- 1Alfred Wegener Institute Helmholtz Centre for Polar and Marine Research (AWI), Bremerhaven, Germany
- 2Centre for Applied Marine Sciences, School of Ocean Sciences, Bangor University, Menai Bridge, United Kingdom
- 3Applied Marine Biology, University of Applied Sciences Bremerhaven, Bremerhaven, Germany
- 4Ecological Aquaculture Foundation, LLC, Biddeford, ME, United States
- 5Ecological Aquaculture Foundation, LLC, Candelaria, Portugal
- 6Kelson Marine Co., Portland, ME, United States
- 7Cawthron Institute, Nelson, New Zealand
- 8Laboratory of Aquaculture and Artemia Reference Center, Ghent University, Ghent, Belgium
- 9Section for Coastal Ecology, National Institute of Aquatic Resources, Technical University of Denmark, Nykøbing Mors, Denmark
- 10Norwegian College of Fisheries, UiT - The Arctic University of Norway, Tromsø, Norway
- 11Department of Aquatic Life Medicine, College of Ocean Science and Technology, Kunsan National University, Gunsan, South Korea
- 12School of Social Sciences, University of Dundee, Dundee, United Kingdom
- 13Department of Sustainable Development, Environmental Science and Engineering (SEED), KTH Royal Institute of Technology, Stockholm, Sweden
- 14The Beijer Institute of Ecological Economics, The Royal Swedish Academy of Sciences, Stockholm, Sweden
- 15Stockholm Resilience Centre, Stockholm University, Stockholm, Sweden
- 16IVL Swedish Environmental Research Institute, Fiskebäckskil, Sweden
- 17RISE Research Institutes of Sweden, Gothenburg, Sweden
To limit compromising the integrity of the planet, a shift is needed towards food production with low environmental impacts and low carbon footprint. How to put such transformative change towards sustainable food production whilst ensuring food security into practice remains a challenge and will require transdisciplinary approaches. Combining expertise from natural- and social sciences as well as industry perspectives, an alternative vision for the future in the marine realm is proposed. This vision includes moving towards aquaculture mainly of low trophic marine (LTM) species. Such shift may enable a blue transformation that can support a sustainable blue economy. It includes a whole new perspective and proactive development of policy-making which considers, among others, the context-specific nature of allocation of marine space and societal acceptance of new developments, over and above the decarbonization of food production, vis á vis reducing regulatory barriers for the industry for LTM whilst acknowledging the complexities of upscaling and outscaling. This needs to be supported by transdisciplinary research co-produced with consumers and wider public, as a blue transformation towards accelerating LTM aquaculture opportunities in a net zero-carbon world can only occur by considering the demands of society.
Introduction
With continued human population expansion, the production and accessibility of healthy and nutritious food (food security) is becoming a top priority in the global context. There is, however, clear evidence that human exploitation of natural resources has exceeded a range of planetary boundaries, thereby jeopardizing the preservation and sustainment of ecosystem functions from the biome level to global scales (Steffen et al., 2015; Newbold et al., 2016; Willett et al., 2019). These consequences are further enhanced by climate change, one of the most severe crises of our time, with far reaching implications on food security worldwide (Fanzo et al., 2018; IPCC, 2019a,b).
In general, food production has a range of impacts such as land conversion, overuse of freshwater resources, greenhouse gas (GHG) emissions, energy use, biodiversity loss and nutrient imbalances (Cordell et al., 2009; Hasegawa et al., 2020; Karstens et al., 2020; Herrero et al., 2021). Currently, food production generates 26% of overall global greenhouse gas emissions, underlining the need to move away from the most environmentally-costly and damaging production systems (Poore and Nemecek, 2018). This challenge will not diminish as human populations are predicted to reach ~10 billion by 2050 (UN, 2019), placing an increasing strain on natural resources and raising the question of how to feed a populated world in a sustainable manner (Aksnes et al., 2017; Willett et al., 2019; FAO, 2020) without exceeding 1.5 degree warming (Rockström et al., 2017; Warszawski et al., 2021).
Thus, a transformation of food production systems is needed to meet the challenge of simultaneously adhering to the planetary dimensions, food security and human health requirements (Gordon et al., 2017; Willett et al., 2019; Kaiser et al., 2021). Transformation is hereby understood as “a fundamental, system-wide reorganization across technological, economic and social factors, including paradigms, goals and values” (Brondizio et al., 2019). As this conceptualization remains rather abstract, we follow the review by Scoones et al. (2020), which identified three basic perspectives on transformation: structural, systemic and enabling transformation. We here focus on the systemic perspectives on transformation dimensions that are rooted in socio-ecological and socio-technical systems thinking and how these surface in marine food production. In concurrence, the UN Sustainable Development Goals (SDG) support this much needed change by provoking new normative reasoning (Leach et al., 2018), raising the question to what extent farming the oceans help provide food security in the Anthropocene and contribute to meeting SDGs (Gentry et al., 2017; Troell et al., 2017).
Using this description of the challenges faced as a baseline, the following sections will describe the co-produced view of what can be done (sections Shifting Food Production to Aquatic Lower Trophic Levels, and The Contribution of Low-Trophic Mariculture to Blue Food Production), and how this can be achieved (sections Defining a Vision for a Sustainable Blue Economy Transformation, Balancing Narratives of LTM Aquaculture Expansion With Societal Realities, and Concerted Implementation of the Blue Transformation), with the aim to provide a framework for integration of LTM aquaculture into future, sustainable, food systems in a net zero-carbon world.
Shifting Food Production to Aquatic Lower Trophic Levels
Global animal protein production (meat, dairy and fish) occupies over 80% of farmland, but produces only 37% of human food protein and 18% of calories (Poore and Nemecek, 2018). It has been argued that the greatest gains in decarbonizing global food production will come from a transition from animal to plant-based foods, with benefits also from intermediate actions in shifting animal production to those farming systems with demonstrated lower environmental impacts and GHG emissions (Poore and Nemecek, 2018; Gephart et al., 2021). Such a shift would not only reduce the direct and indirect climate impacts of animal production but could also release land for biodiversity conservation and climate change mitigation whilst limiting the drawbacks associated with further expansion of agriculture (Cordell et al., 2009; Herrero et al., 2021). To date, guidelines for changing planetary diets to eat less meat, however, remain focused on eating less terrestrial meats in contrast to advocating more strongly shifting meat consumption toward aquatic, low trophic species, meats. This prevailing narrative is, for example, illustrated in the Planetary Health Plate which still pictures a cow as meat source instead of an alternative aquatic species.1
Aquatic food production systems, and in particular farming of extractive (non-fed) low trophic marine (LTM) species (mainly bivalve molluscs and macroalgae), can provide alternatives with lower environmental impacts; i.e., lower GHG emissions and reduced land and freshwater uses (Nijdam et al., 2012; Hilborn et al., 2018; Gephart et al., 2021). LTM species can be grown with lower energy requirements and zero feed or fertilizer inputs, as they extract dissolved nutrients or planktonic/detrital foods directly from the marine environment, and yet are nutrient-dense food sources rich in protein, unsaturated fats and micronutrients (Wright et al., 2018; Hallström et al., 2019; Naylor et al., 2021). Furthermore, LTM aquaculture can also provide a range of valuable non-food ecosystem services such as biodiversity enhancement and eutrophication remediation (van der Schatte Olivier et al., 2018; Gentry et al., 2019; Kotta et al., 2020; Cabre et al., 2021; Naylor et al., 2021; Theuerkauf et al., 2021; The Nature Conservancy, 2022) and may also transform linear nutrient flows from land to the sea into circular systems (Folke and Kautsky, 1992; Petersen et al., 2019; Filippelli et al., 2020; Thomas et al., 2021). Farming of extractive LTM species is one of the most efficient, low-input, low-carbon food production systems, especially when compared to the farming of terrestrial livestock (Hilborn et al., 2018; Gephart et al., 2020). Consequently, redirecting focus from red meat toward aquatic foods with lower environmental impacts and better health profiles should include a larger emphasis on extractive LTM species.
