- Department of Food Science, College of Agriculture and Veterinary Medicine, United Arab Emirates University, Al-Ain, United Arab Emirates
This study investigated in vitro antidiabetic, anti-obesity, and antioxidant activities of chia seed protein hydrolysates (CSPHs) and flaxseed protein hydrolysates (FSPHs) generated using three food-grade proteases at the hydrolysis at intervals of 120 min for 6 h. The inhibitory potentials of CSPHs and FSPHs on the enzymatic biomarkers related to diabetes (α-glucosidase and DPP-4) and obesity (pancreatic lipase and cholesteryl esterase) were determined. The antioxidant capacity of CSPHs and FSPHs was also assessed using ABTS, DPPH, and FRAP antioxidant assays. Increasing the proteolytic reaction time was shown to significantly increase the degree of hydrolysis values of CSPHs and FSPHs. Protein hydrolysates resulted in higher inhibitory potential against enzymatic biomarkers related to metabolic diseases (diabetes and obesity) as well as higher antioxidant activities compared with undigested proteins. Overall, the lowest IC50 inhibition values demonstrated in DPP-IV were observed by alcalase-catalyzed CSPH at 6 h (IC50 = 272.17 μg/mL) and bromelain-catalyzed FSPH at 6 h (IC50 = 338.70 μg/mL). The strongest inhibition of pancreatic lipase was observed in bromelain-catalyzed hydrolysates (IC50 = 292.43 μg/mL and IC50 = 307.62 μg/mL for CSPH and FSPH, respectively) after 6 h. These findings indicate that CSPHs and FSPHs exhibited enhanced antidiabetic, anti-obesity, and antioxidant properties. Therefore, these hydrolysates can be successfully used for their applications in the development of functional foods.
1. Introduction
At present, sedentary lifestyles together with rising rates of unhealthy diets, alcohol consumption, excessive energy intake, tobacco use, and physical inactivity are generally believed to be the main causes of mortality in relatively young populations. In fact, the global burden of lifestyle-related chronic diseases continues to grow. Tackling these lifestyle-related diseases, such as obesity, diabetes, hyperlipidemia, and stroke, presents one of the main challenges for growth in recent time because they are considered a leading threat to global health (Singh et al., 2014, 2021, 2022; Mudgil et al., 2022a). The growing socioeconomic burden, certain side effects associated with certain allopathic drugs, and increased consumer awareness for wellness have resurged the demand for natural or alternative therapies. Therefore, it has been strongly recommended to modify human's lifestyle by incorporating bioactive dietary supplements and/or functional foods in daily meals, which may pave the way to improve overall health or reduce the menace of these life-style related diseases (Mohamed, 2014).
Proteins have dual qualities, whose one aspect is providing nutrients required for growth and the other aspect is modulating specific physiological functions because they conceal peptide sequences in their native form, which are recognized as “biologically active peptides”. Generally, these peptide sequences remain inactive in the intact structure of their precursor protein and become active during their transit through the gastrointestinal tract via hydrolysis by gastrointestinal enzymes and microbial fermentation (Mohamed, 2014; Singh et al., 2021). Once released, peptides could have potential applications in formulating functional foods and nutraceuticals due to their ability to confer different physiological useful functions, such as antimicrobial, immunomodulatory, anticancerous, antidiabetic, antithrombotic, antioxidant, and mineral-binding activities, in the host system (Maqsood et al., 2021). However, among the studies previously reported on these physiological properties of peptides, milk proteins were the major substrates investigated for producing peptides (Silva and Malcata, 2005). Interestingly, proteins from plant sources are currently being actively explored because of their lower-cost and environmental sustainability compared with animal proteins.
In recent times, chia seeds and flaxseeds has emerged as non-conventional sources of protein and have gained scientific attention because of the global demand for sustainable proteins and their excellent nutrient profile. As stated by Kaushik et al. (2016), flaxseed proteins have comparable amino acid profiles to soy proteins and, as such, are considered a desirable source of protein. However, as earlier mentioned, bioactive peptides can be obtained by in vitro gastrointestinal digestion and fermentation. Although acid hydrolysis and enzyme-catalyzed hydrolysis has been widely used. It is worth mentioning that the latter strategy is safer and provides more uniform end-products due to its specificity reactions; hence, it is commonly used for producing bioactive peptides.
At present, numerous studies have shown that the enzymatic hydrolysis of encrypted peptides is important in liberating peptides with potent bioactivity (Mostafa et al., 2022; Mudgil et al., 2022a,b,c; Alnuaimi et al., 2023; Prakash Nirmal et al., 2023). The success of bioactive peptides from plant sources in performing several physiological activities has prompted researchers to be on the lookout for newer or more potent inhibitors of enzymes related to lifestyle-related diseases. Several enzymes such as alpha-amylase, alpha-glucosidase, and dipeptidyl peptidase IV have been implicated directly or indirectly in the development or complications associated with diabetes (Mudgil et al., 2019, 2020; Nongonierma et al., 2019; Ajayi et al., 2021; Baba et al., 2021a,b,c; Kamal et al., 2021). As such, inhibition of these enzymes has been a matter of recent investigations for the invention of newer and safer antidiabetic drugs. Similarly, pancreatic lipase and cholesteryl esterase inhibitors are also targeted as promising anti-obesity treatment (Adisakwattana et al., 2010; Ajayi et al., 2021; Siegień et al., 2021; Mudgil et al., 2022c; Urbizo-Reyes et al., 2022; Alnuaimi et al., 2023). Hence, this current study aimed to explore the potential of chia and flaxseed proteins for producing hydrolysates with inhibitory properties against enzymatic markers related to diabetes and obesity.
2. Materials and methods
2.1. Materials, enzymes, and chemicals
Chia seeds and flaxseeds purchased from LuLu store (Al Ain, United Arab Emirates) were used for this study. The chemicals and reagents used in the study, including substrates and enzymes, such as bromelain (enzyme activity: ≥3 units mg−1 protein, E.C. 3.4.22.33) from pineapple stem, alcalase (enzyme activity: ≥5 Ug−1, E.C. 3.4.21.62) from Bacillus licheniformis, papain (enzyme activity: ≥3.5 units mg−1 powder, E.C. 3.4.22.2) from papaya latex, dipeptidyl peptidase IV (expressed in Sf9 cells, source: human recombinant), and α-glucosidase (enzyme activity: ≥ 10 units mg−1 protein) sourced from Saccharomyces cerevisiae, cholesteryl esterase from porcine pancreas (E.C. 3.1.1.13), and porcine pancreatic lipase (E.C. 3.4.23.1) of >95% purity, were purchased from Sigma-Aldrich (St. Louis, MO, USA). All the chemicals and reagents used in the study were of analytical grade.
2.2. Mucilage extraction from chia seeds and flaxseeds
The experiment began with the extraction of seed mucilage from chia seeds and flaxseeds following the protocols of Campos et al. (2016) and Urbizo-Reyes et al. (2019). Briefly, hydrated seeds (seed: water ratio 20:1) of chia seeds and flaxseeds were heated at 60°C for 30 min to promote mucilage swelling. Sonication treatment was conducted on the aqueous solution in an ultrasonic cell disruptor (Sonifier® Branson 500D Danbury, CT, USA) at 50% power input. Thereafter, seeds were passed through a vacuum-assisted filtration system to eliminate the mucilage and, subsequently, dried in a tray dryer at 40°C for 12 h, and the dried mucilage that adhered to the seeds was further removed by rubbing over a mesh (30 mm).
2.3. Isolation of protein from chia seeds and flaxseeds
The mucilage-free chia seeds and flaxseeds were then converted to fine powder by grinding using a heavy-duty electric grinder (IKA A11 basic, Guangzhou, China), and the resultant powder was further passed through a 3-μm (pore size) sieve equipped with OCTAGON-200CL shaker (Endecotts Ltd., London, UK). To proceed with the experiment, the seed proteins were isolated using the methodology described in the investigation carried out by Ajayi et al. (2021). First, the flours obtained were subjected to double-hexane defatting (ratio of 1:5 (w/v)) for a period of 120 min, and the solution was centrifuged at 4,400 x g for 10 min at 4°C to remove fat. After that, the resultant flour was heated in the oven (40°C, 12 h) to ensure minimal residual hexane. Then, 100 g of dried defatted seed flours was dissolved in 1,000 mL of NaOH (15mM) to obtain a flour-to-solvent ratio of 1:10, and the slurry was stirred continuously at RT (24 ± 2°C) for 2 h with the pH being maintained at 10.0. The resultant slurry was maintained at a temperature of 4°C overnight, followed by homogenization and double centrifugation at 4,400 x g for 30 min and 10,000 x g for 20 min at 4°C, respectively. Then, the supernatant collected was further frozen to remove any fat layer left after the centrifugation process. The protein extracts of chia seeds and flaxseeds were passed through Whatman no. 1 paper, and the filtrate was adjusted to pH 4.5 using 3.2 mol/1 of HCl to precipitate the proteins, followed by centrifugation at 10,000 × g for 10 min at 4°C. The resultant chia and flax seed protein isolates (CSP and FSP) were washed, homogenized, and neutralized to pH 7.0 before being frozen (-20°C) for future use. The crude protein content of the slurry was estimated by Kjeldahl's nitrogen determination method (N × 6.25). The protein content of CSP and FSP slurry was 95.53 mg/mL and 98.4 mg/mL, respectively.
2.4. Production of chia seed and flaxseed protein hydrolysates
The hydrolysis of CSP and FSP obtained earlier was carried out using a similar methodology as reported previously by Alblooshi et al. (2023). In brief, CSP and FSP extract collected in Section 2.3 was thawed and homogenized followed by adjusting the protein content to 4% (w/v). Then, the slurry was transferred into four tubes, where one tube was labeled as the control set and the other three tubes contained three different enzymes at an E/S ratio of 1/100 (w/w). Specifically, the test enzymes, i.e., alcalase, bromelain, and papain, were maintained at their optimum pH of 8.0, 7.0, and 7.0, respectively. Afterward, the tubes containing the samples were incubated in a water bath (50 °C for 6 h) with sequential agitation of 100 ramps/min. Hydrolysates obtained from CSP and FSP substrates were collected after every 2 h of enzyme-catalyzed hydrolysis, and each hydrolysis reaction cycle was terminated by incubating at 95°C for 10 min. Protein hydrolysates (PHs) produced from CSP and FSP were denoted as CSPHs and FSPHs and then centrifuged at 10,000 x g for 10 min at 4°C. The supernatant fractions were collected and stored at −20°C for further analysis.
