Emerging monitoring technologies to reduce illegal fishing activities at sea and prevent entry of fraudulent fish into markets
- 1Biology Department, Loyola Marymount University, Los Angeles, CA, United States
- 2The Food and Agriculture Organization of the United Nations, Rome, Italy
- 3Department of Ecology and Evolutionary Biology, University of California, Los Angeles, Los Angeles, CA, United States
- 4Conservation X Labs, Washington, D.C, United States
- 5Sustainable Management of Fisheries Division, Collecte Localisation Satellites, Ramonville Saint-Agne, France
- 6UMR DECOD, IFREMER, Nantes, France
National and global priorities are increasingly focused on the concurrent marine fisheries challenges of food security, illegal fishing, and declining fisheries resources. Molecular genetics and electronic monitoring technologies can advance solutions to these challenges, particularly in fisheries surveillance and seafood traceability, and a growing number of studies continues to validate the utility of these tools. What is needed next is guidance to support their wider, more conventional adoption and implementation, either complementary to or in the absence of government policies. Here, we synthesize discussion held during the Borchard Foundation Colloquium held in July 2022 in Missillac, France on modernizing global fisheries with emerging technologies. Our aim is to provide perspectives to scientists, resource managers, and policy makers of emerging monitoring technologies, summarize the utility of these technologies in fisheries, and conclude with how the objective to modernize global marine fisheries is a prime opportunity to engage fresh talent in a new era of fisheries innovation.
1. Introduction
In July 2022, a colloquium on modernizing global fisheries with emerging technologies, funded by the Albert and Elaine Borchard Foundation Center on International Education, was held in Missillac, France. Participants of this 3-day colloquium included all co-authors of this manuscript, as well as Dr. Samantha Cheng of the World Wildlife Fund. The aim of the colloquium was to discuss how emerging monitoring technologies could reduce illegal fishing activities at sea and prevent entry of fraudulent fish products into the global marketplace. As presented below, discussions focused on the harms and costs of current marine fisheries challenges, the emergence of traceability technologies for deterring illegal fishing and fraudulent fish trade, how these tools may and are being implemented, and the need for drawing new talent for and in support of tomorrow’s fisheries workforce.
1.1. The harms of marine fisheries challenges, and who bears the cost of the status quo
Since 1960, global consumption of fisheries products has doubled to 20.2 kg per capita (FAO, 2022). While aquaculture now accounts for a growing share of annual global fish production (57.8%; FAO, 2022). steady pressure on capture fisheries (Boyd et al., 2022) has led to a collapse of multiple marine fisheries (Jackson et al., 2001; Hutchings et al., 2010) and severe population declines for 89% of large marine animals relative to historical averages (Lotze and Worm, 2009). This loss threatens healthy functioning of marine ecosystems – including altered food webs (Estes et al., 2011) and carbon cycling (Doughty et al., 2016), and phase shifts (Hughes et al., 2007), with impacts that can cascade throughout marine ecosystems (Meyers et al., 2007; Estes et al., 2011). Such losses also threaten current and future provision of key ecosystem services, including sustainable fisheries (Hammerschlag et al., 2019), particularly under climate change scenarios (Free et al., 2019; Thiault et al., 2019; Cheung et al., 2022).
Fisheries declines pose a major threat to global food security from local to global scales. Aquatic products dwarf all other sources of animal protein (Béné et al., 2015) providing 4.3 billion people with at least 15% of their protein intake worldwide [HLPE, 2014]. Fisheries also support the livelihoods of approximately 600 million people, with a direct contribution of over $400 billion USD to the global economy (FAO, 2022). While fisheries declines have global economic and nutrition impacts, the negative consequences of these declines most heavily impact coastal and developing nations. For example, daily protein intake from fish can exceed 60% in parts of Africa and Southeast Asia (FAO, 2014), where small-scale fisheries play a critical role in meeting nutritional needs, particularly in developing countries (Kawarazuka and Béné, 2010). Selig et al. (2019) demonstrates that countries of West Africa, Southeast Asia and Oceana have a greater nutritional and economic dependence on marine fisheries than North America and Europe, with countries such Indonesia, the Philippines, Nigeria, Malaysia, and Ghana suffering the greatest impacts of fisheries declines. These differential impacts are not only an equity and humanitarian issue, but they are also a political stability issue, with famine playing a common role historically in precipitating unrest and civil war (Devereux, 2000), including contemporary conflicts in Somalia (Ahmed, 2018) and Syria (Ash and Obradovich, 2020), among others.
1.2. Back-casting to reach sustainable fisheries and food security
In the past decade, there has been a significant rise in attention on the potential for technological solutions to ‘solve’ challenges for sustainable and equitable fisheries management and supply chains. These solutions are promising and have already demonstrated potential for impact at multiple scales. However, to be most effective, they need to be deployed thoughtfully and integrated within the broader suite of interventions that aim to address social, economic, and ecological dimensions of sustainability. In this regard, we convened a multi-sector group comprised of practitioners, policymakers, and researchers working on fisheries traceability and sustainable fisheries management and policy across local and global scales – in a ‘back-casting’ exercise to identify key pathways to impact and where traceability technologies are most well-suited for effective impact across scales. The group identified a preferred future state, then investigated the scenarios and pathways most likely to produce that future state. We posited a future in which fisheries are sustainably managed in perpetuity from local to global levels, specifically by the vast reduction of illegal, unreported, and unregulated (IUU) fishing and seafood fraud. This paper focuses on identifying where technologies can complement existing policies and processes for monitoring, control and surveillance (MCS) of fisheries at the global and national levels. As mentioned previously, those who bear the brunt of the cost of unsustainable fishing and the impacts of global threats on fisheries– are often lower and middle-income economies and small-scale fisheries. It is important to acknowledge the use of traceability technologies is likely to exacerbate or create inequities and negative consequences for small-scale fisheries and these risks should be considered in the widescale deployment of these practices.
