- School of Life Sciences, Arizona State University, Tempe, AZ, USA
Though many animal ornaments and signals are sensitive to and encode information about the oxidative balance (OB) of individuals (e.g., antioxidant supplies/activity, reactive oxygen species, cellular oxidative damage/repair), often the environmental and/or physiological sources of such OB are unknown. Urban development is among the most recent, pervasive, and persistent human stressors on the planet and impacts many environmental and physiological parameters of animals. Here we review the mechanistic underpinnings and functional consequences of how human urbanization drives antioxidant/oxidative status in animals and how this affects signal expression and use. Although we find that urbanization has strong negative effects on signal quality (e.g., visual, auditory, chemical) and OB across a range of taxa, few urban ecophysiological studies address signals and oxidative stress in unison, and even fewer in a fitness context. We also highlight particular signal types, taxa, life-histories, and anthropogenic environmental modifications on which future work integrating OB, signals, and urbanization could be centered. Last, we examine the conceptual and empirical framework behind the idea that urban conditions may disentangle signal expression from honesty and affect plasticity and adaptedness of sexually selected traits and preferences in the city.
General Background
Many conspicuous traits in animals, such as songs, odorants, and bright colors, can reveal the quality of individuals and are used as signals in competition for mates or other resources (Andersson, 1994; Bradbury and Vehrencamp, 2011). The fitness-related qualities that such condition-dependent signals reveal include disease resistance (Hamilton and Zuk, 1982; Blount et al., 2003), nutrition (Walker et al., 2013), parental effort (Badyaev and Hill, 2002; Massaro et al., 2003), and oxidative stress (OS; i.e., accumulation of free-radical damage, including lipid peroxidation, protein carbonylation, and/or genotoxic damage). Recent empirical work suggests that oxidative balance (OB; i.e., balance between free-radical molecules and antioxidant defenses; Tomášek et al., 2016) integrates many aspects of individual quality/condition (e.g., disease, diet, stress) and can be an important modulator of signal production (von Schantz et al., 1999; Garratt and Brooks, 2012; Henschen et al., 2015). However, we need a deeper understanding of the extent to which oxidative challenges affect different organisms with different signals, environments, genetics, and life-histories.
A number of natural environmental factors, including heat stress and population density (Costantini, 2010; Costantini et al., 2010), are known to generate oxidative imbalance in organisms, via the accumulation of damaging pro-oxidant byproducts of cellular respiration, disruptions to the activity of endogenous antioxidants (e.g., melatonin, enzymes such as superoxide dismutase), or depletion/availability of exogenous dietary antioxidant supplies (e.g., vitamin E, carotenoids). Only recently have biologists begun to address the effects of more large-scale human-induced rapid environmental changes, such as urbanization, on OB and animal signals (Isaksson, 2015). Many local ecological parameters can be altered by human activities in cities and may disproportionately depress or elevate OB and environmentally sensitive sexually selected signals (Hill, 1995). Indeed, experimental research has demonstrated that many urban-associated environmental factors can cause either a reduction in antioxidant availability/expression, an increase in pro-oxidant production, and/or an increase in OS (Isaksson, 2015). Chemical contaminants (Isaksson, 2010), light pollution (Navara and Nelson, 2007), noise pollution (Demirel et al., 2009), altered diet (Isaksson and Andersson, 2007; Andersson et al., 2015), and changes in social interactions (Beaulieu et al., 2014) are candidate modulators of OB in urban animals. Alternatively, animals may experience fewer negative effects on OB in urban areas, perhaps due to increased resources or lower perceived predation threat (Janssens and Stoks, 2013). Thus, though urbanization may have positive or negative effects on OB (perhaps based on taxon-specific life-history traits, including current physiological condition or behavioral/physiological plasticity; see Figure 1A), we are currently unaware of the prevailing effects of urbanization on OB and signaling. Therefore, we need an overarching evaluation of the linkages between urban environmental pressures, OB, and the expression and evolution of animal signals.
