- 1School of Public Affairs, University of Science and Technology of China, Hefei, China
- 2The College of Humanities, Shandong Management University, Jinan, China
- 3School of Management, University of Science and Technology of China, Hefei, China
- 4Department of Economics and Statistics, University of Salerno, Fisciano, Italy
Food security is a matter of human existence and a top agenda item of United Nations’ sustainable development goals (SDGs); in the wake of the fact that 2.37 billion people are classified as either undernourished or unable to sustain regular intake of nutrient-dense diet, is a matter of prime importance across the globe. Our study investigated the zero-hunger reality through the prism of food security impacted by the consumption of fixed capital (Agriculture, Forestry and Fishing), environmental temperature surges, arable land, agricultural land, nitrogen and phosphate nutrient usage in the context of the most populous Asian countries. We employed the quantitative approach to investigate our problem by relying on the data collected from the FAOstat, World Bank, Economist Impact, and national statistics bureaus spanning 2016 to 2020 for six Asian countries such as China, India, Indonesia, Pakistan, Bangladesh, and Malaysia. This study’s findings explain that environmental temperature surges and arable land negatively affect food security, while fertilizers and fixed capital consumptions positively impact food security. Moreover, the study findings pave the path for policymakers and businesses to find the best approaches to enhancing food security, hygiene, quality, availability and wealth prosperity in these specific countries, which are half the world’s population, i.e., approximately 4 billion.
1 Introduction
The United Nations has made zero hunger one of its top two priorities among its sustainable development goals because food is essential to human existence (De Vries, 2021). The term “food security” garnered global attention during the 1996 World Food Summit, which was organized in response to the first global food crisis; where food security was defined as the access to reasonable, secure, and healthy food that fits the nutritional needs and is based on the choice of an individual or community at all times (Mc Carthy et al., 2018; Ghufran et al., 2024). In the last half-century, humans have made tremendous progress in satisfying the people’s food demand (Ingram, 2020), but still, 2 billion people lacked regular access to nutritious and healthy food in 2019, and 144 million children encountered undergrowth under the age of 5, and 44 million suffered from the food wastage.1 Similarly, according to the United Nations’ Sustainable Development Goals Report 2022, 1/10th of the people worldwide are hungry, and 1/3rd suffer from irregular food access.2 COVID-19 triggered these facts and worsened the situation, such as 811 million people worldwide faced hunger in 2020, up by 161 million from 2019. In 2020, about 2.37 billion people, a rise of 370 million from the previous year, did not have enough food to consume.3 Furthermore, the Ukraine and Russian crisis has strongly hit the emerging countries’ food supply and created food shortage problems: as per the United Nations 2022 report, Ukraine and Russia are contributing 30% wheat, 20% maize and 80% sunflower seed products in global exports.4 Many obstacles stand in the way of eradicating the food shortage problems. The United Nations 2017 and 2018 report versions demonstrated how armed conflict, climatic unpredictability and extremes impede initiatives to eliminate world hunger and food insecurity. Likewise, economic slowdowns and downturns in 2019 also impacted these initiatives adversely. In 2020, the COVID-19 pandemic and massive Desert Locust outbreaks in Eastern Africa were clouding economic prospects (Peng et al., 2020; Xu et al., 2021), which were not anticipated and were further augmented by the delayed public response.
It is worth noting that the food security debate has become more intense while considering the food safety concerns due to foodborne diseases, which are typically caused by the toxic chemical viruses, bacteria and parasites that enter into the human body through contaminated food (Schlundt et al., 2020; Potter, 2021). As stated in the World Health Organization’s 2022 report, more than 200 foodborne diseases, including diarrhea and cancer, impact all age groups, from newborns and young children to the elderly and the chronically ill (Gallo et al., 2020; Schlundt et al., 2020; Potter, 2021). Moreover, 1/10th, about 600 million people worldwide became sick, while 33 million healthy people died after consuming contaminated food. On an economic note, in low and middle-income countries, the public spending is around 110 billion United States dollars each year on medical expenses that emerge from foodborne diseases.5 Therefore, foodborne diseases impose a burden on healthcare systems and negatively impact national economies, tourism and commerce industries. It is vital to address food safety and security issues like food accessibility, sufficiency and hygiene, which are considered fundamental human rights that must be satisfied (Njage et al., 2017; Cadieux et al., 2019; Ghufran et al., 2022a, 2024). Food adequacy, availability, and accessibility are the building blocks of food security (Njage et al., 2017; Masniadi et al., 2020; Ghufran et al., 2024), which are not complete without food safety (Vipham et al., 2020). Standing on the foundation of these elements, nations form their food security apparatus; for instance, the government of Indonesia, regulation No. 17 of 2015, describes food security and safety as a state in a society related to the availability of food that is sufficient in quantity and quality, safe, evenly distributed, and affordable (Setiadi et al., 2022). Thus, this study empirically analyzes the realization of zero-hunger goals in the heavily populated Asian economies, where 418 million people are undernourished, which is approximately half of the world’s malnourished population (768 million) in 2020, while Africa hosts one-third (282 million), Latin America and the Caribbean houses the 60 million undernourished people, and 8 million are in remaining countries as per the FAO 2021 report.6
Previous literature explains food security in two sets of streams: one focusing on the risk, threat and uncertainty that rise because of climate change (resulting in earthquakes, floods, high temperatures, and drought; Tyczewska et al., 2018; Garcia et al., 2020; Hameed et al., 2020; Oskorouchi and Sousa-Poza, 2021; Ghufran et al., 2024), climate pollution (increase in CO2, methane, black carbon and ozone precursors) (Kinney, 2018; Ford et al., 2022), water pollution and soil pollution (dumping plastic and other harmful heavy metals and chemical into the water and soil) (Wu et al., 2017; Andrade et al., 2018; Garcia et al., 2020) which adversely affect the crops productivity and nutrient values and also provides the ideal conditions to harmful elements such as fungus, insects and weeds (Yang et al., 2016; Tyczewska et al., 2018). Second focus on productivity and food safety: such as Ali et al. (2019) explained that genetically modified (GM) seeds have shown high resilience in China and the United States during harsh environments and have provided a high yield (Raman, 2017; Aldemita and Hautea, 2018; Toma et al., 2018; Paarlberg et al., 2024) by consuming minimum natural resources like water, organic or bio-fertilizers, as compared to the traditional and organic food. Likewise, according to the World Health Organization, GM food is also secure and safe to consume which obtains national security and safety approval, and it can be a viable option to subside the food insecurity and safety problem of the entire world (Siegrist and Hartmann, 2020; Ali et al., 2021; Szenkovics et al., 2021; Mutegi et al., 2024; Paarlberg et al., 2024). In a detailed note (Ghufran et al., 2022b) explored that consumer behaviors in China and the United States are also shifting, and they are consuming GM food even if China is importing it from the United States because of the GM food quality and safety while having economic tug-of-war between the two (Ghufran et al., 2022a).
However, the prior researcher did not thoroughly investigate climate change based on the change in temperature, fixed capital stock spending on food security, land utilization and fertilizers for food security in populus Asian countries. For this purpose, we investigate the zero-hunger reality through the prism of food security (FS) impacted by the consumption of fixed Capital (Agriculture, Forestry and Fishing), environmental temperature surges, arable land, agricultural land, nitrogen (N) nutrients, and phosphate (P2O5) nutrient in the context of populous Asian countries.
2 Food security and sustainable development goals indicators
In 2012, the United Nations (UN) organized the conference in Rio de Janeiro, Brazil, on the SDGs, where 191 UN member countries agreed to tackle the world’s collective problems, such as poverty, zero hunger, good health and well-being, which resulted in the form of 17 goals and 196 sub-targets with the objective of their completion by 2030.7 Unfortunately, global calamities like COVID-19, climate change and an armed conflict between Ukraine and Russia seriously threaten the 2030 agenda of SDGs (Ben Hassen and El Bilali, 2022; Hellegers, 2022). As the COVID-19 pandemic enters its third year and while the global systemic recovery from it is underway, the crisis in Ukraine has exacerbated the already severe issues of food insecurity, humanitarian aid, refugees, and energy sustainability during global climate catastrophes like floods, drought and forest fire (Abay et al., 2022; Ben Hassen and El Bilali, 2022; Hellegers, 2022). The COVID-19 pandemic has devastated nearly every facet of our society within 2 years, and we have not even seen the end of it yet. More than 4 years of progress on poverty eradication were lost, and 93 million more people fell into the clutches of extreme poverty by 2020 due to the pandemic, which depleted people’s ability to access good quality food and clean water (see footnote 7, respectively). Essential health services also had unprecedented pressure during this time. As a result, the globe witnessed an increase in mortality from Tuberculosis (TB) and malaria and a decrease in vaccine coverage for the first time in the modern era. Although COVID-19 is a pressing issue (Ghufran et al., 2022b), but the looming danger of global warming has already eclipsed it (Duarte et al., 2020). Already, billions of people and ecosystems worldwide have been impacted by rising temperatures and extreme weather events (Duarte et al., 2020), like more frequent super floods in Pakistan, India, Bangladesh,8 and Malaysia,9 droughts and heat waves in Pakistan and India,10,11 Glacier bursts in Pakistan,12 and forest fires in India and Pakistan. Marine life is also in danger because of rising sea temperatures and ocean acidification (Duarte et al., 2020). On the other hand, the onset of conflict in Ukraine has driven up the cost of food, gasoline, and fertilizer, interrupted supply lines and international trade in all Asian economies, stressed the global financial system and augmented the concerns of a worldwide food shortage (Hellegers, 2022; Markus, 2022; Yeoman, 2022). Consequently, in this research article, we explore the impact of environmental temperature surges, arable land, agricultural land, fertilizer and consumption of fixed capital on food security in the context of populous Asian countries.