1.3. Pathway to outcomes for sustainable fisheries
The dialogue on global sustainability has focused on behavior change and adoption of sustainable practices. In fisheries, this requires coordination and coherence across a wide range of interventions and sectors. For example, supporting small-scale fisheries requires taking a social-ecological approach to encompass the range of complexities and interactions between local stakeholders and economics, ecosystems, and wider economic, political, and social drivers (Lindkvist et al., 2022). In these complex systems, programs and projects from the development, conservation, natural resource management, public health, and impact investing sectors have aimed to address different parts of fisheries supply and value chains including gear types and harvest practices, improved ecosystem and population data and modeling, market access instruments supporting ecological, social and cultural dimensions, and building demand for sustainable products (Gutierrez and Morgan, 2015; Howson, 2020). However, there are still many gaps in understanding how these interventions result in improvements to fish populations, ecosystem health, and human well-being (Eales et al., 2021). In particular, better understanding of how to upscale the adoption of sustainable practices is needed. While many efforts have focused on trainings for fishers at all scales, changing fishing practices does not only require increased capability – but sufficient opportunities, motivations, and capacities to utilize and leverage these new sustainable practices (Michie et al., 2011; Perros et al., 2022), particularly monitoring and traceability technologies.
2. Emerging traceability technologies for deterring illegal fishing and fraudulent fish trade
2.1. Role of traceability technologies
Traceability alone does not deliver improved fish stocks, healthier ecosystems, nor sustained benefits for people. It does improve our ability to understand whether sustainable practices are adopted, identify fraudulent activities, and aid enforcement rules aimed to support greater transparency of the supply chains. By improving cost effectiveness and capacity development to utilize the below described tools, we create the opportunity to lower the startup cost and barriers to adoption and implementation and facilitate pathways to sustainable fisheries and livelihoods.
2.2. Molecular genetic tools
The advent of affordable and more accessible molecular genetics tools and technologies (Willette et al., 2014) has opened opportunities for modernizing marine fisheries surveillance. Traditional DNA barcoding is one such tool that has both been rigorously vetted and widely adopted late in seafood supply chain to detect seafood substitution, including in market and restaurant-focused studies (Naaum et al., 2016; Luque and Josh Donlan, 2019; Willette et al., 2021a). Yet, traditional DNA barcoding methods can be limiting; requiring direct, individual sampling of fish tissues which is not practical early in the supply chain where thousands of fish may be transported and processed at once. The emergence of environmental DNA (eDNA) methods helps circumvent the need for direct sampling of fish tissue by instead targeting the remnant DNA and metabolic waste left behind in their environment, which can then be used to identify multiple present species simultaneously (Deiner et al., 2017). For example, Willette et al. (2021b) demonstrated the potential of eDNA metabarcoding for in situ profiling of fish species composition from the meltwater in the fish holds of industrial and artisanal fishing vessels, revealing more diverse taxa than generally reported by the vessel’s crew. Such applications would not only complement existing surveillance tools but could have a transformative effect in achieving unambiguous species identification and fish supply chain traceability.
New applications of the use of eDNA for addressing a wide range of scientific and management questions emerge every day. Its potential use for supporting fisheries management by supplying abundance indices is increasingly explored (Rourke et al., 2022). However, numerous methodological challenges still need to be overcome and promising novel approaches remain to be tested (see review in Yao et al., 2022). One common result across a diversity of case studies is the high vertical resolution of eDNA signals in stratified marine ecosystems and the overall positive relationship of eDNA derived abundance indices with traditional sampling methods such as trawling, visual counts, echo-sounders, etc. (Rourke et al., 2022). Recent progress has further been made in applying molecular genetics to estimate absolute abundance of exploited fish populations based on genotyping fish tissues samples, identifying related individuals, and applying advanced statistical methods (Bravington et al., 2016; Trenkel et al., 2022). This approach also provided novel insights into demographic connectivity and fine scale population structure, which are essential for both conservation and sustainable exploitation (Hillary et al., 2018; Trenkel et al., 2022). Further, applications of eDNA metabarcoding can also be used to detect rare or threatened species captured as by-catch (Burgess et al., 2018), catalog fish gut content in diet analyses (Leray et al., 2013), and spot instances of farmed fish in wild stocks (Aguirre-Pabon et al., 2015).
Since its earliest application in French wetlands to detect and identify invasive American bullfrogs (Ficetola et al., 2008), eDNA methods have gained traction as a tool for the surveillance and monitoring of biodiversity. This includes in the United States where federal agencies have launched in-house eDNA research labs (Morisette et al., 2021) and have been successful in getting eDNA genetic findings admitted as evidence in litigation (N.D. Illinois 2010, App. Voices v US Dept of Interior 4th Cir Court 2022). This technology is now undergoing a transition from research-focused exploratory trials to more routine use by fisheries agencies (Aylagas et al., 2018; Laroche et al., 2018), with the next necessary step the creation of national and international policies (Lodge, 2022).
