- 1CSIR-Crops Research Institute, Kumasi, Ghana
- 2Department of Agricultural and Biosystems Engineering, KNUST, Kumasi, Ghana
- 3Department of Plant Resources Development, CSIR College of Science and Technology, Kumasi, Ghana
Water availability for irrigation farming is one of the greatest challenges associated with the increasing spatio-temporal effects of climate change and variability on tomato production, especially in tropical regions. This study was conducted to demonstrate the combined effect of irrigation and nutrient management as a water-saving strategy to maximize nutrient and water productivity in tomato production. The research was conducted in a screen house at the CSIR-Crops Research Institute (CSIR-CRI), Kumasi, Ghana using the split-plot design in the 2020 and 2021 cropping seasons. The main plot consisted of a single full irrigation at 100% recommended crop water requirement and two deficit irrigation levels (75 and 50% of the crop water requirement). The subplots consisted of two nutrient amendments (inorganic fertilizer and organic compost) and a control (without any nutrient amendment). Data from the research was used to parameterize the DSSAT CCROPGRO model to simulate the interactive effect of irrigation and nutrient management on the yield of tomatoes. Plant height and stem girth did not have a specific influence on tomato yield, but the number of branches had a positive effect on tomato yield. The combined use of inorganic fertilizer and full irrigation was found to improve tomato yield up to 7691.4 and 9009.9 kg/ha whereas treatment with no fertilizer application at 50% deficit irrigation recorded the lowest tomato yield of 1423.9 and 1739.2 kg/ha in 2020 and 2021, respectively. For the two deficit irrigations (50 and 75% ETc), organic compost produced the highest tomato yield. Deficit irrigation recorded higher crop water productivity (CWP) compared to full irrigation. At 50% deficit irrigation, organic compost recorded the highest CWP of 4.54 kg/m3 in 2020 while inorganic fertilizer recorded the highest CWP of 5.52 kg/m3 in 2021. No fertilizer at full irrigation recorded the lowest CWP of 1.37 and 1.67 kg/m3 in 2020 and 2021, respectively. This study has revealed that deficit irrigation with organic compost has the same effect on yield and water productivity as full irrigation with inorganic fertilizer. The strong agreement observed between the measured and simulated yields under the different irrigation and nutrient management shows that the DSSAT CROPGRO tomato model can be used to simulate tomato fruit yield under future climate scenarios. However, the general overestimation of the measured tomato yield shows the limitations of the model to simulate the real-world complexity of cropping systems under controlled conditions. This calls for more research into crop system modeling in controlled environment agriculture.
1. Introduction
Tomato (Solanum lycopersicum L.) is one of the most extensively irrigated crops in the world (Arah et al., 2015). In irrigated cultivation of tomatoes in Ghana, farmers tend to use more water than the crop requires which reduces water use efficiency and causes fruits to rot as well as other water-borne diseases. Despite the investment made by tomato farmers to achieve high productivity, the sector continues to face challenges, particularly water and nutrient scarcity, which affect sustainable tomato production in Ghana relative to other countries (Melomey et al., 2019). Open-field farming exposes tomatoes to extreme weather conditions including high infestation from insects, pests, and birds as well as fluctuating temperatures, humidity and rainfall due to climate change. Climate change, in particular, has had severe impacts on tomato production causing heat injury, physiological abnormalities such as photosynthesis reduction, tomato growth, and development retardation, as well as a reduction in farmers’ livelihood as crop yields decline (Ayankojo and Morgan, 2020).
Tomato is currently the most common vegetable grown in greenhouses due to extensive studies that have proven that tomatoes grown in greenhouses produce higher quality fruits, which helps to reduce tomato imports. However, farmers in Ghana have identified water and nutrient resources as the main challenges to their adoption of controlled environment agriculture such as greenhouses (Forkuor et al., 2022). This is because these agricultural technologies do not depend on natural inputs such as rainfall and accumulated soil nutrients. Tomato cultivation in greenhouses or screen houses, requires prudent management of water and nutrient resources in order to maximize yield, especially in an era of increasing cost of inorganic fertilizer in the global markets as well as climate change impacts on water resources in tropical regions.
In recent years, controlled alternate partial root-zone irrigation or partial root-zone drying, as well as regulated deficit irrigation are practiced as climate change adaptation measures for tomato production to maximize water and nutrient productivity (Kumar et al., 2017). Deficit irrigation causes a progressive increase in crop water stress by reducing the amount of water provided uniformly. This approach is increasingly utilized to increase fruit crop water use efficiency. A study on a global meta-analysis of the responses of different vegetables to deficit irrigation in 30 countries found that reducing irrigation levels from full irrigation to 35% crop water requirement reduced yield by 50% whereas water productivity improved by up to 30% (Singh et al., 2021).
In tomato production, deficit irrigation techniques were found to have the greatest potential to increase water use efficiency in areas with limited water availability (Al-Ghobari and Dewidar, 2018). Song et al. (2016) also found that irrigation with a water deficit index is more profitable than total irrigation when water is a constraint to agricultural production. A detailed study of modeling the effect of deficit irrigation on fruit quality at various development stages of tomatoes under greenhouse conditions revealed interesting results (Chen et al., 2014). The study found that tomato plants were more sensitive to deficit irrigation at flowing, fruiting, and fruit ripening stages than initial growth stages. Also, fruit quality parameters such as reducing sugar, sugar/acid content ratio, and Vitamin C were more sensitive than other quality parameters such as total soluble solids, organic acids, firmness and color index (Chen et al., 2014). Nonetheless, several research gaps still exist in deficit irrigation studies. These include but are not limited to (i) can deficit irrigation sustain tomato yield at a comparable level to full irrigation? and (ii) does deficit irrigation improve crop water productivity?
Farmers are also experiencing a decrease in soil fertility status (Zhang et al., 2017). Inorganic fertilizers work faster; however, their long-term sustenance is a problem (Aryal et al., 2021). Soil quality and productivity can be improved by implementing effective management practices that can improve tomato yield and reduce chemical fertilization. The current plant nutrition regimes seek to improve crop system performance by providing the crops with optimal nutrients but do not decrease soil nutrient loss nor enhance the sustainability of agricultural systems (Mikkelsen et al., 2012). There is a close link between water and nutrient use in crop production, when the water supply is adequate, plants can make maximum use of the least nutrient and vice versa. The task of boosting tomato production while using less water and other inputs such as nutrients, will not only help sustain production but would also reduce input (water and nutrient) costs, increase the incomes of farmers and improve the food sovereignty of the country.
Several authors have studied the interactive effects of nutrients and water on tomato growth and yield. Rahman and Zhang (2018), for instance, found that optimum plant nutrition enables plants to withstand water stress. Ullah et al. (2021) studied the effects of deficit irrigation and reduced nitrogen on growth and root morphology in soilless media. They found that an increase in water and nitrogen supply resulted in an increase in photosynthesis and therefore biomass, which resulted in increased tomato fruit yield and irrigation water use efficiency. Al-Selwey et al. (2021) also studied the effect of deficit irrigation on total yield as well as fruit physical and nutritional characteristics of four tomato genotypes and found that a reduced crop water requirement or crop evapotranspiration (50% ETc) significantly increased nutritional value for two of the tomato genotypes. The same study also identified 75% ETc at the fruiting stage to be optimal, which did not decrease the total yield of the crop. However, these studies did not follow up to simulate and analyze the potential interactive effects under future climate scenarios and their implication for tomato production, particularly in tropical regions.
For higher productivity, economic returns, and environmental quality priorities, various technologies that improve water use efficiency will need to be matched by real-time data to support future predictions for improved and sustainable production. Research on climate change’s impact on agriculture is supported by crop models, to simulate crop production and economic balance under various climatic and soil management scenarios (Bationo et al., 2012). The Decision Support System for Agro-Technology Transfer (DSSAT) model predicts crop growth, development, and yield in response to variations in meteorological conditions, soil qualities, and management approaches (Jones et al., 2003; Hoogenboom et al., 2019, 2021). The DSSAT Cropping Systems Models (CSMs) have been widely used for different applications by researchers worldwide, which have also served as major data sources for Intergovernmental Panel on Climate Change (IPCC) assessments for agriculture (Gitay et al., 2001; Easterling et al., 2007). By using modern computational instruments, CSMs can increase the knowledge gained from field experiments to simulate crop responses quickly with a lower cost under a large range of experimental conditions for different crops (e.g., Ventrella et al., 2012; Thorp et al., 2014; Chisanga et al., 2015; Adnan et al., 2017; Oteng-Darko et al., 2020). The CROPGRO simulation model of the DSSAT CSM suite is used in this research to assess the combined effect of irrigation and fertilizer management on the growth and yield of the tomato crop under controlled environment agriculture conditions.
The study therefore aims at investigating the interactive effects of different deficit irrigation regimes and soil nutrient amendments on tomato production and to go further to evaluate the performance of crop models in simulating the effects of these interactions on future climate scenarios using measured field data from controlled environment agriculture as benchmark scenarios. The main research questions this study seeks to answer include; (i) what is the combined effect of deficit irrigation and nutrient amendments on tomato growth and yield? (ii) what is the combined effect of deficit irrigation and nutrient amendments on tomato crop water productivity? and (iii) how effective is the DSSAT CROGRO Tomato model to simulate tomato yield under controlled environment agriculture conditions. Simulating tomato yield in controlled environments such as greenhouses using measured climate, soil, and crop data can help optimize water and nutrient efficiency since farmers can control the use of these resources in such agricultural environments and can equally be extended to open-field agriculture if all conditions are met. The results from the simulations could also help direct future research and build optimized recommendations and strategies for current and future water and nutrient use in tomato production.
2. Materials and methods
2.1. Study area and experimental design
The experiment was conducted in a screen house at the research fields of the CSIR-Crops Research Institute, Fumesua, Kumasi, located in the forest agroecological zone of Ghana (6.7177° N, 1.5317° W). The experiment was carried out in the 2020 and 2021 cropping seasons. The meteorological conditions for the two seasons were different. The average temperatures in the screen house were 36°C and 30°C, with average humidity levels of 52 and 68% in 2020 and 2021, respectively. A split-plot experimental design was used for this study. The main plots were allocated to the three irrigation schedules: 50% crop water requirement—ETc (S1), 75% ETc (S2) and full irrigation or 100% ETc (S3). The subplot treatments consisted of three different soil amendments [NPK 15-15-15 inorganic fertilizer (IF) and organic compost (OC)] as nutrients, applied at the same N rate of 90 kg N/ha as well as control subplot treatments, where no fertilizer (NF) was applied. The design produced nine (9) treatments which were replicated thrice, consisting of three potted plants per each replicate. The treatments were randomized across replicates. Table 1 presents details of the treatment combinations of three irrigation schedules (S1, S2, and S3) and three nutrient amendments (IF, OC, and NF) and their acronyms for this study.