Accordingly, a shift to LTM aquaculture has the potential to reduce GHG emissions of food production and support more efficient and sustainable use of available resources. However, the linkages and repercussions between environmental impacts and food security need to be thoroughly investigated and communicated. Comprehensive and balanced scientific knowledge of the impacts of different aquaculture species, including the role of LTM species in less carbon-intensive diets, will be needed to promote consumption and market development of low-impact foods. New narratives need to be developed that harness the contemporary societal debates on how to tackle climate change and ensure food security at the same time. As such, this may present opportunities to improve social acceptability of blue foods as a shift to low-impact “blue alternatives” may entail a transition to more sustainable and more nutritious foods compared to other protein sources (Hallström et al., 2019). Hence, social culture and practices, and more specifically, the cultures where seafood consumption is not fully integrated as in Asia, must occupy a central position alongside the economic and social analysis of whether marine aquaculture can contribute to the sustainable blue transformation reaching its full potential (Simpson, 2011; Krause et al., 2015, 2020; Naylor et al., 2021). We understand this blue transformation as being part of the “blue economy” concept (Silver et al., 2015). Blue economy encompasses ocean-based industries and the natural assets and ecosystem services that the ocean provides (OECD, 2016; Rayner et al., 2019). As such, it emphasizes the multiple economic and social dimensions of the ocean that can be complementary or even reinforcing under a sustainability lens.
The Contribution of Low-Trophic Mariculture to Blue Food Production
The prospect of aquaculture contributing significantly to feeding a growing world population has been a vision since the mid 20th century, with its emergence as a new food production sector in the 1950s (Costello et al., 2019). Worldwide, governments, non-governmental (e.g., WWF, TNC, EDF) and international organizations (e.g., UN-FAO, EU, ICES) are responding to this challenge by promoting the “Blue Revolution”, “Blue Growth” and, more recently, a “sustainable Blue Economy” that emphasizes zero pollution, zero-carbon, circular economies and biodiversity protection (European Commission, 2021). Development of more sustainable and equitable food production systems is emphasized although how to achieve this vision varies among organizations and institutions (Caswell et al., 2020; Wittmer et al., 2021). It includes, among other aims, the rapid spread and full utilization of aquaculture (Krause et al., 2015; Stevens et al., 2018).
However, despite over 70 years of aspirational policy and pioneering investments, outside China most of the world is still a long way from achieving a transformation in farming the oceans (Caswell et al., 2020; Naylor et al., 2021). Ocean aquatic foods production are estimated to comprise only 4–6% of all human foods today (Costa-Pierce, 2016; FAO, 2020). The global distribution of aquaculture production also remains uneven. Whilst being traditionally conducted in Asia throughout centuries, the rest of the world is yet in nascent stages of aquaculture development (Costa-Pierce and Chopin, 2021). This skewed distribution is currently reflected by 92% of all aquaculture (~110 million tons annually) being performed in Asia, with the rest of the world combined producing ~10 million tons (FAO, 2021). Also, much of aquaculture (40%) within Asia is land-based production of freshwater fish. Similarly, the total aquaculture production elsewhere is dominated by diadromous (34%) and freshwater (32%) fish (Naylor et al., 2021). In fact, 73% of the total edible production from global aquaculture originates from freshwater.
Hence, considering aquaculture as a homogenous food production system overlooks the large differences between sub-sectors in terms of potential environmental benefits. Even though the efficiency of fed aquaculture of finfish has improved over time, lowering food conversion ratios and reducing fish meal and fish oil use (Cottrell et al., 2021; Naylor et al., 2021), the production of plant-based feed ingredients may compete with land and water use for human food production (Troell et al., 2014; Gephart et al., 2017). Feeds remain a major contributor of GHG emissions attributed to production of fed aquaculture species (Robb et al., 2017). Emissions from pond farming of catfish, tilapia and shrimp can be higher than pork and chicken and equivalent to that of beef production, while salmon farming has lower impact. However, it is only extractive LTM species that offer the opportunity for substantive reductions in GHG emissions (Hilborn et al., 2018). Hence the greatest transformative potential of aquaculture lies in increasing production and consumption of LTM species as an alternative to continuing to increase red meat consumption.
Despite these potential benefits, aquaculture of extractive LTM species remains in its infancy in most areas outside of Asia, and uptake on a global scale is geographically uneven. Increasing consumption of LTM species as a source of dietary proteins could make a valuable contribution to the transition to a low-carbon food economy. Achieving a significant impact will require a stepwise, transformative change toward farming of LTM species such as bivalve, shellfish and seaweeds. Presently, the combined annual production of these species is only 52 million tons (FAO, 2021), or even less (Porse and Rudolph, 2017), of which less than half may be converted to consumable food (Edwards et al., 2019). This compares to global meat production of 328 million tons in 2020, which is projected to rise to over 374 million tons in the coming decade (FAO, 2021; OECD FAO, 2021). Nevertheless, anticipation of several-fold increase in global LTM production as an alternative to continued expansion of red meat production represents an achievable goal over timeframe that aligns with the urgency of the pathway towards net zero-carbon emissions. One reason for this current imbalance in terrestrial and marine food production is rooted in the terrestrial bias of the human mind that affects human behavior, decision-making and problem-solving (Fuchs et al., (under review); Steinberg and Peters, 2015; Armbrecht and Skallerud, 2019). Although the ocean has long been a food source for humans (especially for coastal communities), it has always been a less accessible and predictable environment for cultivation compared to land. It is from this stance that the oceans on our planet have never been completely included in the thoughts and perception of the majority of humans (Gee, 2019).
Defining a Vision for a Sustainable Blue Economy Transformation
The planetary boundaries framework (Steffen et al., 2011) and the call for societal transformation has spurred discourses on a stronger recognition of intersections between natural and social/cultural dimensions, leading to more inter- and transdisciplinary conversations (Castree, 2014; Blythe et al., 2018; Brondizio et al., 2019; Scoones et al., 2020). This raises an opportunity to reconsider how the oceans are viewed and integrated in socio-economic concepts (Österblom et al., 2017).
Common across competing scenarios of transformative ocean futures (i.e., Gentry et al., 2017; Belton et al., 2020; Costa-Pierce et al., 2021) is the tendency to focus on quantifiable effects of activities, such as the prospects of economic gain or the risk of environmental degradation (Oyinlola et al., 2018). While these are clearly important, responsible ocean development should also consider how the activities affect our ideas about the common good. Indeed, a sustainable blue transformation requires us to reconsider the relationships between the private and public (Steinberg and Peters, 2015; Brugere et al., 2021). This is a crucial question, as the understanding of public-private interactions in marine aquaculture is still blurred. These opposing views surface especially when considering open ocean aquaculture, as this entails investment and operational costs at scale that involves fewer and larger businesses. However, it can be argued that LTM aquaculture systems benefits humanity by offering low-carbon healthy foods, nutrient recapture and clearer water in eutrophic areas, in addition to providing economic profits to a few actors. We recognize that LTM systems will have some environmental costs, the level of which will depend on the geographical context such as oligotrophic vs. eutrophic areas, scale of production, water movements, etc. (Theuerkauf et al., 2021). However, these are substantially less than other forms of food production (including fed aquaculture) and are offset by the benefits (Gephart et al., 2021; Naylor et al., 2021) and are in large reversible within a few years after ceasing the activity.
Thus, the central question is how to put transformative change toward sustainability into practice? It is not only important to develop a vision on what future ocean we want, but also to investigate what needs to be known to change the prevailing and entrenched food systems toward this sustainability vision, how to navigate, nudge and nurture system change, and how to create space for deliberating just transformation (Wittmer et al., 2021). To date, much of the scientific attention has been placed on the technical, engineering and natural sciences, yet as each of the planetary boundary challenges are contested, these cannot be addressed based on only one type of knowledge base. The doughnut economy framework by Raworth (2017) is one noteworthy effort to bridge some of these conceptual islands of knowledge. Indeed, to achieve transformation, different types of knowledge must come together. For example, the global rapid development of marine fed aquaculture over recent decades has been a success story for the triple helix interaction of government, research and industry (Leydesdorff, 2000), since the development of aquaculture is often industry- and technology-led, hand-in-hand with research, and facilitated (or not) by governance arrangements.
