- Department of Animal Sciences, Faculty of Agriculture, Obafemi Awolowo University, Ile-Ife, Nigeria
Climate change, characterized by the increased frequency and intensity of extreme weather events, is the greatest environmental challenge threatening global food systems. Its impacts are particularly severe for livestock production systems in developing countries. In low-and middle-income countries (LMICs), livestock production provides critical livelihoods for millions of vulnerable people and plays a significant role in food security. However, the sector is highly susceptible to the adverse effects of climate change. Climate change in LMICs is associated with erratic rainfall, rising temperatures, flooding, drought, desertification, and a higher frequency of extreme weather events. In particular, when temperatures exceed the thresholds projected by the Intergovernmental Panel on Climate Change (IPCC), livestock are subjected to heat stress, which reduces productivity, lowers conception rates, and can be life-threatening for many species. In response, various climate adaptation strategies have been implemented to enhance resilience in livestock production systems. This review evaluates existing adaptation strategies including their effectiveness in LMICs and proposes simplified and targeted adaptation strategies to build resilience in livestock production systems. Key adaptation measures include genetic improvement and diversification of livestock species, early warning systems, precision livestock farming technologies, climate-smart strategies, institutional and policy frameworks and capacity-building initiatives. Further, key factors influencing adaptation strategies outcomes such as governance, financial investment, community engagement, and technological infrastructure were highlighted. While some strategies such as breeding programs for heat-tolerant livestock and early warning systems have yielded positive results, challenges including limited financial resources, weak institutional frameworks, and resistance to change hinder their widespread adoption. The review also provides recommendations for improving adaptation strategies, including enhanced investment in data-enabled innovations, integration of climate adaptation policies into national development plans, and increased participatory approaches involving local livestock farmers. In conclusion, this study provides a roadmap for building climate-resilient livestock production systems in LMICs to ensure sustainable food production and improved livelihoods under changing climate.
1 Introduction
According to FAO (2006) and the World Bank (2020), the global livestock sector contributes 40% of the world’s agricultural gross domestic product, employing one to three billion people and providing a livelihood base for about one billion individuals living in poverty. Livestock serves as a critical resource for low-income populations, including pastoralists who rely entirely on livestock, agro-pastoralists who raise crops and livestock, and smallholder farmers who primarily depend on crops but also keep livestock. These groups represent key players in complex and interconnected livestock value chains globally. Further, livestock products are also vital to global food security, contributing 17% of global kilocalorie consumption and 33% of global protein consumption (Rosegrant et al., 2009; Godde et al., 2021; Erdaw, 2023). Despite its substantial contribution to global economic development as highlighted, the livestock sector faces numerous challenges, with climate change being one of the most significant (Rojas-Downing et al., 2017; Cheng et al., 2022). Climate change is characterized by the increased frequency and intensity of extreme weather events, representing the greatest environmental challenge and a global threat to food systems especially in low and middle-income countries (LMICs). LMICs, as of 2024, are classified by the World Bank as nations with a gross national income (GNI) per capita of $4,465 or less (World Bank, 2024). These countries are primarily located in Africa, Asia, Latin America, and parts of the Pacific. They are disproportionately affected by climate change due to high dependence on climate-sensitive sectors, such as agriculture and livestock farming. They are also characterized by limited financial and technological resources to implement large-scale adaptation measures to climate change.
Extreme weather events such as droughts, rising temperatures, heat stress, unpredictable rainfall, and increased flooding are likely to adversely affect livestock production both in the short-term and long-term (Godde et al., 2021; Thornton and Gerber, 2010). For example, during the 2011–2012 period, Mexico experienced its most severe drought in 70 years, leading to substantial declines in livestock populations. Specifically, cattle and goat stocks decreased by approximately 3% across the country (Murray-Tortarolo and Jaramillo, 2019). Further, Mongolia’s livestock industry has been recurrently affected by dzud—a climatic phenomenon characterized by harsh winters following dry summers. During the 2009–2010 Dzud crisis, approximately 9,000 families lost all their livestock, with an estimated 17% of the country’s livestock perishing (Otani et al., 2015). Further, climate change poses a significant environmental threat not only to crops and animals but also to the entire human race (Thornton, 2010; Abbass et al., 2022). Its effects have serious implications for agriculture, livestock production, ecosystems, water resources, human health, soil quality, and the atmosphere. In many LMICs in the tropics and subtropics, the impacts of climate change are already evident. Weather-related disasters have become increasingly frequent over the past four decades, a trend that is predicted to deteriorate further (Thomas and López, 2015).
In terms of vulnerability, the agricultural sector, particularly the livestock sub-sector, is highly vulnerable to climate variability and extreme weather (Godde et al., 2021; Cervigini et al., 2013; Ayanlade et al., 2022). Depending on the region, climate change can manifest as fewer wet days, heavier rainfall, flooding, rising surface air temperatures, sea-level rise, and accelerated soil erosion. The Intergovernmental Panel on Climate Change (IPCC) Fourth Assessment Report (IPCC, 2007) identified many LMICs especially those in sub-Saharan Africa as being most vulnerable to climate change. Associated threats include food and nutrition insecurity, environmental degradation, and exacerbated poverty levels. The report further predicted that the frequency of extreme weather events will continue to aggravate their socio-economic conditions. Projections indicate that global average surface temperatures could rise by 1.8 to 4.0°C by 2,100—significantly higher than temperature increases observed in the last century. These challenges are expected to result in increased mortality and morbidity, further worsening poverty levels among millions of households. For instance, the Horn of Africa experienced an unprecedented multi-year drought from 2020 to 2023 which severely affected many livestock-dependent communities. In particular, the drought led to significant livestock deaths, with reports indicating that approximately 13.2 million livestock perished across Somalia, Ethiopia, and Kenya (Henchiri et al., 2024; Odongo et al., 2025). To mitigate the impacts of climate change on critical livelihood assets such as livestock, a variety of adaptation strategies must be implemented. This review presents a novel synthesis of climate change adaptation strategies specifically tailored for livestock production systems in low-and middle-income countries (LMICs). Unlike previous reviews that primarily discuss general adaptation measures, this study integrates emerging innovations such as precision livestock farming, data-enabled decision-making, and climate-smart genetic improvement programs. Additionally, it critically evaluates the effectiveness of existing strategies by incorporating recent case studies and empirical evidence from LMICs, an area that remains underexplored in climate adaptation literature. A key research gap addressed is the lack of region-specific, practical adaptation frameworks that consider the socio-economic and infrastructural constraints faced by livestock farmers in resource-limited settings. These strategies are intended for implementation by key stakeholders, including government agencies, non-governmental organizations (NGOs), livestock keepers, and other actors in the livestock sector, to mitigate the impacts of climate change on livestock productivity. In this narrative review, we synthesized existing knowledge on climate adaptation strategies for livestock production through qualitative comparison, with a focus on low-and middle-income countries (LMICs). Literature was selected from reputable databases, including Google Scholar, Scopus, Web of Science, and institutional reports from FAO, IPCC, and the World Bank. A thematic analysis approach was used to categorize adaptation strategies into key areas and key findings were presented in tabular format to facilitate structured analysis of adaptation measures, their benefits, challenges, and implementation feasibility.
2 Livestock production and climate change
Climate change can result from both natural and human (anthropogenic) influences (IPCC, 2013; Zheng et al., 2021). Among these, the production of greenhouse gases such as methane, carbon dioxide, water vapor, and nitrous oxide stands out as a major anthropogenic driver. According to IPCC (2013), the primary sources of these gases are the burning of fossil fuels and agricultural activities, including livestock production. The FAO (2006) report highlighted the significant role of the livestock production sector, identifying it as a major threat to environmental sustainability and biodiversity. The sector contributes up to 18% of anthropogenic greenhouse gas emissions—a figure reported to exceed emissions from the entire transport sector (Rojas-Downing et al., 2017). However, this claim has been disputed with estimates lower than this (Kristiansen et al., 2020; Twine, 2021; Scoones, 2022). Regardless of these debates, the livestock sector remains both a contributor to and a victim of climate change.
More than 60 billion land animals are reared and slaughtered annually for human consumption worldwide (FAO, 2020). Furthermore, livestock inventories are expected to double by 2050, with the majority of this growth occurring in developing countries (Steinfeld et al., 2006). As livestock numbers rise to meet increasing demand for meat, milk, and eggs, greenhouse gas emissions from the sector are likely to escalate, further exacerbating climate change and its adverse effects on livestock production, human health, and environmental sustainability. In addition to livestock production, other significant sources of greenhouse gas emissions include fossil fuel combustion, land use changes such as deforestation and desertification, and agricultural practices such as bush burning and fertilizer application (Rojas-Downing et al., 2017).
3 Impact of climate change on livestock production
Climate change has both direct and indirect impacts on livelihoods and livestock production systems in LMICs. Direct impacts include heat stress, flooding, and other extreme weather events that affect livestock assets and food systems (Godde et al., 2021). Indirect impacts extend to the economy, food security, and infrastructure. Valtorta (2009) highlighted four primary pathways through which climate change impacts animal production in tropical regions. Firstly, it reduces the availability of livestock feed-grains, leading to increased prices. Secondly, climate change causes declines in both the production and quality of pastures and forage crops, which are essential for livestock nutrition. Thirdly, it alters the distribution of livestock diseases and pests, potentially exposing animals to new threats. Lastly, extreme weather events directly affect animal health, growth, reproduction, and overall performance, further compounding the challenges faced by livestock producers. These impacts can result in significant adverse consequences for livestock production and yields, which in turn affect human livelihoods. Impaired performance and productivity, high mortality rates, and the loss of animals lead to reduced revenues, increased poverty, and hunger for individuals and communities. Rising global temperatures exacerbate these issues, especially for livestock production. Heat stress impairs livestock performance, reduces productivity, lowers conception rates, and can even be life-threatening (Thornton et al., 2022). Additionally, rising sea levels could flood pastures with saltwater, raising salinity levels and negatively affecting livestock feeds, fodders, forages, and grazing fields. Further, temperature changes may introduce vector-borne diseases, parasite infestations, and the transmission of diseases to new areas previously unaffected by these stressors (Thornton and Herrero, 2008). Addressing these challenges is critical to safeguarding livestock production and the livelihoods dependent on it. Detailed case studies of the impact of climate change on livestock production in LMICs are presented in Table 1.
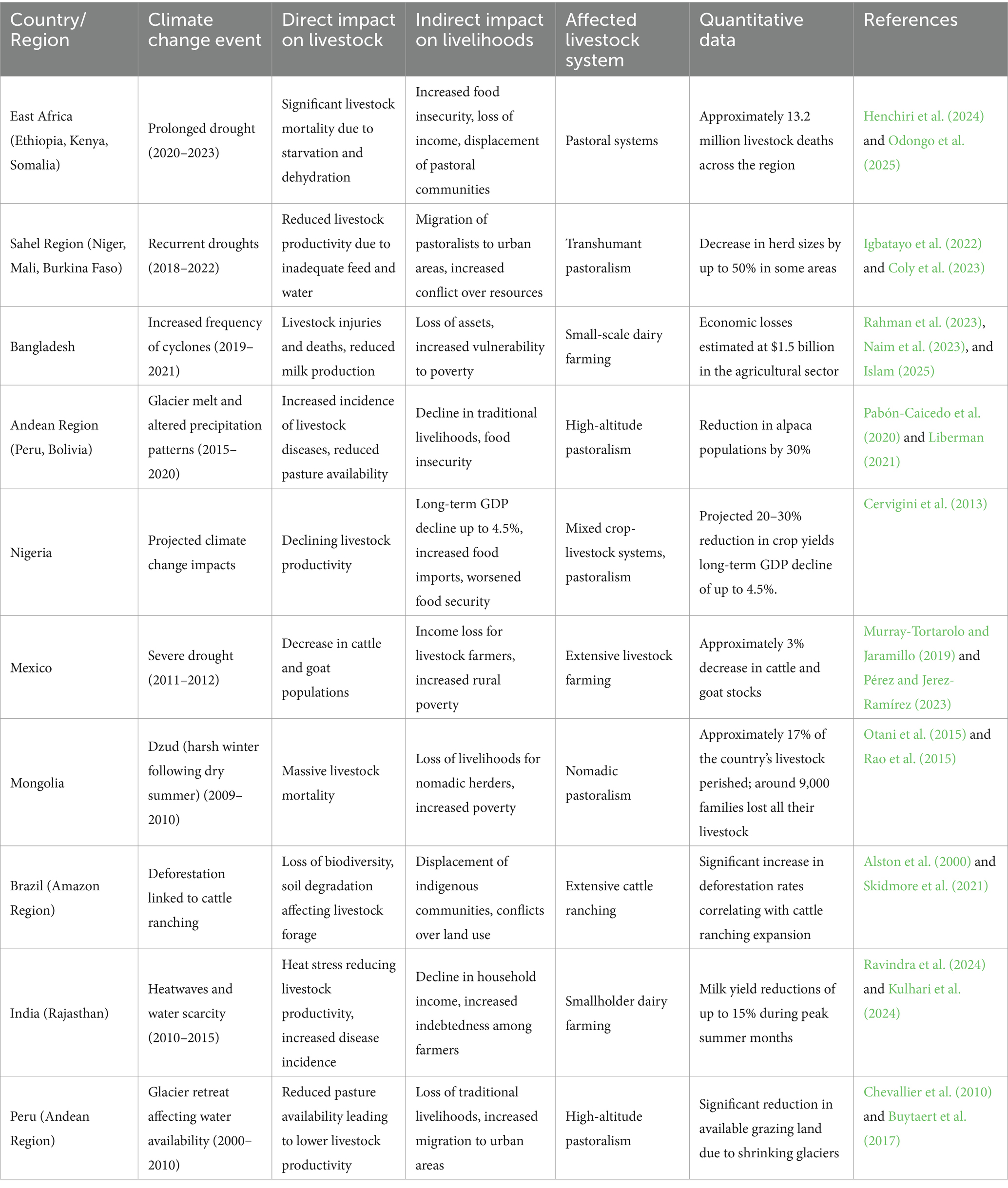
Table 1. Case studies on the impact of climate change on livestock production in low-and middle-income countries (LMICs).
