- 1Departamento de Ingeniería Bioquímica, Escuela Nacional de Ciencias Biológicas, Instituto Politécnico Nacional, Mexico City, Mexico
- 2Facultad de Ciencias Químicas, Universidad Veracruzana, Xalapa, Mexico
- 3Laboratorio de Toxicología, Facultad de Biología, Universidad Veracruzana, Xalapa, Mexico
- 4Instituto de Ciencias Básicas, Universidad Veracruzana, Xalapa, Mexico
Stingless bees are essential to preser tropical ecosystems. They pollinate native flora, producing honey with properties for traditional health uses. Lactic acid bacteria spontaneously ferment honey in stingless bee honey (SBH). This study aims to determine the main physicochemical characteristics of Melipona beecheii, Scraptotrigona pectoralis, Plebeia jatiformis and Plebeia llorentei honey and to isolate and identify FLAB present in SBH samples. The physicochemical properties of SBH, such as color, pH, acidity, sugars, protein, total soluble solids, water activity, total polyphenols, and antioxidant activity, were determined since these parameters can be related to the presence of some bacteria groups, and with health benefits for humans and the hive ecosystems. FLAB harvested from honey, taken directly from storing pots of the hives, were identified by 16S ribosomal RNA sequencing and preserved for future biotechnological use due to their resistance to non-ionic osmotic stress. The results showed significant differences in the physicochemical characteristics of SBH samples. Seven FLAB from four stingless bee species were identified as Fructobacillus pseudoficulneus and F. tropaeoli. In addition, three other strains of Fructilactobacillus spp. were identified only at the genus level. All species showed the ability to grow under different carbon sources, resulting in negative hemolysis and sensitivity to cefuroxime, erythromycin, and chloramphenicol. To the best of our knowledge, this is the first time that the physicochemical and FLAB characterization of SBH from P. jatiformis and P. llorentei has been reported. Therefore, the future following research should be focused on the environmental, health and food biotechnological applications implications of FLAB from SBH.
1. Introduction
Stingless bees, meliponines, or indigenous bees comprise a broad group of social bees (Nordin et al., 2018). They can be found in tropical or subtropical regions, such as Australia, Africa, Southeast Asia, and tropical America, mainly in warm and humid forests. Most of these species are native to Central and South America, and many are still wild. They form a honeycomb with a similar structure (with pots, breeding panels, wax and propolis), as shown in Figure 1. Some species are farmed due to the medicinal and nutritional properties of their honey, royal jelly, and pollen (Leonhardt, 2017). More than 46 species of stingless bees have been reported in Mexico, most of them in the country's southwest (Quezada-Euán, 2018).
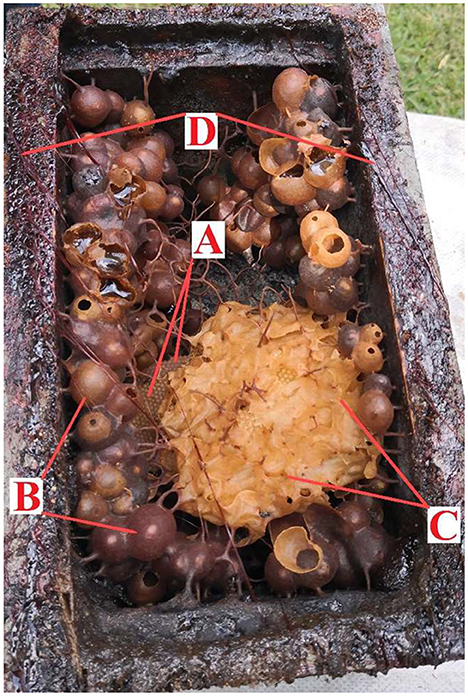
Figure 1. Stingless bee honeycomb structure of Plebeia jorentei: brood nest (A), storing pots of honey and pollen (B), protective wax (C), and propolis to seal (D).
Honey is a natural viscous sweetener made by several bees. The honey produced by the Apis mellifera bee is the most studied worldwide. Generally, it contains 80–85% carbohydrates, 15–17% water, 0.3% proteins, 0.2% ashes and minor quantities of amino acids, phenols, pigments and vitamins (Alvarez-Suarez et al., 2018; Khan et al., 2018). However, over 600 species of Meliponini (stingless bees) produce honey classified as SBH. Significant differences have been found between the honey produced by Apis mellifera honey and the different species of SBH, which difficult their quality regulation and characterization (Nordin et al., 2018). SBH generally has more moisture, a peculiar flavor, a varied color range, and a more pronounced aroma (Rozman et al., 2022).
The SBH has more moisture than Apis spp. honey, so some microorganisms, such as Lactic Acid Bacteria (LAB), consume part of the sugar and transform it into lactic acid through anaerobic fermentation (Souza et al., 2021). Because of the nutritional compounds of honey, the growth of bacteria is allowed without spoiling the honey. These bacteria modulate the properties of honey through fermentation. Thus, an interaction between the food and the living microorganism is generated (Mărgăoan et al., 2020). Some microorganisms contribute to honey conservation and nutrient digestion (Belina-Aldemita et al., 2020; Souza et al., 2021).
Fructophilic lactic acid bacteria (FLAB) are a recently discovered group of previously considered LAB with some unique biochemical characteristics. They prefer fructose as substrate, and their growth in glucose is poor. FLAB use fructose as a substrate and electron acceptor because they usually live in fructose-rich habitats (Filannino et al., 2019). So, they can be found in flowers, fruits, fermented food derived from fruits, and honey (Endo et al., 2018). FLAB are heterofermentative since they generate other products (carbon dioxide, acetate, ethanol and mannitol) in addition to lactate. Moreover, they metabolize different carbohydrates, and some strains can metabolize p-coumaric acid (the main phenolic acid in pollen) to phloretic acid or p-vinylphenol (Endo et al., 2018) and have shown high tolerance to non-ionic osmotic stress (Ali et al., 2017).
The richness of bioactive metabolites in SBH could be responsible for traditional medical and therapeutic uses. For example, honey is rich in antioxidant compounds like flavonoids, phenolic compounds, and bioactive peptides, which can prevent health issues (Nordin et al., 2018). Among the SBH beneficial properties, the antimicrobial activity, the antioxidant and hypolipidemic effect, the protection from injuries caused by dyslipidemia, the anti-inflammatory activity against chronic subclinical systemic inflammation, and the prevention of Staphylococcus aureus infection have been reported (Pimentel et al., 2022). In addition, SBH has been used to prevent and treat diverse diseases in Mexico (Sánchez Cano, 2019), Kenya (Sabella et al., 2022) and Guatemala (de la Roca, 2018), like:
- Respiratory diseases (cough, pneumonia, sinusitis, laryngitis, and asthma);
- Stomach upsets (diarrhea, ulcers, and indigestion);
- Skin problems (wounds, blemishes, measles, allergy, itching, burns, and hemorrhoids);
- Eye conditions (cataracts, carnosities, conjunctivitis);
- Prevent anemia or recover energy during pregnancy or after childbearing.
However, despite its potential importance for health and food, there are few studies about the characterization of fresh SBH to differentiate it from an old SBH. Most of the research has been carried out on the genera Melipona and Scaptotrigona (Jimenez et al., 2016; Ávila et al., 2018), and for the genus Plebeia, there are only three reports to date (Duarte et al., 2012, 2018; Echeverrigaray et al., 2021).
LAB have been studied from the gastrointestinal tract of Apis spp. and some stingless bees, but not in honey samples. In A. mellifera were identified Lactobacillus johnsonii and Enterococcus faecium (Carina Audisio et al., 2011), and in A. dosarta honey, Weissella spp. were found. In addition, Torres-Moreno et al. (2021) recently isolated LAB from the intestine of M. beecheii, S. pectoralis, and P. jatiformis (stingless bees), finding strains of Apilactobacillus spp., Lactiplantibacillus plantarum, W. paramesenteroides and Leuconostoc citreum. All these unique characteristics of FLAB have made them a novel candidate for environmental, food and health biotechnology (Agagündüz et al., 2022). Therefore, this research aimed to determine the main physicochemical characteristics of honey from Melipona beecheii, Scraptotrigona pectoralis, Plebeia jatiformis and Plebeia llorentei (Figure 2) and to isolate and identify FLAB in the honey samples.
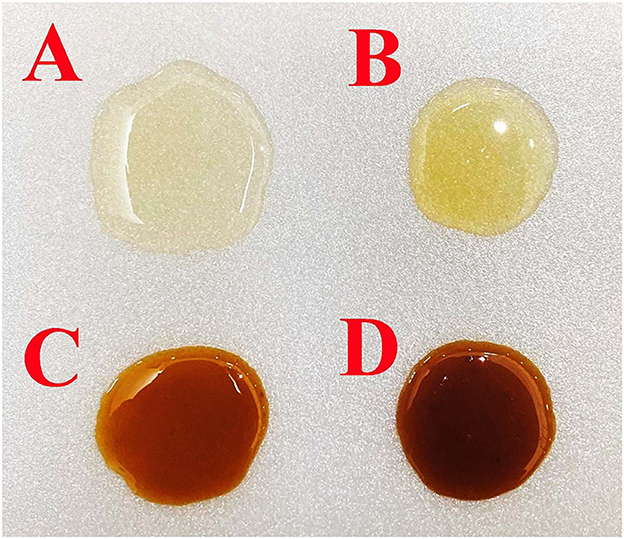
Figure 2. Melipona beecheii (A), Scaptotrigona pectoralis (B), Plebeia jatiformis (C), and Plebeia llorentei (D) honey samples taken from the same honeybee farm.
2. Materials and methods
2.1. Samples
Mr. Juan Pale kindly donated the SBH samples. The samples were collected in September 2020 from honeycombs belonging to “El Rinconcito”, a honeybee farm in Teocelo, Veracruz, Mexico (19.393640787641715, −96.97934590967033). Each sample (30 mL) of SBH was taken with sterile material directly from the honey-storing pots (Figures 1, 3) of four stingless bee specimens and kept in tubes under refrigeration. The bees were previously identified as Melipona beecheii, Scaptotrigona pectoralis, Plebeia llorentei, and Plebeia jatiformis (Torres-Moreno et al., 2021).
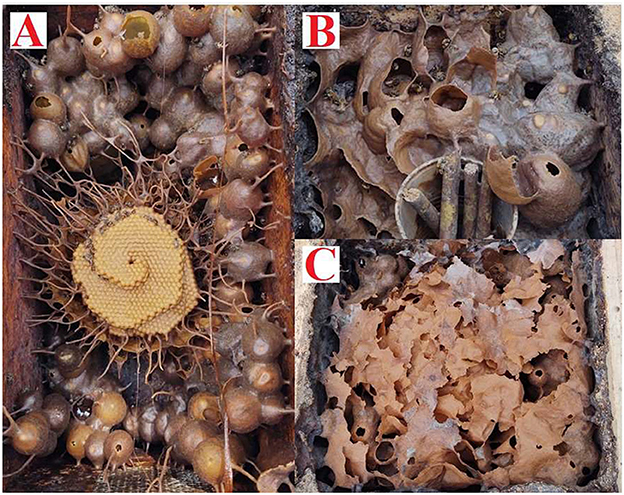
Figure 3. Honeycombs of Plebeia llorentei (A), Melipona beecheii (B), and Scaptotrigona pectoralis (C).