Moreover, to achieve transformation the meaning of the term must first be described. The notion of “transformation” is used differently in politics and in science (Blythe et al., 2018). In politics, it is a wake-up call for bolder, multilateral action. In science, different schools of thought elaborate on the conceptual underpinnings for what transformation to sustainability actually entails: What needs to be transformed? Into what? How fast? Who should do it and how? Yet, ambiguities in the definition and pursuit of transformative change are widespread (O'Brien, 2012; Feola, 2015; Costa-Pierce, 2016, 2021; Blythe et al., 2018). Also, transformation requires proactive (rather than responsive) investments and it should aim for a lasting positive change in dominant power relations by favoring equity, fairness, and justice (Chaffin et al., 2016; Cisneros-Montemayor et al., 2021). Transformation is interpreted as relating to a social-ecological change that addresses the underlying idea that “more of the same” will not solve the growing tensions and socio-economic impacts that result from over-using and degrading ecosystems and resource systems and putting further strain on planetary boundaries (Cisneros-Montemayor et al., 2021; Wittmer et al., 2021). Consequently, transformation is considered as a fundamental change-of-path. However, such large-scale fundamental changes cannot be planned and implemented in one piece but will rather involve a number of steps and iterative evaluation to achieve change that in retrospect can be considered as fundamental.
To date, most of the observable contemporary developments in aquaculture are locked in the 20th century technology-fit pathways. This points out to a fundamental dilemma, as already observed by Collingridge (1980). At an early stage of research and development of a new idea, such as low carbon blue food systems, it is impossible to know what the most important impacts (positive and/or negative) on the sustainability dimensions will be (see e.g., Gephart et al., 2020). However, if attempts are not made to identify, predict and mitigate negative impacts, it will often be too late to handle or control them (Collingridge, 1980). An example of this, is the current situation where climate change impacts of terrestrial food systems were not sufficiently predicted. In this sense, narratives and visions provide an important role as a compass for what a transformed system and a desirable future would look like. Consequently, there needs to be some forward view of the potential outcomes of competing visions of a blue transformation under the umbrella of the blue economy concept. In comparison to terrestrial food production systems, aquaculture has many benefits, however there is a risk of similar path-dependencies. In this article, in expansion to earlier propositions (Gentry et al., 2017; Troell et al., 2017; Belton et al., 2020), and in order to avoid a Collingridge dilemma, we propose an alternative vision for the future. This vision includes moving towards a future development of aquaculture that is focused mainly on LTM systems. By this shift, we predict that a significant contribution towards net zero-carbon food systems may evolve.
Balancing Narratives of LTM Aquaculture Expansion With Societal Realities
In accordance with this vision there is, at least from a theoretical perspective, scope for coastal waters to support a significant increase in LTM production (Buck and Langan, 2017; Theuerkauf et al., 2019; Thomas et al., 2019; Heasman et al., 2020). This potential is apparent even in disparities among those nations that already have some coastal marine aquaculture; for which production ranges from <1 MT km−1 to more than 500 MT km−1 of shoreline (Kapetsky et al., 2013). However, access to this space is by no means pre-emptive (Troell et al., 2017). The barriers to increasing aquaculture in nearshore areas can include, e.g., the availability of suitable sheltered coastal sites that allow cost-effective production, competition for densely-used coastal marine space (Debnath, 2020; Kluger and Filgueira, 2021; St. Gelais et al., 2022), water quality (Cheney et al., 2010; Hassard et al., 2017; Lee et al., 2018; Rodil et al., 2019; Song and Duan, 2019; Xie et al., 2020) and significant regulatory and legislative complexities (Lester et al., 2018). These limitations have led to a focus on marine environments where there may be greater scope for a step change in production through expansion into more exposed coastal sites and more distant open ocean waters.
A narrative has emerged in which optimistic scenarios portray the future of open ocean aquaculture as “the new frontier” for food security (Marra, 2005; Costello et al., 2020). For example, recent analyses have proposed that the suitable ocean space for aquaculture vastly exceeds the requirements for any currently required increase in production—with theoretically more than sufficient space to exceed terrestrial meat production needs many times over (Gentry et al., 2017; Oyinlola et al., 2018). However, such projections are likely over-optimistic as not all this space will be accessible or suitable (Troell et al., 2017, 2022; Theuerkauf et al., 2019). Froehlich et al. (2019) projected 48 million km2 for seaweeds for blue growth to mitigate climate change. Thus, to avoid ending up in a Collingridge dilemma, it is important to recognize the specific requirements, constraints and impacts of LTM aquaculture development. Otherwise, the manner in which LTM aquaculture expansion of both, coastal and offshore exposed areas is moved, puts us at risk of making incorrect assumptions that may undermine meaningful and sustainable expansion, especially when pioneering developments on a very large scale are required to drive a fundamental shift in food supply. Engineering such systems for economic sustainability, within the confines of regulatory and social acceptance, and importance for nutrition and food security (globally), remains a challenge for open ocean LTM aquaculture.
From a technical perspective, the tools and engineering capabilities required to design and de-risk LTM systems for exposed and open-ocean conditions exist (e.g., Dewhurst, 2016; Pribadi et al., 2019; Fredriksson et al., 2020; Heasman et al., 2021; Landmann et al., 2021; Moscicki et al., 2021). This, however, infers significant investments in infrastructure and increases in operational costs compared to nearshore, sheltered areas, representing barriers to entry into the sector for prospective producers of relatively low value commodities such as seaweeds and shellfish. To cope with these increased costs either the value of the end-products must increase and/or the production costs must decrease. To address the latter pushes the sector toward large scale production, which will have to go hand in hand with major shifts in consumer attitudes towards new products and markets in order to achieve large scale incorporation of LTM species into the human food chain and ensure stable markets for the newly produced foods. Whereas in China, early expansion of marine aquaculture focused on LTM species for food security, led by government policy within a socialist market economy (Yu and Han, 2020), elsewhere the evolution of industrial marine aquaculture has tended to focus initially on high value fed species (mostly finfish and shrimp) with potential for high initial returns on investment (Llorente et al., 2020). Consequently, from a societal perspective, if net zero-carbon is a central goal, a fundamental change in the narrative of food system production and re-aligning the contemporary market drivers and incentives will be required.
So far, a contextual approach particularly in terms of what type of aquaculture (nearshore vs. open ocean), and what type of effect at different scales (individual, community, national, regional and international) has been neglected. That said, occupation of large areas of marine space by LTM aquaculture, even in the “distant offshore”, may mobilize objections, especially as the scale of farms is likely to reflect the investment and infrastructure required to achieve economies of scale in open ocean conditions and to make a meaningful impact on global food systems. Moreover, moving LTM aquaculture offshore may cause significant ecological and societal trade-offs. For example, roughly 50–60% of coastal waters suffer from nutrient pollution, causing severe ecosystem degradation and loss of important ecosystem services (Howarth et al., 2000; Grizzetti et al., 2021). The effects are most pronounced in nearshore areas, although the severity vary between regions. Extractive LTM aquaculture has been proposed as a tool to remediate this challenge (Petersen et al., 2016; Kotta et al., 2020; Naylor et al., 2021; Theuerkauf et al., 2021). The latter is reflected in the global movement in aquaculture toward developing financial instruments to recognize the roles of (restoration) aquaculture to enhance ecosystem goods and services (The Nature Conservancy, 2021; Barrett et al., 2022). LTM aquaculture may also contribute to circular economies by recovering finite resources from marine coastal environments (Thomas et al., 2021). For instance, 80% of all phosphorous is used by agriculture, and provided business-as-usual scenario, our phosphorous resources are predicted to be depleted in 50–100 years due to the linear flow of phosphorous from land-based agriculture systems into the sea (Sverdrup et al., 2013; Achary et al., 2017). Such ecosystem service benefits by LTM aquaculture species are additional to the reduction in food-related GHG emissions from replacing terrestrial animal source foods (e.g., Naylor et al., 2021). By increasing the societal understanding of how different types of aquaculture impact and benefit different marine environments, increased acceptance may be achieved on a range of scales. Public social acceptance is needed to embrace the value of the blue transformation toward a sustainable blue economy. Social license, as an outcome of a successful, non-formal, institutional exchange between a company and its public, is needed to enable the development of LTM aquaculture (Shindler et al., 2002; Krause et al., 2020).
