- 1Animal Nutrition and Welfare Service, Department of Animal and Food Sciences, Universitat Autònoma de Barcelona, Bellaterra, Spain
- 2Delacon Biotechnik GmbH, Engerwitzdorf, Austria
- 3Department of Animal Genomics, Centre for Research in Agricultural Genomics, Universitat Autònoma de Barcelona, Bellaterra, Spain
This research aims to determine whether a specific blend of phytogenic compounds (BPC) supplemented in gestating hyperprolific sow diets can promote prenatal maternal effects in terms of piglet gut function and morphology. Twenty-eight (Landrace × Yorkshire) gilts and sows (parity 0 to 7) were randomly distributed by parity number and body weight into two dietary treatments: unsupplemented Control (CON) (n = 14) or CON diet supplemented with 1 g/kg feed of BPC during gestation (n = 14). The BPC supplementation during gestation of sows downregulated the neonate piglets' jejunal genes involved in oxidation (SOD2) and nutrient transport (SLC16A1/MCT1, SLC11A2/DMT1, and SLC39A/ZIP4), while IFNG and CLDN4 related to immune response and barrier function, respectively, were upregulated (q < 0.10). In addition, the jejunal villus height and the ratio of the villus height to crypt depth tended to increase (p < 0.10), while goblet cell volume density was higher (p < 0.05) in BPC compared to CON. In conclusion, dietary supplementation of BPC in gestating diets for hyperprolific sows influences neonatal histomorphology and expression of genes related to the intestinal function and health.
Introduction
In modern pig breeds, hyperprolific dam-line sows are characterized by a high number of fetuses in utero compared to conventional sow lines. This has increased the litter size; however, it has also increased the percentage of piglets affected with varying degrees of intrauterine growth retardation (IUGR) and a low birth weight (LBW) (1). The IUGR characteristics may be derived from a reduced utero-placental blood flow (2), as well as increased oxidative damage, decreased mitochondrial function, impaired angiogenesis, and downregulated protein levels of glucose transporters in placentae for LBW piglets (3). Indeed, limitations in available nutrients supplied by the sow may slow the growth of fetuses and their intestinal development (4), which are the major causes of morbidity and impaired growth performance in LBW piglets (5). Perinatal (fetal/neonatal) gut dysfunction in newborn mammals not only restricts their primary function of nutrient digestion and absorption but also could compromise their epithelial barrier function and the activation and maturation of the submucosal immune system (6). In fact, among the frequent problems suffered by IUGR piglets are intestinal growth and morphology as well as altered gene expression (7).
In previous research, we and other authors have shown that phytogenic compounds (PCs) in the sow's feed can be transferred to the offspring through amniotic fluid and milk (8, 9). This prenatal and postnatal exposure to PCs, through nutritional programming during the perinatal period, influences the oxidative status of sows and piglets (9) and also reduces weaning-associated health and welfare problems in piglets (8, 9). However, we were not able to identify the importance and relevance of early effects promoted by the prenatal dietary treatments to counteract signs of impaired gastrointestinal function in neonatal piglets. In the present study, we explored whether a blend of PCs (BPC) supplemented to the diets of gestating hyperprolific sows influences the small-intestine histomorphology and gene expression in neonate piglets. It was hypothesized that BPC supplementation would enhance the parameters related to gut health and function in neonate piglets.
Materials and Methods
Experimental Design, Animals, and Housing
Twenty-eight DanBred hybrid line (Landrace × Yorkshire) gilts and sows (parity 0 to 7) were randomly distributed by parity number and body weight (BW) into two dietary treatment groups (n = 14 per treatment). After breeding, sows were fed an unsupplemented control diet (CON) or the CON diet supplemented with 1 kg/MT of a blend of PCs (Delacon Biotechnik GmbH, Engerwitzdorf, Austria) during the entire gestation (BPC). During the entire gestation, the sows were individually controlled, while pen was included as a random effect. Sows were assigned to individual cages (1.8 × 0.8 m) from weaning (d 0) until confirmed gestation (day 35). On day 35, sows were allotted by parity and BW into group pens of seven sows/pen (5.0 × 5.0 m) until day 110 of gestation. Thereafter, sows were moved to the farrowing unit, where they were placed in individual farrowing pens (2.0 × 2.6 m) mounted over a partially slatted floor with a heated floor pad for piglets. Water was provided ad libitum through a nipple waterer, and experimental diets were provided to sows in pelleted form. In addition, the sows' reproductive performance was monitored at farrowing.
