- 1School of Chemistry and Chemical Engineering, Guangzhou University, Guangzhou, China
- 2Peats Soil and Garden Supplies, Whites Valley, SA, Australia
- 3Institute for Nano Scale and Technology, College of Science and Engineering, Flinders University, Bedford Park, SA, Australia
- 4College of Engineering, Information Technology & Environment, Charles Darwin University, Darwin, NT, Australia
- 5Institute of Food Science and Technology, Khwaja Fareed University of Engineering and Information Technology, Rahimyar Khan, Pakistan
- 6Institute of Bioresource and Agriculture, Hong Kong Baptist University, Hong Kong, China
- 7Faculty of Agriculture and Life Sciences, Lincoln University, Lincoln City, New Zealand
- 8School of Food Science and Engineering, South China University of Technology, Guangzhou, China
To evaluate the efficiency of the oil-reducing properties of kombucha cellulose, enzymolysis and microwave-assisted enzymolysis methods were developed. The water-holding capacity of the kombucha cellulose hydrolysates formed by these two methods was higher than for the intact kombucha cellulose, while the oil-holding capacity was lower. The hydrolysates of kombucha cellulose and the intact kombucha cellulose were used to make deep-fried donuts. During this process, kombucha cellulose hydrolysates were added instead of 2% flour, and from the results, the oil content of the donut decreased significantly from ~28 to 15%, and the reduction was not related to the processing of the donut. The hardness and brittleness of all samples showed no significant change, and these samples had similar internal micro-structures, confirming texture profile analysis. In vitro digestion results suggested that there would be no adverse health effects from substituting kombucha cellulose hydrolysates in the deep-fried donut formula.
Introduction
Kombucha is a functional fermented tea with a sweet and sour taste. This kind of beverage usually contains sugars, polyphenols, organic food acids, fibers, ethanol, amino acids, vitamins, and essential minerals (Kapp and Sumner, 2018). It is a symbiotic colony of yeasts (mainly Acetobacter xylinoides and Acetobacter ketogenum) and bacteria (Tran et al., 2020). It was first produced in the northeast of China around 220 BC, and in recent years, it has gained tremendous popularity in Europe and America (Kim and Adhikari, 2020). Kombucha is a functional beverage, which has shown the fastest growth in the markets due to its beneficial effect on human health (Kim and Adhikari, 2020). There has been an increase of up to 30% annually to kombucha retail sales worldwide since 2017, and over the next few years, the market in the United States is expected to expand at a pace of 17.5%. During fermentation, a by-product, kombucha cellulose hydrolysates, is formed by acetic acid bacteria as a cellulose biological film in the liquid–air interface. Unlike the celluloses derived from plants, it shows excellent mechanical strength, a high degree of water absorption, high purity, and a high degree of polymerization, and it does not contain hemicellulose or lignin (Oliveira et al., 2017). Kombucha cellulose was first regarded as a waste product in the process of fermentation, although this biomaterial has recently been applied to different fields due to its diverse physical and chemical properties. For example, it has been used in forming hydrogel cellulose (Esa et al., 2014) and in the pyrolytic generation of graphene oxide (Amarasekara and Wang, 2021). It has also been shown to reduce Escherichia coli in the dairy shed effluent (Laurenson et al., 2021) and is used to fabricate electrolyte membranes (Vilela et al., 2018). However, in these previous studies, structural modifications of the cellulose have been chemically derived, and there has been no investigation of kombucha cellulose as a functional food.
Deep frying is a quick and suitable food preparation process. It provides distinctive sensory properties, making the food crunchy on the outside, with a soft and moist interior that customers from different countries love. There are many typical deep-fried desserts in Western countries, and the deep-fried donut is prominent among them. Customers favor the fried donut due to its unique flavor, crispy texture, and sweet taste (Saguy and Dana, 2003).
Because there is a great amount of oil in deep-fried food products, it is considered unhealthy. Studies have found that an excessive oil intake can cause hypertension and obesity (Peter et al., 2018). The oil content of raw fish can be as much as 190 g/kg, up from approximately 20 g/kg after frying (Barbara et al., 2016), while the oil content of deep-fried chips can reach approximately 400 g/kg (Kita and Golubowska, 2007). In general, after deep frying, the oil content of food can reach up to one-third of its overall weight (Mia et al., 2017). According to research, the oil level in deep-fried food products may be successfully lowered by using specific substances in the batter formula. For instance, adding 10 ml/L methylcelluloses and 5 ml/L sorbitol into the batter of deep-fried potatoes can significantly decrease its oil content by 40.6% (Bo et al., 2017). Another study increased the proportion of wheat flour from 1 to 5% in soybean hull-coated frying batter, and the oil content of the final deep-fried food was reduced from 244 to 33 g/kg (Oke et al., 2018). However, few studies have been reported on decreasing the oil content in deep-fried foods and the effects of water- and oil-holding capacities of FPH on the oil content of deep-fried foods.
In this study, cellulose from kombucha was applied as a sample. This study aims to analyze the several preparation procedures for the water-holding and oil-holding capabilities of hydrolysates made from kombucha cellulose. Furthermore, we report the impacts of kombucha cellulose hydrolysates on the reduction of oil content and the texture profiles for deep-fried foods.
Materials and methods
Materials
Constituents bought from a local market were used to prepare the kombucha. First, a sugared liquid solution was prepared by adding 100 g of sucrose in 800 ml of distilled water and heated for 15 min at 98°C. At first, 4 g green tea was soaked in the sugared liquid for 12 min and then filtered to remove leaves. The mixture's temperature was reduced to 25°C, then inoculated with 600 ml kombucha (Shenzhen Care Pack Co. Ltd.) to begin fermentation. The cellulose film for kombucha was produced after a 15-day fermentation of the culture.
