- 1Division of Organic Farming, Department of Sustainable Agricultural Systems, University of Natural Resources and Life Sciences, Vienna, Austria
- 2Faculty of Agriculture and Environmental Sciences, Mountains of the Moon University, Fort Portal, Uganda
- 3Department of Economics and Social Sciences, Institute of Agricultural and Forestry Economics, University of Natural Resources and Life Sciences, Vienna, Austria
Organic matter management (OMM) strategies such as farmyard manure (FYM) application, legume integration, crop residue incorporation, and alley cropping are recognized for improving soil fertility and crop productivity. However, studies on yield and economics of a combination of such strategies on smallholder farms are generally scarce, yet an understanding of such can enhance adoption. This study analyzed the yield and gross margins of crops grown with OMM strategies in comparison to those grown under inorganic fertilizer application on smallholder farms. Field experiments with five treatments over two short rainy (SR) and two long rainy (LR) seasons were conducted from January 2018 to February 2020 on 10 smallholder farms. The treatments (T) included T1 (control): the inorganic fertilizer application strategy that involved maize monocrop with 50 kg/ha Diammonium phosphate (DAP) application and the OMM strategies (T2-T5). T2: cowpea-maize-bean-maize rotation; T3: cowpea-maize-bean-maize rotation + 2.5 tons/ha FYM; T4: Faidherbia albida alleys + cowpea-maize-bean-maize rotation; and T5: Faidherbia albida alleys + cowpea-maize-bean-maize rotation + 2.5 tons/ha FYM. The maize in T3-T5 was intercropped with Mucuna pruriens. The results indicate that the grain and residue yields in LR were not significantly different among all treatments. The total variable costs, which included monetarized labor and annualized capital costs for the establishment of F. albida were significantly higher under T1 than in T2-T5 during LR2018 and not significantly different from what was observed under T3-T5 in LR2019. The accumulated revenues and gross margins for the four seasons were not significantly different between T1 and the OMM strategies. We conclude that the integration of OMM strategies can give gross margins similar to the 50 kg/ha DAP application. Further, based on the price sensitivity analysis, we conclude that the smallholder farmers could adopt T3 and T4 as the gross margins under these treatments are less affected by grain price fluctuations than in T1, T2, and T5. Since the smallholder farmers can access the planting materials, we recommend the adoption of T3 and T4 on smallholder farms.
Introduction
Agriculture in Uganda, where 95 percent of the farmers are smallholders with landholdings averaging two hectares, is characterized by low land productivity (Njeru et al., 2016). Taking an example of maize, which is one of the most important crops grown for food and sale in Uganda (Agona et al., 2001), its productivity in terms of grain yield has been recorded at 1.5 tons/ha compared to the potential of 7 tons/ha (Okoboi et al., 2012; Simtowe et al., 2019). One of the major factors contributing to these low crop yields is the lack of adequate nutrients for crops resulting from soil nutrient depletion (Tadele, 2017), low recirculation of animal manure, low share of legumes, lack of crop residue (straw) management, and low mineral fertilizer application regimes (Odhiambo and Mag, 2008). Soil nutrient depletion emanates from a complex of different non-adapted farming practices including continuous cropping without nutrient input, as well as farmers carrying away crop residues from the fields for feeding livestock without recycling nutrients through farmyard manure (FYM) application (Nkonya et al., 2005; Ronner and Giller, 2013; Tadele, 2017). The productivity is worsened by the reliance of farmers on the natural fertility of the soil since they lack mineral fertilizers and if applied, they are washed out by surface runoff (Woniala and Nyombi, 2014). The low transfer rates of mineral fertilizer nutrients to the crops (nutrient availability) as a result of soil compaction, reduced root growth, negative humus balances, low water holding capacity, and leaching processes (Tadesse et al., 2013; Massah and Azadegan, 2016), can force the farmers to abandon mineral fertilizer application. There is a knowledge gap on how to increase synthetic fertilizer uptake, i.e., productivity via other management strategies. Other reasons for the reliance of farmers on natural soil fertility include the prohibitive prices and limited accessibility of mineral fertilizers by farmers located in remote areas (Nziguheba et al., 2016).
Although trade-offs with family income due to costs of acquisition, transport, and application of organic matter management (OMM) strategies like vermicompost have been reported (Flores-Sánchez et al., 2015), other OMM strategies such as FYM application, alley cropping, forage & grain legume integration, and crop residue incorporation on arable land have been documented for increasing soil characteristics. These soil characteristics include soil carbon, soil water-holding capacity, nutrient storage and availability (Birhane et al., 2018; Beuschel et al., 2019; Bu et al., 2020). In addition, the application of OMM strategies reduces soil compaction and increases crop productivity (Lupwayi et al., 2011; Akmal et al., 2015; Birhane et al., 2018; Beuschel et al., 2019; Bu et al., 2020). Organic matter management therefore, to a certain degree reduces the dependency on mineral fertilizers but also acts as a precondition for optimal utilization and uptake of nutrients by plants (De Moura et al., 2010; Pirhofer-Walzl et al., 2012). The multiple functions OMM contributes to ecosystem services cannot be delivered by commercial mineral fertilizers (Mikha et al., 2017). Moreover, the application of OMM strategies requires less finance (cash) compared to the inorganic mineral fertilizers and the inputs are readily available to most farmers. In contrast to mineral fertilizers, the diverse OMM strategies offer several benefits:
• If forage and alley legume trees such as Faidherbia albida are integrated into the farming system, nitrogen demand can be covered as the trees can contribute about 80.2 kg N/ha/season when they are mature. This is about the recommended average application rate of mineral nitrogen fertilizer for maize production in Uganda (Mugendi et al., 1999; Sunday and Ocen, 2015; Yengwe et al., 2018; Kohler, 2020; Silva-Galicia et al., 2020).
• Organic matter from decomposed biomass protects nutrients against leaching, feeds microorganisms, stores nutrients, and increases their availability (Yang et al., 2018).
• Specifically, forage and alley legume trees with their deep rooting system can uptake nutrients from the subsoil, and transfer them into the main rooting zone, enriching the nutrient concentration (De Moura et al., 2010; Pirhofer-Walzl et al., 2012). This vertical (re-)transfer of the farm nutrient cycle of probably leached nutrients is not an addition of nutrients per se, but an enrichment of soil stock nutrients in the upper layer.
• Organic matter includes not only one or two nutrients, as is usually the case when applying mineral fertilizer, but a broad range of macro and micronutrients that are offered to the soil and crop in a more or less available form.
It should be noted that all nutrients in the biomass are from the soil's nutrient stock if no deposition, mineral fertilizer, organic manure, or forage are added from outside of the farm, except nitrogen, which also results from natural fixation processes.
Although several OMM strategies have been applied to improve soil fertility (Cai et al., 2019; Sánchez-Navarro et al., 2019; Vanlauwe et al., 2019), their systematic and comprehensive application is still an exception, and soil nutrient depletion and low crop productivity have persisted (Woniala and Nyombi, 2014). The reason for the low adoption rate of the OMM strategies such as tillage systems could be related to their high labor requirements (Dahlin and Rusinamhodzi, 2019), and the limited knowledge about the positive impact on yield and economics (costs, revenues, and profits). This could specifically be the case if more than two strategies (for example the application of grain and forage legumes, alley trees, and FYM) are jointly applied. While the positive influence of single OMM strategies is well known and studied (Akmal et al., 2015; Yengwe et al., 2018; Bu et al., 2020), literature on the impact of combined OMM strategies on yield and economic parameters is limited. Moreover, training and advisory services have not been sufficient in extending related information to farmers (Sebaggala and Matovu, 2020). This study tested the effects of combined OMM strategies such as alley cropping, FYM application, as well as grain and forage legume integration compared to inorganic fertilizer application strategy on (a) crop yield, (b) costs and (c) gross margins. This study hypothesizes that the application of OMM strategies would (1) increase crop yield to amounts equal to the inorganic fertilizer application strategy; (2) reduce costs compared to the inorganic fertilizer application strategy; (3) yield gross margins that are comparable to the ones obtained through the inorganic fertilizer application strategy.
Materials and methods
This study applied a systems perspective that involved both natural science and economic perspectives. The first part of this section describes the study area and the field trials including the experimental design, choice of crops, and sampling procedure. This section was adapted from the methodology developed by Ekyaligonza et al. (2022). The second part of this section is the economic analysis of the different OMM strategies (treatments) over four crop-growing seasons in 2018 and 2019.
Study area
A field experiment was conducted on 10 randomly selected farmer fields that are located in Nyabbani Sub-county of Kamwenge district (00°11.17′ N, 30°27.07′ E, 1160 m a.s.l.) in the Rwenzori region of Uganda (Figure 1). The study area experiences a tropical climate with an annual temperature range of 20-25° C and annual rainfall of 700-1,400 mm with a short rainy season (SR) from March to May and a long rainy season (LR) from August to November (FAO, 2005). In 2018, the study area received a total of 524.8 mm of rain in SR and 657.2 mm in LR, while in 2019, a total of 385.6 mm of rain was received in the SR and 578.9 mm in LR (Figure 2). Any possible differences between sites due to climatic conditions were taken care of by the randomization and replication effect of the experimental design. The soils were dominantly sandy clay loamy acrisols (about 57% sand, 19% silt, and 24% clay in the topsoil) and were acidic with a pH (H2O) of approx. 5.1, a cation exchange capacity (CEC) of 5 cmolc/kg, low organic carbon of 0.8%, and bulk density of 1.41 kg/dm3 (FAO, 2012). Such soils require adjustment of pH and organic matter management to increase water holding capacity and plant nutrient uptake.