In the same vein, Figure 1 represents the overall food security situation from 2016 to 2020 (pre and post-COVID-19) in the populous six Asian countries, along with an average of 113 other countries denoted with a dashed line. Figures 1A–F have normalized on a scale of 0 to 100, where 0 represents the worst food security environment while 100 represents the most favorable environment. We grasp the most alarming fact of world food security, which scored between 50 and 65% slab before COVID-19; after that, it slightly tilts downward, indicating that the situation in developed and developing countries became impaired during the pandemic.
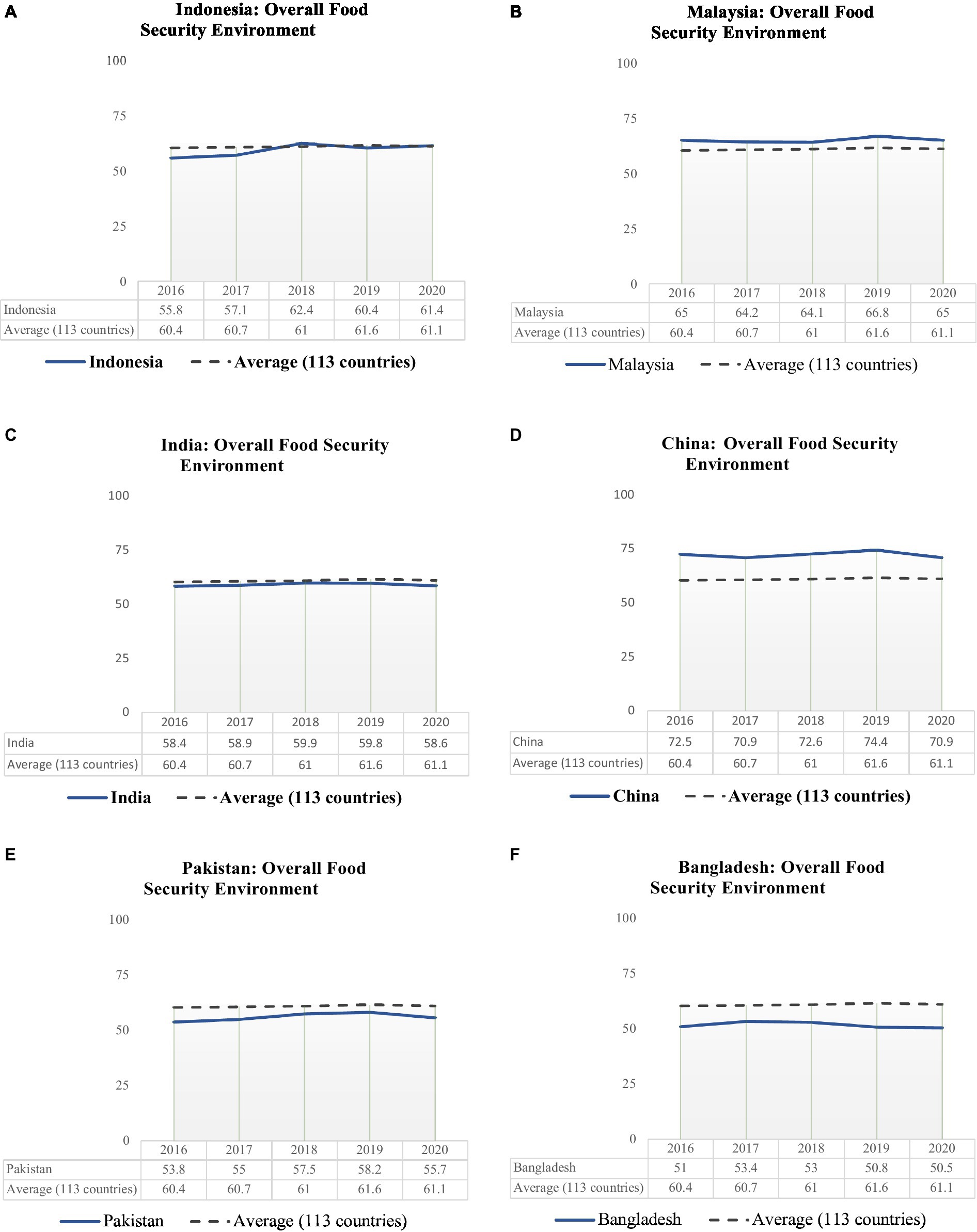
Figure 1. Asian countries’ overall food security environment situation in the world pre and post-COVID-19.
The situation in the six countries studied in this research is intense; for instance, in Figure 1F, Bangladesh was improving and moving toward the global average of 50, but after COVID-19, it again went down. The situation is similar in Pakistan (Figure 1E) and India (Figure 1C). Similarly, in Figure 1A, Indonesia was doing great and improving the overall food security condition, which was progress toward a 60 score, but after COVID-19, it was affected and declining but still better than before COVID-19. On the contrary, China (Figure 1D) and Malaysia (Figure 1B) were already above the average food security score but did not achieve the optimal point, and COVID-19’s devasting impact compelled them to move toward 50.
The current study emphasizes on the food security of 4 billion people (estimated by the United Nations) across six Asian countries, which account for roughly 56% of the total population of the globe and have a food security environment scoring below 50 or around 50. These distressing statistics lead us to the scenario in which we may identify why 418 million people are undernourished in Asia alone, which is half of the world’s population of malnourished people (768 million) in 2020.13 Thus, it is imperative to research the overall relationship between environmental temperature surges, arable land, agricultural land, fertilizer, consumption of fixed capital and food security in the context of populous Asian countries.
2.1 Food security and environmental temperature surges
Temperature is the fundamental component in describing the actual state of climate change in the entire world. Extreme temperature has far-reaching consequences for human and nonhuman life (Choufani et al., 2017; Watts et al., 2018). Since 1880, the average global temperature has risen by 0.14°F (0.08°C) each decade, which is twice the rise after 1981, 0.32°F (0.18°C) each decade. According to the National Oceanic and Atmospheric Administration Agency’s (NOAA) climate change data Wana et al., 2021 had become the sixth warmest year when the average surface temperature was 1.51°F (0.84°Celsius), the warmest in the twentieth century,14 posing severe food security and health risk, especially to those already susceptible to the effects of extreme heat (Choufani et al., 2017; Watts et al., 2018). Likewise, temperature increases lead to more extreme weather events, including droughts, storms, floods and typhoons, which deplete natural resources and threaten food security (including availability, safety, and sustainability; Godde et al., 2021). On a detailed note, global warming, including the increase in day and night temperature (Jha and Tripathi, 2017) and pollution, severely impacted and caused a 5.2% drop in the wheat crop from 1981 to 2009 in India (Gupta et al., 2017). Changes in weather patterns have also had an impact on Pakistan’s agricultural sector. In 2010–2014, Pakistan was hit by a rapid sequence of its four most enormous floods, which resulted in significant losses of cattle, agriculture, forest, and fishing, as well as the destruction of critical agricultural systems (Arora, 2019; Ahmad and Afzal, 2022). The severe flooding in 2022 resulted in an aggregate economic loss of 30 billion United States dollars, damaged an agricultural area equivalent to 1.2 million hectares alone in Sindh, 61% farming community exposed with transboundary animal disease in Baluchistan and 755,000 animals were lost in the flood in Pakistan.15 Similarly, Indonesia, China, Bangladesh, Malaysia are facing the temperature change severely which impact their food security.
2.2 Food security and arable and agricultural land
According to the Food and Agriculture Organization of the United Nations (FAO), arable land is used for short-term agricultural purposes, such as seasonal crops, grazing or home gardening. In contrast, agricultural land refers to long-term agricultural purposes, such as cultivating crops and meadows.16 Agriculture and arable land play a preemptive role in ensuring food security, which combines the three components: availability of food, food access, and food utilization (Aisyah et al., 2020). However, food consumption is expected to rise by 59–102% due to the world population’s rapid rise; in 2018,17 the world population was 7.6 billion, which is expected to reach 9.7 billion in 2050 (Elferink and Schierhorn, 2016; Fukase and Martin, 2020). These high demands are imposing a burden on the agriculture sector to provide enough supply to meet the required demand, which can be done in one of two ways: either by expanding the amount of land used for agriculture or by enhancing the existing agricultural land’s productivity through the use of fertilizer, precision farming and novel technology (Genetically modified crops and food) (Ali et al., 2019; Fukase and Martin, 2020; Pawlak and Kołodziejczak, 2020). Researchers preferably support the idea of increasing the productivity of the existing cultivation land by inducting precision farming and genetically modified crops (which are more resilience to the harsh environment along with high productivity) relative to the expansion of the agriculture and arable land (Ali et al., 2019, 2021; Pawlak and Kołodziejczak, 2020), resulting the deforestation (Bahar et al., 2020). The impact of prudent land management on productivity and food security is an evolving area of investigation in China (Du et al., 2018). In 2019, although the Indian cultivated land area became the largest in the world, however the country’s per unit production remained lower than most of the countries and contributed little to improving the country’s food security situation (Li and Song, 2023). Similarly, Pakistan has experienced land cover and land use increment while facing a production reduction and contributing to the rise of land surface temperature (Hussain et al., 2020). While the Bangladesh facing the challenges of soil salinity, tidal flooding, and erosion which is imparing the production capacity of farmers and pressuring the food security outlook of the country (Parven et al., 2022). Alike Bangladesh Indonesia is also encountering the adverse effect of land-use change on the national food security levels (Chrisendo et al., 2020; Rozaki, 2021). Malaysia is also encountering a land use change phenomenon which is exerting sever pressure on the country’s ability to contribute locally in its food security outlook (Azizan and Hussin, 2015).
