Ultrasound-assisted fortification of yellow sweet potato (Ipomoea batatas) with iron and ascorbic acid
- 1Department of Food Engineering, Universidad Nacional de Frontera, Sullana, Peru
- 2Department of Chemistry, Federal University of Santa Catarina, Florianopolis, Brazil
The objective of this study was to evaluate the effect of ultrasound on the incorporation of iron and ascorbic acid (AA) in sweet potato (Ipomoea batatas) and to optimize the process parameters to obtain a fortified food. The incorporation was carried out using cubes of sweet potato submerged in 0.1% m/v ferrous sulfate and 1% m/v AA solutions, treated at different times and sonication frequencies (37 and 80 kHz), at 100 watts of power and 30 ± 5°C. ANOVA and Tukey’s test at 5% significance were applied to establish significant differences and the process was evaluated using a factorial design. The results revealed that the application of ultrasound influences the content of iron and AA, incorporating greater amount of iron and AA compared to samples not treated with ultrasound. Similarly, longer times led to higher incorporation of iron and AA content in sweet potatoes; the frequency was not statistically significant. The highest iron content was 105.91 ± 0.03 mg/100 g and for AA, it was 392.65 ± 4.84 mg AAE/100 g. The defined ultrasonic process conditions produced an increase of 4928.99 and 610.65%, respectively, in iron and AA content in sweet potato.
1. Introduction
The need and consumption of fortified foods have increased significantly in recent years, there is great concern about the nutritional aspects and processed foods products consumed in the daily diet, this is due to the high nutritional deficiencies in the world population. Iron deficiency anemia is one of the most prevalent nutritional deficiencies worldwide, and is considered as a public health problem (Ministerio de Salud [MINSA], 2017). Worldwide, anemia affects 500 million women of reproductive age (15–49 years), 40% of pregnant women, and 42% of children under five years of age (Meilianti et al., 2023). In Peru, 40% of the population suffers from anemia (Instituto Nacional de Salud [INS], 2020), 33.6% of children under 5 years old, 20.6% of women between 15 and 49 years old by 2022 (Instituto Nacional de Estadística e Informática [INEI], 2023).
This issue can be approach by adhering to a strict diet, containing iron-rich foods, and consuming supplements or fortified and/or enriched foods. Iron fortification is considered the most effective option to prevent malnutrition on a large scale, and this strategy has been applied in different governmental programs (Joshi et al., 2019). Iron is a micronutrient necessary for the human body; it participates in the production of hemoglobin and myoglobin, which are responsible for oxygen transport. Likewise, contributes to the metabolism of certain enzymes and in the synthesis and catabolism of neurotransmitters, therefore this deficiency has an impact on behavioral, mental and motor development, and also induce a slower speed of conduction of the sensory, auditory and visual systems (Rivera et al., 2012; Ministerio de Salud [MINSA], 2017).
Fortification is the addition of a micronutrient to food to increase the content of one or more essential micronutrients, correct or prevent a demonstrated deficiency (dietary, biochemical, functional, and/or clinical) of a nutrient in the population (Latham and FAO, 2002). Iron fortification is one of the most complex, there are different iron compounds concerning their solubility and chemical state; ferrous sulfate is the most widely used source of iron in the industry, due to its high bioavailability of the micronutrient, and its low cost (Shubham et al., 2020). To enhance absorption, iron is combined with vitamin C, which acts as a potentiator. Vitamin C in molar relations with iron greater than 1:1 can double the absorption of non-heme iron, despite the presence of dietary inhibitory factors (Tostado-Madrid et al., 2015). However, when iron incorporated directly into the food matrix, it can oxidize and cause undesirable changes in organoleptic properties (odor, taste, color) (Genevois et al., 2014). For this reason, the optimal fortification of foods with complex structures such as fruits and vegetables, requires a great deal of research, most of the traditional technologies used cause instability in the added nutrients; this is why new technologies such as ultrasound (US) are being used (Carvalho et al., 2021).
Ultrasonic technology has been the subject of numerous studies aimed at improving mass transfer in foods. These studies utilized different media, such as water, osmotic solutions and ethanol, to transmit acoustic waves into the food matrix. This method enables the efficient inclusion of compounds, such as iron, more effectively, improving their homogeneous dispersion of micronutrients (Rojas et al., 2019). Furthermore, the use of this technology aids in decreasing nutrient loss and processing times, favoring the quality of the sensory properties of the product (Bhargava et al., 2021).
Carvalho et al. (2021) demonstrated the efficacy of the combination of ultrasonic technology, microencapsulation, and convection drying in the production of fortified pineapple chips. The authors showed that optimal ultrasonic pre-treatment of ethanol (25 kHz - 30 min), significantly increases the iron content (up to 1,000% more than the control sample) in the final product. Similarly, Bonto et al. (2020) incorporated iron in rice with ultrasonic technology (40 kHz- 5 min). The absorption of iron in the rice sample was 321 ± 13.43 mg/kg of rice, achieving a 28-fold increase when compared to the untreated rice sample. Furthermore, the experiment revealed efficient iron diffusion, and achieved 82.9% retention rate after washing and cooking. Similarly, Rojas et al. (2019) incorporated iron and carotenoid microencapsulates in pumpkin and apple, respectively. The application of ultrasound resulted in a more homogeneous distribution of iron increased iron content by over than 1,000% compared to the control samples.