2.3. Vessel monitoring systems
Vessel monitoring systems (VMS) are a technology that contributed to improving MCS, implementing restricted areas for fishing, and showing a more reliable estimate of fishing effort than before (e.g., Murawski et al., 2005; Mills et al., 2007). VMS are designed to automatically acquire and transmit the position, speed and heading of the vessel. Because the reporting interval is mandatory (currently every hour for vessels above 12 meters in the European Union (EU)), and the VMS equipment cannot be switched off, any interruption of VMS signal reception can raise an alert in the MCS room of the flag state and may be analyzed as potential attempt to fraud. The VMS data set is encoded and sent over satellite link to the only authorized recipients. The flag state is the primary recipient, then sharing on “need to know” basis with coastal states or Regional Fisheries Management Organizations (RFMOs).
Because of its high level of data security, VMS is fit for the purpose of fishing effort estimate, distant control and possible at-sea inspections. Most fishing nations now operate a VMS to monitor their industrial fleet and many are considering monitoring more vessels to include small scale fisheries with cellular VMS (Burgos et al., 2013). The VMS data link is also used to transmit the electronic logbook (also called Electronic Reporting System or ERS), which becomes a mandatory requirement in many states. The electronic logbook is a computer or tablet application used by the ship master to report each fishing trip details (start, end, capture species and quantities, possible bycatch, quantities in hold when entering or exiting a zone). These data are used for quota management and fishing effort statistics.
In the EU, monitoring and control of the Common Fisheries Policy is based on the Council Regulation (EC) No 1224/2009 which requires fishing vessels above 12 meters to carry a VMS and report their catches via an electronic logbook. The VMS systems have been adapted for small scale artisanal boats, with cellular connectivity and solar panels (STARFISH, 2020). All EU member states today operate a Fisheries Monitoring Center (FMC) for their national fleet, to manage a vessel register, monitor the fishing efforts, compare with the licences and authorizations, detect suspicious activities and exchange data with the European Commission and regional fisheries management organizations. Data exchanges through the UN Flux format and cybersecurity are becoming standards in a context where fisheries irregularities can lead to heavy penalties.
2.4. Automatic identification systems
Automatic identification system (AIS) is another technology which has progressively been installed on most vessels above tonnage thresholds under the SOLAS regulation V/19 as a collision avoidance system supporting safety of life at sea. Like VMS, AIS also transmits the vessel position and movements but more frequently and in open broadcast format, so that everyone with an appropriate AIS receiver can pick up the messages. While the initial concept allowed reception in close proximity only (ship-to-ship to avoid collision) or through institutional coastal systems (port authorities), the AIS usage has greatly changed with introduction of web-based AIS data providers and commercial satellite-AIS constellations. These systems now make the vessels movements a commodity which can be purchased by any entity, including fishing companies to monitor their competitors, shipping companies to evaluate the traffic in ports etc. Some fishers prefer not to disclose the best fishing grounds and turn off the system, some others engaged in illegal activities may switch off (Welch et al., 2022) or purposely tamper their AIS device to send false identifiers or positions (called AIS spoofing; Androjna et al., 2021).
The reference data portal providing free access to global AIS data sets is the Global Fishing Watch (Kroodsma et al., 2018), which acquires AIS from satellite operators, publishes them and supports data analytics revealing large scale IUU activities. The portal also shows that AIS tracking is not evenly distributed over the planet. However, the portal gives only a partial view of actual fishing since most small fishing vessels do not have an AIS, or not an AIS model able to be received by satellite.
2.5. Remote electronic monitoring
Remote electronic monitoring (REM) is a technology of interest in deterrence of IUU fishing at sea and appraisal of incidental catches such as marine mammals (Kindt-Larsen et al., 2012). REM consists of a complete VMS, including CCTVs, sensors on winches, conveyors and freezers. The best AIS or VMS will not tell which species and quantity is actually captured, and the real figures may differ from those declared in the electronic reports. The monitoring of bycatch is a prime example demonstrating the capacity of REM to deter IUU fishing. Bycatch represents the non-targeted organisms captured while fishing for specific species. It could be tempting to discard it at sea to save limited hold space for target species, or to avoid a complete fisheries closure in the case of mixed fisheries where the vessel has reached its quota for a given species (the choke species) and cannot take the risk to continue fishing for the other species. Bycatch is by nature underestimated and underreported. In 2019, FAO evaluated discards at 9.1 million tons or 10% of total catches, and fisheries may interact with 20 million individuals of endangered species (sea birds, turtles, sharks etc.; Pérez et al., 2019). Many states have consequently implemented landing obligations for all bycatch, but these measures are complex to monitor unless deploying observers to monitor the deck activities. Where onboard observers were mandatory, their replacement by cameras has been increasingly considered and implemented when COVID pandemic prevented boarding of observers. Presently less than 1% of global fisheries are subject to REM. REM is a complex system to adapt to each individual vessel, and it may generate substantial amount of information, for which artificial intelligence will be necessary to extract relevant events from hours of video footage (Monkman et al., 2019), yet another technology with the potential to transform marine fisheries management and combat IUU fishing.
3. Discussion
3.1. Implementing the future of fisheries MCS technology
Innovation and increasing adoption of emerging technologies will continue to advance our ability to implement MCS global fisheries. VMS and AIS can improve our reach to see where fishing is happening, REM aids in estimating how much different fishes are brought on-board, and eDNA metabarcoding enables us to identify what has been landed from vessels, processed in factories, and sold in wholesale markets – tracking through the seafood supply chain (Figure 1). While these technologies are currently usable or tested in the global fisheries arena, government action is needed to facilitate their rapid adoption and at scale. Urgency to act is further fueled by consumers’ attention on seafood traceability and sustainability, which has catalyzed calls to strengthen international conventions and corporate social responsibility commitments (Mileski et al., 2016). This must include foreign fisheries assistance programs that cover the cost of disseminating the technology and building local capacity in the public and private sector.