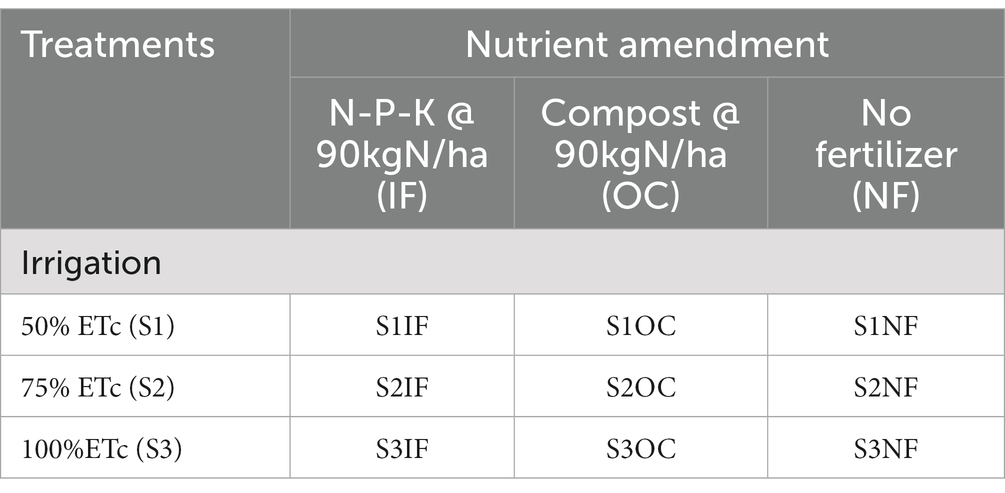
Table 1. Details of the deficit irrigation and nutrient amendment treatment combinations for the experimental plan.
2.2. Agronomic practices
Seeds of the Pectomech tomato variety were nursed in two big bowls in the screen house. The seedlings started to emerge within 3–5 days after the nursery with a germination rate of approximately 84% for the two seasons. The germinated seedlings were transplanted into the buckets filled with sterilized black soil in the screen house 3 weeks after nursing. Three tomato seedlings were planted per bucket. Staking was done to provide support to the tomato stems. Sampyrifos insecticide was used to control whiteflies in the screen house. Weeds were controlled by handpicking.
ACARP© Compost fertilizer was used for the OC treatments which involved the split application of compost. The first application was done 2 weeks before transplanting and the second application was done 10 days after transplanting. Compost was split and applied at 90 kg N/ha for both cropping seasons. It has been found that only 50% of the available nutrients in compost are usually available to the crop during the growing season (Sayara et al., 2020). In order not to disadvantage the crops fertilized with compost, we doubled the amount of compost used to avail 100% of the N requirement of the crop during the growing season. This was done to make more nutrients in the compost available to the crop during the growing season depending on water availability. The compost was bunded at a depth of about 10–15 cm and 5 cm away from the plant within the root zone. The IF treatment was split into halves with the first application occurring 10 days after transplanting while the second application was done at 50% flowering. The N-P-K fertilizer was banded at an application depth of about 15 cm.
2.3. Irrigation scheduling
The CROPWAT 8.0 model developed by the FAO Land and Water Development Division was used to estimate crop water and irrigation requirements (ETc) based on location-specific climate, soil, and crop information (FAO, 2002). The climate data used included monthly means of the minimum and maximum temperatures, humidity, wind, and sunshine hours. The crop data included crop name and potential variety height, crop coefficient values (Kc) for initial, mid, and late seasons, the growth duration for initial, development, mid and late seasons, the critical depletion factor, and the yield response (Table 2). The depletion factor represents the fraction of total available water (TAW) that a crop can extract from the root zone without suffering water stress. These values usually vary from 0.3 for shallow-rooted crops at high rates of ETc (>8 mmd−1) to 0.70 for deep-rooted crops at low rates of ETc (<3 mmd−1). A value of 0.5 is commonly used for many crops (Colaizzi et al., 2017). We used values between 0.3 and 0.5 for initial and late seasons (Table 2). The yield response factor (Ky) which quantifies the response of a crop’s yield to water, relates relative yield decrease (1 – Ya/Ym) to relative evapotranspiration deficit (1 – ETa /ETc), where Ya is the actual yield (corresponding to ETa) [kg/ha], Ym is the maximum theoretical yield (corresponding to ETc) [kg/ha], ETa is the actual crop evapotranspiration and ETc is the potential crop evapotranspiration, and thus the crop water requirement. The crop data used in the CROPWAT 8.0 model are presented in Table 2.
Site-specific soil data (Table 3) were also uploaded for use in the CROPWAT 8.0 model. The experimental soil was mixed extensively and sterilized before transferring to cylindrical shape pots 50 cm deep and 30 cm diameter for the potted experiment. Each pot was then filled with a weight of 8 kg of sterilized soil. Total available soil moisture was determined as the difference between field capacity and wilting point. Field capacity and permanent wilting point were determined in the laboratory using the retention curve method. An infiltration test using a double-ring infiltrometer was carried out to determine the maximum infiltration rate. Since rooting was restricted within the pots, the maximum rooting depth was taken as the height from the potted soil surface to the base of the pot. At planting, pots were irrigated, hence initial soil moisture depletion was assumed to be zero.
The water balance Equation (1) was used to calculate evapotranspiration (ET):
2.4. Data collection
Data collection was initiated 21 days after transplanting and subsequently, weekly until the final harvest. Three plants in each treatment were tagged for data collection. Plant height was measured from the base of the plant just above the soil surface to the tip of the longest stem with a calibrated rule. Stem girth was similarly taken from the three tagged plants using a vernier caliper. Total number of branches were counted on the tagged plants. Number of days to 50% flowering was recorded when more than half of the total number of plants flowered per the treatment. This was useful for the application of the second dose of the N-P-K inorganic fertilizer.
Harvest data was collected from the three tagged plants. Data on fruit weight per plant was collected and used to estimate fruit yield per hectare. Fruit weight per plant was determined by weighing the total number of fruits harvested in all subsequent harvests for the three tagged plants per treatment combinations and the average is taken to represent fruit weight per plant. The fruit yield per hectare was then estimated by multiplying fruit weight per plant by the number of plants per hectare, which is 33,333 plants for the Pectomech tomato variety.
Crop Water Productivity (CWP) was estimated as the ratio of the total fruit yield to the total amount of water used throughout the entire growth period as shown in Equation (2).
2.5. Parameterization of the DSSAT CROPGRO model
The CROPGRO simulation model of the DSSAT CSM suite was used to simulate the measure of fruit yield for the two cropping seasons, taking into account the different irrigation schedules and nutrient amendments. Local soil and weather data were used to calibrate the soil (X-Build) and weather (Weatherman) modules of the DSSAT CROPGRO model (version 4.7.5) respectively. Historical weather data for the period 2000–2020 were obtained from the Ghana Meteorological Agency, Accra. This included relative humidity, minimum and maximum temperatures, rainfall, wind speed and direction, solar radiation, and sunshine hours. The historical weather data was input into the Weatherman model of DSSAT to be recalled during simulations.
About 100 g soil sample was collected and analyzed for soil physical and chemical properties at the Laboratory of the Department of Crop and Soil Sciences, Faculty of Agriculture, KNUST, Kumasi. The soil was analyzed for exchangeable bases and acidity, Phosphorous, percent organic carbon and matter, pH and particle size distribution (Table 4). Particle size distribution of percentage sand, silt and clay content was used to estimate the soil texture, which was identified as sandy loam soil (Table 4). Soil information was input into the S-Build module of DSSAT and recalled for all simulations pertaining to the experiments undertaken. We refer readers to Hoogenboom et al. (2019, 2021) and Jones et al. (2003) for a more complete description and application of the DSSAT and the Cropping System Model.
For validation purposes, the model outputs were tested using a Minimum Data Set (MDS) sourced from the experiments carried out at the CSIR—Crops Research Institute. The minimum crop data set included fruit yield, biomass, plant height, leaf area index and stem girth collected from the stress-free experiment conducted in 2019 under similar environmental conditions as this current research. The validation employed one-year weather data from 2019. The genetic coefficient of the Pectomech tomato cultivar was derived using the trial-and-error method as described by Thirumeninathan et al. (2021). After every trial-and-error simulation run, adjustment was made to match the observed crop phenology and yield with the simulated values. This was done to make the calibrated genetic coefficient lie within the predefined limits for the cultivar.
2.6. Statistical analysis
Data collected on the growth parameters (plant height, stem girth and number of branches), and productivity data (fruit yield and crop water productivity) were subjected to ANOVA (analysis of variance) using the LSD.test function of the Agricole package in the R-studio statistical software (version 4.0.2). Means separation was done using the least significant difference (LSD) at 5% significance level (p < 0.05), thus, 95% confidence level.
To assess the overall performance of the DSSAT CROPGRO model to simulate the tomato yield, the following statistical and model performance measures were used: Mean Bias Error (MBE), Root Mean Square Error (RMSE), Index of Agreement (d), and the RMSE—Observation Standard Deviation (RSR) (Chai and Draxler, 2014; Oteng-Darko et al., 2020). The Nash-Sutcliffe model efficiency score (Nash and Sutcliffe, 1970) between the measured and simulated fruit yields was also used to evaluate the overall model performance.
Suppose m is the number of data points; where is the measured yield values, is the simulated yield values, is the mean measured yield value, and j = 1, …, m:
3. Results
3.1. Effects of deficit irrigation and nutrient amendment on the growth parameters
3.1.1. Plant height
Figure 1 presents the trends of average tomato plant heights from 4 to 12 weeks after transplanting at 2 weeks intervals for the treatment combinations of deficit irrigation and nutrient amendments for the two seasons. The bars represent the least significant difference (LSD) values at 95% confidence interval, above which the differences in the mean plant height values were considered statistically significant. The trends show a systemic increase in plant height for both seasons for all treatment combinations. Tomato plants were generally higher from the start of data collection (4th week after transplanting) for the 2021 cropping season but were higher at harvest (12th week after transplanting) for the 2020 cropping season. There were significant differences (p < 0.05) in plant height between the different treatment combinations for each week of data collection for the 2020 cropping season (Figure 1A). For the 2021 cropping season, there was a significant difference (p < 0.05) in plant height between the different treatment combinations for only the 4th week after transplanting (Figure 1B).
