- 1Faculty of Agricultural and Environmental Sciences, Department of Aquaculture and Sea-Ranching, University of Rostock, Rostock, Germany
- 2Landgesellschaft Mecklenburg-Vorpommern mbH, Renewable Energies Section, Leezen, Germany
- 3Faculty of Agricultural and Environmental Sciences, Department of Waste-and Resource Management, University of Rostock, Rostock, Germany
In Germany, warm water Recirculating Aquaculture Systems (RAS) are generally integrated with biogas plants. The process sludge produced in aquaculture could be utilized to generate energy. This study investigates the potential of process sludge from commercial African catfish (Clarias gariepinus) warm water RAS, alongside associated plant production residues (whole plants and pruning residues) for energy generation through anaerobic digestion. Biogas tests, including batch, semi-Continuous Stirred Tank Reactor (CSTR), and Upflow Anaerobic Sludge Blanket Reactor (UASB) were conducted. In batch, methane yields were 229 L(N) / kg VS from the sludge and 173–184 L(N) / kg VS from the various plant substrates (cucumber, paprika, and tomato plants). During CSTR operation, mono-fermentation of sludge produced a methane yield of 265 L(N) / kg VS. Co-fermentation with 25% cucumber residues, based on VS, increased this value to 381 L(N) / kg VS. Mono-fermentation of sludge in the UASB reactor yielded a maximum of 329 L(N) /kg VS. The relatively low TS content and unfavorable C/N ratio in C. gariepinus sludge, along with the low energy density and occasional high sulfur content in the investigated plant substrates, present challenges for CSTR biogas production. These challenges can be partially mitigated through substrate combination. For mono-fermentation of African catfish RAS sludge, the UASB reactor is recommended. Improved solids separation, extraction, and concentration techniques at aquaculture operations are essential for the efficient utilization of aquaculture sludge, especially from African catfish, in biogas plants.
1 Introduction
Encouraged by the EU’s renewable energy policies the production of biogas has experienced a dynamic levitation in recent years. In 2015 total biogas output in the EU rose to 18 billion m3 methane, contributing to halve of the global biogas production (Scarlat et al., 2018). The German biogas industry is currently at a turning point: for the first plants, the 20-year compensation period is coming to an end, and continued economic operation, despite a 10-year (reduced) extension of the Renewable Energy Sources Act (RES/EEG) compensation, is economically often not feasible. This is aggravated by continuously increasing operational requirements. On basis of the recent geopolitical situation however, there is a compelling need for more energy self-sufficiency and a shift toward reliable, resilient and renewable sources independent from global markets and supply chains. Unfortunately, as of today, the share of fossil fuels in the global energy mix remains obstinately high at around 80% (International Energy Agency, 2022). While the long-term future energy-mix is predicted to be led by wind and solar power, biogas production can still provide a significant contribution. In the context of rising resource scarcity and competition for reasonable farmland use, the biogas industry would be well advised to expand its focus on residues and waste stream materials as substrate for anaerobic digestion rather than relying on primary agricultural crops suitable for human consumption.
Agricultural biogas facilities primarily use pig, cow, and chicken manure with the addition of co-substrates to enhance the content of organic material and produce more gas (Weiland, 2010). Solid wastes originating from uneaten feed and fecal matter are also generated in aquatic animal production (Dauda et al., 2019). Land based recirculating aquaculture systems (RAS) can help sustainably supply fish to the growing global population and mitigate overfishing of wild stocks. This method offers inherent advantages, including sophisticated water and solids treatment, distinguishing it from traditional aquaculture linked to open water bodies prone to environmental pollution. RAS has potential as a large-scale, environmentally sustainable source of aquatic protein (Wik et al., 2009). In Germany, RAS with warm-water species are almost always coupled to biogas plants (BGP) (Pasch and Palm, 2021). This offers various synergies: the BGP operator can take advantage of the combined heat and power (CHP) bonus for heat utilization or sell heat, the aquaculture plant obtains heat, and material cycles are closed by utilizing the aquaculture sludge in the BGP and/or as organic fertilizer for plant production in an agricultural setting. However, the volumetric and energetic share of aquaculture sludge in the total input of common biogas plants so far is very low.Aquaculture stands as the fastest-growing food production sector, with fed aquaculture representing 69.5% of the total 80 million-ton production worldwide (European Commission, 2021; FAO, 2021). In 2019, approximately 41.6 million tons of aquatic feeds were produced, with 10–30% ending up as fecal particulate matter (Chen and Malone, 1991; Global Feed Survey Alltech, 2022), translating to 4.16 to 12.48 million t annually. Despite this, only 5% of the world’s aquaculture production comes from RAS, a share projected to increase to 40% by 2030 (Hatchery International, 2018). Due to the high grade of technologization, RAS entail significant initial investments. Synergies are crucial for cost competitiveness, with heat being a vital cost factor in aquaculture (Le et al., 2020). Loss of low-cost heat supply, particularly via biogas plants, would severely impact existing operations. Utilizing waste heat, especially in warm-water RAS, is essential.
With 1,193 t African catfish (Clarias gariepinus, Burchell, 1822) was the most produced RAS species in 2019 and practically all facilities are linked to biogas plants utilizing CHP waste heat (BMEL, 2019). This species comes with desirable traits for aquaculture, as they grow fast, convert feed effectively, can breathe atmospheric air, thrive well in exceptionally high stocking densities and withstand unfavorable water conditions (Hossain et al., 1998; van de Nieuwegiessen et al., 2009; Schram et al., 2010; Palm et al., 2018). Their fecal sediments are mainly collected in open lagoons and used for field fertigation (Hänisch, 2021). Although this kind of treatment is to be preferred over direct disposal into receiving water bodies, it is assumed, that 17 to 30% of ingoing organic carbon is lost as methane into the atmosphere (Mirzoyan et al., 2010). Because African catfish RAS operators currently work with simple gravimetric sedimentation basins for mechanical filtration, the resulting sludge is characterized by elevated water content and a total solid (TS) fraction ranging from a mere 1–2%.
In Norwegian RAS, sludge dry matter contents of up to 13% have been achieved (Gebauer and Eikebrokk, 2006; Brod et al., 2017) through advanced filter technologies like microsieve drum filters and a more particular constitution of the feces from salmonid fish. Sikora et al. (2019) claim, that with more sophisticated technology, African catfish sludge could be brought up to as much as 6.2 to 8.1% TS. Scientific literature covers the use of aquaculture sludge for biogas production, particularly from cold water salmonid fish like salmon, or from brackish/saline water productions (Lanari and Franci, 1998; Gebauer, 2004; Gebauer and Eikebrokk, 2006; Brod et al., 2017). However, studies focusing on African catfish as a warm-and freshwater species are underrepresented (Goddek et al., 2018; Strauch et al., 2018). In aquaculture related studies, upflow anaerobic sludge blanket reactors (UASB), fixed bed reactors, or their modifications are commonly employed. They are well-suited for low solids substrates (Mainardis et al., 2020). Yogev et al., 2017 reported that UASB technology can save up to 12% of the energy needs of a RAS facility, while Mirzoyan and Gross (2013) assumed that 2–4% of its demand could be met. Because of its high-water content, biogas/methane yield of aquaculture sludge is rather low in relation to the volume input.
