- Center for Food Safety and Applied Nutrition, Food and Drug Administration, College Park, MD, United States
Introduction: The objective of this study was to analyze antimicrobial resistance (AMR), stress resistance, and virulence factors through whole genome sequencing (WGS) of 192 isolates comprising 164 Salmonella isolates, 8 non-O157 Shiga toxin-producing Escherichia coli (STEC) isolates, and 20 Listeria monocytogenes isolates.
Methods: These isolates were sourced from a national survey conducted between 2010 and 2017, involving 31,322 samples of produce (31 isolates), nuts (43 isolates), and spices (118 isolates).
Results: The findings yielded several key insights: (1) Within all Salmonella isolates studied, the most prevalent Salmonella serotypes included Give, Kentucky, Senftenberg, Mbandaka, Anatum, Newport, and Weltevreden. (2) All eight non-O157 STEC isolates were found to carry the genes blaEC, acrF, and mdtM, while all 20 L. monocytogenes isolates possessed fosX and lin genes. The Salmonella isolates displayed diverse AMR gene profiles, with 3.65% exhibiting multi-drug resistance. (3) Both Salmonella and non-O157 STEC isolates were discovered to carry stress genes associated with acid resistance, but none of the L. monocytogenes isolates carried an acid resistance gene. Salmonella isolates were found to carry multiple metal-resistance genes. The non-O157 STEC isolates universally exhibited acid resistance genes, and 4 out of the 20 L. monocytogenes isolates were equipped with resistance genes against biocides. (4) All of the STEC isolates (100%) carried stx1 and stx2 genes, while none of them carried eae and wyz genes. Most L. monocytogenes isolates were found to contain 29 virulence genes and 1 pathogenicity island. All Salmonella isolates carried SPI-9, but lacked SPI-7, SPI-10, SPI-11, SPI-12, mig-5, prefA, tviA, and viaB genes.
Conclusion: These findings on AMR, stress resistance, and virulence factors among the investigated isolates highlight the potential risks they pose to public health and provide the scientific foundation for the development of preventative and control strategies and guidance pertaining to these major foodborne pathogens.
1. Introduction
Foodborne illnesses pose a persistent and significant global public health threat, imposing significant human and economic burdens. In 2010, these illnesses affected 600 million people worldwide, resulting in an estimated 420,000 fatalities (WHO, 2015). In the United States alone, it was reported that there are approximately 9.4 million cases of foodborne illnesses each year, attributed to 31 major pathogens, resulting in 55,961 hospitalizations and 1,351 fatalities (Scallan et al., 2011). Historically, from 1940 to 2004, 30% of emerging human infections were primarily transmitted through food (Jones et al., 2008). The FoodNet program by the Centers for Disease Control and Prevention (CDC) has identified several key foodborne pathogens, including Salmonella spp., Campylobacter spp., Shiga toxin-producing E. coli (STEC), Yersinia, Listeria monocytogenes, Shigella spp., Cryptosporidium, Vibrio, and Cyclospora, as major contributors to foodborne illnesses (CDC, 2017). Furthermore, some of these pathogens demonstrate remarkable persistence and the ability to survive under adverse conditions, such as temperature extremes (heat/cold stress), high sugar concentrations, low water activity, exposure to harsh chemicals, and contact with manure or slurry (Juven et al., 1984; Gibson and Khoury, 1986; Burton et al., 1987; Sorrells et al., 1989; Hirai, 1991; Lin et al., 1995; Tsai and Ingham, 1997; Burnett et al., 2000; Syamaladevi et al., 2016; Komora et al., 2017; Biswas et al., 2018); this resilience poses a significant and ongoing public health challenge worldwide.
The widespread global use of antibiotics to combat bacterial infections in both humans and animals has given rise to a pressing and significant public health crisis—antimicrobial resistance (AMR). What makes this issue even more concerning is the abundance of reports confirming the high prevalence of multi-drug-resistant (MDR) strains among major foodborne pathogens like Salmonella spp., STEC, and L. monocytogenes (Franz et al., 2014; Chang et al., 2015). The World Health Organization (WHO) conducted global surveillance across 194 member states, revealing alarming statistics. For instance, E. coli exhibited a substantial proportion of resistance to third-generation cephalosporins (44%, affecting 86 member states) and fluoroquinolones (47%, impacting 92 member states). Non-typhoidal Salmonella displayed a significant resistance rate to fluoroquinolones (35%, affecting 68 member states) (WHO, 2014). A comprehensive analysis of 48 non-typhoidal Salmonella outbreaks in the United States between 1984 and 2002 unveiled a startling fact that 28% of these outbreaks were caused by antimicrobial-resistant isolates, resulting in infections in 18,698 individuals (Varma et al., 2005). Considering the annual toll of over 2.8 million human illnesses and 35,000 deaths attributed to antibiotic-resistant pathogens in the United States alone, coupled with the conservative estimate of a $55 billion annual economic loss incurred by AMR for both the healthcare system and society at large (CDC, 2017, 2019), it is abundantly clear that the lack of comprehensive surveillance has left significant gaps in our understanding of AMR distribution and its associated infections. Hence, there is an urgent need to investigate and comprehend AMR from all angles, with a particular focus on the genetic level.
Whole genome sequencing (WGS) has found extensive application in routine diagnostic microbiology, including tasks such as detection, identification, drug susceptibility testing, monitoring antimicrobial resistance (AMR), epidemiological typing, outbreak management, and infection prevention (Köser et al., 2012; Alghoribi et al., 2018; Gygli et al., 2019). In addition to its cost-effectiveness and rapid turnaround time, WGS offers the capability to screen for virulence factors, new antibiotic resistance genes, and the identification of novel variants of antibiotic resistance genes in foodborne pathogens (Franz et al., 2014; Laabei et al., 2014; Nijhuis et al., 2015). Currently, laboratories and researchers worldwide are rapidly expanding the use of WGS to comprehensively investigate foodborne pathogens, particularly in the context of outbreaks. Nevertheless, there is a scarcity of WGS-based research addressing foodborne pathogens across large cohorts and diverse food samples as well as confronting the challenges posed by antimicrobial resistance.
In this study, we employed WGS technology to examine the genetic profiles related to antimicrobial resistance (AMR), stress resistance, and virulence factors in primary foodborne pathogens (Salmonella, STEC, and L. monocytogenes). These pathogens were isolated from a wide range of food sources, including domestic and imported varieties of leafy greens, sprouts, cantaloupes, mangoes, cucumbers, tree nuts, and dried spices (Zhang et al., 2017a,b, 2018).
2. Materials and methods
2.1. Sample collection and microbiological assays
Samples were meticulously collected from diverse geographic locations and various types of retail markets in the United States as outlined in Supplementary Tables S1–S3. The collection included the following: (A) a total of 14,183 samples of leafy greens (iceberg lettuce, romaine lettuce, and spinach) spanning the years from 2010 to 2012. (B) 2,652 sprout samples (alfalfa sprouts, bean sprouts, and other varieties such as radish, snow pea, daikon, clover, broccoli, dill, sunflower, green pea, adzuki, lentil, and mixed) collected between 2012 and 2014. (C) 1,160 cucumbers, 1,075 cantaloupes, and 1,176 mangoes were collected in the year 2014. (D) 3,656 samples of tree nuts (cashews, pecans, hazelnuts, macadamia nuts, pine nuts, and walnuts) were collected during the years 2014–2015. (E) 7,250 dried spice samples (basil leaf, black pepper, coriander seed, cumin seed, curry powder, dehydrated garlic, oregano leaf, paprika, red pepper, sesame seed, and white pepper) were collected from 2012 to 2015.
The leafy greens and fruit samples underwent thorough preparation and analysis to detect foodborne pathogens, including Salmonella, L. monocytogenes, E. coli O157:H7, non-O157 STEC, and Shigella. Meanwhile, the nuts and spice samples were also meticulously prepared and examined for the presence of Salmonella. Comprehensive information on sample collection, microbiological analysis methods, and data can be found in our previously published articles (Zhang et al., 2017a,b, 2018). It is worth noting that the WGS data of the isolates obtained from the aforementioned project were not presented in these prior publications.
2.2. Whole genome sequencing
Genomic DNA was extracted from an overnight pure culture of the confirmed isolates incubated at 37°C, using the DNeasy Blood & Tissue kit (Qiagen, Valencia, CA), and DNA concentration was measured using the Qubit fluorometer (Life Technologies, Invitrogen, CA, United States). DNA Libraries were prepared with the Nextera XT DNA Sample Preparation Kit (Illumina, San Diego, CA, United States) according to the manufacturer’s instructions. A paired-end 250-bp DNA sequencing (with a coverage depth of 30-90X) was carried out using the Illumina MiSeq platform (Illumina, San Diego, CA, United States) at the Center for Food Safety and Applied Nutrition (CFSAN) genomics laboratory of the U.S. Food and Drug Administration (FDA).
2.3. Genomic analysis
We selected 192 isolates for this project, including 8 non-O157 STEC, 20 L. monocytogenes, and 164 Salmonella strains. This selection was made with a focus on data quality and the need for reliable sequencing in individual samples. Moreover, this approach was also taken to ensure the accuracy and reliability of our analysis, in accordance with the previous studies (Zhang et al., 2017a,b, 2018). The WGS data of these isolates have been submitted to the National Center for Biotechnology Information (NCBI) and are accessible through the Sequence Read Archive (SRA). To conduct our analysis, we used the complete genomes of reference strains: Salmonella enterica subsp. enterica serovar Typhimurium str. LT2 (NC_003197.2) for Salmonella, Escherichia coli strain D2 (CP010137.1) for non-O157 STEC, and L. monocytogenes strain J1776 (CP006598.1) for L. monocytogenes. The raw sequencing reads were subsequently subjected to de novo assembly using CLC Workbench v12, developed by QIAGEN Bioinformatics (Redwood City, CA). Single nucleotide polymorphism (SNP) of each pathogen was performed following the FDA CFSAN SNP pipeline (Davis et al., 2015), then the phylogenetic trees, based on the whole genome sequences, were inferred using the method of Randomized Axelerated Maximum Likelihood (RAxML) with GTRCAT model, which was facilitated by Galaxytrakr.1 Mega v112 and iTOL v63 were used to visualize and present the resulting trees and serotyping outcomes.
The AMR genes were identified by the NCBI AMRFinder process in the NCBI Pathogen detection system,4 based on the Bacterial Antimicrobial Resistance Reference Gene Database. All isolates were run against more than 5,300 resistance genes/proteins (including antibiotic class aminoglycoside, avilamycin, beta-lactam, bleomycin, colistin, efflux, fluoroquinolone, fosfomycin, fusidic acid, glycopeptide, lincosamide, macrolide, mupirocin, nitroimidazole, phenicol, rifamycin, streptogramin, sulfonamide, tetracycline, thiostrepton, trimethoprim, and tuberactinomycin) to identify the AMR genes that are encoded in the genome sequence, while the alignment exceeded the curated identity threshold of 90% by default, and over 90% of the sequence indicated the presence of the AMR gene (Feldgarden et al., 2021).
