- Unit of Chemical Ecology, Department of Plant Protection Biology, Swedish University of Agricultural Sciences, Alnarp, Sweden
Food availability and nutritional status shape the reproductive activity of many animals. In rodents, hormones such as gonadotropin-releasing hormone (GnRH), restore energy homeostasis not only through regulating e.g., caloric intake and energy housekeeping, but also through modulating sex drive. We investigated whether the insect homolog of the GnRH receptor, the adipokinetic hormone receptor (AKHR) modulates sexual behavior of the fruit fly Drosophila melanogaster depending on nutritional status. We found that AKHR regulates male, but not female sexual behavior in a starvation-dependent manner. Males lacking AKHR showed a severe decrease in their courtship activity when starved, as well as an increase in mating duration when fed. AKHR expression is particularly strong in the subesophageal zone (SEZ, Ito et al., 2014). We found axonal projections from AKHR-expressing neurons to higher brain centers including specific glomeruli in the antennal lobe. Among the glomeruli that received projections were those dedicated to detecting the male specific pheromone cis-vaccenyl acetate (cVA). Accordingly, responses to cVA were dependent on the nutritional status of flies. AKHR was also involved in the regulation of the production of cuticular pheromones, 7,11-heptacosadiene and 7-tricosene. This effect was observed only in females and depended on their feeding state. AKHR has therefore a dual role on both pheromone perception and production. For the first time our study shows an effect of AKHR on insect sexual behavior and physiology. Our results support the hypothesis of a conserved role of the GnRH/AKH pathway on a nutritional state-dependent regulation of reproduction in both vertebrates and invertebrates.
Introduction
Food availability and food deprivation affect the reproduction of a wide range of animals including mammals, fishes, insects, or spiders, through acting on both physiological and behavioral traits (Franssen et al., 2008; Martin et al., 2008; Moskalik and Uetz, 2011). Hormones that are regulated by food intake control many of these reproductive traits (Kauffman et al., 2006; Martin et al., 2008). In rodents, the most widespread variant of the gonadotropin-releasing hormone, GnRH-II, influences female, but not male, sexual behavior depending on food availability (Kauffman and Rissman, 2004). This hormone acts via a specific receptor and is thought to synchronize reproduction with energetically favorable conditions (Kauffman and Rissman, 2004). In invertebrates, the adipokinetic hormone receptor (AKHR) is structurally and evolutionarily related to the mammalian GnRH receptor (Lindemans et al., 2009). AKHR is activated by adipokinetic hormone (AKH), which is, however, homologous to glucagon instead of GnRH. Like glucagon, AKH plays a key role in sugar and lipid metabolism and homeostasis (Lee and Park, 2004). Its hormonal ligand, AKH, is produced and released by corpora cardiaca cells in response to starvation, and is involved in energy mobilization from the fat body, food intake and nutrient absorption in the midgut (Liu et al., 2009; Kodrík et al., 2012).
For the past few decades, the fruit fly D. melanogaster has become a model system to study the genetic basis of fundamental biological processes such as energy metabolism and sexual behavior (Sokolowski, 2001; Baker and Thummel, 2007). In the wild, Drosophila flies aggregate, which is partly mediated by the male-produced pheromone cis-vaccenyl acetate (cVA), a pheromone that is also involved in courtship (Bartelt et al., 1985). Other pheromones also play an important role in courtship, such as 7-tricosene (7-T, mainly produced by males), and 7,11-heptacosadiene (7,11 HD, female-specific, Ferveur, 2005). The synthesis and expression of these pheromones depend, at least partly, on lipid metabolism. As fatty acid derivatives, they are carried in the hemolymph by lipophorin, a general lipid transporter, from their synthesis site (oenocytes) to the cuticle (Pho et al., 1998; Schal et al., 2001; Ferveur, 2005). Moreover, pheromone expression is affected by diet quality (Ferveur, 2005; Sharon et al., 2010; Fedina et al., 2012). Pheromones are detected either by olfactory sensory neurons housed in the antenna (cVA) or gustatory sensory neurons (7-T, 7,11-HD, CH5O3) that project to specific structures in the central nervous system, respectively, the antennal lobes (AL) and the subesophageal zone (SEZ, Pavlou and Goodwin, 2013; Shankar et al., 2015).
Recently, Lindemans et al. (2009) demonstrated that AKH signaling through the GnRH receptor modulates egg-laying behavior in the nematode Caenorhabditis elegans. They therefore hypothesized that the role of the AKH/GnRH pathway on reproduction might have been developed before the divergence of protostomians and deuterostomians. However, involvement of AKH and/or AKHR in modulating sexual behavior in insects has not been demonstrated yet. In the present study, using AKHR-deficient flies, we studied the effect of AKH signaling on courtship, mating, pheromone detection and pheromone production in D. melanogaster.