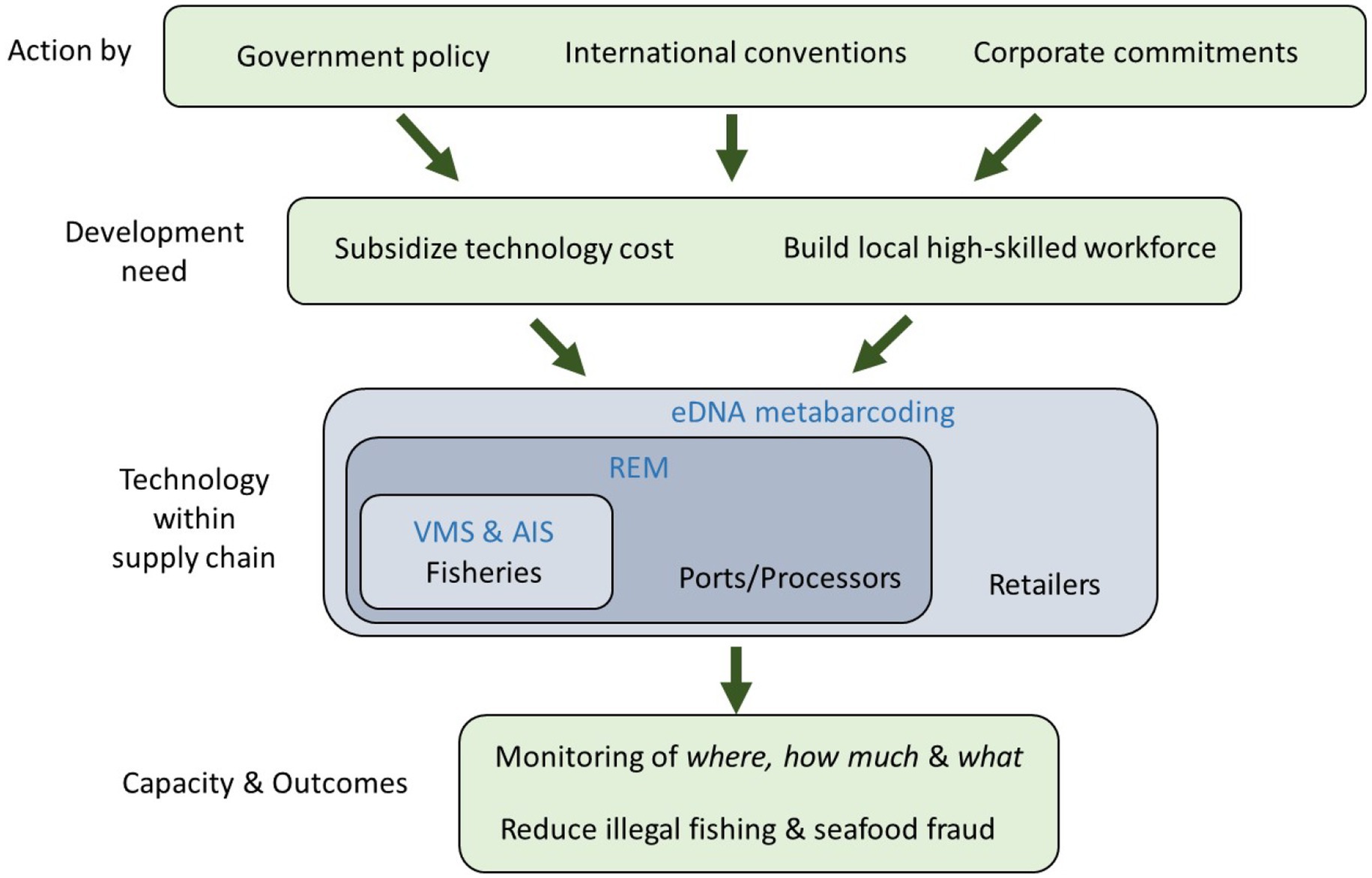
Figure 1. A conceptual framework illustrating how actions by fisheries stakeholders require development input of emerging monitoring technologies throughout the supply chain to monitor for and reduce illegal fishing and seafood fraud.
3.2. Practical and on-board considerations of MCS technology
It is not uncommon for fishers to be critical of data used for fisheries management as insufficient and not reflecting the realities of the global fishing industry (Wilson et al., 2006). Rather, fishers and the fishery industry often offer to contribute their own observations to complement official and research data. The implementation of emerging MCS technologies represent a way fishers may consult and participate in co-management schemes, including demonstration of best practices that may aid in accessing more lucrative fish marketplaces that require traceability and transparency (Nthane et al., 2020).
There are, however, distinctions in both the practical implementation of MSC technology in industrial fishing and small-scale fishing. Industrial fishing is already open to technology that can aid better efficiency, safety and compliance (FAO, 2022). For example, crew members are often trained and proficient in the use of electronic equipment such as AIS/VMS data. In the EU, new control regulation is aiming at increasing the number of vessels under VMS obligations (vessels below 12 m in length) and introduce broader adoption of REM (European Fisheries Control Agency, 2019). In contrast, small scall fisheries are highly diversified by country, regularly combine commercial and subsistence fishing, and is often less exposed to monitoring technologies for an assortment of reasons (lack of investment capital, insufficient workforce training, aging vessels, poorly represented fishers groups at highest decision levels, etc.). Small scale fisheries are data poor and it is difficult to find statistics on how best to promote acceptance of emerging technologies to these groups. Encouragingly recent reporting indicates that the most effective management solutions are based on co-management and empowerment of fishers’ communities in decision process and confirm the need for data and data collecting technology (FAO, Duke University, World Fish, 2023).