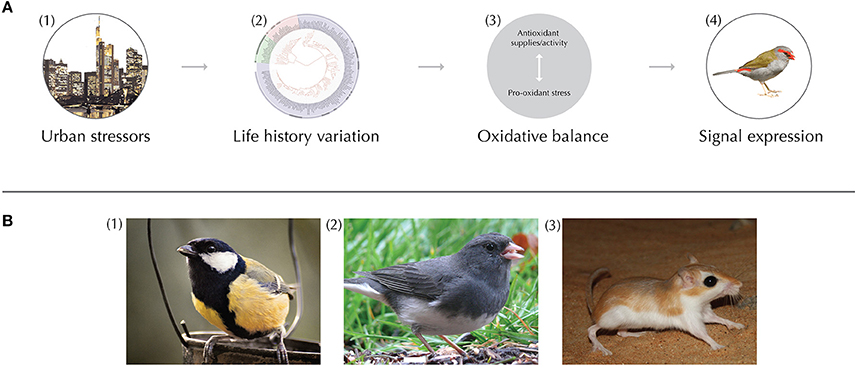
Figure 1. (A) Conceptual diagram depicting urban effects on oxidative balance (OB) and signal expression. (1) Urban environments can produce unique stressors for animals, such as chemical, noise and light pollution, and habitat and diet alteration. (2) Animals respond to urban stressors based on their unique life-histories, which include the physiological and behavioral sensitivity to stressors during particular phases of life (e.g., development, dispersal, breeding). Additionally, within life-history stages, current physiological condition and behavioral/physiological plasticity likely mediate susceptibility to changes in OB. (3) Ultimately, these ‘life-history filters’ govern whether and how OB shifts in urban animals. The relative abundance current antioxidants vs. pro-oxidants contributes to the accumulation of oxidative stress. (4) Therefore, the OB of animals can influence ornament expression and associated traits (e.g., survival, fecundity). (B) Study organisms that exemplify strong past empirical efforts or provide interesting routes for future research on OB and signaling in cities. (1) Oxidative stress, song, and carotenoid- and melanin-based color signals have been studied extensively in urban and rural great tits (Parus major). For example, tits have drabber yellow flanks and increase the frequency of song in the city, both of which are sexually selected traits (Hõrak et al., 2000; Halfwerk et al., 2011). Interestingly, selection on the width of the black breast tie is reversed in urban environments, suggesting this trait may have entered a dishonest signaling state (Senar et al., 2014). (2) Studies of the dark-eyed junco (Junco hyemalis) have revealed significant changes to many modalities of communication, such as tail shading (Yeh, 2004), preen oil composition (Whittaker et al., 2010), and song characteristics (Slabbekoorn et al., 2007). (3) Mammals such as gerbils (Family: Gerbillinae) provide interesting future routes to explore the effects of urbanization on OB and olfactory communication in this clade. The abundance of Indian gerbils (Tatera indica), for example (Prakesh et al., 1998), in both urban and rural environments make this organism a strong candidate for studies of urban chemical signaling and OB in mammals. All images obtained through Wikimedia Commons.
Here we review literature and understudied/untested ideas surrounding three critical questions related to animal signaling and OB in urban environments: (1) How might urbanization have physiological effects on OB and signal expression?; (2) Are different organisms or signal modalities more or less prone to urban-induced change in OB and signal quality?; and (3) How might urbanization shape the links between OB and signal honesty?
Overview of Literature Linking OB, Signals, and Urbanization
We surveyed the literature for studies on the relationship(s) between signal expression, OB, and/or urban conditions in animals. We found a total of 57 studies linking urbanization and signaling: consistent with the early hypothesis advanced by Hill (1995) that sexual ornaments act as indicators of environmental quality, the majority (70%) of published studies on this topic reveal that animals have reduced signal quality in urban settings (Tables 1A,B; Figure 1B). This suggests that urban environments contain pervasive pressures that are stressful to animals across a range of clades and signal types. Urbanization enhanced signal expression in only 14% of studies. Interestingly, 4 of the 6 studies on melanin-based ornaments in birds show increased signal expression in the city; it is noteworthy that expression of melanin ornaments is related to resistance of both disease (Jacquin et al., 2011) and OS (Henschen et al., 2015), which may be beneficial in the pro-disease (Giraudeau et al., 2014a) and pro-oxidant urban environment. Taken together, these studies indicate that urban environments have overall strong negative impacts on the expression of animal signals, though it is not often known if these effects are plastic or adaptive (McDonnell and Hahs, 2015).