4 Climate change adaptation strategies for the livestock sector development in LMICs
Adaptation to climate change, as defined by the IPCC (2001), involves adjustments in natural or human systems to actual or anticipated climatic stimuli and their effects, to mitigate harm or capitalize on beneficial opportunities. Adaptive capacity refers to a system’s ability to adjust to climate change, including variability and extremes, to reduce potential damages, exploit opportunities, or cope with its consequences. The extent to which agricultural systems including the livestock sector are affected by climate change depends significantly on their adaptive capacity (Thornton and Herrero, 2008). Further, the impacts of climate change vary across regions, with some areas more severely affected than others. Climate change “hotspots” are regions where the effects are expected to be most pronounced. Using the Regional Climate Change Index (RCCI), Giorgi (2006) identified Sub-Saharan and Southern Equatorial Africa as primary hotspots in Africa. The RCCI evaluates regional responses to climate change by considering factors such as changes in mean precipitation, mean surface air temperature, and variability in these elements over time.
Africa’s agricultural vulnerability to climate change largely stems from its reliance on rain-fed and underdeveloped farming systems. Most African farmers operate on a small scale, with limited financial resources, inadequate infrastructure, and inconsistent access to information (Thornton et al., 2009). Despite these challenges, the inherent diversity, context specificity, and traditional knowledge within African agricultural systems offer significant resilience to climate change (Thornton et al., 2014). Addressing the threats posed by climate change requires strategies to reduce vulnerabilities and enhance resilience. These adaptation strategies are essential for maintaining or improving livestock productivity in a rapidly changing climate (Herrero et al., 2008). These practices enable individuals and communities to cope with or adjust to climate change impacts (Nyong et al., 2007). In the livestock sector, adaptation measures focus on improving livestock tolerance to heat and their ability to thrive, grow, and reproduce under conditions of poor nutrition, parasites, and diseases exacerbated by climate change (Hoffman and Vogel, 2008). Such strategies are critical for ensuring food and livelihood security for livestock producers. Community-based interventions, like those documented by Oseni and Bebe (2010) in Kenya, have proven effective in building resilience among pastoral communities. Commonly adopted adaptation strategies include the use of emergency fodder during droughts, diversification of herd composition, improved breeding practices, de-stocking to manage heat stress, provision of shade, and supplementary feeding. These measures play a vital role in safeguarding the livelihoods of livestock-dependent communities in the face of evolving climatic conditions.
Adaptation strategies in livestock production systems can be categorized into different levels: herd, communal, national, and regional. At the herd level, strategies are tailored to small-scale livestock keepers and include measures such as documenting and selecting for heat-tolerant breeds, providing shade, and improving feed availability. At the communal level, collective approaches such as shared grazing areas and community breeding programs are emphasized. At the national and regional levels, governments and organizations can implement policies and programs to support sustainable livestock practices and promote resilience to climate change. By employing these simplified strategies, livestock producers can build resilience, increase adaptive capacity, and reduce the impacts of climate change on livestock production systems. These efforts are crucial for ensuring sustainable livelihoods and food security in vulnerable regions. A detailed critical evaluation of these climate change adaptation strategies for livestock production including their benefits, challenges, implementation feasibility, cost implications, stakeholders involved, and scalability are presented in Table 2.
4.1 Short-term adaptation measures
Short-term adaptation measures are immediate, reactive interventions aimed at reducing the negative impacts of climate variability and extreme weather events on livestock production. These strategies are cost-effective, require minimal infrastructure investment, and are critical for preventing sudden losses in productivity and livestock mortality. The following short-term measures can help livestock farmers mitigate climate-induced stress and maintain productivity.
4.1.1 Resilience building and diversification of livestock species and breeds
To enhance resilience and mitigate the impacts of climate change, livestock farming systems in LMICs must adopt alternative options and strategic adjustments. One effective approach that could be adopted is the introduction of mixed farming systems, where farmers integrate crop and livestock production. Mixed farming systems often yield higher overall productivity due to complementary resource use (Sujatha and Bhat, 2015; Low and Meuwissen, 2023). Farmers also benefit from multiple income streams, which improve financial stability and food security. Furthermore, mixed species systems contribute to ecosystem health by maintaining ecological balance and enhancing biodiversity. Mixed farming also promotes nutrient cycling, as crop residues can be used as livestock feed, and livestock manure can enhance soil fertility. Additionally, providing shaded areas can reduce heat stress impacts on livestock, thereby improving their productivity and welfare. Moreover, enhancing livestock management through improved feeding regimes, effective disease control, and better reproductive management is essential for maintaining productivity under stressful conditions. Further, adjusting stocking rates helps prevent overgrazing by modifying the number of animals per unit area, ensuring sustainable pasture use. Implementing rotational grazing systems also allows pastures to recover, maintaining both the availability and quality of forage all-year. At the national level, coordinated guidelines for livestock production adjustments should be established. These standards should reflect the vulnerability and adaptive capacity of each community, ensuring that interventions are context-specific and sustainable. In addition, the development and implementation of climate-smart feed strategies are essential for enhancing feed efficiency and reducing greenhouse gas (GHG) emissions. These strategies include the use of specific feed additives and formulations. Additives such as tannins (Cardoso-Gutiérrez et al., 2021), seaweed extracts (McGurrin et al., 2023), and essential oils (Benetel et al., 2022; Jiménez-Ocampo et al., 2022) have been shown to mitigate methane emissions from ruminants. Precision feeding techniques also play a crucial role by optimizing nutrient intake, thereby reducing waste and environmental impact (Llorens et al., 2024). Additionally, utilizing locally available feed resources, such as crop residues and agro-industrial by-products, can decrease reliance on imported feed, lowering both costs and emissions. Effective manure management techniques through anaerobic digestion and compositing are also vital for reducing emissions and recycling nutrients (Chadwick et al., 2020; Dadrasnia et al., 2021). Anaerobic digestion captures methane from manure and convert it into biogas for energy production (Jameel et al., 2024). Composting, when properly managed, stabilizes nutrients, reduces methane emissions, and produces organic fertilizer. Biogas production systems not only help in emission reduction but also provide renewable energy for farm operations. Incorporating renewable energy into livestock farming systems could potentially reduce the carbon footprint. Solar-powered water pumps, for instance, offer a reliable water source for livestock in remote areas while reducing dependence on fossil fuels. Wind energy systems, through small-scale wind turbines, can power essential farm equipment, supporting sustainable operations. Additionally, bioenergy production from livestock manure and other organic waste helps reduce waste and provides clean, renewable energy.
Diversification of livestock species and breeds is an essential adaptation strategy to mitigate the impacts of climate change on livestock production systems. By keeping more than one species of livestock, farmers can generate a wider variety of livestock products and make better use of available forage in different seasons even in times of crisis. Diversification also mitigates risk by reducing the likelihood of total production failure, as species respond differently to climatic shocks. Examples of diversification practices include multi-species grazing systems, where cattle, sheep, and goats are integrated to optimize forage use and enhance productivity (Tohiran et al., 2023; Slayi and Jaja, 2024). Another practice involves integrating poultry farming with aquaculture, where chicken manure is used to enhance pond productivity (Njoku and Ejiogu, 1999; Shoko et al., 2019). A summary of proposed production adjustments in various livestock systems for climate change adaptation is presented in Table 3.
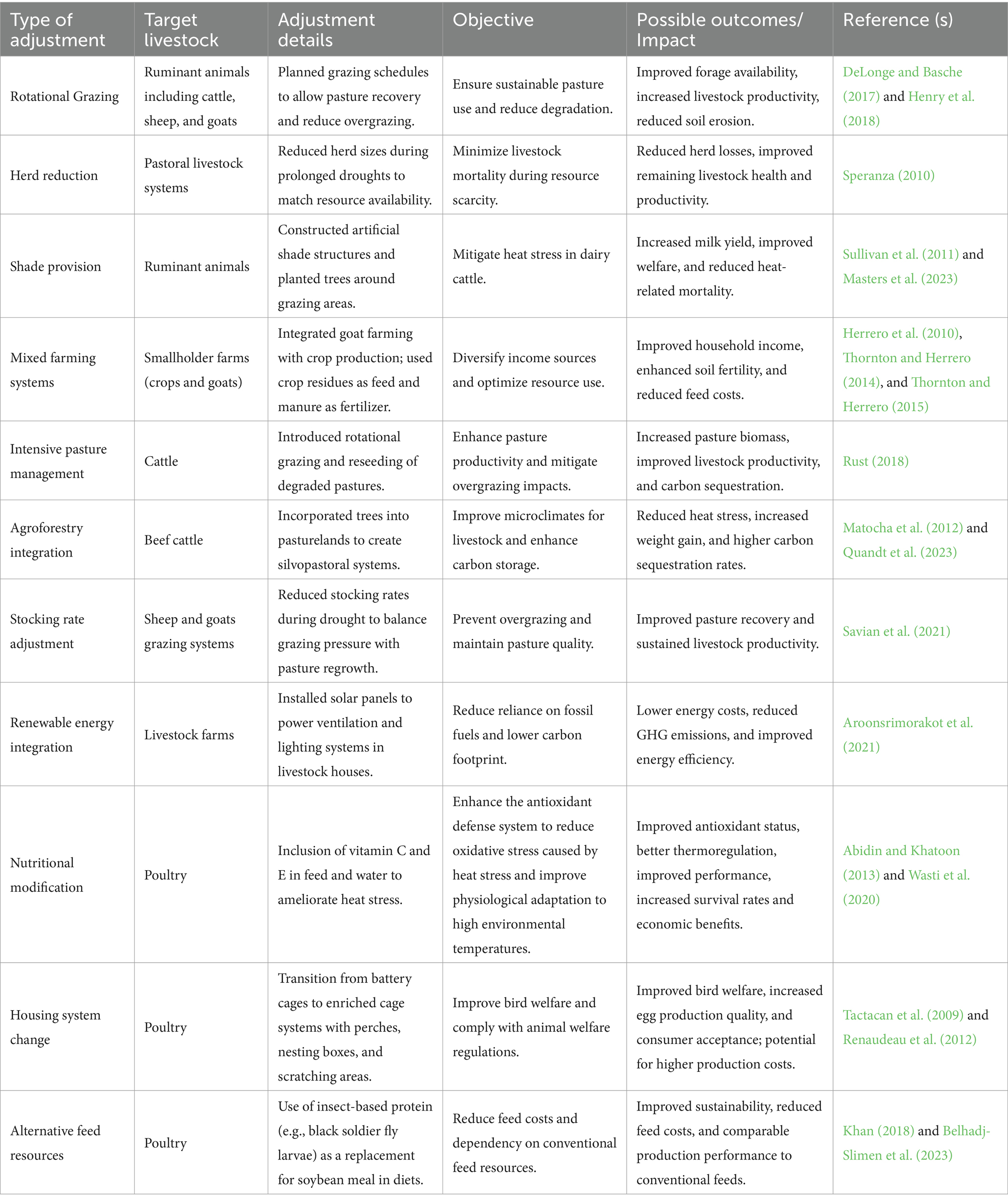
Table 3. Proposed production adjustments in various livestock systems for climate change adaptation.