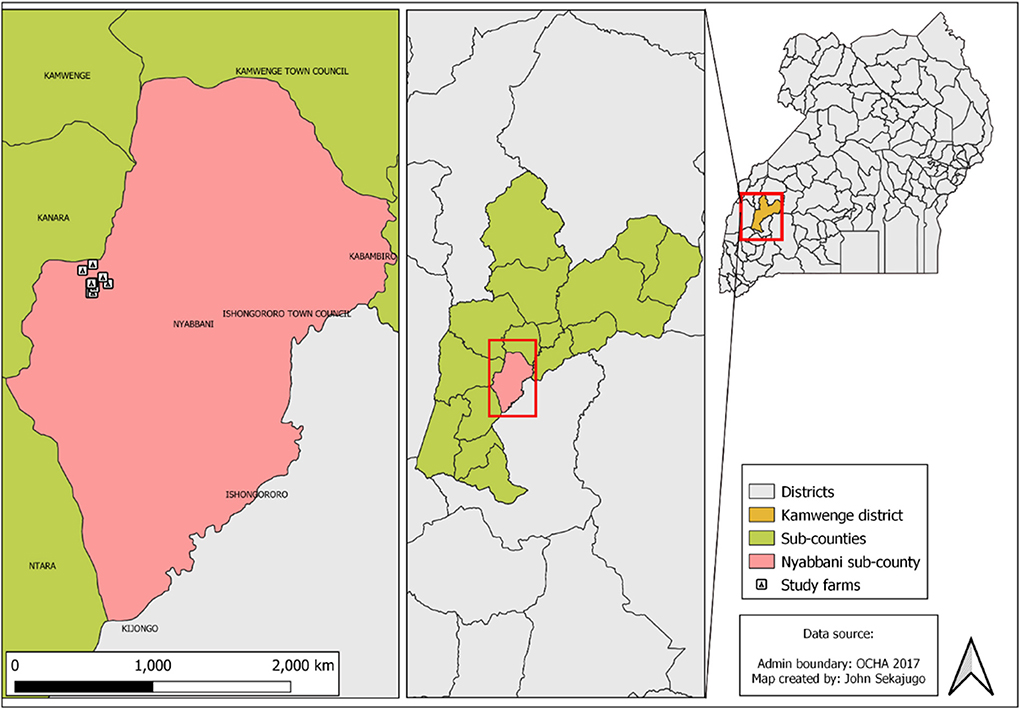
Figure 1. Location of the study farms in Kamwenge district, Uganda. Source Ekyaligonza et al. (2022).
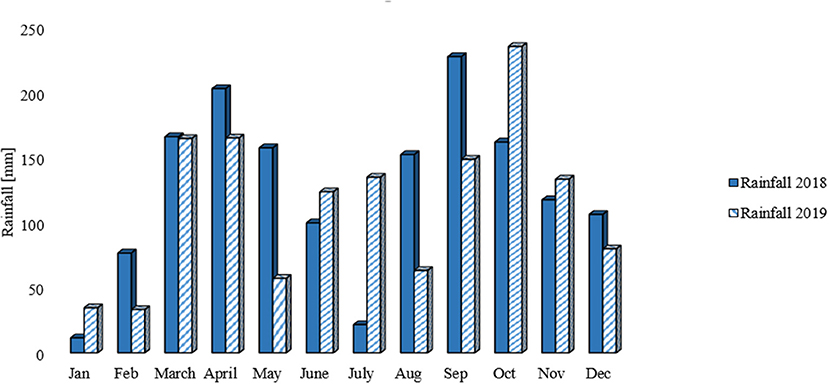
Figure 2. Rainfall distribution of Kamwenge district for the period of two years in 2018 and 2019. Source Ekyaligonza et al. (2022).
Field experiments
Experimental design, treatments, and sampling
Field experiments were conducted for 2 years from January 2018 until February 2020 in four consecutive crop-growing seasons: two for SR and another two for LR. The trials were established on 10 smallholder farms and the owners of the farms fully participated in carrying out all the relevant agronomic practices. A randomized complete block design (RCBD) was established, where each farm was considered a block (n = 10). Five treatments were randomly allocated per block. The five treatments (T1-T5) included:
• T1: maize monocrop with Diammonium phosphate (DAP) fertilizer (18-46-0) at an application rate of 50 kg/ha (N: 9 kg/ha; P2O5: 10.05 kg/ha), a rate affordable to most maize subsistence farmers in the Rwenzori. This was treated as control as it is a common practice to use low doses of fertilizers among the majority of the smallholder maize farmers in the Rwenzori region;
• T2: Cowpea-maize-beans-maize rotation. The initial plan was to rotate cowpea with maize but the farmers opted for beans in the SR2019 following the low cowpea yields realized during SR2018;
• T3: Cowpea-maize/Mucuna pruriens-beans-maize/Mucuna pruriens rotation + farmyard manure (FYM) from cattle containing N: 13 kg/ha; P: 6 kg/ha; K: 18 kg/ha. The FYM was applied at an application rate of 2.5 tons/ha as fresh matter, which is the feasible amount that can be produced by most smallholder farmers in the Rwenzori region;
• T4: Cowpea-maize/Mucuna pruriens-beans-maize/Mucuna pruriens rotation + Faidherbia albida alleys;
• T5 Cowpea-maize/Mucuna pruriens-beans-maize/Mucuna pruriens rotation + FYM + F. albida alleys (see Table 1 for the detailed description of the treatments).
For each farm, the five treatments were allocated to five plots with dimensions of 10 m x 10 m each. These plots were separated by 0.5 m walkways. The plots were tilled with a hand hoe and then seeds of selected crops were sown. Selection of crops and varieties was done according to the following criteria: (i) Maize (Zea mays) was selected because it is one of the major staple foods for the majority of the population in East Africa and it is cropped for both food and cash (Agona et al., 2001). In this study, maize variety Longe 5 at a rate of 24.7 kg/ha was selected because of its high share of protein and its drought tolerance (FAO, 2017). This maize variety matured 115 days after sowing; (ii) The legume cowpea (Vigna unguiculata) produces high biomass and nitrogen in a short time and is known for its high pre-crop value (Omae et al., 2014). The grains and leaves of cowpeas are served as food to some tribes in Uganda. SECOW 5T cowpea variety was planted at a rate of 25 kg/ha during SR2018. This variety was selected due to its ability to survive under the dry climatic conditions of the study area; (iii) The legume M. pruriens var. utilis was selected because it grows very fast providing good soil cover, fixes a high share of nitrogen, and is adapted to dry seasons (Chakoma et al., 2016). M. Pruriens seed was purchased from a farmer group in Kasese district in the Rwenzori region and it was intercropped with the 30-day-old maize at a rate of 20 kg/ha; (iv) The legume tree F. albida was selected because it fixes nitrogen and has reversal phenology, shedding off the leaves during the rainy season and developing leaves during the dry season. This attribute enables the trees to grow well with minimum water supply and competition with other crops (Roupsard et al., 1999). F. albida of Moroto provenance, which was the only seed provenance available at the Uganda National Tree seed center, was planted at a rate of 1000 seedlings/ha (one tree/10 m2); (v). The grain legume beans (Phaseolus vulgaris) were included in the rotation system in SR2019 following the farmers' request to replace the cowpeas which exhibited low yields during SR2018. Beans are rich in proteins and therefore part of human diets both in urban and rural areas (Aseete et al., 2018). Nabe 4 bean variety was selected because it matures early after 80-85 days and has high resistance against anthracnose and the bean common mosaic virus, which are the most prevalent diseases in the Rwenzori region (Kankwatsa, 2018). Nabe 4 was sown in SR2019 at a rate of 20 kg/ha. Harvesting was done for mature crops in such a way that only maize ears or bean/cowpea pods were picked and crop residues (leaves and stalks) left in the field for further organic matter improvement. These crop residues and M. pruriens were chopped with a machete into smaller pieces of about 3 cm in length. The chopped material together with F. albida leaves were then incorporated within 0-15 cm soil depth using a hand hoe during the land tilling process. A combination of crop residues, M. pruriens and F. albida leaves is later referred to as plant residues in the subsequent sections. As indicated in Ekyaligonza et al. (2022), the quantity of plant residues that were incorporated into the soil in T1 was 18, 11, 11 and 15 tons/ha during SR2018, LR2018, SR2019, and LR2019 respectively, while those incorporated into the OMM treatments was 2.9–3.6, 24–137, 61–180, and 15–145 tons/ha during SR2018, LR2018, SR2019 and LR2019 respectively depending on the treatment. The total nutrients in these plant residues is described in Table 2. Land tilling, FYM application, sowing, two times weeding by hand pulling (the uprooted weeds were distributed in the field), and harvesting were done by the farmers under the guidance of the researchers.
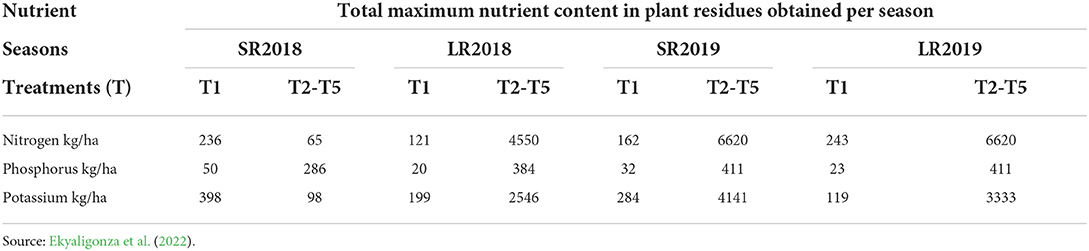
Table 2. Nutrient content of plant residues recycled into the soil in the four crop-growing seasons of 2018 and 2019.
When the crops were mature and ready for harvesting, dry matter (DM) yield was determined. Sample collection was done in such a way that 10 plants from each crop type were randomly selected per plot. The plants within the first two rows from the plot boundary were excluded from the sample to minimize the boundary effect. The grains and crop residues were oven-dried separately at 70°C until a constant weight was obtained. For each plot, the average DM grain yield and DM residue yield were weighed and measured as kg/ha and then converted to ton/ha. A high DM grain yield is expected to improve food and income security, while a high DM residue yield shows the potential of the cropping system to recycle biomass, improve soil organic matter and recycle nutrients.
Soil sampling and testing
Soil was sampled and tested before and after experimentation to find out the contribution of the different cropping systems (treatments) on soil fertility improvement. Initial soil sampling was conducted in January 2018 before the fields were subjected to experimental conditions to understand the level of nutrients in the soil. Final soil sampling was conducted in January 2020 to determine the contribution of each treatment to the improvement of pH, OM, WHC, N, P, and K levels in the soil. N, P, and K are macronutrients which are essential for crop growth enhancement (Lhamo and Luan, 2021), while pH, WHC and OM influence availability of nutrients to crops (Neina, 2019). In January 2018, a composite soil sample from five sub-samples was collected from each of the 10 study farms/blocks. These farms were relatively uniform in terms of terrain and vegetation. The soil sub-samples were randomly collected at 0-15 cm soil depth with an aid of a soil Auger. In January 2020 after the four-season experiment, soil sampling was repeated and a composite sample was collected from each of the 50 experimental plots.