Concerted Implementation of the Blue Transformation
Even though the prospect of open ocean production is often raised as a promising direction, to date the economics, governance and technology of ocean aquaculture favor nearshore environments. Consequently, when matching academic visions with industry and societal realities, the positive vision of open ocean LTM expansion becomes complex. It is not obvious that the predominant scientific perspective on how to move forward is the optimal pathway from either an industry or socio-economic perspective (Figure 1).
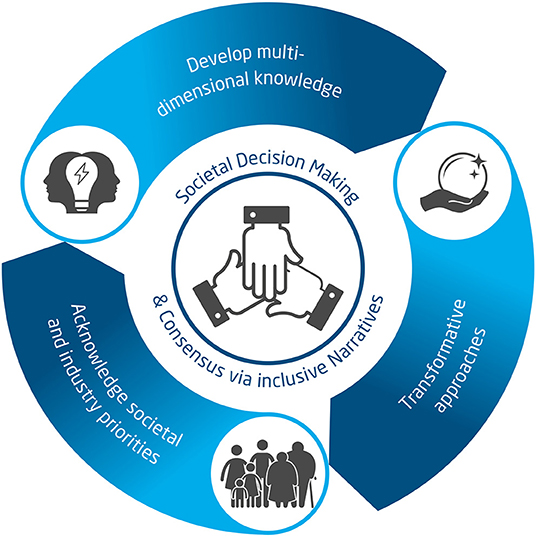
Figure 1. Reflexive co-development toward societal decision-making and consensus via inclusive narratives. ((C) Y. Nowak/AWI).
While new technologies for utilizing natural resources such as farming in open ocean areas are often perceived as a degradation of nature, they can also bring new ways of valuing and relating of societies to marine species and spaces (Cabre et al., 2021). Therefore, we need to identify and consider LTM systems that take advantage of the individual merits found in nearshore and open oceans. For example, open ocean sites will have many of the same social and regulatory challenges of nearshore sites in addition to increased capital costs. Nevertheless, considering the shortcomings of sprawling developments of aquaculture and environmental problems in coastal waters, and the increase in demand for safe seafood, development of aquaculture in the open sea has high validity in many regions of the world.
While food cannot be produced without changing the environment, whether on land, nearshore or in the open ocean, LTM without feed or fertilizer inputs can generate wider benefits beyond the core activity of food production, including a range of ecosystem services (van der Schatte Olivier et al., 2018; Cabre et al., 2021; Naylor et al., 2021; Theuerkauf et al., 2021). These benefits provide powerful policy drivers for allocation of marine space and societal acceptance of new developments, over and above the decarbonization of food production. If the realization of the potential of LTM aquaculture is a priority, the prerequisites for a sustainable expansion of the LTM sector must be addressed by the establishment of enabling conditions for long-term investment and growth of the private sector. This must be enforced by policy and governance in a careful way that supports industry development of LTM aquaculture, as well as optimizing its socio-economic benefits. This could be achieved through a progressive expansion first into traditionally used sheltered and exposed, highly energetic nearshore areas (St. Gelais et al., 2022), and then, successively, into open ocean sites, if required. Such change cannot be planned and implemented in one piece, but will rather involve a number of steps that, in retrospect, can be considered as fundamental. This entails a “strategy of incremental change with a transformative agenda, where a normative focus on sustainability transformations helps to orient incremental efforts (such as policy change) within a broader narrative of transformative change” (Patterson et al., 2017). This thinking is captured in the notions of “progressive incremental” change (Levin et al., 2012), “directed incrementalism” (Grunwald, 2007), or “radical incrementalism” (Göpel, 2016).
In this sense, narratives and visions provide an important role as a compass for what a transformed food production system, and thus what a desirable future for LTM aquaculture would look like, and how these are embedded in a sustainable blue economy setting. At the same time, the exact outcomes of fundamental change cannot be anticipated and there will be many different options to achieve the desired (i.e., more sustainable) outcomes. This highlights the importance of ensuring that transformations are democratically negotiated and debated broadly within society. To this end, with the help of co-developed ocean narratives that match different knowledge realms, new pathways for a blue transformation toward a blue economy are fostered. Embracing low-trophic extractive species, even large-scale open ocean or exposed nearshore aquaculture enterprises can still represent ethical investments, with an opportunity to reframe the public dialogue about aquaculture, emphasizing climate change mitigation, sustainable resource use and ecosystem services (Hoegh-Guldberg et al., 2019). This may help achieve societal acceptance of a blue transformation on a range of scales from coastal communities to broader societies at large (Froehlich et al., 2017; Mather and Fanning, 2019). Gaining social license will require fostering a paradigm shift in public perceptions informed by interdisciplinary and transdisciplinary research approaches in order to avoid any reinforcement of dystopian narratives of industrialized oceans (Merrie et al., 2018).
Conclusions
It needs to be acknowledged that every type of food production does change the environment. Therefore, transformation of contemporary food systems toward net zero-carbon systems will require a pathway that focusses on environmental impacts being better than the alternative rather than elusively aiming for zero environmental impact. This calls for a whole new perspective in policy-making. Under this umbrella, despite overall aquaculture increasing globally, LTM aquaculture remains an underutilized resource with great sustainability potential. So far, blue protein from LTM species is an important, although often forgotten, resource when developing policy and recommendations for a societal transformation of food production and consumption from red meat to green plant-based proteins towards net zero-carbon food systems. Indeed, as pointed out, LTM aquaculture may provide a sustainable food production option to a growing world population, as well as providing both ecological, health and climate benefits. As such, LTM products need to be compared side by side with other marine and terrestrial protein sources in regards to “land” use, ecological and emission impacts in order to support decision making of both, policy makers and consumers. Technical solutions for open-ocean LTM aquaculture exist but a step-wise transformation including expansion in nearshore and open ocean, increased market demand, upscaling, and reduced regulatory barriers for the industry is required for LTM aquaculture to realize this potential and achieve impactful sustainable growth. Overall, to increase the cultivation and consumption of LTM products in society, a transformative change toward a sustainable blue economy is needed of how we perceive, relate to and prioritize the use of coastal and open ocean areas. To this end, a net zero-carbon blue transformation narrative is warranted that includes proactive development and investments by the government, authorities and aquaculture industry that is supported by transdisciplinary research co-produced with consumers and wider public. Indeed, there will be no blue transformation without people—LTM aquaculture opportunities can only be harnessed in tandem with demands of society.
Author Contributions
GK, LLV, and ÅS: conceptualization and methodology. GK, LLV, ÅS, BB, BC-P, TD, KH, NN, PN, KN, KP, MS, J-BT, JW, and AW: investigation and writing—original draft. ÅS, KH, BC-P, and BB: funding acquisition. ÅS: project administration. GK, LLV, ÅS, MT, and FZ: writing—review and editing. All authors contributed to the article and approved the submitted version.
Funding
We would like to thank the KVA fund for internationalization and scientific renewal at the Sven Lovén Centre, the foundation Karin and Herbert Jacobsson, and the European Union's Horizon 2020 Research and Innovation Programme under Grant Agreement No 818173 for financial support for hosting this workshop and preparing this publication. LLV and JW were supported by the Shellfish Centre (Grant Agreement c81364) part-funded by the European Regional Development Fund Operational Programme through the Welsh Government.
Author Disclaimer
This publication reflects the views only of the authors, and the European Union cannot be held responsible for any use which may be made of the information it contains.
Conflict of Interest
TD was employed by Kelson Marine Co. BC-P was employed by Ecological Aquaculture Foundation, LLC, Biddeford, ME, United States and Ecological Aquaculture Foundation, LLC, Candelaria, Portugal.
The remaining authors declare that the research was conducted in the absence of any commercial or financial relationships that could be construed as a potential conflict of interest.
Publisher's Note
All claims expressed in this article are solely those of the authors and do not necessarily represent those of their affiliated organizations, or those of the publisher, the editors and the reviewers. Any product that may be evaluated in this article, or claim that may be made by its manufacturer, is not guaranteed or endorsed by the publisher.