2.2. Analytical methods to determine physicochemical parameters in honey
2.2.1. Color intensity: ABS635nm
The color intensity of SBH was measured according to Smetanska et al. (2021) with slight modifications. First, one gram of each honey sample was diluted 1:1 with distilled water. Then, the homogenized solution was centrifuged at 14,000 × g for 5 min, and the absorbance was measured at 635 nm (spectrophotometer VE-5100UV, Cientifica Velaquin, Mexico). Finally, the color intensity was determined by converting the absorbance value to the Pfund scale with equation (1).
2.2.2. pH, free acidity, lactonic acidity, and total acidity
The pH was determined with a potentiometer. The acidity (free, lactone, and total) was measured according to the AOAC methods (Lee et al., 2005). The free acidity was calculated according to equation (2), the lactonic acidity with equation (3), and the total acidity with equation (4).
Where FA means free acidity, LA means lactonic, and TA is total acidity.
2.2.3. Sugar and soluble protein content
Reducing sugars were determined by the 3,5-dinitrosalicylic acid (DNS) method. First, the sample was diluted to 0.001% (100 mg of honey in 100 mL of distilled water), and 200 μL of the diluted sample were taken and mixed with 200 μL of the DNS reagent (1% 3,5-dinitrosalicylic acid and 12% sodium potassium tartrate in 0.4 M NaOH). The mixture was heated for 15 min at 100°C and then diluted with 8 ml of distilled water in an ice bath. Then, the percentage of reducing sugar was determined by measuring the absorbance of the sample and making a standard curve of 100 mg of glucose in 100 mL of distilled water, which would be 100% glucose, and their respective dilutions (80, 60, 40, 20, and 0%) at 540 nm in a multiplate reader (Multiskan go, Thermoscienfic, USA) (Susilowati and Azkia, 2022).
The total sugar content was determined by the phenol-sulfuric acid method, described by Trinh et al. (2022), with some modifications. First, the honey (1 g) was dissolved in 20 mL of water and then made up to 100 mL with distilled water. The previous solution was mixed, and 1 mL was taken to bring it to a volume of 100 mL with distilled water. Later, 5 mL of concentrated H2SO4 was added to the second dilution of honey (1 mL) in a test tube, mixed carefully and allowed to react for 10 min and placed in an ice bath. When it reached room temperature, phenol 5% (3 mL) was added and mixed. The solution was allowed to stand for 30 min and then, absorbance measured spectrophotometrically at 490 nm. A calibration curve was made with different concentrations of glucose. This assay was repeated three times.
The Bradford method was used to determine the soluble protein content in the SBH samples (Naree et al., 2021). The assay was carried out in a multiplate reader (Multiskan go, Thermoscienfic, USA) at 595 nm. Honey was first diluted 1:1 with distilled water. Then, for the quantification, 10 μL of the diluted sample was placed in the wells, and 190 μL of Bradford reagent was added, allowing the mixture to react for 5 min. The calibration curve used a serial dilution of 0.2 to 1.4 mg of ovalbumin per 1 mL of distilled water.
2.2.5. Total soluble solids (TSS), moisture, and water activity (aw)
The TSS, refractive index, moisture and aw are related parameters that were determined according to Anguebes et al. (2016). The TSS was measured by °Brix of the honey with a refractometer (ATAGO, Pocket Refractometer model PAL-1) at 20°C. Moisture was calculated by subtracting the amount of TSS from the total content of the product to determine the water content in honey (Bogdanov et al., 2002). The water activity (aw) was determined by the dew point method, according to Balzan et al. (2020); the AquaLab 4TE-Decagon equipment (Decagon Devices, Pullman, WA, USA) was used at an ambient temperature of 25°C, calibrating the equipment with activated carbon.
2.2.6. Phenolic compounds and antioxidant activity
The total phenolic content of honey samples was determined using the Folin-Ciocalteu method, according to Alvarez-Suarez et al. (2018) with slight modifications. So, 1 g of honey was diluted to 10 mL with distilled water and filtered through a 0.45 μm pore filter. Total phenolic content results were expressed as mg of gallic acid equivalents (GAE) per 100 g of honey (mg GAE 100 g−1 of honey) with a gallic acid standard curve.
To determine the antioxidant content or antioxidant activity, 2,2′-azinobis (3-ethylbenzothiazoline-6-sulfonic acid) diammonium salt (ABTS), 2,2-diphenyl-2-picrylhydrazyl hydrate (DPPH) and the potassium ferricyanide reduction (FRAP) methods were used (Badrulhisham et al., 2020). The dilution and filtration of the sample were carried out in the same way as in the total polyphenol content method. Ten μL of diluted honey and 190 μL of previously prepared ABTS solution (0.700 ± 0.05 nm) were mixed and incubated in the dark at 30°C for 6 minutes. After the incubation, the absorbance of the mixture was measured at 734 nm. Ten μL of 80% methanol were used as a blank. For DPPH, 10 μL of diluted honey and 290 μL of a previously prepared DPPH solution (0.04 mg mL−1) were mixed and incubated in the dark at room temperature (22°C) for 30 min. As a blank, 80% methanol was used, and the absorbances were measured at 517 nm. For the potassium ferricyanide reduction method, the FRAP reagent was prepared by mixing 1% of K3Fe(CN)6 solution (w/v, in Milli-Q water) with 1 M HCl (v/v, in Milli-Q water), 1% sodium dodecyl sulfate solution (w/v, in Milli-Q water), and 0.2% ferric (III) chloride solution (w/v, in Milli-Q water) at a ratio of 3:3:1:1. Then, 50 μL of the diluted honey sample were mixed with 200 μL of the prepared FRAP, and incubated for 20 min at 50°C. The absorbances were read at 750 nm, using the FRAP reagent as blank. All absorbance measurements were performed in triplicate on a microplate spectrophotometer (Multiskan go, Thermoscienfic, USA).
A Trolox standard curve (0, 5, 15, 25, 40, 55, 70, 90, and 120 μg Trolox mL−1) was performed for ABTS, DPPH and FRAP. The reducing antioxidant power of SBH samples was expressed in milligrams of Trolox equivalents of antioxidant capacity per kilogram of honey (mg TEAC kg−1of honey).
2.2.7. Enumeration of LAB
The enumeration of LAB present in SBH was carried out according to Mathialagan et al. (2018), with some modifications. Plate count was used to enumerate LAB and FLAB populations under aerobic and static conditions. Plates were first prepared with the Man, Rogosa and Sharpe (MRS) agar supplemented with 2% fructose and 0.8% calcium carbonate (CaCO3). Then, serial dilutions were made with 1 ml of the honey sample up to 1 × 10−4 dilution, with the drop plate technique (Naghili et al., 2013), three micro drops of 10 μL were taken from each dilution to place them on the plates. The plates were incubated at 30°C for 3 days. The bacterial colonies were enumerated and expressed as Colony Forming Units per mL (CFU mL−1).
2.5. Isolation of lactic acid bacteria from honey samples
LAB isolation was performed according to Aween et al. (2012), with some modifications. A diluted honey solution was made using 1 mL of fresh honey diluted 1:10 with peptone water (0.1% w/v). To induce bacteria growth, 1 mL of the diluted honey solution was added to 9 mL of MRS broth supplemented with 2% fructose (MRS-2F) and 0.8% of CaCO3. The MRS-2F broth supplemented with CaCO3 and honey was incubated for 2 days under microaerophilic conditions at 30°C. This culture was used to streak in a Petri dish with MRS-2F agar added with 0.8% CaCO3. In addition, striatal inoculation was performed with the diluted honey solution directly to the Petri dishes (MRS-2F agar added with 0.8% CaCO3) without previously inducing growth in broth. All the plates were incubated for three days under aerobic and static conditions at 30°C. Colonies that grew were replated (three to four times) in new Petri dishes (in the same conditions) to ensure isolation. Well-isolated colonies were picked and transferred to MRS-2F broth, adding the same volume of glycerol 1:1 with distilled water. For further studies, the strains were stored at −80°C and maintained on glycerol stocks (Arencibia et al., 2008).
2.6. Identification of isolated colonies
Phenotypic identification of seven isolated bacteria was made to verify that the strains were catalase-negative, Gram-positive and rod-shaped. The size was measured by image analysis using ImageJ 1.53t (public domain, USA). The strains that did not meet the characteristics of LAB were discarded. A carbohydrate fermentation profile with API 50 CHL was carried out for the presumptive LAB under anaerobic and static conditions (Syed Yaacob et al., 2018). A molecular identification was carried out according to Torres-Moreno et al. (2021), with slight modifications. Genomic DNA was extracted using the cetyltrimethylammonium bromide (CTAB) method (Aboul-Maaty and Oraby, 2019), using the 16S rRNA gene to identify LAB. The PCR amplification programs consisted of (1) 7 min at 95°C, (2) 35 cycles of 1 min at 94°C+1 min at 63°C+1 min at 72°C and (4) and a final extension of 10 min at 72°C, using the primer UniBac-Forward (GAT CCT GGC TCA GGA TGA AC) and UniBac-Reverse (GGA CTA CCA GGG TAT CTA ATC) in T100 thermal cycling (BIO-RAD, USA). PCR products were sequenced using the Genetic Analyzer 3130xl sequencer (Applied Biosystems, USA) to obtain approximately 790 bp sequences.
2.7. Phylogenetic analysis of gene 16S rRNA
The obtained sequences were compared to published sequences of 16S rRNA genes from different species of the Order Lactobacillales. Sequences were aligned using the MUSCLE algorithm in UGENE v33.0 (Okonechnikov et al., 2012) and edited with PhyDE-1v0.9971 (http://www.phyde.de/download.html). The phylogenetic analysis was conducted for Bayesian Inference using MrBayes 3.2.5 software (Ronquist et al., 2012) using the model for molecular evolution TVMef +I+G selected according to the best fit using jModelTest v0.1.1 (Posada, 2008). The phylogenetic analysis was run for 10 million generations, sampling every 1000 generations. Bayesian posterior probability values were calculated, considering nodes significantly supported if posterior probabilities were ≥0.95. Trees were visualized in FigTree v1.3.1(http://tree.bio.ed.ac.uk/software/figtree/).
