- 1Division of Agricultural Physics, ICAR-Indian Agricultural Research Institute, New Delhi, India
- 2Division of Soil Science and Agricultural Chemistry, ICAR-Indian Agricultural Research Institute, New Delhi, India
- 3Division of Agronomy, ICAR-Indian Agricultural Research Institute, New Delhi, India
- 4Division of Environmental Science, ICAR-Indian Agricultural Research Institute, New Delhi, India
The sustainability of dominant rice-wheat rotation is under threat due to numerous water-, nutrients-, weeds- and environment-related problems, mainly, due to rice cultivation in north-western Indo-Gangetic Plains (NWIGP) of India. It needs crop- and soil- appropriate management techniques with a focus on conservation agriculture (CA) that can maintain soil health which in turn is essential for long-term sustainability of intensive cereal-based systems. Thus, rice-wheat rotation may be diversified with maize as feasible substitute for rice. But, there is a dearth of comprehensive investigation on the impact of short-term CA in maize-wheat rotation, on soil quality. Hence, an attempt has been made to assess the system productivity (SP) and soil quality in a four-year-old CA-based maize-wheat rotation. Contrasting tillage as the main plot [Conventional tillage (CT), No–tillage (NT)], crop residue mulch as subplot [residue mulch (M+), no residue (M0)], and nitrogen [50 (N1), 100 (N2), 150% (N3) of recommended nitrogen dose] as sub-sub plot was laid out in split-split plot design. Soils sampled from 0–0.05, 0.05– 0.15, and 0.15–0.30 m soil layers were examined to develop a unified soil quality index (SQI) through principal component analysis (PCA) and expert opinion. The available K, P, total organic carbon (TOC), EC, bulk density (BD), dehydrogenase activity (DHA), and soil microbial biomass C (SMBC) were identified as the crucial minimum data set for SQI using PCA. Results showed that, NT and M+ plots had 10.52 and 17.39% higher mean weight diameter (MWD) than CT and M0, respectively at 0–0.05 m soil depth. NT and M+ plots increased TOC by 5.26 and 8% than CT and M0 plots, respectively in 0–0.05 m soil layer. Available K and P were found to be significantly higher in M+ plots than that of M0. NTM+ treatments improved SMBC and DHA than CTM0 treatments. The highest and lowest SQI was registered with NTM + N3 and CTM0N1, respectively. SP was significantly and positively correlated with SQI. Diversification of rice-wheat system with maize-wheat rotation following CA practices appears to have a positive impact on soil health. Therefore, CA-based maize-wheat rotation may be recommended to improve soil quality and system productivity in NWIGP region.
1. Introduction
The overwhelming concern for agricultural sustainability is the numerous changes intensive agriculture brings, including the imbalanced and excessive application of fertilizer, the environmental pollution risk, and the decline of soil and water quality. Most critically, the rice-wheat system in north-western Indo-Gangetic Plain (NWIGP) region has numerous challenges that threaten its long-term sustainability. The main issues are the depletion of natural resources (Chauhan et al., 2012), the deterioration of soil quality (Das et al., 2021), the rapid decline of the water table (Jain et al., 2021), the disruption of the ecological balance (Srinivasarao et al., 2019), and low nutrient productivity. Therefore, the region’s food security is constantly under threat, and the issue is made worse by the changes in the global environment (Fischer et al., 2002; FAO, 2005; Gupta et al., 2016). These negative elements have encouraged the development of alternative crops and farming techniques that are more resource-efficient and environmentally benign (Aulakh et al., 2012). Recently, maize-wheat crop rotation is being encouraged as a substitute to rice-wheat system since they are more suited for a variety of ecologies and produce higher yields with less water consumption (Parihar et al., 2016). Maize has a much-reduced irrigation demand (500–700 mm) in comparison to direct-seeded (1300–1400 mm) and transplanted (2000–2100 mm) rice. This can increase system efficiency and maintain soil and environmental quality. In the NWIGP, maize and wheat are conventionally sown via broadcasting after intensive dry tillage (3–4 harrow passes, 1–2 cultivators, and 1 planking) operations and with flood irrigation (Das et al., 2018). Traditional production methods are not only inefficient with regard to inputs, but they also raise input costs. Around 25% of the overall production cost is attributed to heavy tillage and crop establishment, which lowers net revenue (Hobbs et al., 2007). Therefore, the primary research issue is to create an alternative production method that is input effective, can support agricultural output, and can produce more for less money (Gathala et al., 2011).
Conservation agriculture (CA) have emerged as an exemplar shift in agricultural practices having significant positive impact on soil quality, carbon sequestration and sustainable agricultural production as well as mitigation of climate change (Naresh, 2016). CA is currently being pushed and implemented for sustainable agricultural strengthening (FAO, 2011; Saad et al., 2016). It provides chances for maize and wheat in NWIGP region for water conservation and better crop establishment (Das et al., 2018). Additionally, CA-based methods like zero tillage (ZT) or no tillage (NT) indicate minimal soil disturbances, residue retention, and crop biodiversity through the balanced application of organic and inorganic fertilizers which improves soil health. Moreover, the CA practices lead to enhancement in soil carbon sequestration (Bhattacharyya et al., 2015). CA techniques also avoid soil erosion due to better soil aggregation and the shielding effect of crop mulches (Vanlauwe et al., 2014). CA increases soil quality and plant water use efficiency, making it more productive than CT (Brunel et al., 2013; Muchabi et al., 2014). In comparison to a conventional tilled (CT) system, a 10-year-old CA in the eastern IGP under ZT or permanent beds (PB) indicated significantly greater system productivity (Jat et al., 2019). By increasing the continuous pore system, which in turn leads to a rise in capillary porosity, conservation tillage improves the water-holding capacity (WHC) (Bhattacharyya et al., 2006). The profile distribution and concentration of soil organic carbon (SOC) are improved by long-term ZT in conjunction with optimal fertilizer and residue management, which also leads to positive changes in hydraulic conductivity (HC), porosity, soil aggregation, WHC (Dey et al., 2016; Bhattacharyya et al., 2019). SOC along with the availability of all the nutrients was enhanced by combining CA with correctly balanced nutrition management (Dey et al., 2016; Jat et al., 2019). Additionally, the use of these alternate tillage and crop establishment techniques promotes timely sowing of both crops, which raises yield. Hassan et al. (2005) found a 30 and 65% improvement in yield and water productivity (WP) of maize under NT raised bed than that of CT from a 4–year trial on sandy clay loam soil. Ram et al. (2011) stated a comparable yield but greater economic revenues from a maize-wheat rotation grown on NT raised beds than that of conventional tillage system on a loamy sand.
Despite the fact that CA is becoming more significant in tropical regions, there is still ongoing discussion on the profitability, yield, and advantages of CA over traditional practices. Therefore, establishing soil quality index (SQI) as a management goal in relation to crop system productivity and tracking changes in SQI as a result of various tillage, residue, and nitrogen management practices would undoubtedly provide a convincing declaration about the viability and sustainability of CA under maize-wheat rotation in NWIGP. According to Doran and Parkin (1994), “Soil quality is the capacity of a soil to function within ecosystem and land-use boundaries to sustain biological productivity, maintain environmental quality, and promote plant and animal health.” It is a common practice to quantify the impact of every alteration in management strategy on overall soil quality employing the SQI (Andrews et al., 2002). Improvements in soil microbial diversity, enzymatic activity, and soil microbial biomass carbon (SMBC) are some of the immediate effects of balanced nutrition management in CA (Ghosh et al., 2019). In the upcoming years, variations in those responsive characteristics, which aid as an early cautionary sign of variations in further soil quality factors like nutrient availability, will become apparent (Jia et al., 2014). Additionally, CA has certain negative effects on soil physical qualities during the early years of adoption, such as higher BD, lower oxygen diffusion rates, and decreased soil temperatures (Lampurlanés and Cantero-Martínez, 2003) but in the long run, when the system gets stabilized there is improvement in soil physical health under CA. The soil’s chemical, physical, and biological indicators and their interactions are exceedingly complicated (Karlen et al., 2003). Thus, the most vital phase in the computation of SQI is choosing an adequate minimum data set (MDS) relating to soil functions sensitive to management approaches. Principal component analysis (PCA) is a helpful statistical tool in the selection of MDS for SQI computation (Andrews et al., 2002). The Soil Management Assessment Framework (SMAF) was created by Andrews et al. (2004) to improve the methodology for assessing SQI, and it allows for the selection of indicators dependent on management objectives, linked soil functions, and additional site-specific considerations. In order to determine the sustainability and viability of CA under different cropping system (tropical or subtropical) in India, it is also necessary to measure variations in SQI that result from various management approaches.
In this context, we examined the impact of CA after 4 years on soil quality under a maize-wheat rotation in the hot, subtropical NWIGP agro-ecological region in India. We hypothesized that CA based systems have higher SQI than conventional tillage systems. To test this hypothesis, the specific objective of the current study was (a) to monitor the changes in physical, chemical, and biological soil quality indicators, (b) to assess soil quality index (SQI) and (c) to develop relationship between SQI and system productivity of maize-wheat system. In a novel method, this study relates to the assessment of SQI under the maize-wheat rotation in Inceptisol of NWIGP of India using two approaches, i.e., expert opinion and PCA. The derived methodology might be used to measure soil quality for comparable soils under similar agro-climatic environments worldwide.
2. Materials and methods
2.1. The study site description and climate
A field study was undertaken in a four-year-old continuing CA experiment with a set of management methods under the maize-wheat rotation. The experiment was set up in the ICAR-IARI research farm in New Delhi, India (28°35′ N latitude, 77°12′ E longitude, and at an altitude of 228 m AMSL). The soil is having sandy loam texture, well-drained, and slightly alkaline. The initial representative 0–15 cm soil sample had medium levels of Av-P (7.3 kg ha−1) and K (275.0 kg ha−1) but low levels of organic C (Walkley and Black C) (4.2 g kg−1) and Av-N (290 kg ha−1).
The trial site is mainly dominated by a semi-arid climate with dry, scorching summers and a short, harsh winter. The coldest month (January) prevails a mean monthly minimum and maximum temperature range of 5.9°C and 19.9°C, respectively. The equivalent temperature ranged between 24.4 and 38.6°C in May, which was the hottest month. The average total of precipitation each year is 651 mm. The S-W monsoon (July to September) accounts for 75% of total rainfall.
2.2. Experimental details
The experiment was set up using a split-split plot design, with two main plot tillage combinations (CT and NT), two levels of residue mulch [residue @ 5 Mg ha−1 (M+) and no residue removal (M0)] as the subplot, and three levels of nitrogen [50 (N1), 100 (N2), and 150% (N3) of the recommended nitrogen dose] as the sub-sub plot. Three replications of the treatments were done. Single super phosphate (SSP) and muriate of potash (MOP) fertilizers were administered uniformly to all treatments at sowing time for both the crops. The sub-sub plot measured 4.5 × 5 meters square.
2.3. Crop establishment
The maize crop (cv. PMH1) was sown in the 1st July of 2017 at a seed rate of 20 kg ha−1 with 60 cm row spacing and 20 cm plant spacing. The third week of November 2017 was selected for the wheat (cv. HD2967) sowing with a 22.5 cm row spacing and a seed rate of 100 kg ha−1. Maize and wheat were harvested in October (2nd week) 2017 and April (2nd week) 2018, respectively. In CT, tillage was done twice: once with a disc plough and again with a cultivator with duck-foot tines. After that, the ground was leveled and seed drills were used to plant the seeds. In the case of NT, the seed was sown employing an inverted T-style no-till seed drill. Crop residue from the previous season was used as mulch and spread @ 5 Mg ha−1 in the residue mulch treatments. Fertilizers were administered at rates of 150:75:75 and 120:60:60 kg ha−1 of N: P2O5:K2O for maize and wheat respectively, in accordance with the recommended doses. Urea was applied as a source of N in four splits to maize (20% at sowing, 20% at 4-leaf, 30% at knee-high, and 30% at tasseling) and three splits to wheat [at sowing (50%), CRI stage (25%), and flowering (25%)]. Using the USDA SCS approach, the effective rainfall was subtracted from the precipitation data (Cropwat 8.0). As per the crop water requisite for individual treatment, crops were irrigated at critical stages and in the dry periods. Weeds were managed in the NT plots by spraying glyphosate @ 1.0 kg active ingredient (a.i.) ha−1 2 days before the sowing. Herbicides were used in accordance with best practices to manage weeds in standing crop (Nath et al., 2017).