A promising future variant of aquaculture is the combination with horticultural production, called aquaponics (Palm et al., 2024). Residual material streams from aquaculture and plant production, such as aquaponics or stand-alone nearby greenhouse crop production, might offer promising energy potential for co-digestion (Zhu et al., 2021).
In UASB and related reactors, however, co-digestion with substrates rich in solids is only possible with a very high technical effort. Upstream hydrolysis and separation processes would be conceivable (Tassew et al., 2019). This study examines to what extent the widely used Continuous Stirred Tank Reactor (CSTR) reactor type can be used for African catfish RAS sludge. Prior to this, batch tests were carried out in order to determine basic and comparative data. An additional UASB test provides an indication of the methane yields of commercial African Catfish RAS sludge under mono-fermentation. Implications on the potential use of African catfish sludge in Biogas plants, without or in combination with plant production (aquaponics), are discussed.
2 Materials and methods
2.1 Substrates investigated
The primary substrate, AQ sludge, originated from an aquaculture facility in Mecklenburg-Western Pomerania producing around 300 t of African catfish annually. This sludge was extracted with a pond suction cleaner (Oase Pondovac 4, Conrad Electronics, Hirschau, Germany) from the sedimentation units before the daily cleaning procedure. The sludge suspension was placed in 60 L lidded barrels in a cooling chamber overnight at 4\u00B0C° to facilitate further sedimentation of solids. Through decantation of the supernatant after a 24 h rest in the barrels the dry matter content was increased and adjusted from 0.8–2% to 3.7–5.2%. The leftover sludge from different barrels was then thoroughly mixed to achieve a homogenous product.
To explore the potential benefits of integrated fish and plant production (aquaponics), residues from various common greenhouse crops were co-digested with the AQ-sludge. These residues were sourced from an agricultural company in Mecklenburg-Western Pomerania, producing 5,000 t of fruits and vegetables annually, mainly in greenhouse cultivation. They consisted of cuttings with stems and leaves (cucumber, tomato, and paprika) and pure leaves (tomato only), representing waste materials from ongoing production. All substrates were stored at-15°C until testing or feeding and gently thawed at room temperature before use and utilized in their original state without additional preparation. Only long plant components of the horticultural residues were coarsely shredded to a maximum length of 2 cm.
2.2 Batch experiment
Digested sewage sludge from the Central Wastewater Treatment Plant in Rostock, generally containing a diverse range of biogas-forming microorganisms, served as inoculum. Each batch fermenter (working volume 32.7 L) was mixed with the inoculation sludge to achieve a ratio of approximately 0.3 kg VS (S)/kg VS (I). To minimize oxygen influence, the headspace of the batch reactors was purged with nitrogen before starting the experiment. The experiment entailed triplicates, with pure inoculated sludge and cellulose included as controls. Operating under mesophilic conditions (38°C), the reactors were maintained at temperature in a heating chamber (Figure 1), and the suspension was regularly homogenized using a magnetic stirrer. Biogas generated during the experiment was collected in aluminum ironing bags and manually measured using a Visit 03 gas analyzer (Messtechnik EHEIM GmbH, Schwaigern, Germany). The amount and composition of biogas formed were measured daily, at the experiment’s start, and adjusted as needed with the decreasing rate of biogas formation. In addition to the AQ-sludge, all plant substrates were tested to gather fundamental data for the selection of the most promising candidates for co-digestion in the CSTR trial.
2.3 Continuous stirred tank reactor experiment
Laboratory fermenters, operated semi-continuously, were employed, each with a working volume of 20 liters and filled to a capacity of 10 liters. These fermenters were equipped with agitators featuring variable speed and adjustable agitation intervals. Manual feeding was performed. A bottom ball valve facilitated the removal of fermentation residues. The experimental setup and implementation adhered according to the Association of German Engineers (Verein Deutscher Ingenierure e.V, 2016) guideline 4,630 (Fermentation of organic materials-Characterization of the substrate, sampling, collection of material data, fermentation tests, 2016). Four digesters were grouped together in a shared heating chamber. The schematic structure of a fermenter is illustrated in Figure 2 (Table 1).
Fermenter contents of an agricultural biogas plant were used as inoculum. Alongside cattle manure and silage maize, the plant also uses small amounts of process water from a neighboring RAS, from where the sludge was obtained, too. The fresh mass ratio of inoculum to aquaculture sludge (AQ sludge) was 4:1, with the inoculum having a volatile solids (VS) content in the fresh mass (FM) of 7.7% and the AQ sludge of 3.2%. To minimize oxygen influence, the headspace was purged with nitrogen before the experiment commenced. The initial feeding, consisting of 10 g VS each of silage maize and aquaculture sludge, took place on the third day after filling, following a thermal adjustment period for the biocenosis. Regular feeding started 5 days later, starting on Monday (Table 2).
The experiments were conducted in fourfold repetition (in parallel). The fermenters were grouped and heated to mesophilic levels (38°C) using air heating. Key variables, including air pressure, temperature, and gas formation, were automatically recorded. Gas production was measured with a drum gas meter (TG 0.5), and biogas yield was continuously monitored using the data acquisition software RIGAMO (both from Dr.-Ing. RITTER Apparatebau GmbH & Co. KG, Bochum, Germany). Methane (CH4), carbon dioxide (CO2), hydrogen sulfide (H2S), and oxygen (O2) contents were measured three times a week (Mon, Wed, Fri) in the headspace. Methane and carbon dioxide levels were determined through infrared spectrometry, while electrochemical sensors were used for hydrogen sulfide and oxygen, using a Visit 03 gas analyzer (Messtechnik EHEIM GmbH, Schwaigern, Germany). Due to intensive upstream mixing, the properties of the digestate accurately represent those of the fermenter contents.
A Fibretherm FT 12 (C. Gerhardt GmbH and Co. KG, Königswinter, Germany) method compliant with van Soest fiber fractionation and Weender feed analysis was utilized to determine fiber content [Acidic Detergent Fibre (ADF), Neutral Detergent Fibre (NDF), Acid Detergent Lignin (ADL), and crude fiber (XF)] in the digestate. The analysis of organic acids was conducted using a gas chromatograph with flame ionization detector (GC/FID) Model 7820A (Agilent, Santa Clara, United States).
The ratio of volatile organic acids and reactor buffer capacity relative to calcium carbonate (FOS/TAC) was determined using TitraLab AT1000 (Hach-Lange GmbH, Düsseldorf, Germany). For trelevant basic parameters in aqueous solutions digestate samples werefilterted and centrifuged (0.45 μm at 6000 rpm), respectively, were measured by spectrophotometer Nanocolor (Machery-Nagel, Düren, Germany). Organic dry matter was determined using a muffle furnace at 550°C. For CNS analysis, an elemental analyzer VarioMAX CNS (Elementar Analysensysteme GmbH, Langenselbold, Germany) was used.
The individual fermenters were uniformly treated regarding feeding, temperature, and stirring interval (48/d). An adapted, distributed feeding approach was implemented based on the recommendation in Lincke and Schwarz (Liebetrau et al., 2013), specifying 160% of the mean feeding quantity on Monday, 80% on Tuesday and Wednesday, and 90% on Thursday and Friday. The weekly feeding amounted to 100 g VS, with a daily provision of 20 g VS per working weekday. This resulted in a room load of 0.2 kg VS/(m3d). The quantity and distribution were consistently maintained throughout the entire experiment. During the adaptation phase, the reactors were initially fed with silage maize. Additionally, potential residues from horticulture were explored as carbon sources. Based on the findings from the batch trial, tomato and cucumber plant residues were selected as co-digestion substrates. Throughout the experiment, the proportion of aquaculture sludge was incrementally increased until mono-digestion was achieved (Table 2). After 8 weeks of experimentation, the mono-fermentation phase lasted for 4 weeks, followed by co-digestion with tomato and cucumber plants.