2.5. Determination of the extent of hydrolysis
The extent of protein hydrolysis referred to as the degree of hydrolysis (DH) of the PHs was quantified according to the previous study by Nongonierma et al. (2019). Purposely, 10 μL aliquots of PHs (CSPH and FSPH) were suspended into microplates well containing 100 μL of freshly prepared o-phthaldialdehyde (OPA) reagent. The reagent was composed of 2.5 mL SDS (20 %, w/v), 0.1 mL β-mercaptoethanol, 0.04 g of OPA, and 1000 μL of methanol in 25 mL of 100 mM sodium tetraborate buffer (pH 8.3), the final volume was raised to 50 mL using deionized water. The UV spectrophotometer was set at 340 nm to measure the amount of free amino nitrogen (FAN). A standard curve of tryptone was constructed and was used to estimate the free amino nitrogen content and increase in free amino content upon protein hydrolysis as DH was measured using equation 1.
2.6. Profiling of CSPH and FSPH with RP-UPLC
The obtained hydrolysates (i.e., CSPH and FSPH) were analyzed by RP-UPLC using the modified methodology described by Nongonierma and Fitzgerald (2012). Herein, lyophilized PHs were reconstituted in tubes containing HPLC-grade deionized water to attain 0.2% w protein equivalent/v and mixed in an equal proportion with solvent A, after vigrous vortexing for 5 min samples were then passed through a 0.45-μm filter ahead of sample injection. An HPLC reversed-phase C18 column (XBridge® BEH, C18 Column, Waters Corporation, Ireland) conditioned with solvent A [Milli-Q water in 0.1 % trifluoroacetic acid (TFA)] was used for peptide profiling; 40 μL of samples were passed through the column (2.5 μm, 2.1 × 100 mm column) with a flow rate of 0.3 mL/min at 25°C and separated by a linear gradient elution of 60% acetonitrile in 0.05% TFA (solvent B) from 0 to 80% over 100 min. The eluted fractions of peptides and proteins were recorded on a photodiode array detector (Dionex UltiMate 3000 RS) directly coupled with an HPLC system set at 215 nm.
2.7. In vitro antidiabetic activity via inhibition of enzymatic markers
In vitro antidiabetic activities of CSPH and FSPH were investigated by α-glucosidase and dipeptidyl peptidase IV (DPP-4) inhibitory activity using the earlier reported procedures of Baba et al. (2021c) and Mudgil et al. (2022a), respectively. The α-glucosidase inhibitory capacity of PHs was determined by suspending 80 μL of samples in 0.1 M phosphate buffer (pH 6.8) containing 0.01 M of 4-nitrophenyl-α-D-glucopyranoside (p-NPG) solution. The resulting solution was reacted with 20 μL enzyme solution (0.2 U/mL) at 37°C for 30 min.
To determine the DPP-4 activity, PH samples were diluted to concentrations ranging from 0.025 to 2.5 mg/mL with HPLC water for the assay. Briefly, sample aliquots (25 μL) were applied to a 96-well microplate, and the reaction substrate (Gly-Pro-pNA) of final concentrations of 0.2 mM was added. Thereafter, the mixture was reacted using 0.0025 units mL−1 DPP-4 diluted in 0.1 M Tris-HCl buffer (pH 8.0). Diprotin A at final concentrations ranging from 0.0125 to 12.5 μg/mL was taken to be the positive control, and the negative control was composed of Gly-Pro-pNA and 0.1 M Tris-HCl buffer (pH 8.0). The microplate reader was set at 405 nm to measure the absorbance of the mixture. The percentage of enzyme inhibition was measured using Equation 2. The percentage inhibition was plotted against the concentration of test samples (protein equivalent mg/ml), and half-maximal inhibitory concentration (IC50) values were determined and expressed in μg mL−1 based on the curve.
Here A is the control reaction containing Enzyme, substrate and buffer; B is the control blank containing buffer and substrate only; C is the test reaction containing sample, enzyme, substrate and buffer; and D is the test reaction blank containing sample, substrate and buffer.
2.8. In vitro anti-obesity activity via inhibition of enzymatic markers
The anti-obesity activity of PHs was conducted using the in vitro cholesteryl esterase (CE) and pancreatic lipase (PL) assays according to the previous procedures of Mudgil et al., 2022c and Alnuaimi et al. (2023). The enzyme inhibition was calculated as per Equation (2) and expressed in IC50 (μg/mL) value.
2.9. Antioxidant activity
The ABTS and DPPH radical scavenging activities and ferric reducing antioxidant power (FRAP) activities of the PHs were determined following the methods described in Al-Shamsi et al. (2018) and expressed as micromoles of Trolox equivalents.
2.10. Statistical analysis
The PHs were produced in three batches, and measurements were taken in triplicates. SPSS software (version 28, Chicago, IL, USA) was used to perform one-way ANOVA, and data were subjected to Tukey's multiple range test to determine the significant difference at a significance level of p < 0.05.
3. Results and discussion
3.1. Degree of hydrolysis
To measure the effectiveness of protein hydrolysates (PHs) for their application in the food industry, free amino nitrogen (FAN) content, among other parameters, e.g., solubility, molecular weight, and peptide length, is an important parameter to be studied. As such, it is imperative to monitor the proteolysis reaction of PHs by controlling the extent of hydrolysis reaction. During the production of PHs, enzyme: substrate (E:S) ratio, reaction time, and temperature play a role in achieving the desired level of FAN; thus, these factors should be taken into consideration. The influence of all three enzymes employed for hydrolysis of CSP and FSP on the FAN values are presented in Figures 1A, B. The results suggested that the time of hydrolysis significantly impacted the FAN values. For instance, as the hydrolytic reaction time was increased from the minimum reaction time (2 h) to the maximum time (6 h), the degree of hydrolysis (DH) of the test enzymes increased as shown by increased FAN values, which suggests a time-dependent proteolytic reaction.
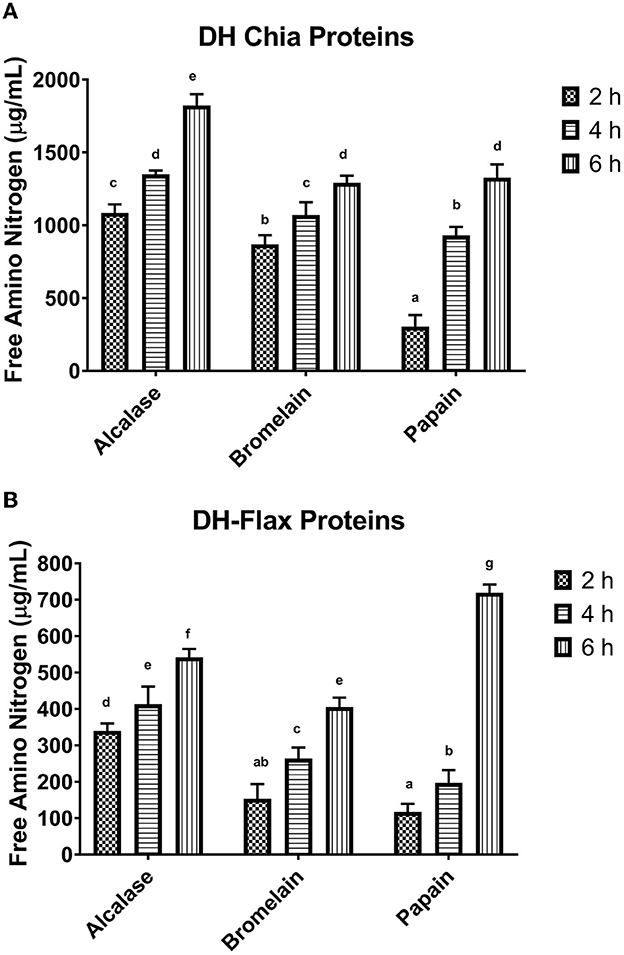
Figure 1. Free amino nitrogen (μg NH3 g−1) of chia (A) and flax (B) seed protein hydrolysates as obtained through hydrolysis by alcalase, bromelain, and papain hydrolysis after 2, 4, and 6 h of hydrolysis. Different small letters in the column indicate significant difference between the different analyzed samples (p < 0.05).
As depicted in Figure 1A, extended hydrolysis of CSP up to a 6-h period released more FAN in comparison to FSP, as evidenced by higher FAN content (1978 μg/mL, 1400 μg/mL, and 1455 μg/mL for alcalase, bromelain, and papain, respectively). Similarly, the FAN content of FSPH also exhibited the same trend, i.e., increased FAN content with the progression of hydrolytic reaction (Figure 1B). Taking all together, the DH data from CSPH and FSPH revealed that increasing the biocatalysis reaction time enabled the hydrolysis of the seed proteins significantly to a higher degree. A possible reason for the high FAN values of CSPH and FSPH could be the high availability of reactive sites. This is because increasing the hydrolysis reaction time upon degradation of native proteins is believed to have resulted in higher reactivity of the enzymes, thus facilitating the degradation of manifold peptide bonds (Ambigaipalan and Shahidi, 2015). Furthermore, the results demonstrate that papain biocatalysis exhibited the strongest hydrolyzing effect on FSP, in which the DH was significantly higher than those PHs produced from bromelain and alcalase enzymes. The FAN values of CSPHs show that alcalase was more efficient at hydrolyzing CSP with their highest FAN content obtained after 6 h of hydrolysis. The enzymatic hydrolysis of both substrates (CSP and FSP) using papain displayed slow actions at some point. For instance, an initial slow hydrolytic reaction was recorded when the proteolytic reaction duration progressed from 2 to 4 h in CSP before showing a rapid increase after 4 h. Similarly, FSP showed rapid hydrolysis during the first 2-h biocatalytic reaction before exhibiting a slow gradual increasing DH when the reaction time progressed to 6 h. The observed results indicate that only a few peptide bonds disintegrate at these slow hydrolysis times, which possibly lowered the hydrolysis efficiency. Interestingly, bromelain and alcalase sharply degraded most of CSP within the first 2 h of hydrolysis. However, only a slight difference was noticed at 4 h and 6 h among different hydrolysates with alcalase being slightly more active than bromelain.
Moreover, enzymatic hydrolysis of CSP had significantly (p < 0.05) higher FAN values when compared to FSP under comparable hydrolysis conditions (Figures 1A, B). One can infer that the hydrolysis efficiency of proteinaceous substrates, especially food proteins, perhaps depends on the substrate and not only on the enzyme selectivity and hydrolysis period. A similar observation was made by other researchers showing that the polypeptide chains of bovine caseins are more prone to enzymatic cleavage than camel caseins (Salami et al., 2008; Mudgil et al., 2022c). Overall, the results from this finding support the existing facts that proteolytic enzyme is effective at hydrolyzing proteinaceous substrate. Quinoa protein hydrolysates produced by Galante et al. (2020) using serine protease sourced from Aspergillus niger after 3 h of hydrolysis exhibited a DH value of 17%. In another study, the rate of hydrolysis in chia expeller papain hydrolysate, however, increased rapidly (by 14.3 % DH) in the first 40 min and then remained constant (Cotabarren et al., 2019). Another study reported higher DH values (42.62 %) of alcalase-catalyzed hydrolysates of flaxseed protein (Logarušić et al., 2020). Similarly, Aluko and Monu (2003) indicated the production of quinoa protein hydrolysates with a DH value of 48% by alcalase catalysis after 4 h of hydrolysis. A previous study on chia seed protein reported that alcalase hydrolysis showed a DH of 19.0 % after 180 min (San Pablo-Osorio et al., 2019). A similar extent of hydrolysis was reported for papain-catalyzed quinoa proteins which yielded free amino acids of 8.5 mg/mL following a 3-h biocatalysis reaction (Nongonierma et al., 2015). The significant difference in the DH value of enzyme-catalyzed CSPHs and FSPHs in comparison with those reported in previous studies was expected as different substrates and enzymes were involved. This disparity indicates that the characteristics of enzymes including specificity, substrate affinity, reaction rates, and catalysis time have a major influence on their proteolytic reaction (Kamal et al., 2021).
