- Department of Sustainable Agriculture, Biodiversity and Ecosystem Management, Nelson Mandela African Institution of Science and Technology, Arusha, Tanzania
The worldwide increase in population continues to threaten the sustainability of agricultural systems since agricultural output must be optimized to meet the global rise in food demand. Sub-Saharan Africa (SSA) is among the regions with a fast-growing population but decreasing crop productivity. Pests and diseases, as well as inadequate nitrogen (N) levels in soils, are some of the biggest restrictions to agricultural production in SSA. N is one of the most important plant-limiting elements in agricultural soils, and its deficit is usually remedied by using nitrogenous fertilizers. However, indiscriminate use of these artificial N fertilizers has been linked to environmental pollution calling for alternative N fertilization mechanisms. Soybean (Glycine max) is one of the most important legumes in the world. Several species of rhizobia from the four genera, Bardyrhizobium, Rhizobium, Mesorhizobium, and Ensifer (formerly Sinorhizobium), are observed to effectively fix N with soybean as well as perform various plant-growth promoting (PGP) functions. The efficiency of the symbiosis differs with the type of rhizobia species, soybean cultivar, and biotic factors. Therefore, a complete understanding of the ecology of indigenous soybean-nodulating rhizobia concerning their genetic diversity and the environmental factors associated with their localization and dominance in the soil is important. This review aimed to understand the potential of indigenous soybean-nodulating rhizobia through a synthesis of the literature regarding their characterization using different approaches, genetic diversity, symbiotic effectiveness, as well as their functions in biological N fixation (BNF) and biocontrol of soybean soil-borne pathogens.
Introduction
Soybean (Glycine max) is among the world's important crops due to its high-quality plant-based protein and oil content (Hartman et al., 2011; Thilakarathna et al., 2019). Worldwide, the crop is grown on about 6% of the arable land (Hartman et al., 2011) and 50% of the legume growing areas (Thilakarathna and Raizada, 2017). Globally, the major soybean-producing countries include the United State of America (USA), Brazil, Argentina, China, and India (Lorito and Woo, 2015). In Africa, different countries, mostly in Sub-Saharan Africa (SSA), grow soybean (Santos, 2019) and the crop is among the most common legumes in SSA (Wilson, 2013; Vanlauwe et al., 2019).
Soybeans establish symbiotic relationships with rhizobia, which form nodules in their roots, leading to biological nitrogen fixation (BNF), or the conversion of atmospheric nitrogen (N2/N) into biologically active forms that can be used by plants. Rhizobia play a fundamental role in N supply to ecosystems through their ability to fix N in symbiosis with legumes and promote the growth of plants. Through effective symbiosis, annually, soybean can fix about 16.4 Tg of N from the atmosphere which is about 77% of the total amount of N fixed by legumes (Hartman et al., 2011).
Soybean is not a crop of origin in Africa, but the demand is increasing tremendously due to its nutritional value (Chianu et al., 2011; Chianu and Mairura, 2019). Despite the increased demand for the crop, the yield obtained by smallholder farmers is not promising as compared to the major soybean growing areas in the world (Santos, 2019). This needs alternative efforts to increase the productivity of crops while lowering the cost of production, which can be achieved through the use of biofertilizers made from effective rhizobia. Several key joint research projects, such as N2-Africa, Consultative Group on International Agricultural Research (CGIAR) (Vanlauwe et al., 2019), together with other related research projects on the legume-rhizobium symbiosis, production, and management strategies related to the application of BNF for smallholder farmers in underdeveloped countries, have made the encouraging progress over the previous decade. However, more effort is required for the isolation of effective indigenous rhizobia, commercialization of their biofertilizers, and creation of community awareness for increased and sustainable production of leguminous crops (Ezeaku et al., 2012; Simon et al., 2014; Dawson et al., 2019).
Although the deficiency of nutrients in the soil is reduced by the supplementation of synthetic fertilizers, the productivity of soil and crop yield in SSA are constrained by many factors (Vanlauwe et al., 2011), including poor soil fertility (Vanlauwe et al., 2011; Snapp et al., 2018), and pests and diseases (Tadele, 2017; Franke et al., 2018; Parveen et al., 2019). Low soil fertility in most farming systems is due to inefficient soil management after harvesting crops, which leads to low levels of essential mineral nutrients (Raimi et al., 2017). Soil infertility is also attributed to alkalinity, which covers almost 17% of the arable land in tropical Africa (Ugboh and Ulebor, 2011). N is one of the major plant-limiting nutrients in agricultural soils due to its gradual depletion from the soil due to continuous crop cultivation (Pasley et al., 2019). N deficiency in agricultural soils has increased the demand for chemical nitrogenous fertilizers (Simon et al., 2014). However, chemical fertilizers are known to cause environmental pollution by producing greenhouse gases in the atmosphere, soil, and water pollution through runoff and contaminating the underground water systems through leaching. According to Patel et al. (2017), nitrate pollution of surface waters results in eutrophication and the unsustainability of agricultural production systems. Cognizant of this, there are efforts worldwide to explore alternative methods of N fertilization of crops, especially through the exploitation of legume-rhizobia symbiotic interactions.
Inoculation with rhizobia has been shown to improve N2-fixation and grain yield in soybean (Rodríguez-Navarro et al., 2003; Fatima et al., 2006; Nyaguthii, 2017; Yuan et al., 2020). However, the effectiveness of newly introduced rhizobial strains is affected by the presence of well-adapted indigenous strains (Burdon et al., 1999; Sannazzaro et al., 2011; Vanlauwe et al., 2019), which may also be inefficient in forming symbiotic nodules with the host plant depending on their N2-fixing capabilities (Musiyiwa et al., 2005). Among the areas where the soybean crop has been introduced, others have shown poor nodulation reports, which may be due to the absence of effective and compatible N2-fixing rhizobial strains (Agoyi et al., 2017; Thilakarathna and Raizada, 2017). This calls for a complete understanding of the ecology, genetic diversity, and the environmental factors associated with the localization and dominance of indigenous soybean-nodulating rhizobia. Therefore, this review aims to discuss the potential of indigenous soybean-nodulating rhizobia by synthesizing the literature on their characterization using different approaches, as well as our current knowledge of their genetic diversity, symbiotic effectiveness, BNF and other plant-growth promoting (PGP) functions, and biocontrol of soybean soil-borne pathogens.
Characterization of Soybean-Nodulating Rhizobia
Morphological Characterization
Morphological characterization entails assessing the cultural properties of rhizobial isolates as essential preliminary tools for confirming and distinguishing them from one another. The colony features (macro-morphology), as well as the gram reaction and cell shape (micro-morphology), can be studied for morphological characterization (Singha et al., 2013; Tyagi et al., 2017; Qudratullah Oryakhil, 2020). Macro-morphological features include colony elevation, color, texture, optical density (Kober and Giongo, 2004; Kapembwa et al., 2016; Khansole, 2016; Nagalingam et al., 2020; Qudratullah Oryakhil, 2020), growth rate, production of mucous/exopolysaccharides, elasticity, and pH changes during growth (Ondieki et al., 2017). On the other hand, micro-morphological characterization involves the response to gram stain and the shapes of rhizobial cells (Naz and Bano, 2009). However, molecular approaches can be utilized directly to characterize rhizobia in small samples with narrow diversity, but the morphological characterization is essential in bigger samples and newly isolated rhizobia. Table 1 shows some morphological features of soybean nodulating rhizobia as described by different studies. The reviewed studies revealed variations in morphological features even for the same species obtained from different locations. Therefore, morphological classification of the newly isolated strains is important in assessing similarities or differences and finally using the information to confirm their identification and classification.
Biochemical Characterization
Biochemical characterization of rhizobia is conducted to test their biochemical features and activities (Singh et al., 2008; Tyagi et al., 2017; Qudratullah Oryakhil, 2020). The biochemical assays are conducted for the intended purpose. Some biochemical features of rhizobia tested for various purposes include the reaction of rhizobia to methylene blue and gentian violet treatments. The two dyes are known for their inhibitory effects on the growth of microorganisms, including rhizobia. The results, either positive (+) or negative (-), indicate bacterial growth or no growth, respectively (Wei et al., 2002; Singh et al., 2008). Other important biochemical tests that are conducted include the ability of rhizobia to utilize different carbohydrates as the sole source of energy for their activities. Some carbohydrate utilization assays include hydrolysis of starch, glucose, lactose, sucrose, and citrate hydrolyses (Singh et al., 2008; Khalid et al., 2020).
A gelatine test is conducted by inoculating the rhizobia in a gelatine medium. The degradation of the medium is an indication of the presence of a gelatinase enzyme in the rhizobia (Kumari et al., 2010; Tyagi et al., 2017; Qudratullah Oryakhil, 2020). Production of the gelatinase enzyme by rhizobia is among the features that promote nodulation and N2 fixation (Kumari et al., 2010; Qudratullah Oryakhil, 2020). Among the products of gelatine hydrolysis by the gelatinase, enzyme are amino acids and peptides. These are the results of reduced inorganic N or fixed N in nodules by rhizobia (Balan et al., 2012). The amino acids produced can also be quantified to determine the amount of N fixed, while peptides can be used as a determinant of interaction between rhizobia and the host plant (Clarke et al., 2014; Tegeder and Masclaux-Daubresse, 2018).
In addition, other biochemical assays conducted to study rhizobia include the urease test, for the determination of the ability of rhizobia to hydrolyze urea into ammonia and carbon dioxide using the urease enzyme (Toffanin et al., 2002). Some rhizobium isolates can grow in urea-supplemented media and change the color of phenol red (a pH indicator) from yellow to pink, indicating that they can secrete urease enzyme (Singh et al., 2011; Mahavidyalaya, 2013). Moreover, Leite et al. (2017) reported on the capability of Bradyrhizobium yuanmingense to secrete urease enzyme as an indication of urea hydrolyzation in NH3 and CO2 by the strain.
Acidity, alkalinity, and temperature tolerance tests are often conducted to test the adaptation of rhizobia to various edaphic factors (Azcon and Barea, 2010; Deora and Singhal, 2010; Gómez Padilla et al., 2016). Tests for tolerance to acidity and alkalinity are conducted by growing the rhizobia in yeast extract mannitol broth (YEMB) broth or agar medium which is supplemented with Bromothymol blue at different pH at 28°C for 7 days, recording the growth (Maria et al., 2001; Shamseldin and Werner, 2005). For tolerance to acidity, the rhizobia change the green color of the plate to yellow, but for alkaline, the color changes to blue (Shamseldin and Werner, 2005; Kapembwa et al., 2016). Alkaline-producing rhizobia are dominant in acidic soils (Oliveira et al., 2010), while those producing acids dominate alkaline soils (Abd-Alla, 1994; Adhikari et al., 2012; Yan et al., 2014). Soybean nodulating rhizobia such as Bradyrhizobium liaoningense and Sinorhizobium fredii have been observed to be capable of inhabiting saline-alkaline soils (Wang et al., 2019). Rhizobia use the production of acid or alkaline as an adaptive feature to balance the external environment with their cells. The rhizobia growth can reveal the tolerance of rhizobia to stress conditions when inoculated on YEMB agar medium and kept at lower temperatures of 12, 17, and 25°C (Berrada et al., 2012; Yuan et al., 2020), as well as higher temperatures, such as 37 and 40°C (Maria et al., 2001). Rhizobia having small heat shock proteins (sHSP) are observed to tolerate high temperatures (Nocker et al., 2001; Alexandre and Oliveira, 2013).
Furthermore, an antibiotic resistance test is often conducted to confirm the genus of rhizobia. For instance, Bradyrhizobium japonicum-YCK294 was observed to be resistant to Kanamycin (100 μg/ml), Gentamycin (250 μg/ml), 100 μg/ml of Streptomycin and Chloramphenicol, Nalidixic acid (50 μg/ml), Polymyxin B sulfate (50 μg/ml), and Trimethoprim (50 μg/ml) (Sharma et al., 2010). The ability of rhizobia to resist antibiotics increases the chances of their survival in the rhizosphere of plants as well as their ability to occupy a larger number of nodules in symbiotic association with legume plants (Naamala et al., 2016). The summary of antibiotic resistance status for different rhizobia species that have symbiotic associations with soybean is presented in Table 2. The biochemical characterization of new strains of isolated rhizobia is important as this will provide accurate biochemical information about the strains and assist in their correct and quick identification. Apart from the importance of biochemical characterization in the identification of soybean rhizobia, it is also worthwhile to conduct various biochemical characterizations for the newly isolated rhizobia species. Biochemical assays such as the growth of rhizobia under different temperature and pH ranges help in the economical and appropriate use of the inoculum based on the climate, soil type, and characteristics under either smallholder or intensive farming.
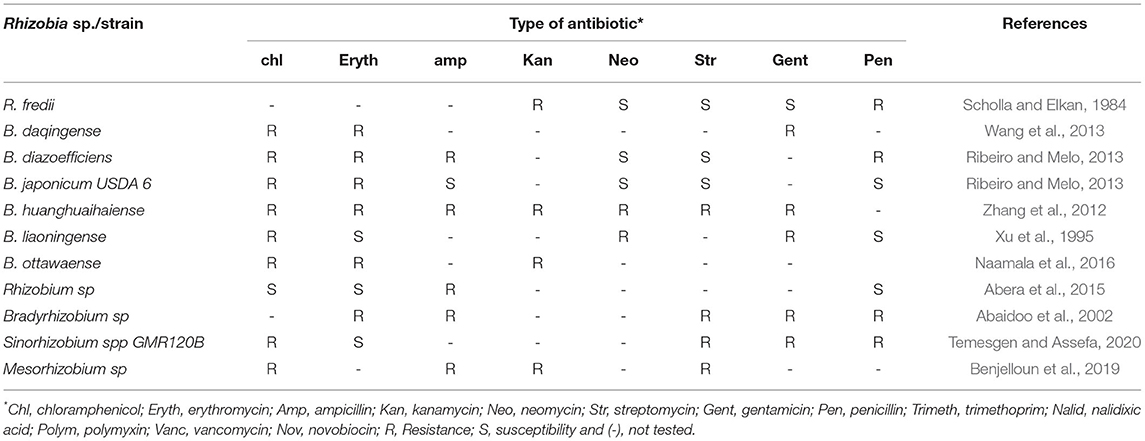
Table 2. Antibiotic resistance and susceptibility of different soybean nodulating rhizobia species isolated from different geographical locations.
Molecular Characterization
Many leguminous plant species interact symbiotically with different rhizobia species (Wei et al., 2002; Ojha et al., 2020). However, soybean is among the legumes that nodulate with a narrow diversity of rhizobia. Despite the narrow diversity of rhizobia which forms symbiotic nodules in soybean, the same rhizobia interact with many legumes making the classification a complicated process (Yang et al., 2010; Hayashi et al., 2014; Wang et al., 2018). This requires appropriate molecular methods for the taxonomic classification of diverse rhizobia (Khalid et al., 2020) as well as genes responsible for different functions (Lebrazi et al., 2018). Several molecular techniques used to characterize soybean nodulating rhizobia are discussed hereunder.
Sequencing of the genome and targeted DNA coding regions of interest have been used in classification and studying the diversity of soybean nodulating rhizobia. Sequencing of targeted DNA coding regions involves the use of specific or universal primers and PCR for amplification of the DNA fragment. Different specific or universal primers targeting certain regions or genes of interest in genomic DNA have been used for taxonomic or diversity characterization of indigenous soybean nodulating rhizobia. These techniques include sequencing of DNA coding regions such as 16S rRNA (Chibeba et al., 2017) or both 16S rRNA and 16S rDNA (Youseif et al., 2014; Gyogluu et al., 2018), as well as the 16S-23s rDNA internal transcribed spacer (ITS) region (Htwe et al., 2015). Other markers include enterobacterial repetitive intergenic consensus (ERIC-PCR) (Jain and Pundir, 2017), and repetitive extragenic palindromic (REP; Tajima et al., 2000) as well as rep-PCR (BOX) (Chibeba et al., 2017). The aforementioned taxonomic and diversity markers have been used together with other markers for genes with specific functions such as symbiotic genes, which are nodA and nifH (Youseif et al., 2014), as well as protein-coding housekeeping genes including dnaK, glnII, gryB, and recA (Chibeba et al., 2017).
Gyogluu et al. (2018) observed the high genetic diversity of 10 native soybeans nodulating Bradyrhizobium strains by characterizing their genomic fingerprinting using ERIC-PCR. Similarly, Tajima et al. (2000) successfully identified and classified 44 B. japonicum, 7 Sinorhizobium meliloti, and 10 Rhizobium leguminosarum in soybean from Japan and Thailand through ERIC-PCR, and REP-PCR, and their combination, ERIC-REP-PCR. Furthermore, ERIC-REP-PCR has been used in the classification of Bradyrhizobium in soybean (Chen et al., 2000; Muiru et al., 2010). Chibeba et al. (2017) used rep-PCR (BOX) and 16S rRNA gene sequencing which their phylogenetic study revealed remarkable genetic diversity among Bradyrhizobium and Agrobacterium/Rhizobium species in soybean. Moreover, Wang et al. (2014) identified the high genetic diversity of rhizobia in the rhizosphere of different soybean cultivars by using T-RFLP and 16S rDNA sequencing. Moreover, soybean nodulating rhizobia have been characterized for other functions through a single or combination of various molecular characterization methods. Youseif et al. (2014) successfully studied the genetic diversity of soybean-nodulating rhizobia in Egyptian soils by using rep-PCR fingerprinting and sequencing of symbiotic genes nifH and nodA and the DNA coding region 16S rDNA.
Other molecular methods have also been used in the characterization of protein-coding housekeeping genes in soybean nodulating rhizobia. Chibeba et al. (2017) used BOX_PCR to amplify the DNA coding region 16S rRNA and protein-coding housekeeping genes, which are dnaK, glnII, gyrB, and recA, to characterize indigenous Bradyrhizobium strains that were effective in fixing N in soybean. The results from 16S rRNA sequence analysis showed a high nucleotide identity of 97–100% among species, and further characterization of protein-coding genes supported the classification of other five species with those identified through 16S rRNA. This demonstrates the importance of using additional molecular methods in the classification and phylogenetic investigations of soybean nodulating rhizobia.
Genome and targeted DNA (PCR amplicon) sequencing have been used to uncover the microsymbionts of different leguminous plants (Wang et al., 2018). These techniques have been useful in the successful identification of different species of rhizobia from groups/genera which nodulate soybean (Yang et al., 2010; Tian et al., 2012; Wang et al., 2018). Genome sequencing has been used to identify the dominant species in certain biogeographical patterns including edaphic factors such as alkaline-saline soil which was dominated by the genus Sinorhizobium in China (Han et al., 2009). Based on the literature, there are various PCR-based procedures, which have been used in studying the taxonomy of soybean nodulating rhizobia for different gene functions. Table 3 summarizes the genetic diversity of soybean nodulating rhizobia studied in different geographical locations as grouped in their specific genera. Of all the methods, 16S rRNA and16S rDNA are the widely used coding regions for identification, because these are the highly conserved regions existing as multigene families or operons and present in almost all bacteria including rhizobia. Therefore, molecular characterization of newly isolated strains of rhizobia is important in the establishment of the genetic information and characteristics of the organisms.