The investigation of stress resistance genes was carried out using the NCBI Pathogen Detection System, screening for 235 stress resistance genes, including 2 acid resistance genes (asr and ymgB), 55 biocide resistance genes, 8 heat resistance genes, and 170 metal resistance genes (Feldgarden et al., 2021). A match with a reference gene of over 90% identity was considered indicative of the presence of the gene. The biocide resistance genes mainly focused on abgT family antimetabolite efflux transporter (mtrF), qacA/B family quaternary ammonium compound efflux MFS transporter (qacA and qacB), SMR family small multidrug resistance efflux protein (qac, emrE, smr, ssmE, bcrB, bcrC, emrE, and qacH), efflux transport transcriptional regulator (ttgR and ttgT), fluoride efflux transporter (crcB), multidrug efflux ABC transporter permease/ATP-binding subunit (smdA and smdB), multidrug efflux MFS transporter (lmrS), multidrug efflux RND transporter periplasmic adaptor subunit (sdeA), multidrug-binding transcriptional regulator (qacR), solvent efflux transporter (srpR and srpS), and tribuyltin resistance regulator (tbtR). The heat resistance genes were hdeD-GI, psi-GI, yfdX1, yfdX2, kefB-GI, trxLHR, hsp20, and shsP. The metal resistance genes were resistant to arsenic, cadmium/lead/zinc, gold, copper, silver, copper/silver, nickel, chromate, mercury, and tellurium (genes arsN/P/R, cadC/D/R, golS, silC/E/F/S, ncrA, arsA/B/C/D/H/P, dpsA, cnrY, nirA/D, nreB, merA/B/C/D/E/F/G/P/F/R, chrA/R, copC/D/S, pcoB/S/E/P, terB/C/D/E/W/Z, klaB/C, and copL).
Regarding the investigation of virulence factors, an extensive approach was used to obtain a more complete picture of the virulence gene landscape. We initially utilized the NCBI detection system, which includes approximately 740 virulence genes. However, recognizing that the majority of these virulence genes were associated with pathogens such as E. coli (621), Staphylococcus (54), and Yersinia (25), which were not directly relevant to the objectives of our current study; hence, we supplemented our analysis with additional typical virulence genes, gene clusters, and Salmonella pathogenicity islands (SPI) for non-O157 STEC (45), L. monocytogenes (32), and Salmonella (57). However, given the vast number of virulence genes screened, we are unable to present all the data in this context. However, we have selectively included only the most significant results in Figures 1–3. These selected virulence factors, which can be encoded on either plasmids or chromosomes and serve various functions, such as regulation, adhesion and invasion, intracellular growth, stress response, intimin, and fimbriae, among others, were chosen as references based on their frequent use in molecular detection methods such as polymerase chain reaction (PCR) and loop-mediated isothermal amplification (LAMP), which are commonly associated with each respective pathogen (Kreft and Vazquez-Boland, 2001; Lomonaco et al., 2008; Grimstrup Joensen et al., 2014; Yoon et al., 2015; Massot et al., 2016; Carroll et al., 2017; Xu et al., 2017). The investigated genes were blasted against the WGS of all isolates using CLC Workbench v12, and any match exceeding 90% identity to the reference gene was considered indicative of the presence of that particular gene. Listed here are the additional virulence factors selected: (A) 45 virulence factors selected for further analysis for non-O157 STEC: aap, aat, adk, aggR, bfpA, clbQ, cnf1, eae, ehly1, ehxA, elt, espP, fimH, fumC, fyuA, gyrB, hlyA, hlyC, hek, ibeA, icd, iha, ireA, iroN, irp, iucC, katP, mdh, neuC, ompT, papC, papG, pic, purA, recA, saa, sat, sfa/foc, stx1, stx2, subAB, traT, uidA, usp., and wzy. (B) 32 virulence factors for L. monocytogenes: 16sRNA, actA, arcA, betL, bsh, clpC, ClpE, clpP, ctsR, dnaK, dnaJ, gadD, groESL, hly, hpt, hrcA, htrA, inlA, inlB, inlC, inlJ, ldh, mpl, opuC, pdgA, plcA, plcB, prfA, sigB, LIPI-1, LIPI-3, and LIPI-4. (C) 57 virulence factors for Salmonella: 16 s rRNA, agf (A, B, C), avrA, bcfD, fhlA, fimICDHF, fur, grvA, hilA, himA, hin, hisJ, invA, invH, iroBC, lpfA, mgtB, mig-5, misL, mutS, orgC, pefA, phoP, ratB, rpoS, safABCD, shdA, sigDE, si0H, sipB, sirA, slyA, sodC, spaM, spaQ, spvRABCD, ssaQRSTU, sseC, sspABCD, stn, ttrRSBCA, tviA, viaB, SPI-1, SPI-2, SPI-3, SPI-4, SPI-5, SPI-6, SPI-7, SPI-8, SPI-9, SPI-10, SPI-11, SPI-12, SPI-13, and SPI-14.
3. Results
3.1. Occurrence of three foodborne pathogens in different types of food
All eight non-O157 STEC isolates that underwent sequencing were derived from leafy greens, specifically Romaine lettuce and spinach, with five of them originating from organic sources as shown in Supplementary Table S1.
The 20 sequenced L. monocytogenes isolates were sourced from a variety of foods, including spinach, Romaine lettuce, iceberg lettuce, alfalfa sprouts, broccoli, mango, and cucumber, with six of them traced back to organic samples, as detailed in Supplementary Table S2.
Among the 164 Salmonella isolates sequenced in this study (Supplementary Table S3), all were classified under Salmonella Group O, and 83 of these isolates were further identified to specific serotypes. Within this group, the most frequently observed Salmonella serotypes were Give (7 isolates), Kentucky (7 isolates), and Senftenberg (7 isolates), following by serotypes Mbandaka (6 isolates), Anatum (5 isolates), Newport (5 isolates), and Weltevreden (5 isolates). Salmonella was predominantly found in samples of macadamia nuts, capsicums, coriander, black pepper, cumin, and sesame. Serotypes Infantis, Newport, Montevideo, Muenchen, and Thompson were listed as the top 20 Salmonella serotypes that led to human infections on FoodNet (Figure 4). Furthermore, mangoes and cantaloupes were found to be contaminated with Salmonella serotypes Minnesota (in mangoes) and 6,7:m,t:- (in cantaloupes), with Newport also identified in cantaloupes. Tree nuts were frequently contaminated with Salmonella serotypes Muenchen, Give, Montevideo, and Senftenberg, with 43 isolates collectively. Among the four samples of nuts contaminated with Salmonella Give, three were organic macadamia nuts, and one was cashews. Salmonella Montevideo was primarily isolated from pistachio samples (three pistachios and one walnut). Additionally, organic macadamia nuts were contaminated by Salmonella serotypes Worthington and Diarizonae, organic pistachios by Salmonella Duisburg, and organic walnuts by Salmonella Thompson (Supplementary Table S3). Furthermore, out of the 118 Salmonella isolates obtained from dried spices, 14 different serotypes were identified. In particular, Salmonella serotypes Potsdam, Telhashomer, Arizonae IIIa 41:z4:-, and Diarizonae IIIb 61 were unique to spices in the United States market. Other serotypes, including Infantis, Meleagridis, Tennessee, Bareilly, Oranienburg, and Sandiego, were observed both in the spices and at the entry points to the United States markets. Kentucky (7 isolates), Anatum (5 isolates), and Newport (5 isolates) were the most frequently occurring serotypes in the spice samples entering the United States markets (Supplementary Table S3).
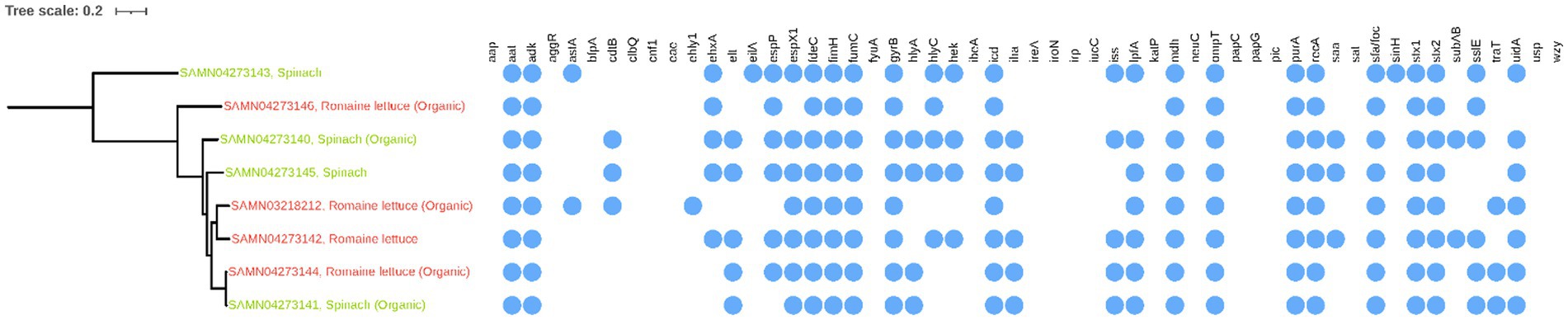
Figure 4. Association between Salmonella serotypes and positive food samples. Serotypes highlighted in yellow represent the top 20 Salmonella serotypes causing infections listed on FoodNet (CDC, 2017), and serotypes highlighted in green represent the top serotypes (≥4 samples) from all Salmonella samples examined in this study.
For more in-depth information regarding the prevalence of serotypes within each food category, please refer to our previous publications (Zhang et al., 2017a,b, 2018).
3.2. Prevalence and diversity of AMR genes
The prevalence of AMR genes was investigated in various pathogenic isolates. Among the non-O157 STEC isolates studied (Figure 5A; Supplementary Table S1), the AMR gene blaEC (associated with cephalosporin resistance) was dominant in all cases, along with two multidrug efflux transporters, acrF and mdtM. One isolate, derived from organic Romaine lettuce (SAMN04273144), exhibited the gyrA_S83L mutation, conferring resistance to fluoroquinolones. Another isolate from spinach carried mutations cyaA_S352T and uhpT_E350Q, responsible for fosmidomycin resistance. In the case of the 20 L. monocytogenes isolates (Figure 5B; Supplementary Table S2), only two resistance genes were identified: fosX (fosfomycin resistance) and lin (lincosamide resistance). The gene abc-f was observed in a single isolate (SAMN02943415) sourced from organic cucumber.
