- 1Department of Crop and Soil Sciences, Faculty of Agriculture, Kwame Nkrumah University of Science and Technology, Kumasi, Ghana
- 2Maize Improvement Program, International Institute of Tropical Agriculture (IITA), Ibadan, Oyo State, Nigeria
In West and Central Africa (WCA), drought and low soil nitrogen (low N) impede increased maize (Zea mays L.) productivity and production. Due to climate change, the two stresses usually occur together, leading to food, nutritional, and economic insecurity in the sub-region. There is, therefore, the need for the development and availability of high-yielding extra-early maturing white Quality Protein Maize (QPM) synthetics and hybrids with resilience to the prevailing stresses through the identification of superior climate smart (extra-early maturing) QPM inbreds under stress (drought and low soil N) conditions. The study was conducted to identify stress-resilient QPM inbred lines for hybrid production and assess the association between grain yield and other studied characters. During the 2012 minor and major rainy seasons, 96 extra-early white QPM inbreds and four (4) normal endosperm maize inbred checks were assessed in multi-location trials under stress and optimal conditions in Nigeria. The experiments were laid out in a 10 × 10 simple lattice design with two replications. Data were recorded on grain yield and other agronomic traits. Significant variations (p < 0.01) were detected among the inbred lines for measured characters, indicating adequate genetic variability among the inbreds to allow for selection and improvement of grain yield and other measured traits. Grain yield was interrelated with all the traits used in the selection index. Moderate to high estimates of heritability were observed for most of the measured traits under stress conditions, indicating that the traits could be easily transmitted to the progenies. Fifty-seven out of the 96 QPM inbreds evaluated exhibited varying degrees of resilience to drought and low N. The QPM inbreds with desired traits may be used as genetic resources for the incorporation of tolerance genes into QPM populations in the tropics, as well as for the development of drought and low N resilient synthetics and hybrids in WCA.
1 Introduction
Maize is an important staple crop for about half of the human population in sub-Saharan Africa (SSA). Due to its economic importance, it is anticipated to become the most important cereal crop by 2025 across the world (FAOSTAT, 2017; Bhadmus et al., 2021). However, factors such as drought and low soil N severely hamper its productivity and production across the sub-region, thereby increasing food and nutritional insecurity (Badu-Apraku et al., 2011; Annor and Badu-Apraku, 2016; Kountche et al., 2019). The current poor maize grain yield of 2.01 t ha−1 in SSA compared to the global estimate of 5.75 t ha−1 (FAOSTAT, 2020) is caused largely by low soil N and drought. Due to climate change and farmers' inability to afford adequate quantities of fertilizers, the effects of the two stresses are very severe under farmers' field conditions in SSA (Annor and Badu-Apraku, 2016; Ertiro et al., 2017).
Maize reaction to low soil nitrogen and drought is controlled by similar mechanisms (Badu-Apraku et al., 2012a,b; Obeng-Bio et al., 2019), and the two stresses frequently occur jointly in farmers' fields (Kim and Adetimirin, 1997; Badu-Apraku et al., 2011; Ertiro et al., 2017). Separately, drought and low N can cause grain output reduction of 40–50% (Wolfe et al., 1988; Amegbor et al., 2017; Annor et al., 2019) and 30–90% (Menkir and Akintunde, 2001; Annor et al., 2019), respectively. When these stresses occur jointly and the cultivated varieties are vulnerable, the combined effect could be a total (100%) grain yield loss (Kim and Adetimirin, 1997; Annor and Badu-Apraku, 2016). Therefore, combined nitrogen use efficiency and resilience to drought must be considered when developing maize genotypes for cultivation in SSA.
Normal endosperm maize grain contains ~10% protein. However, the levels of lysine and tryptophan are inadequate. This makes children fed on non-quality protein maize (non-QPM) without other dietary protein sources suffer growth deformities such as kwashiorkor due to malnutrition (Olakojo et al., 2007; Upadhyay et al., 2009; Mbuya et al., 2011; Annor et al., 2019; Bhadmus et al., 2021). Quality protein maize, however, can provide 73% of the protein needs of human beings and contains nearly twice the quantity of tryptophan and lysine in the non-QPM endosperm (Annor et al., 2019; Bhadmus et al., 2021). Conversely, the normal endosperm maize grain can supply only ~46% of the human protein requirements and has a protein content of ~1.81% lysine and 0.35% tryptophan (Krivanek et al., 2007; Obeng-Bio et al., 2019). Studies aimed at enhancing the creation of stress (combined drought and low N)-resilient extra-early white QPM inbred lines that reach physiological maturity between 80 and 85 days after planting (Oluwaranti et al., 2008; Badu-Apraku et al., 2012a,b) are therefore crucial in reducing nutritional and food insecurity among the rural, peri-urban, and urban populations in SSA. According to Badu-Apraku and Oyekunle (2012), extra-early maize genotypes mature before the onset of the dry season and are capable of escaping tropical drought. Extra-early maturing white QPM genotypes that contain alleles for resilience to low soil N and drought can survive the drought at the flowering and grain-filling periods during the growing seasons. The drought and low-N resilient maize can overcome the effects of climate change in the tropics, resulting in the reduction of the unpredictability of maize grain yields in SSA, particularly in the savannah zones, and the relatively shorter second growing season in the forest agro-ecological zones of the tropics (Badu-Apraku et al., 2013).
Because of the significance of QPM, numerous extra-early white QPM inbreds have been developed by scientists of the International Institute of Tropical Agriculture Maize Improvement Program (IITA-MIP). The major thrust of the maize improvement program has been to develop drought-resilient and high-yielding QPM synthetic cultivars and hybrids that are resilient to the prevailing stresses for the release and commercialization in SSA. However, this objective cannot be promptly achieved without adequate knowledge and a clear understanding of the responses of inbred lines developed in the program to stress conditions, especially low soil N and drought. According to Betrán et al. (2003) and Meseka et al. (2011), hybrid maize potential is largely linked with the performance of the parents, and hybrids or varieties that have one or two stress-resilient parents usually produce higher yields compared to those with both parents susceptible. Information on the responses of the parental inbreds under the prevailing stress conditions would enhance the development of efficient breeding strategies for generating maize genotypes for stress environments in SSA (Meseka et al., 2006). Hence, the reaction of inbred lines to stresses should be considered by breeders when developing stress-resilient hybrids aimed at overcoming the effects of climatic change in the tropics. However, data on the reaction of the existing extra-early maturing white QPM inbred lines to combined low soil N and drought is insufficient. The present study sought to (i) identify climate-smart QPM inbred lines that can tolerate drought and low N stresses and (ii) determine the association between grain yield and other agronomically desirable characters of 96 extra-early maturing white QPM inbred lines and four inbred checks under drought and low-N conditions.
2 Materials and methods
2.1 Experimental materials
The ninety-six (96) QPM inbreds used in the present study were developed from a cross between Pool 15 SR (a QPM donor source) and two extra-early Striga-resistant (SR) white normal endosperm inbreds by scientists of the IITA-MIP in Nigeria. Backcrossing the single cross hybrids (F1 hybrids) to the normal endosperm parents resulted in the first backcross (BC1) and second backcross (BC2) filial generations. Subsequently, the BC2 individuals were repeatedly self-pollinated to obtain 245 extra-early white QPM S6 inbred lines. Based on the assessment of the opaqueness of the maize kernels under a light box and the results of the field evaluations under drought at locations in Nigeria, 96 QPM inbred lines (Table 1) were selected for the present study.
