- 1School of Life Science and Engineering, Southwest University of Science and Technology, Mianyang, Sichuan, China
- 2Animal Breeding and Genetics Key Laboratory of Sichuan Province, Sichuan Animal Science Academy, Chengdu, China
- 3College of Animal Science and Technology, Henan University of Animal Husbandry and Economy, Zhengzhou, Henan, China
Introduction: The persistent African swine fever epidemic has significantly compromised China’s swine production. To accelerate production recovery, commercial farms are increasingly adopting retention of two-way backcross sows (Landrace × Yorkshire × Landrace, LLY) for breeding. This study aimed to investigate the effects of yeast protein, an emerging sustainable protein source, on reproductive performance, immune responses, and gut microbiota in two-way crossbred sows (Landrace × Yorkshire, LY) and LLY sows.
Methods: The experiment employed a 2×2 factorial design evaluating two fixed factors: sow hybrid (LY vs LLY) and yeast protein supplementation (0% vs 2.6%). The four treatment groups were: LY sows without yeast protein supplementation (LY-C), LLY sows without yeast protein supplementation (LLY-C), LY sows with yeast protein supplementation (LY-YP), and LLY sows with yeast protein supplementation (LLY-YP). A total of one hundred healthy sows of 2-6 parities (50 LY sows and 50 LLY sows), were stratified by backfat thickness, body weight, and parity, then randomly allocated to the four treatment groups on day 105 of gestation, with 25 sows in each group. The experimental period lasted from day 106 of gestation to day 18 of lactation.
Results and conclusion: Yeast protein supplementation showed no significant effects on most reproductive parameters of different sow hybrids, but reduced backfat loss by 30.5% during lactation (P < 0.05) and demonstrated a numerical reduction in mummification rate of fetuses (P = 0.06). Immunological assessments revealed that LLY sows exhibited 26.8% lower serum IgM concentration than LY sows (P < 0.05), while yeast protein supplementation significantly reduced serum IL-1β levels by 45.6% (P < 0.05) on day 18 of lactation. 16S rRNA gene sequencing analysis revealed comparable fecal microbial diversity across treatments (P > 0.05), though differences were observed in certain bacterial genera between LY and LLY sows during late gestation and lactation. Yeast protein supplementation enriched beneficial bacteria including Ruminococcaceae_UCG-002, Rikenellaceae_RC9_gut_group, and Christensenellaceae_R_7_group, while suppressing potentially detrimental bacteria such as Family_XIII_AD3011_group (P < 0.05). These findings demonstrate the practical feasibility of retaining LLY sows for commercial breeding. Yeast protein supplementation, as a substitute for fishmeal during late gestation and lactation, significantly reduced lactational backfat loss, moderately attenuated inflammatory response, and enhanced gut microbiome homeostasis through selective microbial enrichment in sows.
1 Introduction
In recent years, China’s swine industry has suffered substantial losses due to African Swine Fever (ASF) outbreaks (Liu et al., 2021). The ASF epidemic caused a significant reduction in the population of two-way crossbred sows (Landrace × Yorkshire, LY), resulting in severe shortage of breeding stock and inflated prices for breeding sows. Official data from the Ministry of Agriculture and Rural Affairs of China (MARA) revealed a 40.5% reduction in hog inventories and a 39.3% decrease in breeding sow populations between August 2018 (ASF onset) and August 2019 (Ma et al., 2021). To expedite production recovery, MARA and local governments have prioritized breeding herd restoration through policy interventions. Consequently, many farms have adopted three-way crossbred sows (Duroc × Landrace × Yorkshire, DLY) or two-way backcross sows (Landrace × Yorkshire × Landrace, LLY) as replacements for traditional LY sows. While existing research extensively compares reproductive performance between DLY and LY sows, studies evaluating LLY versus LY sows remain scarce. Current evidence suggests that although LLY sows exhibit marginally reduced heterosis compared to LY sows, their overall reproductive hybrid vigor remains substantial. Yu et al. (2020) demonstrated that LLY sows maintain genetic stability in both reproductive efficiency and growth traits, retaining sufficient heterosis for commercial breeding applications. However, further research is required to conclusively characterize performance differences between LLY and LY sows.
Additionally, China has long grappled with a chronic deficit in domestic protein feedstuffs, with excessive reliance on imported protein sources posing a significant barrier to sustainable development in both feed and livestock industries (Yin et al., 2019). This critical situation underscores the urgent need to identify alternative protein resources and develop innovative nutritional strategies to strengthen national food security. Among potential solutions, yeast, a single celled eukaryotic organism, and its derivatives, have emerged as promising candidates due to their rich composition of protein, amino acids, cell wall polysaccharides, and bioactive compounds (Shurson, 2018; Agboola et al., 2021). The diverse range of yeast-derived products, including live/dry yeast, purified cell wall components, and fermentation-derived cultures or extracts (Pang et al., 2022), has attracted significant attention from animal nutritionists seeking functional feed additives. Extensive research documents the beneficial effects of yeast products across various species, demonstrating improvements in growth performance, gut health, and immune modulation in poultry (Hofacre et al., 2024; Islam et al., 2024; Qiu et al., 2024; Maina et al., 2025), aquatic species (Jin et al., 2018; Zheng et al., 2021; Kong et al., 2025), and young pigs (Espinosa et al., 2023; Fan et al., 2024; Kim and Duarte, 2024). In sow nutrition, studies demonstrate enhanced reproductive or growth performance, immune function, and gut microbiota profiles in sows or their offspring through supplementation with various yeast products, including live yeast (Xia et al., 2022; Fu et al., 2024), yeast culture (Zhao et al., 2022; Liu et al., 2023), yeast extract (Gao et al., 2021; Tan et al., 2021; dos Santos et al., 2023), and yeast hydrolysates (Chang et al., 2024; Kim and Duarte, 2024), though some studies show limited effects (Chance et al., 2022; Le Floc′h et al., 2022). However, research of yeast products as a major protein source in swine diets remains in its infancy. Given its high digestibility and favorable essential amino acid profile (Fernandes et al., 1998), whole yeast and its derivatives present particular potential as alternative protein sources for swine nutrition, especially in regions experiencing shortage of conventional high-quality proteins like fishmeal and soybean meal. This study therefore aims to investigate the effects of yeast protein supplementation - a single-cell protein derived from hydrolyzed yeast cells - on reproductive performance, immune responses, and gut microbiota in LY and LLY sows. This study addresses two key objectives: to establish scientific foundation for optimizing LLY sow utilization in commercial swine production, and to provide essential insights for yeast protein application in both LY and LLY sows.
2 Materials and methods
2.1 Animal care
The research protocol was approved by The Animal Welfare Committee of Southwest University of Science and Technology under ethic approval number L2023021.
2.2 Animals and experimental design
The experiment employed a 2×2 factorial design evaluating two fixed factors: sow hybrid (LY vs LLY) and yeast protein supplementation (0% vs 2.6%). The four treatment groups were: LY sows without yeast protein supplementation (LY-C), LLY sows without yeast protein supplementation (LLY-C), LY sows with yeast protein supplementation (LY-YP), and LLY sows with yeast protein supplementation (LLY-YP). A total of one hundred healthy sows of 2-6 parities (50 LY sows and 50 LLY sows), were stratified by backfat thickness, body weight, and parity, then randomly allocated to the four treatment groups on day 105 of gestation, with 25 sows in each group. The experimental period lasted from day 106 of gestation to day 18 of lactation.
2.3 Diet and feeding
The experimental diets were formulated to meet the nutrient requirements for gestating and lactating sows as recommended by the NRC (2012), with detailed composition and nutritional levels presented in Table 1. Yeast protein supplementation was incorporated at 2.6%, a level determined by replacing 2.1% fishmeal in the control diet through iso-nitrogenous substitution, thereby constituting 7% of the total dietary protein sources. The yeast protein was produced via polysaccharide removal and subsequent protein concentration from dried yeast, primarily comprising microbial protein and yeast-derived metabolites. The analyzed crude protein and amino acid contents of the yeast protein are provided in Table 2.
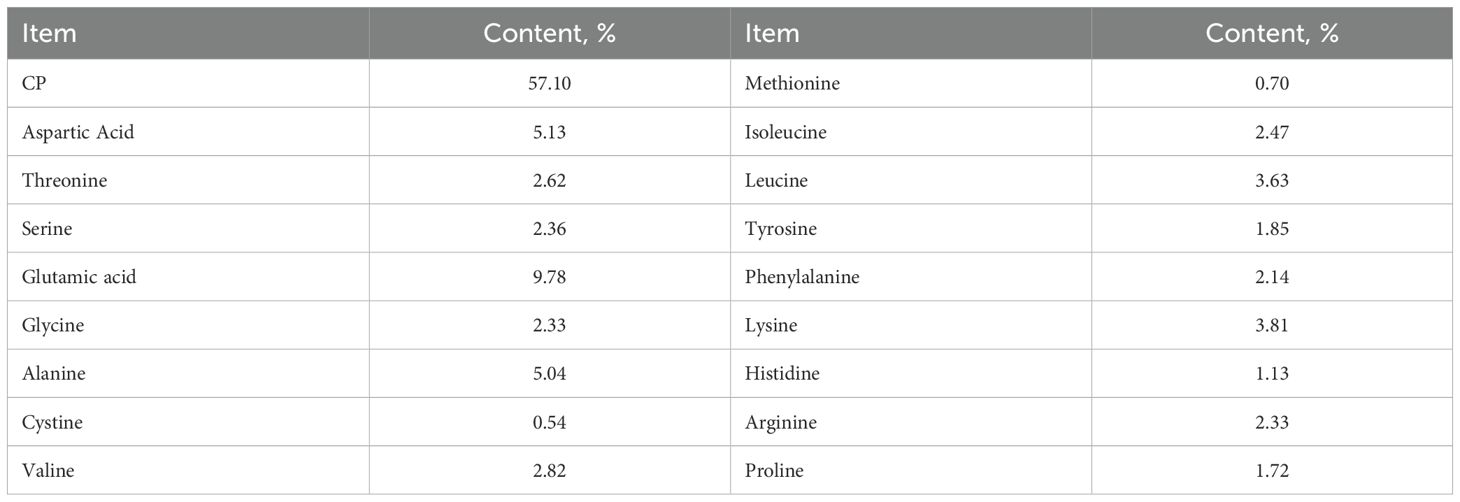
Table 2. The analyzed contents of crude protein and various amino acids in yeast protein used in this experiment.
From day 106 of gestation until parturition, sows were housed in individual crates and offered 3.0 kg/d of corresponding diets. After parturition, the feed allowance was 2 kg initially and increased by 1.0 kg/d until day 5 of lactation. From day 6 onward, all sows had free access to feed and water until weaning.
2.4 Measurements and sample collection
After parturition, the following parameters were recorded for each sow: number of total born piglets, number of live-born piglets, number of normal-born piglets (piglets birth weight > 800 g), and piglet birth weight. These data were used to calculate the litter birth weight, coefficient of variation (CV) of piglet birth weight, stillborn rate, mummification rate, and intrauterine growth restriction (IUGR, piglets birth weight < 800 g) rate. Piglets were weighed individually at birth, and on day 7, 14, and 18 of lactation, to calculate piglet or litter weight gain, and to estimate milk yield following the method of Hansen et al. (2012). Sow backfat thickness was measured 65 mm to the left side of the dorsal midline at the last rib (P2) using an ultrasound scanner (Renco Lean-Meater; Renco Corporation, Minneapolis, MN, USA).
On the day of farrowing, 50 mL of colostrum was collected from two to five teats of each sow. On day 18 of lactation, 0.3 mL of oxytocin was injected intravenously through the ear vein, and then 50 mL of milk was rapidly collected from two to five teats. Both colostrum and milk samples were filtered through sterile gauze and stored at -20°C until further analysis. Fasting blood samples on day 113 of gestation and day 18 of lactation were drawn by jugular vein puncture into two 5 mL tubes without anticoagulant. After 2 h of room temperature coagulation, samples were centrifuged at 2,550 × g at 4°C for 10 min. Serum samples were harvested and stored at -20°C until analysis. Fecal samples (2 g) were aseptically collected from six randomly selected sows per group on day 113 of gestation, day 3 of lactation, and day 18 of lactation. Samples were transported on dry ice to the laboratory and stored at -80°C pending analysis.
2.5 Milk and plasma sample analyses
Milk composition was analyzed for fat, protein, lactose, dry matter, and solids non-fat contents with an automatic milk quality analyzer (CombiFoss FT+, Foss, Denmark). Concentrations of immunoglobulins (IgG, IgA, IgM) and inflammatory cytokines (TNF-α, IL-10, IL-17, IL-1β) in colostrum, milk, and serum were determined with commercial ELISA kits (Nanjing Jiancheng Bio-Engineering Institute, China).
2.6 Fecal microbial analysis
Microbial DNA was extracted from thawed stool samples using the EZNA. ®Stool DNA Kit (D4015, Omega, Inc., Norwalk, CN, USA) following the manufacturer’s protocol. The genomic DNA was measured for purity and integrity before sequencing. The V4 hypervariable region of the 16S rRNA gene was amplified using 515F and 806R primers according to Zhou et al. (2023). The 16S RNA gene sequencing was performed on PacBio Sequel II platform. Sequences with ≥97% similarity were clustered to the same operational taxonomic unit (OTU) using USEARCH (v10.0). Representative sequences for each OTU were selected. The Naive Bayes classifier in QIIME2 (v2020.6) was used for taxonomic classification. The relative abundance of each OTU was examined at different taxonomic levels. Alpha diversity, as well as taxonomic community assessments, were performed by QIIME2 (v2020.6). Beta diversity was analyzed by principal coordinate analysis (PCoA) to assess the diversity in samples using QIIME (v1.9.1) (Mou et al., 2025).
