- 1ICAR-Indian Agricultural Statistics Research Institute, New Delhi, India
- 2Tocklai Tea Research Institute (TRI), Tea Research Association, Jorhat, India
Motivation: Tea, derived from the Camellia sinensis plant, holds the position of being the most widely consumed manufactured beverage globally. Its cultivation necessitates specific agro-climatic conditions, leading to its production being confined to select regions, notably including India. India contributes about 20.81% to the world’s tea output. The production and processing of tea leaves to final product consume energy in terms of machinery, fertilizer, irrigation etc. The energy consumption involved in tea production is a pressing concern, given the associated high costs and CO2 emissions resulting from fossil fuel usage. To achieve a net-zero carbon balance, there is need to pay attention towards promoting renewable energy technologies as a means to mitigate the CO2 emissions stemming from fossil fuels in India’s tea sector.
Objectives: Aligned with the objective of sustainability through the integration of renewable energy sources, a pilot study was conducted in the primary tea-growing regions of northeastern India during 2021–22. The primary aims of this study were twofold: to gauge the quantity of CO2 emissions originating from conventional energy sources and to explore the feasibility of incorporating renewable energy sources as viable substitutes.
Data and methods: Data on various inputs used in tea production were collected from Assam and West Bengal states of India by using a stratified random sampling method with equal probability and without replacement.
Results: The findings of this investigation underscore a noteworthy potential for the adoption of renewable energy, particularly solar energy, within the tea estates situated in the north eastern region of India. Such a transition would yield benefits for both the tea estates themselves and the overall environment.
1 Introduction
Global warming is likely the world’s greatest threat in the current scenario. One of the main sources of global warming is the massive use of fossil fuels, which produces carbon dioxide. Global warming is responsible for global climate change, and the world is facing challenges in terms of sustainability due to it. One of the best ways to prevent climate change is to move away from the use of fossil fuels. Renewable energies, such as solar, wind, biomass, and geothermal, may be alternatives for the same. Renewable energy technologies are being promoted in many parts of the world for various agricultural practices to mitigate CO2 emissions from burning fossil fuels. Renewable energy technologies are those that consume primary energy resources that are not subject to depletion. Along with renewable energy technologies, there was also emphasis on finding ways to net-zero carbon in climate resilient agricultural system. Through various cultivation practices, i.e., conservation tillage, bio-char application, soil and nutrient management etc. net-zero carbon can be achieved in tea plantation. Several works in the past were conducted focusing on the issue of carbon sequestration in tea plantation in the north eastern states of India. Phukan et al. (2018) proposed the algorithm computing CO2 assimilation in tea bushes. This study revealed that tea bushes had potential to assimilate 1243.8–2526.7 kg CO2 ha−1 year−1 and the high yielding tea cultivar absorbed significantly higher amount of CO2 as compared to better quality producing tea cultivar. Pramanik and Phukan (2020a,b) showed tea plants were capable for sequestering 50.8% of the assimilated atmospheric CO2 in their biomass. They have also showed that tea bushes release organic C through the root which was equivalent to 5.9%–8.6% of the assimilated CO2. Further, Pramanik and Phukan (2020a,b) have also quantified the potential of tea plantation to mitigate greenhouse effect by assimilating the atmospheric CO2. They have also compared few high yielding and quality tea producing cultivars and showed that the high yielding cultivars assimilate more CO2 than the quality tea producing cultivars. From the study it was also found that the mature 25–30 years old tea bushes assimilate more CO2 than the younger ones. It was found that, tea bushes sequestrated 5134.4 831.6 kg CO2 in their biomass and had global warming mitigation potential (GWMP) of 3.47 0.64 kg CO2 . Alom et al. (2021) showed that, among various cropping systems, tea-albizzia cropping system had superior performance in carbon sequestration followed by tea-areca palm cropping system with respect to monoculture in the north eastern states of India. While several works were going on regarding the carbon sequestration potential of tea plantations in the north eastern states of India, Kumari et al. (2021) showed the potential of renewable energy technologies to mitigate the problem of global warming in the tea plantations of north eastern India using some basic descriptive statistics on a pilot survey data. The results were usually inconsistent as the data collected through surveys were never identically and individually distributed (iids). Further, Udeagha and Ngepah (2022a,b,c) studied the impact of technological innovation on carbon dioxide (CO2) emissions and focused on energy-growth-CO2 emissions nexus, which were mostly concentrated towards the econometric approach towards sustainability. They have focused on one of the most important topics in the current scenario of global warming and climate change but is not related to our work focused on achieving the net-zero carbon models for development of climate resilient sustainable agriculture systems.
In general, tea production is deeply dependent on the use of fossil fuels for various production activities. India contributes approximately 20.81% in the area of global tea production and is one of the leading countries in the world for the production of tea leaves (FAOSTAT, 2021; Tea Board of India, 2023). The growth and production of tea have been reported to be higher than other plantation crops in the country. It is the most consumed drink after water in the country (Awasom, 2011). Tea is produced from the apical shoot of the plant Camellia sinensis, consisting of one bud and two or three leaves (Hatibaruah et al., 2012; Kumar et al., 2023). According to data published by the Tea Board of India (2023) through the Annual Bulletin of Statistics (2020), the total world tea production was 6012.81 million kg. Chronologically, the five top producers of tea in the world are China, India, Kenya, Sri Lanka, and Türkiye (Annual Bulletin of Statistics, 2020; FAOSTAT, 2021; Kumar et al., 2023; Figure 1).