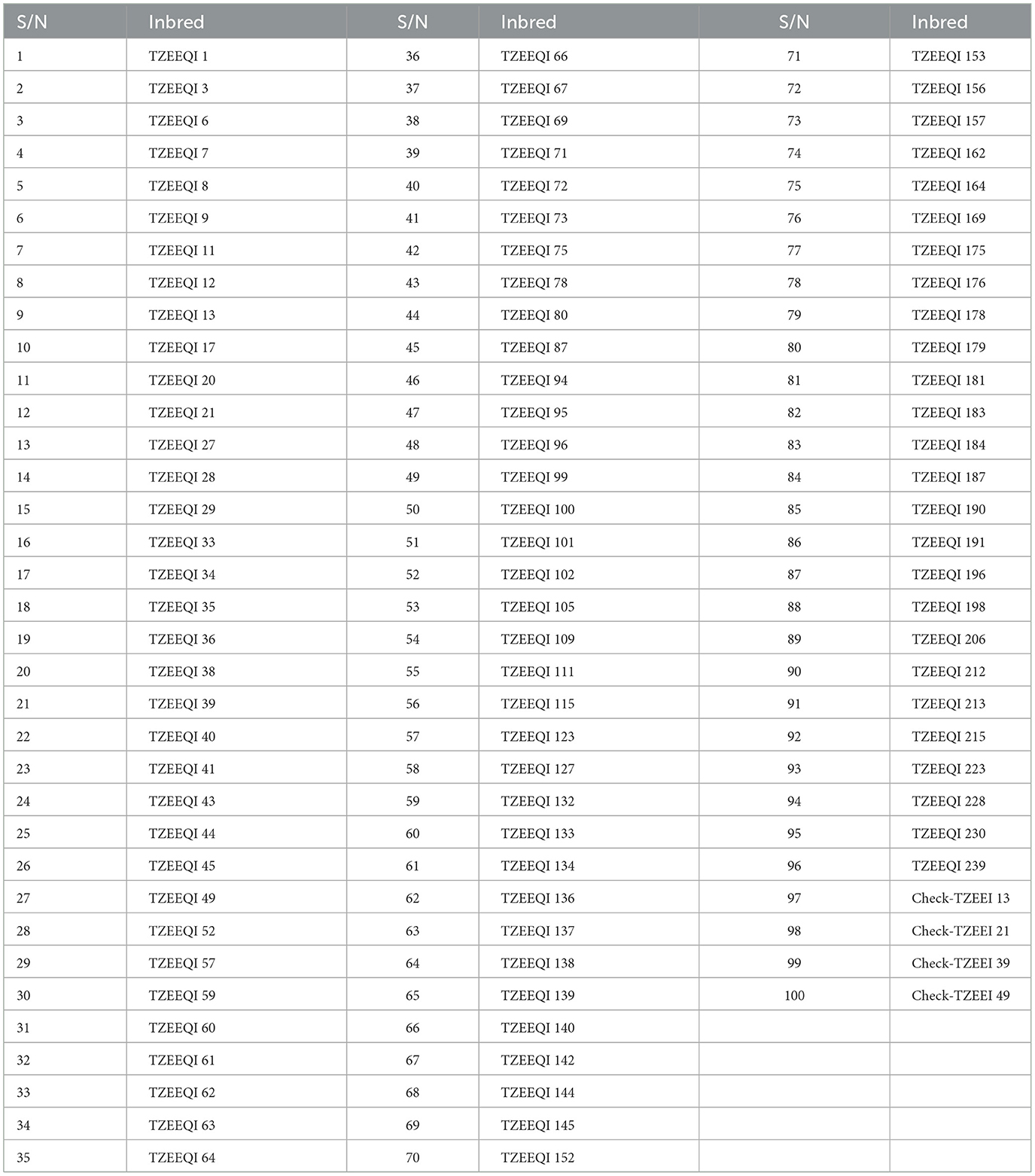
Table 1. List of 96 S6 extra-early maturing white quality protein maize inbred lines and four checks used in the study.
2.2 Field experiments
The 96 extra-early white QPM parental inbreds and four low N and drought-resilient normal endosperm maize checks (Table 1) were assessed in low soil nitrogen and moisture stress conditions in Nigeria. The experiment was conducted in drought environments at Bagauda, which is drought-prone lying at longitude 8°22′E, latitude 12°00′N, with an elevation of 580 m and an annual rainfall of 800 mm, during the 2012 rainy season (July–October).1 It was also conducted under imposed drought stress during the dry season (November–March) of 2012/2013 at the IITA drought research field at Ikenne. Furthermore, the trial was established under low soil nitrogen and optimal nitrogen environments at Mokwa and low soil nitrogen at Ile-Ife during the 2012 rainy season. A 10 × 10 simple lattice design with two (2) replications was utilized for all the experiments at the various sites. The experiments were made up of one-row plots, 4-m long with 0.75-m inter-row spacing and 0.40-m within-row spacing. Three seeds were sown per hole at planting and thinned to two 14 days after planting (DAP) to achieve ~66,000 plants ha−1 population density. The experimental site at Ikenne has ~1,200 mm of annual rainfall and is found in Nigeria at longitude 6°87′N, latitude 3°7′E, and 30 meters elevation. On the other hand, Mokwa is situated at longitude 5°4′E, latitude 9°18′N, 457 m altitude, and has an average annual rainfall of 1,100 mm, whereas Ile-Ife records an average annual rainfall of 1,200 mm and is positioned at a longitude 4°33′ E, latitude 7°28′ N, and 244 m above sea level in Nigeria.
At Ikenne, drought stress was imposed by stopping the irrigation water application 21 DAP to ensure the drought occurred at the bloom and grain-producing stages. Throughout the first 21 days of crop growth and development, a sprinkler irrigation system was utilized to make available 17 mm of water each week, as demonstrated in a similar research conducted by Annor and Badu-Apraku (2016) and Annor et al. (2019). The Ikenne experimental site is characterized as eutric nitisol soils with a high water-holding capacity. At planting, the drought experiments were fertilized by applying 60 kg ha−1 of phosphorus (P), 60 kg ha−1 of nitrogen (N), and 60 kg ha−1 of potassium (K). Additionally, 60 kg ha−1 of nitrogen was applied at 14 DAP. To ensure that the trials were weed-free, gramoxone and atrazine were applied as post- and pre-emergence weedicides at 5 l/ha of paraquat and primextra. The weedicides application was supplemented by manual weeding.
The selected maize inbreds were also assessed at the Mokwa and Ile-Ife experimental sites, which had been continuously depleted of nitrogen for several years by removing the biomass of cultivated maize after each harvesting. To ascertain the amount of nitrogen in the soil, samples of soil were taken from 0 to 15 cm depth for analysis at the soil laboratory of IITA in Ibadan, Nigeria, before planting (after land preparation). The soil analysis was done following the Association of Official Analytical Chemists (AOAC, 1984) procedure. Based on the nitrogen level in the soil (soil analysis results), urea fertilizer was applied to bring the nitrogen content of the low soil nitrogen plots to about 30 kg ha−1, while the level of the optimal nitrogen block was increased to 90 kg ha−1 at 14 DAP. This brought the N, P, and K fertilizers applied to the low N conditions to 30, 60, and 60 kg ha−1, respectively, while the N, P, and K fertilizers applied to the optimum N conditions were 90, 60, and 60 kg ha−1, respectively.
Muriates of potash and single superphosphate were applied to the low soil N and optimal N fields to achieve 60 kg ha−1 of K2O and 60 kg ha−1 of P2O5. To reduce the nitrogen fertilizer movement from one block to the other, the low and optimal nitrogen experiments were carried out in adjacent blocks set apart by a 10-m alley. The other agronomic practices carried out were as reported earlier for the drought trials.