2.7 Statistics
The statistical analysis was performed using the MIXED procedure of SAS software (Version 9.3; SAS Institute Inc., Cary, NC, USA), except for stillborn rate, mummification rate, IUGR rate, and piglet preweaning mortality, where odds ratios of these traits were analyzed using the GENMOD procedure of SAS. The fixed effects in the mixed model include sow hybrid (LY vs LLY), yeast protein supplementation (0% vs 2.6%), and their interaction. Mean values were presented as least square mean ± largest SEM, except for stillborn rate, mummification rate, IUGR rate, and piglet preweaning mortality which were reported as mean and their 95% confidence limits. All variables were considered significant when P < 0.05, whereas 0.05 < P < 0.1 was considered a tendency.
For the 16S rRNA sequencing data, differences in the alpha diversity indexes between groups were analyzed by t-test. The permutational multivariate analysis of variance (PERMANOVA) was used on the Bray-Curtis distance matrices to assess the beta diversity between groups. The Wilcoxon rank-sum test was used to compare data of relative abundance at different taxonomic levels between groups.
3 Results
3.1 Sow and litter performance
Reproductive performance analysis (Table 3) revealed neither main effects of sow hybrid nor yeast protein supplementation, nor their interactive effects on majority of reproductive parameters (P > 0.05). Notably, LLY sows exhibited significantly lower CV of piglet birth weight compared to LY sows (19.37% vs. 22.88%; P < 0.01). Yeast protein supplementation demonstrated a numerical reduction in mummification rate of fetuses (0% vs. 0.87%; P = 0.06).
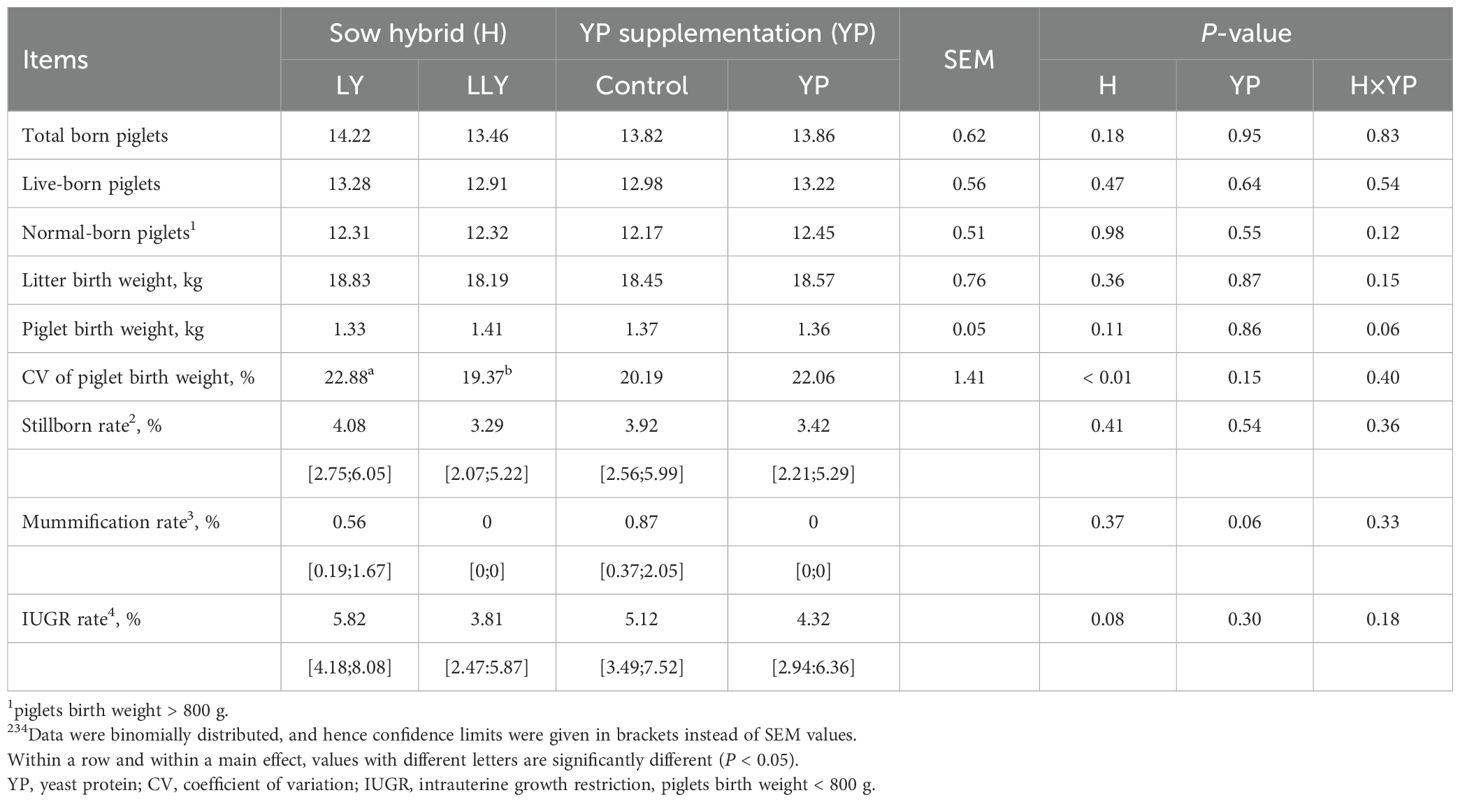
Table 3. The effect of yeast protein supplementation on the farrowing performance of two sow hybrids.
Lactation performance parameters (Table 4) remained unaffected by either sow hybrid or yeast protein supplementation in most measured indices (P > 0.05). However, LLY sows demonstrated a numerical reduction in average daily feed intake (ADFI) (4.91 vs. 5.22 kg/d; P = 0.06). And yeast protein supplementation reduced sow backfat loss by 30.5% during lactation (0.98 vs. 1.41 mm; P < 0.05).
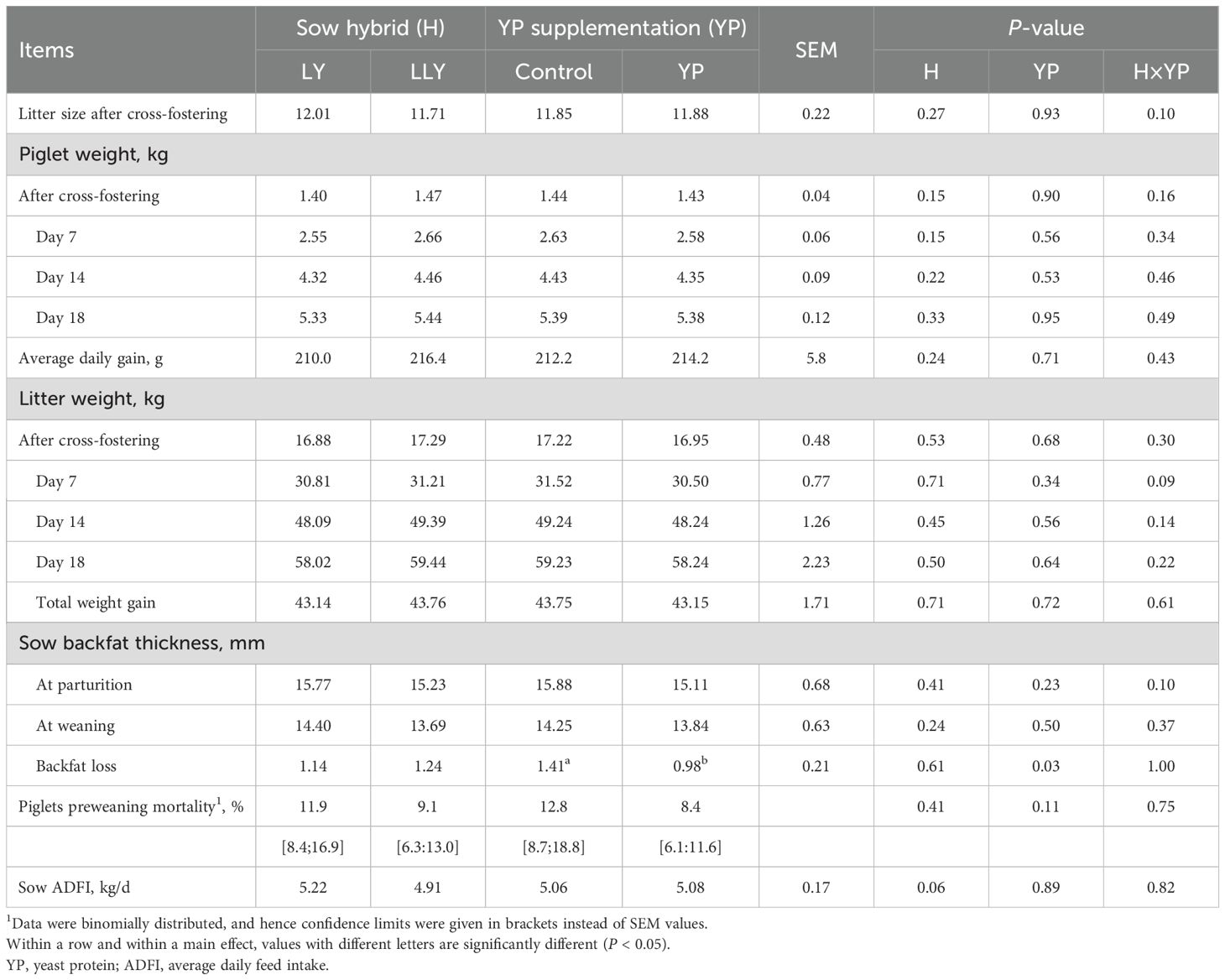
Table 4. The effect of yeast protein supplementation on the lactational performance of two sow hybrids.
3.2 Milk yield and milk composition
Milk composition analysis (Table 5) demonstrated that neither sow hybrid nor yeast protein supplementation exerted significant influence on milk yield or majority of compositional parameters in both colostrum and milk (P > 0.05). Notably, LLY sows exhibited elevated milk fat content compared to LY sows in colostrum (5.78% vs 4.25%) and milk (6.77% vs 5.78%) (P < 0.05). Furthermore, LLY sows showed higher milk dry matter (20.98% vs 19.42%; P < 0.01) and solids non-fat content (14.51% vs 14.12%; P = 0.05) compared to LY sows.
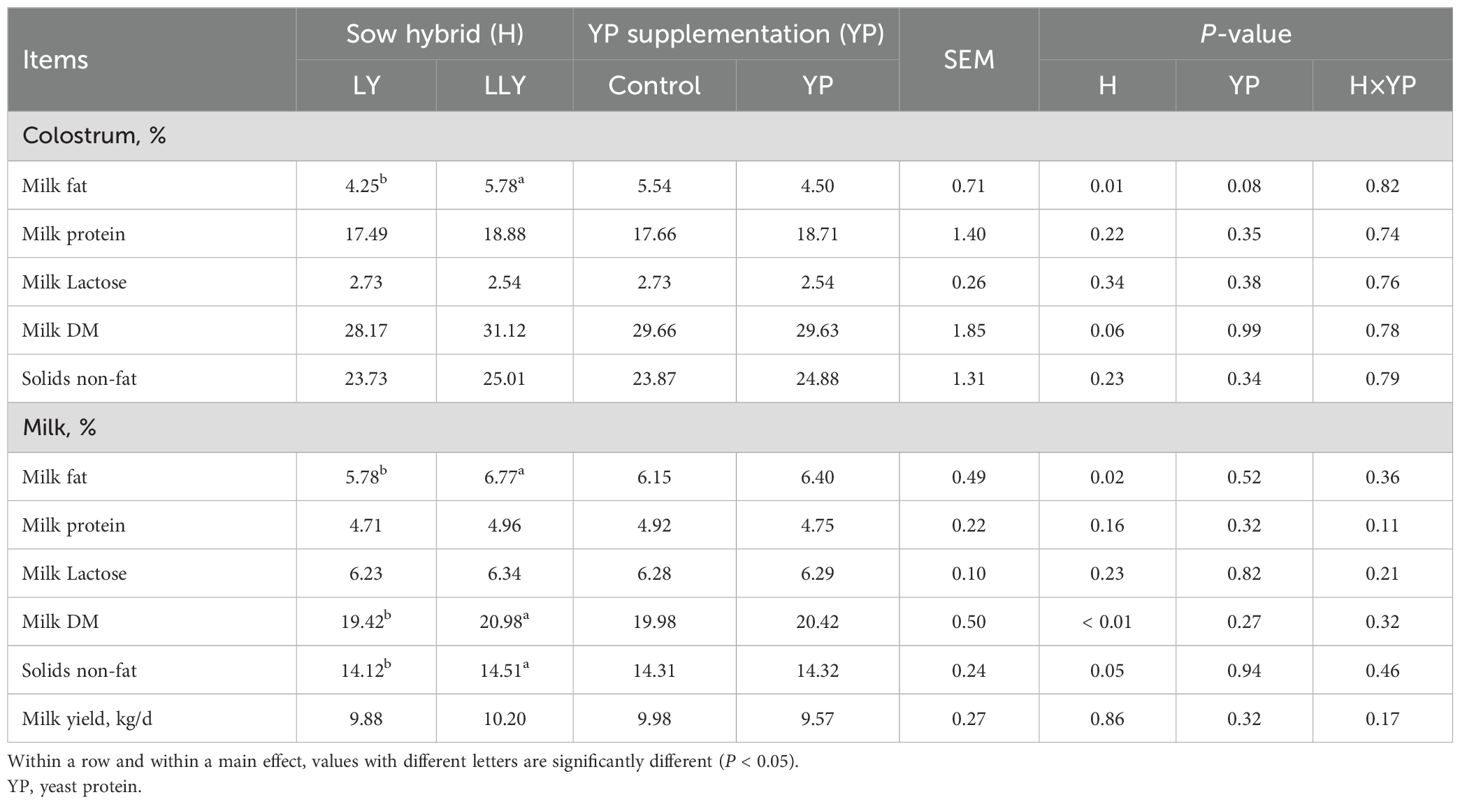
Table 5. The effect of yeast protein supplementation on the milk composition and milk yield of two sow hybrids.