Materials and Methods
Drosophila Stocks and Rearing Conditions
The null mutant Akhrnull was used to study the effect of AKHR and the revertant Akhrrev was used as a control (Bharucha et al., 2008). In mating experiments, the wild-type line Dalby (Ruebenbauer et al., 2008; WT) was used as a mate for Akhrnull and Akhrrev flies. For labeling of AKHR expressing neurons the transgenic Akhr-Gal4 line was crossed with a UAS-mcd8::GFP line (double homozygous on 2nd and 3rd chromosomes). A total of more than 200 fly brains of 3–8 days old were processed, less than half of them showing staining in the antennal lobes. The innervation patterns of AKHR-expressing neurons appeared sexually isomorphic. Flies were reared on a standard sugar-yeast-cornmeal medium at room temperature (19–22°C) and under a 10:14 h L:D photoperiod. Shortly after emergence, virgin flies were briefly anesthetized with carbon dioxide and sexed under a microscope. Fed flies were kept on a standard medium until they were used for experiments. Starved flies were placed on a humidified piece of cotton for 3 days. Mortality rate during starvation was low for both lines. For all the experiments 3-day old flies were used. Akhrnull, Akhrrev and Akhr-Gal4 lines were obtained from K. Bharucha (University of Texas Southwestern Medical School, Dallas, Texas, USA) and the UAS-GFP line was obtained from T. Chertemps (Université Paris 6, Paris, France).
Courtship and Mating Experiments
Mating arenas consisted of a small round inverted cup (45 mm in diameter, 30 mm high) placed on a clean glass plate. An Akhrnull or Akhrrev fly (male or female, fed or starved; n = 35 for each group) was grouped with a fed WT fly of the opposite sex and their behavior was observed for 1 h or until the male dismounted the female following mating. The percentage of males displaying courtship (behavioral sequence including the steps from wing vibration to attempting copulation, Sokolowski, 2001), the percentage of mating pairs and the copulation duration were recorded. The percentage of females being courted by wild-type males was used to estimate their receptiveness.
Pheromone Attraction
Pheromone attraction was tested in a glass Y-tube olfactometer bioassay, similar to the one described in a previous study (Lebreton et al., 2015). Individual Akhrnull and Akhrrev males (n = 31–36, respectively) were released at the entrance of the olfactometer and given a choice between a combination of white wine vinegar odors plus cVA or vinegar odors alone. cVA (1.5 μg/μl, Pherobank, Wageningen, the Netherlands) was applied at a rate of 10 μl/min using a piezoelectric sprayer. Tests lasted 5 min. The time spent by flies in each arm of the olfactometer was recorded. A Preference Index (PI) was then calculated as follows:
The PI thus ranged from 1 (preference for vinegar odors +cVA) to −1 (preference for vinegar odors alone).
Immunostaining
Female and male flies of AKHR-Gal4 × UAS-GFP (double homozygote, see above) were decapitated and heads were fixed in PBS (0.01 M) with 0.25% Triton-X (PBS0.25) +4% formaldehyde for 2.5 h at 4°C, dissected, washed 3 × with PBS containing 0.25% Triton-X (PBS0.25) and incubated with mouse anti-synapsin antibody (1:10, Developmental Studies Hybridoma Bank, University of Iowa). After 24 h at room temperature on the rotator brains were washed 3 × with PBS0.25, and incubated with anti-mouse conjugated with Alexafluor 546 (1:100, Molecular Probes, CA, USA) and anti-GFP antibody conjugated with Alexafluor 488. Following 24 h at RT on the rotator, brains were washed in PBS0.25, and mounted in Vectashield Hard Mount (Vector Laboratories, Burlingame, CA), using spacer rings (0.12 mm thick, Secure-Seal™ imaging spacers, Sigma-Aldrich). Brains were scanned using a Zeiss Laser Scanning Microscope, LSM 510.
Chemical Analysis of Pheromones
Pheromones were extracted from the fly cuticle by placing individual flies in 100 μl of hexane for 5 min at room temperature. Hundred ng of heptadecenyl acetate was added as an internal standard. Extracts of single flies (n = 5 for each group) were concentrated to ca. 10 and 2 μl was injected into a gas chromatograph coupled mass spectrometer (GC-MS; 6890 GC and 5975 MS, Agilent technologies Inc., Santa Clara, CA, USA) equipped with a 5 MS silica capillary column (30 m × 0.25 mm × 0.25 μm film thickness; Agilent technologies Inc.) and temperature-programmed from 150 to 200°C at 8°C/min and from 200 to 300°C at 3°C/min.
Cuticular hydrocarbons (CHCs) were quantified by peak integration and the relative amount was calculated as a proportion of the area of the internal standard peak. Compounds of interest [cVA, 7-tricosene (7-T) and 7,11-heptacosadiene (7,11-HD)] were identified based on their mass spectrum, retention time, comparison with already published works on D. melanogaster CHCs (mainly Everaerts et al., 2010) and injection of synthetic corresponding compound (for cVA). Because 7,11-HD co-eluted with 2-methylhexacosane, the relative amount of 7,11-HD was quantified by peak integration of a diagnostic fragment specific for 7,11-HD (m/z = 278).