2.4 Description of the UASB experiment
The utilized reactor was a combined UASB/EGSB bio-reactor manufactured by “aquaponic manufactur” from Issum, Germany. For this study, only the UASB reactor was operational, and the EGSB unit remained inactive. The test unit comprised the following components: a reaction chamber (UASB/EGSB), gas collection G1/G2, and the analytical areas 1–3 (Figure 3). The microbial processes took place within the reaction chamber, and its contents were recirculated by pumps (P1/P2) and heated to the set temperature using a heating element controlled by a thermostat. The UASB reactor had a working volume of 23.7 liters, with approximately 40% of this volume filled with spherical methane-producing microbial cultures, known as granules, obtained from an IC (internal circulation) reactor at a local sewage treatment plant. These granules were continuously circulated. Biogas production was monitored using a drum gas meter (1) from Dr.-Ing. Ritter KG with control and recording software on a computer (2). The produced gas was stored in a gas collecting ironing bag (3) for later analysis.
A crucial parameter for a stable process and optimal biogas yield and quality in UASB reactors is the organic loading. It was gradually reduced throughout the measurement series by diluting the original substrate, which decreased the VS content. The reactor was fed 5 days a week, and the experiment lasted 28 days. Table 3 presents the basic characteristics of the original substrate and the two dilutions.
3 Results and discussion
3.1 Substrate characterization
The optimal supply of fermentable organic dry matter is crucial for biogas formation. For simplicity, the proportion of organic dry matter was utilized in the present study. The substrates employed in the trials (Batch and CSTR) showed significant fluctuations in total solids (TS), ranging from 3.7 to 55.2%. However, the proportion of organic matter in the dry mass of these diverse substrates showed less variability, falling within the range of 72 to 85% (Table 4).
The determined C/N ratio of the African catfish sludge was consistent with values from previous research (C/N 7.4–7.9: 1 and 8–9: 1 in Prüter et al., 2020 and Strauch et al., 2018) however, its range was wider than the very narrow C/N ratio of sludge from salmon aquaculture reported by Brod et al., 2017 (C/N 2.8: 1; Brod et al., 2017). This also results from the enormous and rapidly changing stocking densities in African catfish RAS (Palm et al., 2018), changing the formation of solid sludge. Higher stocking densities increase the likelihood of anaerobic zones, leading to denitrification processes and resulting in the loss of molecular nitrogen into the atmosphere. As previously highlighted, sludge from African catfish farming has insufficient carbon content for the biogas process relative to the nitrogen content. This also holds true for the C/S- ratio. According to (Weinrich, 2017), a balanced nutrient ratio of C: N: P: S = 3,000: 50: 3: 1 to 600: 15: 5: 1 should be maintained for optimal biogas formation. However, the number of dedicated studies is scarce. A C/N ratio of 20 to 35 is considered optimal for anaerobic degradation (Puñal et al., 2000; Ceron-Vivas et al., 2019). Some authors define the optimal range to be somewhat narrower between 15 to 30 (Koch et al., 2017). The range of the optimal C/S ratio spans from 150 to 1,200.
A very narrow C/N ratio with high nitrogen or low carbon content poses the risk of ammonia inhibition, making it advisable to combine it with additional feedstock rich in carbon. Liquid manure has a similarly narrow C/N ratio to the AQ sludge studied [cattle slurry: 8:1, pig slurry: 10:1 (Koch et al., 2017)]. Co-digesting AQ sludge with substrates rich in carbon and simultaneously low in nitrogen and sulfur can help achieving more balanced ratios. Therefore, silage maize (C/N ratio 31:1) and the available residual plant materials were utilized as co-substrates in the trials. As depicted in Table 4, the use of horticultural co-substrates can enhance the nutrient ratios only marginally. Silage maize, with its comparatively wide C/N ratio of 31:1 and very wide C/S ratio of 862:1 (due to a very low sulfur content) has a better balancing effect. This suggests an optimal use of the African catfish sludge combined with silage maize or other plant residues with wide C/N and C/S ratios.
3.2 Results batch-trial
The biogas formation followed a typical pattern. Table 5 lists the cumulative and averaged results, which are presented both in terms of the original substance (fresh matter) and in terms of the organic dry mass. The horticultural (whole plants) substrates showed a methane yield between 173 and 184 standard liters per kilogram of organic dry matter input (L (N)/kg VS). Tomato leaves produced the lowest methane yield relative to the organic dry matter contained. The methane yield of the AQ sludge alone reached a significantly higher value of 229 L (N)/kg VS. These results fall somewhat midrange among values reported in the literature. Biochemical methane potentials reach from 124–320 to 88–280 L (N) CH4/kg VS for whole tomato and cucumber plant residues, respectively, (Jagadabhi et al., 2011; Oleszek et al., 2016; Li et al., 2018; Wang et al., 2018; Szilágyi et al., 2021). Methane yield from whole paprika plants was reportedly lower at 121 L(N) CH4/kg VS (Li et al., 2018). However, anaerobic digestion of pure fruit residues proved to be more productive with methane yields of 210, 348 and 354 L(N) CH4/kg VS for tomato, cucumber, and paprika fruit leftovers, respectively (Shin et al., 2011). The same authors reported 268 L (N) CH4/kg VS from pure tomato leaf material, contrasting with the 154 L (N) CH4/kg VS in the present experiment.
Conducting direct comparisons between the findings of this study and values documented in existing literature presents challenges attributable to several factors. For the above-mentioned studies, it remains partially uncertain if substrates were carefully segregated. Variations in inocula and pre-treatments, such as crushing, have a substantial impact on methane yields. Furthermore, the proportion of fruit material will influence degradation and methane production, as it contains less lignin (Lowe et al., 2020) and more simple carbohydrates (Morales-Polo et al., 2019). For instance, the BMP of pure cucumber fruit material was found to be 648 L (N)/kg VS (Lowe et al., 2020). In the present study, only very few old and partially broken residual fruits were left in the substrates declared as whole plant material (leaves and stems).
Typically, biogas has a methane content of 50 to 75% (Fachagentur Nachwachsende Rohstoffe e.V. (FNR), 2016). The methane contents of the biogas investigated during fermentation of the substrates in the present study ranged from 54 to 66% (in dry gas, Table 5). Since the biogas, as well as the methane yield of the tomato leaves, just reached that of the inoculum, this material was not used as substrate in the further trials. Cucumber and tomato whole plant material yielded the highest methane output and were therefore used as co-substrates for digestion inside the CSTR-reactors.
The hydrogen sulfide (H2S) contents in the raw biogas provide an indication of the sulfur content of the substrates investigated. They reached maximum values of 1,260 ppm for whole paprika plants, followed by tomato leaves and whole plants with 893 and 260 ppm, respectively. The maximum hydrogen sulfide contents of the other samples ranged from about 50 to 135 ppm. In the headspace, hydrogen sulfide and water vapor combine to form sulfuric acid, posing challenges for biogas utilization. With an increased sulfur content of the applied substrates, higher biogas conditioning costs for internal or external desulfurization must be expected. H2S-content of 200–500 ppm is referred to as a tolerable low load (Khoshnevisan et al., 2017). Since its use as a co-substrate is assumed, the sulfur content of the horticultural residual materials is not considered critical. However, in the case of higher proportions, it must be monitored and potential desulfurization must be adjusted if necessary.