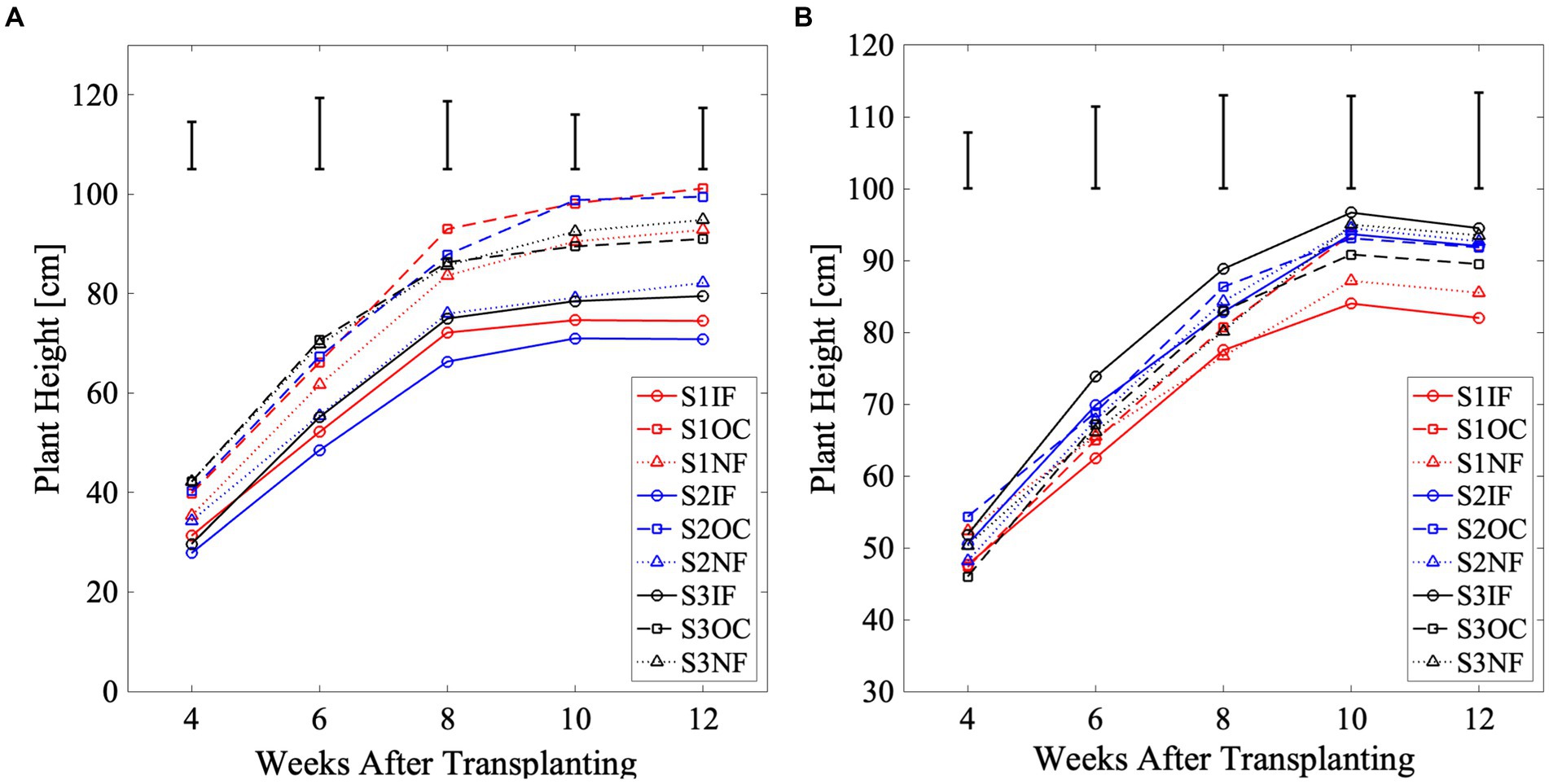
Figure 1. Average plant height of tomatoes for the different treatment combinations for the two seasons (A) 2020 and (B) 2021. Bars indicate LSD (p < 0.05) for each week of data collection.
Tomatoes cultivated under full irrigation schedule with organic compost (S3OC) and no fertilizer (S3NF) recorded significantly, the highest plant height for the 4th and 6th week after transplanting during the 2020 cropping season. Treatments with organic matter under all three irrigation schedules then recorded the highest plant height from the 8th to 12th week after transplanting in 2020, though the mean values were not significantly different from treatments with no fertilizer under all three irrigation schedules. For the 4th week after transplanting, where the mean differences recorded significant differences for the 2021 cropping season, treatment combination of organic compost and 75% ETc deficit irrigation (S2OC) recorded significantly higher plant height than the same nutrient amendment under full irrigation (S3OC). There were however, no significant differences with the other treatment combinations. Treatments with inorganic fertilizer under all three irrigation schedules consistently recorded significantly lowest plant height among the treatment combinations for all weeks of data collection for the 2020 cropping season. However, this treatment combination consistently recorded the highest plant height between the 6th and 12th weeks after transplanting for the 2021 cropping season, though this was not significantly different from the other treatment combination.
3.1.2. Stem girth
The growth trends for the stem girth also showed systemic increases for the 2020 and 2021 cropping seasons under all treatment combinations (Figure 2). Stem girths were generally similar from the start of data collection (4th week after transplanting) for both cropping seasons but were bigger at harvest (12th week after transplanting) for the 2020 cropping season. There were significant differences (p < 0.05) in the number of branches between the different treatment combinations for each week of data collection during the 2020 cropping season and other weeks of data collection during the 2021 cropping season except the 8th week after transplanting. For the 2020 cropping season, treatments with inorganic fertilizer and organic compost under all three irrigation schedules recorded significantly bigger stem girths. The treatment with no fertilizer under all three irrigation schedules recorded the least stem girths (Figure 2A). For the 2021 cropping season, treatments with inorganic fertilizer under full irrigation (S3IF) and 75% ETc deficit irrigation (S2IF) recorded significantly bigger stem girths, whereas organic compost under all three irrigation schedules recording significantly smallest stem girths (Figure 2B). It can also be observed that in 2021 (Figure 2B), stem girths were consistently bigger for treatments with inorganic fertilizer (continuous lines), followed by treatments with no fertilizer (dotted lines) and then treatments with organic fertilizer (dashed lines) under the irrigation schedules for all weeks of data collection.
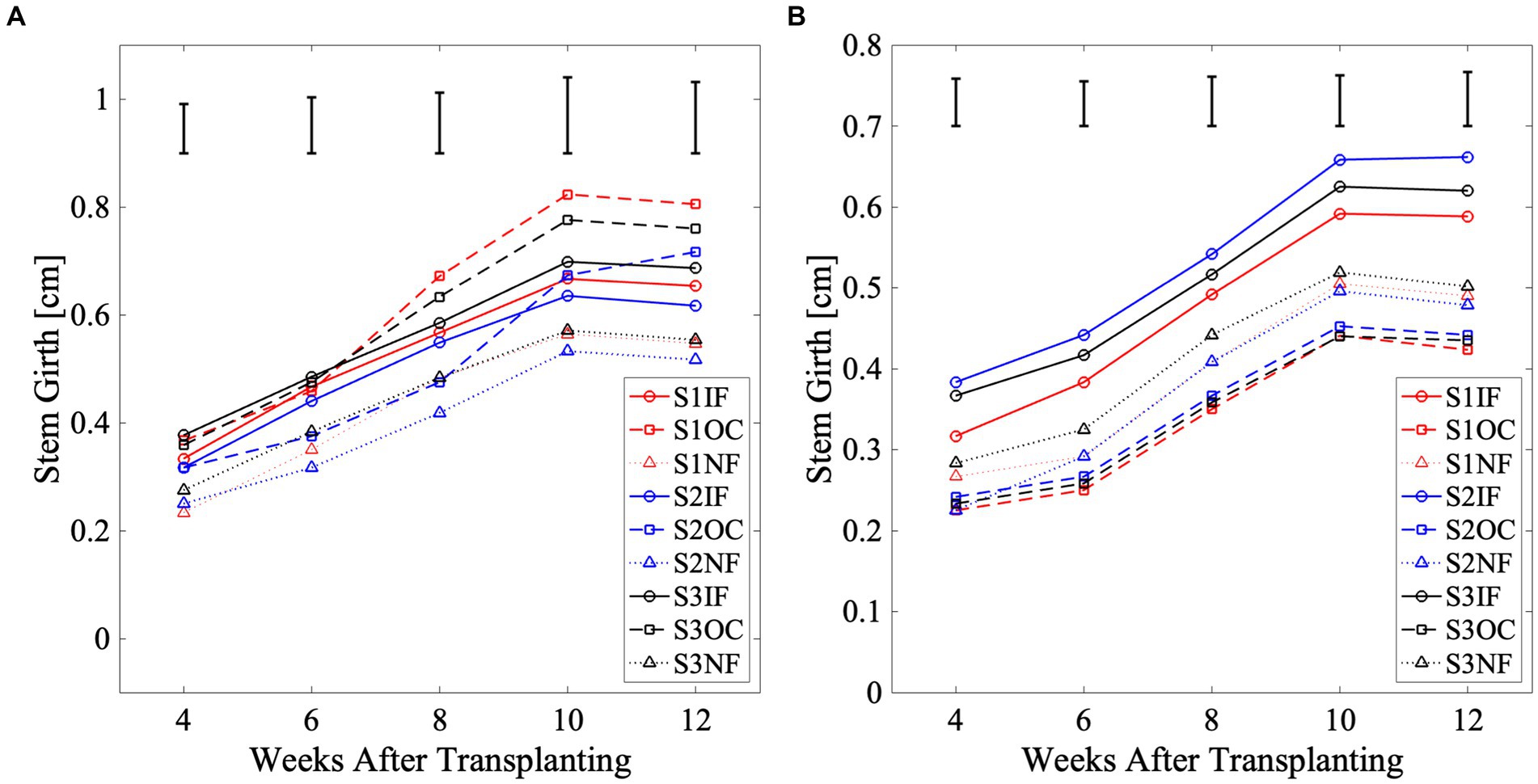
Figure 2. Average stem girth of tomatoes for the different treatment combinations for the two seasons (A) 2020 and (B) 2021. Bars indicate LSD (p < 0.05) for each week of data collection.
3.1.3. Number of branches
Again, the trends of the number of branches showed a systemic increase for both the 2020 and 2021 cropping seasons under all treatment combinations (Figure 3). A number of branches was consistently higher for 2021 compared to 2020 throughout the data collection period. There were significant differences (p < 0.05) in the number of branches between the different treatment combinations for each week of data collection for both cropping seasons. For the 2020 cropping season, treatments with organic compost under 50% ETc deficit irrigation (S1OC) recorded the highest number of branches, but not significantly higher than the other irrigation schedules (75 and 100% ETc) as well as treatments with inorganic fertilizer under both full irrigation (S3IF) and 50% ETc deficit irrigation (S1IF). Treatments with no fertilizer under full irrigation (S3NF) and 50% ETc deficit irrigation (S1NF) consistently recorded the lowest number of branches. For the 2021 cropping season, treatments with inorganic fertilizer under all three irrigation schedules recorded significantly highest number of branches, whereas treatments with organic compost and no fertilizer under 50% ETc deficit irrigation (S1OC and S1NF) recorded the significantly lowest number of branches. Treatments with organic compost and no fertilizer under full irrigation and 75% ETc deficit irrigation consistently recorded intermediary number of branches.
