- Pathology and Mycotoxin Unit, International Institute of Tropical Agriculture (IITA), Ibadan, Nigeria
One of the most elusive food safety problems is the contamination of staple crops with the highly carcinogenic aflatoxins produced by Aspergillus section Flavi fungi. Governments, farmers, institutions, consumers, and companies demand aflatoxin solutions. Many aflatoxin management technologies exist, but their real-life use and effectiveness is determined by diverse factors. Biocontrol products based on atoxigenic isolates of A. flavus can effectively reduce aflatoxins from field to fork. However, development, testing, and registration of this technology is a laborious process. Further, several barriers prevent the sustainable use of biocontrol products. There are challenges to have the products accepted, to make them available at scale and develop mechanisms for farmers to buy them, to have the products correctly used, to demonstrate their value, and to link farmers to buyers of aflatoxin-safe crops. Developing an effective aflatoxin management technology is the first, major step. The second one, perhaps more complicated and unfortunately seldomly discussed, is to develop mechanisms to have it used at scale, sustainably, and converged with other complementary technologies. Here, challenges and actions to scale the aflatoxin biocontrol technology in several countries in sub-Saharan Africa are described with a view to facilitating aflatoxin management efforts in Africa and beyond.
1. Introduction
Crop contamination with aflatoxins has severe negative effects on public health, trade, and income (Bui-Klimke et al., 2014; Ismail et al., 2021; Saha Turna and Wu, 2021; Senghor et al., 2021). Those negative effects are more pronounced in low- and middle-income countries (LMICs) due to agricultural, social, infrastructural, and legislative challenges (Hanlon et al., 2019). Aflatoxins are produced by several spp. within Aspergillus section Flavi. A. flavus is the most common causal agent of contamination in most susceptible crops (Klich, 2007; Frisvad et al., 2019) while other Aspergillus spp. (e.g., A. parasiticus or A. minisclerotigenes) are common in certain cropping and environmental conditions (Cotty and Jaime-Garcia, 2007; Singh et al., 2020). The importance of aflatoxins cannot be overemphasized. Aflatoxin B1, the most common and dangerous of the aflatoxins, is classified as a Class 1 carcinogen by the International Agency for Research on Cancer (JECFA, 2018). Class 1 carcinogens are unequivocally highly carcinogenic compounds. Aflatoxin tolerance thresholds are very low, in parts per billion (ppb), and vary by countries and intended crop use. In high-income countries strict mechanisms and infrastructure prevent trade and consumption of contaminated foods and feeds, while in LMICs, regulations are difficult to implement, if existing (Bandyopadhyay et al., 2016; Matumba et al., 2017). In other words, most of the consumers in LMICs consume products that may be unsafe.
Although A. flavus is the major aflatoxin producer some members of this species cannot produce aflatoxins due to defects in the aflatoxin biosynthesis gene cluster (Chang et al., 2005; Adhikari et al., 2016). Isolates of A. flavus unable to produce aflatoxins have been known for over 50 years (Joffe, 1969). Hereafter isolates of A. flavus lacking abilities to produce aflatoxins are referred to as “atoxigenic,” even though they, as nearly all filamentous fungi, may produce other secondary metabolites. From the diversity in aflatoxin-producing potentials comes an opportunity: the atoxigenicity trait can be exploited as the foundation of a biocontrol technology to outcompete aflatoxin producers in the field (Mehl et al., 2012). Significantly less aflatoxins (~80–100% less) accumulate in crops when atoxigenic isolates are broadcast in the field, at the right crop stage (2–3 weeks before flowering) and using appropriate formulation and dosage (10 kg of product per ha) (Dorner, 2004; Doster et al., 2014; Bandyopadhyay et al., 2019). Biocontrol forming the cornerstone of integrated aflatoxin management programs executed at scale offers many benefits for farmers, consumers, and industries, and can contribute to control other mycotoxins because of use of good agricultural, post-harvest, and processing practices. Furthermore, aflatoxin management strategies centered on biocontrol, when scaled up, can contribute to achieve many objectives of most UN Sustainable Development Goals (Ortega-Beltran and Bandyopadhyay, 2021).
The biocontrol technology based on atoxigenic A. flavus fungi was pioneered by the Agricultural Research Service of the United States Department of Agriculture (USDA-ARS) to reduce aflatoxin in cottonseed grown in the US (Cotty et al., 2007). Later on, it was adapted for use in maize, groundnut, almond, pistachio, and fig grown in the US (Dorner, 2009; Doster et al., 2014; Ortega-Beltran et al., 2019). To date, around 5 million ha of susceptible crops have been treated in the US with the biocontrol product AF36 (Larry Antilla, personal communication). Afla-Guard®, another aflatoxin biocontrol product in the US manufactured by Syngenta, is also used extensively in maize and groundnut for several years and more recently its commercial use began on pistachio and almond. Use of biocontrol, along with other practices, allows growing susceptible crops in regions of the US with conducive conditions for high aflatoxin contamination such as in the southwest. The technology is available for large-scale use in several sub-Saharan Africa (SSA) countries and Italy and is at different stages of development in various countries, including Argentina, China, Mexico, Pakistan, and Serbia (Moral et al., 2020). In SSA countries, the technology was adapted and improved through collaborative efforts of a consortium composed by the International Institute of Tropical Agriculture (IITA), USDA-ARS, and both local and international public and private institutions (Bandyopadhyay et al., 2016; Ortega-Beltran and Bandyopadhyay, 2021). This consortium from hereon will be referred as the Aflasafe Initiative. Institutions in the Aflasafe Initiative are diverse but work closely and rely on each other's comparative advantages. To date, through over 20 years of efforts, various aflatoxin biocontrol products under the tradename Aflasafe have been developed. Fourteen Aflasafe products effective in limiting crop aflatoxin content are registered with biopesticides regulatory authorities and are commercially available for use in 10 countries while products in another 12 countries are being developed (Schreurs et al., 2019; Moral et al., 2020).1 The products are being scaled and commercialized through public-private partnerships stimulated by a science-to-scale approach (Konlambigue et al., 2020; Bandyopadhyay et al., 2022). The diffusion of the biocontrol technology in SSA resulted in improvements to the original formulation of the first aflatoxin biocontrol product, AF36, including using multiple active ingredient atoxigenic fungi (four vs. one), changing the nutritive source and carrier (sorghum grain vs. wheat grain), and a different manufacturing process (dry process vs. wet process).
Many insist on reservations for the use of the aflatoxin biocontrol technology (Ehrlich et al., 2015; Stepman, 2018; Kagot et al., 2019; Pitt, 2019; Moore, 2021). These reservations are sometimes based on perceptions [e.g., assuming that (i) aflatoxin is not a pre-harvest issue and therefore biocontrol is not needed, (ii) farmers will not adopt the technology, (iii) regulators will not approve registration, (iv) biocontrol will never be used at scale, (v) there is no interest among private sector to invest on the technology] not realities on the ground while others mention potential and/or hypothetical risks (e.g., appearance of super toxigenic strains, increased kernel rot as a result of biocontrol treatment) without acknowledging the realized benefits of treating crops with atoxigenic biocontrol products, that is, reduced aflatoxin contamination and farmers obtaining health and economic benefits. On the other hand, there are others who consider current biocontrol efforts as critical components of aflatoxin mitigation strategies (Sarrocco et al., 2019; Nelson, 2020; Matumba et al., 2021). What is more important is that actual users consider the technology vital to achieve their goals and appreciate it because other technologies (either at pre- or post-harvest stage) do not consistently result in crops with safe aflatoxin content. Several private sector companies have made considerable investments to set up factories to manufacture biocontrol products at scale and/or develop distribution networks in various SSA countries (Bandyopadhyay et al., 2022). Success stories have sparked interest in countries lacking the technology to have it developed for their own use or by industries working with susceptible crops but for which the technology must be tested, adapted, and registered (e.g., sesame, sunflower) before widespread use.
This paper emphasizes critical aspects for the aflatoxin biocontrol technology to be correctly used and adopted, and other steps required to scale sustainably after a product is developed. Those topics should be frequently considered but most biocontrol researchers in general and aflatoxin biocontrol in particular have paid little attention to them.
Barring a few exceptions, aflatoxin biocontrol efforts have stayed as concepts in the laboratory or tested on a limited scale in on-station microplot trials with few possible variables. However, much of the information that leads to practical use of aflatoxin biocontrol (and that has allowed product registration with regulatory authorities) comes not from laboratory or microplot trials but from field evaluation by large-scale and/or smallholder farmers and observations made by extension agents, policy makers, private industry, and scientists working with them. Candidate biocontrol agents (whether fungal or bacterial spp.) or compounds must be tested at farmer field level in a wide range of conditions and the results must hold true to those obtained in the controlled conditions. Then, if effective, such agents/compounds must be registered, manufactured, and made available for farmers to purchase and use. Some aflatoxin control efforts span decades (e.g., Abbas et al., 2011a,b; Alaniz Zanon et al., 2013) and, although are found promising, have not been translated into technologies that farmers use at scale. Only few aflatoxin biocontrol products (all being atoxigenic-based) have moved into actual validation, registration, and implementation: AF36 and afla-guard® in the US, 14 Aflasafe products in SSA, and AFX1® in Italy (Moral et al., 2020). To not lose years (perhaps decades) and resources, during the product development stage it is necessary to clearly define strategies (the earliest, the better) on how the technology will be made available to farmers, how and for whom it will create value, and how it will be used sustainably. Those strategies have more chances of positive outcomes when inputs of diverse stakeholders (farmers' organizations, consumers, governments, regulators, donors, scientists, industries) are incorporated into the planning and implementation, as discussed for other contexts seeking to solve plant disease problems of a multilevel complexity (Evans et al., 2020).
Millions of farmers growing susceptible crops currently lack access to effective aflatoxin management tools, including biocontrol. Hopefully aflatoxin management technologies will be rapidly made available for use by both smallholder and large-scale farmers. This would allow increased production of safe crops, reduce the health and economic burdens of contamination, maximize farmers/industries profits, and overcome the once thought insurmountable aflatoxin problem. In this paper, we summarize the strategies implemented by the Aflasafe Initiative in several SSA countries after an effective aflatoxin biocontrol product is developed, tested, registered, and licensed to a commercial partner for the technology to reach the end-users, a process that can take 5–8 years and sometimes more. We thought that it is important to share the lessons learned by the Aflasafe Initiative because these could be valuable to others in Africa and beyond who are planning or at different stages of developing diverse aflatoxin management technologies, including biocontrol. To provide context, a few questions are formulated, and commentaries are provided.
2. Context on aflatoxin and aflatoxin biocontrol
2.1. Is aflatoxin management needed/demanded in Africa?
Aflatoxin contamination of staple crops is a recurrent, common problem across Africa (Udomkun et al., 2017; JECFA, 2018; Ezekiel et al., 2021; Matumba et al., 2021) where the most severe health, trade, and income effects occur. The contamination negatively impacts the development, peace, and prosperity of affected countries (Ortega-Beltran and Bandyopadhyay, 2021) precluding millions of people to have a normal life. Many studies reporting prevalence, management options, and control prospects, and news articles stressing the importance and consequences of aflatoxin contamination and population exposure in Africa emerge continuously. It is rare for a single week to pass by without aflatoxins being in digital or print media.