3.3 Results CSTR-trial
With an increasing input of AQ sludge, the water content in the fermenter increased as expected, significantly influencing the process parameters. The evolution of dry matter content in individual digesters is depicted in Figure 4. The initial dry matter (inoculum + sludge) was 8%. During the run of the experiment, it decreased inside the fermenter from initially 7.3 to approx. 3.0% during the mono-digestion of the sludge. This decrease is attributed to the water-rich AQ sludge and the biological degradation of the organic mass. However, from the curve’s pattern, a stabilization of the dry mass content can be observed.
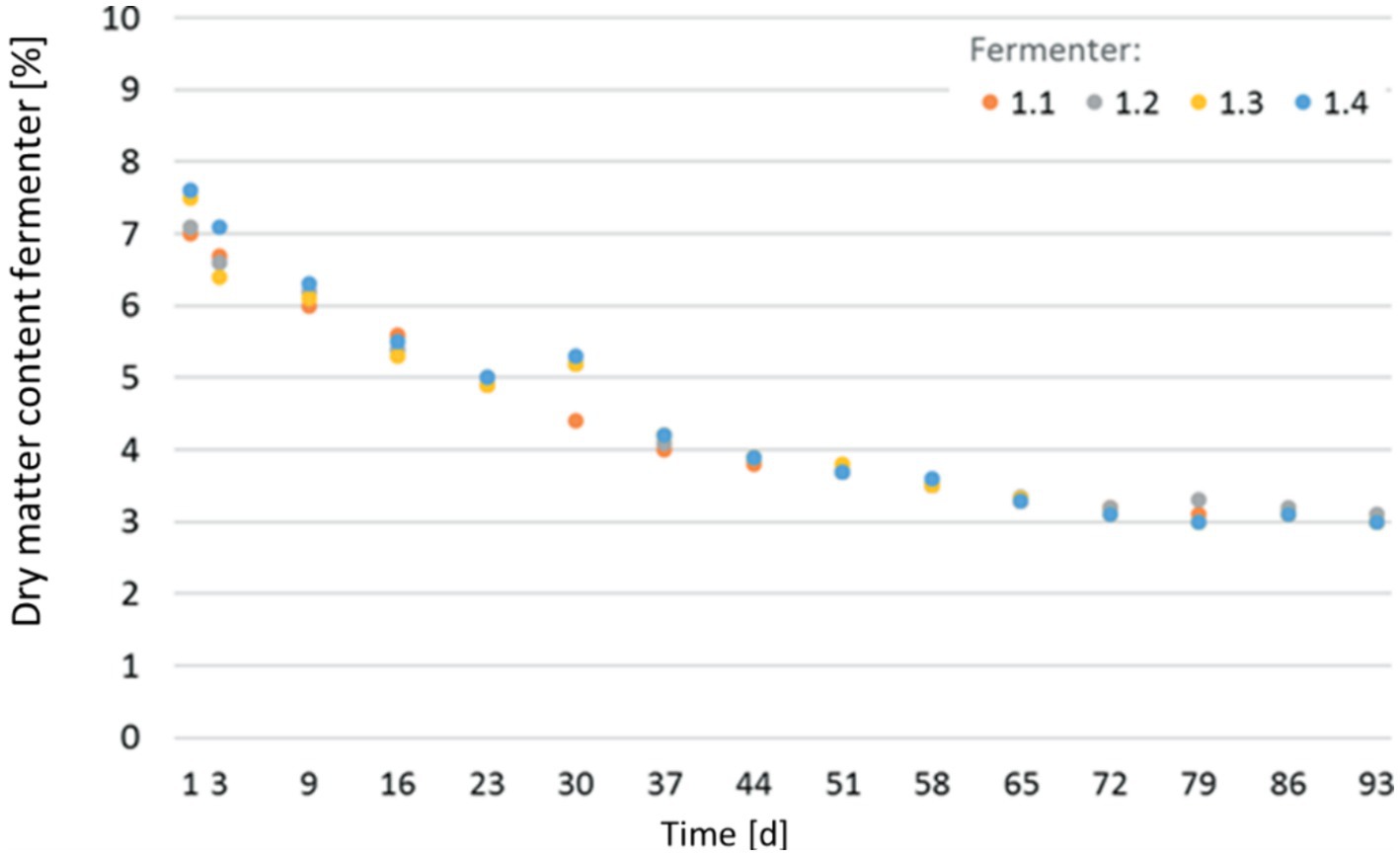
Figure 4. Development of dry matter content in the individual digesters over the course of the CSTR Trial.
Even at high water contents (highest during mono-fermentation), a stable biogas formation process was achieved. The FOS/TAC value, indicating the assessment of the feeding intensity, was consistently low at 0.21 right from the beginning. The target value for a stable operation is a FOS/TAC value of 0.3 to 0.5 [Fachagentur Nachwachsende Rohstoffe e.V. (FNR), 2016]. Throughout the trial, the FOS/TAC oscillated around 0.21, dropping to 0.18 in the phase of mono-digestion of the AQ sludge. Subsequent co-digestion with tomato or cucumber plant residues slightly increased the value to 0.19. Accordingly, the fermenter biology faced a comparatively low supply of fermentable biomass. The pH value changed only slowly during the run of the experiment, remaining in the nearly neutral range between 7.3 and 7.9. Lower values of below 7 were observed for short periods.
Due to the allocated feeding and the weekly fill level correction, the daily feeding quantity varied from 0.13 to 0.28 kg VS/m3 d (Mon-Fri weekly average 0.20 kg VS/m3 d and Mon-Sun weekly average 0.14 kg VS/m3 d). As anticipated, the hydraulic retention time was low due to the high-water input. It decreased on a weekly average from 43 days at the trial’s beginning to 31 days during mono-digestion. A value of 36 days is typical for a biogas plant with a high proportion of liquid manure [Fachagentur Nachwachsende Rohstoffe e.V. (FNR), 2016]. As the substrate exchange rate increases, relevant microorganisms for biogas formation are washed out. At a certain point, the re-growing microorganism biomass is no longer sufficient to break down the supplied substrate, leading to a decrease in biogas or methane yield. Since substrate and microorganism compositions are always specific, no universally applicable minimum residence time can be determined. In the presented experiments, a decrease in specific biogas formation was observed, analogous to the described effect, though the observed reduction was less than originally expected.
A typical curve of the specific methane yield of the four digesters with allocated feeding is illustrated by Figure 5. The consistently lower values of reactor 1 (F11) are clearly visible. Troubleshooting revealed a leak at a connection point, which had no influence on the biocenosis. This is confirmed by the fact that the measured contents in the biogas, as well as fermenter contents, principally corresponded to those of the other reactors. However, this leakage could not be eliminated during the trial without causing serious process disturbances. As a result, the averaging of the specific biogas and methane generation did not include this reactor.