Kombucha cellulose purification
The film was dipped in distilled water for 2 days. Filtration of kombucha was performed by the Buchner funnel to eliminate bacteria and impurities from the mixture. Then, the filtered sample was treated with 1 M NaOH at 50°C for 12 h, which was then neutralized for 1 h by using 1% glacial acetic acid. A neutral pH of washing water was achieved by washing the kombucha cellulose in distilled water and freeze-dried it for further use.
Kombucha cellulose hydrolysates produced using the enzymatic treatment and microwave-intensified enzymatic treatment
Commercial cellulase was used to perform the enzymatic treatment. The cellulase enzyme activity in the kombucha–cellulose mixture with a concentration of 10% (w/v) was 300 leucine aminopeptidase units per gram (LAPU/g). One aminopeptidase unit per gram (LAPU/g) is the amount of enzyme that hydrolyzes 1 μmol of leucine-p-nitroanilide in 60 s. To reach these optimal conditions, the kombucha–cellulose mixture temperature was set to 50°C and the pH was set to 7–8 (adjusted to a neutral pH in 2.2). A water bath was used to perform a 1-h enzymatic treatment with an enzyme:substrate ratio of 1.0% (w/w). A microwave synthesis laboratory station (Milestone S.r.L, Sorisole (BG), Italy) was used to perform the microwave-intensified enzymatic treatment at 500 W. The enzyme:substrate (E:S) ratio was set at 1.0% (w/w) for 10 min.
Following each process, enzyme activity was restricted by boiling at 100°C for 10 min. The processed hydrolyzed solution was centrifuged at 4,000 × g for 30 min, resulting in two layers: an unhydrolyzed, insoluble cellulose precipitate layer at the bottom and a hydrolyzed, soluble cellulose hydrolysate liquid layer on the top. The top layer was collected and freeze-dried. Cellulose hydrolysate powder was stored in sealed 50 ml tubes in a desiccator at room temperature.
Determination of oil-holding capacity
Determination of the oil-holding capabilities was done with the method by Zhang et al. (2015). In brief, the 1 g sample was weighed in a centrifuge tube. A total of 9 g of canola oil was added and well mixed using a vortex mixer and subsequently allowed to stand at room temperature. The oil-holding capacity was recorded by calculating the weight of the oil that was absorbed by the 1 g sample, compared with the original 9 g of canola oil.
Determination of water-holding capacity test
Bowker and Zhuang (2015) method, with a few modifications, was used to determine the water-holding capacity. A total of 1 g of sample was mixed with 5 g of ground fresh carp fish mince, and then added 8 g of tap water. Further, the sample was incubated at 50°C for 1 h, followed by centrifugation at 340 × g for 10 min, and the weight of the supernatant was recorded. The weight difference between the supernatant and added water measured the water-holding capacity.
Particle size distribution of kombucha cellulose and kombucha cellulose hydrolysates
Dynamic light scattering (DLS) (Nano ZS90, Malvern instruments, Worcester, UK), was applied to measure the particle size distribution. The freeze-dried samples were crushed and dispersed in water with a concentration of 2% (w/v) for DLS studies. Based on the particle sizes, the time-dependent fluctuations of light scattered were measured using a Malvern zeta-sizer instrument.
Deep-fried donut production
The dough and donut were prepared by the proposed method of Sirichokworrakita et al. (2015) with slight modifications. To prepare the control sample, 3 g of yeast was weighed, and 10 ml of water was added and incubated for 20 min. Further, the dough's control sample was prepared by adding 40 g of sugar, 2 g of salt, and 3 g of yeast to the 100 g of flour and mixed until the dough base was fully developed. As for the other samples, 2 g of 100 g flour was replaced with kombucha cellulose hydrolysates or kombucha cellulose. In a pan, 60 ml of milk and 15 g of butter were heated. Melted butter was added to the dough mixer. Then, two drops of vanilla extract and a whole egg (110 g) were added in a mixing pan followed by adding the sunflower oil. The dough was covered with a bowl cover and incubated for 2 h at room temperature. Once the dough volume increased, it was placed on a clean flat surface. The 3 cm diameter round donut cutter was used to shape the donuts after being rolled to a thickness of 1.27 cm. Then, donuts were deep fat fried into 2 L of sunflower oil (purchased from a local market) approximately for 4 min at 150°C (Zolfaghari et al., 2013; Arash et al., 2018). Furthermore, we took out the donuts from the frying pan and drained any remaining oil using absorbent paper. The processed donuts were cooled at room temperature, placed in high-density polyethylene, and kept for further analysis.
Measurement of texture profile analysis
Texture profile analysis (TPA) was used to measure deep-fried donuts' hardness (g) and brittleness (mm). After deep frying, the donuts were cut immediately into a cylindrical form. The height of the cut samples was 2 cm, and the diameter was 2.5 cm. Further, the texture of the samples was analyzed by putting them in a specimen of texture analyzer, Perten Instruments 31, SE-126, 53, Hagersten, Sweden) and analysis was performed with a speed of 2.0, 1.0, and 2.0 mm/s, for pre-test, test, and post test, respectively; and 50% sample deformation. After each compression, the force deformation graph was recorded by the analyzer to calculate the hardness and brittleness of the samples (Figure 1).
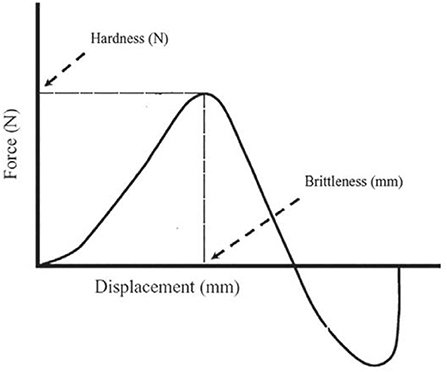
Figure 1. Instrumental texture analysis yielded a force–displacement curve that displayed hardness and brittleness.