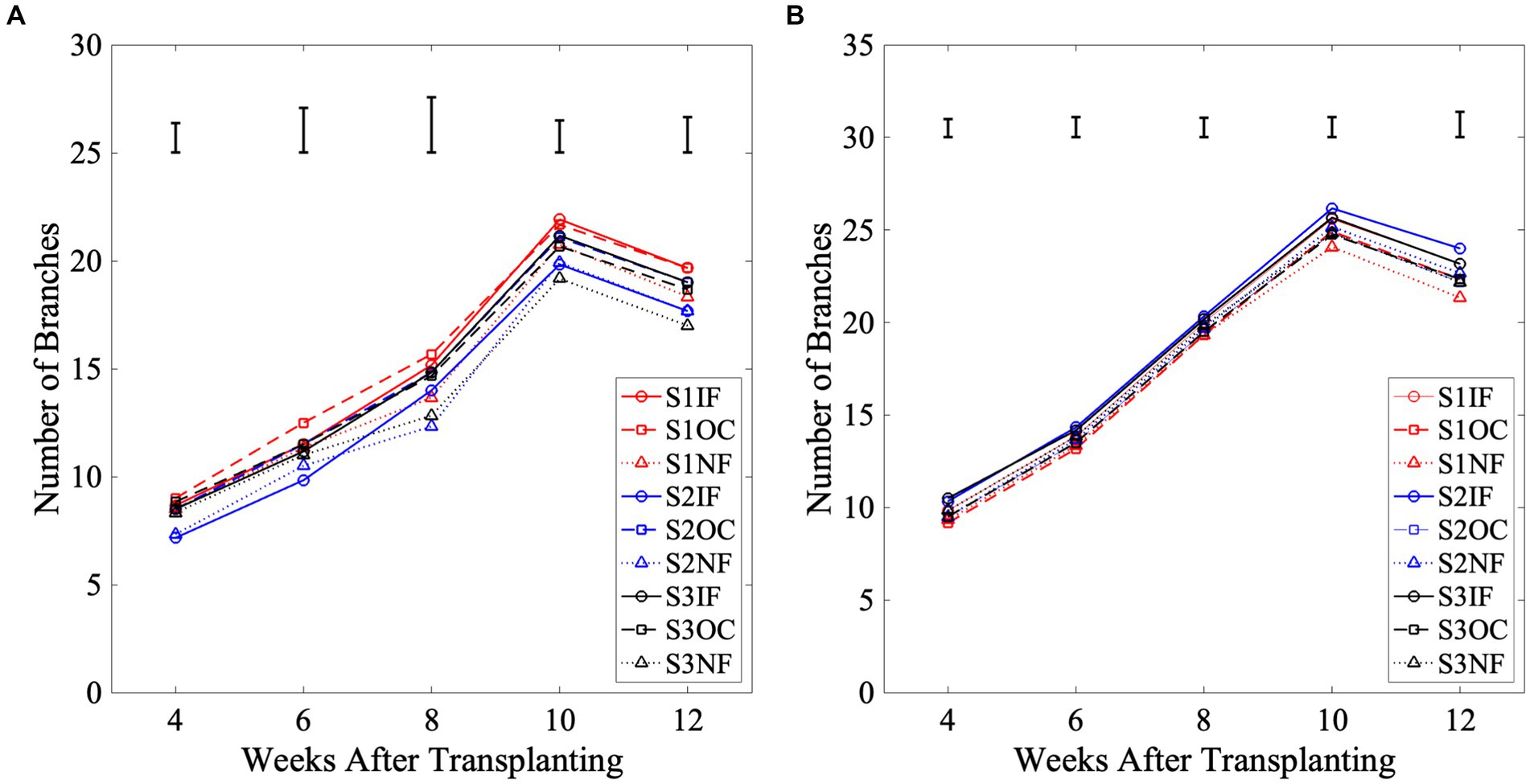
Figure 3. Average number of branches of tomatoes for the different treatment combinations for the two seasons (A) 2020 and (B) 2021. Bars indicate LSD (p < 0.05) for each week of data collection.
3.2. Effects of deficit irrigation and nutrient amendments on productivity parameters
Generally, fruit yield per hectare and crop water productivity was higher for the 2021 cropping season compared to the 2020 cropping season under all treatment combinations (Table 5). Tomato plants cultivated under the treatment combination of full irrigation and inorganic fertilizer (S3IF) produced the highest mean yield per hectare for both the 2020 and 2021 cropping seasons (7691.4 and 9009.9 kg/ha, respectively). Plants cultivated under treatment combination of full irrigation and organic compost (S3OC) recorded the second-highest yield for both cropping seasons (6297.5 and 7892.7 kg/ha, respectively) whereas plants cultivated with no fertilizer application under 50% ETc deficit irrigation (S1NF) recorded the lowest yield for both cropping seasons (1423.9 and 1739.2 kg/ha, respectively) (Table 5). There were no significant differences between the yields from inorganic fertilizer (IF) and organic compost (OC) nutrient amendment treatments under 50% ETc and 75% ETc deficit irrigation (S1 and S2) for both cropping seasons.
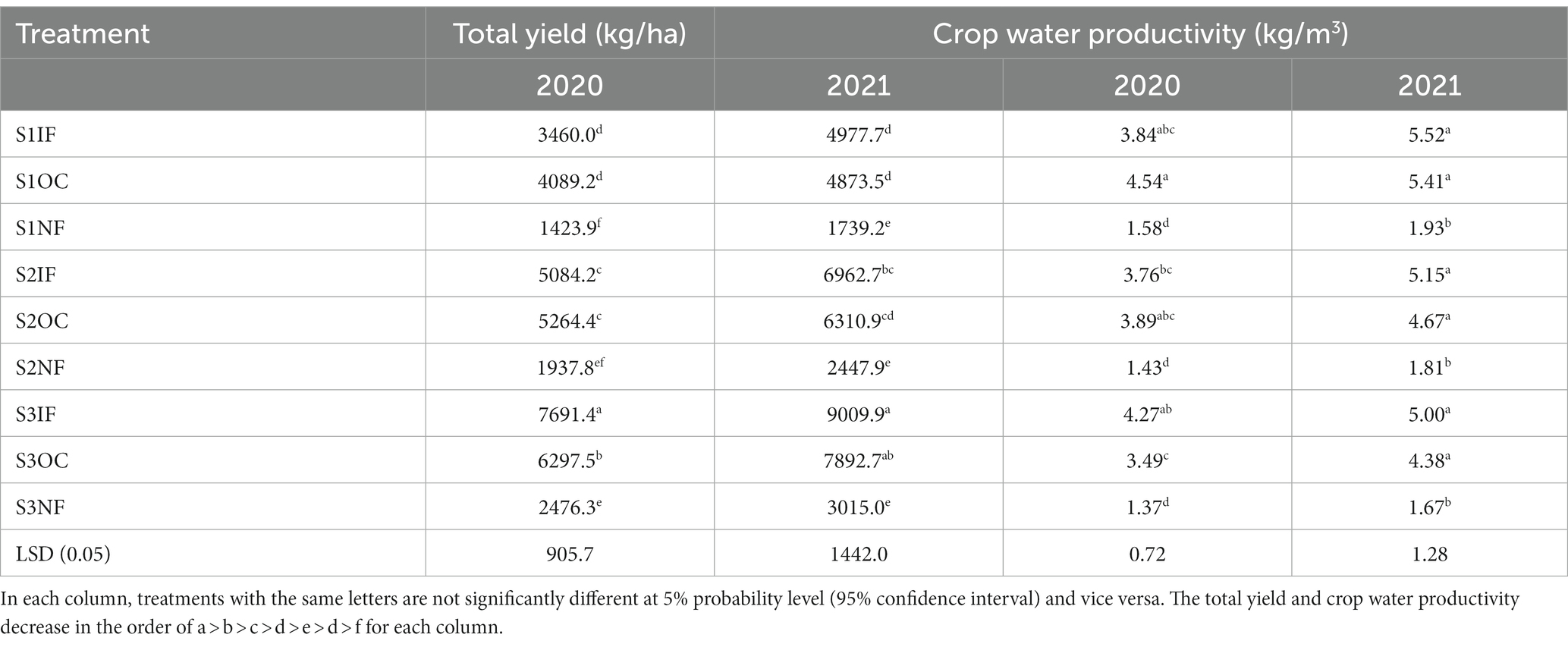
Table 5. Summary of mean fruit yield and crop water productivity for the treatment combinations for the two cropping seasons.
For the 2021 cropping season, treatments with no fertilizer statistically producedthe same mean yield under all three irrigation schedules. For both cropping seasons, tomato plants cultivated under full irrigation (S3) produced the highest fruit yield per hectare for all nutrient amendment treatments followed by 75% ETc deficit irrigation schedule (S2) with 50% ETc deficit irrigation (S1) producing the least yield (Table 5). Nutrient amendment with inorganic fertilizer (IF) also produced the highest fruit yield for all three irrigation schedules, which were not significantly different from mean yield values for treatments with organic compost except for under full irrigation schedule (S3) for 2020. Treatments with no fertilizer application recorded the least fruit yield under all deficit irrigation schedules for both cropping seasons.
The total volume of water supplied to each plant for the three irrigation schedules S1, S2, and S3 were 27.039, 40.559, and 54.079 liters, respectively. In 2020, treatments with organic compost under 50% ETc deficit irrigation (S1OC) recorded the highest crop water productivity (4.54 kg/m3), with inorganic fertilizer under full irrigation (S3IF) recording the second-highest crop water productivity (4.27 kg/m3). There was, however, no significant difference between these two treatment combinations. In 2021, treatments with inorganic fertilizer under 50% ETc deficit irrigation (S1IF) recorded the highest crop water productivity (5.52 kg/m3), with organic compost under 50% ETc deficit irrigation (S1OC) recording the second-highest crop water productivity (5.41 kg/m3). There was no significant difference between these two treatment combinations, as well as with the other two irrigation schedules (S2 and S3) with organic compost and inorganic fertilizer nutrient amendments. Meanwhile, treatments with no fertilizer application under full irrigation (S3NF) produced significantly, the least crop water productivity, of 1.37 and 1.67 kg/m3 for the 2020 and 2021 cropping seasons, respectively (Table 5).
Deficit irrigation of 50% ETc (S1) produced the highest crop water productivity under organic compost (OC) and no fertilizer (NF) treatments for the 2020 cropping season, and under all nutrient amended treatments for the 2021 cropping season followed by 75% ETc deficit irrigation (S2) with full irrigation (S3) producing the least water productivity (Table 5). For inorganic fertilizer (IF) treatments, full irrigation (S3) produced the highest crop water productivity for the 2020 cropping season. Again, treatments with no fertilizer application recorded the least crop water productivity under all irrigation schedules for both cropping seasons. There was no significant difference between crop water productivity for treatments with inorganic fertilizer (IF) and organic compost (OC) under all three irrigation schedules. They were however, significantly higher than treatments with no fertilizer (NF) under all three irrigation schedules.
3.3. Performance evaluation of the DSSAT CROPGRO model
3.3.1. Simulating the effects of deficit irrigation and nutrient amendment on fruit yield
Fruit yields were generally higher in the 2021 cropping season compared to the 2020 cropping season (see Table 5). As stated earlier, full irrigation (S3) recorded the highest fruit yield followed by 75% ETc deficit irrigation (S2) with 50% ETc deficit irrigation (S1) recording the lowest fruit yield for all nutrient amendments. Organic compost (OC) recorded higher fruit yield than inorganic fertilizer (IF) under both 50% ETc (S1) and 75% ETc (S2) deficit irrigation, whiles inorganic fertilizer recorded higher fruit yield than organic compost under full irrigation (S3). Treatments with no fertilizer application (NF) consistently recorded the least fruit yield under the irrigation schedules. The DSSAT CROPGRO model adequately captured all these dynamics in the measured data (Figure 4).