3.2. Profiling of CSPH and FSPH with RP-UPLC
Among the features that describe the bioactivity of peptides and/or protein hydrolysate, amino acid composition, peptide profile, and hydrophobicity are well known to be important. In the present study, CSPH and FSPH generated using alcalase, bromelain, and papain were subjected to peptide profiling using the RP-UPLC technique. Purposely, unhydrolyzed CSP and FSP were analyzed with RP-UPLC in comparison with their hydrolyzed proteins with a gradient elution over a retention of 44 min, as shown in Figures 2, 3. Based on the analysis of chia seeds revealed by RP-UPLC, unhydrolyzed CSP revealed three major peaks eluted between 0 and 2 min, 12 and 16 min, and 22 and 24 min, respectively (Figures 2A–C). Differently, among CSPHs, alcalase-catalyzed hydrolysates displayed a different propensity compared to their bromelain- and papain-catalyzed counterparts, in which several small peaks were eluted around a wide range of retention times. However, in bromelain-catalyzed CSPHs, a few small peaks eluted at a later elution time of 20 min and 32 min. Similarly, small peaks eluted at a later elution time (32 min) were observed in papain-catalyzed hydrolysates. Hanafi et al. (2018) reported that high hydrophilic or polar molecules elute earlier while hydrophobic or non-polar fractions would elute later. This suggests that alcalase-catalyzed CSPHs tend to possess both hydrophobic and hydrophilic amino acid sequences within their sequences. Moreover, higher protein hydrolysis exhibited by alcalase-catalyzed CSPHs in comparison with other hydrolysates could be associated with the greater early-eluted peptide peaks intensity displayed (Figure 2A). These results corroborate with high DH demonstrated in alcalase-catalyzed CSPHs as shown in Figure 1A.
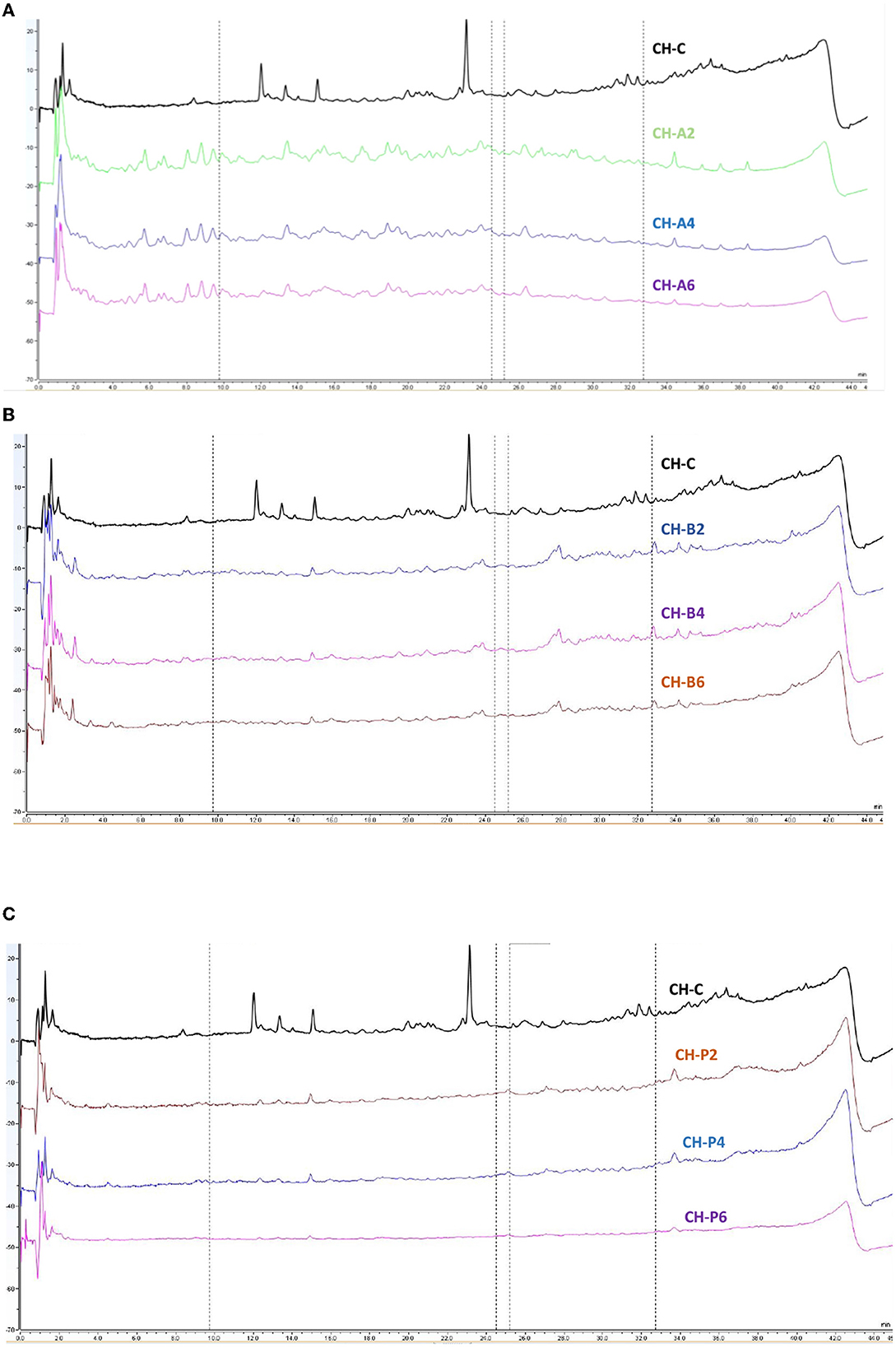
Figure 2. RP-UPLC peptide profiles of chia seeds protein hydrolysates generated by alcalase (A), bromelain (B), and papain (C). CH-C, CH-A, CH-B, and CH-P represent unhydrolyzed chia seeds, hydrolysates generated by alcalase, bromelain, and papain for 2, 4, and 6 h of hydrolysis reaction time, respectively.
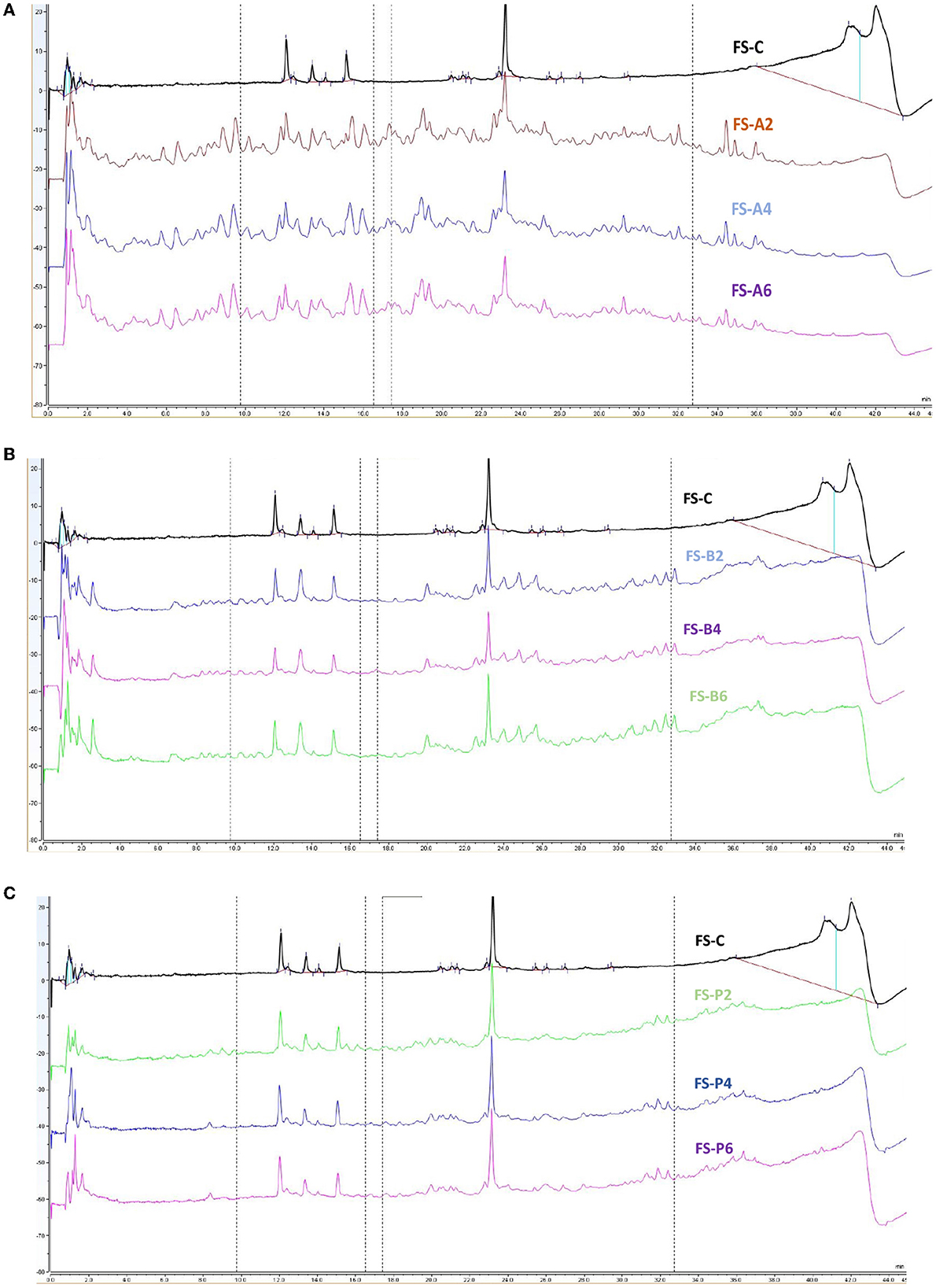
Figure 3. RP-UPLC peptide profiles of flaxseed protein hydrolysates generated by alcalase (A), bromelain (B), and papain (C). FS-C, FS-A, FS-B, and FS-P represent unhydrolyzed flax seed, hydrolysates generated by alcalase, bromelain, and papain for 2, 4, and 6 h of hydrolysis reaction time, respectively.