Determination of donut internal micro-structure
The donut's internal structure was measured with a scanning electron microscope (SEM) (Alto 2100, Gatan Ltd., Abingdon, UK), and by the method followed by Vos et al. The colonial-shaped samples were cut by a Stanley knife with a diameter of 1.5 mm × 1.5 mm, and samples adhered to gold stage. Further, the samples were frozen in liquid nitrogen. In the sample preparation room, the head of the specimen shattered, and furthermore, the sublimation of samples was done at −90°C. The internal structure of the samples was analyzed at 30 kV with 5.0 spot values to see and evaluate the interior micro-structure.
Determination of water and oil contents
The water and oil content was determined with the ethylene to deaminate AOAC procedures 950.46 and 960.3928, respectively.
In vitro digestion
The in vitro digestibility of the sample (donut) was determined with a method by Gao et al. (2016). In brief, the 2.5 g of doughnut and 30 ml of distilled water were mixed in a biopsy pot. Further, we put in a heated magnetic stirring block (IKAAG RT 15, IKA-Werke GmbH & Co., Staufen, Germany), and after 10 min of stirring at 37 °C, we prepared the solution with 0.8 ml HCL (1 M) and 1 ml pepsin (10%) and added in 0.05 M HCl to imitate the stomach digestion. Then we stopped the digestion process after 30 min using 2 ml NaHCO3 (1 M). Therefore, 5 ml sodium maleate (0.1M) buffer having pH 6, and 5 ml of 2.5% pancreatin solution in 0.1 M sodium maleate buffer with pH 6 were added to start small intestine digestion, which was continuously stirred for ~120 min. Moreover, we took 1 ml aliquots before the addition of the pancreatin and added them to 4 ml ethanol at different time intervals such as 20, 60, and 120 min. To analyze reducing sugar content [3,5-dinitrosalicylic acid (DNS) method], samples were kept at 4°C. Allotted into trapezoids, the graph area under the curve (AUC) was determined. After being freeze-dried, the digest supernatants were put in storage at 20°C.
Statistical analysis
After three replications of each measurement, the data were presented as means with standard deviations. To conduct the statistical analysis, Minitab was used to apply a one-way analysis of variance (ANOVA) and the least significant difference (LSD). The F value at a probability (p) ≤ 0.05 was used statistically to determine the significance.
Results and discussion
The oil-holding and water-holding capacities of kombucha cellulose and kombucha cellulose hydrolysates
The oil-holding capacities are shown in Table 1. Any liquid oil can be used in the measurement of oil-holding capacity with the same principle: The oil-holding capacity was recorded by calculating the weight of the oil that was absorbed by 1 g of the sample, compared with the original weight of the oil. Considering that canola oil is one of the cooking oils that is the easiest to be sourced in the market, and many previous studies used canola oil for oil-holding capacity measurement (Li et al., 2020; Hadi and Sudiyono, 2021), this study also selected canola oil for the measurement of oil-holding capacity.
The analysis stated that the oil-holding capacities of kombucha cellulose hydrolysates were significantly reduced as compared to the original kombucha cellulose (16.56 g oil/g). The lowest oil-holding capacity was shown with kombucha cellulose hydrolysates produced by microwave-intensified enzymatic treatment (2.18 g oil/g sample), which is a reduction ratio of ~88%. It has been reported that the size reduction of biomaterials may cause the reduction of its oil-holding capacities. For example, He et al. (2012) reported that the oil-holding capacity dropped from its highest capacity of 14.77 g oil/g sample to the lowest capacity of 4.45 g oil/g in fish protein after its molecular weight was reduced from above 100 kDa to 0–30 kDa via enzymatic hydrolyzation, which is a reduction ratio of ~71%. The oil-holding capacity of the kombucha cellulose hydrolysates that were produced via microwave-intensified enzymatic treatment was significantly lower than other samples (2.18 g/g in comparison with 6.11 and 16.56 g/g). The DLS measurement demonstrated a substantial reduction in particle size from kombucha cellulose (Kashcheyeva et al., 2019) to kombucha cellulose hydrolysates produced from microwave-intensified enzymatic treatment (Kashcheyeva et al., 2019) (Figure 2), which is consistent with previous findings. However, as observed by SEM (Figure 3), the kombucha cellulose showed network micro-structures cross-linked by multiple long chains of intact cellulose fibers. By contrast, after hydrolyzation, the cross-linked network micro-structure was dismantled due to the multiple long chains of intact cellulose fibers being broken down into tiny pieces through hydrolyzation and therefore unable to form a network. The better the cross-linked network of microstructures in the bio-materials, the more oil it could entrap in this micro-structure, increasing its oil-holding capacity (Tanti et al., 2016). This combinatorial effect of a reduction in particle size and dismantling of the cross-linked network micro-structure caused by enzymatic hydrolyzation explains the higher reduction ratio of the oil-holding capacity.
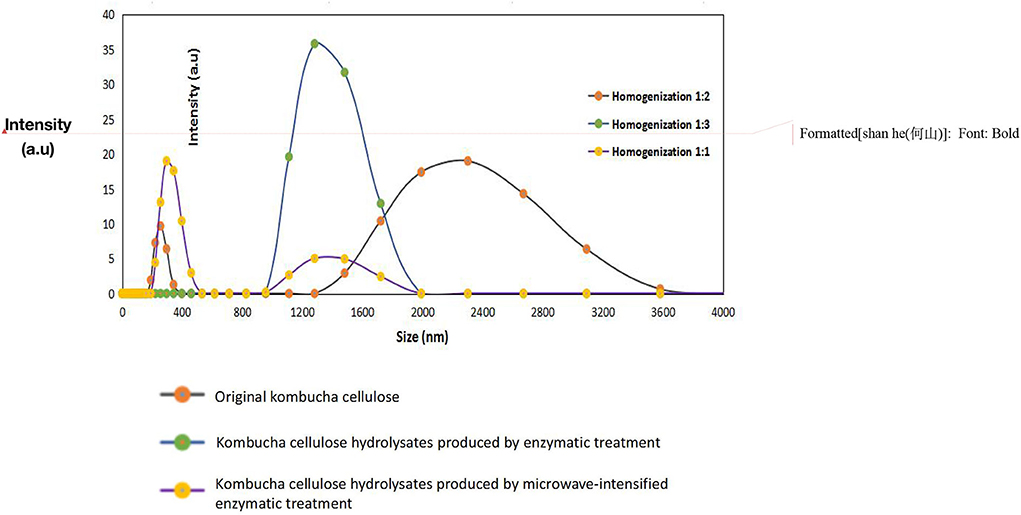
Figure 2. DLS data at 25°C (He–Ne laser 633 nm; detector angle 173°) for original kombucha cellulose, kombucha cellulose hydrolysates produced via enzymatic treatment, and kombucha cellulose hydrolysates produced using microwave-intensified enzymatic treatment.