4.1.2 Early warning systems
Swift responses to perceived threats to livestock are crucial in building resilience and reducing their vulnerability (LEGS, 2014). Prompt interventions, such as relocating animals from affected areas during emergencies like floods and droughts, can significantly help in preserving key livestock assets. The specific intervention required depends on the nature of the emergency, the local context, and the phase of the emergency—whether it is ongoing, in the immediate aftermath, or during recovery or rehabilitation phases (FAO, 2016). The Livestock Emergency Guidelines and Standards (LEGS, 2014) offer comprehensive guidelines aimed at protecting and rebuilding the livestock assets of crisis-affected communities. These guidelines are designed with a focus on livelihoods objectives, providing rapid assistance to support communities in distress. LEGS is particularly valuable for a wide range of stakeholders, including donors, program managers, technical experts, NGOs, policy and decision-makers, educational institutions, and community-based organizations. It helps in identifying the most appropriate livestock interventions during disasters. Typical livestock interventions include the provision of animal health services, emergency feeding and water supplies, and shelter. Additionally, strategies such as destocking help manage livestock numbers during crises, while restocking efforts aim to rebuild herds post-crisis (FAO, 2016). It is crucial to prioritize adaptation efforts in communities where vulnerabilities are highest and the need for resilience is greatest. By focusing resources and efforts on these communities, interventions can be more effective in mitigating the impacts of emergencies and fostering long-term resilience.
4.2 Long-term adaptation measures
Long-term adaptation measures focus on sustainable, proactive strategies that enhance the resilience and productivity of livestock systems in the face of climate change. Unlike short-term interventions, these strategies require systematic planning, investment, and policy support but provide lasting benefits by reducing vulnerability, increasing efficiency, and ensuring food security. The key long-term adaptation measures include the following.
4.2.1 Breeding strategies
Breeding strategies play a pivotal role in enhancing the resilience and productivity of livestock under the increasing pressures of climate change. Significant differences in adaptation exist between livestock breeds and even within breeds, enabling targeted selection and improvement to meet specific environmental challenges. Indigenous livestock breeds are typically more adapted to changing climates (Ahlawat et al., 2015; Mathew and Mathew, 2023). They also have lower feed requirements and can efficiently utilize low-quality pasture and feeds (Ateş et al., 2014). Thus, identifying and strengthening local breeds that have adapted to local climatic stress and feed sources is key to breeding for resilience and adaptation to extreme climatic conditions (Rojas-Downing et al., 2017). For example, breeds such as the Red Maasai sheep (Radeny et al., 2022) and East African shorthorn zebu (Ayalew et al., 2023) demonstrate inherent resilience to harsh climates and diseases. These traits make them invaluable in breeding programs aimed at enhancing climate resilience. Breeding strategies that focus on resilience to heat stress and diseases are especially crucial to adapt to climate change. For example, the development of heat-tolerant cattle breeds, such as the Bonsmara in South Africa (Fedrigo et al., 2021), has shown success in improving resilience to high temperatures and disease resistance. Therefore, designing breeding programs that incorporate adaptation as a major breeding goal could potentially lead to progenies that are hardy, suitable, and well adapted to climate variability. Vulnerable stocks can also be improved through cross-breeding with more adapted breeds. At the herd level, breeding strategies could involve documenting and identifying stocks that have adapted to changing climates and whose performance and productivity are least affected by climate change impacts for breeding purposes. At the communal level, options for nucleus or community-based breeding programmes (CBBPs) should be explored. CBBPs have been utilized over the years under low-input systems in developing countries with considerable success for improving productivity and adaptation (Olaniyan et al., 2024). For instance, the productivity of the indigenous Djallonke sheep was improved in an open nucleus breeding scheme in Ivory Coast (Yapi-Gnaore et al., 1997a; Yapi-Gnaore et al., 1997b). Similarly, Abdel-Salam et al. (2010) reported high genetic gain in milk production of Egyptian Buffalo in open nucleus breeding scheme. Similarly, CBBPs for smallholder farmers in Liberia have resulted in genetic improvements for Liberian goats (Karnuah and Dunga, 2018). These models can be replicated in other regions to enhance climate resilience. Further, these showed that nucleus or community breeding schemes represent unique opportunities for genetic improvement of livestock at the communal level for adaptation to climate change impacts (Shrivastava et al., 2018). At the national and regional levels, investment and collaborative efforts are needed to design and implement breeding programs that incorporate adaptation as a major goal. Additionally, there should be investment in biodiversity conservation. Developing regional gene banks for animal genetic resource conservation can improve breeding programs and serve as an insurance policy against the erosion of valuable indigenous genetic resources. A summary of case studies of how indigenous livestock breeds could enhance resilience and adaptation to climate change is presented in Table 4.
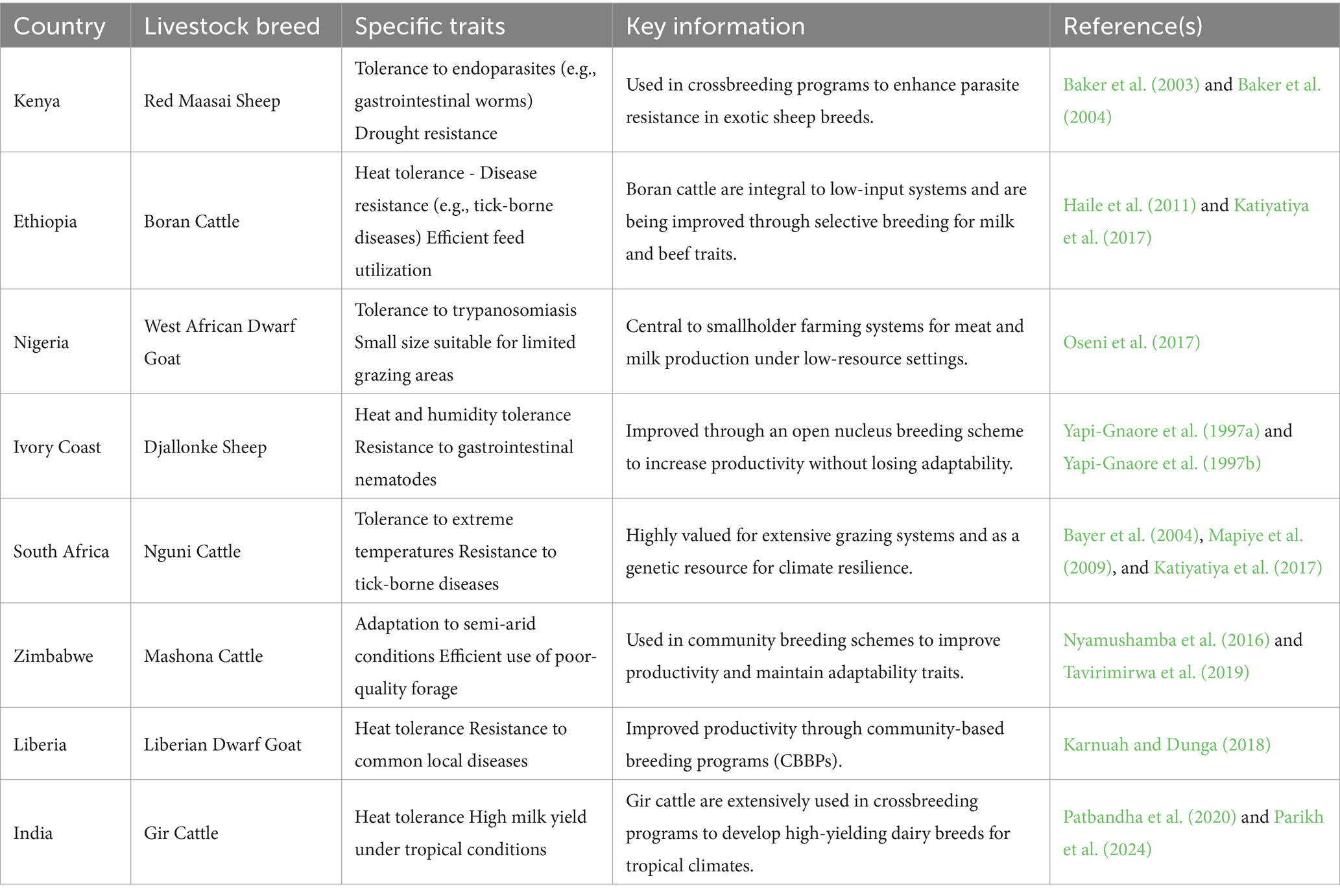
Table 4. Case studies of indigenous livestock breeds enhancing resilience and adaptation to climate change.
4.2.2 Application of science, technology, and innovation in building resilience and adaptation
The Federal, State, and Local Governments in LMICs must make investments in scientific research and development for climate change adaptation. Advancing science and technology is a fundamental requirement for developing effective management strategies to cope with the anticipated impacts of climate change. Both basic and applied research in areas such as breeding and genetics, biotechnology, molecular biology, animal nutrition, pasture and range management, and animal health are essential. These fields will enhance our understanding of the expected impacts of climate change on livestock systems and help devise strategies to reduce their vulnerability. For instance, Oseni (2018) highlighted significant gaps in the application of science, technology, and innovations (STI) in the management of indigenous livestock resources across Eastern, Southern, and Western Africa through the EU-funded iLinova program. One notable area is the development of alternative livestock production systems, such as pasture-based systems, which reduce feed costs by incorporating natural supplements like insects and grasses (Sanusi and Oseni, 2020; Oseni and Bashiru, 2022). Additionally, the use of unconventional feedstuffs and kitchen waste as alternative feed sources has been shown to sustain livestock productivity without adverse effects. The program also emphasized the importance of regional collaboration for the institutionalization of STI in managing indigenous livestock. Such collaborations foster knowledge sharing, resource pooling, and the development of region-specific solutions to common challenges. By prioritizing research and innovation in these areas, a more resilient livestock sector that is better equipped to withstand the pressures of climate change could be attained in LMICs. This will not only protect livelihoods but also contribute to food security and sustainable agricultural development.
Government-led investments in science, technology, and innovation have been demonstrated as effective strategies for enhancing climate adaptation in LMICs. For example, in Bangladesh, the government has implemented the Bangladesh Climate Change Strategy and Action Plan (BCCSAP) to address the increasing threats of flooding and cyclones (Reid et al., 2012; Islam et al., 2013; Akon and Mia, 2024). Further, in Kenya, the Kenya Climate Smart Agriculture Strategy (KCSAS) promotes drought-resistant crop varieties and water-efficient irrigation technologies to mitigate erratic rainfall and prolonged droughts (Kenya Climate Smart Agriculture Strategy, 2025; Waaswa et al., 2024). In Ethiopia, the Sustainable Land Management Program (SLMP) has focused on soil and water conservation, reforestation, and agroforestry to combat land degradation and drought (World Bank, 2020; Schmidt and Tadesse, 2019). Detailed case studies of these investments including their impacts are presented in Table 5.
4.2.3 Capacity building for livestock keepers
There is an urgent need to enhance the capacity of livestock keepers and herders to understand and address the impacts of climate change on livestock production. Mobilizing various local and agrarian communities for climate change adaptation actions is critical to mitigating the adverse effects on key sectors and vulnerable populations. This mobilization should focus on implementing practical strategies and interventions that directly address the challenges posed by climate change. One crucial area for improvement is providing adequate training in heat stress management and fodder production. These skills are essential for ensuring a consistent supply of animal feed, which helps reduce malnutrition and mortality in herds. By equipping livestock producers with the knowledge and tools to manage heat stress and maintain fodder supplies, the resilience of livestock systems can be significantly improved. Strengthening the existing capacities of local authorities, civil society organizations, and the private sector is equally important. This capacity-building effort lays the groundwork for robust climate risk management and facilitates the rapid scaling up of adaptation measures through community-based risk reduction and effective local governance (Nyong et al., 2007). Enhancing these capacities ensures that communities are better prepared to respond to climate-related challenges and can implement sustainable adaptation strategies. For example, the Livestock and Climate Solutions Hub (ILRI, 2025) developed by the International Livestock Research Institute is a platform designed to support LMICs in transitioning to sustainable, climate-smart systems. This hub could potentially be used as a template to build and strengthen the capacity of stakeholders in livestock production in LMICs. The Hub aims to accelerate practical solutions to the challenges posed by climate change to livestock production. This initiative focuses on developing and scaling climate-smart livestock innovations, enhancing resilience and productivity, and guiding countries in meeting their climate goals under the Paris Agreement.