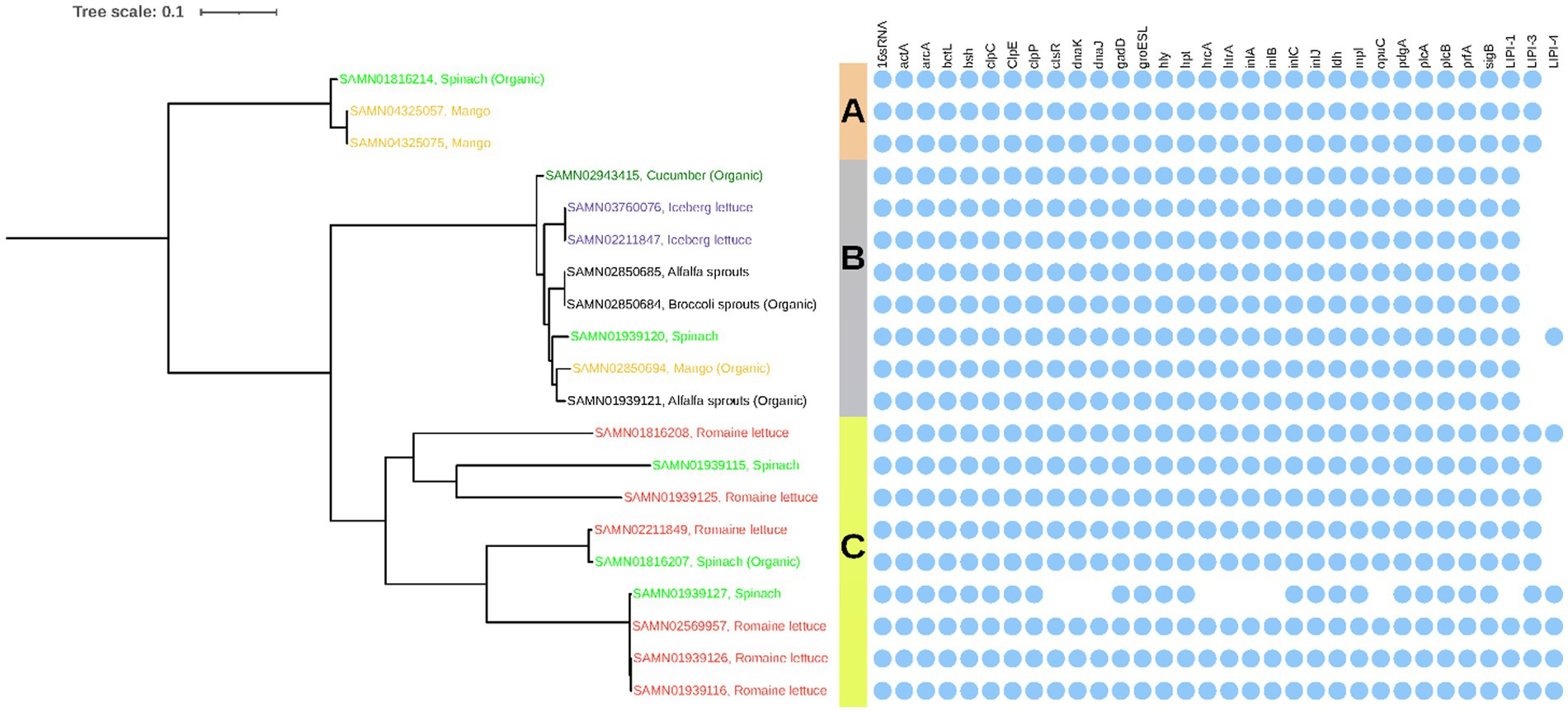
Figure 5. Prevalence of antimicrobial resistance (AMR) genes among the 192 isolates investigated. (A) AMR profiles of non-O157 Shiga toxin-producing E. coli; (B) AMR profiles of Listeria monocytogenes; and (C) AMR profiles of Salmonella.
The majority of isolates in our sample set were Salmonella, and their genomes were analyzed to assess the distribution of AMR genes (Figure 5C; Supplementary Table S3). The identified AMR genes included aac, ant, aph, aar, bla, bleO, cml/floR, dfr, fos, mph, qnr, sul, tet genes, gyrA/parC mutations, and the multidrug efflux RND transporter mdsA/mdsB. MdsA/mdsB genes were the most widespread, accounting for 76.3% of the total 405 AMR genes, followed by AMR genes that were resistant to aminoglycoside (5.43%), fosfomycin (3.95%), sulfonamide (2.47%), and tetracycline (2.47%). Isolates sourced from capsicums (17.53%), cumin (14.81%), and black (white) pepper (12.53%) carried an abundance of AMR genes compared with other matrices (<10%).
Data from Supplementary Table S3 reveal that 29/43 isolates (67.44%) from nuts samples carried only the multidrug efflux transporter, while 8 isolates carried both the fosA7 gene and multidrug efflux transporter. The sole MDR isolate from nuts was originally sourced from cashews. Isolates from oregano, curry powder, red pepper, paprika, and mixed spices exclusively carried the multidrug efflux transporter without other AMR genes. Basil samples contained the gyrA gene with a point mutation associated with quinolone resistance. The gyrA/parC gene was present in isolates from cashews, black pepper, cumin, garlic, capsicums, and sesame seeds. In particular, only one isolate from black pepper was observed to contain the bleO gene (resistant to bleomycin). Beta-lactam-resistance genes were found in isolates from cashews, black pepper, cumin, marjoram, capsicums, and sesame seeds. Sulfonamide and tetracycline resistance genes [sul1, sul2, sul3, tet(A), and tet(M)] were both present in the isolates sourced from cashew, cumin, marjoram, capsicums, and sesame seeds, while tetracycline resistance gene [tet(A) and tet(M)] in isolates from black pepper (Figure 5C; Supplementary Table S3).
Furthermore, seven MDR isolates (≥4 drug classes) were identified among the 164 Salmonella isolates studied. These MDR isolates were found in one cashew sample (sourced from Maryland, US) contaminated with Salmonella Give and six spice samples (two cumin samples with Salmonella Infantis and Mbandaka, one capsicum sample with Salmonella Kentucky, one marjoram sample with Salmonella Havana, one black pepper sample with Salmonella Rissen, and one sesame seed sample with Salmonella Kentucky) imported to the United States. Consequently, MDR isolates accounted for 3.65% of all Salmonella isolates (Supplementary Table S3).
3.3. Prevalence and diversity of stress genes
Among the eight non-O157 STEC isolates under investigation, all were found to possess the ymgB gene, which is associated with acid resistance (Figure 6A). One isolate (SAMN04273145) from a spinach sample was found to carry the acid resistance gene asr. Additionally, three isolates sourced from organic Romaine lettuce (SAMN03218212), conventional Romaine lettuce (SAMN04273142), and spinach (SAMN04273143) contained the emrE gene, conferring resistance to biocides (Class: Quaternary ammonium).
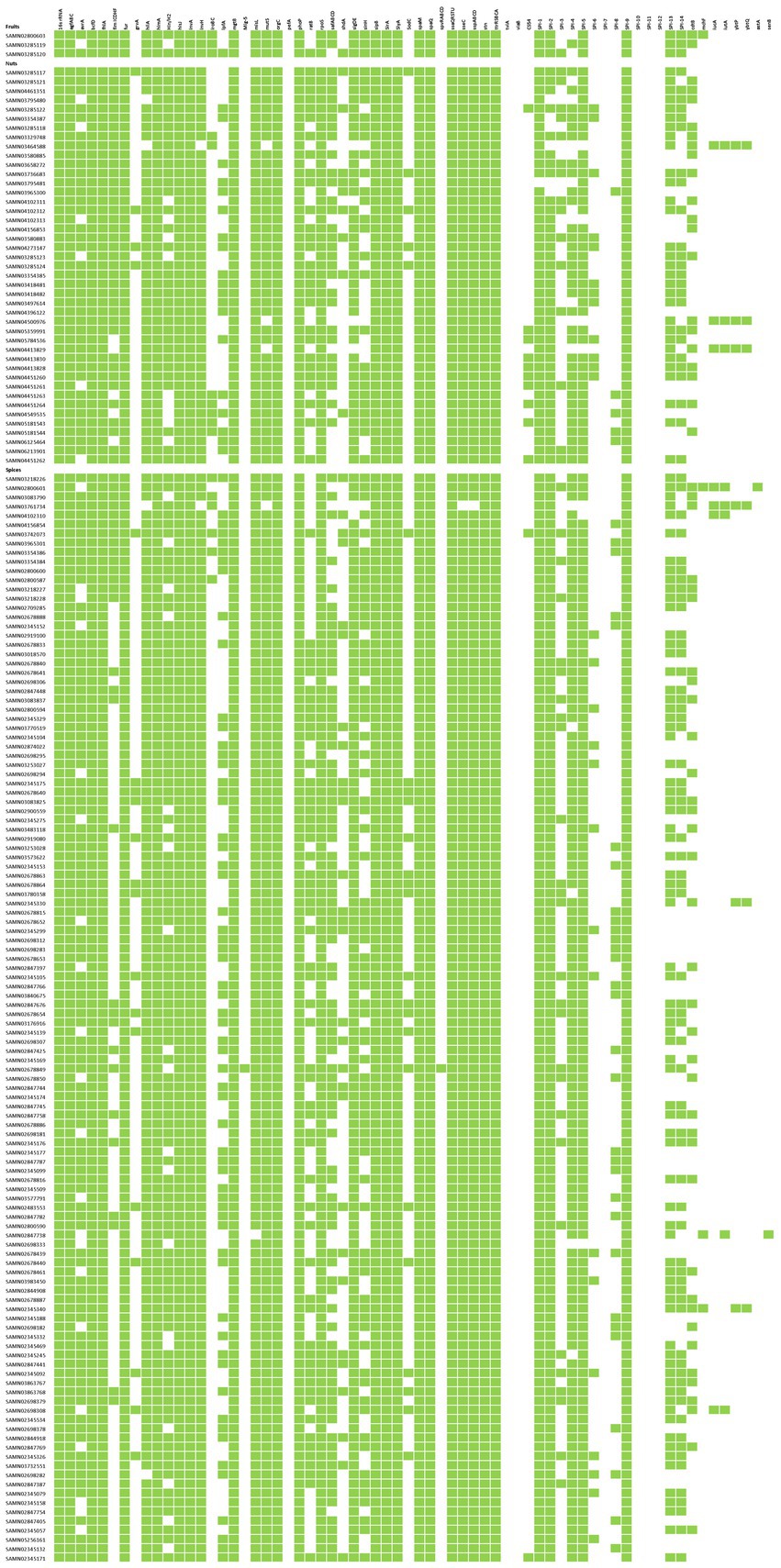
Figure 6. Prevalence of stress genes among the 192 isolates investigated. (A) Stress genes of non-O157 Shiga toxin-producing E. coli; (B) stress gees of Listeria monocytogenes; (C) stress genes of Salmonella; and (D) scatterplot matrix of the stress genes of Salmonella.
In the case of the L. monocytogenes isolates studied (Figure 6B), stress genes bcrB, bcrC, and cadC were identified. Among these, four isolates sourced from iceberg lettuce and mango (SAMN03760076, SAMN02211847, SAMN04325075, and SAMN04325057) carried both bcrB and bcrC genes that are resistant to biocide environment (bacitracin). Furthermore, one isolate (SAMN02850684) obtained from organic broccoli sprouts exhibited high tolerance to cadmium (Cd) conditions due to the presence of the cadC gene. No stress genes were detected in the remaining L. monocytogenes isolates studied.
In the examination of stress genes among Salmonella isolates (Figure 6C), metal resistance genes were observed most frequently (80.29%, 656 out of all 817 presented genes), with 157 isolates carrying these metal resistance genes among the 164 Salmonella isolates studied. Following closely were acid resistance genes (18.85%, 154/817) found in 154 out of 164 isolates and biocide resistance genes (0.86%, 7/817) identified in 7 out of 164 isolates. Salmonella isolates from fruit samples were found to carry stress genes that are resistant to acid (asr gene) and metals (arsenic, arsA/B/C/D/H/R gene; copper/gold, golS, and golT gene). Isolates sourced from nut samples that possessed the asr, golS, golT, pcoA/B/C/D/E/R/S, silA/B/C/F/P/R/S, and silE genes exhibited high tolerance to acid, copper, gold, and silver environments. Similarly, isolates obtained from spice samples and carrying genes such as asr, arsA/B/C/D/H/R, golS, golT, pcoA/B/C/D/E/R/S, silA/B/C/F/P/R/S, and silE were highly resistant to acid, arsenic, copper, gold, mercury, and silver (Figure 6D).