2.3 Food security and fertilizers
Fertilizers are generally classified as either organic or inorganic, where organic fertilizers are made up of enhanced organic matter derived from plants or animals, while inorganic fertilizers are made up of manufactured chemicals like nitrogen, phosphate, and potash (Heinrich, 2000) which are used as soil additive that provides certain nutritional levels to the soil to enhance the productivity of the crop (Hammed et al., 2019). Theriault et al. (2018) found that in the West African Sahel, fertilizers boost land productivity and crops yield 24.1 kg per ha by including nitrogen each kg per ha. Likewise, Belete et al. (2018) explained that wheat varieties Menze, ET-13 and Tsehay produced a high yield because of the fertilizer (nitrogen). Some researchers also argued that overuse of fertilizers does not increase productivity; sometimes, it increases the productivity of some products and sometimes does not have a significant effect (Sheahan and Barrett, 2017; Belete et al., 2018; Van Wesenbeeck et al., 2021). Therefore, it is crucial to understand the land nutrition values and crops that farmers will cultivate. For instance, wheat needs 17 essential nutritional components, three of which are hydrogen, carbon and oxygen, while the remaining 14 come from the soil and mostly from plants, animals, compost, manure, or mineral fertilizers (Panhwar et al., 2019). In this case, nitrogen (N) is only required at the time of the deficiency (Panhwar et al., 2019). China classifies its cultivated land through a 10-grade classification system where grade 1 classifies the best land for cultivation and the 10 attributes to the low-quality land. In 2019, the average grade of the land was 4.76, and two-thirds of the soil was of low to medium quality, indicating the deficiencies that needed to be covered to increase land productivity.18 Similarly, in India, 55% of the soil is nitrogen (N) deficient, and 42% is deficient in phosphorus (P), making India an intensive user of fertilizers to augment productivity.19 Soil nutrient deficiency, which has been attributed as a vital factor in low yielding, has also been reported by Pakistan. The deficient nutrients in Pakistani soil, like India, are also nitrogen (N), phosphorus (P), and potassium (K) (Saber et al., 2009; Thanh et al., 2018). The soil nutrient deficiency has been recorded in indonesia as well where the studies have reported the deficiency of nitrogen (N), phosphorus (P), potassium (K), and organic carbon; needing various areas to employ different proportions of fertilizers for the enhancement of land productivity (Prasetyo et al., 2022). In Bangladesh, significant reduction in potassium (K), boron (B), copper (Cu), iron (Fe), manganese (Mn), and zinc (Zn), whereas an increase in calcium (Ca), and magnesium (mg) was recorded; indicating the proportions of fartilizers needed for enhancing crop yields (Shamim et al., 2019). Malaysia is also encountering the potassium (K), phosphorus (P), sulfur (S), and boron (B) deficiency; requiring it to use fartilizers for augmenting productivity (Lai et al., 2019).
2.4 Food security and consumption of fixed capital
According to traditional consumption-based accounting research, fixed capital consumption is the fulfilment of the actual demand for household needs (Kanemoto et al., 2020; Kobayakawa, 2022) by food products from agriculture, fishing and forest that are the bare necessities and embedded in food security (Garcia et al., 2020). Likewise, the production and consumption of fixed capital are tightly intertwined (Södersten et al., 2018). Therefore, fixed capital allows us to utilize resources to enhance agricultural productivity, which assists in subsiding the food shortage problems (Fukase and Martin, 2020). Developing populous countries like China, India, Pakistan, Bangladesh, Indonesia, and Malaysia encounter the challenges of forming fixed capital and increasing consumption in the sector where the masses need it the most; they can overcome these shortcomings through foreign direct investment and strategic technology sharing in the food sector (Liu, 2014; Slimane et al., 2016) to attain the United Nations sustainable development goal of “Zero Hunger” and attain the food security for the masses.
3 Methodology
3.1 Data sample and processing
The study investigates the impact of environmental temperature surges, arable land, agricultural land, fertilizer and consumption of fixed capital on food security in the context of six populous Asian countries: China, India, Indonesia, Pakistan, Bangladesh, and Malaysia. The study analyzes these indicators spanning from 2016 to 2020. The study relies on the data available on public databases such as FAOstat,20 World Bank,21 Economist Impact22 and national statistics bureaus (see Table 1). The United Nations (UN) database reasonably defines the grasp of distinct indicators and explains the methods opted for their computation and the measures employed for the validation; the same criteria are considered while employing the data obtained from sources other than the UN. Moreover, the study analyzes the models through the panel data analysis approach, a widely used methodology that primarily accommodates the analysis entailing both spatial and temporal dimensions (Yaffee, 2003; Chen et al., 2019).
3.2 Model selection
This article goes through the most common panel data analysis models in academic papers (Greene, 2003; Baltagi, 2008; Hsiao, 2022). The panel data approach sheds light on both temporal and spatial dimensions. The temporal dimension covers the data collected through periodic measurements, and the spatial dimension presents the level at which we observe phenomena such as individual actions and reactions, national and organizational decisions and trends (Wooldridge, 2015; Hsiao, 2022).
Mathematically generic regression model of panel data looks like this:
Where j is the observation unit, t time, k indicates the kth explanatory variable, β0 intercept, βk represents the coefficient of the kth explanatory variable, and is the error term which is divided into two sub-components like representing the unit-specific cross-sectional error that refers to the fixed effects, unobserved heterogeneity and also the unobserved effect in the literature, which does not vary over time and represent the idiosyncratic error that changes with time and cross-sectional units (Baltagi and Kao, 2001; Greene, 2003; Baltagi, 2008; Wooldridge, 2015; Hsiao, 2022). Moreover, using panel data and separating the error terms into two categories allows us to alleviate some concerns about bias in omitted variables due to unquantified unit-specific characteristics.
Thus, by integrating Equations (1, 2):
Similarly, the model employed in this study is as follows:
In Equation 4, FS represents food security, TS is environmental temperature surges, ARL is arable land, AGL is agricultural land, N is Nutrient nitrogen, PH is Nutrient phosphate P2O5, and CFC is the consumption of fixed capital.
3.2.1 Fixed effects model
Researchers frequently employ the fixed effects model to account for unobserved heterogeneity, unobserved effect, or fixed effects these factors remain constant over time but vary among units and are generally ignored in the pooled OLS model. Notably, the fixed effect model allows us to determine the Equation (3), along with the unit-specific cross-sectional error and idiosyncratic error which shows that is correlated with and idiosyncratic errors are independent of the (Greene, 2003; Xu et al., 2007; Wooldridge, 2015; Hsiao, 2022). Moreover, to obtain more accurate estimates, we can eliminate the unobserved heterogeneity through the widely used technique know as the time demeaning or the within transformation (Wooldridge, 2015), which also means reducing biases due to omitted variables. Thus, we have more robust results from the fixed effect model. The fixed effect model estimation relies on the ordinary least squares (OLS) technique for generating results.
3.2.2 Random effects model
It is a common practice to employ the fixed effects model to eliminate the unobserved heterogeneity when we are analyzing panel data due to the assumption of correlation between unobserved heterogeneity and any explanatory variables On the other hand, when unobserved heterogeneity is not dependent on any of the explanatory variables; using the fixed effects model to get rid of unobserved heterogeneity will become an ineffective estimator (Greene, 2003; Xu et al., 2007; Baltagi, 2008). In this situation, the random effect model becomes more effective because it treats the unobserved heterogeneity randomly as compared to the fixed effect model, it is also known as variance components model (Baltagi and Kao, 2001; Baltagi, 2008). Moreover, the random effects model was measured in this study using the generalized least squares (GLS) method; if the variance structure across categories is known and unknown, then we use the feasible generalized least squares (FGLS) technique (Greene, 2003; Hsiao, 2022).
3.2.3 Adopted approach for model selection
In this study, we employed the fixed effect and the random effect models to analyze the proposed relationship significance level on time-varying explanatory factors, whether the fixed effects model is suitable or the random effect model is checked through the researchers’ recommend Hausman test, which allows identifying the unobserved heterogeneity is independent of any of the explanatory variables or not under the shed of the null hypothesis (Wooldridge, 2002; Xu et al., 2007; Baltagi, 2008). Random effects are preferred if the null hypothesis is accepted; otherwise, the fixed effects model is superior to random effects (Xu et al., 2007).
4 Results
4.1 Data distribution, descriptives, and normality
Scholars often assume the data has a Gaussian or normal distribution and conduct analyses like Pearson correlation, regression, and variance that rely on this assumption to draw valid conclusions about the results of the proposed models (Wooldridge, 2015; Hsiao, 2022). However, this assumption can backfire if the data does not actually follow a Gaussian or normal distribution; if this is the case, the scholars have invested their time and effort in the wrong direction instead of employing the non-parametric techniques such as the spearman correlation test, polynomial regression, Wilcoxon rank sum test, the Mann–Whitney U test, and the Kruskal test (Ghasemi and Zahediasl, 2012; Field, 2013; Derrick et al., 2020). Therefore, first, we checked the normal distribution of the data, which helped us to transform the data as per the valid and accurate transformation technique. Thus, in this study, we normalized (Yu et al., 2009) the data on the 0 to 100 scale and performed the normality test to confirm our assumption was valid, and Table 2 shows that Shapiro Wilk W results meet the minimum criteria (Ghasemi and Zahediasl, 2012; Derrick et al., 2020).