Exploring opportunities for grantsmanship in capacity building for climate change adaptation is another critical avenue. Grants from developed countries can play a significant role in not only building the human capacity necessary to address climate change impacts but also in fostering resilience, improving infrastructure, and raising the standard of living for livestock keepers. These grants can support training programs, infrastructure development, and the adoption of innovative practices that help communities adapt to the changing climate and safeguard their livelihoods.
4.2.4 Institutional policies for climate-smart livestock systems
Strengthening institutional and policy frameworks is critical for enhancing the adaptive capacity of the livestock sector in the face of climate change (USDA, 2013). Effective policies provide a structured approach to implementing adaptation strategies, fostering resilience, and ensuring long-term sustainability. Enacting favorable legislation, subsidies, grants, and insurance schemes can support livestock keepers in adopting climate-smart practices, mitigate financial risks, and secure their livelihoods. For example, in Malawi, the government has integrated Climate-Smart Agriculture (CSA) into national policies to enhance agricultural resilience (World Bank, 2025). This integration includes promoting sustainable land management practices and supporting livestock keepers in adopting climate-resilient strategies. However, in Uganda, efforts to implement climate change adaptation policies in the livestock sector have faced institutional challenges (Ampaire et al., 2017). This highlights the need for coherent policy frameworks and effective institutional coordination to support livestock keepers in adapting to climate change. A well-defined “Climate Change Adaptation Strategy for the Livestock Sector” should be developed to serve as a guiding framework for national, regional, and local governments, as well as research institutions and private stakeholders. This strategy should outline specific actions, allocate resources, and establish measurable goals to ensure effective implementation of climate adaptation initiatives. Integrating climate-smart principles into agricultural policies will not only support livestock resilience but also promote sustainable land use and resource conservation.
The Climate-Smart Agriculture (CSA) approach provides a comprehensive framework for adaptation and mitigation within the livestock sector (Lipper and Zilberman, 2017). By optimizing resource use and reducing greenhouse gas emissions, CSA strategies enhance productivity while minimizing environmental impact (Sekaran et al., 2021; Jalón et al., 2016). Key interventions include precision breeding for resilience, improving feed efficiency, and promoting integrated crop-livestock systems. The adoption of water-efficient irrigation methods, enhanced pasture management, and afforestation programs further contribute to sustainability. A critical component of climate-smart policies is the promotion of sustainable land management and biodiversity conservation. Encouraging agroforestry, rotational grazing, and pasture rehabilitation can help restore degraded lands, enhance carbon sequestration, and improve livestock productivity. Additionally, leveraging data-driven innovations, such as remote sensing and predictive modeling, will enable better decision-making in climate risk management. To ensure the successful implementation of climate-smart policies, multi-stakeholder collaboration is essential. Governments, research institutions, the private sector, and civil society organizations must work together to develop and enforce policies that support climate adaptation. Providing financial incentives for sustainable practices, investing in early warning systems for climate-related risks, and fostering regional cooperation will further strengthen resilience in the livestock sector. By embedding climate adaptation strategies within national policies and leveraging innovative agricultural practices, LMICs can build a more resilient livestock industry, ensuring food security and sustainable development amid evolving climate challenges.
4.2.5 Precision livestock farming and data-enabled innovations for climate change adaptation
The rapid advancements in technology offer unprecedented opportunities to transform livestock production and make it more adaptive and resilient to the impacts of climate change. Precision livestock farming (PLF), coupled with data-enabled innovations, provides tools to monitor, manage, and optimize livestock production systems in real-time. These innovations enhance decision-making, improve resource use efficiency, and mitigate the adverse effects of climate variability (Pardo et al., 2022; Papakonstantinou et al., 2024). One of the most promising developments in PLF is the deployment of Internet of Things (IoT)-enabled sensors, which are cost-effective and scalable for use in low-resource settings. These sensors provide continuous monitoring of key environmental and animal health parameters, enabling farmers to track temperature, humidity, and other climatic variables within livestock housing systems. For instance, early detection of heat stress conditions allows farmers to implement cooling measures such as misting or ventilation adjustments (Islam et al., 2021). Further, Oseni et al. (2025) utilized low-cost IoT sensors to monitor environmental parameters, such as temperature, humidity and noxious gases, for optimal health and welfare of broiler chickens raised under tropical conditions in Nigeria. In addition, wearable sensors on livestock can monitor physiological metrics such as heart rate, body temperature, and activity levels (Neethirajan, 2017; Alipio and Villena, 2023). These data help detect early signs of illness, or heat stress, enabling timely interventions. Furthermore, sensors can also be integrated into feed bins that can measure feed intake in real-time (Shelley et al., 2016; Gonzalez et al., 2018), allowing precise adjustments to meet nutritional requirements while minimizing waste and greenhouse gas emissions. IoT devices can also monitor water quality and consumption, ensuring that livestock have access to clean water, especially during periods of drought or extreme heat.
Data collected through IoT sensors are analyzed using advanced analytics and machine learning algorithms to provide actionable insights for farmers. Decision support systems (DSS) built on these platforms can be applied for weather forecasts (Ahmad and Hossain, 2019) and historical climate data integrated with livestock performance metrics can predict risks such as heatwaves or disease outbreaks (Bett et al., 2017) to help farmers plan preventive measures. In addition, DSS tools can recommend optimal stocking densities, grazing schedules, and rotational grazing practices based on real-time pasture conditions. Data on animal performance and genetic traits can also guide selective breeding efforts to develop heat- and disease-tolerant livestock. However, while PLF and IoT innovations hold great promise, challenges such as high initial costs, limited internet connectivity in rural areas, and low technical expertise among farmers must be addressed. Governments, NGOs, and private sector stakeholders should collaborate to provide subsidies and financial incentives for adopting PLF technologies, invest in infrastructure to improve internet access in rural areas and organize training programs to build farmers’ capacity to use these technologies effectively. Furthermore, IoT-enabled systems can be powered by renewable energy sources, such as solar panels, to ensure sustainability in regions with limited access to electricity. For instance, solar-powered water pumps and ventilation systems can be automated based on sensor inputs, reducing dependency on fossil fuels while ensuring animal welfare.
5 Analysis of climate change adaptation strategies and challenges in their implementation
5.1 Comparative analysis of climate adaptation strategies in livestock production: key factors for success
Climate adaptation strategies in livestock production vary widely across regions, with differing degrees of success. These strategies are influenced by financial resources, community engagement, technical support, and environmental conditions. Therefore, analyzing these strategies provides valuable insights into the critical factors determining their effectiveness. A major determinant of success is technical and financial support. For example, silvopastoral systems (SPS) in Montería, Colombia, achieved success due to the integration of innovative grazing techniques, financial investment, and technical expertise, which enhanced pasture growth and improved carbon sequestration (Rivera et al., 2019; Chará et al., 2017). Similarly, breeding improvement programs in Northern Kenya benefited from structured training, favorable environmental conditions, and community participation, leading to increased productivity (Ojango et al., 2023). In contrast, adaptation strategies such as destocking during droughts in Namibia failed due to low herd sizes, unfavorable market conditions, and cultural barriers (Siririka et al., 2025). Another key factor is community engagement and acceptance. Disease management training in Northern Kenya was successful due to the active participation of pastoral communities and the integration of veterinary services, resulting in improved livestock health (Ojango et al., 2023). Conversely, climate-resilient livestock housing in Bangladesh failed due to high maintenance costs and low farmer adoption, leading to 40% of shelters being abandoned (Rahman, 2022). Institutional and policy support also plays a crucial role. Water management strategies in Namibia had mixed success, highlighting the need for stronger government support and financial assistance (Siririka et al., 2025). Similarly, climate change adaptation efforts in Northeastern Iran were hindered by regulatory weaknesses and inadequate insurance mechanisms, emphasizing the necessity of comprehensive policy frameworks (Sharafatmandrad et al., 2024). Finally, technology integration has shown promising results. Mobile climate advisory services in Uganda effectively reduced livestock losses and increased farm income through real-time weather and market information, facilitated by strong mobile network infrastructure and public-private partnerships (Tuheirwe-Mukasa et al., 2019). A detailed summary of these strategies and their effectiveness is presented in Table 6.
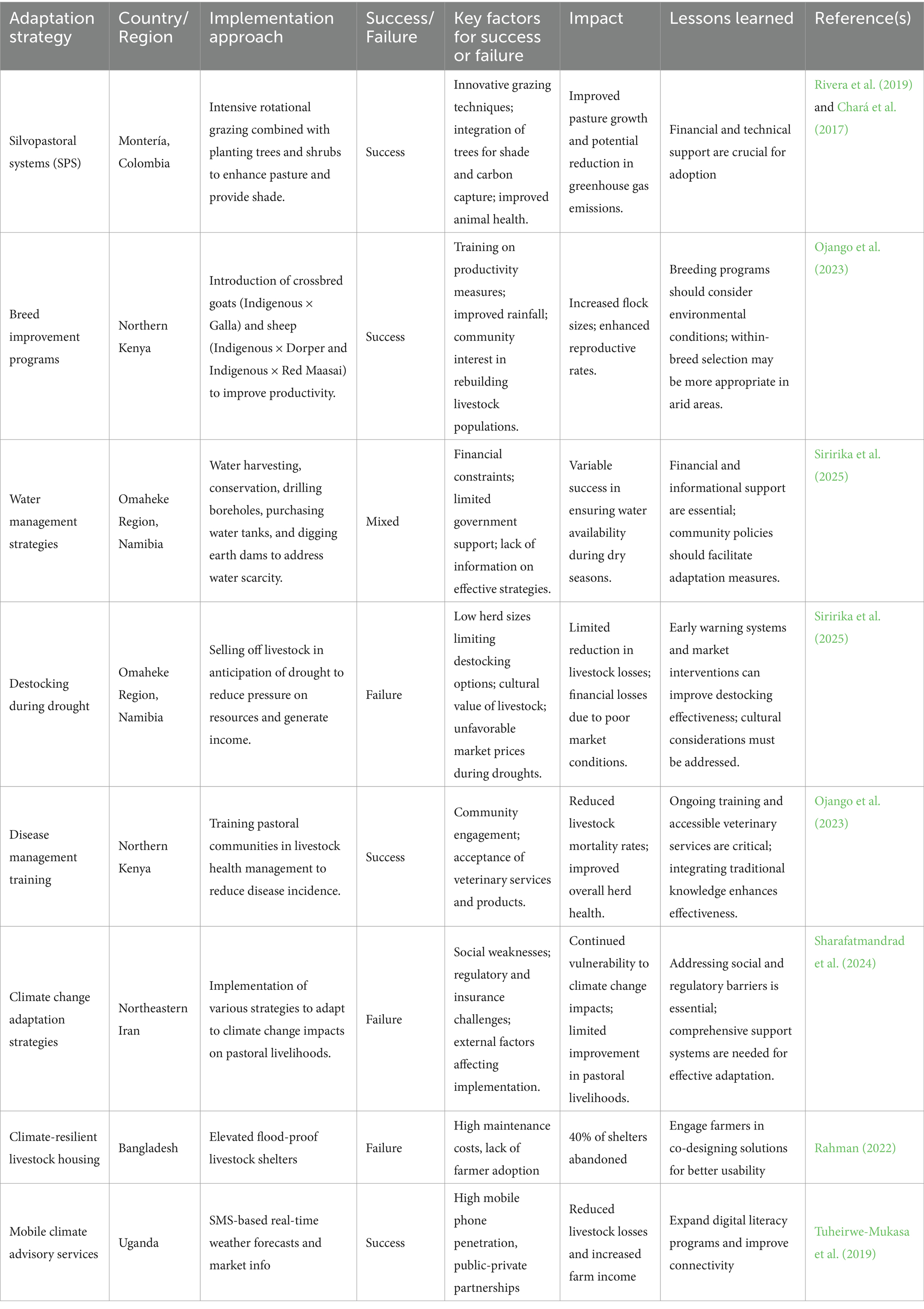
Table 6. Comparative analysis of climate adaptation strategies in livestock production across different regions.