2.8. Safety of the strains
Hemolysis and antibiotic resistance assays were determined (Mohammad et al., 2020) by activating the strains with MRS-2F broth until reaching 1 × 108 CFU mL−1. Subsequently, each strain was seeded on the entire surface of blood agar for the hemolysis test and MRS-2F agar for the antibiotic susceptibility test, placing on the MRS-2F agar the usual antibiotic disks used for Gram-positive bacteria immediately after the strains were spread. All plates were incubated for 48 h at 37°C under aerobic conditions. Staphylococcus aureus ATCC 6538 and Listeria monocytogenes ATCC7644 were positive controls for α-hemolysis and β-hemolysis. For antibiotic resistance, the diameter (mm) of growth inhibition of each antibiotic was measured.
2.9. Statistical analysis
All measurements were performed (n = 3) to obtain the mean, standard deviation, and one-way analysis of variance (ANOVA). For honey, the independent variable was the origin (species of bee) since the samples were taken simultaneously and in the same place. Comparisons between species were made in pairs using the Tukey test with a confidence of α = 0.05. All statistical analyzes were performed using SigmaPlot 14.0 (Systat Software Inc., London, UK).
3. Results and discussion
3.1. Physicochemical characterization of stingless bee honey
The physicochemical data of the honey produced by M. beecheii, S. pectoralis, P. llorentei, and P. jatiformis is shown in Table 1. Most of the parameters are within the range of the results reported by other authors. Different factors influence the composition of honey, like the bee species, the environment and the extraction method. In this study, to reduce these parameters, the procedure was standardized to reduce variability, since all the samples were taken freshly from one pot from each hive belonging to each bee specie (Mohammed, 2020). The physicochemical parameters of the SBH were essential to predict the presence of different LAB and FLAB in the hives and their stress-related characteristics (Braghini et al., 2021).
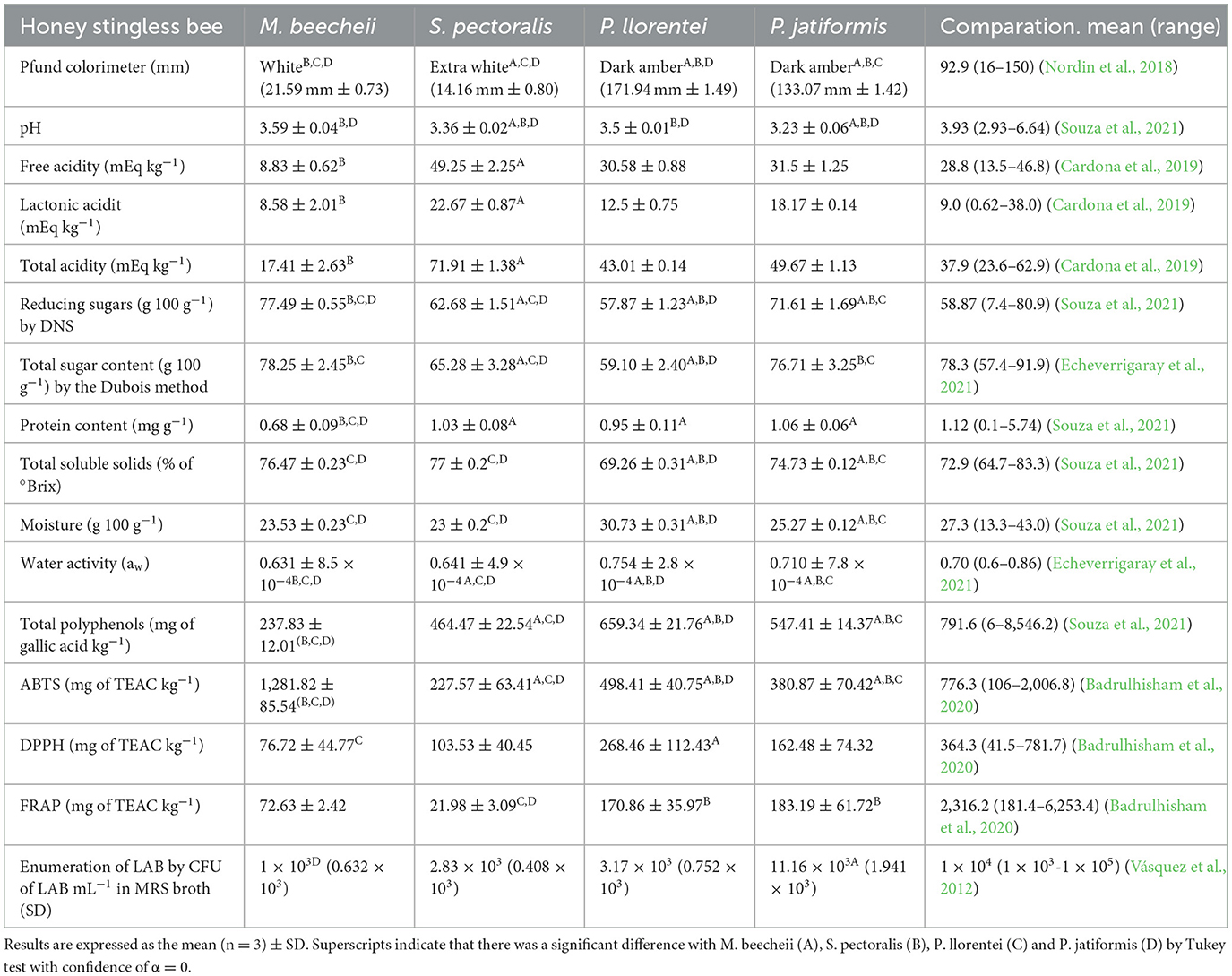
Table 1. Physicochemical characterization of the honey produced by stingless honeybees: Melipona beecheii, Scaptotrigona pectoralis, Plebeia llorentei, and Plebeia jatiformis.
The Pfund scale determined the color of the samples. All SBH samples were statistically different (p ≤ 0.001). There are no food regulations regarding the color of SBH (Tan et al., 2021). In this research, the P. llorentei and P. jatiformis honey was dark, and lighter in the samples from S. pectoralis and M. beecheii. Nordin et al. (2018) and Ávila et al. (2019) analyzed the color of 24 honey samples from the Melipona spp. with a color ranking from clear to dark (0.3 to 152 mm Pfund units) and eight S. bipunctata samples (5.87 to 118.03 mm). These results indicated that the color of the honey is independent of the bee species and that it is normal to find light-colored honey like the one found in this work (S. pectoralis honey with 14.5 mm Pfund units). Finally, the color of Plebeia molesta has been described between light and dark amber without a Pfund measurement, so it is important to perform the color assay (Geisa et al., 2021).
3.1.1. Free acidity, lactonic acidity, total acidity, and pH
The pH of S. pectoralis and P. jatiformis honey was statistically different (p = 0.012), while the pH of P. llorentei and M. beecheii was similar (p = 0.07). All the pH values were lower than the average value reported by Souza et al. (2021). Nectar, mandibular bee substances, plant species, and soil composition influence the pH. Low pH prevents undesirable microorganisms (Nascimento et al., 2015).
The lactonic and total acidity were statistically different in all honey produced by the tested bee species (p ≤ 0.014). For free acidity, M. beecheii and S. pectoralis were statistically different (p ≤ 0.001), but free acidity values between P. jatiformis and P. llorentei had no significant difference (p = 0.851). The free acidity is an indicator of freshness, and the maximum limit, according to International Honey Commission for Apis mellifera, is 50 mEq kg−1. So, the samples could be considered fresh (Bogdanov et al., 2002). In addition, M. beecheii honey from Mexico had 5.9 mEq kg−1, as previously found (Santiesteban-Hernández et al., 2003). Therefore, lactonic acid is another indicator of the freshness in honeybee. The reported values were also like those previously reported (Lage et al., 2012; Cardona et al., 2019; Scholz et al., 2020). Therefore, results indicated the freshness of the honeybee samples collected directly from the beehives.
3.1.2. Sugar and soluble protein content
Table 1 shows the differences of sugar and protein content in the samples of Melipona beecheii, Scaptotrigona pectoralis, Plebeia llorentei, and Plebeia jatiformis honey.
All the reducing sugar results were statistically different (p < 0.001). The average of reducing sugars previously found was between 7.45 to 97.10 g 100 g−1 (Ávila et al., 2018; Nordin et al., 2018; Souza et al., 2021). The reduced sugar concentration was like that previously reported by Trinh et al. (2022), who found a range between 74.28 to 88.47 g 100 g−1 in 13 honey samples. Moreover, as expected, the total sugar content was higher than the reducing sugars because of the presence of non-reducing sugars such as sucrose, trehalose and raffinose, carbohydrates that can be metabolized by FLAB (Mahmood and Abbas, 2020). For total sugars, all the honey samples were statistically different (p ≤ 0.007), except for M. beecheii and P. jatiformis, which were statistically similar (p = 0.789). Honey is a carbohydrate source but not a food rich in protein. However, the protein present in the honey is vital for the quality of life in the hives to feed the bees (Camilli et al., 2020). Stingless bees are more selective in collecting pollen because it is their honey's primary protein source. However, enzymes involved in sugar metabolism (for example, glucose oxidase, amylases, invertases and glucosidases) produced by the hypopharyngeal glands of stingless bees also count in the protein concentration of honey (Ahmad et al., 2021). Generally, the samples analyzed here had higher soluble protein values than previous reports of SBH (Villacrés-Granda et al., 2021) and like those found in Taiwan and Thailand (Chen et al., 2019).
Furthermore, the difference in the protein content of SBH produced by S. pectoralis, P. jatiformis and P. llorentei was not statistically significant (p ≤ 0.001). In contrast, the reduced protein content in M. beecheii honey was significantly different (p ≥ 0.194) compared to the honey of the other bee species. The samples were taken in the same geographical region, and the environment where the bees look for the nectar is the same, but even so, the honey turned out to have differences as Ávila et al. (2018), demonstrating that there are variations in the chemical composition of SBH of the same species. The protein from pollen in honey is important because it modulates and enhances the development and growth of LAB, such as Fructobacillus spp. and Lactobacillus spp. (di Cagno et al., 2019).
3.1.3. Total soluble solids, moisture, and water activity
The results of total soluble solids (TSS) (%), moisture (%), and water activity (aw) in the samples of Melipona beecheii, Scaptotrigona pectoralis, Plebeia llorentei, and Plebeia jatiformis honey are shown in Table 1.
All the samples were statistically different (p ≤ 0.001) in TSS, moisture and aw except for M. beecheii and S. pectoralis (p ≥ 0.074) where TSS and moisture were different (p ≥ 0.074). The results in this research were similar to those previously reported by Nordin et al. (2018), Cardona et al. (2019), and Echeverrigaray et al. (2021). In general, Plebeia spp. had a higher aw and P. jatiformis had a higher bacteria count. Furthermore, the aw of SBH was higher than that of A. mellifera (Shamsudin et al., 2019). The results obtained showed that when moisture increases or the number of soluble solids decreases, aw increases. Therefore, high levels of moisture and aw, coupled with favorable external conditions, could cause fermentation and alter the acidity, pH, bacterial load, and amount of carbohydrates and protein in the honey (Makhloufi et al., 2021).
