- 1Laboratory of Agronomy, Department of Crop Science, Agricultural University of Athens, Athens, Greece
- 2Department of Crop Science, University of Patras, Mesolonghi, Greece
Sustainable agriculture confronts significant challenges such as soil degradation, intensification of agricultural production, and the impact of climate change. Legume intercropping is an “underestimated” cultural practice that harnesses the unique ecosystem services of legumes to enhance resilience and productivity of cropping systems. Legumes contribute to biodiversity by supporting beneficial insects, including pollinators, which can enhance crop productivity. Additionally, through nitrogen fixation, legumes reduce synthetic fertilizer inputs, promote efficient nutrient cycling, and improve soil health. The introduction of legumes in intercropping schemes has beneficial effects on soil texture, microbial diversity, water retention, crop growth, and yield. This cultural practice also contributes to reduced emissions of greenhouse gases, carbon sequestration, and lower pesticide inputs. In addition, legume intercropping promotes biodiversity while facilitating natural pest control and weed suppression. Overall, the inclusion of legumes in intercropping schemes and diversified cropping systems can support food security and mitigate environmental risks related to climate change.
1 Introduction
The sustainable use of soil is crucial for human life and ecological stability, as soil supports agriculture, fosters plant development, serves as habitats for wildlife, preserves biodiversity, soil organic carbon storage, and improves the overall quality of the environment (Pereira et al., 2018). These functions are vital for maintaining biodiversity and ecological resilience, especially in an era when the constantly increasing population raises food demands and puts immense pressure on natural resources' availability (Smith et al., 2016).
Intensive agriculture causes biodiversity loss and extensive land degradation that now affects approximately 30% of global land area and impacts three billion people; each year, an estimated 10 million hectares of arable land are rendered unproductive (Hossain et al., 2020). This intensified land use, along with a heavy reliance on pesticide inputs and fertilizers, gradually diminishes soil fertility and disrupts ecosystem balance (Krasilnikov et al., 2022). Climate change exacerbates these challenges, accelerating resource depletion and posing additional threats to food security and safety (Miraglia et al., 2009; Farooq and Pisante, 2019). Accordingly, the adoption of sustainable agricultural practices is key to strengthening resilience and long-term productivity in agricultural systems. Biodiversity restoration through diversified agroecosystems plays a vital role in maintaining soil fertility and facilitating key ecological processes such as pollination and pest control. Intercropping, as an agronomic practice, is one of the best “fits” in such diversified agroecosystems, referring to a multiple cropping system where two or more crop species are cultivated together in the same field during overlapping periods of their growing season (Scherr and McNeely, 2008). Intercropping has demonstrated the ability to increase yields across various crop combinations while reducing reliance on chemical inputs and promoting the cultivation of high-quality food (Lulie, 2017). It also improves crop protection and facilitates efficient use of soil resources (Hauggaard-Nielsen et al., 2008). In contrast to crop monocultures, which deplete biodiversity and lead to overreliance on agrochemicals, intercrops reduce soil erosion, increase yield stability, and improve pest-weed management (Figure 1).
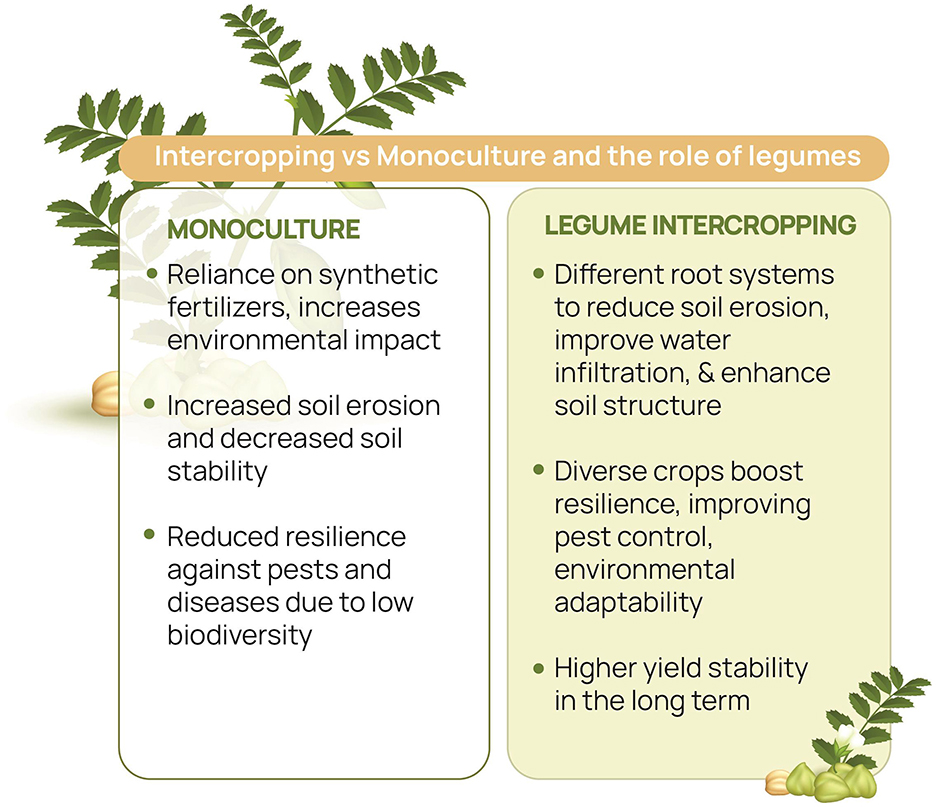
Figure 1. Benefits of legume intercropping vs. monoculture: enhancing soil health, resilience, and productivity.