5.2 Challenges in implementing climate change adaptation strategies in LMICs
Several challenges hinder the effective implementation of climate change adaptation strategies. A primary challenge is limited financial resources which significantly impede the adoption of adaptation measures. Many LMICs struggle to allocate sufficient funds for climate initiatives due to competing development priorities. According to Nelson et al. (2016), high poverty levels and limited access to education in LMICs can reduce the capacity of communities to adopt new adaptation strategies. Limited research and development capacity in LMICs also restricts the generation of context-specific adaptation solutions (Obe et al., 2025). This knowledge gap hinders the development and implementation of effective strategies. Furthermore, as noted by Biesbroek et al. (2013), weak institutional frameworks and governance structures also obstruct the coordination and execution of adaptation policies in LMICs. Challenges such as bureaucratic inefficiencies and lack of clear mandates often lead to ineffective adaptation efforts. In addition, inadequate integration of climate adaptation into national policies and development plans can result in fragmented efforts (Lee et al., 2022). Limited access to technology and technical expertise required for some strategies such as data-enable innovations pose significant challenges. Timely response of communities in LMICs climate risks is also limited due to insufficient access to climate information and early warning systems (Guja and Bedeke, 2024). In addition, traditional beliefs and resistance to change can hinder the acceptance of new adaptation practices especially if cultural norms conflict with proposed strategies (Masud et al., 2017). Further, market failures, such as lack of access to credit and insurance, can deter investments in adaptation while geographical challenges, such as susceptibility to natural disasters, can limit the feasibility of certain adaptation strategies. Addressing these challenges requires a multifaceted approach, including strengthening institutional frameworks, enhancing financial mechanisms, improving access to information and technology, and fostering community engagement. Tailored strategies that consider local contexts and actively involve stakeholders are essential for effective climate change adaptation in LMICs.
6 Interdisciplinary approaches to climate change adaptation in livestock production
A successful climate change adaptation strategy for livestock production must be multidisciplinary. This approach should incorporate various fields including agricultural sciences, economics, sociology, policy studies, and environmental sciences. Governments and research institutions must foster collaborations across these disciplines to develop policies, technological solutions, and farmer support programs that align with social, economic, and environmental sustainability. By adopting an interdisciplinary approach, LMICs can enhance their adaptive capacity, ensure food security, and promote sustainable livestock production systems in the face of climate change challenges (Sargison, 2020). Social sciences play a crucial role in adaptation efforts by ensuring that strategies align with the knowledge, traditions, and needs of local communities. Effective adaptation requires participatory approaches where livestock farmers, extension officers, and policymakers collaborate to design context-specific solutions (Andrieu et al., 2019). This could be particularly helpful to encourage farmers to participate in initiatives like the community-based breeding programs suggested. In addition, farmer cooperatives and knowledge-sharing networks can enhance resource pooling and dissemination of best practices for adaptation (Eise et al., 2021). Social perspectives could also be key to addressing resistance to new technologies through behavioral change campaigns. Economic strategies are also essential in making adaptation measures financially viable and attractive to livestock producers in LMICs. Governments, financial institutions, and international organizations should invest in mechanisms that support adaptation at different scales. For example, climate insurance and credit access that provide livestock farmers with insurance schemes against climate-induced losses can enhance resilience and encourage investment in climate-smart technologies (Kramer, 2023). Further, market incentives for climate-smart livestock products such as the implementation of certification programs and premium pricing for sustainably produced meat, dairy, and eggs can encourage farmers to adopt adaptive practices. Public-private partnerships (PPPs) in form of collaboration between governments, research institutions, and agribusinesses can also facilitate investment in precision livestock farming, renewable energy integration, and early warning systems. Sustainable adaptation strategies should focus on minimizing the environmental footprint of livestock farming while improving resilience to climate stressors. This could be through agroecological approaches by integrating silvopastoral systems (trees, shrubs, and pasture) into livestock production systems. This can enhance carbon sequestration, improve forage quality, and provide shade to reduce heat stress in livestock. Further, effective water and feed resource management through promotion of rainwater harvesting, efficient irrigation systems, and the use of climate-resilient fodder crops can ensure sustainable feed availability. Further, utilization of livestock waste for biogas energy can reduce methane emissions while providing renewable energy sources for rural farmers.
7 Future research directions
Future studies should explore a range of methodological approaches to deepen our understanding of climate adaptation strategies in livestock production. One promising avenue is field experiments, which can be used to test the effectiveness of various climate adaptation measures, such as precision feeding, heat stress mitigation strategies, and improved pasture management systems. For instance, on-farm trials incorporating climate-resilient livestock breeds could provide empirical evidence on their performance under changing climatic conditions. Farmer surveys and participatory research are also critical for capturing the lived experiences of livestock keepers and their adaptation strategies. Surveys could explore factors influencing the adoption of climate-smart practices, including socio-economic barriers, institutional support, and access to resources. Longitudinal studies tracking how farmers respond to climate variability over time could offer valuable insights into the sustainability of different adaptation strategies.
Moreover, climate modeling and geospatial analysis can be leveraged to predict the impact of climate change on livestock production at regional and national scales. High-resolution climate models can be integrated with livestock productivity data to simulate potential future scenarios which would allow policymakers to design targeted interventions. Additionally, remote sensing and Geographic Information System (GIS) technologies can be employed to monitor changes in rangeland conditions, water availability, and vegetation cover, all of which are crucial for sustainable livestock production. Given the increasing role of technology in climate adaptation, future research should also focus on data-driven innovations such as Internet of Things (IoT)-enabled livestock monitoring, machine learning applications for prediction, and the use of big data analytics to optimize livestock production systems under climate change. Collaborative research involving multidisciplinary teams including animal scientists, climatologists, economists, and social scientists will also be essential in developing holistic and effective adaptation strategies.
8 Conclusion
The livestock sector in LMICs faces significant challenges from climate change, including heat stress, reduced feed availability, increased disease prevalence, and extreme weather events. Addressing these issues requires the implementation of simplified and context-specific adaptation strategies tailored to the unique environmental and socio-economic conditions of LMICs. Key strategies such as the exploitation of indigenous livestock genetic resources, adoption of climate-smart technologies, precision livestock farming and data-enabled innovations, and diversification of livestock species and breeds are essential for building resilience. Successful case studies indicate that when adaptation strategies are well-funded, community-driven, and supported by strong policies, they yield significant improvements in productivity, resilience, and sustainability. However, fragmented policies, socio-economic constraints, and infrastructure gaps remain significant obstacles to adoption and scaling up these initiatives in many LMICs. Targeted investments in research, capacity building, and policy integration are crucial for bridging these gaps. Further, strengthening institutional frameworks, increasing financial support, and fostering public-private partnerships will be key to accelerating climate adaptation efforts. Additionally, ensuring that adaptation strategies are tailored to local contexts through participatory approaches could enhance their effectiveness and long-term sustainability.
Author contributions
HB: Writing – original draft, Writing – review & editing. SO: Writing – original draft, Writing – review & editing.
Funding
The author(s) declare that no financial support was received for the research and/or publication of this article.
Acknowledgments
The study benefitted from research facilities provided by the Climate Change Adaptation in Africa (CCAA) Programme of the IDRC (Canada) and the DFID (United Kingdom), and coordinated by the global START, Washington, DC, USA as part of a post-doctoral research fellowship awarded to the second author. This is gratefully acknowledged.
Conflict of interest
The authors declare that the research was conducted in the absence of any commercial or financial relationships that could be construed as a potential conflict of interest.
Generative AI statement
The authors declare that no Gen AI was used in the creation of this manuscript.
Publisher’s note
All claims expressed in this article are solely those of the authors and do not necessarily represent those of their affiliated organizations, or those of the publisher, the editors and the reviewers. Any product that may be evaluated in this article, or claim that may be made by its manufacturer, is not guaranteed or endorsed by the publisher.
References
Abbass, K., Qasim, M., Song, H., Murshed, M., Mahmood, H., and Younis, I. (2022). A review of the global climate change impacts, adaptation, and sustainable mitigation measures. Environ. Sci. Pollut. Res. Int. 29, 42539–42559. doi: 10.1007/s11356-022-19718-6
Abdel-Salam, S., Sayed, A. I., Elsayed, M., and Abou-Bakr, S. (2010). Genetic gain in open nucleus breeding scheme to improve milk production in Egyptian buffalo. Livest. Sci. 131, 162–167. doi: 10.1016/j.livsci.2010.03.013
Abidin, Z. U., and Khatoon, A. (2013). Heat stress in poultry and the beneficial effects of ascorbic acid (vitamin C) supplementation during periods of heat stress. Worlds Poult. Sci. J. 69, 135–152. doi: 10.1017/s0043933913000123
Ahlawat, S. P. S., Kumar, P. K. P., Shrivastava, K., and Sahoo, N. R. (2015). Indigenous livestock resources in a changing climate: Indian perspective. Livest. Prod. Clim. Change 214-228. doi: 10.1079/9781780644325.0214
Ahmad, S., and Hossain, F. (2019). A web-based decision support system for smart dam operations using weather forecasts. J. Hydroinf. 21, 687–707. doi: 10.2166/hydro.2019.116
Akon, M. S., and Mia, M. J. (2024). Performance of JICA-funded cyclone shelter in the coastal areas of Bangladesh: an empirical analysis from Pirojpur district. Prog. Disaster Sci. 23:100342. doi: 10.1016/j.pdisas.2024.100342
Alipio, M. I., and Villena, M. L. (2023). Intelligent wearable devices and biosensors for monitoring cattle health conditions: a review and classification. Smart Health 27:100369. doi: 10.1016/j.smhl.2022.100369
Alston, L. J., Libecap, G. D., and Mueller, B. (2000). Land reform policies, the sources of violent conflict, and implications for deforestation in the Brazilian Amazon. J. Environ. Econ. Manag. 39, 162–188. doi: 10.1006/jeem.1999.1103
Ampaire, E., Jassogne, L., Providence, H., Acosta, M., Twyman, J., Winowiecki, L., et al. (2017). Institutional challenges to climate change adaptation: a case study on policy action gaps in Uganda. Environ. Sci. Pol. 75, 81–90. doi: 10.1016/j.envsci.2017.05.013
Andrieu, N., Howland, F., Acosta-Alba, I., Coq, J. L., García, A. M. O., Barón, D. M., et al. (2019). Co-designing climate-smart farming systems with local stakeholders: a methodological framework for achieving large-scale change. Front Sustain Food Syst 3, 1–19. doi: 10.3389/fsufs.2019.00037
Aroonsrimorakot, S., Laiphrakpam, M., and Paisantanakij, W. (2021). Application of innovative eco-friendly energy technology for sustainable agricultural farming. Green Technol. Innov. Sustain. Smart Soc. 211-231. doi: 10.1007/978-3-030-73295-0_10
Ateş, S., Keleş, G., İnal, F., Güneş, A., and Dhehibi, B. (2014). Performance of indigenous and exotic×indigenous sheep breeds fed different diets in spring and the efficiency of feeding system in crop–livestock farming. J. Agric. Sci. 153, 554–569. doi: 10.1017/s0021859614000677
Ayalew, W., Wu, X., Tarekegn, G. M., Chen, M., Liang, C., Tessema, T. S., et al. (2023). Signatures of positive selection for local adaptation of African native cattle populations: a review. J. Integr. Agric. 22, 1967–1984. doi: 10.1016/j.jia.2023.01.004
Ayanlade, A., Oluwaranti, A., Ayanlade, O. S., Borderon, M., Sterly, H., Sakdapolrak, P., et al. (2022). Extreme climate events in sub-Saharan Africa: a call for improving agricultural technology transfer to enhance adaptive capacity. Clim. Serv. 27:100311. doi: 10.1016/j.cliser.2022.100311
Baker, R. L., Mugambi, J. M., Audho, J. O., Carles, A. B., and Thorpe, W. (2004). Genotype by environment interactions for productivity and resistance to gastrointestinal nematode parasites in red Maasai and Dorper sheep. Anim. Sci. 79, 343–353. doi: 10.1017/s1357729800090214
Baker, R. L., Nagda, S., Rodriguez-Zas, S. L., Southey, B. R., Audho, J. O., Aduda, E., et al. (2003). Resistance and resilience to gastrointestinal nematode parasites and relationships with productivity of red Maasai, Dorper and their crossbred lambs in the sub-humid tropics. Anim. Sci. 76, 119–136. doi: 10.1017/s1357729800053388
Bayer, W., Alcock, R., and Gilles, P. (2004). “Going backwards? Moving forward? Nguni cattle in communal KwaZulu–Natal” in Paper presented at: Rural poverty reduction through research for development and transformation (Berlin, Germany: Humboldt–Univ).