Acknowledgments
The industry perspectives presented in this perspective piece is based on the outcomes from an industry-oriented workshop “State of the Art and Future Development of Low Trophic Level Species Culture in High Energy Environments”, hosted by IVL Swedish Environmental Research Institute, University of Gothenburg, University of New England, Alfred Wegener Institute Helmholtz Center for Polar and Marine Research, Cawthron Institute and the H2020 consortium AquaVitae at the Kristineberg Center, Sweden, with 31 participants from 11 nations on October 16th−18th 2019. We would like to acknowledge the importance of the information extracted from, and by, the participants at the workshop.
Footnote
1. ^eatforum.org (accessed April 13, 2022).
References
Achary, V. M. M., Ram, B., Manna, M., Datta, D., Bhatt, A., Reddy, M. K., et al. (2017). Phosphite: a novel P fertilizer for weed management and pathogen control. Plant Biotechnol. J. 15, 1493–1508. doi: 10.1111/pbi.12803
Aksnes, L. D., Holm, P., Bavinck, M., Biermann, F., Donovaro, R., Harvey, P., et al. (2017). Food from the Oceans - How can more food and biomass be obtained from the oceans in a way that does not deprive future generations of their benefits?. Science Advice for Policy by European Academies (SAPEA). Berlin, Germnany. Available online at: https://www.sapea.info/wp-content/uploads/FFOFINALREPORT-1.pdf
Armbrecht, J., and Skallerud, K. (2019). Attitudes and intentional reactions towards mariculture development -local residents' perspective. Ocean. Coast. Manag. 174, 56–62. Available online at: https://munin.uit.no/bitstream/handle/10037/15281/article.pdf?sequence=3&isAllowed=n
Barrett, L. T., Theuerkauf, S. J., Rose, J. M., Alleway, H. K., Bricker, S. B., Parker, M., et al. (2022). Sustainable growth of non-fed aquaculture can generate valuable ecosystem benefits. Ecosyst. Serv. 53, 101396. doi: 10.1016/j.ecoser.2021.101396
Belton, B., Little, D. C., Zhang, W., Edwards, P., Skladany, M., and Thilsted, S. H. (2020). Farming fish in the sea will not nourish the world. Nat. Commun. 11, 5804. doi: 10.1038/s41467-020-19679-9
Blythe, J., Silver, J., Evans, L., Armitage, D., Bennett, N. J., Moore, M-L, Morrison, T. H., et al. (2018). The dark side of transformation: latent risks in contemporary sustainability discourse. Antipode 50, 1206–1223. doi: 10.1111/anti.12405
Brondizio, E. S., Settele, J., Díaz, S., and Ngo, H. T. (2019). Global Assessment Report on Biodiversity and Ecosystem Services of the Intergovernmental Science-Policy Platform on Biodiversity and Ecosystem Services (IPBES). Bonn, Germany: IPBES Secretariat.
Brugere, C., Troell, M., and Eriksson, H. (2021). More than fish: Policy coherence and benefit sharing as necessary conditions for equitable aquaculture development. Mar. Policy 123, 104271. doi: 10.1016/j.marpol.2020.104271
Buck, B. H., and Langan, R. (2017). Aquaculture Perspective of Multi-Use Sites in the Open Ocean: The Untapped Potential for Marine Resources in the Anthropocene (Cham: Springer).
Cabre, L. M., Hosegood, P., Attrill, M. J., Bridger, D., and Sheehan, E. V. (2021). Offshore longline mussel farms: a review of oceanographic and ecological interactions to inform future research needs, policy and management. Rev. Aquacult. 13, 1864–1887. doi: 10.1111/raq.12549
Castree, N. (2014). The anthropocene and geography III: future directions. Geogr. Compass 8, 464–476. doi: 10.1111/gec3.12139
Caswell, B., Klein, E., Ball, J., Alleway, H., Cardinale, M., Eero, M., et al. (2020). Something old, something new: lessons from history for today's blue growth agendas. Fish Fish. 21, 774–796. doi: 10.1111/faf.12460
Chaffin, B. C., Garmestani, A. S., Gunderson, L. H., Benson, M. H., Angeler, D. G., Arnold, C. A., et al. (2016). Transformative environmental governance. Annu. Rev. Environ. Resour. 41, 399–423. doi: 10.1146/annurev-environ-110615-085817
Cheney, D., Langan, R., Heasman, K., Friedman, B., and Davis, J. (2010). Shellfish culture in the open ocean: lessons learned for offshore expansion. Mar. Technol. Soc. J. 44, 55–67. doi: 10.4031/MTSJ.44.3.6
Cisneros-Montemayor, A. M., Moreno-Báez, M., Reygondeau, G., Cheung, W. W. L., González-Espinosa, P. C., Lam, V. W. Y., et al. (2021). Enabling conditions for an equitable and sustainable blue economy. Nature 591, 396–401. doi: 10.1038/s41586-021-03327-3
Cordell, D., Drangert, J.-O., and White, S. (2009). The story of phosphorus: global food security and food for thought. Glob. Environ. Change 19, 292–305. doi: 10.1016/j.gloenvcha.2008.10.009
Costa-Pierce, B. A. (2016). “Ocean foods ecosystems for planetary survival in the Anthropocene” in World Nutrition Forum: Driving the Protein Economy, ed E. M. Binder (Austria: Erber AG), 301–320.
Costa-Pierce, B. A. (2021). The social ecology of aquaculture in its new geographies. World Aquacult. 52, 43–50.
Costa-Pierce, B. A., and Chopin, T. (2021). The hype, fantasies and realities of aquaculture development globally and in its new geographies. World Aquacult. 52, 23–35.
Costa-Pierce, B. A., Bockus, A. B., Buck, B. H., van den Burg, S. W. K., Chopin, T., Ferreira, J. G., et al. (2021). A fishy story promoting a false dichotomy to policy-makers: it's not freshwater vs. marine aquaculture. Rev. Fish. Sci. Aquacult. 1–18.
Costello, C., Cao, L., Gleich, S., Cisneros-Mata, M. Á., Free, C. M., Froehlich, H. E., et al. (2019). The Future of Food From the Sea. Washington, DC: World Resources Institute. Available online at: https://www.oceanpanel.org/blue-papers/future-food-sea (accessed November 1, 2021).
Costello, C., Cao, L., Gleich, S., Cisneros-Mata, M. Á., Free, C. M., Froehlich, H. E., et al. (2020). The future of food from the sea. Nature 588, 95–100. doi: 10.1038/s41586-020-2616-y
Cottrell, R. S., Metian, M., Froehlich, H. E., Blanchard, J. L., Jacobsen, N. S., McIntyre, P. B., et al. (2021). Time to rethink trophic levels in aquaculture policy. Rev. Aquacult. 13, 1583–1593. doi: 10.1111/raq.12535
Debnath, R. (2020). Conflicts over water and land use in Coastal Aquaculture vis-à-vis Fisheries. ESFA J. Fish. Aquacult. 1, 12–14.
Dewhurst, T. (2016). Dynamics of a Submersible Mussel Raft. University of New Hampshire. Available online at: https://scholars.unh.edu/dissertation/1380 (accessed December 16, 2021).
Edwards, P., Zhang, W., Belton, B., and Little, D. C. (2019). Misunderstandings, myths and mantras in aquaculture: its contribution to world food supplies has been systematically over reported. Mar. Policy 106, 103547. doi: 10.1016/j.marpol.2019.103547
European Commission (2021). Communication From the Commission to the European Parliament, The Council, The European Economic and Social Committee and the Committee of the Regions on a New Approach for a Sustainable Blue Economy in the EU Transforming the EU's Blue Economy for a Sustainable Future. COM/2021/240 final. Available online at: https://eur-lex.europa.eu/legal-content/EN/TXT/?uri=COM:2021:240:FIN (accessed June 6, 2021).
Fanzo, J., Davis, C., McLaren, R., and Choufani, J. (2018). The effect of climate change across food systems: Implications for nutrition outcomes. Global Food Secur. 18, 12–19. doi: 10.1016/j.gfs.2018.06.001
FAO (2021). Fishery and Aquaculture Statistics. Global aquaculture production 1950-2019. FishstatJ. Rome: FAO dataset. Available online at: www.fao.org/fishery/statistics/software/fishstatj/en (accessed December 12, 2021).