4.2 Baseline regression
We obtained the baseline regression results by relying on the more appropriate model between the fixed and the random; the Hausman test results (Baltagi, 2008; Bartels, 2008) provided the foundation for the model selection. For this reason, we utilized STATA 17 to analyze the model selection and proposed relationship association. First, in STATA, we set the data as a panel time series data, then we performed the fixed effect regression and stored their values. Afterwards, we performed the random effect regression and stored their values; then, we used the Hausman test command on the stored values. Results of the Hausman test favored the fixed effect because the p value of the Chi-square is less than 0.05 (see Table 3; Hausman, 1978; Baltagi, 2008; Bartels, 2008). Hence, the fixed effect is more suitable for the problem investigated in this study. However, it is also imperative to determine whether the time-fixed effect contributes to the baseline regression outcomes. Thus, we performed the testparm yearly and found Prob > F is non-significant, meaning that accounting for the time-fixed effect is not needed in the present situation (Torres-Reyna, 2007).
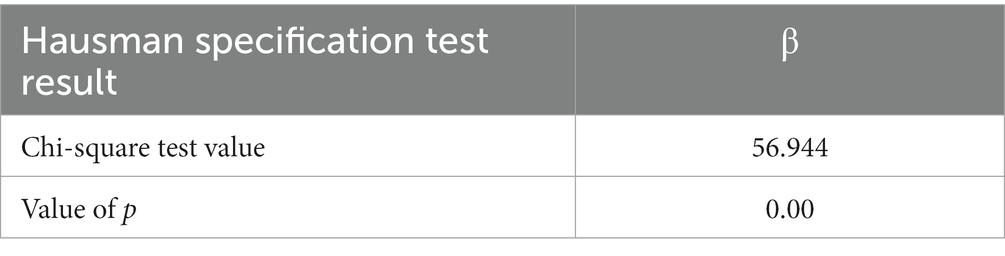
Table 3. Hausman (1978) specification test.
Finally, we selected the fixed effect model to investigate the impact of the change in temperature, arable land, agricultural land, fertilizers such as nutrient nitrogen and phosphate and the consumption of the fixed capital on food security. We found that temperature change and arable land have a significantly adverse effect on food security; meanwhile, expansion of the agricultural land, use of fertilizers based on the soil nutrient information and consumption of the fixed capital in the agriculture sector have a positive impact on food security (see Table 4).
4.3 Post estimation diagnostic
4.3.1 Multicollinearity test
Moreover, we checked the multicollinearity via the variance inflation factor (VIF), and the VIF range is between 1.068 and 1.754, which falls under the threshold and indicates that the basic conditions have been satisfied. Model estimates and their respective p values are valid for concluding the problem statement (see Table 5).
4.3.2 Cross-sectional dependence check via Breusch-pagan LM test of independence
As per Baltagi (2008), small data does not have a cross-sectional dependency or contemporaneous correlation concern, and it has statistical significance if the data is of more than 20 or 30 years. Even though in this study, the time dimension is short (t = 5 years), we still have performed the Breusch-Pagan LM test of independence, and the non-significant Pr value indicates that the data does not have cross-sectional dependence (see Table 6).
4.3.3 Heteroskedasticity check
Heteroskedasticity is a source of bias in regression analysis (Baltagi, 2008). So, It is vital to check for heteroskedasticity to present a legitimate analysis. Therefore, we checked for the heteroskedasticity via Breuscha Pagan/ Cookac Weisberg test and found Prob>chi2 > 0.05, indicating that heteroskedasticity adjustment is not required in the present scenario (see Table 7; Robinson, 1991; Torres-Reyna, 2007; Baltagi, 2008).
4.3.4 Serial correlation check
When investigators deal with data that spans more than 20 years or is on a macro scale, serial correlation issues arise. These issues can significantly alter the outcome and impair the practical applicability of the findings (Baltagi, 2008). Thus, autocorrelation is not a problem because the study’s data, which span 5 years, are not long-span data. Additionally, the Wooldridge test we conducted revealed no first-order autocorrelation, and Prob>F is non-significant (see Table 8), which meets the threshold requirements (Robinson, 1991; Torres-Reyna, 2007; Baltagi, 2008).
5 Discussion and conclusion
5.1 Theoretical implications
United Nations established 17 sustainable development goals along with 169 sub-targets as an urgent call for the globe to actively pursue the agenda of the greater good for the betterment of humanity and the entire world’s sustainability and prosperity. In this regard, the United Nations encourages developed and developing nations to have a global partnership to eradicate the world’s most critical problems; one of these critical problems is global hunger for the eradication of which the UN devised its second SDG with the focus of attaining “zero hunger” by 2030. Thus, this study investigates zero hunger through the lens of food security in populous Asia from the perspectives of the pre-and post-pandemic eras. The findings of this study explain that temperature change negatively affects food security. The temperature surge breeds unfavorable conditions for agricultural products and human life, evidenced by the low productivity of staple crops like wheat in India and Pakistan during unprecedented heat waves and loss of life due to heat strokes pointing toward the far-reaching consequences (Choufani et al., 2017; Watts et al., 2018). Notably, our findings are aligned with previous research, which highlights that the temperature changes (rise 0.08°C to 0.84°Celsius) around the globe cause unprecedented weather events like floods, drought, storms, and typhoons which are the primary source of natural resources’ impairment and threaten food security (including availability, safety, and sustainability) (Choufani et al., 2017; Watts et al., 2018; Godde et al., 2021). For instance, from 1981 to 2009, India saw a 5.2% decline in its wheat crop due to global warming, soil nutrient deficiency (Gupta et al., 2017), and related factors such as rising day and nighttime temperatures (Jha and Tripathi, 2017). In Pakistan, the impact was more devastating; it endured damage to 78,000 square kilometers (30,000 square miles) of agricultural land throughout 81 districts, and more than 80 per cent of the nation’s crops were ravaged. The Sindh province, which provides a significant portion of the nation’s food, is among the most severely impacted.23 We saw the same temperature rise trend, threatening the food supply in places like Indonesia, China, Bangladesh, and Malaysia.
Second, the results of this study show that arable land negatively affects food security due to the expansion of arable land and its use for grazing or home gardening and tree cultivation, indirectly affecting agricultural crop production. In contrast, agricultural land positively influences food security because it is the primary source of food production. While the world has an overpopulation burden, in 2018 global population was 7.6 billion which is projected to reach 9.7 billion by 2050 (Elferink and Schierhorn, 2016; Fukase and Martin, 2020); especially Asia contributing a significant portion of this rise. As a result, food consumption is bound to depict a surge of 59 to 102%.24 The impact of these issues can be diminished by extending the agricultural land area for agricultural production or boosting land productivity by utilizing fertilizers based on the soil nutrient deficiencies as it is garnering positive results in the under-instigation countries, coupled with the new technologies such as genetically modified (GM) crops and food in the agriculture sector (Ali et al., 2019; Fukase and Martin, 2020; Pawlak and Kołodziejczak, 2020). The adoption of GM crops is seeing a rising trend in China, India, and Pakistan mostly because of the tendency of these crops to perform in even harsh climatic conditions, protect themselves from insect attacks, have the reputation of a safe product, and be nutritionally rich (Ali et al., 2019, 2021). The option of agricultural land expansion can trigger deforestation (Bahar et al., 2020), harming the environment, ecosystem and food security. For a sustainable solution, experts usually suggest the mass production of crops and food on the existing land through innovative techniques such as drip irrigation, drone seeding, technologically modified crops and food cultivation without utilizing extensive natural resources (Ali et al., 2019, 2021; Pawlak and Kołodziejczak, 2020).
Third, this study’s findings show that nitrogen and phosphate positively influence food security. Fertilizers are considered soil additives that enhance land nutrient values that support the crop’s productivity (Hammed et al., 2019). Similarly, our findings align with previous research (Belete et al., 2018; Theriault et al., 2018; Hammed et al., 2019; Panhwar et al., 2019; Ghufran et al., 2024), explaining that fertilizers boost land productivity and crop yield. Evidence from all under-investigation countries (China, Pakistan, India, Bangladesh, Indonesia, Malaysia) also supports that using fertilizers based on soil nutrient deficiencies augments land productivity. The approach promoted by several experts for effective fertilizer utilization while warning about the misusage of fertilizers, causing productivity loss and adverse effects on human health (Sheahan and Barrett, 2017; Belete et al., 2018; Van Wesenbeeck et al., 2021). Hence, our findings endorse fertilizer utilization to cover the soil nutrient gap, bolstering land productivity and subsiding human health risks.
Fourth, in this study, we find that fixed capital consumption substantially influences food security because it is tightly intertwined (Södersten et al., 2018) and satisfies genuine household needs, such as essential foods products derived from agriculture, fishing, and forestry that contribute to food security (Garcia et al., 2020; Kanemoto et al., 2020; Kobayakawa, 2022). As a result, the availability of fixed capital enables economies to capitalize on opportunities for enhancing agricultural output and curtailing the challenges like food scarcity (Fukase and Martin, 2020). Hence, foreign direct investment and strategic technology sharing in the food sector can augment developing and underdeveloped countries’ ability to form fixed capital and direct its consumption in the sector where they need it the most (Liu, 2014; Slimane et al., 2016) to achieve the United Nations sustainable development goal of “Zero Hunger” from the perspective of food security.