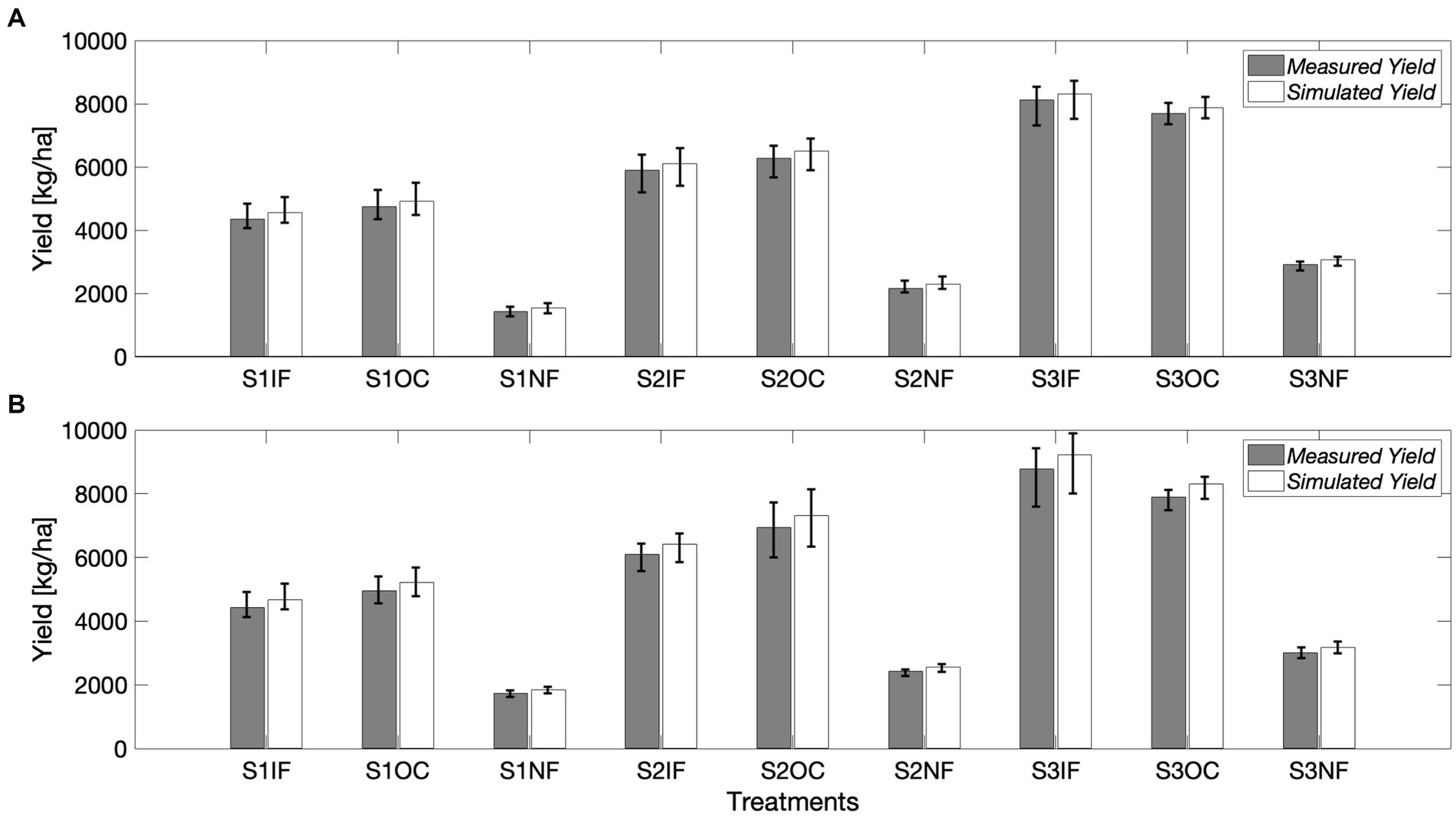
Figure 4. Mean tomato fruit yields as measured from the experiment and simulated using the DSSAT CROPGRO model for the different treatment combinations for the two seasons (A) 2020 and (B) 2021. Standard error bars are also indicated.
However, the model generally overestimated the measured fruit yield for both cropping seasons (Figure 4). This overestimation was higher for the 2021 cropping season compared to the 2020 cropping season for all treatment combinations. For 2020, model overestimation was highest for 75% ETc deficit irrigation (S2) followed by full irrigation (S3), whereas full irrigation recorded higher overestimation than 75% ETc deficit irrigation in 2021. Deficit irrigation with 50% ETc (S1) recorded the lowest overestimation for both cropping seasons. In terms of nutrient amendments, treatments with inorganic fertilizer (IF) recorded higher overestimation than treatments with organic compost (OC) in 2020, with the opposite dynamics in 2021. Treatments with no fertilizer (NF) recorded the lowest overestimation for both cropping seasons.
For 2020, highest model overestimation of 218.5 kg/ha was recorded under treatment combination of 75% ETc deficit irrigation with inorganic fertilizer (S2IF), with treatment under the same irrigation schedule with organic compost (S2OC) recording the second highest model overestimation of 218.0 kg/ha. For 2021, highest model overestimation of 448.5 kg/ha was recorded under treatment combination of full irrigation with inorganic fertilizer (S3IF), with treatment under the same irrigation schedule with organic compost (S3OC) recording the second highest model overestimation of 407.6 kg/ha. Consistently, treatment combination of 50% ETc deficit irrigation and no fertilizer application (S1NF) recorded the lowest model overestimation of 103.6 and 102.8 kg/ha for the 2020 and 2021 cropping seasons, respectively.
3.3.2. Model performance metrics for tomato yield simulation
The model performance metrics for simulating the measured tomato fruit yields in both the 2020 and 2021 cropping seasons are presented in Table 6. The metrics include the Mean Bias Error (MBE), Root Mean Square Error (RMSE), Index of Agreement (d), and RMSE – Observation Standard Deviation (RSR). Average MBE values of 177 and 272 kg/ha and RMSE values of 293 and 467 kg/ha were recorded for the 2020 and 2021 cropping seasons, respectively. Higher MBE and RMSE values were recorded for the 2021 cropping season compared to the 2020 cropping season, which confirms the overestimation dynamics of the model (see Figure 4). On the other hand, the average index of agreement values of 0.83 and 0.82 and RSR values of 0.36 and 0.38 for the 2020 and 2021 cropping seasons, respectively, confirm high correlation between measured and simulated fruit yield values and therefore high model performance for the 2020 cropping season compared to the 2021 cropping season.
For the model performance on the different treatment combinations, MBE values greater than 200 kg/ha were recorded for treatments with inorganic fertilizer (IF) under both 50% ETc (S1) and 75% ETc (S2) deficit irrigation as well as organic compost (OC) under 75% ETc deficit irrigation for both cropping seasons (Table 6). However, more than 300 kg/ha MBE values were recorded for treatments with inorganic fertilizer (IF) under both 75% ETc deficit irrigation (S2) and full irrigation (S3) as well as organic compost (OC) under full irrigation for the 2021 cropping season. MBE values less than 150 kg/ha were recorded for treatments with no fertilizer application (NF) under both 50% ETc (S1) and 75% ETc (S2) deficit irrigation for both cropping seasons.
The model recorded RMSR values of more than 300 kg/ha for treatments with inorganic fertilizer (IF) and organic compost (OC) under both 50% ETc deficit irrigation (S1) and full irrigation (S3) for both cropping seasons (Table 6). However, more than 500 kg/ha RMSR values were recorded for treatments with inorganic fertilizer (IF) and organic compost (OC) under both 75% ETc deficit irrigation (S2) and full irrigation (S3) for the 2021 cropping season. RMSE values less than 200 kg/ha were recorded for treatments with no fertilizer application (NF) under 50% ETc deficit irrigation (S1) for both cropping seasons.
The index of agreement for the 2020 and 2021 cropping seasons generally ranged between 0.8–0.9 and 0.6–0.9, respectively. The index of agreement was up to 0.9 for treatments with inorganic fertilizer (IF) under both 50% ETc (S1) and 75% ETc (S2) deficit irrigation as well as treatments with no fertilizer application (NF) under 50% ETc deficit irrigation for the 2020 cropping season (Table 6). The index of agreement was also up to 0.9 for treatments with organic compost (OC) under all three irrigation schedules as well as treatments with inorganic fertilizer under full irrigation and no fertilizer application under 50% ETc deficit irrigation for the 2021 cropping season. It is worth noting that for the 2021 cropping season, low index of agreements of 0.6 and 0.7 were recorded for treatments with no fertilizer (NF) under both 75% ETc deficit irrigation (S2) and full irrigation (S3), respectively (Table 6).
Generally, a relatively high index of agreement translates to a low RSR. RSR values for the 2020 and 2021 cropping seasons generally ranged between 0.3–0.4 and 0.3–0.6, respectively. The dynamics of the RSR values are similar (but opposite in magnitude) to that of the index of agreement. Low RSR values of 0.3 were recorded for treatments with a high index of agreements (0.9), whereas RSR values of 0.4 were recorded for treatments with an index of agreements of 0.8. It is also worth mentioning that for the 2021 cropping season, high RSR values of 0.6 and 0.5 were recorded for the treatment with no fertilizer (NF) under both 75% ETc deficit irrigation (S2) and full irrigation (S3) schedules, which also recorded relatively low index of agreements of 0.6 and 0.7, respectively (Table 6).
4. Discussion
4.1. Plant growth parameters under different irrigation schedules and nutrient amendment
Growth performance (i.e., plant height, stem girth, and number of branches) were generally not consistent with the different treatment combinations of irrigation schedules and nutrient amendments. For instance, plant height was higher for treatments with organic fertilizer and no fertilizer application compared to treatments with inorganic fertilizer during the 2020 cropping season, especially under full irrigation. However, plant height was higher for treatments with inorganic fertilizer compared to treatments with organic fertilizer and no fertilizer application during the 2021 cropping season, particularly under 75% ETc deficit irrigation.
Stem girths were also similar for treatments with inorganic fertilizer and organic manure but higher than treatments with no fertilizer application under all three irrigation schedules during the 2020 cropping season. Treatments with inorganic fertilizer, however, recorded higher stem girth than treatments with organic compost as well as treatments with no fertilizer application during the 2021 cropping season, particularly under 75% ETc deficit irrigation and full irrigation. On the other hand, treatments with organic compost and inorganic fertilizer recorded a similar number of branches, but higher than treatments with no fertilizer application under 50% ETc deficit irrigation and full irrigation during the 2020 cropping season. For the 2021 cropping seasons, treatment with inorganic fertilizer, however, recorded a higher number of branches than treatments with organic fertilizer as well as treatments with no fertilizer application, especially under 50% ETc deficit irrigation.
Different fertilizer sources can have different effects on the vegetative growth of plants. For instance, Fidiyawati et al. (2022) reported increasing rice plant height for treatments with inorganic fertilizer compared to that of organic compost. Abdul-Rahman (2019) however, observed an increase in plant height and spikelet number per panicle with the application of both organic compost and inorganic fertilizers. The application of mineral fertilizer has also been reported to increase plant height compared to no fertilizer application (Mahmud et al., 2016). Some studies have also reported insignificant differences between rice plant height from the application of inorganic fertilizer and organic manure in isolation, but maximum plant height was attained from the combination of these two fertilizer sources (e.g., Moe et al., 2017). The increase in height of plants obtained by the use of inorganic fertilizer could have been due to the highly stimulating effect of readily available nitrogen on various physiological phases in cell division and cell elongation (Razaq et al., 2017).