Statistical Analysis
The percentage courtship and mating observed between Akhrnull and Akhrrev were analyzed using a Fisher's exact test. Mating duration and pheromone production were analyzed by a non-parametric Mann-Whitney U test. Concerning cVA attraction, PIs were compared to a theoretical value of 0 (no attraction) using a Wilcoxon's signed-rank test and were compared between the two lines with a Mann-Whitney U test.
The statistical analyses were performed in R (R 2.1.1, R Development Core Team, Free Software Foundation Boston, MA, USA).
Results
AKHR Affects Male Mating Behavior in a Starvation Dependent Manner
When lacking AKHR, fewer starved males courted WT females (Fisher test, p < 0.001) (Figure 1A). This effect disappeared when males were previously fed ad-libitum (Fisher test, p = 0.014, Figure 1B). However, fed Akhrnull males increased their mating duration compared to Akhrrev males (W = 85, p < 0.001) (Figure 1B). The mating success (proportion of males that copulated) was not affected (starved males: p = 0.3; fed males: p = 0.62). Females were not affected in their attractiveness (WT males courted both Akhrnull and Akhrrev females equally much) or sexual receptivity (both females accepted to mate in the same proportion), independent of nutritional state (Figures 1A,B).
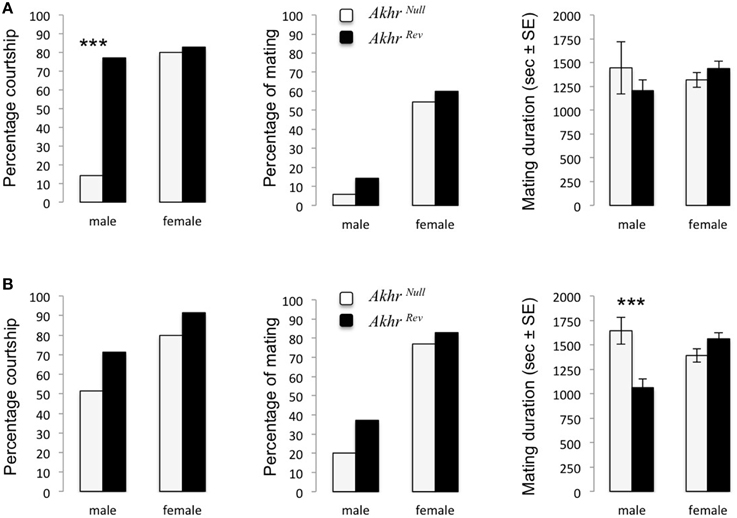
Figure 1. Courtship and mating behavior of AKHR deficient flies. Proportion of couples (an Akhrnull or Akhrrev male or female with a wild-type fly of the opposite sex) courting, mating and the corresponding mating duration. Akhrnull and Akhrrev flies were either (A) starved or (B) fed. ***p < 0.001.
AKHR is Expressed in Neurons that Connect to Pheromone Sensing Glomeruli and Affects Pheromone Response
Immunostaining using an Akhr-Gal4 line show profuse staining in the subesophageal zone (SEZ, Bharucha et al., 2008), from where ascending collaterals were found projecting to the antennal lobes and higher brain centers (Figures 2A–C; Bharucha et al., 2008). A total of nine antenna lobe glomeruli were observed receiving projections from these neurons, among which three glomeruli with relatively profuse arborizations: the cVA sensing glomeruli DA1 and DL3, as well as the glomerulus VA2 (Figures 2A,C).
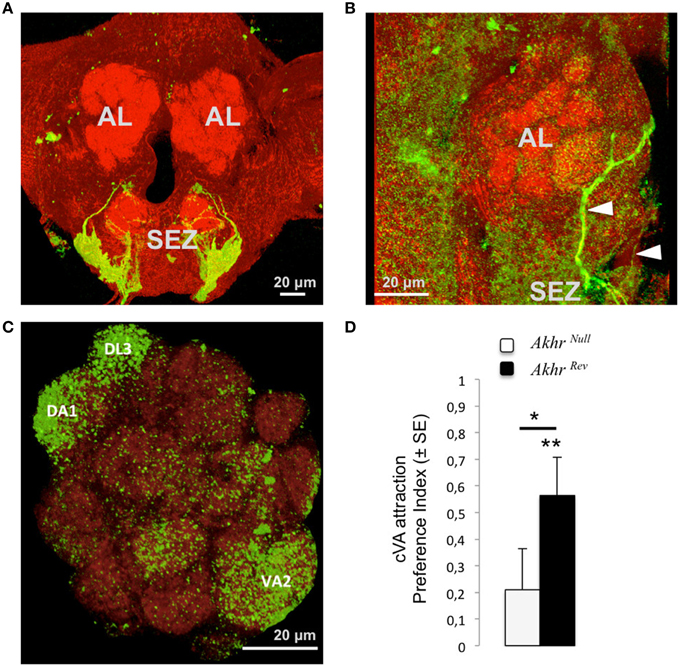
Figure 2. Effect of AKHR on pheromone perception. (A–C) Innervation pattern of AKHR-sensitive neurons (using AKHR-Gal4 to drive GFP expression, green) within a background of anti-synapsin staining (red). (A) Expression in the subesophageal zone (SEZ). (B) Arrowheads show the projections of the AKHR-expressing neurons from the SEZ to the antennal lobes (AL). (C) The glomeruli in which a consistent expression of AKHR was observed are annotated. (D) Attraction to cVA for Akhrnull and Akhrrev males. A positive preference index shows a preference for a blend of cVA and vinegar over vinegar alone. Asterisks show significant attraction to cVA (above bars) or significant differences between flies (between bars), *p < 0.05, **p < 0.01. Scale bar in each confocal image represents 20 μm.