Feola, G. (2015). Societal transformation in response to global environmental change: a review of emerging concepts. Ambio 44, 376–390. doi: 10.1007/s13280-014-0582-z
Filippelli, R., Termansen, M., Hasler, B., Timmermann, K., and Petersen, J. K. (2020). Cost-effectiveness of mussel farming as a water quality improvement measure: agricultural, environmental and market drivers. Water Resources Econ. 32, 100168. doi: 10.1016/j.wre.2020.100168
Folke, C., and Kautsky, N. (1992). Aquaculture with its environment: prospects for sustainability. Ocean Coastal Manag. 17, 5–24. doi: 10.1016/0964-5691(92)90059-T
Fredriksson, D. W., Dewhurst, T., Drach, A., Beaver, W., St. Gelais, A. T., Johndrow, K., et al. (2020). Hydrodynamic characteristics of a full-scale kelp model for aquaculture applications. Aquacult. Eng. 90, 102086. doi: 10.1016/j.aquaeng.2020.102086
Froehlich, H., Afflerbach, J. C., Frazier, M., and Halpern, B. S. (2019). Blue growth potential to mitigate climate change through seaweed offsetting. Curr. Biol. 29, 3087–3093. doi: 10.1016/j.cub.2019.07.041
Froehlich, H., Gentry, R. R., Rust, M., Grimm, D., and Halpern, B. S. (2017). Public perceptions of aquaculture: evaluating spatiotemporal patterns of sentiment around the world. PLoS ONE 12, e0169281. doi: 10.1371/journal.pone.0169281
Fuchs, N., Meunier, C., and Krause, G. (under review). Lose sight of the woods for the trees – why Oceans need a new narrative. Mar. Policy.
Gee, K. (2019). “The ocean perspective” in Maritime Spatial Planning, eds J. Zaucha, K. Gee (Cham: Palgrave Macmillan), 23–45.
Gentry, R. R., Alleway, H. K., Bishop, M. J., Gillies, C. L., Waters, T., and Jones, R. (2019). Exploring the potential for marine aquaculture to contribute to ecosystem services. Rev. Aquacult. 12, 499–512. doi: 10.1111/raq.12328
Gentry, R. R., Froehlich, H. E., Grimm, D., Kareiva, P., Parke, M., Rust, M., et al. (2017). Mapping the global potential for marine aquaculture. Nat. Ecol. Evolut. 1, 1317–1324. doi: 10.1038/s41559-017-0257-9
Gephart, J. A., Golden, C. D., Asche, F., Belton, B., Brugere, C., Froehlich, H. E., et al. (2020). Scenarios for global aquaculture and its role in human nutrition. Rev. Fish. Sci. Aquacult. 29, 122–138. doi: 10.1080/23308249.2020.1782342
Gephart, J. A., Henriksson, P. J. G., Parker, R. W. R., Shepon, A., Gorospe, K. D., Bergman, K., et al. (2021). Environmental performance of blue foods. Nature 597, 360–365. doi: 10.1038/s41586-021-03889-2
Gephart, J. A., Troell, M., Henriksson, P. J. G., Beveridge, M. C. M., Verdegem, M., Metian, M., et al. (2017). The 'seafood gap' in the food-water nexus literature — issues surrounding freshwater use in seafood production chains. Adv. Water Resour. 110, 505–514. doi: 10.1016/j.advwatres.2017.03.025
Gordon, L. J., Bignet, V., Crona, B., Henriksson, P. J. G., Van Holt, T., Jonell, M., et al. (2017). Rewiring food systems to enhance human health and biosphere stewardship. Environ. Res. Lett. 12, 100201. doi: 10.1088/1748-9326/aa81dc
Grizzetti, B., Vigiak, O., Udias, A., Aloe, A., Zanni, M., Bouraoui, F., et al. (2021). How EU policies could reduce nutrient pollution in European inland and coastal waters. Global Environ. Change 69, 102281. doi: 10.1016/j.gloenvcha.2021.102281
Grunwald, A. (2007). Working towards sustainable development in the face of uncertainty and incomplete knowledge. J. Environ. Policy Plan. 9, 245–262. doi: 10.1080/15239080701622774
Hallström, E., Bergman, K., Mifflin, K., Parker, R., Tyedmers, P., Troell, M., et al. (2019). Combined climate and nutritional performance of seafoods. J. Clean. Prod. 230, 402–411. doi: 10.1016/j.jclepro.2019.04.229
Hasegawa, T., Sands, R., Brunelle, T., Cui, Y., Frank, S., Fujimori, S., et al. (2020). Food security under high bioenergy demand. Clim. Change 163, 1587–1601. doi: 10.1007/s10584-020-02838-8
Hassard, F., Sharp, J. H., and Taft, H. (2017). Critical review on the public health impact of norovirus contamination in shellfish and the environment: a UK perspective. Food Environ. Virol. 9, 123–141. doi: 10.1007/s12560-017-9279-3
Heasman, K. G., Scott, N., Ericson, J. A., Taylor, D. I., and Buck, B. H. (2020). Extending New Zealand's marine shellfish aquaculture into exposed environments – adapting to modern anthropogenic challenges. Front. Mar. Sci. 7, 565686. doi: 10.3389/fmars.2020.565686
Heasman, K. G., Scott, N., Smeaton, M., Goseberg, N., Hildebrandt, A., Vitasovich, P., et al. (2021). New system design for the cultivation of extractive species at exposed sites - Part 1: system design, deployment and first response to high-energy environments. Appl. Ocean Res. 110, 102603. doi: 10.1016/j.apor.2021.102603
Herrero, M., Thornton, P., Mason-D'Croz, D., Palmer, J., Bodirsky, B., Pradhan, P., et al. (2021). Articulating the impact of food systems innovation on the sustainable development goals. Lancet Planetary Health 5, e50–62. doi: 10.1016/S2542-5196(20)30277-1
Hilborn, R., Banobi, J., Hall, S. J., Pucylowski, T., and Walsworth, T. E. (2018). The environmental cost of animal source foods. Front. Ecol. Environ. 16, 329–335. doi: 10.1002/fee.1822
Hoegh-Gulderbg, O., Northrop, E., and Lubchenco, J. (2019). The ocean is key to achieving climate and societal goals. Science 365, 1372–1374. doi: 10.1126/science.aaz4390
Howarth, R. W., Anderson, D. B., Cloern, J. E., Elfring, C., Hopkinson, C. S., Lapointe, B., et al. (2000). Nutrient pollution of coastal rivers, bays, and seas. Issues Ecol. 7, 1–16.
IPCC (2019a). Climate Change and Land: An IPCC Special Report on Climate Change, Desertification, Land Degradation, Sustainable Land Management, Food Security, and Greenhouse Gas Fluxes in Terrestrial Ecosystems. IPCC. Available online at: https://www.ipcc.ch/srccl/ (accessed October 4, 2021).
IPCC (2019b). IPCC Special Report on the Ocean and Cryosphere in a Changing Climate. IPCC. Available online at: https://www.ipcc.ch/srocc/ (accessed October 4, 2021).
Kaiser, M., Goldson, S., Buklijas, T., Gluckman, P., Allen, K., Bardsley, A., et al. (2021). Towards post-pandemic sustainable and ethical food systems. Food Ethics 6, 4. doi: 10.1007/s41055-020-00084-3
Kapetsky, J. M., Aguilar-Manjarrez, J., and Jenness, J. (2013). A Global Assessment of Potential for Offshore Mariculture Development From a Spatial Perspective. Rome: FAO Technical Paper No. 549.