2.4. Soil sampling and methods of analysis
Subsequently, after the maize harvest in 2017, soil samples were collected and subjected to various analysis. Soil was sampled from each plot of 12 treatments that were replicated three times at three depths (0–0.05, 0.05–0.15, and 0.15–0.30 m) (thus total number of samples was 108). For the examination of soil biological, physical, and chemical properties, each composite sample was split into three groups. Examination of biological parameters, for the first batch of the composite soil samples, was done by maintaining it at 4°C in a refrigerator. The other two sets were air-dried, processed, ground, and passed through a sieve (2 mm) and were used to analyze soil physical and chemical properties following standard procedures. For assessing soil bulk density, 108 single intact soil cores (diameter = 5 cm and length = 5 cm) were collected (Black and Hartge, 1971). In the realm of physical attributes to evaluate the soil physical health, MWD, porosity, and BD of the soil were examined. Following laboratory measurements of aggregate stability employing the wet sieving method, the MWD was estimated (Kemper and Rosenau, 1986), and porosity was calculated using BD and particle density (Hati et al., 2007). To determine the chemical composition of the soil, we assessed TOC using an automatic analyzer (Vario EL, Elementar Analysensysteme GmbH, Hanau, Germany), available-P (Av-P) (Olsen, 1954), available–N (Av–N) (Subbiah and Asija, 1956), available-K (Av-K) (Hanway and Heidel, 1952), electrical conductivity (EC), and pH (Jackson, 1973). Under the biological parameters we analyzed SMBC and DHA using the chloroform fumigation-extraction method (Vance et al., 1987), and the release of triphenyl formazan from the reduction of 2,3, 5-triphenyl tetrazolium chloride (Dick et al., 1996), respectively.
2.5. Maize–wheat system productivity
Maize crop was harvested at the physiological maturity in October (2nd week) 2017 while wheat was in April (2nd week) 2018. From net plots that were harvested, the grain yields were estimated (2-border rows and 0.50 m in the perpendicular direction were excluded). The grain yield was calculated and expressed as Mg ha−1 at 12% moisture content. Wheat yield was expressed in terms of maize equivalent yield (MEY). Using Eq. 1, the wheat yield was transformed into MEY to determine system productivity. The MEY for wheat was calculated using the minimum support price (MSP) for wheat announced by the Indian Government.
The cumulative of maize yield and MEY of wheat, a measure of system productivity, was employed in this study to evaluate soil quality in accordance with Lal et al. (2017).
2.6. Determination of soil quality index (SQI)
2.6.1. Identification of minimum dataset
The Andrews et al. (2004) “Soil Management Assessment Framework (SMAF)” was used to choose, analyze, and combine indicator measures into a unified SQI. In order to create a minimum data set (MDS), the first phase of the SMAF is to identify essential soil properties. Principal component analysis (PCA) and the conceptual framework (CF), or expert opinion (EO), were the two methods employed in the current study to create an MDS. “Productivity” was selected as the primary management goal for the expert opinion-based SQI (EO-SQI) in this study (Andrews et al., 2004). The soil functions selected under the “productivity” objective included “nutrient cycling,” “physical stability and support,” “water relations,” “resistance, and resilience” (Table 1). On the other hand, to determine the most sensitive parameter, PCA of all the soil parameters was also performed. High eigenvalue principal components (PCs) would effectively represent system variance (Brejda et al., 2000). Therefore, only PCs having eigenvalues greater than one were included in this study (Kaiser, 1960). Based on the highest factor loading, the most sensitive parameter in each PC was chosen, and parameters that varied by <5% were included. When multiple variables were present in a single PC, the correlation was utilized to fix whether redundant variables may be removed from the MDS for SQI assessment (Andrews et al., 2001).
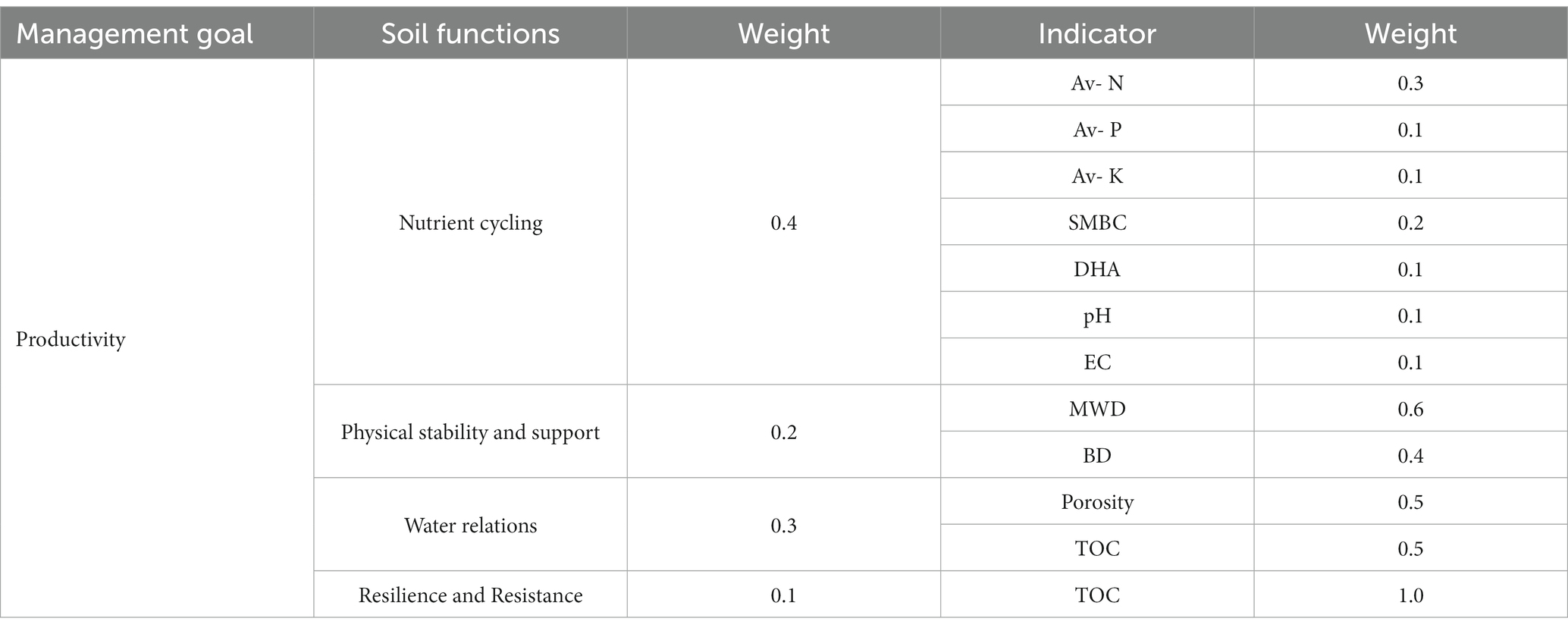
Table 1. Indicators and soil functions with assigned weights in conceptual framework or expert opinion.
2.6.2. Weight of the soil indicators
According to the EO-SQI assessment, each soil function received a distinct numerical weight based on its significance in achieving the overarching objective (productivity) of sustaining soil quality (Table 1). The weights for indicators indicating distinct soil functions were chosen based on the published literature and expert opinion (EO) (Andrews et al., 2004). All soil function weights added together equals to 1.00. On the other hand, the weightage of all the key components utilized for PCA-SQI, was determined by dividing the variation (%) of every principal component by the total variation (%) (Biswas et al., 2017).
2.6.3. Scoring of the soil indicators
Adopting the approach by Sharma et al. (2008), the MDS was modified using a linear scoring function, where the “more is better” philosophy was included for the majority of the MDS indicators. However, for the BD, pH (alkaline soil), and EC, “less is better” function was accepted. With the exemption of BD, pH, and EC, all other soil parameters were given scores of 1.0 for the maximum experimental value, meaning that they had the greatest values (more is better), while for the others the experimental value was divided by the maximum value for normalization. For BD, pH, and EC, the score 1.0 was assumed to be related to the least value and for others, the lowest value was divided by the other observed values for normalization.
2.6.4. Computation of SQI
After the normalization, the SQI value (Eq. 2) was computed by summing the multiplication of the weightage of the MDS variables (Wi) with the score (Si) of those variables.
Here, it was assumed that a higher SQI value would indicate higher soil quality and improved performance of soil functions.
2.7. Statistical analysis
Using SAS (Statistical Analysis System) software, the analysis of variance (ANOVA) approach was used to statistically examine all of the data using the split-split plot design (Gomez and Gomez, 1984). F-test was employed to determine the significance of the treatment effects and the significant difference between the means was computed employing Duncan’s Multiple Range Test (DMRT) at p-value<0.05. Correlation analysis amongst the soil parameters was exercised using the “ggplot2” package (Wickham and Chang, 2015) in R version 4.2.1. The PCA analysis was performed employing “factoextra” (Kassambara and Mundt, 2017) and “FactoMineR” (Lê et al., 2008) packages in R studio (Version 4.2.1).
3. Results and discussion
3.1. Soil physical quality parameters
It was observed that BD (Mg m−3) under NT (1.56) at 0–0.05 m was 4.0% higher than CT (1.50) whereas, at 0.05–0.15 and 0.15–0.30 m, BD (1.64, 1.72) under NT were higher than that of CT (1.58, 1.67) by 3.8 and 3%, respectively (Table 2); although the variations in BD between NT and CT were not statistically significant. Hati et al. (2015) and Martín-Lammerding et al. (2013) have also reported similar findings. At 0–0.05 m soil layer, the BD with residue mulch plots was 4.0% lower (p ≤ 0.05) than that with no mulch treatment. This may be because mulched plots have significantly more organic matter and increased earthworm activity, which enriched soil aggregation and porosity (Acharya et al., 2005). The MWD was between 0.73 and 1.17 mm, 0.74 and 1.07 mm, and 0.61 and 0.96 mm for soil depths of 0–0.05, 0.05–0.15, and 0.15–0.30 m, respectively (data not shown). At top soil and 0.05–0.15 m, plots under NT had MWD improvements of 10.52 and 8.5% compared to CT plots. Additionally, MWD in mulched plots (1.08 mm) increased significantly over no mulch treatment (0.92 mm) by 17.39% in the topsoil (Table 2). However, at lower depths residue retention had no substantial effect on the MWD. The use of organic residues contributed to the increased aggregate stability, as shown by the higher MWD values. Additionally, delaying the macroaggregate turnover rate due to reduced tillage in NT encouraged the production of stable soil aggregates (Six et al., 2000; Hati et al., 2015; Acar et al., 2018; Adak et al., 2019) leading to improved MWD at 0–0.05 m soil layer (Parihar et al., 2020). However, varied management practices had no substantial effect on soil porosity (Table 2).
3.2. Soil chemical quality parameters
Different tillage, residue, and nitrogen management practices had a significant impact on soil chemical parameters in various soil layers (Tables 3, 4). The TOC, a soil quality measure, was positively and significantly affected by the tillage and residue mulching (Table 3). Plots under NT demonstrated a higher TOC than that of CT by 5.26 and 5.48% at 0–0.05 and 0.05–0.15 m soil depths, respectively. In comparison to no mulch treatment, the residue retention treatment considerably increased TOC by 1.08–fold in the topsoil and 1.07–fold at 0.05–0.15 m soil layer. Under an NT regime, higher SOC may be caused by better soil aggregation in top soil layer (Mohammad et al., 2012; Hati et al., 2015; Fernández et al., 2017; Naab et al., 2017). The accumulation of organic matter in the soil through crop residues was also responsible for a higher TOC content in the surface layer. Applying crop residue mulch shields the soil from the effect of raindrops, which enhances soil aggregation (Iqbal et al., 2011; Das et al., 2021). Nevertheless, the impact of tillage and residue mulching was not substantial for lower soil depth while the effect of nitrogen management was also non-significant.
The experimental field’s soil pH ranged from 7.38 to 7.89, 7.55 to 7.9, and 7.81 to 8.26 at soil depths of 0–0.05, 0.05–0.15, and 0.15–0.30 m, respectively (data not shown). Tillage had no discernible impact on soil pH, whereas treatments with residue mulching had significantly decreased soil pH than that of no residue treatments at all soil depths (Table 3). This might be caused by the release of organic acid throughout the breakdown of crop residue (Mrabet et al., 2001). At all soil depths, the higher nitrogen dose caused a significant drop in soil pH. Lower soil pH may be caused by localized nitrogen mineralization with greater nitrogen doses (Ghimire et al., 2017). EC varied from 0.31 to 0.57 dS m−1 (0–0.05 m), 0.32 to 0.53 dS m−1 (0.05–0.15 m), and 0.27 to 0.40 dS m−1 (0.15–0.30 m) (data not shown). The impact of various management practices on EC, however, was not statistically significant (Table 3).
The effect of tillage, residue mulch, and N management on the availability of nutrients are presented in Table 4. However, the tillage and mulching effect on Av-N was not significant. This outcome is consistent with that of Monsefi et al. (2014), who reported that tillage treatments had no discernible effect on plant Av-N. However, the mulching treatments increased available-N more in the 0–0.15 m soil layer than in the 0.15–0.30 m soil layer (Das et al., 2014). As anticipated, the increase in nitrogen doses led to a considerable increase in the Av-N content in all layers. Tillage had no discernible effect on Av-P in any of the soil layers. At 0–0.05 and 0.05–0.15 m soil layers, plots with residue mulch treatment exhibited considerably higher Av-P compared to those without mulch treatment by 13.56 and 30.7%, respectively (Table 4). Higher Av-P could result from organic acid release during the decomposition of residues and native P solubilization in residue-mulched plots (Piegholdt et al., 2013; Dorneles et al., 2015). Similarly, results showed no significant effect of tillage on Av-K (Table 4). Plots under residue retention had 16.32, 16.0, and 24.1% more Av-K than residue removal plots at topsoil, 0.05–0.15, and 0.15–0.30 m soil depths, respectively. Meena et al. (2018) investigated that under CA, cereal residues provide a better amount of K to the soil by means of decomposition since their biomass contains a higher concentration of K.