The soil samples were tested for pH, OM, WHC, and the plant-available forms of N, P, and K. These tests can help to explain the impact of our experiments on land productivity. Soil pH was determined through an electrometric method using a pH meter (Eutech pH 700 meter). In the procedure for pH determination, fifty (50) ml of distilled water was added to a beaker containing 20 g of air-dried soil of 2 mm particle size. The content of the beaker was stirred for 5 min. The grass electrode was then calibrated using a standard buffer of pH = 7 and pH = 4. The electrodes were then dipped into a beaker containing the soil-water suspension with constant stirring. The pH meter was then switched to pH reading and the pH value was then taken after 30 s. Organic matter was determined through the ignition of the samples at high temperatures (up to 550 °C) (Pawar et al., 2009). In the procedure for OM determination, 20 g of soil was dried at 104 °C for 24 h. It was placed on an aluminum foil and then put in an oven to dry at 550 °C for 8 h. The soil was then left to cool and then weighed. The weight difference between soils weighted before and after being subjected to very hot conditions is the OM of the soil. For WHC, 20 g of air-dried soil of 2 mm particle size was placed on a filter paper that was fixed onto the internal floor of a perforated dish. The filter paper prevents soil particles from escaping through the dish perforations. The perforated dish with its content was then placed on a trough containing water and allowed to stand for 6 h so that the soil can get saturated with water. Water holding capacity was determined by calculating the difference between the weight of air-dried soil and that of the soil saturated with water (Pawar et al., 2009). The soil N in plant-available form was determined through the hot hydrogen peroxide/potassium chloride (H2O2/KCl) extraction method following Sahrawat (1982) and Tie et al. (2013) where the soil was air-dried and sieved through a 2 mm sieve. Fifty (50) ml of 25% H2O2 was added to a 300 ml conical flask containing 5 g of sieved soil. The conical flask with its content was then placed into a ventilated oven and heated for 6 h at a temperature of 60°C. The suspension was cooled, 1 M KCl was added and the mixture rotated for 30 min. Ammonium Nitrogen (NH4+-N) from the filtrate was determined through distillation. The plant available form of phosphorus was determined through Bray 1 extraction method in the procedure by Kovar and Pierzynski (2009) where 20 ml of Bray1 extraction solution [0.025 M hydrochloric acid (HCl) in 0.03 M ammonium fluoride (NH4F)] was added to 2 g of soil in a 50 ml conical flask. The flask with its contents was shaken at 200 revolutions per minute at room temperature. The plant-available P was measured from the filtrate by use of a spectrophotometer (VWR- UV- 6300PC) at a wavelength of 880 nm (Kovar and Pierzynski, 2009). Potassium (K) in plant-available form was determined following a flame photometry procedure with ammonium acetate (NH4OAc) extractant (Okalebo et al., 2002; Pawar et al., 2009) where 100 ml of NH4OAc was added to a conical flask containing 5 g of air-dry soil sample. The flask and its content were shaken at 200 oscillations per min for 30 min and the solution was left to stand for 30 min. The supernatant was filtrated through the Whatman No. 42 filter paper and the extracted solution was diluted 10 times. Five (5) ml of the solution was pipetted into a 50 ml volumetric flask and 1 ml of lanthanum chloride solution was added. The contents were then diluted with NH4OAc extraction solution to the mark. Potassium (K) content was determined by spraying the soil extract, lanthanum chloride, and ammonium acetate solution onto the flame of the flame photometer (PFP7 model).
Farmyard manure was analyzed for N, P, and K using Kjeldahl, Bray-1 and flame photometry methods respectively. For N, analysis followed the procedure by Hendershot et al. (1993) where 3 ml of deionized H2O was added to a dry digestion tube containing 2 g of ground manure. Three and a half (3.5) g of K2SO4: CuSO4 was mixed with the contents of the digestion tube and one selenized and one non-selenized Hengar granule were added. Ten (10) ml of concentrated sulphuric acid (H2SO4) was added and the content of the digestion tube was digested for 1.5 h at 220 °C. Air condensers were put onto the digestion tubes in the block and the temperature raised further to 360° C for 3.5 h. The samples were then cooled overnight in the block. The air condenser was then removed. Slowly and with swirling, 25 ml of deionized water was added to each cooled digestion tube. The content in the digestion tube was then transferred to a 0.5 l round bottom distillation flask and connected it to the steam distillation apparatus. One hundred (100) ml of the graduated beaker with 5 ml of 2% boric acid (H3BO3) was placed under the condenser. Very slowly, 30 ml of excess of 10 M sodium hydroxide (NaOH) was added through the distillation head. Forty (40) ml of distillate was collected. The distillate was titrated with 0.01 M H2SO4. The color change at the endpoint is from green to pink. Nitrogen was calculated from the molarity of H2SO4, the volume of H2SO4 used during titration of the sample, the volume of standard H2SO4 used during titration of the blank, and the mass of the oven-dried soil sample. The procedure for testing P and K was the same as that of soil.
Economic analysis
Grain revenue and gross margin calculations were conducted for each season and the total for the entire four-season rotation to establish the economic impact of the OMM strategies. The grain revenues were calculated from the yield and selling price of the grains (Equation 1). The prices used in calculating revenues were the farm gate prices of the grains in the study area in 2018 (Table 3). These prices were determined by middlemen as they are the main actors who buy produce directly from the farmers. Gross margins were calculated from the total variable costs and revenues, following other studies (Beuchelt and Zeller, 2011; Uddin et al., 2016) (Equations 1 and 2):
The total variable costs included all the input costs, monetarized labor costs, and the total annualized costs of establishing F. albida alleys during the first year. Opportunity costs for the land (lease or rent), administration costs, and other fixed costs were however excluded from cost calculations, as they were fixed and therefore common factors for all treatments. The input costs were the actual costs incurred in buying inputs (Table 3). These were the costs incurred in buying maize and legume seeds, as well as fertilizers. Family labor that was used to conduct all the agronomic practices such as land preparation, manure collection and application, sowing of maize and legume seeds, and harvesting was also determined. Labor was considered a key factor hindering the establishment of OMM strategies as different treatments have different labor requirements. The labor costs were valued in terms of money as farming was the only income-generating activity for all the farming families that participated in the study. These labor costs were estimated by the farmers based on the work per completed task, which is one of the main ways of determining labor within the study area (Table 3). These labor costs were based on what hired labor would be paid for similar tasks. For the annualized costs, all the costs associated with F. albida tree alleys during the first year (two seasons) such as purchasing the seedlings, pitting, planting the trees as well as providing extra care not to damage the trees during land preparation and weeding of fields were considered. These costs were annualized for 20 years. A lending interest rate of 20 % per year was used, as indicated by UBOS (2019) and the World Bank database of 2018. Since there are two crop-growing seasons per year, the annualized costs were calculated for 40 seasons (20 years), using an interest rate of 10 % per season. Annualizing costs of establishing the trees, assuming a useful life of 20 years, allows comparison of establishment costs of alley cropping systems with cropping systems that solely involve annual crops. Furthermore, it also enables farmers to know how much they need to pay back per season in case they received the total establishment costs as a loan. The annualized capital costs per season for the establishment of an alley cropping system were calculated following equation 3.
Where,
C = Annualized capital costs per season
EC = Establishment costs
r = interest rate per season (10 %)
n = number of periods (40 seasons)
Since price fluctuation is one of the challenges that affect Uganda's agricultural production (Bamwesigye et al., 2020), a sensitivity analysis was conducted to determine the impact of price fluctuation on gross margins. This was conducted for each of the five treatments per season. For each of the three studied food crops (cowpea, beans, and maize), price scenarios were set and these included the highest, lowest, and the 2018 food prices since the harvests from our field experiment were sold starting with the year 2018. The highest, the lowest, and the 2018 prices of maize and beans were the farm gate prices. For cowpeas, the highest and the minimum prices were obtained from Ddungu et al. (2015) as the crop was traditionally not grown on a large scale in the study area. For maize, the lowest price was 0.14 US$ in 2012 (83% of the 2018 maize price) and the highest maize price was 0.29 US$ in 2015 (167% of the 2018 maize price). The lowest bean price was 0.19 US$ in 2014 (22% of the 2018 price). On the other hand, the highest bean price was the same as that of 2018. For the cowpeas, the lowest price was in 2018 while the highest was 0.63 US$ in 2009-2011 (110% of the 2018 cowpea price).
Statistical analysis
A linear mixed model (LMM) was used to analyze data as it was continuous, having “treatment” as a fixed factor and “block (farm)” as a random factor. Moreover, LMM has been documented as a suitable analysis procedure for continuous data with both the fixed factor and the random factor effects (Molenberghs and Verbeke, 2001). This data was analyzed in IBM SPSS Statistics (version 26) software using the SPSS mixed command. The dependent variables were the soil physical-chemical properties, grain yield, residue yield, grain revenue and gross margins, while the independent variable was the treatments. Data for each season was analyzed separately as the cropping systems involved rotation of different crops. The results in the table “Type III Tests of Fixed Effects” enabled us to understand whether there were significant differences in soil physical-chemical properties, grain yield, residue yield, grain revenue and gross margins among treatments while the “estimates of Fixed Effects” table enabled us to come up with pairwise comparisons of the grain yield, residue yield, grain revenue and gross margins among the treatments.
Data for the variable costs were however analyzed using an independent samples nonparametric test, as the outputs with LMM analysis could not be obtained. In addition, the data did not conform to the normality and homogeneity of variance conditions even after being subjected to transformation processes such as logarithm, natural logarithm, and Cosine.
Descriptive analysis was conducted to compare yields, costs, revenues, and gross margins among treatments and was used as a supplement to LMM and the independent samples' non-parametric tests.
Results
We compared the soil parameters in soils collected at the end of the experiment to determine the effect of the different cropping systems on soil nutrient content. In addition, we compared the parameters between the initial and final soil samples to determine any possible residual effects created by the different treatments. The yields, costs, revenues, and gross margins were compared between treatments of the same season. However, yield comparisons between seasons for the different treatments were only possible between LR2018 and LR2019 since they involved the same crops. In addition, the accumulated revenues and gross margins for each treatment across the four seasons were calculated to identify the most profitable cropping system.