Belhadj-Slimen, I., Yerou, H., Ben-Larbi, M., M’Hamdi, N., and Najar, T. (2023). Insects as an alternative protein source for poultry nutrition: a review. Front. Vet. Sci. 10:1200031. doi: 10.3389/fvets.2023.1200031
Benetel, G., Silva, T. S., Fagundes, G. M., Welter, K. C., Melo, F. A., Lobo, A. A., et al. (2022). Essential oils as in vitro ruminal fermentation manipulators to mitigate methane emission by beef cattle grazing tropical grasses. Molecules 27:2227. doi: 10.3390/molecules27072227
Bett, B., Kiunga, P., Gachohi, J., Sindato, C., Mbotha, D., Robinson, T., et al. (2017). Effects of climate change on the occurrence and distribution of livestock diseases. Prev. Vet. Med. 137, 119–129. doi: 10.1016/j.prevetmed.2016.11.019
Biesbroek, R., Klostermann, J., Termeer, C., and Kabat, P. (2013). On the nature of barriers to climate change adaptation. Reg. Environ. Chang. 13, 1119–1129. doi: 10.1007/s10113-013-0421-y
Buytaert, W., Moulds, S., Acosta, L., Bièvre, B. D., Olmos, C. F. G., Villacís, M., et al. (2017). Glacial melt content of water use in the tropical Andes. Environ. Res. Lett. 12:114014. doi: 10.1088/1748-9326/aa926c
Cardoso-Gutiérrez, E., Aranda-Aguirre, E., Jiménez, L. E. R., Castelán-Ortega, O. A., Muñoz-Osorio, G. A., Foggi, G., et al. (2021). Effect of tannins from tropical plants on methane production from ruminants: a systematic review. Vet. Anim. Sci. 14:100214. doi: 10.1016/j.vas.2021.100214
Cervigini, R., Valentini, R., and Santini, M. (2013). Towards climate-resilient development in Nigeria. Washington, DC: Int. Bank Reconstr. Dev.
Chadwick, D. R., Williams, J. R., Lu, Y., Ma, L., Bai, Z., Hou, Y., et al. (2020). Strategies to reduce nutrient pollution from manure management in China. Front. Agric. Sci. Eng. 7, 45–57. doi: 10.15302/j-fase-2019293
Chará, J., Rivera, J. E., Rosales, R. B., Murgueitio, E., Deblitz, C., Reyes, E., et al. (2017). Intensive silvopastoral systems: economics and contribution to climate change mitigation and public policies. Adv Agrofor 12, 2–16. doi: 10.1007/978-3-319-69371-2_16
Cheng, M., McCarl, B. A., and Fei, C. (2022). Climate change and livestock production: a literature review. Atmos. 13:140. doi: 10.3390/atmos13010140
Chevallier, P., Pouyaud, B., Suárez, W., and Condom, T. (2010). Climate change threats to environment in the tropical Andes: glaciers and water resources. Reg. Environ. Chang. 11, 179–187. doi: 10.1007/s10113-010-0177-6
Coly, S. M., Zorom, M., Lèye, B., Karambiri, H., and Guiro, A. (2023). Learning from history of natural disasters in the Sahel: a comprehensive analysis and lessons for future resilience. Environ. Sci. Pollut. Res. 31, 40704–40716. doi: 10.1007/s11356-023-28989-6
Dadrasnia, A., Muñoz, I. B., Hernández, E., Lamkaddam, I. U., Mora, M., Ponsá, S., et al. (2021). Sustainable nutrient recovery from animal manure: a review of current best practice technology and the potential for freeze concentration. J. Clean. Prod. 315:128106. doi: 10.1016/j.jclepro.2021.128106
DeLonge, M. S., and Basche, A. (2017). Managing grazing lands to improve soils and promote climate change adaptation and mitigation: a global synthesis. Renew. Agric. Food Syst. 33, 267–278. doi: 10.1017/s1742170517000588
Du, H., Dang, K. K., Nguyễn, H. Q., and Rijswick, H. V. (2022). A framework for reviewing laws and policies for climate resilience: the case of the Vietnamese Mekong Delta. J. Environ. Plan. Manag. 66, 1280–1304. doi: 10.1080/09640568.2022.2026308
Eise, J., Lambert, N., and Wiemer, E. C. (2021). Leveraging communities’ network strengths to support climate change adaptation information-sharing: a study with coffee farmers in Risaralda, Colombia. Clim. Chang. 168, 1–19. doi: 10.1007/s10584-021-03206-w
Erdaw, M. M. (2023). Contribution, prospects and trends of livestock production in sub-Saharan Africa: a review. Int. J. Agric. Sustain. 21, 1–15. doi: 10.1080/14735903.2023.2247776
FAO (2016) in Livestock-related interventions during emergencies: The how-to-do-it manual. eds. P. Ankers, S. Bishop, S. Mack, and K. Dietze (Rome: FAO Animal Production and Health Manual No. 18).
FAO (2020). FAOSTAT statistical database. Rome: Food and Agriculture Organization of the United Nations.
Fedrigo, J. K., Báez, F., Cruz, R. S., and Viñoles, C. (2021). Heat tolerance in cows of British breeds and their crosses with Bonsmara under grazing conditions. J. Therm. Biol. 102:103118. doi: 10.1016/j.jtherbio.2021.103118
Godde, C., Mason-D’Croz, D., Mayberry, D., Thornton, P. K., and Herrero, M. (2021). Impacts of climate change on the livestock food supply chain: a review of the evidence. Glob. Food Secur. 28:100488. doi: 10.1016/j.gfs.2020.100488
Gonzalez, L., Kyriazakis, I., and Tedeschi, L. (2018). Review: precision nutrition of ruminants: approaches, challenges and potential gains. Anim. 12, s246–s261. doi: 10.1017/s1751731118002288
Guja, M. M., and Bedeke, S. B. (2024). Smallholders’ climate change adaptation strategies: exploring effectiveness and opportunities to be capitalized. Environ. Dev. Sustain. doi: 10.1007/s10668-024-04750-y
Haile, A., Joshi, B. K., Ayalew, W., Tegegne, A., and Singh, A. (2011). Genetic evaluation of Ethiopian Boran cattle and their crosses with Holstein Friesian for growth performance in Central Ethiopia. J. Anim. Breed. Genet. 128, 133–140. doi: 10.1111/j.1439-0388.2010.00882.x
Henchiri, M., Zhang, J., Li, S., Essifi, B., and Kalisa, W. (2024). Comprehensive assessment of drought vulnerability and resilience over north and West Africa during 1980–2100. Agric. Water Manag. 296:108804. doi: 10.1016/j.agwat.2024.108804
Henry, B., Eckard, R., and Beauchemin, K. A. (2018). Adaptation of ruminant livestock production systems to climate changes. Anim. 12, s445–s456. doi: 10.1017/s1751731118001301
Herrero, M., Thornton, P. K., Kruska, R., and Reid, R. S. (2008). Systems dynamics and the spatial distribution of methane emissions from African domestic ruminants to 2030. Agric. Ecosyst. Environ. 126, 122–137. doi: 10.1016/j.agee.2008.01.017
Herrero, M., Thornton, P. K., Notenbaert, A. M., Wood, S., Msangi, S., Freeman, H. A., et al. (2010). Smart investments in sustainable food production: revisiting mixed crop-livestock systems. Science 327, 822–825. doi: 10.1126/science.1183725
Hills to Ocean (2023). Available online at: https://2023.snrd-asia.org/mekong-delta-climate-resilience-programme/ (Accessed March 18, 2025)
Hoffman, M. T., and Vogel, C. (2008). Climate change impacts on African rangelands. Rangelands 30, 12–17. doi: 10.0.8.63/1551-501X(2008)30[12:CCIOAR]2.0.CO;2
Igbatayo, S., Afolabi, B., Danladi, J. D., Awoyemi, B. O., and Babalola, O. (2022). Exploring disaster risk reduction strategies in the Sahel: a multicountry study of Burkina Faso, Chad, Mauritania, Niger, and Senegal. Disaster Risk Reduct. Resil. 333-359. doi: 10.1007/978-3-030-72196-1_13
ILRI (2025). The livestock and climate solutions hub. Available online at: https://www.ilri.org/research/projects/livestock-and-climate-solutions-hub. (Accessed 21 March, 2025)
IPCC (2001). Climate change: Synthesis report. Contribution of working groups I, II, and III to the third assessment report of the intergovernmental panel on climate change. Cambridge: Cambridge University Press.
IPCC (2007). Climate change: The physical science basis. Contribution of working group I to the fourth assessment report of the intergovernmental panel on climate change. Cambridge: Cambridge University Press.
IPCC (2013). Climate change: The physical science basis. Contribution of working group I to the fifth assessment report of the intergovernmental panel on climate change. Cambridge: Cambridge Univ. Press.
Islam, M. R. (2025). Tropical cyclones in Bangladesh: retrospective analysis of storm information, disaster statistics, and preparedness. Environ. Res. Commun. 7:015003. doi: 10.1088/2515-7620/ada239
Islam, M. A., Lomax, S., Doughty, A., Islam, M. K., Jay, O., Thomson, P., et al. (2021). Automated monitoring of cattle heat stress and its mitigation. Front. Anim. Sci. 2:737213. doi: 10.3389/fanim.2021.737213
Islam, A., Shaw, R., and Mallick, F. (2013). Bangladesh climate change strategy and action plans. Clim. Change Adapt. Actions Bangladesh 1–20. doi: 10.1007/978-4-431-54249-0_7
Jalón, S. G. D., Silvestri, S., and Barnes, A. P. (2016). The potential for adoption of climate-smart agricultural practices in sub-Saharan livestock systems. Reg. Environ. Chang. 17, 399–410. doi: 10.1007/s10113-016-1026-z
Jameel, M. K., Mustafa, M. A., Ahmed, H. S., Mohammed, A. J., Ghazy, H., Shakir, M. N., et al. (2024). Biogas: production, properties, applications, economic and challenges: a review. Results Chem. 7:101549. doi: 10.1016/j.rechem.2024.101549
Jiménez-Ocampo, R., Montoya-Flores, M. D., Pámanes-Carrasco, G. A., Herrera-Torres, E., Arango, J., Estarrón-Espinosa, M., et al. (2022). Impact of orange essential oil on enteric methane emissions of heifers fed Bermudagrass hay. Front. Vet. Sci. 9:863910. doi: 10.3389/fvets.2022.863910
Karnuah, A. B., and Dunga, G. (2018). Community-based breeding program for improved goat production in Liberia. MOJ Curr. Res. Rev. 1, 216–221. doi: 10.15406/mojcrr.2018.01.00036
Katiyatiya, C. L., Bradley, G., and Muchenje, V. (2017). Thermotolerance, health profile, and cellular expression of hsp90ab1 in Nguni and Boran cows raised on natural pastures under tropical conditions. J. Therm. Biol. 69, 85–94. doi: 10.1016/j.jtherbio.2017.06.009
Kenya Climate Smart Agriculture Strategy (2025). Available online at: https://www.kcsap.go.ke/home (Accessed 19 March, 2025)
Khan, S. (2018). Recent advances in the role of insects as an alternative protein source in poultry nutrition. J. Appl. Anim. Res. 46, 1144–1157. doi: 10.1080/09712119.2018.1474743
Kramer, B. (2023). Climate insurance: opportunities for improving agricultural risk management in Kenya. Food Syst Transform Kenya 285.
Kristiansen, S., Painter, J., and Shea, M. M. (2020). Animal agriculture and climate change in the US and UK elite media: volume, responsibilities, causes and solutions. Environ. Commun. 15, 153–172. doi: 10.1080/17524032.2020.1805344
Kulhari, K., Singh, B. V. R., and Mishra, R. (2024). Investigating the trend and pattern of heat waves in North-Western Rajasthan (2012–2023), in the context of climate variability. Adv. Geogr. Environ. Sci. 316-330. doi: 10.1007/978-981-96-0605-4_18
Lee, S., Paavola, J., and Dessai, S. (2022). Towards a deeper understanding of barriers to national climate change adaptation policy: a systematic review. Clim. Risk Manag. 35:100414. doi: 10.1016/j.crm.2022.100414
LEGS (2014). Livestock emergency guidelines and standards. 2nd Edn. Rugby, UK: Practical Action Publishing.