Diversity of Soybean-Nodulating Rhizobia From Single Species B. japonicum to Multiple Species of Rhizobia
Rhizobia is made up of various species that are taxonomically classified as proteobacteria, specifically in the classes α-proteobacteria, which includes many species, and some in β-roteobacteria (Gyaneshwar et al., 2011). There are 17 genera of rhizobia which are categorized into different families (Figure 1). The families include Rhizobiaceae, Phyllobacteriaceae, Brucellaceae, Methylobacteriaceae, Bradyrhizobiaceae, Xanthobacteraceae, Hyphomicrobiaceae, and Burkholderiaceae. Members of the Rhizobiaceae include Rhizobium, Ensifer (formerly Sinorhizobium), Allorhizobium, Pararhizobium, Neorhizobium, and Shinella. Phyllobacteriaceae include Mesorhizobium, Aminobacter, and Phyllobacterium. Brucellaceae include Ochrobactrum. Methylobacteriaceae include Methylobacterium and Microvirga (Berrada, 2014; Lindstrom and Mousavi, 2020). Methylobacteriaceae include Methylobacterium and Microvirga (Berrada, 2014; Youseif et al., 2014; Jain and Pundir, 2017; Lindstrom and Mousavi, 2020). Bradyrhizobiaceae include Bradyrhizobium. Xanthobacteraceae include Azorhizobium. Hyphomicrobiaceae include Devosia. Finally, Burkholderiaceae include Paraburkholderia and Cupriavidus (Berrada, 2014; Jain and Pundir, 2017; Volpiano et al., 2019; Lindstrom and Mousavi, 2020). However, soybean forms symbiotic nodules with a narrow range of rhizobia species. For a long time, the single species B. japonicum was known to accommodate all the strains of rhizobia which nodulate and fix N with soybean. The advancement in DNA sequencing and phylogenetic analyses from different studies revealed that soybean nodulating rhizobia do not fall into the same species due to their heterogeneity. Through DNA homologies, the new species Bradyrhizobium elkanii (Hollis et al., 1981; Berrada, 2014) was developed, and hence the third species, B. liaoningense, was isolated in China from the soybean nodules. B. japonicum and Bradyrhiszobium elkanii are categorized as slow growers, but B. liaoningense is categorized as a very slow grower (Xu et al., 1995). After the classification of the above three strains, a novel species called Bradyrhizobium huanghuaihaiense was isolated in different sites in China (Zhang et al., 2012).
Due to advancements in phylogenetic studies, different soybean-nodulating rhizobia species were classified into other genera, which are Rhizobium, Mesorhizobium, and Ensifer, formerly known as Sinorhizobium. The genera Rhizobium and Ensifer (formerly Sinorhizobium), together with the genus Shinella, were considered as one branch that included PGPR (Chen et al., 1988; Lajudie et al., 1994). However, due to differences in cultural, biochemical, and molecular characteristics, the genus Rhizobium was separated from the genus Ensifer (Sinorhizobium). Apart from other strains, the soybean nodulating rhizobia such as R. leguminosarum (Martinez-romero et al., 1991) were observed to fall under the genus Rhizobium. Ensifer (Sinorhizobium) was then treated as the new genus of fast-growing rhizobia, including those which nodulate soybeans, such as S. fredii, S. meliloti, and S. xingianense (Scholla and Elkan, 1984; Lajudie et al., 1994). The fourth genus of soybean nodulating rhizobia with intermediate growth is Mesorhizobium, which was proposed in 1997 (Jarvis et al., 1997). Soybean nodulating rhizobia which were placed in this genus include M. loti (Jarvis et al., 1982) and M. tianshanense (Jarvis et al., 1997).
Through further studies conducted in different geographical locations, different species of soybean nodulating rhizobia have been identified under the four genera (Table 3). Bradyrhizobium japonicum (Jordan, 1982; Hungria et al., 2001; Musiyiwa et al., 2005; Youseif et al., 2014; Htwe et al., 2019), B. elkanii (Hungria et al., 2001; Youseif et al., 2014). Bradyrhizobium yuanmingense (Appunu et al., 2008), B. liaoningense (Xu et al., 1995), and R. leguminosarum (Schaechter, 2009) and later its biovars R. leguminosarum bv. Trifolii, R. leguminosarum bv. Viciae (Tian et al., 2012; Youseif et al., 2014) as well as R. leguminosarum bv. Phaseoli (Youseif et al., 2014) have been observed to nodulate and fix N effectively in soybean.
The diversity of soybean nodulating rhizobia is affected by host specificity and the narrow range of rhizobia species, which forms an effective symbiosis with soybean (Xu et al., 1995). Climatic conditions and soil properties (Suzuki et al., 2008; Han et al., 2009; Adhikari et al., 2012) and stress conditions such as acidity/alkalinity, salinity, and high temperature (Chibeba et al., 2017) are other factors that affect the effectiveness of soybean nodulating rhizobia. Different land uses and management of crops (Yan et al., 2014), geographical location (Shiro et al., 2013) as well, influence the diversity of soybean nodulating rhizobia. The literature reveals that the rhizobia species which nodulate effectively with soybean are taxonomically placed in four genera, or groups, including Bradyrhizobium, Rhizobium, Sinorhizobium, and Mesorhizobium, as summarized in Figure 1. Further research is required to explore soybean nodulating rhizobia in regions such as SSA, which currently have inadequate research on BNF studies for different leguminous crops, including soybean. This may be helpful in discovering new rhizobia species capable of forming effective nodules in soybean. Moreover, understanding rhizobial diversity and the associated environmental factors affecting their performance is of paramount importance as, collectively, they affect their distribution in the ecosystem.
Effectiveness of Indigenous Soybean Nodulating Rhizobia
Most leguminous plants have the capability of associating with symbiotic rhizobia and forming nodules for N fixation (Oldroyd et al., 2011; Mathu et al., 2012). Soybean exhibits a high degree of specificity for effective symbiosis with N fixers (Figure 2). Bradyrhizobium is the major symbiont leading to an effective symbiosis with soybean (Merrill et al., 2017; Thilakarathna and Raizada, 2017). Sinorhizobium fredii grows fast and has the ability to establish symbiosis for N2 fixation with soybeans and other legumes (Fan et al., 2017). Although S. fredii and other rhizobia can nodulate with a wide range of legumes (Fan et al., 2017), some strains nodulate selectively with a narrow range of legume genotypes or species (Wang et al., 2012).
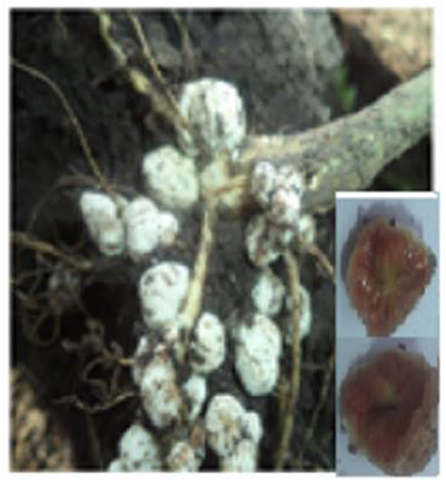
Figure 2. Symbiotic nodules in soybean adapted from Temesgen and Assefa (2020).
However, the type of soybean cultivar can affect the effectiveness of nodulation process and N fixation. Effective nodulation depends on plant recognition of Nod factors (NFs) by the host plant (Oldroyd et al., 2011) for the infection of plant roots and nodule formation (Radutoiu et al., 2003; Okazaki et al., 2013). The structural diversity of NFs produced by bacteria is the key determinant of specific symbiotic associations between legumes and rhizobia species (Radutoiu et al., 2007). Some studies have demonstrated the influence of soybean cultivars on the symbiotic effectiveness of the rhizobia species. Wang et al. (2014) observed the influence of soybean cultivars on the composition and diversity of rhizobia in the rhizosphere. The study conducted by Waswa et al. (2014) revealed that the inoculation of soybean seeds by local strain Bradyrhizobium sp increased the grain yield from 1.1 to 1.5 t ha−1 in promiscuous soybean SB19 and 1.3–1.4 t ha−1 in specific a soybean variety (Safari) over the commercial strain USDA110. The difference in yield increase demonstrates the influence of the soybean cultivars in effective nodulation and N fixation as revealed by the variation of seed yield between promiscuous and specific varieties of soybean.
On the other hand, indigenous rhizobia have been observed to be more effective in fixing N than the commercial inoculants which have been previously used in soybean (Musiyiwa et al., 2005; Thuita et al., 2012; Thilakarathna and Raizada, 2017). The study conducted by Ndusha et al. (2019) demonstrated the outperformance of indigenous species of B. japonicum over the commercial strain Bradyrhizobium diazoefficiens USDA110 by increasing the yield of soybean by 68.7 and 70.8% under greenhouse and field conditions, respectively. Similarly, Chibeba et al. (2017) observed effective nodulation of 85 indigenous soybean nodulating rhizobia isolates under greenhouse conditions, whereby 10 isolates performed better than the best commercial reference strain, B. diazoefficiens USDA 110. However, the symbiotic effectiveness of commercial inoculum in soybean can be limited by the presence of indigenous rhizobial strains which are well-adapted and competitive but ineffective in N2-fixation (Ampomah et al., 2008; Mathu et al., 2012; Kim et al., 2014). Despite the development of promiscuous cultivars that can nodulate with indigenous rhizobial strains (Merrill et al., 2017), it is important to search for indigenous strains that are capable of nodulating a wide range of soybean cultivars. It is also worthwhile to identify the site-specific indigenous soybean nodulating rhizobia to maintain effective nodulation and N fixation. For the improvement of symbiotic interaction between different soybean varieties and rhizobia, it is important to understand the genetic and molecular basis for the host specificity, especially for the new isolates collected from the natural environment.
Factors Affecting the Effectiveness of BNF in Farming Areas
Temperature Stress
Elevated temperature directly affects the formation and functioning of nodules and, indirectly affects the metabolism of the host plant (Hungria and Vargas, 2000; Aranjuelo et al., 2008). One of the indirect effects of high temperature is the depletion of photosynthetic activity (Long et al., 2004; Islam, 2015) caused by stomatal pores closure (Aranjuelo et al., 2014) under elevated carbon dioxide (CO2) and temperature (Aranjuelo et al., 2008). Under high temperatures and elevated levels of CO2, the photosynthetic efficiency of plant leaves decreases, resulting in the inhibition of Rubisco activity, which causes a reduction in N assimilation and reduced carbohydrate concentration in the nodules, resulting in inefficient N fixation (Aranjuelo et al., 2008, 2009). Additionally, high temperatures cause an increased respiration rate, resulting in less substrate availability for the nodules and hence nodule senescence (Hungria and Vargas, 2000; Mohammadi et al., 2012).
Assimilation of N is affected by a decrease in the activities of glutamine synthetase and glutamate synthase (Lea and Miflin, 2018). This results in lower production of allantoin (C4H6N4O3) and allantoic acid (C4H8N4O4), the products of N2 fixation in root nodules of soybean and other tropical and sub-tropical legumes (Bikash et al., 2016; Liu et al., 2018) are transported to the shoot via xylem vessels (Ono et al., 2021). At a root level, elevated temperature affects N2 fixation by decreasing the formation of root hairs, reducing the sites of nodulation, and altering bacterial adherence to root hairs (Hungria and Vargas, 2000; Aranjuelo et al., 2007; Mohammadi et al., 2012). Additionally, high temperature affects molecular signals exchange between the host plant and rhizobia through a decrease in nod-gene inducers (Mabood and Smith, 2005; Mohammadi et al., 2012).
Other factors that are mostly affected by high temperatures include root infection, nodule initiation, and nodule function. Under high-temperature conditions, the survival of rhizobia in the soil is reduced, genetic modification or loss of plasmid occurs, as well as the poor physiological state of surviving rhizobia leading to failure of them to infect root hairs. For the host plant, under high temperatures, the physiological activities are affected, and the development of roots is reduced, resulting in reduced infection sites and leading to failure of nodule initiation. The exchange of molecular signals, especially nod factors and signaling compounds such as flavonoids, between host plants and rhizobia is also affected by high temperature (Hungria and Vargas, 2000; Miransari and Smith, 2009; Mandal et al., 2010; Mario et al., 2015).
However, some soybean nodulating rhizobia have been observed to tolerate high-temperature conditions. Yuan et al. (2020) found that 13 out of 77 isolates of soybean nodulating rhizobia were able to grow under a high temperature of 44°C. Through the phylogenetic analysis, the strains were observed to fall within the Bradyrhizobium and Rhizobium genera. Similarly, Asadi et al. (2009) isolated 56 Bradyrhizobium sp from soils and tested them in an in vitro environment for their ability to tolerate higher temperature conditions, finding that 19 isolates could grow at 38°C and 10 at 41°C. For the effectiveness of N fixation in areas with high temperatures, it is important to isolate the well-adapted indigenous soybean nodulating rhizobia for commercialization.
Soil Moisture Content
Moisture availability of the soil is determined by soil moisture-retaining capacity (Hungria and Vargas, 2000). Moisture stress in the soil results in the reduction of nodule weight and nitrogenase activity and hence affects the N2 fixation process (Siczek and Lipiec, 2011; Dabessa et al., 2018; Santachiara et al., 2019). Although some rhizobial populations occur in desert soils and are effective in nodulation, viable strains cannot survive or function under high moisture stress (Lucrecia et al., 2003; Bashan et al., 2014; Dabessa et al., 2018). Drought is among the major environmental stresses which affect the survival of N2-fixing bacteria and the nodule initiation stage because plants need more adequate moisture during this stage than other stages (Monica et al., 2013). Furthermore, some studies bio-prospect the tolerance of some rhizobial strains under moisture deficiency.
Mnasri et al. (2007) observed that Ensifer meliloti bv. Mediterranense 4H41, a salt-tolerant strain was highly competitive and effective in nodulating common bean (Phaseolus vulgaris) under conditions of water scarcity. Through PCR-RFLP of 16S rRNA and nodC, the same study revealed that drought stress narrows the genetic diversity of nodulating rhizobia. Similarly, Hungria and Vargas (2000) reported the ability of Rhizobium tropici to fix N under water stress conditions. Rampa et al. (2019) found that the soybean nodulating Bradyrhizobium diazoefficiens was capable of fixing N under drought conditions. Similarly, Kunert et al. (2016) reported the ability of Bradyrhizobium sp to form an effective symbiotic association with soybean under water deficit conditions. The consortium of B. japonicum–Trichoderma Harzianum is also observed to increase the productivity of soybean under drought conditions (Nahrawy et al., 2020). More research is needed to investigate the capabilities of indigenous soybean nodulating on N fixation in drought environments, as well as the survival rates of the cells in the soil.
Soil Acidity, Alkalinity, and Salinity
Soil pH determines the availability of nutrients for plant uptake in the soil (Roem and Berendse, 2000; Stark et al., 2012; Penn, 2019). Soil phosphorus (P), acidity, alkalinity, and salinity are among the abiotic factors which determine biogeographical patterns of soybean nodulating rhizobia (Han et al., 2009, 2020; Zhang et al., 2018). The rhizobia population and activities vary among different soil conditions. P is a very important nutrient in the symbiotic association process as it is involved in plants' energy (ATP) acquisition, storage, and utilization (Mmbaga et al., 2014). Iron (Fe) and aluminum (Al) abundance in acidic soil, as well as calcium (Ca) and magnesium (Mg) abundance in alkaline soil, fix soil P in the form of oxides and hydroxides, rendering P unavailable for plant uptake (Dabessa et al., 2018).
Acidic soil causes root injury, leading to reduced water and nutrient uptake as well as endangering the survival of soil microorganisms (Kidd and Proctor, 2001). Furthermore, soil acidity has been observed to negatively affect the process of BNF by altering cell-membrane permeability caused by excess H,+ which induces cation efflux and hence impairing nutrients availability to plants (Ferreira et al., 2016). Alkalinity in soil reduces the abundance, activities, and diversity of rhizobia (Li et al., 2011a,b; Zhang et al., 2017, 2018).
Both extreme soil acidity and alkalinity affect the activities of rhizobia in the soil by inhibiting the growth and the process of nodule initiation (Slattery et al., 2001; Azcon and Barea, 2010; Gómez Padilla et al., 2016). The nod genes of rhizobia are suppressed, the rate of multiplication of rhizobia and root infection is reduced, the distribution and recolonization of the soil are also restricted (Slattery et al., 2001; Miransari and Smith, 2009; Mario et al., 2015). Studies have reported a few species of soybean nodulating rhizobia are tolerant to abiotic stresses. R. tropici and Bradyrhizobium sp. are tolerant to soil acidity (Graham et al., 1994) and B. liaoningense and B. yuanmingense species are tolerant to soil alkalinity (Gómez Padilla et al., 2016).
Tian et al. (2012) conducted a study on the comparative genomics of soybean nodulating rhizobia Bradyrhizobium and Sinorhizobium for gene adaptations to acidic, neutral, and saline-alkaline soils. The results demonstrated clear biogeographic patterns that S. fredii dominated in alkaline-saline soil, while Bradyrhizobium species dominated in neutral to acidic soil. Several other studies have also confirmed that genus Sinorhizobium are dominant in alkaline–saline soils, whereby those which are Bradyrhizobium, dominate neutral to acidic soils (Li et al., 2011a; Zhang et al., 2011, 2017, 2018), observed S. sojae as soybean microsymbionts that inhabit the regions of alkaline soils. Therefore, for the effective symbiosis of indigenous rhizobia that nodulate soybean, it is important to explore the biogeographic patterns, underlying effective nodulation.
Macronutrients
Nitrogen
Nitrogen is the most limiting essential nutrient for plant growth and development (Pasley et al., 2019). The element partakes in numerous metabolic pathways as well as in the synthesis of molecules such as proteins (Bruijn, 2015), nucleic acids, hormones, and chlorophyll (Saturno et al., 2017; Dabessa et al., 2018). Various sources of N in the soil include mineralized organic matter (Ray et al., 2006; Salvagiotti et al., 2008; Ramakrishnan, 2015), BNF, industrial chemical N fertilizers (Ramakrishnan, 2015), lightning, volcanism, and combustion of fossil fuels (Saturno et al., 2017). High levels of N in the soil or that from inorganic fertilizers are reported to inhibit the growth of soil rhizobia (Dabessa et al., 2018), nodule formation, Nase activity, reduce infection threads (Liu et al., 2009; Guan et al., 2013; Saturno et al., 2017), and hence limit N2 fixation (Weisany et al., 2013; Dabessa et al., 2018). When the level of mineral N is higher in the soil, the plants use lesser energy to take up N from the soil than through fixation, hence shifting from symbiotic to inorganic N nutrition (Liu et al., 2009). In legume production, inorganic N is required during the N hunger period for the healthy growth of plants, which facilitates nodule formation (Marino et al., 2013). The study conducted by Kassam (2019) and Wang et al. (2020) revealed that higher levels of N in the soil from supplementation by industrial fertilizers affected the nodulation efficiency of Bradyrhizobium sp with soybean.
Phosphorus
Phosphorus is an important essential nutrient element in the N2-fixation process as it is involved in the plants' energy acquisition, storage, and utilization for the N2-fixation process (Mmbaga et al., 2014). The availability of P results in an increase in the number, size, and weight of nodules (Abebe, 2017). Inadequate levels of P in the soil inhibit symbiotic association between plants and rhizobia, reduction in the mass of nodules, N2-fixation, as well as the yield of leguminous crops (Abebe, 2017; Dabessa et al., 2018). Unavailability of P for plants' uptake may be due to extreme soil pH. Under extreme acidity, P is adsorbed or held in active sites of the soil particles surfaces (Idris and Ahmed, 2012; Mbene et al., 2017). In acid soils, P reacts with oxides and hydroxides of Fe and Al, while in alkaline soils, P reacts with the oxides and hydroxides of cCa (Dabessa et al., 2018). Under such conditions, nodulation and N fixation are inhibited.