All legumes have the potential to fix atmospheric nitrogen (N) due to their symbiotic association with rhizobacteria of the genus Rhizobium spp. (Mahieu, 2008; Patel and Shah, 2014). N-fixation contributes about 20–22 million tons of N annually to the globe. Therefore, legume presence can reduce fertilizer inputs and nutrient leaching to groundwater (Büchi et al., 2015). Moreover, legumes can enhance microbial abundance and activity, which contributes to improved soil health (Tang et al., 2014). In addition to these benefits, legumes also support climate change mitigation by reducing greenhouse gas emissions associated with synthetic fertilizer use and enhancing soil carbon sequestration, a function that deserves greater recognition (Hassen et al., 2017). They further contribute to improved nutrient cycling and natural pest regulation, reinforcing their multifunctional role in sustainable agriculture.
Their integration into cropping systems offers a pathway toward reducing input dependence, optimizing ecological performance, and promoting productive, biologically based farming practices that align with long-term sustainability goals.
2 Ecosystem services provided by legumes
Incorporating legumes into existing monoculture and simplified crop rotations is a common strategy for improving agricultural diversity globally (Hufnagel et al., 2020), recognized for its multiple social and ecological advantages. These advantages are referred to as ecosystem services (ES), which are the benefits that people receive from ecosystems crucial for life on Earth, including both biological functions and agricultural aspects (Zhang et al., 2007). Globally, cereal–legume intercropping often pairs major cereals with grain legumes; for example, maize is commonly grown alongside soybean and wheat with field pea (Landschoot et al., 2024). In temperate regions, annual legumes such as pea, vetches, and white lupin have long been intercropped with cereals, representing one of the oldest and most established practices for improving both forage and grain production in diversified systems (Mikić et al., 2015).
2.1 Human-centered ecosystem services provided by legumes
Beyond their environmental and agronomic benefits, legumes also provide significant social advantages, particularly in improving food security, public health, and economic resilience. In many regions, limited access to nutritious food remains a major issue, affecting over 2 billion people globally, including vulnerable populations in developed nations. Legumes, with their high protein and micronutrient content, offer an affordable and accessible alternative to expensive animal-based proteins, improving dietary diversity and reducing the risk of malnutrition-related health issues (Boye et al., 2010; Marinangeli et al., 2017). However, despite their nutritional and ecological advantages, legumes remain underutilized in both diets and large-scale agricultural production systems (Foyer et al., 2016).
Despite growing awareness of their benefits, legumes have historically been overlooked in global food security policies. However, targeted public procurement initiatives have shown promising results. In Portugal, a national food policy successfully increased institutional legume consumption, demonstrating how policy-driven interventions can promote healthier and more sustainable dietary habits (Graca et al., 2018).
Beyond nutrition and policy, legumes also offer economic and public health benefits. Research in Canada has shown that encouraging legume consumption (100 g cooked legumes/day for 50% of the population) could reduce healthcare costs by $370 million per year, primarily by lowering the prevalence of cardiovascular disease and type 2 diabetes (Abdullah et al., 2017). Additionally, legumes support local food systems and community-based agriculture, such as home gardens, school meal programs, and cooperative farming projects, making nutritious food more accessible and affordable (Keatinge et al., 2012).
Given their potential to enhance food security, economic resilience, and public health, legumes should be recognized as a cornerstone of sustainable food security strategies. Strengthening policy support, institutional procurement programs, and consumer awareness would help maximize their social benefits, ensuring they contribute more effectively to global food security and sustainable agriculture.
2.2 Enhancing nitrogen fixation through legume intercropping
The use of legumes in intercropping systems is justified by their natural ability to exploit atmospheric nitrogen (N2), which makes them particularly valuable in cropping systems with nitrogen deficiency (Bedoussac et al., 2015). These legumes enhance nitrogen fixation through facilitative interactions among root systems that improve nutrient availability, including nitrogen and phosphorus, especially under environmental stress (Latati et al., 2016). Their ability to fix nitrogen not only supplies an extra source of nitrogen but also minimizes competition between various crop species and legumes for nitrogen uptake. Research has demonstrated that intercropped legumes significantly benefit adjacent cereal crops, with estimates of nitrogen fixation by legumes ranging from 10 to 38 g N m−2 a−1 and nitrogen transfer to cereals varying from 0 to 73% (Thilakarathna et al., 2016). Intercropped legumes can store between 40 and 100 kg N/ha in their above ground biomass, which can lead to higher yields for subsequent crops. For instance, a 30% increase in maize yield has been positively correlated with the development of legumes and nitrogen accumulation in legume tissues (Amossé et al., 2014). Furthermore, the land equivalent ratio (LER) for intercropped systems often exceeds 1, indicating increased productivity compared to sole crops (Mead and Willey, 1980). Legumes exhibit adaptive traits that allow them to optimize nitrogen fixation based on soil nitrogen availability; their nitrogen fixation rate is negatively correlated with the inorganic nitrogen content in the soil, meaning they fix more nitrogen when soil nitrogen is low (Mahieu, 2008). Additionally, research indicates that intercropped cereals absorb 54–64% more soil nitrogen than those grown in monoculture (Rodriguez et al., 2020). Legume intercropping can significantly reduce synthetic fertilizer dependency through several mechanisms. In research conducted by Li et al. (2020), it is shown that intercropping practices can result in 16% to 29% higher grain production per hectare while requiring 19% to 36% less fertilizer per unit of output compared to conventional monocropping systems, this not only enhances profitability for farmers but also reduces environmental impacts, contributing to a more secure food supply.