If emerging technology comes in support of better fisheries management, better sales prices, improved access to better quality markets, reduced waste, or reduced operational costs, then it may be accepted. The virtuous cycle is selecting and implementing technologies for data collection, improving management based on science-based choices, improving the fisheries sustainability and profitability, and then developing a circle of confidence. This process may proceed slowly or too slowly to gain support. We must recognize that in many cases the defiance of adopting technologies is in fact a defiance of decision makers. For fishers who are required to carry REM onboard, they may ask if it will be used to reduce time at sea or avoid areas because of risk of encounters with dolphins? Technology for compliance purposes will be more difficult to implement effectively and require costs be offset by subsidies. Likewise, the concern of privacy in recording technology has been raised, however, a growing number of jurisdiction implement policies and specifications for REM including hiding personal faces and aiming devices at hands, belts and fish storage areas (European Fisheries Control Agency, 2019). If, however, technology could concurrently be used for commercial purposes, then it would be more acceptable. A successful example is the South African company Abalobi and their use of traceability and fish catch inventory by fishers (Nthane et al., 2020).
3.3. Drawing talent for tomorrow’s fisheries workforce
Fishing is a prehistoric practice dating back at least 40,000 years. It has evolved over time to become an activity practiced by almost 40 million people worldwide, using around 4.1 million vessels, of which two third are deployed in Asia (~1.8 million vessels), Africa (~635,000 vessels) and the Americas (~240,000 vessels). Approximately 600 million people worldwide are supported directly by fisheries (FAO, 2022).
Fisheries are a major engine of the global economy. Yet as employment opportunities worldwide have diversified, young graduates have moved away from the primary production sectors of fisheries, to embrace the more lucrative sectors of finance, high tech industries and services. Furthermore, employment in fisheries has carried a reputation of being lower skilled, of modest means, or an unfashionable activity. Fisheries of the 21st century, however, are changing. As more countries embrace science-based fisheries management policies, increase adoption of international instruments, and utilize monitoring technologies for regulatory enforcement, there is growing demand for highly skilled personnel to complement the traditional fisheries workforce. This is particularly true to facilitate and maximize the implementation of technological MCS innovations including AIS, VMS, REM, and molecular genetic tools. These jobs will require young professionals from the disciplines of genetics, biotechnology, information technology, computer science, and data management.
Technologies cannot solve all the issues of IUU fishing and fish fraudulent practices, but they have now demonstrated the capacity to detect IUU fishing activities at sea, which was nearly impossible 20 years ago (Dunn et al., 2018; Kroodsma et al., 2018). Progressive digital transition and more integrated data exchanges have also made it possible to improve fisheries management, reduce the catch documentation analysis workload, produce more reliable risk analysis based on vessel history, and allow much faster verification prior to landing. Concurrently, Millennials and Generation Z have collectively developed a deep interest in environmental issues, including the urgent necessity to protect marine ecosystems and biodiversity while simultaneously seeking to provide healthy food to the global population. As a result, many national and international programs for training and education in areas related to fisheries have been upgraded and adapted to address this new paradigm. These efforts aim to prepare future generations of fisheries experts well versed in these scientific and technological areas, who are now entering the profession and charting the course for the future of global marine fisheries.
Data availability statement
The original contributions presented in the study are included in the article. Any inquiries can be directed to the corresponding author.
Author contributions
All authors listed have made a substantial, direct, and intellectual contribution to the work and approved it for publication.
Funding
Funding for this paper’s associated colloquium and publication charges were generously provided through a grant from the Albert and Elaine Borchard Foundation Center on International Education to DW.
Conflict of Interest
The authors declare that the research was conducted in the absence of any commercial or financial relationships that could be construed as a potential conflict of interest.
Publisher’s note
All claims expressed in this article are solely those of the authors and do not necessarily represent those of their affiliated organizations, or those of the publisher, the editors and the reviewers. Any product that may be evaluated in this article, or claim that may be made by its manufacturer, is not guaranteed or endorsed by the publisher.
Acknowledgments
The authors thank Janna Beling, Director of the Borchard Foundation Center on International Education for hosting this paper’s associated colloquium. DW thanks Margarita Joaquin for logistical support.
References
Aguirre-Pabon, J. C., Orozco Berdugo, G. Jr., and Barandica, J. C. N. (2015). Genetic status, source and establishment risk of the giant tiger shrimp (Penaeidae: Penaeus monodon), an invasive species in Colombian Caribbean waters. Acta Biol. Colomb. 20, 117–127. doi: 10.15446/abc.v20n1.41946
Ahmed, Hassan Omar (2018). “The Somali civil war: root causes.” Master’s thesis, İstanbul Ticaret Üniversitesi Sosyal Bilimler Enstitüsü.