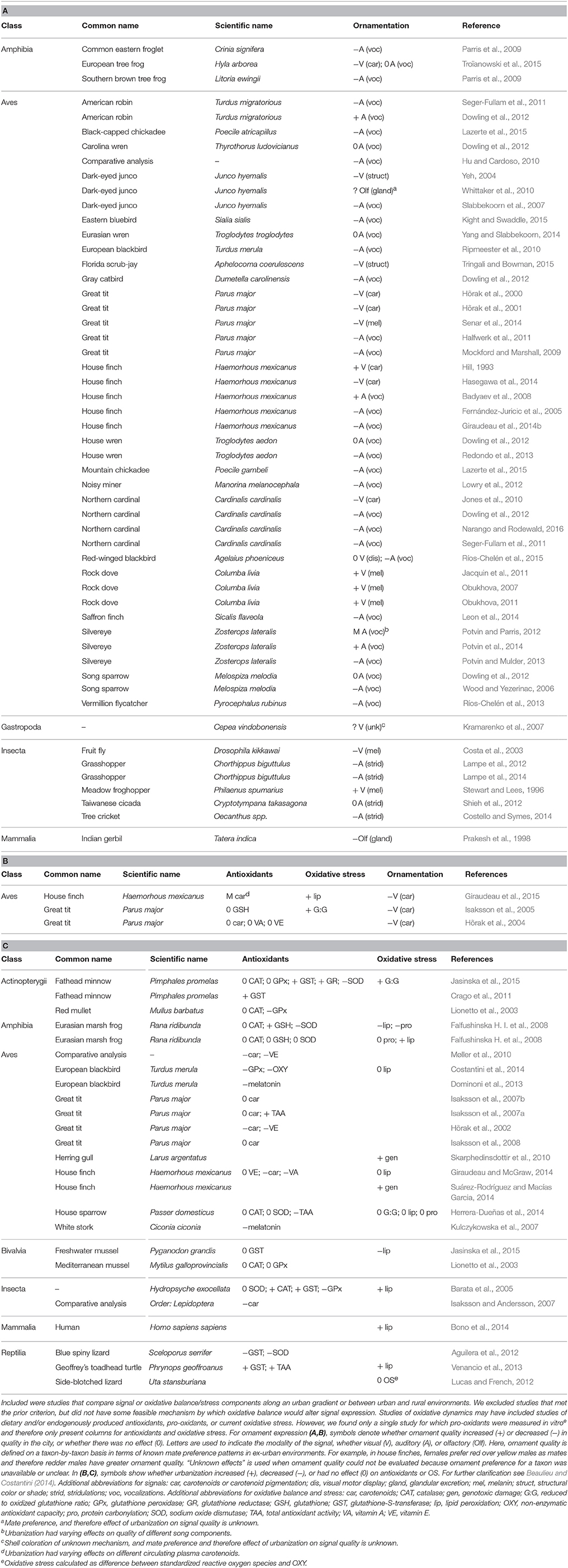
Table 1. Summary of studies that associate urbanization with (A) animal signals, (B) both animal signals and oxidative stress and/or antioxidants, and (C) just oxidative stress and/or antioxidants.
Of the nine studies that specifically tested for urban-rural differences in dietary antioxidants, 44% showed antioxidant reductions in urban compared to rural environments, and none showed an increase (Tables 1B,C). Urban antioxidant depletion may arise if dietary antioxidants are harder to procure in urban environments (Isaksson, 2009) and/or to maintain in the body as they are destroyed by elevated pro-oxidants (reviewed in Seifried et al., 2007). The fact that antioxidants were depleted in nearly half of the studies on urban animals is consistent with results of a large comparative study by Møller et al. (2010), who found that urban birds have lower levels of liver carotenoids and vitamin E than rural counterparts. We also found that endogenous antioxidant enzyme activity showed variable differences between urban and rural environments, as they both decreased and increased in a handful of studies (27 and 33% of total, respectively) (Tables 1B,C). The failure to find consistent urban-rural variation in antioxidants could reflect how organisms tend to up-regulate endogenous antioxidant activity after an oxidative challenge, but how prolonged and intense oxidative challenge may eventually overpower and deplete endogenous defenses (Finkel and Holbrook, 2000; Finkel, 2003). Alternatively, this pattern may reflect differences among studies in the timing of antioxidant measurement (e.g., due to seasonal or life-history-stage-related fluctuations; Barata et al., 2005; Falfushinska H. et al., 2008; Giraudeau and McGraw, 2014) or the use of different antioxidant tests (Beaulieu and Costantini, 2014). Therefore we stress that (1) more consistent tests of antioxidants should be conducted, all with strong biological rationale for the question(s) being asked, and (2) studies should combine measurements of past oxidative damage, current oxidative threats, as well as the actions, intake, and mobilized stores of various antioxidants, as it is difficult to interpret each independently (Beaulieu and Costantini, 2014; Costantini, 2016).
We located 15 published studies that investigated urban-rural differences in OS, 60% of which showed that urban environments have negative effects on OS (Tables 1B,C). These studies used a variety of OS metrics, and based on this evidence it appears that urbanization increases lipid peroxidation and genotoxic damage, but the only two studies on protein carbonylation failed to find similar trends. One exciting study shows that novel resource use can generate a trade-off between OS and parasite avoidance; although urban house finches (Haemorhous mexicanus) that line nests with cigarette butts experience reduced parasitism, they suffer increased genotoxic damage, presumably due to chemical contaminant exposure (Suárez-Rodríguez and Macías Garcia, 2014). In addition to the aforementioned evidence that cities disrupt signal quality, the fact that cities affect overall OS provides deeper evidence that urban environments alter environmental and physiological conditions that are key for the production and maintenance of quality signals. Still, there may be taxon- or life-history-specific effects of urbanization, given that not all studies found urban impacts on OS.