2.3 Improving legume intercropping for enhanced soil health
Healthy soil is foundational to sustainable agriculture, and legume intercropping contributes to key ecosystem services, such as soil stability, water retention, and microbial health. Intercropping legumes with cereals enhances soil structure by leveraging complementary root-zone effects, as legumes' deeper root systems break up compacted layers, improve soil aeration, and promote stronger soil aggregates (Garland et al., 2017). Additionally, intercropping a variety of crops helps prevent soil erosion by minimizing the impact of precipitation on the soil surface, thereby reducing the sealing of surface pores, enhancing water infiltration, and decreasing runoff volume (Seran and Brintha, 2010). Specifically, intercropping sorghum with cowpea reduced surface runoff by 20–30% compared to growing sorghum alone and by 45–55% compared to growing cowpea alone. Furthermore, soil loss was cut by 50% when sorghum and cowpea were intercropped rather than cultivated as monocultures (Zougmore et al., 2000). Incorporating legumes into intercropped systems has been found to significantly boost the diversity of soil microbial communities, which is essential for effective nutrient cycling and overall soil health due to the specialized roles played by different microbial species (Lai et al., 2022). Research demonstrates that intercropping cereals with legumes increases microbial biomass and activity (Latati et al., 2014). All this evidence supports the idea that intercropping is vital for promoting soil biodiversity and helps select functional microbial communities that rely on carbon fluxes from plant roots and signaling molecules, which encourage mutualistic relationships (Bartelt-Ryser et al., 2005; Philippot et al., 2013).
2.4 Building climate resilience with legume intercropping
Sustainable agricultural practices are essential for climate change adaptation and mitigation. Legume intercropping has been shown to promote agricultural sustainability, providing both mitigation and adaptation benefits by reducing greenhouse gas emissions (Soussana and Lemaire, 2014) and replacing synthetic nitrogen fertilizers with symbiotic nitrogen fixation (Lüscher et al., 2014). According to Jensen et al. (2012), a study on grass-clover intercropping revealed significantly lower mean annual N2O emissions (0.54 kg N2O–N/ha) compared to N-fertilized pasture grass (4.49 kg N2O–N/ha) and pure legume stands of white clover (0.79 kg N2O–N/ha), highlighting the potential of legume intercropping to reduce nitrogen-based emissions. Similarly, Senbayram et al. (2015) reported that seasonal N2O emissions were 35% lower in a wheat-faba bean intercrop compared to N-fertilized wheat in Germany, demonstrating further reductions in fertilizer-derived N2O emissions through intercropping. Additionally, soil carbon sequestration, which has the technical capacity to mitigate up to 89% of greenhouse gas emissions, is a promising climate change mitigation strategy (Fischlin et al., 2007). Over a 7-year experiment, Cong et al. (2015) found that intercropping improved soil organic carbon by up to 4% in the top 20 cm of soil compared to monoculture systems, underscoring its potential to support long-term carbon storage and climate resilience. Another climate-smart approach involves adopting crop varieties and species adapted to specific environmental conditions. This includes introducing new or heritage crop varieties, which diversify agricultural production and can enhance resilience against climate variability (Chimonyo et al., 2020). Given the ecological diversity across regions, tailored planting strategies and crop selections are necessary to address varying climates and weather conditions effectively.
2.5 Pollination and biodiversity support in legume intercropping
Pollination is a key ecological function that plays a crucial role in preserving biodiversity. Many species within the Fabaceae family rely on animal-mediated pollination, offering floral resources such as nectar and pollen that attract a wide range of insect pollinators (Proctor et al., 1996). This function is especially important as the decline in floral resources within modern agricultural landscapes is recognized as a major factor driving pollinator losses (Rhodes, 2018). Consequently, as a potential solution, legume intercropping may help mitigate pollinator losses and contribute to maintaining essential crop pollination services. Growing legume species alongside non-insect-pollinated crops, such as cereals, has been shown to increase the diversity and abundance of flower-visiting insects compared to monoculture systems (Brandmeier et al., 2021). Additionally, intercropping legumes with cereals improves the overall productivity of neighboring crops by attracting shared pollinators (Galloni et al., 2007). Although legume-cereal intercropping is expected to receive fewer pollinators than legume monoculture due to a lower floral density, the impact on pollination rates may be less significant than anticipated (Beyer et al., 2022). However, in a fava bean (Vicia faba L.)—wheat intercropping system, the reduced number of Vicia faba inflorescences did not decrease the total number of bee pollinator visits compared to sole fava bean cultivation. This suggests that both cropping systems are equally attractive to foraging insects. Additionally, Vicia faba plants in intercropping systems produced 46.7% higher yields compared to monoculture, highlighting how intercropping optimizes resource use, reduces plant competition, and enhances overall growth and productivity (Kirsch et al., 2023). Legume intercropping not only supports pollinator conservation but also improves the sustainability of agricultural systems by means of the provision of various ecosystem services (Figure 2).