Liberman, M. (2021). Climate change, wetland management and alpaca pastoralism in the Bolivian high Andes Mountains. Geobot. Stud. 65-98. doi: 10.1007/978-3-030-74950-7_5
Lipper, L., and Zilberman, D. (2017). A short history of the evolution of the climate-smart agriculture approach and its links to climate change and sustainable agriculture debates. Nat. Resour. Manag. Policy 52, 13–30. doi: 10.1007/978-3-319-61194-5_2
Llorens, B., Pomar, C., Goyette, B., Rajagopal, R., Andretta, I., Latorre, M. A., et al. (2024). Precision feeding as a tool to reduce the environmental footprint of pig production systems: a life cycle assessment. J. Anim. Sci. 102. doi: 10.1093/jas/skae225
Low, G., and Meuwissen, M. (2023). Mixed farming and agroforestry systems: a systematic review on value chain implications. Agric. Syst. 206:103606. doi: 10.1016/j.agsy.2023.103606
Mapiye, C., Chimonyo, M., Dzama, K., Raats, J., and Mapekula, M. (2009). Opportunities for improving Nguni cattle production in the smallholder farming systems of South Africa. Livest. Sci. 124, 196–204. doi: 10.1016/j.livsci.2009.01.013
Masters, D. G., Blache, D., Lockwood, A., Maloney, S. K., Norman, H. C., Refshauge, G., et al. (2023). Shelter and shade for grazing sheep: implications for animal welfare and production and for landscape health. Anim. Prod. Sci. 63, 623–644. doi: 10.1071/an22225
Masud, M. M., Azam, M. N., Mohiuddin, M., Banna, H., Akhtar, R., Alam, A. S. A. F., et al. (2017). Adaptation barriers and strategies towards climate change: challenges in the agricultural sector. J. Clean. Prod. 156, 698–706. doi: 10.1016/j.jclepro.2017.04.060
Mathew, E. J., and Mathew, L. (2023). Conservation of landraces and indigenous breeds: an investment for the future. Sustain. Dev. Biodivers. 30, 291–321. doi: 10.1007/978-981-19-5841-0_12
Matocha, J., Schroth, G., Hills, T., and Hole, D. (2012). Integrating climate change adaptation and mitigation through agroforestry and ecosystem conservation. Adv. Agrofor. 9, 105–126. doi: 10.1007/978-94-007-4676-3_9
McGurrin, A., Maguire, J., Tiwari, B. K., and Garcia-Vaquero, M. (2023). Anti-methanogenic potential of seaweeds and seaweed-derived compounds in ruminant feed: current perspectives, risks, and future prospects. J. Anim. Sci. Biotechnol. 14:145. doi: 10.1186/s40104-023-00946-w
Murray-Tortarolo, G. N., and Jaramillo, V. J. (2019). The impact of extreme weather events on livestock populations: the case of the 2011 drought in Mexico. Clim. Chang. 153, 79–89. doi: 10.1007/s10584-019-02373-1
Naim, Z., Asaduzzaman, M., Akter, M., and Islam, M. A. (2023). Impact of climate change on livestock production in Bangladesh - a review. Bangladesh J. Anim. Sci. 52, 1–14. doi: 10.3329/bjas.v52i1.65311
National Strategy on Climate Change and Low Carbon Development for Rwanda. (2011). Baseline Report. Available online at: https://greenfund.rw/sites/default/files/2021-06/Rwanda%27s%20Climate%20Change%20%26%20Low%20Carbon%20Development%20Strategy%20Baseline%20Report.pdf (Accessed 20 March, 2025)
Neethirajan, S. (2017). Recent advances in wearable sensors for animal health management. Sens. Bio-Sens. Res. 12, 15–29. doi: 10.1016/j.sbsr.2016.11.004
Nelson, D. R., Lemos, M. C., Eakin, H., and Lo, Y. (2016). The limits of poverty reduction in support of climate change adaptation. Environ. Res. Lett. 11:094011. doi: 10.1088/1748-9326/11/9/094011
Njoku, D., and Ejiogu, C. O. (1999). On-farm trials of an integrated fish-cum-poultry farming system using indigenous chickens. Aquac. Res. 30, 399–408. doi: 10.1046/j.1365-2109.1999.00325.x
Nyamushamba, G. B., Mapiye, C., Tada, O., Halimani, T. E., and Muchenje, V. (2016). Conservation of indigenous cattle genetic resources in southern Africa’s smallholder areas: turning threats into opportunities—a review. Asian Australas. J. Anim. Sci. 30, 603–621. doi: 10.5713/ajas.16.0024
Nyong, A., Adesina, F. A., and Elasha, B. O. (2007). The value of indigenous knowledge in climate change mitigation and adaptation strategies in the African Sahel. Mitig. Adapt. Strateg. Glob. Change 12, 787–797. doi: 10.1007/s11027-007-9099-0
Obe, M. M., Kpadé, C. P., and Singbo, A. (2025). Identifying and overcoming barriers to climate change adaptation innovations among smallholder farmers in developing countries: a literature review and meta-analysis. Clim. Chang. 178, 49–61. doi: 10.1007/s10584-025-03892-w
Odongo, R. A., Schrieks, T., Streefkerk, I., Moel, H. D., Busker, T., Haer, T., et al. (2025). Drought impacts and community adaptation: perspectives on the 2020–2023 drought in East Africa. Int. J. Disaster Risk Reduct. 119:105309. doi: 10.1016/j.ijdrr.2025.105309
Ojango, J. M., Gitau, J., Ndiwa, N., Recha, J., Gachora, J., and Muigai, A. W. T. (2023). Integration and adoption of climate-resilient management practices for enhanced productivity of sheep and goats in pastoral communities of northern Kenya. Pastoralism 13, 1–12. doi: 10.1186/s13570-023-00277-5
Olaniyan, O. F., Oladejo, O. A., Adeola, A. C., Bello, S. F., Bashiru, H. A., and Oseni, S. O. (2024). Integration of genomics into community-based breeding programmes for chickens: an overview of opportunities, challenges, and potential benefits. Worlds Poult. Sci. J. 80, 977–997. doi: 10.1080/00439339.2024.2354194
Oseni, S.O. (2018). Indigenous Livestock Innovations in Africa (iLINOVA): A Project Report. In I.C. Okoli, I.P. Ogbuewu, O.O. Emenalom, & B.O. Esonu (Eds.), Proceedings of the 43rd Annual Conference of the Nigerian Society for Animal Production, March 18–22, 2018, Federal University of Technology, Owerri, Nigeria. Available online: https://mail.njap.org.ng/index.php/njap/article/view/4252/3093 (Accessed March 25, 2025).
Oseni, S. O., and Bashiru, H. A. (2022). Some productivity indices of heterogeneous rabbits under a pastured system. Niger. J. Anim. Sci. 24, 79–90.
Oseni, S., Bashiru, H., Lawal, R., Ajayi, A., Akintaro, O., and Munir, K. (2025). Monitoring environmental factors for optimal health and welfare of broiler chickens using sensor technology in Southwest Nigeria. Lect. Notes Comput. Sci 15511, 377–393. doi: 10.1007/978-3-031-83472-1_25
Oseni, S., and Bebe, O. (2010). Climate change, genetics of adaptation and livestock production in low-input systems. Proceedings of the 2nd International Conference: Climate, Sustainability and Development in Semi-Arid Regions (ICID+18), August 16–20, 2010, Fortaleza, Ceará, Brazil.
Oseni, S. O., Yakubu, A., and Aworetan, A. R. (2017). Nigerian West African Dwarf Goats. In: Simões, J., Gutiérrez, C. (eds) Sustainable Goat Production in Adverse Environments: Volume II. Springer, 91–110. doi: 10.1007/978-3-319-71294-9_8
Otani, S., Mu, H., Onishi, K., Kurozawa, Y., and Shinoda, M. (2015). Risk prediction of the effects of severe winter disasters (dzud) on nomadic health and society in Mongolia with regard to loss of livestock. Int. J. Epidemiol. 44:i178. doi: 10.1093/ije/dyv096.260
Pabón-Caicedo, J. D., Arias, P. A., Carril, A. F., Espinoza, J. C., Fita, L., Goubanova, K., et al. (2020). Observed and projected hydroclimate changes in the Andes. Front. Earth Sci. 8, 1–29. doi: 10.3389/feart.2020.00061
Papakonstantinou, G. I., Voulgarakis, N., Terzidou, G., Fotos, L., Giamouri, E., and Papatsiros, V. G. (2024). Precision livestock farming technology: applications and challenges of animal welfare and climate change. Agriculture 14:620. doi: 10.3390/agriculture14040620
Pardo, G. O., Prado, A. D., Álvarez, J. F., Yáñez-Ruíz, D. R., and Belanche, A. (2022). Influence of precision livestock farming on the environmental performance of intensive dairy goat farms. J. Clean. Prod. 351:131518. doi: 10.1016/j.jclepro.2022.131518
Parikh, S. S., Patbandha, T. K., Gamit, P. M., and Savaliya, B. D. (2024). Seasonal influence on reproductive traits in Gir (Bos indicus) heifers. Biol. Rhythm. Res. 55, 509–519. doi: 10.1080/09291016.2024.2399561
Patbandha, T., Sabapara, G. P., Savaliya, B. D., Dash, S. K., Parikh, S., and Ali, M. (2020). Physical characteristics and production performance of Gir cattle in India. Int. J. Livest. Res. 10, 1–7. doi: 10.5455/ijlr.20200608100242
Pérez, J. L. H., and Jerez-Ramírez, D. O. (2023). The impacts of drought disasters on Mexican agriculture: an interpretation from the perspective of the political economy of disasters. Clim. Change Agric. Soc. 65-84. doi: 10.1007/978-3-031-28251-5_4
Quandt, A., Neufeldt, H., and Gorman, K. (2023). Climate change adaptation through agroforestry: opportunities and gaps. Curr. Opin. Environ. Sustain. 60:101244. doi: 10.1016/j.cosust.2022.101244
Radeny, M. A., Rao, E., Ogada, M. J., Recha, J. W., and Solomon, D. (2022). Impacts of climate-smart crop varieties and livestock breeds on the food security of smallholder farmers in Kenya. Food Secur. 14, 1511–1535. doi: 10.1007/s12571-022-01307-7
Rahman, R. (2022). Community resilience to climate change through disaster-resilient housing practice in coastal areas: a case study of Dacope Upazila in Khulna. MSc thesis, Institute of Water and Flood Management (IWFM), Bangladesh University of Engineering and Technology. Available online at: http://lib.buet.ac.bd:8080/xmlui/handle/123456789/6540 (Accessed 24 March, 2025).
Rahman, M. M., Arif, M., Hossain, M. T., Almohamad, H., Dughairi, A. A. A., Al-Mutiry, M., et al. (2023). Households’ vulnerability assessment: empirical evidence from cyclone-prone area of Bangladesh. Geosci. Lett. 10:26. doi: 10.1186/s40562-023-00280-z
Rao, M. P., Davi, N., D’Arrigo, R., Skees, J. R., Nachin, B., Leland, C., et al. (2015). Dzuds, droughts, and livestock mortality in Mongolia. Environ. Res. Lett. 10:074012. doi: 10.1088/1748-9326/10/7/074012
Ravindra, K., Bhardwaj, S., Ram, C., Goyal, A., Singh, V., Venkataraman, C., et al. (2024). Temperature projections and heatwave attribution scenarios over India: a systematic review. Heliyon 10:e26431. doi: 10.1016/j.heliyon.2024.e26431
Reid, H., Ampomah, G., Olazábal Prera, M. I., Rabbani, G., and Zvigadza, S. (2012). Southern voices on climate policy choices: Analysis of and lessons learned from civil society advocacy on climate change. London: Int. Inst. Environ. Dev.
Renaudeau, D., Collin, A., Yahav, S., Basilio, V. D., Gourdine, J., and Collier, R. J. (2012). Adaptation to hot climate and strategies to alleviate heat stress in livestock production. Anim. 6, 707–728. doi: 10.1017/s1751731111002448
Rivera, J. E., Chará, J., Murgueitio, E., Molina, J. J., and Rosales, R. B. (2019). Feeding Leucaena to dairy cows in intensive silvopastoral systems in Colombia and Mexico. Trop Grassl-Forrajes Trop 7, 370–374. doi: 10.17138/tgft(7)370-374
Rojas-Downing, M. M., Nejadhashemi, A. P., Harrigan, T. M., and Woznicki, S. A. (2017). Climate change and livestock: impacts, adaptation, and mitigation. Clim. Risk Manag. 16, 145–163. doi: 10.1016/j.crm.2017.02.001
Rosegrant, M. W., Fernandez, M., and Sinha, A. (2009). “Looking into the future for agriculture and AKST” in Int. assess. Agric. Knowl. Sci. Technol. Dev. (IAASTD). Agric. Crossroads. eds. B. D. McIntyre, H. R. Herren, J. Wakhungu, and R. T. Watson (Washington, DC: Island Press), 307–376.