For flaxseeds, the undigested FSP showed a major peak in the region between 0 and 2 min, 12 and 16 min, and 22 and 24 min, respectively (Figures 3A–C). After the hydrolysis reaction with bromelain and papain, a more or less similar profile was obtained with some new peptide peaks being eluted between 24 and 38 min of retention time (Figures 3B, C). However, for alcalase-catalyzed FSPHs, distinct intense peptide peaks were eluted over an extensive retention time ranging from 2.0 to 36.0 min, thus reflecting higher range of hydrophobicity of the peptides and diverse peptide being generated. Alcalase-catalyzed FSPHs also showed the highest DH after 2 h and 4 h reaction time (Figure 1B). Previous studies have also pointed out that eluted peaks obtained halfway or at the end of the retention time of RP-HPLC confer high inhibitory activities due to the high amount of hydrophobic amino acid within their sequences (Rui et al., 2013; Hanafi et al., 2018). As unhydrolyzed FSP and FSPHs eluted peaks around these retention peaks regardless of the proteases, this implies that flaxseed contains inhibitory peptides. Overall, the data observed from the RP-UPLC profiling of proteases-generated hydrolysates clearly showed that they might contain a wide range of hydrophobic amino acids. However, further fractionation of the derived hydrolysates should be carried out to validate the bioactivity of the peptides.
3.3. Antidiabetic activity
3.3.1. α-glucosidase inhibitory activity
High-level blood glucose is one of the prevailing metabolic disorders throughout the world. In the last few decades, α-glucosidase inhibitors have already been used as antidiabetic drugs in clinical therapy due to their ability to inhibit postprandial blood glucose elevation. To confirm the antidiabetic activities of peptides released from CSPHs and FSPHs, their α -glucosidase inhibitory activity was investigated. The α-glucosidase inhibitory potency of CSPHs and FSPHs generated using three proteolytic enzymes and their intact proteins are presented in Tables 1, 2. The hydrolysis of chia seed and flaxseed proteins led to a multifold improvement in the IC50 values of α-glucosidase, suggesting an overall improved inhibitory potential. Based on the data recorded, enzyme-catalyzed CSPHs and FSPHs indicated higher inhibitory potential for α-glucosidase than their native intact proteins. For CSPHs, maximum inhibitory potential toward α-glucosidase was displayed in bromelain (CS-B6) with an IC50 of 260.92 μg/mL, followed by papain hydrolysate (CS-P6; 264.48 μg/mL), while the activity of papain-digested hydrolysates after 4 h of hydrolysis was minimal (CS-P4; 283.25 μg/mL), as detailed in Table 1. Similarly, among FSPHs, hydrolysates catalyzed by papain after 6-h biocatalysis showed the highest activity toward α-glucosidase with an IC50 value of 562.02 μg/mL, followed by FS-B4 and FS-B6 with IC50 values of 562.02 μg/mL and 573.02 μg/mL, respectively (Table 2).
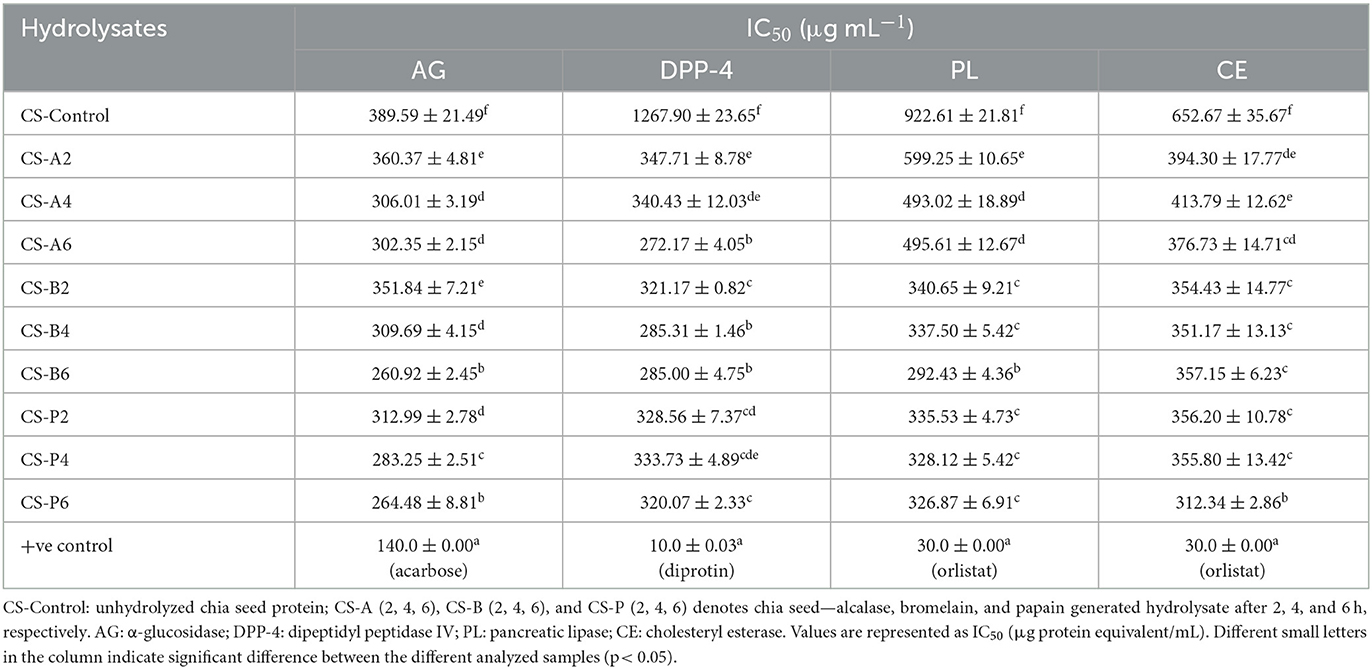
Table 1. In-vitro antidiabetic and anti-obesity inhibitory activities of chia seed protein hydrolysates obtained using different proteolytic enzymes.
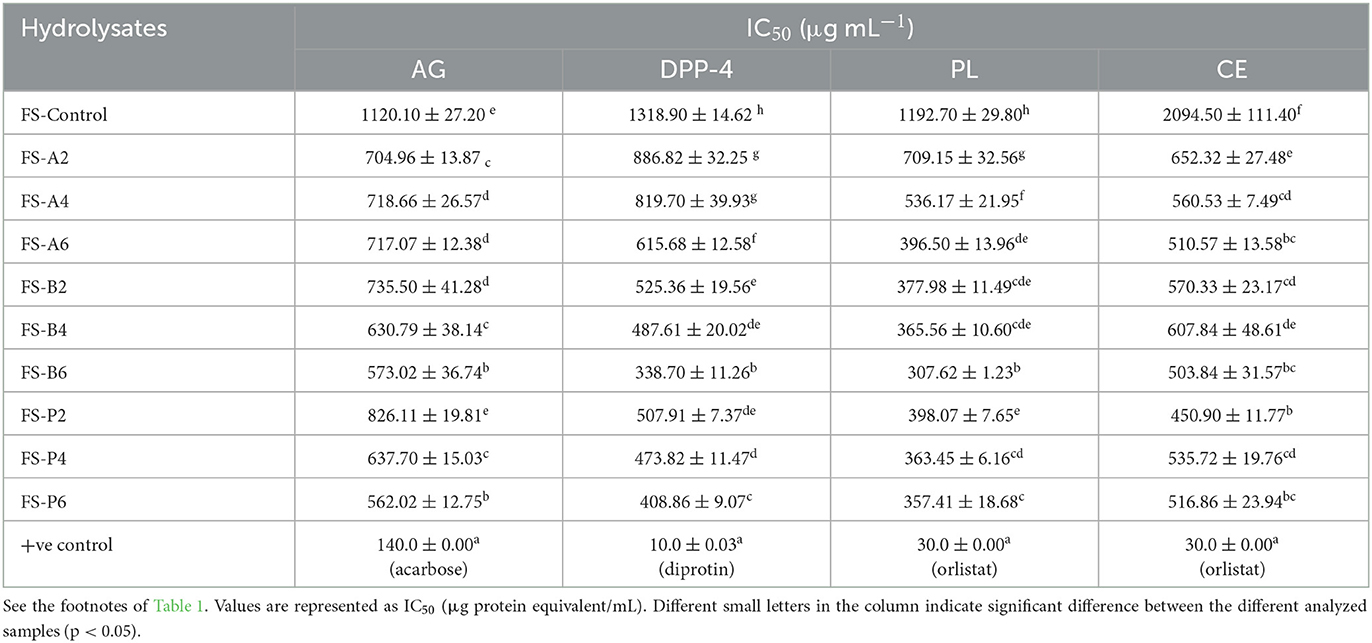
Table 2. In-vitro antidiabetic and anti-obesity inhibitory activities of flax seed protein hydrolysates obtained using different proteolytic enzymes.
As prolonged hydrolysis reaction time at 6 h was found to exert the strongest activity, having shown a continuous decline in the IC50 values with the progression of hydrolysis, our study opines that employing extended hydrolysis time is desirable. Therefore, hydrolyzing chia and flaxseed proteins at 6 h is best suited for producing peptides with potent α-glucosidase inhibitions. However, the content of FAN released from the hydrolysates as a function of the reaction time showed no correlation with the resulting α-glucosidase inhibition. Given the significance of shorter peptides in accessing the active sites of specific enzymes, we assumed that alcalase-catalyzed hydrolysates would possess the maximum α-glucosidase inhibitions because of the higher FAN content exhibited. Surprisingly, papain-catalyzed PHs released the most potent inhibitory activity despite having a lower content of FAN, and this suggests that protease specificity or the mechanism of cleaving peptide bonds and their sequence played an important role. This finding aligns with the dogma that plant proteases have broad spectra of amino acid specificity (Ji et al., 2020) and are more effective in releasing α-glucosidase inhibitory peptides. However, experimental studies related to the α-glucosidase inhibitory potential of chia seed and flaxseed proteins are limited from what we know. However, according to the in silico analysis conducted, the presence of some peptides with potential α-glucosidase inhibitory peptides among proteins was linked with general carbohydrates and lipid metabolism (Grancieri et al., 2019; Valenzuela Zamudio et al., 2022). Many other studies, however, have identified the α-glucosidase inhibitory potential of plant protein-derived hydrolysates (Vilcacundo et al., 2017; Ayyash et al., 2018; Obaroakpo et al., 2019; Mudgil et al., 2020; Valenzuela Zamudio et al., 2022). For instance, a study conducted on buckwheat protein hydrolysates via alcalase-catalyzed hydrolysis produced α-glucosidase inhibition of ~90% (Tao et al., 2019). In the same way, simulated gastrointestinal digestion of quinoa protein displayed α-glucosidase inhibitory activity with IC50 values in the range of 1.45–1.81 mg protein/mL (Vilcacundo et al., 2017). Similarly, bromelain-catalyzed amaranth protein hydrolysates yielded peptides with α-glucosidase IC50 of 1.17 mg protein equivalent (Kamal et al., 2021).