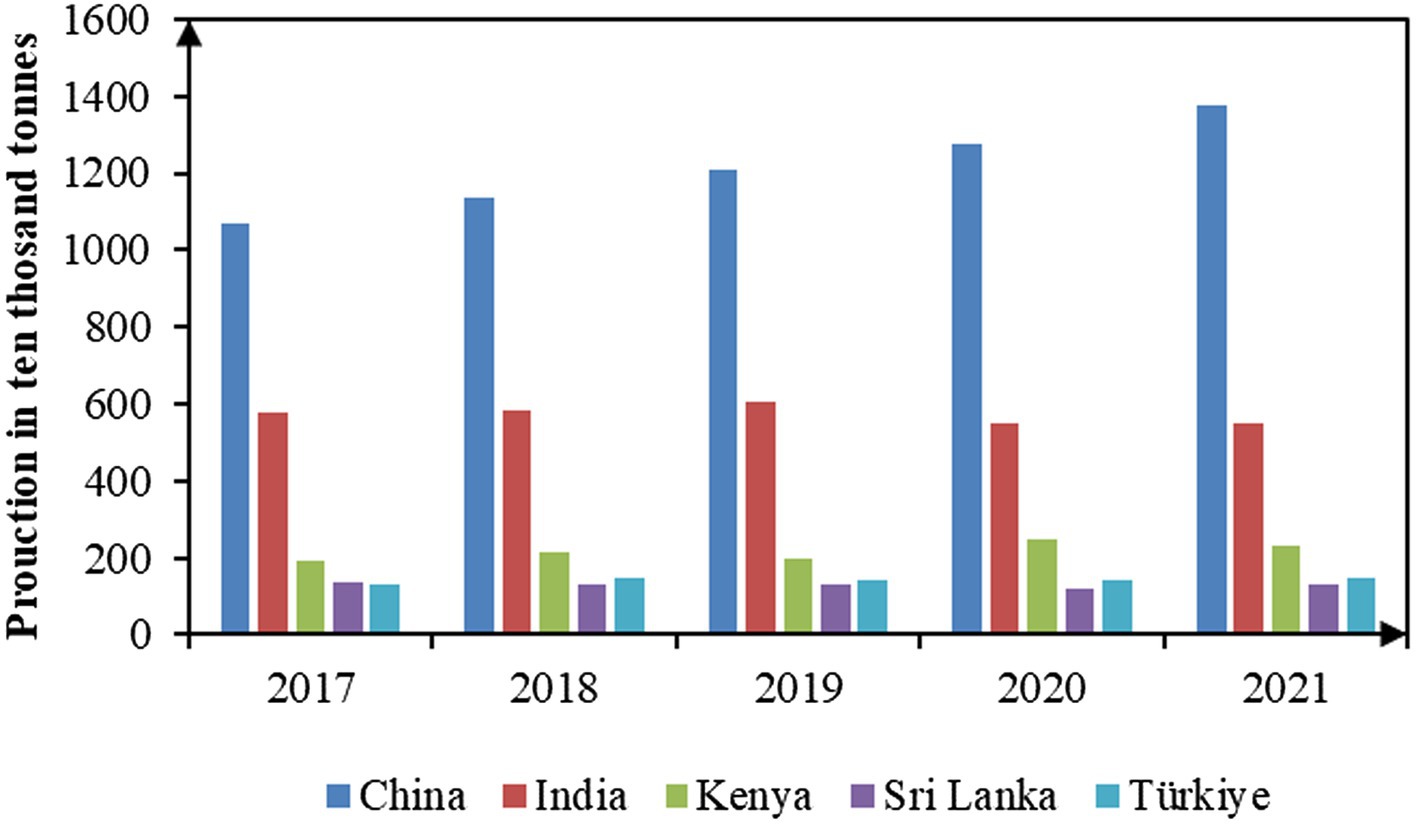
Figure 1. Top tea leaves producing countries in world (FAOSTAT, 2021).
Over the past few decades, the production and consumption of tea have steadily increased, and it has become one of the main economic pillars in countries such as China, India, Sri Lanka, and Kenya (Tularam, 2016). The growing of tea plants and its processing into made tea requires energy, generated mostly from fossil fuels in developing countries like India which on the contrary leads to heavy emission of greenhouse gases (GHG). However, the ever-increasing demand for energy has led to the depletion of non-renewable energy sources, and it is predicted that energy resources will significantly reduce by the 2030s–40s (Ulyanin et al., 2018). The continuous use of conventional non-renewable energy sources also poses several challenges, such as environmental pollution and greenhouse gas (GHG) emissions that contribute towards global warming and climate change. One of the best ways to mitigate the problem of climate change occurring from the GHG emissions is to shift to some alternative renewable energy sources which prevents CO2 and other GHG emissions in long term. Therefore, the adoption of renewable energy is a crucial opportunity for Indian tea factories to grow sustainably (Shah and Patel, 2016). Table 1 presents data on the use of energy in agriculture and the emission of CO2 and CH4 in India over the last 3 years (FAOSTAT, 2021). Both CO2 and CH4 are major contributors to GHG emissions that are directly related to burning biomass or fossil fuels, along with the use of synthetic fertilizers for better crop growth.
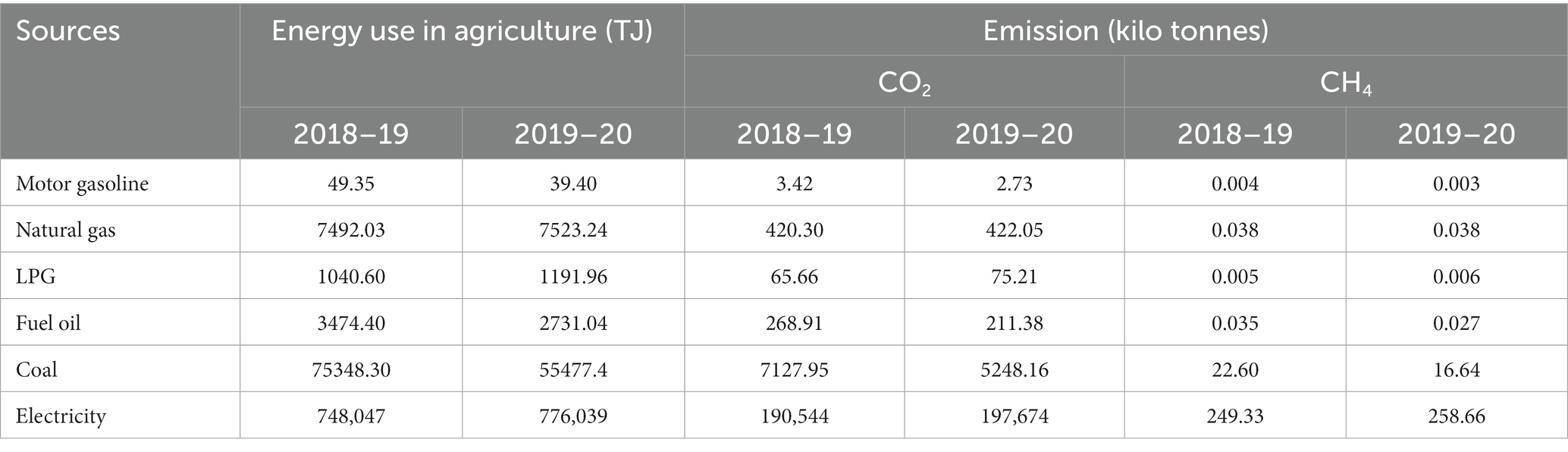
Table 1. The use of energy in agriculture and emission of CO2 and CH4 in India (Source: FAOSTAT, 2021).