5.2 Practical implications
5.2.1 For policymakers
This study provides essential guidance to multi-stakeholders – and opens the door for policymakers to understand the pressing issue of food scarcity, especially in Asia’s most populous countries, including India, Pakistan, China, Bangladesh, Indonesia, and Malaysia, which have a population of 4 billion and houses nearly 418 million undernourished people almost half the world’s famished population (768 million).25 In addition, these six Asian nations, accounting for approximately 56% of the world’s population, have a food security environment ranking below or around the global food security average of 50. Therefore, enhanced focus and segregation of the policy instruments and their implementation on various levels are paramount. First, policymakers of these nations and global stakeholders should keenly notice the situation among these countries and ensure the effective utilization of the existing and newly added agricultural land, enhancing crops and food production. Secondly, strongly discourage and strictly prohibit infrastructure development like housing schemes on agricultural land, impairing food security outlook and threatening human survival. Building infrastructure on agricultural land poses significant environmental and socio-economic challenges. The conversion of fertile and arable land for construction projects leads to the loss of valuable agricultural resources, diminishing the capacity for food production and compromising food security (Foley et al., 2011). The impact extends to biodiversity loss as the conversion disrupts ecosystems supporting diverse flora and fauna (Tsiafouli et al., 2015). Furthermore, the construction activities contribute to soil degradation through compaction, erosion, and contamination, rendering the land less suitable for future agricultural use (Montgomery, 2007). Water scarcity becomes a concern as irrigation systems are disrupted, affecting both agricultural and non-agricultural water needs (Liu et al., 2013). The displacement of traditional farming communities results in social and economic challenges, while the conversion of agricultural land contributes to increased greenhouse gas emissions and influences local climate patterns, exacerbating the impacts of climate change (Lobell et al., 2008; UNICEF, 2021). Sustainable land-use planning and environmental impact assessments are essential to mitigate these issues and promote alternative development practices that balance the need for infrastructure with the preservation of vital agricultural resources. Third, sharing information with farmers about soil nutrient deficiencies and advising about the correct proportion of fertilizer utilization, enhancing land productivity and subsiding the effects of excessive fertilizer usage on human health. Lastly, they also have to allocate fixed capital for research and development in the agriculture sector to determine the resilient seeds against the harsh environment and employ the vertical farming concept for enhancing production levels without deforestation and damage to the ecosystem.
5.2.2 For businesses
Asian nations are considered agricultural because of the cultivated area, which is highly suited for agricultural production and cultivation. Surprisingly, they face food insecurity and undernourishment, and their agricultural imports are rising: in China, 80% of consumed soybeans are imported;26 in Malaysia, 60% of consumed food is imported;27 in Indonesia, imports bills exceeded 24 billion dollars in 2021.28 Pakistan also imports food products, including wheat, rice, soybeans, and cooking oil, worth $1.65 billion,29 and Bangladesh imported 6.6 million tonnes of foodgrains, the highest amount in 3 years.30 These crucial facts give local and international investors opportunities to invest in the agriculture sector to enhance agricultural productivity by employing the latest agriculture tools and cultivation methods. In this regard, businesses can maximize their wealth by collaborating with the farmers and enhancing their social standing in the market by serving the greater good.
5.2.3 Future research and limitations
In this research, we have captured the overall impact on food security based on the data of six populous Asian countries from 2016 to 2020. Future research may add more years, use different attributes like corporate social responsibility, sustainable finance options, and compare the impact of different attributes among different countries. Furthermore, this research is Asia-focused future research may check the food security environment in other regions and capture a much deeper outlook by analyzing the situation at the city level in different countries. At last, future research may consider GM’s role in food security. We have provided some important standing of these six Asian countries. In detail, the augmenting demand for agricultural products (food and non-food) has encouraged considering GM products to fill this demand gap across all (China, India, Pakistan, Bangladesh, Malaysia, and Indonesia). According to Wang et al. (2023), the Chinese Ministry of Agriculture’s assurances that certified genetically modified (GM) food products are safe to consume even though most consumers are reluctant to purchase GM foods. Moreover, they found that Chinese consumers’ awareness, products’ available information, trust in sellers, long product life, GM foods’ taste, and consumers’ experience in buying GM food products are positively related to their willingness to buy GM foods. In 2022, China expanded the commercial adoption of genetic modification (GM) to two staple crops, corn and soybeans, making major strides in its plan to ensure food security despite persistent public controversy over the technology.31 Adopting GM corn and soybean is likely to increase average yields, possibly by as much as 50 per cent, effectively reducing China’s demand for large volumes of these two feeds.32
India has debated using genetically modified (GM) crops for over two decades. The Supreme Court of India has been hearing constitutional challenges to India’s regulatory regime for GM crops for nearly two decades.33 In 2022, the Union Ministry of Environment, Forests and Climate Change approved the University of Delhi’s GM mustard seeds for cultivation, which would have allowed the university to release GM mustard seeds to farmers. However, environmentalists immediately appealed against the approval before the Supreme Court, which temporarily halted the approval on 3 November 2022 (see footnote 34, respectively). Supreme Court eventually approved GM mustard, which will become India’s first GM food crop (see footnote 34, respectively). According to the Center for Science and Environment 2018, 21 out of 65 food samples tested from Delhi, Gujarat, and Punjab were GM positive.34 The study formed the basis of the latest regulations, which mandate that no person shall manufacture, store, distribute, sell or import any food or food ingredient derived from Genetically Modified Organisms in India.
Riansah et al. (2023) explained that genetically modified (GM) food is controversial in Indonesia, but the Indonesian government has regulations in place to ensure consumer protection by labeling food products with GM ingredients. However, Indonesia’s regulations on GM are still dependent on worldwide statements, and the country has limited capability to enforce regulations for the biosafety of genetically engineered (GE) products (Riansah et al., 2023). GM food is one of the results of a breakthrough in the application of biotechnology to increase food production. In Indonesia, many of these products have been mainly imported fruits and meat, as well as imported vegetables and food products. Most GM products contain vegetable and fruit products such as apples, oranges, bananas, and many more (Riansah et al., 2023). In 2017, the United States exported nearly $1.6 billion genetically engineered products to Indonesia. These products included Bt cotton, herbicide-tolerant soybeans and meal, Bt corn, and various food products derived from GE crops.35 The government of Indonesia and local universities are researching a number of GE crops, including virus resistance for tomatoes, rice, potatoes, and sugar canes (see footnote 36, respectively).
Wana et al. (2021) explained that Malaysia has regulations on genetically modified organisms (GMOs) and their presence in the marketplace. The presence of an unauthorized GMO in the marketplace is regarded as a regulatory non-compliance, to which the Malaysian government has a zero-tolerance approach.36 On the other hand, the Department of Biosafety in the Ministry of Natural Resources and Environment Malaysia, under the National Biosafety Act 2007, has approved eight genetically modified (GM) maize/corn products and 5 GM soybean products for food, feed, and processing purposes.37 Besides these, approvals have also been given for the field trials of GM mosquitoes and papaya and the release of GM products for use as pesticides and fertilizers.
In 2005, Pakistan established its federal regulatory framework for biotechnology, designed to approve emerging technologies under the Environmental Protection Act of 1997. This initiative led to the creation of the Pakistan Biotechnology Regulatory (PBR) in April 2005.38 In 2019, Pakistan imported approximately 3.73 million bales (375 lbs./bale) of cotton, with a major share sourced from the United States and Brazil (see footnote 39, respectively). Furthermore, Pakistan is a notable importer of various agricultural products, including soybeans, soybean meal, soybean oil, canola, and distillers dried grains (DDGs) derived from genetically engineered (GE) crops originating from the United States, Brazil, Canada, and Argentina (see footnote 39, respectively). Specifically, in CY 2019, Pakistan’s soybean imports amounted to about 1.92 million metric tons, valued at approximately $690 million. Around 60 per cent of these imports were sourced from the United States (see footnote 39, respectively).
Bangladesh has become the first South Asian country to approve commercial cultivation of a genetically modified (GM) food crop – brinjal (also known as eggplant or aubergine) spliced with a gene from the soil bacterium Bacillus thuringiensis.39 Moreover, In Bangladesh, the imminent approval of commercially cultivating genetically modified (GM) crops for food and feed raises concerns due to the lack of specific biosafety regulations.40 Existing laws covering agriculture, medicine, and the environment inadequately address GM-related issues (see footnote 41, respectively). Outdated environmental regulations and a lack of differentiation between GM and non-GM crops contribute to challenges (see footnote 41, respectively). Despite consumer rights in the present Consumer Protection Act, there is a dearth of research on the effects of GM crops (see footnote 41, respectively).
Data availability statement
The original contributions presented in the study are included in the article/supplementary material, further inquiries can be directed to the corresponding author.
Author contributions
MJ, JA, PX, and SA: conceptualization. MG, MJ, and JA: methodology, software, and formal analysis. SA and PX: validation, resources, visualization, and supervision. MG, JA, and SA: investigation and writing–original draft preparation. MG and MJ: data curation. SA, JA, and PX: writing–review and editing. PX, MJ, and SA: project administration. MJ and PX: funding acquisition. All authors contributed to the article and approved the submitted version.
Conflict of interest
The authors declare that the research was conducted in the absence of any commercial or financial relationships that could be construed as a potential conflict of interest.
Publisher’s note
All claims expressed in this article are solely those of the authors and do not necessarily represent those of their affiliated organizations, or those of the publisher, the editors and the reviewers. Any product that may be evaluated in this article, or claim that may be made by its manufacturer, is not guaranteed or endorsed by the publisher.