Despite the multitude of articles and frequent rejections of susceptible crops within and outside Africa, the importance of aflatoxins has been questioned, sometimes because only ~25% of harvested crops contain unsafe levels. Aflatoxin levels at harvest significantly increase if post-harvest storage is sub-optimal (Hell et al., 2000; Walker et al., 2018; Ezekiel et al., 2021). It is estimated that up to 80% of susceptible crops contain varying levels of mycotoxins (Eskola et al., 2020). Clearly, effective aflatoxin mitigation strategies converging technical, institutional, and policy options are needed across Africa. Due to the importance of aflatoxins, there is an African Union Commission program (the Partnership for Aflatoxin Control in Africa2; PACA) dedicated to solving the aflatoxin problem in Africa through a comprehensive set of policies and programs. Aflatoxin policies to prevent aflatoxin contamination and exposure have been launched by Regional Economic Communities [East African Community (EAC)3 and Economic Community of West African States (ECOWAS)4], and individual Governments (e.g., Ghana5). Evidently, Governments, consumers, institutions, and industries affected by aflatoxin need development and delivery of effective technologies to reduce aflatoxin contamination and exposure. For example, the major impediment to produce ready-to-use therapeutic foods in Nigeria by UNICEF partners is to find locally produced aflatoxin-safe groundnut; UNICEF has repeatedly called for local production of safe groundnut. Therefore, a short answer is yes, aflatoxin management at both pre- and post-harvest level is needed, and many farmers, consumers, industries, NGOs, and governments across Africa demand it.
2.2. Are current atoxigenic-based aflatoxin biocontrol products effective in Africa?
Some deem the atoxigenic-based biocontrol technology as inconsistent, doubtful, and/or ineffective. This has occurred in a few cases when testing has been limited (both number of fields and area treated), or multiple formulations were evaluated, or certain environmental factors were unfavorable. Testing the technology in those scenarios may provide unpredictable results but that does not negate the effectiveness of the technology everywhere. Apart from the known effectiveness in the US (Cotty et al., 2007; Dorner, 2009; Doster et al., 2014; Grubisha and Cotty, 2015), over a decade of field effectiveness trials and commercial usage in various SSA countries has demonstrated that biocontrol products successfully limit aflatoxin in a wide range of environments and cropping conditions, predominantly within tolerance thresholds (Bandyopadhyay et al., 2019; Agbetiameh et al., 2020; Senghor et al., 2020, 2021; Ortega-Beltran et al., 2021; Mahuku et al., 2022; Ola et al., 2022). For example, nearly 100,000 ha of maize were treated in Nigeria during a 10-year period and most (>95%) of the >300,000 tons of harvested maize had <4 ppb total aflatoxin and, in general, treated crops contained >80% less aflatoxin than untreated maize. This is high level effectiveness.
Essential aspects to develop effective aflatoxin biocontrol products for use in SSA included the identification of native atoxigenic isolates, testing several isolates in multiple fields in multiple crops to select the best candidate isolates, constitute products with superior atoxigenic isolates (four per product); and testing products using a farmer participatory approach in multiple fields of multiple zones, during several years. Effectiveness of the products have been tested in maize and groundnut, and in some countries products have been tested in sorghum (Ghana, Tanzania, Kenya, Mali, Niger, Rwanda, and Sudan), sunflower (Tanzania), sesame (Sudan) and chilies (Nigeria) (Bandyopadhyay et al., 2016; Moral et al., 2020). The farmer participatory approach in hundreds of fields is critical. It allows farmers and other key national partners, including the private sector, to be involved in the product testing process and become familiar with the technology by evaluating products in their farms. It allows scientists and extension agents to listen and learn from the farmers' knowledge to improve the overall biocontrol strategy and reveals what the technology can and cannot do. Inferences from aflatoxin biocontrol effectiveness trials on few fields are unreliable because of variability in aflatoxin content in untreated fields (around 60% of the untreated crops will contain low aflatoxin levels at harvest). To reduce error, the evaluations should be conducted in many fields, over multiple agroecological zones, and preferably over multiple years. Conducting the trials in controlled microplot trials alone does not reveal the true value of the technology.
The effectiveness of the aflatoxin biocontrol technology based on atoxigenic fungi, when developed and used appropriately, is beyond proof of concept. Emphasis should be placed on use of appropriate testing protocols, further development in the commercialization pathway, including manufacturing and distribution, to effectively reduce aflatoxin contamination (Moral et al., 2020). Additionally, equally important is to determine if the technology will be indeed available for use at scale and if farmers will adopt it. On the other hand, biocontrol treatment sometimes may not result in crops with safe aflatoxin content (e.g., 20 ppb). It could be that avg. aflatoxin content in untreated crops grown in the same area was high (e.g., 500 ppb), and the biocontrol treatment was insufficient to limit aflatoxin below tolerance thresholds although in the hypothetical example a >95% reduction occurred, which is indeed a significant aflatoxin reduction. Another example: drought conditions during the application time may cause low sporulation of the active ingredients on the carrier and, subsequently, displacement of aflatoxin producers resident in the field is likely to be poor. There are many other scenarios in which biocontrol may not provide the required level of protection for a treated crop to be considered safe. Appropriate adjustments to the technology are, therefore, needed. In addition, the products will not be effective if broadcast before or after the application window, or if the dosage is inadequate. Of course, the full potential of biocontrol is unrealized when biocontrol is not integrated with other practices that discourage aflatoxin contamination in the field and throughout storage. A short answer to the question of the subtitle is: yes, aflatoxin biocontrol products are effective (80% to 100% less aflatoxins in treated crops compared to untreated crops) when developed, produced, and used appropriately, within reasonable limits for a technology based on living organisms.
2.3. Are there proposals to improve current atoxigenic-based aflatoxin biocontrol efforts?
Any technology, including aflatoxin biocontrol, must be sought to be improved. Aflatoxin biocontrol must be more effective, cheaper, and resilient using pragmatic and/or risk-taking approaches. In this vein, various research programs aim to improve the technology, predominantly looking for more effective isolates. However, proposed improvements or novel ideas must be rigorously analyzed, evaluated, and taken to practice. Then, the new and old strategies/products must be compared in large-scale testing. Atoxigenic isolates of A. flavus more effective than active ingredients of commercial products used in the US have been reported after conducting on-station microplot trials (Abbas et al., 2011a,b; Molo et al., 2019; Weaver and Abbas, 2019; Carbone, 2021) or laboratory experiments (Huang et al., 2011; Moore et al., 2019; Sweany and Damann, 2020). The practical value of those new biocontrol approaches in commercial fields and/or storage conditions must be tested and demonstrated. Aflatoxin control products/technologies should be judged based on their demonstrated effectiveness, in real life farming situations.
Products containing atoxigenic fungi that could recombine in nature with field resident fungi have been proposed (Molo et al., 2019; Carbone, 2021) although field recombination has been detected either when fastidious laboratory conditions are used and in limited number of isolates (Horn et al., 2014) or in on-station field trials (Molo et al., 2022). Traditionally, the possibility of field recombination between biocontrol isolates and field populations was seen as a serious drawback because of concerns on emergence of highly toxigenic, competitive strains (Ehrlich, 2014; Ehrlich et al., 2015) or strains with novel toxin profiles (Olarte et al., 2015). That strategy, called a population genetics approach (Carbone, 2021),6 hypothesizes that reduced application regimes would be needed compared to other formulations (Andrews et al., 2020). Although carry-over effects have been detected in large-scale farmer fields (Cotty, 2000, 2006; Jaime et al., 2017), smallholder farmer fields (Atehnkeng et al., 2022), and on-station trials managed by researchers (Weaver and Abbas, 2019; Molo et al., 2022), atoxigenic isolates applied during a cropping season are not expected to remain for a long term in a treated field. A large portion of the applied fungi and resulting progeny, if or when produced, would move from the treated field, and would be replaced by genotypes arriving from other locations due to climatic events and/or agronomic practices. Cropping systems in areas receiving treatment influence the prevalence of applied atoxigenic fungi. Movement of atoxigenic fungi from treated cotton fields has been reported almost two decades ago (Bock et al., 2004) and recently harvesting of maize was reported to influence densities of A. flavus in cotton fields (Michaels and McDonald, 2021). Only if area-wide programs are implemented it could be expected that atoxigenic fungi would commute across treated fields, providing the required levels of protection if treatment is skipped for 1 or 2 seasons (Bock et al., 2004; Jaime et al., 2017).
Selecting isolates to compose a product based on mating-type profile of Aspergillus fungi resident in a single field has been proposed (Moore et al., 2013; Lewis et al., 2019). However, fungal communities are dynamic. Profiles detected at one point of time most likely will change within fields, and of course from field to field. Deciding which isolates to use in a country or region based on data from a single field, or even a few fields, is not practical. Having multiple products based on multiple potential fungal profiles is complicated. Several products would have to be tested, registered, licensed, manufactured, marketed, and made available when farmers need them, based on the mating-type profiles of Aspergillus spp. in their fields, which are constantly changing. How would the mating-type profiles of individual fields be determined? For example, 7 million farmers plant maize in Nigeria, each of them planting on an average a little bit over 1 ha. Even using representative fields for specific areas is difficult. Who would collect the samples, conduct, and pay for those molecular analyses, and communicate the results to farmers and the biocontrol manufacturers? Also, extension agents need to be trained to advise farmers on reasons for using one or another product. The industries also would need to know which products need to be manufactured and have a rapid distribution system to the interested buyers. This is a very difficult, impractical strategy that does not consider the realities on the ground. Recently, this strategy has been proposed in a study discussing the active ingredient fungi of the Aflasafe product used in Nigeria (Chang, 2022). The product has been effective across multiple areas, over multiple years (Bandyopadhyay et al., 2019; Ola et al., 2022) without determining the mating-type profile in “the field.” Incidentally, in 2023 over 150,000 ha of susceptible crops will be treated by nearly 100,000 smallholder farmers across a wide swath of Nigeria. It will be an unrealistic and exorbitantly expensive task for conducting the microbiological and/or molecular analyses needed to determine the mating-type profiles in those 150,000 ha. Such a huge task would be extremely complicated, and impractical for the smallholder farmers or businesses dealing with biocontrol scale-up.
There are proposals to use isolates emitting volatiles or secreting compounds that could discourage aflatoxin formation either in the field or during storage (Sweany and Damann, 2020; Moore, 2021; Sweany et al., 2021). Repeating concepts from previous paragraphs, potential new active ingredients/modes of action must be tested extensively in the field/storage, under a diverse range of conditions to determine if they would offer reliable performance in the large array of conditions that farmers face/use. On the basis of existing evidence, it is not possible to discern if those volatiles and/or compounds are effective in real-life conditions.
Many assume the effectiveness of new approaches for the prospects of aflatoxin biocontrol based on limited laboratory and on-station field experiments that do not reflect the conditions that farmers face. The practical utility of promising results reported under controlled conditions must be verified in real farming situations before such new strategies are adopted by biocontrol programs for developing new active ingredients. The cost to spend time and resources in changing strategies to include new criteria to select active ingredient would be very high. For new aflatoxin biocontrol products in SSA countries, regulatory agencies would demand new sets of effectiveness trials and conduct the regulatory process from scratch. Commercialization strategies for new products would be required, as well as new licensing, manufacturing, commercialization, and communication efforts. This would take more than a decade. As mentioned in the introduction, millions of farmers and billions of consumers are in need of aflatoxin management now. The available products developed following well-tested and rigorous processes are already effective, licensed, and commercialized. Farmers trust these products as they result in reduced aflatoxin contamination, a precursor for access to premium markets (Bandyopadhyay et al., 2019; Agbetiameh et al., 2020; Konlambigue et al., 2020; Senghor et al., 2020, 2021; Ortega-Beltran et al., 2021; Ola et al., 2022).