The major contributors to CO2 and CH4 emissions in agriculture are electricity and coal, compared to other sources (FAOSTAT, 2021). Electricity is generally produced in countries like India mostly using the combustion of fossil fuels or diesel generators, which produces a huge amount of GHG emissions. In rural areas of India, due to a shortage of power supply and energy poverty, people mostly rely on diesel generators for electricity generation. For these reasons, electricity is among the major contributors to GHG emissions, along with coal. Electricity is required in gardens for irrigation, pruning, power spray, plucking, etc., and in tea factories for converting tea leaves into tea products using machinery. Thermal energy (heat) is required in tea factories for moisture removal from the tea leaves during withering and drying (Kumar et al., 2021, 2023). According to Mitra and Totan (2016), 0.679 kWh of electrical energy and 28.39 MJ of thermal energy are required to produce 1 kg of tea. In tea plantations and factories, conventional fuels such as coal and diesel are used to meet the thermal energy requirements for various operations, and these energy sources pollute the environment. These conventional fossil fuels could be replaced by suitable renewable energy resources to meet the energy demand in tea plantations and factories. Shifting from the use of fossil fuels to renewable energy sources like solar energy or wind turbines, requires a huge amount of initial investment. It is easier for large tea industries or estates to adopt these alternative renewable energy sources while it is difficult for smallholder farmers due to unavailability of sufficient funds and poverty. Based on some past studies in South Africa, it was found that in case of the small holder farmers, sustainability towards climate change and global warming can be achieved through optimizing various management and agricultural practices in crop production (Kephe et al., 2022) or through proper institutional support (Kephe et al., 2021). Although, in tea sector in India, smallholder farmers are mostly involved in cultivation and production of tea leaves, the GHG emissions occurring out of the smallholder farms were considerable very less as compared to huge tea estates and factories prevailing in the area.
Renewable energy has become a popular option for meeting energy needs across different sectors of the economy. The Government of India intends to achieve 500 GW of renewable energy capacity by 2030. They are also planning to replace fossil fuels with solar energy up to 50%. A recent article in Mongabay (Goswami, 2022) titled “Assam tea gardens experiment with solar for reliable power supply and cutting costs, emissions” highlights the opportunities for adopting solar or other renewable energy sources in the northeastern states of India (Kumar et al., 2023). Most of the studies conducted in connection to climate resilient and sustainable agricultural practices towards tea cultivation and production are based on either carbon sequestration or feasibility studies for greener technologies based on mere naïve descriptive statistics, there is a need for a detailed study using proper statistical methodology for impact analysis of GHG emission from extensive use of fossil fuel and implementation of renewable energy practices and technologies as an substitute. To fill up this gap, in this study, a detailed survey was conducted in two major tea-producing states of India, namely Assam and West Bengal, which account for 52.08% and 30.40% of the country’s total production capacity, respectively. The major aim of the survey was to assess the feasibility of integrating solar energy as an alternative energy source. The study also focuses on quantifying the amount of GHG emissions during the tea processing and production using proper random sampling methodologies. The estimates of energy produced under this study are consistent and unbiased with respect to the population parameters (Sukhatme et al., 1984). Conventional fuel sources such as coal and diesel are conventionally used to generate heat for the tea factory processes. The rising cost of fossil fuels and the emissions of greenhouse gases are major drawbacks that contribute to the increase in tea production costs. Additionally, sustainable development goal (SDG) 7.0 of the United Nations, which focuses on “Ensuring access to affordable, reliable, sustainable, and modern energy for all,” highlights the importance of reliable alternative renewable energy sources, specifically targeted in SDG 7.2. In agricultural sector, sustainability revolves around achieving a delicate balance between increasing crop productivity, preserving economic stability, minimizing the use of limited natural resources, and mitigating unfavorable environmental effects (Chel and Kaushik, 2011). Hence, to achieve sustainability in tea production, there is a need to give emphasis on net-zero carbon models to maintain the soil organic carbon content through carbon sequestration along with mitigating the problems occur from CO2 emissions through various cultivation and production practices. As more emphasis was given on finding the potential of tea plantations for carbon sequestration from around the world and India, little focus was laid on the GHG emissions from the same during various stages of tea production. Given the importance of these aspects, the present study focuses on environmental impact of CO2 emissions from the tea industries of northeastern states of India. Combination of the results of both the studies on carbon sequestration by tea plantation and measures of CO2 emissions from the tea industries will pave the way in better policy planning for net-zero carbon in climate resilient and sustainable agricultural systems among tea producers in India.
2 Materials and methods
2.1 Study area
The tea crop requires a specific type of agro-climatic environment that is only available in tropical and subtropical areas (Kumar et al., 2021). The tea plant thrives in a hot and humid climate with temperatures typically ranging from 10°C to 30°C. It requires a minimum annual precipitation of 1,250 mm, acidic soils, ideally slopes ranging from 0.5 to 10 degrees, and elevations up to 2,000 meters (Ahmed et al., 2017). As a result, tea production is limited to a few regions around the world, and it is highly sensitive to environmental fluctuations during its developmental stages. It is important to note that the ideal growing conditions for tea are at high risk due to changing climatic conditions worldwide. Assam, which is the single largest tea-growing state in the world, contributes to 57% of India’s tea production and produces some of the finest black tea globally (Biggs et al., 2018). Therefore, Assam and West Bengal were purposely selected for this study as they are major tea-producing states in India.