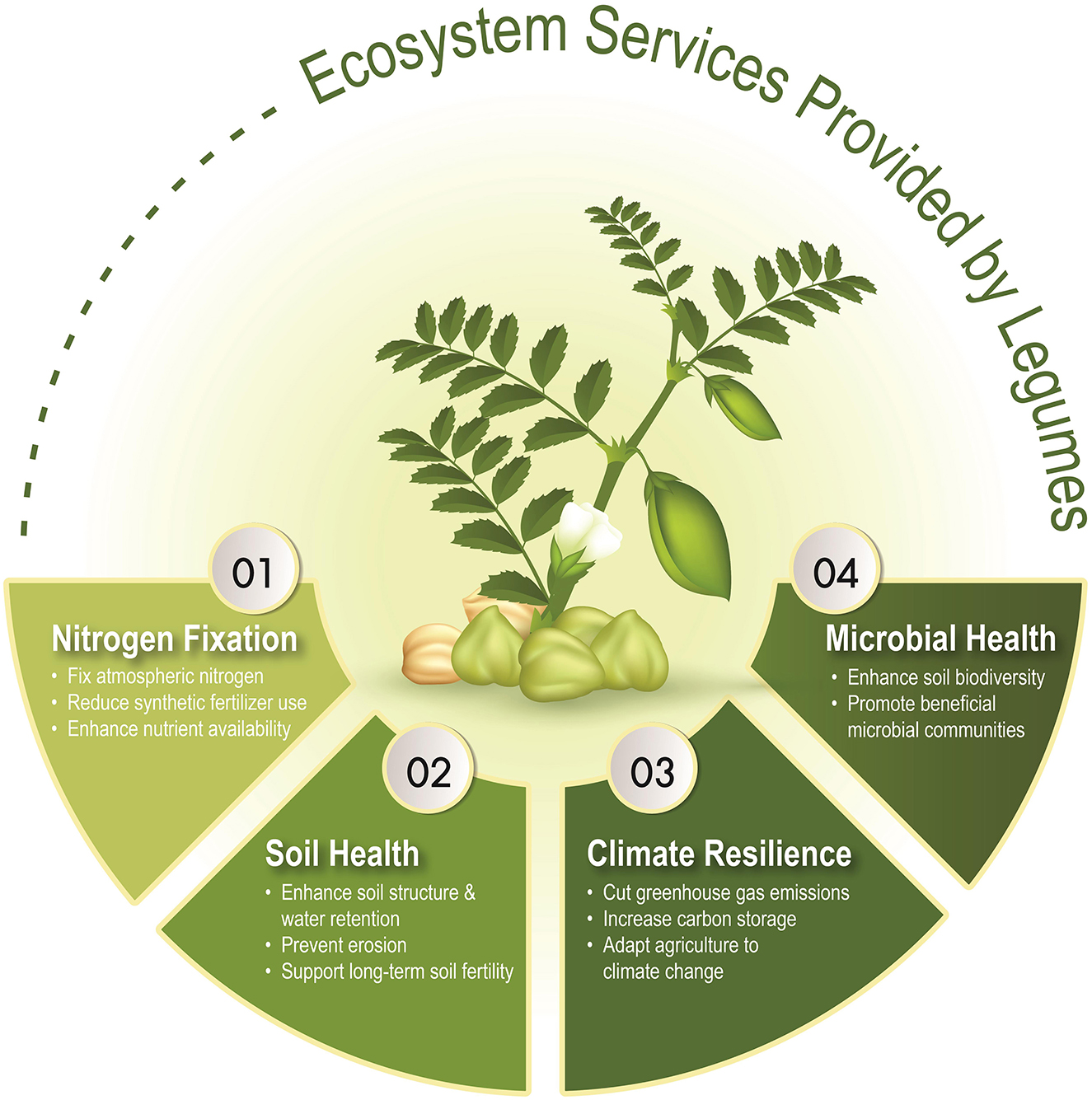
Figure 2. Ecosystem services of legumes in intercropping systems: contributions to soil health, nitrogen fixation, microbial activity, and climate resilience.
3 Discussion
When legumes are included in agricultural systems, farmers are able to take advantage of the natural nitrogen-fixing abilities of these species and, therefore, reduce their reliance on synthetic fertilizers (Crews and Peoples, 2004). This cultural practice mitigates certain nutrient depletion concerns linked to monoculture and also contributes to an increase in the overall productivity of intercropped systems. Some research has shown that intercropping also improves N-use efficiency and further reduce nitrogen leaching, thus lessening the ecological impact of farming (Li et al., 2006). Furthermore, the key ecosystem services provided by legume intercropping contribute to the sustainability of agricultural practices. Synergistic interactions among the root systems of legumes and cereals enhance soil structure and stability, which increases water-holding capacity and reduces the tendency toward soil erosion (Stagnari et al., 2017). Besides, their beneficial effect on soil microbial communities makes nutrient cycling and pest control more efficient, enhancing the resilience of the cropping system (Cardinale et al., 2012).