3.3. Soil biological quality parameters
Soil microbiological activities viz., SMBC and DHA were positively affected by different tillage, residue retention, and N management practices (Garcia et al., 1997; Roldan, 2003). Average over residue and nitrogen treatment, at 0–0.05, 0.05–0.15, and 0.15–0.30 m soil layers, it was found that SMBC under NT was significantly greater than CT by 22.54, 29.91, and 6.56%, respectively. Residue mulching also significantly increased SMBC by 5.03, 34.07, and 13.61% as compared to residue removal treatment for 0–0.05, 0.05–0.15, and 0.15–0.30 m, respectively (Table 5). Plots under N3 resulted in significantly higher SMBC by 26.3 and 34.1% as compared to N1 plots at 0–0.05 and 0.05–0.15 m soil depth, respectively. Higher SMBC with an increase in nitrogen doses may have been caused by microbial proliferation in the presence of better nitrogen availability. Similar findings were recorded about DHA. The experimental data of DHA was found to be higher in NT than that of CT at topsoil, 0.05–0.15, and 0.15–0.30 m soil layers by 13.7, 4.2, and 11.2%, respectively. Crop residue retention had a similar impact on DHA. It was 19.1 and 13.3% higher than residue removal plots at 0–0.05 and 0.15–0.30 m soil depths, respectively. Our observations are in agreement with Govaerts et al. (2007), who found that residue retention considerably enhanced surface soil MBC compared to the no residue treated plots during a long-term experiment on subtropical soil. The microorganisms may obtain their energy from the continuous influx of C provided by crop waste. This could explain why the residue-amended plots had higher enzyme activity than the residue-removal plots.
3.4. SQI based on expert opinion (EO-SQI)
The “conceptual framework (CF) or expert opinion (EO)” based SQI was calculated for all three depths (Figure 1). In the CF-SQI, all 11 soil indicators were taken into account and they have been weighted based on their contribution to crop productivity. Av-N was given the highest weight. Nitrogen was considered as one of the most important key indicators of soil quality due to its direct association with crop growth (Andrews et al., 2002). The weights assigned for soil functions, i.e., nutrient cycling, physical stability & support, water relations, and resistance & resilience were 0.4, 0.2, 0.3, and 0.1, respectively (Table 1). The EO-SQI was calculated using Eq. 3.
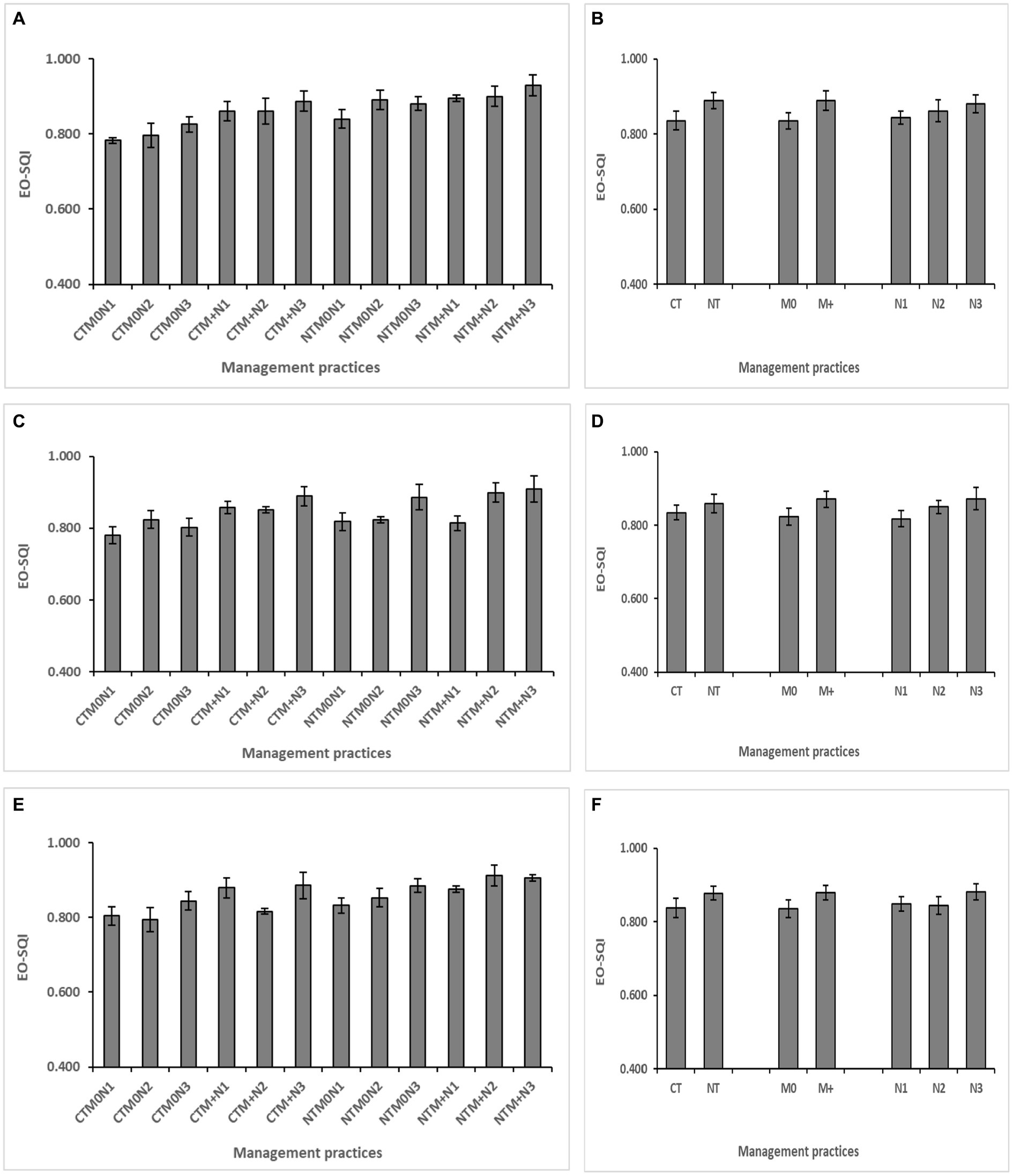
Figure 1. Effect of tillage, residue mulch, and N management on EO-SQI in 0–0.05 (A,B), 0.05–0.15 (C,D), and 0.15–0.30 (E,F) m soil layers. CTM0N1, conventional tillage (CT) + residue removal + 50% recommended nitrogen dose (RND); CTM0N2, CT + residue removal + 100% RND; CTM0N3, CT + residue removal + 150% RND; CTM+N1, CT + residue mulching + 50% RND; CTM+N2, CT + residue mulching + 100% RND; CTM+N3, CT + residue mulching + 150% RND; NTM0N1, no tillage (NT) + residue removal + 50% recommended nitrogen dose (RND); NTM0N2, NT + residue removal + 100% RND; NTM0N3, NT + residue removal + 150% RND; NTM+N1, NT + residue mulching + 50% RND; NTM+N2, NT + residue mulching + 100% RND; NTM+N3, NT + residue mulching + 150% RND.
The EO-SQI varied from 0.783 to 0.930, and 0.780 to 0.909 for the soil layers of 0–0.05, 0.05–0.15 m, respectively while it ranged from 0.794 to 0.913 at 0.15–0.30 m soil layer. The highest EO-SQI was recorded in NTM + N3, NTM + N3, and NTM + N2 for topsoil, 0.05–0.15 and 0.15–0.30 m soil layers, respectively (Figures 1A,C,E). The effect of tillage and residue mulching was found to be significant (p < 0.05) on EO-SQI. For soil depths 0–0.05 m, NT has enhanced the EO-SQI by 6.5% compared to CT, while it was determined to be 3.0 and 4.8% for other soil depths (0.05–0.15 and 0.15–0.30 m, respectively) (Figures 1D−F). EO-SQI under residue mulch treatments was improved over no mulch plots by 6.3 and 5.8% for topsoil and 0.05–0.15 m soil layer, respectively. Das et al. (2016) reported a similar result, where the treatment with crop residue and NPK displayed the highest SQI value because of significant improvements in the soil’s physical characteristics. Mohanty et al. (2007) also observed the most beneficial impact of ZT on soil quality in wheat.
3.5. SQI based on principal component analysis (PCA-SQI)
3.5.1. Key soil indicators and PCA-SQI for 0–0.05 m soil layer
In order to compare the soil quality indices between the treatments, PCA was also used to analyze 11 soil quality indicators of 0–0.05 m soil layer (Figure 2). Only three principal components (PCs) with eigenvalues >1 were identified by PCA as main components, accounting for 79% of the variation in the MDS (Table 6). Based on these, the Av-K was found to be the variable with the highest weight in PC1 and was followed by SMBC, while in PC2 and PC3, TOC and EC were qualified as the highest-weighted variables, respectively (Table 6). Since Av-K and SMBC had a significant correlation (r = 0.72**) (Figure 3A), Av-K was kept in MDS since it had the highest factor loading and SMBC was not considered. After that, linear scoring functions were employed to normalize and translate the sensitive key indicators, i.e., Av-K, TOC, and EC (Sharma et al., 2008). For PC1, PC2, and PC3, the weighted factors were 0.559, 0.251, and 0.190, respectively. The PCA-SQI for 0–0.05 m soil layer was calculated by the summation of the product of weightage and observed scores of each weighted indicator in MDS using Eq. 4.
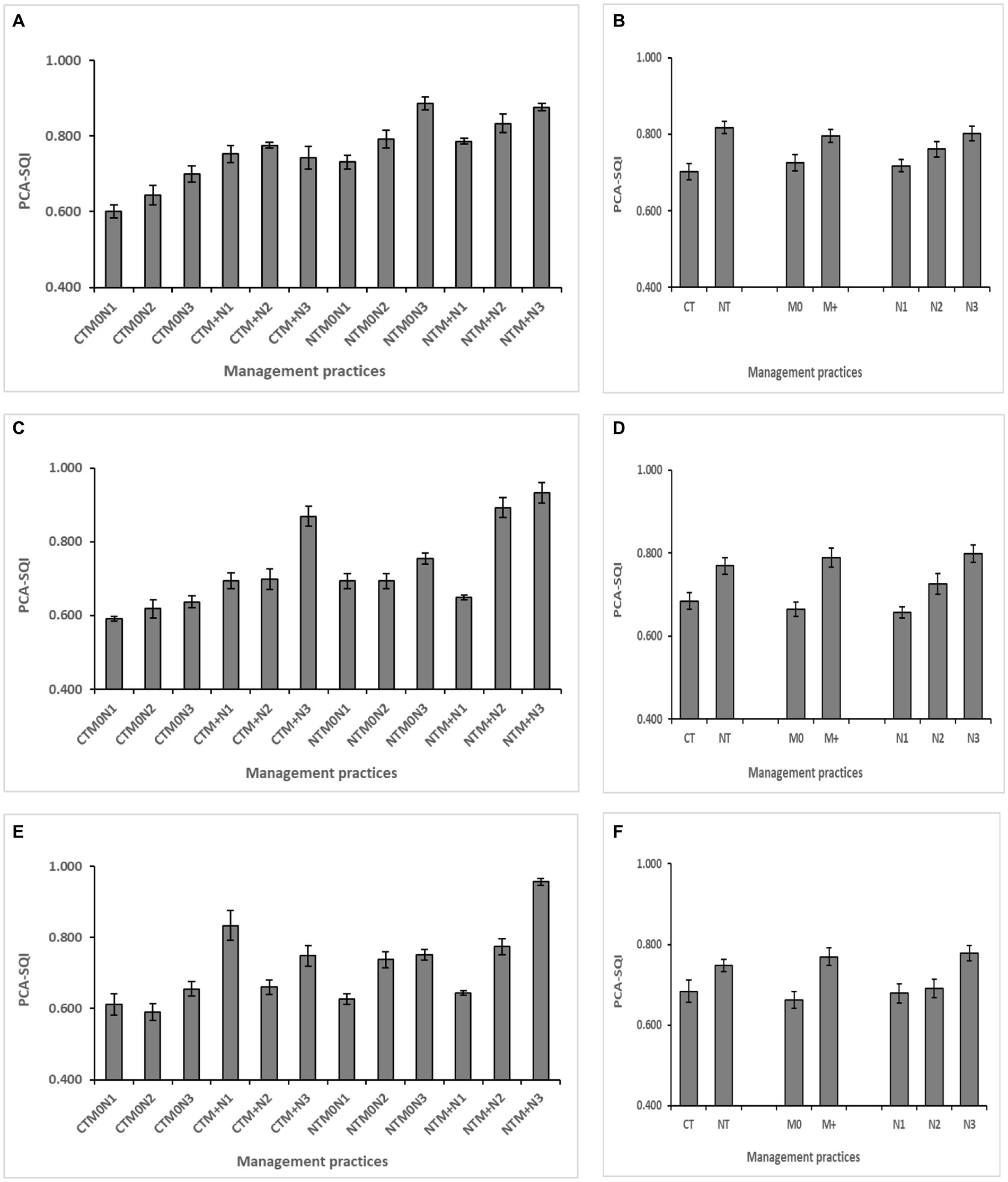
Figure 2. Effect of tillage, residue mulch, and N management on PCA-SQI in 0–0.05 (A,B), 0.05–0.15 (C,D), and 0.15–0.30 (E,F) m soil layers. CTM0N1, conventional tillage (CT) + residue removal + 50% recommended nitrogen dose (RND); CTM0N2, CT + residue removal + 100% RND; CTM0N3, CT + residue removal + 150% RND; CTM+N1, CT + residue mulching + 50% RND; CTM+N2, CT + residue mulching + 100% RND; CTM+N3, CT + residue mulching + 150% RND; NTM0N1, no tillage (NT) + residue removal + 50% recommended nitrogen dose (RND); NTM0N2, NT + residue removal + 100% RND; NTM0N3, NT + residue removal + 150% RND; NTM+N1, NT + residue mulching + 50% RND; NTM+N2, NT + residue mulching + 100% RND; NTM+N3, NT + residue mulching + 150% RND.