Mashkour et al. (2018) incorporated iron into whole potatoes by vacuum impregnation in combination with ultrasonic technology (37 kHz - 45 min) as a pre-treatment. Their study showed that the combination of IV (vacuum impregnation) with the US (ultrasonic waves), resulted in higher iron incorporation of 210%. In addition, no significant effects on color and texture parameters were observed. Miano and Augusto (2018) incorporated iron during the bean hydration process with ultrasound application (91 W/L; 25 kHz), after 510 min, the incorporation obtained with ultrasonic application was 60.1 mg Fe/100 g, compared to 34.4 mg Fe/100 g without ultrasonic application. Mason et al. (2015) mentioned that ultrasound generates positive effects on processed food, this effect occurs due to the collapse of cavitation bubbles that are caused by pressure fluctuations, exerted by the passage of acoustic waves from the US bath. This cell disruption can produce a higher mass transfer (solids gain and water loss), allowing better impregnation of the solvent and thus facilitating the incorporation of micronutrients in foods (Bonto et al., 2018; Yılmaz and Ersus Bilek, 2018).
The incorporation of iron in different foods has been investigated with positive results, reflected in the increase of the content of this micronutrient. To this extent, it is interesting to investigate the incorporation in food matrices, being sweet potato is a food of great interest and has great potential to become a food vehicle, since it ranks seventh in most produced food. Sweet potatoes are one of the most significant crops worldwide, producing over 104 million tons in 2014 (FAOSTAT data, 2017). In addition, production cost is relatively low (Grozo and INEI, 2021). Likewise, this food has valuable nutritional content and is highly rich in nutrients, such as vitamin C, Fe, K, and Ca. It is a member of the Convolvulaceae family, genus Ipomoea, and the type species Ipomea batatas L. The orange-fleshed sweet potato has antioxidant properties, anti-inflammatory effects, high iron and zinc content and a low glycemic index being a great ally in countries suffering food shortages (Oladejo and Ma, 2016; Renee et al., 2018; Nyathi et al., 2019). Although heme iron contained in animals is more bioavailable than non-heme iron (found in vegetables), many countries have low meat consumption and high prevalence of iron deficiency anemia, therefore, non-heme iron is an alternative option to increase iron intake and combat iron deficiency. Furthermore, vegetable sources may contain a significant content of AA, which favors a higher bioavailability of iron (Andre et al., 2018). Although studies on ultrasound-assisted food fortification are reviewed in the literature, there are no studies that propose the incorporation of iron and AA in sweet potato. Consequently, considering the great importance of iron-fortified foods for the world population, the objective of this work was to study the effect of ultrasound on the incorporation of iron and AA in sweet potato (Ipomoea batatas) and to evaluate the ultrasound parameters for obtaining an fortified food.
2. Materials and methods
2.1. Reagents
All the reagents were of analytical grade, for the determination of ascorbic acid (AA) we used 2,4-dinitrophenylhydrazine (Lobachemie, India), glacial acetic acid (Scharlau, Spain), bromine water, thiourea, sodium acetate, metaphosphoric acid, sulfuric acid (98%, m/v), the reagents were acquired from Movilab (Lima, Peru). For iron determination, 1, 10-phenanthroline (Biolab, Argentina), ammonium iron (II) sulfate (Fe(SO4)(NH4)2(SO4)6H2O) (Movilab), hydroxylamine hydrochloride (Scharlau, Spain), hydrochloric acid (37% m/v) was purchased from (J.T.Baker, Mexico), nitric acid (65% m/v) was purchased from (Merck, Germany). AA C6H8O6 and ferrous sulfate (FeSO4) were purchased from Movilab.
2.2. Raw material and sample preparation
Yellow sweet potatoes (Ipomoea batatas) were acquired from a local supermarket in Sullana (Peru) and kept in storage at 27 ± 3°C for 2 to 3 days prior to utilization. The sample had a soluble solids content of 10 ± 1 °Brix and a moisture content of 79.8 ± 1.5%. The sweet potato samples were sliced into pieces measuring11 mm x 11 mm x 5 mm for further processing.
2.3. Ultrasound-assisted incorporation of iron and acorbic acid
Iron and AA were incorporated following the methodologies reported by Carvalho et al. (2021) and Rojas et al. (2019). First, the solution was prepared containing ferrous sulfate at 0.1% w/v and AA at 1% w/v (FA). Next, sweet potato slices were added to a beaker containing FA solution in a 1:5 w/v ratio. The above samples were placed in an the ultrasonic bath (Elmasonic P 30H, Germany) and treated at different frequencies (37 and 80 kHz) for 10, 20, 30, 40, 50, and 60 min., and ultrasound power of 100 watts and temperature inside the ultrasonic bath was 30°C. A control sample (without ultrasonic treatment) was also prepared in parallel. Subsequently, both the ultrasound-treated and control samples were drained, and dehydrated in a tray dryer (Dehydrator ST-04, 30–90°C, 0 − 15 h), at 45 ± 2°C for 8 h at air circulation speed (2.5 m/s). The Schematic diagram of the ultrasound-assisted incorporation of iron and AA in sweet potatoes is shown in Figure 1.