Some N-fixing bacteria through interaction with legumes such as soybean are capable of solubilizing P in soil and increasing P use efficiency by plants as well as facilitating the BNF process (Drevon and Abadie, 2015). Mallaiah (2009) observed the ability of Rhizobium isolates/strains from different legumes to solubilize P from different compounds. The study conducted by (Fatima et al., 2006) demonstrated that Rhizobium strains capable of nodulating soybean can solubilize P in the soil for utilization by plants. Similarly, Igiehon et al. (2019) investigated the capability of soybean nodulating Rhizobium sp, Rhizobium tropici, Rhizobium cellulosilyticum, Rhizobium taibaishanense, and Ensifer meliloti in solubilizing P in the soil. Among the five strains, Rhizobium tropici exhibited the bigger halo-zone of 17.3 mm in pikovskaya's agar medium whereby the smallest diameter of halo-zone (10.7 mm) was exhibited by Ensifer meliloti. From the study, the author declared that all five strains of Rhizobium possess the traits of phosphate solubilization. Therefore, as phosphorous is an important element in numerous molecular processes in N fixation, its availability is crucial in the establishment of the symbiotic relationship between the host legume and rhizobia.
Potassium and Sulfur
Symbiotic organisms, including rhizobia, are sensitive to low levels of K when compared to their leguminous host plants. Conversely, potassium ion (K+) plays a significant role as an osmolyte for adaptation in saline soils. Irrigation triggers salinity problems in soils. To ensure the suitability of irrigated soils for cultivating symbiotic legumes, the levels of exchangeable K+ need special consideration (Bonilla and Bolaños, 2009; Wakeel et al., 2011). K is important in the uptake of water and growth of roots in plants, and these influence the nodulation process by increasing the nodulation sites (Youssef et al., 2001; Mfilinge et al., 2014). Studies have reported improved N fixation in the presence of K (Sherwood, 1970).
On the other hand, sulfur (S) is an essential nutrient for plant growth and development. However, the nutrient has received little attention in BNF research, despite the fact that plants that rely on N fixed by rhizobia require more S than plants that rely on mineral N supplements (Divito and Sadras, 2014). S is essential for buffering soil alkalinity and improving rhizobia activities by increasing the number of nodules, N2 fixation, and thus legume yield (Anandham et al., 2007). Some studies have shown that S deficiency lowers BNF because of reduced nodule development as well as Nase and leghemoglobin. The reduction of nodule development, nitrogenase, and leghemoglobin is caused by the N-feedback mechanism, which has been demonstrated to reduce the absorption of mineral N. When the absorption of mineral N is reduced, the fate is the prolonged reduction of N-assimilation, uptake, and fixation by plants in S-deficient soils. This is due to increased levels of N-rich amino acids, especially asparagine, which are responsible for feedback inhibition of N-assimilation. The same study has demonstrated the increase of N-concentration in the nodules as well as N-absorption and fixation upon sulfate application (Varin et al., 2010). The study conducted by Getachew et al. (2016) revealed that the application of S in soil resulted in the improvement of N fixation, improved plant growth, and nutrient uptake by rhizobia inoculated soybean. Similarly, Weisany et al. (2013) observed less growth of Bradyrhizobium sp in media supplemented with a low concentration of S, indicating that S is a very important nutrient for effective nodulation. More studies are required in the roles of K and S on N fixation for the new strains to be identified.
Calcium
Calcium is one of the secondary macronutrients in the soil (Dar et al., 2014). Amongst its roles in plant growth, Ca is essential in the development of plant roots (Hepler, 2005). In the symbiotic association between leguminous plants and rhizobia, Ca enhances the attachment of rhizobia to root hairs, infecting the root and hence nodulation (Dabessa et al., 2018). High levels of Ca in the soil enhance the higher abundance of rhizobia which results in a higher number of nodules in leguminous plants (Bonilla and Bolaños, 2009). The essentiality of Ca in early infection events (El-Hamdaoui et al., 2003) is an indication that the ion is important in plant-bacteria signaling and recognition of nod factors from rhizobia. The activity of Nod genes increases with the levels of Ca in soil being higher (CapoCapoen et al., 2009). This is because, Ca increases the levels of flavonoids in plant root exudates, and the flavonoids are responsible for the induction of nod genes due to signal perception by rhizobia (Miwa et al., 2006). Ca in plants enhances the activity of phenylalanine ammonia-lyase which is the key enzyme responsible for the pathway of flavonoids synthesis (Debona et al., 2017).
The presence of Ca ions enhances bacterial exopolysaccharides in forming a gel as a device for rhizobia to attach to root hairs. Ca strengthens the activities of plant-binding proteins (lectins) as well as rhizobial Ca-dependent adhesion by acting as a ligand (Bonilla and Bolaños, 2009; Janczarek et al., 2015). This signifies that Ca is essential for the optimal colonization of root hair by rhizobia (Fauvart and Michiels, 2008; Abdian et al., 2013).
Concerning the importance of Ca ions in root hair development and infection interactions, the spiking of Ca2+ is crucial in the transduction of signals during the perception of NFs and the organogenesis of nodules. Ca has been revealed as a second messenger in NFs signal transduction following its detectable effect of influx at the root hair tip leading to efflux of chloride ions (Cl−) and hence membrane depolarization (Cullimore et al., 2001; Oldroyd and Downie, 2004; Crespi and Frugier, 2009). The influx of Ca2+ in the root hair tip results in Ca oscillation in the root hair zone. This has been observed to facilitate faster nodulation than the normal invasion of root hair by rhizobia. Rhizobia can enter into their host through cracks formed during lateral root development, but this has been observed to be associated with the death of cells responsible for the formation of infection pockets (Cullimore et al., 2001). At the root hair tip, Ca becomes one of the aqueous components of the cell cytoplasm. The dynamics of Ca may be related to the infection events such as the swelling of root hair tip, vacuolation, and alignment of endoplasmic reticulum with the plasma membrane, movement of nuclear to the swelling root hair, and the inner growth of the cell wall for the initiation of infection threads (Bonilla and Bolaños, 2009). More studies are required on the role of Ca in influencing the activities of isolated but uncharacterized indigenous rhizobia that nodulate and effectively fix N with soybean.
Magnesium
Magnesium is required by rhizobia to stabilize cell membranes, nucleic acids, and ribosomes and has an important role as an enzyme cofactor. Mg serves as a cofactor for many enzymes, complexes with ATP, and has key roles in stabilizing ribosomes and cell walls. Mg-deficient cells of some gram-negative bacteria are very sensitive to environmental stress such as drought and die very quickly (O'Hara, 2001). In biological N fixation, Mg influences nodule formation (Kiss et al., 2004; Ramesh and Winkler, 2010). It is an important nutrient element in the process of photosynthesis as it is involved in enzymatic reactions, enabling them to perform properly, including urease and nitrogenase, which are involved in N fixation (Cakmak and Yazici, 2010; Gransee and Führs, 2013). Mg is reported to be important in the metabolism of rhizobia by facilitating the alteration of carbohydrate partitioning and transport into nodules (Peng et al., 2018). Some reports revealed that the addition of Mg together with other nutrient elements contributed to an increase in nodulation as well as the yield and quality of soybean seeds inoculated with Bardyrhizobium sp (Okereke and Onochie, 1996; Masunga et al., 2016). Similarly, Khaitov (2018) found a significant increase in the shoot and root biomass pod numbers as well as increased Mg levels in the nodules upon the addition of optimum levels of Mg and inoculation by Bradyrhizobium sp. To investigate the further roles of Mg on nodulation and plant growth and development, it is important to test the effects of Mg and inoculation of soybean seeds with different species of newly isolated indigenous rhizobia that effectively fix N with soybean.
Micronutrients
Iron (Fe) and Molybdenum (Mo)
Iron and Molybdenum are the important micronutrients in nitrogenase enzyme activities and cofactors. The two nutrients are the major components of the nitrogenase enzyme system (Bonilla and Bolaños, 2009), which are MoFe protein (MoFe heterotetramer) products of nifDK, Fe-protein a product of nifH which is an oxygen-sensitive metalloenzyme complex, and molybdenum-nitrogenase encoded by nifHDK (Boyd et al., 2011). The nifH genes are responsible for the encoding of nitrogenase enzyme activities. Iron is a cofactor for other proteins inside the nodule which are important in N2-fixation, including leghemoglobin, cytochromes, or ferredoxin (Bonilla and Bolaños, 2009). Fe and Mo at optimum levels in the soil were demonstrated to elevate the concentration of N, proteins, and leghemoglobin in Cicer arietinum Land Vigna radiata (L) (Khan et al., 2014a; Lalita and Kumar, 2017). Between these two nutrients, Mo availability is also hindered by the acidity in the soil as well as the acidifying effect of nitrogenase activity (Doerge et al., 1985). Moreover, Mo is crucial in plant N uptake and N fixation due to the activities of nitrate reductase and nitrogenase enzymes for which Mo acts as a co-factor (Mendel, 2013; Oburger et al., 2018). Therefore, the supply of Fe and Mo is very important for nodulation and N2-fixation. For these reasons, the supplementation of soils with optimum levels of these nutrients can improve the BNF process in legumes as well as increase yield (Bonilla and Bolaños, 2009; Khan et al., 2014a). Rotaru and Sinclair (2009) found that higher levels of iron in the nodules resulted in a significant increase in the N fixation rate and export of ureides from the nodules to the leaves of soybean inoculated with B. japonicum. Bambara and Ndakidemi (2010) reported that the application of Molybdenum significantly improved the symbiotic performance of a leguminous crop in the field.
Copper (Cu) and Cobalt (Co)
Copper (Cu) and Cobalt (Co) has been demonstrated to be involved in the synthesis of leghemoglobin in the nodules due to the presence of vitamin B12, whose concentration in the pinkish nodules is higher as compared with white nodules. The increase in the size of nodules with the increasing levels of Co while Cu has been observed to increase the concentration of N and yield of subterranean clover (Hallsworth et al., 1960; O'Hara, 2001). The study conducted by Wahab et al. (1996) demonstrated the contribution of Cu and Co to nodulation and yield parameters of faba beans. Cobalt was observed to increase the number and weight of nodules while Cu increased the nodule mass. Both metals were observed to increase nitrogenase activity, leghaemoglobin content, dry matter, and total N content of shoots and roots. In the differentiation of bacteroids, Co is essential in the endoreduplication of rhizobia due to its composition in ribonucleotide reductases (Ronald, 2011). Copper ion is also observed to be responsible for the transduction of ATP/energy in microaerobic environments (González-Guerrero et al., 2014).
Nickel (Ni), Manganese (Mn), and Zinc (Zn)
Nickel (Ni) has been indicated as an important metal in BNF due to its significance in the expression and biosynthesis of hydrogenase enzyme (Lavres et al., 2016) which recycles the H2 produced during N2−fixation to conserve energy (González-Guerrero et al., 2014). Ni has been noticed to increase the growth of rhizobia on yeast extract mannitol and the minimum medium with glutamate as an N source. At optimum concentration, Ni is reported to contribute to the increase of the number of nodules, the activity of nitrogenase enzyme, and the plant dry weight in pigeon pea and green gram (Singh and Rao, 1997). According to Lavres et al. (2016), the optimum levels of Ni have significant importance in BNF as they relate to N of N uptake, activities of Nase and urease enzymes, nodulation, plant, and grain dry matter yield of soybean. The recommended concentrations of Mn and Zn should be used to avoid their toxicity and inhibition of rhizobial growth (Wilson and Reisenauer, 1970). More studies are required on the physiological roles of Ni, manganese (Mn), and zinc (Zn) in biological N fixation.
For effective BNF in soybeans and other legumes, it is critical to understand soil characteristics such as soil type, nutrient levels, and climate, particularly temperature and moisture content. Another very important factor is the assessment of soil acidity, alkalinity, and salinity, which are determined by soil pH. In situ trials in different soil types and conditions for the commercial inoculants, which are made from the newly isolated and identified rhizobia, are very important so as to avoid uneconomical and inappropriate use under various soil types. It is also worth isolating site-specific rhizobia species, which can form effective symbioses, especially for problematic soils such as acidic, alkaline, and saline soils as well as extreme temperatures and drought. Considering all these factors will help to avoid compromising the effectiveness of rhizobia inoculants and also ensure the economical and appropriate use of inoculum for smallholder farmers as well as under intensive farming.
Plant Growth-Promoting Functions of Soybean-Nodulating Rhizobia
Apart from the main and most commonly referred to PGP role of rhizobia, BNF, rhizobia possess other PGP traits such as nutrient solubilization such as P, K, and Zn solubilization, as well as siderophore production for iron solubilization. Others include the production of phytohormones such as indole-acetic-acid (IAA) (Igiehon et al., 2019; Aloo et al., 2021), exopolysaccharide (EPS), 1-aminocyclopropane-1-carboxylate (ACC) (Igiehon et al., 2019), cytokinins, gibberellins, riboflavin, and lumichrome (Jaiswal et al., 2021). Apart from promoting the growth in soybean as its symbionts, some species of soybean nodulating rhizobia promote the growth in non-leguminous plants directly or indirectly. Among others, some of the PGP functions in non-leguminous plants such as the production of Abscisic acid (ABA) by Bradyrhizobium japonicum USDA110 along with gibberellins, can serve the purpose of alleviating water stress in maize plants. The nutrients solubilized and released in soil solution for instance P (Khan et al., 2014b), K (Kalkar, 2014; Choudhary et al., 2018; Sattar et al., 2019), and Zn (Rani et al., 2020) available for uptake by all plants including non-leguminous plants. For this review, four of the PGP functions, including BNF, nutrients solubilization, siderophore, and indole-acetic-acid (IAA), will be discussed hereunder.
Biological Nitrogen Fixation
Like all other symbiotic associations with leguminous plants, biological N2 fixation is the most important function of soybean nodulating rhizobia. Biological N2 fixation is an inexpensive and environmentally-friendly alternative source of N2 in which atmospheric N is transformed into biologically active N that can be utilized by plants (Bhattacharjee and Dey, 2014; Simon et al., 2014; Gupta et al., 2015; Suhag, 2016). Symbiotic N2 fixation plays a central role in the production of a million tons of total biological N. The significance of these microbes is evident from the fact that although a total of 100 million metric tons of synthetic N is produced per year, N2 fixing microbes convert about 200 million tons of N to ammonia yearly (Glazer and Nikaido, 2007). The study conducted by Nyaguthii (2017) found that the inoculation of soybean by rhizobia made the grain yield up to 2 tons per hectare. Similarly, Heerwaarden et al. (2017) found that in Sub-Saharan Africa, the inoculation of soybean seeds with rhizobia increased the yield from 1,227 to 1,343 kg/ha in field trials conducted from 2010 to 2015.
Phosphate Solubilization
Phosphorus is the second essential and limiting nutrient element in the soil after N. In soil, the availability of P is rarely adequate for satisfying the plants' requirements due to its fixation in oxides or hydroxides of aluminum/iron or Ca/Mg in the soil, hence unavailable for plant uptake. It is estimated that the deficiency of P in soil reduces the yield of crops by 30–40%, which leads to excessive application of P fertilizers (Malhotra et al., 2018). In soil, the concentration of P is about 2 × 103 higher than the amount required by plants, but only 15–20% is available for plants' uptake while the rest remains unavailable and leaches to surface and groundwater, leading to eutrophication (Smith et al., 2000). For the P to be available to plants, it must be in soil solution in the form of soluble P. Solubility of P in the soil can be increased by plants through exploration of nutrients by roots and exudates such as organic anions and phosphatases (Richardson et al., 2009). For the P to be available in the soil solution, it has to undergo the processes of solubilization and mineralization for inorganic and organic P, respectively, influenced by the microbial and physicochemical structure of the soil as well as plant exudates (Khan et al., 2014b).
Phosphate solubilizing organisms use the same approach of solubilizing insoluble P compounds such as tricalcium phosphate (Ca3PO4)2, aluminum phosphate (Al3PO4), iron phosphate (Fe3PO4), and others, hence making them available for uptake by plants (Gupta et al., 2007). Phosphate solubilizing microorganisms secrete low molecular mass organic acids (OA), which chelate with mineral ions and hence release P in the soil solution. Inorganic acids such as HCl, HNO3, and H2SO4 are also documented as another mechanism used by PS microorganisms to chelate mineral ions and release P in soil solution (Khan et al., 2014b). Another mechanism used by microbes is the secretion of enzymes, such as phosphatase and phytases (Maougal et al., 2014), which converts organic P to inorganic form, which is also available for uptake by plants (Yadav and Tarafdar, 2003; Maougal et al., 2014). Several types of bacteria, including rhizobia in the soil, can solubilize a high proportion of P (Elkoca et al., 2008; Collavino and Sansberro, 2010; Kapri and Tewari, 2010; Hajjam and Cherkaoui, 2017). Bradyrhizobium sp (Mahavidyalaya, 2013) and B. japonicum (Wasule et al., 2003), which nodulates soybean, are capable of solubilizing P. Therefore, apart from the main role of fixing N in the soil, rhizobia can serve the dual purpose of fixing N, solubilizing P in the soil, and hence reducing the excessive application of synthetic fertilizers such as phosphates. It is worthwhile to investigate new rhizobia species that are successful in P solubilization, especially in difficult soils such as acidic, alkaline, and saline soils, where P is fixed in the oxides and hydroxides of Fe, Al, Ca, and Mg and therefore unavailable for plant uptake.
Potassium Solubilization
Potassium is the third macronutrient, following N and P. Its importance is increasing significantly due to increased requirements caused by its continuous production of crops (Dotaniya et al., 2016; Choudhary et al., 2018). Although the element is abundant in soil, it is less available for uptake by plants (Bhattacharyya et al., 2016). This is due to fixation in the soil where almost all K remains as insoluble rocks, necessitating a large reliance on synthetic fertilizers to meet plant requirements (David, 2010; Yadegari and Mosadeghzad, 2012; Choudhary et al., 2018). In the soil, microorganisms including rhizobia (Sessitsch et al., 2013) use organic ligands, enzymes, hydroxyl anions, and biofilms to solubilize K from the minerals such as mica, illite, biotite, and muscovite (Das and Pradhan, 2016) and hence contribute to the availability of the nutrient for uptake by plants (Alikhani, 2017). The process of K solubilization by bacteria involves acidolysis, chelation, complexolysis, and exchange reactions (Kalkar, 2014; Choudhary et al., 2018; Sattar et al., 2019). However, the effectiveness of bacteria to solubilize K depends on the type of soil, bacterial strain, and the form of insoluble K. Some rhizobia, which fixes N in leguminous plants, have been observed to solubilize K in agricultural soils. This is drawing attention to the aspect of sustainability in cropping systems by increasing the availability of K in agricultural soils through a sustainable and reliable source but also environmentally friendly (Meena et al., 2015). According to a study by Htwe et al. (2019), inoculation of soybeans with a combination of Bradyrhizobium sp and Streptomyces griseoflavus increased K uptake at three unfolded trifoliate leaves (V3) in Japan soybean varieties, and at V3 and R2 (full bloom) in Myanmar soybean varieties. This indicates that the two bacteria have a greater ability to solubilize K when inoculated together. Sinorhizobium meliloti one of the soybean nodulating rhizobia have been reported to solubilize K (Deshwal, 2020). With regard to the above studies revealing the capabilities of some soybean nodulating rhizoa in solubilizing K, more research is needed to explore diverse indigenous soybean nodulating rhizobia that are effective in solubilizing K, in order to develop a biofertilizer that will reduce deficiency while also ensuring a sustainable and environmentally friendly supply of K in agricultural soils.