2.2 Sampling scheme
A detailed survey was conducted in the two major tea-growing states in India to gather information on the adoption of renewable energy for tea cultivation. To conduct the survey, a sampling frame was prepared based on available data from the Tea Board of India (2023). As of 2020, the Toklai Tea Research Institute in Jorhat, Assam reported a total of 459 major tea estates/gardens in Assam and 70 in Darjeeling, West Bengal. These states were treated as independent subpopulations based on specific ethnic criteria. The survey was planned and carried out between March 2021 and June 2021. However, due to the severe impact of the COVID-19 pandemic in India during that period, contacting and obtaining responses from all the tea estates/gardens was challenging. As a result, only 95 tea estates/gardens (20.6%) from Assam and 37 tea estates/gardens (52.8%) from Darjeeling agreed to participate in the survey. Based on the available sampling frame at the stratum level, a sample size of 25% was randomly selected without replacement from the list of participating major tea estates/gardens in Assam and Darjeeling. The sample sizes and population sizes in the states are provided in Table 2.

Table 2. Population and sample sizes selected for detailed survey on renewable energy adoption in the states of Assam and West Bengal for tea crop.
2.3 Data collection
Three questionnaires were prepared for the survey:
(i) Listing/enumeration questionnaire,
(ii) List of selected tea estates/gardens, and
(iii) Detailed survey questionnaire on energy inputs and outputs in the selected tea estates/gardens.
The questionnaires were designed based on a pilot study conducted in the north eastern states of India during May, 2020 to June, 2020. Data on data were collected from randomly selected eight tea gardens (four from Assam and four from northern area of West Bengal) and seven tea processing industries and the developed questionnaires were communicated to them (Kumari et al., 2021; Goswami, 2022). After collection of the data, analysis of the data was done using basic descriptive statistics to assess the scope of implementation of renewable energy technologies in the north eastern states of India. As the data were collected through random sampling and as only descriptive statistics were used for draw conclusion, the results cannot be treated as consistent and unbiased as the data points are not independently distributed. Hence, a bigger survey was planned with proper sampling design to generate the design unbiased and consistent estimates through a stochastic sampling design. This is another major reason behind this study. To comply with COVID-19 travel restrictions during the survey period, all the questionnaires were converted into Google Forms and emailed to the respondents. The listing/enumeration questionnaire was designed to obtain a comprehensive list of tea estates/gardens in Assam and West Bengal willing to participate. It was sent to all the tea estates/gardens (459 in Assam and 70 in Darjeeling, West Bengal). Based on the number of respondents who replied to the questionnaires, a final list of participating estates/gardens was compiled and the sampling frame was constructed. Based on the thumb rule of random sampling, of taking 15%–25% of the survey population units in sample for better acceptable estimates, a sample size of 25% of the units were taken into consideration.
The second questionnaire involved preparing a list of tea estates and gardens at the institute level, which was then communicated to the participating estates/gardens. Once the tea gardens were selected in the second questionnaire, the third questionnaire, designed for a detailed survey, was sent to the selected tea estates/gardens in the sample.
The third questionnaire collected data on energy consumption during various cultural practices involved in tea cultivation, such as planting, sowing, irrigation, fertilizer and chemical application, and harvesting. Additionally, information on energy consumption during various operations of tea production, including withering, fermentation, sorting, and drying, was gathered from the tea factories. Details such as the types of machines used, their capacity (kW/hp), working hours, fuel types (petrol/diesel/gas), total energy value, and associated costs were also collected in the third questionnaire.
2.4 Statistical analysis
A stratified random sampling design was employed to estimate the total fossil fuel consumption during different stages of tea production and the amount of greenhouse gas (GHG) emissions generated throughout the process.
For calculation of GHG equivalent (kg CO2 eq per/acre) the estimates of the inputs (MJ/acre) were multiplied with the green house gas (GHG) coefficients (kg CO2 eq/MJ). The GHG coefficients were given in the following Table 3.
The probability sampling design was chosen over model-based predictions because model-based approaches often overlook the inclusion of survey design weights when calculating estimates. Failing to account for design weights, which are the inverse of the probabilities used in selecting sampling units, can result in inconsistent estimates and an inaccurate representation of the target population.
Moreover, model-based predictions rely on assumptions that may be challenging to meet in real-life survey data. In contrast, using a sampling design allows for the generation of robust estimates that are not solely reliant on model assumptions. To ensure the consistency of the estimates for total fossil fuel consumption during various stages of tea production, a random sampling design was proposed. Once the total fuel consumption during different stages of tea production was estimated, these figures were multiplied by corresponding GHG equivalent multipliers to calculate the carbon footprint of each process.
Let, a finite population U = {1,…, k,…, N} consisting of N units. A sample s of size n is drawn without replacement according to a probabilistic sampling plan with inclusion probabilities which are assumed to be strictly positive and known.
Then, the Horvitz–Thompson estimator (Horvitz and Thompson, 1952) of the population total be
where, yi be the value of the variable of interest, y, for the ith population element. The study variable y is observed for each unit in the sample hence is known for all is the sampling design weight, defined as the inverse of the inclusion probability for unit i (Sarndal et al., 1992). The study variable concerns with the values of each energy inputs that were used for tea production and cultivation is the normalized survey weight for ith unit and is survey weight for ith unit in the sample. The proposed Horvitz–Thompson estimator is a design based estimator and this is why it is assumption free. According to Sukhatme et al. (1984), the Horvitz–Thompson estimator is a design unbiased and consistent estimator under equal probability without replacement sampling design.
The unbiased estimator of variance under the sampling design is obtained as:
3 Results and discussion
The purpose of the survey was to identify energy-intensive management practices during the production of tea leaves in tea gardens and the processing of tea leaves into the final product in tea factories. Data were collected on energy consumption, specifically the amount of fossil fuel consumed, during various operations in tea gardens and tea factories. The collected data were analyzed based on the proposed sampling design.