Diets and Feeding
The Control diet offered in gestation was formulated to meet or exceed nutrient requirements for DanBred sows (10), with adaptations based on Spanish recommendations for gestating sows (11). The BCP experimental diet was the CON diet plus 1 g/kg of BPC supplement. The BPC contained 45 g/kg essential oils (EO) (EO composition described in Table 1). Based on individual body condition, sows were fed their corresponding diet as follows: 2.1 kg/d from weaning to service, an average of 2.9 kg/d from service to day 35 of gestation, and 2.5 kg/d from day 35 to day 110 of gestation (flat line). Each gestating pen was equipped with a mechanical free-access self-closing semi-cage without pneumatic actuators (Rotecna, Lleida, Spain) to keep animals individually monitored during feeding. From day 110 of gestation, sows were fed their corresponding treatment diet with ad libitum intake.
Sampling
Eight piglets per treatment (one piglet per liter, the animal representing the median BW within each litter) were selected and euthanized at farrowing (without sucking colostrum) to obtain jejunum tissue samples. Before euthanasia, piglets were anesthetized by intramuscular injection of 100 mg Telazol, 50 mg ketamine, and 50 mg xylazine per 1 ml/22 kg of BW. Thereafter, pigs were euthanized by jugular vein injection using sodium pentobarbital 0.5 ml/kg of BW. The jejunum tissue obtained for the gene expression analysis (~1.5 cm2) was placed in 1 ml of RNAlater (Applied Biosystems, Foster City, CA, USA) and stored at room temperature (25°C) during the first 24 h after collection. Thereafter, the tissue samples were stored at −80°C until analysis. Additional jejunum tissue samples (~2 cm2) were placed in individual tubes containing formaldehyde solution for histomorphology determination.
Jejunal Gene Expression Study by qPCR
Piglet jejunum gene expression of 56 genes related to intestinal health and functionality was quantified by RT-qPCR using an OpenArray Real-Time PCR Platform as specified by Reyes-Camacho et al. (9). Briefly, total RNA was extracted from 50 mg of jejunum tissue using the Ambion RiboPure Kit (Life Technologies, Carlsbad, CA, USA) by following the manufacturer's protocol. RNA concentration was measured using a NanoDrop ND-1000 spectrophotometer (NanoDrop products, Wilmington, DE, USA), and RNA quality was checked using Agilent 2100 Bioanalyzer equipment (Agilent Technologies, Santa Clara, CA, USA). Primers were designed by spanning exon–exon boundaries using the Primer Express 2.0 software (Applied Biosystems), and genomic DNA amplification and primer dimer formation were controlled. One replicate per sample was run in a TaqMan OpenArray gene expression custom plate format for gene expression with 56 assays of 48 samples per plate (OpenArray plate) in a QuantStudio 12K Flex Real-Time PCR System (Applied Biosystems, Foster City, CA). A total of 56 genes were previously selected based on the bibliography, including four reference genes. Details regarding genes and primers can be found in previously published work by González-Solé et al. (12).
Jejunal Histomorphology Measurement
For the histomorphology evaluation, jejunum samples were fixed for 24–48 h in neutral-buffered 10% formalin. After dehydration and embedding in paraffin wax, sections of ~3 μm were stained with hematoxylin and eosin. Villus height (VH), crypt depth (CD), number of intraepithelial lymphocyte (IEL), and number of goblet cells (GC) per 100 micrometers of villus height were measured blinded in 10 well-oriented villi and crypts per sample by the same person using a light microscope (BHS, Olympus, Barcelona, Spain).
Statistical Analyses
The individual newborn piglet was considered as the experimental unit. All data were analyzed considering the treatment as the main effect. The results are presented as means with their corresponding SEM. For morphology, data were analyzed by using the TTEST procedure of the statistical package SAS 9.4 (SAS Inst. Inc., Cary, NC, USA), and significant differences were declared at p < 0.05, while 0.05 < p < 0.10 were considered significant tendencies.