3.3 Concentration of immunoglobulins and inflammatory cytokines in serum
Serum immunological profiling (Table 6) revealed no main effects of sow hybrid nor yeast protein supplementation on serum immunoglobulins or inflammatory cytokines on day 113 of gestation (P > 0.05). However, on day 18 of lactation, LLY sows exhibited 26.8% lower serum IgM concentration than LY sows (2.18 vs. 2.98 mg/mL; P < 0.05), and yeast protein supplementation decreased the serum IgG by 22.6% (2.43 vs. 3.14 mg/mL; P < 0.05) and IL-1β by 45.6% (70.40 vs. 129.53 pg/mL; P < 0.05) in sows. In addition, a significant interactive effect was observed between the main effects on the serum IL-10 content on day 18 of lactation (P = 0.02).
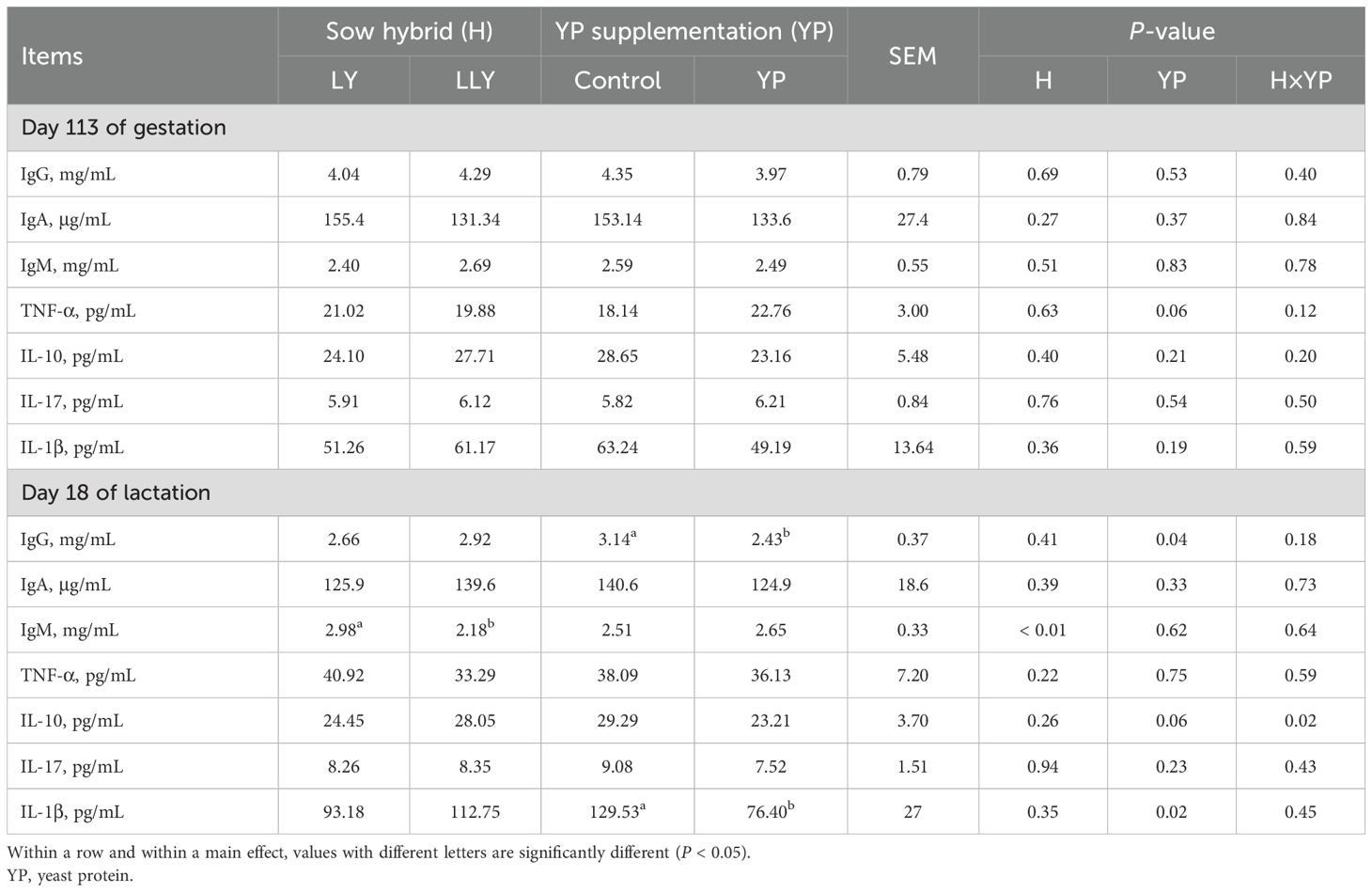
Table 6. The effect of yeast protein supplementation on concentrations of immunoglobulins and inflammatory cytokines in sow serum.
3.4 Concentration of immunoglobulins and inflammatory cytokines in colostrum and milk
Colostrum and milk immunological profiling (Table 7) demonstrated neither sow hybrid nor yeast protein supplementation significantly influenced majority of immunoglobulins and inflammatory cytokines in both colostrum and milk (P > 0.05). However, yeast protein supplementation reduced the IgG concentration in colostrum by 34.3% (2.90 vs. 4.42 mg/mL; P < 0.01).
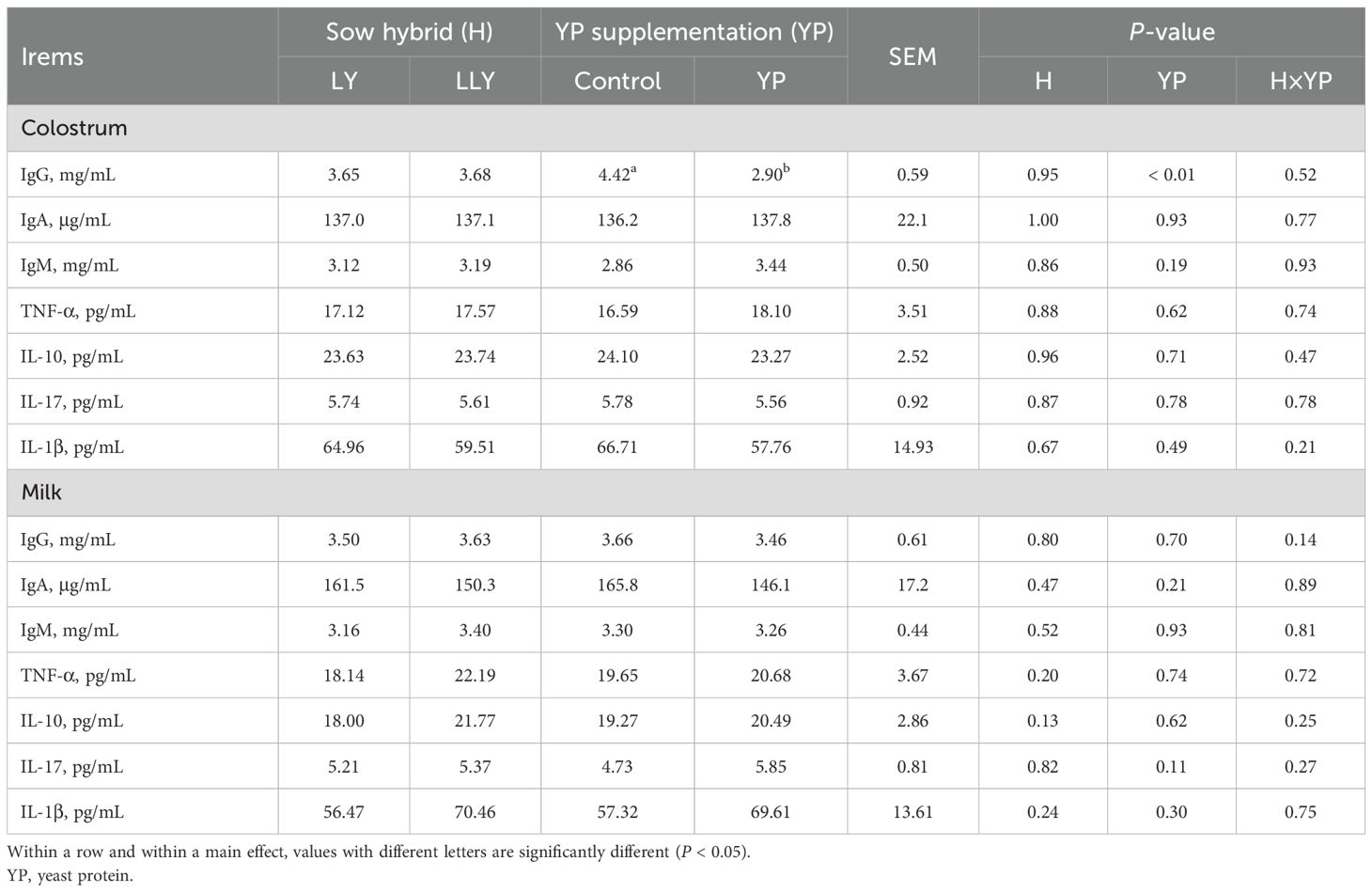
Table 7. The effect of yeast protein supplementation on concentrations of immunoglobulins and inflammatory cytokines in sow milk.
3.5 Analysis of the differences in fecal microbiota between two sow hybrids
The alpha diversity analysis revealed no significant differences in the ACE index, Chao1 index, Shannon index, and Simpson index between LY and LLY sows on day 113 of gestation, day 3 of lactation, and day 18 of lactation (Figures 1A–C; P > 0.05). Beta-diversity assessment through PCoA based on the Bray-Curtis distance matrices demonstrated no clear clustering between the two different sow hybrids at any sampling timepoint (P > 0.05; Figures 2A–C).
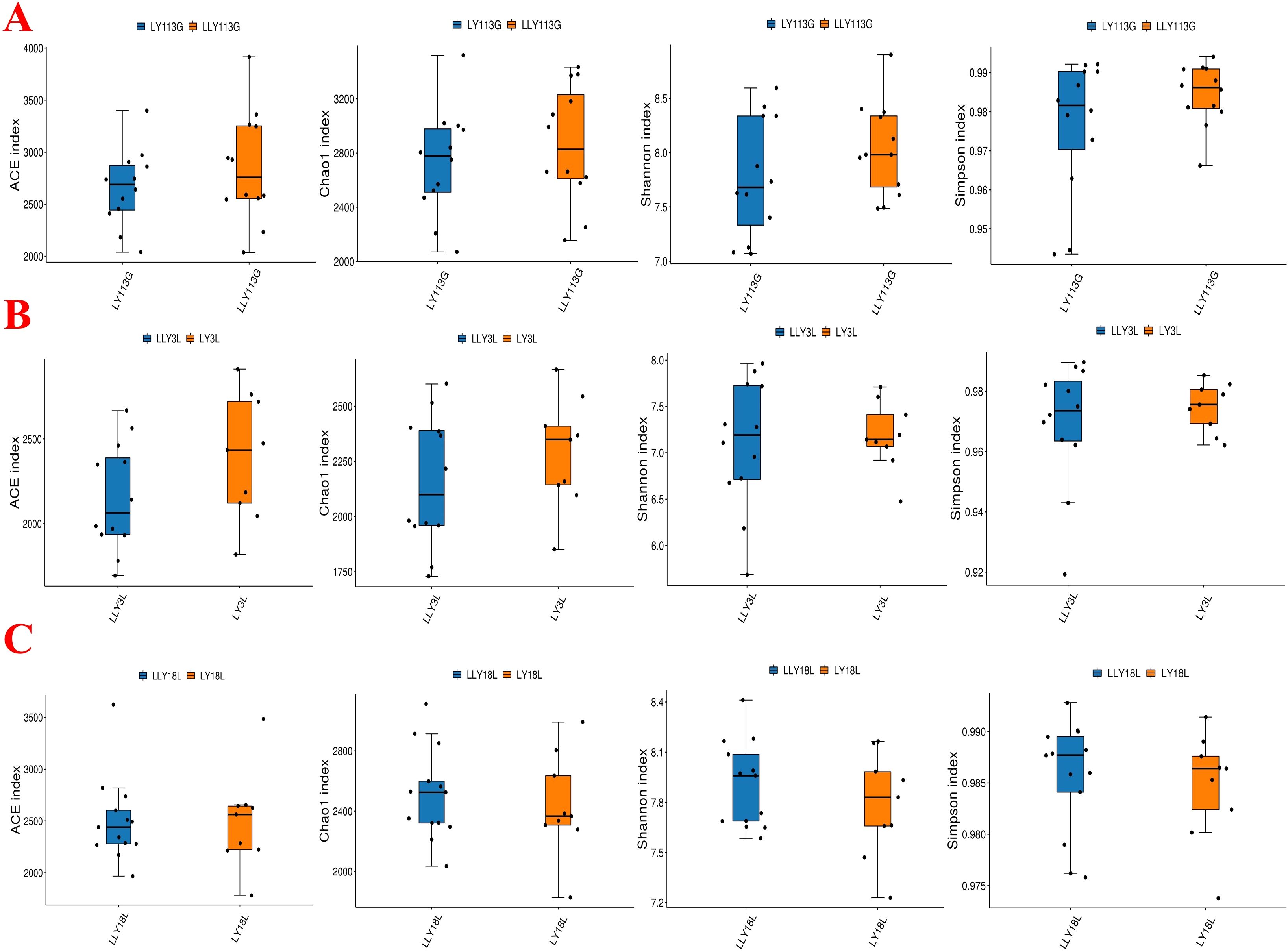
Figure 1. Alpha diversity index of fecal microbiota in two sow hybrids at different stages. (A) 113G, day 113 of gestation. (B) 3L, day 3 of lactation. (C) 18L, day 18 of lactation. LY, Landrace × Yorkshire; LLY, Landrace × Yorkshire × Landrace.