Impact of OMM strategies on soil physical-chemical properties
The soil pH was moderately acidic (pH ranges between 5.3 and 6.0) both before and after experimentation (Pawar et al., 2009) (Tables 4, 5). In addition, there were no significant differences in soil pH among all the five treatments after the experiment (P > 0.05) (Table 5). Like pH, the WHC was not significantly different among all the treatments after the study period (P > 0.05), but the amounts were higher after than before the soil was subjected to the different treatments (Tables 4, 5). The OM content of soil tested before the experiment was high, while that of soils tested after the experiment was very high (Tables 4, 5) (Pawar et al., 2009). However, the OM content of the soil collected after the experiment was not significantly different among all treatments (P > 0.05) (Table 5). The plant-available N content can be described as low (140–280 kg/ha) in soils tested before the experiment but high (562–700 kg/ha) in soils tested after the experiment (Pawar et al., 2009) (Tables 4, 5). In addition, plant-available nitrogen in soil collected after the experiment was not significantly different among all the five treatments (P > 0.05). For the plant-available P, the content was within the medium range (13–22 kg/ha) before and after subjecting the fields to the experimental conditions (Pawar et al., 2009) (Tables 4, 5). The P content in soils collected after the experiment was however not significantly different among all the treatments (P > 0.05) (Table 5). The plant-available K content was moderate (181–240 kg/ha) before the study and moderately high (241–300 kg/ha) after the study (Pawar et al., 2009) (Tables 4, 5). From the soil samples collected after the experimental period, there were no significant differences in plant-available K content observed among all the treatments (P > 0.05) (Table 5).
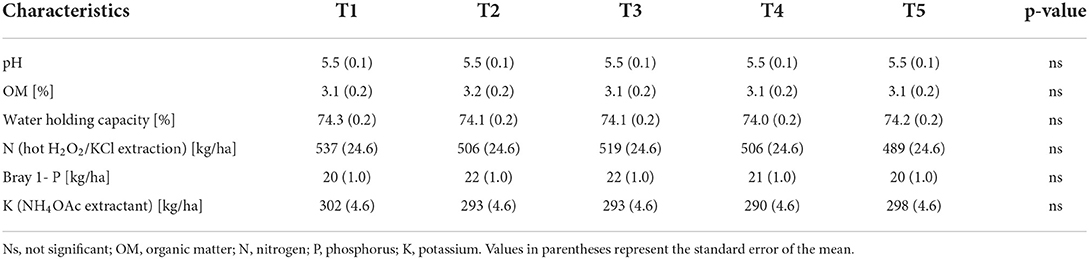
Table 5. Comparison of soil physical-chemical characteristics between treatments after the experiments in January 2020.
Impact of OMM strategies on dry matter yield of crops
A season-per-season yield comparison for different treatments showed that DM grain and residue yield were significantly higher under T1 than in OMM treatments (T2-T5) during the SR (P > 0.05) (Table 6). The DM grain and residue yields in T1 were however not significantly different from those in the OMM treatments during the LR except for T3 were grain yields were lowest in comparison to other treatments (P > 0.05) (Table 6). Considering the OMM treatments (T2-T5) in SR alone, there were no significant differences in DM grain yield and DM residue yield observed (P > 0.05) (Table 6). Since the treatments in LR2018 and LR2019 were similar, DM grain and DM residue yields were compared between the two seasons. The DM grain and DM residue yield however increased from LR2018 to LR2019. For instance, treatments T4 and T5 showed the highest grain yield increase of 46.3 and 42.6%, respectively from LR2018 to LR2019. T1 had the lowest grain yield increase of 24.2 %, whereas, in T2 and T3, the yield increase amounted to 29.0 and 25.9 %, respectively from LR2018 to LR2019 (Table 6). The crop residue yield increased by 25.2, 36.4, 36.9, 45.0, and 46.1% for T1, T2, T5, T4, and T3, respectively from LR2018 to LR2019 (Table 6).
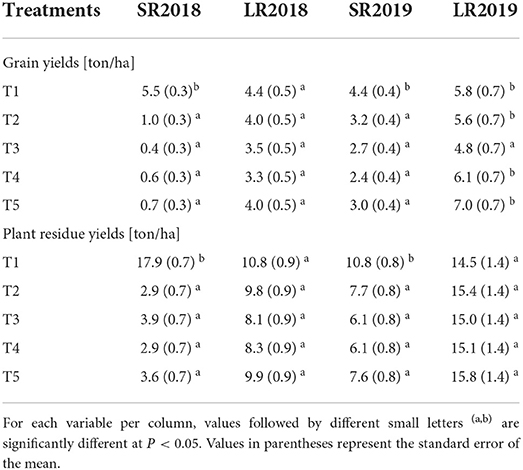
Table 6. Comparison of grain and residue yields between treatments over 4 crop-growing seasons during 2018 and 2019.
Costs of establishment and maintenance of OMM strategies
Generally, the accumulated total variable costs for the entire four-season rotation system, which included an additional annualized capital cost of 25 US$ to T4 and T5 for the establishment and maintenance of F. albida alleys were significantly lower in T2 than in other treatments (T1 and T3-T5) (P < 0.05). The total variable costs for the entire four-season rotation were however not significantly different between T1, T3 andT4 (P > 0.05) (Table 7). In a season-per-season cost comparison, the total variable costs were significantly higher under T3 and T5 than in other treatments during SR (P < 0.05). For the LR, the total variable costs were on the other hand significantly higher under T1 than in treatments with OMM strategies during LR2018 but these costs were not significantly different between T1 and T3-T5 during LR2019 (P < 0.05) (Table 7).
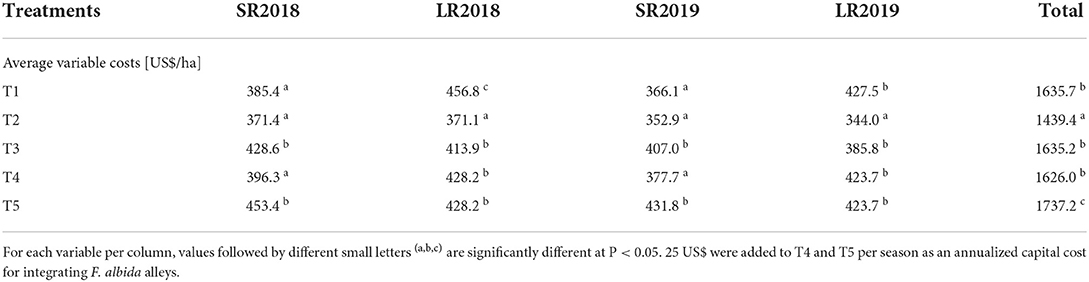
Table 7. Comparison of total variable costs incurred during implementation of treatments in the four crop-growing seasons during 2018 and 2019.
Revenues and gross margins generated through establishment and maintenance of OMM strategies
The total grain revenues for the entire four-season rotation system were not significantly different among T1, T2 and T5. It was significantly higher under T1, T2 and T5 than in T3 and T4. From the season-per-season grain revenue comparison, it can be seen that the grain revenue in SR2018 was significantly higher under T1 than in treatments T2-T5 with the OMM strategies (Table 8). For SR2019, grain revenue was significantly higher in T2-T5 than in T1 (P < 0.05), and no significant differences in grain revenues were observed among treatments T2- T5 (P > 0.05). For the LR2018 and LR2019, no significant differences in grain revenues were observed among all the treatments (T1- T5) except T3, which yielded lower revenues than in other treatments during LR2019 (Table 8).
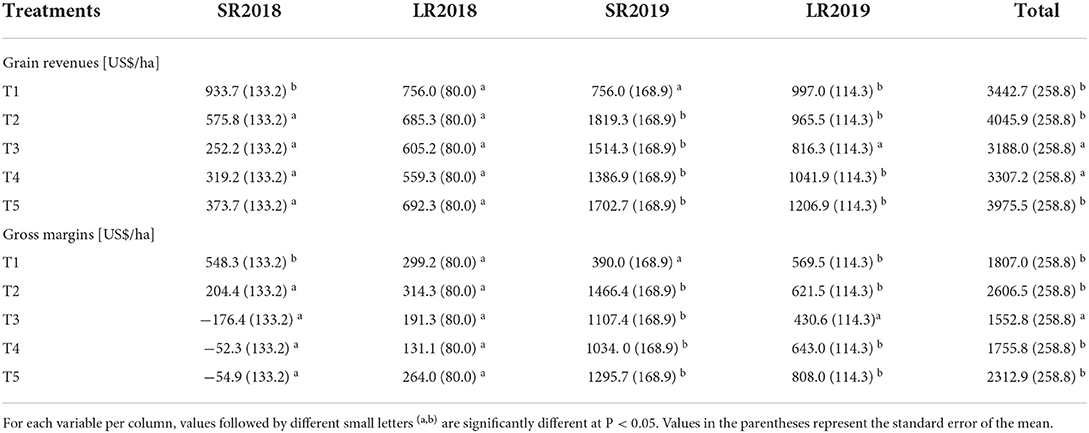
Table 8. Comparison of grain revenues and gross margins between treatments over 4 crop-growing seasons during 2018 and 2019.
Gross margins followed a similar trend as the grain revenues except for the entire rotation where T3 resulted into the lowest gross margins in comparison to other treatments (P < 0.05). The gross margins were reduced by 28.9% for T1 and increased by 86.0, 115.9, 105.0, and 104.0% for T2, T3, T4, and T5, respectively from SR2018 to SR2019. For the long rains, the gross margins increased for all treatments by 48.0, 49.0, 56.0, 79.0, and 67.0%, and for T1, T2, T3, T4, and T5, respectively, from LR2018 to LR2019 (Table 8).