Gene expression statistical analysis was performed as specified by González-Solé et al. (12). Briefly, data were collected and analyzed using the Thermo Fisher Cloud software 1.0 (Applied Biosystems) applying the applying the 2−ΔΔCt method for relative quantification (RQ). The normal distribution of the RQ values was checked with the Shapiro.test function of R 3.5.3 software, and log2 transformation was applied if required. One-way ANOVA was performed, and the Benjamini–Hochberg false discovery rate (FDR) was used for multiple-testing correction of p-values, defining the model:
where Yij is each observation of the outcome variable, μi is the global mean, treatj is the main effect of the treatment, and εij is the experimental error term. Significant gene expression differences between functional groups were accepted at p < 0.05, whereas significant gene expression differences between treatments were accepted at FDR (q-values) < 0.10.
Results
The Piglets' Jejunum Histomorphology
The jejunal histomorphology results (Table 2) showed that, compared to the CON group, BPC tended to increase the VH (p = 0.072) and VH:CD ratio (p = 0.060) in neonate piglets. The GC density improved in the BPC group (p = 0.033). However, no significant differences (p > 0.05) were observed in CD or IEL density.
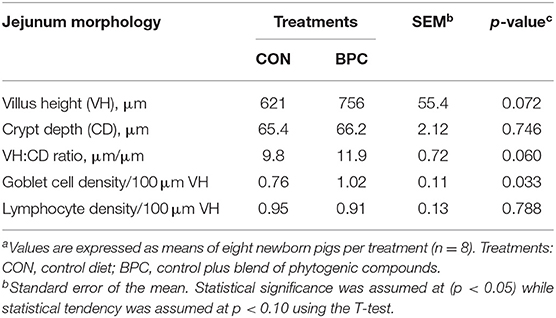
Table 2. Effects of BPC supplementation during gestation of hyperprolific sows on jejunal histomorphology of neonate pigletsa.
The Piglets' Jejunum Gene Expression
The results for the jejunum gene expression analysis of neonate piglets are shown in Table 3. Although only six genes showed statistical differences between treatments (p < 0.05, q < 0.10), the other six genes that showed statistical differences (p < 0.05) between functional groups are presented. Compared to the CON, a downregulation (q < 0.10) was observed in the BPC treatment for the SOD2 gene from the oxidation group and the SLC16A1/MCT1, SLC11A2/DMT1, and SLC39A/ZIP4 genes from the nutrient transport group, while from the immune response and barrier function groups an upregulation (q < 0.10) was observed for IFNG and CLDN4, respectively. Furthermore, there were no significant differences between treatments (p < 0.05, q > 0.10) for SLC15A1/PEPT1, ALPI, IDO1, DAO1, CCK, and OCLN from nutrient transport, immune response, enzyme/hormone, and barrier function group, respectively.
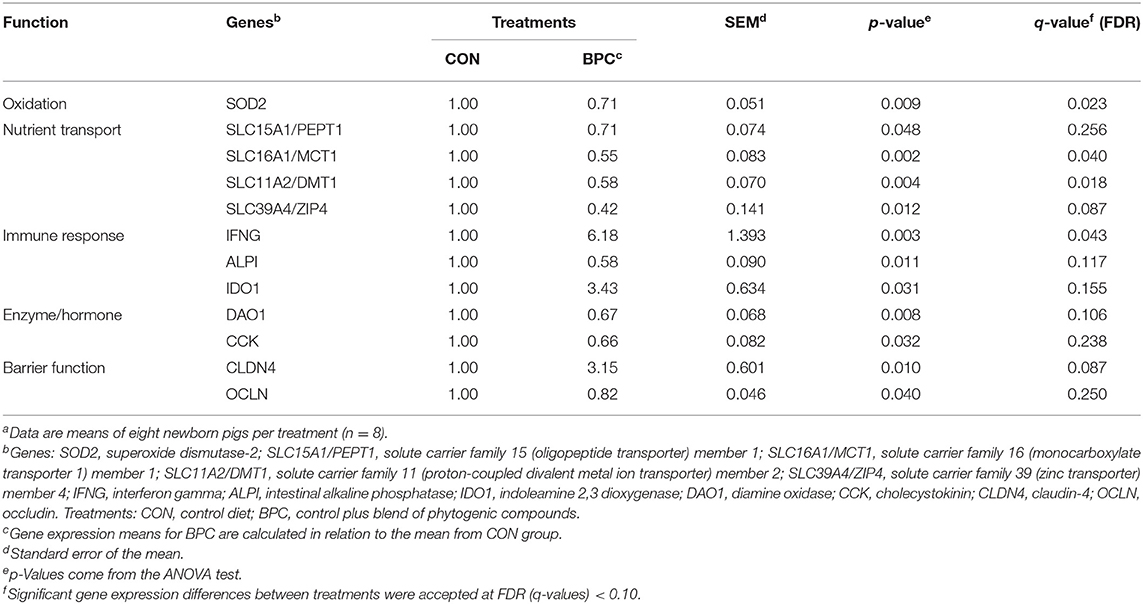
Table 3. Effects of BPC supplementation during gestation of hyperprolific sows on related jejunal health-function mRNA relative expression of neonate pigletsa.
