 10.3390/biology9070163
Terzo, S., Mulè, F., Caldara, G. F., Baldassano, S., Puleio, R., Vitale, M., et al. (2020). Pistachio consumption alleviates inflammation and improves gut microbiota composition in mice fed a high-fat diet. Int. J. Mol. Sci. 21, 365. doi: 10.3390/ijms21010365
Thengyai, S., Thiantongin, P., Sontimuang, C., Ovatlarnporn, C., and Puttarak, P. (2020). α-Glucosidase and α-amylase inhibitory activities of medicinal plants in Thai antidiabetic recipes and bioactive compounds from Vitex glabrata R. Br. stem bark. J. Herb. Med. 19, 100302. doi: 10.1016/j.hermed.2019.100302
Thomas, S. S., Kim, M., Lee, S. J., and Cha, Y. S. (2018). Antiobesity effects of purple perilla (Perilla frutescens var. acuta) on adipocyte differentiation and mice fed a high-fat diet. J. Food Sci. 83, 2384–2393. doi: 10.1111/1750-3841.14288
Thorning, T. K., Bertolt, C. J., Nielsen, M. S., Ritz, C., Astrup, A., Raben, A., et al. (2020). Potato fibers have positive effects on subjective appetite sensations in healthy men, but not on fecal fat excretion: a randomized controlled single-blind crossover trial. Nutrients 12, 3496. doi: 10.3390/nu12113496
Tung, Y. C., Chang, W. T., Li, S., Wu, J. C., Badmeav, V., Ho, C. T., et al. (2018). Citrus peel extracts attenuated obesity and modulated gut microbiota in mice with high-fat diet-induced obesity. Food Funct. 9, 3363–3373. doi: 10.1039/C7FO02066J
Umar, W., Hameed, M. K., Aziz, T., Maqsood, M. A., Bilal, H. M., Rasheed, N., et al. (2021). Synthesis, characterization and application of ZnO nanoparticles for improved growth and Zn biofortification in maize. Arch. Agron. Soil Sci. 67, 1164–1176. doi: 10.1080/03650340.2020.1782893
Unuofin, J. O., Otunola, G. A., and Afolayan, A. J. (2018). In vitro α-amylase, α-glucosidase, lipase inhibitory and cytotoxic activities of tuber extracts of Kedrostis africana (L.) Cogn. Heliyon 4, e00810. doi: 10.1016/j.heliyon.2018.e00810
Vezza, T., Rodríguez-Nogales, A., Algieri, F., Garrido-Mesa, J., Romero, M., Sánchez, M., et al. (2019). The metabolic and vascular protective effects of olive (Olea europaea L.) leaf extract in diet-induced obesity in mice are related to the amelioration of gut microbiota dysbiosis and to its immunomodulatory properties. Pharm. Res. 150, 104487. doi: 10.1016/j.phrs.2019.104487
Vishnupradeep, R., Bruno, L. B., Taj, Z., Karthik, C., Challabathula, D., Kumar, A., et al. (2022). Plant growth promoting bacteria improve growth and phytostabilization potential of Zea mays under chromium and drought stress by altering photosynthetic and antioxidant responses. Environ. Technol. Innov. 25, 102154. doi: 10.1016/j.eti.2021.102154
Wang, G., Yang, X., Wang, J., Zhong, D., Zhang, R., Zhang, Y., et al. (2021). Walnut green husk polysaccharides prevent obesity, chronic inflammatory responses, nonalcoholic fatty liver disease and colonic tissue damage in high-fat diet fed rats. Int. J. Biol. Macromol. 182, 879–898. doi: 10.1016/j.ijbiomac.2021.04.047
Wang, J., Suo, Y., Zhang, J., Zou, Q., Tan, X., Yuan, T., et al. (2019). Lycopene supplementation attenuates western diet-induced body weight gain through increasing the expressions of thermogenic/mitochondrial functional genes and improving insulin resistance in the adipose tissue of obese mice. J. Nutr. Biochem. 69, 63–72. doi: 10.1016/j.jnutbio.2019.03.008
Wang, M. Y., Ma, W. Y., Wang, Q. L., Yang, Q., Yan, X. X., Tang, H., et al. (2022). Flavonoid-enriched extract from Millettia speciosa Champ prevents obesity by regulating thermogenesis and lipid metabolism in high-fat diet–induced obese C57BL/6 mice. Food Sci. Nutr. 10, 445–459. doi: 10.1002/fsn3.2664
Wei, S., Zheng, Y., Zhang, M., Zheng, H., and Yan, P. (2018). Grape seed procyanidin extract inhibits adipogenesis and stimulates lipolysis of porcine adipocytes in vitro. J. Anim. Sci. 96, 2753–2762. doi: 10.1093/jas/sky158
WHO (2019). Stunting, Wasting, Overweight and Underweight. Available online at: https://apps.who.int/nutrition/landscape/help.aspx?menu=0&helpid=391&lang=EN (accessed July 25, 2022).
WHO (2020). WHOGlobal Health Observatory: Estimates of Prevalence of Overweight in Adults. Available online at: https://data.worldobesity.org/maps/?mapid=56&agegroup=AT&area=maps (accessed July 27, 2022).
WHO (2021a). Malnutrition. Available online at: https://www.who.int/news-room/fact-sheets/detail/malnutrition (accessed July 25, 2022).