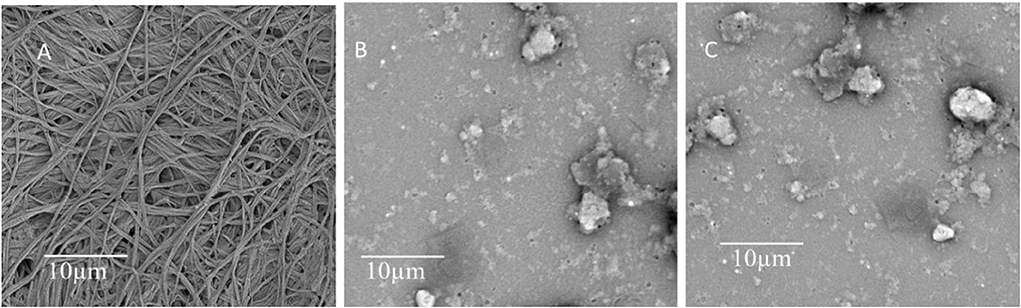
Figure 3. The internal images of (A) original kombucha cellulose. (B) Kombucha cellulose hydrolysates produced via enzymatic treatment. (C) Kombucha cellulose hydrolysates produced via microwave-intensified enzymatic treatment.
The kombucha cellulose hydrolysates showed a higher water-holding capacity than the kombucha cellulose. It has been reported that kombucha cellulose has a high level of hydrophilicity (Guzel and Akpinar, 2020). This refers to abundant hydrophilic groups of kombucha cellulose that are exposed to the surroundings. The increased hydrophilic groups during the hydrolyzation process are the reason for the improved water-holding ability of kombucha cellulose. Furthermore, a higher frequency of exposed hydrophilic groups led to a lower frequency of exposed hydrophobic groups, and after hydrolyzation, it also contributes to the reduction of oil-holding capacity.
It is noteworthy that though the microwave-intensified enzymatic processing period (10 min) was significantly shorter than the time taken for enzymatic treatment (1 h), it was more efficient in terms of hydrolyzation, as indicated by the smaller particle size and the oil-binding capacity of the kombucha cellulose hydrolysates produced. The heat is transmitted from the outside, which caused delays with traditional heating techniques (such as heating water baths). This delay was quickly reduced by the electromagnetic energy heating of microwave irradiation (Thostenson and Chou, 1999). Microwaves heat evenly from the inside samples (Su et al., 2022).
Donuts water and oil contents
Table 3 lists the water and oil content of deep-fried donuts processed using different preparation methods. The oil content of the donuts processed with kombucha cellulose hydrolysates was ~15.32%, while control samples had an oil content of 28.11%. There was no discernible change between the donut oil content of the control sample and that of the sample with the addition of the original kombucha cellulose. The oil-holding capacity is very important in food products, and ingredients with different oil-holding capacities have been studied and employed to control the oil content and structure of food products (Rajan et al., 2014). It is reasonable to believe that the oil contents of deep-fried donuts with FPH from various treatments would be positively correlated with the oil-holding capacities of samples from different treatments. However, this correlation could not be established due to the model of the deep-fried food products. First, while frying, the donut was not evenly in contact with the oil; moreover, the major inner part of the donut was not in contact with the oil. The mechanism for the volume ratio of the outside (crust) to the inner part of deep-fried foods has been studied by (Kumar et al., 2014), and the oil does not penetrate deeply into the interior during deep frying. The thickness of the food crust during deep frying is ~6 mm, while the oil in the outside crust can be 6–10 times greater than that which is absorbed by the inner parts. On average, there may be no discernible variation in the oil content of the complete deep-fried food product.
However, Table 3 indicated a positive correlation between the oil content in the donut crust and the oil-holding capacity of the samples. It is also noticeable that the oil content in the crust was much higher than that in the entire donut after deep frying (Table 3), while a lower water content was observed in the whole donut (Table 2).
The oil content of the deep-fried donuts (Table 1) and their water-holding capabilities (Table 2) were correlated. The samples' ability to retain water increases as oil concentration decreases. Because of the higher water-holding capacity, the water content after deep frying was higher. The oil content of fried food is affected by its water content. An increase in oil content is related to a reduction in water content. After the water is removed from fried food, oil can easily penetrate into it. The temperature of the food surface rapidly rises with the addition of heated oil, resulting in immediate boiling of the water on the food surface (Giuseppe and Camilla, 2015). This leads to low water content and surface porosity on the crust of the deep-fried food (Table 3). The crust structure has many voids for oil to penetrate the interior. Free water is more easily evaporated than bound water. Kombucha cellulose hydrolysates have a strong water-holding capacity. The water inside the donut evaporates more slowly, lowering the number of holes for oil to enter, resulting in low oil content.