Discussion
Although the study did not aim to research the sow's reproductive performance, our findings indicate that BPC improved both the total number of piglets born (19.9 vs. 17.8) and the number born alive (17.2 vs. 14.7), while the neonate piglet BW tended to decrease (1.17 vs. 1.33 kg) (Supplementary Table 1) in agreement with previous studies with a larger sample size in commercial conditions (9). LBW in piglets has been associated with a certain degree of IUGR (1), with impaired intestinal structure, and with a transcriptomic profile and bacterial colonization in neonatal IUGR piglets (13). In previous studies by our group, it has been observed that at birth, the jejunum expression of 10 genes, involved in the immune, digestive, and stress responses, is clearly different according to the piglet BW category between half-sibling piglets (light vs. average littermates) (unpublished data).
In this study we explored the effects of prenatal BPC exposure on histomorphology, and gene expression related to the health and functioning of the newborn piglet jejunum from hyperprolific dam-line sows. The jejunum plays an important role in nutrient uptake as well as immune system programming and metabolic programming in the pigs' early life (14). To our knowledge, there are no studies reporting the effects of PCs on the intestinal gene expression and morphology of neonate pigs. Positive findings were observed in histomorphology in neonate piglets of the BPC group, which showed improvements in the VH, the ratio of VH:CD, and the goblet cell density compared to newborn piglets from the CON group. The effects promoted by BPC appear to attenuate the early effects derived from IUGR, which is known to affect the intestinal cell proliferation–apoptosis balance in neonate piglets (5). They also affect the intestinal growth and morphology in association with the altered gene expression of growth-related proteins (7) and modify the volume density and function of epithelial cell types such as goblet cells, which are important constituents of the innate defense system (15). An increased VH and ratio VH:CD may suggest an improvement in the digestive and absorptive function of the intestine as a result of an increased absorptive surface, an expression of brush border enzymes, and nutrient transport systems (16).
In highly prolific sows, uterine capacity or insufficiency can affect fetal growth when competition by littermates for limited uterine space and nutrients becomes increasingly critical (17). Evidence in human studies has shown that placental uptake and transport of nutrients such as thiamin, folic acid, and glucose by BeWo cells are modulated by PCs, such as polyphenols (18). The solute carrier (SLC) genes are a large family of protein transporters in mammals, and their gene expression is notably affected if there is a deprivation of nutrients such as amino acids (AAs), which implies an effect on the capacity of the SLC to regulate intracellular nutrient concentrations and, in addition, detect alterations in extracellular nutrient levels (19). For instance, the gene expression of the SLC38 family was upregulated in hypothalamic cells N25/2 of mice after AA starvation (20). Glucose deprivation increases MCT1 protein expression and their interaction in oxidative tumor cells (21). Moreover, the hypoxia induced an increase in MCT1 plasma membrane expression in glioma cells, both in in vitro and in vivo models (22). The gene expression analysis of the current study showed that prenatal exposure of the fetus to BPC led to downregulation in the jejunum of nutrient transport-related genes SLC16A1/MCT1, SLC11A2/DMT1, and SLC39A/ZIP4 in newborn piglets. This may indicate that compared to BPC, potential deprivation of nutrients, especially for Fe2+ (23), short-chain fatty acids (24), and zinc (25), respectively, induced an adaptative upregulation of the abovementioned SLC genes in newborn piglets from the CON group.