Androjna, A., Perkovič, M., Pavic, I., and Mišković, J. (2021). AIS data vulnerability indicated by a spoofing case-study. Appl. Sci. 11:5015. doi: 10.3390/app11115015
Ash, K., and Obradovich, N. (2020). Climatic stress, internal migration, and Syrian civil war onset. J. Confl. Resolut. 64, 3–31. doi: 10.1177/0022002719864140
Aylagas, E., Borja, Á., Muxika, I., and Rodríguez-Ezpeleta, N. (2018). Adapting metabarcoding-based benthic biomonitoring into routine marine ecological status assessment networks. Ecol. Indic. 95, 194–202. doi: 10.1016/j.ecolind.2018.07.044
Béné, C., Barange, M., Subasinghe, R., Pinstrup-Andersen, P., Merino, G., Hemre, G.-I., et al. (2015). Feeding 9 billion by 2050–Putting fish back on the menu. Food Secur. 7, 261–274. doi: 10.1007/s12571-015-0427-z
Boyd, C. E., McNevin, A. A., and Davis, R. P. (2022). The contribution of fisheries and aquaculture to the global protein supply. Food Secur. 14, 805–827. doi: 10.1007/s12571-021-01246-9
Bravington, M. V., Skaug, H. J., and Anderson, E. C. (2016). Close-kin mark-recapture, Statist. Sci 31, 259–274. doi: 10.1214/16-STS552
Burgess, M. G., McDermott, G. R., Owashi, B., Peavey, L. E., Reeves, T. C., Ovando, D., et al. (2018). Protecting marine mammals, turtles, and birds by rebuilding global fisheries. Science 359, 1255–1258. doi: 10.1126/science.aao4248
Burgos, C., Gil, J., and del Olmo, L. A. (2013). The Spanish blackspot seabream (Pagellus bogaraveo) fishery in the Strait of Gibraltar: spatial distribution and fishing effort derived from a small-scale GPRS/GSM based fisheries vessel monitoring system. Aquat. Living Resour. 26, 399–407. doi: 10.1051/alr/2013068
Cheung, W. W. L., Palacios-Abrantes, J., Frölicher, T. L., Palomares, M. L., Clarke, T., Lam, V. W. Y., et al. (2022). Rebuilding fish biomass for the world's marine ecoregions under climate change. Glob. Chang. Biol. 28, 6254–6267. doi: 10.1111/gcb.16368
Deiner, K., Bik, H. M., Mächler, E., Seymour, M., Lacoursière-Roussel, A., Altermatt, F., et al. (2017). Environmental DNA metabarcoding: transforming how we survey animal and plant communities. Mol. Ecol. 26, 5872–5895. doi: 10.1111/mec.14350
Devereux, S. (2000). Famine in the twentieth century. IDS Working Paper 105, Brighton: IDS, http://bldscat.ids.ac.uk/cgi-bin/koha/opac-detail.pl?biblionumber=119124
Doughty, Christopher E., Roman, Joe, Faurby, Søren, Wolf, Adam, Haque, Alifa, Bakker, Elisabeth S., et al. (2016). "Global nutrient transport in a world of giants." in Proceedings of the National Academy of Sciences 113, no. 4. pp. 868–873.
Dunn, D. C., Jablonicky, C., Crespo, G. O., McCauley, D. J., Kroodsma, D. A., Boerder, K., et al. (2018). Empowering high seas governance with satellite vessel tracking data. Fish Fish. 19, 729–739. doi: 10.1111/faf.12285
Eales, J., Bethel, A., Fullam, J., Olmesdahl, S., Wulandari, P., and Garside, R. (2021). What is the evidence documenting the effects of marine or coastal nature conservation or natural resource management activities on human well-being in South East Asia? A systematic map. Environ. Int. 151:106397. doi: 10.1016/j.envint.2021.106397
Estes, J. A., Terborgh, J., Brashares, J. S., Power, M. E., Berger, J., Bond, W. J., et al. (2011). Trophic downgrading of planet Earth. Science 333, 301–306. doi: 10.1126/science.1205106
European Fisheries Control Agency. (2019). “Technical guidelines and specifications for the implementation of Remote Electronic Monitoring (REM) in EU Fisheries ”. Vigo, Spain.
FAO, Duke University, World Fish. (2023). “Illuminating Hidden Harvests – The contributions of small scale fisheries to sustainable development ”. Rome. doi: 10.4060/cc4576en
FAO. (2022). “Aquaculture Department, The State of World Fisheries and Aquaculture.” Towards Blue Transformation. Rome, FAO. doi: 10.4060/cc0461en
Ficetola, G. F., Miaud, C., Pompanon, F., and Taberlet, P. (2008). Species detection using environmental DNA from water samples. Biol. Lett. 4, 423–425. doi: 10.1098/rsbl.2008.0118
Free, C. M., Thorson, J. T., Pinsky, M. L., Oken, K. L., Wiedenmann, J., and Jensen, O. P. (2019). Impacts of historical warming on marine fisheries production. Science 363, 979–983. doi: 10.1126/science.aau1758
Gutierrez, A. T., and Morgan, S. K. (2015). The influence of the Sustainable Seafood Movement in the US and UK capture fisheries supply chain and fisheries governance. Front. Mar. Sci. 2:72. doi: 10.3389/fmars.2015.00072
Hammerschlag, N., Schmitz, O. J., Flecker, A. S., Lafferty, K. D., Sih, A., Atwood, T. B., et al. (2019). Ecosystem function and services of aquatic predators in the Anthropocene. Trends Ecol. Evol. 34, 369–383. doi: 10.1016/j.tree.2019.01.005
Hillary, R. M., Bravington, M. V., Patterson, T. A., Grewe, P., Bradford, R., Feutry, P., et al. (2018). Genetic relatedness reveals total population size of white sharks in eastern Australia and New Zealand. Sci. Rep. 8:2661. doi: 10.1038/s41598-018-20593-w