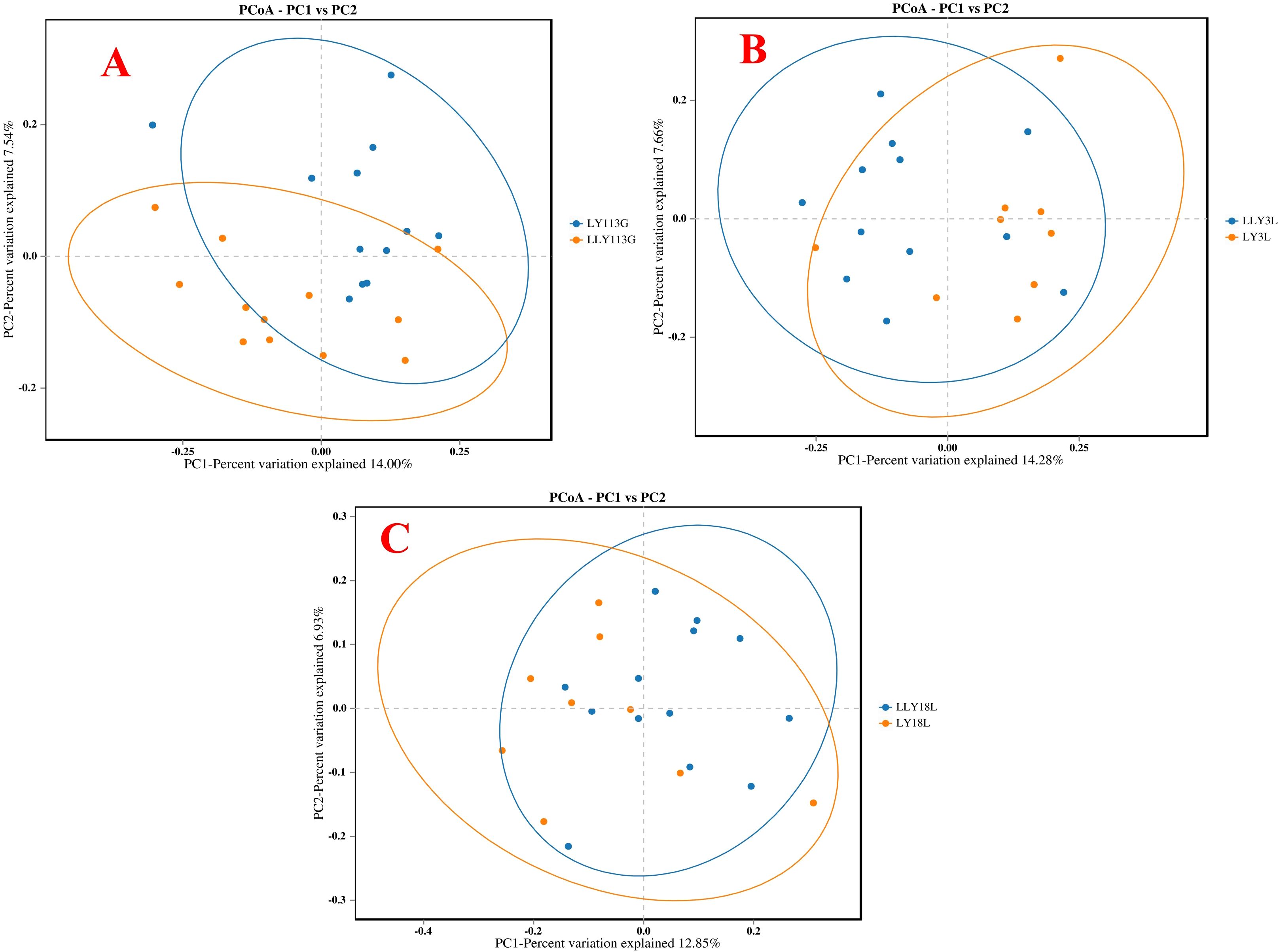
Figure 2. The Principal Coordinate Analysis (PCoA) of bacterial communities in two sow hybrids. (A) 113G, day 113 of gestation. (B) 3L, day 3 of lactation. (C) 18L, day 18 of lactation. LY, Landrace × Yorkshire; LLY, Landrace × Yorkshire × Landra.
Community composition at the phylum level showed Firmicutes as the primary dominant phylum and Bacteroidetes as the secondary dominant phylum across all samples (Figure 3A). The bacterial community composition of the top 10 genera is displayed in Figure 3B. In the two sow hybrids, Rikenellaceae_RC9_gut_group, unclassified_p_2534_18B5_gut_group, and Lactobacillus were the top three prevalent genera on day 113 of gestation. These dominant genera shifted to Christensenellaceae_R_7_group, Lachnospiraceae_NK4A136_group, and Rikenellaceae_RC9_gut_group on day 3 of lactation, and further changed to Rikenellaceae_RC9_gut_group, Christensenellaceae_R_7_group, and uncultured_rumen_bacterium on day 18 of lactation.
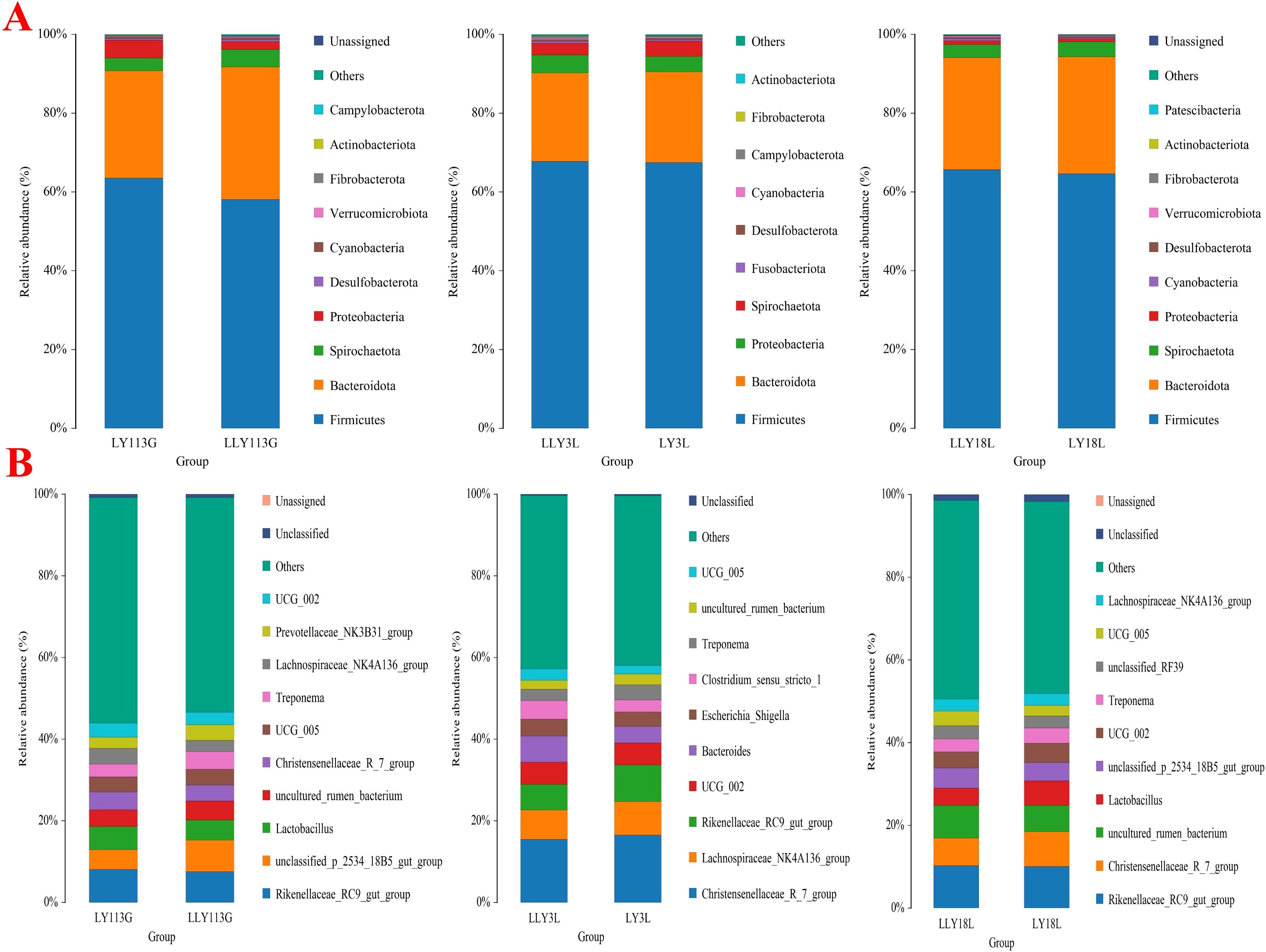
Figure 3. Relative abundances of top 10 bacteria at levels of phyla (A) and genera (B) in two sow hybrids at different stages. LY, Landrace × Yorkshire; LLY, Landrace × Yorkshire × Landrace; 113G, day 113 of gestation; 3L, day 3 of lactation; 18L, day 18 of lactation.
Wilcoxon rank-sum test for the differential microbial genera in the feces of different sow hybrids are shown in Figure 4. On day 113 of gestation, LLY sows exhibited reduced relative abundances of Lachnospiraceae_NK4A136_group, Lachnospiraceae_AC2044_group, and unclassified_Ruminococcaceae, contrasting with elevated abundances of Prevotellaceae_NK3B31_group, Prevotella, and unclassified_UCG_010 compared to LY sows (P < 0.05; Figure 4A). On day 3 of lactation, LLY sows demonstrated enriched relative abundances of Ruminococcus but depleted Lachnospiraceae_AC2044_group compared to LY sows (P < 0.05; Figure 4B). On day 18 of lactation, LLY sows showed significantly higher abundances of unclassified_[Eubacterium]_coprostanoligenes_group, unclassified_Ruminococcaceae, Phascolarctobacterium, and Catenibacterium, alongside reduced dgA_11_gut_group and Limosilactobacillus abundances compared to LY sows (P < 0.05; Figure 4C).
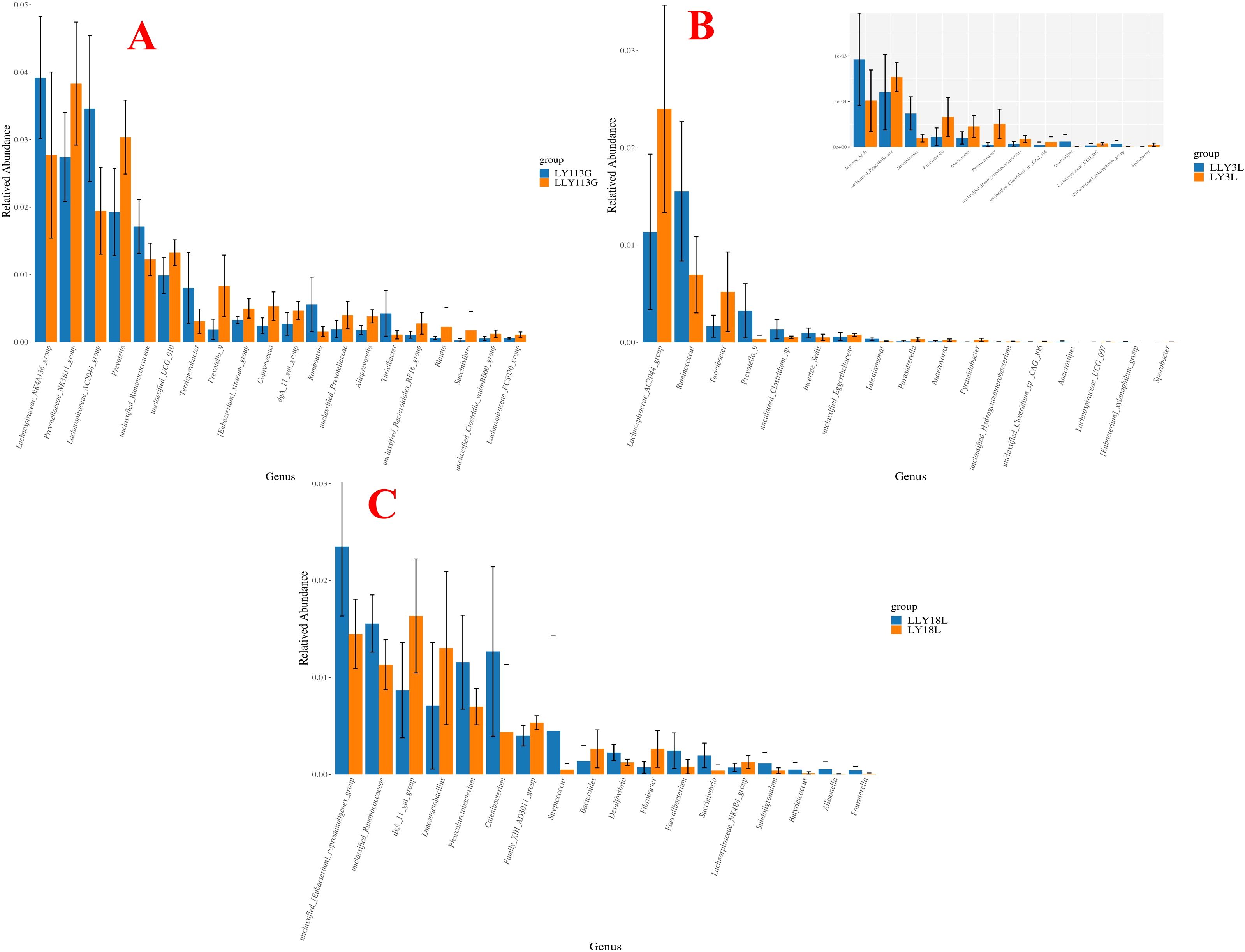
Figure 4. Analysis of differential bacterial genera in fecal microbiota of two sow hybrids at different stages. (A) 113G, day 113 of gestation. (B) 3L, day 3 of lactation. (C) 18L, day 18 of lactation. LY, Landrace × Yorkshire; LLY, Landrace × Yorkshire × Landrace.