A specific methane yield of 265 L(N)/kg VS was determined in the CSTR experiment during mono-digestion. This was higher than the 229 L(N)/kg VS found in the batch experiment and comparable to values found in the literature, as reported by Gebauer and Eikebrokk (2006) (0.260–0.281 L/g VS added; salmon aquaculture, CSTR). However, the very high methane yields for AQ sludge from rainbow trout of 318 ± 29 mL CH4/g VS (Batch-BMP; Suhr et al., 2015) or 400 to 460 mL CH4/g VS (fixed bed reactor; Lanari and Franci, 1998) were not achieved. Differences in the mechanical filtration units of the aquaculture facilities (i.e., sedimentation vs. drum/sieve filters) and therefore longer resting times with inherent leaching and energy loss of the sludge can be considered. Moreover, within African catfish RAS, the notably high stocking densities contribute to denitrification in anoxic zones, consequently altering the C/N ratios. Additionally, it is essential to account for various factors such as metabolism, feed conversion efficiency, composition, and energy content of the input feed, which vary between fish species (e.g., Salmonids vs. African catfish).
The methane yield of the investigated AQ sludge was comparable to that of liquid manure. Cattle manure has an average methane yield of 210 Nm3/t VS (range 110–275), pig manure of 250 Nm3/t VS (range 180–360), and silage maize of 340 Nm3/t VS [range 234–364) (FNR), 2016]. Co-digestion with silage maize and AQ sludge yielded an average methane output of more than 280 L(N)/kg VS. When comparing weekly measurements, it could be observed that the methane yield of the AQ sludge mono-digestion was only around 15% lower than co-digestion with 12.5 to 37.5 percent silage maize (Figure 6).
Co-digestion of AQ sludge with horticultural plant residues followed the four-week mono-digestion of AQ sludge. Process inhibition during mono-fermentation could not be observed, in contrast to the experiments in (Gebauer and Eikebrokk, 2006). However, with a higher volumetric load, longer mono-fermentation and accumulation effects, this impact cannot be ruled out.
Comparatively high methane yields of 381 L(N)/kg VS were achieved with the addition of cucumber plant residues (Table 6). This substantial increase in methane yield can be attributed to positive effects of balanced nutrient ratios and the balancing of deficiency symptoms. However, the minimal balancing of the C/N ratio alone cannot explain the observed increase in specific methane yield. A further explanation might be subsequent changes in the biocenosis after adding of cucumber substrates to the reactors. Wang et al. (2018) found the share of added cucumber residues changed the bacteria and archea species composition and diversity, which was positively correlated with methane yields.
The reduced methane yield in the co-digestion of tomato plant residues could be due to an inhibitory effect of potentially toxic compounds such as α-solanine or α-tomatin contained in tomato plants (Janick, 2001) or other inhibitory substances, which could also explain the low yield in the batch experiment. Restricted biodegradability of the carbon contained or N-inhibition could be also conceivable. Nonetheless, the negative effect of α-tomatin on the biogas yield was confirmed in an earlier batch-fermentation experiment by Szilágyi et al. (2021).
During the course of the experiment, an increase in methane content from 53% to up to 59% (56.9–58.7%) was observed during mono-digestion with an increase in the AQ sludge content (Figure 7). The lower contents of fermenter 4 stand out, for reasons that cannot be explained. In the subsequent co-digestion, the methane content in the biogas decreased to 56.6% (56.0–56.9%), which can be explained by the increased carbon dioxide formation from the decomposition of the increased carbohydrate content. The methane content in the biogas from digester 4 at this stage is at the same level as the other digester.
3.4 Results UASB trial
In total, 84.6 liters of biogas were converted from 438.45 g VS over 28 days. It is noticeable that the first week produced much lower methane contents compared to the 4th week (Table 7; Figure 7). As the dry matter content in the aquaculture sludge decreased due to dilutions of the original substance, there was an observable increase in biogas yield and methane content. The biogas yield rose from 112 (N)L/kg VS to 515 (N)L/kg VS. Simultaneously, the methane yield increased from 49 to 329 (N)L/kg VS. The methane content in the biogas grew from 43.5 to 63.9%. Particularly noteworthy is the significant surge in both biogas and methane formation during the final week of the test series. Various studies support these findings, as they indicate that increasing loading rates lead to a decrease in biogas and methane yields (Torkian et al., 2003; Chaisri et al., 2007; Yun et al., 2016; Magdalena et al., 2020). At higher organic loading rates, accumulation effects cause inhibitions in the process and the added organic dry matter cannot be efficiently decomposed, leading to accumulation. The ongoing recovery after the reduction of the organic load was likely reflected in the yields in weeks 2 and 3. This process was stable in week 4., as supported by the values from the accompanying analysis (Figure 8).
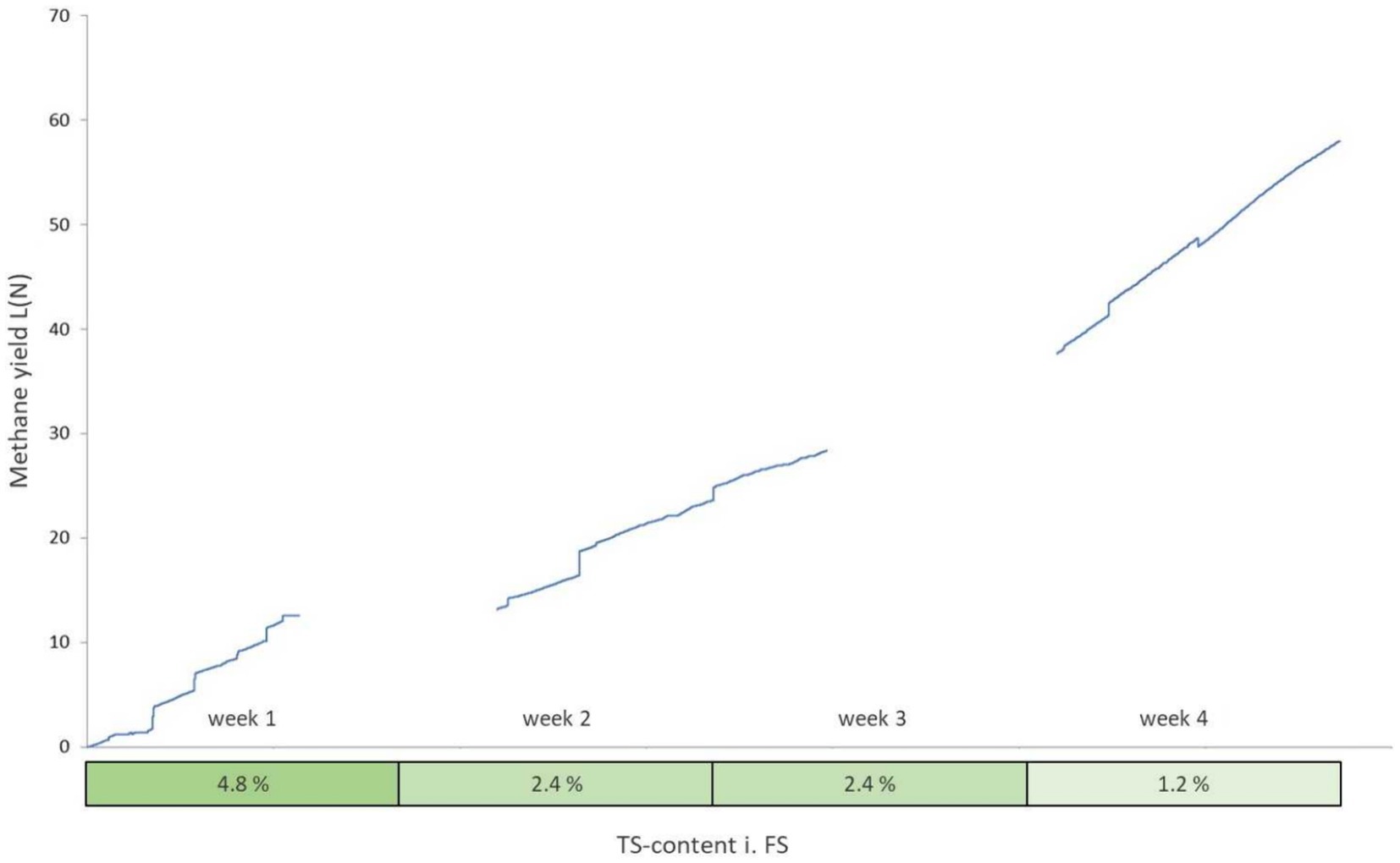
Figure 8. Cumulative methane yield over the course of the UASB trial (gaps represent measurement errors).