Texture analysis of donuts
A texture analyzer was used to measure the influences of water and oil content on the texture of deep-fried donuts. While testing, the samples were compressed two times to mimic chewing behavior by a human. Amada et al. subjected different extruded snacks to TPA and a sensory test (Di et al., 2014) and analyzed the adhesiveness, hardness, fracturability, crispness, and chewiness of extruded snacks, although different snack food shapes could also cause different sensory profiles. The two most crucial parameters in the TPA test for foods produced using flour were hardness and brittleness (Amanda and Ana, 2014). Table 4 shows the hardness and brittleness of kombucha cellulose, kombucha cellulose treated with enzyme and microwave, and deep-fried kombucha cellulose without cellulose as a control sample. The results showed that the brittleness of kombucha cellulose treated by an enzyme (13.88 ± 3.60) and microwave (11.76 ± 1.71) increased as compared to control sample (10.83 ± 2.63). while it could be noticed that brittleness of microwave-assisted kombucha cellulose decreased as compared to enzymatic treatment. Further analysis of the hardness of these samples revealed that enzymatic- (17.21 ± 1.73) and microwave (17.31 ± 1.39)-treated kombucha cellulose exhibits more hardness compared to the control sample (16.88 ± 2.03). The characteristics of deep-fried donuts manufactured using various procedures, and these two parameters revealed no significant changes across samples, although the water and oil content of the deep-fried donuts made using various methods differed (Table 2). Deep-fried food products' textures are impacted mainly by their soft and wet core, while the exterior crust offers sensory qualities, that is, color and flavor. It also shows that their impact on the water lost during the deep-frying process is primarily free water (Huanghuang et al., 2017).
However, there was no more water loss from the control sample (with no additional ingredients) when compared with the deep-fried donut produced with the addition of kombucha cellulose or cellulose hydrolysates. According to (Koerten et al., 2017), a significant shift in the free water percentage from 50 to 30% had little impact on the food items' textural characteristics, and they demonstrated how the mechanically trapped water primarily influenced the food textural attributes.
Micro-structures of donuts
Scanning electron microscopy is a dependable method for exploring the inner micro-structures of matrices in food products (Koerten et al., 2017). The texture profile of the food can be determined by using structured matrices (Wilkinson et al., 2000). Shan et al. (2010) found that steam-cooked kamaboko with a pre-treatment of carp mince washed with aqueous H2O2 under optimal conditions possessed the highest degree of chewiness and hardness because of its more regular, cross-linked inner micro-structure. Figure 4 shows the images of deep-fried donuts produced using different processes. There were no significant inner structural differences, indicating the similarity of textures in deep-fried donuts produced using different methods. This finding was in accordance with the relationship between the texture of deep-fried kamaboko and its inner structure reported by Zeng et al. (2018).
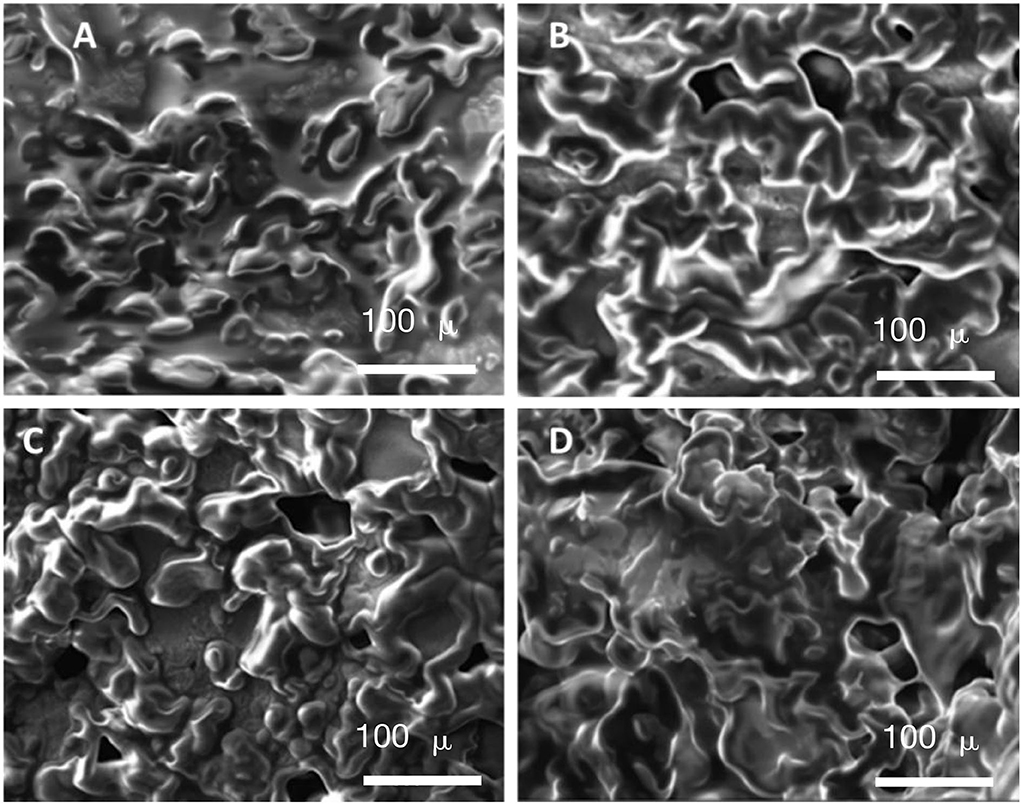
Figure 4. The micro-structures of deep-fried donuts are produced using different processing methods. (A) Kombucha cellulose, (B) Kombucha cellulose produced via enzymatic treatment, (C) Kombucha cellulose produced via microwave-intensified treatment, and (D) Control (deep-fried donuts without cellulose) using a scanning electron microscope (×3,000).
In vitro digestion
The amount of reducing sugar released by in vitro digestion of deep-fried donuts is shown in Figure 5. The risk of obesity and dental decay can be decreased by reducing the intake of sugar (Stanhope, 2016). The results of this in vitro digestion show that the release of reducing sugars was unaffected by the different treatments. This is different from protein-based materials. Xi et al. (2021) reported that in comparison with cereal protein, cereal protein hydrolysate increased the release of reducing sugars when it was applied as a food supplement in bakery products. However, the cellulose-based materials presented in this study do not show this negative health impact.