2.3 Data collection
Data were taken on: days to 50% silking (DS) = the number of days between planting and when 50% of the plants' silks appeared; days to 50% anthesis (DA) = the number of days from planting and when 50% of the plants began to shed pollen grains. The anthesis-silking interval (ASI) was estimated as the difference between DA and DS; plant height (cm) = the distance between the plant's base and the height of the first tassel branch (for 10 randomly selected plants); ear height (cm) = the distance between the plant's base and the height of the node carrying the upper ear (mean of 10 randomly selected plants); root lodging was taken as the percentage of plants that leaned more than 30 degrees from the vertical; stalk lodging = the proportion of plants that had broken at or below the highest ear node; husk cover = measurement of how tight or loose the ear tip was (rating was on a scale of 1 to 9, with 1 indicating husks densely packed and extending beyond the ear tip and 9 indicating ear tips exposed); ear aspect = the overall look of the ears without the husks (ear aspect was graded on a scale of 1–9 based on ear size, uniformity of size, color, and texture, level of grain filling, and insect and disease damage); ears per plant (EPP) = the number of ears collected per plot divided by the number of plants in a plot at harvest; plant aspect was scored on a scale of 1 to 9 (Amegbor et al., 2017) based on the assessment of overall design of plants in a plot as they appealed to sight; and stay-green characteristic was rated at 70 DAP (10 WAP) on a scale of 1–9 (Annor and Badu-Apraku, 2016) based on the percentage of dead leaves in the low N and drought trials. Harvested ears from each plot were shelled, and grain weight was assessed in tests conducted under low N and drought conditions. The moisture content of the grains was tested using the Kett moisture tester PM-450. Grain yield in kg ha−1 was determined using shelled grain weight and a moisture level of 15%. However, for the optimal experiment, a shelling percentage of 80% for inbred lines per plot was assumed, and grain yield (obtained from ear weight converted to kg ha−1) was adjusted to 15% moisture content.
2.4 Statistical analysis
The square root transformation method was used to transform the data recorded for stalk lodging, ear rot, and root lodging after converting them to percentages. The Statistical Analysis System (SAS) (SAS Institute, 2011) was used for the analysis of variance (ANOVA) of the data taken across the stresses (low N and drought) and under optimal conditions. The entries (inbreds) were considered fixed factors, while the environments, incomplete blocks within replicates × environment interactions, and replicates within the environments were considered random factors in the ANOVA across the stress conditions. The means that were estimated with SAS were separated by the standard error (S.E).
To identify stress-resilient inbred lines for the present study, a base index (BI), which comprised grain yield, plant aspect (PASP), ears per plant (EPP), anthesis-silking interval (ASI), stay-green characteristic (STGR), and ear aspect (EASP), was used as reported by Annor and Badu-Apraku (2016). The stress BI was estimated as follows:
To minimize the effects of the different scales, a mean of zero and a standard deviation of BI were used to standardize the traits. A negative BI estimate was an indicator of the susceptibility of an inbred, while a positive estimate was a sign of an inbred's resilience to stress, as reported by other researchers (Annor and Badu-Apraku, 2016).
The SAS PROC Varcomp was used to compute the heritability in the broad sense (H2) for the observed variables under stress conditions by estimating the phenotypic and genetic variation of the inbreds. The heritability values were obtained using the formula below:
where is the genotype x environment interaction, is the genotypic variance, is the error variance, r is the number of replicates per environment, and e is the number of environments (Fehr, 1991).
The association between the measured traits was determined by estimating the correlation coefficients using the PROC CORR in SAS. Employing the grain yield data for the inbreds, the genotype main effects plus genotype x environment interaction (GGE) biplot analysis was performed to break down the inbred x environment interactions into their components and to obtain the information on the most promising inbreds across the research conditions as described by Yan (2001).
3 Results
3.1 Variance analysis of grain yield and other agronomic parameters of 96 extra-early white quality protein maize inbred lines and checks
The combined ANOVA over four stress environments of the inbred lines revealed differences (P < 0.01) among inbreds and environments for measured parameters in the study. Under optimum conditions, there was genotypic variation for all parameters except for ear and plant heights. The ANOVA also revealed that inbred x environment interaction was highly variable for measured parameters apart from the number of ears per plant (Table 2).
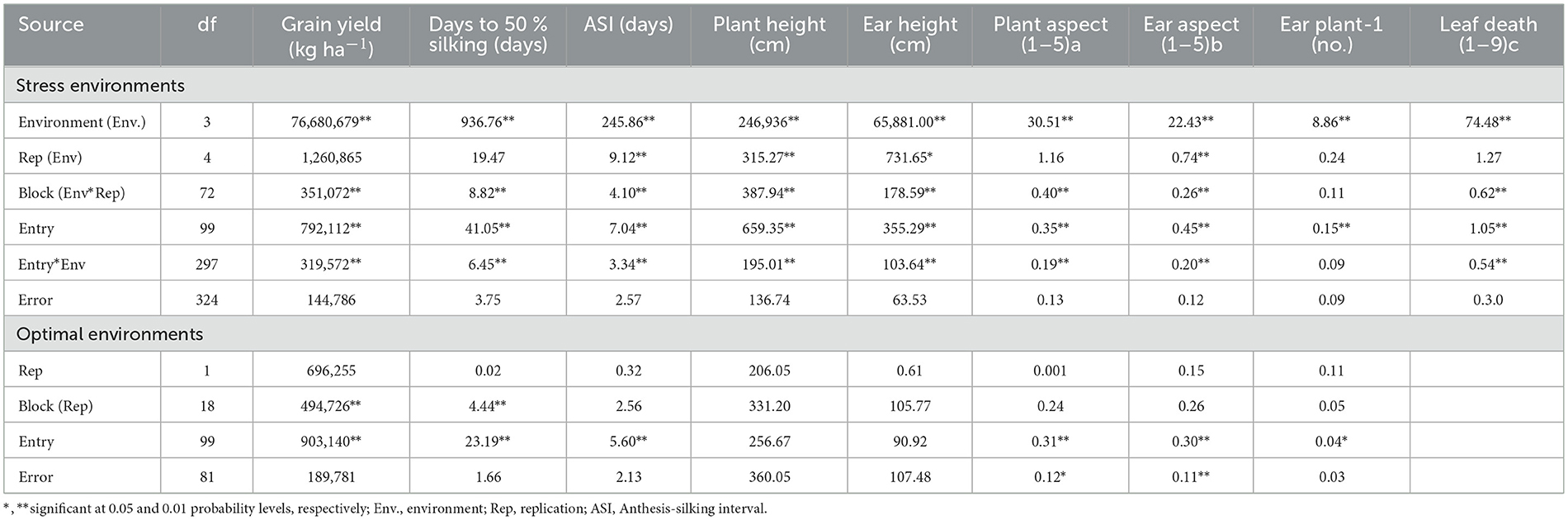
Table 2. Grain yield and other agronomic parameters of 96 extra-early white QPM maize inbreds and four checks assessed under low soil nitrogen, drought stress, and optimal growing conditions in Nigeria, 2012.