The availability of soil moisture content can also affect the absorption rate of available nutrients. For instance, Amina et al. (2023) reported that the use of inorganic fertilizer at 100% recommended water produced higher stem girth compared to the application of the same fertilizer at 50% recommended water. Also, nitrogen addition has been reported to significantly increase Arabidopsis thaliana stem diameter, cortical thickness, flower ring radius, mid-rib thickness, and leaf and stem vascular size, while phosphorus addition significantly increased the stem xylem thickness (Cai et al., 2017). These findings indicate that different plants have different optimal ranges of nutrient demands. Different concentrations, ratios and even different nutrient availability can have quite different effects on plant growth and development.
The dynamics in plant growth parameters recorded in our study might be due to the different microclimatic conditions in the screen house for the two cropping seasons. With the same irrigation schedule for the two cropping seasons, high temperatures and low relative humidity in 2020 compared to 2021 might have influenced evapotranspiration through the dynamics of soil temperature and moisture content. Several studies have reported the impacts of soil temperature and moisture content on plant growth. Ideally, optimum soil conditions eventually improve plant growth (Ashrafuzzaman et al., 2011; Li et al., 2018; Yang et al., 2018). This is usually achieved through the adoption of appropriate irrigation schedules (Adekaldu et al., 2021). Our study focused on the atmospheric conditions in the screen house, which have impacts on the fruiting and flowing of vegetable plants (e.g., Reddy et al., 2017). Perhaps, monitoring soil temperature and moisture content dynamics could have helped to better explain the dynamics in the plant growth parameters.
4.2. Yield and crop water productivity under different deficit irrigation and nutrient amendment
Generally, tomato yields increased with increasing water application for both cropping seasons. Full irrigation produced the highest tomato yield, followed by 75% ETc deficit irrigation, with 50% ETc deficit irrigation producing the least yield for all the nutrient amendment treatments. This can be ascribed to the conducive growth conditions, namely optimum soil temperature, adequate soil moisture, and favorable microclimate for microbial activities achieved with the application of recommended irrigation rate (Sekhon et al., 2005; Arora et al., 2011; Adekaldu et al., 2021). Optimum soil moisture contents from the application of full crop water requirement also enhance root growth and proliferation, and, thus, increased nutrient availability and uptake by the crop roots eventually improved growth and yield (Sarkar and Singh, 2007). In the case of tomato crops, yield is likely to drop significantly when recommended water is not applied to adequately replenish evapotranspiration, as observed in our study. Reduced irrigation has been found to result in lower crop yields (Jianming et al., 2017).
Interestingly, the treatment combination of inorganic fertilizer and full irrigation schedule produced a higher yield compared to the same irrigation schedule with organic compost for both cropping seasons. However, for the deficit irrigation schedules (50 and 75% ETc), treatments with organic compost produced higher yields compared to inorganic fertilizer for both cropping seasons. This is due to the ability of organic fertilizers to improve the water holding capacities of soils even at a lower water supply (e.g., Elanchezhian et al., 2020). Organic composts generally have a slow effect on tomato output despite their ability to retain soil qualities whereas inorganic fertilizers are expensive but easier to utilize and react fast, especially with adequate moisture content (Koul et al., 2022) though, they are not environmentally friendly and pose a number of risks to human health and the environment (Gong et al., 2011; Bisht and Chauhan, 2021).
Low fruit yield recorded for the control treatments with no fertilizer application was expected since the limited soil nitrogen is not replenished over the growing season. Mineral nutrition of tomato plants from inorganic fertilizer and organic compost application has been shown to boost tomato yield and nutrient uptake by several folds when compared to no fertilization (Hu et al., 2022). The increase in the number of branches observed for the treatments with inorganic fertilizer compared to treatments with both organic manure and no fertilizer application could have resulted from the direct effect of the applied potassium nitrate in the NPK inorganic fertilizer, which promotes bud development (Cetinbas and Koyuncu, 2006; Khayyat et al., 2010). The number of branches has been found to increase the number of active fruit-bearing nodes and photosynthetic rates of crops, and subsequently, fruit yields (Adekaldu et al., 2021).
Deficit irrigation at 50% ETc and 75% ETc saved water up to 50 and 25% of the water used for full irrigation (100% ETc), respectively. Although full irrigation produced the highest fruit yield under all three nutrient amendments, the depiction of the water use efficiency, represented by the crop water productivity is different. Fruit yield produced with the same quantity of water was higher under deficit irrigation (50 and 75% ETc) than under full irrigation (100% ETc). Deficit irrigation was more efficient in terms of water use efficiency than full irrigation in this study.
This study supports the findings of a global meta-analysis of the responses of vegetables to deficit irrigation, which found that reducing irrigation levels reduced yield but improved water productivity (Singh et al., 2021). These findings also support those of Chand et al. (2020), who found that irrigation deficits of 30 and 50% result in higher crop water productivity in a greenhouse. This is practically important for regions with limited water resources such as arid and semi-arid tropical regions. However, farmers usually want to maximize profitability and therefore tend to focus more on total fruit yield than crop water productivity. This effect can be corrected if farmers can increase planting density in greenhouses or screen houses (Zhang et al., 2017) or perhaps, increase their farm size in open-field agriculture to realize higher yield with the use of deficit irrigation, especially in tropical environments.
The high yield and crop water productivity for the 2021 cropping season compared to the 2020 cropping season can also be explained by the disparities in temperature and relative humidity in the screen house. High temperatures (>35°C) have been identified to induce poor pollination and flower abortion, so high temperatures during the key blooming phase might have lowered tomato yield during the cropping season in 2020, with high temperatures compared to the 2021 cropping season (Ventrella et al., 2012). It has been reported that fruit yields are reduced drastically by extreme variabilities in weather conditions (Boote et al., 2012; Hatfield and Prueger, 2015). Usually, low temperature and high relative humidity suppress the impacts of deficit irrigation by reducing evapotranspiration. This ultimately increases soil moisture content that will enhance plant growth and yield in the face of the limited water resources associated with deficit irrigation. For instance, Zhang et al. (2017) reported an increase in yield and crop water productivity when midday temperature substantially decreased from 38.5°C in high vapor pressure deficit area to 31.3°C in low vapor pressure deficit area in a green house.
Treatments with inorganic fertilizer and organic compost produced statistically the same crop water productivity for the two cropping seasons under all the irrigation schedules used, whereas treatments with no fertilizer application produced the least crop water productivity. The higher crop water productivity recorded by the inorganic fertilizer and organic compost can be explained by the role of nitrogen, phosphorus and potassium in water uptake and utilization (Barłóg et al., 2022; Ru et al., 2022). Indeed, different irrigation and fertilization levels have been found to significantly affect crop water productivity (e.g., Zou et al., 2020). However, the effect of irrigation treatment on the crop water productivity was found to be greater than the effect of fertilization, which is consistent with our experimental results. One reason might be that fertilization promoted tomato root growth and development, thereby improving the capacity of the root system to absorb water and nutrients. Another reason may be that tomato water consumption improved during growth, causing the roots to increase the efficiency of soil water absorption and to further improve crop water productivity (Ye et al., 2022).
4.3. Model simulation of tomato yield as affected by climate variability
The overestimation of measured tomato yield by the DSSAT CROPGRO model is expected since the model produces potential yield, which is always higher than the actual yield, resulting from especially, fluctuations in soil and weather conditions over the growing stages compared to the use of initial soil and weather parameters as required in the model (Boote et al., 2012). Positive high Mean Bias Error (MBE) and Root Mean Square Error (RMSE) performance metrics of the DSSAT CROPGRO model indicate a general overestimation of the measured fruit yield by the model. A high index of agreement coupled with low RMSE-Observations Standard Deviation (RSR) also indicates a high correlation between the measured and simulated fruit yields, and eventually, high model performance in simulating the dynamics of the measured fruit yield for the different treatment combinations of irrigation schedules and nutrient amendments.
According to Moriasi et al. (2007), model performance is considered adequate if the Nash-Sutcliffe efficiency score (NSE) is less than or equal to 0.65, and RSR is less than or equal to 0.60. The NSE for the model performance between the measured and simulated yields was greater than 0.90 whereas RSR was less than or equal to 0.60 for both years (see Table 6). This is consistent with a study by Adnan et al. (2017), who reported low RSR of the DSSAT model for simulating maize yield for the irrigated and rainfed plots, indicating very good performance rating of the model. This observation is also similar to that reported by Oteng-Darko et al. (2020), who found that the DSSAT CERES Maize model has a very good performance rating for simulating maize biomass for irrigated plots, based on similar model metrics.
In comparison to stressful situations, the simulated model output demonstrated that larger yields can be obtained under adequate conditions (nutrient and water). The more water is available for crop use at the optimal temperature, the better the yields, and vice versa. However, the DSSAT CROPGRO tomato model tends to overestimate yields in soil with high water content and underestimate yields in deficit conditions. This is because the DSSAT CROPGRO tomato model is sensitive to severe conditions, with overestimation of yields in the case of water availability and underestimation of yields in the case of stress (Boote et al., 2012). Hence, fruit yields improve drastically when given the right doses of nutrients and water (Deligios et al., 2017). This may have accounted for the highest simulated fruit yield by the treatment combination of inorganic fertilizer and full irrigation for both cropping seasons, whereas the treatment combination of no fertilizer at 50% deficit irrigation recorded the lowest simulated fruit yield in both years (Table 6). In Zambia, similar experiments on enhanced fertilizer and irrigation schedules utilized in the DSSAT-CERES maize model resulted in higher maize yields (Chisanga et al., 2015).
With the increasing utilization of climate models, the DSSAT CROPGRO model can be used to simulate potential tomato yields based on different projected climate scenarios. The current experiment in the screen house provides a basis to validate such models since the microclimatic environments and water and nutrient supply can be controlled in such agricultural production systems to simulate the impact of different climatic conditions. It should be acknowledged that practically, modeling the impacts of climate change on tomato yield involves numerous issues of data availability and quality as well as scaling from global climate change data to the plot scale, where crop models are usually implemented. In addition, models have limitations relating to processes they consider, contrasting with the real-world complexity of cropping systems. However, such results will ultimately provide a solid foundation for tomato growers, agricultural investors, and agricultural policymakers to make informed decisions about early warning systems and climate adaptation measures in tomato production.