When inflammation occurs in the gastrointestinal tract (GIT), this can result in a decrease in digestive efficiency and reduced absorption of nutrients (26). In this study, the prenatal exposure of the fetus to BPC induced an upregulation of the immune response IFNG and barrier function CLDN4 genes. IFNG is critical in increasing and mediating intestinal immunity because of its role in recognizing and eliminating pathogens. IFNG can have several functions; it can exhibit its immunomodulatory effects by controlling inflammatory response, enhancing antigen processing and presentation, increasing leukocyte trafficking, inducing an antiviral state, boosting the antimicrobial functions, and affecting cellular proliferation and apoptosis (27). On the other hand, expression of Claudin proteins such as CLDN4 within the small intestine of newborn piglets plays a vital role in controlling barrier function and mucosal homeostasis (epithelial tight junctions), particularly on the apical aspect of lateral surfaces of intestinal epithelial cells where they help regulate ion and macromolecule movement across the intestinal epithelium (28). It has been described that transcription levels of IFNG and CLDN4 showed a lower expression in LBW than normal birth weight (NBW) piglets (29). Thus, results shown in the BPC group suggest that upregulation of IFNG and CLDN4, together with the development in jejunal histomorphology, could help to improve the gut health of LBW piglets.
In addition, the induction of mitochondrial oxidative stress during periods of nutrient deprivation in animals has been associated with decreased metabolic requirements, higher mitochondrial membrane potential, and increased superoxide production at the level of the complex III of the electron transport chain (30). Since SOD2 activity is regulated in response to mitochondrial superoxide production, the upregulation of mitochondrial SOD activity results in an overproduction of hydrogen peroxide as a product of the disproportionation reaction of superoxide anion catalyzed by SOD (31). In the present study, the SOD2 gene was downregulated in the jejunum of BPC newborn piglets, which may indicate that compared with CON, undue intrauterine oxidative stress was avoided in newborn piglets from the BPC group. In swine, it has been described that oxidative damage to DNA (8-hydroxy-20-deoxyguanosine, 8-OHdG) and proteins (carbonyls) measured in plasma increased after the stress period such as weaning, and this coincides with a rise in enzymatic antioxidant activity including SOD. Furthermore, oxidative damage to macromolecules by mitochondrial dysfunction and cellular oxidative stress is more important in LBW than in NBW piglets, as measured concentrations of 8-OHdG and carbonyls are significantly higher (32). Thus, it suggests that upregulation of the oxidation SOD2 gene shown in CON may indicate a defense mechanism against mitochondrial dysfunction and cellular oxidative stress conditions.
It has also been reported that several dietary plants and their bioactive components have the potential to modulate human transcriptome profiles of various biological pathways involved in oxidative, immune response, glucose and fatty acid metabolism, and inflammatory and cell signaling pathways (33). In previous studies, we have observed that perinatal exposure to a blend of PCs containing eucalyptol, p-cymene, linalool, anethole, and thymol influenced the overexpression of the barrier function (MUC2), and immune response (PPARGC-α, TNF-α, TGF-β1, IDO1, and IL-10) genes in suckling piglets (9). In this study, we have observed that these effects may be anticipated by administering BPC in the sow gestation diet. In terms of fetal programming, maternal nutrition status during fetal development may result in a permanent imprint by changing the epigenetic state of the fetal genome and its gene expression (34). Although the action modes of several PCs are not yet fully clarified, the main action modes of the major compounds used in BPC, such as trans-anethole (35), 1.8-cineole (36), and Camphor (37), exhibit important biological properties. These include anti-inflammatory, antioxidant, and antimicrobial properties that could have influenced the changes on the intestinal histomorphology and gene expression of neonate piglets in this study. Therefore, the prenatal exposure of the fetus to PCs may indicate a positive means to attenuate the intestinal dysfunction in LBW piglets, especially in terms of structure and functionality.
In conclusion, BPC supplemented to hyperprolific sows during gestation influenced the prenatal programming of some intestinal biological functions. This was evidenced by the improved histomorphology and gene expression related to the nutrient absorption, immune response, intestinal integrity, and oxidative stress in the jejunum of neonate piglets.
Data Availability Statement
The raw data supporting the conclusions of this article will be made available by the authors, without undue reservation.
Ethics Statement
The animal study was reviewed and approved by CEEAH-Univesritat Autònoma de Barcelona.