3.2 Performance of inbred lines under stress and optimum conditions
Under stress conditions, the mean grain production of the inbreds varied from 447 kg ha−1 for TZEEQI 223–1,567 kg ha−1 for TZEEQI 60, with an overall mean of 1,020 kg ha−1. Under optimum conditions, grain yield ranged from 744 kg ha−1 for TZEEQI 239–2,582 kg ha−1 for TZEEQI 7, with a mean of 1,838 kg ha−1. Among the best 15 and worst 10 of the 96 extra-early QPM inbred lines selected based on their performance under stress conditions using the IITA base index, viz. inbreds TZEEQI 60, TZEEQI 7, TZEEQI 111, TZEEQI 78, and TZEEQI 137 with mean grain yield of 1,560, 1,404, 1,529, 15,667, 1,494, and 1,382 kg ha−1, respectively, proved to be superior in terms of grain yield and resilience to drought and low nitrogen stresses in comparison with the four normal endosperm drought and low nitrogen-resilient normal maize checks (TZEEI 13, TZEEI 21, TZEEI 39, and TZEEI 49) (Table 3). The yield of these QPM inbred lines except TZEEQI 137 was also significantly higher than those of the most stress-resilient normal endosperm inbred check, TZEEI 21 (based on the base index), with a mean grain yield of 1122 kg ha−1. However, the grain yield produced by the QPM inbreds was not significantly different from that of TZEEI 49, which recorded the highest yield among the checks. The grain yield produced by the QPM inbreds TZEEQI 78, TZEEQI 7, TZEEQI 60, and TZEEQI 111 were also significantly higher than those of TZEEI 13 and TZEEI 39. Under optimum conditions, the QPM inbred line, TZEEQI 7, produced much more grain (2,582 kg ha−1) than all the checks except TZEEI 21 (2,362 kg ha−1). An average yield decrease of 44% was revealed for the inbred lines across drought and low nitrogen stresses. The grain yield reduction was accompanied by fewer ears per plant, a rise in ASI, days to silking, and poor ear and plant aspects (Table 3). Based on the base index, 57 out of the 96 QPM inbred lines assessed across low nitrogen and drought stresses showed variable degrees of stress resilience (Table 4).
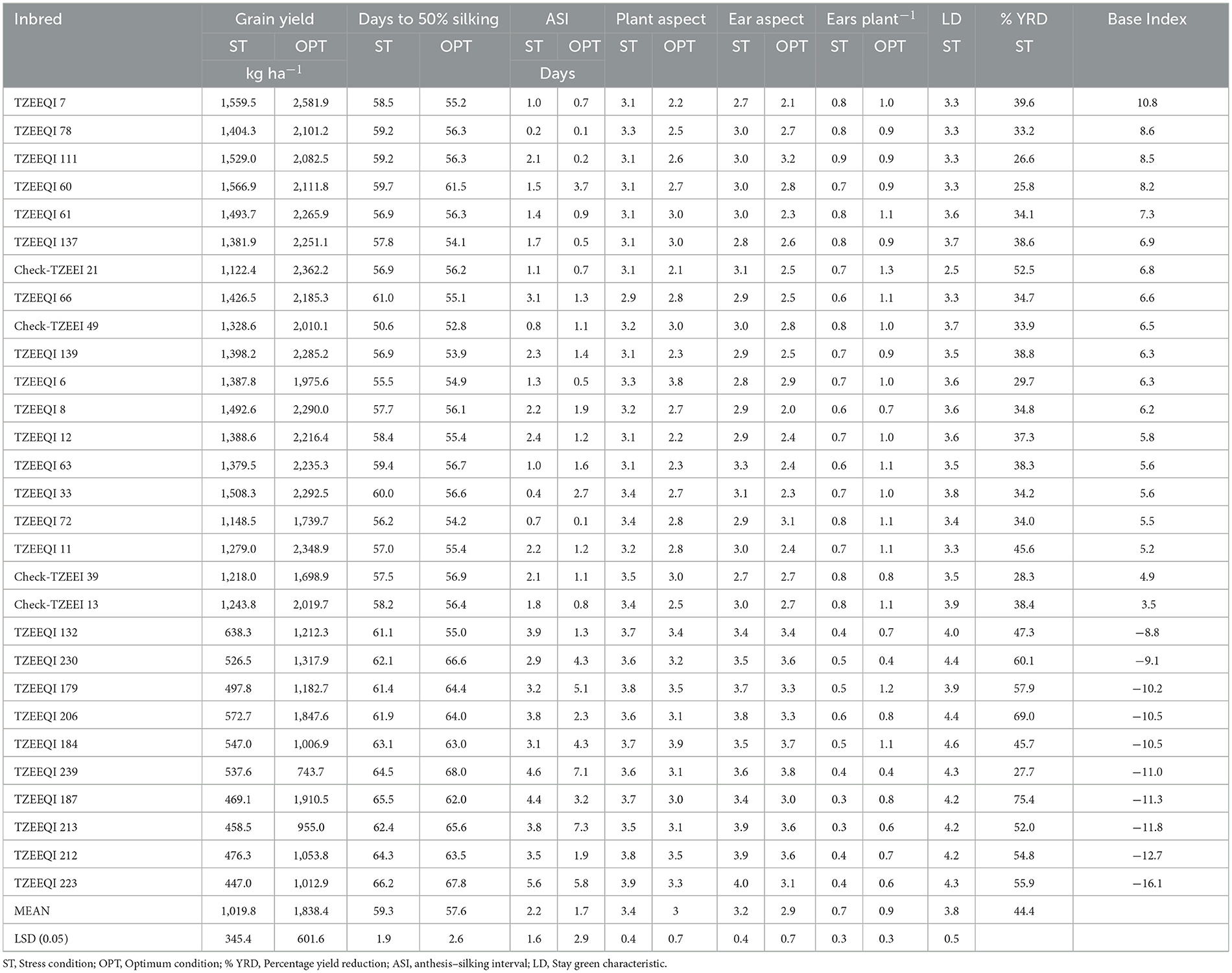
Table 3. Grain yield and other agronomic traits of etra-early white quality protein maize inbred lines (the best 15 and the worst 10 based on base index) and four normal checks evaluated under low -N, drought stress, and optimum conditions in Nigeria, 2012.
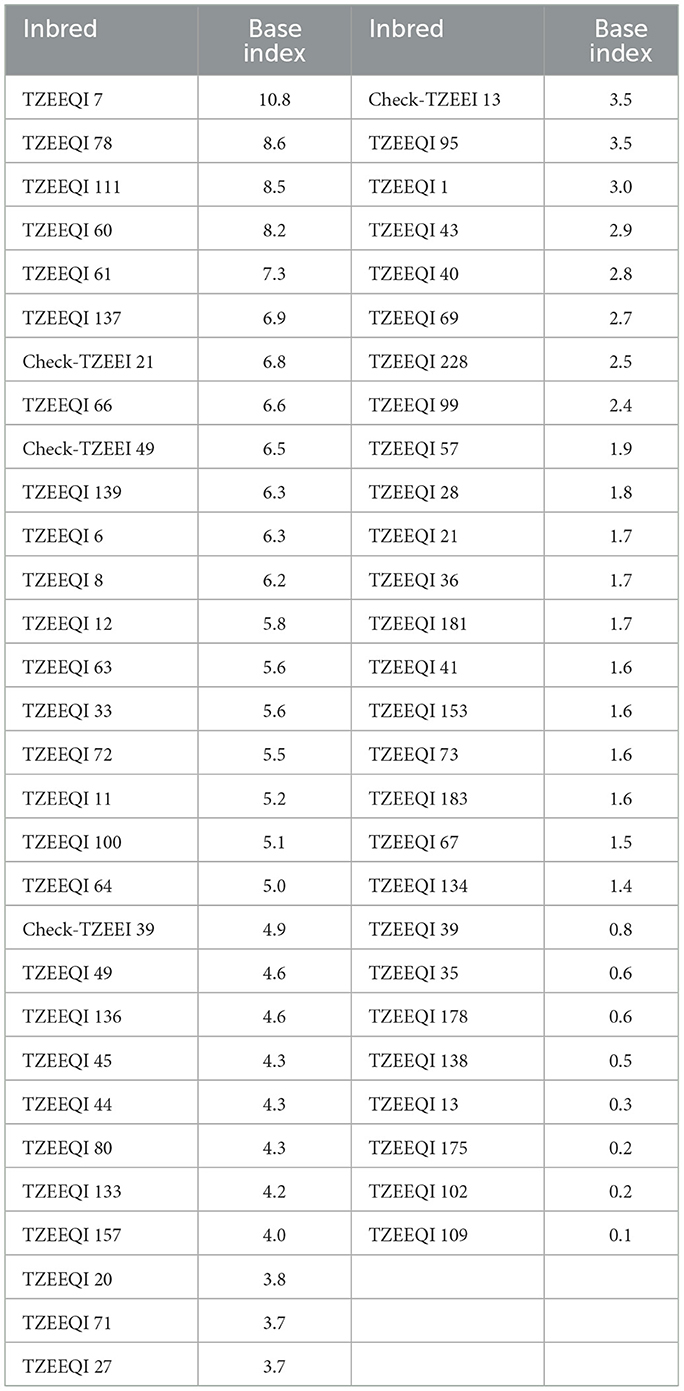
Table 4. List of 53 S6 extra-early maturing white quality protein maize inbred lines and four checks identified in the present study.