5. Conclusion
This study investigated the combined effects of deficit irrigation and nutrient amendments on the growth and yield of the Pectomech tomato variety cultivated in sandy loam soils in a screen house during the 2020 and 2021 cropping seasons in the forest agroecological zone of Ghana. The combined use of inorganic fertilizer and full irrigation was found to improve tomato yield. However, for the two deficit irrigations (50 and 75% ETc), organic compost produced the highest tomato yield. Plant height and stem girth did not have a specific influence on tomato productivity, but the number of branches, which influence bud development required for flowering and subsequently fruiting had a positive effect on tomato fruit yield. Deficit irrigation recorded improved crop water productivity, thus the number of tomato fruits produced with the same amount of water compared to full irrigation. This study has revealed that the use of readily available nutrient-holding mineral fertilizers such as NPK can maximize productivity with full crop water requirement since high moisture content is required to enable the release of nutrients. On the other hand, the use of slow response organic compost can as well maximize productivity with deficit irrigation schedules since organic compost can improve soil water hold capacity which can improve water and nutrient absorption even under water stress conditions. The strong agreement observed between the measured and simulated yields under the deficit irrigation and nutrient amendments shows that the DSSAT CROPGRO tomato model can be used to simulate tomato fruit yield under future climate scenarios. Increasing the amount of information to agricultural decision makers through the use of crop simulation models such as DSSAT CROPGRO tomato model can help to develop plans and policies that meet sustainable food security goals in tropical regions, especially in an era of climate change and uncertainties.
Data availability statement
The weather data for the DSSAT CROPGRO model cannot be shared by the authors but can be requested from the Ghana Meteorological Agency (https://www.meteo.gov.gh/gmet/). The remaining raw data supporting the conclusions of this article will be made available by the authors, without undue reservation.
Author contributions
PA-Y and WA conceptualized and designed the research. PA-Y, FAA, and SY implemented the research. FAA, MBB and AKK collected data. WA, FAA, MBB and AKK analyzed the data. FAA, PA-Y, SY and WA drafted the manuscript. MBB and AKK edited the manuscript. All authors contributed to the article and approved the submitted version.
Acknowledgments
We wish to express our gratitude to the Crops Research Institute of the Council of Scientific and Industrial Research, Fumesua, Kumasi and the Kwame Nkrumah University of Science and Technology, Kumasi, Ghana for providing the enabling environment for this project. The authors wish to express their gratitude to the Ghana Meteorological Agency, Accra, for providing the weather data for the DSSAT CROPGRO model. The data cannot be shared by the authors but can be requested from the Ghana Meteorological Agency (https://www.meteo.gov.gh/gmet/).
Conflict of interest
The authors declare that the research was conducted in the absence of any commercial or financial relationships that could be construed as a potential conflict of interest.
Publisher’s note
All claims expressed in this article are solely those of the authors and do not necessarily represent those of their affiliated organizations, or those of the publisher, the editors and the reviewers. Any product that may be evaluated in this article, or claim that may be made by its manufacturer, is not guaranteed or endorsed by the publisher.
References
Abdul-Rahman, A.-B. I. (2019). Combined effect of organic and inorganic fertilizers on growth of rice plants United Nations University Land Restoration Training Programme, [final project] Available at: https://www.grocentre.is/static/gro/publication/719/document/abdul-rahman2019.pdf.
Adekaldu, E., Amponsah, W., Tuffour, H. O., Adu, M. O., and Agyare, W. A. (2021). Response of Chilli pepper to different irrigation schedules and mulching Technologies in Semi-Arid Environments. J Agricult Food Res 6:100222. doi: 10.1016/j.jafr.2021.100222
Adnan, A. A., Jibrin, J. M., Kamara, A. Y., Abdulrahman, B. L., Shaibu, A. S., and Garba, I. I. (2017). CERES–maize model for determining the optimum planting dates of early maturing maize varieties in northern Nigeria. Front. Plant Sci. 8:1118. doi: 10.3389/fpls.2017.01118
Al-Ghobari, H. M., and Dewidar, A. Z. (2018). Integrating deficit irrigation into surface and subsurface drip irrigation as a strategy to save water in arid regions. Agric. Water Manag. 209, 55–61. doi: 10.1016/j.agwat.2018.07.010
Al-Selwey, W. A., Alsadon, A. A., Al-Doss, A. A., Solieman, T. H., Dewir, Y. H., and Ibrahim, A. A. (2021). Effect of deficit irrigation on Total yield, fruit physical characteristics, and nutritional value of four drought tolerant tomato (Solanum lycopersicum L.). Genotypes. J. Agr. Sci. Tech. 23, 1105–1118.
Amina, I. O., Bake, I. D., and Omar, A. W. H. (2023). Effects of different rates of NPK 15:15:15 fertilizer on growth and yield of okra (Abelmoschus Esculentus (L.) Moench) in Mubi north local government area of Adamawa state, Nigeria. Afr. J. Agricult. Sci. Food Res. 10, 1–13.
Arah, I. K., Kumah, E. K., Anku, E. K., and Amaglo, H. (2015). An overview of post-harvest losses in tomato production in Africa: causes and possible prevention strategies. J. Biol. Agric. Healthc. 5, 78–88.
Arora, V. K., Singh, C. B., Sidhu, A. S., and Thind, S. S. (2011). Irrigation, tillage and mulching effects on soybean yield and water productivity in relation to soil texture. Agric. Water Manag. 98, 563–568. doi: 10.1016/j.agwat.2010.10.004
Aryal, P. J., Sapkota, B. T., Krupik, J. T., Rahut, B. D., Jat, M. L., and Stirling, M. C. (2021). Factors affecting farmers’ use of organic and inorganic fertilizers in South Asia. Environ. Sci. Pollut. Res. 28, 51480–51496. doi: 10.1007/s11356-021-13975-7
Ashrafuzzaman, M., Halim, M. A., Ismail, M. R., Shahidullah, S. M., and Hossain, M. A. (2011). Effect of plastic mulch on growth and yield of chilli (capsicum annuum L.). Braz. Arch. Biol. Technol. 54, 321–330. doi: 10.1590/S1516-89132011000200014
Ayankojo, I. T., and Morgan, K. T. (2020). Increasing air temperatures and its effects on growth and productivity of tomato in South Florida. Plan. Theory 9:1245. doi: 10.3390/plants9091245
Barłóg, P., Grzebisz, W., and Łukowiak, R. (2022). Fertilizers and fertilization strategies mitigating soil factors constraining efficiency of nitrogen in plant production. Plan. Theory 11:1855. doi: 10.3390/plants11141855
Bationo, A., Hartemink, A. E., Lungu, O. I., Naimi, M., Okoth, P. F., Smaling, E. M., et al. (2012). “Knowing the African soils to improve fertilizer recommendations” in Improving soil fertility recommendations in Africa using the decision support system for Agrotechnology transfer (DSSAT). eds. J. Kihara, D. Fatondji, J. Jones, G. Hoogenboom, R. Tabo, and A. Bationo (Dordrecht: Springer)
Bisht, N., and Chauhan, P. S. (2021). “Excessive and disproportionate use of chemicals cause soil contamination and nutritional stress” in Soil contamination - threats and sustainable solutions. eds. M. L. Larramendy and S. Soloneski (IntechOpen)
Boote, K. J., Rybak, M. R., Scholberg, J. M. S., and Jones, J. W. (2012). Improving the CROPGRO-tomato model for predicting growth and yield response to temperature. HortScience 47, 1038–1049. doi: 10.21273/HORTSCI.47.8.1038
Cai, Q., Ji, C., Yan, Z., Jiang, X., and Fang, J. (2017). Anatomical responses of leaf and stem of Arabidopsis thaliana to nitrogen and phosphorus addition. J. Plant Res. 130, 1035–1045. doi: 10.1007/s10265-017-0960-2
Cetinbas, M., and Koyuncu, F. (2006). Improving germination of Prunus avium L. seeds by gibberellic acid, potassium nitrate and thiourea. J. Hortic. Sci 33, 119–123. doi: 10.17221/3750-HORTSCI
Chai, T., and Draxler, R. R. (2014). Root mean square error (RMSE) or mean absolute error (MAE)? –arguments against avoiding RMSE in the literature. Geosci. Model Dev. 7, 1247–1250. doi: 10.5194/gmd-7-1247-2014
Chand, J., Hewa, G., Hassanli, A., and Mayers, B. (2020). Evaluation of deficit irrigation and water quality on production and water productivity of tomato in greenhouse. Agriculture 10:297. doi: 10.3390/agriculture10070297
Chen, J., Kang, S., Du, T., Guo, P., Qiu, R., Chen, R., et al. (2014). Modeling relations of tomato yield and fruit quality with water deficit at different growth stages under greenhouse condition. Agric. Water Manag. 146, 131–148. doi: 10.1016/j.agwat.2014.07.026
Chisanga, C. B., Phiri, E., Shepande, C., and Sichingabula, H. (2015). Evaluating CERES- maize model using planting dates and nitrogen fertilizer in Zambia. J. Agric. Sci. 7, 1–19. doi: 10.5539/jas.v7n3p79
Colaizzi, P. D., O’Shaughnessy, S. A., Evett, S. R., and Mounce, R. B. (2017). Crop evapotranspiration calculation using infrared thermometers aboard center pivots. Agric. Water Manag. 187, 173–189. doi: 10.1016/j.agwat.2017.03.016
Deligios, P. A., Cossu, M., Murgia, L., Sirigu, A., Urracci, G., Pazzona, A., et al. (2017). Modelling tomato growth and production in a photovoltaic greenhouse in southern Italy. Acta Hortic. 1182, 203–210. doi: 10.17660/ActaHortic.2017.1182.24
Easterling, W. E., Aggarwal, P. K., Batima, P., Brander, K. M., Erda, L., Howden, S. M., et al. (2007). Food, fibre and forest products. Climate change 2007: Impacts, adaptation and vulnerability. Contribution of working group II to the fourth assessment report of the intergovernmental panel on climate change, M. L. Parry, O. F. Canziani, J. P. Palutikof, P. J. Lindenvan der, and C. E. Hanson, (eds.), Cambridge University Press, Cambridge, 273–313.
Elanchezhian, A., Khan, F., Basak, J. K., Park, J., Okyere, F. G., Lee, Y. J., et al. (2020). Analysis of water retention capacities of various compost and its relationship to strawberry moisture level. Acta Hortic. 1296, 899–906. doi: 10.17660/ActaHortic.2020.1296.114
FAO (2002), Crop water requirements and irrigation scheduling. Irrigation manual module, 4, FAO sub-regional Office for East and Southern Africa, Harare, 2002.
Fidiyawati, E., Latifah, E., Krismawati, A., Sihombing, D., Setyorini, D., Bakar, A., et al. (2022). Effectivity of inorganic fertilizer NPK (15-15-6) to growth and yield of lowland rice (Oryza sativa L.) on alfisol soil. IOP Conf. Series Earth Environ. Sci. 980:012014. doi: 10.1088/1755-1315/980/1/012014
Forkuor, G., Amponsah, W., Oteng-Darko, P., and Osei, G. (2022). Safeguarding food security through large-scale adoption of agricultural production technologies: the case of greenhouse farming in Ghana. Cleaner Eng. Technol. 6:100384. doi: 10.1016/j.clet.2021.100384
Gitay, H., Brown, S., Easterling, W., Jallow, B., Ande, J., Apps, M., et al. (2001). “Ecosystems and their goods and services” in Climate change 2001: Impacts, adaptation and vulnerability to climate change. Contribution of working group II to the third assessment report of the intergovernmental panel on climate change. eds. M. C. JJ , Canziani OF , N. A. Leary, D. J. Dokken, and K. S. White (Cambridge: Cambridge University Press), 236–342.