The results of the price sensitivity analysis indicate that the total gross margins under T1, T2, T3, T4, and T5 that would be obtained from the lowest prices of the crops grown in the entire rotation system would be 34, 60, 85, 72, and 66% lower than the gross margins obtained from the 2018 farm gate prices respectively. The gross margins that were calculated from the highest prices under T1, T2, T3, T4, and T5 would be 132, 45, 63, 62, and 56% higher than the ones obtained from the 2018 farm gate prices respectively (Figure 3). For the season-per-season comparison, the lowest maize price would result in 29% lower gross margins whereas the highest maize price would result in 120% higher gross margins than those obtained from the 2018 price during SR2018. For cowpeas, the price in 2018 was the lowest but the highest price would result in a 29, 15, 15, and 24% increase in gross margins for T2, T3, T4, and T5 respectively. During LR2018, the lowest maize price would result in 37, 40, 58, 86, and 52% lower gross margins for T1, T2, T3, T4, and T5 respectively than those obtained with the 2018 farm gate price. The highest maize price would result in 180, 151, 219, 287, and 177% higher gross margins for T1, T2, T3, T4, and T5 respectively than those obtained from the 2018 farm gate price. For SR2019, the lowest maize price would result in a 37% reduction in gross margins while the highest maize price would result in a 133% increase in gross margins. For beans, the lowest price would result in 84, 93, 91, and 89% lower gross margins than those obtained from the 2018 farm gate price for T2, T3, T4, and T5 respectively. The 2018 bean price was the highest farm gate price from 2010 to 2018. In LR2019, the lowest maize price would result in 35, 33, 41, 32, and 29% lower gross margins while the maximum maize price would result in 118, 103, 125, 110, and 101% higher gross margins than those obtained from the 2018 farm gate price for T1, T2, T3, T4, and T5 respectively (Figure 3).
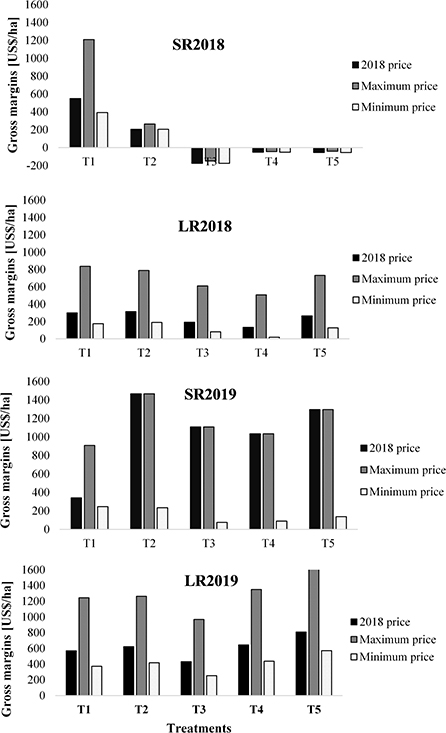
Figure 3. Comparison of gross margins (US$/ha) with different price scenarios among treatments over 4 crop-growing seasons during 2018 and 2019.
Discussion
Impact of OMM strategies on soil physical-chemical properties
The higher plant-available form of N in soils sampled after the experiment than those sampled before could be attributed to the legume-maize rotation practice for treatments T1-T4 with OMM practices and the DAP together with crop residue application for T5. Moreover, there is evidence that the inclusion of legumes into a cropping system can improve soil N and other nutrients that are crucial for crop growth (Houngnandan et al., 2000; Imoro et al., 2013; Nassary et al., 2020). The nutrients accumulated by such systems could have improved crop growth with higher grain and crop residue yields. The incorporation of crop residues into the soil could have further increased the OM and soil nutrients. These results are in agreement with a study by Bu et al. (2020) where crop residue incorporation increased soil organic carbon and total N concentrations by 18.81 and 22.73%, respectively in a 12-year experiment that was established in 2007. Water holding capacity was higher after the experiment (74.0–74.3 %) than before (62%). This could be explained by the organic matter formed after the decomposition of the crop residues incorporated in all the treatments. The pH levels were however within the same range before and after the experiment, possibly, because both soil sample sets were collected in the same month although in different years (January 2018 and 2020). The plant-available P content from DAP in T1 and the different combinations of OMM strategies in the other treatments did not substantially change, compared to the situation before the trials. The reason might be the low nutrient contribution by the applied 50 kg/ha DAP for T1 and the organic materials such as crop residues incorporated in T2-T5 or export of nutrients via crop harvest for all treatments.
The similar effect in pH, WHC, and the plant-available forms of N, P, and K after the experiment could be explained by the nutrient uptake by the crops during the crop growing season as the soil was sampled 1 week after harvesting the grain.
The soil after our experiments had higher N and K levels (489–537 and 290–302 kg/ha respectively) compared with the recommended nutrients (180, 74 and 157 kg/ha of N, P and K respectively) that are required for proper maize growth (MAAIF, 2019). However, the P levels (20–22 kg/ha) were below the recommended levels. If phosphorus levels were increased, the yields would probably increase further. Since this study only focused on macronutrients, future studies should also test the impact of OMM strategies on soil micronutrients.
Impact of OMM strategies on dry matter yield of crops
Organic matter management is an important strategy for improving soil fertility and DM crop yield (Lupwayi et al., 2011; Akmal et al., 2015; Beuschel et al., 2019; Bu et al., 2020). The significantly higher DM grain and residue yield in T1 than in the OMM treatments (T2-T5) during SR could be explained by the different crops integrated into the different treatments as T1 involved maize while T2-T5 had cowpeas during SR2018 and beans in SR2019. In the LR, the observed DM maize grain yields for all the treatments were 1.8–5.5 tons/ha higher than the 1.5 tons/ha average yield reported on some Ugandan farms that neither employ hired labor nor apply organic manure and mineral fertilizers (Simtowe et al., 2019). This could be explained by the multiple pre-crop effects on soil characteristics and nutrient supply for treatments T2-T5, and the crop residues and DAP fertilizer that were applied under T1. For instance, the higher maize yield of 4 tons/ha in T2 during LR2018 than the 1.5 tons/ha average Ugandan farmers' yield could be related to the N2 fixed and other nutrients added to the soil by cowpea biomass accumulated during SR2018. These results are in agreement with Vesterager et al. (2007) in a Tanzanian farm where maize yield doubled in a field when maize followed a cowpea pre-crop compared to a maize mono-cropping system.
A similar effect in DM grain and residue yields during LR among all the five treatments is not surprising. It could be attributed to the improved soil OM and availability of N and K that resulted from the application of the OMM strategies for T2-T5 and the inorganic fertilizer DAP and maize residues for T1. This similar effect is an indicator that the cereal-legume rotation system and crop residue incorporation for treatments with OMM strategies can result in yields that are similar to the ones in T1 with 50 kg/ha DAP fertilizer and maize-crop residue incorporation.
The higher maize grain and residue yield in LR2019 than in LR2018 could be explained by several factors. First, the better rainfall distribution in LR2019 than in LR2018 could have supported crop growth hence higher yields in LR2019. In addition, the lower maize yield in LR2018 than in LR2019 might be an effect of the leaching processes that resulted from the higher rainfall in LR2018 (plus 78.2 mm) in a period where the soil was still not fully covered by organic matter. Secondly, the higher DM yields in the LR2019 could also be attributed to the decomposition of plant residues as reported by Bu et al. (2020) in a study where soil organic carbon, organic matter, and macro aggregates increased after the decomposition of the crop residues. Moreover, the plant residues in the OMM strategies contained up to 6620, 411, and 3333 kg/ha of total N, P and K respectively while those under the fertilizer application strategy contained 243, 23 and 119 kg/ha of total N, P and K respectively (Ekyaligonza et al., 2022). For T2-T5, the higher maize yield during LR2019 than in LR2018 could have occurred as feedback on the incorporated crop residues of the bean pre-crop in SR2019 and its N2 fixation (see also Nassary et al., 2020). Thirdly, the organic matter accumulation by the OMM treatments could have improved soil nutrients and crop growth hence higher maize yields. Moreover, OMM strategies such as FYM application can improve soil nutrients that would favor crop growth (Motsi et al., 2019). In addition, FYM application improves soil organic carbon, which in turn influences the soil's physical properties responsible for improving crop growth (Xiao et al., 2022). The impact of FYM on yield was also observed in trials by Shibabaw et al. (2018) where FYM was applied in the field at a rate of 5 tons/ha, and Kumar et al. (2011), where it was applied at a rate of 12 tons/ha. Fourthly, the higher crop yield under the treatments with F. albida could be explained by nitrogen fixation as F. albida trees are known to fix about 39 kg N/ha/year (Toure et al., 1998; Umar et al., 2013), but the amount of nitrogen fixed is expected to increase as the trees grow. F. albida is also known for improving soil organic carbon (SOC), total N, available P, and total K (Birhane et al., 2018; Yengwe et al., 2018), but also for other nutrients accumulated in the branches. The maize yield in treatments with F. albida alleys was however higher than the amounts observed in Yengwe et al. (2018) where maize yield in fields with 8, 12, and 22-year F. albida trees with DM litter-fall of 0.9–3.9 ha−1 from 2014–2016 was analyzed. The yield difference between what is observed in the current study and other studies could be attributed to the additional role of other OMM strategies such as crop rotation, integration of M. pruriens, and crop residue incorporation that were applied into the field. Crop yields are expected to increase when F. albida alleys attain an age of about 20 years (Dilla et al., 2019). Fifth, M. pruriens which was intercropped with maize in treatments T3-T5 could have also contributed to nitrogen fixation, which in other trials in Africa is estimated to fix up to 177 kg N/ha, as well as increasing the SOC, and other minerals in plant-available forms (Houngnandan et al., 2000; Imoro et al., 2013). Under climate conditions similar to those of the study area, the decomposition of M. pruriens can be attained within 6–7 weeks (Saria et al., 2018). An increase in DM maize grain yield in LR2019 for the treatments with OMM strategies is an indicator that the strategies can improve both food and income security.
For the DM residue yield, its increase under the OMM treatments during the LR2019 shows that the applied cropping systems have the potential to increase biomass, which when incorporated into the soil can recycle the nutrients hence improving the soil organic matter. In the subsequent years, it can be expected that the OMM strategies' additional biomass, nitrogen, and higher availability of nutrients can lead to higher soil fertility with positive impacts on crop yields than what we observed in LR2018 and LR019.
Costs of establishment and maintenance of OMM strategies
The application of OMM strategies is expected to improve crop yield with lower costs incurred than the inorganic fertilizer application strategy. Our results show that T2 involved significantly lower accumulated variable costs than all the treatments during the entire four-season rotation. This could be explained by the lowest labor and seedling costs incurred during the establishment and maintenance of the cropping system in comparison to other treatments. Indeed, T2 only involved the application of simple OMM strategies; majorly maize-legume rotation and crop residue incorporation without incurring any cost of purchasing DAP, as well as preparing and applying FYM.