3.2. Phenolic compounds and antioxidant activity
The total polyphenol compounds are shown in Table 1. All the results were statistically different (p < 0.001). Biluca et al. (2017) reported a higher content of polyphenols in the honey of S. bicunctata (≈500 mg of gallic acid kg−1) compared to M. bicolor, M. quadrifasciata, M. mondury and M. scutellaris, as well as in this work. However, the results of the polyphenol content of this research are lower than the polyphenol content reported by Souza et al. (2021). Nonetheless, it is normal because the polyphenol content depends on the geographical origin. It turns out that honey samples taken in highlands (mountain areas), such as those in this research, have lower total polyphenol content values because the floral variety and availability are less accessible than in the lowlands (Yayinie et al., 2022).
The antioxidant activity with ABTS, DPPH and FRAP is shown in Table 1. Although all the results of ABTS were statistically different (p ≤ 0.03), M. beecheii had the highest antioxidant activity by ABTS, followed by P. llorentei and P. jatiformis. For DPPH assay, the honey samples were not statistically different (p ≥ 0.085), except for P. llorentei (p ≤ 0.023), which was different from the others and with greater antioxidant activity. In the FRAP method, samples were similar. There was no significant difference between P. jatiformis and P. llorentei (p = 0.932), showing a high antioxidant activity, compared to M. beecheii and S. pectoralis, which also had no significant statistical difference between them (p = 0.099).
Previous reports have shown a higher ABTS activity on honey from S. bipuncata and S. mexicana compared to the results from this research (Jimenez et al., 2016; Ávila et al., 2019). Furthermore, as in this report, A. mellifera honey usually has higher ABTS activity than DPPH (Attanzio et al., 2016). Alvarez-Suarez et al. (2018) found greater antioxidant activity in M. beecheii with 440.05 ± 27.10 mg of TEACkg−1 by FRAP and similar antioxidant activity by DPPH with 105.69 ± 4.15 mg of TEAC kg−1.
3.3. Enumeration of LAB from honey and its isolation
Each colony was counted to measure the bacterial load of LAB, then it was corroborated under an optical microscope since some yeasts can grow even in the presence of CaCO3 and be confused with LAB colonies. Table 1 shows the total LAB count of the different samples.
There was no significant difference in the CFU of bacterial load between M. beecheii, S. pectoralis and P. llorentei honey samples (p > 0.05), but there was a significant difference for P. jatiformis honey (p < 0.001). It was not possible to appreciate any trend or relationship between the bacterial load and the physicochemical properties of honey since P. jatiformis honey had the highest bacterial count with 11.16 × 103 CFU mL−1, almost four times higher than P. llorentei honey, even though these were the most similar honey sharing some similar properties (Table 1). As shown in Table 2, four strains from S. pectoralis were isolated, two from P. jatiformis and one from M. beecheii. Although P. jatiformis honey had a higher bacterial load, more bacteria could be isolated from S. pectoralis honey with greater bacterial diversity since it showed more LAB species such as F. tropaeoli H-Sp-04, F. pseudoficulneus H-Sp-01 and two Fructilactobacillus spp. (H-Sp-02 and H-Sp-03). A greater bacterial diversity in S. pectoralis honey could be due to the physicochemical conditions favorable to the development of LAB. Therefore, this honey presents the highest lactonic (22.67 mEq kg−1) and total (71.91 mEq kg−1) acidity, which are related to the freshness, maturation and fermentation of honey.
Unfortunately, LAB cannot be isolated from P. llorentei since, during the replanting process, the colonies stopped growing, probably due to a lack of nutrients or by its obligate anaerobe metabolism. Torres-Moreno et al. (2021) showed that LAB could not be isolated from the stomach of P. llorentei bees, suggesting that this honey contains LAB that cannot be cultivated in MRS medium, probably because some nutrient is missing or because this LAB is used only for honey's physicochemical properties and does not adapt to different conditions. The physicochemical properties of P. llorentei honey compared with P. jatiformis honey are statistically different since P. llorentei has the least amount of total and reducing sugars, the highest moisture and aw, the lowest °Brix value, the highest content of polyphenols and antioxidant activity by DPPH. It may be that the difference between some or the combination of these properties has interfered in the null isolation of bacteria from P. llorentei honey. Obligate anaerobic bacteria could be present in SBH. Therefore, this characteristic could have prevented their cultivation in a conventional culturing attempt since they do not grow in atmospheric oxygen concentrations (Mattila et al., 2012). The need for an anaerobic environment may explain the lack of bacteria identified in P. llorentei honey.
3.4. Characterization of LAB isolated from honey samples
The interest in isolating LAB from SBH is because honey is a stressful environment for bacteria. Therefore, this food should have resistant bacteria, as demonstrated by Reale et al. (2020), who isolated LAB from sourdough, a food that shares some of the characteristics of honey. After all, it is an acid product with low moisture, high solute content, and contains fructose. Reale et al. (2020) found resistant bacteria, such as F. sanfranciscensis, that can be used in fermentation technology. In addition, it is known that honey has phenolic compounds with antioxidant activity. According to Cortés-Rodríguez et al. (2019) some LAB can grow and develop in the presence of phenolic compounds, while pathogenic bacteria cannot. Thus, there will be a biocontrol of pathogens due to resistant bacteria such as desirable, beneficial or probiotic LAB in food rich in polyphenols.
The genetic identification (Figures 4, 5) and optical microscope photos (Figures 6–8) of the strains from SBH indicated three of the isolates of Fructilactobacillus spp. could not be identified to the species level. Moreover, three Fructobacillus pseudoficulneus and one Fructobacillus tropaeoli species were identified. All the isolates were Gram-positive, catalase-negative, able to grow at 30°C, non-hemolytic and had a size around 2 μm for Fructilactobacillus spp. and 1 μm for Fructobacillus spp. (Table 2). The identified strains were added to the GenBank (https://www.ncbi.nlm.nih.gov/genbank/) database with the following access numbers: OP941514, OP941513, OP941512, OP941511, OP941515, OP941516 and OP941510 as shown in Table 2.
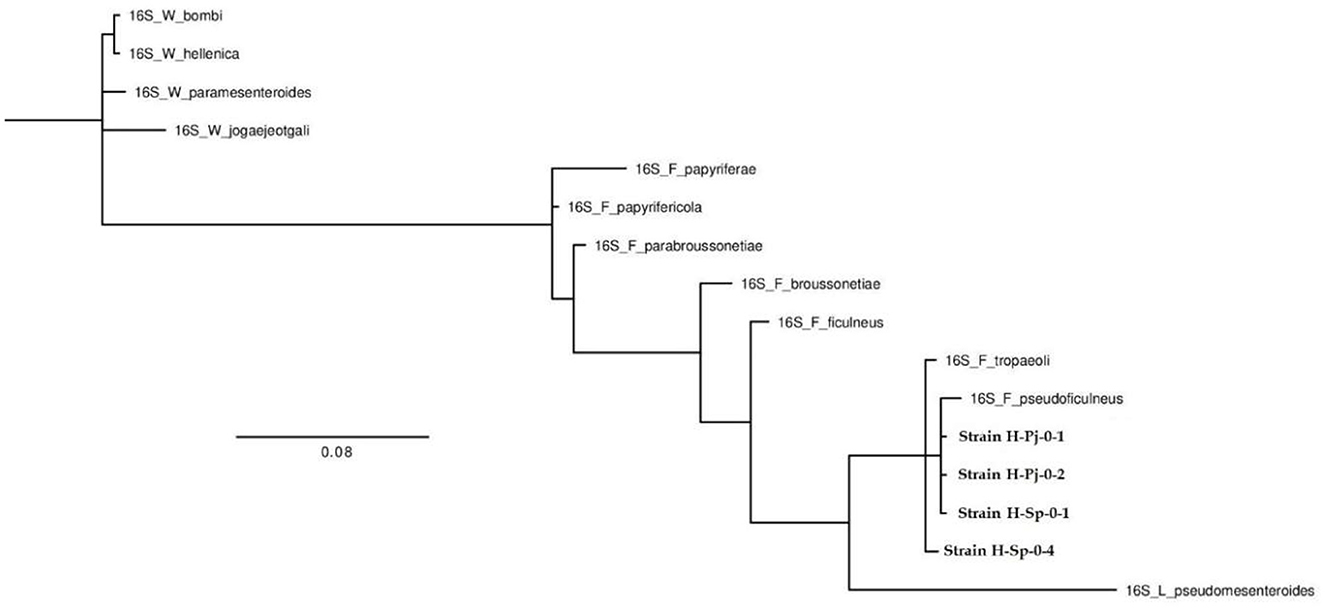
Figure 4. Phylogenetic tree of the strains found in honey belonging to the genus Fructobacillus (H-Pj-01, H-Pj-02, H-Sp-01, and H-Sp-04).
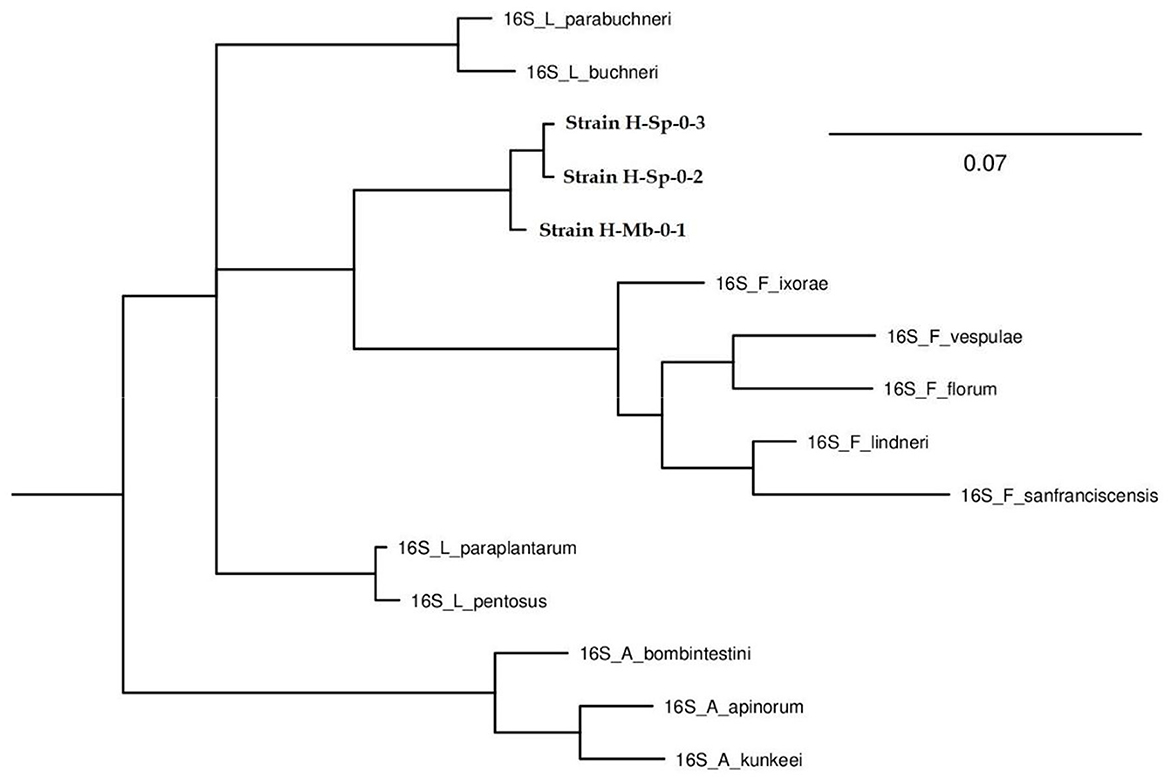
Figure 5. Phylogenetic tree of the strains found in honey belonging to the genus Fructilactobacillus (H-Sp-02, H-Sp-03, and H-Mb-01).