3.3.2. DPP-4 inhibitory activity
For further validation purposes of the hydrolysates, the DPP-4 inhibitory activities of both substrates were determined. Of all the proteases used for the biocatalysis of chia seeds, alcalase-catalyzed hydrolysates obtained after 6 h of hydrolysis (CS-A6) displayed the highest DPP-4 inhibitory potential with an IC50 value of 272.17 μg protein/mL, followed by bromelain-catalyzed hydrolysates at 4 h (CS-B4) and 6 h (CS-B6), respectively. The obtained IC50 results in the present study are equivalent to those obtained by another researcher, where the authors observed a similar DPP-4 extent of inhibition for alcalase-digested seed proteins. In one study reported by Wang et al. (2015), oat glutelins catalyzed using alcalase produced more pronounced DPP-IV inhibition displaying an IC50 value of 0.13 mg/mL. The authors concluded that alcalase was more potent in hydrolyzing cereal proteins; therefore, it has the potential worth considering as an antidiabetic functional ingredient (Wang et al., 2015). Moreover, a significant hypoglycemic effect in streptozotocin-induced diabetic mice was observed for alcalase-catalyzed amaranth glutelin hydrolysates due to the maximum in vitro DPP-4 inhibitory activity (IC50 of 0.12 mg/mL) displayed (Jorge et al., 2015). In another study conducted on pearl millet protein hydrolysates derived via alcalase hydrolysis following a 9-h reaction, an IC50 value of 3.44 μg/mL was reported for the DPP-4 activity (Mudgil et al., 2022a). As can be observed from this current study as well as the previous studies, alcalase can cause prominent hydrolysis of its substrates that results in shorter peptides with potent DPP-4 inhibition.
Differently, among FSP and their hydrolysates, maximum DPP-4 inhibition was displayed by bromelain (FS-B6; IC50 = 338.70 μg/mL), followed by FS-P6 (IC50 = 408.86 μg/mL) and FS-P4 (IC50 = 473.82 μg/mL). The DPP-4 inhibition activity was lowest for alcalase-generated hydrolysates among all the FSPHs with the inhibition capacity in terms of IC50 values ranging between 615.68 and 886.82 μg/mL and could be attributed to the lower values of FAN content among these hydrolysates. In particular, hydrolysates from chia seeds displayed better DPP-4 IC50 values in comparison with their flaxseed counterparts. Moreover, all the proteases used in this study could release inhibitory peptides from both chia and flaxseed against DPP-4 in comparison with their intact proteins that recorded less potent ability, i.e., high IC50 values (1267.9 and 1318.9 μg/mL for CSP and FSP, respectively).
3.4. Anti-obesity activity
3.4.1. Pancreatic lipase inhibition activity
Pancreatic lipase plays a vital role in the metabolism of dietary cholesterol esters. In obesity, inhibiting the enzyme is a major panacea in reducing its incidence. Lipase inhibitors suppress the release of hydrolyzed fats into the digestive system (Lunagariya et al., 2014). The results regarding the inhibitory concentration of CSP and FSP (unhydrolyzed) and their hydrolysates are shown in Tables 1, 2, respectively. It appears that the pancreatic lipase inhibitory potentials of enzyme-catalyzed hydrolysates from chia seed and flaxseed proteins were higher compared with the unhydrolyzed proteins. This observation confirms the release of pancreatic lipase inhibitory peptides following the biocatalysis of proteins and indicates that the proteolytic reaction of enzymes was the primary effect for the release of relatively short peptides (Alnuaimi et al., 2023). The biocatalysis reaction duration was also measured concerning the release of pancreatic lipase inhibitory properties. As shown in the data (Tables 2, 3), there was a steadily declining trend in IC50 values (enhanced inhibition) with increased reaction time for both hydrolysates produced from chia seed and flaxseed proteins. The constant rise observed in the pancreatic lipase inhibitory activity of the hydrolysates clearly showed that prolonged reaction time facilitated the release of higher concentrations of shorter peptides with smaller MW fractions. Interestingly, bromelain-catalyzed CSPHs and FSPHs after 6 h reaction time yielded more potent lipase inhibitors (IC50 = 292.43 μg/mL and 307.62μg/mL, respectively, for CSPH and FSPH). On a similar note, alcalase-catalyzed hydrolysates yielded the minimum lipase inhibitory activity (CSPH IC50 = 599.25 μg/mL: FSPH IC50 = 709.15 μg/mL) after 2 h of hydrolysis. The obtained results demonstrated that potent inhibition was at its highest at extended hydrolysis time at 6 h when the hydrolysates are likely to have fully degraded. Moreover, comparing the potency of chia seed and flaxseed proteins in generating pancreatic lipase inhibitory peptides, increased inhibition with minimum IC50 values, implying a very strong inhibition potential, was recorded in CSPHs. This could be explained by the high DH values displayed by CSPHs (Figure 1A) causing the release of encrypted peptides, which resulted in increased potency toward pancreatic lipase. There are scant studies on the pancreatic lipase inhibitory potentials of seed protein. However, higher pancreatic lipase inhibitory activity with an IC50 of 12.72 μg/mL was derived from the extracts of sorghum (Irondi et al., 2019). Similarly, pepsin biocatalysis of tea proteins after 3 h of hydrolysis time demonstrated pancreatic lipase inhibition showing an IC50 value of 0.153 mg/mL (Wang et al., 2022). Overall, hydrolysates generated from both chia seed and flaxseed proteins suggest that they possess anti-obesity activity via pancreatic lipase inhibition.
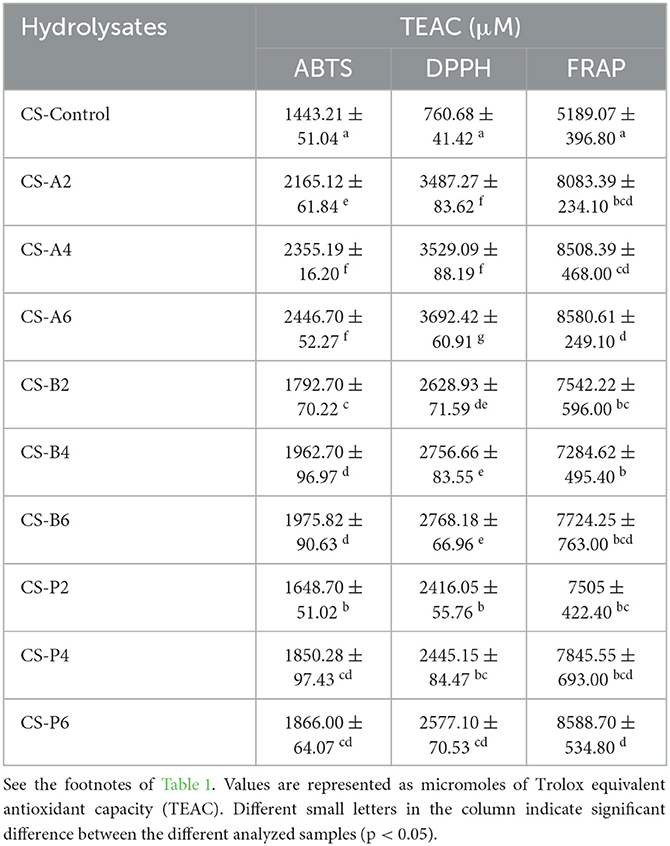
Table 3. Antioxidant activities of chia seed protein hydrolysates obtained using different proteolytic enzymes.
3.4.2. Cholesteryl esterase inhibition activity
In addition to the metabolism of dietary cholesterol, cholesterol esterase catalyzes the hydrolysis of dietary cholesterol ester in the lumen, thus accelerating the liberation of free cholesterol. The cholesterol esterase enzyme boosts the bioavailability of derived cholesterol by incorporating free cholesterol with cholesterol present in the primary bile secretions to form micelles, which are transported to the enterocytes for absorption (Heidrich et al., 2004). Clinical trials have demonstrated that inhibitors of cholesterol esterase that limit the absorption of free cholesterol liberated are advantageous for managing the incidence of obesity. Many bioactive compounds derived from plants such as phytic acids and dietary fibers are extensively elaborated for their role in inhibiting cholesterol esterase. However, the clinical functions of proteins and peptides in the inhibition of cholesteryl esterase are still under-explored. Cholesteryl esterase inhibition properties of CSP, FSP, and their hydrolysates as IC50 values in μg protein/mL are shown in Tables 1, 2. Low inhibition activity toward cholesteryl esterase was noticed with CSP and FSP in their intact form with IC50 values of 652.67 and 2094.5 μg protein/mL, respectively. Overall, the IC50 values nearly declined by 2-fold with papain-catalyzed hydrolysates (i.e., CS-P6 and FS-P2) displaying the highest cholesteryl esterase inhibition activities (312.34 and 450.90 μg protein/mL, respectively). As mentioned previously, there are sparse studies related to the inhibition of cholesteryl esterase by protein and peptides; however, recent reports had proven that hydrolysates derived from plant proteins have a high capacity to inhibit cholesterol esterase enzyme activity. It is worth revealing that the present study is among the first few studies that have explored the cholesteryl esterase inhibition potential of chia seed and flaxseed proteins and their hydrolysates. The IC50 values of cholesterol esterase inhibition activity recorded for CSPHs and FSPHs align with the values previously reported for bromelain-catalyzed amaranth protein hydrolysates (IC50 value of 0.47 mg/mL) by Ajayi et al. (2021).
Conversely, our observed IC50 values against cholesteryl esterase are less potent than those obtained from hydrolysis of seed protein from bitter apple (Citrullus colocynthis) via chymotrypsin, alcalase, and bromelain. The results from a very recent study indicated that Citrullus hydrolysates obtained from chymotrypsin, bromelain, and alcalase biocatalysis possessed high in vitro cholesteryl esterase inhibition at IC50 value of 13.68 μg/mL, 14.55 μg/mL, and 16.5 μg/mL, respectively (Alblooshi et al., 2023). Similarly, Alnuaimi et al. (2023) studied the anti-hypercholesterolemic activity of hydrolysates derived from young and mature soybean and reported that flavourzyme hydrolysis effectively inhibited cholesteryl esterase with an IC50 value of 27.08 and 32.96 μg/mL, respectively. In another investigation, alcalase-generated date seed protein hydrolysates presented efficient IC50 performances (70.35 μg/mL) (Mostafa et al., 2022). Similarly, tea protein hydrolysates obtained from pepsin hydrolysis indicated an IC50 of 0.549 mg/mL (Ye et al., 2023). The authors also identified three novel tripeptides (FLF, QIF, and IYF) that interacted with the active sites of cholesteryl esterase enzymes by van der Waals forces, hydrophobic interactions, hydrogen, and π-π bonds are responsible for their hypolipidemic activities. Usually, it is indicated that peptides with cholesterol inhibitory action exert their effect by interrupting the formation of micelles. Moreover, various peptides, specifically the ones with hydrophobic residues at their terminal ends, are reported to interact with cholesteryl esterase active sites, i.e., a catalytic triad (comprised of Asp 320, His435, and Ser194) and an oxyanion hole (comprised of Ala 108, Ala 195, and Gly107) well-known to perform a vital role in the catalytic function by disrupting active site structure, consequently leading to enzyme inhibition. However, to confirm the mechanism of action for CSPHs and FSPHs, further investigations into the identification of peptides and further study related to molecular docking and synthetic peptides are warranted.