3.6 Effects of yeast protein supplementation on the fecal microbiota of sows
The alpha diversity analysis revealed no significant differences in the ACE index, Chao1 index, Shannon index, and Simpson index between control and yeast protein-supplemented sows on day 113 of gestation, day 3 of lactation, and day 18 of lactation (Figures 5A–C; P > 0.05). Beta-diversity assessment through PCoA based on the Bray-Curtis distance matrices demonstrated no clear clustering between the two groups at any sampling timepoint (P > 0.05; Figures 6A–C).
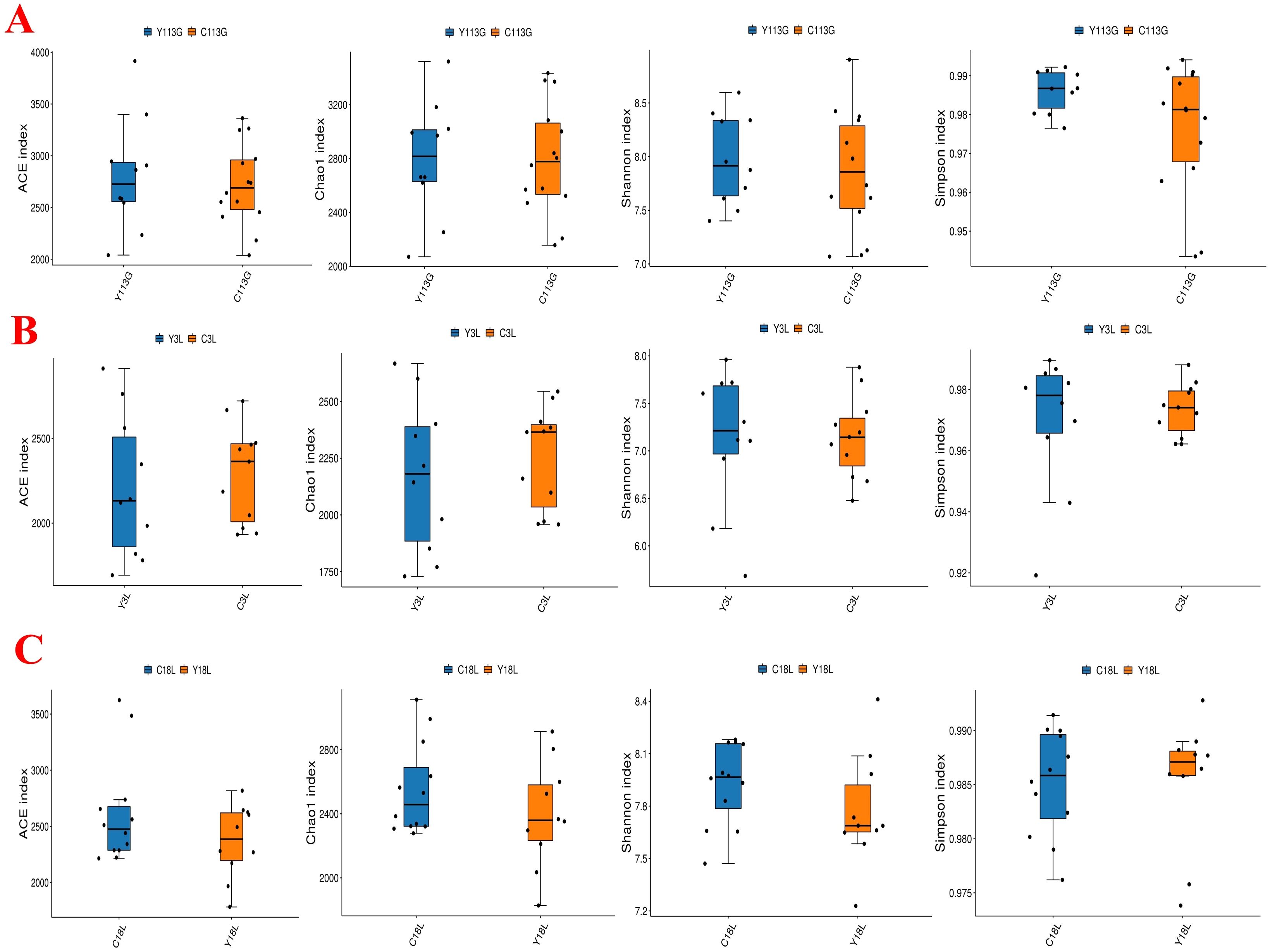
Figure 5. The effect of yeast protein supplementation on the alpha diversity index of fecal microbiota in sows at different stages. (A) 113G, day 113 of gestation. (B) 3L, day 3 of lactation. (C) 18L, day 18 of lactation. C, sows fed with control diet; Y, sows fed with yeast protein-supplemented diet.
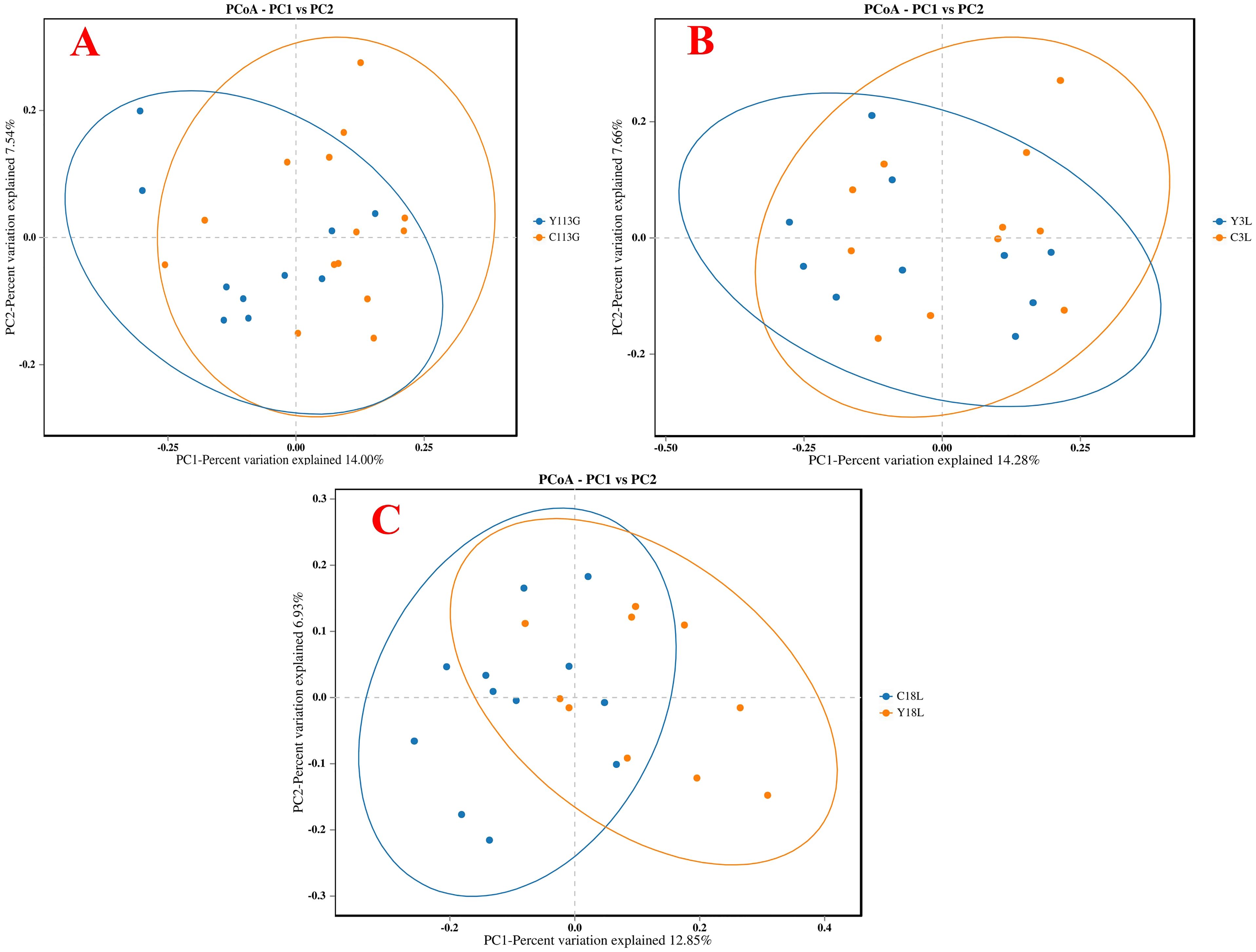
Figure 6. The Principal Coordinate Analysis (PCoA) of bacterial communities in sows fed either a control diet or a yeast protein-supplemented diet. (A) 113G, day 113 of gestation. (B) 3L, day 3 of lactation. (C) 18L, day 18 of lactation. C, sows fed with control diet; Y, sows fed with yeast protein-supplemented diet.
The community composition at the phylum level in both control sows and yeast protein-supplemented sows demonstrated Firmicutes and Bacteroidetes as the dominant phyla across all samples (Figure 7A), consistent with previous observations in different sow hybrids. The bacterial community composition of the top 10 genera during late gestation or lactation is displayed in Figure 7B, which shows the same pattern as previously observed in different sow hybrids.
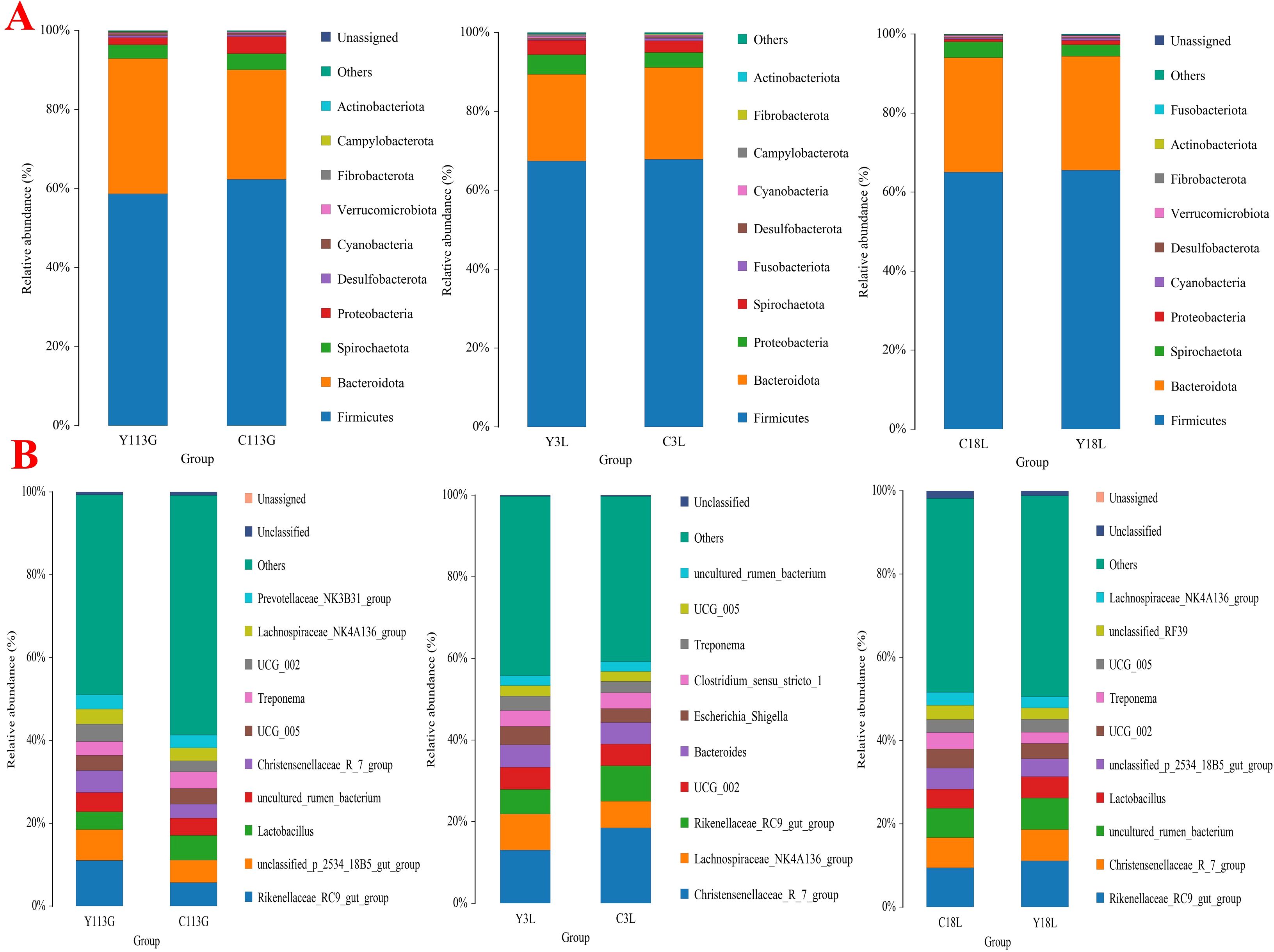
Figure 7. Relative abundances of top 10 bacteria at levels of phyla (A) and genera (B) in sows fed either a control diet or a yeast protein-supplemented diet at different stages. C, sows fed with control diet; Y, sows fed with yeast protein-supplemented diet.