Abbreviations
SDG, Sustainable development goals; WHO, World Health Organization; GM, Genetically modified; P2O5, Phosphate; N, Nitrogen; FS, Food security; UN, United Nations; NOAA, National Oceanic and Atmospheric Administration Agency’s; FAO, Food and Agriculture Organization of the United Nations; OLS, Ordinary least squares; FGLS, Feasible generalized least squares; GLS, Generalized least squares
Footnotes
1. ^https://www.un.org/sustainabledevelopment/wp-content/uploads/2016/08/2_Why-It-Matters-2020.pdf
2. ^https://www.un.org/sustainabledevelopment/wp-content/uploads/2022/07/Goal-2-infographic.pdf
3. ^https://www.fao.org/3/cb4474en/cb4474en.pdf
4. ^https://www.un.org/sustainabledevelopment/wp-content/uploads/2022/07/Goal-2-infographic.pdf
5. ^https://www.who.int/health-topics/foodborne-diseases#tab=tab_2
6. ^https://www.fao.org/3/cb4474en/cb4474en.pdf
7. ^https://unstats.un.org/sdgs/report/2022/The-Sustainable-Development-Goals-Report-2022.pdf
8. ^https://insideclimatenews.org/news/02082022/flooding-flooding-monsoons-india-pakistan-bangladesh/
9. ^https://ahacentre.org/flash-update/flash-update-no-01-flooding-and-landslides-in-malaysia-6-march-2023/
10. ^https://www.worldbank.org/en/news/feature/2023/08/17/india-managing-the-complex-problem-of-floods-and-droughts
11. ^https://www.nbcnews.com/science/environment/south-asias-intense-heat-wave-sign-things-come-rcna30239
12. ^https://apnews.com/article/floods-peshawar-pakistan-glaciers-56a830b87746447385e59e261a07b18d
13. ^https://www.fao.org/3/cb4474en/cb4474en.pdf
14. ^https://www.ncei.noaa.gov/news/global-climate-202112
15. ^https://www.wsj.com/articles/pakistans-flood-losses-deal-crushing-blow-to-ailing-economy-11663250402
16. ^https://unstats.un.org/unsd/environment/agriculturalland.htm
17. ^https://www.fao.org/faostat/en/#data/OA
18. ^https://chinadialogue.net/en/pollution/china-launches-first-national-soil-survey-in-40-years/#:~:text=China%20uses%20a%2010%2Dgrade,land%20is%20continuing%20to%20degrade
19. ^https://www.deccanherald.com/opinion/soil-health-crisis-urgent-reforms-needed-for-sustainable-agriculture-1234300.html
20. ^Food and Agriculture Organization (FAO), (2020, December 14), Government Regulation of the Republic of Indonesia No. 17 of 2015 concerning Food and Nutrition Security. FAOLEX Database. https://www.fao.org/faolex/results/details/en/c/LEX-FAOC169453
21. ^https://data.worldbank.org/indicator/SN.ITK.MSFI.ZS?end=2018&start=2015&view=chart
22. ^Global Food Security Index (GFSI) (economist.com).
23. ^https://www.aljazeera.com/opinions/2022/10/28/floods-are-tipping-pakistan-into-a-food-crisis
24. ^https://www.fao.org/faostat/en/#data/OA
25. ^https://www.fao.org/3/cb4474en/cb4474en.pdf
26. ^https://www.weforum.org/agenda/2022/11/china-domestic-food-stability-amid-global-food-crises/
27. ^https://www.businesstoday.com.my/2022/05/21/malaysia-and-our-food-security-conundrum/
28. ^https://www.trade.gov/country-commercial-guides/indonesia-agriculture#:~:text=Indonesian%20agricultural%20imports%20surpassed%20%2424,ingredients%20were%20the%20main%20categories
29. ^https://www.business-standard.com/article/international/flood-hit-pakistan-s-food-imports-surge-65-during-first-two-months-of-fy23-122091600051_1.html
30. ^https://archive.dhakatribune.com/bangladesh/2021/07/03/bangladesh-s-food-import-hits-second-highest-in-10-years
31. ^https://www.scmp.com/economy/china-economy/article/3238523/china-adopts-gm-technology-corn-soybeans-major-food-security-manoeuvre
32. ^https://phys.org/news/2022-01-china-shifting-gm-policy-corn.html
33. ^https://in.boell.org/en/gm-crops-india
34. ^https://timesofindia.indiatimes.com/life-style/food-news/what-are-gm-foods-and-how-have-they-secretly-become-part-of-our-diet/photostory/77099285.cms
35. ^https://apps.fas.usda.gov/newgainapi/api/report/downloadreportbyfilename?filename=Agricultural%20Biotechnology%20Annual_Jakarta_Indonesia_11-20-2017.pdf
36. ^https://www.statista.com/statistics/983765/malaysia-willingness-to-eat-gm-foods/
37. ^http://www.biosafety.nre.gov.my/country_decision/app_plmo.shtml
38. ^https://apps.fas.usda.gov/newgainapi/api/Report/DownloadReportByFileName?fileName=Agricultural%20Biotechnology%20Annual_Islamabad_Pakistan_10-20-2020
39. ^https://www.scidev.net/asia-pacific/news/bangladesh-takes-to-gm-food-crops/
40. ^https://foodcomplianceinternational.com/industry-insight/scholarly-articles/3148-bangladesh-legal-regime-of-genetically-modified-food-developing-country-in-the-context
References
Abay, K. A., Breisinger, C., Glauber, J. W., Kurdi, S., Debucquet, D. L., and Siddig, K. (2022). The Russia-Ukraine crisis: Implications for global and regional food security and potential policy responses. Washington, DC: The International Food Policy Research Institute.
Ahmad, D., and Afzal, M. (2022). Flood hazards and agricultural production risks management practices in flood-prone areas of Punjab, Pakistan. Environ. Sci. Pollut. Res. 29, 20768–20783. doi: 10.1007/s11356-021-17182-2
Aisyah, I. N. T., Purnomo, E. P., and Kasiwi, A. N. (2020). Analisis Kebijakan Ketahanan Pangan di Kabupaten Bantul. Int. J. Demos 2:40. doi: 10.37950/ijd.v2i2.40
Aldemita, R. R., and Hautea, R. A. (2018). Biotech crop planting resumes high adoption in 2016. GM Crops Food 9, 1–12. doi: 10.1080/21645698.2018.1428166
Ali, S., Ghufran, M., Nawaz, M. A., and Hussain, S. N. (2019). The psychological perspective on the adoption of approved genetically modified crops in the presence of acceptability constraint: the contingent role of passion. GM Crops Food 10, 220–237. doi: 10.1080/21645698.2019.1680241
Ali, S., Nawaz, M. A., Ghufran, M., Hussain, S. N., and Hussein Mohammed, A. S. (2021). GM trust shaped by trust determinants with the impact of risk/benefit framework: the contingent role of food technology neophobia. GM Crops Food 12, 170–191. doi: 10.1080/21645698.2020.1848230
Andrade, L., O'Dwyer, J., O'Neill, E., and Hynds, P. (2018). Surface water flooding, groundwater contamination, and enteric disease in developed countries: a scoping review of connections and consequences. Environ. Pollut. 236, 540–549. doi: 10.1016/j.envpol.2018.01.104
Arora, N. K. (2019). Impact of climate change on agriculture production and its sustainable solutions. 2. Berlin: Springer, pp. 95–96.
Azizan, M. U., and Hussin, K. (2015). Understanding the pressure on agriculture land as a safeguard for food security in Malaysia. International J. Sustain. Built Environ, 2.
Bahar, N. H., Lo, M., Sanjaya, M., Van Vianen, J., Alexander, P., Ickowitz, A., et al. (2020). Meeting the food security challenge for nine billion people in 2050: what impact on forests. Glob. Environ. Chang. 62:102056. doi: 10.1016/j.gloenvcha.2020.102056
Baltagi, B. H., and Kao, C. (2001). Nonstationary panels, cointegration in panels and dynamic panels: A survey. Nonstationary panels, panel cointegration, and dynamic panels. Bingley, UK: Emerald Group Publishing Limited.
Bartels, B. (2008). Beyond fixed versus random effects: a framework for improving substantive and statistical analysis of panel, time-series cross-sectional, and multilevel data. Soc. Polit. Methodol. 9, 1–43.
Belete, F., Dechassa, N., Molla, A., and Tana, T. (2018). Effect of nitrogen fertilizer rates on grain yield and nitrogen uptake and use efficiency of bread wheat (Triticum aestivum L.) varieties on the Vertisols of central highlands of Ethiopia. Agric. Food Secur. 7:78. doi: 10.1186/s40066-018-0231-z
Ben Hassen, T., and El Bilali, H. (2022). Impacts of the Russia-Ukraine war on global food security: towards more sustainable and resilient food systems? Food Secur. 11:2301. doi: 10.3390/foods11152301
Cadieux, B., Goodridge, L. D., and Spink, J. (2019). Gap analysis of the Canadian food fraud regulatory oversight and recommendations for improvement. Food Control 102, 46–55. doi: 10.1016/j.foodcont.2019.03.012
Chen, C., Park, T., Wang, X., Piao, S., Xu, B., Chaturvedi, R. K., et al. (2019). China and India lead in greening of the world through land-use management. Nat. Sustain. 2, 122–129. doi: 10.1038/s41893-019-0220-7
Choufani, J., Davis, C., McLaren, R., and Fanzo, J. (2017) Climate change and variability: What are the risks for nutrition, diets, and food systems? Washington, DC: The International Food Policy Research Institute.
Chrisendo, D., Krishna, V. V., Siregar, H., and Qaim, M. (2020). Land-use change, nutrition, and gender roles in Indonesian farm households. Forest Policy and Economics, 118, 102245.