Perhaps billions of people are exposed to aflatoxins in their daily meals, sometimes at exceedingly high concentrations. It is a true tragedy that farmers in many regions/countries cannot access effective tools to produce safe foods. This should not be taken lightly. Although improvements in biocontrol technology is a welcome long-term objective, affected people simply cannot wait for “more effective” technologies to be developed, tested, validated, registered, and made available while being continuously exposed to unsafe aflatoxin concentrations. Many have lost/will lose their lives due to repeated aflatoxin exposure. Farmers, governments, and industries cannot indefinitely wait for readily available aflatoxin management tools. A short answer to the question of the subtitle is: yes, there are several proposed improvements to current biocontrol strategies. Perhaps those improvements and others to be discovered could offer great advantages. Therefore, research groups, universities, governments, private sector actors can develop better, more effective technologies (regardless of the criteria to select the biocontrol agents/compounds) and have them available for farmers' uptake without delay. However, any aflatoxin management technology must overcome several hurdles before used at scale.
2.4. Are prospects of novel strategy-based aflatoxin biocontrol products scalable, cheaper, and acceptable by farmers, aggregators, industries, governments?
Oftentimes reports emerge on strategies to make aflatoxin management products/technologies cheaper and more effective, and resilient. Many reports portray certain products or technologies will solve the aflatoxin problem. Perhaps, technically the proposed solutions can solve the aflatoxin problem as can other technologies. However, little is discussed about how farmers can access and adopt them in a sustainable manner, especially in LMICs. Availability of a technology does not result in farmers invariably adopting it, especially smallholder farmers. Developers of technologies intending smallholder farmers being the end-users must keep the technologies simple and design scalability strategies having the smallholder farmers at the center and forefront (Bunch, 2000). However, the downstream scalability and scaling approaches, which are extremely important (Schut et al., 2020), are rarely discussed or practically evaluated. In the case of aflatoxin biocontrol, there are challenges to obtain registration and to gain acceptance of both the product and treated crops by governments, farmers, farmers' organizations, industries, consumers, and several others in the crop value chain. For new products or technologies, once registered, there must be willingness of industries to refine and/or manufacture such products, volatiles, or secreted compounds. More importantly, it must be known whether farmers in any given country will be willing to pay (and at what price) for other set of products or novel compounds. Testing atoxigenic isolates in on-station experiments or characterizing compounds that limit fungal growth and/or aflatoxin production in the laboratory is an initial step that must be further pursued in the discovery to delivery pathway to make new aflatoxin management tools operational. In Figure 1, we present a set of steps that have allowed the sustainable use of registered aflatoxin biocontrol products in SSA. Those steps are applicable to any aflatoxin management technology, grosso modo.
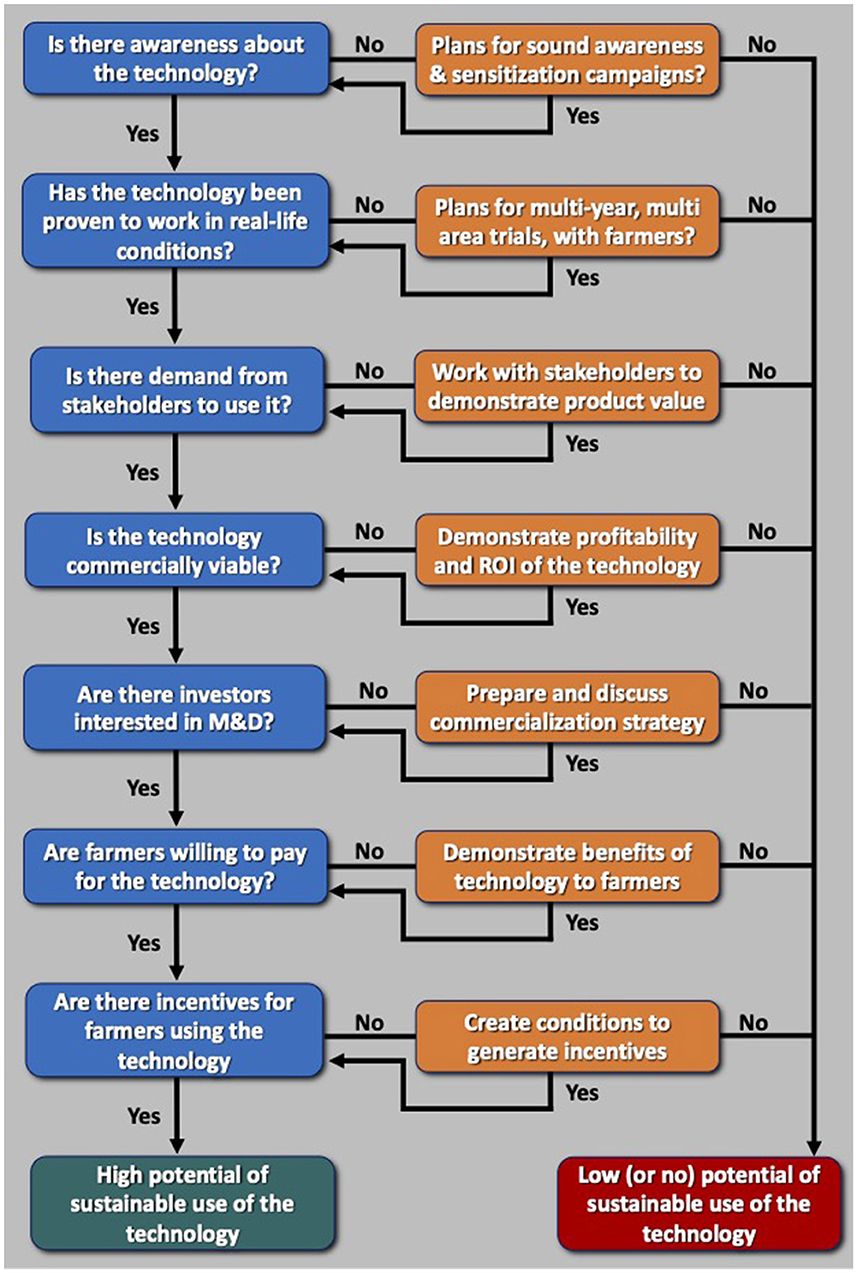
Figure 1. A “code” that has been proven to work for the sustainable use of registered aflatoxin biocontrol products by smallholder farmers in African countries. This basic process, with modifications where necessary, applies for any other aflatoxin management technology.
To be at the current stage, apart from the large scale effectiveness trials (Bandyopadhyay et al., 2019; Agbetiameh et al., 2020; Senghor et al., 2020, 2021; Ortega-Beltran et al., 2021; Mahuku et al., 2022), it was necessary to conduct toxicological and ecotoxicological studies, willingness-to-pay studies, adoption projections, environmental impact assessments, among other studies (Bandyopadhyay et al., 2016; Ayedun et al., 2017; Johnson et al., 2018, 2019; Schreurs et al., 2019). All biocontrol products/technologies must be subjected to studies to know if they are effective, safe, and scalable. Absence of those studies may preclude their registration and therefore their scaling. Other significant requirements are the development of commercialization strategies and business plans to identify who (and how and where) is best positioned to manufacture and distribute the products, and how farmers will access them (Konlambigue et al., 2020; Bandyopadhyay et al., 2022). Market linkages must be facilitated between producers of aflatoxin-reduced crops and aflatoxin-conscious buyers. In short, it will take more than scientific work at the laboratory and on-station level to make any form of aflatoxin control moving to commercial, sustainable usage (Figure 1). A multi-disciplinary approach by a team composed of biological scientists, engineers, social scientists, communication experts, extension agencies, policymakers, regulators, business development specialists, private sector investors, among others is needed to allow sustainable use of the aflatoxin biocontrol technology. Thus, aflatoxin management should be considered multi-disciplinary in nature. A diverse, multi-disciplinary team brings creativity to overcome impediments for successful uptake of the technology.
For the question posed in the subtitle, we cannot elaborate an opinion about the prospects of biocontrol products based on new approaches since the sustainable use of the biocontrol technology is not quite that simple and will certainly require more than partnerships between academic and industry scientists, as proposed recently (Andrews et al., 2020).
2.5. Is a single strategy needed?
Biocontrol itself does not completely solve the aflatoxin problem. Other practices and technologies must be combined with biocontrol to bring aflatoxin levels to the lowest possible. Aflatoxin contamination occurs across the whole value chain and, therefore, there needs to be convergence of multiple technological, institutional, and policy options. This has been repeatedly stressed over the years (Hell et al., 2008; Bandyopadhyay et al., 2016; Ayalew et al., 2017; Logrieco et al., 2018; Ortega-Beltran and Bandyopadhyay, 2021). Recently, similar suggestions were made (Moore, 2021). Pending is to investigate the extent to which diverse aflatoxin management technologies complement each other. In briefest terms, it would be naïve to assume that a single technology (e.g., biocontrol, sorting, or use of tarps for drying) will provide the required level of protection to prevent aflatoxin contamination from field to fork.
3. Preamble to challenges and actions for effective scaling of aflatoxin biocontrol
Before explaining difficulties to have aflatoxin biocontrol products used at scale, we consider worthwhile making an analogy. Several challenges to have smallholder farmers adopting improved seeds exist (Fisher et al., 2015; Sinyolo, 2020). Perhaps the major barrier is simply that the improved seeds are not available in agro-dealer stores. When available, there can be challenges if information on the benefits of the improved seeds is not properly conveyed. Even how packages of improved seeds are displayed by agro-dealers influences technology uptake7 Other reasons include perceived high price, or that the improved seed is of a type not preferred by the farmer and her/his family, and/or the market. There are books and several articles, even programs within institutions, dedicated at understanding and proposing how to disentangle barriers preventing uptake of improved seeds (Langyintuo et al., 2010; Abdoulaye et al., 2018; McEwan et al., 2021; Rutsaert et al., 2021). If there are challenges to have farmers buying seeds that increase yield and/or tolerate various stresses, what are the prospects of farmers paying for products that may increase crop quality, but such higher quality is not visible and for which reward mechanisms are very limited?
Biocontrol acceptance and uptake is difficult because aflatoxins are usually less known, do not reduce yield, and markets typically do not discriminate crops in LMICs even if containing high levels. Acceptance and uptake are of course much more limited if there is no clear, sound strategy for scaling. Years can pass by, and ideas, concepts, and recommendations on aflatoxin biocontrol may never be implemented because the realities on the ground may not allow for it. The scaling of agricultural technologies, particularly those developed for smallholder farmers, is a whole science requiring more than developing an effective technology (Woltering et al., 2019; Schut et al., 2020). For aflatoxin biocontrol, it cannot be expected that farmers will invariably adopt the technology. Even if an effective product is available and fully registered, several challenges for its use in aflatoxin management must be overcome even after commercialization strategies have been defined, manufacturing and distribution agreements have been signed–respecting the Convention on Biodiversity–, infrastructure to manufacture a product is available, and distribution channels are established. Political stability, local or regional conflicts, infrastructure, effective communication campaigns, timely reception of materials for manufacturing, proper training of manufacturers, timely production and distribution, correct training of farmers on how to use the product, and many other factors must be placed in the equation. Moreover, crop and market trends as well as government policies can define whether farmers and organizations decide investing on an aflatoxin management technology.