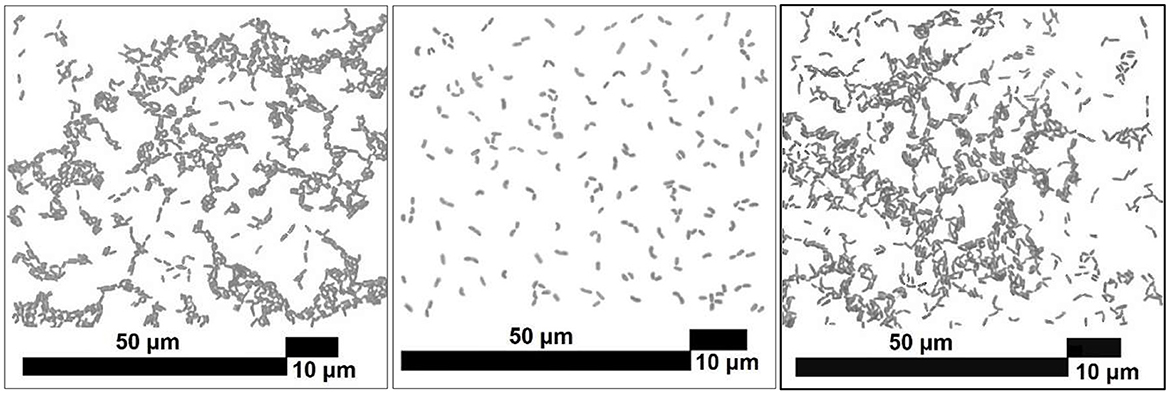
Figure 6. Optical microscope micrograph of the Fructobacillus pseudoficulneus H-Pj-0-2 (left), Fructobacillus tropaeoli H-Sp-04 (center) and Fructobacillus pseudoficulneus H-Pj-0-1 (right).
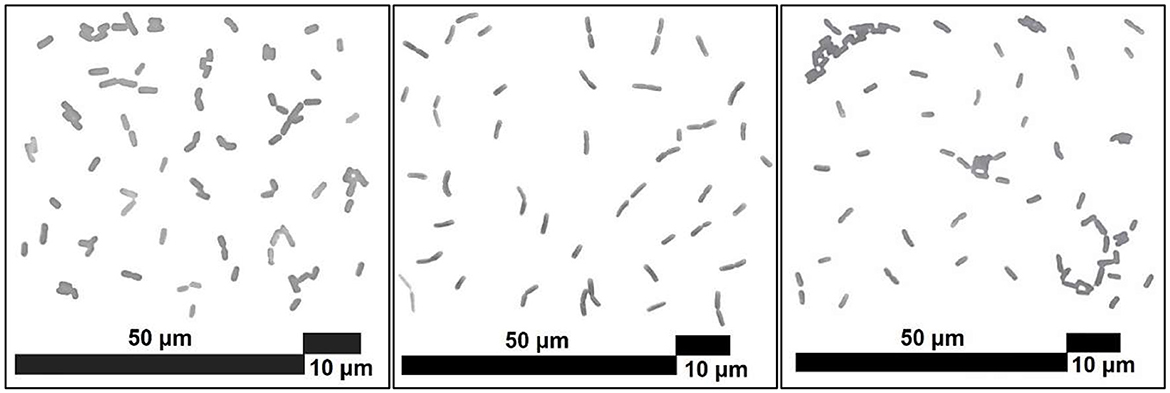
Figure 7. Optical microscope micrograph of the Fructilactobacillus spp. H-Sp-0-3 (left), Fructilactobacillus spp. H-Mb-01 (center) and Fructilactobacillus spp. H-Sp-0-2 (right).
Due to the taxonomic rearrangement, the genus Fructilactobacillus is derived from the genus Lactobacillus, and both belong to the Lactobacillaceae family (Zheng et al., 2020). On the other hand, the Fructobacillus genus forms monophyletic clades with the Oenococcus genus since they belong to the Leuconostocaceae family (Bello et al., 2022). The comparative genomic analyses found four new species of the genus Fructobacillus (F. broussonetiae, F. parabroussonetiae, F. papyriferae and F. papyrifericola). Therefore it is believed that new species of the genus Fructilactobacillus can also be found, and the fact that some bacteria could not be identified to the species level in this research was because many species have not yet been reported (Lin et al., 2022). Torres-Moreno et al. (2021) isolated LAB (three strains of Apilactobacillus spp., two of Lactiplantibacillus plantarum, three of Weissella paramesenteroides, two Leuconostoc citreum and two undetermined LAB) at the same time and place, from the same beehives of the same bee species, with the only difference that the honey sample was taken from the honey stomach of stingless bees and not from the honey of the beehive. Since no bacteria of the genus Fructobacillus or Fructilactobacillus were found in the research carried out by Torres-Moreno et al. (2021), it is believed that these LAB and FLAB proceed from the environment or the beehive and not from the gastrointestinal tract of honeybees because bacteria of these genera were found in all samples of honey from stingless bees taken from the hives.
3.4.1. Carbohydrate metabolism of LAB isolated from honey samples
F. tropaeoli H-Pj-04, F. pseudoficulneus H-Pj-0-1 and F. pseudoficulneus H-Sp-0- are FLAB (Table 3) because they grew faster with fructose (one day) than glucose (2 days). Moreover, all the Fructobacillus spp. grew in D-xylose (from 3 to 5 days) and with potassium 5-ketogluconate (from 2 to 3 days), and the bacteria of the genus Fructilactobacillus usually cannot, although they grow with squalene (in 1 day). Finally, some reports indicated that Lactobacillaceae could ferment potassium 5-ketogluconate (Buron-Moles et al., 2019). However, in general, Fructobacillus spp. are unable to ferment this carbohydrate (Endo et al., 2011; Ma'unatin et al., 2020). F. tropaeoli H-Pj-04 could ferment D-trehalose but not D-raffinose as F. pseudoficulneus H-Pj-0-1, H-Pj- 0-2 and H-Sp-01. F. pseudoficulneus H-Sp-0-1 differs from F. pseudoficulneus H-Pj-0-1 and H-Pj-0-2 in that it does not ferment mannose or D-turanose. The strain Fructilactobacillus spp. H-Sp-0-2 is the only one that can ferment erythritol (within 5 days), and it is also the only one that cannot metabolize methyl-αD-glucopyranoside. The strain Fructilactobacillus spp. H-Sp-0-3 differs from the other two strains of the same genus in that it can grow on D-maltose (within 3 days) but does not grow with D-raffinose.
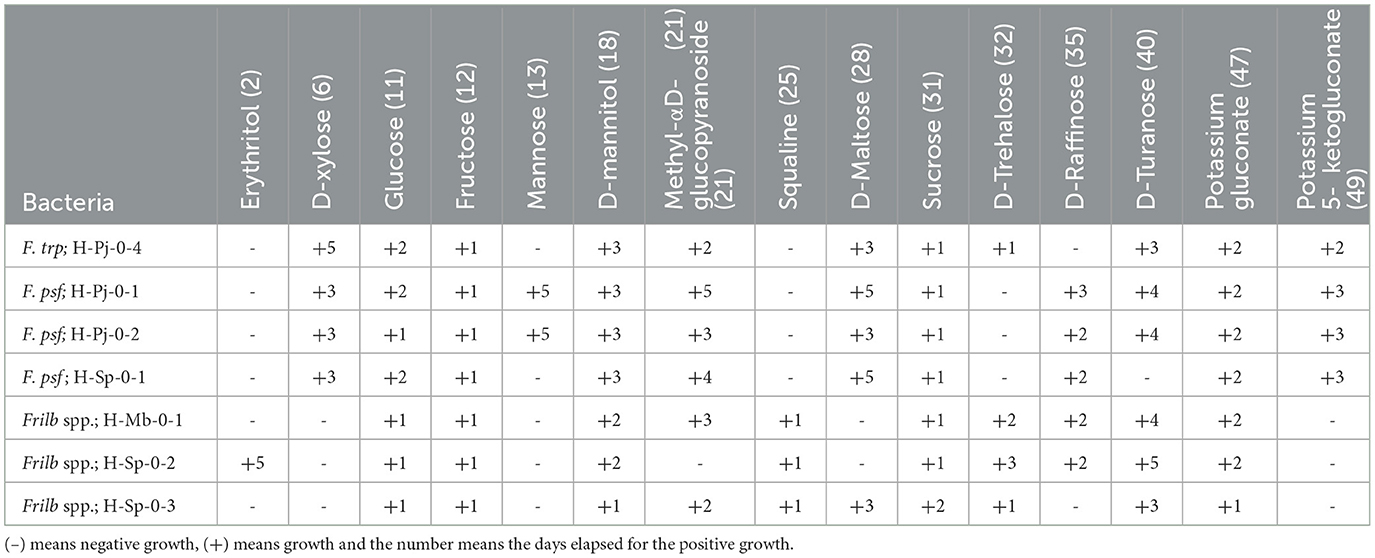
Table 3. Results of bacterial growth in anaerobiosis with different carbon sources with the API 50 CHL.
3.4.2. Haemolytic activities and susceptibility to antibiotics of LAB isolated from honey
The ability of seven LAB and FLAB to induce hemolysis in vitro was tested. Non-hemolytic activity is considered a safety requirement for biotechnological or probiotic use in the food industry; positive hemolytic activity is considered a typical virulence factor in pathogenic microorganisms (Hazwani Hasali et al., 2018). The results indicated that all bacteria did not exhibit β-hemolytic activity, meaning negative hemolysis or that the bacteria exhibited γ-hemolysis.