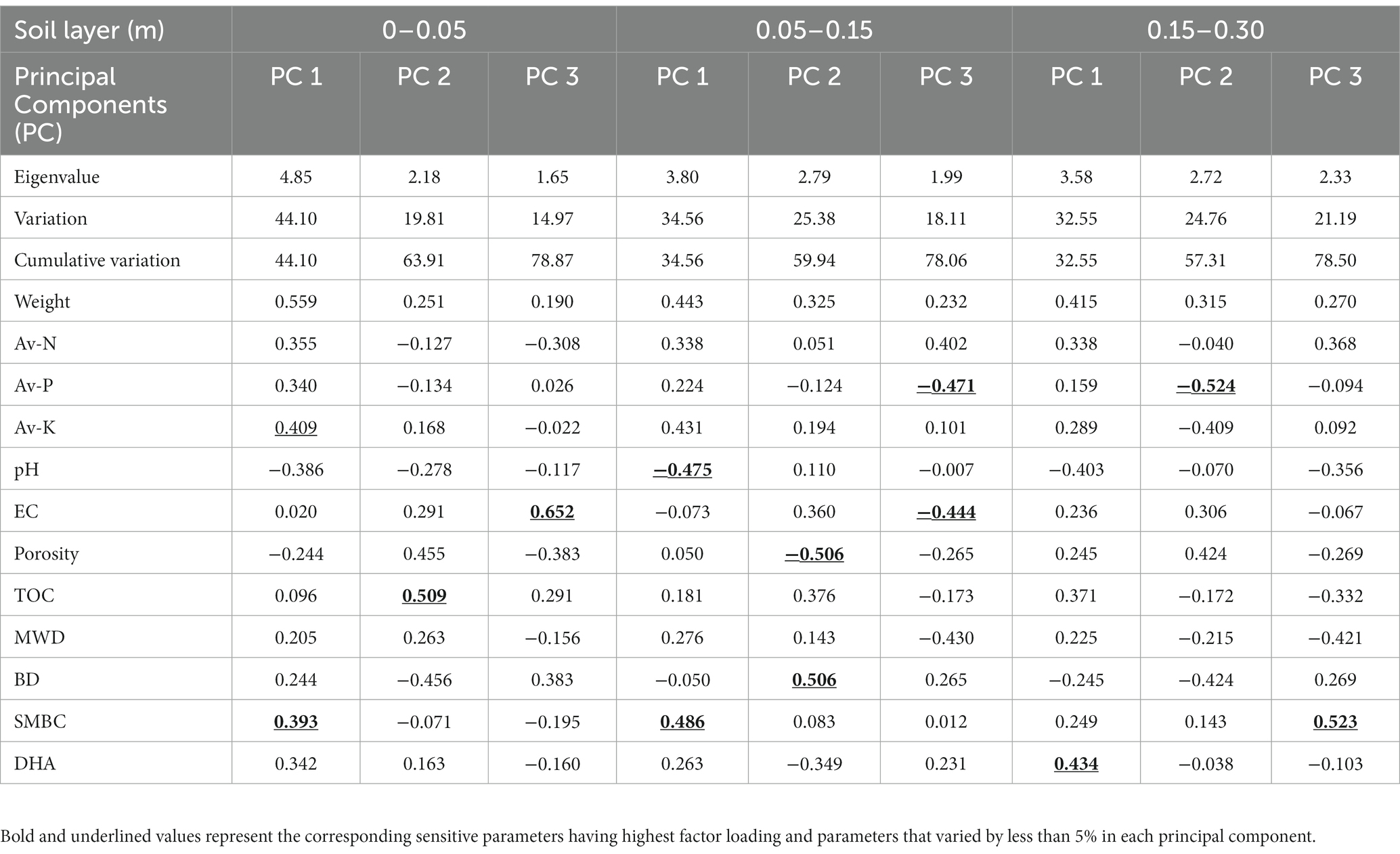
Table 6. PCA of significant soil attributes of all three soil layers during the fourth year of maize-wheat system.
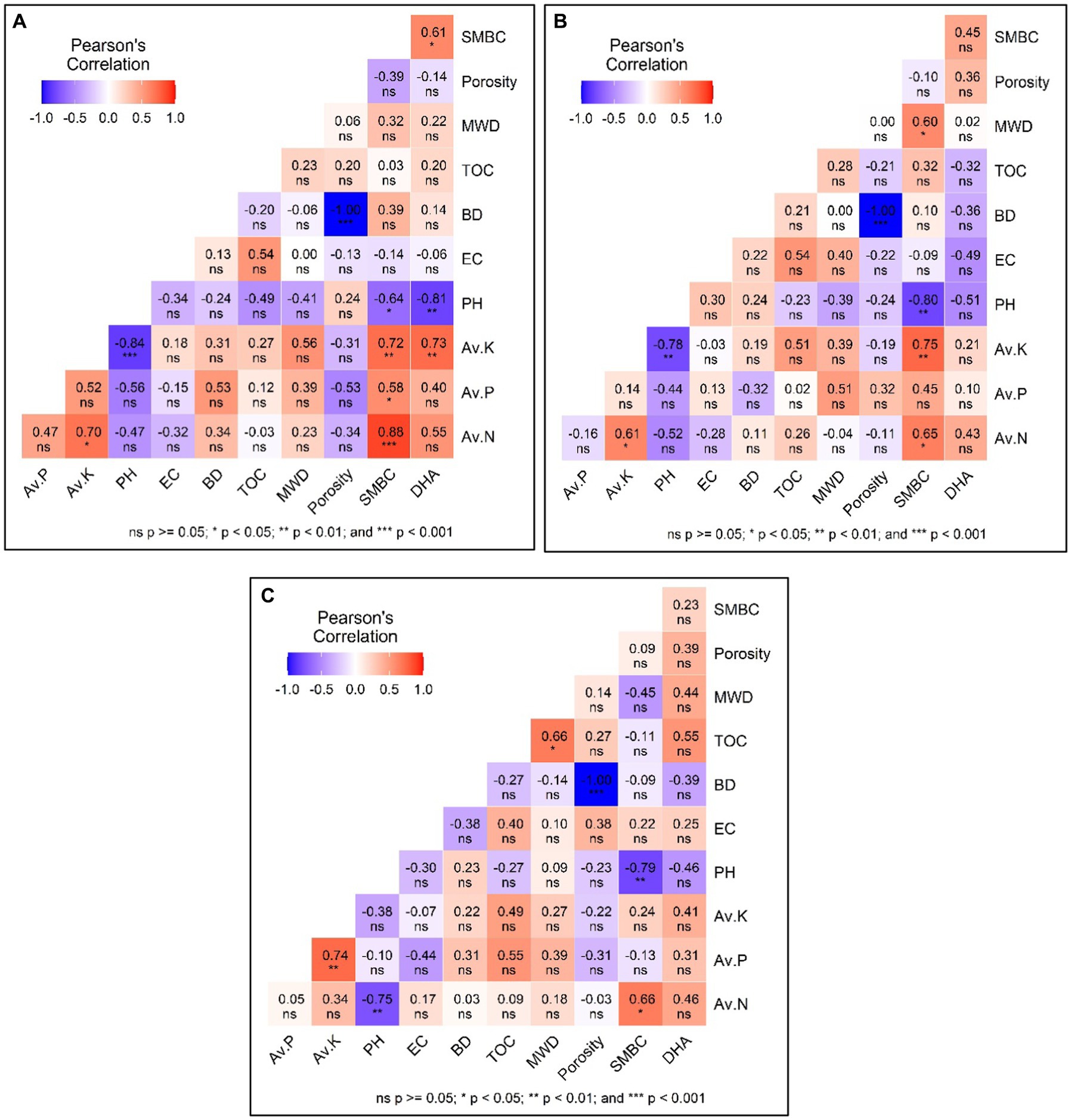
Figure 3. Values of correlation coefficient (r) between various soil quality parameters at 0–0.05 (A), 0.05–0.15 (B), and 0.15–0.30 (C) m soil layers.
The PCA-SQI varied throughout the management practices, ranging from 0.601 (CTM0N1) to 0.887 (NTM0N3) (Figure 2A) for 0–0.05 m soil layer. In comparison to CT, plots under NT increased the PCA-SQI by 16.38%, while residue retention led to a 9.51% improvement in PCA-SQI (Figure 2B). This finding clearly shows the advantage of following NT and residue retention for improving soil quality in the surface (0–0.05 m) layer.
3.5.2. Key soil indicators and PCA-SQI for 0.05–0.15 m soil layer
The 11 important soil indicators from the 0.05–0.15 m soil layer was also taken into account for PCA, however, only three PCs with eigenvalues >1, which account for 78.06% of the variation in the data set, were deemed as major components (Table 6). In PC1, SMBC was determined to be the variable with the highest weight, followed by pH. Similar to this, PC2 defined BD and porosity as highly weighted factors, while PC3 showed Av-P as the highest weighted factor. As there was a high correlation between SMBC and pH (r = −0.80**), BD and porosity (r = −1.00***) (Figure 3B), pH and porosity were not considered for MDS as they had relatively lower factor loading compared to SMBC and BD, respectively. The weighted components that emerged for PC1, PC2, and PC3 were 0.443, 0.325, and 0.232, respectively (Table 6), followed by transformation of data using a linear scoring method. The weighted MDS indicator scores for each observation were added up using Eq. 5 to derive the SQI for 0.05–0.15 m soil depth.
The highest and lowest PCA-SQI was observed under NTM + N3 (0.933) and CTM0N1 (0.592), respectively (Figure 2C). It was also evident that, compared to CT-based plots, the NT-based plots exhibited a 12.38% improvement in PCA-SQI. The SQI was also improved by 18.75% with the crop residue mulch plots compared to residue removal plots (Figure 2D). So, the effect of NT and crop residue mulch on improvement in SQI was experienced in 0.05–0.15 m soil depth also.
3.5.3. Key soil indicators and PCA-SQI for 0.15–0.30 m soil layer
In the 0.15–0.30 m soil layer, DHA, Av-P, and SMBC were found to be the highest weighted factors for PC1, PC2, and PC3, respectively. These three PCs with eigenvalues >1 explained a 78.5% variation in the data set (Table 6). There was no significant correlation between DHA, Av-P and SMBC (Figure 3C). Then these data were transformed by employing linear scoring functions. The weighted factors that emerged were 0.415, 0.315, and 0.270 for PC1, PC2, and PC3, respectively (Table 6). Using Eq. 6, the weighted MDS indicator scores for every observation were added up to produce PCA-SQI for 0.15–0.30 m soil depth.
The measured PCA-SQI ranged from 0.591 (CTM0N2) to 0.957 (NTM + N3) depending on the management practices at the sub-surface layer (0.15–0.30 m) in the maize-wheat rotation (Figure 2E). PCA-SQI improved by 9.53% in NT plots compared to CT, while increased by 16.15% in residue mulching areas compared to no mulch plots (Figure 2F). NT and crop residue mulch were also found to improve the PCA-SQI in the sub-surface soil layer.