3.4. Prevalence and diversity of virulence factors
3.4.1. Non-O157 STEC
Virulence genes (aat, adk, fimH, fumC, gyrB, icd, mdh, ompT, purA, recA, sfa/foc, stx1, stx2, and uidA) were widely observed in the non-O157 STEC isolates obtained from all Romaine lettuce and spinach samples (Figure 6B). There was only one isolate (SAMN04273146) from organic Romaine lettuce that lacked the uidA gene, while the remaining seven isolates all carried the uidA gene. The ehly1 gene only occurred in one isolate (SAMN03218212) from organic Romaine lettuce. Although all eight STEC isolates in this study harbored both stx1 and stx2 genes, none of these stx-positive isolates from leafy green sources exhibited the presence of eae and wyz genes.
3.4.2. Listeria monocytogenes
The majority of the L. monocytogenes isolates examined were found to possess 30 out of the 32 selected virulence factors as shown in Figure 2. They exhibited variability in the presence of LIPI-3 and LIPI-4, with the exception of isolate SAMN01939127 sourced from spinach in Clade C, which lacked ctsR, dnaK, dnaJ, hrcA, htrA, inlA, inlB, opuC, and LIPI-1. All three isolates in Clade A carried 31 out of the 32 factors except for LIPI-4. In Clade B, all isolates excluded LIPI-3 and LIPI-4, except for isolate SAMN01939120, sourced from spinach, which possessed 31 virulence factors but lacked LIPI-4. Within the group of nine isolates classified under Clade C, four of them (SAMN01816208, SAMN02569957, SAMN01939126, and SAMN01939116), all sourced from Romaine lettuce, carried all 32 virulence factors. On the other hand, four isolates (SAMN01939115, SAMN01939125, SAMN02211849, and SAMN01816207) possessed 31 virulence factors, with the exception of LIPI-4.
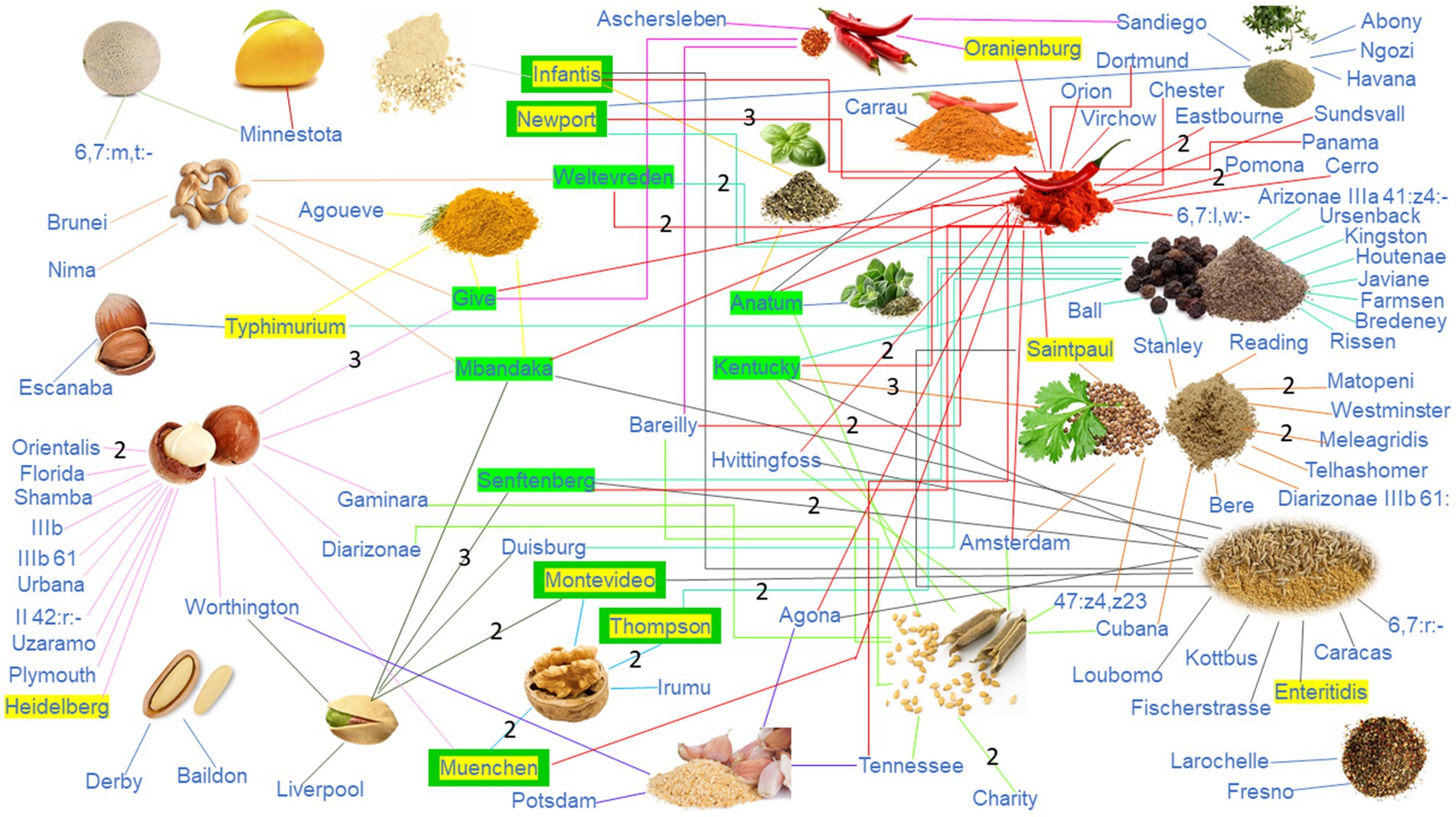
Figure 2. Phylogenetic analysis and the presence/absence of virulence factors of Listeria monocytogenes.
3.4.3. Salmonella
Figure 3 illustrates that all 164 Salmonella isolates contained the genes/gene clusters 16sRNA, agfABC, bcfD, flnA, fur, himA, hisJ, invA, mgtB, misL, orgC, phoP, rpoS, sipB, sirA, slyA, spaM, spaQ, stn, ttrSBCA, and ssaQRSTU. In particular, none of the isolates contained the mig-5, prefA, tviA, and viaB genes. There was only one Salmonella Enteritidis (SAMN02678849) isolated from ground cumin from Pakistan that contained spvRABCD, and one Salmonella Diarizonae (SAMN03761734) isolated from coriander from the United Kingdom lacked both sseC and sspABCD. Meanwhile, two isolates (SAMN03464588, macadamia nuts, Salmonella IIIb; and SAMN03761734, coriander, Salmonella Diarizonae) lacked the invH gene, while four isolates (SAMN03761734, SAMN03464588, SAMN04500976, macadamia nuts, Salmonella IIIb; and SAMN04413829, macadamia nuts, Salmonella Diarizonae) lacked the mutS gene. Furthermore, four isolates (SAMN03761734, SAMN03464588, SAMN03795480, and SAMN02698282) lacked the hilA gene, and another four isolates (SAMN03464588, SAMN004500976, SAMN04413829, and SAMN03761743) did not possess the sigDA and sioH genes. Two isolates (SAMN04102311, macadamia nuts, Salmonella Uzaramo; SAMN04102310, coriander, Salmonella Telhashomer) were missing the sigDA gene, and another two isolates (SAMN0284738, sesame seed, Salmonella Amsterdam; and SAMN04102313, macadamia nuts, Salmonella II 42:r:-) lacked the sioH gene. Additionally, a total of 28 isolates did not contain the avrA and hin genes, while 20 isolates carried the rva gene, and 25 isolates possessed the sodC gene. In terms of the SPI 1–14 investigated, all isolates presented with SPI-9 and lacked SPI-7, SPI-8, SPI-10, SPI-11, and SPI-12. However, one isolate obtained from nuts (SAMN03795481, macadamia nuts, Salmonella Gaminara) was missing SPI-1, and six isolates sourced from nuts lacked SPI-2, while seven isolates from nuts and spices were missing SPI-5. The number of isolates missing SPI-3, SPI-4, SPI-6, SPI-13, and SPI-14 were 121, 17, 137, 56, and 73 out of 164, respectively, with these absences occurring randomly across all the samples.
3.5. Genetic diversity by phylogenetic analysis
3.5.1. Non-O157 STEC
In Figure 1, it can be observed that the non-O157 STEC isolate from Romaine lettuce (SAMN04273144) and from spinach (SAMN04273141), both collected from organic samples in CA, were genetically identical. In contrast, isolate SAMN04273143 from spinach, also collected in CA, exhibited significant genetic divergence from the other non-O157 STEC isolates studied.
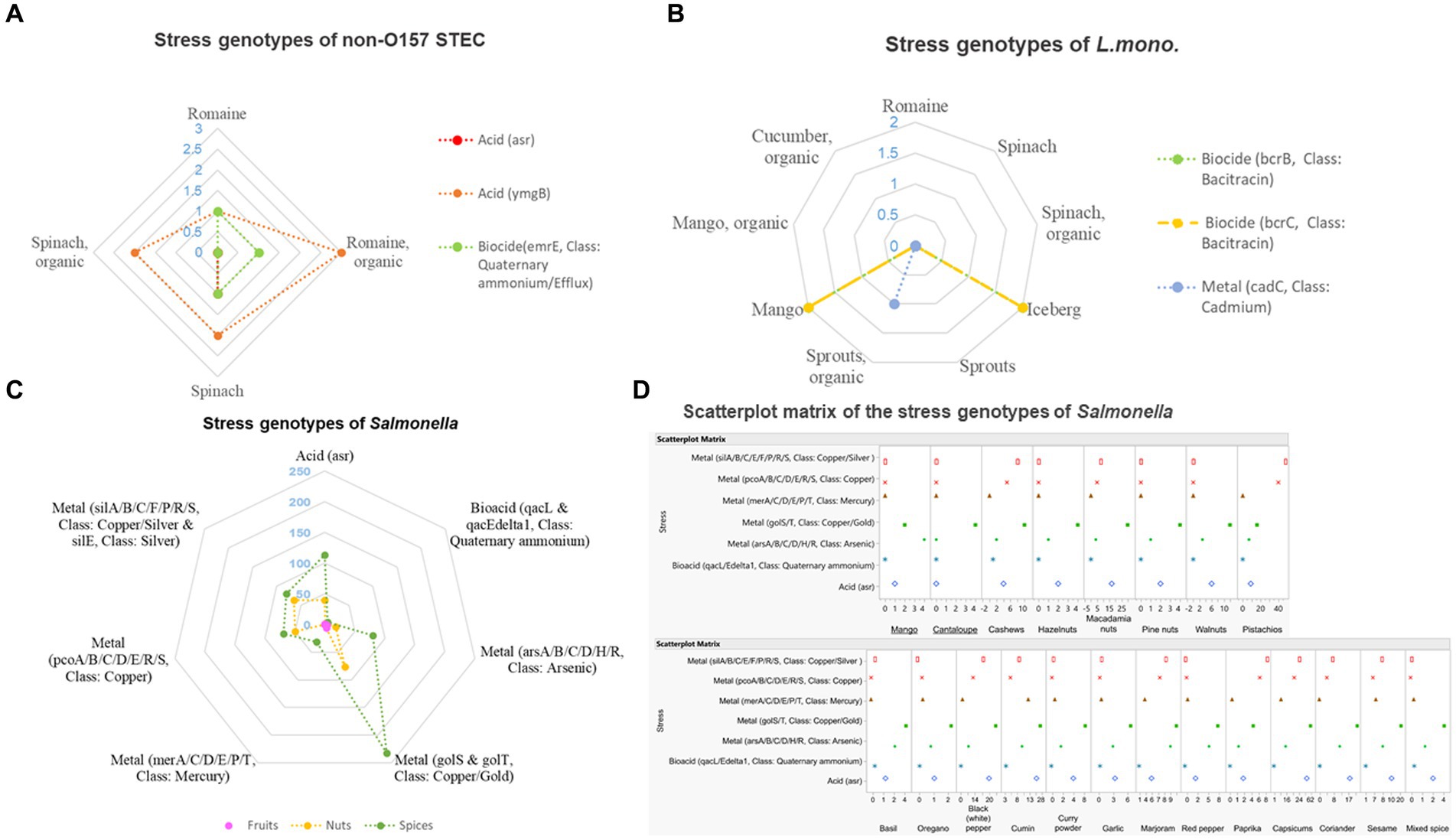
Figure 1. Phylogenetic analysis and the presence/absence of virulence factors of non-O157 Shiga toxin-producing E. coli isolates.