3.5. Antioxidant activity
Chia seeds and flaxseeds are considered to possess high antioxidant capacity because it is loaded with a high amount of phenolic compounds (Orona-Tamayo et al., 2015). However, the antioxidant activities of chia seed- and flaxseed-derived peptides from their protein fractions are not fully evaluated to date. Accordingly, in this current study, the ABTS, DPPH radical scavenging, and FRAP activities of CSP, FSP, and their hydrolysates were performed to provide more insights into their antioxidant activities, and the results obtained are presented in Tables 3, 4, respectively.
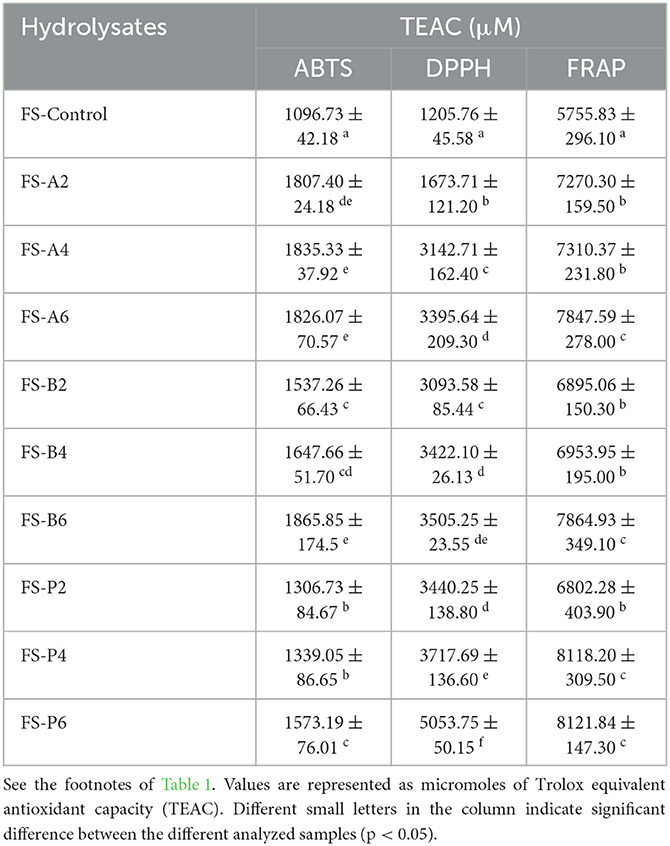
Table 4. Antioxidant activities of flax seed protein hydrolysates obtained using different proteolytic enzymes.
3.5.1. ABTS and DPPH radical scavenging activity
The ABTS and DPPH activities of hydrolysates produced from chia seed and flaxseed proteins via biocatalysis exhibited significant improvement in their radical scavenging activities compared with their intact proteins. The results regarding the ABTS radical scavenging activities of intact CSP and FSP showed lower values of 1443.21 ± 51.04 and 1096.73 ± 42.18 μM TEAC, respectively. However, higher scavenging activities ranging from 1792.70 to 2446.70 μM TEAC and 1306.73 to 1865.85 μM TEAC were recorded in CSPHs and FSPHs, respectively. This further supports the assertion that the presence of small MW peptides can enhance the antioxidant activity of seeds. Among all the CSPHs, alcalase-catalyzed hydrolysates showed the highest TEAC values to inactivate the ABTS radicals. In particular, the trend for scavenging activity displayed by CSPHs showed a parallel increment with the progression of a substrate hydrolysis reaction. Here, the maximum ABTS scavenging activity was displayed by CS-A6, which correlated with the results recorded in the extent of hydrolysis (Section 3.1). The results recorded in FSPHs were contrary to CSPHs, in which bromelain-catalyzed hydrolysate after 6 h of hydrolysis (FS-B6) displayed the maximum scavenging activity, however, which was not different from the activities of FS-A6 and FS-A4 (P > 0.05).
In yet another aspect of antioxidant activity, the DPPH assay was carried out to establish the scavenging activity of CSP, FSP, and their hydrolysates (Tables 3, 4). A similar trend of improved radical scavenging activities was recorded in CSPHs and FSPHs, compared with their intact proteins. In fact, as shown in Tables 3, 4, enzymatic hydrolysis of the proteinaceous substrates significantly improved the scavenging of free radicals by approximately 5-fold and 4-fold for CSP and FSP, respectively. For CSPHs, hydrolysate CS-A6 showed the highest DPPH scavenging activity for CSPH (3692.42 ± 60.91 μM TEAC), whereas hydrolysate CS-P2 demonstrated the least scavenging activity (2416.05 ± 55.76 μM TEAC). Contrarily, maximum DPPH scavenging activity obtained in FSPHs was obtained in hydrolysate FS-P6 (5053.75 ± 50.15 μM TEAC), followed by FS-P4 (3717.69 ± 136.6 μM TEAC), while the activity displayed by hydrolysate CS-A2 was the lowest (1673.71 ± 121.2 μM TEAC). Similar effects were demonstrated by Orona-Tamayo et al. (2015) where they observed an ABTS IC50 value of 266.5 mg/mL in papain-catalyzed chia flour showing the most superior activity (Orona-Tamayo et al., 2015). Cotabarren et al. (2019) found that a 3–15-kDa peptide fraction of chia expeller papain hydrolysate exhibited IC50 values within the range of 562.3 μg/ml to 398.1 μg/mL for DPPH and 44.7 μg/mL to 25.1 μg/mL for ABTS, respectively. Moreover, the antioxidant activity of peptide fractions from alcalase hydrolyzed flaxseed showed 62% DPPH radical scavenging at 50 mg/mL of concentration (Sarabandi and Jafari, 2020). Overall, these findings suggest that the biocatalysis reaction appears to have increased the ABTS radical scavenging activity compared with the DPPH radical scavenging of CSPHs and FSPHs. Differences in the stereoselectivity of the radicals with different interaction mechanisms may have been be the cause for the difference among the scavenging activities (Al-Shamsi et al., 2018).
In addition, the concentrations of ABTS and DPPH activities of chia seed and flaxseed hydrolysates could be highly influenced by the length of the proteolytic reaction, because shorter peptides generated at extended time could hinder their ability to donate an electron. In addition to the reaction time, the presence of high amounts of hydrophobic amino acids within the peptides and rich acidic amino acid and their amides (Glu, Asp, and Asn) in chia globulin proteins could degrade their scavenging ability (Siow and Gan, 2016). In particular, in the current study, papain- and alcalase-catalyzed CSPHs and FSPHs scavenged DPPH and ABTS radicals effectively, which suggests that hydrolyzing chia seed and flaxseed proteins improved the antioxidant activity more than their parent proteins.
3.5.2. Ferric reducing antioxidant power
The ability of hydrolysates and/or cleaved peptides to donate electrons and protons are usually measured from their FRAP or reducing antioxidant potential (Sonawane and Arya, 2017). As shown in Tables 3, 4, significantly higher reducing antioxidant potentials were obtained in CSPHs and FSPHs compared with their intact proteins (i.e., CSP and FSP). Most of the hydrolysates exhibited nearly similar TEAC values with papain and alcalase obtained at 6 h displaying the maximum activity. Comparing the different proteolytic enzymes, alcalase- and papain-digested CSPs and FSPs showed greater FRAP values, while bromelain-catalyzed hydrolysates also showed comparable FRAP potential displaying increased TEAC values concerning the time of hydrolysis (p > 0.05). The FRAP values of hydrolysates yielded from enzyme-catalyzed CSP and FSP in our study were contradictory to the findings of Villanueva-Lazo et al. (2021). They reported a significant downward shift in the FRAP values of chia proteins digested with alcalase and flavourzyme. One of the possible reasons for these variations is the different duration and degree of hydrolysis reaction as well as sequential differences between the peptides obtained. However, the results are comparable to those obtained from pearl millet protein hydrolysates where hydrolysis by trypsin enhanced the FRAP activity of the generated peptides (Agrawal et al., 2016). Additionally, in an earlier study, the degree of hydrolysis of hydrolysates derived from Mexican chia seeds catalyzed by an alcalase–flavourzyme mixture showed a positive correlation with the FRAP activity (Chim-Chi et al., 2018). Apparently, enzyme-catalyzed hydrolysates from CSP and FSP contain higher reducing potential; therefore, they could strongly confer primary and secondary antioxidant properties.
4. Conclusion
Chia seeds and flaxseeds are consumed largely as functional food products owing to their benefits through omega fatty acid contents, minerals, and several other bioactive compounds that contribute to health.
In this study, a biocatalysis reaction involving three proteolytic enzymes was applied to generate hydrolysates from chia seed and flaxseed proteins as well as to evaluate the antidiabetic, antioxidant, and anti-obesity activity of the obtained hydrolysates. The degree of hydrolysis of the enzyme-catalyzed chia seed and flaxseed proteins increased as a function of time, as evidenced by notable FAN values. Overall, the biocatalysis reaction of both substrates highly increased their α-glucosidase, DPP-4, pancreatic lipase, and cholesteryl esterase inhibitory activities. Hydrolysates had higher α-glucosidase, DPP-4, pancreatic lipase, and cholesteryl esterase inhibitory activities compared to intact fractions. Among the hydrolysates, bromelain-catalyzed hydrolysates of chia seed and flaxseed proteins had higher α-glucosidase, DPP-4, and pancreatic lipase inhibitory activities. In terms of antioxidant properties, alcalase-catalyzed hydrolysates displayed higher values than bromelain- and papain-catalyzed hydrolysates. It is interesting that CSPHs recorded the most potent antidiabetic and anti-obesity activities as well as maximum ABTS radical scavenging activity than FSPHs. These findings have proven that chia seed and flaxseed proteins and their generated enzyme-catalyzed hydrolysates might be beneficial in mitigating blood glucose and cholesterol, thus acting as a potential source of functional ingredients with glucose and cholesterol-lowering activities. Nonetheless, further research regarding the in vitro and in vivo effects of the derived peptides for the observed bioactivities, as well as peptide profiling and characterization should be carried out.
Data availability statement
The original contributions presented in the study are included in the article/supplementary material, further inquiries can be directed to the corresponding author.