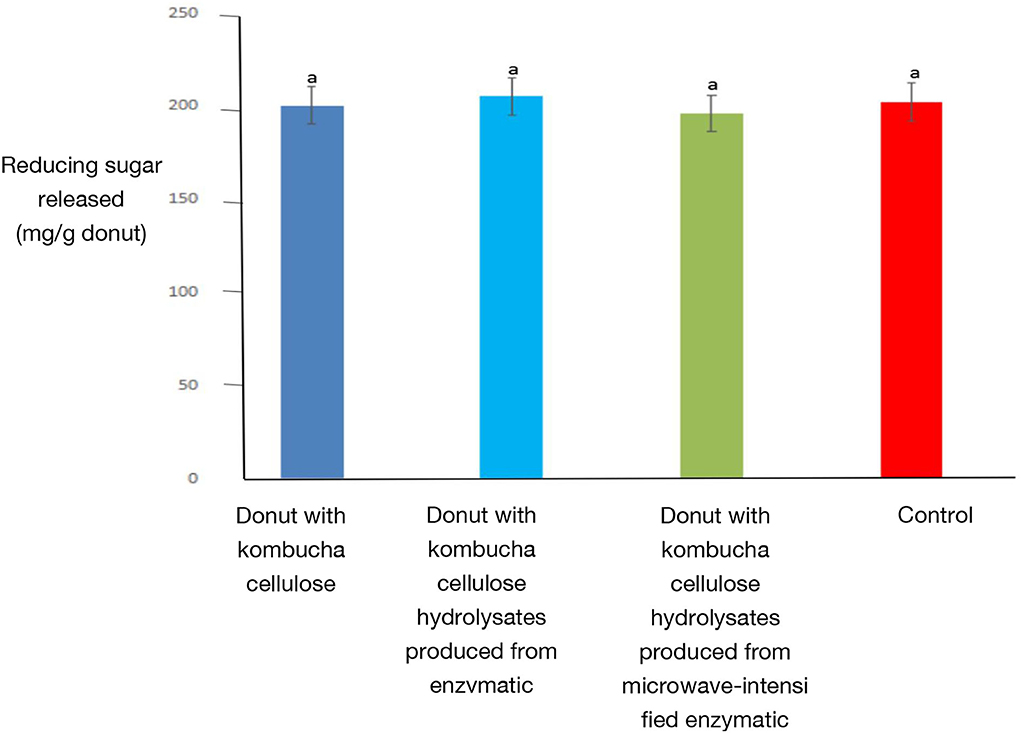
Figure 5. The AUC of deep-fried donuts produced by different treatments. Error bars represent the standard deviation of replicates (n = 3). Values with different letters indicate significant differences (p < 0.05). Minitab was used to apply a one-way analysis of variance (ANOVA) and the least significant difference (LSD).Within each trial, different letters indicate significant differences (p < 0.05), according to one-way ANOVA and LSD test.
Conclusions
Kombucha cellulose hydrolysates were developed by enzymatic treatment and a microwave-assisted enzymatic treatment as well. The water-holding and oil-holding capacities of kombucha cellulose hydrolysates created from both processes showed all higher and lower capacities, respectively, compared to intact kombucha cellulose. By replacing flour with 2% kombucha cellulose hydrolysates in the production of deep-fried donuts, the oil contents of the deep-fried donuts could be significantly decreased from ~28 to 15%. The texture profile analysis results showed slight differences, that is increasing brittleness and hardness, as compared to the deep-fried donut samples without cellulose. SEM analysis found that the deep-fried samples' inner microstructures were also similar or exhibit negligible differences. In vitro digestion test did not change the release of reducing sugars. So, this study concludes that kombucha cellulose hydrolysates in deep-fried donuts would not show adverse health effects.
Data availability statement
The raw data supporting the conclusions of this article will be made available by the authors, without undue reservation.
Author contributions
SH, YZ, JG, and CG designed the study protocol and wrote the manuscript's first draft. YW, MF, and DY conducted sample selection and data management. JW and KC managed the literature searches and analysis. BT, AK, and AR edited the manuscript. SH supervised the work. All authors contributed to and have approved the final manuscript.
Funding
The study was funded by the National Natural Science Foundation of China (32150410363), S&T projects of Guangzhou City (Project No.: 202102020731), Guangzhou City University Alliance Fundamental Research Fund (Fund No.: 20210210486), and the S&T projects of China's Ministry (QN2021163001L). The authors are also thankful for the funding provided by the German Research Foundation (DFG, Deutsche Forschungsgemeinschaft) as part of Germany's Excellence Strategy—EXC 2050/1—Project ID 390696704—Cluster of Excellence, Centre for Tactile Internet with Human-in-the Loop (CeTI) of Technische University Dresden.
Conflict of interest
Authors SH and KC shared an affiliation with Peats Soil and Garden Supplies.
The remaining authors declare that the research was conducted in the absence of any commercial or financial relationships that could be construed as a potential conflict of interest.
Publisher's note
All claims expressed in this article are solely those of the authors and do not necessarily represent those of their affiliated organizations, or those of the publisher, the editors and the reviewers. Any product that may be evaluated in this article, or claim that may be made by its manufacturer, is not guaranteed or endorsed by the publisher.