Karstens, K., Bodirsky, B., Dietrich, J., Dondini, M., Heinke, J., Kuhnert, M., et al. (2020). Management induced changes of soil organic carbon on global croplands. Biogeosci. Discuss. 2020, 1–30. doi: 10.5194/bg-2020-468
Kluger, L. C., and Filgueira, R. (2021). Thinking outside the box: embracing social complexity in aquaculture carrying capacity estimations. ICES J. Mar. Sci. 78, 435–442. doi: 10.1093/icesjms/fsaa063
Kotta, J., Futter, M., Kaasik, A., Liversage, K., Rätsep, M., Barboza, F., et al. (2020). Cleaning up seas using blue growth initiatives: Mussel farming for eutrophication control in the Baltic Sea. Sci. Total Environ. 709, 136144. doi: 10.1016/j.scitotenv.2019.136144
Krause, G., Billing, S-L, Dennis, J., Grant, J., Fanning, L., Filgueira, R., Miller, M., et al. (2020). Visualizing the social in aquaculture: how social dimension components illustrate the effects of aquaculture across geographic scales. Mar. Policy 118, 103985. doi: 10.1016/j.marpol.2020.103985
Krause, G., Brugere, C., Diedrich, A., Ebeling, M. W., Ferse, S. C., Mikkelsen, E., et al. (2015). A revolution without people? Closing the people-policy gap in aquaculture development. Aquaculture 447, 44–55. doi: 10.1016/j.aquaculture.2015.02.009
Landmann, J., Fröhling, L., Gieschen, R., Buck, B. H., Heasman, K., Scott, N., et al. (2021). New system design for the cultivation of extractive species at exposed sites - Part 2: Experimental modelling in waves and currents. Appl. Ocean Res. 113, 102749. doi: 10.1016/j.apor.2021.102749
Leach, M., Reyers, B., Bai, X., Brondizio, E. S., Cook, C., Díaz, S., et al. (2018). Equity and sustainability in the Anthropocene: a social–ecological systems perspective on their intertwined futures. Global Sustain. 1, e13. doi: 10.1017/sus.2018.12
Lee, J., Park, K.-T., Lim, J.-H., Yoon, J.-E., and Kim, I.-N. (2018). Hypoxia in Korean coastal waters: a case study of the natural Jinhae Bay and Artificial Shihwa Bay. Front. Mar. Sci. 5, 70. doi: 10.3389/fmars.2018.00070
Lester, S. E., Gentry, R. R., Kappel, C. V., White, C., and Gaines, S. D. (2018). Offshore aquaculture in the United States: Untapped potential in need of smart policy. PNAS 115, 7162–7165. doi: 10.1073/pnas.1808737115
Levin, K., Cashore, B., Bernstein, S., and Auld, G. (2012). Overcoming the tragedy of super wicked problems: Constraining our future selves to ameliorate global climate change. Policy Sci. 45, 123–152. doi: 10.1007/s11077-012-9151-0
Leydesdorff, L. (2000). The triple helix: an evolutionary model of innovations. Res. Policy 29, 243–255, doi: 10.1016/S0048-7333(99)00063-3
Llorente, I., Fernández-Polanco, J., Baraibar-Diez, E., Odriozola, M. D., Bjørndal, T., Asche, F., et al. (2020). Assessment of the economic performance of the seabream and seabass aquaculture industry in the European Union. Mar. Policy 117, 103876. doi: 10.1016/j.marpol.2020.103876
Mather, C., and Fanning, L. (2019). Social licence and aquaculture: towards a research agenda. Mar. Policy 99, 275–282. doi: 10.1016/j.marpol.2018.10.049
Merrie, A., Keys, P., Metian, M., and Österblom, H (2018). Radical ocean futures-scenario development using science fiction prototyping. Futures 95, 22–32. doi: 10.1016/j.futures.2017.09.005
Moscicki, Z., Dewhurst, T., Macnicoll, M., Lynn, P., Sullivan, C., Chambers, M., et al. (2021). Using Finite Element Analysis for the Design of a Modular Offshore Macroalgae Farm. MARINE - Computational Methods in Marine Engineering XVII.
Naylor, R. L., Hardy, R. W., Buschmann, A. H., Bush, S. R., Cao, L., Klinger, D. H., et al. (2021). A 20-year retrospective review of global aquaculture. Nature 591, 551–563. doi: 10.1038/s41586-021-03308-6
Newbold, T., Hudson, L. N., Arnell, A. P., Contu, S., De Palma, A., Ferrier, S., et al. (2016). Has land use pushed terrestrial biodiversity beyond the planetary boundary? A global assessment. Science 353, 288–291. doi: 10.1126/science.aaf2201
Nijdam, D., Rood, T., and Westhoek, H. (2012). The price of protein: review of land use and carbon footprints from life cycle assessments of animal food products and their substitutes. Food Policy 37, 760–770. doi: 10.1016/j.foodpol.2012.08.002
O'Brien, K. (2012). Global environmental change II: from adaptation to deliberate transformation. Prog. Hum. Geogr. 36, 667–676. doi: 10.1177/0309132511425767
Österblom, H., Crona, B. I., Folke, C., Nyström, M., and Troell, M. (2017). Marine ecosystem science on an intertwined planet. Ecosystems 20, 54–61. doi: 10.1007/s10021-016-9998-6
Oyinlola, M. A., Reygondeau, G., Wabnitz, C. C. C., Troell, M., and Cheung, W. W. L. (2018). Global estimation of areas with suitable environmental conditions for mariculture species. PLoS ONE 13, e0191086. doi: 10.1371/journal.pone.0191086
Patterson, J., Schulz, K., Vervoort, J., van der Hel, S., Widerberg, O., Adler, C., et al. (2017). Exploring the governance and politics of transformations towards sustainability. Environ. Innovat. Soc. Trans. 24, 1–16. doi: 10.1016/j.eist.2016.09.001
Petersen, J. K., Holmer, M., Termansen, M., and Hasler, B. (2019). “Nutrient extraction through bivalves” in Goods and Services of Marine Bivalves, eds A. Smaal, J. Ferreira, J. Grant, J. Petersen, Ø. Strand (Cham: Springer).
Petersen, J. K., Saurel, C., Nielsen, P., and Timmermann, K. (2016). The use of shellfish for eutrophication control. Aquacult. Int. 24, 857–878. doi: 10.1007/s10499-015-9953-0
Poore, J., and Nemecek, T. (2018). Reducing food's environmental impacts through producers and consumers. Science 360, 987–992. doi: 10.1126/science.aaq0216
Porse, H., and Rudolph, B. (2017). The seaweed hydrocolloid industry: 2016 updates, requirements, and outlook. J. Appl. Phycol. 29, 2187–2200. doi: 10.1007/s10811-017-1144-0
Pribadi, A. B. K., Donatini, L., and Lataire, E. (2019). Numerical modelling of a mussel line system by means of lumped-mass approach. J. Mar. Sci. Eng. 7, 309. doi: 10.3390/jmse7090309
Raworth, K. (2017). Doughnut Economics: Seven Ways to Think Like a 21st-Century Economist (London: Chelsea Green Publishing).
Rayner, R., Jolly, C., and Gouldman, C. (2019). Ocean observing and the blue economy. Front. Mar. Sci. 6, 330. doi: 10.3389/fmars.2019.00330
Robb, D. H. F., MacLeod, M., Hasan, M. R., and Soto, D. (2017). Greenhouse Gas Emissions From Aquaculture: A Life Cycle Assessment of Three Asian Systems. Rome: FAO. Available online at: https://www.fao.org/documents/card/en/c/056ca0ea-e495-45bc-b23e-91fa4a370a34/ (accessed June 25, 2021).
Rockström, J., Gaffney, O., Rogelj, J., Meinshausen, M., Nakicenovic, N., and Schellnhuber, H. J. (2017). A roadmap for rapid decarbonization. Science 355, 1269–1271. doi: 10.1126/science.aah3443
Rodil, R., Villaverde-de-Sáa, E., Cobas, J., Quintana, J. B., Cela, R., and Carro, N. (2019). Legacy and emerging pollutants in marine bivalves from the Galician coast (NW Spain). Environ. Int. 129, 364–375. doi: 10.1016/j.envint.2019.05.018
Scoones, I., Stirling, A., Abrol, D., Atela, J., Charli-Joseph, L., Eakin, H., et al. (2020). Transformations to sustainability: combining structural, systemic and enabling approaches. Curr. Opin. Environ. Sustain. 42, 65–75. doi: 10.1016/j.cosust.2019.12.004
Shindler, B. A., Brunson, M., and Stankey, G. H. (2002). Social Acceptability of Forest Conditions and Management Practices: A Problem Analysis. (Gen. Tech. Rep. PNW-GTR-537. Portland, OR: U.S. Department of Agriculture, Forest Service, Pacific Northwest Research Station. 68 p.