Gong, P., Liang, L., and Zhang, Q. (2011). China must reduce fertilizer use too. Nature 473, 284–285. doi: 10.1038/473284e
Hatfield, J. L., and Prueger, J. H. (2015). Temperature extremes: effect on plant growth and development. Weather Clim. Extrem. 10, 4–10. doi: 10.1016/j.wace.2015.08.001
Hoogenboom, G., Porter, C. H., Boote, K. J., Shelia, V., Wilkens, P. W., Singh, U., et al. (2019). The DSSAT crop modeling ecosystem. In: K.J. Boote , editor. Advances in Crop Modeling for a Sustainable Agriculture. Burleigh Dodds Science Publishing, Cambridge, 173–216.
Hoogenboom, G., Porter, C. H., Shelia, V., Boote, K. J., Singh, U., White, J. W., et al. (2021). Decision support system for Agrotechnology transfer (DSSAT) version 4.8 (DSSAT.net). DSSAT Foundation, Gainesville, Florida.
Hu, G. Q., Ma, X.-X., Li, X. H., and Wang, H. (2022). Evaluation of organic substitution based on vegetable yield and soil fertility. Environ. Pollut. Bioavailab. 34, 162–170. doi: 10.1080/26395940.2022.2064335
Jianming, L., Xiangyu, F., Fangfang, Y., Hui, L., and Dongsheng, C. (2017). Effect of different irrigation amount based on transpiration model on yield and quality of muskmelon. Trans. Chin. Soc. Agricult. Eng. 33, 156–162. doi: 10.11975/j.issn.1002-6819.2017.21.018
Jones, J. W., Hoogenboom, G., Porter, C. H., Boote, K. J., Batchelor, W. D., Hunt, L. A., et al. (2003). DSSAT Cropping System Model. Eur. J. Agron. 18, 235–265. doi: 10.1016/S1161-0301(02)00107-7
Khayyat, M., Rajaee, S., Shayesteh, M., Sajadinia, A., and Moradinezhad, F. (2010). Effect of potassium nitrate on breaking bud dormancy in strawberry (Fragaria ananassa Duch.) plants. J. Plant Nutr. 33, 1601–1611. doi: 10.1080/01904167.2010.496885
Koul, B., Yakoob, M., and Shah, M. P. (2022). Agricultural waste management strategies for environmental sustainability. Environ. Res. 206:112285. doi: 10.1016/j.envres.2021.112285
Kumar, A., Nayak, A. K., Sah, R. P., Sanghamitra, P., and Das, B. S. (2017). Effects of elevated CO2 concentration on water productivity and antioxidant enzyme activities of rice (Oryza sativa L.) under water deficit stress. Field crops research, 212:61-72. Agric. Water Manag. 212, 61–72. doi: 10.1016/j.fcr.2017.06.020
Li, S., Li, Y., Lin, H., Feng, H., and Dyck, M. (2018). Effects of different mulching technologies on evapotranspiration and summer maize growth. Agric. Water Manag. 201, 309–318. doi: 10.1016/j.agwat.2017.10.025
Mahmud, A. J., Shamsuddoha, A. T. M., and Haque, N. (2016). Effect of organic and inorganic fertilizer on the growth and yield of rice (Oryza sativa L.). Nat. Sci. 14, 45–54. doi: 10.7537/marsnsj14021607
Melomey, L. D., Danquah, A., Offei, S. K., Ofori, K., Danquah, E., and Osei, M. (2019). “Review on tomato (Solanum lycopersicum, L.) improvement programmes in Ghana” in Recent advances in tomato breeding and production. eds. S. T. Nyaku and A. Danquah (IntechOpen)
Mikkelsen, R., Jensen, T. L., Snyder, C., and Bruulsema, T. W. (2012). “Nutrient management planning and accountability” in 4R plant nutrition manual: A manual for improving the Management of Plant Nutrition, chapter 9. eds. T. W. Bruulsema, P. E. Fixen, and G. D. Sulewski (Norcross, GA: International Plant Nutrition Institute)
Moe, K., Mg, K. W., Win, K. K., and Yamakawa, T. (2017). Combined effect of organic manures and inorganic fertilizers on the growth and yield of hybrid rice (palethwe-1). Am. J. Plant Sci. 8, 1022–1042. doi: 10.4236/ajps.2017.85068
Moriasi, D. N., Arnold, J. G., Van Liew, M. W., Bingner, R. L., Harmel, R. D., and Veith, T. L. (2007). Model evaluation guidelines for systematic quantification of accuracy in watershed simulations. Trans. ASABE 50, 885–900. doi: 10.13031/2013.23153
Nash, J. E., and Sutcliffe, J. V. (1970). River flow forecasting through conceptual models: part 1. A discussion of principles. J. Hydrol. 10, 282–290. doi: 10.1016/0022-1694(70)90255-6
Oteng-Darko, P., Yeboah, S., Agyei Obeng, E., Amponsah, W., Ennin, S. A., Sarpong, F., et al. (2020). Simulative evaluation of the response of maize and some dual-purpose legumes to water and nutrient amendments. Agricult. Food Sci. J. Ghana 13, 1292–1310.
Rahman, K. M. A., and Zhang, D. (2018). Effects of fertilizer broadcasting on excessive use of inorganic fertilizers and environmental sustainability. Sustainability 10:759. doi: 10.3390/su10030759
Razaq, M., Zhang, P., and Shen, H. L. and Salahuddin (2017). Influence of nitrogen and phosphorous on the growth and root morphology of Acer mono. PLoS One 12:e0171321. doi: 10.1371/journal.pone.0171321
Reddy, G., Hebbar, S. S., Nair, A. K., Raghupathy, H. B., Gowda, A. M., and Umesha, K. (2017). Growth and yield performance of hybrid hot pepper, chilli (Capsicum annuum L.) as influenced by fertigation and polyethylene mulching. J. Hortic. Sci. 11, 151–155.
Ru, C., Hu, X., Chen, D., Song, T., Wang, W., Lv, M., et al. (2022). Nitrogen modulates the effects of short-term heat, drought and combined stresses after anthesis on photosynthesis, nitrogen metabolism, yield, and water and nitrogen use efficiency of wheat. Water 14:1407. doi: 10.3390/w14091407
Sarkar, S., and Singh, S. R. (2007). Interactive effect of tillage depth and mulch on soil temperature, productivity and water use pattern of rainfed barley (Hordium vulgare L.). Soil Tillage Res. 92, 79–86. doi: 10.1016/j.still.2006.01.014
Sayara, T., Basheer-Salimia, R., Hawamde, F., and Sanchez, A. (2020). Recycling of organic wastes through composting: process performance and compost application in agriculture. Agronomy 10:1838. doi: 10.3390/agronomy10111838
Sekhon, N. K., Hira, G. S., Sidhu, A. S., and Thind, S. S. (2005). Response of soyabean (Glycine max Mer.) to wheat straw mulching in different cropping seasons. Soil Use Manag. 21, 422–426. doi: 10.1079/SUM2005356
Singh, M., Singh, P., Singh, S., Saini, R. K., and Angadi, S. V. (2021). A global meta-analysis of yield and water productivity responses of vegetables to deficit irrigation. Sci. Rep. 11:22095. doi: 10.1038/s41598-021-01433-w
Song, W. Z., Yuan, Y., Jiang, Y. Z., Lei, X. H., and Shu, D. C. (2016). Rule-based water resource allocation in the Central Guizhou Province. China. Ecol. Eng. 87, 194–202. doi: 10.1016/J.ECOLENG.2015.11.051
Thirumeninathan, S., Pazhanivelan, S., Sudarmanian, N. S., Ragunath, K. P., Gurusamy, A., and Sritharan, N. (2021). Calibration and validation of DSSAT CROPGRO Peanut model for yield and yield attributing characters of groundnut varieties in northern agro-climatic zone of Tamil Nadu. Int. J. Environ. Clim. Change 11, 207–215. doi: 10.9734/ijecc/2021/v11i1130535
Thorp, K. R., Barnes, E. M., Hunsaker, D. J., Kimball, B. A., White, J. W., Nazareth, V. J., et al. (2014). Evaluation of CSM-CROPGRO-cotton for simulating effects of management and climate change on cotton growth and evapotranspiration in an arid environment. Trans. ASABE 57, 1627–1642. doi: 10.13031/trans.57.10612
Ullah, I., Mao, H., Rasool, G., Gao, H., Javed, Q., Sarwar, A., et al. (2021). Effect of deficit irrigation and reduced N fertilization on plant growth, root morphology and water use efficiency of tomato grown in soilless culture. Agronomy 11:228. doi: 10.3390/agronomy11020228
Ventrella, D., Charfeddine, M., Giglio, L., and Castellini, M. (2012). Application of DSSAT models for an agronomic adaptation strategy under climate change in southern of Italy: optimum sowing and transplanting time for winter durum wheat and tomato. Ital. J. Agron. 7, 16–115. doi: 10.4081/ija.2012.e16
Yang, Y., Ding, J., Zhang, Y., Wu, J., Zhang, J., Pan, X., et al. (2018). Effects of tillage and mulching measures on soil moisture and temperature, photosynthetic characteristics and yield of winter wheat. Agric. Water Manag. 201, 299–308. doi: 10.1016/j.agwat.2017.11.003
Ye, T., Ma, J., Zhang, P., Shan, S., Liu, L., Tang, L., et al. (2022). Interaction effects of irrigation and nitrogen on the coordination between crop water productivity and nitrogen use efficiency in wheat production on the North China plain. Agricult. Water Manag. 271, 107787–103774. doi: 10.1016/j.agwat.2022.107787
Zhang, D., Du, Q., Zhang, Z., Jiao, X., Song, X., and Li, J. (2017). Vapour pressure deficit control in relation to water transport and water productivity in greenhouse tomato production during summer. Sci. Rep. 7:43461. doi: 10.1038/srep43461
Keywords: deficit irrigation, water use efficiency, soil amendments, crop simulation models, DSSAT model
Citation: Amankwaa-Yeboah P, Aruna Akoriko F, Amponsah W, Yeboah S, Brempong MB and Keteku AK (2023) Combining deficit irrigation and nutrient amendment enhances the water productivity of tomato (Solanum lycopersicum L.) in the tropics. Front. Sustain. Food Syst. 7:1199386. doi: 10.3389/fsufs.2023.1199386
Edited by:
Krishna Devkota, International Center for Agricultural Research in the Dry Areas (ICARDA), MoroccoReviewed by:
Prosanta K. Dash, Texas A&M University at Qatar, QatarPratapsingh Khapte, National Institute of Abiotic Stress Management (ICAR), India
Copyright © 2023 Amankwaa-Yeboah, Aruna Akoriko, Amponsah, Yeboah, Brempong and Keteku. This is an open-access article distributed under the terms of the Creative Commons Attribution License (CC BY). The use, distribution or reproduction in other forums is permitted, provided the original author(s) and the copyright owner(s) are credited and that the original publication in this journal is cited, in accordance with accepted academic practice. No use, distribution or reproduction is permitted which does not comply with these terms.
*Correspondence: Patricia Amankwaa-Yeboah, p.amankwaa-yeboah@cropsresearch.org