3.3 Relationship among the measured characters across stress environments
In the current study, grain yield had a high positive association with EPP but significant and negative relationships with days to 50% anthesis, ASI, days to 50% silking, ear height, plant aspect, ear aspect, and leaf death scores across the two (2) stress environments (Table 5). Heritability estimates for the measured traits varied from 0.00 for husk cover to 0.85 for days to 50% silking. The results revealed low heritability for root lodging (0.14), husk cover (0.00), and stalk lodging (0.26). Moderately high heritability estimates were observed for plant aspect (0.46), anthesis-silking interval (0.50), ear aspect (0.56), number of ears per plant (0.39), leaf death (0.47), and ear rot (0.42), while grain yield (0.65), days to 50% silking (0.85), days to 50% anthesis (0.84), ear height (0.73), and plant height (0.72) recorded very high heritability estimates across the two stress conditions (Table 5).
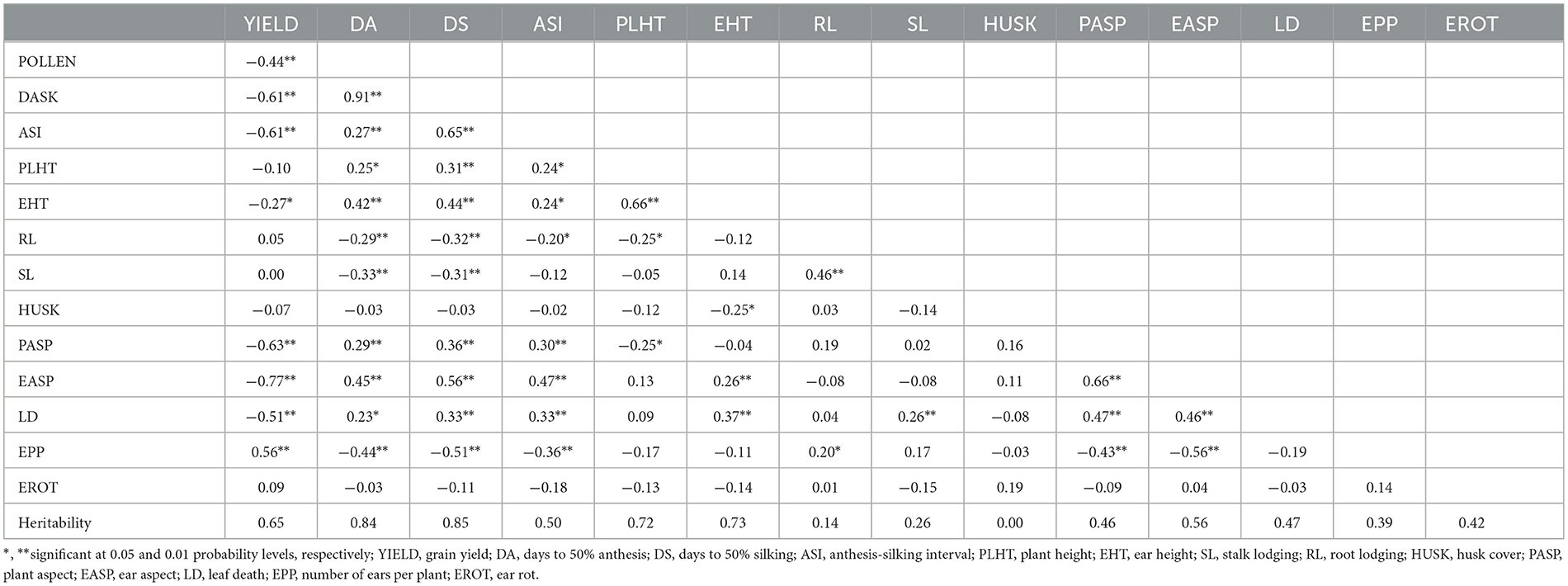
Table 5. Heritability estimates and Pearson's correlation coefficients for grain yield and other agronomic characters of 96 extra-early white quality protein maize inbred lines and four checks evaluated across drought and low N environments in Nigeria, 2012.
3.4 Grain yield performance and stability of selected QPM inbred lines across stress and optimum growing conditions
The significant genotype x environment interactions and genotypes for most measured traits across stress and optimum conditions justified using the GGE biplot to examine the yield performance and stability of the QPM inbreds across test environments. The GGE biplot is based on the grain yield performance of the 25 (20 best and five worst) QPM inbreds and four normal endosperm inbred checks studied across the stress and optimal conditions. The stability of inbred lines is determined by their projections onto the ATC abscissa. Thus, the longer the projection of an inbred line onto the ATC, the less the stability of the line. Based on these criteria, TZEEQI 7 (Entry 1) was the most stable and highest yielding, followed by inbred lines TZEEQI 60 (Entry 2) and TZEEQI 12 (Entry 10). Contrarily, TZEEI 63 (Entry 11) and TZEEQI 6 (Entry 13) were the least stable and lowest across test environments (Figure 1).