3.5.2. Listeria monocytogenes
The 20 L. monocytogenes isolates in our project (Figure 2) were divided into three Clades: A (3 isolates), B (8 isolates), and C (9 isolates). The majority of these isolates sourced from organic samples (3 of all 5 organic isolates) fell within Clade B, whereas all six isolates obtained from Romaine lettuce were grouped in Clade C. The two isolates recovered from mango (SAMN04325075, GA) and mango (SAMN04325057, GA) were identified as the same L. monocytogenes. In Clade B, the isolate from cucumber (SAMN02943415, FL) presented a far distance from others obtained from iceberg lettuce, broccoli sprouts, alfalfa sprouts, mango, and spinach. Conversely, the remaining five isolates in this clade were genetically similar. Among them, two isolates sourced from iceberg lettuce in different locations (SAMN03760076, CO and SAMN02211847, AZ) were found to be genetically identical based on WGS data. Similarly, two isolates obtained from alfalfa sprouts (SAMN02850685, FL) and organic broccoli sprouts (SAMN02850684, FL) were also genetically identical. In Clade C, it was observed that isolates sourced from organic spinach (SAMN01816207, CA) and Romaine lettuce (SAMN02211849, CA) belonged to the same strain. Similarly, three isolates sourced from spinach (SAMN01939127, CA) and Romaine lettuce (SAMN01939126, CA; SAMN01939116, NC; and SAMN02569957, CA) were genetically identical.
3.5.3. Salmonella
The 164 Salmonella isolates were classified into five distinct Clades, denoted as Clade A through Clade E (Figure 7). Clades A, B, and C each contained 16 isolates, while Clade D comprised 66 isolates, and Clade E contained 55 isolates. All three isolates originating from fruits were grouped into Clade B (cantaloupe) and Clade E (cantaloupe and mango). Salmonella isolates from nuts were predominantly distributed in Clade D and Clade E. Most isolates sourced from coriander were displayed in Clade D. Isolates sourced from capsicums in this study were distributed across all five Clades. Additionally, the finished genomes obtained from organic samples were observed in all Clades except Clade B.
In Figure 7, the following key findings were observed: (1) All five Salmonella Newport isolates sourced from capsicums, marjoram, and black pepper in this study were classified in Clade A. (2) The sole Salmonella Enteritidis among all isolates studied was shown in Clade B as well as all three Salmonella Bareilly sourced from red pepper and capsicums. Similarly, two Salmonella Stanley among all isolates studied were both located in Clade B, and these two isolates sourced from coriander (India, 2014) and black pepper (China: Hong Kong, 2013) were genetically related to an isolate (SAMN03285120, 6,7:m,t:-) obtained in 2014 from cantaloupe in Connecticut, United States. Furthermore, two Salmonella Hvittingfoss isolates from capsicums were identified as the same strain, and they were isolated from neighboring countries (SAMN02345329, Vietnam, 2012; SAMN03770519, Thailand, 2015) in different years. (3) Clade C included Salmonella serotypes Escanaba, Irumu, Potsdam, Anatum, Thompson, 6,7:r:-, Heidelberg, Saintpaul, and Typhimurium. Salmonella Anatum isolates from sesame seed (India), basil (Egypt), capsicums (Mexico), oregano (Mexico), and paprika (Canada), and three Salmonella Typhimurium isolates from black pepper (Belgium), hazelnuts (USA:GA), and curry powder (United Kingdom) were grouped in Clade C. (4) Serotypes Senftenberg (7 isolates), Kentucky (7 isolates), Mbandaka (6 isolates), Weltevreden (5 isolates), Amsterdam (3 isolates), Agona (3 isolates), and Tenseness (3 isolates) obtained in this project were only observed in Clade D (Figure 7), and some isolates belonging to serotypes, such as Worthington, Diarizonae, Cubana, Fresno, Rissen, Meleagridis, were also seen in Clade D. Interestingly, we found that one Salmonella Senftenberg (SAMN02345177) sourced from cumin was genetically distant from the other six Salmonella Senftenberg isolates. (5) Clade E exhibited significant diversity, comprising 55 Salmonella isolates with 35 distinct serotypes. More than 50% of these 55 isolates were obtained from nuts, capsicums, and black pepper.
4. Discussion
Numerous foodborne pathogens, such as Salmonella, E. coli O157:H7, Campylobacter, L. monocytogenes, Shigella, and Staphylococcus, have their reservoirs in healthy food animals and spread to an increasing variety of foods worldwide, which give rise to the changing epidemiology of foodborne disease (Tauxe, 1997). This study considered the current landscape of surveillance and explores the potential utility of WGS in monitoring the prevalence of major foodborne pathogens, AMR, stress genes, and virulence factors associated with these pathogens. The research involved the analysis of a sufficiently large sample size that better represents the overall population. This approach not only minimizes the impact of outliers or extreme data points but also widens the scope of available data, providing a more comprehensive understanding of the genetic profiles of these pathogens.
4.1. Distribution features of the foodborne pathogens investigated
Among the 192 isolates recovered from a total of 31,322 food samples in the United States and entry into the United States, Salmonella emerged as the most frequent foodborne bacteria and accounted for 85.42% of the total isolates obtained, compared to other pathogens investigated (L. monocytogenes and non-O157 STEC), which aligns with the previous official reports (CDC, 2017). The Foodborne Disease Outbreak Surveillance System (CDC, 2013) discovered that the majority of Salmonella-related outbreaks in the United States during 1998–2008 were primarily linked to four serotypes: Enteritidis, Typhimurium, Newport, and Heidelberg. Our study revealed a high occurrence of Salmonella serotypes Give, Kentucky, Senftenberg, Mbandaka, Anatum, Newport, and Weltevreden among the investigated samples, while Salmonella serotypes Newport, Montevideo, Thompson, and Muenchen were the serotypes listed on the top 20 Salmonella serotypes leading to human infections on FoodNet list (CDC, 2017), which implied that the landscape of Salmonella serotypes causing outbreaks may undergo changes in the near future. This could be attributed to the genetic diversity and prevalence dynamics of dominant pathogen types in food sources.
4.2. AMR and MDR landscape of the foodborne pathogens investigated
In recent decades, the increasing levels of AMR in foodborne pathogens have raised significant concerns, as treatment effectiveness can be compromised when bacteria are resistant to prescribed agents and antibiotics. Consequently, AMR genes are now regarded as emerging pollutants, and the prevalence of AMR among enteric organisms in food animals varies across different countries (de Jong et al., 2009). WGS has significantly facilitated the detection of resistant microorganisms and the identification of AMR determinants (and their genomic background) within surveillance schemes (Punina et al., 2015). In this study, we provided a glimpse of the diversity of AMR genes in non-O157 STEC, Salmonella, and L. monocytogenes in various food sources. Our findings revealed that all eight non-O157 STEC isolates contained the AMR gene blaEC conferring resistance to cephalosporin, as well as multiple drug resistance genes acrF and mdtM. Among the 20 L. monocytogenes isolates studied, all of them carried resistance genes for fosfomycin and lincosamide (fosX and lin). However, the occurrence and distribution of AMR genes in Salmonella isolates were highly diverse, and these isolates displayed a high frequency of multidrug efflux transporter, followed by AMR genes resistant to aminoglycoside (ant/aac/aph gene), fosfomycin (fos gene), sulfonamide (sul gene), and tetracycline (tet gene). Thus, from an AMR perspective, Salmonella showed a higher level of pollution, posing potentially greater risks to the ecosystem and human health than non-O157 STEC and L. monocytogenes isolates in this study. By comparing different Salmonella enterica serovars from dairy cattle and humans by WGS, Carroll et al. (2017) discovered 42 different groups of AMR genes in 90 Salmonella genomes. The most common genes belonged to groups associated with resistance to penicillins [penicillin-binding protein (PBP) gene], aminoglycosides [aac(6)-Iaa, strA, and strB], phenicols (floR), tetracyclins [tet(A) and tet(R)], cephalosporins (CMY), and sulphonamides (sul2) (Carroll et al., 2017). Using WGS, Wilson et al. (2018) investigated the AMR profiles of 100 L. monocytogenes isolates from dairy, meat, vegetables, food, seafood, and dairy farm environment samples in Australian food production chains between 1988 and 2016, all isolates were found to harbor the fosfomycin resistance gene (fosX) and the lincomycin resistance gene (lmrB), and no tetracycline [tet(A), tet(K), tet(L), tet(M), and tet(S)], trimethoprim (dfrD and dfrG), or vancomycin (vanA and vanB) resistance-associated genes (Wilson et al., 2018).