The operations in tea gardens encompass several activities such as land preparation, planting, plant protection, pruning of tea plants, and plucking of tea leaves. Tea plants can be propagated through cuttings or seeds, and it is crucial to clean the land before planting to ensure successful establishment of the tea garden. Immediate mulching of the soil surface is recommended after planting, along with providing the necessary filtered shade for tea plantation. Plant protection, fertilizer application, and irrigation are also essential at different stages of tea plant growth. Pruning plays a vital role in tea gardens as it helps the plants establish well during their growth. After these operations, the tea leaves are harvested, which is a labor-intensive process in tea gardens. Table 4 and Figure 2 present the estimates of annual CO2 production (in kilograms per hectare) by tea gardens for each operation, including land preparation and planting, irrigation, fertilizer and chemical application, pruning of tea plants, plucking of tea leaves, harvest, and transportation.
The tea gardens primarily relied on fossil or petroleum-based fuels for their farming operations. Once the tea leaves were plucked from the gardens, they were sent to the tea factories for further processing to obtain the final product. The major energy-consuming operations in the tea factories are depicted in Figure 3 and are described as follows:
(i) Withering: This process involves temporarily storing the harvested shoots to partially remove moisture. It causes changes in the texture of the shoots, making the leaves flaccid. The chemical changes that occur during withering contribute to the tea’s flavor, aroma, color, and taste. The sampled factory used equipment such as a centrifugal fan, heater, coal stove, axial fan, and withering trough for withering. The estimated energy consumption for withering per 1,000 kg of made tea was 20.27 liters of diesel, 43.93 kg of coal, and 87.17 kWh of electricity. According to Sharma et al. (2019), for tea production of 100 kg, the specific electrical consumption required for withering was calculated as 5.54 kWh, and the required thermal energy was 179.11 MJ.
(ii) Rolling: This operation imparts a specific shape and size to the withered leaves, resulting in a tea product that is acceptable to consumers. There are two types of black tea produced based on the rolling method: orthodox tea and CTC (crush, tear, and curl) tea. Orthodox tea is rolled to give it a twisted form, while CTC tea is macerated by chopping the leaves into uniform granulated particles. For 1,000 kg of made tea, the estimated electrical energy consumption for rolling was 190 kWh, and the thermal energy consumption was 574.63 MJ. Tea-processing units also rely on diesel generators for electricity during power cuts.
(iii) Fermentation: This process involves the oxidation of tea leaves. The sampled factory used equipment such as CFM machines, humidifiers, floor fermentation, fermenting troughs, electric fans, and blowers for fermentation. Tea is classified into different types, including green, white, oolong, and black, based on the extent of oxidation. Estimates of energy consumption for fermentation per 1,000 kg of made tea were 0.79 liters of diesel and 89.65 kWh of electricity. According to Sharma et al. (2019), for tea production of 100 kg, the required electrical consumption for fermentation was calculated as 8.63 kWh.
Figure 3 illustrates the steps involved in the production of various types of tea.
(iv) Drying: The drying process is carried out to reduce the moisture content of the tea leaves to an appropriate standard (usually 2.8%–3% w.b.) in order to halt enzymatic reactions and create a stable tea product. It is one of the most energy-intensive processes in tea production. For every 1,000 kg of made tea, the estimated energy consumption for drying is 16.08 liters of diesel, 630.22 kg of coal, and 103.41 kWh of electricity. In terms of thermal energy, the drying process requires 8.41 times more energy compared to the withering process. This finding is supported by Sharma et al., 2019, who also demonstrated that tea processing factories have higher thermal energy requirements for the drying process compared to the withering process.
(v) Sorting and Packaging: After the tea leaves have been dried, they undergo sorting, where any fiber and stalky substances are separated and the tea is sieved and graded based on particle size. The final step in tea manufacturing is packaging, which is crucial for protecting the tea from moisture and contaminants. These processes combined require an energy consumption of 61.72 kWh of electricity and 161.48 MJ of thermal energy per 1,000 kg of made tea. It is worth noting that the energy requirements in these processes are comparatively lower than those in other stages of tea processing in factories.
It is important to highlight that all of these operations heavily rely on conventional energy sources and electricity. Table 5 provides estimates of the thermal and electrical energy requirements per kilogram of made tea, as well as the corresponding CO2 emissions in tea factories at various stages of tea processing (Figure 4).
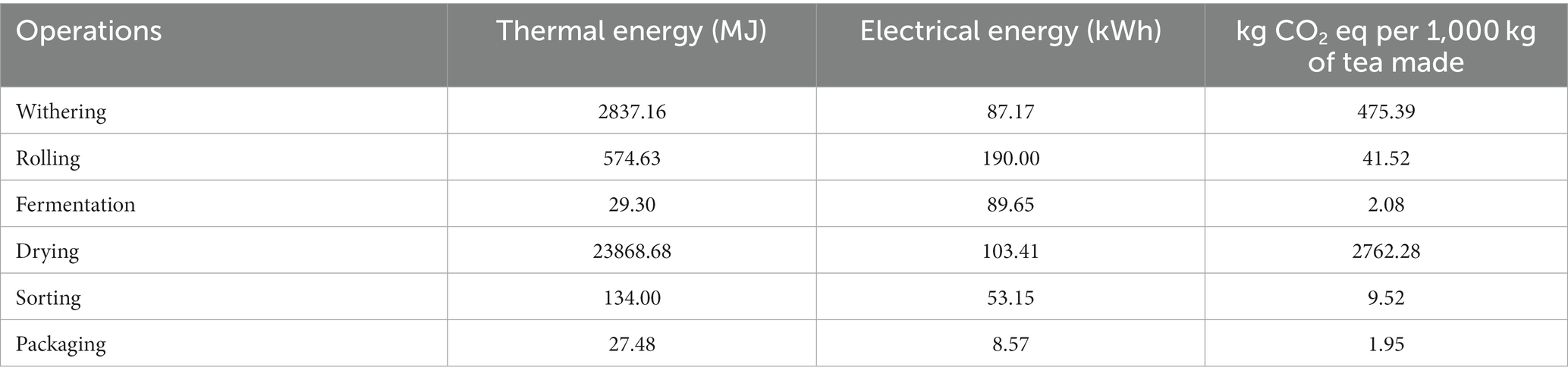
Table 5. Estimates of consumption of diesel, natural gas, coal and electricity per 1,000 kg of tea made.