There is no single approach to stimulate uptake of aflatoxin management technologies, including biocontrol. Agriculture within and among regions is dramatically diverse and therefore both aflatoxin contamination and implementation of possible solutions are highly complex. There is the need to identify local actors, adapt strategies to their practices and the context, consider their concerns, and comply with local customs and regulations to design appropriate programs to effectively control aflatoxins in complex cropping system mosaics. Otherwise, the efforts will be of little to no practical value in solving the aflatoxin problem at a significant level. An aflatoxin biocontrol product can be highly effective, but such effectiveness cannot compensate for absence of a sound scaling strategy and the potential benefits of the product thus cannot be realized. By now, it should be recognized the truism that aflatoxin biocontrol uptake is not an easy task due to the nature of the problem, the characteristics of the product, and the multiple challenges on the ground. Similar challenges apply to other aflatoxin management technologies.
4. Challenges and actions to make aflatoxin biocontrol products available to smallholder farmers
With the context given above, challenges faced to have aflatoxin biocontrol sustainably used at scale in various SSA countries are described. Similar challenges are likely in countries where aflatoxin biocontrol is being developed or where new products are sought. For each challenge, actions conducted by the Aflasafe Initiative to overcome it are outlined. Some of the challenges and actions have been discussed in other publications, and therefore are briefly mentioned here.
4.1. Awareness continues being low
A technology cannot be popularized among farmers if they do not know the problem that the technology is designed to solve. Many farmers and consumers do not know what aflatoxins are, the causal agents of contamination, and/or ways to prevent the contamination (Stepman, 2018). Further, if consumers do not know about the problem, they cannot persuade farmers, aggregators, industries to conduct actions to manage aflatoxin contamination throughout the value chain. In this sense, the Aflasafe Initiative has placed efforts to raise awareness among stakeholders on the dangers posed by aflatoxins and possible solutions, including biocontrol (Bandyopadhyay et al., 2016; Schreurs et al., 2019; Ortega-Beltran and Bandyopadhyay, 2021). These efforts many times are affected by unfounded doubts about the technology, including the notion that biocontrol is not effective in African environments, assuming that registration is impossible due to the absence of appropriate frameworks, claiming that there is no need for protecting crops (Pitt, 2019) (an aflatoxin denialism), and assuming that few farmers will adopt the products. Farmers, regulators, scientists, extension agents, industries, health specialists, and government officers, among others are sensitized on the need to implement aflatoxin management strategies and the benefits of implement such programs using biocontrol-centric approaches. Developers of an aflatoxin management technology need to place considerable efforts to explain in different fora, and over many years, what the technology is about; its safety, benefits, and limitations; how to use it; how to make it commercially available; and should be prepared to receive criticism from different sectors, many times repeatedly responding to the same concerns.
4.2. Policies not fully implemented
In most LMICs, policies preventing commercialization of contaminated crops are either non-existent, poorly articulated, or poorly enforced. Thus, absence of effective policies restricts farmers' willingness to invest in aflatoxin management technologies. Similarly, lack of effective, enforced policies discourage industries to invest in production of technologies to reduce aflatoxins, such as biocontrol products. As mentioned before (Bandyopadhyay et al., 2022), policies to enforce standards based on dietary habits must be developed and implemented. It is necessary to raise awareness among key stakeholders on control strategies and regulations plus develop mechanisms to assess food safety risks. Absence of effectively enforced policies precludes countries from achieving food safety and this is a failure to protect the wellbeing of their populations. Poor food safety policy implementation is an impediment to the sustainable use of any aflatoxin management technology (improved seeds, biocontrol, drying, sorting, hermetic storage, nixtamalization, etc.). Scientists/industries developing aflatoxin management solutions need to establish close collaborations with regulators and key stakeholders to formulate appropriate policies that encourage the promotion and adoption of the solutions to prevent consumption of contaminated foods and feeds.
An example of policy actions led by the Aflasafe Initiative: the United States Agency for International Development (USAID) East Africa Regional Mission has worked intensively with EAC (Burundi, Kenya, Rwanda, Tanzania, Uganda) to implement the mandate of PACA. In this context, USAID supported the formation of the Aflatoxin Policy and Program for the East Africa Region (APPEAR) which was implemented by EAC and IITA. EAC brought together Ministers of Health, Agriculture and Trade, their Deputies, and other key actors in the public and private sector to review technical materials, conduct deliberations, and formulate and disseminate policy recommendations. Through APPEAR, 11 technical papers to decrease the negative impacts of aflatoxins were developed. The papers covered topics such as pre- and post-harvest technologies, standards for food and feeds, nutrition, health, communication, biocontrol using atoxigenic isolates, trade, and disposal. The 11 papers were turned into nine policy briefs that outline key strategic policy recommendations and actions to prevent and control aflatoxin contamination along food and feed value chains in EAC.8 Also, a communication strategy was developed for use of aflatoxin management strategies centered on Aflasafe in Kenya. The communication strategy is planned to be adapted for use in other EAC countries. Further, the 36th Meeting of the EAC Council of Ministers directed Partner States to mainstream EAC Aflatoxin Prevention and Control Strategy priorities in their national budgets and national agriculture investment plans to facilitate implementation of proposed interventions which included biocontrol and key post-harvest practices. However, although considerable progress has been made, much more needs to be done to have those policies successfully enforced.
4.3. Regulations for registration of biocontrol products
An aflatoxin biocontrol product must be registered for large-scale use. It is necessary to conduct the effectiveness trials under the guidance of the regulatory agency that will provide the registration. A problem stemmed during the initial testing and registration of aflatoxin biocontrol products in SSA since there were no precedents for registration of biocontrol products containing atoxigenic isolates of A. flavus as active ingredients, except in the US. In some cases, the same guidelines implemented for registration of chemical pesticides were planned to be used for aflatoxin biocontrol agents. However, guidelines for both type of products should be inherently different because of the nature of the active ingredients (chemical vs. biological). Therefore, because of the lack of precedents, members of regulatory agencies and policymakers were consulted and sensitized on all relevant aspects of the biocontrol technology prior to starting the product development (Bandyopadhyay et al., 2016).
For products aiming to exploit the population genetics approach, extensive sensitization and research efforts will be needed to demonstrate that (i) the active ingredient isolates mate in nature and decrease the average aflatoxin production potentials of the resulting communities (Molo et al., 2022) compared to the potentials prior to treatment, (ii) that the progenies will not pose a risk to future cropping systems, the environment, and organisms interacting in areas surrounding fields receiving treatment (environmental impact assessment); (iii) logistic feasibility for both field sampling and conduct the molecular assays for mating-type profile determination, and (iv) plans for manufacturing and distributing multiple products when farmers need them. For the fungus-free strategy (e.g., production, and concentration and packaging of inhibitory compounds for field/storage application), the regulatory process could be similar as for chemical pesticides and will require extensive toxicological and ecotoxicological studies. For most Aflasafe products, waivers were granted for toxicological and ecotoxicological studies on the basis of evidence of studies conducted in the US (AF36 and afla-guard), Nigeria (Aflasafe™), and Kenya (Aflasafe KE01™) with isolates for which the main mechanism is competitive displacement of aflatoxin producers via production of large amounts of conidia. For the population genetics approach and the fungus-free compounds, waivers for toxicological and ecotoxicological studies could be difficult to obtain since the modes of action are different.
4.4. Market discrimination
As mentioned above, in most LMICs the policies to prevent trade and consumption of contaminated foods and feeds are either non-existent or yet to be appropriately enforced. Therefore, many markets do not pay premium prices for aflatoxin-safe crops and most farmers do not have incentives to invest in technologies for production of aflatoxin-safe crops. Apart from efforts to develop, test, register, and transfer effective technologies to address aflatoxin contamination, it is necessary to invest significant resources to ensure that the technology will indeed be used. In the case of aflatoxin biocontrol, this will only occur when markets stimulate farmers to produce safe crops or governments/development investors incentivize the technology to promote adoption (Narayan and Geyer, 2022). Otherwise, the technology will fail to bridge the last mile. Some publications may be produced, the technology could be transferred, but with no reward mechanism the technology is bound to be abandoned. We have discussed that many industries and food aid agencies demand production of aflatoxin-reduced crops, but in many cases, there is a lack of reward mechanisms to motivate farmers for investing in aflatoxin management technologies. Little to no market discrimination is an impediment for the sustainable use of any aflatoxin management technology, even if the technology is a silver bullet. To alleviate this constraint, it is necessary to identify industries seeking aflatoxin-reduced crops and explain the benefits of using biocontrol treated crops compared to other options (e.g., use of toxin binders, import of safe crops). Then those industries need to be linked with farmers able to produce the crops meeting the required standards. More information on this topics has been published (Bandyopadhyay et al., 2019, 2022; Hoffmann et al., 2022).
4.5. Manufacturing capacity
The biocontrol product needs manufacturing at scale for use by hundreds of thousands of farmers to make significant impact in reducing crop aflatoxin content. There are only few aflatoxin biocontrol manufacturing facilities: two in the US, and one each in Italy, Nigeria, Senegal, Kenya, Tanzania, and Pakistan. Others are under construction in the Democratic Republic of Congo, Mozambique, Sudan, Rwanda, and Burundi. All those in SSA countries have been or are being constructed under the guidance of the Aflasafe Initiative. The one in Pakistan has input from members of the Aflasafe Initiative. In Nigeria, during the initial years of the Aflasafe Initiative, the product was manufactured using a laboratory-scale methodology that could produce ~300 kg/week, which can treat 30 ha. Thereafter, an efficient industrial manufacturing process was designed taking into consideration the realities on the ground (Bandyopadhyay et al., 2016). After technology transfer, the industrial process is allowing production of large quantities of biocontrol product. Manufacturing capacity in factories set up in Nigeria, Senegal, Tanzania, and Kenya is 5 tons/h. In an 8 h shift, enough biocontrol product can be manufactured to treat 4,000 ha of susceptible crops. This capacity can increase by adding more production modules (Bandyopadhyay et al., 2019, 2022). Similarly, products or technologies being developed must consider how, where and by whom the production will take place. Local production, or in neighboring countries (e.g., in Senegal and sent by road to Mali), is preferred since it is not practical to send products to treat hundreds of thousands of hectares via air or sea freight.
4.6. The scaling challenges of aflatoxin management
As mentioned above, even the most effective aflatoxin management technology must be scaled to reach the end users, the farmers, who will ultimately use it to produce safe crops. The scale up of aflatoxin biocontrol products in SSA after their registration has been achieved following the process briefly explained below.
Determining whether the technology is commercially feasible. In countries where Aflasafe products are commercially available, commercialization strategies were developed to demonstrate how the product could become a marketable farm input for sale. In each country, it is necessary to conduct a study to understand the country context, analyze market potential, forecast biocontrol uptake, review manufacturing potential, and identify potential investors. The study provides a clear understanding of how to commercialize a biocontrol product in the country, and at what price. Depending on the country, the cost to treat one hectare is 12–19 USD, which is influenced by currency exchange rate and price of sorghum grain, polymer for spores to stick to sorghum, the blue dye used to differentiate the product from regular sorghum, and laboratory materials and reagents (Bandyopadhyay et al., 2016). Potential private sector partners use the study to make an informed judgement on whether to invest in the technology. For determining the initial commercialization opportunities, it is necessary to prioritize market segments that are aflatoxin-conscious and are more likely to implement aflatoxin management strategies centered on the biocontrol product. One key output of the study is to understand the needs from investors in terms of capacity and expertise for setting up a manufacturing facility and develop marketing and distribution strategies to reach the end users. Such a study is not conducted by scientists but by appropriate firms with expertise in agribusiness, strategical thinking, and finance and that are supported by others involved in the product development, testing, and registration process. More on this can be found at: https://newint.iita.org/wp-content/uploads/2021/03/150221Market-Assessment-and-Strategy-Development-Guide.pdf.