In this study, isolates sourced from capsicums, cumin, and black (white) pepper carried an abundance of AMR genes, in comparison with isolates from other matrices, which suggested a potential interaction between spices and conventional antibiotics. However, there is a limited body of research currently addressing the mechanisms/relationships between spices and traditional antibiotics. Moore et al. (2019) conducted a study involving 27 spice varieties sampled from retail sales in Al Ain and Dubai. They examined these spices with four antibiotics and 15 bacterial pathogens, and the in vitro study showed that when a combination of 27 spices was introduced at a low concentration (circa 0.02 percent [w/v]; 200 ppm), antibiotic susceptibility increased with four major classes of antibiotic: β-lactams (amoxicillin, piperacillin/tazobactam), macrolides (erythromycin), polymyxins (colistin), and tetracyclines (doxycycline) (Moore et al., 2019). Another study also proved the positive interaction between spices and conventional antibiotics in the observation of five isolates of Mycobacterium abscessus (5/10; 50%) that failed to grow on the spice-enriched medium, which included four clinical isolates and the National Culture Type Collection (NCTC) Reference Strain, and both the inhibition zones of amikacin and linezolid were increased with the inclusion of the spices (Moore et al., 2018). The higher abundance of AMR genes in spices compared to other food matrices can be attributed to several factors (Forsberg et al., 2012; Manaia, 2017; Oniciuc et al., 2018); for example, spices are often procured from diverse regions characterized by varying agricultural practices and hygiene standards. Factors such as contaminated water, soil, or improper handling throughout the cultivation, harvesting, processing, and packaging phases can introduce AMR bacteria and genes into spices. Furthermore, as spices are frequently traded on a global scale, there is an increased potential for contamination with AMR bacteria from different sources and regions. Cross-border trade can facilitate the dissemination of AMR genes. Additionally, spices come into contact with natural microbial communities in their environment, including soil and water, where AMR genes may naturally exist. These genes have the potential to transfer to bacteria that colonize spices. Therefore, given the global burden of AMR and the significant consumption of spices in human diets, and the fact that few studies have focused on the interaction between spices and conventional antibiotics, it is crucial to explore the food safety aspects of spice consumption and elucidate the specific mechanisms behind why isolates from certain spices harbor a higher abundance of AMR genes.
Furthermore, the observed frequency (3.65%) of MDR Salmonella isolates in this study was lower than in some previous reports, where the MDR rates of Salmonella isolates were reported as: 47.4% (129/272) in retail chicken in 2009 and 50.0% (10/20) in retail pork in 2010 in the United States (FDA, 2023); 68.6% (35/51) in raw food samples of pork, chicken carcasses, shell eggs, and ready-to-eat foods (sausages, ham, salami, and fresh chicken meat samples) in Romania between 2016 and 2018 (Tîrziu et al., 2020); 73.44% in pig farms in Spain from 2012 to 2014 (Cameron-Veas et al., 2018); and 43.34% (46/106) in fecal and environmental samples (from different animal hosts including cattle, sheep, goats, pigs, ducks, and chickens) in South Africa in 2018 (Mthembu et al., 2019), respectively. This discrepancy may be related to the strictly controlled use of antimicrobials in the production of vegetables, fruits, nuts, and spices plants. Moreover, the high MDR rates in animal or animal-related samples could be attributed to the heavy use of antimicrobials in infection treatment and animal growth promotion. For instance, cephalosporins and fluoroquinolones are primary antimicrobial treatment options for salmonellosis in animals, and β-lactams and fluoroquinolones are the most widely used classes of antibacterial agents for treating bacterial infections in animals, accounting for approximately 73% of the prescriptions (Folster et al., 2015; Bush and Bradford, 2016). The development of MDR in Salmonella has a noteworthy influence on antibiotic therapy against this pathogen. The presence of AMR, particularly MDR, in foodborne pathogens poses a significant global public health threat. High prevalence rates of AMR and MDR genes in Salmonella underscore the urgency of addressing this issue on a worldwide scale.
4.3. Stress characteristics analysis of the foodborne pathogens investigated
The recent emergence of outbreaks associated with acidic foods has brought attention to the acid tolerance of foodborne pathogens. Typically, bacteria enter the stationary phase in their natural environment, during which cells display pH-dependent acid tolerance, enhancing their resistance to acidity. Moreover, the ability of bacteria to survive in acidic foods can induce cross-protection against other environmental stresses encountered during food processing (Garren et al., 1998; Kim et al., 2015). In this study, all eight non-O157 STEC isolates exhibited genes related to acid resistance. Similarly, Salmonella isolates displayed resistance to acidic conditions, with 18.85% of the 817 resistance genes observed in the 154 Salmonella isolates investigated being associated with acid resistance. However, it is noteworthy that we did not identify any L. monocytogenes isolates carrying genes related to acid resistance. These results indicated that Salmonella and non-O157 STEC may possess a higher tolerance to acidified environments than L. monocytogenes, which is an incredibly significant discovery. As plenty of reports focused on the survival of E. coli O157:H7 under acidified environments encountered in various foods, stomach, and in vitro, investigators also reported strains of E. coli O157:H7 did not have superior acid resistance abilities compared to non-O157 STEC (Berry et al., 2004; Kim et al., 2015). Our results also underscored the importance of examining the acid resistance of non-O157 STEC, considering the lack of research on acid resistance of non-O157 STEC serogroups despite the globally high incidence of infections caused by these pathogens. Moreover, the notable prevalence of acid resistance among Salmonella isolates, as well as the high percentage of isolates exhibiting acid resistance in our study, raise concerns. This phenomenon might be explained by reports that Salmonella is skilled at adapting to, growing, and/or surviving within a wide pH range from 3.99 to 9.5 (D’Aoust and Maurer, 2007). Such adaptability can significantly impact the survival of Salmonella throughout various stages of food processing, preparation, and storage, as well as its passage through the host organisms.
Biocides play a critical role in controlling the spread of environmentally transmitted pathogens in healthcare and food-processing environments. Quaternary ammonium compounds are commonly used as disinfectants that can lead to the development of resistant organisms. They interact with the cytoplasmic membrane of bacteria and the plasma membrane of yeasts and also interact with intracellular targets and bind to DNA (Zinchenko et al., 2004; Gerba, 2015). In our study, 37.5% of all non-O157 STEC isolates were found to harbor stress genes resistant to biocide (Class: Quaternary ammonium), whereas Salmonella isolates showed a lower prevalence (0.86%) of quaternary ammonium-resistance genes. However, 20% of the 20 L. monocytogenes isolates investigated harbored bcrB and bcrC genes that are resistant to biocide environments (Class: Bacitracin). Previous research has also demonstrated the occurrence of L. monocytogenes resistance to quaternary ammonium compounds in farms, food manufacture, food transport, and food retail sites (Ortiz et al., 2014).
The ability to sense, respond, and adapt to metal environments is critical to the epidemiology and virulence of foodborne pathogens, but the survival of these pathogens in a host also depends upon their ability to populate many metalloproteins with the correct metal cofactors (Wojcicki et al., 2021). Our study observed one L. monocytogenes isolate from broccoli sprouts encoded the cadC gene, indicating its high tolerance to cadmium (Cd) conditions. None of the non-O157 STEC and L. monocytogenes isolates from other leafy greens/fruits were found to carry metal resistance genes. However, among the 164 Salmonella isolates investigated, 157 (95.7%) isolates were found to carry metal resistance genes. Salmonella isolates harboring stress genes from fruit samples were mostly resistant to arsenic and copper/gold; isolates sourced from nuts samples exhibited high tolerance to copper, gold, and silver; and isolates sourced from spices samples were highly resistant to arsenic, copper, gold, mercury, and silver. These results implied that the tolerance and resistance of pathogens to metals may be related to specific plant types, a phenomenon worthy of further investigation. Additionally, Salmonella may have a higher ability to accumulate metals than non-O157 STEC and L. monocytogenes, and comprehensive, well-designed experiments are needed to better understand the mechanisms underlying this phenomenon.
4.4. Virulence characteristics of the foodborne pathogens investigated
Isolates carrying stx1 and stx2 genes can produce Shiga toxins, which can lead to various health issues, such as diarrhea, hemorrhagic colitis, and hemolytic uremic syndrome (HUS) (Friedrich et al., 2007). It has been highlighted that the carriage of eae gene and the capacity of stx-positive strains have a strong relationship in causing human diseases, particularly HUS (Zahraei Salehi et al., 2007). Our analysis of WGS data revealed that 100% percentage of the STEC isolates (which were all non-O157 from leafy green sources) in this study carried both stx1 and stx2 genes, and 87.5% of these isolates carried uidA gene, but none of these isolates were positive for eae and wzy genes. Based on these data, we speculate that leafy greens may serve as a natural reservoir for STEC, albeit occasionally lacking some of the more virulent genes, such as eae and wzy. Therefore, these isolates do pose a risk to public health, but at a lower level compared with isolates having stx genes and other virulent genes (e.g., eae and wzy). Furthermore, it is worth noting that only one organic Romaine lettuce isolate contained the ehly1 gene, which codes for enterohemolysin, while the other seven isolates did not have this gene. As the ehly1 gene is located on a plasmid, we hypothesize that this particular isolate may have acquired the gene through horizontal gene transfer (HGT) from other organisms (Zahraei Salehi et al., 2007).
In our study, which analyzed 31,322 food samples (including leafy greens, sprouts, melons, cantaloupes, mangoes, cucumbers, tree nuts, and spices), we detected and isolated more L. monocytogenes (20 isolates) than STEC (8 isolates). In recent years, listeriosis outbreaks have occurred frequently, with nearly all sporadic and epidemic human cases linked to the consumption or use of contaminated foods, such as cheese, mushrooms, eggs, ice cream, frozen vegetables, raw milk, sprouts, cantaloupe, crustaceans, shellfish, mollusks, and stone fruits (Buchanan et al., 2017; CDC, 2019). Our study also revealed that 19 out of the 20 L. monocytogenes isolates carried 30 of the 32 virulence genes/clusters shown in Figure 2, including LIPI-1, a cluster of six genes (prfA, plcA, hly, mpl, actA, and plcB) crucial for the infection cycle of L. monocytogenes. A total of 86.67% of these 30 genes were located on the chromosome, indicating their conservation and reduced susceptibility to loss compared to factors found on plasmids. Interestingly, the presence or absence of LIPI-3 (a cluster of eight genes encoding listeriolysin S, a post-translationally modified cytolytic peptide) and LIPI-4 (a cluster of six genes encoding a sugar transport system involved in neural and placental infection) appeared to correspond with the Clades in the phylogenetic tree (Figure 2). LIPI-3 was detected in all isolates belonging to Clades A and C but was missing from all isolates in Clade B. LIPI-4 was absent from Clade A, seven out of eight isolates in Clade B, and four out of nine isolates in Clade C. These results suggest that LIPI-3 and LIPI-4 may be associated with specific genetic types of isolates, which may imply that some isolate groups are more virulent than others due to serotypes, other typings, or other genetic makeups. A previous study reported that all 33 L. monocytogenes isolates from the 2010–2015 multi-state ice cream-outbreak carried LIPI-1, but no other pathogenicity islands (LIP3 and LIP4), and outbreak isolates from stone fruit, taffy apples, and leafy green salad had LIPI-1 and LIPI-3 (Li et al., 2017). Further research is needed to explore these speculations in large-scale experiments with appropriate isolates.
The genomic data obtained through WGS revealed genetic similarities among some Salmonella serotypes from different countries and across various years. For example, Salmonella Weltevreden, isolated from capsicums in Bangladesh in 2013, exhibited nearly identical WGS profiles to an isolate from the same country in 2015. Salmonella Duisburg, obtained from organic pistachios in California in 2016, shared identical genomic characteristics with an isolate from black pepper in Washington in 2014. Furthermore, Salmonella Sandiego, isolated from marjoram in Mexico in 2013, and red pepper in Colorado in 2014, displayed genetic identity when analyzed using WGS. This phenomenon may be attributed to the growing intensity of domestic and global food product trade.