Zinc Solubilization
Zinc is among the seventeen essential plant nutrients in which it is a micronutrient (Mousavi et al., 2013). In agricultural soils, the deficiency of Zn is reduced by supplementation of synthetic fertilizers such as ZnSO4, ZnNO3, ZnO, (ZnCl2), or ZnCO3 to meet crop requirements. However, a greater proportion of the supplemented Zn (Mclaughlin, 2014) is fixed to insoluble complexes (zincate) especially alkaline soils (Udeigwe et al., 2016). This makes it unavailable for uptake by plants (Mclaughlin, 2014; Hacisalihoglu, 2020). In soil, Zn solubilizing bacteria (ZNB) produce organic acids such as gluconic and ketogluconate, which combine with soil mineral surfaces and form compounds with Zn and hence chelation of Zn through carboxyl and hydroxyl groups, which then facilitate the solubility of Zn (Rani et al., 2020). Some non-rhizobia endophytic bacteria (NREB), in soybean nodules, Pseudomonas sp have been observed to solubilize Zn under in vitro conditions and their synergistic effect with N2 fixing rhizobia promotes the growth of soybean plants. Poonam et al. (2019) demonstrated that NREB has a good ability to solubilize Zn and the synergistic effect of Bradyrhizobium sp (LSBR-3) and NREB, Pseudomonas aeruginosa (LSE-2) improved the growth of soybean plants as compared to inoculation by Bradyrhizobium sp (LSBR-3) alone and non-inoculated treatment. Similarly, (2014) conducted a co-inoculation study of soybean seeds with B. japonicum and each of Nocardia alba S4301, Nonomuraea rubra S3304, and Actinomadura glauciflava significantly increased the yield of soybean as well as nutrients concentration including Zn in plant samples. However, there is limited information about the solubilization of Zn by soybean nodulating rhizobia. Therefore, isolation of diverse soybean nodulating rhizobia from different soil types to obtain the effective species with the dual purpose of fixing N and Zn solubilization or a consortium inoculation to save both purposes is important for sustainable availability of Zn to plants in agricultural soils.
Siderophore Production
Iron (Fe) is an important nutrient in the BNF process as it is a co-factor of leghemoglobin, cytochromes, ferredoxin (Bonilla and Bolaños, 2009), and nitrogenase enzyme (Paudyal et al., 2007). Although Fe is adequate in most soils, its substantial amount is inaccessible to plants due to the low solubility of its oxides, making Fe deficient in many agricultural soils (Colombo et al., 2014; Arora and Verma, 2017). Siderophores are low-molecular-weight secondary metabolites that chelate with iron (Arora and Verma, 2017). Siderophores are unique compounds with functional groups and electron-rich side chains (Verma et al., 2011; Ghavami et al., 2017) that bind ferric ions and transport them across the cell membrane (Niehus et al., 2017). In soils with inadequate iron, bacteria, including rhizobia, produce siderophores, which chelate the iron and subsequently solubilize it into complexes that are easily available for uptake by plants (Mathew et al., 2014; Boiteau et al., 2016). The ability of microorganisms to produce siderophores can be a sustainable solution to making iron readily available for uptake by plants in iron-deficient soils. Catecholates, carboxylates, hydroxamates, and combinations of these are produced by a variety of bacteria, including rhizobia (Anastasiou et al., 2014; Mimmo et al., 2014; Aznar et al., 2015; Arora and Verma, 2017). Siderophore production is one of the key PGP functions of bacteria, including rhizobia (Verma et al., 2011). Apart from mobilizing iron uptake by plants, siderophores produced by rhizobia increase nodulation and N fixation, as well as improve the adaptation of plants to disease-causing pathogens and stress (Jaiswal et al., 2021). Different studies have revealed the higher abilities of soybean nodulating rhizobia in the production of siderophores. Igiehon et al. (2019) demonstrated the higher levels of siderophore production by different species of soybean nodulating rhizobia, including Rhizobium sp., R. tropici, R. cellulosilyticum, R. taibaishanense, and E. meliloti. Similarly, Khandelwal et al. (2002) observed the production of siderophores by B. japonicum and improved nodulation in soybean under a sick-pot experiment. Regarding the ability of soybean nodulating rhizobia in siderophore production, it is worth exploring them by isolating them from diverse ecologies to obtain the most effective species that can produce siderophores for effective nodulation and N2 fixation in soybean.
Indole-3-Acetic Acid (IAA) Production
Indole-3-acetic acid (IAA) is a naturally occurring auxin hormone with broad physiological effects. The hormone is produced through different pathways, which are indole-3-acetamide (IAM), indole-3-pyruvate (IPyA), tryptamine, and indole-3-acetonitrile (Lambrecht et al., 2000). It is well-documented that IAA is responsible for vascular tissues differentiation, elongation growth, apical dominance, lateral root initiation, fruit setting, and ripening (Dilfuza, 2011), whereas exogenous application of IAA from rhizobia promotes nodulation, root growth, and plant development in leguminous plants (Jaiswal et al., 2021). Several studies have identified different soybean nodulating rhizobia, which produces IAA associated with the promotion of nodulation and increased N fixation. Appunu et al. (2009) conducted an in vitro test of the IAA production of soybean nodulating rhizobia and observed that the species of rhizobia from the Ensifer group and B. elkanii demonstrated the good capability of producing IAA. Fukuhara et al. (1994) revealed that the exogenous application of B. elkanii, which produces IAA, increased the number of nodules in soybean. Similarly, Shi et al. (2002) investigated the production of IAA by soybean nodulating rhizobia. The species demonstrated different capabilities in synthesizing IAA such that the reference strains B. japonicum SEMIA 566 and SEMIA 5080 produced 1.00–15.55 μM, which was rated as low. Among the test isolates, 23 produced 16.6–50.0 μM rated as medium, while the other 8 isolates, together with B. elkanii SEMIA 587 and SEMIA 5019, and S. fredii USDA 205 and CCBAU 114, high concentrations (50–130 μM of reference strains. Depending on the reviewed studies, it is worth conducting more studies to obtain the rhizobia strains, which are highly effective in the production of IAA solely or together with N2 fixation, and commercialize them as inoculum.
Role of Soybean-Nodulating Rhizobia in the Suppression of Soybean Soil-Borne Pathogens
Along with their ability to fix N, rhizobia also have a good ability to suppress soil-borne pathogens affecting plants (Farhat et al., 2017). Some species like R. japonicum (Al-Ani et al., 2012), S. meliloti, B. japonicum, and R. leguminosarum have been observed to suppress the common root-rot diseases caused by Phytophthora clandestine, Pythium ultimum, Fusarium solani, Fusarium oxysporum, Pythium sp., and Rhizoctonia bataticola (Parveen et al., 2019). Soybean plants are among the legumes which are highly affected by soil-borne pathogens which cause root-rot diseases which limit the growth and yield of plants (Yang and Feng, 2001). Macrophomina phaseolina and F. solani are among the most important soybean root pathogens (Al-Ani et al., 2012). Some rhizobial species, including Bradyrhizobium sp, S. meliloti, and Rhizobium sp, have been observed to suppress F. solani, Macrophomina phaseolina, F. oxysporum, and R. solani in the rhizosphere of soybean. Some of the mechanisms reported include rhizobia producing antibiotics, hydrocyanic acid, and mycolytic enzymes, hence limiting the growth of soil pathogens (Das et al., 2017). These rhizobial species were observed to be effective in suppressing F. solani, M. phaseolina, F. oxysporum, and R. solani in the rhizosphere of soybean when applied in combination with mustard oil cakes and Trichoderma harzianum (Parveen et al., 2019). Trichoderma harzianum has been observed to be effective in the biocontrol of root rot pathogens (Afzal et al., 2013). Mustard oil cake is used as organic nitrogenous fertilizer with a high content of N, P, and K (NPK), increasing uptake of N by plants as well as protecting plants from infection by nematodes, soil insects, and parasites (Parveen et al., 2019). Therefore, it is important to explore the new rhizobia species, which are capable and effective in suppressing the root rot nematodes and fungal pathogens causing the losses in the yield of soybean. It is also worth testing the multiple roles of rhizobia in fixing N, solubilizing P, suppressing root rot diseases, or preparing the inoculum with different species or co-inoculation of rhizobia with different roles and testing the compatibility effect. For instance, an inoculum containing different rhizobia species that fix N, solubilize P and suppress root rot disease causing-pathogens.
Effect of Soybean Nodulating Rhizobia in Plant Growth and Development
Soybean plants form symbiotic associations with rhizobia in the soil to form root nodules. The rhizobia fix atmospheric N2, which is then used by the host plant. Apart from the symbiotic association, soybean roots can extract N from the soil or fertilizers (Simon et al., 2014; Takuji et al., 2017). The amount of N that is taken in the shoot of soybean plants is correlated with the yield of soybean seeds regardless of the N. The availability of N is very important for the improved and sustainable production of soybean. This can be achieved by investing in biofertilizers made from the rhizobia species, which are effective in fixing N. This is an affordable, environmentally friendly, and highly effective source of N in agricultural soils (Shi et al., 2002; Takuji et al., 2017; Reckling et al., 2021). Kassam (2019) reported that the potential of soybean to fix N is about 88-188 kg N/ha/year. When there is a low level of N in the soil, soybean can fix up to 300 kg of N/ha under inoculation with effective Bradyrhizobiaum sp (Ntambo et al., 2017). On the other hand, Rhizobium spp and S. fredii SMH12 are observed to increase soybean seed N up to 193 and 175 kg ha−1 under Oxisol soil with pH 5.4 as compared with non-inoculated treatment with a seed yield N of 168 kg ha−1 (Hungria et al., 2001).
The symbiotic performance of soybean nodulating rhizobia is assessed by evaluating the effect of nodulation on different plant growth parameters as well as the determination of plant N percentage in the shoot. For nodulation, the assessment is mainly on the number and dry weight of nodules per plant, while for plant growth parameters, the assessment is done on plant height, a number of leaves, root length, shoot and root dry weight, seed yield, N content in above-ground biomass and harvest index (Rodriguez-Navarro et al., 2011; Elkoca et al., 2015). Rodriguez-Navarro et al. (2011) conducted the field experiment on the inoculation of soybean with eight S. fredii strains against the standard inoculum of B. japonicum USDA110 (currently: B. diazoefficiens USDA110) strain, N-fertilized plants, and non-fertilized plants. An increase in the number of nodules per plant from zero in N fertilizer and none-inoculated soybean plants to a maximum of 106 for B. japonicum and 163 for S. fredii was observed. The dry weight of nodules per plant increased from 441 to 780 mg, the seed yield increased from 2,226 kg/ha in non-inoculated plants and 3,732 kg/ha in N-fertilized plants to an astonishing value of 4,041 and 4,338 kg/ha following B. japonicum and S. fredii inoculation, respectively. The seed N content of N-fertilized plants was 197 kg/ha, while in plants inoculated with B. japonicum and S. fredii, the seed N content was 252 and 230 kg/ha, respectively.
On the other hand, Chibeba et al. (2017) tested the symbiotic performance of indigenous Bradyrhizobium sp and Rhizobium sp in Mozambican soils and observed a significant relationship (p < 0.05) between the nodule numbers and their dry weight, nodule dry weight with shoot dry weight and total N of the shoot, as well as between shoot dry weight and total N. Similarly, Iturralde et al. (2019) reported a positive relationship (p < 0.05) between nodulation and plant growth parameters including shoot and dry weight per plant, above and below-ground biomass ratio, the N content of shoot and root, as well as ureides in the plant leaves. Similarly, Alam et al. (2015) observed a significant (p ≤ 0.05) increase in soybean pod, stover, and seed yield in three of four soybean genotypes that were inoculated with Rhizobium sp. BARIRGm901 under field conditions. The results from the above studies imply that the soybean nodulating rhizobia strains which are effective in fixing N outperform the use of nitrogenous fertilizers for the attainment of an optimum yield of soybean. However, the performance of rhizobia is affected by many factors, including soil nutrients, soil reaction (acidity and alkalinity), and climate. These factors need careful consideration for the successful use of biofertilizers for the growth promotion function.
Concluding Remarks
Soybean-nodulating rhizobia are important parts of the ecosystem due to their symbiotic N2-fixing activities with legumes. With the goal of bettering our understanding of this unique group of bacteria, the present review summarizes the approaches employed in characterization and studies of rhizobial diversity, symbiotic effectiveness, plant growth-promoting (PGP) functions, and suppression of soybean soil-borne pathogens. To obtain the most effective rhizobia species for a given application, extensive morphological, biochemical, and molecular characterization is required. Differentiating the rhizobia for further investigation requires morphological characterization, including colony characteristics and the gram reaction, as well as many biochemical studies. Essential biochemical assays such as antibiotic resistance are done to measure the survival and competitiveness of rhizobia in the soil. Carbohydrates utilization assays are undertaken to determine the potential of rhizobia to utilize different sources of carbon as their only source of energy because carbon is a crucial substrate for microbial activities, including rhizobia. Production of nitrogenase enzyme by rhizobia is tested to evaluate the capability of rhizobia in fixing N. Solubilization of essential nutrients such as P, K, and Zn as well as production of siderophores and IAA are tested as markers of PGP activities. Apart from these PGP functions, rhizobia's capacity to suppress pathogens that cause root rot disease is being explored as a biological control agent. Genetic identification and taxonomic classification of the discovered organism are the primary goals of molecular characterization. Furthermore, molecular approaches are used to investigate gene activities such as symbiotic genes like nodC and nifH. It is apparent that although the inoculation of soybean with rhizobia can improve N2 fixation, nutrients solubilization, and promote plant growth, these tend to differ with the type of rhizobia species. Despite notable research efforts into the ability of soybean nodulating in the suppression of soil-borne pathogens, there is still limited information about the availability of commercial rhizobia inoculum that suppresses root rot disease in soybean. Therefore, a complete understanding of the ecology of indigenous soybean-nodulating rhizobia concerning their genetic diversity and the environmental factors associated with their effectiveness is required. Such information can ultimately result in comprehensive knowledge about these soybean symbionts and increase their large-scale application for soybean inoculation and sustainability of agricultural systems.
Author Contributions
MDN, PAN, and PBV: conceptualization and methodology. MDN: original draft preparation. PAN and PBV: review and editing. All authors have read and agreed to the published version of the manuscript.
Conflict of Interest
The authors declare that the research was conducted in the absence of any commercial or financial relationships that could be construed as a potential conflict of interest.
Publisher's Note
All claims expressed in this article are solely those of the authors and do not necessarily represent those of their affiliated organizations, or those of the publisher, the editors and the reviewers. Any product that may be evaluated in this article, or claim that may be made by its manufacturer, is not guaranteed or endorsed by the publisher.
Acknowledgments
The authors thank all staff and technical experts from Nelson Mandela African Institution of Science and Technology (NM-AIST), Arusha-Tanzania for their guidance and support during the preparation of this paper.
References
Abaidoo, R. C., Keyser, H. H., Singleton, P. W., and Borthakur, D. (2002). Comparison of molecular and antibiotic resistance profile methods for the population analysis of Bradyrhizobium spp. (TGx) isolates that nodulate the new TGx soybean cultivars in Africa. J. Appl. Microbiol. 92, 109–117. doi: 10.1046/j.1365-2672.2002.01518.x
Abd-Alla, M. H. (1994). Phosphatases and the utilization of organic phosphorus by Rhizobium leguminosarum biova r viceae. Lett. Appl. Microbil. 10, 294–296. doi: 10.1111/j.1472-765X.1994.tb00873.x
Abdian, P. L., Caramelo, J. J., Ausmees, N., and Zorreguieta, A. (2013). RapA2 is a calcium-binding lectin composed of two highly conserved cadherin-like domains that specifically recognize Rhizobium leguminosarum acidic exopolysaccharides. J. Biol. Chem. 288, 2893–2904. doi: 10.1074/jbc.M112.411769
Abebe, Z. (2017). On-farm yield variability and responses of common bean (Phaseolus vulgaris L.) varieties to rhizobium inoculation with inorganic fertilizer rates. J. Anim. Plant Sci. 32, 5120–5133.
Abera, T., Semu, E., and Debele, T. (2015). Determination soil rhizobium populations, intrinsic antibiotic resistance, nodulation and seed yield of faba bean and soybean in Western Ethiopia. World J. Agri Sci. 11, 311–324.
Adhikari, D., Kaneto, M., Itoh, K., Suyama, K., Pokharel, B. B., and Gaihre, Y. K. (2012). Genetic diversity of soybean-nodulating rhizobia in Nepal in relation to climate and soil properties. Plant Soil. 357, 131–145. doi: 10.1007/s11104-012-1134-6
Afzal, S., Tariq, S., Sultana, V., Ara, J., and Ehteshamul-Haque, S. (2013). Managing the root diseases of okra with endo-root plant growth promoting pseudomonas and trichoderma viride associated with healthy okra roots. Pak. J. Bot. 45, 1455–1460.
Agoyi, E. E., Odong, T. L., Tumuhairwe, J. B., Chigeza, G., Diers, B. W., and Tukamuhabwa, P. (2017). Genotype by environment effects on promiscuous nodulation in soybean (Glycine max L. Merrill). Agri. Food Secur. 6, 1–14.
Alam, F., Bhuiyan, M. A. H., Alam, S. S., Waghmode, T. R., Kim, P. J., and Lee, Y. B. (2015). Effect of Rhizobium sp. BARIRGm901 inoculation on nodulation, nitrogen fi xation and yield of soybean (Glycine max) genotypes in gray terrace soil. Biosci. Biotechnol. Biochem. 79, 1–9. doi: 10.1080/09168451.2015.1044931
Al-Ani, R. A., Adhab, M. A., Mahdi, M. H., and Abood, H. M. (2012). Rhizobium japonicum as a biocontrol agent of soybean root rot disease caused by Fusarium solani and Macrophomina phaseolina. Plant Protect. Sci. 48, 149–155. doi: 10.17221/16/2012-PPS
Alexandre, A., and Oliveira, S. (2013). Response to temperature stress in rhizobia. Crit. Rev. Microbiol. 39, 219–228. doi: 10.3109/1040841X.2012.702097
Alikhani, H. E. S. E. H. A. (2017). Potassium solubilizing bacteria (KSB): mechanisms, promotion of plant growth, and future prospects - a review. J. Soil Sci. Plant Nutr. 17, 897–911. doi: 10.4067/S0718-95162017000400005
Aloo, B. N., Mbega, E. R., and Makumba, B. A. (2021). “Sustainable food production systems for climate change mitigation: indigenous rhizobacteria for potato bio-fertilization in Tanzania,” in African Handbook of Climate Change Adaptation, eds W. L. Filho, N. Oguge, D. Ayal, L. Adeleke, and I. da Silva (Cham: Springer), doi: 10.1007/978-3-030-45106-6_276
Ampomah, O. Y., Ofori-ayeh, E., Solheim, B., and Svenning, M. M. (2008). Host range, symbiotic effectiveness and nodulation competitiveness of some indigenous cowpea bradyrhizobia isolates from the transitional savanna zone of Ghana. Afri. J. Biotechnol. 7, 988–996.