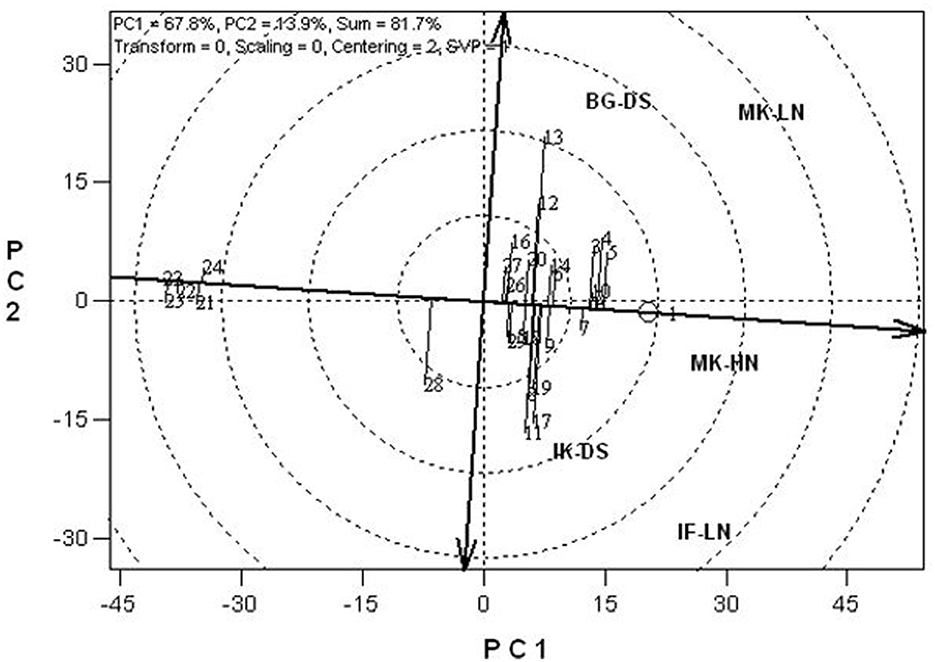
Figure 1. The yield performance and stability of 25 extra-early maturing white QPM inbred lines and four normal endosperm extra-early maize inbred checks evaluated under drought, low N, and optimum growing conditions at Bagauda, Ikenne, Ile-Ife, and Mokwa (1 = TZEEQI 7; 2 = TZEEQI 60; 3 = TZEEQI 33; 4 = TZEEQI 8; 5 = TZEEQI 6; 6 = TZEEQI 111; 7 = TZEEQI 66; 8 = TZEEQI 139; 9 = TZEEQI 137; 10= TZEEQI 12; 11 = TZEEQI 63; 12 = TZEEQI 78; 13 = TZEEQI 6; 14 = TZEEQI 11; 15 = TZEEQI 136; 16 = TZEEQI 73; 17 = TZEEQI 64; 18 = TZEEQI 134; 19 = TZEEQI 35; 20 = TZEEQI 133; 21 = TZEEQI 179; 22 = TZEEQI 212; 23 = TZEEQI 239; 24= TZEEQI 213; 25 = TZEEQI 223; 26 = Check-TZEEI 13; 27 = Check-TZEEI 21; 28 = Check-TZEEI 39; 29 = Check-TZEEI 49; IF-LN = Ile-Ife low nitrogen; MK-LN = Mokwa low nitrogen; MK-HN = Mokwa high nitrogen; BG-DS = Baguada drought stress; IK-DS = Ikenne drought stress).
4 Discussion
To reduce malnutrition and improve food security in SSA, there is a pressing need for drought-resilient QPM inbreds with high nitrogen utilization efficiency (resilient to low N) and genes for drought resilience. Such inbred lines could be utilized to develop high grain-producing QPM hybrids and synthetic cultivars resilient to both stresses. The differences detected among the inbreds, inbred x environment interactions (GEI), and environments observed in this study for most measured traits under stress and optimum conditions suggested that the test environments varied enough to enable the selection of inbred lines with outstanding performance, as indicated by Badu-Apraku et al. (2011, 2016). The results also suggested that adequate genetic variation existed among the genotypes to allow them to be utilized as sources of beneficial alleles for the development of extra-early white QPM breeding populations for resilience to low soil N and drought conditions. The current result agrees with the reports of Badu-Apraku and Oyekunle (2012) and Adu et al. (2021). Moreover, the varied GEI and environments revealed for most of the measured parameters of the inbreds across stress conditions indicated that there could be differences in the expression of the traits under the stress conditions. The implication is that the evaluations of the inbreds in more stressful environments would be required for the identification of the most outstanding inbred lines, as stated by other researchers under drought stress conditions (Badu-Apraku et al., 2011; Edmeades, 2013; Adu et al., 2021) and under low soil N (Meseka et al., 2006). This result endorses the substantial role that the growing environment plays in detecting genotypes with an outstanding performance across the two stress conditions.
The most important aim of the current study was to discover outstanding inbred lines for generating climate-smart synthetic cultivars and hybrids for SSA. Such outstanding inbred parents could also be used as sources of valuable alleles for stress resilience. Stress-resilient inbred lines would be priceless in maize breeding programs to develop stress-resilient and high grain-producing synthetic cultivars and hybrids (Betrán et al., 2003; Meseka et al., 2011) for cultivation in SSA. Fifty-seven (57) out of the 96 QPM inbred lines assessed under the stress conditions were found to be resilient to stress at different levels. The stress-resilient hybrids identified also had high grain yield, superior ear aspect, short ASI, high number of ears per plant, good stay-green characteristics, and better plant aspect. Therefore, the superior inbreds identified under stress situations would be valuable for the development of QPM populations in addition to the generation of high-yielding and stress-resilient synthetic cultivars and hybrids for commercialization in SSA. They will also be a good source of invaluable alleles for stress resilience.
The QPM inbreds TZEEQI 60 and TZEEQ1 7 were the best in terms of stability and yield across environments. The most stable and highest yielding QPM inbred, TZEEQI 7, out-yielded the best low nitrogen and drought-resilient normal endosperm check, TZEEI 21, by 28.0% under stress and 8.5% under optimum conditions. The low levels of yield reduction observed for the top-yielding inbred lines, compared to the checks, indicated the presence of genes for stress resilience in the inbred lines evaluated. The reduction in grain yield, along with an increase in ASI and days to silking, poor plant and ear aspects, and few ears per plant, suggested that the selection for reduced days to anthesis, shorter ASI, increased ears per plant, and good plant and ear aspects is the best method for breeding for stress resilience in the set of inbred lines used. The results in the current study concord with those by Badu-Apraku et al. (2011) and Adu et al. (2021), who suggested that the stay-green characteristic, plant aspect, ASI, and EPP should be considered for selecting genotypes in drought environments. Guei and Wassom (1992) and Badu-Apraku et al. (2023) also indicated that improvement in stress tolerance requires a good stay-green characteristic, shorter ASI, and an improved number of ears per plant. Short ASI, delayed leaf senescence, and an increased number of ears per plant in stress environments, according to Edmeades et al. (1993) and Zhao et al. (2022), are signs of better growth rates of ovules under stress and increased allocation of assimilates to the emerging ear. The grain yield losses of 44.4% for inbreds under stress conditions in the current study is within the range reported by workers in other studies (NeSmith and Ritchie, 1992; Menkir and Akintunde, 2001; Derera et al., 2008; Badu-Apraku et al., 2011).
The moderate to very high estimates of heritability (≥0.30) recorded in the current study for the plant aspect, anthesis-silking interval, grain yield, days to 50% anthesis, ear aspect, number of ears per plant, leaf death, ear rot, days to 50% silking, ear, and plant heights in stress environments showed that the parameters might be easily transferred to the progenies and phenotypic selection would also be effective since additive gene action is more important than non-additive gene action for most of the measured traits. The results also suggested that early generation selection for the characters under stress could be effective, as reported in other studies by Bänziger et al. (1999), Badu-Apraku et al. (2013), and Annor and Badu-Apraku (2016). The results of the present study are at variance with those of Badu-Apraku et al. (2011), who evaluated a set of inbred lines in stress environments and reported that selection for grain yield directly would not be effective due to the low heritability recorded for the trait. The low heritability recorded for husk cover, stalk lodging, and root lodging under stress conditions implied that phenotypic selection of the traits might not be promising, and therefore, the use of selection indices or indirect selection method would be required to assess the genetic values of the traits as indicated by Mhike et al. (2011).