Moreover, all 164 Salmonella isolates in our study carried the genes/gene cluster 16sRNA, agfABC, bcfD, flnA, fur, himA, hisJ, invA, mgtB, misL, orgC, phoP, rpoS, sipB, sirA, slyA, spaM, spaQ, stn, ttrSBCA, ssaQRSTU, and SPI-9. Many of these isolates, spanning different serotypes and originating from various food categories, also carried various other virulence genes and pathogenicity islands. This underscores the inherent virulence of Salmonella in nature and its significant threat to public health, explaining why Salmonella is the leading cause of foodborne illnesses, hospitalizations, and deaths in the United States (CDC, 2022).
To sum up, our comprehensive study, which examined a large number and variety of food samples from different regions and countries, has uncovered high genetic diversity and genetic relationships among major foodborne pathogens (Salmonella, STEC, and L. monocytogenes), as well as unveiled the presence of AMR, virulence, and stress resistance genes/factors within these pathogens. These findings not only highlighted the inherent risks and challenges associated with these pathogens, but also signify the importance of the One Health Initiative, which is a collaborative, multisectoral, and transdisciplinary approach, recognizing the interconnection among animals, plants, people, and their shared environment, with the collaboration at the local, regional, national, and global levels, with the goal to achieve optimal health outcomes.5
Author’s note
Mention of trade names or commercial products in the study is solely for the purpose of providing scientific information and does not imply recommendation or endorsement by the U. S. Food and Drug Administration.
Data availability statement
The datasets presented in this study can be found in online repositories. The names of the repository/repositories and accession number(s) can be found in the article/Supplementary material.
Author contributions
LH: Conceptualization, Data curation, Formal analysis, Investigation, Methodology, Software, Writing – original draft, Writing – review & editing. EB: Writing – review & editing. GZ: Conceptualization, Investigation, Project administration, Supervision, Writing – original draft, Writing – review & editing.
Funding
The author(s) declare financial support was received for the research, authorship, and/or publication of this article. This study was supported by funding from the Center for Food Safety and Applied Nutrition, U.S. Food and Drug Administration.
Conflict of interest
The authors declare that the research was conducted in the absence of any commercial or financial relationships that could be construed as a potential conflict of interest.
Publisher’s note
All claims expressed in this article are solely those of the authors and do not necessarily represent those of their affiliated organizations, or those of the publisher, the editors and the reviewers. Any product that may be evaluated in this article, or claim that may be made by its manufacturer, is not guaranteed or endorsed by the publisher.
Supplementary material
The Supplementary material for this article can be found online at: https://www.frontiersin.org/articles/10.3389/fsufs.2023.1281005/full#supplementary-material
Footnotes
References
Alghoribi, M. F., Balkhy, H. H., Woodford, N., and Ellington, M. J. (2018). The role of whole genome sequencing in monitoring antimicrobial resistance: a biosafety and public health priority in the Arabian peninsula. J. Infect. Public Health 11, 784–787. doi: 10.1016/j.jiph.2018.08.001
Berry, E. D., Barkocy-Gallagher, G. A., and Siragusa, G. R. (2004). Stationary-phase acid resistance and injury of recent bovine Escerichia coli O157 and non-O157 biotype I Escherichia coli isolates. J. Food Prot. 67, 583–590. doi: 10.4315/0362-028x-67.3.583
Biswas, S., Niu, M., Pandey, P., Appuhamy, J., Leytem, A. B., Kebreab, E., et al. (2018). Effect of dairy manure storage conditions on the survival of E. coli O157:H7 and Listeria. J. Environ. Qual. 47, 185–189. doi: 10.2134/jeq2017.06.0224
Buchanan, R. L., Gorris, L. G. M., Hayman, M. M., Jackson, T. C., and Whiting, R. C. (2017). A review of Listeria monocytogenes: an update on outbreaks, virulence, dose-response, ecology, and risk assessments. Food Control 75, 1–13. doi: 10.1016/j.foodcont.2016.12.016
Burnett, S. L., Gehm, E. R., Weissinger, W. R., and Beuchat, L. R. (2000). Survival of Salmonella in peanut butter and peanut butter spread. J. Appl. Microbiol. 89, 472–477. doi: 10.1046/j.1365-2672.2000.01138.x
Burton, G. A. Jr., Gunnison, D., and Lanza, G. R. (1987). Survival of pathogenic bacteria in various freshwater sediments. Appl. Environ. Microbiol. 53, 633–638. doi: 10.1128/aem.53.4.633-638.1987
Bush, K., and Bradford, P. A. (2016). β-Lactams and β-lactamase inhibitors: an overview. Cold Spring Harb. Perspect. Med. 6:a025247. doi: 10.1101/cshperspect.a025247
Cameron-Veas, K., Fraile, L., Napp, S., Garrido, V., Grillo, M. J., and Migura-Garcia, L. (2018). Multidrug resistant Salmonella enterica isolated from conventional pig farms using antimicrobial agents in preventative medicine programmes. Vet. J. 234, 36–42. doi: 10.1016/j.tvjl.2018.02.002
Carroll, L. M., Wiedmann, M., den Bakker, H., Siler, J., Warchocki, S., Kent, D., et al. (2017). Whole-genome sequencing of drug-resistant Salmonella enterica isolates from dairy cattle and humans in New York and Washington states reveals source and geographic associations. Appl. Environ. Microbiol. 83, e00140–e00117. doi: 10.1128/AEM.00140-17
CDC (2013). Surveillance for foodborne disease outbreaks-United States, 1998-2008. Surveillance summaries. Morb. Mortal. Wkly Rep. 62, 1–34.
CDC . Foodborne diseases active surveillance network (FoodNet): FoodNet 2015 surveillance report (final data) (2017). Available at: https://www.cdc.gov/foodnet/pdfs/FoodNet-Annual-Report-2015-508c.pdf (Accessed Feburary 13, 2022).
CDC . Antibiotic resistance threats in the United States (2019). Available at: https://www.cdc.gov/drugresistance/pdf/threats-report/2019-ar-threats-report-508.pdf (Accessed October 11, 2022).
CDC . Foodborne germs and illnesses (2022). Available at: https://www.cdc.gov/foodsafety/foodborne-germs.html (Accessed January 18, 2023).
Chang, H. H., Cohen, T., Grad, Y. H., Hanage, W. P., O'Brien, T. F., and Lipsitch, M. (2015). Origin and proliferation of multiple-drug resistance in bacterial pathogens. Microbiol. Mol. Biol. Rev. 79, 101–116. doi: 10.1128/mmbr.00039-14
D’Aoust, J.-Y., and Maurer, J. (2007). “Salmonella species” in the Food microbiology: Fundamentals and Frontiers. eds. M. P. Doyle and L. R. Beuchat 3rd Edn (Washington, D.C.: ASM Press), 187–236.
Davis, S., Pettengill, J. B., Luo, Y., Payne, J., Shpuntoff, A., Rand, H., et al. (2015). CFSAN SNP pipeline: an automated method for constructing SNP matrices from next-generation sequence data. Peer J. Comput. Sci. 1:e20. doi: 10.7717/peerj-cs.20
de Jong, A., Bywater, R., Butty, P., Deroover, E., Godinho, K., Klein, U., et al. (2009). A pan-European survey of antimicrobial susceptibility towards human-use antimicrobial drugs among zoonotic and commensal enteric bacteria isolated from healthy food-producing animals. J. Antimicrob. Chemother. 63, 733–744. doi: 10.1093/jac/dkp012
FDA . NARMS Now: Integrated data. Multidrug Resistance (2023). Available at: https://www.fda.gov/animal-veterinary/national-antimicrobial-resistance-monitoring-system/narms-now-integrated-data (Accessed January 18, 2023).
Feldgarden, M., Brover, V., Gonzalez-Escalona, N., Frye, J. G., Haendiges, J., Haft, D. H., et al. (2021). AMRFinderPlus and the reference gene catalog facilitate examination of the genomic links among antimicrobial resistance, stress response, and virulence. Sci. Rep. 11:12728. doi: 10.1038/s41598-021-91456-0
Folster, J. P., Campbell, D., Grass, J., Brown, A. C., Bicknese, A., Tolar, B., et al. (2015). Identification and characterization of multidrug-resistant Salmonella enterica serotype Albert isolates in the United States. Antimicrob. Agents Chemother. 59, 2774–2779. doi: 10.1128/aac.05183-14
Forsberg, K. J., Reyes, A., Wang, B., Selleck, E. M., Sommer, M. O., and Dantas, G. (2012). The shared antibiotic resistome of soil bacteria and human pathogens. Science 337, 1107–1111. doi: 10.1126/science.1220761
Franz, E., Delaquis, P., Morabito, S., Beutin, L., Gobius, K., Rasko, D. A., et al. (2014). Exploiting the explosion of information associated with whole genome sequencing to tackle Shiga toxin-producing Escherichia coli (STEC) in global food production systems. Int. J. Food Microbiol. 187, 57–72. doi: 10.1016/j.ijfoodmicro.2014.07.002
Friedrich, A. W., Zhang, W., Bielaszewska, M., Mellmann, A., Köck, R., Fruth, A., et al. (2007). Prevalence, virulence profiles, and clinical significance of Shiga toxin-negative variants of enterohemorrhagic Escherichia coli O157 infection in humans. Clin. Infect. Dis. 45, 39–45. doi: 10.1086/518573
Garren, D. M., Harrison, M. A., and Russell, S. M. (1998). Acid tolerance and acid shock response of Escherichia coli O157:H7 and non-O157:H7 isolates provide cross protection to sodium lactate and sodium chloride. J. Food Prot. 61, 158–161. doi: 10.4315/0362-028x-61.2.158
Gerba, C. P. (2015). Quaternary ammonium biocides: efficacy in application. Appl. Environ. Microbiol. 81, 464–469. doi: 10.1128/AEM.02633-14
Gibson, L. F., and Khoury, J. T. (1986). Storage and survival of bacteria by ultra-freeze. Lett. Appl. Microbiol. 3, 127–129. doi: 10.1111/j.1472-765X.1986.tb01565.x
Grimstrup Joensen, K., Scheutz, F., Lund, O., Hasman, H., Kaas, R., Nielsen, E., et al. (2014). Evaluation of real-time WGS for routine typing, surveillance and outbreak detection of verotoxigenic Escherichia coli. J. Clin. Microbiol. 52, 1501–1510. doi: 10.1128/JCM.03617-13
Gygli, S. M. K. P., Ballif, M., Blöchliger, N. H. R., Reinhard, M., Loiseau, C., Ritter, C., et al. (2019). Whole-genome sequencing for drug resistance profile prediction in Mycobacterium tuberculosis. Antimicrob. Agents Chemother. 63, e02175–e02118. doi: 10.1128/AAC.02175-18