The antibiogram (Table 4) reveals that all strains of the genus Fructilactobacillus were sensitive to amoxicillin and piperacillin (both β-lactam antibiotics); azithromycin (macrolide antibiotic); and sulfamethoxazole-trimethoprim (sulfonamide), while all the strains of the genus Fructobacillus showed resistance to these antibiotics. Resistance to the antibiotics mentioned above that inhibit beta-lactamase, protein synthesis, and purine binding for DNA formation could be risky for Fructobacillus spp. Furthermore, it was found that F. tropaeoli and A. kunkeei isolated from bee gut were resistant to kanamycin, streptomycin, erythromycin and clindamycin, drawing attention to the health industry (Simsek et al., 2022). Furthermore, all strains of the Fructobacillus genus of this research have similar results except F. tropaeoli H-Sp-0-4 with an inhibition diameter of 2 mm for cefuroxime, while all strains of F. pseudoficulneus were inhibited by 0.5 mm and because it was the only one which was inhibited by ciprofloxacin (0.5 mm). The strain Fructilactobacillus spp. H-Sp-0-3 was more sensitive than all the others because it has larger diameters of inhibition, especially with amoxicillin (7 mm), cefixime (5 mm), cefuroxime (5 mm), sulfamethoxazole-trimethoprim (7 mm), and penicillin (3 mm).
According to Hoerr et al. (2016), the sensitivity of bacteria to cephalosporins such as cefazolin and cefuroxime (β-lactam antibiotics more stable against β-lactamases) is due to a fragile cell wall since the mechanism of action of the antibiotic is to inhibit the synthesis and binding of peptidoglycan causing cell lysis. Also, all strains were sensitive to erythromycin, the most used macrolide, since it inhibits the protein synthesis by binding to the 50S subunit of the ribosome, causing bacteriostatic activity. However, azithromycin was only effective for strains of the genus Fructilactobacillus spp. This genus was also sensitive to amoxicillin and piperacillin, which are β-lactam derivatives of penicillin that differ from cephalosporin because 7-cephalosporanic acid is replaced with 6-aminopenicillanic acid and they are less stable against β-lactamase, making Fructobacillus spp. strains more resistant to antibiotics than strains of Fructilactobacillus spp.
Most of the LAB isolates of this research were resistant to ofloxacin and ciprofloxacin (both synthetic quinolone antibiotics of second generation), and penicillin (β-lactam antibiotic). Although first and second-generation quinolones are usually more effective against some Gram-positive bacteria than third and fourth-generation quinolones, these drugs are used mostly for Gram-negative bacteria (Pham et al., 2019). Unfortunately, there is an increasing resistance to penicillin by Gram-positive bacteria, even pathogenic bacteria, because of horizontal spreading of penicillinase plasmids or by horizontal gene transfer that synthesizes penicillin-binding protein (Jubeh et al., 2020). Then, more vigilance is required for these risk factors such as prohibiting the use of antibiotics in beekeeping, although there is a trend to allow the limited presence of antibiotics in honey and honey bee colonies in Belgium, France, United Kingdom, Switzerland, United States of America, Canada, India, and Argentina (Pachla et al., 2021).
The safety of LAB concerning antibiotic resistance is due to the possible lateral transfer of antibiotic resistance genes such as tetracycline, erythromycin, and chloramphenicol; clinically relevant antibiotics to which FLAB isolates from this research were sensitive (Li et al., 2019). Nevertheless, it is always necessary to characterize the resistance to antibiotics of LAB for future biotechnological purposes or as probiotics in food to guarantee public health. Therefore, using bacteria that are sensitive to a greater number of antibiotics is recommended so that they are not infectious bacteria.
4. Conclusion
This work shows significant differences between the stingless bee species regarding the physicochemical characteristics of the freshly honey samples collected from the same place simultaneously. Since bees foraged in the same area, these differences can be attributed to the foraging preference of each bee genus and species. Since fresh honey was characterized, the results obtained can be used as quality indicators in Meliponini honey. Furthermore, the antioxidant activity depended on the stingless species because the foraged area was the same. Then, the Meliponini bees can produce honey with a different antioxidant activity.
Finally, a total of seven LAB were identified from the SBH, three of them, of the genus Fructobacillus, with fructophilic character (FLAB), three bacteria whose 16S rRNA genes did not match the previously reported species, leaving the possibility that they belong to new species of the genus Fructilactobacillus. SBH is a natural sweetener of interest for its healthy properties and beneficial microbiota. However, more studies are necessary to characterize the bacteria present in it, as well as to determine which compounds are the ones that generate its antioxidant activity.
Data availability statement
The original contributions presented in the study are publicly available. The identified strains were added to the GenBank (https://www.ncbi.nlm.nih.gv/Genbank/) database with the following access numbers OP941514, OP941513, OP941512, OP941511, OP941515, OP941516, and OP941510.
Author contributions
GM-L: conceptualization, supervision, formal analysis, writing the original draft, writing review, editing, and supervision. AA-V: formal analysis, research, and writing the original draft. HH-S: conceptualization, supervision, writing the original draft, writing review, editing, and supervision. LD-Á: conceptualization and supervision. BP-S and RT-M: research, methodology, and writing the original draft. DH-R: research, methodology, formal analysis, writing the original draft, and editing. All authors contributed to the article and approved the submitted version.
Funding
This research was supported by CONACYT (Consejo Nacional de Ciencia y Tecnologia) with project number 283954 (CB-2016-01). In addition, AA-V received a doctoral grant from CONACYT with the number 73406 and DH-R received a postdoctoral grant from CONACYT with project number I1200/224/2021.
Conflict of interest
The authors declare that the research was conducted in the absence of any commercial or financial relationships that could be construed as a potential conflict of interest.
Publisher's note
All claims expressed in this article are solely those of the authors and do not necessarily represent those of their affiliated organizations, or those of the publisher, the editors and the reviewers. Any product that may be evaluated in this article, or claim that may be made by its manufacturer, is not guaranteed or endorsed by the publisher.
References
Aboul-Maaty, N. A.-F., and Oraby, H. A.-S. (2019). Extraction of high-quality genomic DNA from different plant orders applying a modified CTAB-based method. Bull. Natl. Res. Cent. 43, 1. doi: 10.1186/s42269-019-0066-1
Agagündüz, D., Yilmaz, B., Koçak, T., Altintaş Başar, H. B., Rocha, J. M., and Özogul, F. (2022). Novel candidate microorganisms for fermentation technology: from potential benefits to safety issues. Foods. 11, 19. doi: 10.3390/foods11193074
Ahmad, S., Khan, S. A., Khan, K. A., and Li, J. (2021). Novel insight into the development and function of hypopharyngeal glands in honey bees. Front. Physiol. 11, 615830. doi: 10.3389/fphys.2020.615830
Ali, H., Alqarni, A. S., Owayss, A. A., Hassan, A. M., and Smith, B. H. (2017). Osmotic concentration in three races of honey bee, Apis mellifera L. under environmental conditions of arid zone. Saudi J. Biol. Sci. 24, 1081–1085. doi: 10.1016/j.sjbs.2016.12.006
Alvarez-Suarez, J. M., Giampieri, F., Brenciani, A., Mazzoni, L., Gasparrini, M., González-Paramás, A. M., et al. (2018). Apis mellifera vs Melipona beecheii Cuban polifloral honeys: a comparison based on their physicochemical parameters, chemical composition and biological properties. LWT. 87, 272–279. doi: 10.1016/j.lwt.2017.08.079
Anguebes, F., Pat, L., Ali, B., Guerrero, A., Córdova, A., Abatal, M., et al. (2016). Application of multivariable analysis and FTIR-ATR spectroscopy to the prediction of properties in campeche honey. J. Anal. Methods Chem. 2016, 5427526. doi: 10.1155/2016/5427526
Arencibia, D. F., Rosario, L. A., and Gámez, R. (2008). Métodos generales de conservación de microorganismos. Available onliine at: https://www.researchgate.net/publication/262724715 (accessed January 02, 2020).
Attanzio, A., Tesoriere, L., Allegra, M., and Livrea, M. A. (2016). Monofloral honeys by Sicilian black honeybee (Apis mellifera ssp. sicula) have high reducing power and antioxidant capacity. Heliyon. 2, 11. doi: 10.1016/j.heliyon.2016.e00193
Ávila, S., Beux, M. R., Ribani, R. H., and Zambiazi, R. C. (2018). Stingless bee honey: quality parameters, bioactive compounds, health-promotion properties and modification detection strategies. Trends Food Sci. Tec. 81, 37–50. doi: 10.1016/j.tifs.2018.09.002
Ávila, S., Lazzarotto, M., Hornung, P. S., Teixeira, G. L., Ito, V. C., Bellettini, M. B., et al. (2019). Influence of stingless bee genus (Scaptotrigona and Melipona) on the mineral content, physicochemical and microbiological properties of honey. J. Food Sci. Technol. 56, 4742–4748. doi: 10.1007/s13197-019-03939-8
Aween, M. M., Hassan, Z., Muhialdin, B. J., Eljamel, Y. A., Al-Mabrok, A. S. W., and Lani, M. N. (2012). Antibacterial activity of lactobacillus acidophilus strains isolated from honey marketed in Malaysia against selected multiple antibiotic resistant (MAR) gram-positive bacteria. J. Food Sci. 77, 7. doi: 10.1111/j.1750-3841.2012.02776.x
Badrulhisham, N. S. R., Ab Hamid, S. N. P., Ismail, M. A. H., Yong, Y. K., Zakuan, N. M., Harith, H. H., et al. (2020). Harvested locations influence the total phenolic content, antioxidant levels, cytotoxic, and anti-inflammatory activities of stingless bee honey. J. Asia-Pac. Entomol. 23, 950–956. doi: 10.1016/j.aspen.2020.07.015
Balzan, S., Carraro, L., Merlanti, R., Lucatello, L., Capolongo, F., Fontana, F., et al. (2020). Microbial metabarcoding highlights different bacterial and fungal populations in honey samples from local beekeepers and market in north-eastern Italy. Int. J. Food Microbiol. 334, 108806. doi: 10.1016/j.ijfoodmicro.2020.108806
Belina-Aldemita, M. D., Schreiner, M., and D'Amico, S. (2020). Characterization of phenolic compounds and antioxidative potential of pot-pollen produced by stingless bees (Tetragonula biroi Friese) from the Philippines. J. Food Biochem. 44, e13102. doi: 10.1111/jfbc.13102
Bello, S., Rudra, B., and Gupta, R. S. (2022). Phylogenomic and comparative genomic analyses of Leuconostocaceae species: identification of molecular signatures specific for the genera Leuconostoc, Fructobacillus and Oenococcus and proposal for a novel genus Periweissella gen. nov. Int. J. Syst. Evol. Microbiol. 72, 3. doi: 10.1099/ijsem.0.005284
Biluca, F. C., de Gois, J. S., Schulz, M., Braghini, F., Gonzaga, L. V., Maltez, H. F., et al. (2017). Phenolic compounds, antioxidant capacity and bioaccessibility of minerals of stingless bee honey (Meliponinae). J. Food Composit. Anal. 63, 89–97. doi: 10.1016/J.JFCA.2017.07.039
Bogdanov, S., Martin, P., and Lullmann, C. (2002). Harmonised Methods of the International Honey Commission. Liebefeld: Swiss bee research Centre. p. 1–62.