Wilcoxon rank-sum test for the differential microbial genera in the feces of control sows and yeast protein-supplemented sows are shown in Figure 8. On day 113 of gestation, yeast protein supplementation exhibited elevated relative abundances of Rikenellaceae_RC9_gut_group, Christensenellaceae_R_7_group, and UCG_002 compared to control sows (P < 0.05; Figure 8A). On day 3 of lactation, yeast protein supplementation demonstrated enriched relative abundances of Ruminococcus and unclassified_[Eubacterium]_coprostanoligenes_group, but reduced Family_XIII_AD3011_group compared to LY sows (P < 0.05; Figure 8B). On day 18 of lactation, yeast protein supplementation significantly increased the relative abundance of unclassified_[Eubacterium]_coprostanoligenes_group, but decreased the abundances of unclassified_Lachnospiraceae and Lachnospiraceae_XPB1014_group compared to LY sows (P < 0.05; Figure 8C).
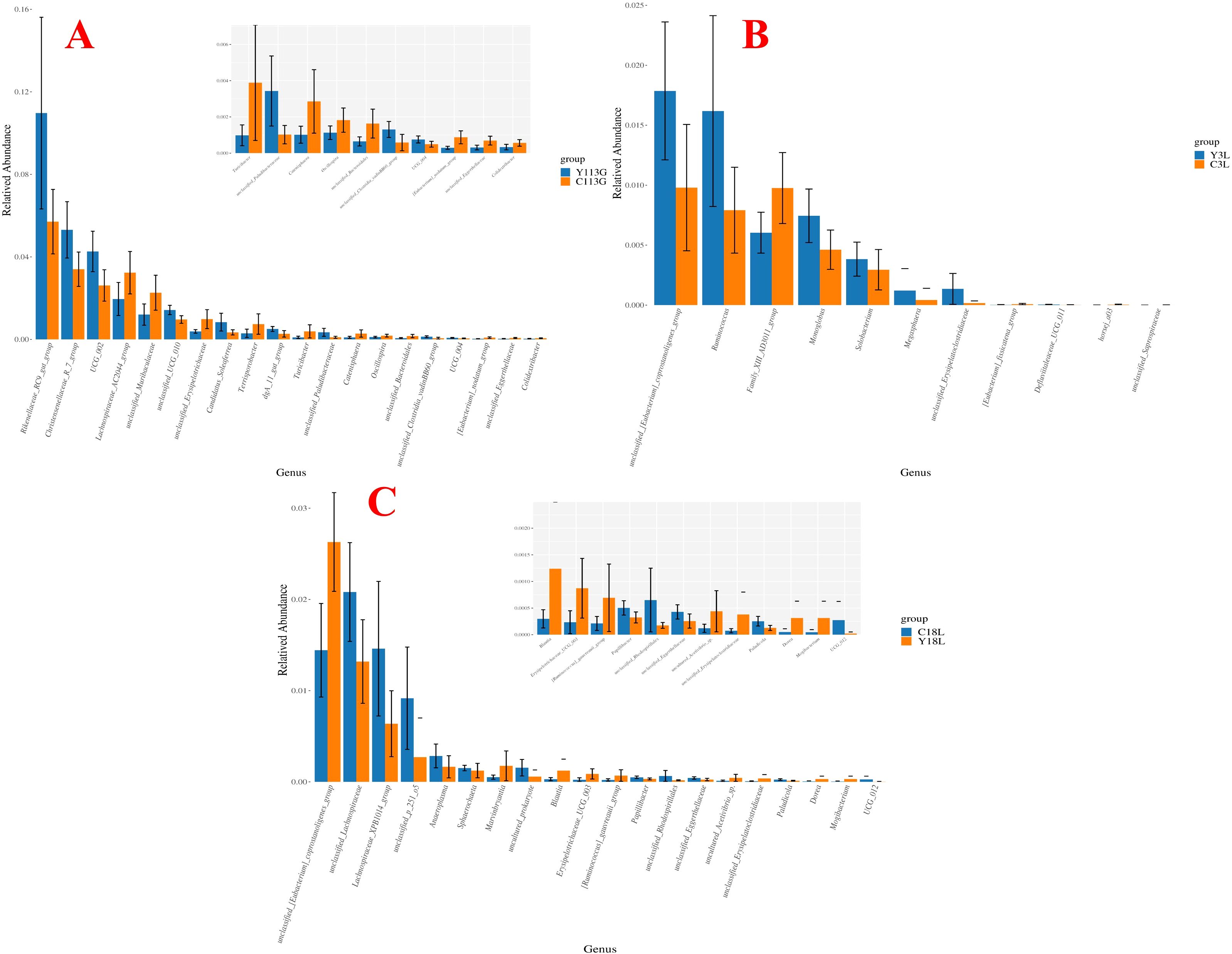
Figure 8. The effect of yeast protein supplementation on the differential bacterial genera in fecal microbiota of sows at different stages. (A) 113G, day 113 of gestation. (B) 3L, day 3 of lactation. (C) 18L, day 18 of lactation. C, sows fed with control diet; Y, sows fed with yeast protein-supplemented diet.
4 Discussion
The present study demonstrated no significant differences in most reproductive and lactation parameters between LY and LLY sows, consistent with earlier research by Sun et al. (2020) and Wang et al. (2021). Furthermore, our study revealed replacement of fishmeal with yeast protein (at a 2.6% inclusion rate) during late gestation to weaning tended to reduce the mummification rate of fetuses, although no statistically significant effects were observed on other reproductive and lactation performance parameters. While previous studies have highlighted the beneficial effects of yeast products as functional additives in improving sow performance, the impact of yeast protein substitution for fishmeal on sow productivity remains elusive (Chen et al., 2024). Our findings align with previous studies reporting comparable reproductive and lactation performance in sows supplemented with either live yeast (Le Floc′h et al., 2022; Xia et al., 2022) or yeast-derived products (Chance et al., 2022) at low-dose ranges of 0.01% to 0.125%. This consistency extends to recent research by Chen et al. (2024), who observed similar outcomes when replacing fishmeal with 0.5% to 2.0% yeast protein supplementation from day 103 of gestation to weaning. However, conflicting evidence exists in the literature. Several studies have reported increased numbers of live-born piglets (Hasan et al., 2018; Bass et al., 2019) and enhanced piglet birth weight (Taylor-Pickard et al., 2017) following supplementation with live yeast or yeast derivatives supplementation at doses ranging from 0.08% to 0.2%. Notably, in addition to variations in yeast product types, the timing of supplementation may contribute to these discrepancies. The aforementioned studies demonstrating improved farrowing outcomes implemented supplementation throughout the entire gestation, whereas our intervention commenced during late gestation. This temporal difference suggests that earlier initiation of yeast protein supplementation during gestation might enhance embryonic survival and consequently increase live-born piglets.
In addition, our study indicated that yeast protein supplementation exerted no significant effects on sow lactation performance, contrasting with several previous research. Previous studies have demonstrated increased sow feed intake (Tan et al., 2021; Zhao et al., 2022), elevated milk yield and composition (Peng et al., 2020; Zhao et al., 2022; Chen et al., 2024), and improved pre-weaning piglet growth performance (Zhao et al., 2022; Liu et al., 2023; Chen et al., 2024; Kim and Duarte, 2024) by utilizing various yeast-based products, including live yeast, yeast culture, yeast extract, yeast hydrolysates, and yeast protein. The absence of significant alterations in colostrum composition and milk yield observed in our study may directly explain the unchanged piglet growth performance during lactation. Notably, we identified that yeast protein supplementation significantly attenuated sow backfat loss during lactation. Excessive loss of backfat and body mobilization may compromise subsequent reproductive performance through increasing the weaning to estrus interval of sows (Thaker and Bilkei, 2005) and reducing ovulation rates and embryonic survivals (Van den Brand et al., 2000; Vinsky et al., 2006). Our results align with previous research documenting the adipose-preserving effects of yeast-derived supplements, including yeast extracts and live yeast (Tan et al., 2021; Sun et al., 2022). As proposed by Sun et al. (2022), the metabolic demands of parturition drive substantial energy expenditure that precipitates backfat depletion. The supplementation of yeast fermentation or culture products, characterized by high nutrient density and bioavailability, appears to enhance the energy reserves of sows, effectively counterbalancing this catabolic process with no effect on feed intake as evidenced in our results.
Serum immunoglobulin concentrations serve as critical indicator of humoral immune response in animals. Changes in these protein levels have been demonstrated to affect animal productivity and immunity. IgA, IgG, and IgM are the main immunoglobulins in the body fluids of humans and animals. IgA governs mucosal immunity within the gastrointestinal tract, IgG is the most important immune factor in secondary immune responses, and IgM is the main immune factor involved in primary immune responses (Hăbeanu et al., 2022). Our study revealed a statistically significant reduction in serum IgM concentrations in LLY sows compared to LY sows on day 18 of lactation. Given the pivotal role of IgM in innate immunity, particularly its ability to neutralize pathogens and act as a cell and pathogen signaler for lysis by complementary cells (Keyt et al., 2020), the observed deficiency suggests diminished immunocompetence in LLY sows during late lactation. Additionally, we found that yeast protein supplementation reduced IgG concentration in both serum on day 18 of lactation and colostrum. This result contrasts with previous studies utilizing yeast-derived product supplementation, which reported either no alterations in colostrum immunoglobulin profiles (Hasan et al., 2018; Bass et al., 2019; Le Floc′h et al., 2022; Xu et al., 2023) or elevated immunoglobulin levels in blood (Xia et al., 2022; Zhao et al., 2022) or colostrum (Quinn et al., 2001; Jang et al., 2013; Zanello et al., 2013; dos Santos et al., 2023). The observed discrepancies may stem from the type of yeast-derived products and the length and rate of inclusion. The yeast protein used in our study, derived from dried yeast through polysaccharide-depletion enrichment, primarily consists of yeast cell proteins and metabolic products. In contrast, yeast products in previous studies retained β-glucan-rich cell walls, known to enhance both innate and adaptive immunity (Zhen et al., 2020; Byrne et al., 2021; Bi et al., 2022; Rhayat et al., 2023). Notably, yeast protein supplementation reduced serum IL-1β concentration by 45.6% in sows on day 18 of lactation, a key pro-inflammatory cytokine linked to systemic inflammation (Parrilla et al., 2020). This anti-inflammatory effect aligns with findings by Fu et al. (2023) and Fan et al. (2024), who observed that supplementation with yeast hydrolysate or yeast fermentation products reduced serum IL-1β concentrations in weaned piglets challenged with lipopolysaccharide or Salmonella typhimurium.
The gut microbiota plays a critical role in animal health by regulating key physiological functions, including nutrient metabolism, growth and development, intestinal barrier maintenance, immune modulation, and protection against pathogen invasion (Barathan et al., 2024). On day 18 of lactation, the relative abundance of dgA_11_gut_group and Limosilactobacillus was significantly lower in LLY sows. DgA-11_gut_group is involved in metabolism of amino acids, energy, and lipids (Sun et al., 2019). A recent study by Li et al. (2024) demonstrated that supplementation with mulberry 1-deoxynijirimycin increased the relative abundance of dgA-11_gut_group, thereby reducing inflammatory responses in rabbits. Chang et al. (2024) demonstrated that the relative abundance of Limosilactobacillus positively correlated with the serum IL-6 concentrations in sows. And this genus has been shown to possess antimicrobial properties and intestinal immune functions, mitigating inflammation and colitis through NF-kB signaling pathway regulation (Liu et al., 2022). Thus, the reduced dgA-11_gut_group and Limosilactobacillus abundance in the feces of LLY sows may explain their lower serum IgM concentrations compared to LY sows observed on day 18 of lactation in this study.