Surprisingly, we found only three studies (all on carotenoid color signals of birds) that simultaneously tested urban-rural differences in OB and signals (Hõrak et al., 2004; Isaksson et al., 2005; Giraudeau et al., 2015) (Table 1B). Both Isaksson et al. (2005) and Giraudeau et al. (2015) report drabber plumage coloration and increased levels of OS in Swedish great tits (Parus major) and house finches, respectively. In breeding Estonian great tits, Hõrak et al. (2004) found a strong trend that city birds are drabber in color, but no urban-rural difference in levels of dietary antioxidants. This limited dataset also suggests that OB is a potential critical constraint on signal production in the city. Taken altogether, studies testing urban effects on OB and signals both independently and simultaneously reveal the general pattern that urban animals experience both impaired OB and signal expression. However, we urge more comprehensive testing of this idea across animal clades and signaling modalities (see more below).
Signal Modalities and Taxonomic Perspectives
Urban environments are inhabited by a diversity of animals that have different communication modalities, life-history traits, developmental histories, and adaptabilities. Thus, cities may present unique oxidative and signaling challenges to specific taxa or ornament types. In other words, urban stressors ‘filter’ through taxon-specific life-history variation (e.g., metamorphosis, home-range size, current physiological state, behavioral/physiological plasticity) before ultimately influencing OB and signal quality (Figure 1A). Based on such differences in oxidative susceptibility, some animals may inherently rely more on either endogenous or exogenous antioxidants or suffer more oxidative damage. In the same vein, some ornament modalities may be more sensitive to cities and OB disruptions than others, and one hypothesis is that those signals that are both directly (e.g., song masking by noise; Gil et al., 2015) and indirectly (i.e., OS) affected by urban environmental conditions will be most disrupted.
Despite the potential ecological and evolutionary insights gained by studying urban effects on signaling and OB in diverse taxa, studies have overwhelmingly been performed on the ornaments and OB of birds (73% of total), with a notable paucity of studies on mammals, reptiles, fish, and invertebrates. Moreover, among the various animal-signaling modalities (e.g., visual, auditory, olfactory), studies of acoustic signals (68% of total) and colors (32% of total; some studies include multiple ornaments and therefore sum >100%) dominate the types of ornaments studied in relation to urbanization and OB to date (see Figure 1B for breakdown of strong past and future study systems). Only a handful of studies were performed on other modalities, such as motor displays (Ríos-Chelén et al., 2015) or olfactory (Whittaker et al., 2010) communication.
Studies of some signaling modalities have better considered the impacts of OB than others. As referenced above, studies of plumage coloration in birds like house finches and great tits have best wrapped together both signals and OB (Isaksson et al., 2007a; Giraudeau and McGraw, 2014), perhaps because of the natural links between carotenoid nutrition, OB, and plumage coloration (Blount, 2004; Svensson and Wong, 2011). Conversely, despite the large proportion of urban studies on auditory communication, the majority are considered in the context of short-term behavioral plasticity in noisy environments, few are in the context of individual quality, and none are from an OB perspective (Narango and Rodewald, 2016). Therefore, we stand to gain from reciprocally testing the current knowledge gaps for each modality: the behavioral/physiological plasticity of color ornaments (e.g., combined use in behavioral display, rapidly changeable bare parts) and how acoustic signals co-vary with urban oxidative conditions. For example, song learning and quality may be disrupted in the city, due to oxidative damage suffered to brain or vocal musculature during development (von Schantz et al., 1999; Nowicki et al., 2002; Buchanan et al., 2004) or adulthood (Garratt and Brooks, 2012).
Relatively little attention has been paid to other important signal modalities that may also be affected by cities and OB, such as chemical and electrical signals. The rare studies to date of this sort serve as exciting building-blocks; for example both male and female Indian gerbils (Tatera indica) are far less likely to express mature scent-marking glands in urban environments (Prakesh et al., 1998), perhaps due to increased gregariousness and reliance on close-quarters communication. The unique chemoscape of the city (e.g., chemical masking) may provide heretofore unconsidered selective pressures for animals that rely on chemical communication. Moreover, only in lab studies have links been made between OB and chemical signaling; Garratt et al. (2014) showed that both the mass of preputial glands and molecular composition of urine is depressed in knockout mice for superoxide dismutase, an endogenous antioxidant. We must now put this work in urban-ecological context and consider natural co-variation in OB and olfactory signals. Another understudied but exciting direction for future research is urban effects on OB and electrical signals of aquatic animals (Stoddard, 2002). Electrical signals may be anthropogenically altered via oxidative-stress pathways either through disruption of Ca2+ ionic homeostasis (van der Vliet and Bast, 1992) or man-made electro-magnetic fields (Consales et al., 2012). However, whether sexually selected electrical signals are sensitive to OB or electromagnetic pollution has yet to be tested in an ecological or urban context.