HLPE. (2014). “Sustainable fisheries and aquaculture for food security and nutrition.” A report by the High Level Panel of Experts on Food Security and Nutrition of the Committee on World Food Security, Rome
Howson, P. (2020). Building trust and equity in marine conservation and fisheries supply chain management with blockchain. Mar. Policy 115:103873. doi: 10.1016/j.marpol.2020.103873
Hughes, T. P., Rodrigues, M. J., Bellwood, D. R., Ceccarelli, D., Hoegh-Guldberg, O., McCook, L., et al. (2007). Phase shifts, herbivory, and the resilience of coral reefs to climate change. Curr. Biol. 17, 360–365. doi: 10.1016/j.cub.2006.12.049
Hutchings, J. A., Minto, C., Ricard, D., Baum, J. K., and Jensen, O. P. (2010). Trends in the abundance of marine fishes. Can. J. Fish. Aquat. Sci. 67, 1205–1210. doi: 10.1139/F10-081
Jackson, J. B. C., Kirby, M. X., Berger, W. H., Bjorndal, K. A., Botsford, L. W., Bourque, B. J., et al. (2001). Historical overfishing and the recent collapse of coastal ecosystems. Science 293, 629–637. doi: 10.1126/science.1059199
Kawarazuka, N., and Béné, C. (2010). Linking small-scale fisheries and aquaculture to household nutritional security: an overview. Food Secur. 2, 343–357. doi: 10.1007/s12571-010-0079-y
Kindt-Larsen, L., Dalskov, J., Stage, B., and Larsen, F. (2012). Observing incidental harbour porpoise Phocoena phocoena bycatch by remote electronic monitoring. Endanger. Species Res. 19, 75–83. doi: 10.3354/esr00455
Kroodsma, D. A., Mayorga, J., Hochberg, T., Miller, N. A., Boerder, K., Ferretti, F., et al. (2018). Tracking the global footprint of fisheries. Science 359, 904–908. doi: 10.1126/science.aao5646
Laroche, O., Wood, S. A., Tremblay, L. A., Ellis, J. I., Lear, G., and Pochon, X. (2018). A cross-taxa study using environmental DNA/RNA metabarcoding to measure biological impacts of offshore oil and gas drilling and production operations. Mar. Pollut. Bull. 127, 97–107. doi: 10.1016/j.marpolbul.2017.11.042
Leray, M., Yang, J. Y., Meyer, C. P., Mills, S. C., Agudelo, N., Ranwez, V., et al. (2013). A new versatile primer set targeting a short fragment of the mitochondrial COI region for metabarcoding metazoan diversity: application for characterizing coral reef fish gut contents. Front. Zool. 10, 34–14. doi: 10.1186/1742-9994-10-34
Lindkvist, E., Pellowe, K. E., Alexander, S. M., O'Neill, E. D., Finkbeiner, E. M., Girón-Nava, A., et al. (2022). Untangling social–ecological interactions: A methods portfolio approach to tackling contemporary sustainability challenges in fisheries. Fish Fish. 23, 1202–1220. doi: 10.1111/faf.12678
Lodge, D. M. (2022). Policy action needed to unlock eDNA potential. Front. Ecol. Environ. 20:2563. doi: 10.1002/fee.2563
Lotze, H. K., and Worm, B. (2009). Historical baselines for large marine animals. Trends Ecol. Evol. 24, 254–262. doi: 10.1016/j.tree.2008.12.004
Luque, G. M., and Josh Donlan, C. (2019). The characterization of seafood mislabeling: a global meta-analysis. Biol. Conserv. 236, 556–570. doi: 10.1016/j.biocon.2019.04.006
Meyers, G., McIntosh, P., Pigot, L., and Pook, M. (2007). The years of El Niño, La Niña, and interactions with the tropical Indian Ocean. J. Clim. 20, 2872–2880. doi: 10.1175/JCLI4152.1
Michie, S., Van Stralen, M. M., and West, R. (2011). The behaviour change wheel: a new method for characterising and designing behaviour change interventions. Implement. Sci. 6, 1–12. doi: 10.1186/1748-5908-6-42
Mileski, J. P., Galvao, C. B., and Forester, Z. D. (2016). Human trafficking in the commercial fishing industry: A multiple case study analysis. Marine Policy 116. doi: 10.1016/j.marpol.2019.103616
Mills, C. M., Townsend, S. E., Jennings, S., Eastwood, P. D., and Houghton, C. A. (2007). Estimating high resolution trawl fishing effort from satellite-based vessel monitoring system data. ICES J. Mar. Sci. 64, 248–255. doi: 10.1093/icesjms/fsl026
Monkman, G. G., Hyder, K., Kaiser, M. J., and Vidal, F. P. (2019). Using machine vision to estimate fish length from images using regional convolutional neural networks. Methods Ecol. Evol. 10, 2045–2056. doi: 10.1111/2041-210X.13282
Morisette, J., Burgiel, S., Brantley, K., Daniel, W. M., Darling, J., Davis, J., et al. (2021). Strategic considerations for invasive species managers in the utilization of environmental DNA (eDNA): steps for incorporating this powerful surveillance tool. Manag. Biol. Invasions 12, 747–775. doi: 10.3391/mbi.2021.12.3.15
Murawski, S. A., Wigley, S. E., Fogarty, M. J., Rago, P. J., and Mountain, D. G. (2005). Effort distribution and catch patterns adjacent to temperate MPAs. ICES J. Mar. Sci. 62, 1150–1167. doi: 10.1016/j.icesjms.2005.04.005
Naaum, A. M., Warner, K., Mariani, S., Hanner, R. H., and Carolin, C. D. (2016). “Seafood mislabeling incidence and impacts” in Seafood Authenticity and Traceability, eds. Amanda M. Naaum and Robert H. Hanner (Cambridge, Massachusetts: Academic Press), 3–26.