References
Amanda, M. P., and Ana, C. C. (2014). Texture profile and correlation between sensory and instrumental analyses on extruded snacks. J. Food Eng. 121, 9–14. doi: 10.1016/j.jfoodeng.2013.08.007
Amarasekara, A. S., and Wang, D. (2021). Pyrolysis route for the conversion of bacterial cellulose to graphene oxide. ACS Sustain Chem. Eng. 9, 113–119. doi: 10.1021/acssuschemeng.0c05400
Arash, G., Arash, K., Mohebbat, M., and Michael, O. N. (2018). Effect of deep fat and hot air frying on doughnuts physical properties and kinetic of crust formation. J. Cereal Sci. 83, 25–31. doi: 10.1016/j.jcs.2018.07.006
Barbara, N., Encarnacion, G., Maria, J. M., and Maria, D. G. (2016). The influence of frying technique, cooking oil and fish species on the changes occurring in fish lipids and oil during shallow-frying, studied by 1H NMR. Food Res. Int. 84, 150–159. doi: 10.1016/j.foodres.2016.03.033
Bo, J., Daming, F., Jinwei, L., Zhanhua, D., and Liuping, F. (2017). Effect of Guar gum with sorbitol coating on the properties and oil absorption of French fries. Int. J. Mol. Sci. 18, 2700. doi: 10.3390/ijms18122700
Bowker, B., and Zhuang, H. (2015). Relationship between water-holding capacity and protein denaturation in broiler breast meat. Poult. Sci. 94, 1657–1664. doi: 10.3382/ps/pev120
Di, W., De-Wen, S., and Yong, H. (2014). Novel non-invasive distribution measurement of texture profile analysis (TPA) in salmon fillet by using visible and near infrared hyperspectral imaging. Food Chem. 145, 417–426. doi: 10.1016/j.foodchem.2013.08.063
Esa, F., Tasirin, S. M., and Rahman, N. A. (2014). Overview of bacterial cellulose production and application. Agric. Agric. Sci. Procedia 2, 113–119. doi: 10.1016/j.aaspro.2014.11.017
Gao, J., Brennan, M. A., Mason, S. L., and Brennan, C. S. (2016). Effect of sugar replacement with stevianna and inulin on the texture and predictive glycaemic response of muffins. Int. J. Food Sci. Technol. 51, 1979–1987. doi: 10.1111/ijfs.13143
Giuseppe, L., and Camilla, M. (2015). Fried food and prostate cancer risk: systematic review and meta-analysis. Int. J. Food Sci. Nutr. 66, 587–589. doi: 10.3109/09637486.2015.1056111
Guzel, M., and Akpinar, O. (2020). Preparation and characterization of bacterial cellulose produced from fruit and vegetable peels by Komagataeibacter hansenii GA2016. Int. J. Biol. Macromol. 162, 1579–1604. doi: 10.1016/j.ijbiomac.2020.08.049
Hadi, R. F., and Sudiyono, Wati, A. K. (2021). Physical quality of local duck meat with addition of different vegetable oils in the ration. IOP Conf. Ser. Earth Environ. 637, 012083. doi: 10.1088/1755-1315/637/1/012083
He, S., Franco, C., and Zhang, W. (2012). Process optimisation and physicochemical characterisation of enzymatic hydrolysates of proteins from co-products of Atlantic Salmon (Salmo salar) and Yellowtail Kingfish (Seriola lalandi). Int. J. Food Sci. 47, 2397–2404.
Huanghuang, H., Jing, Y., Xueqing, Z., Yayan, H., Xiaohui, L., and Meitian, X. (2017). K-Carrageenan/locust bean gum as hard capsule gelling agents. Carbohydr. Polym. 175, 417–424. doi: 10.1016/j.carbpol.2017.07.049
Kapp, J. M., and Sumner, W. (2018). Kombucha: a systematic review of the empirical evidence of human health benefit. Ann. Epidemiol. 30, 66–70. doi: 10.1016/j.annepidem.2018.11.001
Kashcheyeva, E. I., Gladysheva, E. K., Skiba, E. A., and Budaeva, V. V. (2019). A study of properties and enzymatic hydrolysis of bacterial cellulose. Cellulose 26, 2255–2265. doi: 10.1007/s10570-018-02242-7
Kim, J., and Adhikari, K. (2020). Current trends in kombucha: marketing perspectives and the need for improved sensory research. Beverages. 6, 15. doi: 10.3390/beverages6010015
Kita, A., and Golubowska, G. (2007). The effect of oils and frying temperatures on the texture and fat content of potato crisps. Food Chem. 102, 1–5. doi: 10.1016/j.foodchem.2005.08.038
Koerten, K. N. V., Schutyser, M., Somsen, D., and Boom, R. (2015). A pore inactivation model for describing oil uptake of French fries during pre-frying. J. Food Eng. 146, 92–98. doi: 10.1016/j.jfoodeng.2014.09.010
Koerten, K. N. V., Somsen, D., Room, R. M., and Schutyser, A. (2017). Modelling water evaporation during frying with an evaporation dependent heat transfer coefficient. J. Food Eng. 197, 60–67. doi: 10.1016/j.jfoodeng.2016.11.007
Kumar, K. S., Ganaesan, K., Selvarag, K., and Rao, P. S. (2014). Studies on thefunctional properties of protein concentrate of Kappaphycus alvarezii (Doty) doty - an edible seaweed. Food Chem. 153, 353–360. doi: 10.1016/j.foodchem.2013.12.058
Laurenson, S., Villamizar, L., Ritchie, W., Scott, S., and Mackay, M. (2021). Novel use of Kombucha consortium to reduce Escherichia coli in dairy shed effluent. J. Sci. Food Agric. 101, 6143–46. doi: 10.1002/jsfa.11250
Li, X., John, S., Martin, S., Sophia, J. X., and John, L. (2020). Effects of pretreatments on physicochemical and structural properties of proteins isolated from canola seeds after oil extraction by supercritical-CO2 process. LWT. 2020, 137. doi: 10.1016/j.lwt.2020.110415
Mia, K., Mario, S., and Kata, G. (2017). Edible coating minimize fat uptake in deep fat fried products: a review. Food Hydrocoll. 71, 225–235. doi: 10.1016/j.foodhyd.2017.05.006