Derrick, B., White, P., and Toher, D. (2020). Parametric and non-parametric tests for the comparison of two samples which both include paired and unpaired observations. J. Mod. Appl. Stat. Methods 18, 2–23. doi: 10.22237/jmasm/1556669520
Duarte, C. M., Agusti, S., Barbier, E., Britten, G. L., Castilla, J. C., Gattuso, J. P., et al. (2020). Rebuilding marine life. Nature 580, 39–51. doi: 10.1038/s41586-020-2146-7
Du, X., Zhang, X., and Jin, X. (2018). Assessing the effectiveness of land consolidation for improving agricultural productivity in China. Land use policy, 70, 360–367.
Elferink, M., and Schierhorn, F. (2016). Global demand for food is rising. Can we meet it. Harv. Bus. Rev. 7:11.
Foley, J. A., Ramankutty, N., Brauman, K. A., Cassidy, E. S., Gerber, J. S., Johnston, M., et al. (2011). Solutions for a cultivated planet. Nature 478, 337–342. doi: 10.1038/nature10452
Ford, H. V., Jones, N. H., Davies, A. J., Godley, B. J., Jambeck, J. R., Napper, I. E., et al. (2022). The fundamental links between climate change and marine plastic pollution. Sci. Total Environ. 806:150392. doi: 10.1016/j.scitotenv.2021.150392
Fukase, E., and Martin, W. (2020). Economic growth, convergence, and world food demand and supply. World Dev. 132:104954. doi: 10.1016/j.worlddev.2020.104954
Gallo, M., Ferrara, L., Calogero, A., Montesano, D., and Naviglio, D. (2020). Relationships between food and diseases: what to know to ensure food safety. Food Res. Int. 137:109414. doi: 10.1016/j.foodres.2020.109414
Garcia, S. N., Osburn, B. I., and Jay-Russell, M. T. (2020). One health for food safety, food security, and sustainable food production. Front. Sustain. Food Syst. 4:1. doi: 10.3389/fsufs.2020.00001
Ghasemi, A., and Zahediasl, S. (2012). Normality tests for statistical analysis: a guide for non-statisticians. Int. J. Endocrinol. Metab. 10, 486–489. doi: 10.5812/ijem.3505
Ghufran, M., Aldieri, L., Pyka, A., Ali, S., Bimonte, G., Senatore, L., et al. (2024). Food security assessment in the light of sustainable development goals: a post-Paris agreement era. Environ. Dev. Sustain. 2024:89. doi: 10.1007/s10668-023-04089-w
Ghufran, M., Ali, S., Ariyesti, F. R., Nawaz, M. A., Aldieri, L., and Xiaobao, P. (2022a). Impact of COVID-19 to customers switching intention in the food segments: the push, pull and mooring effects in consumer migration towards organic food. Food Qual. Prefer. 99:104561. doi: 10.1016/j.foodqual.2022.104561
Ghufran, M., Ashraf, J., Ali, S., Xiaobao, P., and Aldieri, L. (2022b). Effect of consumption value on consumer willingness to consume GM food: a post-COVID-19 analysis. Food Secur. 11:2918. doi: 10.3390/foods11182918
Godde, C., Mason-D’Croz, D., Mayberry, D., Thornton, P. K., and Herrero, M. (2021). Impacts of climate change on the livestock food supply chain; a review of the evidence. Glob. Food Sec. 28:100488. doi: 10.1016/j.gfs.2020.100488
Gupta, R., Somanathan, E., and Dey, S. (2017). Global warming and local air pollution have reduced wheat yields in India. Clim. Chang. 140, 593–604. doi: 10.1007/s10584-016-1878-8
Hameed, M., Ahmadalipour, A., and Moradkhani, H. (2020). Drought and food security in the middle east: an analytical framework. Agric. For. Meteorol. 281:107816. doi: 10.1016/j.agrformet.2019.107816
Hammed, T. B., Oloruntoba, E. O., and Ana, G. R. E. E. (2019). Enhancing growth and yield of crops with nutrient-enriched organic fertilizer at wet and dry seasons in ensuring climate-smart agriculture. Int. J. Recycl. Org. Waste Agric. 8, 81–92. doi: 10.1007/s40093-019-0274-6
Hausman, J. A. (1978). Specification tests in econometrics. Econometrica 46, 1251–1271. doi: 10.2307/1913827
Hussain, S., Mubeen, M., Ahmad, A., Akram, W., Hammad, H., and Nasim, W. (2020). Using GIS tools to detect the land use/land cover changes during forty years in Lodhran District of Pakistan. Environ. Sci. Pollut. Res. 27, 39676–39692.
Heinrich, W. (2000). Scherer “fertilizers” in Ullmann’s encyclopedia of industrial chemistry. Weinheim: Wiley-VCH.
Hellegers, P. (2022). Food security vulnerability due to trade dependencies on Russia and Ukraine. Food Secur. 14, 1503–1510. doi: 10.1007/s12571-022-01306-8
Jha, B., and Tripathi, A. (2017). How susceptible is India’s food basket to climate change? Soc. Change 47, 11–27. doi: 10.1177/0049085716681902
Kanemoto, K., Shigetomi, Y., Hoang, N. T., Okuoka, K., and Moran, D. (2020). Spatial variation in household consumption-based carbon emission inventories for 1200 Japanese cities. Environ. Res. Lett. 15:114053. doi: 10.1088/1748-9326/abc045
Kinney, P. L. (2018). Interactions of climate change, air pollution, and human health. Curr. Environ. Health Rep. 5, 179–186. doi: 10.1007/s40572-018-0188-x
Kobayakawa, T. (2022). The carbon footprint of capital formation: an empirical analysis on its relationship with a country's income growth. J. Ind. Ecol. 26, 522–535. doi: 10.1111/jiec.13199
Lai, C. H., Settinayake, A. R. H., Yeo, W. S., Lau, S. W., and Jong, T. K. (2019). Crop Nutrients review and the impact of fertilizer on the plantation in malaysia: a mini review. Communications in Soil Science and Plant Analysis, 50, 2089–2105.
Li, H., and Song, W. (2023). Spatial transformation of changes in global cultivated land. Science of The Total Environment, 859, 160194.
Liu, J., Hull, V., Batistella, M., DeFries, R., Dietz, T., Fu, F., et al. (2013). Framing sustainability in a telecoupled world. Ecol. Soc. 18:226. doi: 10.5751/ES-05873-180226
Liu, P. (2014). Impacts of foreign agricultural investment on developing countries: evidence from case studies. FAO Commodity and Trade Policy Research Working Papers, No. 47.
Lobell, D. B., Burke, M. B., Tebaldi, C., Mastrandrea, M. D., Falcon, W. P., and Naylor, R. L. (2008). Prioritizing climate change adaptation needs for food security in 2030. Science 319, 607–610. doi: 10.1126/science.1152339
Markus, S. (2022). Long-term business implications of Russia’s war in Ukraine. Asian Bus. Manag. 21, 483–487. doi: 10.1057/s41291-022-00181-7
Masniadi, R., Angkasa, M. A. Z., Karmeli, E., and Esabella, S. (2020). Telaah kritis ketahanan pangan Kabupaten Sumbawa dalam menghadapi pandemi covid-19. Indones. J. Soc. Sci. Hum. 1, 109–120.
Mc Carthy, U., Uysal, I., Badia-Melis, R., Mercier, S., O'Donnell, C., and Ktenioudaki, A. (2018). Global food security – issues, challenges and technological solutions. Trends Food Sci. Technol. 77, 11–20. doi: 10.1016/j.tifs.2018.05.002
Montgomery, D. R. (2007). Soil erosion and agricultural sustainability. Proc. Natl. Acad. Sci. 104, 13268–13272. doi: 10.1073/pnas.0611508104
Mutegi, J., Adolwa, I., Kiwia, A., Njoroge, S., Gitonga, A., Muthamia, J., et al. (2024). Agricultural production and food security implications of Covid-19 disruption on small-scale farmer households: lessons from Kenya. World Dev. 173:106405. doi: 10.1016/j.worlddev.2023.106405
Njage, P. M. K., Sawe, C. T., Onyango, C. M., Habib, I., Njagi, E. N., Aerts, M., et al. (2017). Microbial performance of food safety control and assurance activities in a fresh produce processing sector measured using a microbial assessment scheme and statistical modeling. J. Food Prot. 80, 177–188. doi: 10.4315/0362-028X.JFP-16-233
Oskorouchi, H. R., and Sousa-Poza, A. (2021). Floods, food security, and coping strategies: evidence from Afghanistan. Agric. Econ. 52, 123–140. doi: 10.1111/agec.12610
Paarlberg, R., Bhattacharya, A., Huang, J., Karembu, M., Pray, C., and Wesseler, J. (2024). The uptake of new crop science: explaining success, and failure. Food Policy 122:102572. doi: 10.1016/j.foodpol.2023.102572
Panhwar, Q. A., Ali, A., Naher, U. A., and Memon, M. Y. (2019). “Fertilizer management strategies for enhancing nutrient use efficiency and sustainable wheat production” in Organic farming. eds. S. Chandran, M. R. Unni, and S. Thomas (Sawston, UK: Woodhead Publishing), 17–39.
Parven, A., Pal, I., Witayangkurn, A., Pramanik, M., Nagai, M., Miyazaki, H., et al. (2022). Impacts of disaster and land-use change on food security and adaptation: Evidence from the delta community in Bangladesh. Int. J. Disaster Risk Reduct., 78, 103119.