The relationship found between grain yield and EPP suggested that the indirect selection of an increased number of ears per plant could result in improved grain yield across the two stress environments. Musila et al. (2010) also reported a strong positive connection between grain yield and the number of ears per plant across stress conditions. Contrarily, a negative relationship was displayed between grain yield and ASI, days to 50% anthesis, ear height, days to 50 % silking, plant aspect, leaf death, and ear aspect, indicating that selection for reduced ASI, ear height, days to 50% anthesis, plant aspect, days to 50% silking, ear aspect, and leaf death could improve grain yield in stress environments. A reliable secondary character for selecting genotypes for stress tolerance must have a strong relationship with grain yield, be highly heritable, and be very simple to record (Bänziger et al., 2000; Adu et al., 2021). Hence, grain yield, ASI, ear height, days to 50% anthesis, plant aspect, days to 50% silking, ear aspect, EPP, and leaf death could be very useful for selecting stress-resilient genotypes among the QPM inbred lines utilized in the current study. This report supported the discoveries of Betrán et al. (2003), Badu-Apraku et al. (2011), Badu-Apraku and Oyekunle (2012), Adu et al. (2021) that the most dependable characters for the identification of high yielding maize inbreds in stress environments were plant aspect, ASI, EPP, leaf death, ear aspect, and grain yield. Inbred lines TZEEQI 7, TZEEQI 12, and TZEEQI 60 were found to have exceptional stability and yield across the research environments and could be employed to produce outstanding QPM synthetics and hybrids for commercialization in SSA.
5 Conclusion
The inbred lines exhibited differences in the ability to tolerate low N and drought, with an average grain yield reduction of 44.4%. Moderate to high estimates of heritability were displayed by the majority of the studied characters across low N and drought, indicating that several measured characters studied could be easily transferred from the parental inbreds to the progenies and that selection could be done directly. Fifty-seven QPM inbred lines were found to be stress-resilient and could be easily employed as genetic resources for the incorporation of low N and drought-resilient genes into QPM populations in the tropics and the development of stress-resilient synthetic and hybrid cultivars in SSA.
Data availability statement
The datasets presented in this study can be found in online repositories. The names of the repository/repositories and accession number(s) can be found below: the datasets used in the present study have been deposited at the IITA CKAN repository http://data.iita.org.
Author contributions
BA and BB-A were involved in the experimental design, data collection, analysis, interpretation, and the entire write-up.
Funding
This research was supported by the Bill & Melinda Gates Foundation (OPP1134248) through the Stress Tolerant Maize for Africa (STMA) and Accelerated Genetic Gain (AGG) in Maize and Wheat Projects.
Acknowledgments
The authors are grateful to the Bill & Melinda Gates Foundation for providing financial support for this research through the Stress Tolerant Maize for Africa (STMA) Project. The authors are also grateful to the Alliance for a Green Revolution in Africa (AGRA) and the International Institute of Tropical Agriculture (IITA) for the financial support provided for this research. Special thanks are also extended to the late Prof. M.E. Aken'Ova, the first author's MSc project supervisor, and the field workers of the IITA Maize Improvement Program in Ibadan for their contributions to the effective implementation of this research.
Conflict of interest
The authors declare that the research was conducted in the absence of any commercial or financial relationships that could be construed as a potential conflict of interest.
Publisher's note
All claims expressed in this article are solely those of the authors and do not necessarily represent those of their affiliated organizations, or those of the publisher, the editors and the reviewers. Any product that may be evaluated in this article, or claim that may be made by its manufacturer, is not guaranteed or endorsed by the publisher.
Footnotes
1. ^Although the data were collected about a decade ago, this study is still very relevant because there are no high-yielding extra-early white QPM hybrids combining drought and low-N resilience currently released and commercialized in the sub-region. It is therefore important to make any information that could aid the development and identification of outstanding extra-early QPM varieties available to all relevant stakeholders across the globe.
References
Adu, G. B., Badu-Apraku, B., and Akromah, R. (2021). Strategies for selecting early maturing maize inbred lines for hybrid production under low soil nitrogen and Striga infestation. Agronomy 11, 1309. doi: 10.3390/agronomy11071309
Amegbor, I. K., Badu-Apraku, B., and Annor, B. (2017). Combining ability and heterotic patterns of extra-early maturing white maize inbreds with genes from Zea diploperennis under multiple environments. Euphytica 213, 24. doi: 10.1007/s10681-016-1823-y
Annor, B. B, Badu-Apraku, D., Nyadanu, R., Akromah, M. A. B., and Fakorede (2019). Testcross performance and combining ability of early maturing maize inbreds under multiple-stress environments. Sci. Rep. 9, 13809. doi: 10.1038/s41598-019-50345-3
Annor, B., and Badu-Apraku, B. (2016). Gene action controlling grain yield and other agronomic traits in extra-early quality protein maize under stress and non-stress conditions. Euphytica 212, 213–228. doi: 10.1007/s10681-016-1757-4
AOAC (1984). Official Methods of Analysis. 14th Ed. Washington, DC: Association of Official Agricultural Chemists, 9–23
Badu-Apraku, B., Akinwale, R. O., Ajala, S. O., Menkir, A., Fakorede, M. A. B., and Oyekunle, M. (2011). Relationships among traits of tropical early maize cultivars in contrasting environments. Agronomy J. 103, 717–729. doi: 10.2134/agronj2010.0484
Badu-Apraku, B., Akinwale, R. O., Franco, J., and Oyekunle, M. (2012a). Assessment of reliability of secondary traits in selecting for improved grain yield in drought and low- nitrogen environments. Crop Sci. 52, 2050–2062. doi: 10.2135/cropsci2011.12.0629
Badu-Apraku, B., Fakorede, M. A. B., Menkir, A., and Sanogo, D. (2012b). Conduct and Management of Maize Field Trials. Ibadan, Nigeria: IITA, 59.
Badu-Apraku, B., Fakorede, M. A. B., Nelimor, C., Osuman, A. S., Bonkoungou, T. O., Oyekunle, M., et al. (2023). Recent advances in breeding maize for drought, heat and combined heat and drought stress tolerance in sub-saharan africa. CABI Rev. 2023. doi: 10.1079/cabireviews.2023.0011
Badu-Apraku, B., Fakorede, M. A. B., Talabi, A. O., Oyekunle, M., Akaogu, I. C., Akinwale, R. O., et al. (2016). Gene action and heterotic groups of early white quality protein maize inbreds under multiple stress environments, Crop Sci. 56, 183–199. doi: 10.2135/cropsci2015.05.0276
Badu-Apraku, B., Oyekunle, M., Akinwale, R. O., and Aderounmu, M. (2013). Combining ability and genetic diversity of extra-early white maize inbreds under stress and nonstress environments. Crop Sci. 53, 9–26. doi: 10.2135/cropsci2012.06.0381
Badu-Apraku, B., and Oyekunle., M. (2012). Genetic analysis of grain yield and other traits of extra-early yellow maize inbreds and hybrid performance under contrasting environments. Field Crops Res. 129, 99–110. doi: 10.1016/j.fcr.2012.01.018
Bänziger, M., Edmeades, G. O., Beck, D., and Bellon, M. (2000). Breeding for Drought and Nitrogen Stress Tolerance in Maize: From Theory to Practice. Mexico: CIMMYT, 17–23.