Based on the findings, thermal energy accounted for approximately 85.65% of the total energy consumption in tea factories. The primary sources of thermal energy were coal, firewood, natural gas, and briquetted biomass. Previous studies on energy consumption in tea factories have shown that the ratio of thermal to electrical energy consumption is around 85:15 (Sharma et al., 2019). In India, the specific electrical energy consumption ranges from 0.4 to 0.7 kWh/kg of tea, while the total thermal energy consumption ranges from 4.45 to 6.84 kWh/kg of tea (Silva, 1994). According to Vidanagama and Lokupitiya (2018), the total greenhouse gas emissions range from 514.27 ± 68.66 kg CO2/ton (in high yielding variety grown areas) to 603.10 ± 191.58 kg CO2/ton (in quality tea growing areas) of made tea. Among the greenhouse gas emissions in tea factories, 63% can be attributed to electricity consumption, while the remaining emissions are divided between fossil fuel usage (23%) and biomass usage (14%).
Tea growers often rely on chemical inputs to maintain high tea yields, but this practice poses significant risks to the environment, crops, and public safety. It can lead to issues such as soil erosion, soil acidification, pesticide residues, loss of soil organic carbon and in totality loss of biodiversity (Chen et al., 2022). To mitigate these risks, the application of modern agricultural practices, including conservation tillage, bio-char application, and soil and nutrient management, becomes crucial for sustaining a net-zero carbon sink and preserving soil biodiversity. This approach contributes to the establishment of a resilient agricultural system in the face of climate challenges. Research conducted by Phukan et al. (2018) highlighted that tea bushes exhibit the potential to absorb a range of 1243.8–2526.7 kg CO2/ha per year. Interestingly, higher-yielding tea cultivars displayed a notably greater capacity to assimilate CO2 compared to cultivars focused on better quality production. Additional work by Pramanik and Phukan (2020a,b) demonstrated that tea plants can sequester approximately 50% of assimilated atmospheric CO2 within their biomass. Their findings also indicated that tea bushes release organic carbon through roots, equivalent to 5.9%–8.6% of assimilated CO2. The study also revealed that mature tea bushes aged 25–30 years assimilated more CO2 than their younger counterparts, with an estimated sequestration of around 5134.4 ± 831.6 kg CO2/ha per year in their biomass.
Further investigations revealed that for every ton of tea produced, tea factories contributed around 3,292 ± 493.91 kg of CO2 emissions, while tea gardens contributed about 13.61 kg CO2/ha. Based on data from the Tea Board of India (2023), the total tea production from the northeastern states in July 2021 amounted to 157.23 million kgs (equivalent to 154746.7 imperial tonnes). Consequently, the total CO2 emissions from tea factory production during that month reached 509540.67 tonnes. Similarly, according to the Indian Tea Association (2023), the total tea crop area in the northeastern states covered 330,030 ha, resulting in a total CO2 emission of 4491.708 tonnes during tea cultivation. Collectively, the overall CO2 emissions during cultivation and production in July 2021 summed up to 514032.38 tonnes. On the other hand, the tea crop’s atmospheric CO2 sequestration in the same year, calculated following the algorithm proposed by Phukan et al. (2018), amounted to approximately 410491.3 tonnes. This discrepancy of 103541.1 tonnes between CO2 generation through tea production and sequestration by tea plantations underscores the urgent need for the replacement of non-renewable energy sources with renewable alternatives such as solar thermal energy and wind energy. This transition is essential to mitigate CO2 emissions and sustain the net-zero carbon balance in the tea production process.
Solar energy can be harnessed through two main methods: solar photovoltaic systems, which convert sunlight into electricity, and solar thermal systems, which capture heat energy. Drying tea leaves using solar heat is one of the earliest and most common uses of solar energy. A study conducted by Kumar et al. (2021) also found that tea estates preferred adopting solar energy as an alternative source. Solar panels are relatively easy to install and require a one-time initial investment compared to the higher costs associated with installing a hydropower station.
Renewable fuels, such as biodiesel derived from vegetable oils and animal fats, and ethanol produced from crops like corn, barley, and wheat, can be utilized for transportation and other tea factory processes. Encouraging tea farmers to adopt these renewable energy sources requires raising awareness and providing incentives. The government can play a role by offering subsidies and implementing skill development programs for tea farmers. These initiatives would motivate farmers to embrace sustainable energy practices, making their agricultural operations more profitable. This contributes to local and national energy security and sustainability.
The use of solar energy and other renewable sources as an alternative to fossil fuels not only improves profitability but also reduces greenhouse gas emissions and maintains the net zero carbon system, thus mitigating the risks associated with global warming and degradation of soil and environment. Implementation of renewable energy sources have one constraint, that it is costly during the initial period of implementation, i.e., solar energy and due to this fact most of the small holder growers avoid using these technologies as they were unable spend the needed money. Large industries and tea estates have shown their eagerness towards these technologies as it needs a good investment in the initial stage and then it will run free of cost at the later stages. Still there is need for few future studies to investigate the economic feasibility and long-term sustainability of renewable energy systems and its adoption in tea estates along with their adoption among smallholder tea growers like Kephe et al. (2021, 2022) for long term sustainability towards climate change. Further, there is need for exploring the potential synergies between different renewable energy sources and their impact on tea industry. There is further need to extend the study to other parts of the country where tea is produced. By efficiently utilizing various available renewable energy sources like solar energy, fuel-efficient air heaters, biomass gasifiers (Datta and Baruah, 2014), and mini hydro power plants and adopting sustainable modern climate resilient agricultural practices, tea growers can contribute more towards environmental friendly and sustainable tea production industry.