Future Directions: Broad Implications for Understanding Urban Evolution of Honest Signals
Honest communication requires that a signal provide fitness benefits to both sender and receiver (Maynard Smith and Harper, 2003). However, if environmental conditions change rapidly, then signals of mate quality may become uncoupled from individual quality and animals may choose low-quality mates as a result (Robinson et al., 2008; Bro-Jørgensen, 2010; Robertson et al., 2013). A classic example of this is the high rates of parasitism and mortality experienced by calling males (and females that prefer calling males) in newly introduced Polynesian field crickets (Teleogryllus oceanicus) in Hawaii (Zuk et al., 2006; Tinghitella and Zuk, 2009); this eventually led to the rapid loss of a sexually selected trait in this species. Rapid environmental alterations in cities may also perturb conditions, including via OB, in a way that leads to dishonest signaling systems.
To date, the majority of studies on signals in urban settings operate under the assumption that signals remain condition-dependent. To ask whether signal honesty persists or degrades in urban environments, we must know if and how ornament expression is related to condition of the signal sender (i.e., OB) or fitness in both rural and urban environments, how signal receivers base mate choice on ornaments, and their resulting fitness consequences. Thus, if signal honesty degrades in urban environments, we predict that (1) ornament expression will be positively related to condition only in natural/rural environments, and there will be either no relationship or a negative relationship in urban environments, and (2) signal receivers retain a preference for the exaggerated trait, a choice that (3) ultimately impairs fitness. The mechanisms that generate dishonesty may be diverse in nature. For example, Candolin (1999) showed that three-spined sticklebacks (Gasterosteus aculeatus) in extremely poor condition invested heavily into ornamentation, perhaps as a last-ditch effort to acquire a mate before dying; a similar scenario may occur given that urban environments generally have negative effects on OB. Alternatively, low-quality males may produce elaborate ornaments if they exploit urban-specific resources that provide surplus mate-choice currency (e.g., carotenoid-rich human-provided foods) but no real benefit to viability or ultimately do not reflect genetic quality.
The few published studies that address this question indeed point to dishonest signaling as a potential consequence of urbanization. Perhaps the best evidence comes from work on plumage coloration of Florida scrub-jays (Aphelocoma coerulescens). Prior to breeding, suburban and rural jays immigrate to the same location, allowing for pairing amongst jays of urban and ex-urban origin. Suburban jays had UV-shifted plumage relative to rural jays, and suburban jays were more likely to achieve breeder status, suggesting that UV-rich plumage is preferred (Tringali and Bowman, 2015). Importantly, reproductive success was lower per unit effort in nests of suburban than rural immigrants, thereby suggesting that suburban jays dishonestly exaggerated signals of quality. Senar et al. (2014) showed that rural great tits with large melanin-based ornaments (width of the ventral black tie) were more likely to survive, whereas large-tied great tits in urban settings were less likely to survive. Though this suggests that the condition-dependent expression of tie width is altered in the city, further work is necessary to test whether urban female great tits that choose males with large black ties experience reduced fitness, and if so, whether females adaptively (or plastically; Qvarnström et al., 2000) reverse mate selection for ornament size. Unlike in these previous two cases, urbanization may also prevent the formation of dishonest signaling. For example, Amur honeysuckle (Lonicera maackii) is an invasive nest- and food-plant for both urban and rural populations of Northern cardinal (Cardinalis cardinalis) and substantially increases plumage brightness of birds that nest in and forage from it, but amplifies nest predation, ultimately lowering reproductive output of females that choose “high-quality” males. However, only in rural, but not urban landscapes do redder males prefer to nest in honeysuckle (Rodewald et al., 2011). Therefore, in the city, females that choose red males tend to avoid amplified predation rates imposed by honeysuckle. Though these few examples highlight potential dishonest signaling systems, further work is clearly needed within these systems to meet the full criteria we outlined, and to expand the diversity of modalities and taxa studied.
We have previously established that few studies investigate the mechanistic role of OB for signal production in the city. One major utility of these and future studies will be for examining the relationship between individual quality, signal quality, and signal use in both urban and rural environments. To investigate the potential for dishonest signaling systems, future studies should examine the covariation between OB and signals at the time of both ornament production and ornament use (i.e., during mate choice). For example, in urban environments, ornaments may honestly reflect individual quality at the time of production, but become dishonest before/during mate selection if the costs of bearing high-quality ornaments are disproportionately high in the city. These studies perhaps make best sense in the framework of relatively non-labile signals (e.g., dead integumentary ornaments, skeletal armaments like horns/antlers), whereas rapidly changeable signals may be less prone to dishonesty (e.g., song, bare part coloration, odorants; Hutton et al., 2015). Long-term studies of ornamentation, OB, and mate choice on species that advertise dishonestly in the city will be extremely productive for understanding urban plasticity and evolution of mate choice tactics. Additionally, species that bear multiple signals may flexibly choose mates based on those signal components/modalities that retain their reliability in the city (Troïanowski et al., 2015).