Finding investors willing to manufacture and/or distribute the technology. Potential investors are identified when a commercialization and market analysis study are conducted, as described above. Findings of the study are presented in an open investor's forum. After the forum, potential investors elicit express interest to manufacture and/or distribute the technology. The capabilities of the potential investors are then evaluated, and selection of the most appropriate candidate for local manufacturing and/or marketing and distribution of aflatoxin biocontrol products occurs through a competitive process. Companies with proven business expertise, having established and sustainable distribution channels (or with capabilities to develop them), with innovative marketing strategies, and commitment to persevere to deal with a product that solves an unseen problem are selected. More on this can be found at: http://www.iita.org/wp-content/uploads/2021/03/150221Investor-Selection-Guide.pdf.
Structuring the Business Relationship. This relationship needs to conform with policies and practices of the institution developing the technology but also consider the financial motivations of the investor(s) selected as manufacturing and/or distribution partners. Extensive discussions take place to produce a legal document that allows the transfer of the technology. Aflasafe products have been transferred to the private sector through a Technology Transfer and Licensing Agreement (TTLA) because this legal document allows one organization (i.e., the selected private company) to obtain a license to use a patented technology or intellectual property developed by another organization (e.g., university, research organization). The TTLA allows a public institution (licensor) to receive fees or royalties and reinvest them into future research products and innovations while also ensuring its product is accessible and successfully scaled. In turn, the licensee leverages its marketing knowledge and business acumen to reap financial benefits from the commercialized product of research. For Aflasafe products, there is a balance to allow private sector investors to make profit without making it impossible for smallholder farmers to access the product. More on this can be found at: http://www.iita.org/wp-content/uploads/2021/03/150221Structuring-the-Business-Relationship-Guide.pdf.
Implementation of the Business Development Strategy. Once a TTLA has been signed, there is the need to share relevant information with the selected investor and support them during the initial aflatoxin biocontrol business development and marketing strategies. This is critical because Aflasafe requires a unique marketing strategy because of the realities on the ground explained above. Traditional retail sales, branding, and advertising approaches do not work for aflatoxin biocontrol. There is the need to create market demand for a new product category, one that address a problem that many are not aware of or that they are not stimulated to use. More on this can be found at: http://www.iita.org/wp-content/uploads/2021/03/150221Implementation-of-the-Business-Development-Strategy-Guide.pdf.
4.7. Technical backstopping to manufacturers
The correct manufacture of the aflatoxin biocontrol technology requires significant, continuous support to licensees. The technology is new in most countries and licensees require that their employees receive training on how to manipulate the active ingredient fungi, set-up the manufacturing facility, and make it operational. The Aflasafe Initiative even works closely with architects and engineers who design and construct both the manufacturing facility and accompanying laboratories. Several consultations with on-site visits and frequent virtual calls are done before, during, and after the construction to ensure that the biocontrol product will be/is produced in optimal conditions. In addition, as mentioned above, there is the need to work together with the licensees to transfer the know-how with respect to awareness creation, farmer training, training material, demonstrating utility of the product in commercial settings (see in-situ testing below), and promoting institutional linkages. Failure to backstop licensees can result in incorrect manufacturing, deficient training to farmers, and overall biocontrol product failure.
4.8. In-situ testing
It is not possible to determine the aflatoxin content of a crop by visual inspection. Thus, it is difficult for quality-sensitive buyers to quickly identify aflatoxin-safe crops for purchase. The Aflasafe Initiative promotes an in-situ aflatoxin testing and sampling system for use in the field by licensees and value chain actors. The system demonstrates the effectiveness of integrated aflatoxin management and risks of not protecting crops with aflatoxin management practices. A laboratory protocol was adapted for use in the field/warehouses. Quantifying aflatoxin at harvest or at any stage of storage is now possible without having to send samples to a laboratory. Farmers and processors trust the technology when participating and/or witnessing the quantification process (Senghor et al., 2021).
4.9. Addressing concerns of researchers and value chain actors
As mentioned above, sometimes there are interactions with stakeholders that do not trust/believe in the technology (for diverse reasons) and that in many cases advocate for preventing its use. Several concerns expressed by such stakeholders have been addressed (Bandyopadhyay et al., 2016). Additional concerns addressed include (i) whether products stand a chance of being adopted [farmers indeed are willing to pay for the products (Ayedun et al., 2017; Bandyopadhyay et al., 2019; Migwi et al., 2020)], (ii) whether product usage affect total soil microbiome [recent evidence showing no overall changes in total microbiome composition of treated and untreated crops (Bhandari et al., 2020)], and (iii) higher accumulation of other metabolites, such as fumonisin, in treated crops [treated and untreated crops contain the same fumonisin levels (Ortega-Beltran et al., 2020)]. Other concerns are briefly discussed below.
One product for multiple mycotoxins. Aflatoxin biocontrol has been criticized for not addressing all mycotoxin problems that affect a crop (e.g., maize). One product, developed specifically to address a single problem, in this case aflatoxins, cannot be the solution for all mycotoxins. Similarly, it cannot be expected that a single insecticide will prevent damage by all kind of insects. We have discussed above that perhaps other mycotoxins are reduced when using integrated aflatoxin management strategies because of use of good agricultural practices and improved harvest and post-harvest practices. However, the value of aflatoxin biocontrol should not be undermined if other toxins are not specifically controlled.
Crops left behind. It has been mentioned that biocontrol has been targeted for use in a few crops while there are over 50 crops susceptible to aflatoxin contamination grown in African countries. Adapting the technology for use in a crop requires significant human, infrastructural, and financial resources. There is the need to obtain public and/or private capital to test and register aflatoxin biocontrol products for use in additional crops. Obtaining those funds also takes considerable effort and time. See below the ‘Reduced Investments' section. The Aflasafe Initiative focused in two major staple crops that are highly susceptible to aflatoxin contamination (maize and groundnut) and as much as possible covered other crops (i.e., sorghum, sunflower, sesame, chili pepper). Hopefully, in future there will be no crop left behind from aflatoxin protection.
Semantics. Concerns have been raised for using the term “atoxigenic” because atoxigenic fungi may produce other secondary metabolites (Moore, 2021). However, the term “atoxigenic” is generally accepted as the antonomasia both for non-aflatoxin-producing fungi and isolates used in biocontrol formulations to reduce aflatoxin content. Farmers, consumers, and industries suffering from aflatoxin surely are not worried about the semantics—they are worried about the accumulation of aflatoxins in the crops that they produce, transform, feed to their livestock/pets, commercialize, and…consume. The production and consumption of safe foods/feeds is possible when using biocontrol products regardless of naming the active ingredient fungi “atoxigenic,” “non-aflatoxin-producing fungi,” “non-aflatoxigenic,” “Non Tox,” “non-tox,” or any other term. Farmers in different countries name biocontrol fungi/products in different manners. For example, some farmers in Malawi use the term “chuku chabwino” for the biocontrol product which roughly means “good aflatoxin.” Once again, what is important is that the aflatoxin biocontrol technology when developed, manufactured, and used correctly reduces the accumulation of one of the deadliest compounds found in nature.
4.10. Reduced investments
Bernardo (2014) cited the 1936 USDA Yearbook of Agriculture as: “the field of breeding and genetics has become so large, it is so dependent on progress in basic research and it requires such continuous effort on projects running over many years or even more than one generation, that it obviously becomes a function of government institutions capable of devoting the necessary money and time to the work and doing it with a sufficiently disinterested attitude.” Thus, breeding efforts for a crop of interest are continuous and require both public and private investment for the development of improved varieties with utility in target environments. Similarly, aflatoxin biocontrol although being effective in many regions is at its infancy relative to many other research areas and needs to be continuously improved, tested, and adapted for use in new regions, crops, or cropping systems. In other words, the technology is an active area of research and development. Food safety efforts, in this case through biocontrol, require significantly higher amounts of both public and private investment than what has been devoted already (Unnevehr, 2022). Unfortunately, the level of investment for food safety has been significantly less than that for breeding, agronomy, soil science or nutrition, despite its importance for progress and wellbeing in areas prone to food safety crises. For aflatoxin management, even if correctly implemented, it is very difficult to demonstrate the positive results within a short time since the benefits become apparent over long-term. Therefore, it is very difficult to obtain enough resources for its control. Hopefully governments, private sector, and funding agencies will continue supporting efforts to make staple crops safe from aflatoxin contamination through biocontrol-centric approaches.
4.11. Silver bullets
Often single strategies are promoted as the solution to the aflatoxin problem. Unfortunately, no single strategy can solve the aflatoxin problem because of its complexity and the many points across the value chain that are vulnerable to the production of aflatoxins. Any technology portrayed as the solution will suffer when failing to provide the required protection. Promoters of any single solution run the risk of users losing confidence in the solution when it does not show the desired results of aflatoxin reduction. Once again, we advocate for the use of all available practices in a cost-effective manner from field to fork to increase chances of reducing aflatoxin to the lowest possible level. A specific silver bullet example is provided below:
Are GMOs the answer now? There are publications and/or news articles9 showcasing GMOs as the panacea for aflatoxin control. Transgenic events can lead to reduced aflatoxin levels in genetically modified maize and groundnut under controlled conditions (Thakare et al., 2017; Sharma et al., 2018; Schmidt et al., 2021) but such testing has not been done in field conditions. Programs using transgenic crops to reduce aflatoxin contamination, when and if commercially available, must know basic information about transgenes to effectively communicate with stakeholders and must provide science-based answers in a layman language to address questions on the use and safety of transgenic cultivars. Further, several groups will oppose the use of transgenics. Bernardo (2014) groups them as follows: (1) those opposing because the transgenic technology is an unnatural process and cannot accept it because of their ethical, moral, or religious backgrounds; (2) those considering that using transgenics creates a forced dependency on for-profit, large corporations; and (3) those concerned about the safety of transgenics for humans, animals, and the environment. Of course, transgenic aflatoxin-resistant crops need to satisfy regulatory approvals, which is long, arduous, and difficult to obtain in many countries. More importantly, the transgenic events need to be introduced or stacked (if multiple) into breeding populations for the development of stable, high yielding cultivars (whether hybrids, OPVs, or synthetics). Then, the improved transgenic cultivars need to be commercialized and adopted by farmers, which has its own sets of challenges as mentioned above, and most importantly, the grains/nuts from transgenic crops must be accepted by the consumers, which is another challenge. Regardless, if transgenic cultivars become available, registered, and accepted, they would provide another arsenal in the management of aflatoxins but should be combined with other management options in case conditions in the field/transport/storage favor aflatoxin contamination and the transgenic events are not enough to discourage it.