The FOS/TAC ratio increased significantly from the start of the experiment with increasing room load, rising from an initial value of 0.836 to 1.585. This indicates excessive feeding intensity. The free organic acid content increased sharply, in contrast to the substantial decrease in carbon buffer capacity. This value slowly recovered after reducing the room load to 1.372 in week 3 and 0.636 in week 4, remaining stable thereafter. The pH value changed only gradually throughout the experiment and remained in the almost neutral range between 7.09 and 7.84, with lower values measured for a short period. This could also be an indication of an increasingly poor C/N ratio. As mentioned before narrow C/N ratios (substrates with little carbon) bear the risk of inhibition by ammonia, although this was not supported by the accompanying analysis.
With a maximum methane production of 329 L/kg VS, the present trial established a new peak value in UASB treatment of AQ sludge, surpassing previous studies where CH4 yields were considerably lower. It is important to note that those studies are limited and primarily focused on treating brackish/saline water AQ sludge (Mirzoyan et al., 2008; Mirzoyan and Gross, 2013). Additionally, it remains to be demonstrated whether long-term operation with consistent yields can be achieved. Comparing the results from the present study with the literature, particularly in the context of batch and CSTR trials, it is evident that the African catfish AQ sludge with low TS content can be more energetically advantageous when utilized in UASB reactors. Satisfactory results, especially at loads below 1 kg VS m3*d, can be attained. It is worth mentioning that the substrate used for the UASB trial was not exactly the same batch as in the other trials. However, VS content of 3.7% of FS falls within the range observed in both, the Batch and CSTR trials (2.8–4.5% of FS). Given that samples were obtained from a commercial catfish farm, variations or rapid changes in stocking densities are common (Palm et al., 2018), leading to major fluctuations in the amount of fish feed introduced into the system. Moreover, changes in the brand or type of fish feed can occur regularly due to the volatile nature of the market and susceptibility to price fluctuations (Hardy, 2010; Luthada-Raswiswi et al., 2021). Given that feeding non-thickened sludge also requires larger reactor volumes and space, for future studies, a comparative techno-economic analysis of CSTR and UASB systems would be of interest.
4 Conclusion
African catfish sludge can be used for biogas production and energy generation. This research, provides data on the anaerobic digestion of AQ sludge with constant methane production, offering a potential contribution to the energy requirements of aquaculture facilities. In the context of the CSTR technology, co-digestion with substrates featuring a narrow C/N ratio and higher VS content can be recommended. Suitable options include horticultural or agricultural residues, such as those from cucumber production. Silage maize is to be considered an ideal choice. However, for structurally rich substrates, higher processing costs and the identification of potentially inhibiting substances must be factored in, possibly including desulfurization. Phytosanitary aspects must be examined in the case of a conceivable circulation of the fermentation residues in horticulture or aquaponics. Methods for dewatering Catfish sludge seem to be essential on order to reasonably harness its energetic potential in CSTR fermentation. For non-thickened sludge, UASB fermentation is a viable option. Sludges with around 1% TS content, as obtained in the present study, are suitable for direct utilization in UASB. The approximate percentage of total energy recovery from AQ-Sludge via methane production was 41% for Batch, 48% for CSTR, and 60% for UASB treatments, respectively. As land-based aquaculture production continues to grow, effective effluent treatment and reasonable sludge utilization will become crucial for the sustainable persistence of the industry.
Data availability statement
The original contributions presented in the study are included in the article/supplementary material, further inquiries can be directed to the corresponding author.
Author contributions
JK: Conceptualization, Data curation, Investigation, Writing – original draft, Writing – review & editing, Formal analysis, Methodology. AS: Conceptualization, Formal analysis, Methodology, Project administration, Supervision, Visualization, Writing – original draft, Writing – review & editing. SF: Conceptualization, Data curation, Formal analysis, Visualization, Writing – original draft, Investigation. JS: Conceptualization, Methodology, Resources, Supervision, Writing – review & editing. AB: Conceptualization, Data curation, Supervision, Validation, Writing – review & editing. MN: Conceptualization, Funding acquisition, Project administration, Resources, Supervision, Writing – review & editing. HP: Conceptualization, Funding acquisition, Project administration, Resources, Supervision, Writing – original draft, Writing – review & editing.
Funding
The author(s) declare that financial support was received for the research, authorship, and/or publication of this article. This project was funded through the project “Performance and process water management in commercial (integrated) aquaculture systems with African catfish (Clarias gariepinus) in Mecklenburg–Western Pomerania” (MV-II.1-LM-007: 139030000103, EMFF: 7302).
Acknowledgments
We want to thank Kersten Eckermann (Bioenergietechnikum, Universität Rostock) for the technical support. We would like to thank regional African catfish producers in Mecklenburg–Western Pomerania for providing access and information. In particular we want to thank Sukower Bioenergie und Welsfarm GmbH and operational manager Kalle Hänisch.
Conflict of interest
The authors declare that the research was conducted in the absence of any commercial or financial relationships that could be construed as a potential conflict of interest.
Publisher’s note
All claims expressed in this article are solely those of the authors and do not necessarily represent those of their affiliated organizations, or those of the publisher, the editors and the reviewers. Any product that may be evaluated in this article, or claim that may be made by its manufacturer, is not guaranteed or endorsed by the publisher.
References
BMEL (2019). Jahresbericht zur Deutschen Binnenfischerei und Binnenaquakultur 2019. Bonn, Germany: Bundesministerium für Ernährung und Landwirtschaft, 47.
Brod, E., Oppen, J., Kristoffersen, A. Ø., Haraldsen, T. K., and Krogstad, T. (2017). Drying or anaerobic digestion of fish sludge: nitrogen fertilisation effects and logistics. Ambio Springer Netherlands 46, 852–864. doi: 10.1007/s13280-017-0927-5
Ceron-Vivas, A., Cáceres-Cáceres, K. T., Rincón-Pérez, A., and Cajigas, A. A. (2019). Influence of pH and the C/N ratio on the biogas production of wastewater. Cronaca del Magnetismo Anim. 92, 70–79. doi: 10.17533/udea.redin.20190627
Chaisri, R., Piyarat, B., Prasertsan, P., and Sumate, C. (2007). Effect of organic loading rate on methane and volatile fatty acids productions from anaerobic treatment of palm oil mill effluent in UASB and UFAF reactors. Songklanakarin J. Sci. Technol. 29, 311–323,
Chen, S., and Malone, R. F. (1991) Suspended solids control in recirculating Aquacultural systems. In: Northeast regional agricultural engineering service. Engineering aspects of intensive aquaculture. Proceedings from the aquaculture symposium. New York, pp. 170–186.