Overall, it was found that Av-K, SMBC, TOC, and EC were the sensitive key indicators for PCA-SQI in the topsoil layer. In addition, PCA-SQI in the 0.05–0.15 m layer of soil revealed SMBC, pH, BD, Av-P, and porosity as the primary indicators. Whereas DHA, Av-P, and SMBC were the key indicators for the sub-surface layer (0.15–0.30 m). Analogous findings were reported by Das et al. (2021). According to Bünemann et al. (2018), the most often suggested soil quality indicators included TOC, Av-P, pH, soil water-related parameters, Av-K, and Av-N. In our study, BD and porosity were found to be the most significant indicators of soil physical quality. Due to its significant influence on soil water, porosity, and other factors, BD is a significant indicator that regulates the soil water-air connection, particularly the soil pore’s arrangement and the macropore’s endurance (Bhattacharya et al., 2020). Consequently, it needs to be checked on a regular basis (Dam et al., 2005; Paz-Kagan et al., 2014). Greater HC is caused by the increase and connectivity of soil pores, which facilitate water infiltration also (Mishra et al., 2015).
The most significant indicators among soil chemical characteristics were TOC, Av-K, Av-P, pH, and EC (Askari and Holden, 2015; Salomé et al., 2016). The subtropical region of India suffered from poor soil quality as a result of high temperatures and repeated cultivation (Mandal et al., 2008). It has been demonstrated that TOC is a very effective soil quality indicator (Biswas et al., 2017; Liu et al., 2017). Additionally, soil aggregates were greatly impacted by TOC (Bhattacharyya et al., 2004; Mandal et al., 2008). The increase in soil water holding capacity can be attributed to the higher TOC level in the soil (Das et al., 2016). In farming systems based on wheat, Av-K is a crucial soil quality indicator (Shahid et al., 2013; Basak et al., 2016). Av-K helps plants withstand drought, which is vital for the osmoregulation of plants. Plants may become vulnerable to drought because of a deficit of Av-K in soil. Cereal residues with greater K concentration improve soil K through the breakdown of plant residue in CA (Meena et al., 2018). Crop demand and soil K supply in the CA plots were coordinated. The significant effects of tillage, residue, and nutrient management on Av-K established it as an SQI indicator (Parihar et al., 2020). Av-P, which is crucial for crop growth due to its involvement in energy transfer reactions, was one of the significant chemical indicators that were carefully chosen. There is the direct involvement of phosphorus in processes such as respiration, photosynthesis, other metabolic pathways, root formation, grain quality, etc. (Tisdale et al., 1993). Soil pH is regarded as yet additional sensitive key indicator (Salomé et al., 2016; Biswas et al., 2017), possibly because of its effect on numerous soil properties and processes leading to nutrient availability. In addition to controlling nutrient availability (Schoenholtz et al., 2000), pH also controls microbial growth and the organic matter’s turnover (Arias et al., 2005). Another important indicator was EC, which quantifies the salt content of the soil. It influences crop yields, crop compatibility, plant nutrient availability, and soil microbial activity. EC has been linked to quantities of nitrates, potassium, ammonia, etc. even though it does not directly detect any particular ions or salt compounds.
Soil microbial biomass C and DHA were identified in the current investigation as important biological indicators. In agroecosystems, nutrient cycling depends heavily on microbial biomass (van der Heijden et al., 2008; Mbuthia et al., 2015). Microbial activity, one of the most sensitive indices of soil quality (Liu et al., 2017), is significantly affected by soil management methods (Masto et al., 2008). Plant nutrition is significantly influenced by the mineralization of organic materials in the soil. Additionally, mineralization aids in predicting system yield, nitrate extraction from soils, and risk assessment for environmental pollution (Hirzel et al., 2012). Overall, the SQI was much greater under NT compared to CT. On the other hand, compared to plots with residue removal, residue mulching considerably increased the SQI.
3.6. Maize-wheat system productivity
The experimental data revealed that maize grain yield was significantly affected by tillage, residue, N management and their interaction (Figures 4A,B). NT improved the maize yield by 21.41% than that of CT, while it was 37.66% higher under M+ plots as compared on residue plots. N3 and N2 treatment had 49.08 and 25.53% higher yield than that of N1 treatment. Though there was comparable wheat yield under different tillage and residue management, while it increased significantly with the increase in the doses of nitrogen (Figures 4C,D). Similar findings were also reported by Adak et al. (2021). The wheat yield was expressed in terms of maize equivalent yield (MEY) and was added to the yield of maize to find out system productivity. The effect of different management practices on system productivity was significant (Figures 4E,F). The highest (13.90 Mg ha−1) and lowest (6.94 Mg ha−1) system productivity were noted under NTM + N3 and CTM0N1 treatments, respectively. 15.17% improvement in the system productivity was observed under NT as compared to CT. Similarly, M+ also increased the system productivity by 24.15% than that of M0. The increased grain yield under the NTM+ might be attributed to favorable biological tillage and mulching effects. Under a no-tillage with residue retention condition, there was more infiltration, conservation of soil moisture, lower erosion and run-off, moderation of temperature, prevention of weed development, and higher microbial activity (Baghel et al., 2020; Ghosh et al., 2022).
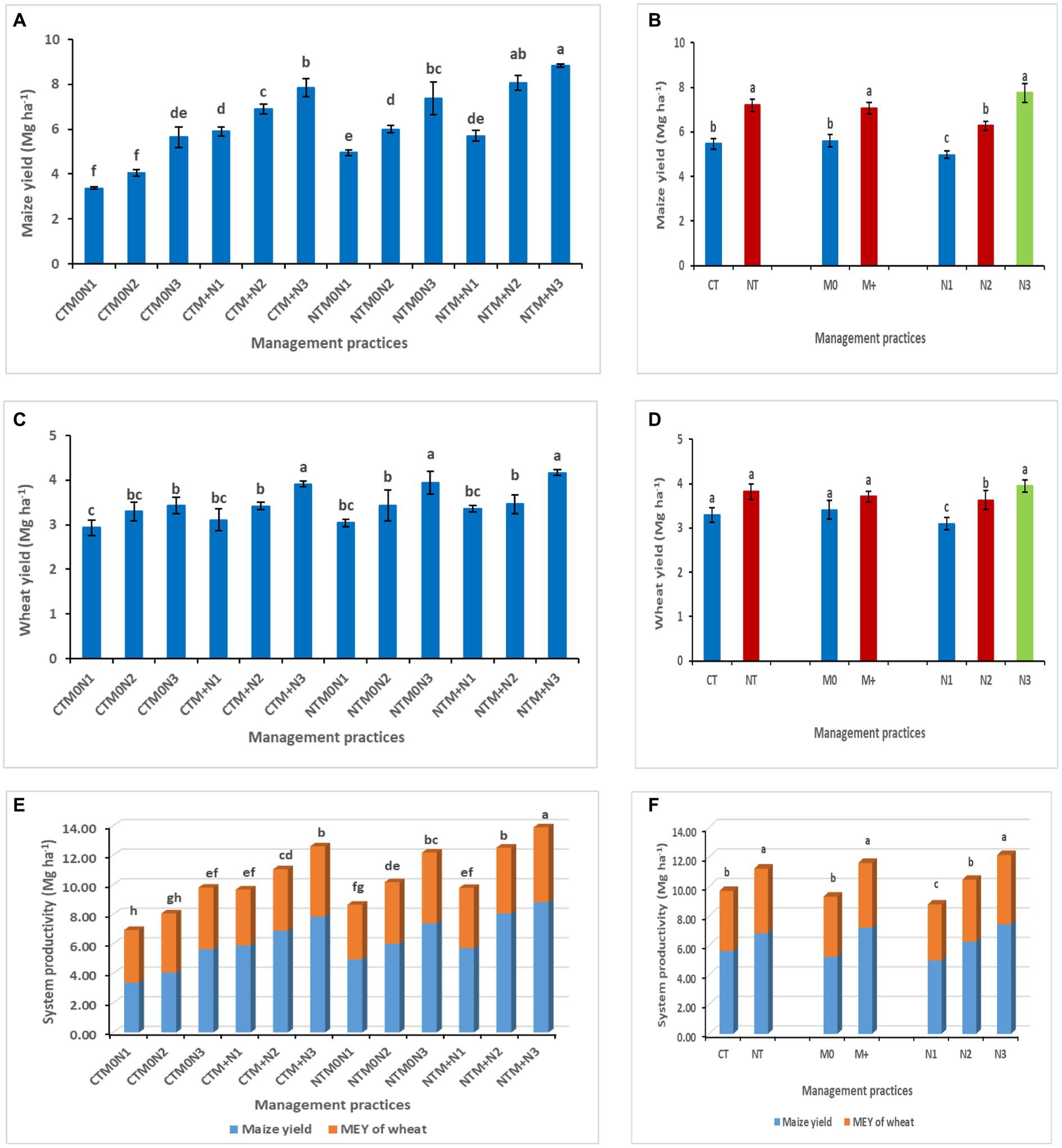
Figure 4. Effect of tillage, residue mulch, and N management on maize (A,B) and wheat (C,D) yield and system productivity (E,F). CTM0N1, conventional tillage (CT) + residue removal + 50% recommended nitrogen dose (RND); CTM0N2, CT + residue removal + 100% RND; CTM0N3, CT + residue removal + 150% RND; CTM+N1, CT + residue mulching + 50% RND; CTM+N2, CT + residue mulching + 100% RND; CTM+N3, CT + residue mulching + 150% RND; NTM0N1, no tillage (NT) + residue removal + 50% recommended nitrogen dose (RND); NTM0N2, NT + residue removal + 100% RND; NTM0N3, NT + residue removal + 150% RND; NTM+N1, NT + residue mulching + 50% RND; NTM+N2, NT + residue mulching + 100% RND; NTM+N3, NT + residue mulching + 150% RND.
3.7. Quantitative relationship between SQI and system productivity
A predictive and quantitative relationship was developed between system productivity in terms of maize equivalent yield (MEY) and EO-SQI (Figures 5A–C) and PCA-SQI (Figures 6A–C) of each soil layer after the fourth year of long-term CA practices. The system productivity in 2017–18 was considered for validation purposes of the impact of improved SQI on system productivity (Figures 4E,F). System productivity was considered as the dependent variable (y) and SQI as the independent variable (x) under various management techniques independently for each soil layer to fit the regression equations. It showed a significant and positive relationship between system productivity and SQI. The estimated regression coefficients between system productivity and EO-SQI were significant, with R2 = 0.75 for topsoil, and R2 = 0.83 for 0.05–0.15 m layer (Figures 5A,B) whereas R2 = 0.72, and 0.82 for 0–0.05 and 0.05–0.15 m soil layers, respectively between system productivity and PCA-SQI (Figures 6A,B). However, the regression coefficients for both EO-SQI and PCA-SQI were lower with R2 = 0.65 and 0.60, respectively for 0.15–0.30 m soil layer (Figures 5C, 6C). Thus EO-SQI and PC-SQI showed similar trends and a similar relationship with system productivity. Thus, soil quality indices at topsoil and 0.05–0.15 m soil layer could account for 72–83% variation in the system productivity. These findings demonstrated that the soil’s physical and biochemical indicators are influenced by tillage-based crop establishment practices with various residue management strategies. Overall, it was observed that no-tillage and crop residue mulching had a positive effect on the soil’s characteristics and system productivity (Saurabh et al., 2021). Use of crop residues as surface mulch assisted in improving soil biological activity, reducing weed growth, and moderating soil temperature. Higher crop productivity was the outcome of better soil health under these NT plots (Parihar et al., 2017a,b).