During SR, the total variable costs incurred were higher under T3 and T5 than all other treatments, and this could be attributed to the extra labor costs of 57.15 and 54.05 US$/ha that were incurred for each of the two treatments during SR2018 and SR2019, respectively. These extra costs resulted from dung collection from the livestock farm and the preparation and application of FYM in the field. These extra labor costs can however enable farmers to generate additional income on their farms. Conversely, the higher total variable costs under T1 than the treatments T2-T5 with OMM strategies during LR2018 could be explained by the cost incurred in purchasing DAP fertilizer and the labor costs for applying it in the field. This study shows that the costs involved in the application of OMM treatments begin to reduce by the second season when the same crop is grown.
Revenues and profits generated through establishment and maintenance of OMM strategies
During the SR2018, the higher revenues and gross margins obtained in T1 than in the treatments T2-T5 with OMM strategies could be explained by the different crops planted in the different treatments since T1 involved maize monocrop while treatments T2-T5 involved cowpeas. The maize and cowpeas differed in yield and price, hence affecting the grain revenues and gross margins. The lower revenues and gross margins under T1 than in treatments with OMM strategies in SR2019 could be explained by the lower selling price of maize (0.17 $/kg) that was planted under T1 than the beans (0.86 $/kg) planted under T2-T5.
Obtaining a similar effect in terms of grain revenues and gross margins among treatments T1, T2, T4 and T5 during the LR where maize was a common crop for all treatments could be explained by the impact of the different soil amendment strategies. Moreover, the yields obtained were not significantly different among the same treatments in the LR. From the gross margin results obtained during the LR and the total gross margins after the entire four-season rotation, this study suggests that the OMM strategies, while contributing more to soil fertility, as shown in several studies (Lupwayi et al., 2011; Akmal et al., 2015; Birhane et al., 2018; Beuschel et al., 2019; Bu et al., 2020), are as profitable as the inorganic fertilizer application strategy.
The price sensitivity analysis showed that all the crops in this study are affected by price fluctuation, which would in turn affect gross margins. Based on the range of gross margins in SR2018 and LR2018, the results indicate that T1 has a higher sensitivity to price fluctuation while T4 has the least in comparison to other treatments. This could be explained by the higher maize price fluctuation in T1 than in cowpeas that were planted in T2-T5. In SR2019, T2 showed the highest sensitivity to price fluctuation while T1 showed the least in comparison to other treatments. This could be explained by the higher bean price fluctuation compared to the maize. In the LR2019, T5 showed the highest sensitivity to price fluctuation while T3 showed the least in comparison to other treatments. The total gross margins of the entire rotation system however indicate that T1 has the highest sensitivity to price fluctuation while T3 has the least, in comparison to other treatments. Based on the gross margin trends per season and the total gross margins for the entire rotation system, it can be seen that T1 is the riskiest strategy while T3 and T4 are the least risky strategies in comparison to T2 and T5. The lower sensitivity levels of gross margins under T3 and T4 than the maize monocropping system in T1 could be explained by the wide maize price fluctuations in comparison to the diversified rotation systems in T3 and T4. This implies that T3 and T4 would have a higher adoption potential by the smallholder farmers than T1, T2, and T5.
Conclusion
During this two-year study, the OMM strategies attained similar maize crop yield as the mono-cropping system with DAP and maize residue application. Future effects of OMM strategies on soil productivity can be expected on this sandy, low-pH soil with a low buffer capacity. In addition, the revenues and gross margins from the maize yields under the treatments with OMM strategies were similar to the mono-cropping / inorganic fertilizer application approach. Moreover, the total variable costs, inclusive of monetarized family labor were higher in the treatment with inorganic fertilizer application than in the treatments with OMM strategies during LR2018 and they were not significantly different from the OMM strategies of T3-T5 during LR2019. In addition, the application of OMM strategies proved to be as profitable as the inorganic fertilizer application strategy. The continuation of OMM is expected to increase soil fertility indicators and consequently crop yield and farm economy. The price sensitivity analysis however showed that the gross margins under T3, which involved FYM application, maize-M. pruriens intercrop and a legume-maize rotation; and T4, which involved F. albida alleys, maize-M. pruriens intercrop and a legume-maize rotation are less sensitive to price fluctuation. Due to this advantage, T3 and T4 have a high adoption potential by smallholder farmers. Moreover, smallholder farmers can access the planting materials for T3 and T4.
The results of this study were however based on only four crop-growing seasons. Both cropping systems are at risk of soil-borne diseases and need further development. Future studies should be conducted for at least eight seasons (four years), involving at least four food crop families or different cereals to ascertain whether the OMM strategies will increase the yields and profits in subsequent crop-growing seasons, as well as analyze the effects on soil fertility indicators. Another study should consider varying FYM and nutrient levels to determine whether the results will be different from what we observed. The soil micronutrient levels should also be determined in future studies. Since phosphorus was low in all treatments before and after experimentation, future studies should also consider the inclusion of an input with liming effect for the inorganic fertilizer application treatment and the plant materials that can increase phosphorus in the OMM treatments.
Data availability statement
The original contributions presented in the study are included in the article/supplementary material, further inquiries can be directed to the corresponding author.
Author contributions
DE conceptualized the idea, established the field trials, conducted data collection, and conducted the statistical analysis. DE and ME conducted the economic analysis. DE and TT wrote the first draft. JK, JF, and BF supervised the research. DE, TT, PD, JK, ME, JF, and BF reviewed and edited the manuscript. All authors contributed to the article and approved the submitted version.
Funding
This research was funded by the Austrian Partnership Programme in Higher Education and Research for Development (APPEAR), a programme of the Austrian Development Cooperation (ADC) that is implemented by the Austria's Agency for Education and Internationalization (OeAD-GmbH).
Acknowledgments
The authors acknowledge Professor Erwin Lautsch for guiding data analysis and Akoraebirungi Bendicto for assisting in data collection. The farmers of Nyabbani Sub County are acknowledged for availing their land for the experiment and fully participating in the experiment.
Conflict of interest
The authors declare that the research was conducted in the absence of any commercial or financial relationships that could be construed as a potential conflict of interest.
Publisher's note
All claims expressed in this article are solely those of the authors and do not necessarily represent those of their affiliated organizations, or those of the publisher, the editors and the reviewers. Any product that may be evaluated in this article, or claim that may be made by its manufacturer, is not guaranteed or endorsed by the publisher.
References
Agona, A., Nabawanuka, J., and Muyinza, H. (2001). An overview of maize in Uganda. Post-harvest Programme, NARO Uganda. Available online at: http://citeseerx.ist.psu.edu/viewdoc/download?doi=10.1.1.580.5899andrep=rep1andtype=pdf (accessed on March 2, 2022).
Akmal, M., Shah, A., Zaman, R., Afzal, M., and Ul Amin, N. (2015). Carryover response of tillage depth, legume residue and nitrogen-rates on maize yield and yield contributing traits. Int. J. Agric. Biol. 17, 961–968. doi: 10.17957/IJAB/15.0010
Aseete, P., Katungi, E., Bonabana-Wabbi, J., Birachi, E., and Ugen, M. A. (2018). Consumer demand heterogeneity and valuation of value-added pulse products: a case of precooked beans in Uganda. Agric. Food Secur. 7, 51. doi: 10.1186/s40066-018-0203-3
Bamwesigye, D., Doli, A., Adamu, K. J., and Mansaray, S. K. (2020). A review of the political economy of agriculture in Uganda: Women, property rights, and other challenges. Univ. J. Agric. Res. 8, 1–10. doi: 10.13189/ujar.2020.080101
Beuchelt, T. D., and Zeller, M. (2011). Profits and poverty: certification's troubled link for Nicaragua's organic and fairtrade coffee producers. Ecol. Econ. 70, 1316–1324. doi: 10.1016/j.ecolecon.2011.01.005
Beuschel, R., Piepho, H. P., Joergensen, R. G., and Wachendorf, C. (2019). Similar spatial patterns of soil quality indicators in three poplar-based silvo-arable alley cropping systems in Germany. Biol. Fertil. Soils 55, 1–14. doi: 10.1007/s00374-018-1324-3
Birhane, E., Gebremeskel, K., Taddesse, T., Hailemariam, M., Hadgu, K. M., Norgrove, L., et al. (2018). Integrating Faidherbia albida trees into a sorghum field reduces striga infestation and improves mycorrhiza spore density and colonization. Agrofor. Syst. 92, 643–653. doi: 10.1007/s10457-016-0027-8
Bu, R., Ren, T., Lei, M., Liu, B., Li, X., Cong, R., et al. (2020). Tillage and straw-returning practices effect on soil dissolved organic matter, aggregate fraction and bacteria community under rice-rice-rapeseed rotation system. Agric. Ecosyst. Environ. 287, 106681. doi: 10.1016/j.agee.2019.106681
Cai, A., Xu, M., Wang, B., Zhang, W., Liang, G., Hou, E., et al. (2019). Manure acts as a better fertilizer for increasing crop yields than synthetic fertilizer does by improving soil fertility. Soil Tillage Res. 189, 168–175. doi: 10.1016/j.still.2018.12.022
Chakoma, I., Manyawu, G. J., Gwiriri, L., Moyo, S., and Dube, S. (2016). The Agronomy and Use of Mucuna Pruriens in Smallholder Farming Systems in Southern Africa. ILRI extension brief. Available online at: https://cgspace.cgiar.org/bitstream/handle/10568/78515/extension_brief_mucuna.pdf?sequence=1 (accessed October 8, 2022).