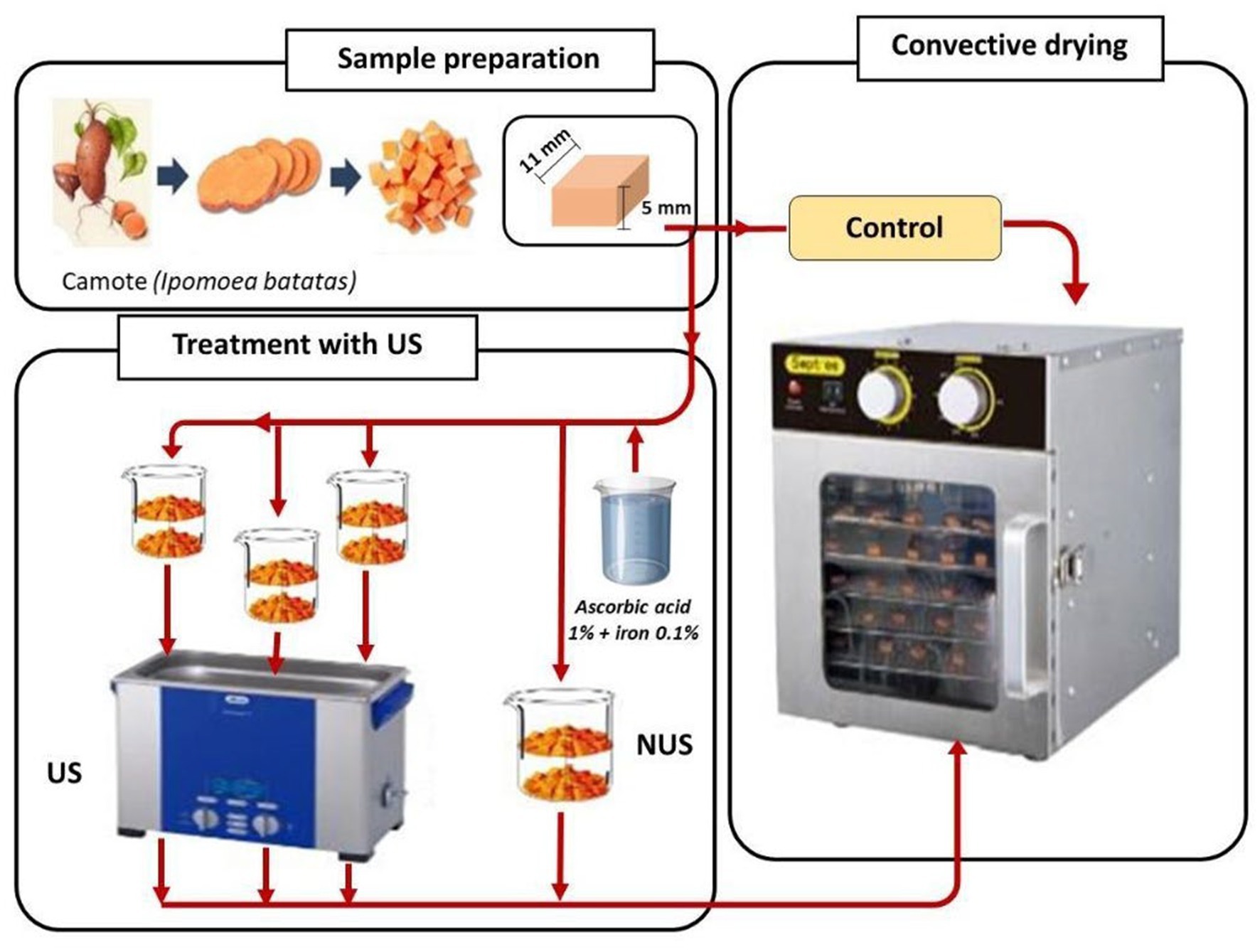
Figure 1. Schematic diagram of ultrasound-assisted incorporation of iron and AA in sweet potato (Ipomoea batatas). US, Ultrasonic treatment; NUS, No ultrasonic treatment.
2.4. Determination of AA
Total AA (ascorbic acid + dehydroascorbic acid) was quantified using the UV spectrophotometric method described by Rahman et al. (2007). Ascorbic acid is oxidized to dehydroascorbic acid by the action of bromine water in an acidic medium, generated by the presence of acetic acid. The AA was extracted from the samples; for this, 1 g of the sample was mixed with 20 mL of 5% w/v metaphosphoric acid solution and 10% v/v acetic acid centrifuged at 2500 rpm for 4 min. Next, the obtained mixture was filtered with Whatman No. 1 filter. Next, 3 mL of filtered solution (SF) was reacted with 0.15 mL of bromine water, and then gently stirred. Additionally, 0.80 mL of 10% m/v thiourea was added to remove excess bromine, then 1 mL of 2,4- dinitrophenylhydrazine solution was added and gently stirred. To finish the reaction, the samples were maintained in an incubator (Usamed, DNP-9052A) at 37°C temperature for 3 h; subsequently, the samples solutions were immediately cooled in an ice bath, added 1 mL of 85% m/v H2SO4 with constant stirring and made up to 5 mL with distilled water. The absorbance of the colored solution obtained was measured at 521 nm in a UV–visible spectrophotometer (Thermo Scientific, model Genesys 150). The calibration curves were obtained with standard solutions of AA ranging from 2.5 to 20 mg/L, following the same procedure used for the samples, and the concentrations were expressed in mg of AA (AAE) /100 g. All determinations were performed in triplicate and all steps were repeated for the blank sample.
2.5. Determination of total iron
The determination of total iron was performed according to the AOAC (1994) method (944.02) with adaptations. In this method, Fe2+ reacts with 1,10-phenanthroline to form a characteristic red-orange complex that absorbs strongly in the region around 510 nm of the visible spectrum. Hydroxylamine hydrochloride solution was applied as a reducing agent to reduce Fe 3+ to Fe 2+.
To perform the analysis, a crucible was used to weigh 1 g of the sample and which was subsequently placed in a muffle furnace (Sel-Horn “R- 8 L) at 550 ± 15°C for a period of 5 h. Next, 0.1 mL of nitric acid was added to the ashes obtained and evaporated on a hot plate. Next, 1 mL of hydrochloric acid was added and again evaporated on a hot plate. Finally, 0.2 mL of hydrochloric acid was added, and the volume was filled up to 10 mL with distilled water. For quantification, an aliquot of 2 mL of the above solution was reacted with 0.6 mL of hydroxylamine hydrochloride, then allowed to stand in a dark place for 5 min, followed by the addition of 1.5 mL of acetate buffer and 0.6 mL of 1,10-phenanthroline, stirred gently and distilled water was added to make up the 5 mL. Finally, the solution was agitated in a vortex (Cole-Parmer, model SA8) at 1000 rpm × 10s and kept at rest for 10 min. The absorbance was measured at 510 nm in a UV–visible spectrophotometer. The calibration curves were obtained with standard solutions of Fe2+ ranging from 0.5 to 5.0 mg/L, following the same procedure applied to the samples, and the concentrations were expressed in mg /100 g. All determinations were performed in triplicate and all steps were repeated for the blank sample.