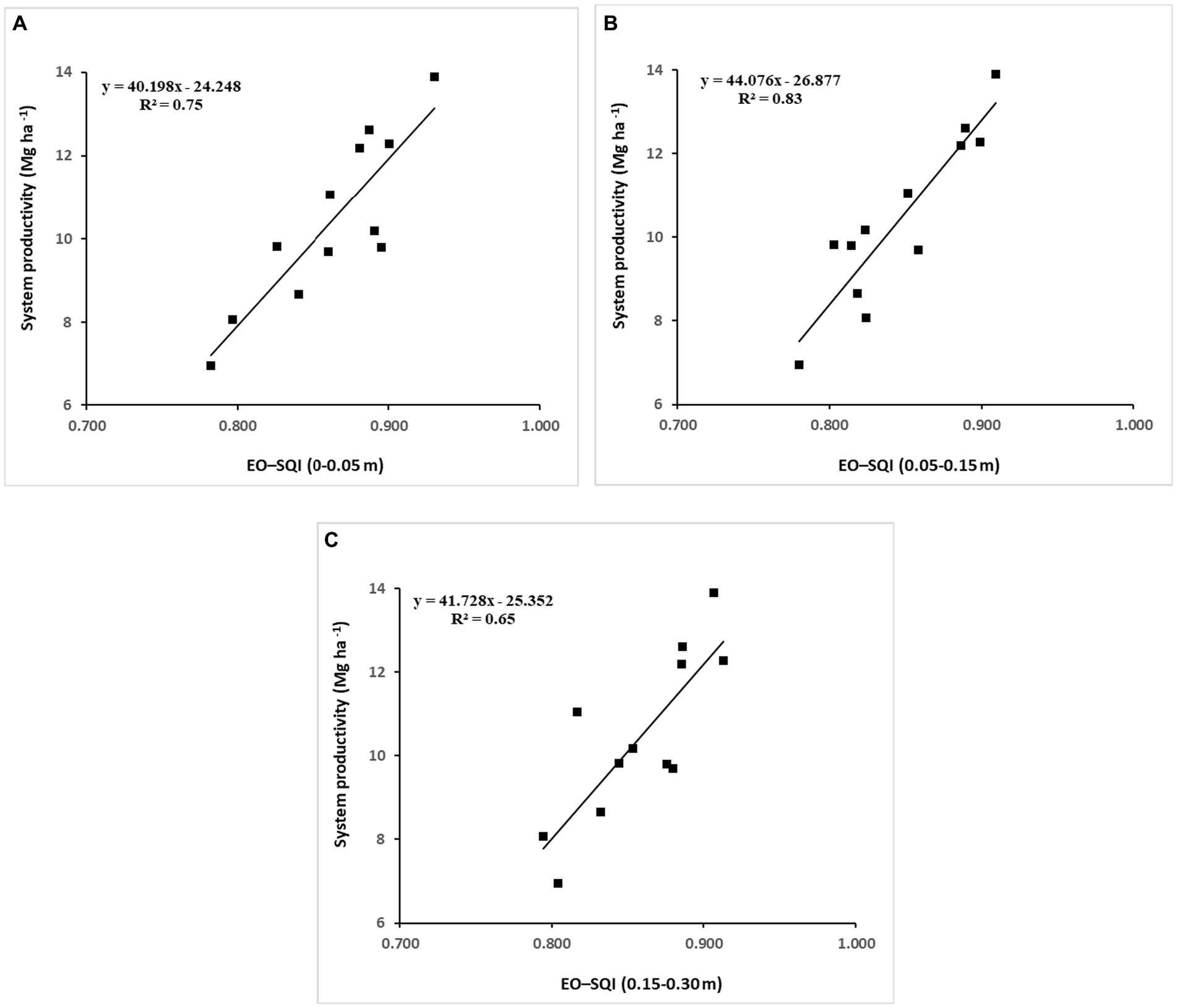
Figure 5. Relationship between EO-SQI of 0–0.05 (A), 0.05–0.15 (B), and 0.15–0.30 (C) m soil layers with system productivity.
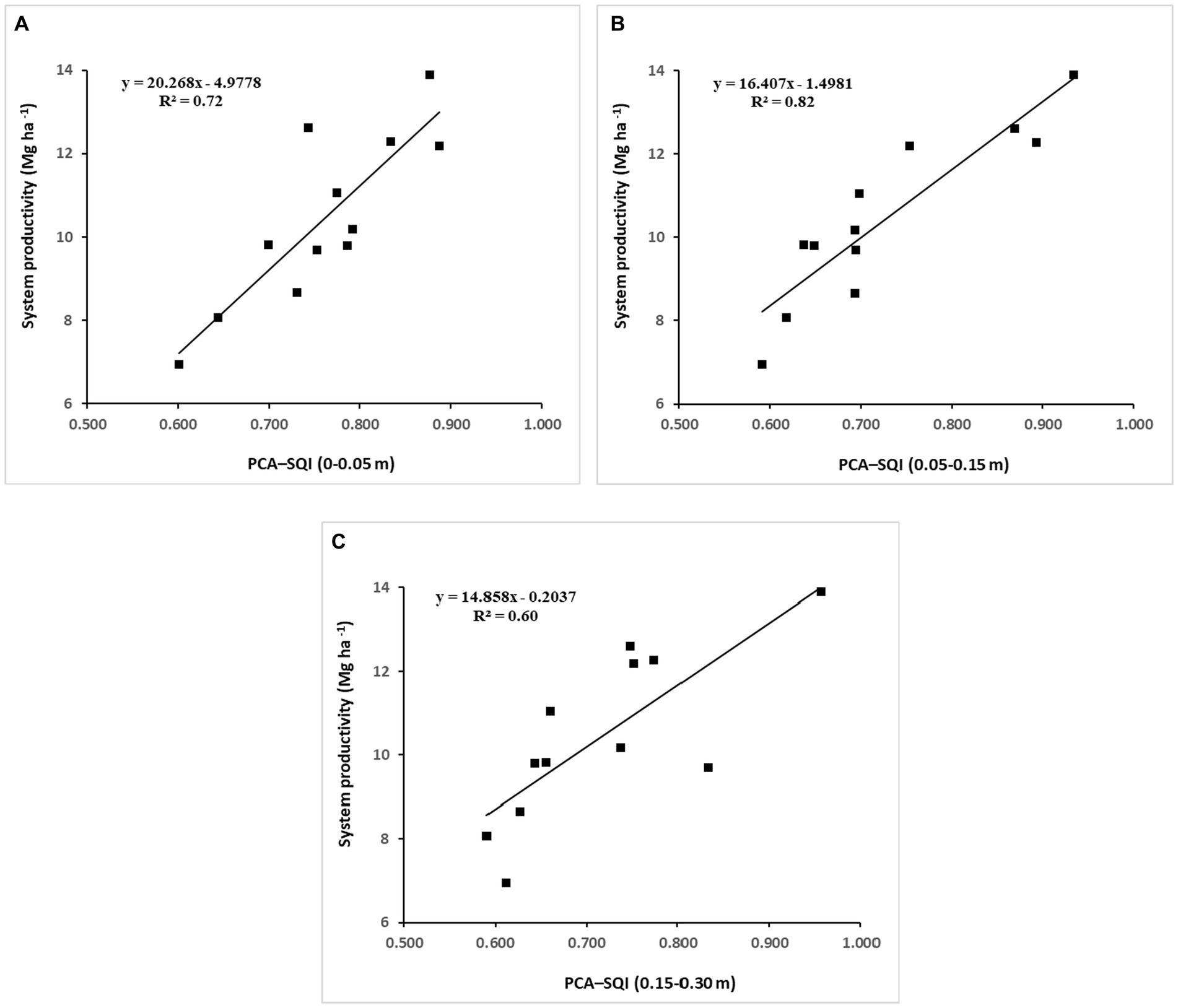
Figure 6. Relationship between PCA-SQI of 0–0.05 (A), 0.05–0.15 (B), and 0.15–0.30 (C) m soil layers with system productivity.
4. Conclusion
This study showed that conservation agriculture, even when it was only 4 years old, significantly improved soil quality, as measured by better soil biological, chemical, and physical health indices in the top soil and in soil depths of 0.05–0.15 m. Soil aggregation and organic carbon content were improved by agricultural residue retention and minimal soil disturbance. Additionally, residue retention increased the available P, K, and biological activity in the soil. The soil physical indicator viz., BD and porosity; the soil chemical indicators viz., TOC, Av-K, Av-P, pH, and EC and the biological indicators viz., SMBC and DHA were identified as key indicators of soil quality for the surface and sub-surface soil layers in Inceptisol. These indicators can be used to detect changes in soil quality; however, they are soil-specific, and hence they should be used with caution. The plots under No tillage, residue retention and 150% RDN treatment led to highest soil quality index. Soil quality indices at top 0–0.05 and 0.05–0.15 m soil layer could account for 74–80% variation in the system productivity. The significant and positive relationship between system productivity and SQI is also indicative for the sustainable crop production under conservation agriculture. The relationship between SQI and system productivity will also be helpful for the prediction of crop yield using SQI in maize-wheat rotation. Our research suggests that no tillage, and residue retention with balanced fertilizer doses can be a practical substitute for conventional tillage for sustainable production with concurrent improvement in soil quality under maize-wheat rotation in NWIGP region of India. The findings from this study also will be crucial for enhancing soil health and achieving the objectives of sustainable development goal.
Data availability statement
The raw data supporting the conclusions of this article will be made available by the authors, without undue reservation.
Author contributions
SA and KB conducted the investigation. SA, KB, TP, and SS implemented all the analysis. KB, PK, RS, and MS conceptualized the study. SA and KB prepared the original draft. KB supervised the experiment. All authors contributed to the article and approved the submitted version.
Acknowledgments
The authors thank the Division of Agricultural Physics, Soil Science and Agricultural Chemistry and Environmental Science, New Delhi, India. SA acknowledges the support received from Department of Science and Technology, GoI during the study period. It is happily acknowledged that during the study time, the Director, IARI, provided logistical help.
Conflict of interest
The authors declare that the research was conducted in the absence of any commercial or financial relationships that could be construed as a potential conflict of interest.
Publisher’s note
All claims expressed in this article are solely those of the authors and do not necessarily represent those of their affiliated organizations, or those of the publisher, the editors and the reviewers. Any product that may be evaluated in this article, or claim that may be made by its manufacturer, is not guaranteed or endorsed by the publisher.
References
Acar, M., Celik, I., and Günal, H. (2018). Effects of long-term tillage systems on aggregate-associated organic carbon in the eastern Mediterranean region of Turkey. Eurasian J. Soil Sci. 7, 51–58. doi: 10.18393/ejss.335329
Acharya, C. L., Hati, K. M., and Bandyopadhyay, K. K. (2005). “Mulches” in Encyclopedia of soils in the environment. eds. D. Hillel, C. Rosenzweig, D. S. Powlson, K. M. Scow, M. J. Singer, and D. L. Sparks, et al. (Amsterdam: Elsevier Publication), 521–532.
Adak, S., Bandyopadhyay, K. K., Sahoo, R. N., Mridha, N., Shrivastava, M., and Purakayastha, T. J. (2021). Prediction of wheat yield using spectral reflectance indices under different tillage, residue and nitrogen management practices. Curr. Sci. 121, 402–413. doi: 10.18520/cs/v121/i3/402-413
Adak, S., Bandyopadhyay, K. K., Sahoo, R., Purakayastha, T. J., Shrivastava, M., and Mridha, N. (2019). Soil physical characteristics, productivity and input use efficiency of wheat (Triticum aestivum) as affected by different tillage, residue mulch and nitrogen management in maize-wheat cropping system. J. Agric. Phys. 19, 239–250.
Andrews, S. S., Karlen, D. L., and Cambardella, C. A. (2004). The soil management assessment framework. Soil Sci. Soc. Am. J. 68, 1945–1962. doi: 10.2136/sssaj2004.1945
Andrews, S. S., Karlen, D. L., and Mitchell, J. P. (2002). A comparison of soil quality indexing methods for vegetable production systems in northern California. Agric. Ecosyst. Environ. 90, 25–45. doi: 10.1016/s0167-8809(01)00174-8
Andrews, S. S., Mitchell, J. P., Mancinelli, R., Karlen, D. L., Hartz, T. K., Horwath, W. R., et al. (2001). On-farm assessment of soil quality in California’s central valley. Agron. J. 94:12. doi: 10.2134/agronj2002.0012
Arias, M. E., González-Pérez, J. A., González-Vila, F. J., and Ball, A. S. (2005). Soil health: a new challenge for microbiologists and chemists. Int. Microbiol. 8, 13–21.
Askari, M. S., and Holden, N. M. (2015). Quantitative soil quality indexing of temperate arable management systems. Soil Tillage Res. 150, 57–67. doi: 10.1016/j.still.2015.01.010
Aulakh, M. S., Manchanda, J. S., Garg, A. K., Kumar, S., Dercon, G., and Nguyen, M. L. (2012). Crop production and nutrient use efficiency of conservation agriculture for soybean-wheat rotation in the indo-gangetic plains of north-western India. Soil Tillage Res. 120, 50–60. doi: 10.1016/j.still.2011.11.001
Baghel, J. K., Das, T. K., Pankaj,, Mukherjee, I., Nath, C. P., Bhattacharyya, R., et al. (2020). Impacts of conservation agriculture and herbicides on weeds, nematodes, herbicide residue and productivity in direct-seeded rice. Soil Tillage Res. 201:104634. doi: 10.1016/j.still.2020.104634
Basak, N., Datta, A., Biswas, S., Mitran, T., and Mandal, B. (2016). Organic amendment influences soil quality in farmers’ field under rice-based cropping systems in indo-Gangetic plains of India. J. Indian Soc. Soil Sci. 64:138. doi: 10.5958/0974-0228.2016.00018.9
Bhattacharya, P., Maity, P. P., Mowrer, J., Maity, A., Ray, M., Das, S., et al. (2020). Assessment of soil health parameters and application of the sustainability index to fields under conservation agriculture for 3, 6, and 9 years in India. Heliyon 6:e05640. doi: 10.1016/j.heliyon.2020.e05640
Bhattacharyya, R., Das, T. K., Das, S., Dey, A., Patra, A. K., Agnihotri, R., et al. (2019). Four years of conservation agriculture affects topsoil aggregate-associated 15nitrogen but not the 15nitrogen use efficiency by wheat in a semi-arid climate. Geoderma 337, 333–340. doi: 10.1016/j.geoderma.2018.09.036
Bhattacharyya, R., Das, T. K., Sudhishri, S., Dudwal, B., Sharma, A. R., Bhatia, A., et al. (2015). Conservation agriculture effects on soil organic carbon accumulation and crop productivity under a rice-wheat cropping system in the western indo-Gangetic Plains. Eur. J. Agron. 70, 11–21. doi: 10.1016/j.eja.2015.06.006
Bhattacharyya, P., Pal, R., Chakrabarti, K., and Chakraborty, A. (2004). Effect of composting on extractability and relative availability of heavy metals present in Calcutta municipal solid waste. Arch. Agron. Soil Sci. 50, 181–187. doi: 10.1080/03650340310001627694
Bhattacharyya, R., Prakash, V., Kundu, S., and Gupta, H. S. (2006). Effect of tillage and crop rotations on pore size distribution and soil hydraulic conductivity in sandy clay loam soil of the Indian Himalayas. Soil Tillage Res. 86, 129–140. doi: 10.1016/j.still.2005.02.018
Biswas, S., Hazra, G. C., Purakayastha, T. J., Saha, N., Mitran, T., Singha Roy, S., et al. (2017). Establishment of critical limits of indicators and indices of soil quality in rice-rice cropping systems under different soil orders. Geoderma 292, 34–48. doi: 10.1016/j.geoderma.2017.01.003
Black, G. R., and Hartge, K. H. (1971). Methods of soil analysis-part 1: bulk density. Agronom. Monogr. 9, 363–375.