Akhrnull males were not attracted to cVA (V = 289.5, p = 0.23), whereas Akhrrev males were (Figure 2D; V = 374.5, p = 0.002). The attraction index of Akhrnull males was also significantly reduced compared to that of the control Akhrrev males (W = 316, p = 0.031).
AKHR Modulates Female Pheromone Production Depending on Their Starvation State
The total amount of CHCs was not affected by the akhr mutation (starved males: W = 20, p = 0.15; fed males: W = 16, p = 0.55; starved females: W = 5, p = 0.15; fed females: W = 19, p = 0.22). Starved male and female Akhrrev flies produced less CHCs than fed flies (males: W = 0, p = 0.008; females: W = 1, p = 0.016). In contrast, only a tendency was observed in starved mutant males (W = 3, p = 0.056), whereas starvation did not affect CHC production in mutant females (W = 8, p = 0.42).
We then looked more specifically at the main CHCs present on the male and female cuticle (respectively 7-T and 7,11-HD) as well as the male specific pheromone cVA. The amount of cVA and 7-T produced by males was not significantly affected by the lack of AKHR, neither when fed nor when starved (Figure 3). In contrast, in females, production of 7-T and 7,11-HD was affected by the mutation. This effect depended on the feeding status. Akhrnull females produced significantly more 7-T than Akhrrev females when starved (W = 0, p = 0.008) and significantly less 7,11-HD when fed (W = 23, p = 0.03) (Figure 3). In Akhrrev females, the amount of both 7-T and 7,11-HD decreases during starvation (7-T: W = 1, p = 0.016; 7,11-HD: W = 0, p = 0.008). Interestingly, this is not the case in Akhrnull females (7-T: W = 15, p = 0.69; 7,11-HD: W = 0.06).
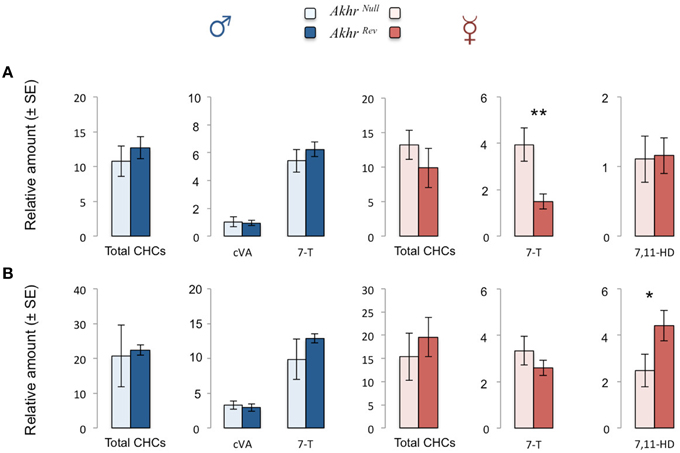
Figure 3. Effect of AKHR on CHC and cVA production in starved (A) and fed (B) flies. Relative amounts of total CHCs produced by Akhrnull and Akhrrev flies as well as cVA and 7-Tricosene (7-T) produced by males and 7-T and 7,11-Heptacosadiene (7,11-HD) produced by females. *p < 0.05, **p < 0.01.
Discussion
Animals modulate their behavior depending on their physiological state. For instance, food deprivation promotes food-searching behaviors over reproductive behaviors. Modulation generally relies on hormonal pathways that are activated in response to either food intake or starvation. Here we show that the receptor for AKH has an important modulatory effect on D. melanogaster sexual traits. AKH is known to regulate sugar and lipid homeostasis, food-searching (Lee and Park, 2004) and feeding behaviors (Bharucha et al., 2008) in response to starvation. Our results show that when starved, males lacking AKHR exhibit a dramatically reduced level of courtship. This suggests that, perhaps unexpectedly, AKH signaling during starvation does not decrease sexual activity, but instead is required to keep a reasonable level of courtship regardless of feeding status.