Anandham, R., Sridar, R., Nalayini, P., Poonguzhali, S., and Madhaiyan, M. (2007). Potential for plant growth promotion in groundnut (Arachis hypogaea L.) cv. ALR-2 by co-inoculation of sulfur-oxidizing bacteria and Rhizobium. Microbiol. Res. 162, 139–153. doi: 10.1016/j.micres.2006.02.005
Anastasiou, E., Lorentz, K. O., Stein, G. J., and Mitchell, P. D. (2014). Prehistoric schistosomiasis parasite found in the Middle East. Lancet Infect. Dis. 14, 553–554. doi: 10.1016/S1473-3099(14)70794-7
Appunu, C., N'Zoue, A., and Laguerre, G. (2008). Genetic diversity of native bradyrhizobia isolated from soybeans (Glycine max L.) in different agricultural-ecological-climatic regions of India. Appl. Environ. Microbiol. 74, 5991–5996. doi: 10.1128/AEM.01320-08
Appunu, C., Sasirekha, N., Prabavathy, V. R., and Nair, S. (2009). A significant proportion of indigenous rhizobia from India associated with soybean (Glycine max L.) distinctly belong to Bradyrhizobium and Ensifer genera. Biol. Fertil. Soils 46, 57–63. doi: 10.1007/s00374-009-0405-8
Aranjuelo, I., Arrese-Igor, C., and Molero, G. (2014). Nodule performance within a changing environmental context. J. Plant Physiol. 171, 1076–1090. doi: 10.1016/j.jplph.2014.04.002
Aranjuelo, I., Irigoyen, J. J., Nogués, S., and Sánchez-Díaz, M. (2009). Elevated CO2 and water-availability effect on gas exchange and nodule development in N2-fixing alfalfa plants. Environ. Exp. Bot. 65, 18–26. doi: 10.1016/j.envexpbot.2008.06.006
Aranjuelo, I., Irigoyen, J. J., and Sánchez-Díaz, M. (2007). Effect of elevated temperature and water availability on CO2 exchange and nitrogen fixation of nodulated alfalfa plants. Environ. Exp. Bot. 59, 99–108. doi: 10.1016/j.envexpbot.2005.10.008
Aranjuelo, I., Irigoyen, J. J., Sánchez-Díaz, M., and Nogués, S. (2008). Carbon partitioning in N2 fixing Medicago sativa plants exposed to different CO2 and temperature conditions. Funct. Plant Biol. 35, 306–317. doi: 10.1071/FP07296
Arora, N. K., and Verma, M. (2017). Modified microplate method for rapid and efficient estimation of siderophore produced by bacteria. Biotech. 7, 1–9. doi: 10.1007/s13205-017-1008-y
Asadi, H. R. N., Saleh-rastin, and Khavazi, K. (2009). Selection of thermotolerant bradyrhizobial strains for nodulation of soybean (Glycine max L.) in semi-arid regions of Iran. World J. Microbiol. Biotechnol. 25, 591–600. doi: 10.1007/s11274-008-9927-8
Azcon, R., and Barea, J.-M. (2010). “10 mycorrhizosphere interactions for legume improvement,” in Microbes for Legume Improvement, eds M. S. Khan, J. Musarrat and A. Zaidi (Vienna: Springer), 237–271. doi: 10.1007/978-3-211-99753-6_10
Aznar, A., Chen, N. W. G., Thomine, S., and Dellagi, A. (2015). Immunity to plant pathogens and iron homeostasis. Plant Sci. 240, 90–97. doi: 10.1016/j.plantsci.2015.08.022
Balan, S. S., Nethaji, R., Sankar, S., and Jayalakshmi, S. (2012). Production of gelatinase enzyme from Bacillus spp isolated from the sediment sample of Porto Novo Coastal sites. Asian Pacific J. Trop. Biomed. 2(3Suppl.):60500. doi: 10.1016/S2221-1691(12)60500-0
Bambara, S., and Ndakidemi, P. A. (2010). Phaseolus vulgaris response to Rhizobium inoculation, lime and molybdenum in selected low ph soil in Western Cape, South Africa. Afri. J. Agri. Res. 5, 1804–1811. doi: 10.5897/AJAR09.584
Bashan, Y., Luz, E., de-Bashan, P. S., and Juan-Pablo, H. (2014). Advances in plant growth-promoting bacterial inoculant technology: formulations and practical perspectives. Plant Soil. 378, 1–33. doi: 10.1007/s11104-013-1956-x
Benjelloun, I., Alami, I. T., Douira, A., Udupa, S. M., and Ramírez-bahena, M. H. (2019). Phenotypic and genotypic diversity among symbiotic and non-symbiotic bacteria present in chickpea nodules in Morocco. Front. Microbiol. 10, 1–19. doi: 10.3389/fmicb.2019.01885
Berrada, H., Nouioui, I., Houssaini, M. I., Ghachtouli, N. E. L., Gtari, M., and Benbrahim, K. F. (2012). Phenotypic and genotypic characterizations of rhizobia isolated from root nodules of multiple legume species native of Fez, Morocco. Afri. J. Microbiol. Res. 6, 5314–5324. doi: 10.5897/AJMR11.1505
Berrada, H. K. F.-B. (2014). Taxonomy of the Rhizobia: current perspectives. Br. Microbiol. Res. J. 4, 616–639. doi: 10.9734/BMRJ/2014/5635
Bhattacharjee, R., and Dey, U. (2014). Biofertilizer, a way towards organic agriculture : a review. Afri. J. Microbiol. Res. 8, 2332–2342. doi: 10.5897/AJMR2013.6374
Bhattacharyya, P. N., Goswami, M. P., and Bhattacharyya, L. H. (2016). Perspective of beneficial microbes in agriculture under changing climatic scenario : a review. J. Phytol. 8, 26–41. doi: 10.19071/jp.2016.v8.3022
Bikash, A., Jaime, B., and Teixeira, A. (2016). Early signaling, synthesis, transport and metabolism of ureides. J. Plant Physiol. 193:97–109.
Boiteau, R. M., Mende, D. R., Hawco, N. J., McIlvin, M. R., Fitzsimmons, J. N., Saito, M. A., et al. (2016). Siderophore-based microbial adaptations to iron scarcity across the eastern Pacific Ocean. Proc. Natl. Acad. Sci. U. S. A. 113, 14237–14242. doi: 10.1073/pnas.1608594113
Bonilla, I., and Bolaños, L. (2009). Mineral nutrition for legume-rhizobia symbiosis: B, Ca, N, P, S, K, Fe, Mo, Co, and Ni: a review. Sustain. Agri. Rev. 1, 253–274. doi: 10.1007/978-1-4020-9654-9_13
Boyd, E. S., Hamilton, T. L., and Peters, J. W. (2011). An alternative path for the evolution of biological nitrogen fixation. Front. Microbiol. 2, 1–11. doi: 10.3389/fmicb.2011.00205
Bruijn, F. J. De. (2015). “Biological nitrogen fixation,” in Principles of Plant-Microbe Interactions, ed B. Lugtenberg (Cham: Springer International Publishing), 215–224. doi: 10.1007/978-3-319-08575-3_23
Burdon, J. J., Gibson, A. H., Searle, S. D., Woods, M. J., and Brockwell, J. (1999). Variation in the effectiveness of symbiotic associations between native rhizobia and temperate Australian Acacia: within-species interactions. J. Appl. Ecol. 36, 398–408. doi: 10.1046/j.1365-2664.1999.00409.x
Cakmak, I., and Yazici, A. M. (2010). Magnesium: a forgotten element in crop production. Better Crops 94, 23–25.
CapoCapoen, W., Den Herder, J., Sun, J., Verplancke, C., De Keyser, A., De Rycke, R., et al. (2009). Calcium spiking patterns and the role of the calcium/calmodulin-dependent kinase CCaMK in lateral root base nodulation of Sesbania rostrata. Plant Cell 21, 1526–1540. doi: 10.1105/tpc.109.066233
Chen, L. S., Figueredo, A., Pedrosa, F. O., and Hungria, M. (2000). Genetic characterization of soybean rhizobia in Paraguay. Appl. Environ. Microbiol. 66, 5099–5103. doi: 10.1128/AEM.66.11.5099-5103.2000
Chen, W. X., Yan, H., and Li, J. L. (1988). Numerical taxonomic study of fast-growing soybean rhizobia and a proposal that Rhizobium fredii be assigned to Sinorhizobium gen. nov. Int. J. Systemat. Bacteriol. 38, 392–397. doi: 10.1099/00207713-38-4-392
Chianu, J., Nkonya, E., Mairura, F., Chianu, J., and Akinnifesi, F. (2011). Biological nitrogen fixation and socioeconomic factors for legume production in sub-Saharan Africa: a review biological nitrogen fixation and socioeconomic factors for legume production in sub-Saharan Africa: a review. Agron. Sustain. Dev. 31, 139–154. doi: 10.1051/agro/2010004
Chianu, N., and Mairura, F. (2019). Soybean Situation and Outlook Analysis: The Case of Tanzania. Available online at: https://www.academia.edu/21091023
Chibeba, A. M., Kyei-Boahen, S., Guimarães, M. de. F., Nogueira, M. A., and Hungria, M. (2017). Isolation, characterization and selection of indigenous Bradyrhizobium strains with outstanding symbiotic performance to increase soybean yields in Mozambique. Agri. Ecosyst. Environ. 246, 291–305. doi: 10.1016/j.agee.2017.06.017
Choudhary, M., Ghasal, P. C., Yadav, R. P., Meena, V. S., Mondal, T., and Bisht, J. K. (2018). “Towards plant-beneficiary rhizobacteria and agricultural sustainability,” in Role of Rhizospheric Microbes in Soil, ed V. S. Meena (Singapore: Springer Nature Singapore Pte Ltd), 1. doi: 10.1007/978-981-13-0044-8_1
Clarke, V. C., Loughlin, P. C., Day, D. A., and Smith, P. M. C. (2014). Transport processes of the legume symbiosome membrane. Front. Plant Sci. 5, 1–9. doi: 10.3389/fpls.2014.00699
Collavino, M. M., and Sansberro, P. A. (2010). Comparison of in vitro solubilization activity of diverse phosphate-solubilizing bacteria native to acid soil and their ability to promote Phaseolus vulgaris growth. Biol. Fertil. Soils 46, 727–738. doi: 10.1007/s00374-010-0480-x
Colombo, C., Palumbo, G., He, J. Z., Pinton, R., and Cesco, S. (2014). Review on iron availability in soil: interaction of Fe minerals, plants, and microbes. J. Soils Sediment. 14, 538–548. doi: 10.1007/s11368-013-0814-z
Crespi, M., and Frugier, F. (2009). Erratum: De novo organ formation from differentiated cells: root nodule organogenesis (Sci. Signal. (2009) 2 (er1)). Sci. Signal. 2, 1–8. doi: 10.1126/scisignal.149re11
Cullimore, J. V., Ranjeva, R., and Bono, J. J. (2001). Perception of lipo-chitooligosaccharidic Nod factors in legumes. Trends Plant Sci. 6, 24–30. doi: 10.1016/S1360-1385(00)01810-0
Dabessa, A., Abebe, Z., and Bekele, S. (2018). Limitations and strategies to enhance biological nitrogen fixation in sub-humid tropics of Western Ethiopia. J. Agri. Biotechnol. Sustain. Dev. 10, 122–131. doi: 10.5897/JABSD2018.0318
Dar, M. A., Wani, J. A., Bangroo, S. A., Aslam, M., Dar, M. A., Bhat, M. Y., et al. (2014). Secondary nutrient status in pear orchard soils of Kashmir at different altitudes. Res. J. Agri. Sci. 5, 410–415. doi: 10.13140/RG.2.2.17415.24480
Das, I., and Pradhan, M. (2016). Potassium-Solubilizing Microorganisms and Their Role in Enhancing Soil Fertility and Health. Springer India. doi: 10.1007/978-81-322-2776-2_20
Das, K., Prasanna, R., and Saxena, A. K. (2017). Rhizobia: a potential biocontrol agent for soilborne fungal pathogens. Folia Microbiol. 62, 425–435. doi: 10.1007/s12223-017-0513-z
David, A. C. M. (2010). Mineral sources of potassium for plant nutrition. A review. Agron. Sustain. Dev. 30, 281–294. doi: 10.1051/agro/2009023
Dawson, I. K., Park, S. E., Attwood, S. J., Jamnadass, R., Powell, W., Sunderland, T., et al. (2019). Contributions of biodiversity to the sustainable intensification of food production. Glob. Food Secur. 21, 23–37. doi: 10.1016/j.gfs.2019.07.002
Debona, D., Cruz, M. F. A., and Rodrigues, F. A. (2017). Calcium-triggered accumulation of defense-related transcripts enhances wheat resistance to leaf blast. Trop. Plant Pathol. 42, 309–314. doi: 10.1007/s40858-017-0144-6
Deora, G. S., and Singhal, K. (2010). Isolation, biochemical characterization and preparation of biofertilizers using Rhizobium strains for commercial use. Biosci. Biotechnol. Res. Commun. 2010, 132–136.
Deshwal, V. K. (2020). Influence of the co-inoculation rhizobium sr-9 and pseudomonas sp-8 on growth of soybean crop. Develop. Microbiol. Mole. Biol. 2, 67–74.
Dilfuza, E. (2011). “Indole acetic acid production by root associated bacteria and its role in plant growth and development,” in Auxins: Structure, Biosynthesis, eds A. H. Keller and M. D. Fallon (Hauppauge, NY: Nova Science Publishers, Inc.), 1–13.
Divito, G. A., and Sadras, V. O. (2014). How do phosphorus, potassium and sulphur affect plant growth and biological nitrogen fixation in crop and pasture legumes? A meta-analysis. Field Crops Res. 156, 161–171. doi: 10.1016/j.fcr.2013.11.004
Doerge, T. A., Bottomley, P. J., and Gardner, E. H. (1985). Molybdenum limitations to alfalfa growth and nitrogen content on a moderately acid, high-phosphorus soil 1. Agron. J. 77, 895–901. doi: 10.2134/agronj1985.00021962007700060015x
Dotaniya, M. L., Meena, V. D., Basak, B. B., and Meena, R. S. (2016). Potassium uptake by crops as well as microorganisms. Proc. Natl. Acad. Sci. India Sec. B 85, 267–280. doi: 10.1007/978-81-322-2776-2_19
Drevon, J., and Abadie, J. (2015). Phosphorus use efficiency for N2 fixation in the rhizobial symbiosis. Biol. Nitr. Fixat. 1, 455–464. doi: 10.1002/9781119053095.ch46
El-Hamdaoui, A., Redondo-Nieto, M., Rivilla, R., Bonilla, I., and Bolaños, L. (2003). Effects of boron and calcium nutrition on the establishment of the Rhizobium leguminosarum-pea (Pisum sativum) symbiosis and nodule development under salt stress. Plant Cell Environ. 26, 1003–1011. doi: 10.1046/j.1365-3040.2003.00995.x
Elkoca, E., Kantar, F., and Sahin, F. (2008). Influence of nitrogen fixing and phosphorus solubilizing bacteria on the nodulation, plant growth, and yield of chickpea. J. Plant Nutr. 31, 157–171. doi: 10.1080/01904160701742097
Elkoca, E., Kocli, T., Gunes, A., Turan, M., Arietinum, C., and Of, L. C. (2015). The symbiotic performance and plant nutrient uptake of certain nationally registered chickpea (Cicer arietinum L.) cultivars of Turkey. J. Plant Nutr. 4167, 1427–1448. doi: 10.1080/01904167.2014.983123
Ezeaku, P. I., Unagwu, B. O., and Sc, E. (2012). Sustainable agricultural development strategies in increasing sub-saharan Africa. Int. J. Basic Appl. Sciencesci. 1, 272–288.
Fan, Y., Liu, J., Lyu, S., Wang, Q., Yang, S., Zhu, H., et al. (2017). The soybean Rfg1 gene restricts nodulation by Sinorhizobium fredii. Front. Microbiol. 8, 1–9. doi: 10.3389/fpls.2017.01548
Farhat, H., Urooj, F., Shafique, H. A., Sultana, V., and Ehteshamul-Haque, S. (2017). Rhizobia suppress the root knot nematode and root rotting fungi on Mungbean. Int. J. Biol. Res. 5, 71–75.
Fatima, Z., Zia, M., and Chaudhary, F. M. (2006). Effect of Rhizobium strains and Phosphorus on growth of soybean (Glycine Max) and survival of Rhizobium and P solubilization bacteria. Pak. J. Bot. 38, 459–464.
Fauvart, M., and Michiels, J. (2008). Rhizobial secreted proteins as determinants of host specificity in the rhizobium-legume symbiosis. FEMS Microbiol. Lett. 285, 1–9. doi: 10.1111/j.1574-6968.2008.01254.x
Ferreira, T. C., Aguilar, J. V., Souza, L. A., Justino, G. C., Aguiar, L. F., and Camargos, L. S. (2016). pH effects on nodulation and biological nitrogen fixation in Calopogonium mucunoides. Revista Brasileira de Botanica. 39, 1015–1020. doi: 10.1007/s40415-016-0300-0
Franke, A. C., van den Brand, G. J., Vanlauwe, B., and Giller, K. E. (2018). Sustainable intensification through rotations with grain legumes in Sub-Saharan Africa: a review. Agri. Ecosyst. Environ. 261, 172–185. doi: 10.1016/j.agee.2017.09.029
Fukuhara, H., Minakawa, Y., Akao, S., and Minamisawa, K. (1994). The involvement of indole-3-acetic acid produced by Bradyrhizobium elkanii in nodule formation. Plant Cell Physiol. 35, 1261–1265. doi: 10.1093/oxfordjournals.pcp.a078722
Getachew, Z., Abera, G., and Beyene, S. (2016). Sulfur fertilizer and rhizobia inoculation improved soybean (Glycine max L. Merill) growth performance and N2 fixation on acidic Nitisols of western Ethiopia. Biology 8, 1–11.
Ghavami, N., Alikhani, H. A., and Pourbabaei, A. A. (2017). Effects of two new siderophore producing rhizobacteria on growth and iron content of maize and canola plants. J. Plant Nutr. 40, 736–746. doi: 10.1080/01904167.2016.1262409
Glazer, A. N., and Nikaido, H. (2007). Microbial Biotechnology: Fundamentals of Applied Microbiology - Alexander N. Glazer, Hiroshi Nikaido - Google Books. Available online at: https://books.google.co.tz/books?hl=en&lr=&id=o3HnX18eU3AC&oi=fnd&pg=PA8&dq=(Glazer+and+Nikaido,+2007)&ots=yZsJ6ZkR44&sig=c_g3FneHhLZfl7sf0aJBuS8h4eo&redir_esc=y#v=onepage&q=(GlazerandNikaido%2C~2007)&f=false (accessed April 22, 2021).
Gómez Padilla, E., Ruiz-Díez, B., Fernández-Pascual, M., López Sánchez, R., Bloem, E., and Eichler-Löbermann, B. (2016). Inoculation with native bradyrhizobia strains improved growth of cowpea plants cultivated on a saline soil. Commun. Soil Sci. Plant Anal. 47, 2218–2224. doi: 10.1080/00103624.2016.1228950
González-Guerrero, M., Matthiadis, A., Sáez, Á., and Long, T. A. (2014). Fixating on metals: new insights into the role of metals in nodulation and symbiotic nitrogen fixation. Front. Plant Sci. 5, 1–6. doi: 10.3389/fpls.2014.00045
Graham, P. H., Draeger, K. J., Ferrey, M. L., Conroy, M. J., Hammer, B. E., Martinez, E., et al. (1994). Acid pH tolerance in strains of Rhizobium and Bradyrhizobium, and initial studies on the basis for acid tolerance of Rhizobium trpici UMR1899. Can. J. Microbiol. 40, 198–207. doi: 10.1139/m94-033
Gransee, A., and Führs, H. (2013). Magnesium mobility in soils as a challenge for soil and plant analysis, magnesium fertilization and root uptake under adverse growth conditions. Plant Soil. 368, 5–21. doi: 10.1007/s11104-012-1567-y
Guan, D., Stacey, N., Liu, C., Wen, J., Mysore, K. S., Torres-Jerez, I., et al. (2013). Rhizobial infection is associated with the development of peripheral vasculature in nodules of Medicago truncatula. Plant Physiol. 162, 107–115. doi: 10.1104/pp.113.215111
Gupta, G., Ahirwar, N. K., Gandhi, M., and Gramoday, C. (2015). Plant Growth Promoting Rhizobacteria (PGPR): current and future prospects microbial & biochemical technology plant growth promoting rhizobacteria (PGPR): current and future prospects for development of sustainable agriculture. J. Microb. Biochem. Technol. 7, 96–102. doi: 10.4172/1948-5948.1000188
Gupta, N., Sabat, J., Parida, R., and Kerkatta, D. (2007). Solubilization of tricalcium phosphate and rock phosphate by microbes isolated from chromite, iron and manganese mines. Acta Botanica Croatica 66, 197–204.