Bänziger, M., Edmeades, G. O., and Lafitte, H. R. (1999). Selection for drought tolerance increases maize yields across a range of nitrogen levels. Crop Sci. 39, 1035–1040. doi: 10.2135/cropsci1999.0011183X003900040012x
Betrán, F. J., Bänziger, M., and Edmeades, G. O. (2003). Secondary traits in parental inbreds and hybrids under stress and non-stress environments in tropical maize. Field Crops Res. 83, 51–56. doi: 10.1016/S0378-4290(03)00061-3
Bhadmus, O. A., Badu-Apraku, B., Adeyemo, O. A., and Ogunkanmi, A. L. (2021). Genetic analysis of early white quality protein maize inbreds and derived hybrids under low-nitrogen and combined drought and heat stress environments. Plants 10, 2596. doi: 10.3390/plants10122596
Derera, J., Tongoona, P., Vivek, B. S., and Laing, M. D. (2008). Gene action controlling grain yield and secondary traits in southern African maize hybrids under drought and non-drought environments. Euphytica 162, 411–422 doi: 10.1007/s10681-007-9582-4
Edmeades, G. O. (2013). “Progress in achieving and delivering drought tolerance in maize- an update,” in The International Service for the Acquisition of Agri-biotech Applications (ISAAA). Ithaca, NY: Global Status of Commercialized Biotech/GM Crops.
Edmeades, G. O., Bolaños, J., Hernández, M., and Bello, S. (1993). Causes for silk delay in a lowland tropical maize population. Crop Sci. 33, 1029–1035. doi: 10.2135/cropsci1993.0011183X003300050031x
Ertiro, B. T., Beyene, Y., Das, B., Mugo, S., Olsen, M., Oikeh, S., et al. (2017). Combining ability and testcross performance of drought-tolerant maize inbred lines under stress and non-stress environments in Kenya. Plant Breed. 136, 197–205. doi: 10.1111/pbr.12464
FAOSTAT (2017). FAOSTAT. Food and Agriculture Organization of the United Nations. Available online at: http://faostat.fao.org/ (accessed 29 April, 2019).
FAOSTAT (2020). Crops. Food and Agriculture Organization of the United Nations. Available online at: https://www.fao.org/faostat/en/#country/81 (accessed September, 2021).
Fehr, W. R. (1991). “Principles of cultivar development,” in Theory and Technique. New York, NY: Macmillan.
Guei, R. G., and Wassom, C. E. (1992). Inheritance of some drought adaptive traits in maize: interrelationships between yield, flowering, and ears per plant. Maydica 37, 157–164.
Kim, S. K., and Adetimirin, V. O. (1997). Responses of tolerant and susceptible maize hybrids to timing and rate of nitrogen under Striga hermonthica infestation. Agron. J. 89, 38–44. doi: 10.2134/agronj1997.00021962008900010006x
Kountche, B. A., Jamil, M., Yonli, D., Nikiema, M. P., Blanco-Ania, D., Asami, T., et al. (2019). Suicidal germination as a control strategy for Striga hermonthica (Benth.) in smallholder farms of sub-Saharan Africa. Plants People Planet 1, 107–118. doi: 10.1002/ppp3.32
Krivanek, A. F., De Groote, H., Gunaratna, N. S., Diallo, A. O., and Friesen, D. (2007). Breeding and disseminating quality protein maize (QPM) for Africa. Afr. J. Biotechnol. 6, 312–324.
Mbuya, K., Nkongolo, K. K., and Kalonji-Mbuyi, A. (2011). Nutrition analysis of quality protein maize selected for agronomic characteristics in breeding program. Int. J. Plant Breed. Genet. 2, 325–332. doi: 10.3923/ijpbg.2011.317.327
Menkir, A., and Akintunde, A. O. (2001). Evaluation of the performance of maize hybrids improved open-pollinated and farmers' local varieties under well-watered and drought-stress conditions. Maydica 46, 227–238.
Meseka, S. K., Menkir, A., and Ajala, S. (2011). Genetic analysis of performance of maize inbred lines under drought stress. J. Crop Improvem. 25, 521–539 doi: 10.1080/15427528.2011.592570
Meseka, S. K., Menkir, A., Ibrahim, A. E. S., and Ajala, S. O. (2006). Genetic analysis of performance of maize inbred lines selected for tolerance to drought under low nitrogen. Maydica 51, 487–495.
Mhike, X., Lungu, D. M., and Vivek, B. (2011). Combining ability studies amongst AREX and CIMMYT maize (Zea mays L.) inbred lines under stress and non-stress conditions. Afr. J. Agric. Res. 6, 1952–1957.
Musila, R. N., Diallo, O. A., Makumbi, D., and Njoroge, K. (2010). Combining ability of early-maturing quality protein maize inbred lines adapted to Eastern Africa. Field Crops Res. 119, 231–237. doi: 10.1016/j.fcr.2010.07.009
NeSmith, D. S., and Ritchie, J. T. (1992). Effects of soil water-deficits during tassel emergence on development and yield components of maize (Zea mays L.). Field Crops Res. 28, 251–256. doi: 10.1016/0378-4290(92)90044-A
Obeng-Bio, E. B., Badu-Apraku, B. E., Ifie, A., Danquah, E., Blay, T., and Annor, B. (2019). Genetic analysis of grain yield and agronomic traits of early provitamin A quality protein maize inbred lines in contrasting environments. J. Agricult. Sci. 157, 1–21. doi: 10.1017/S0021859619000753
Olakojo, S. A., Omueti, O., Ajomale, K., and Ogunbodede, B. A. (2007). Development of quality protein maize: biochemical and agronomic evaluation. Trop. Subtrop. Agroecosyst. 7, 97–104.
Oluwaranti, A., Fakorede, M. A. B., and Badu-Apraku, B. (2008). Grain yield of maize varieties of different maturity groups under marginal rainfall conditions. J. Agricult. Sci. 53, 183–191. doi: 10.2298/JAS0803183O
Upadhyay, S., Gurung, D. B., Paudel, D. C., Koirala, K. B., Sah, S. N., Prasad, R. C., et al. (2009). Evaluation of quality protein maize (QPM) genotypes under rainfed mid-hill environments of Nepal. Nepal J. Sci. Technol. 10, 9–14. doi: 10.3126/njst.v10i0.2803
Wolfe, D. W., Henderson, D. W., Hsiao, T. C., and Alvio, A. (1988). Interactive water and nitrogen effects on maize. II. Photosynthetic decline and longevity of individual leaves. Agron. J. 80, 865–870. doi: 10.2134/agronj1988.00021962008000060005x
Yan, W. (2001). GGE biplot: a windows application for graphical analysis of multi-environment trial data and other types of two-way data. Agron. J. 93, 1111–1118. doi: 10.2134/agronj2001.9351111x
Keywords: climate change, drought, indirect selection for high yield, low soil nitrogen, quality protein maize, stress resilient maize
Citation: Annor B and Badu-Apraku B (2024) Selection of extra-early white quality protein maize (Zea mays L.) inbred lines for drought and low soil nitrogen resilient hybrid production. Front. Sustain. Food Syst. 7:1238776. doi: 10.3389/fsufs.2023.1238776
Received: 13 June 2023; Accepted: 31 December 2023;
Published: 30 January 2024.
Edited by:
Muhammad Saqlain Zaheer, Khwaja Fareed University of Engineering and Information Technology (KFUEIT), PakistanReviewed by:
Jean Mubalama Mondo, Université Evangélique en Afrique, Democratic Republic of CongoNemera Geleta Shargie, Agricultural Research Council of South Africa (ARC-SA), South Africa
Copyright © 2024 Annor and Badu-Apraku. This is an open-access article distributed under the terms of the Creative Commons Attribution License (CC BY). The use, distribution or reproduction in other forums is permitted, provided the original author(s) and the copyright owner(s) are credited and that the original publication in this journal is cited, in accordance with accepted academic practice. No use, distribution or reproduction is permitted which does not comply with these terms.
*Correspondence: Baffour Badu-Apraku, Yi5iYWR1LWFwcmFrdUBjZ2lhci5vcmc=