Finally, the most robust urban-ecological studies on OB and signal expression/honesty will consider the variability and heterogeneity of the cities themselves (i.e., “not all cities and their oxidative stressors are created equal”). Urban stressors and selective pressures may differ in type, timing, and intensity across diverse urban-rural landscape gradients (e.g., desert city, forest city) and within individual cities based on spatiotemporal changes in development and infrastructure, and therefore we will benefit greatly from increasingly global perspectives. Ultimately, urban environments serve as excellent, long-term and continuing “natural experiments” for understanding basic ecological, physiological, and evolutionary mechanisms underlying signal honesty, and the evolution and plasticity of mate preferences as a function of signal honesty/dishonesty (Hahs and Evans, 2015).
Author Contributions
Both PH and KJM drafted and intellectually contributed to the work and approved it for publication.
Funding
This material is based upon work supported by the National Science Foundation under grant number BCS-1026865, Central Arizona-Phoenix Long-Term Ecological Research (CAP LTER).
Conflict of Interest Statement
The authors declare that the research was conducted in the absence of any commercial or financial relationships that could be construed as a potential conflict of interest.
Acknowledgments
We thank Mathieu Giraudeau for helpful conversations during the early conception of this piece. Additionally, we thank David Costantini and Fabrice Helfenstein for organizing the “oxidative stress and signal honesty” research topic. Lastly, we thank Keila DeZeeuw for assistance and thoughts on figure construction.
References
Aguilera, C., del Pliego, P. G., Alfaro, R. M., Lazcano, D., and Cruz, J. (2012). Pollution biomarkers in the spiny lizard (Sceloporus spp.) from two suburban populations of Monterrey, Mexico. Ecotoxicology 21, 2103–2112. doi: 10.1007/s10646-012-0978-0
Andersson, M. N., Wang, H., Nord, A., Salmón, P., and Isaksson, C. (2015). Composition of physiologically important fatty acids in great tits differs between urban and rural populations on a seasonal basis. Front. Ecol. Evol. 3:93. doi: 10.3389/fevo.2015.00093
Badyaev, A. V., and Hill, G. E. (2002). Paternal care as a conditional strategy: distinct reproductive tactics associated with elaboration of plumage ornamentation in the house finch. Behav. Ecol. 13, 591–597. doi: 10.1093/beheco/13.5.591
Badyaev, A. V., Young, R. L., Oh, K. P., and Addison, C. (2008). Evolution on a local scale: developmental, functional, and genetic bases of divergence in bill form and associated changes in song structure between adjacent habitats. Evolution 62, 1951–1964. doi: 10.1111/j.l558-5646.2008.00428.x
Barata, C., Lekumberri, I., Vila-Escalé, M., Prat, N., and Porte, C. (2005). Trace metal concentration, antioxidant enzyme activities and susceptibility to oxidative stress in the tricoptera larvae Hydropsyche exocellata from the Llobregat river basin (NE Spain). Aquat. Toxicol. 74, 3–19. doi: 10.1016/j.aquatox.2005.04.002
Beaulieu, M., and Costantini, D. (2014). Biomarkers of oxidative status: missing tools in conservation physiology. Conserv. Physiol. 2, 1–16. doi: 10.1093/conphys/cou014
Beaulieu, M., Mboumba, S., Willaume, E., Kappeler, P. M., and Charpentier, M. J. E. (2014). The oxidative cost of unstable social dominance. J. Exp. Biol. 217, 2629–2632. doi: 10.1242/jeb.104851
Blount, J. D. (2004). Carotenoids and life-history evolution in animals. Arch. Biochem. Biophys. 430, 10–15. doi: 10.1016/j.abb.2004.03.039
Blount, J. D., Metcalfe, N. B., Birkhead, T. R., and Surai, P. F. (2003). Carotenoid modulation of immune function and sexual attractiveness in zebra finches. Science 300, 125–127. doi: 10.1126/science.1082142
Bono, R., Bellisario, V., Romanazzi, V., Pirro, V., Piccioni, P., Pazzi, M., et al. (2014). Oxidative stress in adolescent passive smokers living in urban and rural environments. Int. J. Hyg. Environ. Health 217, 287–293. doi: 10.1016/j.ijheh.2013.06.008
Bradbury, J., and Vehrencamp, S. (2011). Principles of Animal Communication, 2nd Edn. Sunderland, MA: Sinauer Associates Inc.