Rust, J. M. (2018). The impact of climate change on extensive and intensive livestock production systems. Anim. Front. 9, 20–25. doi: 10.1093/af/vfy028
Sanusi, A., and Oseni, S. O. (2020). Nigerian Fulani ecotype chickens: estimation of growth curve parameters. Gabj. 4, 1–13. doi: 10.46325/gabj.v4i1.70
Sargison, N. (2020). The critical importance of planned small ruminant livestock health and production in addressing global challenges surrounding food production and poverty alleviation. N. Z. Vet. J. 68, 136–144. doi: 10.1080/00480169.2020.1719373
Savian, J. V., Schons, R. M. T., Filho, W. S., Zubieta, Á. S., Kindlein, L., Bindelle, J., et al. (2021). ‘Rotatinuous’ stocking as a climate-smart grazing management strategy for sheep production. Sci. Total Environ. 753:141790. doi: 10.1016/j.scitotenv.2020.141790
Schmidt, E., and Tadesse, F. (2019). The impact of sustainable land management on household crop production in the Blue Nile Basin. Ethiopia. Land Degrad. Dev. 30, 777–787. doi: 10.1002/ldr.3266
Scoones, I. (2022). Livestock, methane, and climate change: the politics of global assessments. WIREs. Clim. Chang. 14:e790. doi: 10.1002/wcc.790
Sekaran, U., Lai, L., Ussiri, D. A., Kumar, S., and Clay, S. A. (2021). Role of integrated crop-livestock systems in improving agriculture production and addressing food security – a review. J. Agric. Food Res. 5:100190. doi: 10.1016/j.jafr.2021.100190
Sharafatmandrad, M., Sarvestani, A. A., Shahraki, M., and Nafooti, M. H. (2024). Uncovering the reasons behind the failure of pastoralists in adopting climate change adaptation strategies. Sci. Rep. 14:20602. doi: 10.1038/s41598-024-70818-4
Shelley, A. N., Lau, D. L., Stone, A. E., and Bewley, J. (2016). Short communication: measuring feed volume and weight by machine vision. J. Dairy Sci. 99, 386–391. doi: 10.3168/jds.2014-8964
Shoko, A. P., Limbu, S. M., Lamtane, H. A., Kishe-Machumu, M. A., Sekadende, B. C., Ulotu, E. E., et al. (2019). The role of fish-poultry integration on fish growth performance, yields and economic benefits among smallholder farmers in sub-Saharan Africa. Tanzania. Afr. J. Aquat. Sci. 44, 15–24. doi: 10.2989/16085914.2018.1555512
Shrivastava, K., Sinha, R., Pathak, P., and Nayak, V. K. (2018). Open nucleus breeding system to improve livestock population: a review. Int. J. Pure Appl. Biosci. 6, 892–899. doi: 10.18782/2320-7051.6579
Singh, A. K., Mishra, Y. D., Gupta, S., and Priyanka, J. (2022). Impact of climate-resilient agricultural technologies and social interaction under NICRA project in Madhya Pradesh. Pharma Innov. J. 11, 817–819. doi: 10.22271/tpi.2022.v11.i9Sj.15399
Siririka, N. A. T., Charamba, V., Mupangwa, J., Shipandeni, M. N. T., and Kahumba, A. (2025). Climate change adaptation strategies among smallholder livestock farmers in Namibia's Omaheke region. Discov. Sustain. 6, 1–19. doi: 10.1007/s43621-025-00963-x
Skidmore, M. E., Moffette, F., Rausch, L., Christie, M., Munger, J., and Gibbs, H. K. (2021). Cattle ranchers and deforestation in the Brazilian Amazon: production, location, and policies. Glob. Environ. Change 68:102280. doi: 10.1016/j.gloenvcha.2021.102280
Slayi, M., and Jaja, I. F. (2024). Integrating mixed livestock systems to optimize forage utilization and modify woody species composition in semi-arid communal rangelands. Land 13:1945. doi: 10.3390/land13111945
Speranza, C. I. (2010). Drought coping and adaptation strategies: understanding adaptations to climate change in agro-pastoral livestock production in Makueni district. Kenya. Eur. J. Dev. Res. 22, 623–642. doi: 10.1057/ejdr.2010.39
Steinfeld, H., Gerber, P., Wassenar, T., Castel, V., Rosales, M., and De Haan, C. (2006). Livestock’s long shadow: Environmental issues and options. Rome: FAO.
Sujatha, S., and Bhat, R. (2015). Resource use and benefits of mixed farming approach in arecanut ecosystem in India. Agric. Syst. 141, 126–137. doi: 10.1016/j.agsy.2015.10.005
Sullivan, M. J., Cawdell-Smith, A. J., Mader, T. L., and Gaughan, J. B. (2011). Effect of shade area on performance and welfare of short-fed feedlot cattle. J. Anim. Sci. 89, 2911–2925. doi: 10.2527/jas.2010-3152
Tactacan, G. B., Guenter, W., Lewis, N. J., Rodríguez-Lecompte, J. C., and House, J. D. (2009). Performance and welfare of laying hens in conventional and enriched cages. Poult. Sci. 88, 698–707. doi: 10.3382/ps.2008-00369
Tavirimirwa, B., Manzungu, E., Washaya, S., Ncube, S., Ncube, S., Mudzengi, C. P., et al. (2019). Efforts to improve Zimbabwe communal grazing areas: a review. Afr. J. Range Forage Sci. 36, 73–83. doi: 10.2989/10220119.2019.1602566
Thomas, V., and López, R. (2015). Global increase in climate-related disasters. SSRN Electron. J. doi: 10.2139/ssrn.2709331
Thornton, P. K. (2010). Livestock production: recent trends, future prospects. Philos. Trans. R. Soc.Lond. B Biol. Sci. 365, 2853–2867. doi: 10.1098/rstb.2010.0134
Thornton, P. K., Ericksen, P. J., Herrero, M., and Challinor, A. J. (2014). Climate variability and vulnerability to climate change: a review. Glob. Change Biol. 20, 3313–3328. doi: 10.1111/gcb.12581
Thornton, P. K., and Gerber, P. (2010). Climate change and the growth of the livestock sector in developing countries. Mitig. Adapt. Strateg. Glob. Change 15, 169–184. doi: 10.1007/s11027-009-9210-9
Thornton, P. K., and Herrero, M. (2008). Climate change, vulnerability, and livestock keepers: Challenges for poverty alleviation. May, Tunisia: Livest. Glob. Clim. Change Conf. Proc.
Thornton, P. K., and Herrero, M. (2014). Climate change adaptation in mixed crop-livestock systems in developing countries. Glob. Food Secur. 3, 99–107. doi: 10.1016/j.gfs.2014.02.002
Thornton, P. K., and Herrero, M. (2015). Adapting to climate change in the mixed crop and livestock farming systems in sub-Saharan Africa. Nat. Clim. Chang. 5, 830–836. doi: 10.1038/nclimate2754
Thornton, P. K., Nelson, G. C., Mayberry, D., and Herrero, M. (2022). Impacts of heat stress on global cattle production during the 21st century: a modelling study. Lancet Planet. Health 6, e192–e201. doi: 10.1016/s2542-5196(22)00002-x
Thornton, P. K., van de Steeg, J., Notenbaert, A. M. O., and Herrero, M. (2009). The impacts of climate change on livestock and livestock systems in developing countries: a review of what we know and what we need to know. Agric. Syst. 101, 113–127. doi: 10.1016/j.agsy.2009.05.002
Tohiran, K. A., Nobilly, F., Zulkifli, R., Yahya, M. S., Norhisham, A. R., Rasyidi, M. Z., et al. (2023). Multi-species rotational grazing of small ruminants regenerates undergrowth vegetation while controlling weeds in the oil palm silvopastoral system. Agric. Syst. 210:103720. doi: 10.1016/j.agsy.2023.103720
Tuheirwe-Mukasa, D., Haveraaen, M., Sansa-Otim, J., Kanagwa, B., and Mujuni, G. (2019). The efficacy of ICT in weather forecast information dissemination: evidence from farming communities in Mbale and Rakai districts. Uganda. Clim Change Manag 571-593. doi: 10.1007/978-3-030-12974-3_25
Twine, R. (2021). Emissions from animal agriculture—16.5% is the new minimum figure. Sustain. For. 13:6276. doi: 10.3390/su13116276
USDA (2013). Climate change and agriculture in the United States: Effects and adaptation. Washington, DC: USDA technical bulletin.
Valtorta, S. E. (2009). Animal production in a changing climate: Impacts and mitigation. Rafaela, Argentina: National Institute of Agricultural Technology.
Venkateswarlu, B., Maheswari, M., Srinivasa Rao, M., Rao, V. U. M., Srinivasa Rao, C., Reddy, K. S., et al. (2013). National Initiative on climate resilient agriculture (NICRA), research highlights (2012–13). Hyderabad: Cent. Res. Inst. Dryl. Agric, 111.
Waaswa, A., Nkurumwa, A. O., Kibe, A., and Kipkemoi, J. N. (2024). Adapting agriculture to climate change: institutional determinants of adoption of climate-smart agriculture among smallholder farmers in Kenya. Cogent Food Agric. 10, 1–18. doi: 10.1080/23311932.2023.2294547
Wasti, S. S., Sah, N., and Mishra, B. (2020). Impact of heat stress on poultry health and performances, and potential mitigation strategies. Animals 10:1266. doi: 10.3390/ani10081266
World Bank (2020). Sustainable Land Management Program. Available online at: https://documents1.worldbank.org/curated/en/126731603826296434/pdf/Ethiopia-Sustainable-Land-Management-Project-I-and-II.pdf (Accessed 18th March, 2025).
World Bank. (2020). World development indicators. The World Bank, Washington, DC 20433, USA. Available online at: https://datacatalog.worldbank.org/dataset/world-development-indicators (Accessed November 18, 2024).
World Bank (2024). World Bank country classifications by income level for 2024-2025. Available online at: https://blogs.worldbank.org/en/opendata/world-bank-country-classifications-by-income-level-for-2024-2025 (Accessed 21st March, 2025).
World Bank (2025). Climate-Smart Agriculture in Malawi. Available online at: https://climateknowledgeportal.worldbank.org/sites/default/files/2019-06/CSA%20_Profile_Malawi.pdf (Accessed 21 March, 2025)
Yapi-Gnaore, C. V., Rege, J. E. O., and Alemayehu, N. (1997b). Analysis of an open nucleus breeding programme for Djallonke sheep in the Ivory Coast. 2. Response to selection on body weights. Anim. Sci. 64, 301–307. doi: 10.1017/s1357729800015873
Yapi-Gnaore, C. V., Rege, J. E. O., and Dagnogo, B. (1997a). Analysis of an open nucleus breeding programme for Djallonke sheep in the Ivory Coast. 1. Examination of non-genetic factors. Anim. Sci. (UK) 64, 291–300. doi: 10.1017/s1357729800015861
Keywords: climate change, livestock production, adaptation strategies, resilience, food security, indigenous breeds, precision livestock farming
Citation: Bashiru HA and Oseni SO (2025) Simplified climate change adaptation strategies for livestock development in low-and middle-income countries. Front. Sustain. Food Syst. 9:1566194. doi: 10.3389/fsufs.2025.1566194
Edited by:
Fisayo Oretomiloye, Dalhousie University, CanadaReviewed by:
Mhlangabezi Slayi, University of Fort Hare, South AfricaAlpha Kargbo, University of the Gambia, Gambia
Bessy Kathambi, University of Nairobi, Kenya
Copyright © 2025 Bashiru and Oseni. This is an open-access article distributed under the terms of the Creative Commons Attribution License (CC BY). The use, distribution or reproduction in other forums is permitted, provided the original author(s) and the copyright owner(s) are credited and that the original publication in this journal is cited, in accordance with accepted academic practice. No use, distribution or reproduction is permitted which does not comply with these terms.
*Correspondence: Hameed Akande Bashiru, aGJhc2hpcnVAb2F1aWZlLmVkdS5uZw==