The use of intercropping reduces the overreliance on chemical fertilizers and significantly lowers greenhouse gas emissions resulting from nitrogen fertilization. Further, this capacity of legumes' carbon sequestration potential in soil makes a quite important role in efforts aimed at mitigating climate change (Yao et al., 2023). The results show that the adoption of sustainable practices enhances not only the efficiency and resilience of agricultural systems but also soil-plant biodiversity, thus making agricultural practices in line with broader environmental goals. Further research is needed to optimize legume intercropping systems to ensure adaptability across diverse agroecosystems, as regional variables such as soil composition, climatic conditions, and water accessibility strongly influence their performance.
Despite these well-documented benefits, several practical challenges continue to hinder the widespread adoption of legume intercropping. Managing intercrops typically involves higher complexity compared to monoculture systems, as it requires precise coordination of planting times, compatible crop selection, and harvest synchronization, thus increasing the demand for specialized knowledge, careful monitoring, and additional labor (Bedoussac et al., 2015). Additionally, poorly designed intercrop combinations or inadequate management can lead to undesirable competition for critical resources such as nutrients, water, and sunlight, which might negatively affect crop yields and productivity (Yu et al., 2015). Furthermore, economic barriers, including increased initial investment costs, greater labor inputs, and limited market incentives or infrastructure, often discourage wider farmer adoption, especially where supportive policy frameworks are lacking (Mamine and Farès, 2020). Addressing these practical challenges is essential to fully exploit the potential of legume intercropping in diverse agricultural contexts.
While legume intercropping has demonstrated multiple benefits in terms of soil fertility, biodiversity, and yield stability, research findings are not always consistent across different agroecosystems. Some studies report significant improvements in productivity and nitrogen-use efficiency due to complementary plant interactions (Raseduzzaman and Jensen, 2017), while others highlight cases where intercropping showed minimal or even negative impacts on yield due to interspecific competition and environmental variability (Hauggaard-Nielsen et al., 2009). Similarly, while nitrogen fixation benefits are well-documented, research suggests that phosphorus availability and uptake may not always improve under legume-based intercropping systems, depending on soil conditions and species selection (Li et al., 2020). These inconsistencies emphasize the importance of region-specific research and tailored management strategies to optimize intercropping performance.
In summary, legume intercropping reinforces its value in sustainable agriculture. Nonetheless, future research should focus on optimizing cropping systems tailored to regional conditions, addressing existing management complexities, and strengthening policy support and market incentives. These steps will ensure broader adoption and enable legume intercropping systems to fully deliver their potential for resilient and sustainable global agriculture.
Author contributions
MK: Conceptualization, Writing – original draft. IG: Writing – review & editing. MD: Data curation, Visualization, Writing – original draft. VK: Data curation, Visualization, Writing – original draft. PK: Data curation, Visualization, Writing – original draft. IT: Conceptualization, Project administration, Writing – review & editing.
Funding
The author(s) declare that financial support was received for the research and/or publication of this article. This work was funded by the European Union under the grant agreement no. 101135472 (VALERECO project).
Acknowledgments
The authors want to thank the Editorial Board for welcoming this work.
Conflict of interest
The authors declare that the research was conducted in the absence of any commercial or financial relationships that could be construed as a potential conflict of interest.
The author(s) declared that they were an editorial board member of Frontiers, at the time of submission. This had no impact on the peer review process and the final decision.
Generative AI statement
The author(s) declare that no Gen AI was used in the creation of this manuscript.
Publisher's note
All claims expressed in this article are solely those of the authors and do not necessarily represent those of their affiliated organizations, or those of the publisher, the editors and the reviewers. Any product that may be evaluated in this article, or claim that may be made by its manufacturer, is not guaranteed or endorsed by the publisher.
References
Abdullah, M. M., Marinangeli, C. P., Jones, P. J., and Carlberg, J. G. (2017). Canadian potential healthcare and societal cost savings from consumption of pulses: a cost-of-illness analysis. Nutrients 9:793. doi: 10.3390/nu9070793