Silver, J., Gray, N. J., Campbell, L. M., Fairbanks, L. W., and Gruby, R. L. (2015). Blue economy and compeiting disoucrse in interantional oceans governance. J. Environ. Dev. 24, 135–160. doi: 10.1177/1070496515580797
Simpson, S. (2011). The blue food revolution. Sci. Am. 304, 54–61. doi: 10.1038/scientificamerican0211-54
Song, J., and Duan, L. (2019). “The yellow sea” in World Seas: An Environmental Evaluation. Volume II: the Indian Ocean to the Pacific, ed C. Sheppard (Elsevier Publication), chap. 18, 395–413.
Steffen, W., Persson, A., Deutsch, L., Zalasiewicz, J., Williams, M., Richardson, K., et al. (2011). The anthropocene: from global change to planetary stewardship. Ambio 40, 739–761. doi: 10.1007/s13280-011-0185-x
Steffen, W., Richardson, K., Rockström, J., Cornell, S. E., Fetzer, I., Bennett, E. M., et al. (2015). Sustainability. Planetary boundaries: guiding human development on a changing planet. Science 347, 1259855. doi: 10.1126/science.1259855
Steinberg, P., and Peters, K. (2015). Wet ontologies, fluid spaces: giving depth to volume through oceanic thinking. Environ. Plan. D Soc. Space 33, 247–264. doi: 10.1068/d14148p
Stevens, J. R., Newton, R. W., Tlusty, M., and Little, D. C. (2018). The rise of aquaculture by-products: Increasing food production, value, and sustainability through strategic utilisation. Mar. Policy 90, 115–124. doi: 10.1016/j.marpol.2017.12.027
St. Gelais, A. T., Fredriksson, D. W., Dewhurst, T., Miller-Hope, Z. S., Costa-Pierce, B. A., and Johndrow, K. (2022). Engineering a low-cost kelp aquaculture system for community-scale seaweed farming at nearshore exposed sites via user-focused, social ecological design process. Front. Sustain. Food Syst. 6, 848035. doi: 10.3389/fsufs.2022.848035
Sverdrup, H., Koca, D., and Ragnarsdottir, K. V. (2013). Peak metals, minerals, energy, wealth, food and population: urgent policy considerations for a sustainable society. J. Environ. Sci. Eng. 499–533.
The Nature Conservancy (2021). Global Principles of Restorative Aquaculture. Arlington, VA: The Nature Conservancy.
The Nature Conservancy (2022). Global Principles of Restorative Aquaculture. Arlington, VA: The Nature Conservancy.
Theuerkauf, S. J., Barrett, L. T., Alleway, H. K., Costa-Pierce, B. A., St. Gelais, A., and Jones, R. C. (2021). Habitat value of bivalve shellfish and seaweed aquaculture for fish and invertebrates: pathways, synthesis and next steps. Rev. Aquacult. 14, 54–72. doi: 10.1111/raq.12584
Theuerkauf, S. J., Morris, JA, Waters, T. J., Wickliffe, L. C., Alleway, H. K., and Jones, R. C. (2019). A global spatial analysis reveals where marine aquaculture can benefit nature and people. PLoS ONE 14, e0222282. doi: 10.1371/journal.pone.0222282
Thomas, J. B. E., Silva Ramos, F., and Gröndahl, F. (2019). Identifying suitable sites for macroalgae cultivation on the Swedish West Coast. Coastal Manage. 47, 88–106. doi: 10.1080/08920753.2019.1540906
Thomas, J. B. E., Sinha, R., Strand, Å., Söderqvist, T., Stadmark, J., Franzen, F., et al. (2021). Marine biomass for a circular blue-green bioeconomy? A life cycle perspective on closing nitrogen and phosphorus land-marine loops. J. Indus. Ecol. 1–18. doi: 10.1111/jiec.13177
Troell, M., Jonell, M., and Henriksson, P. (2017). Ocean space for seafood. Nat. Ecol. Evol. 1, 1224–1225. doi: 10.1038/s41559-017-0304-6
Troell, M., Naylor, R. L., Metian, M., Beveridge, M., Tyedmers, P. H., Folke, C., et al. (2014). Does aquaculture add resilience to the global food system? PNAS 111, 13257–13263. doi: 10.1073/pnas.1404067111
Troell, M., Henriksson, P. J. G., Buschmann, A. H., Chopin, T., and Quahe, S. (2022). Farming the ocean – seaweeds as a quick fix for the climate? Rev. Fish. Sci Aquac. 1–11. doi: 10.1080/23308249.2022.2048792
UN (2019). World Population Prospects of the UN (2019). Probabilistic Population Projections Rev. 1 based on the World Population Prospects 2019 Rev. 1. Available online at: http://population.un.org/wpp/ (accessed Auguest 31, 2021).
van der Schatte Olivier, A., Jones, L., Le Vay, L., Christie, M., Wilson, J., and Malham, S. K. (2018). A global review of the ecosystem services provided by bivalve aquaculture. Rev. Aquacult. 12, 3–25. doi: 10.1111/raq.12301
Warszawski, L., Kriegler, E., Lenton, T. M., Gaffney, O., Jacob, D., Klingenfeld, D., et al. (2021). All options, not silver bullets, needed to limit global warming to 1.5° C: a scenario appraisal. Environ. Res. Lett. 16, 064037. doi: 10.1088/1748-9326/abfeec
Willett, W., Rockström, J., Loken, B., Springmann, M., Lang, T., Vermeulen, S., et al. (2019). Food in the anthropocene: the EAT–lancet commission on healthy diets from sustainable food systems. Lancet Commission 393, 447–492. doi: 10.1016/S0140-6736(18)31788-4
Wittmer, H., Berghöfer, A., Büttner, L., Chakrabarty, R., Förster, J., Khan, S., et al. (2021). “Transformative change for a sustainable management of global commons — biodiversity, forests and the ocean. Recommendations for international cooperation based on a review of global assessment reports and project experience,” in UFZ-Report 3/2021.
Wright, A. C., Fan, Y., and Baker, G. L. (2018). Nutritional value and food safety of bivalve molluscan shellfish. J. Shellfish Res. 37, 695–708. doi: 10.2983/035.037.0403
Xie, H., Du, J., and Chen, J. (2020). Concerted efforts are needed to control and mitigate antibiotic pollution in coastal waters of China. Antibiotics 9, 88. doi: 10.3390/antibiotics9020088
Keywords: marine aquaculture, food security, zero-carbon, transdisciplinarity, blue transformation, narrative, private sector
Citation: Krause G, Le Vay L, Buck BH, Costa-Pierce BA, Dewhurst T, Heasman KG, Nevejan N, Nielsen P, Nielsen KN, Park K, Schupp MF, Thomas J-B, Troell M, Webb J, Wrange AL, Ziegler F and Strand Å (2022) Prospects of Low Trophic Marine Aquaculture Contributing to Food Security in a Net Zero-Carbon World. Front. Sustain. Food Syst. 6:875509. doi: 10.3389/fsufs.2022.875509
Received: 14 February 2022; Accepted: 06 May 2022;
Published: 26 May 2022.
Edited by:
Pankaj Kumar Arora, Babasaheb Bhimrao Ambedkar University, IndiaReviewed by:
Michael Phillips, WorldFish, MalaysiaBerchie Asiedu, University of Energy and Natural Resources, Ghana
Copyright © 2022 Krause, Le Vay, Buck, Costa-Pierce, Dewhurst, Heasman, Nevejan, Nielsen, Nielsen, Park, Schupp, Thomas, Troell, Webb, Wrange, Ziegler and Strand. This is an open-access article distributed under the terms of the Creative Commons Attribution License (CC BY). The use, distribution or reproduction in other forums is permitted, provided the original author(s) and the copyright owner(s) are credited and that the original publication in this journal is cited, in accordance with accepted academic practice. No use, distribution or reproduction is permitted which does not comply with these terms.
*Correspondence: Gesche Krause, gesche.krause@awi.de