Bro-Jørgensen, J. (2010). Dynamics of multiple signalling systems: animal communication in a world in flux. Trends Ecol. Evol. 25, 292–300. doi: 10.1016/j.tree.2009.11.003
Buchanan, K. L., Leitner, S., Spencer, K. A., Goldsmith, A. R., and Catchpole, C. K. (2004). Developmental stress selectively affects the song control nucleus HVC in the zebra finch. Proc. R. Soc. B 271, 2381–2386. doi: 10.1098/rspb.2004.2874
Candolin, U. (1999). The relationship between signal quality and physical condition: is sexual signalling honest in the three-spined stickleback? Anim. Behav. 58, 1261–1267. doi: 10.1006/anbe.1999.1259
Consales, C., Merla, C., Marino, C., and Benassi, B. (2012). Electromagnetic fields, oxidative stress, and neurodegeneration. Int. J. Cell Biol. 2012, 1–16. doi: 10.1155/2012/683897
Costa, B., Rohde, C., and Valente, V. (2003). Temperature, urbanization and body color polymorphism in South Brazilian populations of Drosophila kikkawai (Diptera, Drosophilidae). Iheringia Ser. Zool. 93, 381–393. doi: 10.1590/S0073-47212003000400005
Costantini, D. (2010). Redox physiology in animal function: the struggle of living in an oxidant environment. Curr. Zool. 56, 687–702. doi: 10.1111/j.1365-2435.2010.01746.x
Costantini, D. (2016). Oxidative stress ecology and the d-ROMs test: facts, misfacts and an appraisal of a decade's work. Behav. Ecol. Sociobiol. 70, 809–820. doi: 10.1007/s00265-016-2091-5
Costantini, D., Greives, T. J., Hau, M., and Partecke, J. (2014). Does urban life change blood oxidative status in birds? J. Exp. Biol. 217, 2994–2997. doi: 10.1242/jeb.106450
Costantini, D., Rowe, M., Butler, M. W., and McGraw, K. J. (2010). From molecules to living systems: historical and contemporary issues in oxidative stress and antioxidant ecology. Funct. Ecol. 24, 950–959. doi: 10.1111/j.1365-2435.2010.01746.x
Costello, R. A., and Symes, L. B. (2014). Effects of anthropogenic noise on male signalling behaviour and female phonotaxis in Oecanthus tree crickets. Anim. Behav. 95, 15–22. doi: 10.1016/j.anbehav.2014.05.009
Crago, J., Corsi, S. R., Weber, D., Bannerman, R., and Klaper, R. (2011). Linking biomarkers to reproductive success of caged fathead minnows in streams with increasing urbanization. Chemosphere 82, 1669–1674. doi: 10.1016/j.chemosphere.2010.11.011
Demirel, R., Akkaya, M., and Uygur, R. (2009). Noise induces oxidative stress in rat. Eur. J. Gen. Med. 6, 20–24.
Dominoni, D. M., Goymann, W., Helm, B., and Partecke, J. (2013). Urban-like night illumination reduces melatonin release in European blackbirds (Turdus merula): implications of city life for biological time-keeping of songbirds. Front. Zool. 10:60. doi: 10.1186/1742-9994-10-60
Dowling, J. L., Luther, D. A., and Marra, P. P. (2012). Comparative effects of urban development and anthropogenic noise on bird songs. Behav. Ecol. 23, 201–209. doi: 10.1093/beheco/arr176
Falfushinska, H., Loumbourdis, N., Romanchuk, L., and Stolyar, O. (2008). Validation of oxidative stress responses in two populations of frogs from Western Ukraine. Chemosphere 73, 1096–1101. doi: 10.1016/j.chemosphere.2008.07.060
Falfushinska, H. I., Romanchuk, L. D., and Stolyar, O. B. (2008). Different responses of biochemical markers in frogs (Rana ridibunda) from urban and rural wetlands to the effect of carbamate fungicide. Comp. Biochem. Physiol. C 148, 223–229. doi: 10.1016/j.cbpc.2008.05.018
Fernández-Juricic, E., Poston, R., De Collibus, K., Morgan, T., Bastain, B., Martin, C., et al. (2005). Microhabitat selection and singing behavior patterns of male house finches (Carpodacus mexicanus) in urban parks in a heavily urbanized landscape in the western U.S. Urban Habitats 3, 49–69.