Brejda, J. J., Karlen, D. L., Smith, J. L., and Allan, D. L. (2000). Identification of regional soil quality factors and indicators II. Northern Mississippi Loess Hills and Palouse prairie. Soil Sci. Soc. Am. J. 64, 2125–2135. doi: 10.2136/sssaj2000.6462125x
Brunel, N., Seguel, O., and Acevedo, E. (2013). Conservation tillage and water availability for wheat in the dryland of central Chile. J. Soil Sci. Plant Nutr. 13, 622–637. doi: 10.4067/s0718-95162013005000050
Bünemann, E. K., Bongiorno, G., Bai, Z., Creamer, R. E., De Deyn, G., de Goede, R., et al. (2018). Soil quality – a critical review. Soil Biol. Biochem. 120, 105–125. doi: 10.1016/j.soilbio.2018.01.030
Chauhan, B. S., Mahajan, G., Sardana, V., Timsina, J., and Jat, M. L. (2012). Productivity and sustainability of the rice-wheat cropping system in the indo-Gangetic Plains of the Indian subcontinent: problems, opportunities, and strategies. Adv. Agron. 117, 315–369. doi: 10.1016/B978-0-12-394278-4.00006-4
Dam, R. F., Mehdi, B. B., Burgess, M. S. E., Madramootoo, C. A., Mehuys, G. R., and Callum, I. R. (2005). Soil bulk density and crop yield under eleven consecutive years of corn with different tillage and residue practices in a sandy loam soil in Central Canada. Soil Tillage Res. 84, 41–53. doi: 10.1016/j.still.2004.08.006
Das, S., Bhattacharyya, R., Das, T. K., Sharma, A. R., Dwivedi, B. S., Meena, M. C., et al. (2021). Soil quality indices in a conservation agriculture based rice-mustard cropping system in north-western indo-Gangetic Plains. Soil Tillage Res. 208:104914. doi: 10.1016/j.still.2020.104914
Das, B., Chakraborty, D., Singh, V. K., Ahmed, M., Singh, A. K., and Barman, A. (2016). Evaluating fertilization effects on soil physical properties using a soil quality index in an intensive rice-wheat cropping system. Pedosphere 26, 887–894. doi: 10.1016/s1002-0160(15)60093-5
Das, A., Ghosh, P. K., Lal, R., Saha, R., and Ngachan, S. (2014). Soil quality effect of conservation practices in maize-rapeseed cropping system in eastern Himalaya. Land Degrad. Dev. 28, 1862–1874. doi: 10.1002/ldr.2325
Das, T. K., Saharawat, Y. S., Bhattacharyya, R., Sudhishri, S., Bandyopadhyay, K. K., Sharma, A. R., et al. (2018). Conservation agriculture effects on crop and water productivity, profitability and soil organic carbon accumulation under a maize-wheat cropping system in the north-western indo-Gangetic Plains. Field Crops Res. 215, 222–231. doi: 10.1016/j.fcr.2017.10.021
Dey, A., Dwivedi, B. S., Bhattacharyya, R., Datta, S. P., Meena, M. C., Das, T. K., et al. (2016). Conservation agriculture in a rice-wheat cropping system on an alluvial soil of north-western indo-Gangetic plains: effect on soil carbon and nitrogen pools. J. Indian Soc. Soil Sci. 64, 246–254. doi: 10.5958/0974-0228.2016.00034.7
Dick, L. R., Cruikshank, A. A., Grenier, L., Melandri, F. D., Nunes, S. L., and Stein, R. L. (1996). Mechanistic studies on the inactivation of the proteasome by lactacystin. J. Biol. Chem. 271, 7273–7276. doi: 10.1074/jbc.271.13.7273
Doran, J. W., and Parkin, T. B. (1994). “Defining and assessing soil quality” in Defining soil quality for a sustainable environment. eds. W. Doran, D. C. Coleman, D. F. Bezdicek, and B. A. Stewart, vol. 35 (Madison: SSSA Special Publications), 1–21.
Dorneles, E. P., Lisboa, B. B., Abichequer, A. D., Bissani, C. A., Meurer, E. J., and Vargas, L. K. (2015). Tillage, fertilization systems and chemical attributes of a Paleudult. Sci. Agric. 72, 175–186. doi: 10.1590/0103-9016-2013-0425
FAO (2005). Save and grow in practice: maize, rice, wheat. Available at: http://www.fao.org/publications/save-and-grow/maize-rice-wheat/en/.
FAO (2011). Conservation agriculture: principles, sustainable land management and ecosystem services. FAO: Rome.
Fernández, R., Frasier, I., Noellemeyer, E., and Quiroga, A. (2017). Soil quality and productivity under zero tillage and grazing on Mollisols in Argentina – a long-term study. Geoderma Reg. 11, 44–52. doi: 10.1016/j.geodrs.2017.09.002
Fischer, R. A., Santiveri, F., and Vidal, I. R. (2002). Crop rotation, tillage and crop residue management for wheat and maize in the sub-humid tropical highlands: I. Wheat and legume performance. Field Crops Res. 79, 107–122. doi: 10.1016/S0378-4290(02)00157-0
Garcia, C., Roldan, A., and Hernandez, T. (1997). Changes in microbial activity after abandonment of cultivation in a semiarid mediterranean environment. J. Environ. Qual. 26, 285–292. doi: 10.2134/jeq1997.00472425002600010040x
Gathala, M. K., Ladha, J. K., Saharawat, Y. S., Kumar, V., Kumar, V., and Sharma, P. K. (2011). Effect of tillage and crop establishment methods on physical properties of a medium-textured soil under a seven-year rice−wheat rotation. Soil Sci. Soc. Am. J. 75, 1851–1862. doi: 10.2136/sssaj2010.0362
Ghimire, R., Machado, S., and Bista, P. (2017). Soil pH, soil organic matter, and crop yields in winter wheat-summer fallow systems. Agron. J. 109, 706–717. doi: 10.2134/agronj2016.08.0462
Ghosh, A., Bhattacharyya, R., Dey, A., Dwivedi, B. S., Meena, M. C., Manna, M. C., et al. (2019). Long-term fertilisation impact on temperature sensitivity of aggregate associated soil organic carbon in a sub-tropical inceptisol. Soil Tillage Res. 195:104369. doi: 10.1016/j.still.2019.104369
Ghosh, S., Das, T. K., Shivay, Y. S., Bandyopadhyay, K. K., Bhatia, A., and Yeasin, M. (2022). Weed interference and wheat productivity in a conservation agriculture-based maize-wheat-mungbean system. J. Crop Weed 18, 111–119. doi: 10.22271/09746315.2022.v18.i1.1540
Gomez, K. A., and Gomez, A. A. (1984). Statistical procedures for agricultural research. New York: John Wiley & Sons.
Govaerts, B., Fuentes, M., Mezzalama, M., Nicol, J. M., Deckers, J., Etchevers, J. D., et al. (2007). Infiltration, soil moisture, root rot and nematode populations after 12 years of different tillage, residue and crop rotation managements. Soil Tillage Res. 94, 209–219. doi: 10.1016/j.still.2006.07.013
Gupta, D. K., Bhatia, A., Kumar, A., Das, T. K., Jain, N., Tomer, R., et al. (2016). Mitigation of greenhouse gas emission from rice-wheat system of the indo-Gangetic plains: through tillage, irrigation and fertilizer management. Agric. Ecosyst. Environ. 230, 1–9. doi: 10.1016/j.agee.2016.05.023
Hanway, J. J., and Heidel, H. (1952). Soil analysis methods as used in Iowa state college soil testing laboratory. Iowa State College Bull. 57, 1–131.
Hassan, I., Hussain, Z., and Akbar, G. (2005). Effect of permanent raised beds on water productivity for irrigated maize-wheat cropping system. Australian Centre for International Agriculture Research Proceeding 121, of a workshop on “Evaluation and Shah, Anwar-Ul-Hassan, Ghafoor and Bakhsh 21 performance of permanent raised bed cropping systems in Asia, Australia and Mexico”. Grifith, NSW.
Hati, K. M., Chaudhary, R. S., Mandal, K. G., Bandyopadhyay, K. K., Singh, R. K., Sinha, N. K., et al. (2015). Effects of tillage, residue and fertilizer nitrogen on crop yields, and soil physical properties under soybean-wheat rotation in vertisols of Central India. Agric. Res. 4, 48–56. doi: 10.1007/s40003-014-0141-7
Hati, K. M., Swarup, A., Dwivedi, A. K., Misra, A. K., and Bandyopadhyay, K. K. (2007). Changes in soil physical properties and organic carbon status at the topsoil horizon of a vertisol of Central India after 28 years of continuous cropping, fertilization and manuring. Agric. Ecosyst. Environ. 119, 127–134. doi: 10.1016/j.agee.2006.06.017
Hirzel, J., Cordero, K., Fernández, C., Acuña, J., Sandoval, M., and Zagal, E. (2012). Soil potentially mineralizable nitrogen and its relation to rice production and nitrogen needs in two paddy rice soils of Chile. J. Plant Nutr. 35, 396–412. doi: 10.1080/01904167.2012.639920
Hobbs, P. R., Sayre, K., and Gupta, R. (2007). The role of conservation agriculture in sustainable agriculture. Philos. Trans. R. Soc. B 363, 543–555. doi: 10.1098/rstb.2007.2169
Iqbal, M., Ul-Hassan, A., and Van Es, H. M. (2011). Influence of residue management and tillage systems on carbon sequestration and nitrogen, phosphorus, and potassium dynamics of soil and plant and wheat production in semi-arid region. Commun. Soil Sci. Plant Anal. 42, 528–547. doi: 10.1080/00103624.2011.546929
Jain, M., Fishman, R., Mondal, P., Galford, G. L., Bhattarai, N., Naeem, S., et al. (2021). Groundwater depletion will reduce cropping intensity in India. Sci. Adv. 7:eabd2849. doi: 10.1126/sciadv.abd2849
Jat, S. L., Parihar, C. M., Dey, A., Nayak, H. S., Ghosh, A., Parihar, N., et al. (2019). Dynamics and temperature sensitivity of soil organic carbon mineralization under medium-term conservation agriculture as affected by residue and nitrogen management options. Soil Tillage Res. 190, 175–185. doi: 10.1016/j.still.2019.02.005
Jia, G.-M., Xi, Y., Zhang, B.-L., and Chen, F. (2014). Soil labile organic carbon and microbial activity changes with age in citrus (Citrus sinensis Osb.) plantations in China. Aust. For. 77, 153–158. doi: 10.1080/00049158.2014.897921
Kaiser, H. F. (1960). The application of electronic computers to factor analysis. Educ. Psychol. Meas. 20, 141–151. doi: 10.1177/001316446002000116
Karlen, D. L., Ditzler, C. A., and Andrews, S. S. (2003). Soil quality: why and how? Geoderma 114, 145–156. doi: 10.1016/s0016-7061(03)00039-9
Kassambara, A., and Mundt, F. (2017). Factoextra: extract and visualize the results of multivariate data analyses. R package version 1, 337–354.