Dauda, A. B., Ajadi, A., Tola-Fabunmi, A. S., and Akinwole, A. O. (2019). Waste production in aquaculture: sources, components and managements in different culture systems. Aquac Fish 4, 81–88. doi: 10.1016/j.aaf.2018.10.002
European Commission. (2021). “Directorate-general for maritime affairs and fisheries,” in A new strategic vision for sustainable aquaculture production and consumption in the European union - blue farming in the European Green Deal. Publications Office of the European Union.
Fachagentur Nachwachsende Rohstoffe e.V. (FNR) (2016). Leitfaden Biogas. Von der Gewinnung zur Nutzung: Gulzow Fachagentur Nachwachsende Rohstoffe ISBN 3-00-014333-5.
FAO (2021). The state of world fisheries and aquaculture 2020. Sustainability in action. Rome Inform. doi: 10.4060/ca9229en
Gebauer, R. (2004). Mesophilic anaerobic treatment of sludge from saline fish farm effluents with biogas production. Bioresour. Technol. 93, 155–167. doi: 10.1016/j.biortech.2003.10.024
Gebauer, R., and Eikebrokk, B. (2006). Mesophilic anaerobic treatment of sludge from salmon smolt hatching. Bioresour. Technol. 97, 2389–2401. doi: 10.1016/j.biortech.2005.10.008
Global Feed Survey Alltech (2022). Available at: https://www.alltech.com/2021-global-feed-survey (Accessed January 22, 2023).
Goddek, S., Delaide, B. P. L., Joyce, A., Wuertz, S., Jijakli, M. H., Gross, A., et al. (2018). Nutrient mineralization and organic matter reduction performance of RAS-based sludge in sequential UASB-EGSB reactors. Aquac. Eng. 83, 10–19. doi: 10.1016/j.aquaeng.2018.07.003
Hardy, R. W. (2010). Utilization of plant proteins in fish diets: effects of global demand and supplies of fishmeal. Aquac. Res. 41, 770–776. doi: 10.1111/j.1365-2109.2009.02349.x
Hatchery International (2018). Strong forecast for RAS. Available at: https://www.hatcheryinternational.com/strong-forecast-for-ras-3183/ (Accessed February 25, 2023).
Hossain, M. A. R., Beveridge, M. C. M., and Haylor, G. S. (1998). The effects of density, light and shelter on the growth and survival of African catfish (Clarias gariepinus Burchell, 1822) fingerlings. Aquaculture 160, 251–258. doi: 10.1016/S0044-8486(97)00250-0
International Energy Agency (2022). Executive summary–world energy outlook 2022–analysis–IEA. Available at: https://www.iea.org/reports/world-energy-outlook-2022/executive-summary (Accessed January 15, 2023).
Jagadabhi, P. S., Kaparaju, P., and Rintala, J. (2011). Two-stage anaerobic digestion of tomato, cucumber, common reed and grass silage in leach-bed reactors and upflow anaerobic sludge blanket reactors. Bioresour. Technol. 102, 4726–4733. doi: 10.1016/j.biortech.2011.01.052
Khoshnevisan, B., Tsapekos, P., Alfaro, N., Díaz, I., Fdz-Polanco, M., Rafiee, S., et al. (2017). A review on prospects and challenges of biological H2S removal from biogas with focus on biotrickling filtration and microaerobic desulfurization. Biofuel Res. J. 4, 741–750. doi: 10.18331/BRJ2017.4.4.6
Koch, K., Post, M., Auer, M., and Lebuhn, M. (2017) Einsatzstoffspezifische Besonderheiten in der Prozessführung. Biogas Forum Bayern III, p. 18.
Lanari, D., and Franci, C. (1998). Biogas production from solid wastes removed from fish farm effluents. Aquat. Living Resour. 11, 289–295. doi: 10.1016/S0990-7440(98)80014-4
Le, A. T., Wang, L., Wang, Y., Vu, N. T., and Li, D. (2020). Experimental validation of a low-energy-consumption heating model for recirculating aquaponic systems. Energies 13:1958. doi: 10.3390/en13081958
Li, Y., Lu, J., Xu, F., Li, Y., Li, D., Wang, G., et al. (2018). Reactor performance and economic evaluation of anaerobic co-digestion of dairy manure with corn Stover and tomato residues under liquid, hemi-solid, and solid state conditions. Bioresour. Technol. 270, 103–112. doi: 10.1016/j.biortech.2018.08.061
Liebetrau, J., Pfeiffer, D., and Thrän, D. (2013) Messmethodensammlung Biogas Methoden zur Bestimmung von analytischen und prozessbeschreibenden Parametern im Biogasbereich. Messmethodensammlung Biogas Methoden zur Bestimmung von analytischen und prozessbeschreibenden Parametern im Biogasbereich, No. 213.
Lowe, T. B., Hatch, B. T., Antle, C., Nartker, S., and Ammerman, M. L. (2020). ‘One- and two-stage anaerobic co-digestion of cucumber waste and sewage sludge. Environ. Technol. 41, 3157–3165. doi: 10.1080/09593330.2019.1601262
Luthada-Raswiswi, R., Mukaratirwa, S., and O’brien, G. (2021). Animal protein sources as a substitute for fishmeal in aquaculture diets: a systematic review and meta-analysis. Appl. Sci. 11:854. doi: 10.3390/app11093854
Magdalena, J. A., Greses, S., and González-Fernández, C. (2020). Anaerobic degradation of protein-rich biomass in an UASB reactor: organic loading rate effect on product output and microbial communities dynamics. J. Environ. Manag. 274:111201. doi: 10.1016/j.jenvman.2020.111201
Mainardis, M., Buttazzoni, M., and Goi, D. (2020). Up-flow anaerobic sludge blanket (Uasb) technology for energy recovery: a review on state-of-the-art and recent technological advances. Bioengineering 7:43. doi: 10.3390/bioengineering7020043
Mirzoyan, N., and Gross, A. (2013). Use of UASB reactors for brackish aquaculture sludge digestion under different conditions. Water Res. 47, 2843–2850. doi: 10.1016/j.watres.2013.02.050
Mirzoyan, N., Parnes, S., Singer, A., Tal, Y., Sowers, K., and Gross, A. (2008). Quality of brackish aquaculture sludge and its suitability for anaerobic digestion and methane production in an upflow anaerobic sludge blanket (UASB) reactor. Aquaculture 279, 35–41. doi: 10.1016/j.aquaculture.2008.04.008
Mirzoyan, N., Tal, Y., and Gross, A. (2010). Anaerobic digestion of sludge from intensive recirculating aquaculture systems: review. Aquaculture 306, 1–6. doi: 10.1016/j.aquaculture.2010.05.028
Morales-Polo, C., Cledera-Castro, M. D. M., and Soria, B. Y. M. (2019). Biogas production from vegetable and fruit markets waste-compositional and batch characterizations. Sustainability (Switzerland) 11, 1–22. doi: 10.3390/su11236790
Oleszek, M., Tys, J., Wiącek, D., Król, A., and Kuna, J. (2016). The possibility of meeting greenhouse energy and CO2 demands through utilisation of cucumber and tomato residues. Bioenergy Res. 9, 624–632. doi: 10.1007/s12155-015-9705-z