The reduced sexual activity in males deficient for AKHR can be, at least partly, due to a change in cVA sensing. Indeed, AKHR is expressed in neurons that send projections to only few glomeruli in the AL, the first olfactory processing center in the insect brain. Among these glomeruli, AKHR neurons project to DA1 and DL3, two glomeruli involved in cVA detection (Ejima et al., 2007; Schlief and Wilson, 2007; van der Goes van Naters and Carlson, 2007; Wang and Anderson, 2010; Liu et al., 2011). In addition, cVA attraction is known to depend on feeding status and insulin-like peptides, that are known to interact with the AKH signaling pathway, partly mediate this modulation (Lebreton et al., 2015). Here we show that also AKH signaling appears important for flies in cVA attraction, since AKHR mutant males showed a significantly reduced attraction. cVA is transferred to females during mating and is used by males to recognize already mated females (Zawistowski and Richmond, 1986; Everaerts et al., 2010). Maintaining sensitive to cVA is therefore beneficial for males regarding both mate finding and sexual selection. As feeding co-occurs spatially with meeting and mating, cVA could also be used as a proxy for feeding sites and as such modulated by AKH. Finally, the reduced courtship activity of Akhrnull mutants, suggests that AKH could act at several levels to promote mating behavior. Previous studies showed that the DA1 glomerulus and its corresponding receptor Or67d mediate direct response to exposure to cVA (Schlief and Wilson, 2007; Wang and Anderson, 2010), whereas DL3 and the Or65a receptor seem to modulate cVA response over a longer period through experience (Zawistowski and Richmond, 1986; Everaerts et al., 2010; Liu et al., 2011; Lebreton et al., 2014; Dekker et al., 2015). AKHR is expressed in neurons with cell bodies in the SEZ. Ascending axons project to both DA1 and DL3, but it remains unknown what type of interactions occur at the synapse and how this modulates the response to cVA.
Whereas AKH modulates male attraction to cVA through modulation of DA1 and DL3 output by AKHR neurons, suppression of male courtship is unlikely mediated through this route, as virgin females do not carry cVA (Everaerts et al., 2010). However, AKHR expressing neurons also projects to other glomeruli, most notably to VA2. VA2 appeared, although sensitive to food odors, also involved in courtship behavior. Sphinx, a lineage-specific non-coding RNA gene that is involved in male courtship behavior (Dai et al., 2008), is specifically expressed in this glomerulus (Chen et al., 2011). This suggests that VA2 may also regulate male sexual behaviors. The effect of AKHR on male courtship we presented in this study can therefore also be due to the effect of AKH on VA2 signaling. Bharucha et al. (2008) demonstrated a very strong expression of AKHR in the SEZ (see also Figure 2A), which receives input from gustatory neurons responsible for food and contact pheromones perception. However, expression was restricted to neurons that are involved in sugar, but not pheromone, sensing, which may make the SEZ an unlikely target of AKHR-mediated regulation of sexual behaviors.
AKHR does not only regulate pheromone perception, but also pheromone production. In general, fed flies produce more CHCs than starved flies. However, mutant females no longer showed feeding status dependent CHC production, which could be a secondary effect of the lack of AKHR on lipid metabolism. In addition, production of specific CHCs appeared to be affected: starved AKHR mutant females carried larger amount of the anti-aphrodisiac pheromone 7-T compared to control flies, and smaller amount of the courtship promoting pheromone 7,11-HD when fed. This effect was sex-specific: no effect was found in males, neither for cVA nor for 7-T. It would be interesting to test whether the AKH signaling regulates the production of other pheromonal compounds such as 9-tricosene, 7-pentacosene, 7,11-non-acosadiene or CH503 (Antony et al., 1985; Ferveur and Sureau, 1996; Yew et al., 2009; Lin et al., 2015). We expected that less 7,11-HD (aphrodisiac), but more 7-T (anti-aphrodisiac) would reduce the attractiveness of females lacking AKHR. However, in our assays wild-type males exhibit similar behaviors toward mutant and control females. As our experiments were done in a no-choice condition with “one male/one female” tests, the difference in the pheromone profile between mutant and control females can still play a role in sexual selection when males have the choice between several receptive females. AKH acts with other hormones, mainly insulin-like peptides, in adjusting sugar and lipid metabolism in response to the nutritional state (Buch et al., 2008). Interestingly, it has recently been shown that the insulin-signaling pathway influences female cuticular hydrocarbon expression and therefore attractiveness (Fedina et al., 2012; Kuo et al., 2012). Insulin also regulates female sexual receptivity by modulating remating rate, but it had no effect on the receptivity of virgin females (Wigby et al., 2011). We did not find any effect of the AKH pathway on the receptivity of virgin females. It would be interesting to test if, similar to insulin, AKH can affect the receptivity of mated females.
We showed multiple effects of the AKH hormonal pathway (AKHR) on both pheromone perception and pheromone production. It has recently been reported that the gene, desat1, which codes for a desaturase, is involved in both the biosynthesis of unsaturated CHCs (like 7-T and 7,11-HD) and pheromone perception (Bousquet et al., 2012). Desat1 is expressed in, among other structures, oenocytes (production site of cuticular hydrocarbon, Billeter et al., 2009), the fat body (FB) and the sensory neurons that project to the DA1 glomerulus in the AL (Bousquet et al., 2012). AKH acts on FB to mobilize lipid resources (Lee and Park, 2004), but the precise function of desat1 in the FB and the AL is not known. An interesting future study could investigate whether AKH, through the activation of its receptor AKHR, could affect the activity or expression of desat1, and in turn modulate pheromone perception and production. In addition, the role of AKH in modulating food odor sensitivity deserves attention. We have not in detail looked at the response of mutant flies to food odors, although both AKHRnull and AKHRrev flies were attracted to these. However, since VA2 and a number of other glomeruli received input from AKHR sensitive neurons, we postulate AKH to modulate food odor sensitivity as well.