Gyaneshwar, P., Hirsch, A. M., Moulin, L., Chen, W. M., Elliott, G. N., Bontemps, C., et al. (2011). Legume-nodulating betaproteobacteria: diversity, host range, and future prospects. Mol. Plant Microbe Interact. 24, 1276–1288. doi: 10.1094/MPMI-06-11-0172
Gyogluu, C., Jaiswal, S. K., Kyei-Boahen, S., and Dakora, F. D. (2018). Identification and distribution of microsymbionts associated with soybean nodulation in Mozambican soils. Systemat. Appl. Microbiol. 41, 506–515. doi: 10.1016/j.syapm.2018.05.003
Hacisalihoglu, G. (2020). Zinc (Zn): the last nutrient in the alphabet and shedding light on Zn efficiency for the future of crop production under suboptimal Zn. Plants 9, 1–9. doi: 10.3390/plants9111471
Hajjam, Y., and Cherkaoui, S. (2017). The influence of phosphate solubilizing microorganisms on symbiotic nitrogen fixation : perspectives for sustainable agriculture. J. Mater. Environ. Sci. 8, 801–808.
Hallsworth, E. G., Wilson, S. B., and Greenwood, E. A. N. (1960). Copper and cobalt in nitrogen fixation. Nature 8, 8–9. doi: 10.1038/187079a0
Han, L. L., Wang, E. T., Han, T. X., and Liu, J. (2009). Unique community structure and biogeography of soybean rhizobia in the saline-alkaline soils of Xinjiang, China. Plant Soil 324, 291–305. doi: 10.1007/s11104-009-9956-6
Han, Q., Ma, Q., Chen, Y., Tian, B., Xu, L., Bai, Y., et al. (2020). Variation in rhizosphere microbial communities and its association with the symbiotic eficiency of rhizobia in soybean. ISME J. 14, 1915–1928. doi: 10.1038/s41396-020-0648-9
Hartman, G. L., West, E. D., and Herman, T. K. (2011). Crops that feed the World 2. Soybean—worldwide production, use, and constraints caused by pathogens and pests. Food Secur. 3, 5–17. doi: 10.1007/s12571-010-0108-x
Hayashi, M., Shiro, S., Kanamori, H., Mori-hosokawa, S., Sasaki-yamagata, H., Sayama, T., et al. (2014). A thaumatin-like protein, Rj4, controls nodule symbiotic specificity in soybean. Plant Cell Physiol. 55, 1679–1689. doi: 10.1093/pcp/pcu099
Heerwaarden, J., Van Baijukya, F., Kyei-boahen, S., Adjei-nsiah, S., Ebanyat, P., Kamai, N., et al. (2017). Agriculture, ecosystems and environment soyabean response to rhizobium inoculation across sub-Saharan Africa: patterns of variation and the role of promiscuity. Agri. Ecosyst. Environ. 1, 1–8. doi: 10.1016/j.agee.2017.08.016
Hepler, P. K. (2005). Calcium: a central regulator of plant growth and development. Plant Cell 17, 2142–2155. doi: 10.1105/tpc.105.032508
Hollis, A. B., Kloos, W. E., and Elkan, G. . (1981). DNA: DNA hybridization studies of rhizobiumjaponicum and related rhizobiaceae. J. Gen. Microbiol. 123, 215–222. doi: 10.1099/00221287-123-2-215
Htwe, A. Z., Moh, S. M., Soe, K. M., Moe, K., and Yamakawa, T. (2019). Effects of biofertilizer produced from Bradyrhizobium and Streptomyces griseoflavus on plant growth, nodulation, nitrogen fixation, nutrient uptake, and Seed Yield of Mung Bean, Cowpea, and Soybean. Agronomy 9, 1–15. doi: 10.3390/agronomy9020077
Htwe, A. Z., Yamakawa, T., Sarr, P. S., and Sakata, T. (2015). Diversity and distribution of soybean-nodulating bradyrhizobia isolated from major soybean-growing regions in Myanmar. Afri. J. Microbiol. Res. 9, 2183–2196. doi: 10.5897/AJMR2015.7702
Hungria, M., Campo, R. J., Chueire, L. M. O., Grange, L., and Megías, M. (2001). Symbiotic effectiveness of fast-growing rhizobial strains isolated from soybean nodules in Brazil. Biol. Fertil. Soils 33, 387–394. doi: 10.1007/s003740100338
Hungria, M., and Vargas, M. A. T. (2000). Environmental factors affecting N2 xation in grain legumes in the tropics, with an emphasis on Brazil. Field Crops Res. 65, 151–164. doi: 10.1016/S0378-4290(99)00084-2
Idris, O. A. A., and Ahmed, H. S. (2012). Phosphorus sorption capacity as a guide ror phosphorus availability. Afri. Crop Sci. J. 20, 59–65.
Igiehon, N. O., Babalola, O. O., and Aremu, B. R. (2019). Genomic insights into plant growth promoting rhizobia capable of enhancing soybean germination under drought stress. BMC Microbiol. 19, 1–22. doi: 10.1186/s12866-019-1536-1
Islam, M. D. T. (2015). Effects of high temperature on photosynthesis and yield in mungbean. Bangl. J. Bot. 44, 451–454. doi: 10.3329/bjb.v44i3.38553
Iturralde, E. T., Covelli, J. M., Alvarez, F., Pérez-Giménez, J., Arrese-Igor, C., and Lodeiro, A. R. (2019). Soybean-nodulating strains with low intrinsic competitiveness for nodulation, good symbiotic performance, and stress-tolerance isolated from soybean-cropped soils in Argentina. Front. Microbiol. 10, 1–17. doi: 10.3389/fmicb.2019.01061
Jain, P., and Pundir, R. K. (2017). “Recent trends in identification and molecular characterization of rhizobia species,” in Rhizobium Biology and Biotechnology, ed A. P. Hansen (Springer International Publishing AG), 135–164. doi: 10.1007/978-3-319-64982-5_8
Jaiswal, S. K., Mohammed, M., Ibny, F. Y. I., and Dakora, F. D. (2021). Rhizobia as a source of plant growth-promoting molecules: potential applications and possible operational mechanisms. Front. Sustain. Food Syst. 4, 1–14. doi: 10.3389/fsufs.2020.619676
Janczarek, M., Rachwał, K., Marzec, A., Grzadziel, J., and Palusińska-Szysz, M. (2015). Signal molecules and cell-surface components involved in early stages of the legume-rhizobium interactions. Appl. Soil Ecol. 85, 94–113. doi: 10.1016/j.apsoil.2014.08.010
Jarvis, B. D. W., Berkum, P. V. A. N., Chen, W. X., Nour, S. M., Fernandez, M. P., and Gillis, M. (1997). Transfer of Rhizobium loti, Rhizobium huakuii, Rhizobium ciceri, Rhizobium mediterraneum, and Rhizobium tianshanense to Mesorhizobium gen. nov. Int. J. Systemat. Bacteriol. 3, 895–898. doi: 10.1099/00207713-47-3-895
Jarvis, B. D. W., Pankhurst, C. E., and Patel, J. J. (1982). Rhizobiurn loti, a new species of legume root nodule bacteria. Int. J. Systemat. Bacteriol. 3, 378–380. doi: 10.1099/00207713-32-3-378
Jida, M., and Assefa, F. (2012). Phenotypic diversity and plant growth promoting characteristics of Mesorhizobium species isolated from chickpea (Cicer arietinum L.) growing areas of Ethiopia. J. Biotechnol. 11, 7483–7493. doi: 10.5897/AJB11.4109
Jordan, D. C. (1982). Transfer of Rhizobium japonicum Buchanan 1980 to Bradyrhizobium gen. nov., a genus of slow-growing, root nodule bacteria from leguminous plants. Int. J. Systemat. Bacteriol. 32, 136–139. doi: 10.1099/00207713-32-1-136
Kalkar, S. A. (2014). Potassium solublisers: occurrence, mechanism and their role as competent biofertilizers original research article potassium solublisers: occurrence, mechanism and their role as competent biofertilizers. Int. J. Curr. Microbiol. Appl. Sci. 3, 622–629.
Kapembwa, R., Mweetwa, A. M., Ngulube, M., Yengwe, J., and Yengwe, J. (2016). Morphological and biochemical characterization of soybean nodulating rhizobia indigenous to Zambia. Sustain. Agri. Res. 5:84. doi: 10.5539/sar.v5n3p84
Kapri, A., and Tewari, L. (2010). Phosphate solubilization potential and phosphatase activity of rhizospheric Trichoderma SPP. Brazil. J. Microbiol. 41:5000001. doi: 10.1590/S1517-83822010005000001
Kassam, A. H. (2019). World Resources 2000–2001: People and Ecosystems: The Fraying Web of Life. Washington, DC: World Resources Institute, 389, GBP 22.95. ISBN 1-56973-443-7. 38(2002), 111–113. doi: 10.1017/S0014479702210194
Khaitov, B. (2018). Effects of Rhizobium inoculation and magnesium application on growth and nodulation of soybean (Glycine max L.). J. Plant Nutr. 41, 2057–2068. doi: 10.1080/01904167.2018.1485164
Khalid, R., Zhang, X. X., Hayat, R., and Ahmed, M. (2020). Molecular characteristics of rhizobia isolated from arachis hypogaea grown under stress environment. Sustainability 12, 1–15. doi: 10.3390/su12156259
Khan M. S., Zaidi A., and Ahmad E. (2014b). “Mechanism of phosphate solubilization and physiological functions of phosphate-solubilizing microorganisms,” in Phosphate Solubilizing Microorganisms, eds M. Khan, A. Zaidi, and J. Musarrat (Cham: Springer). doi: 10.1007/978-3-319-08216-5_2
Khan, N., Tariq, M., Ullah, K., Muhammad, D., Khan, I., Rahatullah, K., et al. (2014a). The effect of molybdenum and iron on nodulation, nitrogen fixation and yield of chickpea genotypes (Cicer Arietinum L.). IOSR J. Agri. Vet. Sci. 7, 63–79. doi: 10.9790/2380-07136379
Khandelwal, S. R., Manwar, A. V., Chaudhari, B. L., and Chincholkar, S. B. (2002). Siderophoregenic Bradyrhizobia boost yield of soybean. Appl. Biochem. Biotechnol. A Enzyme Eng. Biotechnol. 102–103, 155–168. doi: 10.1385/ABAB:102-103:1-6:155
Khansole, G. (2016). Morphological, cultural and biochemical characteristics of Rhizobium japonicum syn and Bradyrhizobium japonicum of soybean. Biosci. Discov. 2, 1–5.
Kidd, P. S., and Proctor, J. (2001). Why plants grow poorly on very acid soils : are ecologists missing the obvious? J. Exp. Bot. 52:357. doi: 10.1093/jexbot/52.357.791
Kim, D. H., Kaashyap, M., Rathore, A., Das, R. R., Parupalli, S., Upadhyaya, H. D., et al. (2014). Phylogenetic diversity of Mesorhizobium in chickpea. J. Biosci. 39, 513–517. doi: 10.1007/s12038-014-9429-9
Kiss, S. A., Stefanovits-Bányai, E., and Takács-Hájos, M. (2004). Magnesium-content of rhizobium nodules in different plants: the importance of magnesium in nitrogen-fixation of nodules. J. Am. Coll. Nutr. 23, 751–753. doi: 10.1080/07315724.2004.10719422
Kober, M. D. V., and Giongo, A. (2004). Characterization of variants of Bradyrhizobium elkanii and B. japonicum and symbiotic behaviour in soybeans. Sci. Rural 34, 1459–1464. doi: 10.1590/S0103-84782004000500020
Kumari, B. S., Ram, M. R., and Mallaiah, K. V. (2010). Studies on nodulation, biochemical analysis and protein profile of Rhizobium isolated from Indigofera species. Malays. J. Microbiol. 6, 133–139. doi: 10.21161/mjm.20109
Kunert, K. J., Vorster, B. J., Fenta, B. A., Kibido, T., and Davis, T. S. (2016). Drought stress responses in soybean roots and nodules. Front. Plant Sci. 7, 1–7. doi: 10.3389/fpls.2016.01015
Lajudie, P. D. E., Willems, A., Pot, B., Dewettinck, D., Maestrojuan, G., Ney, M., et al. (1994). Polyphasic taxonomy of rhizobia : emendation of the genus sinorhizobium and description of Sinorhizobium meliloti and Sinorhizobium teranga sp. nov. Int. J. Systemat. Bacteriol. 44, 715–733. doi: 10.1099/00207713-44-4-715
Lalita, S., and Kumar, A. (2017). Review article influence of micronutrients (molybdenum and iron) applied in combination with rhizobium on biochemical parameters of Vigna radiata (L.). Int. J. Curr. Res. 9, 59639–59643.
Lambrecht, M., Okon, Y., Broek, A., and Vanderleyden, J. (2000). Indole-3-acetic acid : a reciprocal signalling molecule in bacteria – plant interactions. Trends Microbiol. 8, 299–300. doi: 10.1016/S0966-842X(00)01732-7
Lavres, J., Franco, G. C., and de Sousa Câmara, G. M. (2016). Soybean seed treatment with nickel improves biological nitrogen fixation and urease activity. Front. Environ. Sci. 4, 1–11. doi: 10.3389/fenvs.2016.00037
Lea, P. J., and Miflin, B. J. (2018). Nitrogen assimilation and its relevance to crop improvement. Ann. Plant Rev. Online 42, 1–40. doi: 10.1002/9781119312994.apr0448
Lebrazi, S., Chraibi, M., Fadil, M., Barkai, H., and Fikri-Benbrahim, K. (2018). Phenotypic, genotypic and symbiotic characterization of rhizobial isolates Nodulating acacia sp. in Morocco. J. Pure Appl. Microbiol. 12, 249–263. doi: 10.22207/JPAM.12.1.30
Leite, J., Ribeiro, S., Simões-araújo, J. L., Gouvêa, N., Ribeiro, G., and Édson, J. (2017). Genomic identification and characterization of the elite strains Bradyrhizobium yuanmingense BR 3267 and Bradyrhizobium pachyrhizi BR 3262 recommended for cowpea inoculation in Brazil. Brazili. J. Microbiol. 49, 703–713. doi: 10.1016/j.bjm.2017.01.007
Li, Q. Q., Wang, E. T., Chang, Y. L., Zhang, Y. Z., Sui, X. H., Chen, W. F., et al. (2011a). Ensifer sojae sp. nov., isolated from root nodules of Glycine max grown in saline-alkaline soils. Int. J. Systemat. Evolut. Microbiol. 61, 1981–1988. doi: 10.1099/ijs.0.025049-0
Li, Q. Q., Wang, E. T., Zhang, Y. Z., Zhang, Y. M., Tian, C. F., Sui, X. H., et al. (2011b). Diversity and biogeography of rhizobia isolated from root nodules of Glycine max grown in Hebei Province, China. Microbial. Ecol. 61, 917–931. doi: 10.1007/s00248-011-9820-0
Lindstrom, K., and Mousavi, S. A. (2020). Effectiveness of nitrogen fixation in rhizobia. Microbial. Biotechnol. 13, 1314–1335. doi: 10.1111/1751-7915.13517
Liu, A., Contador, C. A., Fan, K., Lam, H., Dicenzo, G. C., and Dunn, M. F. (2018). Interaction and regulation of carbon, nitrogen, and phosphorus metabolisms in root nodules of legumes. Front. Plant Sci. 9, 1–18. doi: 10.3389/fpls.2018.01860
Liu, Y., Wu, L., Baddeley, J. A., and Watson, C. A. (2009). Models of biological nitrogen fixation of legumes. Sustain. Agri. 2, 883–905. doi: 10.1007/978-94-007-0394-0_39
Long, S. P., Ainsworth, E. A., Rogers, A., and Ort, D. R. (2004). Rising atmospheric carbon dioxide: plants FACE the future. Ann. Rev. Plant Biol. 55, 591–628. doi: 10.1146/annurev.arplant.55.031903.141610
Lorito, M., and Woo, S. (2015). “Trichoderma: A multi-purpose tool for integrated pest management,” in Principles of Plant-Microbe Interactions, eds B. Lugtenberg (Cham: Springer). doi: 10.1007/978-3-319-08575-3_36
Lucrecia, M., Ramos, G., Parsons, R., Sprent, J. I., and James, E. K. (2003). Effect of water stress on nitrogen fixation and nodule structure of common bean. Plant Physiol. 38, 339–347. doi: 10.1590/S0100-204X2003000300002
Mabood, F., and Smith, D. L. (2005). Pre-incubation of Bradyrhizobium japonicum with jasmonates accelerates nodulation and nitrogen fixation in soybean (Glycine max) at optimal and suboptimal root zone temperatures. Physiol. Plant. 125, 311–323. doi: 10.1111/j.1399-3054.2005.00559.x
Mahavidyalaya, R. S. (2013). Isolation of rhizobia from soybean cultivated in latur area & study of its phosphate solubilization activity. Biosci. Discov. 4, 100–103.