Pawlak, K., and Kołodziejczak, M. (2020). The role of agriculture in ensuring food security in developing countries: considerations in the context of the problem of sustainable food production. Sustain. For. 12:5488. doi: 10.3390/su12135488
Peng, W., Ma, N. L., Zhang, D., Zhou, Q., Yue, X., Khoo, S. C., et al. (2020). A review of historical and recent locust outbreaks: links to global warming, food security and mitigation strategies. Environ. Res. 191:110046. doi: 10.1016/j.envres.2020.110046
Prasetyo, H., Setyobudi, R. H., Adinurani, P. G., Vincēviča-Gaile, Z., Fauzi, A., Pakarti, T. A., et al. (2022). Assessment of Soil Chemical Properties for Monitoring and Maintenance of Soil Fertility in Probolinggo, Indonesia: Assessment of Soil Chemical Properties in Probolinggo, Indonesia. Proceedings of the Pakistan Academy of Sciences: B. Life and Environmental Sciences, 59, 99–113.
Raman, R. (2017). The impact of genetically modified (GM) crops in modern agriculture: a review. GM Crops Food 8, 195–208. doi: 10.1080/21645698.2017.1413522
Riansah, D., Zmudczynska, E., and Andoko, E. (2023) The controversial case study: genetically modified food in Indonesia the controversial case study: genetically modified food in Indonesia.
Robinson, P. M. (1991). Testing for strong serial correlation and dynamic conditional heteroskedasticity in multiple regression. J. Econ. 47, 67–84. doi: 10.1016/0304-4076(91)90078-R
Rozaki, Z. (2021). Food security challenges and opportunities in Indonesia post COVID-19. Advances in food security and sustainability, 6, 119–168.
Saber, W. I. A., Ghanem, K. M., and El-Hersh, M. S. (2009). Rock phosphate solubilization by two isolates of Aspergillus niger and Penicillium sp. and their promotion to mung bean plants. Res. J. Microbiol., 4, 235–250.
Schlundt, J., Tay, M. Y. F., Chengcheng, H., and Liwei, C. (2020). “Food security: microbiological and chemical risks” in Global Health security: Recognizing vulnerabilities, creating opportunities. eds. A. J. Masys, R. Izurieta, and M. Reina Ortiz (Cham: Springer International Publishing), 231–274.
Setiadi, R., Artiningsih, A., Sophianingrum, M., and Satriani, T. (2022). The dimension of rural-urban linkage of food security assessment: an Indonesian case study. Asian Geogr. 39, 113–131. doi: 10.1080/10225706.2020.1768570
Shamim, A. H. M., Moniruzzaman, M., Siddiquy, M., and Shaibur, M. R. (2019). Status of some nutrients at Tejgaon soil series in 2001 and in 2018: A study from Bangladesh. Environ Biol Res, 1, 44–53.
Sheahan, M., and Barrett, C. B. (2017). Ten striking facts about agricultural input use in sub-Saharan Africa. Food Policy 67, 12–25. doi: 10.1016/j.foodpol.2016.09.010
Siegrist, M., and Hartmann, C. (2020). Consumer acceptance of novel food technologies. Nat. Food 1, 343–350. doi: 10.1038/s43016-020-0094-x
Slimane, M. B., Huchet-Bourdon, M., and Zitouna, H. (2016). The role of sectoral FDI in promoting agricultural production and improving food security. Int. Econ. 145, 50–65. doi: 10.1016/j.inteco.2015.06.001
Södersten, C.-J. H., Wood, R., and Hertwich, E. G. (2018). Endogenizing capital in MRIO models: the implications for consumption-based accounting. Environ. Sci. Technol. 52, 13250–13259. doi: 10.1021/acs.est.8b02791
Szenkovics, D., Tonk, M., and Balog, A. (2021). Can genetically modified (GM) crops act as possible alternatives to mitigate world political conflicts for food? Food Energy Secur. 10:e268. doi: 10.1002/fes3.268
Thanh, T., Ton, P. H., Hai, V. D., Luong, N. T., Hai, T. V., and Khanh, T. D. (2018). Detection of bacterial leaf blight resistance genes in indigenous glutinous rice landraces. J. hortic. plant res. 2, 1–9.
Theriault, V., Smale, M., and Haider, H. (2018). Economic incentives to use fertilizer on maize under differing agro-ecological conditions in Burkina Faso. Food Secur. 10, 1263–1277. doi: 10.1007/s12571-018-0842-z
Toma, L., Barnes, A., Sutherland, L. A., Thomson, S., Burnett, F., and Mathews, K. (2018). Impact of information transfer on farmers’ uptake of innovative crop technologies: a structural equation model applied to survey data. J. Technol. Transf. 43, 864–881. doi: 10.1007/s10961-016-9520-5
Torres-Reyna, O. (2007). Panel data analysis fixed and random effects using Stata (v. 4.2). Data Stat. Serv. Priceton Univ. 112:49.
Tsiafouli, M. A., Thébault, E., Sgardelis, S. P., de Ruiter, P. C., Van der Putten, W. H., Birkhofer, K., et al. (2015). Intensive agriculture reduces soil biodiversity across Europe. Glob. Chang. Biol. 21, 973–985. doi: 10.1111/gcb.12752
Tyczewska, A., Woźniak, E., Gracz, J., Kuczyński, J., and Twardowski, T. (2018). Towards food security: current state and future prospects of Agrobiotechnology. Trends Biotechnol. 36, 1219–1229. doi: 10.1016/j.tibtech.2018.07.008
Van Wesenbeeck, C. F. A., Keyzer, M. A., van Veen, W. C. M., and Qiu, H. (2021). Can China's overuse of fertilizer be reduced without threatening food security and farm incomes? Agric. Syst. 190:103093. doi: 10.1016/j.agsy.2021.103093
Vipham, J. L., Amenu, K., Alonso, S., Ndahetuye, J. B., Zereyesus, Y., Nishimwe, K., et al. (2020). No food security without food safety: lessons from livestock related research. Glob. Food Sec. 26:100382. doi: 10.1016/j.gfs.2020.100382
Wana, I. N., Johnny, A., and Nitty, K. (2021). Low level presence of GMO in Malaysia’s import: the alternative tolerance and its economic effects. J. Sustain. Sci. Manage. 16, 162–173. doi: 10.46754/jssm.2021.06.014
Wang, W., Gan, C., Le Trang, A. D., and Nguyen, Q. T. T. (2023). The decision to buy genetically modified foods in China: what makes the difference? Environ. Dev. Sustain. 2023:246. doi: 10.1007/s10668-023-03246-5
Watts, N., Amann, M., Ayeb-Karlsson, S., Belesova, K., Bouley, T., Boykoff, M., et al. (2018). The lancet countdown on health and climate change: from 25 years of inaction to a global transformation for public health. Lancet 391, 581–630. doi: 10.1016/S0140-6736(17)32464-9
Wooldridge, J. M. (2002). Econometric analysis of cross section and panel data. 108. Cambridge, MA: MIT Press, pp. 245–254.
Wooldridge, J. M. (2015). Introductory econometrics: A modern approach. Boston, MA: Cengage Learning.
Wu, L., Taylor, M. P., and Handley, H. K. (2017). Remobilisation of industrial lead depositions in ash during Australian wildfires. Sci. Total Environ. 599-600, 1233–1240. doi: 10.1016/j.scitotenv.2017.05.044
Xu, H., Hwan Lee, S., and Ho Eom, T. (2007). Introduction to panel data analysis. Miller/Handbook of Research Methods in Public Administration, pp. 571–590.
Xu, Z., Elomri, A., El Omri, A., Kerbache, L., and Liu, H. (2021). The compounded effects of COVID-19 pandemic and desert locust outbreak on food security and food supply chain. Sustain. For. 13:1063. doi: 10.3390/su13031063
Yaffee, R. (2003) A primer for panel data analysis. Connect: Information Technology at NYU, pp. 1–11.
Yang, Y., Sornaraj, P., Borisjuk, N., Kovalchuk, N., and Haefele, S. M. (2016). “Transcriptional network involved in drought response and adaptation in cereals” in Abiotic and Biotic Stress in Plants-Recent Advances and Future Perspectives. eds. A. K. Shanker and C. Shanker (London: IntechOpen), 3–29.
Yeoman, I. (2022). Ukraine, price and inflation. J. Rev. Pric. Manage. 21, 253–254. doi: 10.1057/s41272-022-00378-7
Keywords: food security, consumption of fixed capital, environmental temperature surges, arable land, agricultural land, fertilizers
Citation: Ashraf J, Jun M, Ali S, Ghufran M and Xiaobao P (2024) Zero-hunger through the lens of food security in populous Asia: pre and post-pandemic. Front. Sustain. Food Syst. 8:1210385. doi: 10.3389/fsufs.2024.1210385
Edited by:
Aleyda Pérez Herrera, National Council of Science and Technology (CONACYT), MexicoReviewed by:
Liliana Londono Hernandez, National Open and Distance University, ColombiaPing Fang, Guangxi Minzu University, China
Copyright © 2024 Ashraf, Jun, Ali, Ghufran and Xiaobao. This is an open-access article distributed under the terms of the Creative Commons Attribution License (CC BY). The use, distribution or reproduction in other forums is permitted, provided the original author(s) and the copyright owner(s) are credited and that the original publication in this journal is cited, in accordance with accepted academic practice. No use, distribution or reproduction is permitted which does not comply with these terms.
*Correspondence: Sumran Ali, c3VtcmFuYWxpQG1haWwudXN0Yy5lZHUuY24=
†These authors share first authorship