4 Conclusion
Tea farming serves as a source of employment and income for countless small-scale cultivators worldwide. It is imperative that the industry upholds environmental, social, and economic sustainability standards. An endeavor was undertaken to assess the energy consumption across various stages of tea leaf production, from cultivation to the final tea product. The outcomes reveal a substantial utilization of conventional energy sources, encompassing fossil fuels and electricity, throughout both tea cultivations in gardens and the subsequent tea production in factories. This incurs significant costs in terms of both environmental impact and financial resources. Furthermore, there exists a pressing necessity to adopt net-zero carbon models for crop production in order to achieve climate-resilient agriculture. While tea crops possess the capacity to absorb a noteworthy amount of greenhouse gas emissions, it falls short of offsetting the emissions stemming from the combustion of fossil fuels during energy generation for tea production. Further, this study is mainly focused on the north eastern states of India whereas there is a need to assess the same using all the tea estates, i.e., those in the southern parts of India under consideration for more detailed conclusion in future studies. Given the global progression toward an impending energy crisis, a viable solution emerges in the form of transitioning to renewable energy alternatives, such as solar energy, energy-efficient air heating systems, biomass gasifiers, and mini hydro power plants. Such a shift has the potential to considerably curtail greenhouse gas emissions without compromising the energy requisites of the tea industry or the overall environment. The recommendations of this study hold valuable implications for tea factories and have the capacity to invigorate the Indian tea economy.
Data availability statement
The raw data supporting the conclusions of this article will be made available by the authors, without undue reservation.
Ethics statement
Ethical review and approval was not required for the study on human participants in accordance with the local legislation and institutional requirements. Written informed consent from the participants was not required to participate in this study in accordance with the national legislation and the institutional requirements.
Author contributions
KA, RK, and Bharti: data curation, formal data analysis, and drafting of the paper. SS: data collection and data curation. All authors contributed to the article and approved the submitted version.
Funding
The author(s) declare financial support was received for the research, authorship, and/or publication of this article. It is to be mentioned that the research is funded by ICAR-Indian Agricultural Statistics Research Institute, New Delhi, India.
Acknowledgments
All the authors are grateful to all the reviewers for their valuable comments which helped in improvement of the paper to a great extent. The authors are also grateful to Late Hukum Chandra, ICAR-National Fellow, New Delhi who have perished due to COVID-19 in 2021, for his immense contribution in the work.
Conflict of interest
The authors declare that the research was conducted in the absence of any commercial or financial relationships that could be construed as a potential conflict of interest.
Publisher’s note
All claims expressed in this article are solely those of the authors and do not necessarily represent those of their affiliated organizations, or those of the publisher, the editors and the reviewers. Any product that may be evaluated in this article, or claim that may be made by its manufacturer, is not guaranteed or endorsed by the publisher.
References
Ahmed, T., Ali, M., Rana, M. M., and Hossen, M. I. (2017). Effect of global warming and climate change on the tea plantation. Tea J. Bangladesh 46, 42–51.
Alom, S., Das, R., Baruah, U., Das, S., and Bhuyan, R. P. (2021). Carbon sequestration potential under tea based cropping system. J. Environ. Biol. 42, 687–693. doi: 10.22438/jeb/42/3/MRN-1498
Awasom, I. (2011). Commodity of the quarter: tea. J. Agric. Food Inf. 12, 12–22. doi: 10.1080/10496505.2011.540552
Biggs, E. M., Gupta, N., Saikia, S. D., and Duncan, J. M. A. (2018). The tea landscape of Assam: multi-stakeholder insights into sustainable livelihoods under a changing climate. Environ. Sci. Policy 82, 9–18. doi: 10.1016/j.envsci.2018.01.003
Chel, A., and Kaushik, G. (2011). Renewable energy for sustainable agriculture. Agron. Sustain. Dev. 31, 91–118. doi: 10.1051/agro/2010029
Chen, Y., Chen, S., and Chen, Z. (2022). Willingness of tea farmers to adopt ecological agriculture techniques based on the UTAUT extended model. Int. J. Environ. Res. Public Health 19:15351. doi: 10.3390/ijerph192215351
Datta, P. P., and Baruah, D. C. (2014). Possibility of biomass gasification in tea manufacturing industries in Assam, India. Int. J. Renew. Energy Res. 5:310. doi: 10.1504/IJRET.2014.065374
FAOSTAT. Food and Agriculture Organization of the United Nations. (2021) Available at: https://www.fao.org/faostat/en/#data/QCL (Accessed on 13 January 2023)
Goswami, R. (2022). Assam tea gardens experiment with solar for reliable power supply and cutting costs, emissions. Mongabay. Available at: https://india.mongabay.com/2022/02/assam-tea-gardens-experiment-with-solar-for-reliable-power-supply-and-cutting-costs-emissions/
Hatibaruah, D., Baruah, D. C., and Sanyal, S. (2012). Microwave drying characteristics of Assam CTC tea (Camellia assamica). J. Food Process. Preserv. 37, 366–370. doi: 10.1111/j.1745-4549.2011.00656.x
Horvitz, D., and Thompson, D. (1952). A generalization of sampling without replacement from a finite universe. J. Am. Stat. Assoc. 47, 663–685. doi: 10.1080/01621459.1952.10483446
Indian Tea Association. (2023). Available at: https://www.indiatea.org/tea_growing_regions (Accessed on August 16, 2023)
Kephe, P. N., Petja, B. M., and Kwabena, A. K. (2021). Examining the role of institutional support in enhancing smallholder oilseed producers’ adaptability to climate change in Limpopo province, South Africa. OCL 28:14. doi: 10.1051/ocl/2021004
Kephe, P. N., Siewe, L. C., Lekalakala, R. G., Kwabena, A. K., and Petja, B. M. (2022). Optimizing smallholder farmers’ productivity through crop selection, targeting and prioritization framework in the Limpopo and Free State provinces, South Africa. Front. Sustain. Food Syst. 6:738267. doi: 10.3389/fsufs.2022.738267
Kumar, R., Dashora, K., Krishnan, N., Sanyal, S., Chandra, H., Dharmaraja, S., et al. (2021). Feasibility assessment of renewable energy resources for tea plantation and industry in India—a review. Renew. Sust. Energ. Rev. 145:111083. doi: 10.1016/j.rser.2021.111083
Kumar, K. R., Dashora, K., Kumar, S., Dharmaraja, S., Sanyal, S., Aditya, K., et al. (2023). A review of drying technology in tea sector of industrial, non-conventional and renewable energy based drying systems. Appl. Therm. Eng. 224:120118. doi: 10.1016/j.applthermaleng.2023.120118
Kumar, A., Singh, D., and Mahapatra, S. K. (2022). Energy and carbon budgeting of the pearl millet-wheat cropping system for environmentally sustainable agricultural land use planning in the rainfed semi-arid agro-ecosystem of Aravalli foothills. Energy 246:123389. doi: 10.1016/j.energy.2022.123389
Kumari, V., Chandra, H., Aditya, K., Kumar, K. R., Dashora, K., Krishnan, N., et al. (2021). Feasibility study on renewable energy system in tea (Camellia sinensis) estates of north-east India. Indian J. Agric. Sci. 91, 1631–1635. doi: 10.56093/ijas.v91i11.118574
Mitra, R., and Totan, S. (2016). An energy audit in a tea manufacturing industry at North Bengal, India. Int. Res. J. Eng. Technol. 5, 83–89. doi: 10.15623/ijret.2016.0506017
Ozbek, O., Gokdogan, O., and Baran, M. F. (2021). Investigation on energy use efficiency and greenhouse gas emissions (GHG) of onion cultivation. Fresenius Environ. Bull. 30, 1125–1133.