Malhotra, H., Sharma, S. V., and Pandey, R. (2018). “Nutrients and abiotic stress tolerance,” in Nutrients and Abiotic Stress Tolerance, eds M. Hasanuzzaman, M. Fujita, H. Oku, K. Nahar, and B. Hawrylak-Nowak (Singapore: Springer Nature Singapore Pte Ltd). p. 171–190. doi: 10.1007/978-981-10-9044-8
Mallaiah, M. S. K. V. (2009). Phosphate solubilization by Rhizobium strains. Ind. J. Microbiol. 49, 98–102. doi: 10.1007/s12088-009-0005-1
Mandal, S. M., Chakraborty, D., Dey, S., Mandal, S. M., Chakraborty, D., and Dey, S. (2010). Phenolic acids act as signaling molecules in plant- microbe symbioses Phenolic acids act as signaling molecules in plant-microbe symbioses. Plant Signal. Behav. 5, 359–368. doi: 10.4161/psb.5.4.10871
Maougal, R. T., Brauman, A., Plassard, C., Abadie, J., Djekoun, A., and Drevon, J. (2014). Bacterial capacities to mineralize phytate increase in the rhizosphere of nodulated common bean (Phaseolus vulgaris) under P deficiency. Eur. J. Soil Biol. 62, 8–14. doi: 10.1016/j.ejsobi.2014.02.006
Maria, Â., Chueire, D. O., Coca, R. G., Megõ, M., and Hungria, M. (2001). Preliminary characterization of fast growing rhizobial strains isolated from soyabean nodules in Brazil. Soil Biol. Biochem. 33, 1349–1361. doi: 10.1016/S0038-0717(01)00040-2
Marino, D., Damiani, I., Gucciardo, S., Mijangos, I., Pauly, N., and Puppo, A. (2013). Inhibition of nitrogen fixation in symbiotic Medicago truncatula upon Cd exposure is a local process involving leghaemoglobin. J. Exp. Bot. 64, 5651–5660. doi: 10.1093/jxb/ert334
Mario, J. R., Lira, A., Nascimento, L. R. S., and Fracetto, G. G. M. (2015). Legume-rhizobia signal exchange: promiscuity and environmental effects. Front. Microbiol. 6, 1–9. doi: 10.3389/fmicb.2015.00945
Martinez-romero, E., Segovia, L., Mercante, M., Franc, A. A., Graham, P., and Pardo, M. A. (1991). Rhizobium tropici, a novel species nodulating Phaseolus vulgaris L. Beans and Leucaena sp. trees. Int. J. Systemat. Bacteriol. 41, 417–426. doi: 10.1099/00207713-41-3-417
Masunga, R. H., Uzokwe, V. N., Mlay, P. D., Odeh, I., Singh, A., Buchan, D., et al. (2016). Nitrogen mineralization dynamics of different valuable organic amendments commonly used in agriculture. Appl. Soil Ecol. 101, 185–193. doi: 10.1016/j.apsoil.2016.01.006
Mathew, A., Eberl, L., and Carlier, A. L. (2014). A novel siderophore-independent strategy of iron uptake in the genus Burkholderia. Mol. Microbiol. 91, 805–820. doi: 10.1111/mmi.12499
Mathu, S., Herrmann, L., Pypers, P., Matiru, V., Lesueur, D., Mwirichia, R., and Lesueur, D. (2012). Potential of indigenous bradyrhizobia versus commercial inoculants to improve cowpea (Vigna unguiculata L. walp.) and green gram (Vigna radiata L. wilczek.) yields in Kenya Potential of indigenous bradyrhizobia. Soil Sci. Plant Nutr. 58:763. doi: 10.1080/00380768.2012.741041
Mbene, K., Suhtening, A., Suh, C. E., Fomenky, N. N., and Che, V. B. (2017). Phosphorus fixation and its relationship with physicochemical properties of soils on the Eastern flank of Mount Cameroon. Afri. J. Agri. Res. 12, 2742–2753. doi: 10.5897/AJAR2017.12530
Mclaughlin, T. M. M. M. J. (2014). Efficacy of zinc oxides as fertilisers Plant Soil 374, 843–855. doi: 10.1007/s11104-013-1919-2
Meena, V. S., Maurya, B. R., Verma, J. P., Aeron, A., Kumar, A., Kim, K., et al. (2015). Potassium solubilizing rhizobacteria (KSR): isolation, identification, and K-release dynamics from waste mica. Ecol. Eng. 81, 340–347. doi: 10.1016/j.ecoleng.2015.04.065
Mendel, R. R. (2013). The molybdenum cofactor. J. Biol. Chem. 288, 13165–13172. doi: 10.1074/jbc.R113.455311
Merrill, L., Agoyi, E. E., Odong, T. L., Tumuhairwe, J. B., Chigeza, G., and Diers, B. W. (2017). Genotype by environment effects on promiscuous nodulation in soybean (Glycine max). Agri. Food Secur. 29, 1–14. doi: 10.1186/s40066-017-0107-7
Mfilinge, A., Mtei, K., and Ndakidemi, P. A. (2014). Effects of Rhizobium inoculation and supplementation with P and K, on growth, leaf chlorophyll content and nitrogen fixation of bush bean varieties. Am. J. Res. Commun. 2, 49–87.
Mimmo, T., Del Buono, D., Terzano, R., Tomasi, N., Vigani, G., Crecchio, C., et al. (2014). Rhizospheric organic compounds in the soil-microorganism-plant system: their role in iron availability. Eur. J. Soil Sci. 65, 629–642. doi: 10.1111/ejss.12158
Miransari, M., and Smith, D. L. (2009). Original article alleviating salt stress on soybean (Glycine max L. Merr.) – Bradyrhizobium japonicum symbiosis, using signal molecule genistein. Eur. J. Soil Biol. 45, 146–152. doi: 10.1016/j.ejsobi.2008.11.002
Miwa, H., Sun, J., Oldroyd, G. E. D., and Downie, J. A. (2006). Analysis of Nod-factor-induced calcium signaling in root hairs of symbiotically defective mutants of Lotus japonicus. Mol. Plant-Microbe Interact. 19, 914–923. doi: 10.1094/MPMI-19-0914
Mmbaga, G. W., Mtei, K. M., and Ndakidemi, P. A. (2014). Extrapolations on the use of rhizobium inoculants supplemented with phosphorus (P) and potassium (K) on growth and nutrition of legumes. Agri. Sci. 05, 1207–1226. doi: 10.4236/as.2014.512130
Mnasri, B., Aouani, M. E., and Mhamdi, R. (2007). Nodulation and growth of common bean (Phaseolus vulgaris) under water deficiency. Soil Biol. Biochem. 39, 1744–1750. doi: 10.1016/j.soilbio.2007.01.030
Mohammadi, K., Sohrabi, Y., Heidari, G., and Khalesro, S. (2012). Effective factors on biological nitrogen fixation. Afri. J. Agri. Res. 7, 1782–1788. doi: 10.5897/AJARX11.034
Monica, N., Vidican, R., Pop, R., and Rotar, I. (2013). Stress factors affecting symbiosis activity and nitrogen fixation by rhizobium cultured in vitro. Environ 6, 42–45.
Mousavi, S. R., Galavi, M., and Rezaei, M. (2013). Zinc (Zn) importance for crop production - a review zinc (Zn) importance for crop production – a review. Int. J. Agron. Plant Prod. 4, 64–68.
Muiru, W. M., Mutitu, E. W., and Kimenju, J. W. (2010). Use of Repetitive Extragenic Palindromic (REP), Enterobacterial Repetitive Intergenic Consensus (ERIC) and BOX sequences to fingerprint Exserohilum turcicum isolates. J. Appl. Biosci., 1828–1838.
Musiyiwa, K., Mpepereki, S., and Giller, K. E. (2005). Symbiotic effectiveness and host ranges of indigenous rhizobia nodulating promiscuous soyabean varieties in Zimbabwean soils. Soil Biol. Biochem. 37, 1169–1176. doi: 10.1016/j.soilbio.2004.12.004
Naamala, J., Jaiswal, S. K., and Dakora, F. D. (2016). Antibiotics resistance in rhizobium: type, process, mechanism and benefit for agriculture. Curr. Microbiol. 72, 804–816. doi: 10.1007/s00284-016-1005-0
Nagalingam, S., Nithya, T. V., Gayathri, D., Sagarika, A. S., Supriya, G., Vidya, D., et al. (2020). Morphological, biochemical and plant growth promoting characterization of rhizobia isolated from root nodule of cajanus cajan l. Plant Archiv. 20, 1293–1299.
Nahrawy, S. E.-, Elbagory, M., and Omara, A. E.-D. (2020). Biocompatibility effect of Bradyrhizobium japonicum and trichoderma strains on growth, nodulation and physiological traits of soybean (Glycine max L.) under water deficit conditions. J. Adv. Microbiol. 20, 52–66. doi: 10.9734/jamb/2020/v20i1130300
Naz, I., and Bano, A. (2009). Morphological, biochemical and molecular characterization of rhizobia from halophytes of khewra salt range and attock. Pak. J. Bot. 41, 3159–3168.
Ndusha, B. N., Onwonga, R. N., Nabahungu, L. S., Mushagalusa, N. G., Matendo, R. E., and Keya, S. O. (2019). Indigenous rhizobia strains: the silver bullet for enhanced biological nitrogen fixation and soybean (Glycine max L. Merr.) yield under different soil conditions in South Kivu province, Democratic Republic of Congo. Afri. J. Agri. Res. 14, 2038–2047. doi: 10.5897/AJAR2019.14457
Niehus, R., Picot, A., Oliveira, N. M., Mitri, S., and Foster, K. R. (2017). The evolution of siderophore production as a competitive trait. Evolution 71, 1443–1455. doi: 10.1111/evo.13230
Nocker, A., Krstulovic, N. P., Perret, X., and Narberhaus, F. (2001). ROSE elements occur in disparate rhizobia and are functionally interchangeable between species. Arch. Microbiol. 176, 44–51. doi: 10.1007/s002030100294
Ntambo, M. S., Chilinda, I. S., Taruvinga, A., Hafeez, S., Anwar, T., Sharif, et al. (2017). The effect of Rhizobium inoculation with nitrogen fertilizer on growth and yield of soybeans (Glycine max L.). Int. J. Biosci. 10, 163–172. doi: 10.12692/ijb/10.3.163-172
Nyaguthii, M. C. (2017). Soybean (Glycine max) Response to Rhizobia Inoculation as Influenced by soil Nitrogen Levels. Nairobi: Kenyatta University.
Oburger, E., Cid, C. V., Preiner, J., Hu, J., Hann, S., Wanek, W., et al. (2018). pH-dependent bioavailability, speciation, and phytotoxicity of tungsten (W) in soil affect growth and molybdoenzyme activity of nodulated soybeans. Environ. Sci. Technol. 52, 6146–6156. doi: 10.1021/acs.est.7b06500
Odee, D. W., Sutherland, J. M., Makatiani, E. T., Mcinroy, S. G., and Sprent, J. I. (1997). Phenotypic characteristics and composition of rhizobia associated with woody legumes growing in diverse Kenyan conditions. Plant Soil 188, 65–75. doi: 10.1023/A:1004204413140
O'Hara, G. W. (2001). Nutritional constraints on root nodule bacteria affecting symbiotic nitrogen fixation: a review. Austr. J. Exp. Agri. 41, 417–433. doi: 10.1071/EA00087
Ojha, A., Tak, N., Rathi, S., Chouhan, B., Rao, S. R., Barik, S. K., et al. (2020). Molecular characterization of novel Bradyrhizobium strains nodulating Eriosema chinense and Flemingia vestita, important unexplored native legumes of the sub-. Systemat. Appl. Microbiol. 1, 1–4. doi: 10.1016/j.syapm.2017.06.003
Okazaki, S., Kaneko, T., Sato, S., and Saeki, K. (2013). Hijacking of leguminous nodulation signaling by the rhizobial type III secretion system. Proc. Natl. Acad. Sci. U. S. A. 110, 17131–17136. doi: 10.1073/pnas.1302360110
Okereke, G. U., and Onochie, C. C. (1996). Screening of native and foreign Bradyrhizobium japonicum strains for high N2 fixation in soybean. World J. Microbiol. Biotechnol. 12, 639–641. doi: 10.1007/BF00327729
Oldroyd, G. E. D., and Downie, J. A. (2004). Calcium, kinases and nodulation signalling in legumes. Nat. Rev. Mol. Cell Biol. 5, 566–576. doi: 10.1038/nrm1424
Oldroyd, G. E. D., Murray, J. D., Poole, P. S., and Downie, J. A. (2011). The rules of engagement in the legume-rhizobial symbiosis. Ann. Rev. Genet. Is 45, 119–144. doi: 10.1146/annurev-genet-110410-132549
Oliveira, A. N., De Oliveira, L. A., and De Andrade, J. S. (2010). Production and some properties of crude alkaline proteases of indigenous central amazonian rhizobia strains. Food/Feed Sci. Technol. 53, 1185–1195. doi: 10.1590/S1516-89132010000500024
Ondieki, D. K., Nyaboga, E. N., Wagacha, J. M., and Mwaura, F. B. (2017). Morphological and genetic diversity of rhizobia nodulating cowpea (Vigna unguiculata L.) from agricultural soils of lower Eastern Kenya. Int. J. Microbiol. 1, 1–10. doi: 10.1155/2017/8684921
Ono, Y., Fukasawa, M., Sueyoshi, K., Ohtake, N., Sato, T., and Tanabata, S. (2021). Application of nitrate, ammonium, or urea changes the concentrations of ureides, urea, amino acids and other metabolites in xylem sap and in the organs of soybean plants (Glycine max L. Merr.). Int. J. Mol. Sci. 22, 1–27. doi: 10.3390/ijms22094573
Parveen, G., Noreen, R., Shafique, H. A., Sultana, V., Ehteshamul-Haque, S., and Athar, M. (2019). Role of rhizobia in suppressing the root diseases of soybean under soil amendment. Planta Daninha 37, 1–8. doi: 10.1590/s0100-83582019370100038
Pasley, H. R., Cairns, J. E., Camberato, J. J., and Vyn, T. J. (2019). Nitrogen fertilizer rate increases plant uptake and soil availability of essential nutrients in continuous maize production in Kenya and Zimbabwe. Nutrient Cycl. Agroecosyst. 115, 373–389. doi: 10.1007/s10705-019-10016-1
Patel, A., Vyas, R. V., Mankad, M., and Subhash, N. (2017). Isolation and biochemical characterization of rhizobia from rice rhizosphere and their effect on rice growth promotion. Int. J. Pure Appl. Biosci. 5, 441–451. doi: 10.18782/2320-7051.5439
Paudyal, S. P., Aryal, R. R., Maheshwari, D. K., Campus, T., Buddha, L., and Foundation, E. (2007). Effect of heavy metals on growth of rhizobium strains and symbiotic efficiency of two species of tropical legumes. Sci. World 5, 27–32. doi: 10.3126/sw.v5i5.2652
Peng, W. T., Zhang, L. D., Zhou, Z., Fu, C., Chen, Z. C., and Liao, H. (2018). Magnesium promotes root nodulation through facilitation of carbohydrate allocation in soybean. Physiol. Plant. 163, 372–385. doi: 10.1111/ppl.12730
Penn, C. J. (2019). A critical review on soil chemical processes that control how soil pH affects phosphorus availability to plants. Agriculture 9, 1–18. doi: 10.3390/agriculture9060120
Poonam, K. C. K., Asmita, S., Inderjeet, S., Gill, S. B. S., Singh, U., and Saharan, K. (2019). Synergism of Pseudomonas aeruginosa (LSE-2) nodule endophyte with Bradyrhizobium sp. (LSBR-3) for improving plant growth, nutrient acquisition and soil health in soybean. World J. Microbiol. Biotechnol. 35, 2–17. doi: 10.1007/s11274-019-2622-0
Qudratullah Oryakhil, M. A. I. (2020). Morphological biochemical and plant growth promoting characterization of rhizobia isolated from root nodule of cicer. Trop. Agroecosyst. 1, 59–63. doi: 10.26480/taec.02.2020.59.63
Radutoiu, S., Madsen, L. H., Madsen, E. B., Felle, H. H., Umehara, Y., Grønlund, M., et al. (2003). Plant recognition of symbiotic bacteria requires two LysM receptor-like kinases. Nature 425, 585–592. doi: 10.1038/nature02039
Radutoiu, S., Madsen, L. H., Madsen, E. B., Jurkiewicz, A., Fukai, E., Quistgaard, E. M. H., et al. (2007). LysM domains mediate lipochitin-oligosaccharide recognition and Nfr genes extend the symbiotic host range. EMBO J. 26, 3923–3935. doi: 10.1038/sj.emboj.7601826
Raimi, A., Adeleke, R., and Roopnarain, A. (2017). Soil fertility challenges and Biofertiliser as a viable alternative for increasing smallholder farmer crop productivity in sub-Saharan Africa. Cogent Food Agri. 3, 1–26. doi: 10.1080/23311932.2017.1400933
Ramakrishnan, V. V. G. A. (2015). Nitrogen sources and cycling in the ecosystem and its role in air, water and soil pollution: a critical review. J. Poll. Effects Control 3, 1–28. doi: 10.4172/2375-4397.1000136
Ramesh, A., and Winkler, W. C. (2010). Magnesium-sensing riboswitches in bacteria. RNA Biol. 7, 77–83. doi: 10.4161/rna.7.1.10490
Rampa, T. K., Kunert, K. J., Makgopa, M., and Greve, M. (2019). Improvement of rhizobium - soybean symbiosis and nitrogen fixation under drought. Food Energy Securitynergy Secur. 1, 1–14. doi: 10.1002/fes3.177
Rani, N., Kaur, R., and Kaur, S. (2020). Zinc solubilizing bacteria to augment Soil Fertility – a comprehensive review. Int. J. Agri. Sci. Vet. Med. 8, 38–44.
Ray, J. D., Heatherly, L. G., and Fritschi, F. B. (2006). Influence of large amounts of nitrogen on nonirrigated and irrigated soybean. Crop Sci. 46, 52–60. doi: 10.2135/cropsci2005.0043
Reckling, M., Omari, R. A., Bellingrath-kimura, S. D., Bachinger, J., and Bloch, R. (2021). Soybean nodulation response to cropping interval and inoculation in european cropping systems. Front. Plant Sci. 12, 1–9. doi: 10.3389/fpls.2021.638452
Ribeiro, R. A., and Melo, I. S. (2013). Polyphasic evidence supporting the reclassification of Bradyrhizobium japonicum group Ia strains as. Int. J. Systemat. Evol. Microbiol. 63, 3342–3351. doi: 10.1099/ijs.0.049130-0
Richardson, A. E., Hocking, P. J., Simpson, R. J., and George, T. S. (2009). Plant mechanisms to optimise access to soil phosphorus. Crop Pasture Sci. 60, 124–143. doi: 10.1071/CP07125
Rodríguez-Navarro, D. N., Bellogín, R., Camacho, M., Daza, A., Medina, C., Ollero, F. J., et al. (2003). Field assessment and genetic stability of Sinorhizobium fredii strain SMH12 for commercial soybean inoculants. Eur. J. Agron. 19, 299–309. doi: 10.1016/S1161-0301(02)00076-X
Rodriguez-Navarro, D. N., Oliver, I. M., Contreras, M. A., and Ruiz-Sainz, J. E. (2011). Review article soybean interactions with soil microbes, agronomical and molecular aspects. Agron. Sustain. Dev. 31, 173–190. doi: 10.1051/agro/2010023
Roem, W. J., and Berendse, F. (2000). Soil acidity and nutrient supply ratio as possible factors determining changes in plant species diversity in grassland and heathland communities. Biol. Conserv. 92, 151–161. doi: 10.1016/S0006-3207(99)00049-X
Ronald, P. (2011). Plant genetics, sustainable agriculture and global food security. Genetics 188, 11–20. doi: 10.1534/genetics.111.128553
Rotaru, V., and Sinclair, T. R. (2009). Interactive influence of phosphorus and iron on nitrogen fixation by soybean. Remote Sens. Environ. 66, 94–99. doi: 10.1016/j.envexpbot.2008.12.001
Saeki, Y., Kaneko, A., Hara, T., Suzuki, K., Yamakawa, T., Minh, T. N., et al. (2005). Phylogenetic analysis of soybean-nodulating rhizobia isolated from alkaline soils in Vietnam. Soil Sci. Plant Nutr. 51, 1043–1052. doi: 10.1111/j.1747-0765.2005.tb00143.x
Salvagiotti, F., Cassman, K. G., Specht, J. E., Walters, D. T., Weiss, A., and Dobermann, A. (2008). Nitrogen uptake, fixation and response to fertilizer N in soybeans: a review. Field Crops Res. 108, 1–13. doi: 10.1016/j.fcr.2008.03.001
Sannazzaro, A. I., Bergottini, V. M., Paz, R. C., Castagno, L. N., Menéndez, A. B., Ruiz, O. A., et al. (2011). Comparative symbiotic performance of native rhizobia of the Flooding Pampa and strains currently used for inoculating Lotus tenuis in this region. Antonie van Leeuwenhoek 99, 371–379. doi: 10.1007/s10482-010-9502-9
Santachiara, G., Salvagiotti, F., and Rotundo, J. L. (2019). Nutritional and environmental effects on biological nitrogen fixation in soybean : a meta-analysis. Field Crops Res. 240, 106–115. doi: 10.1016/j.fcr.2019.05.006
Santos, M. (2019). Soybean varieties in sub-Saharan Africa.Afri. J Food Agri. Nutr. Develop. 19, 15136–5139. doi: 10.18697/ajfand.88.SILFarmDoc06
Sattar, A., Naveed, M., Ali, M., Zahir, Z. A., Nadeem, S. M., Yaseen, M., et al. (2019). Perspectives of potassium solubilizing microbes in sustainable food production system: a review. Appl. Soil Ecol. 133, 146–159. doi: 10.1016/j.apsoil.2018.09.012
Saturno, D. F., Cerezini, P., Moreira da Silva, P., Oliveira, A. B., de Oliveira, M. C. N., de Hungria, M., et al. (2017). Mineral nitrogen impairs the biological nitrogen fixation in soybean of determinate and indeterminate growth types. J. Plant Nutr. 40, 1690–1701. doi: 10.1080/01904167.2017.1310890
Scholla, M. H., and Elkan, G. H. (1984). Rhizobium fredii sp. nov. a fast-growing species that effectively nodulates soybeans. Int. J. Systemat. Bacteriol. 34, 484–486. doi: 10.1099/00207713-34-4-484
Sessitsch, A., Kuffner, M., Kidd, P., Vangronsveld, J., Wenzel, W. W., Fallmann, K., et al. (2013). The role of plant-associated bacteria in the mobilization and phytoextraction of trace elements in contaminated soils. Soil Biol. Biochem. 60, 182–194. doi: 10.1016/j.soilbio.2013.01.012
Shamseldin, A., and Werner, D. (2005). High salt and high pH tolerance of new isolated Rhizobium etli strains from Egyptian soils. Curr. Microbiol. 50, 11–16. doi: 10.1007/s00284-004-4391-7
Sharma, M. P., Srivastava, K., and Sharma, S. K. (2010). Biochemical characterization and metabolic diversity of soybean rhizobia isolated from Malwa region of central India. Plant Soil Environ. 56, 375–383. doi: 10.17221/247/2009-PSE
Sherwood, M. T. (1970). Improved synthetic medium for the growth of rhizobium. J. Appl. Bacteriol. 33, 708–713. doi: 10.1111/j.1365-2672.1970.tb02253.x
Shi, L., Antonio, C., and Hugo, F. (2002). Diversity and symbiotic effectiveness of rhizobia isolated from field-grown soybean nodules in Paraguay. Biol. Fertil. Soils 35, 448–457. doi: 10.1007/s00374-002-0493-1
Shiro, S., Matsuura, S., Saiki, R., Sigua, G. C., Yamamoto, A., Umehara, Y., et al. (2013). Genetic diversity and geographical distribution of indigenous soybean-nodulating bradyrhizobia in the United States. Appl. Environ. Microbiol. 79, 3610–3618. doi: 10.1128/AEM.00236-13
Siczek, A., and Lipiec, J. (2011). Soybean nodulation and nitrogen fixation in response to soil compaction and surface straw mulching. Soil Tillage Res. 114, 50–56. doi: 10.1016/j.still.2011.04.001
Simon, Z., Mtei, K., Gessesse, A., and Ndakidemi, P. A. (2014). Isolation and characterization of nitrogen fixing rhizobia from cultivated and uncultivated soils of northern tanzania. Am. J. Plant Sci. 5, 4050–4067. doi: 10.4236/ajps.2014.526423
Singh, A. K., Prasad Bhatt, R., Pant, S., Kaur Bedi, M., and Naglot, A. (2011). Characterization of Rhizobium isolated from root nodules of Trifolium alexandrinum. J. Agri. Technol. 7, 1705–1723.