2.6. Experimental design and statistical analysis
For screening purposes, a 22 full factorial design, with two levels (−1 and + 1) was applied to the two independent factors frequency (37–80 kHz) and ultrasound exposure time (10 and 60 min) in the responses of iron (mg/100 g) content and AA (mg AAE/100 g), in a total of 4 runs (Table 1). All the experiments were performed in triplicate.
Apart from the factorial design, aliquots were evaluated every 10 min (20, 30, 40, and 50 min) in 37 and 80 kHz to process control, in a total of 8 runs. For the analysis of results, the Analysis of Variance method (ANOVA) and Tukey’s test were used to determine differences between different treatments. The process was evaluated using factorial design with Statgraphics Centurion XVI software and the analysis was performed with IBM-SPSS software.
3. Results and discussion
3.1. Incorporation of iron and AA
Table 2 presents the iron and AA content in the dehydrated sweet potato after the different treatments with and without ultrasound. The control dehydrated sweet potato (CT) presented an iron content of 2.15 ± 0.01 mg/100 g and an AA content of 63.61 ± 0.50 mg/100 g, values higher than those found by Amagloh et al. (2022), who reported an AA content in fresh sweet potato ranging from 3.040 to 16.698 mg AAE/100 g. Peruvian food composition tables indicated an even lower AA content of 0.80 mg/100 g was reported for dehydrated sweet potato; however, the iron values were 2.90 mg/100 g (María et al., 2017); which is in agreement with the findings of the present study. Both ultrasonic (US) and non-ultrasonic (NUS) treatments were effective in incorporating iron and AA into the sweet potato. Longer treatments resulted increased the content of both iron and AA content; however, ultrasonic frequency, as well as the interaction (frequency versus time), did not influence AA content; on the other hand, in the case of iron content, time and interaction were significant, as observed in Table 2.
According to Mashkour et al. (2018), the use of a 37 kHz frequency generated higher micronutrient incorporation compared to an 80 kHz frequency, due to the cavitation phenomenon induced by the US, the cavitational collapse produces the cellular rupture in the plant tissue, which allows the increased of cell permeability, resulting in better mass transfer. Lower US frequencies generate longer wavelengths, and longer compression cycles and produce a violent cavitational implosion, resulting in greater cell disruption. In contrast, Yu et al. (2016) testing three different frequencies (25, 45, and 100 kHz) to enrich peanuts with resveratrol, used US as a pretreatment, the optimal frequency was found to be 100 kHz. The difference in these results may be attributed in the different in the food matrix, pretreatment methods, and frequencies utilized.
The iron content was incorporated in greater quantity in the treatments with longer exposure times (Figure 2B), for instance (Table 2) the treatment at 37 kHz for 50 and 60 min, showed an iron content of 72.96 and 105.91 mg/100 g. This represents an increase of 3,293 and 4,826% with respect to the control, and at 80 kHz the values were 60.52 and 77.98 mg/100 g in both times showing an increase in iron content of up to 2,715 and 3,427%, respectively. These results were higher than those observed at the same times in NUS. Incorporating iron in sweet potato with US at 30 min allows to obtain values equivalent to those demonstrated in the twice the duration in NUS, this demonstrates the effectiveness of the application of US. The iron content shown in each treatment studied, supplements the recommended daily intake of iron for pregnant women, which recommendation requires the consumption of 23 to 27 mg of iron/day. Additionally, the dietary iron requirement for adolescents is also covered, which ranges varies from 8 to 15 mg/day, depending on age and sex (Ministerio de Salud [MINSA], 2016).
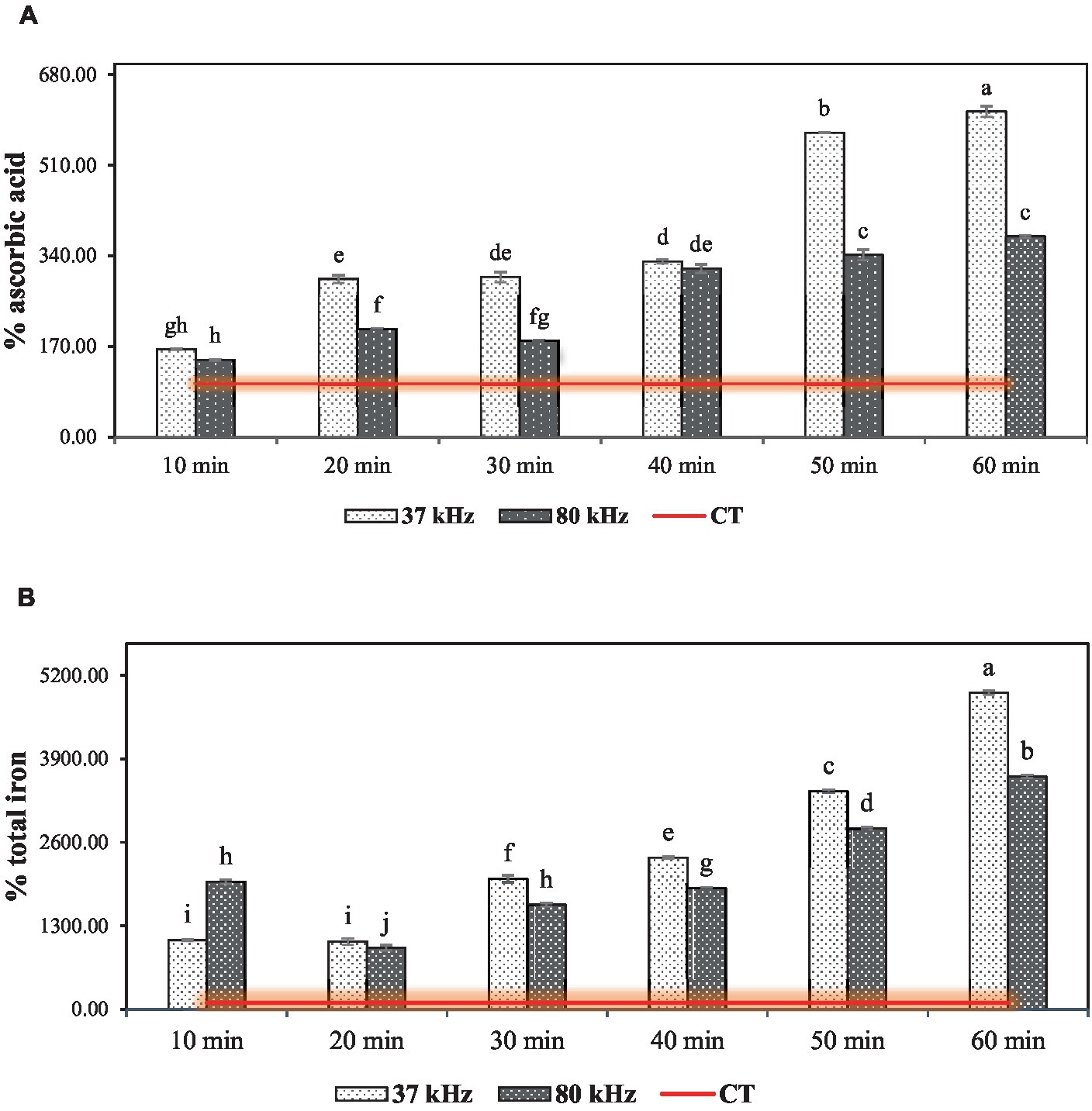
Figure 2. Ascorbic acid (A) and total iron (B) content expressed in percentage, using different times and frequencies of ultrasound treatment. CT, control sample. Values (mean ± SD) within each treatment with different letters differ significantly (p ≤ 0.05).