Pathak, H., and Wassmann, R. (2007). Introducing greenhouse gas mitigation as a development objective in rice-based agriculture: I. Generation of technical coefficients. Agric. Syst. 94, 807–825. doi: 10.1016/j.agsy.2006.11.015
Phukan, M., Savapondit, D., Hazra, A., Das, S., and Pramanik, P. (2018). Algorithmic derivation of CO2 assimilation based on some physiological parameters of tea bushes in north-east India. Ecol. Indic. 91, 77–83. doi: 10.1016/j.ecolind.2018.03.091
Pramanik, P., and Phukan, M. (2020a). Assimilating atmospheric carbon dioxide in tea gardens of northeast India. J. Environ. Manag. 256:109912. doi: 10.1016/j.jenvman.2019.109912
Pramanik, P., and Phukan, M. (2020b). Potential of tea plants in carbon sequestration in north-east India. Environ. Monit. Assess. 192:211. doi: 10.1007/s10661-020-8164-y
Sarndal, C. E., Swensson, B., and Wretman, J. (1992). Model assisted survey sampling. Springer, New York
Shah, S. K., and Patel, V. A. (2016). Tea production in India: challenges and opportunities. J. Tea Sci. Res. 6, 1–6. doi: 10.5376/jtsr.2016.06.0005
Sharma, A., Dutta, A. K., Bora, M. K., and Dutta, P. P. (2019). Study of energy management in a tea processing industry in Assam, India. AIP Conf. Proc. 2091:020012. doi: 10.1063/1.5096503
Silva, W. C. A. (1994). Status review of energy utilization by the tea industry in Sri Lanka. Sri Lanka J. Tea Sci. 63, 46–58.
Sukhatme, P. V., Sukhatme, B. V., Sukhatme, S., and Asok, C. (1984). Sampling theory of surveys with applications. Indian Society of Agricultural Statistics, New Delhi.
Tea Board of India. (2023). Available at: https://teaboard.gov.in Accessed on August 16, 2023
Tularam, G. A. (2016). The tea industry and a review of its price modelling in major tea producing countries. Strateg. Manag. J. 7, 21–36. doi: 10.5430/jms.v7n1p21
Udeagha, M. C., and Ngepah, N. (2022a). Disaggregating the environmental effects of renewable and non-renewable energy consumption in South Africa: fresh evidence from the novel dynamic ARDL simulations approach. Econ. Chang. Restruct. 55, 1767–1814. doi: 10.1007/s10644-021-09368-y
Udeagha, M. C., and Ngepah, N. (2022b). Dynamic ARDL simulations effects of fiscal decentralization, green technological innovation, trade openness, and institutional quality on environmental sustainability: evidence from South Africa. Sustainability 14:10268. doi: 10.3390/su141610268
Udeagha, M. C., and Ngepah, N. (2022c). The asymmetric effect of technological innovation on CO2 emissions in South Africa: new evidence from the QARDL approach. Front. Environ. Sci. 10:985719. doi: 10.3389/fenvs.2022.985719
Ulyanin, Y. A., Kharitonov, V. V., and Yurshina, D. Y. (2018). Forecasting the dynamics of the depletion of conventional energy resources. Stud. Russ. Econ. Dev. 29, 153–160. doi: 10.1134/S1075700718020156
Keywords: net-zero carbon balance, carbon sequestration, renewable energy, CO2 emissions, tea industry, north eastern India
Citation: Aditya K, Kumar R, Bharti and Sanyal S (2023) Environmental impact of green house gas emissions from the tea industries of northeastern states of India. Front. Sustain. Food Syst. 7:1220775. doi: 10.3389/fsufs.2023.1220775
Edited by:
Priscilla Ntuchu Kephe, Potsdam Institute for Climate Impact Research (PIK), GermanyReviewed by:
Florencia Ricard, National University of La Pampa, ArgentinaPartha Dutta, Tezpur University, India
Maxwell Chukwudi Udeagha, University of Johannesburg, South Africa
Copyright © 2023 Aditya, Kumar, Bharti and Sanyal. This is an open-access article distributed under the terms of the Creative Commons Attribution License (CC BY). The use, distribution or reproduction in other forums is permitted, provided the original author(s) and the copyright owner(s) are credited and that the original publication in this journal is cited, in accordance with accepted academic practice. No use, distribution or reproduction is permitted which does not comply with these terms.
*Correspondence: Kaustav Aditya, a2F1c3Rhdi5hZGl0eWFAaWNhci5nb3YuaW4=; a2F0dTQ0OTNAZ21haWwuY29t