Braghini, F., Biluca, F. C., Schulz, M., Gonzaga, L., Costa, A. C. O., and Fett, R. (2021). Stingless bee honey: a precious but unregulated product - reality and expectations. Food Rev. Int. 38, 683–712. doi: 10.1080/87559129.2021.1884875
Buron-Moles, G., Chailyan, A., Dolejs, I., Forster, J., and Mikš, M. H. (2019). Uncovering carbohydrate metabolism through a genotype-phenotype association study of 56 lactic acid bacteria genomes. Appl. Microbiol. Biotechnol. 103, 3135–3152. doi: 10.1007/s00253-019-09701-6
Camilli, M. P., de Barros, D. C. B., Justulin, L. A., Tse, M. L. P., Orsi, R., and de, O. (2020). Protein feed stimulates the development of mandibular glands of honey bees (Apis mellifera). Journal of Apicultural Research. 60, 165–171. doi: 10.1080/00218839.2020.1778922
Cardona, Y., Torres, A., and Hoffmann, W. (2019). Colombian stingless bee honeys characterized by multivariate analysis of physicochemical properties. Apidologie. 50, 881–892. doi: 10.1007/s13592-019-00698-5
Carina Audisio, M., Torres, M. J., Sabaté, D. C., Ibarguren, C., and Apella, M. C. (2011). Properties of different lactic acid bacteria isolated from Apis mellifera L. bee-gut. Microbiol. Res. 166, 1–13. doi: 10.1016/j.micres.2010.01.003
Chen, C. T., Chen, B. Y., Nai, Y. S., Chang, Y. M., Chen, K. H., and Chen, Y. W. (2019). Novel inspection of sugar residue and origin in honey based on the 13C/12C isotopic ratio and protein content. J. Food Drug Anal. 27, 175–183. doi: 10.1016/j.jfda.2018.08.004
Cortés-Rodríguez, V., Dorantes-Alvarez, L., Hernández-Sánchez, H., Paniagua-Castro, N., Aparicio-Ozores, G., López-Villegas, E. O., et al. (2019). Effect of sodium cinnamate, coumarate, caffeate and ferulate mixtures on the viability, morphometry and ultrastructure of lactic-acid bacteria and Listeria monocytogenes. LWT. 112, 108240. doi: 10.1016/j.lwt.2019.06.007
de la Roca, P. (2018). Las abejas mesoamericanas y la miel en la historia, cosmovisión y medicina de los pueblos mayas. Estudios Interétnicos. 29, 139−160. Retrieved from: https://ideipi.usac.edu.gt/wp-content/uploads/2021/07/29-Revista-IDEI.pdf (accessed January 02, 2020).
di Cagno, R., Filannino, P., Cantatore, V., and Gobbetti, M. (2019). Novel solid-state fermentation of bee-collected pollen emulating the natural fermentation process of bee bread. Food Microbiol. 82, 218–230. doi: 10.1016/j.fm.2019.02.007
Duarte, A. W. F., dos Santos Vasconcelos, M. R., de Menezes, A. P. D., da Silva, S. C., Oda-Souza, M., and López, A. M. Q. (2012). Composition and antioxidant activity of honey from Africanized and stingless bees in Alagoas (Brazil): a multivariate analysis. J. Apic. Res. 51, 23–35. doi: 10.3896/IBRA.1.51.1.04
Duarte, A. W. F., Vasconcelos, M. R. D. S., Oda-Souza, M., de Olivera, F. F., and López, A. M. Q. (2018). Honey and bee pollen produced by meliponini (Apidae) in alagoas, brazil: Multivariate analysis of physicochemical and antioxidant profiles. Food Sci. Technol. (Brazil). 38, 493–503. doi: 10.1590/fst.09317
Echeverrigaray, S., Scariot, F. J., Foresti, L., Schwarz, L. V., Rocha, R. K. M., da Silva, G. P., et al. (2021). Yeast biodiversity in honey produced by stingless bees raised in the highlands of southern Brazil. Int. J. Food Microbiol. 347, 109200. doi: 10.1016/j.ijfoodmicro.2021.109200
Endo, A., Irisawa, T., Futagawa-Endo, Y., Sonomoto, K., Itoh, K., Takano, K., et al. (2011). Fructobacillus tropaeoli sp. nov., a fructophilic lactic acid bacterium isolated from a flower. Int. J. Syst. Evol. Microbiol. 61, 898–902. doi: 10.1099/ijs.0.023838-0
Endo, A., Maeno, S., Tanizawa, Y., Kneifel, W., Arita, M., Dicks, L., et al. (2018). Fructophilic lactic acid bacteria, a unique group of fructose-fermenting microbes. Appl. Environ. Microbiol. 84, e01290–18. doi: 10.1128/AEM.01290-18
Filannino, P., di Cagno, R., Tlais, A. Z. A., Cantatore, V., and Gobbetti, M. (2019). Fructose-rich niches traced the evolution of lactic acid bacteria toward fructophilic species. Crit. Rev. Microbiol. 45, 65–81. doi: 10.1080/1040841X.2018.1543649
Geisa, M. G., Ciappini, M. C., and Hilgert, N. I. (2021). Sensory attributes of native stingless bee honey (Plebeia molesta Puls, 1869): first approaches to the characterization and preference of local consumers. Ethnobiol. Conserv. 10. doi: 10.15451/ec2021-06-10.27-1-15
Hazwani Hasali, N., Izzwan Zamri, A., Nizam Lani, M., and Mubarak, A. (2018). Antibiotic susceptibility, antibacterial activity and probiotic characterisation of isolated Lactobacillus brevis strains from Heterotrigona itama honey. Malays. Appl. Biol. 47, 105–112. doi: 10.3892/etm.2016.3545
Hoerr, V., Duggan, G. E., Zbytnuik, L., Poon, K. K. H., Große, C., Neugebauer, U., et al. (2016). Characterization and prediction of the mechanism of action of antibiotics through NMR metabolomics. BMC Microbiol. 16, 1. doi: 10.1186/s12866-016-0696-5
Jimenez, M., Beristain, C. I., Azuara, E., Mendoza, M. R., and Pascual, L. A. (2016). Physicochemical and antioxidant properties of honey from Scaptotrigona mexicana bee. J. Apic. Res. 55, 151–160. doi: 10.1080/00218839.2016.1205294
Jubeh, B., Breijyeh, Z., and Karaman, R. (2020). Resistance of gram-positive bacteria to current antibacterial agents and overcoming approaches. Molecules. 25, 12. doi: 10.3390/molecules25122888
Khan, S. U., Anjum, S. I., Rahman, K., Ansari, M. J., Khan, W. U., Kamal, S., et al. (2018). Honey: Single food stuff comprises many drugs. Saudi J. Biol. Sci. 25, 320. doi: 10.1016/j.sjbs.2017.08.004
Lage, L. G. A., Coelho, L. L., Resende, H. C., Tavares, M. G., Campos, L. A. O., and Fernandes-Salomão, T. M. (2012). Honey physicochemical properties of three species of the brazilian Melipona. An Acad Bras Cienc. 84, 3. doi: 10.1590/S0001-37652012005000051
Lee, J., Durst, R., and Wrolstad, R. (2005). “AOAC official method 2005.02: total monomeric anthocyanin pigment content of fruit juices, beverages, natural colorants, and wines by the pH differential method,” in Official Methods of Analysis of AOAC International, 18th Edn, ed H. Horowitz (Washington, DC: AOAC).
Leonhardt, S. D. (2017). Chemical Ecology of Stingless Bees. J. Chem. Ecol. 43, 385–402. doi: 10.1007/s10886-017-0837-9
Li, Y., Li, L., Kromann, S., Chen, M., Shi, L., and Meng, H. (2019). Antibiotic resistance of Lactobacillus spp. and Streptococcus thermophilus isolated from chinese fermented milk products. Foodborne Pathogens Dis. 16, 221–228. doi: 10.1089/fpd.2018.2516
Lin, S. T., Guu, J. R., Wang, H. M., Tamura, T., Mori, K., Huang, L., et al. (2022). Fructobacillus papyriferae sp. nov., Fructobacillus papyrifericola sp. nov., Fructobacillus broussonetiae sp. nov. and Fructobacillus parabroussonetiae sp. nov., isolated from paper mulberry in Taiwan. Int. J. Syst. Evolutionary Microbiol. 72, 2. doi: 10.1099/ijsem.0.005235
Mahmood, Q. H., and Abbas, K. M. (2020). Determining the ratio of carbohydrates and some types of reducing and non-reducing sugars in honey feed honey bees on citrus flowers (citrus singensis) and christ thorn jujube's flowers (Ziziphus Spina _Christi). Eur. J. Mol. Clinical Med. 7, 11. Retrieved from: https://www.ejmcm.com/article_5054.html (accessed January 02, 2020).
Makhloufi, C., Ait Abderrahim, L., and Taibi, K. (2021). Characterization of some algerian honeys belonging to different botanical origins based on their physicochemical properties. Iran. J. Sci. Technol. Trans. A Sci. 45, 189–199. doi: 10.1007/s40995-020-01047-3
Mărgăoan, R., Cornea-Cipcigan, M., Topal, E., and Kösoglu, M. (2020). Impact of fermentation processes on the bioactive profile and health-promoting properties of bee bread, mead and honey vinegar. Processes. 8, 9. doi: 10.3390/pr8091081
Mathialagan, M., Thangaraj Edward, Y. S. J., David, P. M. M., Senthilkumar, M., Srinivasan, M. R., and Mohankumar, S. (2018). Isolation, characterization and identification of probiotic lactic acid bacteria (LAB) from honey bees. Int. J. Curr. Microbiol. Appl Sci. 7, 894–906. doi: 10.20546/ijcmas.2018.704.096
Mattila, H. R., Rios, D., Walker-Sperling, V. E., Roeselers, G., and Newton, I. L. G. (2012). Characterization of the active microbiotas associated with honey bees reveals healthier and broader communities when colonies are genetically diverse. PLoS ONE. 7, 3. doi: 10.1371/journal.pone.0032962
Ma'unatin, A., Harijono, H., Zubaidah, E., and Rifa, M. (2020). The isolation of exopolysaccharide-producing lactic acid bacteria from lontar (Borassus flabellifer L.) sap. Iran. J. Microbiol. 12, 5. doi: 10.18502/ijm.v12i5.4605
Mohammad, S. M., Mahmud-Ab-Rashid, N. K., and Zawawi, N. (2020). Probiotic properties of bacteria isolated from bee bread of stingless bee Heterotrigona itama. J. Apic. Res. 2020, 284575. doi: 10.1155/2020/2845757
Mohammed, M. E. A. (2020). Factors affecting the physicochemical properties and chemical composition of bee's honey. Food Rev. Int. 38, 1330–1341. doi: 10.1080/87559129.2020.1810701
Naghili, H., Tajik, H., Mardani, K., Mehdi, S., Rouhani, R., Ehsani, A., et al. (2013). Validation of drop plate technique for bacterial enumeration by parametric and nonparametric tests. Vet. Res Forum. 4, 179-83.