Currently, there are no precedents for incorporating iron into sweet potatoes; however, in other foods, it has been possible to incorporate iron and other micronutrients into other foods using the same technology. Carvalho et al. (2021) propose the iron fortification of pineapple chips by using microencapsulation, ethanol, ultrasound, and convective drying. The authors mentioned that the longer time (30 min) of pre-treatment in ethanol and US increased the iron content up to 1157.5%; however, compared to the pre-treatment in ethanol, the US treatment did not significantly improve the iron incorporation. Rojas et al. (2019) showed a similar result for the incorporation of iron and microencapsulated carotenoids in pumpkin and apple. The authors found that by applying US treatment for 30 min, an increase of more than 1,000% of iron incorporation was found and its application helped to improve the dispersion of ethanol microparticles, despite this, the US did not significantly affect the results compared to the treatment without US. This is consistent with Miano and Augusto (2018) found that the iron content in beans increases significantly at longer exposure times, they obtained an increase of 1418.6 mg/kg of iron during 510 min of processing.
The amount of AA incorporation in sweet potato depends significantly on the time factor, as shown in Table 2, which ranges from 93.32 to 152.73 mg AAE/100 g in NUS; US at 37 kHz from 103.71 to 392.65 mg AAE/100 g and US at 80 kHz from 91.82 to 235.70 mg AAE/100 g is observed; despite the frequency, factor did not evidence a significant statistical influence, at 37 kHz the highest AA ranges were observed (Figure 2A). Showing effective incorporation of AA from 63 to 517%, with respect to the control. In fact, 20 min of treatment was sufficient to exceed 60 min in NUS, achieving 50% higher AA. In similar studies, under the vitamin fortification approach in other food matrices, similar behavior was observed; for instance, Tiozon et al. (2021) incorporated folic acid (vitamin B) into rice using ultrasound as a pretreatment for 1, 3, and 5 min, found that longer sonication times resulted in greater incorporation and increased folic acid levels by 1,982% in brown rice and by 4,054 times in milled rice.; in addition, US improved the micronutrient retention capacity after cooking, by 93.53% (brown) and 86.52% (milled); however, the sonication time to be used should be evaluated according to the nature of the micronutrient, food matrix, among other factors. The incorporation of vitamin B5 in rice was study for different durations of 5, 15, 25, and 35 min; 25 min of sonication produced the greatest mass transfer by the rupturing of the cell walls (changes in cell microstructure), which provided an absorption of up to 140% more pantothenic acid than in the untreated sample; because a long time produced the gelatinization due to temperature variation (42–45°C) (Bonto et al., 2018).
3.2. Ultrasound-assisted fortification
The aim of the optimization of the process was to obtain the parameters that allow a greater increase in the iron and AA content, as shown in the surface response plot in Figure 3. As observed, the lowest frequency (37 kHz) and the longest exposure time (60 min) were the optimal parameters for the incorporation of iron and AA, obtaining an iron content of 105.91 ± 0.03, an increase of 4928.99% and an AA content of 392.65 ± 4.84, an increase of 610.65% compared to its initial content. The basis for these results, as mentioned above, is due to the increased cell permeability of the feed tissue as a result of the cavitation collapse caused by the ultrasonic treatment. Similar results were obtained by Oladejo and Ma (2016), in their study optimized the US-assisted osmotic dehydration process in sweet potato (Ipomoea batatas) using methodology response surface (MRS); the optimized values were the following, frequency of 33.93 kHz, sucrose concentration 35.69% and the exposure time was 30 min, this time was the maximum value, concluding that the use of US allowed more mass transfer and that the lower frequency favors the results.