Our study demonstrated that yeast protein supplementation did not alter the α-diversity or β-diversity of gut microbiota in sows, indicating no effects on microbial species diversity and richness in either LLY or LY sows. These findings align with reports by Hasan et al. (2018) and Zhao et al. (2022), though contradictory results exist. Notably, Ma et al. (2023) observed significant increases in Shannon, Simpson, and Sobs indices following gestational yeast culture supplementation. We propose these discrepancies may stem from variations in yeast product types and the timing of supplementation. Our supplementation spanned late gestation to weaning, while Ma’s study lasted from day 30 of gestation to weaning. Our results revealed yeast protein supplementation significantly increased relative abundance of Rikenellaceae_RC9_gut_group, Christensenellaceae_R_7_group, and UCG_002 in sows on day 113 of gestation. These findings align with previous research by Ma et al. (2023), who reported that yeast culture supplementation effectively increased intestinal abundances of Rikenellaceae_RC9_gut_group and Prevotellaceae_NK3B31_group in lactating sows. Cai et al. (2021) reported significantly reduced Rikenellaceae_RC9_gut_group abundance in mice with intestinal inflammation and malnutrition. Christensenellaceae_R_7_group has been identified as a potential beneficial bacterium contributing to gut homeostasis and immune regulation (Kong et al., 2016). These microbial shifts suggest yeast protein benefits late-gestation gut health in sows. During lactation, yeast protein supplementation increased Ruminococcus and unclassified_[Eubacterium]coprostanoligenes_group abundances in sows on day 3, with sustained elevation of the latter through day 18. Concurrently, it reduced Family_XIII_AD3011_group abundance on day 3 and decreased unclassified_Lachnospiraceae and Lachnospiraceae_XPB1014_group on day 18. These findings align with previous studies. Ma et al. (2023) documented similar reductions in Lachnospiraceae_XPB1014_group and Terrisporobacter in yeast culture-supplemented sows, while Zhao et al. (2022) observed increased Ruminococcus and decreased Bacteroidales abundances with yeast culture supplementation. The Ruminococcus genus, comprising two fiber-degrading species, hydrolyzes plant fibers into cellulose and hemicellulose, which are subsequently fermented to volatile fatty acids (VFAs) (Jami and Mizrahi, 2012). This suggests yeast protein-induced increases in Ruminococcus abundance may affect cellulose digestion, VFA production, and energy utilization efficiency, as observed in Tibetan sheep (Ovis aries) supplemented with selenium yeast (Cui et al., 2021). Bai et al. (2024) demonstrated that Eubacterium coprostanoligenes could stimulate mucin production in goblet cells, enhancing intestinal mucus barrier integrity to prevent microbial invasion and reduce the inflammatory response. Notably, Family_XIII_AD3011_group shows negative correlations with acetic acid concentrations (Shi et al., 2020) and impaired disease resistance in Tibetan pigs (Shang et al., 2022). As a conditional pathogen, this genus may induce hypoglycemia through dysbiosis-driven insulin hypersecretion and has been associated with human depression and metabolic disorders (Zhang et al., 2017). In growing pigs, Lachnospiraceae_XPB1014_group abundance negatively correlates with body fat weight (Hu et al., 2019), potentially explaining our observed 30.5% reduction in lactational backfat loss in sows with yeast supplementation through its suppression. Collectively, these microbial shifts likely account for the 45.6% reduction in serum IL-1β concentrations observed in yeast-supplemented sows on day 18 of lactation, indicating yeast protein promotes beneficial bacterial proliferation, and suppresses pathogenic proliferation, thereby improving gut health in lactating sows.
5 Conclusion
LY and LLY sows exhibited comparable reproductive performance, immune function, and gut microbiota, demonstrating the practical feasibility of retaining LLY sows for commercial breeding. Yeast protein supplementation as a substitute for fishmeal during late gestation and lactation significantly reduced lactational backfat loss and moderately attenuated inflammatory response. This effect was likely mediated through selective gut microbiota modulation by promoting beneficial genera such as Christensenellaceae_R_7_group, Ruminococcus and Eubacterium coprostanoligenes, while suppressing specific genera including Family_XIII_AD3011_group and Lachnospiraceae_XPB1014_group. These findings indicate that yeast protein substitution for conventional high-protein ingredients not only reduces feed costs but also improves reproductive performance, immune function, and gut microbiome homeostasis in sows.
Data availability statement
The datasets presented in this study can be found in online repositories. The names of the repository/repositories and accession number(s) can be found below: https://www.ncbi.nlm.nih.gov/, PRJNA1245321.
Ethics statement
The animal study was approved by The Animal Welfare Committee of Southwest University of Science and Technology under ethic approval number L2023021. The study was conducted in accordance with the local legislation and institutional requirements.
Author contributions
PZ: Conceptualization, Formal analysis, Funding acquisition, Writing – original draft. QL: Conceptualization, Formal analysis, Software, Writing – review & editing. YAZ: Conceptualization, Data curation, Visualization, Writing – review & editing. YW: Conceptualization, Data curation, Writing – review & editing. JS: Conceptualization, Writing – review & editing. TD: Conceptualization, Data curation, Writing – review & editing. LC: Conceptualization, Writing – review & editing. YZ: Conceptualization, Writing – review & editing. HY: Conceptualization, Funding acquisition, Supervision, Writing – review & editing.
Funding
The author(s) declare that financial support was received for the research and/or publication of this article. The research was funded by the National Key R&D Program of China (2023YFD1300802), Sichuan Science and Technology Program (2022YFH0063, 2022YFH0064), the Key Project of Science and Technology of Henan Province (242102111021), and the Natural Science Foundation of Southwest University of Science and Technology (19zx7147).
Conflict of interest
The authors declare that the research was conducted in the absence of any commercial or financial relationships that could be construed as a potential conflict of interest.
Generative AI statement
The author(s) declare that no Generative AI was used in the creation of this manuscript.
Publisher’s note
All claims expressed in this article are solely those of the authors and do not necessarily represent those of their affiliated organizations, or those of the publisher, the editors and the reviewers. Any product that may be evaluated in this article, or claim that may be made by its manufacturer, is not guaranteed or endorsed by the publisher.
References
Agboola, J. O., Øverland, M., Skrede, A., Hansen, J. Ø. (2021). Yeast as major protein-rich ingredient in aquafeeds: a review of the implications for aquaculture production. Rev. Aquaculture 13, 949–970. doi: 10.1111/raq.12507
Bai, D., Zhao, J., Wang, R., Du, J., Zhou, C., Gu, C., et al. (2024). Eubacterium coprostanoligenes alleviates chemotherapy-induced intestinal mucositis by enhancing intestinal mucus barrier. Acta Pharm. Sin. B 14, 1677–1692. doi: 10.1016/j.apsb.2023.12.015
Barathan, M., Ng, S. L., Lokanathan, Y., Ng, M. H., Law, J. X.. (2024). The profound influence of gut microbiome and extracellular vesicles on animal health and disease. Int. J. Mol. Sci. 25, 4024. doi: 10.3390/ijms25074024
Bass, B. E., Tsai, T. C., Yang, H., Perez, V., Holzgraefe, D., Chewning, J., et al. (2019). Influence of a whole yeast product (Pichia guilliermondii) fed throughout gestation and lactation on performance and immune parameters of the sow and litter. J. Anim. Sci. 97, 1671–1678. doi: 10.1093/jas/skz060
Bi, S., Zhang, J., Zhang, L., Huang, K., Li, J., Cao, L.. (2022). Yeast cell wall upregulated cell-mediated immune responses to Newcastle disease virus vaccine. Poultry Sci. 101, 101712. doi: 10.1016/j.psj.2022.101712
Byrne, K. A., Tuggle, C. K., Loving, C. L. (2021). Differential induction of innate memory in porcine monocytes by β-glucan or bacillus Calmette-Guerin. Innate Immun. 27, 448–460. doi: 10.1177/1753425920951607
Cai, B., Pan, J., Chen, H., Chen, X., Ye, Z., Yuan, H., et al. (2021). Oyster polysaccharides ameliorate intestinal mucositis and improve metabolism in 5-fluorouracil-treated S180 tumour-bearing mice. Carbohydr. Polymers 256, 117545. doi: 10.1016/j.carbpol.2020.117545
Chance, J. A., DeRouchey, J. M., Amachawadi, R. G., Ishengoma, V., Nagaraja, T. G., Goodband, R. D., et al. (2022). Effects of yeast-based pre-and probiotics in lactation diets of sows on litter performance and antimicrobial resistance of fecal Escherichia coli of sows. J. Anim. Sci. 100, skac165. doi: 10.1093/jas/skac165
Chang, J., Jia, X., Liu, Y., Jiang, X., Che, L., Lin, Y., et al. (2024). Microbial mechanistic insight into the role of yeast-derived postbiotics in improving sow reproductive performance in late gestation and lactation sows. Animals 14, 162. doi: 10.3390/ani14010162
Chen, Z., Li, B., Zhuo, Y., Zhang, Y., Chen, G. (2024). Beneficial effects of maternal supplementation of yeast single-cell protein on suckling piglets by altering sow gut microbiome and milk metabolome. Fermentation 10, 643. doi: 10.3390/fermentation10120643
Cui, X., Wang, Z., Tan, Y., Chang, S., Zheng, H., Wang, H., et al. (2021). Selenium yeast dietary supplement affects rumen bacterial population dynamics and fermentation parameters of Tibetan sheep (Ovis aries) in alpine meadow. Front. Microbiol. 12, 663945. doi: 10.3389/fmicb.2021.663945
dos Santos, M. C., da Silva, K. F., Bastos, A. P. A., Félix, A. P., de Oliveira, S. G., Maiorka, A. (2023). Effect of yeast extracted β-glucans on the immune response and reproductive performance of gilts in the adaptation, gestation, and lactation periods. Livestock Sci. 275, 105289. doi: 10.1016/j.livsci.2023.105289
Espinosa, C. D., Torres-Mendoza, L. J., Stein, H. H. (2023). Torula yeast may improve intestinal health and immune function of weanling pigs. J. Anim. Sci. 101, skad087. doi: 10.1093/jas/skad087
Fan, G., Zhao, Y., Suo, X., Li, Y., Yang, X. (2024). Effects of supplementing yeast fermentation products on growth performance, colonic metabolism, and microbiota of pigs challenged with salmonella Typhimurium. Animals 14, 3675. doi: 10.3390/ani14243675
Fernandes, E., Nepomuceno, N., Trevizam, A., Amorim, H. (1998). From potential to reality: yeasts derived from ethanol production for animal nutrition. J. radioanalytical Nucl. Chem. 234, 113–119. doi: 10.1007/BF02389757
Fu, Y., Li, E., Casey, T. M., Johnson, T. A., Adeola, O., Ajuwon, K. M. (2024). Impact of maternal live yeast supplementation to sows on intestinal inflammatory cytokine expression and tight junction proteins in suckling and weanling piglets. J. Anim. Sci. 102, skae008. doi: 10.1093/jas/skae008
Fu, R., Liang, C., Chen, D., Tian, G., Zheng, P., He, J., et al. (2023). Yeast hydrolysate attenuates lipopolysaccharide-induced inflammatory responses and intestinal barrier damage in weaned piglets. J. Anim. Sci. Biotechnol. 14, 44. doi: 10.1186/s40104-023-00835-2
Gao, L., Xie, C., Liang, X., Li, Z., Li, B., Wu, X., et al. (2021). Yeast-based nucleotide supplementation in mother sows modifies the intestinal barrier function and immune response of neonatal pigs. Anim. Nutr. 7, 84–93. doi: 10.1016/j.aninu.2020.06.009
Hăbeanu, M., Lefter, N. A., Gheorghe, A., Ropota, M., Toma, S. M., Pistol, G. C., et al. (2022). Alterations in essential fatty acids, immunoglobulins (IgA, IgG, and IgM), and enteric methane emission in primiparous sows fed hemp seed oil and their offspring response. Veterinary Sci. 9, 352. doi: 10.3390/vetsci9070352
Hansen, A. V., Strathe, A. B., Kebreab, E., France, J., Theil, P. K. (2012). Predicting milk yield and composition in lactating sows: a Bayesian approach. J. Anim. Sci. 90, 2285–2298. doi: 10.2527/jas.2011-4788
Hasan, S., Junnikkala, S., Peltoniemi, O., Paulin, L., Lyyski, A., Vuorenmaa, J., et al. (2018). Dietary supplementation with yeast hydrolysate in pregnancy influences colostrum yield and gut microbiota of sows and piglets after birth. PloS One 13, e0197586. doi: 10.1371/journal.pone.0197586