Conclusion
Here we report on effects of AKHR on D. melanogaster sexual behavior by regulating both pheromone perception and production. The effects of AKHR are sex-specific (males being affected in their sexual activity and pheromone perception and females in their pheromone production) and depend on the fly's nutritional status. Further studies are needed to understand the complexity of the mode of action of the AKH pathway on Drosophila sexual behavior and its possible interactions with insulin and desat1. This is nevertheless, to our knowledge, the first evidence that the AKH pathway is involved in the regulation of sexual behavior in insects. In line with previous results on mammals (Kauffman and Rissman, 2004) and nematodes (Lindemans et al., 2009), it seems that the AKH/GnRH pathway is conserved across taxa to match reproductive functions with food availability.
Author Contributions
SL and JB performed the behavioral experiments. TD, SM, and JB performed the immunostaining. SL performed, and JB contributed to, the chemical analysis. SL and TD analyzed the data and wrote the manuscript.
Conflict of Interest Statement
The authors declare that the research was conducted in the absence of any commercial or financial relationships that could be construed as a potential conflict of interest.
Acknowledgments
We would like to thank Kamal Bharucha for providing the AKHR fly lines and Thomas Chertemps for providing the GFP line. This work was supported by the Linnaeus Grant from the Swedish Research Council for Environment, Agricultural Sciences and Spatial Planning (FORMAS) to the unit of Chemical Ecology. SL was supported by Carl Tryggers Stiftelse för Vetenskaplig Forskning.
References
Antony, C., Davis, T. L., Carlson, D. A., Pechine, J.-M., and Jallon, J.-M. (1985). Compared behavioral responses of male Drosophila melanogaster (Canton-S) to natural and synthetic aphrodisiacs. J. Chem. Ecol. 11, 1617–1629. doi: 10.1007/BF01012116
Baker, K. D., and Thummel, C. S. (2007). Diabetic larvae and obese flies-emerging studies of metabolism in Drosophila. Cell Metab. 6, 257–266. doi: 10.1016/j.cmet.2007.09.002
Bartelt, R. J., Schaner, A. M., and Jackson, L. L. (1985). cis-vaccenyl acetate as an aggregation pheromone in Drosophila melanogaster. J. Chem. Ecol. 11, 1747–1756. doi: 10.1007/BF01012124
Bharucha, K. N., Tarr, P., and Zipursky, S. L. (2008). A glucagon-like endocrine pathway in Drosophila modulates both lipid and carbohydrate homeostasis. J. Exp. Biol. 211, 3103–3110. doi: 10.1242/jeb.016451
Billeter, J.-C., Atallah, J., Krupp, J. J., Millar, J. G., and Levine, J. D. (2009). Specialized cells tag sexual and species identity in Drosophila melanogaster. Nature 461, 987–991. doi: 10.1038/nature08495
Bousquet, F., Nojima, T., Houot, B., Chauvel, I., Chaudy, S., Dupas, S., et al. (2012). Expression of a desaturase gene, desat1, in neural and nonneural tissues separately affects perception and emission of sex pheromones in Drosophila. Proc. Natl. Acad. Sci. U.S.A. 109, 249–254. doi: 10.1073/pnas.1109166108
Buch, S., Melcher, C., Bauer, M., Katzenberger, J., and Pankratz, M. J. (2008). Opposing effects of dietary protein and sugar regulate a transcriptional target of Drosophila insulin-like peptide signaling. Cell Metab. 7, 321–332. doi: 10.1016/j.cmet.2008.02.012
Chen, Y., Dai, H., Chen, S., Zhang, L., and Long, M. (2011). Highly tissue specific expression of Sphinx supports its male courtship related role in Drosophila melanogaster. PLoS ONE 6:e18853. doi: 10.1371/journal.pone.0018853
Dai, H., Chen, Y., Chen, S., Mao, Q., Kennedy, D., Landback, P., et al. (2008). The evolution of courtship behaviors through the origination of a new gene in Drosophila. Proc. Natl. Acad. Sci. U.S.A. 105, 7478–7483. doi: 10.1073/pnas.0800693105
Dekker, T., Revadi, S., Mansourian, S., Ramasamy, S., Lebreton, S., Becher, P. G., et al. (2015). Loss of Drosophila pheromone reverses its role in sexual communication in Drosophila suzukii. Proc. Biol. Sci. 282:20143018. doi: 10.1098/rspb.2014.3018
Ejima, A., Smith, B. P. C., Lucas, C., van der Goes van Naters, W., Miller, C. J., Carlson, J. R., et al. (2007). Generalization of courtship learning in Drosophila is mediated by cis-vaccenyl acetate. Curr. Biol. 17, 599–605. doi: 10.1016/j.cub.2007.01.053
Everaerts, C., Farine, J.-P., Cobb, M., and Ferveur, J.-F. (2010). Drosophila cuticular hydrocarbons revisited: mating status alters cuticular profiles. PLoS ONE 5:e9607. doi: 10.1371/journal.pone.0009607