Amossé, C., Jeuffroy, M. H., Mary, B., and David, C. (2014). Contribution of relay intercropping with legume cover crops on nitrogen dynamics in organic grain systems. Nutr. Cycl. Agroecosyst. 98, 1–14. doi: 10.1007/s10705-013-9591-8
Bartelt-Ryser, J., Joshi, J., Schmid, B., Brandl, H., and Balser, T. (2005). Soil feedbacks of plant diversity on soil microbial communities and subsequent plant growth. Perspect. Plant Ecol. Evol. Syst. 7, 27–49. doi: 10.1016/j.ppees.2004.11.002
Bedoussac, L., Journet, E. P., Hauggaard-Nielsen, H., Naudin, C., Corre-Hellou, G., Jensen, E. S., et al. (2015). Ecological principles underlying the increase of productivity achieved by cereal-grain legume intercrops in organic farming. A review. Agron Sustain Dev 35, 911–935. doi: 10.1007/s13593-014-0277-7
Beyer, N., Gabriel, D., and Westphal, C. (2022). Landscape composition modifies pollinator densities, foraging behavior and yield formation in faba beans. Basic Appl. Ecol. 61, 30–40. doi: 10.1016/j.baae.2022.03.002
Boye, J., Zare, F., and Pletch, A. (2010). Pulse proteins: processing, characterization, functional properties and applications in food and feed. Int. Food Res 43, 414–431. doi: 10.1016/j.foodres.2009.09.003
Brandmeier, J., Reininghaus, H., Pappagallo, S., Karley, A. J., Kiær, L. P., and Scherber, C. (2021). Intercropping in high input agriculture supports arthropod diversity without risking significant yield losses. Basic Appl. Ecol. 53, 26–38. doi: 10.1016/j.baae.2021.02.011
Büchi, L., Gebhard, C. A., Liebisch, F., Sinaj, S., Ramseier, H., and Charles, R. (2015). Accumulation of biologically fixed nitrogen by legumes cultivated as cover crops in Switzerland. Plant Soil 393, 163–175. doi: 10.1007/s11104-015-2476-7
Cardinale, B. J., Duffy, J. E., Gonzalez, A., Hooper, D. U., Perrings, C., Venail, P., et al. (2012). Biodiversity loss and its impact on humanity. Nature 486, 59–67. doi: 10.1038/nature11148
Chimonyo, V. G. P., Wimalasiri, E. M., Kunz, R., Modi, A. T., and Mabhaudhi, T. (2020). Optimizing traditional cropping systems under climate change: a case of maize landraces and bambara groundnut. Front. Sustain. Food Syst. 4:562568. doi: 10.3389/fsufs.2020.562568
Cong, W. F., Hoffland, E., Li, L., Six, J., Sun, J. H., Bao, X. G., et al. (2015). Intercropping enhances soil carbon and nitrogen. Glob. Chang. Biol. 21, 1715–1726. doi: 10.1111/gcb.12738
Crews, T. E., and Peoples, M. B. (2004). Legume versus fertilizer sources of nitrogen: Ecological tradeoffs and human needs. Agric. Ecosyst. Environ. 102, 279–297. doi: 10.1016/j.agee.2003.09.018
Farooq, M., and Pisante, M. (2019). Innovations in sustainable agriculture. New York: Springer International Publishing.
Fischlin, A., Midgley, G. F., Price, J. T., Leemans, R., Gopal, B., Turley, C., et al. (2007). “Ecosystems, their properties, goods, and services,” in Climate Change 2007: Impacts, Adaptation and Vulnerability, eds. M. L. Parry, O. F. Canziani, J. P. Palutikof, P. J. van der Linden, and C. E. Hanson (Cambridge: Cambridge University Press), 211–272.
Foyer, C. H., Lam, H. M., Nguyen, H. T., Siddique, K. H., Varshney, R. K., Colmer, T. D., et al. (2016). Neglecting legumes has compromised human health and sustainable food production. Nat. Plants 2, 1–10. doi: 10.1038/nplants.2016.112
Galloni, M., Podda, L., Vivarelli, D., and Cristofolini, G. (2007). Pollen presentation, pollen-ovule ratios, and other reproductive traits in Mediterranean Legumes (Fam. Fabaceae - Subfam. Faboideae). Pl. Syst. Evol. 266, 147–164. doi: 10.1007/s00606-007-0526-1
Garland, G., Bünemann, E. K., Oberson, A., Frossard, E., and Six, J. (2017). Plant-mediated rhizospheric interactions in maize-pigeon pea intercropping enhance soil aggregation and organic phosphorus storage. Plant Soil 415, 37–55. doi: 10.1007/s11104-016-3145-1
Graca, P., Gregorio, M. J., De Sousa, S. M., Bras, S., Penedo, T., Carvalho, T., et al. (2018). A new interministerial strategy for the promotion of healthy eating in Portugal: implementation and initial results. Health Res. Policy Sys. 16, 1–16. doi: 10.1186/s12961-018-0380-3
Hassen, A., Talore, D. G., Tesfamariam, E. H., Friend, M. A., and Mpanza, T. D. E. (2017). Potential use of forage-legume intercropping technologies to adapt to climate-change impacts on mixed crop-livestock systems in Africa: a review. Reg. Environ. Change 17, 1713–1724. doi: 10.1007/s10113-017-1131-7
Hauggaard-Nielsen, H., Gooding, M., Ambus, P., Corre-Hellou, G., Crozat, Y., Dahlmann, C., et al. (2009). Pea–barley intercropping for efficient symbiotic N2-fixation, soil N acquisition and use of other nutrients in European organic cropping systems. Field Crops Res. 113, 64–71. doi: 10.1016/j.fcr.2009.04.009
Hauggaard-Nielsen, H., Jørnsgaard, B., Kinane, J., and Jensen, E. S. (2008). Grain legume - Cereal intercropping: The practical application of diversity, competition and facilitation in arable and organic cropping systems. Renew. Agric. Food Syst. 23, 3–12. doi: 10.1017/S1742170507002025