Dahlin, A. S., and Rusinamhodzi, L. (2019). Yield and labor relations of sustainable intensification options for smallholder farmers in sub-Saharan Africa. A meta-analysis. Agron. Sustain. Dev. 39, 1–18. doi: 10.1007/s13593-019-0575-1
Ddungu, S., Ekere, W., Bisikwa, J., Kawooya, R., Kalule, D. O., and Biruma, M. (2015). Marketing and market integration of cowpea (Vigna unguiculata L. Walp) in Uganda. J. Dev. Agric. Econ. 7, 1–11. doi: 10.5897/JDAE2014.0577
De Moura, E. G., Serpa, S. S., Dos Santos, J. G. D., Sobrinho, J. R. S. C., and Aguiar, A. D. C. F. (2010). Nutrient use efficiency in alley cropping systems in the Amazonian periphery. Plant Soil 335, 363–371. doi: 10.1007/s11104-010-0424-0
Dilla, A. M., Smethurst, P. J., Barry, K., Parsons, D., and Denboba, M. A. (2019). Tree pruning, zone and fertiliser interactions determine maize productivity in the Faidherbia albida (Delile) A. Chev parkland agroforestry system of Ethiopia. Agrof. Syst. 93, 1897–1907. doi: 10.1007/s10457-018-0304-9
Ekyaligonza, D. M., Kahigwa, T. T., Dietrich, P., Akoraebirungi, B., Kagorora, J. P., Friedel, J. K., et al. (2022). Biomass contribution and nutrient recycling of organic matter management practices in tropical smallholder annual farming systems. Acta Agric. Scand. 72, 945–956. doi: 10.1080/09064710.2022.2134819
FAO (2005). New_LocClim; Local Climate Estimator. Available online at: http://www.fao.org/nr/climpag/pub/en3_051002_en.asp (Accessed July 22, 2020).
FAO (2012). Harmonised World Soil Database Viewer (Version 1.2). FAO, IIASA, ISRIC and ISS-CAS, JRC FAO. Rome, Italy and IIASA, Laxenburg, Austria. Available online at: https://harmonized-world-soil-database.software.informer.com/1.2 (accessed March 20, 2019).
FAO (2017). Drought-tolerant maize varieties in Uganda. Available online at: http://www.fao.org/3/CA2545EN/ca2545en.pdf (accessed on July 22, 2020).
Flores-Sánchez, D., Groot, J. C., Lantinga, E. A., Kropff, M. J., and Rossing, W. A. (2015). Options to improve family income, labor input and soil organic matter balances by soil management and maize–livestock interactions. Exploration of farm-specific options for a region in Southwest Mexico. Renew. Agric. Food Syst. 30, 373–391. doi: 10.1017/S1742170514000106
Hendershot, W. H., Lalande, H., and Duquette, M. (1993). Soil reaction and exchangeable acidity. In: Soil Sampling and Methods of Analysis. Soon, Y. K., and Hendershot, W. H., eds. Taylor and Francis Group, LLC. Available online at: https://books.google.com/books?hl=en&lr=&id=54lYLsV49zIC&oi=fnd&pg=PA141&dq=Hendershot,+W.+H.,+Lalande,+H.,+and+Duquette,+M.+(1993).+Soil+reaction+and+exchangeable+acidity.+In:+Soil+Sampling+and+Methods+of+Analysis.+Soon,+Y.+K.+and+Hendershot,+W.+H.,+eds.+Taylor+and+Francis+Group,+LLC.&ots=K4kN-JJ5DR&sig=rp64UHFSyKCZevEC1rC9iCU0Lt4 (accessed May 28, 2019).
Houngnandan, P., Sanginga, N., Woomer, P., Vanlauwe, B., and Van Cleemput, O. (2000). Response of Mucuna pruriens to symbiotic nitrogen fixation by rhizobia following inoculation in farmers' fields in the derived savanna of Benin. Biol. Fertil. Soils 30, 558–565. doi: 10.1007/s003740050036
Imoro, A. Z., Gakpo, P. S., and Aikins, T. K. (2013). Assessing soil amendment potentials of Mucuna pruriens and Crotalaria juncea when used as fallow crops. J. Soil Sci. Environ. Manag. 4, 28–33. doi: 10.5897/JSSEM12.064
Kankwatsa, P. (2018). Efficacy and cost-benefit analysis of indigenous technical knowledge versus recommended integrated pest and disease management technologies on common beans in South Western Uganda. Open Access Lib. J. 5, 1. doi: 10.4236/oalib.1104589
Kohler, J. (2020). Assessment of Fertilizer Distribution Systems and Opportunities for Developing Fertilizer Blends. Available online at: https://scholar.google.com/scholar?hl=enandas_sdt=0%2C5andq=Assessment+of+Fertilizer+Distribution+Systems+and++Opportunities+for+Developing+Fertilizer+Blends+UGANDAandbtnG= (accessed August 10, 2020).
Kovar, J. L., and Pierzynski, G. M. (2009). Methods of phosphorus analysis for soils, sediments, residuals, and waters second edition. South. Coop. Ser. Bull. 408. Available online at: https://www.researchgate.net/profile/Lucas-Braos/post/How_to_determine_phosphorus_in_lake_water/attachment/59d6591979197b80779aebd5/AS%3A541032828096514%401506003686846/download/P_Methods2ndEdition2009.pdf (accessed April 05, 2022).
Kumar, B., Verma, S. K., and Singh, H. (2011). Effect of temperature on seed germination parameters in Kalmegh (Andrographis paniculata Wall. ex Nees.). Ind. Crops Prod. 34, 1241–1244. doi: 10.1016/j.indcrop.2011.04.008
Lhamo, D., and Luan, S. (2021). Potential networks of nitrogen-phosphorus-potassium channels and transporters in arabidopsis roots at a single cell resolution. Front. Plant Sci. 12, 1189. doi: 10.3389/fpls.2021.689545
Lupwayi, N. Z., Kennedy, A. C., and Chirwa, R. M. (2011). Grain legume impacts on soil biological processes in sub-Saharan Africa. Afr. J. Plant Sci. 5, 1–7. Available online at: https://academicjournals.org/journal/AJPS/article-full-text-pdf/B9689532400 (accessed February 04, 2020).
MAAIF (2019). Maize Training Manual for Extension workers in Uganda. Ministry of Agriculture Animal Industry and Fisheries. Available online at: https://www.agriculture.go.ug/wp-content/uploads/2019/09/Maize-training-manual-for-extension-workers-in-Uganda.pdf (accessed October 16, 2022).
Massah, J., and Azadegan, B. (2016). Effect of chemical fertilizers on soil compaction and degradation. Agric. Mech. Asia Afr. Latin Am. 47, 44–50. Available online at: https://www.researchgate.net/profile/Jafar-Massah/publication/303568416_Effect_of_Chemical_Fertilizers_on_Soil_Compaction_and_Degradation/links/57e7fb7808aed7fe466bcc1d/Effect-of-Chemical-Fertilizers-on-Soil-Compaction-and-Degradation.pdf (accessed November 22, 2021).
Mikha, M. M., Benjamin, J. G., Vigil, M. F., and Poss, D. J. (2017). Manure and tillage use in remediation of eroded land and impacts on soil chemical properties. PLoS ONE 12, e0175533. doi: 10.1371/journal.pone.0175533
Molenberghs, G., and Verbeke, G. (2001). A review on linear mixed models for longitudinal data, possibly subject to dropout. Stat. Model. 1, 235–269. doi: 10.1177/1471082X0100100402
Motsi, T., Kugedera, A., and Kokerai, L. (2019). Role of cattle manure and inorganic fertilizers in improving maize productivity in semi-arid areas of Zimbabwe. Oct. Jour. Env. Res 7, 122–129. Available online at: https://www.researchgate.net/profile/Andrew-Tapiwa-Kugedera/publication/336603476_International_Peer-Reviewed_Journal_ISSN_2321_3655_Oct/links/5da8077192851caa1baa6f6c/International-Peer-Reviewed-Journal-ISSN-2321-3655-Oct.pdf (accessed July 02, 2022).
Mugendi, D., Nair, P., Mugwe, J., O'neill, M., and Woomer, P. (1999). Alley cropping of maize with calliandra and leucaena in the subhumid highlands of Kenya. Part 2. Biomass decomposition, N mineralization, and N uptake by maize. Agrof. Syst. 46, 51–64. doi: 10.1023/A:1006269217882
Nassary, E. K., Baijukya, F., and Ndakidemi, P. A. (2020). Intensification of common bean and maize production through rotations to improve food security for smallholder farmers. J. Agric. Food Res. 2, 100040. doi: 10.1016/j.jafr.2020.100040
Neina, D. (2019). The role of soil pH in plant nutrition and soil remediation. Appl. Environ. Soil Sci. 2019:9. doi: 10.1155/2019/5794869
Njeru, E., Grey, S., and Kilawe, E. (2016). Eastern Africa climate-smart agriculture scoping study: Ethiopia, Kenya and Uganda. Food Agriculture Organization of the United Nations, Addis Ababa. Available online at: http://www.fao.org/3/a-i5485e.pdf (accessed June 10, 2019).
Nkonya, E., Kaizzi, C., and Pender, J. (2005). Determinants of nutrient balances in a maize farming system in eastern Uganda. Agric. Syst. 85, 155–182. doi: 10.1016/j.agsy.2004.04.004
Nziguheba, G., Zingore, S., Kihara, J., Merckx, R., Njoroge, S., Otinga, A., et al. (2016). Phosphorus in smallholder farming systems of sub-Saharan Africa: implications for agricultural intensification. Nutr. Cycling Agroecosyst. 104, 321–340. doi: 10.1007/s10705-015-9729-y
Odhiambo, J. J., and Mag, V. N. (2008). An assessment of the use of mineral and organic fertilizers by smallholder farmers in Vhembe district, Limpopo province, South Africa. Afr. J. Agric. Res. 3, 357–362. Available online at: https://academicjournals.org/journal/AJAR/article-full-text-pdf/02AC30936202 (accessed February 14, 2022).
Okalebo, J. R., Gathua, K. W., and Woomer, P. L. (2002). Laboratory Methods of Soil and Plant Analysis: A Working Manual Second Edition. Nairobi, Kenya: TSBF-CIAT and SACRED Africa.