5. Summary
The deadliest carcinogen in nature commonly contaminates staple crops and is being consumed daily by millions, at sub-lethal, and even lethal levels. Although challenging, aflatoxin contamination and exposure is preventable using an integrated field-to-fork strategy converging policy, institutional, and technical options, including aflatoxin biocontrol containing atoxigenic isolates of A. flavus. We discussed challenges and actions taken by the Aflasafe Initiative to have integrated aflatoxin management strategies used at scale. Collectively, farmers and industries making relatively small investments and efforts can save hundreds of thousands of lives by successfully mitigating aflatoxin throughout the value chain. The use of effective, available aflatoxin management technologies must be promoted to positively impact current and future generations in affected areas. However, the implementation of aflatoxin management programs is not easy; time, patience, and perseverance are required since their implementation is generally slow. In addition to having an effective technology and the know-how, the ecosystem (agricultural, social, regulatory, industrial, political) must be navigated judiciously, and there must be determination to succeed in having the technology adopted. Hopefully readers will realize that aflatoxin management programs using biocontrol centric approaches are difficult to implement. We think that the lessons and experiences shared could help others to design/improve programs to effectively reduce aflatoxin contamination throughout the value chain. Finally, the Aflasafe Initiative will continue to evaluate all promising options and adapt/adopt them, if their implementation is feasible. In the short to medium term, the Aflasafe Initiative will continue promoting the aflatoxin biocontrol technology and delivery system that has been proven to work.
Author contributions
AO-B and RB conceptualized the idea and wrote the manuscript. Both authors contributed to the article and approved the submitted version.
Funding
Several institutions and governments have funded the aflatoxin control program of IITA: The Bill and Melinda Gates Foundation (BMGF; OPP1007117 and OPP1133356); the United States Agency for International Development (USAID); Meridian Institute on behalf of the Partnership for Aflatoxin Control in Africa (PACA); both Agricultural Research Service and Foreign Agricultural Service of USDA; Bundesministerium für wirtschaftliche Zusammenarbeit und Entwicklung (BMZ, German Federal Ministry for Economic Cooperation and Development); AgResults [a collaborative initiative between BMGF, Australia's Department of Foreign Affairs and Trade (DFAT), the Department for International Development of the United Kingdom (DFID), Global Affairs Canada, and USAID]; MycoGlobe, MycoRed, and MycoKey funded by the European Union Commission; Austrian Development Cooperation; Commercial Agriculture Development Program of the Government of Nigeria; French Development Agency; Royal Government of Norway through the Climate Smart Agricultural Technologies (CSAT; MLI-17/0008 and NER-17/0005) for Mali and Niger; AGRA; CGIAR A4NH Research Program, CGIAR MAIZE Research Program; and One CGIAR Plant Health Initiative.
Acknowledgments
We are grateful to all farmer, governments, donors, researchers, and institutions that strongly supported the aflatoxin biocontrol technology and have made possible its development, registration, commercialization, and technology transfer for sustainable use of aflatoxin biocontrol products in multiple countries.
Conflict of interest
The authors receive no direct financial benefit from the manufacturing and marketing of any of the aflatoxin biocontrol products mentioned in this article. The Aflasafe name is a Trademark of the International Institute of Tropical Agriculture (IITA). IITA used to manufacture and commercialize Aflasafe for use in Nigeria, Senegal, Burkina Faso, The Gambia, and Ghana. Manufacturing and distribution responsibilities have been licensed to the private or public sector. IITA charges a small licensing fee to manufacturers for use of the Aflasafe name and cost associated with technology transfer and technical backstopping. This fee is reinvested for providing reasonable technical support to the manufacturer and distributor when needed, to develop new products/practices for more farmers to use the technology, as well as for further research to improve the aflatoxin biocontrol technology. A portion of the fee is also set aside for fulfilling reasonable Access and Benefit Sharing obligations related to the Convention on Biodiversity. Both authors are employed by IITA.
Publisher's note
All claims expressed in this article are solely those of the authors and do not necessarily represent those of their affiliated organizations, or those of the publisher, the editors and the reviewers. Any product that may be evaluated in this article, or claim that may be made by its manufacturer, is not guaranteed or endorsed by the publisher.
Footnotes
1. ^Dossiers for registration of Aflasafe products for use in Rwanda and Mali are currently being evaluated by regulatory authorities. Once approved, the number of countries where Aflasafe products are commercially available will be 12.
2. ^https://www.aflatoxinpartnership.org
3. ^https://www.eac.int/documents/category/aflatoxin-prevention-and-control
4. ^https://www.aflatoxinpartnership.org/sites/default/files/2018-06/ECOWAS%20Action%20Plan-%20FINAL%20DRAFT.pdf
5. ^https://allafrica.com/stories/202210130396.html
6. ^Over a decade ago, this strategy was showcased as the solution to eliminate aflatoxin contamination. Link accessed on 20 Oct 2022 https://www.youtube.com/watch?v=ToahNhrRcrE.
7. ^AGG, Webinar 1: The challenge of introducing new varieties: Learning from agro-dealers and farmers (https://www.youtube.com/watch?v=Ldpuf9QBs7g).
8. ^The nine policy briefs can be accessed at: https://www.eac.int/documents/category/aflatoxin-prevention-and-control.
9. ^https://www.forbes.com/sites/andrewwight/2021/12/29/meet-the-indian-researcher-helping-to-solve-the-deadly-aflatoxin-puzzle/?sh=6cc836bc6f30
References
Abbas, H. K., Weaver, M., Horn, B., Carbone, I., Monacell, J., and Shier, T. (2011a). Selection of Aspergillus flavus isolates for biological control of aflatoxins in corn. Toxin Rev. 30, 59–70. doi: 10.3109/15569543.2011.591539
Abbas, H. K., Zablotowicz, R. M., Horn, B. W., Phillips, N. A., Johnson, B. J., Jin, X., et al. (2011b). Comparison of major biocontrol strains of non-aflatoxigenic Aspergillus flavus for the reduction of aflatoxins and cyclopiazonic acid in maize. Food Addit. Contam. Part A Chem. Anal. Control. Expo. Risk Assess. 28, 198–208. doi: 10.1080/19440049.2010.544680
Abdoulaye, T., Wossen, T., and Awotide, B. (2018). Impacts of improved maize varieties in Nigeria: ex-post assessment of productivity and welfare outcomes. Food Secur. 10, 369–379. doi: 10.1007/s12571-018-0772-9
Adhikari, B. N., Bandyopadhyay, R., and Cotty, P. J. (2016). Degeneration of aflatoxin gene clusters in Aspergillus flavus from Africa and North America. AMB Express 6, 62. doi: 10.1186/s13568-016-0228-6
Agbetiameh, D., Ortega-Beltran, A., Awuah, R. T., Atehnkeng, J., Elzein, A., Cotty, P. J., et al. (2020). Field efficacy of two atoxigenic biocontrol products for mitigation of aflatoxin contamination in maize and groundnut in Ghana. Biol. Control 150, 104351. doi: 10.1016/j.biocontrol.2020.104351
Alaniz Zanon, M. S., Chiotta, M. L., Giaj-Merlera, G., Barros, G., and Chulze, S. (2013). Evaluation of potential biocontrol agent for aflatoxin in Argentinean peanuts. Int. J. Food Microbiol. 162, 220–225. doi: 10.1016/j.ijfoodmicro.2013.01.017
Andrews, M., Carbone, I., Binder, A., Breakfield, N., Duckworth, O., Francis, K., et al. (2020). Agriculture and the Microbiome. CAST Issue Paper. Ames, IA: Council for Agricultural Science and Technology.
Atehnkeng, J., Ojiambo, P. S., Ortega-Beltran, A., Augusto, J., Cotty, P. J., and Bandyopadhyay, R. (2022). Impact of frequency of application on the long-term efficacy of the biocontrol product Aflasafe in reducing aflatoxin contamination in maize. Front. Microbiol. 13, 1049013. doi: 10.3389/fmicb.2022.1049013
Ayalew, A., Kimanya, M., Matumba, L., Bandyopadhyay, R., Menkir, A., and Cotty, P. J. (2017). “Controlling aflatoxins in maize in Africa: strategies, challenges and opportunities for improvement,” in Achieving Sustainable Cultivation of Maize. Volume 2: Cultivation Techniques, Pest and Disease Control, ed D. Watson (Cambridge; Burleigh Dodds Science Publishing Limited), 371–394. doi: 10.19103/AS.2016.0002.23
Ayedun, B., Okpachu, G., Manyong, V., Atehnkeng, J., Akinola, A., Abu, G. A., et al. (2017). An assessment of willingness to pay by maize and groundnut farmers for aflatoxin biocontrol product in Northern Nigeria. J. Food Prot. 80, 1451–1460. doi: 10.4315/0362-028X.JFP-16-281
Bandyopadhyay, R., Atehnkeng, J., Ortega-Beltran, A., Akande, A., Falade, T. D. O., and Cotty, P. J. (2019). “Ground-truthing” efficacy of biological control for aflatoxin mitigation in farmers' fields in Nigeria: from field trials to commercial usage, a 10-year study. Front. Microbiol. 10, 2528. doi: 10.3389/fmicb.2019.02528
Bandyopadhyay, R., Ortega-Beltran, A., Akande, A., Mutegi, C., Atehnkeng, J., Kaptoge, L., et al. (2016). Biological control of aflatoxins in Africa: current status and potential challenges in the face of climate change. World Mycotoxin J. 9, 771–789. doi: 10.3920/WMJ2016.2130
Bandyopadhyay, R., Ortega-Beltran, A., Konlambigue, M., Kaptoge, L., Falade, T. D. O., and Cotty, P. J. (2022). “Development and scale-up of bioprotectants to keep staple foods safe from aflatoxin contamination in Africa,” in Microbial Bioprotectants for Plant Disease Management, eds J. Köhl, and W. Ravensberg (Cambridge: Burleigh Dodds Science Publishing), 1–41. doi: 10.19103/AS.2021.0093.16
Bhandari, K. B., Longing, S. D., and West, C. P. (2020). Soil microbial communities in corn fields treated with atoxigenic Aspergillus flavus. Soil Syst. 4, 35. doi: 10.3390/soilsystems4020035
Bock, C. H., Mackey, B., and Cotty, P. J. (2004). Population dynamics of Aspergillus flavus in the air of an intensively cultivated region of south-west Arizona. Plant Pathol. 53, 422–433. doi: 10.1111/j.0032-0862.2004.01015.x
Bui-Klimke, T. R., Guclu, H., Kensler, T. W., Yuan, J. M., and Wu, F. (2014). Aflatoxin regulations and global pistachio trade: insights from social network analysis. PLoS ONE 9, e92149. doi: 10.1371/journal.pone.0092149
Bunch, R. (2000). Keeping it simple: what resource-poor farmers will need from agricultural engineers during the next decade. J. Agric. Eng. Res. 76, 305–308. doi: 10.1006/jaer.2000.0581
Carbone, I. (2021). A population genetics approach to biological control of mycotoxin production. US patent 20210235706A1. Available online at: https://patents.google.com/patent/US20210235706A1/en (accessed December 5 2022).
Chang, P. K. (2022). Aspergillus flavus La3279, a component strain of the Aflasafe™ biocontrol product, contains a partial aflatoxin biosynthesis gene cluster followed by a genomic region highly variable among A. flavus isolates. Int. J. Food Microbiol. 366, 109559. doi: 10.1016/j.ijfoodmicro.2022.109559
Chang, P. K., Horn, B. W., and Dorner, J. W. (2005). Sequence breakpoints in the aflatoxin biosynthesis gene cluster and flanking regions in nonaflatoxigenic Aspergillus flavus isolates. Fungal Genet. Biol. 42, 914–923. doi: 10.1016/j.fgb.2005.07.004
Cotty, P. J. (2000). “Stability of modified Aspergillus flavus communities: need for area-wide management,” in Proceedings of the Beltwide Cotton Conference (Cordova, TN: National Cotton Council of America), 148. Available online at: https://www.cotton.org/beltwide/proceedings/getPDF.cfm?year=2000&paper=077.pdf (accessed December 5, 2022).