Hirai, Y. (1991). Survival of bacteria under dry conditions; from a viewpoint of nosocomial infection. J. Hosp. Infect. 19, 191–200. doi: 10.1016/0195-6701(91)90223-U
Jones, K. E., Patel, N. G., Levy, M. A., Storeygard, A., Balk, D., Gittleman, J. L., et al. (2008). Global trends in emerging infectious diseases. Nature 451, 990–993. doi: 10.1038/nature06536
Juven, B. J., Cox, N. A., Bailey, J. S., Thomson, J. E., Charles, O. W., and Shutze, J. V. (1984). Survival of Salmonella in dry food and feed. J. Food Prot. 47, 445–448. doi: 10.4315/0362-028X-47.6.445
Kim, G. H., Breidt, F., Fratamico, P., and Oh, D. H. (2015). Acid resistance and molecular characterization of Escherichia coli O157:H7 and different non-O157 Shiga toxin-Producing E. coli serogroups. J. Food Sci. 80, M2257–M2264. doi: 10.1111/1750-3841.12996
Komora, N., Bruschi, C., Magalhães, R., Ferreira, V., and Teixeira, P. (2017). Survival of Listeria monocytogenes with different antibiotic resistance patterns to food-associated stresses. Int. J. Food Microbiol. 245, 79–87. doi: 10.1016/j.ijfoodmicro.2017.01.013
Köser, C. U., Ellington, M. J., Cartwright, E. J., Gillespie, S. H., Brown, N. M., Farrington, M., et al. (2012). Routine use of microbial whole genome sequencing in diagnostic and public health microbiology. PLoS Pathog. 8:e1002824. doi: 10.1371/journal.ppat.1002824
Kreft, J., and Vazquez-Boland, J. A. (2001). Regulation of virulence genes in Listeria. Int. J. Med. Microbiol. 291, 145–157. doi: 10.1078/1438-4221-00111
Laabei, M., Recker, M., Rudkin, J. K., Aldeljawi, M., Gulay, Z., Sloan, T. J., et al. (2014). Predicting the virulence of MRSA from its genome sequence. Genome Res. 24, 839–849. doi: 10.1101/gr.165415.113
Li, Z., Perez-Osorio, A., Wang, Y., Eckmann, K., Glover, W. A., Allard, M. W., et al. (2017). Whole genome sequencing analyses of Listeria monocytogenes that persisted in a milkshake machine for a year and caused illnesses in Washington state. BMC Microbiol. 17:134. doi: 10.1186/s12866-017-1043-1
Lin, J., Lee, I. S., Frey, J., Slonczewski, J. L., and Foster, J. W. (1995). Comparative analysis of extreme acid survival in Salmonella typhimurium, Shigella flexneri, and Escherichia coli. J. Bacteriol. 177, 4097–4104. doi: 10.1128/jb.177.14.4097-4104.1995
Lomonaco, S., Chen, Y., and Knabel, S. J. (2008). Analysis of additional virulence genes and virulence gene regions in Listeria monocytogenes confirms the epidemiologic relevance of multi-virulence-locus sequence typing. J. Food Prot. 71, 2559–2566. doi: 10.4315/0362-028x-71.12.2559
Manaia, C. M. (2017). Assessing the risk of antibiotic resistance transmission from the environment to humans: non-direct proportionality between abundance and risk. Trends Microbiol. 25, 173–181. doi: 10.1016/j.tim.2016.11.014
Massot, M., Daubie, A. S., Clermont, O., Jaureguy, F., Couffignal, C., Dahbi, G., et al. (2016). Phylogenetic, virulence and antibiotic resistance characteristics of commensal strain populations of Escherichia coli from community subjects in the Paris area in 2010 and evolution over 30 years. Microbiology 162, 642–650. doi: 10.1099/mic.0.000242
Moore, R. E., Millar, B. C., Panickar, J. R., and Moore, J. E. (2018). Interaction of south Asian spices with conventional antibiotics: implications for antimicrobial resistance for mycobacterium abscessus and cystic fibrosis. Int. J. Mycobacteriol. 7, 257–260. doi: 10.4103/ijmy.ijmy_72_18
Moore, R. E., Millar, B. C., Panickar, J. R., and Moore, J. E. (2019). Microbiological safety of spices and their interaction with antibiotics: implications for antimicrobial resistance and their role as potential antibiotic adjuncts. Food Qual. Saf. 3, 93–97. doi: 10.1093/fqsafe/fyz008
Mthembu, T. P., Zishiri, O. T., and El Zowalaty, M. E. (2019). Molecular detection of multidrug-resistant Salmonella isolated from livestock production systems in South Africa. Infect. Drug Resist. 12, 3537–3548. doi: 10.2147/IDR.S211618
Nijhuis, R. H. T., Oueslati, S., Zhou, K., Bosboom, R. W., Rossen, J. W. A., and Naas, T. (2015). OXY-2-15, a novel variant showing increased ceftazidime hydrolytic activity. J. Antimicrob. Chemother. 70, 1429–1433. doi: 10.1093/jac/dkv002
Oniciuc, E. A., Likotrafiti, E., Alvarez-Molina, A., Prieto, M., Santos, J. A., and Alvarez-Ordóñez, A. (2018). The present and future of whole genome sequencing (WGS) and whole metagenome sequencing (WMS) for surveillance of antimicrobial resistant microorganisms and antimicrobial resistance genes across the food chain. Genes 9:268. doi: 10.3390/genes9050268
Ortiz, S., Lopez, V., and Martinez-Suarez, J. V. (2014). Control of Listeria monocytogenes contamination in an Iberian pork processing plant and selection of benzalkonium chloride-resistant strains. Food Microbiol. 39, 81–88. doi: 10.1016/j.fm.2013.11.007
Punina, N. V., Makridakis, N. M., Remnev, M. A., and Topunov, A. F. (2015). Whole-genome sequencing targets drug-resistant bacterial infections. Hum. Genomics 9:19. doi: 10.1186/s40246-015-0037-z
Scallan, E., Hoekstra, R. M., Angulo, F. J., Tauxe, R. V., Widdowson, M. A., Roy, S. L., et al. (2011). Foodborne illness acquired in the United States--major pathogens. Emerg. Infect. Dis. 17, 7–15. doi: 10.3201/eid1701.p11101
Sorrells, K. M., Enigl, D. C., and Hatfield, J. R. (1989). Effect of pH, acidulant, time, and temperature on the growth and survival of Listeria monocytogenes. J. Food Prot. 52, 571–573. doi: 10.4315/0362-028X-52.8.571
Syamaladevi, R. M., Tang, J., Villa-Rojas, R., Sablani, S., Carter, B., and Campbell, G. (2016). Influence of water activity on thermal resistance of microorganisms in low-moisture foods: a review. Compr. Rev. Food Sci. Food Saf. 15, 353–370. doi: 10.1111/1541-4337.12190
Tauxe, R. V. (1997). Emerging foodborne diseases: an evolving public health challenge. Emerg. Infect. Dis. 3, 425–434. doi: 10.3201/eid0304.970403
Tîrziu, E., Bărbălan, G., Morar, A., Herman, V., Cristina, R. T., and Imre, K. (2020). Occurrence and antimicrobial susceptibility profile of Salmonella spp. in raw and ready-to-eat foods and Campylobacter spp. in retail raw chicken meat in Transylvania, Romania. Foodborne Pathog. Dis. 17, 479–484. doi: 10.1089/fpd.2019.2738
Tsai, Y.-W., and Ingham, S. C. (1997). Survival of Escherichia coli O157: H7 and Salmonella spp. in acidic condiments. J. Food Prot. 60, 751–755. doi: 10.4315/0362-028X-60.7.751
Varma, J., Greene, K., Ovitt, J., Barrett, T., Medalla, F., and Angulo, F. (2005). Hospitalization and antimicrobial resistance in Salmonella outbreaks, 1984–2002. Emerg. Infect. Dis. 11, 943–946. doi: 10.3201/eid1106.041231
WHO . Antimicrobial resistance: global report on surveillance 2014 (2014). Available at: https://www.who.int/drugresistance/documents/surveillancereport/en/ (Accessed Feburary 3, 2022).
WHO . WHO estimates of the global burden of foodborne diseases (2015). Available at: https://apps.who.int/iris/bitstream/handle/10665/199350/9789241565165_eng.pdf?sequence=1 (Accessed Feburary 6, 2022).
Wilson, A., Gray, J., Chandry, P. S., and Fox, E. M. (2018). Phenotypic and genotypic analysis of antimicrobial resistance among Listeria monocytogenes isolated from Australian food production chains. Genes 9:80. doi: 10.3390/genes9020080
Wojcicki, M., Swider, O., Daniluk, K. J., Srednicka, P., Akimowicz, M., Roszko, M. L., et al. (2021). Transcriptional regulation of the multiple resistance mechanisms in Salmonella-a review. PathoGenetics 10:801. doi: 10.3390/pathogens10070801
Xu, Y., Bai, X., Jin, Y., Hu, B., Wang, H., Sun, H., et al. (2017). High prevalence of virulence genes in specific genotypes of atypical enteropathogenic Escherichia coli. Front. Cell. Infect. Microbiol. 7:109. doi: 10.3389/fcimb.2017.00109
Yoon, S. H., Park, Y. K., and Kim, J. F. (2015). PAIDB v2.0: exploration and analysis of pathogenicity and resistance islands. Nucleic Acids Res. 43, D624–D630. doi: 10.1093/nar/gku985
Zahraei Salehi, T., Safarchi, A., Peighambari, S. M., Mahzounieh, M., and Rabbani Khorasgani, M. (2007). Detection of stx1, stx2, eae, espB and hlygenes in avian pathogenic Escherichia coli by multiplex polymerase chain reaction. J. Vet. Res. 62, 37–42.
Zhang, G., Chen, Y., Hu, L., Melka, D., Wang, H., Laasri, A., et al. (2018). Survey of foodborne pathogens, aerobic plate counts, total coliform counts, and Escherichia coli counts in leafy greens, sprouts, and melons marketed in the United States. J. Food Prot. 81, 400–411. doi: 10.4315/0362-028X.JFP-17-253
Zhang, G., Hu, L., Melka, D., Wang, H., Laasri, A., Brown, E. W., et al. (2017a). Prevalence of Salmonella in cashews, hazelnuts, macadamia nuts, pecans, pine nuts, and walnuts in the United States. J. Food Prot. 80, 459–466. doi: 10.4315/0362-028x.Jfp-16-396
Zhang, G., Hu, L., Pouillot, R., Tatavarthy, A., Doren, J. M. V., Kleinmeier, D., et al. (2017b). Prevalence of Salmonella in 11 spices offered for sale from retail establishments and in imported shipments offered for entry to the United States. J. Food Prot. 80, 1791–1805. doi: 10.4315/0362-028x.Jfp-17-072
Keywords: Salmonella , Listeria monocytogenes , Escherichia coli , WGS, food, AMR, stress, virulence
Citation: Hu L, Brown EW and Zhang G (2023) Diversity of antimicrobial resistance, stress resistance, and virulence factors of Salmonella, Shiga toxin-producing Escherichia coli, and Listeria monocytogenes from produce, spices, and tree nuts by whole genome sequencing. Front. Sustain. Food Syst. 7:1281005. doi: 10.3389/fsufs.2023.1281005
Edited by:
Eunice Ndegwa, Virginia State University, United StatesReviewed by:
Donald W. Schaffner, Rutgers, The State University of New Jersey, United StatesKebede Amenu, Addis Ababa University, Ethiopia
Copyright © 2023 Hu, Brown and Zhang. This is an open-access article distributed under the terms of the Creative Commons Attribution License (CC BY). The use, distribution or reproduction in other forums is permitted, provided the original author(s) and the copyright owner(s) are credited and that the original publication in this journal is cited, in accordance with accepted academic practice. No use, distribution or reproduction is permitted which does not comply with these terms.
*Correspondence: Guodong Zhang, R3VvZG9uZy5aaGFuZ0BmZGEuaGhzLmdvdg==