Nthane, T. T., Saunders, F., Gallardo Fernández, G. L., and Raemaekers, S. (2020). Toward Sustainability of South African Small-Scale Fisheries Leveraging ICT Transformation Pathways. Cultural Landscapes Preservation and Social–Ecological Sustainability 12, 743. doi: 10.3390/su12020743
Pérez, R., Gilman, E., Huntington, T., Kennelly, S. J., Suuronen, P., Chaloupka, M., et al. (2019). Third assessment of global marine fisheries discards, Rome, Italy: FAO.
Perros, T., Allison, A. L., Tomei, J., and Parikh, P. (2022). Behavioural factors that drive stacking with traditional cooking fuels using the COM-B model. Nat. Energy 7, 886–898. doi: 10.1038/s41560-022-01074-x
Rourke, M. L., Fowler, A. M., Hughes, J. M., Broadhurst, M. K., DiBattista, J. D., Fielder, S., et al. (2022). Environmental DNA (eDNA) as a tool for assessing fish biomass: A review of approaches and future considerations for resource surveys. Environ. DNA 4, 9–33. doi: 10.1002/edn3.185
Selig, E. R., Hole, D. G., Allison, E. H., Arkema, K. K., McKinnon, M. C., Chu, J., et al. (2019). Mapping global human dependence on marine ecosystems. Conserv. Lett. 12:e12617. doi: 10.1111/conl.12617
STARFISH (2020). 4.0: Making Small Scale Fisheries more sustainable. Available at: https://cinea.ec.europa.eu/news-events/news/starfish-40-making-small-scale-fisheries-more-sustainable-2020-11-19_en (Accessed 14 February 2023).
Thiault, L., Mora, C., Cinner, J. E., Cheung, W. W. L., Graham, N. A. J., Januchowski-Hartley, F. A., et al. (2019). Escaping the perfect storm of simultaneous climate change impacts on agriculture and marine fisheries. Sci. Adv. 5:eaaw9976. doi: 10.1126/sciadv.aaw9976
Trenkel, V. M., Charrier, G., Lorance, P., and Bravington, M. V. (2022). Close-kin mark–recapture abundance estimation: practical insights and lessons learned. ICES J. Mar. Sci. 79, 413–422. doi: 10.1093/icesjms/fsac002
Welch, H., Clavelle, T., White, T. D., Cimino, M. A., Van Osdel, J., Hochberg, T., et al. (2022). Hot spots of unseen fishing vessels. Sci. Adv. 8:eabq2109. doi: 10.1126/sciadv.abq2109
Willette, D. A., Allendorf, F. W., Barber, P. H., Barshis, D. J., Carpenter, K. E., Crandall, E. D., et al. (2014). So, you want to use next-generation sequencing in marine systems? Insight from the Pan-Pacific Advanced Studies Institute. Bull. Mar. Sci. 90, 79–122. doi: 10.5343/bms.2013.1008
Willette, D. A., Esteves, S. C., Fitzpatrick, B., Smith, M. L., Wilson, K., and Yuan, X. (2021a). The last mile challenge: Certified seafood and federal labeling laws out of sync at the end of the supply chain in Los Angeles, California. Mar. Policy 125:104380. doi: 10.1016/j.marpol.2020.104380
Willette, D. A., Navarrete-Forero, G., Gold, Z., Apollo, M. D., Lizano, L. G.-S., and Sotil, G. (2021b). Characterizing Industrial and Artisanal Fishing Vessel Catch Composition Using Environmental DNA and Satellite-Based Tracking Data. Foods 10:1425. doi: 10.3390/foods10061425
Wilson, D. C., Mafuzuddin, A., Siar, S. V., and Kanagaratnam, U. (2006). Cross-scale linkages and adaptive management: Fisheries co-management in Asia. Mar. Policy 30, 523–533. doi: 10.1016/j.marpol.2005.07.001
Keywords: automatic identification systems, environmental DNA, illegal fishing, vessel monitoring, remote electronic monitoring, seafood fraud, vessel monitoring systems
Citation: Willette DA, Ababouch L, Barber PH, Bunje PME, Cauzac J-P, Conchon A and Trenkel VM (2023) Emerging monitoring technologies to reduce illegal fishing activities at sea and prevent entry of fraudulent fish into markets. Front. Sustain. Food Syst. 7:1166131. doi: 10.3389/fsufs.2023.1166131
Edited by:
Roberto Anedda, Parco Scientifico e Tecnologico della Sardegna, ItalyReviewed by:
Brooks A. Kaiser, University of Southern Denmark, DenmarkLiliana Mihaela Moga, Dunarea de Jos University, Romania
Copyright © 2023 Willette, Ababouch, Barber, Bunje, Cauzac, Conchon and Trenkel. This is an open-access article distributed under the terms of the Creative Commons Attribution License (CC BY). The use, distribution or reproduction in other forums is permitted, provided the original author(s) and the copyright owner(s) are credited and that the original publication in this journal is cited, in accordance with accepted academic practice. No use, distribution or reproduction is permitted which does not comply with these terms.
*Correspondence: Demian A. Willette, demian.willette@lmu.edu