Cotty, P. J. (2006). “Biocompetitive exclusion of toxigenic fungi,” in The Mycotoxin Factbook, eds D. Barug, D. Bhatnagar, H. P. van Egdmond, J. W. van der Kamp, W. A. van Osenbruggen, and A. Visconti (The Netherlands: Wageningen Academic Publishers), 179–197. doi: 10.3920/978-90-8686-587-1
Cotty, P. J., Antilla, L., and Wakelyn, P. J. (2007). “Competitive exclusion of aflatoxin producers: farmer-driven research and development,” in Biological Control: A Global Perspective, eds C. Vincent, M. S. Goettel, and G. Lazarovits (Oxfordshire: CABI), 241–253. doi: 10.1079/9781845932657.0241
Cotty, P. J., and Jaime-Garcia, R. (2007). Influences of climate on aflatoxin producing fungi and aflatoxin contamination. Int. J. Food Microbiol. 119, 109–115. doi: 10.1016/j.ijfoodmicro.2007.07.060
Dorner, J. W. (2004). Biological control of aflatoxin contamination of crops. J. Toxicol. Toxin Rev. 23, 425–450. doi: 10.1081/TXR-200027877
Dorner, J. W. (2009). Development of biocontrol technology to manage aflatoxin contamination in peanuts. Peanut Sci. 36, 60–67. doi: 10.3146/AT07-002.1
Doster, M. A., Cotty, P. J., and Michailides, T. J. (2014). Evaluation of the atoxigenic Aspergillus flavus strain AF36 in pistachio orchards. Plant Dis. 98, 948–956. doi: 10.1094/PDIS-10-13-1053-RE
Ehrlich, K. C. (2014). Non-aflatoxigenic Aspergillus flavus to prevent aflatoxin contamination in crops: advantages and limitations. Front. Microbiol. 5, 50. doi: 10.3389/fmicb.2014.00050
Ehrlich, K. C., Moore, G. G., Mellon, J. E., and Bhatnagar, D. (2015). Challenges facing the biological control strategy for eliminating aflatoxin contamination. World Mycotoxin J. 8, 225–233. doi: 10.3920/WMJ2014.1696
Eskola, M., Kos, G., Elliott, C. T., Hajšlová, J., Mayar, S., and Krska, R. (2020). Worldwide contamination of food-crops with mycotoxins: validity of the widely cited FAO estimate of 25%. Crit. Rev. Food Sci. Nutr. 60, 2773–2789. doi: 10.1080/10408398.2019.1658570
Evans, K. J., Scott, J. B., and Barry, K. M. (2020). Pathogen incursions - integrating technical expertise in a socio-political context. Plant Dis. 104, 3097–3109. doi: 10.1094/PDIS-04-20-0812-FE
Ezekiel, C. N., Ayeni, K. I., Akinyemi, M. O., Sulyok, M., Oyedele, O. A., Babalola, D. A., et al. (2021). Dietary risk assessment and consumer awareness of mycotoxins among household consumers of cereals, nuts and legumes in North-Central Nigeria. Toxins 13, 635. doi: 10.3390/toxins13090635
Fisher, M., Abate, T., Lunduka, R. W., Asnake, W., Alemayehu, Y., and Madulu, R. B. (2015). Drought tolerant maize for farmer adaptation to drought in sub-Saharan Africa: determinants of adoption in eastern and southern Africa. Clim. Change 133, 283–299. doi: 10.1007/s10584-015-1459-2
Frisvad, J. C., Hubka, V., Ezekiel, C. N., Hong, S. B., Nováková, A., Chen, A. J., et al. (2019). Taxonomy of Aspergillus section Flavi and their production of aflatoxins, ochratoxins and other mycotoxins. Stud. Mycol. 93, 1–63. doi: 10.1016/j.simyco.2018.06.001
Grubisha, L. C., and Cotty, P. J. (2015). Genetic analysis of the Aspergillus flavus vegetative compatibility group to which a biological control agent that limits aflatoxin contamination in U.S. crops belongs. Appl. Environ. Microbiol. 81, 5889–5899. doi: 10.1128/AEM.00738-15
Hanlon, P. R., Bandyopadhyay, R., and Brorby, G. P. (2019). Evaluating the applicability of a risk-based approach (Decision Tree) to mycotoxins mitigation. Food Prot. Trends 39, 406–416. Available online at: https://www.foodprotection.org/files/food-protection-trends/sep-oct-19-hanlon.pdf
Hell, K., Cardwell, K. F., Setamou, M., and Poehling, H. M. (2000). The influence of storage practices on aflatoxin contamination in maize in four agroecological zones of Benin, west Africa. J. Stored Prod. Res. 36, 365–382. doi: 10.1016/S0022-474X(99)00056-9
Hell, K., Fandohan, P., Bandyopadhyay, R., Kiewnick, S., Sikora, R., and Cotty, P. J. (2008). “Pre- and post-harvest management of aflatoxin in maize: an African perspective,” in Mycotoxins: Detection Methods, Management, Public Health and Agricultural Trade (Wallingford: CAB International), 219–229.
Hoffmann, V., Kariuki, S., Pieters, J., and Treurniet, M. (2022). Upside risk, consumption value, and market returns to food safety. Am. J. Agric. Econ. 1–26. doi: 10.1111/ajae.12349
Horn, B. W., Sorensen, R. B., Lamb, M. C., Sobolev, V. S., Olarte, R. A., Worthington, C. J., et al. (2014). Sexual reproduction in Aspergillus flavus sclerotia naturally produced in corn. Phytopathology 104, 75–85. doi: 10.1094/PHYTO-05-13-0129-R
Huang, C., Jha, A., Sweany, R., DeRobertis, C., and Damann, K. E. (2011). Intraspecific aflatoxin inhibition in Aspergillus flavus is thigmoregulated, independent of vegetative compatibility group and is strain dependent. PLoS ONE 6, 23470. doi: 10.1371/journal.pone.0023470
Ismail, A., Naeem, I., Gong, Y. Y., Routledge, M. N., Akhtar, S., Riaz, M., et al. (2021). Early life exposure to dietary aflatoxins, health impact and control perspectives: a review. Trends Food Sci. Technol. 112, 212–224. doi: 10.1016/j.tifs.2021.04.002
Jaime, R., Antilla, L., Liesner, L., and Cotty, P. J. (2017). “Options to reduce costs of aflatoxin control in commercial cotton with area-wide programs utilizing atoxigenic biocontrol agents,” in 2017 APS Annual Meeting. St. Paul: APSNET.
JECFA (2018). Safety Evaluation of Certain Contaminants in Food: Prepared by the Eighty-Third Meeting of the Joint FAO/WHO Expert Committee on Food Additives (JECFA), ed WHO Food A. World Health Organization and Food and Agriculture Organization of the United Nations, Geneva. doi: 10.1016/j.ijfoodmicro.2007.01.001
Joffe, A. Z. (1969). Aflatoxin produced by 1,626 isolates of Aspergillus flavus from groundnut kernels and soils in Israel. Nature 221, 492. doi: 10.1038/221492a0
Johnson, A. M., Abdoulaye, T., Ayedun, B., Fulton, J. R., Widmar, N. J. O., Adebowale, A., et al. (2019). Willingness to pay of Nigerian poultry producers and feed millers for aflatoxin-safe maize. Agribusiness 36, 299–317. doi: 10.1002/agr.21621
Johnson, A. M., Fulton, J. R., Abdoulaye, T., Ayedun, B., Widmar, N. J. O., Akande, A., et al. (2018). Aflatoxin awareness and Aflasafe adoption potential of Nigerian smallholder maize farmers. World Mycotoxin J. 11, 437–446. doi: 10.3920/WMJ2018.2345
Kagot, V., Okoth, S., De Boevre, M., and De Saeger, S. (2019). Biocontrol of Aspergillus and Fusarium mycotoxins in Africa: benefits and limitations. Toxins 11, 109. doi: 10.3390/toxins11020109
Klich, M. A. (2007). Aspergillus flavus: the major producer of aflatoxin. Mol. Plant Pathol. 8, 713–722. doi: 10.1111/j.1364-3703.2007.00436.x
Konlambigue, M., Ortega-Beltran, A., Bandyopadhyay, R., Shanks, T., Landreth, E., and Jacob, O. (2020). Lessons learned on scaling Aflasafe® through commercialization in Sub-Saharan Africa. A4NH Strat. Brief. doi: 10.2499/p15738coll2.133956
Langyintuo, A. S., Mwangi, W., Diallo, A. O., MacRobert, J., Dixon, J., and Bänziger, M. (2010). Challenges of the maize seed industry in eastern and southern Africa: a compelling case for private-public intervention to promote growth. Food Policy 35, 323–331. doi: 10.1016/j.foodpol.2010.01.005
Lewis, M. H., Carbone, I., Luis, J. M., Payne, G. A., Bowen, K. L., Hagan, A. K., et al. (2019). Biocontrol strains differentially shift the genetic structure of indigenous soil populations of Aspergillus flavus. Front. Microbiol. 10, 1–17. doi: 10.3389/fmicb.2019.01738
Logrieco, A. F., Miller, J. D., Eskola, M., Krska, R., Ayalew, A., Bandyopadhyay, R., et al. (2018). The mycotox charter: increasing awareness of, and concerted action for, minimizing mycotoxin exposure worldwide. Toxins 10, 149. doi: 10.3390/toxins10040149
Mahuku, G., Mauro, A., Pallangyo, B., Nsami, E., Boni, S. B., Koyano, E., et al. (2022). Atoxigenic-based technology for biocontrol of aflatoxin in maize and groundnuts for Tanzania. World Mycotoxin J. 1–16. doi: 10.3920/WMJ2021.2758
Matumba, L., Namaumbo, S., Ngoma, T., Meleke, N., De Boevre, M., Logrieco, A. F., et al. (2021). Five keys to prevention and control of mycotoxins in grains: a proposal. Glob. Food Sec. 30, 100562. doi: 10.1016/j.gfs.2021.100562
Matumba, L., Van Poucke, C., Njumbe Ediage, E., and De Saeger, S. (2017). Keeping mycotoxins away from the food: does the existence of regulations have any impact in Africa? Crit. Rev. Food Sci. Nutr. 57, 1584–1592. doi: 10.1080/10408398.2014.993021
McEwan, M. A., Almekinders, C. J. M., Andrade-Piedra, J. J. L., Delaquis, E., Garrett, K. A., Kumar, L., et al. (2021). Breaking through the 40% adoption ceiling: mind the seed system gaps. A perspective on seed systems research for development in One CGIAR. Outlook Agric. 50, 5–12. doi: 10.1177/0030727021989346
Mehl, H. L., Jaime, R., Callicott, K. A., Probst, C., Garber, N. P., Ortega-Beltran, A., et al. (2012). Aspergillus flavus diversity on crops and in the environment can be exploited to reduce aflatoxin exposure and improve health. Ann. N. Y. Acad. Sci. 1273, 7–17. doi: 10.1111/j.1749-6632.2012.06800.x
Michaels, R., and McDonald, D. W. (2021). Large Peaks of Aspergillus flavus Propagules Observed at Cotton Fields. Available online at: https://www.phenotypescreening.com/click_files/Poster4.pdf (accessed December 5, 2022).