Fedina, T. Y., Kuo, T.-H., Dreisewerd, K., Dierick, H. A., Yew, J. Y., and Pletcher, S. D. (2012). Dietary effects on cuticular hydrocarbons and sexual attractiveness in Drosophila. PLoS ONE 7:e49799. doi: 10.1371/journal.pone.0049799
Ferveur, J.-F. (2005). Cuticular hydrocarbons: their evolution and roles in Drosophila pheromonal communication. Behav. Genet. 35, 279–295. doi: 10.1007/s10519-005-3220-5
Ferveur, J.-F., and Sureau, G. (1996). Simultaneous influence on male courtship of stimulatory and inhibitory pheromones produced by live sex-mosaic Drosophila melanogaster. Proc. R. Soc. B Biol. Sci. 263, 967–973. doi: 10.1098/rspb.1996.0143
Franssen, C. M., Tobler, M., Riesch, R., García de León, F. J., Tiedemann, R., Schlupp, I., et al. (2008). Sperm production in an extremophile fish, the cave molly (Poecilia mexicana, Poeciliidae, Teleostei). Aquat. Ecol. 17, 685–692. doi: 10.1007/s10452-007-9128-9
Ito, K., Shinomiya, K., Ito, M., Armstrong, J. D., Boyan, G., Hartenstein, V., et al. (2014). A systematic nomenclature for the insect brain. Neuron 81, 755–765. doi: 10.1016/j.neuron.2013.12.017
Kauffman, A. S., Bojkowska, K., Wills, A., and Rissman, E. F. (2006). Gonadotropin-releasing hormone-II messenger ribonucleic acid and protein content in the mammalian brain are modulated by food intake. Endocrinology 147, 5069–5077. doi: 10.1210/en.2006-0615
Kauffman, A. S., and Rissman, E. F. (2004). A critical role for the evolutionarily conserved gonadotropin-releasing hormone II: mediation of energy status and female sexual behavior. Endocrinology 145, 3639–3646. doi: 10.1210/en.2004-0148
Kodrík, D., Vinokurov, K., Tomčala, A., and Socha, R. (2012). The effect of adipokinetic hormone on midgut characteristics in Pyrrhocoris apterus L. (Heteroptera). J. Insect Physiol. 58, 194–204. doi: 10.1016/j.jinsphys.2011.11.010
Kuo, T.-H., Fedina, T. Y., Hansen, I., Dreisewerd, K., Dierick, H. A., Yew, J. Y., et al. (2012). Insulin signaling mediates sexual attractiveness in Drosophila. PLoS Genet. 8:e1002684. doi: 10.1371/journal.pgen.1002684
Lebreton, S., Grabe, V., Omondi, A. B., Ignell, R., Becher, P. G., Hansson, B. S., et al. (2014). Love makes smell blind: mating suppresses pheromone attraction in Drosophila females via Or65a olfactory neurons. Sci. Rep. 4, 7119. doi: 10.1038/srep07119
Lebreton, S., Trona, F., Borrero-Echeverry, F., Bilz, F., Grabe, V., Becher, P. G., et al. (2015). Feeding regulates sex pheromone attraction and courtship in Drosophila females. Sci. Rep. 5, 13132. doi: 10.1038/srep13132
Lee, G., and Park, J. H. (2004). Hemolymph sugar homeostasis and starvation-induced hyperactivity affected by genetic manipulations of the adipokinetic hormone-encoding gene in Drosophila melanogaster. Genetics 167, 311–323. doi: 10.1534/genetics.167.1.311
Lin, C.-C., Prokop-Prigge, K. A., Preti, G., and Potter, C. J. (2015). Food odors trigger Drosophila males to deposit a pheromone that guides aggregation and female oviposition decisions. Elife 4:e08688. doi: 10.7554/eLife.08688
Lindemans, M., Liu, F., Janssen, T., Husson, S. J., Mertens, I., Gäde, G., et al. (2009). Adipokinetic hormone signaling through the gonadotropin-releasing hormone receptor modulates egg-laying in Caenorhabditis elegans. Proc. Natl. Acad. Sci. U.S.A. 106, 1642–1647. doi: 10.1073/pnas.0809881106
Liu, W., Liang, X., Gong, J., Yang, Z., Zhang, Y.-H., Zhang, J.-X., et al. (2011). Social regulation of aggression by pheromonal activation of Or65a olfactory neurons in Drosophila. Nat. Neurosci. 14, 896–902. doi: 10.1038/nn.2836
Liu, Y., Liu, H., Liu, S., Wang, S., Jiang, R.-J., and Li, S. (2009). Hormonal and nutritional regulation of insect fat body development and function. Arch. Insect Biochem. 71, 16–30. doi: 10.1002/arch.20290
Martin, B., Golden, E., Carlson, O. D., Egan, J. M., Mattson, M. P., and Maudsley, S. (2008). Caloric restriction: impact upon pituitary function and reproduction. Ageing Res. Rev. 7, 209–224. doi: 10.1016/j.arr.2008.01.002
Moskalik, B., and Uetz, G. W. (2011). Female hunger state affects mate choice of a sexually selected trait in a wolf spider. Anim. Behav. 81, 715–722. doi: 10.1016/j.anbehav.2010.12.016
Pavlou, H. J., and Goodwin, S. F. (2013). Courtship behavior in Drosophila melanogaster: towards a “courtship connectome.” Curr. Opin. Neurobiol. 23, 76–83. doi: 10.1016/j.conb.2012.09.002
Pho, D. B., Pennanec'h, M., and Jallon, J. M. (1998). Purification of adult Drosophila melanogaster lipophorin and its role in hydrocarbon transport. Arch. Insect Biochem. Physiol. 3, 289–303.