Okoboi, G., Muwanga, J., and Mwebaze, T. (2012). Use of improved inputs and its effect on maize yield and profit in Uganda. Afr. J. Food Agric. Nutr. Dev. 12. doi: 10.18697/ajfand.55.10505
Omae, H., Saidou, A., and Tobita, S. (2014). Improving millet-cowpea productivity and soil fertility with crop rotation, row arrangement and cowpea density in the Sahel, West Africa. J. Agric. Environ. Sci 14, 110–115. doi: 10.5829/idosi.aejaes.2014.14.02.12307
Pawar, D. R., Randhe, D. B., Shaikh, M., Korde, A. K., Shinde, K. A., Yadav, M. P., et al. (2009). Laboratory Testing Procedure for Soil and Water Sample Analysis. Water Resources Department Directorate of Irrigation Research and Development, Pune. Government of Maharashtra. Available online at: https://www.google.com/url?sa=t&rct=j&q=&esrc=s&source=web&cd=&ved=2ahUKEwji0pPSjK37AhVNUMAKHdiYBMUQFnoECAsQAQ&url=https%3A%2F%2Fwww.researchgate.net%2Fprofile%2FArvind_Singh56%2Fpost%2FHow_we_can_determine_the_N_P_K_Ca_Na_and_Mg_content_of_soil_sample_for_Pisum_species_growing_areas%2Fattachment%2F59d641dd79197b807799dbed%2FAS%253A436663110377472%25401481120006055%2Fdownload%2F2.pdf&usg=AOvVaw1Lj9oCHb5CX4JudP4QEAZt (accessed May 29, 2018).
Pirhofer-Walzl, K., Rasmussen, J., Høgh-Jensen, H., Eriksen, J., Søegaard, K., and Rasmussen, J. (2012). Nitrogen transfer from forage legumes to nine neighbouring plants in a multi-species grassland. Plant Soil 350, 71–84. doi: 10.1007/s11104-011-0882-z
Ronner, E., and Giller, K. E. (2013). Background Information on Agronomy, Farming Systems and Ongoing Projects on Grain Legumes in Uganda. Milestone reference number: S 1.2.3. Available online at: https://docplayer.net/36009472-Background-information-on-agronomy-farming-systems-and-ongoing-projects-on-grain-legumes-in-uganda-milestone-reference-number-s-1-2.html (accessed June 10, 2019).
Roupsard, O., Ferhi, A., Granier, A., Pallo, F., Depommier, D., Mallet, B., et al. (1999). Reverse phenology and dry-season water uptake by Faidherbia albida (Del.) A. Chev. in an agroforestry parkland of Sudanese west Africa. Funct. Ecol. 13, 460–472. doi: 10.1046/j.1365-2435.1999.00345.x
Sahrawat, K. (1982). Evaluation of some chemical indexes for predicting mineralizable nitrogen in tropical rice soils. Commun. Soil Sci. Plant Anal. 13, 363–377. doi: 10.1080/00103628209367275
Sánchez-Navarro, V., Zornoza, R., Faz, Á., and Fernández, J. A. (2019). Comparing legumes for use in multiple cropping to enhance soil organic carbon, soil fertility, aggregates stability and vegetables yields under semi-arid conditions. Sci. Hortic. 246, 835–841. doi: 10.1016/j.scienta.2018.11.065
Saria, A., Sibuga, K., Semu, E., and Jensen, H. H. (2018). Soil Fertility Dynamics of Ultisol as influenced by greengram and mucuna green manures. J. Plant Sci. Agric. Res. 2:14. Available online at: https://www.google.com/url?sa=t&rct=j&q=&esrc=s&source=web&cd=&ved=2ahUKEwjH67eWnK37AhW1gP0HHXPUARoQFnoECAoQAQ&url=https%3A%2F%2Forbit.dtu.dk%2Ffiles%2F149220679%2FPaper_Manuscript_For_Plant_Sci_and_Agric_Res.pdf&usg=AOvVaw0OjIyjb6Pq05-HB8uKrITj (accessed April 11, 2020).
Sebaggala, R., and Matovu, F. (2020). Effects of Agricultural Extension Services on Farm Productivity in Uganda. African Economic Research Consortium, Nairobi. Research Paper 379.
Shibabaw, A., Alemayehu, G., Adgo, E., Asch, F., and Freyer, B. (2018). Effects of organic manure and crop rotation system on potato (Solanum tuberosum L.) tuber yield in the highlands of Awi Zone. EJST. 11, 1–18. doi: 10.4314/ejst.v11i1.1
Silva-Galicia, A., Álvarez-Espino, R., Sosa-Montes, E., and Ceccon, E. (2020). Fertilisation schemes based on organic amendments; decomposition and nutrient contribution of traditionally used species in an indigenous region of southern Mexico. Biol. Agric. Horticult. 37, 55–70. doi: 10.1080/01448765.2020.1837673
Simtowe, F., Amondo, E., Marenya, P., Sonder, K., and Erenstein, O. (2019). Impacts of drought-tolerant maize varieties on productivity, risk, and resource use: Evidence from Uganda. Land Use policy 88, 104091. doi: 10.1016/j.landusepol.2019.104091
Sunday, G., and Ocen, D. (2015). Fertilizer consumption and fertilizer use by crop in Uganda. Ministry of Agriculture, Animal. Available online at: https://africafertilizer.org/wp-content/uploads/2017/05/FUBC-Uganda-final-report-2015.pdf (accessed June 10, 2019).
Tadele, Z. (2017). Raising crop productivity in Africa through intensification. Agronomy 7, 22. doi: 10.3390/agronomy7010022
Tadesse, T., Dechassa, N., Bayu, W., and Gebeyehu, S. (2013). Effects of farmyard manure and inorganic fertilizer application on soil physico-chemical properties and nutrient balance in rain-fed lowland rice ecosystem. Am. J. Plant Sci. 4, 309–316. doi: 10.4236/ajps.2013.42041
Tie, T. B., Diby, L. N., Seyo, E., and Assa, A. (2013). Estimating soil available nitrogen with a hot H2O2/KCl extraction. Sci. Res. Essays 5, 1455–1462. Available online at: https://www.google.com/url?sa=t&rct=j&q=&esrc=s&source=web&cd=&cad=rja&uact=8&ved=2ahUKEwjnjMONna37AhXG3KQKHcESCwkQFnoECAgQAQ&url=https%3A%2F%2Fwww.researchgate.net%2Fpublication%2F264195507_Estimating_soil_available_nitrogen_with_a_hot_H_2_O_2_KCl_extraction&usg=AOvVaw3K-72Tw2Hp9R9hyRd_2qSv (accessed April 05, 2020).
Toure, O., Dasilva, M., Badji, S., Dianda, M., Ndoye, I., and Gueye, M. (1998). Nitrogen fixation improvement in Faidherbia albida. Available online at: https://worldwidescience.org/topicpages/t/tree+acacia+albida.html (accessed March 2, 2022).
UBOS (2019). Statistical abstract. Uganda Bureau of Statistics. Available online at: https://www.ubos.org/wp-content/uploads/publications/01_20202019_Statistical_Abstract_-Final.pdf (accessed July 15, 2022).
Uddin, M., Dhar, A., and Islam, M. (2016). Adoption of conservation agriculture practice in Bangladesh: Impact on crop profitability and productivity. J. Bangladesh Agric. Univ. 14, 101–112. doi: 10.3329/jbau.v14i1.30604
Umar, B. B., Aune, J. B., and Lungu, O. I. (2013). Effects of Faidherbia albida on the fertility of soil in smallholder conservation agriculture systems in eastern and southern Zambia. Afr. J. Agric. Res. 8, 173–183. doi: 10.5897/AJAR11.2464
Vanlauwe, B., Hungria, M., Kanampiu, F., and Giller, K. (2019). The role of legumes in the sustainable intensification of African smallholder agriculture: lessons learnt and challenges for the future. Agric. Ecosyst. Environ. 284, 106583. doi: 10.1016/j.agee.2019.106583
Vesterager, J. M., Nielsen, N. E., and Hoslash, H. (2007). Nitrogen budgets in crop sequences with or without phosphorus-fertilised cowpea in the maize-based cropping systems of semi-arid eastern Africa. Afr. J. Agric. Res. 2, 261–268.
Woniala, J., and Nyombi, K. (2014). Soil fertility management by smallholder farmers and the impact on soil chemical properties in Sironko district, Uganda. Res. J. Agric. For. Sci. 2, 5–10. Available online at: https://www.researchgate.net/profile/Kenneth-Nyombi/publication/264540943_Soil_fertility_management_by_smallholder_farmers_and_the_impact_on_soil_chemical_properties_in_Sironko_district_Uganda/links/53e364880cf23a7ff7494637/Soil-fertility-management-by-smallholder-farmers-and-the-impact-on-soil-chemical-properties-in-Sironko-district-Uganda.pdf (accessed August 22, 2020).
Xiao, Y., Zhou, M., Li, Y., Zhang, X., Wang, G., Jin, J., et al. (2022). Crop residue return rather than organic manure increases soil aggregate stability under corn–soybean rotation in surface mollisols. Agriculture 12, 265. doi: 10.3390/agriculture12020265
Yang, S., Wang, Y., Liu, R., Xing, L., and Yang, Z. (2018). Improved crop yield and reduced nitrate nitrogen leaching with straw return in a rice-wheat rotation of ningxia irrigation district. Sci. Rep. 8, 1–8. doi: 10.1038/s41598-018-27776-5
Keywords: alley cropping, annualized capital costs, Faidherbia albida, farmyard manure, gross margins, labor costs, Mucuna pruriens, organic matter management
Citation: Ekyaligonza DM, Tibasiima TK, Dietrich P, Kagorora JP, Friedel JK, Eder M and Freyer B (2022) Short-term trade-offs of organic matter management strategies for smallholder farms. Front. Sustain. Food Syst. 6:1035822. doi: 10.3389/fsufs.2022.1035822
Received: 03 September 2022; Accepted: 07 November 2022;
Published: 24 November 2022.
Edited by:
Cosmas Parwada, Zimbabwe Open University, ZimbabweReviewed by:
Ruth Wilhem Mukhongo, Makerere University, UgandaPatrice Autfray, Institut National de la Recherche Agronomique (INRA), France
Copyright © 2022 Ekyaligonza, Tibasiima, Dietrich, Kagorora, Friedel, Eder and Freyer. This is an open-access article distributed under the terms of the Creative Commons Attribution License (CC BY). The use, distribution or reproduction in other forums is permitted, provided the original author(s) and the copyright owner(s) are credited and that the original publication in this journal is cited, in accordance with accepted academic practice. No use, distribution or reproduction is permitted which does not comply with these terms.
*Correspondence: Deous Mary Ekyaligonza, bWFyeS5reWFsaWdvbnphQG1tdS5hYy51Zw==