Migwi, B., Mutegi, C., Mburu, J., Wagacha, J., Cotty, P. J., Bandyopadhyay, R., et al. (2020). Assessment of willingness-to-pay for Aflasafe KE01, a native biological control product for aflatoxin management in Kenya. Food Addit. Contam. Part A Chem. Anal. Control. Expo. Risk Assess. 37, 1951–1962. doi: 10.1080/19440049.2020.1817571
Molo, M. S., Heiniger, R. W., Boerema, L., and Carbone, I. (2019). Trial summary on the comparison of various non-aflatoxigenic strains of Aspergillus flavus on mycotoxin levels and yield in maize. Agron. J. 111, 942–946. doi: 10.2134/agronj2018.07.0473
Molo, M. S., White, J. B., Cornish, V., Gell, R. M., Baars, O., Singh, R., et al. (2022). Asymmetrical lineage introgression and recombination in populations of Aspergillus flavus: implications for biological control. PLoS ONE 17, e0276556. doi: 10.1371/journal.pone.0276556
Moore, G. G. (2021). Practical considerations will ensure the continued success of pre-harvest biocontrol using non-aflatoxigenic Aspergillus flavus strains. Crit. Rev. Food Sci. Nutr. 62, 4208–4224. doi: 10.1080/10408398.2021.1873731
Moore, G. G., Elliott, J. L., Singh, R., Horn, B. W., Dorner, J. W., Stone, E. A., et al. (2013). Sexuality generates diversity in the aflatoxin gene cluster: evidence on a global scale. PLoS Pathog. 9, 1003574. doi: 10.1371/journal.ppat.1003574
Moore, G. G., Lebar, M. D., and Carter-Wientjes, C. H. (2019). The role of extrolites secreted by nonaflatoxigenic Aspergillus flavus in biocontrol efficacy. J. Appl. Microbiol. 126, 1257–1264. doi: 10.1111/jam.14175
Moral, J., Garcia-Lopez, M. T., Camiletti, B. X., Jaime, R., Michailides, T. J., Bandyopadhyay, R., et al. (2020). Present status and perspective on the future use of aflatoxin biocontrol products. Agronomy 10, 491. doi: 10.3390/agronomy10040491
Narayan, T. A., and Geyer, J. (2022). Can results-based prizes to private sector incentivize technology adoption by farmers? Evidence from the AgResults Nigeria project that uses prizes to incentivize adoption of Aflasafe™. Agric. Food Secur. 11, 1–11. doi: 10.1186/s40066-022-00377-2
Nelson, R. (2020). International plant pathology: past and future contributions to global food security. Phytopathology™ 110, 245–253. doi: 10.1094/PHYTO-08-19-0300-IA
Ola, O. T., Ogedengbe, O. O., Raji, T. M., Eze, B., Chama, M., Ilori, O. N., et al. (2022). Aflatoxin biocontrol effectiveness in the real world — private sector-led efforts to manage aflatoxins in Nigeria through biocontrol-centered strategies. Front. Microbiol. 13, 977789. doi: 10.3389/fmicb.2022.977789
Olarte, R. A., Worthington, C. J., Horn, B. W., Moore, G. G., Singh, R., Monacell, J. T., et al. (2015). Enhanced diversity and aflatoxigenicity in interspecific hybrids of Aspergillus flavus and Aspergillus parasiticus. Mol. Ecol. 24, 1889–1909. doi: 10.1111/mec.13153
Ortega-Beltran, A., Agbetiameh, D., Atehnkeng, J., Falade, T. D. O., and Bandyopadhyay, R. (2020). Does the use of atoxigenic biocontrol products to mitigate aflatoxin in maize increase fumonisin content in grains? Plant Dis. 105, 2196–2201. doi: 10.1094/PDIS-07-20-1447-RE
Ortega-Beltran, A., and Bandyopadhyay, R. (2021). Contributions of integrated aflatoxin management strategies to achieve the sustainable development goals in various African countries. Glob. Food Sec. 30, 100559. doi: 10.1016/j.gfs.2021.100559
Ortega-Beltran, A., Kaptoge, L., Senghor, A. L., Aikore, M., Jarju, P., Momanyi, H., et al. (2021). Can it be all more simple? Manufacturing aflatoxin biocontrol products using dry spores of atoxigenic isolates of Aspergillus flavus as active ingredients. Microb. Biotechnol. 15, 901–914. doi: 10.1111/1751-7915.13802
Ortega-Beltran, A., Moral, J., Picot, A., Puckett, R. D., Cotty, P. J., and Michailides, T. J. (2019). Atoxigenic Aspergillus flavus isolates endemic to almond, fig, and pistachio orchards in California with potential to reduce aflatoxin contamination in these crops. Plant Dis. 103, 905–912. doi: 10.1094/PDIS-08-18-1333-RE
Pitt, J. (2019). The pros and cons of using biocontrol by competitive exclusion as a means for reducing aflatoxin in maize in Africa. World Mycotoxin J. 12, 103–112. doi: 10.3920/WMJ2018.2410
Rutsaert, P., Donovan, J., and Kimenju, S. (2021). Demand-side challenges to increase sales of new maize hybrids in Kenya. Technol. Soc. 66, 101630. doi: 10.1016/j.techsoc.2021.101630
Saha Turna, N., and Wu, F. (2021). Estimation of tolerable daily intake (TDI) for immunological effects of aflatoxin. Risk Anal. 42, 431–438. doi: 10.1111/risa.13770
Sarrocco, S., Mauro, A., and Battilani, P. (2019). Use of competitive filamentous fungi as an alternative approach for mycotoxin risk reduction in staple cereals: state of art and future perspectives. Toxins. 11, 701. doi: 10.3390/toxins11120701
Schmidt, M. A., Mao, Y., Opoku, J., and Mehl, H. L. (2021). Enzymatic degradation is an effective means to reduce aflatoxin contamination in maize. BMC Biotechnol. 21, 70. doi: 10.1186/s12896-021-00730-6
Schreurs, F., Bandyopadhyay, R., Kooyman, C., Ortega-Beltran, A., Akande, A., Konlambigue, M., et al. (2019). “Commercial products promoting plant health in African agriculture,” in Critical Issues in Plant Health: 50 Years of Research in African Agriculture (Cambridge, UK: Burleigh Dodds Science Publishing), 345–364. doi: 10.19103/AS.2018.0043.14
Schut, M., Leeuwis, C., and Thiele, G. (2020). Science of scaling: understanding and guiding the scaling of innovation for societal outcomes. Agric. Syst. 184, 102908. doi: 10.1016/j.agsy.2020.102908
Senghor, A. L., Ortega-Beltran, A., Atehnkeng, J., Jarju, P., Cotty, P. J., and Bandyopadhyay, R. (2021). Aflasafe SN01 is the first biocontrol product approved for aflatoxin mitigation in two nations, Senegal and The Gambia. Plant Dis. 105, 1461–1473. doi: 10.1094/PDIS-09-20-1899-RE
Senghor, L. A., Ortega-Beltran, A., Atehnkeng, J., Callicott, K. A., Cotty, P. J., and Bandyopadhyay, R. (2020). The atoxigenic biocontrol product Aflasafe SN01 is a valuable tool to mitigate aflatoxin contamination of both maize and groundnut cultivated in Senegal. Plant Dis. 104, 510–520. doi: 10.1094/PDIS-03-19-0575-RE
Sharma, K. K., Pothana, A., Prasad, K., Shah, D., Kaur, J., Bhatnagar, D., et al. (2018). Peanuts that keep aflatoxin at bay: a threshold that matters. Plant Biotechnol. J. 16, 1024–1033. doi: 10.1111/pbi.12846
Singh, P., Callicott, K. A., Orbach, M. J., and Cotty, P. J. (2020). Molecular analysis of S-morphology aflatoxin producers from the United States reveals previously unknown diversity and two new taxa. Front. Microbiol. 11, 1236. doi: 10.3389/fmicb.2020.01236
Sinyolo, S. (2020). Technology adoption and household food security among rural households in South Africa: the role of improved maize varieties. Technol. Soc. 60, 101214. doi: 10.1016/j.techsoc.2019.101214
Stepman, F. (2018). Scaling-up the impact of aflatoxin research in Africa. The role of social sciences. Toxins 10, 136. doi: 10.3390/toxins10040136
Sweany, R., Mack, B., Moore, G., Gilbert, M., Cary, J., Rajasekaran, K., et al. (2021). Genetic responses and aflatoxin inhibition during interaction between aflatoxigenic and non-aflatoxigenic Aspergillus flavus. Toxins 13, 794. doi: 10.3390/toxins13110794
Sweany, R. R., and Damann, K. E. (2020). Influence of neighboring clonal-colonies on aflatoxin production by Aspergillus flavus. Front. Microbiol. 10, 3038. doi: 10.3389/fmicb.2019.03038
Thakare, D., Zhang, J., Wing, R. A., Cotty, P. J., and Schmidt, M. A. (2017). Aflatoxin-free transgenic maize using host-induced gene silencing. Sci. Adv. 3, e1602382. doi: 10.1126/sciadv.1602382
Udomkun, P., Wiredu, A. N., Nagle, M., Bandyopadhyay, R., Müller, J., and Vanlauwe, B. (2017). Mycotoxins in sub-Saharan Africa: present situation, socio-economic impact, awareness, and outlook. Food Control 72, 110–122. doi: 10.1016/j.foodcont.2016.07.039
Unnevehr, L. J. (2022). Addressing food safety challenges in rapidly developing food systems. Agric. Econ. 53, 529–539. doi: 10.1111/agec.12724
Walker, S., Jaime, R., Kagot, V., and Probst, C. (2018). Comparative effects of hermetic and traditional storage devices on maize grain: mycotoxin development, insect infestation and grain quality. J. Stored Prod. Res. 77, 34–44. doi: 10.1016/j.jspr.2018.02.002
Weaver, M. A., and Abbas, H. K. (2019). Field displacement of aflatoxigenic Aspergillus flavus strains through repeated biological control applications. Front. Microbiol. 10, 1788. doi: 10.3389/fmicb.2019.01788
Keywords: effective aflatoxin biocontrol, technology uptake, scaling up, food safety, food security
Citation: Ortega-Beltran A and Bandyopadhyay R (2023) Aflatoxin biocontrol in practice requires a multidisciplinary, long-term approach. Front. Sustain. Food Syst. 7:1110964. doi: 10.3389/fsufs.2023.1110964
Received: 29 November 2022; Accepted: 18 January 2023;
Published: 10 February 2023.
Edited by:
Chibundu Ngozi Ezekiel, Babcock University, NigeriaReviewed by:
John M. Wagacha, University of Nairobi, KenyaAmare Ayalew, African Union Commission, Ethiopia
Copyright © 2023 Ortega-Beltran and Bandyopadhyay. This is an open-access article distributed under the terms of the Creative Commons Attribution License (CC BY). The use, distribution or reproduction in other forums is permitted, provided the original author(s) and the copyright owner(s) are credited and that the original publication in this journal is cited, in accordance with accepted academic practice. No use, distribution or reproduction is permitted which does not comply with these terms.
*Correspondence: Alejandro Ortega-Beltran, YS5iZWx0cmFuQGNnaWFyLm9yZw==