Author contributions
PM supervised the investigation, analyzed the data, and wrote and edited the manuscript. FA wrote the original draft and interpreted data for the study. AA and MA conducted the investigation, collected data, and wrote the first draft of the manuscript. BPS reviewed and edited the manuscript for important intellectual content. SM conceptualized and supervised the study, obtained funding, and revised the manuscript. All authors read and approved the final manuscript.
Funding
The research was funded by the SURE-plus grant (Grant number: G00003182) awarded to PI-SM by UAEU.
Acknowledgments
The authors would like to acknowledge the financial support of the United Arab Emirates University (UAEU).
Conflict of interest
The authors declare that the research was conducted in the absence of any commercial or financial relationships that could be construed as a potential conflict of interest.
Publisher's note
All claims expressed in this article are solely those of the authors and do not necessarily represent those of their affiliated organizations, or those of the publisher, the editors and the reviewers. Any product that may be evaluated in this article, or claim that may be made by its manufacturer, is not guaranteed or endorsed by the publisher.
References
Adisakwattana, S., Moonrat, J., Srichairat, S., Chanasit, C., Tirapongporn, H., Chanathong, B., et al. (2010). Lipid-lowering mechanisms of grape seed extract (Vitis vinifera L) and its antihyperlidemic activity. J. Med. Plant Res. 4, 2113–2120.
Agrawal, H., Joshi, R., and Gupta, M. (2016). Isolation, purification and characterization of antioxidative peptide of pearl millet (Pennisetum glaucum) protein hydrolysate. Food Chem. 204, 365–372. doi: 10.1016/j.foodchem.2016.02.127
Ajayi, F. F., Mudgil, P., Gan, C. Y., and Maqsood, S. (2021). Identification and characterization of cholesterol esterase and lipase inhibitory peptides from amaranth protein hydrolysates. Food Chem. 12, 100165. doi: 10.1016/j.fochx.2021.100165
Alblooshi, M., Devarajan, A. R., Singh, B. P., Ramakrishnan, P., Mostafa, H., Kamal, H., et al. (2023). Multifunctional bioactive properties of hydrolysates from colocynth (Citrullus colocynthis) seeds derived proteins: characterization and biological properties. Plant Physiol. Biochem. 194, 326–334. doi: 10.1016/j.plaphy.2022.11.026
Alnuaimi, A., Ajayi, F. F., Hamdi, M., Mudgil, P., Kamal, H., Gan, C. Y., et al. (2023). A comparative analysis of anti-lipidemic potential of soybean (Glycine max) protein hydrolysates obtained from different ripening stages: Identification, and molecular interaction mechanisms of novel bioactive peptides. Food Chem. 402, 134192. doi: 10.1016/j.foodchem.2022.134192
Al-Shamsi, K. A., Mudgil, P., Hassan, H. M., and Maqsood, S. (2018). Camel milk protein hydrolysates with improved technofunctional properties and enhanced antioxidant potential in in vitro and in food model systems. J. Dairy Sci. 101, 47–60. doi: 10.3168/jds.2017-13194
Aluko, R., and Monu, E. (2003). Functional and bioactive properties of quinoa seed protein hydrolysates. J. Food Sci. 68, 1254–1258. doi: 10.1111/j.1365-2621.2003.tb09635.x
Ambigaipalan, P., and Shahidi, F. (2015). Date seed flour and hydrolysates affect physicochemical properties of muffin. Food Biosci. 12, 54–60. doi: 10.1016/j.fbio.2015.06.001
Ayyash, M., Johnson, S. K., Liu, S. Q., Al-Mheiri, A., and Abushelaibi, A. (2018). Cytotoxicity, antihypertensive, antidiabetic and antioxidant activities of solid-state fermented lupin, quinoa and wheat by Bifidobacterium species: IN-vitro investigations. LWT 95, 295–302. doi: 10.1016/j.lwt.2018.04.099
Baba, W. N., Baby, B., Mudgil, P., Gan, C. Y., Vijayan, R., Maqsood, S., et al. (2021a). Pepsin generated camel whey protein hydrolysates with potential antihypertensive properties: Identification and molecular docking of antihypertensive peptides. LWT 143, 111135. doi: 10.1016/j.lwt.2021.111135
Baba, W. N., Mudgil, P., Baby, B., Vijayan, R., Gan, C. Y., Maqsood, S., et al. (2021b). New insights into the cholesterol esterase-and lipase-inhibiting potential of bioactive peptides from camel whey hydrolysates: Identification, characterization, and molecular interaction. J. Dairy Sci. 104, 7393–7405. doi: 10.3168/jds.2020-19868
Baba, W. N., Mudgil, P., Kamal, H., Kilari, B. P., Gan, C. Y., Maqsood, S., et al. (2021c). Identification and characterization of novel α-amylase and α-glucosidase inhibitory peptides from camel whey proteins. J. Dairy Sci. 104, 1364–1377. doi: 10.3168/jds.2020-19271
Campos, B. E., Dias Ruivo, T., Silva Scapim, D., Madrona, M. R. G. S., De, C., and Bergamasco, R. (2016). Optimization of the mucilage extraction process from chia seeds and application in ice cream as a stabilizer and emulsifier. LWT - Food Sci. Technol. 65, 874–883. doi: 10.1016/j.lwt.2015.09.021
Chim-Chi, Y., Gallegos-Tintoré, S., Jiménez-Martínez, C., Dávila-Ortiz, G., and Chel-Guerrero, L. (2018). Antioxidant capacity of Mexican chia (Salvia hispanica L.) protein hydrolyzates. J. Food Measurem. Charact. 12, 323–331. doi: 10.1007/s11694-017-9644-9
Cotabarren, J., Rosso, A. M., Tellechea, M., García-Pardo, J., Rivera, J. L., Obregón, W. D., et al. (2019). Adding value to the chia (Salvia hispanica L.) expeller: production of bioactive peptides with antioxidant properties by enzymatic hydrolysis with Papain. Food Chem. 274, 848–856. doi: 10.1016/j.foodchem.2018.09.061
Galante, M., Flaviis, D., Boeris, R. V., and Spelzini, D. (2020). Effects of the enzymatic hydrolysis treatment on functional and antioxidant properties of quinoa protein acid-induced gels. LWT 118, 108845. doi: 10.1016/j.lwt.2019.108845
Grancieri, M., Martino, H. S. D., and Gonzalez De Mejia, E. (2019). Chia Seed (Salvia hispanica L.) as a source of proteins and bioactive peptides with health benefits: a review. Compreh. Rev. Food Sci. Food Safet. 18, 480–499. doi: 10.1111/1541-4337.12423
Hanafi, M. A., Hashim, S. N., Chay, S. Y., Ebrahimpour, A., Zarei, M., Muhammad, K., et al. (2018). High angiotensin-I converting enzyme (ACE) inhibitory activity of Alcalase-digested green soybean (Glycine max) hydrolysates. Food Res. Int. 106, 589–597. doi: 10.1016/j.foodres.2018.01.030
Heidrich, J. E., Contos, L. M., Hunsaker, L. A., Deck, L. M., and Vander Jagt, D. L. (2004). Inhibition of pancreatic cholesterol esterase reduces cholesterol absorption in the hamster. BMC Pharmacol. 4, 1–9. doi: 10.1186/1471-2210-4-5
Irondi, E. A., Adegoke, B. M., Effion, E. S., Oyewo, S. O., Alamu, E. O., Boligon, A. A., et al. (2019). Enzymes inhibitory property, antioxidant activity and phenolics profile of raw and roasted red sorghum grains in vitro. Food Sci. Hum. Wellness 8, 142–148. doi: 10.1016/j.fshw.2019.03.012
Ji, D., Xu, M., Udenigwe, C. C., and Agyei, D. (2020). Physicochemical characterisation, molecular docking, and drug-likeness evaluation of hypotensive peptides encrypted in flaxseed proteome. Curr. Res. Food Sci. 3, 41–50. doi: 10.1016/j.crfs.2020.03.001
Jorge, S. S., Raúl, R. B., Isabel, G.-. L, and Edith Bernardo (2015). Dipeptidyl peptidase IV inhibitory activity of protein hydrolyzates from Amaranthus hypochondriacus L. grain and their influence on postprandial glycemia in streptozotocin-induced diabetic mice. Afr. J. Trad. Compl. Alt. Med. 12, 90–98. doi: 10.4314/ajtcam.v12i1.13
Kamal, H., Mudgil, P., Bhaskar, B., Fisayo, A. F., Gan, C. Y., Maqsood, S., et al. (2021). Amaranth proteins as potential source of bioactive peptides with enhanced inhibition of enzymatic markers linked with hypertension and diabetes. J. Cereal Sci. 101, 103308. doi: 10.1016/j.jcs.2021.103308
Kaushik, P., Dowling, K., Mcknight, S., Barrow, C. J., Wang, B., Adhikari, B., et al. (2016). Preparation, characterization and functional properties of flax seed protein isolate. Food Chem. 197, 212–220. doi: 10.1016/j.foodchem.2015.09.106
Logarušić, M., Radošević, K., Bis, A., Panić, M., Slivac, I., Gaurina Srček, V., et al. (2020). Biological potential of flaxseed protein hydrolysates obtained by different proteases. Plant Foods Hum. Nutr. 75, 518–524. doi: 10.1007/s11130-020-00841-z
Lunagariya, N. A., Patel, N. K., Jagtap, S. C., and Bhutani, K. K. (2014). Inhibitors of pancreatic lipase: state of the art and clinical perspectives. EXCLI J. 13, 897–921.
Maqsood, S., Jafar, S., Mushtaq, M., and Mudgil, P. (2021). “Bioactive Peptides Derived from Different Sources,” in Food Biopolymers: Structural, Functional and Nutraceutical Properties, eds. A. Gani and B.A. Ashwar (Cham: Springer International Publishing), 231–249.