Naree, S., Ellis, J. D., Benbow, M. E., and Suwannapong, G. (2021). The use of propolis for preventing and treating Nosema ceranae infection in western honey bee (Apis mellifera Linnaeus, 1787) workers. J. Apic. Res. 60, 686–696. doi: 10.1080/00218839.2021.1905374
Nascimento, A., Marchini, L., Carvalho, C., Araújo, D., Olinda, R., and Silveira, T. (2015). Physical-chemical parameters of honey of stingless bee (Hymenoptera: Apidae). Am. Chem. Sci. J. 7, 139–149. doi: 10.9734/ACSJ/2015/17547
Nordin, A., Sainik, N. Q. A. V., Chowdhury, S. R., Saim, A., and bin, Idrus, R. B. H. (2018). Physicochemical properties of stingless bee honey from around the globe: a comprehensive review. J. Food Composit. Anal. 73, 91–102. doi: 10.1016/j.jfca.2018.06.002
Okonechnikov, K., Golosova, O., Fursov, M., Varlamov, A., Vaskin, Y., Efremov, I., et al. (2012). Unipro UGENE: a unified bioinformatics toolkit. Bioinformatics. 28, 8. 1166–1167. doi: 10.1093/bioinformatics/bts091
Pachla, A., Ptaszyńska, A. A., Wicha, M., Kunat, M., Wydrych, J., Oleńska, E., et al. (2021). Insight into probiotic properties of lactic acid bacterial endosymbionts of Apis mellifera L. derived from the Polish apiary. Saudi J. Biol. Sci. 28, 1890–1899. doi: 10.1016/j.sjbs.2020.12.040
Pham, T. D. M., Ziora, Z. M., and Blaskovich, M. A. T. (2019). Quinolone antibiotics. Med. Chem. Comm. 10, 1719–1739. doi: 10.1039/C9MD00120D
Pimentel, T. C., Rosset, M., de Sousa, J. M. B., de Oliveira, L. I. G., Mafaldo, I. M., Pintado, M. M. E., et al. (2022). Stingless bee honey: an overview of health benefits and main market challenges. J. Food Biochem. 46, e13883. doi: 10.1111/jfbc.13883
Posada, D. (2008). ModelTest: Phylogenetic model averaging. Mol. Biol. Evol. 25, 1253–1256. doi: 10.1093/molbev/msn083
Quezada-Euán, J. J. G. (2018). “Taxonomy and diversity of the stingless bees,” in Stingless Bees of Mexico (Mexico City). p. 1–40. doi: 10.1007/978-3-319-77785-6_1
Reale, A., Zotta, T., Ianniello, R. G., Mamone, G., and di Renzo, T. (2020). Selection criteria of lactic acid bacteria to be used as starter for sweet and salty leavened baked products. LWT. 133, 110092. doi: 10.1016/j.lwt.2020.110092
Ronquist, F., Teslenko, M., van der Mark, P., Ayres, D. L., Darling, A., Höhna, S., et al. (2012). Mrbayes 3.2: efficient bayesian phylogenetic inference and model choice across a large model space. Syst. Biol. 61, 539–542. doi: 10.1093/sysbio/sys029
Rozman, A. S., Hashim, N., Maringgal, B., and Abdan, K. (2022). A comprehensive review of stingless bee products: phytochemical composition and beneficial properties of honey, propolis, and pollen. Appl. Sci. 12, 6370. doi: 10.3390/app12136370
Sabella, J. K., Gladys, M., Bartholomew, N. O., Charles, M., and Sammy, K. (2022). Therapeutic uses of stingless bee honey by traditional medicine practitioners in Baringo County, Kenya. J. Pharmacognosy Phytother. 14, 27–36. doi: 10.5897/JPP2022.0618
Sánchez Cano, J. E. (2019). Desarrollo sostenible de zonas áridas y semiáridas frente al cambio climático. Available online at: www.colef.mx
Santiesteban-Hernández, A., Cuadrillero, J. A., Loper, G., and Honduras, C. (2003). “Comparación de parámetros físico-químicos de mieles de abejas sin aguijón y Apis mellifera de la región del Sonocusc, Chiapas, México,” in III Seminario Mesoamericano Sobre Abejas Sin Aguijón. (Tapachula), p. 60–61.
Scholz, M. B., dos, S., Quinhone Júnior, A., Delamuta, B. H., Nakamura, J. M., Baudraz, M. C., et al. (2020). Indication of the geographical origin of honey using its physicochemical characteristics and multivariate analysis. J. Food Sci. Technol. 57, 1896–1903. doi: 10.1007/s13197-019-04225-3
Shamsudin, S., Selamat, J., Sanny, M., Abd. Razak, S. B., Jambari, N. N., Mian, Z., et al. (2019). Influence of origins and bee species on physicochemical, antioxidant properties and botanical discrimination of stingless bee honey. Int. J. Food Properties. 22, 238–263. doi: 10.1080/10942912.2019.1576730
Simsek, D., Kiymaci, M. E., Tok, K. C., Gumustas, M., and Altanlar, N. (2022). Investigation of the probiotic and metabolic potential of Fructobacillus tropaeoli and Apilactobacillus kunkeei from apiaries. Arch. Microbiol. 204, 432–445. doi: 10.1007/s00203-022-03000-x
Smetanska, I., Alharthi, S. S., and Selim, K. A. (2021). Physicochemical, antioxidant capacity and color analysis of six honeys from different origin. J. King Saud Univ. Sci. 33, 101447. doi: 10.1016/j.jksus.2021.101447
Souza, E. C. A., Menezes, C., and Flach, A. (2021). Stingless bee honey (Hymenoptera, Apidae, Meliponini): a review of quality control, chemical profile, and biological potential. Apidologie. 52, 113–132. doi: 10.1007/s13592-020-00802-0
Susilowati, F., and Azkia, M. N. (2022). “Prebiotic potential of oligosaccharides: In vitro study of indonesian local honey from Apis spp. and Trigona spp. bees,” in 6th International Conference of Food, Agriculture, and Natural Resource (Atlantis Press), 190–198. doi: 10.2991/absr.k.220101.025
Syed Yaacob, S. N., Huyop, F., Kamarulzaman Raja Ibrahim, R., and Wahab, R. A. (2018). Identification of Lactobacillus spp. and Fructobacillus spp. isolated from fresh Heterotrigona itama honey and their antagonistic activities against clinical pathogenic bacteria. J. Apic. Res. 57, 395–405. doi: 10.1080/00218839.2018.1428047
Tan, S. H., Pui, L. P., Solihin, M. I., Keat, K. S., Lim, W. H., and Ang, C. K. (2021). Physicochemical analysis and adulteration detection in Malaysia stingless bee honey using a handheld near-infrared spectrometer. J. Food Proc. Preservat. 45, 7. doi: 10.1111/jfpp.15576
Torres-Moreno, R., Hemández-Sánchez Humberto, S., Méndez-Tenorio, A., Palmeros-Sánchez, B., and Melgar-Lalanne, G. (2021). Characterization and identification of lactic acid bacteria from Mexican stingless bees (Apidae: Meliponini). IOP Conference Series: Earth and Environm. Sci. 858, 012010. doi: 10.1088/1755-1315/858/1/012010
Trinh, N. T. N., Tuan, N. N., Thang, T. D., Kuo, P. C., Thanh, N. B., Tam, L. N., et al. (2022). Chemical composition analysis and antioxidant activity of coffea robusta monofloral honeys from Vietnam. Foods. 11(3). doi: 10.3390/foods11030388
Vásquez, A., Forsgren, E., Fries, I., Paxton, R. J., Flaberg, E., Szekely, L., et al. (2012). Symbionts as major modulators of insect health: lactic acid bacteria and honeybees. PLoS ONE. 7, 3. doi: 10.1371/annotation/3ac2b867-c013-4504-9e06-bebf3fa039d1
Villacrés-Granda, I., Coello, D., Proaño, A., Ballesteros, I., Roubik, D. W., Jijón, G., et al. (2021). Honey quality parameters, chemical composition and antimicrobial activity in twelve Ecuadorian stingless bees (Apidae: Apinae: Meliponini) tested against multiresistant human pathogens. LWT. 140, 10737. doi: 10.1016/j.lwt.2020.110737
Yayinie, M., Atlabachew, M., Tesfaye, A., Hilluf, W., Reta, C., and Alemneh, T. (2022). Polyphenols, flavonoids, and antioxidant content of honey coupled with chemometric method: geographical origin classification from Amhara region, Ethiopia. Int. J. Food Propert. 25, 76–92. doi: 10.1080/10942912.2021.2021940
Zheng, J., Wittouck, S., Salvetti, E., Franz, C. M. A. P., Harris, H. M. B., Mattarelli, P., et al. (2020). A taxonomic note on the genus Lactobacillus: Description of 23 novel genera, emended description of the genus Lactobacillus beijerinck 1901, and union of Lactobacillaceae and Leuconostocaceae. Int. J. Syst. Evol. Microbiol. 70, 2782–2858. doi: 10.1099/ijsem.0.004107
Keywords: honey characterization, fructophilic lactic acid bacteria, stingless bees, antioxidant properties, 16S ribosomal RNA, stingless honey
Citation: Andrade-Velásquez A, Hernández Sánchez H, Dorantes-Álvarez L, Palmeros-Sánchez B, Torres-Moreno R, Hernández-Rodríguez D and Melgar-Lalanne G (2023) Honey characterization and identification of fructophilic lactic acid bacteria of fresh samples from Melipona beecheii, Scaptotrigona pectoralis, Plebeia llorentei, and Plebeia jatiformis hives. Front. Sustain. Food Syst. 7:1113920. doi: 10.3389/fsufs.2023.1113920
Received: 01 December 2022; Accepted: 17 February 2023;
Published: 09 March 2023.
Edited by:
Guadalupe Virginia Nevárez-Moorillón, Autonomous University of Chihuahua, MexicoReviewed by:
Suzy Munir Salama, University of Malaya, MalaysiaLilia Arely Prado Barragan, Metropolitan Autonomous University, Mexico
Copyright © 2023 Andrade-Velásquez, Hernández Sánchez, Dorantes-Álvarez, Palmeros-Sánchez, Torres-Moreno, Hernández-Rodríguez and Melgar-Lalanne. This is an open-access article distributed under the terms of the Creative Commons Attribution License (CC BY). The use, distribution or reproduction in other forums is permitted, provided the original author(s) and the copyright owner(s) are credited and that the original publication in this journal is cited, in accordance with accepted academic practice. No use, distribution or reproduction is permitted which does not comply with these terms.
*Correspondence: Guiomar Melgar-Lalanne, Z21lbGdhckB1di5teA==