Author Contributions
DR-C performed the animal trial, the statistical analyses, and writing—original draft preparation. JP and DS-O were the principal investigator and contributed to conceptualization and experimental design. EP and JV conducted the conceptualization and experimental design. TA supervised the interpretation of the study results and reviewed the draft. LC-M and JF contributed to the design and set-up of the gene expression studies. All authors contributed to the article and approved the submitted version.
Funding
Financial support for this research from DELACON Biotechnik GmbH. (Engerwitzdorf, Austria) is appreciated.
Conflict of Interest
EV, TA, and JV are employees of DELACON Biotechnik GmbH. (Engerwitzdorf, Austria) which is a global supplier of phytogenic compounds.
The remaining authors declare that the research was conducted in the absence of any commercial or financial relationships that could be construed as a potential conflict of interest.
Acknowledgments
The authors are grateful to the staff of the Centre de Recerca en Sanitat Animal from the IRTA for support received in the histomorphology analysis.
Supplementary Material
The Supplementary Material for this article can be found online at: https://www.frontiersin.org/articles/10.3389/fvets.2021.639719/full#supplementary-material
Abbreviations
BPC, blend of phytogenic compounds; GIT, gastrointestinal tract; IUGR, intrauterine growth retardation; LWB, low birth weight; NBW, normal birth weight; PCs, phytogenic compounds; VH, Villus height.
References
1. Matheson SM, Walling GA, Edwards SA. Genetic selection against intrauterine growth retardation in piglets: a problem at the piglet level with a solution at the sow level. Genet Sel Evol. (2018) 50:46. doi: 10.1186/s12711-018-0417-7
2. Reynolds LP, Caton JS, Redmer DA, Grazul-Bilska AT, Vonnahme KA, Borowicz PP, et al. Evidence for altered placental blood flow and vascularity in compromised pregnancies. J Physiol. (2006) 572:51–8. doi: 10.1113/jphysiol.2005.104430
3. Hu C, Yang Y, Deng M, Yang L, Shu G, Jiang Q, et al. Placentae for low birth weight piglets are vulnerable to oxidative stress, mitochondrial dysfunction, and impaired angiogenesis. Oxid Med Cell Longev. (2020) 2020:8715412. doi: 10.1155/2020/8715412
4. Zhang S, Heng J, Song H, Zhang Y, Lin X, Tian M, et al. Role of maternal dietary protein and amino acids on fetal programming, early neonatal development, and lactation in Swine. Animals. (2019) 9:19. doi: 10.3390/ani9010019
5. D'Inca R, Kloareg M, Gras-Le Guen C, Le Huërou-Luron I. Intrauterine growth restriction modifies the developmental pattern of intestinal structure, transcriptomic profile, and bacterial colonization in neonatal pigs. J Nutr. (2010) 140:925–31. doi: 10.3945/jn.109.116822
6. Weström B, Arévalo Sureda E, Pierzynowska K, Pierzynowski SG, Pérez-Cano FJ. The immature gut barrier and its importance in establishing immunity in newborn mammals. Front Immunol. (2020) 11:1153. doi: 10.3389/fimmu.2020.01153
7. Wang T, Yong JH, Shi F, Ruo JX, Hutz RJ. Effects of intrauterine growth retardation on development of the gastrointestinal tract in neonatal pigs. Biol Neonate. (2005) 88:66–72. doi: 10.1159/000084645
8. Val-Laillet D, Elmore JS, Baines D, Naylor P, Naylor R. Long-term exposure to sensory feed additives during the gestational and postnatal periods affects sows' colostrum and milk sensory profiles, piglets' growth, and feed intake 1. J Anim Sci. (2018) 96:3233–48. doi: 10.1093/jas/sky171
9. Reyes-Camacho D, Vinyeta E, Pérez JF, Aumiller T, Criado L, Palade LM, et al. Phytogenic actives supplemented in hyperprolific sows: effects on maternal transfer of phytogenic compounds, colostrum and milk features, performance and antioxidant status of sows and their offspring, and piglet intestinal gene expression. J Anim Sci. (2020) 98:skz390. doi: 10.1093/jas/skz390
10. Tybirk PER. Nutrient Recommendations for Pigs in Denmark. 17th ed. Copenhagen, Denmark: SEGES-VSP Danish Pig Research Centre (2015).
11. FEDNA. Necesidades nutricionales para ganado porcino. 2nd ed. Madrid, Spain: Fundación Española para el Desarollo de la Nutrición Animal (2013).