Singh, B., Kaur, R., and Singh, K. (2008). Characterization of Rhizobium strain isolated from the roots of Trigonella foenumgraecum (fenugreek). Afri. J. Biotechnol. 7, 3674–3679.
Singh, R. K., and Rao, P. J. M. (1997). Biological significance of nickel on the nitrogen fixing ability of cowpea Bradyrhizobium. J. Plant Nutr. 20, 1449–1455. doi: 10.1080/01904169709365346
Singha, B., Das, P., and Mazumder, P. B. (2013). Morphological and biochemical characterization of rhizobia isolated from root nodule of Crotolaria junceae L. grown in Assam. Int. J. Sci. Res. 14, 2319–7064.
Slattery, J. F., Coventry, D. R., and Slattery, W. J. (2001). Rhizobial ecology as affected by the soil environment. Austr. J. Exp. Agri. 41, 289–298. doi: 10.1071/EA99159
Smith, F. W., Rae, A. L., and Hawkesford, M. J. (2000). Molecular mechanisms of phosphate and sulphate transport in plants. Biochem. Biophys. Acta 1465, 236–245. doi: 10.1016/S0005-2736(00)00141-3
Snapp, S., Rahmanian, M., and Batello, C. (2018). Pulse crops for sustainable farms in Sub-Saharan Africa. UN-Library 57:6795bfaf-en. doi: 10.18356/6795bfaf-en
Stark, S., Eskelinen, A., and Ma, M. K. (2012). Regulation of microbial community composition and activity by soil nutrient availability, soil pH, and herbivory in the Tundra. Ecosystems 15, 18–33. doi: 10.1007/s10021-011-9491-1
Suhag, M. (2016). Potential of biofertilizers to replace chemical fertilizers. Int. Adv. Res. J. Sci. Eng. Technol. 3, 163–167. doi: 10.17148/IARJSET.2016.3534
Suzuki, K., Oguro, H., Yamakawa, T., Yamamoto, A., Akao, S., and Saeki, Y. (2008). Diversity and distribution of indigenous soybean-nodulating rhizobia in the Okinawa islands, Japan. Soil Sci. Plant Nutr. 54, 237–246. doi: 10.1111/j.1747-0765.2007.00236.x
Tadele, Z. (2017). Raising crop productivity in Africa through intensification. Agronomy 7, 1–30. doi: 10.3390/agronomy7010022
Tajima, S., Hirashita, T., Yoshihara, K., Bhromsiri, A., and Nomura, M. (2000). Application of repetitive extragenic palindromic (REP)-PCR and enterobacterial repetitive intergenic consensus (ERIC)-PCR analysis to the identification and classification of Japan and thai local isolates of Bradyrhizobium japonicum, Shinorhizobium melilo. Soil Sci. Plant Nutr. 46, 241–247. doi: 10.1080/00380768.2000.10408779
Takuji, O., Kaushal, T., Shinji, I., Kazuya, T., Satoshi, K., Yuki, O., et al. (2017). “Role of nitrogen on growth and seed yield of soybean and a new fertilization and technique to promote nitrogen fixation and seed yield,” in Soybean - The Basis of Yield, Biomass and Productivity (Licensee InTech), 153–185.
Tegeder, M., and Masclaux-Daubresse, C. (2018). Source and sink mechanisms of nitrogen transport and use. New Phytol. 217, 35–53. doi: 10.1111/nph.14876
Temesgen, D., and Assefa, F. (2020). Inoculation of native symbiotic effective Sinorhizobium spp. enhanced soybean [Glycine max (L.) Merr.] grain yield in Ethiopia. Environ. Syst. Res. 9, 1–19. doi: 10.1186/s40068-020-00205-1
Thilakarathna, M. S., Chapagain, T., Ghimire, B., and Pudasaini, R. (2019). Evaluating the effectiveness of rhizobium inoculants and micronutrients as technologies for nepalese common bean smallholder farmers in the real-world context of highly variable hillside environments and indigenous farming practices. Agriculture 9, 1–17. doi: 10.3390/agriculture9010020
Thilakarathna, M. S., and Raizada, M. N. (2017). A meta-analysis of the effectiveness of diverse rhizobia inoculants on soybean traits under field conditions. Soil Biol. Biochem. 105, 177–196. doi: 10.1016/j.soilbio.2016.11.022
Thuita, M., Pypers, P., Herrmann, L., Okalebo, R. J., Othieno, C., Muema, E., et al. (2012). Commercial rhizobial inoculants significantly enhance growth and nitrogen fixation of a promiscuous soybean variety in Kenyan soils. Biol. Fertil. Soils 48, 87–96. doi: 10.1007/s00374-011-0611-z
Tian, C. F., Zhou, Y. J., Zhang, Y. M., Li, Q. Q., Zhang, Y. Z., Li, D. F., et al. (2012). Comparative genomics of rhizobia nodulating soybean suggests extensive recruitment of lineage-specific genes in adaptations. Proc. Natl. Acad. Sci. U. S. A. 109, 8629–8634. doi: 10.1073/pnas.1120436109
Toffanin, A., Cadahia, E., Imperial, J., Ruiz-Argüeso, T., and Palacios, J. (2002). Characterization of the urease gene cluster from Rhizobium leguminosarum bv. viciae. Archiv. Microbiol. 177, 290–298. doi: 10.1007/s00203-001-0392-0
Tyagi, A., Kumar, V., Shottam, P., and Tomar, A. (2017). Isolation, identification, biochemical and antibiotic sensitivity characterization of rhizobium strains from Vigna mungo (L) Hepper, Cicer arietinum L. and Vigna radiata (L.) R Wilczek in Muzaffarnagar, Uttar Pradesh, India. Int. J. Curr. Microbiol. Appl. Sci. 6, 2024–2035. doi: 10.20546/ijcmas.2017.612.233
Udeigwe, T. K., Eichmann, M., and Menkiti, M. C. (2016). Fixation kinetics of chelated and non-chelated zinc in semi-arid alkaline soils: application to zinc management. Solid Earth 7, 1023–1031. doi: 10.5194/se-7-1023-2016
Ugboh, O., and Ulebor, J. U. (2011). Application of integrated soil fertility approach in the improvement of soil fertility in semi arid ecology. J. Agri. Soc. Res. 11, 1689–1699.
Vanlauwe, B., Descheemaeker, K., Giller, K. E., Huising, J., Merckx, R., Nziguheba, G., et al. (2011). Integrated soil fertility management in sub-Saharan Africa: unravelling local adaptation. Soil Discus. 1, 1239–1286. doi: 10.5194/soil-1-491-2015
Vanlauwe, B., Hungria, M., Kanampiu, F., and Giller, K. E. (2019). The role of legumes in the sustainable intensification of African smallholder agriculture: lessons learnt and challenges for the future. Agri. Ecosyst. Environ. 284, 106–583. doi: 10.1016/j.agee.2019.106583
Varin, S., Cliquet, J. B., Personeni, E., Avice, J. C., and Lemauviel-Lavenant, S. (2010). How does sulphur availability modify N acquisition of white clover (Trifolium repens L.)? J. Exp. Bot. 61, 225–234. doi: 10.1093/jxb/erp303
Verma, V. C., Singh, S. K., and Prakash, S. (2011). Bio-control and plant growth promotion potential of siderophore producing endophytic Streptomyces from Azadirachta indica A. Juss. J. Basic Microbiol. 51, 550–556. doi: 10.1002/jobm.201000155
Volpiano, C. G., Lisboa, B. B., Granada, C. E., Freitas, J., São, B., Mara, A., et al. (2019). Microbiome in plant health and disease. Microb. Plant Health Dis. 14, 315–336. doi: 10.1007/978-981-13-8495-0_14
Wahab, A. M. A., Abd-Alla, M. H., and El-Enany, A. E. (1996). “Stimulation of nodulation, nitrogen fixation and plant growth of faba bean by cobalt and copper additions,” in Fertilizers and Environment, ed C. Rodriguez-Barrueco (New York, NY: Kluwer Academic Publishers), 127–130. doi: 10.1007/978-94-009-1586-2_23
Wakeel, A., Schubert, S., Wakeel, A., Farooq, M., Qadir, M., and Schubert, S. (2011). Critical reviews in plant sciences potassium substitution by sodium in plants. Crit. Rev. Plant Sci. 30, 401–413. doi: 10.1080/07352689.2011.587728
Wang, D., Yang, S., Tang, F., and Zhu, H. (2012). Microreview symbiosis specificity in the legume – rhizobial mutualism. Cell Microbiol. 14, 334–342. doi: 10.1111/j.1462-5822.2011.01736.x
Wang, E. T., Chen, W. F., Tian, C. F., Young, J. P. W. Y., and Chen, W. F. (2019). Ecology and Evolution of Rhizobia Principles and Applications. Singapore: Springer Nature Singapore Pte Ltd. p. 1–273. doi: 10.1007/978-981-32-9555-1
Wang, H., Gu, C., Liu, X., Yang, C., and Li, W. (2020). Impact of soybean nodulation phenotypes and nitrogen fertilizer levels on the rhizosphere bacterial community. Front. Microbiol. 11, 1–10. doi: 10.3389/fmicb.2020.00750
Wang, H., Wang, S. D., Jiang, Y., Zhao, S. J., Chen, W. X., Wang, H., et al. (2014). Diversity of rhizosphere bacteria associated with different soybean cultivars in two soil conditions. Soil Sci. Plant Nutr. 60, 630–639. doi: 10.1080/00380768.2014.942212
Wang, Q., Liu, J., and Zhu, H. (2018). Genetic and molecular mechanisms underlying symbiotic specificity in legume-rhizobium interactions. Front. Plant Sci. 9, 1–8. doi: 10.3389/fpls.2018.00313
Wang, R., Li, Y., Tao, W., Zhang, D., Xia, X., Hua, X., et al. (2013). Bradyrhizobium arachidis sp. nov., isolated from effective nodules of Arachis hypogaea grown in China. Systemat. Appl. Microbiol. 36, 101–105. doi: 10.1016/j.syapm.2012.10.009
Wasule, D. L., Wadyalkar, S. R., and Buldeo, A. (2003). Effect of Phosphate Solubilizing Rhizobium on nodulation by Soybean, Vela' zquez, E and Rodrýguez-Barrueco. C. Dordrecht: Springer. p. 239–242.
Waswa, M. N., Karanja, N. K., Woomer, P. L., and Mwenda, G. M. (2014). Identifying elite rhizobia for soybean (Glycine max) in. Afri. J. Crop Sci. 2, 60–66.
Wei, G. H., Wang, E. T., Tan, Z. Y., Zhu, M. E., and Chen, W. X. (2002). Rhizobium indigoferae sp. nov. and Sinorhizobium kummerowiae sp. nov., respectively isolated from Indigofera spp. and Kummerowia stipulacea. Int. J. Systemat. Evol. Microbiol. 52, 2231–2239. doi: 10.1099/ijs.0.02030-0
Weisany, W., Raei, Y., and Allahverdipoor, K. H. (2013). Role of some of mineral nutrients in biological nitrogen fixation. Bullet. Environ. Pharmacol. Life Sci. 2, 77–84.
Wilson, D. O., and Reisenauer, H. M. (1970). Effect of manganese and zinc ions on the growth of Rhizobium. J. Bacteriol. 102, 729–732. doi: 10.1128/jb.102.3.729-732.1970
Wilson, R. T. (2013). The Red Meat Value Chain in Tanzania A report from the Southern Highlands Food Systems Programme. Available online at: www.fao.org/publications. (accessed October 23, 2021).
Xu, L. M., Ge, C., Cui, Z., Li, J., and Fan, H. (1995). Bradyhizobium liaoningense sp. nov., Isolated from the root nodules of soybeans. Int. Union Microbiol. Soc. 45, 706–711. doi: 10.1099/00207713-45-4-706
Yadav, R. S., and Tarafdar, J. C. (2003). Phytase and phosphatase producing fungi in arid and semi-arid soils and their efficiency in hydrolyzing different organic P compounds. Soil Biol. Biochem. 35, 1–7. doi: 10.1016/S0038-0717(03)00089-0
Yadegari, M., and Mosadeghzad, Z. (2012). Biofertilizers effects on quantitative and qualitative yield of Thyme (Thymus vulgaris). Afri. J. Agri. Res. 7, 4716–4723. doi: 10.5897/AJAR11.2347
Yan, J., Han, X. Z., Ji, Z. J., Li, Y., Wang, E. T., Xie, Z. H., et al. (2014). Abundance and diversity of soybean-nodulating rhizobia in black soil are impacted by land use and crop management. Appl. Environ. Microbiol. 80, 5394–5402. doi: 10.1128/AEM.01135-14
Yang, S., Tang, F., Gao, M., Krishnan, H. B., and Zhu, H. (2010). R gene-controlled host specifi city in the legume – rhizobia symbiosis. Plant Biol. 107, 18735–18740. doi: 10.1073/pnas.1011957107
Yang, X. B., and Feng, F. (2001). Ranges and diversity of soybean fungal diseases in North America. Phytopathology 91, 769–775. doi: 10.1094/PHYTO.2001.91.8.769
Youseif, S. H., Abd El-Megeed, F. H., Ageez, A., Mohamed, Z. K., Shamseldin, A., and Saleh, S. A. (2014). Phenotypic characteristics and genetic diversity of rhizobia nodulating soybean in Egyptian soils. Eur. J. Soil Biol. 60, 34–43. doi: 10.1016/j.ejsobi.2013.10.008
Youssef, G., Yanni, A., Rizk, Y., Rizk, A., Faiza, K., Abd El-Fattah, A., et al. (2001). The beneficial plant growth-promoting association of Rhizobium leguminosarum bv. trifolii with rice roots. Austr. J. Plant Physiol. 28, 845–870. doi: 10.1071/PP01069
Yuan, K., Reckling, M., Ramirez, M. D. A., Djedidi, S., Fukuhara, I., Ohyama, T., et al. (2020). Characterization of rhizobia for the improvement of soybean cultivation at cold conditions in Central Europe. Microbes Environ. 35, 1–13. doi: 10.1264/jsme2.ME19124
Zhang, B., Du, N., Li, Y., Shi, P., and Wei, G. (2018). Distinct biogeographic patterns of rhizobia and non-rhizobial endophytes associated with soybean nodules across China. Sci. Tot. Environ. 643, 569–578. doi: 10.1016/j.scitotenv.2018.06.240
Zhang, X. X., Guo, H. J., Jiao, J., Zhang, P., Xiong, H. Y., Chen, W. X., et al. (2017). Pyrosequencing of rpoB uncovers a significant biogeographical pattern of rhizobial species in soybean rhizosphere. J. Biogeogr. 44, 1491–1499. doi: 10.1111/jbi.12891
Zhang, Y. M., Li Jr, Y., Chen, W. F., Wang, E. T., Tian, C. F., Li, Q. Q., et al. (2011). Biodiversity and biogeography of rhizobia associated with soybean plants grown in the North China Plain. Appl. Environ. Microbiol. 77, 6331–6342. doi: 10.1128/AEM.00542-11
Keywords: biological nitrogen fixation, genetic diversity, rhizobia, soybean, soybean-nodulating rhizobia, soybean root rot pathogens, symbiotic effectiveness
Citation: Nakei MD, Venkataramana PB and Ndakidemi PA (2022) Soybean-Nodulating Rhizobia: Ecology, Characterization, Diversity, and Growth Promoting Functions. Front. Sustain. Food Syst. 6:824444. doi: 10.3389/fsufs.2022.824444
Received: 29 November 2021; Accepted: 11 March 2022;
Published: 14 April 2022.
Edited by:
Fanuel Kawaka, Jaramogi Oginga Odinga University of Science and Technology, KenyaReviewed by:
Ana I. F. Ribeiro-Barros, University of Lisbon, PortugalJohn M. Maingi, Kenyatta University, Kenya
Juan José Cabrera, Experimental Station of Zaidín (CSIC), Spain
Olubukola Oluranti Babalola, North-West University, South Africa
Osama Ali, Menoufia University, Egypt
Ahmed Idris Hassen, Agricultural Research Council of South Africa (ARC-SA), South Africa
Yasser A. El-Tahlawy, Agricultural Research Center, Egypt
Copyright © 2022 Nakei, Venkataramana and Ndakidemi. This is an open-access article distributed under the terms of the Creative Commons Attribution License (CC BY). The use, distribution or reproduction in other forums is permitted, provided the original author(s) and the copyright owner(s) are credited and that the original publication in this journal is cited, in accordance with accepted academic practice. No use, distribution or reproduction is permitted which does not comply with these terms.
*Correspondence: Monica D. Nakei, bmFrZWltQG5tLWFpc3QuYWMudHo=