Ruebenbauer, A., Schlyter, F., Hansson, B. S., Löfstedt, C., and Larsson, M. C. (2008). Genetic variability and robustness of host odor preference in Drosophila melanogaster. Curr. Biol. 18, 1438–1443. doi: 10.1016/j.cub.2008.08.062
Schal, C., Sevala, V., Capurro, M. L., Snyder, T. E., Blomquist, G. J., and Bagnères, A. G. (2001). Tissue distribution and lipophorin transport of hydrocarbons and sex pheromones in the house fly, Musca domestica. J. Insect Sci. 1:12. doi: 10.1673/031.001.1201
Schlief, M. L., and Wilson, R. I. (2007). Olfactory processing and behavior downstream from highly selective receptor neurons. Nat. Neurosci. 10, 623–630. doi: 10.1038/nn1881
Shankar, S., Chua, J. Y., Tan, K. J., Calvert, M. E. K., Weng, R., Ng, W. C., et al. (2015). The neuropeptide tachykinin is essential for pheromone detection in a gustatory neural circuit. Elife 4:e06914. doi: 10.7554/eLife.06914
Sharon, G., Segal, D., Ringo, J. M., Hefetz, A., Zilber-Rosenberg, I., and Rosenberg, E. (2010). Commensal bacteria play a role in mating preference of Drosophila melanogaster. Proc. Natl. Acad. Sci. U.S.A. 107, 20051–20056. doi: 10.1073/pnas.1009906107
Sokolowski, M. B. (2001). Drosophila: genetics meets behaviour. Nat. Rev. Genet. 2, 879–890. doi: 10.1038/35098592
van der Goes van Naters, W., and Carlson, J. R. (2007). Receptors and neurons for fly odors in Drosophila. Curr. Biol. 17, 606–612. doi: 10.1016/j.cub.2007.02.043
Wang, L., and Anderson, D. J. (2010). Identification of an aggression-promoting pheromone and its receptor neurons in Drosophila. Nature 463, 227–231. doi: 10.1038/nature08678
Wigby, S., Slack, C., Grönke, S., Martinez, P., Calboli, F. C. F., Chapman, T., et al. (2011). Insulin signalling regulates remating in female Drosophila. Proc. R. Soc. B Biol. Sci. 278, 424–431. doi: 10.1098/rspb.2010.1390
Yew, J. Y., Dreisewerd, K., Luftmann, H., Müthing, J., Pohlentz, G., and Kravitz, E. A. (2009). A new male sex pheromone and novel cuticular cues for chemical communication in Drosophila. Curr. Biol. 19, 1245–1254. doi: 10.1016/j.cub.2009.06.037
Keywords: adipokinetic hormone, courtship, pheromones, cis-vaccenyl acetate, nutritional status, sexual dimorphism
Citation: Lebreton S, Mansourian S, Bigarreau J and Dekker T (2016) The Adipokinetic Hormone Receptor Modulates Sexual Behavior, Pheromone Perception and Pheromone Production in a Sex-Specific and Starvation-Dependent Manner in Drosophila melanogaster. Front. Ecol. Evol. 3:151. doi: 10.3389/fevo.2015.00151
Received: 26 September 2015; Accepted: 14 December 2015;
Published: 06 January 2016.
Edited by:
Stefano Colazza, University of Palermo, ItalyReviewed by:
Freddie-Jeanne Richard, Université de Poitiers, FranceJoanne Yew, University of Hawaii at Manoa, USA
Copyright © 2016 Lebreton, Mansourian, Bigarreau and Dekker. This is an open-access article distributed under the terms of the Creative Commons Attribution License (CC BY). The use, distribution or reproduction in other forums is permitted, provided the original author(s) or licensor are credited and that the original publication in this journal is cited, in accordance with accepted academic practice. No use, distribution or reproduction is permitted which does not comply with these terms.
*Correspondence: Sébastien Lebreton, c2ViYXN0aWVuLmxlYnJldG9uQHVuaXYtYW11LmZy
†Present Address: Sébastien Lebreton, Aix-Marseille Université, Centre National de la Recherche Scientifique, UMR7288, Institut de Biologie du Développement de Marseille, Marseille, France