12. González-Solé F, Criado-Mesas L, Villodre C, García WC, Farré M, Borda E, et al. Porcine digestible peptides (Pdp) in weanling diets regulates the expression of genes involved in gut barrier function, immune response and nutrient transport in nursery pigs. Animals. (2020) 10:1–21. doi: 10.3390/ani10122368
13. Jiang L, Feng C, Tao S, Li N, Zuo B, Han D, et al. Maternal imprinting of the neonatal microbiota colonization in intrauterine growth restricted piglets: a review. J Anim Sci Biotechnol. (2019) 10:1–8. doi: 10.1186/s40104-019-0397-7
14. Schokker D, Hulsegge I, Woelders H, Rebel JMJ. Plasticity of intestinal gene expression profile signatures reflected by nutritional interventions in piglets. BMC Genomics. (2019) 20:1–12. doi: 10.1186/s12864-019-5748-4
15. Bischoff SC, Barbara G, Buurman W, Ockhuizen T, Schulzke JD, Serino M, et al. Intestinal permeability - a new target for disease prevention and therapy. BMC Gastroenterol. (2014) 14:1–25. doi: 10.1186/s12876-014-0189-7
16. Pluske JR, Hampson DJ, Williams IH. Factors influencing the structure and function of the small intestine in the weaned pig: a review. Livest Prod Sci. (1997) 51:215–36. doi: 10.1016/S0301-6226(97)00057-2
17. Wang J, Feng C, Liu T, Shi M, Wu G, Bazer FW. Physiological alterations associated with intrauterine growth restriction in fetal pigs: causes and insights for nutritional optimization. Mol Reprod Dev. (2017) 84:897–904. doi: 10.1002/mrd.22842
18. Martel F, Monteiro R, Calhau C. Effect of polyphenols on the intestinal and placental transport of some bioactive compounds. Nutr Res Rev. (2010) 23:47–64. doi: 10.1017/S0954422410000053
19. Beltrán Piña BG, González Castro MI, Rivas García F. Influence of amino acids that come from the diet in the expression of genes. Nutr Hosp. (2019) 36:173–82. doi: 10.20960/nh.1986
20. Hellsten S V., Lekholm E, Ahmad T, Fredriksson R. The gene expression of numerous SLC transporters is altered in the immortalized hypothalamic cell line N25/2 following amino acid starvation. FEBS Open Bio. (2017) 7:249–64. doi: 10.1002/2211-5463.12181
21. De Saedeleer CJ, Porporato PE, Copetti T, Pérez-Escuredo J, Payen VL, Brisson L, et al. Glucose deprivation increases monocarboxylate transporter 1 (MCT1) expression and MCT1-dependent tumor cell migration. Oncogene. (2014) 33:4060–8. doi: 10.1038/onc.2013.454
22. Miranda-Gonçalves V, Granja S, Martinho O, Honavar M, Pojo M, Costa BM, et al. Hypoxia-mediated upregulation of MCT1 expression supports the glycolytic phenotype of glioblastomas. Oncotarget. (2016) 7:46335–53. doi: 10.18632/oncotarget.10114
23. Hansen SL, Trakooljul N, Liu H-C, Moeser AJ, Spears JW. Iron transporters are differentially regulated by dietary iron, and modifications are associated with changes in manganese metabolism in young pigs. J Nutr. (2009) 139:1474–9. doi: 10.3945/jn.109.105866
24. Villodre Tudela C, Boudry C, Stumpff F, Aschenbach JR, Vahjen W, Zentek J, et al. Down-regulation of monocarboxylate transporter 1 (MCT1) gene expression in the colon of piglets is linked to bacterial protein fermentation and pro-inflammatory cytokine-mediated signalling. Br J Nutr. (2015) 113:610–7. doi: 10.1017/S0007114514004231
25. Sargeant HR, Miller HM, Shaw MA. Inflammatory response of porcine epithelial IPEC J2 cells to enterotoxigenic E. coli infection is modulated by zinc supplementation. Mol Immunol. (2011) 48:2113–21. doi: 10.1016/j.molimm.2011.07.002
26. Celi P, Verlhac V, Pérez Calvo E, Schmeisser J, Kluenter AM. Biomarkers of gastrointestinal functionality in animal nutrition and health. Anim Feed Sci Technol. (2019) 250:9–31. doi: 10.1016/j.anifeedsci.2018.07.012