Palm, H. W., Knaus, U., and Kotzen, B. (2024). Aquaponics nomenclature matters: it is about principles and technologies and not as much about coupling. Rev. Aquac. 16, 473–490. doi: 10.1111/raq.12847
Palm, H. W., Knaus, U., Wasenitz, B., Bischoff, A. A., and Strauch, S. M. (2018). Proportional up scaling of African catfish (Clarias gariepinus Burchell, 1822) commercial recirculating aquaculture systems disproportionally affects nutrient dynamics. Aquaculture 491, 155–168. doi: 10.1016/j.aquaculture.2018.03.021
Pasch, J., and Palm, H. W. (2021). Economic analysis and improvement opportunities of African catfish (Clarias gariepinus) aquaculture in northern Germany. Sustainability (Switzerland) 13:569. doi: 10.3390/su132413569
Prüter, J., Strauch, S. M., Wenzel, L. C., Klysubun, W., Palm, H. W., and Leinweber, P. (2020). Organic matter composition and phosphorus speciation of solid waste from an African catfish recirculating aquaculture system. Agriculture 10, 1–14. doi: 10.3390/agriculture10100466
Puñal, A., Trevisan, M., Rozzi, A., and Lema, J. M. (2000). Influence of C:N ratio on the start-up of up-flow anaerobic filter reactors. Water Res. 34, 2614–2619. doi: 10.1016/S0043-1354(00)00161-5
Scarlat, N., Dallemand, J. F., and Fahl, F. (2018). Biogas: developments and perspectives in Europe. Renew. Energy 129, 457–472. doi: 10.1016/j.renene.2018.03.006
Schram, E., Roques, J. A. C., Abbink, W., Spanings, T., de Vries, P., Bierman, S., et al. (2010). The impact of elevated water ammonia concentration on physiology, growth and feed intake of African catfish (Clarias gariepinus). Aquaculture 306, 108–115. doi: 10.1016/j.aquaculture.2010.06.005
Shin, K.-S., Kim, C. H., Lee, S. E., and Yoon, Y. M. (2011). Biochemical methane potential of agricultural byproduct in greenhouse vegetable crops. Korean J. Soil Sci. Fertil. 44, 1252–1257. doi: 10.7745/kjssf.2011.44.6.1252
Sikora, J., Niemiec, M., Szeląg-Sikora, A., Mudryk, K., Kurpaska, S., Latała, H., et al. (2019). Evaluation of the properties of waste from African catfish (Clarias gariepinus B.) farming in the context of using it for agricultural purposes. IOP Conf. Ser. Earth Environ. Sci. 214:2034. doi: 10.1088/1755-1315/214/1/012034
Strauch, S. M., Wenzel, L., Bischoff, A., Dellwig, O., Klein, J., Schüch, A., et al. (2018). Commercial African catfish (Clarias gariepinus) recirculating aquaculture systems: assessment of element and energy pathways with special focus on the phosphorus cycle. Sustain. For. 10:1805. doi: 10.3390/su10061805
Suhr, K. I., Letelier-Gordo, C. O., and Lund, I. (2015). Anaerobic digestion of solid waste in RAS: effect of reactor type on the biochemical acidogenic potential (BAP) and assessment of the biochemical methane potential (BMP) by a batch assay. Aquac. Eng. 65, 65–71. doi: 10.1016/j.aquaeng.2014.12.005
Szilágyi, Á., Bodor, A., Tolvai, N., Kovács, K. L., Bodai, L., Wirth, R., et al. (2021). A comparative analysis of biogas production from tomato bio-waste in mesophilic batch and continuous anaerobic digestion systems. PLoS One 16, 1–17. doi: 10.1371/journal.pone.0248654
Tassew, F. A., Bergland, W. H., Dinamarca, C., Kommedal, R., and Bakke, R. (2019). Granular sludge bed processes in anaerobic digestion of particle-rich substrates. Energies 12, 1–20. doi: 10.3390/en12152940
Torkian, A., Eqbali, A., and Hashemian, S. J. (2003). The effect of organic loading rate on the performance of UASB reactor treating slaughterhouse effluent. Resour. Conserv. Recycl. 40, 1–11. doi: 10.1016/S0921-3449(03)00021-1
van de Nieuwegiessen, P. G., Olwo, J., Khong, S., Verreth, J. A. J., and Schrama, J. W. (2009). Effects of age and stocking density on the welfare of African catfish, Clarias gariepinus Burchell. Aquaculture 288, 69–75. doi: 10.1016/j.aquaculture.2008.11.009
Verein Deutscher Ingenierure e.V. (2016). Gesellschaft Energie und umwelt (VDI 4630), fermentation of organic materials-characterization of the substrate, sampling, collection of material data, fermentation tests.
Wang, Y., Li, G., Chi, M., Sun, Y., Zhang, J., Jiang, S., et al. (2018). Effects of co-digestion of cucumber residues to corn Stover and pig manure ratio on methane production in solid state anaerobic digestion. Bioresour. Technol. 250, 328–336. doi: 10.1016/j.biortech.2017.11.055
Weiland, P. (2010). Biogas production: current state and perspectives. Appl. Microbiol. Biotechnol. 85, 849–860. doi: 10.1007/s00253-009-2246-7
Wik, T. E. I., Lindén, B. T., and Wramner, P. I. (2009). Integrated dynamic aquaculture and wastewater treatment modelling for recirculating aquaculture systems. Aquaculture 287, 361–370. doi: 10.1016/j.aquaculture.2008.10.056
Yogev, U., Sowers, K. R., Mozes, N., and Gross, A. (2017). Nitrogen and carbon balance in a novel near-zero water exchange saline recirculating aquaculture system. Aquaculture 467, 118–126. doi: 10.1016/j.aquaculture.2016.04.029
Yun, J., Lee, S. D., and Cho, K. S. (2016). Biomethane production and microbial community response according to influent concentration of molasses wastewater in a UASB reactor. Appl. Microbiol. Biotechnol. 100, 4675–4683. doi: 10.1007/s00253-016-7314-1
Keywords: RAS, sludge, biogas, methane, aquaculture, anaerobic digestion, aquaponics
Citation: Klein J, Schüch A, Foth S, Sprafke J, Bischoff A, Nelles M and Palm HW (2024) Enhancing energy recovery from aquaculture residual materials: a focus on anaerobic digestion of African catfish (Clarias gariepinus) sediment sludge. Front. Sustain. Food Syst. 8:1397491. doi: 10.3389/fsufs.2024.1397491
Edited by:
Çağrı Akyol, Ghent University, BelgiumReviewed by:
E. Gozde Ozbayram, Istanbul University, TürkiyeNagore Guerra, University of Vic - Central University of Catalonia, Spain
Copyright © 2024 Klein, Schüch, Foth, Sprafke, Bischoff, Nelles and Palm. This is an open-access article distributed under the terms of the Creative Commons Attribution License (CC BY). The use, distribution or reproduction in other forums is permitted, provided the original author(s) and the copyright owner(s) are credited and that the original publication in this journal is cited, in accordance with accepted academic practice. No use, distribution or reproduction is permitted which does not comply with these terms.
*Correspondence: Jan Klein, amFuLmtsZWluM0B1bmktcm9zdG9jay5kZQ==