Oke, E., Idowu, M., Sobudola, O., Adeyeye, S., and Akinsola, A. (2018). Frying of food: a critical review. J. Culin. Sci. Technol. 16, 107–127. doi: 10.1080/15428052.2017.1333936
Oliveira, J. P. D., Bruni, G. P., Lima, K. O., Halal, S. L. M. E., Rosa, G. S. D., Dias, A. R. G., et al. (2017). Cellulose fibers extracted from rice and oat husks and their application in hydrogel. Food Chem. 221, 153–160. doi: 10.1016/j.foodchem.2016.10.048
Peter, H., Yanping, L., and Hannia, C. (2018). Fried food intake and risk of nonfatalacute myocardial infarction in the Costa Rica heart study. PLoS ONE. 13, e0192960. doi: 10.1371/journal.pone.0192960
Rajan, P., Meena, K., and Abbul, B. K. (2014). Recent advances in the applications of ionic liquids in protein stability and activity: a review. Appl. Biochem. Biotechnol. 172, 3701–3720. doi: 10.1007/s12010-014-0813-6
Saguy, I. S., and Dana, D. (2003). Integrated approach to deep fat frying: engineering, nutrition, health and consumer aspects. J. Food. Eng. 56, 143–152. doi: 10.1016/S0260-8774(02)00243-1
Shan, H., Gorczyca, E., Kasapis, S., and Lopata, A. (2010). Optimization of hydrogen-peroxide washing of common carp kamaboko using response surface methodology. LWT Food Sci. Technol. 43, 765–770. doi: 10.1016/j.lwt.2009.12.012
Sirichokworrakita, S., Phetkhuta, J., and Khommoona, A. (2015). Effect of partial substitution of wheat flour with riceberry flour on quality of noodles. Procedia Soc. Behav. Sci. 197, 1006–1012. doi: 10.1016/j.sbspro.2015.07.294
Stanhope, K. L. (2016). Sugar consumption, metabolic disease and obesity: the state of the controversy. Crit. Rev. Clin. Lab. Sci. 53, 52–67. doi: 10.3109/10408363.2015.1084990
Su, T., Zhang, W., Zhang, Z., Wang, X., and Zhang, S. (2022). Energy utilization and heating uniformity of multiple specimens heated in a domestic microwave oven. Food Bioprod. Process. 132, 35–51. doi: 10.1016/j.fbp.2021.12.008
Tanti, R., Barbut, S., and Marangoni, A. G. (2016). Oil stabilization of natural peanut butter using food grade polymers. Food Hydrocoll. 61, 399–408. doi: 10.1016/j.foodhyd.2016.05.034
Thostenson, E. T., and Chou, T. W. (1999). Microwave processing: fundamentals and applications. Composites Part A. 30, 1055–1071. doi: 10.1016/S1359-835X(99)00020-2
Tran, T., Grandvalet, C., Verdier, F., Martin, A., Alexandre, H., Tourdot-Maréchal, R., et al. (2020). Microbial dynamics between yeasts and acetic acid bacteria in kombucha: impacts on the chemical composition of the beverage. Foods. 9, 963. doi: 10.3390/foods9070963
Vilela, C., Martins, A. P. C., Sousa, N., Silvestre, A. J. D., Figueiredo, F. M. L., Freire, C. S. R., et al. (2018). Poly(bis[2-(methacryloyloxy)ethyl] phosphate)/Bacterial cellulose nanocomposites:preparation, characterization and application as polymer electrolyte membranes. Appl. Sci. 8, 1145. doi: 10.3390/app8071145
Wilkinson, C., Dijksterhuis, G. B., and Minekus, M. (2000). From food structure to texture. Trends Food Sci. Technol. 11, 442–450. doi: 10.1016/S0924-2244(01)00033-4
Xi, G., Xiaodan, H., Gang, W., James, D. M., Margaret, A. B., Charles, S. B., et al. (2021). In vitro digestion characteristics of cereal protein concentrates as assessed using a pepsin-pancreatin digestion model - ScienceDirect. Food Res. Int. 152. doi: 10.1016/j.foodres.2021.110715
Zeng, Q., Dai, B., Yang, Y., Su, D., Feng, S., He, S., et al. (2018). Significant fat reduction in deep-fried kamaboko by fish protein hydrolysates derived from common carp (Cyprinus carpio). J. Sci. Food Agric. 99, 3255–3263. doi: 10.1002/jsfa.9538
Zhang, Z., Yang, Y., Tang, X., Chen, Y., and You, Y. (2015). Chemical forces and water holding capacity study of heat-induced myofibrillar protein gel as affected by high pressure. Food Chem. 188, 111–118. doi: 10.1016/j.foodchem.2015.04.129
Keywords: kombucha cellulose hydrolysates, deep-fried donut, oil content reduction, texture profile, in vitro digestion
Citation: He S, Zhang Y, Gu C, Wu Y, Farooq MA, Young DJ, Wong JWC, Chang K, Tian B, Kumari A, Rahaman A and Gao J (2022) Impact of different processing techniques on reduction in oil content in deep-fried donuts when using kombucha cellulose hydrolysates. Front. Sustain. Food Syst. 6:997097. doi: 10.3389/fsufs.2022.997097
Received: 18 July 2022; Accepted: 22 August 2022;
Published: 07 October 2022.
Edited by:
Rabia Siddique, Government College University, Faisalabad, PakistanReviewed by:
Muhammad Waheed Iqbal, Jiangsu University, ChinaOmnia Badr, Benha University, Egypt
Sunayana Syed, National Institutes of Health (NIH), United States
Copyright © 2022 He, Zhang, Gu, Wu, Farooq, Young, Wong, Chang, Tian, Kumari, Rahaman and Gao. This is an open-access article distributed under the terms of the Creative Commons Attribution License (CC BY). The use, distribution or reproduction in other forums is permitted, provided the original author(s) and the copyright owner(s) are credited and that the original publication in this journal is cited, in accordance with accepted academic practice. No use, distribution or reproduction is permitted which does not comply with these terms.
*Correspondence: Jingrong Gao, Z2FvamluZ3JvbmcyMTE2QHNpbmEuY29t
†These authors have contributed equally to this work and share first authorship