27. Kak G, Raza M, Tiwari BK. Interferon-gamma (IFN-γ): exploring its implications in infectious diseases. Biomol Concepts. (2018) 9:64–79. doi: 10.1515/bmc-2018-0007
28. Deluco B, Fourie KR, Simko OM, Wilson HL. Localization of claudin-3 and claudin-4 within the small intestine of newborn piglets. Physiol Rep. (2021) 9:e14717. doi: 10.14814/phy2.14717
29. Ayuso M, Irwin R, Walsh C, Van Cruchten S, Van Ginneken C. Low birth weight female piglets show altered intestinal development, gene expression, and epigenetic changes at key developmental loci. FASEB J. (2021) 35:1–17. doi: 10.1096/fj.202002587R
30. Salin K, Villasevil EM, Anderson GJ, Auer SK, Selman C, Hartley RC, et al. Decreased mitochondrial metabolic requirements in fasting animals carry an oxidative cost. Funct Ecol. (2018) 32:2149–57. doi: 10.1111/1365-2435.13125
31. Zelko IN, Mariani TJ, Folz RJ. Superoxide dismutase multigene family: a comparison of the CuZn-SOD (SOD1), Mn-SOD (SOD2), and EC-SOD (SOD3) gene structures, evolution, and expression. Free Radic Bio Med. (2002) 33:337–49. doi: 10.1016/S0891-5849(02)00905-X
32. Novais AK, Martel-Kennes Y, Roy C, Deschêne K, Beaulieu S, Bergeron N, et al. Tissue-specific profiling reveals modulation of cellular and mitochondrial oxidative stress in normal- and low-birthweight piglets throughout the peri-weaning period. Animal. (2020) 14:1014–24. doi: 10.1017/S1751731119002829
33. Jamshidi N, Mantri N, Cohen MM. Acute effects of dietary plant nutrients on transcriptome profiles: evidence from human studies. Crit Rev Food Sci Nutr. (2020) 60:1869–80. doi: 10.1080/10408398.2019.1608154
34. Wu G, Bazer F, Cudd T, Meininger C. Recent advances in nutritional sciences-maternal nutrition and fetal development. J Nutr. (2004) 134:2169–72. doi: 10.1093/jn/134.9.2169
35. Pandit K, Kaur S, Kumar A, Bhardwaj R, Kaur S. trans-Anethole abrogates cell proliferation and induces apoptosis through the mitochondrial-mediated pathway in human osteosarcoma cells. Nutr Cancer. (2020) 72:1–19. doi: 10.1080/01635581.2020.1803927
36. Seol GH, Kim KY. Eucalyptol and its role in chronic diseases. Adv Exp Med Biol. (2016) 2016:389–98. doi: 10.1007/978-3-319-41342-6_18
Keywords: hyperprolific sows, prenatal exposure, neonatal programming, phytogenic compounds, piglet gut health
Citation: Reyes-Camacho D, Pérez JF, Vinyeta E, Aumiller T, Criado-Mesas L, Folch JM, Van der Klis JD and Solà-Oriol D (2021) Phytogenic Compounds Supplemented to Gestating Hyperprolific Sows Affects the Gut Health-Related Gene Expression and Histological Responses in Neonate Piglets. Front. Vet. Sci. 8:639719. doi: 10.3389/fvets.2021.639719
Received: 09 December 2020; Accepted: 07 May 2021;
Published: 14 June 2021.
Edited by:
Pietro Celi, Adisseo, FranceReviewed by:
Cesar Augusto Pospissil Garbossa, University of São Paulo, BrazilRyan Dilger, University of Illinois at Urbana-Champaign, United States
Copyright © 2021 Reyes-Camacho, Pérez, Vinyeta, Aumiller, Criado-Mesas, Folch, Van der Klis and Solà-Oriol. This is an open-access article distributed under the terms of the Creative Commons Attribution License (CC BY). The use, distribution or reproduction in other forums is permitted, provided the original author(s) and the copyright owner(s) are credited and that the original publication in this journal is cited, in accordance with accepted academic practice. No use, distribution or reproduction is permitted which does not comply with these terms.
*Correspondence: David Solà-Oriol, ZGF2aWQuc29sYUB1YWIuY2F0