Kemper, W. D., and Rosenau, R. C. (1986). Aggregate stability and size distribution. Methods of soil analysis: Part 1. Phys. Mineral. Methods 5, 425–442. doi: 10.2136/sssabookser5.1.2ed.c17
Lal, B., Gautam, P., Panda, B. B., Raja, R., Singh, T., Tripathi, R., et al. (2017). Crop and varietal diversification of rainfed rice based cropping systems for higher productivity and profitability in eastern India. PLoS One 12:e0175709. doi: 10.1371/journal.pone.0175709
Lampurlanés, J., and Cantero-Martínez, C. (2003). Soil bulk density and penetration resistance under different tillage and crop management systems and their relationship with barley root growth. Agron. J. 95, 526–536. doi: 10.2134/agronj2003.5260
Lê, S., Josse, J., and Husson, F. (2008). FactoMineR: an R package for multivariate analysis. J. Stat. Softw. 25, 1–18. doi: 10.18637/jss.v025.i01
Liu, Z., Rong, Q., Zhou, W., and Liang, G. (2017). Effects of inorganic and organic amendment on soil chemical properties, enzyme activities, microbial community and soil quality in yellow clayey soil. PLoS One 12:e0172767. doi: 10.1371/journal.pone.0172767
Mandal, B., Majumdar, B., Adhya, T. K., Bandyopadhyay, P. K., Gangopadhyay, A., Sarkar, D., et al. (2008). Potential of double-cropped rice ecology to conserve organic carbon under subtropical climate. Glob. Chang. Biol. 14, 2139–2151. doi: 10.1111/j.1365-2486.2008.01627.x
Martín-Lammerding, D., Tenorio, J. L., Albarrán, M. M., Zambrana, E., and Walter, I. (2013). Influence of tillage practices on soil biologically active organic matter content over a growing season under semiarid Mediterranean climate. Span. J. Agric. Res. 11, 232–243. doi: 10.5424/sjar/2013111-3455
Masto, R. E., Chhonkar, P. K., Purakayastha, T. J., Patra, A. K., and Singh, D. (2008). Soil quality indices for evaluation of long-term land use and soil management practices in semi-arid sub-tropical India. Land Degrad. Dev. 19, 516–529. doi: 10.1002/ldr.857
Mbuthia, L. W., Acosta-Martínez, V., DeBruyn, J., Schaeffer, S., Tyler, D., Odoi, E., et al. (2015). Long term tillage, cover crop, and fertilization effects on microbial community structure, activity: implications for soil quality. Soil Biol. Biochem. 89, 24–34. doi: 10.1016/j.soilbio.2015.06.016
Meena, M. C., Dwivedi, B. S., Mahala, D., Das, S., and Dey, A. (2018). “Nutrient dynamics and management under conservation agriculture,” in System based conservation agriculture. eds. V.K. Singh and B. Gangwar (Westville Publishing House: New Delhi), 43–57.
Mishra, A. K., Aggarwal, P., Bhattacharyya, R., Das, T. K., Sharma, A. R., and Singh, R. (2015). Least limiting water range for two conservation agriculture cropping systems in India. Soil Tillage Res. 150, 43–56. doi: 10.1016/j.still.2015.01.003
Mohammad, W., Shah, S. M., Shehzadi, S., and Shah, S. A. (2012). Effect of tillage, rotation and crop residues on wheat crop productivity, fertilizer nitrogen and water use efficiency and soil organic carbon status in dry area (rainfed) of north-west Pakistan. J. Soil Sci. Plant Nutr. 12, 715–727. doi: 10.4067/s0718-95162012005000027
Mohanty, M., Painuli, D., Misra, A., and Ghosh, P. (2007). Soil quality effects of tillage and residue under rice-wheat cropping on a vertisol in India. Soil Tillage Res. 92, 243–250. doi: 10.1016/j.still.2006.03.005
Monsefi, A., Sharma, A. R., Rang Zan, N., Behera, U. K., and Das, T. K. (2014). Effect of tillage and residue management on productivity of soybean and physico-chemical properties of soil in soybean-wheat cropping system. Int. J. Plant Prod. 8, 429–440. doi: 10.22069/IJPP.2014.1618
Mrabet, R., Saber, N., El-Brahli, A., Lahlou, S., and Bessam, F. (2001). Total, particulate organic matter and structural stability of a Calcixeroll soil under different wheat rotations and tillage systems in a semiarid area of Morocco. Soil Tillage Res. 57, 225–235. doi: 10.1016/s0167-1987(00)00180-x
Muchabi, J., Lungu, O. I., and Mweetwa, A. M. (2014). Conservation agriculture in Zambia: effects on selected soil properties and biological nitrogen fixation in soya beans (Glycine max (L.) Merr). Sustain. Agric. Res. 3:28. doi: 10.5539/sar.v3n3p28
Naab, J. B., Mahama, G. Y., Yahaya, I., and Prasad, P. V. V. (2017). Conservation agriculture improves soil quality, crop yield, and incomes of smallholder farmers in North Western Ghana. Front. Plant Sci. 8:996. doi: 10.3389/fpls.2017.00996
Naresh, R. K. (2016). Soil conservation practices for sustainability of rice-wheat system in subtropical climatic conditions: a review. Int. J. Pure Appl. Biosci. 4, 133–165. doi: 10.18782/2320-7051.2162
Nath, C. P., Das, T. K., Rana, K. S., Bhattacharyya, R., Pathak, H., Paul, S., et al. (2017). Weed and nitrogen management effects on weed infestation and crop productivity of wheat-mungbean sequence in conventional and conservation tillage practices. Agric. Res. 6, 33–46. doi: 10.1007/s40003-017-0246-x
Olsen, S. R. (1954). Estimation of available phosphorus in soils by extraction with sodium bicarbonate. USDA Circular No. 939. Washington, DC: US Department of Agriculture.
Parihar, C. M., Jat, S. L., Singh, A. K., Ghosh, A., Rathore, N. S., Kumar, B., et al. (2017a). Effects of precision conservation agriculture in a maize-wheat-mungbean rotation on crop yield, water-use and radiation conversion under a semiarid agro-ecosystem. Agric. Water Manag. 192, 306–319. doi: 10.1016/j.agwat.2017.07.021
Parihar, C. M., Jat, S. L., Singh, A. K., Kumar, B., Yadvinder-Singh,, Pradhan, S., et al. (2016). Conservation agriculture in irrigated intensive maize-based systems of North-Western India: effects on crop yields, water productivity and economic profitability. Field Crops Res. 193, 104–116. doi: 10.1016/j.fcr.2016.03.013
Parihar, C. M., Jat, S. L., Singh, A. K., Majumdar, K., Jat, M. L., Saharawat, Y. S., et al. (2017b). Bio-energy, water-use efficiency and economics of maize-wheat-mungbean system under precision-conservation agriculture in semi-arid agro-ecosystem. Energy 119, 245–256. doi: 10.1016/j.energy.2016.12.068
Parihar, C. M., Singh, A. K., Jat, S. L., Dey, A., Nayak, H. S., Mandal, B. N., et al. (2020). Soil quality and carbon sequestration under conservation agriculture with balanced nutrition in intensive cereal-based system. Soil Tillage Res. 202:104653. doi: 10.1016/j.still.2020.104653
Paz-Kagan, T., Shachak, M., Zaady, E., and Karnieli, A. (2014). A spectral soil quality index (SSQI) for characterizing soil function in areas of changed land use. Geoderma 230-231, 171–184. doi: 10.1016/j.geoderma.2014.04.003
Piegholdt, C., Geisseler, D., Koch, H. J., and Ludwig, B. (2013). Long-term tillage effects on the distribution of phosphorus fractions of loess soils in Germany. J. Plant. Nutr. Soil Sci. 176, 217–226. doi: 10.1002/jpln.201200393
Ram, H., Singh, Y., Saini, K. S., Kler, D. S., Timsina, J., and Humphreys, E. J. (2011). Agronomic and economic evaluation of permanent raised beds, no tillage and straw mulching for an irrigated maize-wheat system in northwest India. Exp. Agric. 48, 21–38. doi: 10.1017/s0014479711000809
Roldan, A. (2003). No-tillage, crop residue additions, and legume cover cropping effects on soil quality characteristics under maize in Patzcuaro watershed (Mexico). Soil Tillage Res. 72, 65–73. doi: 10.1016/s0167-1987(03)00051-5
Saad, A. A., Das, T. K., Rana, D. S., Sharma, A. R., Bhattacharyya, R., and Lal, K. (2016). Energy auditing of a maize-wheat-greengram cropping system under conventional and conservation agriculture in irrigated north-western indo-Gangetic Plains. Energy 116, 293–305. doi: 10.1016/j.energy.2016.09.115
Salomé, C., Coll, P., Lardo, E., Metay, A., Villenave, C., Marsden, C., et al. (2016). The soil quality concept as a framework to assess management practices in vulnerable agroecosystems: a case study in Mediterranean vineyards. Ecol. Indic. 61, 456–465. doi: 10.1016/j.ecolind.2015.09.047
Saurabh, K., Rao, K. K., Mishra, J. S., Kumar, R., Poonia, S. P., Samal, S. K., et al. (2021). Influence of tillage based crop establishment and residue management practices on soil quality indices and yield sustainability in rice-wheat cropping system of eastern indo-Gangetic Plains. Soil Tillage Res. 206:104841. doi: 10.1016/j.still.2020.104841
Schoenholtz, S. H., Miegroet, H. V., and Burger, J. A. (2000). A review of chemical and physical properties as indicators of forest soil quality: challenges and opportunities. For. Ecol. Manag. 138, 335–356. doi: 10.1016/s0378-1127(00)00423-0
Shahid, M., Nayak, A. K., Shukla, A. K., Tripathi, R., Kumar, A., Mohanty, S., et al. (2013). Long-term effects of fertilizer and manure applications on soil quality and yields in a sub-humid tropical rice-rice system. Soil Use Manag. 29, 322–332. doi: 10.1111/sum.12050
Sharma, K. L., Grace, J. K., Mandal, U. K., Gajbhiye, P. N., Srinivas, K., Korwar, G. R., et al. (2008). Evaluation of long-term soil management practices using key indicators and soil quality indices in a semi-arid tropical Alfisol. Soil Res. 46, 368–377. doi: 10.1071/sr07184
Six, J., Elliott, E. T., and Paustian, K. (2000). Soil macroaggregate turnover and microaggregate formation: a mechanism for C sequestration under no-tillage agriculture. Soil Biol. Biochem. 32, 2099–2103. doi: 10.1016/s0038-0717(00)00179-6
Srinivasarao, C., Kundu, S., Kumpawat, B. S., Kothari, A. K., Sodani, S. N., Sharma, S. K., et al. (2019). Soil organic carbon dynamics and crop yields of maize (Zea mays)-black gram (Vigna mungo) rotation-based long term manurial experimental system in semi-arid Vertisols of western India. Trop. Ecol. 60, 433–446. doi: 10.1007/s42965-019-00044-x
Subbiah, B. V., and Asija, G. L. (1956). A rapid procedure for the determination available nitrogen in soils. Curr. Sci. 25, 259–260.
Tisdale, S.L., Nelson, W.L., Beaton, J.D., and Havlin, J.L. (1993). Soil fertility and fertilizers. New York: Macmillan. 176–229.
Van Der Heijden, M. G., Bardgett, R. D., and Van Straalen, N. M. (2008). The unseen majority: soil microbes as drivers of plant diversity and productivity in terrestrial ecosystems. Ecol. Lett. 11, 296–310. doi: 10.1111/j.1461-0248.2007.01139.x
Vance, E. D., Brookes, P. C., and Jenkinson, D. S. (1987). An extraction method for measuring soil microbial biomass C. Soil Biol. Biochem. 19, 703–707. doi: 10.1016/0038-0717(87)90052-6
Vanlauwe, B., Wendt, J., Giller, K. E., Corbeels, M., Gerard, B., and Nolte, C. (2014). A fourth principle is required to define conservation agriculture in sub-Saharan Africa: the appropriate use of fertilizer to enhance crop productivity. Field Crops Res. 155, 10–13. doi: 10.1016/j.fcr.2013.10.002
Keywords: maize-wheat rotation, conservation agriculture, soil quality parameters, soil quality index, principal component analysis, expert opinion, system productivity
Citation: Adak S, Bandyopadhyay K, Purakayastha TJ, Sen S, Sahoo RN, Shrivastava M and Krishnan P (2023) Impact of contrasting tillage, residue mulch and nitrogen management on soil quality and system productivity under maize-wheat rotation in the north-western Indo-Gangetic Plains. Front. Sustain. Food Syst. 7:1230207. doi: 10.3389/fsufs.2023.1230207
Edited by:
David Lapen, Agriculture and Agri-Food Canada (AAFC), CanadaReviewed by:
Nirmalendu Basak, Central Soil Salinity Research Institute (ICAR), IndiaNarendra Kumar, Indian Institute of Pulses Research (ICAR), India
Copyright © 2023 Adak, Bandyopadhyay, Purakayastha, Sen, Sahoo, Shrivastava and Krishnan. This is an open-access article distributed under the terms of the Creative Commons Attribution License (CC BY). The use, distribution or reproduction in other forums is permitted, provided the original author(s) and the copyright owner(s) are credited and that the original publication in this journal is cited, in accordance with accepted academic practice. No use, distribution or reproduction is permitted which does not comply with these terms.
*Correspondence: Kalikinkar Bandyopadhyay, a2suYmFuZHlvcGFkaHlheUBnbWFpbC5jb20=