WHO (2021b). Obesity and Overweight. Available online at: https://www.who.int/news-room/fact-sheets/detail/obesity-and-overweight (accessed July 25, 2022).
World Obesity (2022). Prevalence of Obesity. Available online at: https://www.worldobesity.org/about/about-obesity/prevalence-of-obesity (accessed July 25, 2022).
Wu, T., Gao, Y., Hao, J., Geng, J., Zhang, J., Yin, J., et al. (2020). Capsanthin extract prevents obesity, reduces serum TMAO levels and modulates the gut microbiota composition in high-fat-diet induced obese C57BL/6J mice. Food Res. Int. 128, 108774. doi: 10.1016/j.foodres.2019.108774
Xie, J., Wang, Y., Jiang, W. W., Luo, X. F., Dai, T. Y., Peng, L., et al. (2018). Moringa oleifera leaf petroleum ether extract inhibits lipogenesis by activating the AMPK signaling pathway. Front. Pharmacol. 9, 1447. doi: 10.3389/fphar.2018.01447
Xu, C., Mathews, A. E., Rodrigues, C., Eudy, B. J., Rowe, C. A., O'Donoughue, A., et al. (2018). Aged garlic extract supplementation modifies inflammation and immunity of adults with obesity: a randomized, double-blind, placebo-controlled clinical trial. Clin. Nutr. ESPEN 24, 148–155. doi: 10.1016/j.clnesp.2017.11.010
Xu, L., Nagata, N., and Ota, T. (2018). Glucoraphanin: a broccoli sprout extract that ameliorates obesity-induced inflammation and insulin resistance. Adipocyte 7, 218–225. doi: 10.1080/21623945.2018.1474669
Yimam, M., Jiao, P., Hong, M., Brownell, L., Lee, Y. C., Hyun, E. J., et al. (2019a). Evaluation of natural product compositions for appetite suppression. J. Diet. Suppl. 16, 86–104. doi: 10.1080/19390211.2018.1429518
Yimam, M., Jiao, P., Hong, M., Brownell, L., Lee, Y. C., Kim, H. J., et al. (2019b). Morus alba, a medicinal plant for appetite suppression and weight loss. J. Med. Food 22, 741–751. doi: 10.1089/jmf.2017.0142
Yu, S., Li, H., Cui, T., Cui, M., Piao, C., Wang, S., et al. (2021). Onion (Allium cepa L.) peel extract effects on 3T3-L1 adipocytes and high-fat diet-induced obese mice. Food Biosci. 41, 101019. doi: 10.1016/j.fbio.2021.101019
Yun, J. M., Jung, J., Park, S. H., Seo, Y. H., Lee, J. K., Bae, M. H., et al. (2022). Sunflower (Helianthus annuus) seed extract suppresses the lipogenesis pathway and stimulates the lipolysis pathway in high-fat diet-induced obese mice. Food Nutr. Res. 66, 8587. doi: 10.29219/fnr.v66.8587
Yuniarto, A., Putri, A. Z., Selifiana, N., and Adnyana, I. K. (2020). Preventive effect of ethanol extract of red spinach (Amaranthus tricolor L.) on diet-induced obese Zebrafish. Red 17, 18. doi: 10.9734/ejmp/2020/v31i2030354
Yusefi-Tanha, E., Fallah, S., Rostamnejadi, A., and Pokhrel, L. R. (2020). Zinc oxide nanoparticles (ZnONPs) as a novel nanofertilizer: Influence on seed yield and antioxidant defense system in soil grown soybean (Glycine max cv. Kowsar). Sci. Total Environ. 738, 140240. doi: 10.1016/j.scitotenv.2020.140240
Zhang, Q., de Mejia, E. G., Luna-Vital, D., Tao, T., Chandrasekaran, S., Chatham, L., et al. (2019). Relationship of phenolic composition of selected purple maize (Zea mays L.) genotypes with their anti-inflammatory, anti-adipogenic and anti-diabetic potential. Food Chem. 289, 739–750. doi: 10.1016/j.foodchem.2019.03.116
Keywords: agriceuticals, anti-obesogenic, biofertilization, nanofertilization, obesity
Citation: Guardiola-Márquez CE and Jacobo-Velázquez DA (2022) Potential of enhancing anti-obesogenic agriceuticals by applying sustainable fertilizers during plant cultivation. Front. Sustain. Food Syst. 6:1034521. doi: 10.3389/fsufs.2022.1034521
Received: 01 September 2022; Accepted: 14 November 2022;
Published: 30 November 2022.
Edited by:
Hamid El Bilali, International Centre for Advanced Mediterranean Agronomic Studies, ItalyReviewed by:
Lokesh Kumar Mishra, Central Agricultural University, IndiaMuhammad Adil Farooq, Khwaja Fareed University of Engineering and Information Technology (KFUEIT), Pakistan
Copyright © 2022 Guardiola-Márquez and Jacobo-Velázquez. This is an open-access article distributed under the terms of the Creative Commons Attribution License (CC BY). The use, distribution or reproduction in other forums is permitted, provided the original author(s) and the copyright owner(s) are credited and that the original publication in this journal is cited, in accordance with accepted academic practice. No use, distribution or reproduction is permitted which does not comply with these terms.
*Correspondence: Daniel A. Jacobo-Velázquez, ZGphY29ib3ZAdGVjLm14
†ORCID: Daniel A. Jacobo-Velázquez orcid.org/0000-0002-9478-2570