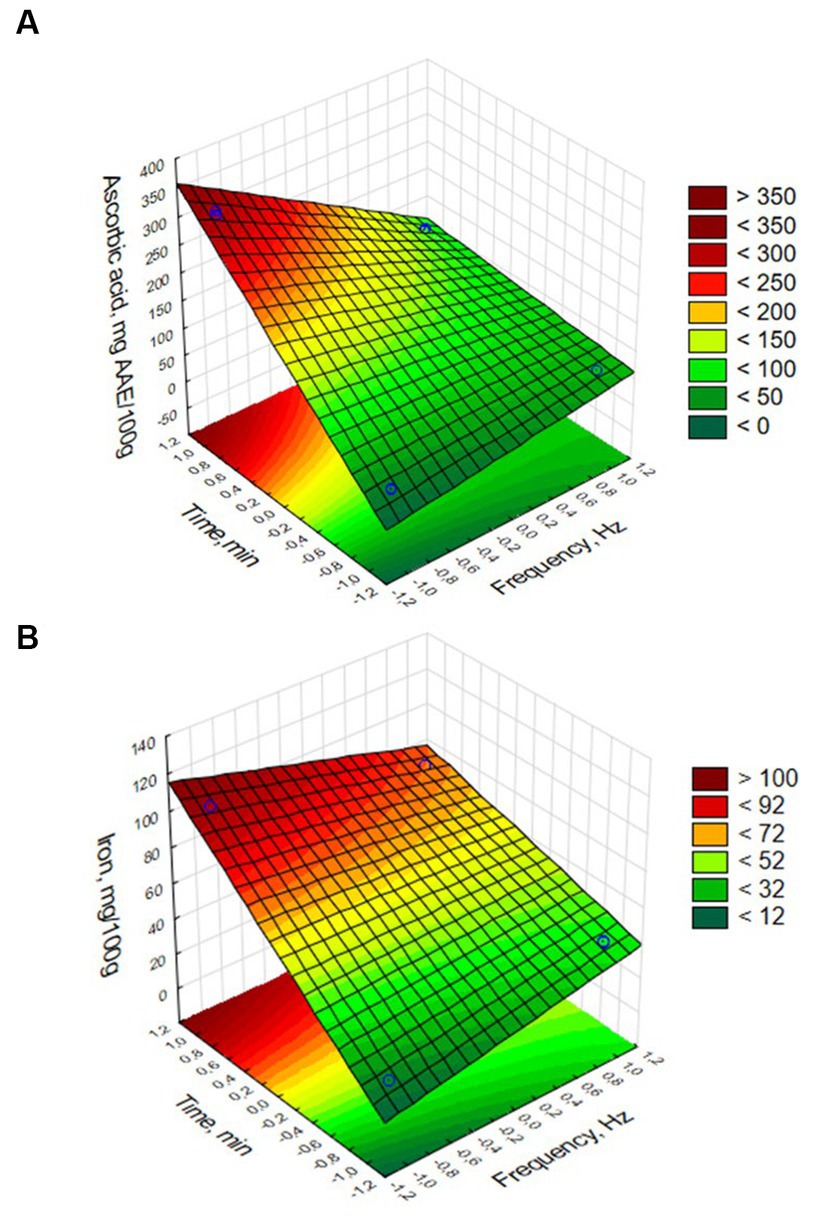
Figure 3. Surface response plot of frequency and time on AA (A) and iron (B) content in the ultrasound-treated sweet potato sample.
Similarly, Azarpazhooh et al. (2020) investigated the impregnation of grape pomace phenols in aloe vera using ultrasound-assisted osmotic dehydration (25 kHz, 200–400 W), and optimized the process using a MRS. The optimum values were 50% sucrose, 50°C, 59% amplitude, 20% grape pomace extract and 173 min exposure, with the maximum time value being 210 min. They study concluded that that a low frequency (25 kHz) with an exposure time of 173 min achieves a higher gain of solids, leading to increase the content and retention of phenolic compounds.
Sucheta et al. (2020), optimized the process of spice impregnation in black carrots assisted with US (37 kHz), they used a MRS design, whose optimum values were 8.18% NaCl, 4.30% spice mixture, and 14.47 min of ultrasound exposure. It should be noted that the maximum exposure time was 15 min, and the US improved mass transfer.
Mashkour et al. (2018) obtained different results, they optimized the process of iron fortification of potatoes by vacuum impregnation and US pre-treatment, the optimized values were; 37 kHz frequency and 24 min exposure time. The authors conclude that longer exposure time affect the nutritional content as they observed leakage of compounds from the potato; however, this is a side effect of the vacuum impregnation technique which was enhanced by the US. Similar results were reported by Maleki et al. (2020), implying that prolonged ultrasound exposure time damages carrot 303 microtissues by reducing mass transfer, the optimum values were 25 kHz frequency and 10 min.
4. Conclusion
The study introduces a novel approach to AA and iron fortification, achieved satisfactory results, showed that both US and NUS applications were effective for the incorporation of AA and iron in sweet potato (Ipomoea batatas). However, US treatments with longer exposure significantly influenced the content of AA and iron content. The frequency factor and the interaction (frequency vs. time) did not have a significantly influence on AA content. For iron content, the time factor and the interaction (time vs. frequency) had a significant effect. The optimized conditions of incorporation favored an increase of 4928.99% in iron content and 610.65% in AA; in comparison with the NUS treatment during the same time. It is concluded that, on a laboratory scale, the US application significantly increased the iron content from the minimum time of treatment.
Data availability statement
The raw data supporting the conclusions of this article will be made available by the authors, without undue reservation.