Hofacre, C. L., Baxter, J., Berghaus, R., Bonato, M. A., de Souza, F. A. (2024). Yeast cell wall supplementation affects the Salmonella enteretidis load in the ceca and ovaries of layer pullets. Poultry Sci. 103, 104187. doi: 10.1016/j.psj.2024.104187
Hu, C., Li, F., Duan, Y., Yin, Y., Kong, X. (2019). Glutamic acid supplementation reduces body fat weight in finishing pigs when provided solely or in combination with arginine and it is associated with colonic propionate and butyrate concentrations. Food Funct. 10, 4693–4704. doi: 10.1039/C9FO00520J
Islam, M. M., Das, P., Samanta, I., Roy, B., Mukherjee, A., Mondal, T. (2024). Effect of Selenium-Yeast supplementation on gut microbiome, intestinal histomorphology and immunity in broilers. Microbe 3, 100071. doi: 10.1016/j.microb.2024.100071
Jami, E., Mizrahi, I. (2012). Composition and similarity of bovine rumen microbiota across individual animals. PloS One 7, e33306. doi: 10.1371/journal.pone.0033306
Jang, Y. D., Kang, K. W., Piao, L. G., Jeong, T. S., Auclair, E., Jonvel, S., et al. (2013). Effects of live yeast supplementation to gestation and lactation diets on reproductive performance, immunological parameters and milk composition in sows. Livestock Sci. 152, 167–173. doi: 10.1016/j.livsci.2012.12.022
Jin, M., Xiong, J., Zhou, Q. C., Yuan, Y., Wang, X., Sun, P. (2018). Dietary yeast hydrolysate and brewer’s yeast supplementation could enhance growth performance, innate immunity capacity and ammonia nitrogen stress resistance ability of Pacific white shrimp (Litopenaeus vannamei). Fish shellfish Immunol. 82, 121–129. doi: 10.1016/j.fsi.2018.08.020
Keyt, B. A., Baliga, R., Sinclair, A. M., Carroll, S. F., Peterson, M. S. (2020). Structure, function, and therapeutic use of IgM antibodies. Antibodies 9, 53. doi: 10.3390/antib9040053
Kim, S. W., Duarte, M. E. (2024). Saccharomyces yeast postbiotics supplemented in feeds for sows and growing pigs for its impact on growth performance of offspring and growing pigs in commercial farm environments. Anim. Bioscience 37, 1463. doi: 10.5713/ab.23.0467
Kong, Y., Gao, Q., Zhou, D., Feng, Q., Ding, Z., Limbu, S. M., et al. (2025). Dietary yeast hydrolysate improves growth performance, antioxidant capacity, immunity, and intestinal microbiota of juvenile giant freshwater prawn (Macrobrachium rosenbergii). Anim. Feed Sci. Technol. 319, 116196. doi: 10.1016/j.anifeedsci.2024.116196
Kong, F., Hua, Y., Zeng, B., Ning, R., Li, Y., Zhao, J. (2016). Gut microbiota signatures of longevity. Curr. Biol. 26, R832–R833. doi: 10.1016/j.cub.2016.08.015
Le Floc′h, N., Achard, C. S., Eugenio, F. A., Apper, E., Combes, S., Quesnel, H. (2022). Effect of live yeast supplementation in sow diet during gestation and lactation on sow and piglet fecal microbiota, health, and performance. J. Anim. Sci. 100, skac209. doi: 10.1093/jas/skac209
Li, S., Li, T., Jiang, Z., Hou, Q., Serrano, B. R., et al. (2024). Dietary Mulberry leaf 1-deoxynijirimycin supplementation shortens villus height and improves intestinal barrier in fattening rabbits. Anim. Bioscience 37, 2101. doi: 10.5713/ab.24.0109
Liu, Y., Jia, X., Chang, J., Jiang, X., Che, L., Lin, Y., et al. (2023). Effect of yeast culture supplementation in sows during late gestation and lactation on growth performance, antioxidant properties, and intestinal microorganisms of offspring weaned piglets. Front. Microbiol. 13, 1105888. doi: 10.3389/fmicb.2022.1105888
Liu, B., Yang, L., Wu, Y., Zhao, X. (2022). Protective effect of Limosilactobacillus fermentum HFY06 on dextran sulfate sodium-induced colitis in mice. Front. Microbiol. 13, 935792. doi: 10.3389/fmicb.2022.935792
Liu, Y., Zhang, X., Qi, W., Yang, Y., Liu, Z., An, T., et al. (2021). Prevention and control strategies of African swine fever and progress on pig farm repopulation in China. Viruses 13, 2552. doi: 10.3390/v13122552
Ma, M., Wang, H. H., Hua, Y., Qin, F., Yang, J. (2021). African swine fever in China: Impacts, responses, and policy implications. Food Policy 102, 102065. doi: 10.1016/j.foodpol.2021.102065
Ma, Z., Wu, Z., Wang, Y., Meng, Q., Chen, P., Li, J., et al. (2023). Effect of yeast culture on reproductive performance, gut microbiota, and milk composition in primiparous sows. Animals 13, 2954. doi: 10.3390/ani13182954
Maina, A. N., Schulze, H., Kiarie, E. G. (2025). Effects of lifetime feeding of hydrolyzed yeast to broiler breeders on egg production, quality, and hatchling attributes. Poultry Sci. 104, 104826. doi: 10.1016/j.psj.2025.104826
Mou, D., Ding, D., Pu, J., Zhou, P., Cao, E., Zhang, X., et al. (2025). Effects of dietary pretreatment with All-trans lycopene on lipopolysaccharide-induced jejunal inflammation: a multi-pathway phenomenon. Foods 14, 794. doi: 10.3390/foods14050794
Pang, Y., Zhang, H., Wen, H., Wan, H., Wu, H., Chen, Y., et al. (2022). Yeast probiotic and yeast products in enhancing livestock feeds utilization and performance: An overview. J. Fungi 8, 1191. doi: 10.3390/jof8111191
Parrilla, I., Martinez, C. A., Cambra, J. M., Lucas, X., Ferreira-Dias, G., Rodriguez-Martinez, H., et al. (2020). Blastocyst-bearing sows display a dominant anti-inflammatory cytokine profile compared to cyclic sows at day 6 of the cycle. Animals 10, 2028. doi: 10.3390/ani10112028
Peng, X., Yan, C., Hu, L., Huang, Y., Fang, Z., Lin, Y., et al. (2020). Live yeast supplementation during late gestation and lactation affects reproductive performance, colostrum and milk composition, blood biochemical and immunological parameters of sows. Anim. Nutr. 6, 288–292. doi: 10.1016/j.aninu.2020.03.001
Qiu, Q., Zhan, Z., Zhou, Y., Zhang, W., Gu, L., Wang, Q., et al. (2024). Effects of yeast culture on laying performance, antioxidant properties, intestinal morphology, and intestinal flora of laying hens. Antioxidants 13, 779. doi: 10.3390/antiox13070779
Quinn, P. R., Funderburke, D. W., Tibbetts, G. W. (2001). Effects of dietary supplementation with mannan oligosaccharides on sow and litter performance in a commercial production system. J. Anim. Sci. 79, 212.
Rhayat, L., Even, G., Kiros, T. G., Kuhn, G., Lebrun-Ruer, S., Audebert, C., et al. (2023). The effect of yeast-derived β-glucans in reducing the adverse outcome of Lawsonia intracellularis in finishing pigs. Front. Anim. Sci. 4, 1245061. doi: 10.3389/fanim.2023.1245061
Shang, P., Wei, M., Duan, M., Yan, F., Chamba, Y. (2022). Healthy gut microbiome composition enhances disease resistance and fat deposition in Tibetan pigs. Front. Microbiol. 13, 965292. doi: 10.3389/fmicb.2022.965292
Shi, J., Zhao, D., Song, S., Zhang, M., Zamaratskaia, G., Xu, X., et al. (2020). High-meat-protein high-fat diet induced dysbiosis of gut microbiota and tryptophan metabolism in Wistar rats. J. Agric. Food Chem. 68, 6333–6346. doi: 10.1021/acs.jafc.0c00245
Shurson, G. C. (2018). Yeast and yeast derivatives in feed additives and ingredients: Sources, characteristics, animal responses, and quantification methods. Anim. feed Sci. Technol. 235, 60–76. doi: 10.1016/j.anifeedsci.2017.11.010
Sun, H., De Laguna, F. B., Wang, S., Liu, F., Shi, L., Jiang, H., et al. (2022). Effect of Saccharomyces cerevisiae boulardii on sows’ farrowing duration and reproductive performance, and weanling piglets’ performance and IgG concentration. J. Anim. Sci. Technol. 64, 10–22. doi: 10.5187/jast.2021.e106
Sun, X., Shen, J., Liu, C., Li, S., Peng, Y., Chen, C., et al. (2019). L-arginine and N-carbamoylglutamic acid supplementation enhance young rabbit growth and immunity by regulating intestinal microbial community. Asian-Australasian J. Anim. Sci. 33, 166. doi: 10.5713/ajas.18.0984
Sun, Z., Zhang, L., Dong, Y., Yang, H. (2020). National survey and analysis of breeding sow productivity and production recommendations for the future. China Swine Industry (Chinese Version) 15, 12–16. doi: 10.16174/j.cnki.115435.2020.05.003
Tan, C. Q., Li, J. Y., Ji, Y. C., Yang, Y. Y., Zhao, X. C., Chen, M. X., et al. (2021). Effects of dietary supplementation of different amounts of yeast extract on oxidative stress, milk components, and productive performance of sows. Anim. Feed Sci. Technol. 274, 114648. doi: 10.1016/j.anifeedsci.2020.114648
Taylor-Pickard, J., McArdle, T., Icely, S. (2017). Effect of feeding Actigen™ to sows during gestation and lactation and on piglet performance. J. Appl. Anim. Nutr. 5, e1. doi: 10.1017/Jan.2017.2
Thaker, M. Y. C., Bilkei, G. (2005). Lactation weight loss influences subsequent reproductive performance of sows. Anim. Reprod. Sci. 88, 309–318. doi: 10.1016/j.anireprosci.2004.10.001
Van den Brand, H., Dieleman, S. J., Soede, N. M., Kemp, B. (2000). Dietary energy source at two feeding levels during lactation of primiparous sows: I. Effects on glucose, insulin, and luteinizing hormone and on follicle development, weaning-to-estrus interval, and ovulation rate. J. Anim. Sci. 78, 396–404. doi: 10.2527/2000.782396x
Vinsky, M. D., Novak, S., Dixon, W. T., Dyck, M. K., Foxcroft, G. R. (2006). Nutritional restriction in lactating primiparous sows selectively affects female embryo survival and overall litter development. Reproduction fertility Dev. 18, 347–355. doi: 10.1071/RD05142
Wang, Y., Ai, J., Xie, J. (2021). Feeding and management strategies for two-breed backcross sows. China Swine Industry (Chinese Version) 16, 35–40. doi: 10.16174/j.cnki.115435.2021.02.007
Xia, T., Yin, C., Comi, M., Agazzi, A., Perricone, V., Li, X., et al. (2022). Live yeast supplementation in gestating and lactating primiparous sows improves immune response in dams and their progeny. Animals 12, 1315. doi: 10.3390/ani12101315
Xu, S., Jia, X., Liu, Y., Pan, Xu., Chang, J., Wei, W., et al. (2023). Effects of yeast-derived postbiotic supplementation in late gestation and lactation diets on performance, milk quality, and immune function in lactating sows. J. Anim. Sci. 101, skad201. doi: 10.1093/jas/skad201
Yin, J., Liu, H., Li, T., Yin, Y. (2019). Current situation and developmental suggestions on shortage of feeding protein resources in Chinese pig industry. Bull. Chin. Acad. Sci. (Chinese Version) 34, 89–93. doi: 10.16418/j.issn.1000-3045.2019.01.011
Yu, C., Hu, X., Yang, H. (2020). The application of reciprocal crossbreeding in pig production-a review of the Muyuan reciprocal two-breed breeding system. Swine Sci. (Chinese Version) 37, 56–58.
Zanello, G., Meurens, F., Serreau, D., Chevaleyre, C., Melo, S., Berri, M., et al. (2013). Effects of dietary yeast strains on immunoglobulin in colostrum and milk of sows. Veterinary Immunol. immunopathology 152, 20–27. doi: 10.1016/j.vetimm.2012.09.023
Zhang, Q., Xiao, X., Li, M., Yu, M., Ping, F., Zheng, J., et al. (2017). Vildagliptin increases butyrate-producing bacteria in the gut of diabetic rats. PloS One 12, e0184735. doi: 10.1371/journal.pone.0184735
Zhao, Y., Wang, Q., Zhou, P., Li, Z., Zhong, W., Zhuo, Y., et al. (2022). Effects of yeast culture supplementation from late gestation to weaning on performance of lactating sows and growth of nursing piglets. Animal 16, 100526. doi: 10.1016/j.animal.2022.100526
Zhen, W., Shao, Y., Wu, Y., Li, L., Pham, V., Abbas, W., et al. (2020). Dietary yeast β-glucan supplementation improves eggshell color and fertile eggs hatchability as well as enhances immune functions in breeder laying hens. Int. J. Biol. Macromolecules 159, 607–621. doi: 10.1016/j.ijbiomac.2020.05.134
Zheng, L., Xie, S., Zhuang, Z., Liu, Y., Tian, L., Niu, J. (2021). Effects of yeast and yeast extract on growth performance, antioxidant ability and intestinal microbiota of juvenile Pacific white shrimp (Litopenaeus vannamei). Aquaculture 530, 735941. doi: 10.1016/j.aquaculture.2020.735941
Keywords: yeast protein, reproductive performance, immune response, gut microbiota, sow hybrid
Citation: Zhou P, Liu Q, Zhao Y, Wu Y, Shen J, Duan T, Che L, Zhang Y and Yan H (2025) Yeast protein as a fishmeal substitute: impacts on reproductive performance, immune responses, and gut microbiota in two sow hybrids. Front. Cell. Infect. Microbiol. 15:1579950. doi: 10.3389/fcimb.2025.1579950
Received: 19 February 2025; Accepted: 24 March 2025;
Published: 22 April 2025.
Edited by:
Yang Li, Shandong Agricultural University, ChinaCopyright © 2025 Zhou, Liu, Zhao, Wu, Shen, Duan, Che, Zhang and Yan. This is an open-access article distributed under the terms of the Creative Commons Attribution License (CC BY). The use, distribution or reproduction in other forums is permitted, provided the original author(s) and the copyright owner(s) are credited and that the original publication in this journal is cited, in accordance with accepted academic practice. No use, distribution or reproduction is permitted which does not comply with these terms.
*Correspondence: Honglin Yan, aG9uZ2xpbnlhbkBzd3VzdC5lZHUuY24=
†These authors have contributed equally to this work