Hossain, A., Krupnik, T. J., Timsina, J., Mahboob, M. G., Chaki, A. K., Farooq, M., et al. (2020). “Agricultural Land Degradation: Processes and Problems Undermining Future Food Security,” in Environment, Climate, Plant and Vegetation Growth, (Newyork: Springer International Publishing), 17–61. doi: 10.1007/978-3-030-49732-3_2
Hufnagel, J., Reckling, M., and Ewert, F. (2020). Diverse approaches to crop diversification in agricultural research. A review. Agron. Sustain. Dev. 40. doi: 10.1007/s13593-020-00617-4
Jensen, E. S., Peoples, M. B., Boddey, R. M., Gresshoff, P. M., Henrik, H. N., Alves, B. J. R., et al. (2012). Legumes for mitigation of climate change and the provision of feedstock for biofuels and biorefineries. A review. Agron. Sustain. Dev. 32, 329–364. doi: 10.1007/s13593-011-0056-7
Keatinge, J. D., Chadha, M. L., Hughes, J. D. A., Easdown, W. J., Holmer, R. J., Tenkouano, A., et al. (2012). Vegetable gardens and their impact on the attainment of the Millennium Development Goals. Biol. Agric. Hortic. 28, 71–85. doi: 10.1080/01448765.2012.681344
Kirsch, F., Hass, A. L., Link, W., and Westphal, C. (2023). Intercrops as foraging habitats for bees: Bees do not prefer sole legume crops over legume-cereal mixtures. Agric. Ecosyst. Environ. 343:108268. doi: 10.1016/j.agee.2022.108268
Krasilnikov, P., Taboada, M. A., and Amanullah (2022). Fertilizer use, soil health and agricultural sustainability. Agriculture 12:462. doi: 10.3390/agriculture12040462
Lai, H., Gao, F., Su, H., Zheng, P., Li, Y., and Yao, H. (2022). Nitrogen distribution and soil microbial community characteristics in a legume–cereal intercropping system: a review. Agronomy 12:1900. doi: 10.3390/agronomy12081900
Landschoot, S., Zustovi, R., Dewitte, K., Randall, N. P., Maenhout, S., and Haesaert, G. (2024). Cereal-legume intercropping: a smart review using topic modelling. Front. Plant Sci. 14:1228850. doi: 10.3389/fpls.2023.1228850
Latati, M., Bargaz, A., Belarbi, B., Lazali, M., Benlahrech, S., Tellah, S., et al. (2016). The intercropping common bean with maize improves the rhizobial efficiency, resource use and grain yield under low phosphorus availability. Eur. J. Agron. 72, 80–90. doi: 10.1016/j.eja.2015.09.015
Latati, M., Blavet, D., Alkama, N., Laoufi, H., Drevon, J. J., Gérard, F., et al. (2014). The intercropping cowpea-maize improves soil phosphorus availability and maize yields in an alkaline soil. Plant Soil 385, 181–191. doi: 10.1007/s11104-014-2214-6
Li, C., Hoffland, E., Kuyper, T. W., Yu, Y., Zhang, C., Li, H., et al. (2020). Syndromes of production in intercropping impact yield gains. Nature Plants 6, 653–660. doi: 10.1038/s41477-020-0680-9
Li, L., Sun, J., Zhang, F., Guo, T., Bao, X., Smith, A. F., et al. (2006). Root distribution and interactions between intercropped species. Oecologia 147, 280–290. doi: 10.1007/s00442-005
Lulie, B. (2017). Intercropping practice as an alternative pathway for sustainable agriculture: a review. ARJASR 5, 440–452. doi: 10.14662/ARJASR2017.057
Lüscher, A., Mueller-Harvey, I., Soussana, J. F., Rees, R. M., and Peyraud, J. L. (2014). Potential of legume-based grassland-livestock systems in Europe: a review. Grass Forage. Sci. 69, 206–228. doi: 10.1111/gfs.12124
Mahieu, S. (2008). Assessment of the Below Ground Contribution of Field Grown Pea (Pisum sativum L.) to the Soil N Pool. Université d'Angers, 128.
Mamine, F., and Farès, M. H. (2020). Barriers and levers to developing wheat–pea intercropping in Europe: a review. Sustainability 12:6962. doi: 10.3390/su12176962
Marinangeli, C. P., Curran, J., Barr, S. I., Slavin, J., Puri, S., Swaminathan, S., et al. (2017). Enhancing nutrition with pulses: defining a recommended serving size for adults. Nutr. Rev. 75, 990–1006. doi: 10.1093/nutrit/nux058
Mead, R., and Willey, R. W. (1980). The concept of a “land equivalent ratio” and advantages in yields from intercropping. Cambridge: Cambridge University Press.
Mikić, A., Cupina, B., Rubiales D, Mihailović V., Šarunaite, L., Fustec, J., et al. (2015). Models, developments, and perspectives of mutual legume intercropping. Adv. Agron. 130, 337–419. doi: 10.1016/bs.agron.2014.10.004
Miraglia, M., Marvin, H. J. P., Kleter, G. A., Battilani, P., Brera, C., Coni, E., et al. (2009). Climate change and food safety: an emerging issue with special focus on Europe. Food. Chem. Toxicol. 47, 1009–1021. doi: 10.1016/j.fct.2009.02.005
Patel, S., and Shah, D. B. (2014). Phylogeny in few species of Leguminosae family based on matK sequence. Comput. Mol. Biol. 4, 1–5 doi: 10.5376/cmb.2014.04.0006
Pereira, P., Bogunovic, I., Muñoz-Rojas, M., and Brevik, E. C. (2018). Soil ecosystem services, sustainability, valuation and management. Curr. Opin. Environ. Sci. Health 5, 7–13. doi: 10.1016/j.coesh.2017.12.003
Philippot, L., Raaijmakers, J. M., Lemanceau, P., and Van Der Putten, W. H. (2013). Going back to the roots: The microbial ecology of the rhizosphere. Nat. Rev. Microbiol. 11, 789–799. doi: 10.1038/nrmicro3109
Proctor, M., Yeo, P., and Lack, A. (1996). The natural history of pollination. London: HarperCollins Publishers, 479 pp.