Mohamed, S. (2014). Functional foods against metabolic syndrome (obesity, diabetes, hypertension and dyslipidemia) and cardiovasular disease. Trends Food Sci. Technol. 35, 114–128. doi: 10.1016/j.tifs.2013.11.001
Mostafa, H., Al-Ahbabi, N., Adiamo, O. Q., Mudgil, P., and Maqsood, S. (2022). Phoenix dactylifera L. seed protein hydrolysates as a potential source of peptides with antidiabetic and anti-hypercholesterolemic properties: an in vitro study. Food Biosci. 49, 101916. doi: 10.1016/j.fbio.2022.101916
Mudgil, P., Alblooshi, M., Singh, B. P., Devarajan, A. R., and Maqsood, S. (2022a). Pearl millet protein hydrolysates exhibiting effective in-vitro antioxidant, antidiabetic and anti-lipidemic properties as potential functional food ingredient. Int. J. Food Sci. Technol. 58, 3264–3272. doi: 10.1111/ijfs.16151
Mudgil, P., Baba, W. N., Alneyadi, M., Ali Redha, A., and Maqsood, S. (2022b). Production, characterization, and bioactivity of novel camel milk-based infant formula in comparison to bovine and commercial sources. LWT 154, 112813. doi: 10.1016/j.lwt.2021.112813
Mudgil, P., Baba, W. N., Kamal, H., Fitzgerald, R. J., Hassan, H. M., Ayoub, M. A., et al. (2022c). A comparative investigation into novel cholesterol esterase and pancreatic lipase inhibitory peptides from cow and camel casein hydrolysates generated upon enzymatic hydrolysis and in-vitro digestion. Food Chem. 367, 130661. doi: 10.1016/j.foodchem.2021.130661
Mudgil, P., Baby, B., Ngoh, Y. Y., Vijayan, R., Gan, C. Y., Maqsood, S., et al. (2019). Identification and molecular docking study of novel cholesterol esterase inhibitory peptides from camel milk proteins. J. Dairy Sci. 102, 10748–10759. doi: 10.3168/jds.2019-16520
Mudgil, P., Kilari, B. P., Kamal, H., Olalere, O. A., Fitzgerald, R. J., Gan, C. Y., et al. (2020). Multifunctional bioactive peptides derived from quinoa protein hydrolysates: Inhibition of α-glucosidase, dipeptidyl peptidase-IV and angiotensin I converting enzymes. J. Cereal Sci. 96, 103130. doi: 10.1016/j.jcs.2020.103130
Nongonierma, A. B., Cadamuro, C., Le Gouic, A., Mudgil, P., Maqsood, S., Fitzgerald, R. J., et al. (2019). Dipeptidyl peptidase IV (DPP-IV) inhibitory properties of a camel whey protein enriched hydrolysate preparation. Food Chem. 279, 70–79. doi: 10.1016/j.foodchem.2018.11.142
Nongonierma, A. B., and Fitzgerald, R. J. (2012). Tryptophan-containing milk protein-derived dipeptides inhibit xanthine oxidase. Peptides 37, 263–272. doi: 10.1016/j.peptides.2012.07.030
Nongonierma, A. B., Le Maux, S., Dubrulle, C., Barre, C., and Fitzgerald, R. J. (2015). Quinoa (Chenopodium quinoa Willd.) protein hydrolysates with in vitro dipeptidyl peptidase IV (DPP-IV) inhibitory and antioxidant properties. J. Cereal Sci. 65, 112–118. doi: 10.1016/j.jcs.2015.07.004
Obaroakpo, J. U., Liu, L., Zhang, S., Lu, J., Pang, X., Lv, J., et al. (2019). α-Glucosidase and ACE dual inhibitory protein hydrolysates and peptide fractions of sprouted quinoa yoghurt beverages inoculated with Lactobacillus casei. Food Chem. 299, 124985. doi: 10.1016/j.foodchem.2019.124985
Orona-Tamayo, D., Valverde, M. E., Nieto-Rendón, B., and Paredes-López, O. (2015). Inhibitory activity of chia (Salvia hispanica L.) protein fractions against angiotensin I-converting enzyme and antioxidant capacity. LWT-Food Sci. Technol. 64, 236–242. doi: 10.1016/j.lwt.2015.05.033
Prakash Nirmal, N., Singh Rajput, M., Bhojraj Rathod, N., Mudgil, P., Pati, S., Bono, G., et al. (2023). Structural characteristic and molecular docking simulation of fish protein-derived peptides: Recent updates on antioxidant, anti-hypertensive and anti-diabetic peptides. Food Chem. 405, 134737. doi: 10.1016/j.foodchem.2022.134737
Rui, X., Boye, J. I., Simpson, B. K., and Prasher, S. O. (2013). Purification and characterization of angiotensin I-converting enzyme inhibitory peptides of small red bean (Phaseolus vulgaris) hydrolysates. J. Funct. Foods 5, 1116–1124. doi: 10.1016/j.jff.2013.03.008
Salami, M., Yousefi, R., Ehsani, M. R., Dalgalarrondo, M., Chobert, J. M., Haertlé, T., et al. (2008). Kinetic characterization of hydrolysis of camel and bovine milk proteins by pancreatic enzymes. Int. Dairy J. 18, 1097–1102. doi: 10.1016/j.idairyj.2008.06.003
San Pablo-Osorio, B., Mojica, L., and Urías-Silvas, J. E. (2019). Chia seed (Salvia hispanica L.) pepsin hydrolysates inhibit angiotensin-converting enzyme by interacting with its catalytic site. J. Food Sci. 84, 1170–1179. doi: 10.1111/1750-3841.14503
Sarabandi, K., and Jafari, S. M. (2020). Fractionation of flaxseed-derived bioactive peptides and their influence on nanoliposomal carriers. J. Agric. Food Chem. 68, 15097–15106. doi: 10.1021/acs.jafc.0c02583
Siegień, J., Buchholz, T., Popowski, D., Granica, S., Osińska, E., Melzig, M. F., et al. (2021). Pancreatic lipase and α-amylase inhibitory activity of extracts from selected plant materials after gastrointestinal digestion in vitro. Food Chem. 355, 129414. doi: 10.1016/j.foodchem.2021.129414
Silva, S. V., and Malcata, F. X. (2005). Caseins as source of bioactive peptides. Int. Dairy J. 15, 1–15. doi: 10.1016/j.idairyj.2004.04.009
Singh, B. P., Aluko, R. E., Hati, S., and Solanki, D. (2021). Bioactive peptides in the management of lifestyle-related diseases: Current trends and future perspectives. Crit. Rev. Food Sci. Nutr. 1–14. doi: 10.1080/10408398.2021.1877109
Singh, B. P., Bangar, S. P., Albaloosh, M., Ajayi, F. F., Mudgil, P., Maqsood, S., et al. (2022). Plant-derived proteins as a sustainable source of bioactive peptides: recent research updates on emerging production methods, bioactivities, and potential application. Crit. Rev. Food Sci. Nutr. 2, 1–22. doi: 10.1080/10408398.2022.2067120
Singh, B. P., Vij, S., and Hati, S. (2014). Functional significance of bioactive peptides derived from soybean. Peptides 54, 171–179. doi: 10.1016/j.peptides.2014.01.022
Siow, H. L., and Gan, C. Y. (2016). Extraction, identification, and structure–activity relationship of antioxidative and α-amylase inhibitory peptides from cumin seeds (Cuminum cyminum). J. Funct. Foods 22, 1–12. doi: 10.1016/j.jff.2016.01.011
Sonawane, S. K., and Arya, S. S. (2017). Citrullus lanatus protein hydrolysate optimization for antioxidant potential. J. Food Measurement Characterization 11, 1834–1843. doi: 10.1007/s11694-017-9565-7
Tao, T., Pan, D., Zheng, Y. Y., and Jun Ma, T. (2019). “Optimization of hydrolyzed crude extract from Tartary buckwheat protein and analysis of its hypoglycemic activity in Vitro”, in IOP Conference Series: Earth and Environmental Science (New York, NY: IOP Publishing).
Urbizo-Reyes, U., Liceaga, A. M., Reddivari, L., Kim, K.-. H, and Anderson, J. M. (2022). Enzyme kinetics, molecular docking, and in silico characterization of canary seed (Phalaris canariensis L.) peptides with ACE and pancreatic lipase inhibitory activity. J. Funct. Foods 88, 104892. doi: 10.1016/j.jff.2021.104892
Urbizo-Reyes, U., San Martin-González, M. F., Garcia-Bravo, J., López Malo Vigil, A., and Liceaga, A. M. (2019). Physicochemical characteristics of chia seed (Salvia hispanica) protein hydrolysates produced using ultrasonication followed by microwave-assisted hydrolysis. Food Hydrocolloids 97, 105187. doi: 10.1016/j.foodhyd.2019.105187
Valenzuela Zamudio, F., Hidalgo-Figueroa, S. N., Ortíz Andrade, R. R., Hernández Álvarez, A. J., and Segura Campos, M. R. (2022). Identification of antidiabetic peptides derived from in silico hydrolysis of three ancient grains: Amaranth, Quinoa and Chia. Food Chem. 394, 133479. doi: 10.1016/j.foodchem.2022.133479
Vilcacundo, R., Martínez-Villaluenga, C., and Hernández-Ledesma, B. (2017). Release of dipeptidyl peptidase IV, α-amylase and α-glucosidase inhibitory peptides from quinoa (Chenopodium quinoa Willd.) during in vitro simulated gastrointestinal digestion. J. Funct. Foods 35, 531–539. doi: 10.1016/j.jff.2017.06.024
Villanueva-Lazo, A., Paz, S. M. D. L., Rodriguez-Martin, N. M., Millan, F., Carrera, C., Pedroche, J. J., et al. (2021). Antihypertensive and antioxidant activity of chia protein techno-functional extensive hydrolysates. Foods 10, 2297. doi: 10.3390/foods10102297
Wang, F., Yu, G., Zhang, Y., Zhang, B., and Fan, J. (2015). Dipeptidyl peptidase IV inhibitory peptides derived from oat (Avena sativa L.), buckwheat (Fagopyrum esculentum), and highland barley (Hordeum vulgare trifurcatum (L.) Trofim) proteins. J. Agric. Food Chem. 63, 9543–9549. doi: 10.1021/acs.jafc.5b04016
Wang, X., Ai, X., Zhu, Z., Zhang, M., Pan, F., Yang, Z., et al. (2022). Pancreatic lipase inhibitory effects of peptides derived from sesame proteins: In silico and in vitro analyses. Int. J. Biol. Macromol. 222, 1531–1537. doi: 10.1016/j.ijbiomac.2022.09.259
Keywords: flaxseed, chai seed, protein hydrolysates, antioxidant, antidiabetic, anti-obesity
Citation: Mudgil P, Ajayi FF, Alkaabi A, Alsubousi M, Singh BP and Maqsood S (2023) Flaxseed- and chia seed-derived protein hydrolysates exhibiting enhanced in vitro antidiabetic, anti-obesity, and antioxidant properties. Front. Sustain. Food Syst. 7:1223884. doi: 10.3389/fsufs.2023.1223884
Received: 16 May 2023; Accepted: 10 July 2023;
Published: 03 August 2023.
Edited by:
Husnain Raza, Shenzhen University, ChinaReviewed by:
Mehdi Mohammadian, University of Tehran, IranLena Gálvez Ranilla, Universidad Catolica De Santa Maria, Peru
Copyright © 2023 Mudgil, Ajayi, Alkaabi, Alsubousi, Singh and Maqsood. This is an open-access article distributed under the terms of the Creative Commons Attribution License (CC BY). The use, distribution or reproduction in other forums is permitted, provided the original author(s) and the copyright owner(s) are credited and that the original publication in this journal is cited, in accordance with accepted academic practice. No use, distribution or reproduction is permitted which does not comply with these terms.
*Correspondence: Sajid Maqsood, U2FqaWQubUB1YWV1LmFjLmFl