Author contributions
KP-S: conceptualization, experimentation, literature review, and writing the original draft. LR-F: experimentation, writing original draft, literature review, and data analysis. ZS: conceptualization, data analysis, supervision, data curation, and review and editing. EC: conceptualization, supervision, review and editing. ME-D: conceptualization, supervision, formal analysis, and review and editing.
Funding
This work was funded by the Universidad Nacional de Frontera (Sullana, Peru). Through the program “Research Projects with Competitive Funds”.
Acknowledgments
The authors thank the support of Universidad Nacional de Frontera and the Brazilian Agency National Council of Technological and Scientific Development (CNPq).
Conflict of interest
The authors declare that the research was conducted in the absence of any commercial or financial relationships that could be construed as a potential conflict of interest.
Publisher’s note
All claims expressed in this article are solely those of the authors and do not necessarily represent those of their affiliated organizations, or those of the publisher, the editors and the reviewers. Any product that may be evaluated in this article, or claim that may be made by its manufacturer, is not guaranteed or endorsed by the publisher.
References
Amagloh, F. C., Kaaya, A. N., Yada, B., Chelangat, D. M., Katungisa, A., Amagloh, F. K., et al. (2022). Bioactive compounds and antioxidant activities in peeled and unpeeled sweetpotato roots of different varieties and clones in Uganda. Future Foods 6:100183. doi: 10.1016/j.fufo.2022.100183
Andre, C. M., Burgos, G., Ziebel, J., Guignard, C., Hausman, J.-F., Felde, T., et al. (2018). In vitro iron bioaccessibility and uptake from orange-fleshed sweet potato (Ipomoea batatas (L.) lam.) clones grown in Peru. J. Food Compos. Anal. 68, 79–86. doi: 10.1016/j.jfca.2017.07.035
Azarpazhooh, E., Sharayei, P., and Ramaswamy, H. S. (2020). Optimization of ultrasound-assisted osmotic treatment of Aleo vera gel impregnated with grape pomace phenolic compounds using response surface methodology. Agric. Eng. Int. CIGR J. 22, 202–2012.
Bhargava, N., Mor, R. S., Kumar, K., and Sharanagat, V. S. (2021). Advances in application of ultrasound in food processing: a review. Ultrason. Sonochem. 70:105293. doi: 10.1016/j.ultsonch.2020.105293
Bonto, A. P., Camacho, K. S. I., and Camacho, D. H. (2018). Increased vitamin B5 uptake capacity of ultrasonic treated milled rice: a new method for rice fortification. LWT 95, 32–39. doi: 10.1016/j.lwt.2018.04.062
Bonto, A. P., Jearanaikoon, N., Sreenivasulu, N., and Camacho, D. H. (2020). High uptake and inward diffusion of iron fortificant in ultrasonicated milled rice. LWT - Food Science and Technology, 128. doi: 10.1016/j.lwt.2020.109459
Carvalho, G. R., Massarioli, A. P., Alvim, I. D., and Augusto, P. E. D. (2021). Iron-fortified pineapple chips produced using microencapsulation, ethanol, ultrasound and convective drying. Food Eng. Rev. 13, 726–739. doi: 10.1007/s12393-020-09259-4
Grozo, J. (2021). Costos de Producción para Actividad: agricultura, ganadería, caza y silvicultura en base a la Encuesta Nacional Agraria (ENA-2018). Lima: Instituto Nacional de Estadística e Informática.
FAOSTAT data (2017). Food and agriculture organization of the United Nations. Statistical database. http://www.fao.org/faostat/en/#data (accessed, March 2017).
Genevois, C., Flores, S., and de Escalada Pla, M. (2014). Effect of iron and ascorbic acid addition on dry infusion process and final color of pumpkin tissue. LWT 58, 563–570. doi: 10.1016/j.lwt.2014.03.020
Instituto Nacional de Estadística e Informática [INEI] (2023). Perú: Encuesta Demográfica y de Salud Familiar 2022 - Nacional y Departamental. Lima, Perú: INEI Available at: www.inei.gob.pe (accessed August 5, 2023).
Instituto Nacional de Salud [INS] (2020). Situación actual de la anemia: Prevalencia de anemia en niños menores de 6 a 35 meses según departamentos. Ministerio de Salud. Available at: https://anemia.ins.gob.pe/situacion-actual-de-la-anemia-c1 (accessed February 23, 2023).
Joshi, A., Prajapatii, U., Sethi, S., Arora, B., and Sharma, R. R. (2019). “Fortification in fresh and fresh-cut horticultural products”, in Fresh-Cut Fruits and Vegetables: Technologies and Mechanisms for Safety Control. Elsevier Inc. 183–204.
Latham, M. C., FAO (2002). Human nutrition in the developing world. Food and agriculture Organization of the United Nations Available at: https://www.fao.org/3/w0073s/w0073s10.htm#bm36x.
Maleki, M., Shahidi, F., Varidi, M. J., and Azarpazhooh, E. (2020). Hot air drying kinetics of novel functional carrot snack: impregnated using polyphenolic rich osmotic solution with ultrasound pretreatment. J. Food Process Eng. 43. doi: 10.1111/jfpe.13331
María, R. G., Iván, G.-S. P., and Cecilia, E. B. (2017). Tablas peruanas de composición de alimentos. 10 ma. Lima: Ministerio de Salud, Instituto Nacional de Salud Available at: www.minsa.gob.pe.