Raseduzzaman, M. D., and Jensen, E. S. (2017). Does intercropping enhance yield stability in arable crop production? A meta-analysis. Eur. J. Agron. 91, 25–33. doi: 10.1016/j.eja.2017.09.009
Rhodes, C. J. (2018). Pollinator decline–an ecological calamity in the making? Sci. Prog. 101, 121–160. doi: 10.3184/003685018X15202512854527
Rodriguez, C., Carlsson, G., Englund, J. E., Flöhr, A., Pelzer, E., Jeuffroy, M. H., et al. (2020). Grain legume-cereal intercropping enhances the use of soil-derived and biologically fixed nitrogen in temperate agroecosystems. A meta-analysis. Eur. J. Agron. 118:126077. doi: 10.1016/j.eja.2020.126077
Scherr, S. J., and McNeely, J. A. (2008). Biodiversity conservation and agricultural sustainability: Towards a new paradigm of “ecoagriculture” landscapes. Phil. Trans. R. Soc. B. 363, 477–494. doi: 10.1098/rstb.2007.2165
Senbayram, M., Wenthe, C., Lingner, A., Isselstein, J., Steinmann, H., Kaya, C., et al. (2015). Legume-based mixed intercropping systems may lower agricultural born N2O emissions. Energy Sustain. Soc. 6, 1–9. doi: 10.1186/s13705-015-0067-3
Seran, T. H., and Brintha, I. (2010). Review on maize based intercropping. J. Agron. 9, 135–145. doi: 10.3923/ja.2010.135.145
Smith, P., House, J. I., Bustamante, M., Sobock,á, J., Harper, R., Pan, G., et al. (2016). Global change pressures on soils from land use and management. Glob. Chang. Biol. 22, 1008–1028. doi: 10.1111/gcb.13068
Soussana, J. F., and Lemaire, G. (2014). Coupling carbon and nitrogen cycles for environmentally sustainable intensification of grasslands and crop-livestock systems. Agric. Ecosyst. Environ. 190, 9–17. doi: 10.1016/j.agee.2013.10.012
Stagnari, F., Maggio, A., Galieni, A., and Pisante, M. (2017). Multiple benefits of legumes for agriculture sustainability: an overview. Chem. Biol. Technol. Agric. 4, 1–13. doi: 10.1186/s40538-016-0085-1
Tang, X., Bernard, L., Brauman, A., Daufresne, T., Deleporte, P., Desclaux, D., et al. (2014). Increase in microbial biomass and phosphorus availability in the rhizosphere of intercropped cereal and legumes under field conditions. Soil Biol. Biochem. 75, 86–93. doi: 10.1016/j.soilbio.2014.04.001
Thilakarathna, M. S., McElroy, M. S., Chapagain, T., Papadopoulos, Y. A., and Raizada, M. N. (2016). Belowground nitrogen transfer from legumes to non-legumes under managed herbaceous cropping systems. A review. Agron. Sustain. Dev. 36. doi: 10.1007/s13593-016-0396-4
Yao, F., Wu, Y., Liu, X., Cao, Y., Lv, Y., Wei, W., et al. (2023). Research progress and development trends of greenhouse gas emissions from cereal–legume intercropping systems. Agronomy 13:4. doi: 10.3390/agronomy13041085
Yu, Y., Stomph, T. J., Makowski, D., and van Der Werf, W. (2015). Temporal niche differentiation increases the land equivalent ratio of annual intercrops: a meta-analysis. Field Crops Res. 184, 133–144. doi: 10.1016/j.fcr.2015.09.010
Zhang, W., Ricketts, T. H., Kremen, C., Carney, K., and Swinton, S. M. (2007). Ecosystem services and dis-services to agriculture. Ecol. Econ 64, 253–260. doi: 10.1016/j.ecolecon.2007.02.024
Keywords: legumes, ecosystem services, biodiversity, nitrogen fixation, climate adaptation, pollination
Citation: Kokkini M, Gazoulis I, Danaskos M, Kontogeorgou V, Kanatas P and Travlos I (2025) Enhancing ecosystem services in agriculture: the special role of legume intercropping. Front. Sustain. Food Syst. 9:1547879. doi: 10.3389/fsufs.2025.1547879
Received: 18 December 2024; Accepted: 14 April 2025;
Published: 30 April 2025.
Edited by:
Sudhir Kumar Rajpoot, Banaras Hindu University, IndiaReviewed by:
Silvia Fogliatto, University of Turin, ItalyInga Grinfelde, Latvia University of Agriculture, Latvia
Copyright © 2025 Kokkini, Gazoulis, Danaskos, Kontogeorgou, Kanatas and Travlos. This is an open-access article distributed under the terms of the Creative Commons Attribution License (CC BY). The use, distribution or reproduction in other forums is permitted, provided the original author(s) and the copyright owner(s) are credited and that the original publication in this journal is cited, in accordance with accepted academic practice. No use, distribution or reproduction is permitted which does not comply with these terms.
*Correspondence: Ilias Travlos, dHJhdmxvc0BhdWEuZ3I=; Metaxia Kokkini, a29raW5pbWV0YXhpYTIwMDBAZ21haWwuY29t