Mashkour, M., Maghsoudlou, Y., Kashaninejad, M., and Aalami, M. (2018). Effect of ultrasound pretreatment on iron fortification of potato using vacuum impregnation. J. Food Process. Preserv. 42. doi: 10.1111/jfpp.13590
Mason, T. J., Chemat, F., and Ashokkumar, M. (2015). Power ultrasonics for food processing. Power Ultrason. Appl. High Intens. Ultras., 815–843. doi: 10.1016/B978-1-78242-028-6.00027-2
Meilianti, S., John, C., Duggan, C., O’campo, L., and Bates, I. (2023). How can pharmacists contribute to anaemia management? A review of literature and exploratory study on pharmacists’ role in anaemia. Exp. Res. Clin. Soc. Pharm. 9:100231. doi: 10.1016/j.rcsop.2023.100231
Miano, A. C., and Augusto, P. E. D. (2018). The ultrasound assisted hydration as an opportunity to incorporate nutrients into grains. Food Res. Int. 106, 928–935. doi: 10.1016/j.foodres.2018.02.006
Ministerio de Salud [MINSA] (2016). Guía técnica: Guía de práctica clínica para el diagnóstico y tratamiento de la anemia por deficiencia de hierro en niñas, niños y adolescentes en establecimientos de salud de primer nivel de atención-RM No028-2015/MINSA. Available at: http://www.minsa.gob.pe/
Ministerio de Salud [MINSA] (2017). Norma técnica - manejo terapéutico y preventivo de la anemia en niños, adolescentes, mujeres gestantes y puérperas. Available at: http://www.minsa.gob.pe/.
Nyathi, M. K., Du Plooy, C. P., Van Halsema, G. E., Stomph, T. J., Annandale, J. G., and Struik, P. C. (2019). The dual-purpose use of orange-fleshed sweet potato (Ipomoea batatas var. Bophelo) for improved nutritional food security. Agric. Water Manag. 217, 23–37. doi: 10.1016/j.agwat.2019.02.029
Oladejo, A. O., and Ma, H. (2016). Optimisation of ultrasound-assisted osmotic dehydration of sweet potato (Ipomea batatas) using response surface methodology. J. Sci. Food Agric. 96, 3688–3693. doi: 10.1002/jsfa.7552
Rahman, M. M., Khan, M. M. R., and Hosain, M. M. (2007). Analysis of vitamin C (ascorbic acid) contents in various fruits and vegetables by UV-spectrophotometry. Bang. J. Sci. Indus. Res. 42, 417–424. doi: 10.3329/bjsir.v42i4.749
Renee, A. V., Zaucedo-Zuñiga, A. L., and De Ramos-García, M. (2018). Propiedades nutrimentales del camote (Ipomoea batatas L.) y sus beneficios en la salud humana. Revista Iberoamericana de Tecnología Postcosecha 19.
Rivera, I. C., Rivera, M. F., and Rivera, R. (2012). Deficiencia de hierro y su relación con la función cognitiva en escolares. Revista de Ciencia y Tecnología. doi: 10.5377/rct.v0i10.1063
Rojas, M. L., Alvim, I. D., and Augusto, P. E. D. (2019). Incorporation of microencapsulated hydrophilic and lipophilic nutrients into foods by using ultrasound as a pre-treatment for drying: a prospective study. Ultrason. Sonochem. 54, 153–161. doi: 10.1016/j.ultsonch.2019.02.004
Shubham, K., Anukiruthika, T., Dutta, S., Kashyap, A., Moses, J. A., and Anandharamakrishnan, C. (2020). Iron deficiency anemia: A comprehensive review on iron absorption, bioavailability and emerging food fortification approaches. Trends Food Sci. Technol. 99, 58–75. doi: 10.1016/j.tifs.2020.02.021
Sucheta,, Misra, N. N., and Yadav, S. K. (2020). Extraction of pectin from black carrot pomace using intermittent microwave, ultrasound, and conventional heating: Kinetics, characterization and process economics. Food Hydrocolloids 102. doi: 10.1016/j.foodhyd.2019.105592
Tiozon, R. N., Camacho, D. H., Bonto, A. P., Oyong, G. G., and Sreenivasulu, N. (2021). Efficient fortification of folic acid in rice through ultrasonic treatment and absorption. Food Chem. 335:127629. doi: 10.1016/j.foodchem.2020.127629
Tostado-Madrid, T., Benítez-Ruiz, I., Pinzón-Navarro, A., Bautista-Silva, M., and Ramírez-Mayans, J. A. (2015). Actualidades de las características del hierro y su uso en pediatría. Acta Pediátrica de México 36, 189–200. doi: 10.18233/APM36No3pp189-200
Yılmaz, F. M., and Ersus Bilek, S. (2018). Ultrasound-assisted vacuum impregnation on the fortification of fresh-cut apple with calcium and black carrot phenolics. Ultrason. Sonochem. 48, 509–516. doi: 10.1016/j.ultsonch.2018.07.007
Keywords: ultrasound, iron, ascorbic acid, fortified sweet potato, food processing
Citation: Purizaca-Santisteban K, Ruiz-Flores LA, Sócola Z, Chaves ES and Espinoza-Delgado MdP (2023) Ultrasound-assisted fortification of yellow sweet potato (Ipomoea batatas) with iron and ascorbic acid. Front. Sustain. Food Syst. 7:1193496. doi: 10.3389/fsufs.2023.1193496
Edited by:
Elliot Berry, Hebrew University of Jerusalem, IsraelReviewed by:
Flora Christine Amagloh, CSIR – Savanna Agricultural Research Institute, GhanaDewa Ngurah Suprapta, Udayana University, Indonesia
Copyright © 2023 Purizaca-Santisteban, Ruiz-Flores, Sócola, Chaves and Espinoza-Delgado. This is an open-access article distributed under the terms of the Creative Commons Attribution License (CC BY). The use, distribution or reproduction in other forums is permitted, provided the original author(s) and the copyright owner(s) are credited and that the original publication in this journal is cited, in accordance with accepted academic practice. No use, distribution or reproduction is permitted which does not comply with these terms.
*Correspondence: Milagros del Pilar Espinoza-Delgado, mpespinoza@unf.edu.pe