Finkel, T. (2003). Oxidant signals and oxidative stress. Curr. Opin. Cell Biol. 15, 247–254. doi: 10.1016/S0955-0674(03)00002-4
Finkel, T., and Holbrook, N. J. (2000). Oxidants, oxidative stress and the biology of ageing. Nature 408, 239–247. doi: 10.1038/35041687
Garratt, M., and Brooks, R. C. (2012). Oxidative stress and condition-dependent sexual signals: more than just seeing red. Proc. R. Soc. B 279, 3121–3130. doi: 10.1098/rspb.2012.0568
Garratt, M., Pichaud, N., Glaros, E. N., Kee, A. J., and Brooks, R. C. (2014). Superoxide dismutase deficiency impairs olfactory sexual signaling and alters bioenergetic function in mice. Proc. Natl. Acad. Sci. U.S.A. 111, 8119–8124. doi: 10.1073/pnas.1322282111
Gil, D., Honarmand, M., Pascual, J., Perez-Mena, E., and Macias Garcia, C. (2015). Birds living near airports advance their dawn chorus and reduce overlap with aircraft noise. Behav. Ecol. 26, 435–443. doi: 10.1093/beheco/aru207
Giraudeau, M., and McGraw, K. J. (2014). Physiological correlates of urbanization in a desert songbird. Integr. Comp. Biol. 54, 622–632. doi: 10.1093/icb/icu024
Giraudeau, M., Chavez, A., Toomey, M. B., and McGraw, K. J. (2015). Effects of carotenoid supplementation and oxidative challenges on physiological parameters and carotenoid-based coloration in an urbanization context. Behav. Ecol. Sociobiol. 69, 957–970. doi: 10.1007/s00265-015-1908-y
Giraudeau, M., Mousel, M., Earl, S., and McGraw, K. (2014a). Parasites in the city: degree of urbanization predicts poxvirus and coccidian infections in house finches (Haemorhous mexicanus). PLoS ONE 9:e86747. doi: 10.1371/journal.pone.0086747
Giraudeau, M., Nolan, P. M., Black, C. E., Earl, S. R., Hasegawa, M., and McGraw, K. J. (2014b). Song characteristics track bill morphology along a gradient of urbanization in house finches (Haemorhous mexicanus). Front. Zool. 11:8. doi: 10.1186/s12983-014-0083-8
Hahs, A. K., and Evans, K. L. (2015). Expanding fundamental ecological knowledge by studying urban ecosystems. Funct. Ecol. 29, 863–867. doi: 10.1111/1365-2435.12488
Halfwerk, W., Bot, S., Buikx, J., van der Velde, M., Komdeur, J., ten Cate, C., et al. (2011). Low-frequency songs lose their potency in noisy urban conditions. Proc. Natl. Acad. Sci. U.S.A. 108, 14549–14554. doi: 10.1073/pnas.1109091108
Hamilton, W. D., and Zuk, M. (1982). Heritable true fitness and bright birds: a role for parasites? Science 218, 384–387. doi: 10.1126/science.7123238
Hasegawa, M., Ligon, R. A., Giraudeau, M., Watanabe, M., and McGraw, K. J. (2014). Urban and colorful male house finches are less aggressive. Behav. Ecol. 25, 641–649. doi: 10.1093/beheco/aru034
Henschen, A. E., Whittingham, L. A., and Dunn, P. O. (2015). Oxidative stress is related to both melanin and carotenoid-based ornaments in the common yellowthroat. Funct. Ecol. doi: 10.1111/1365-2435.12549. [Epub ahead of print].
Herrera-Dueñas, A., Pineda, J., Antonio, M. T., and Aguirre, J. I. (2014). Oxidative stress of house sparrow as bioindicator of urban pollution. Ecol. Indic. 42, 6–9. doi: 10.1016/j.ecolind.2013.08.014
Hill, G. E. (1993). Geographic variation in the carotenoid plumage pigmentation of male house finches (Carpodacus mexicanus). Biol. J. Linn. Soc. 49, 63–86.
Hõrak, P., Ots, I., Vellau, H., Spottiswoode, C., and Møller, A. P. (2001). Carotenoid-based plumage coloration reflects hemoparasite infection and local survival in breeding great tits. Oecologia 126, 166–173. doi: 10.1007/s004420000513
Hõrak, P., Surai, P. F., and Møller, A. P. (2002). Fat-soluble antioxidants in the eggs of great tits Parus major in relation to breeding habitat and laying sequence. Avian Sci. 2, 123–130.
Hõrak, P., Surai, P. F., Ots, I., and Møller, A. P. (2004). Fat soluble antioxidants in brood-rearing great tits Parus major: relations to health and appearance. J. Avian Biol. 35, 63–70. doi: 10.1111/j.0908-8857.2004.03167.x
Hõrak, P., Vellau, H., Ots, I., and Møller, A. P. (2000). Growth conditions affect carotenoid-based plumage coloration of great tit neslings. Naturwissenschaften 87, 460–464. doi: 10.1007/s001140050759
Hu, Y., and Cardoso, G. C. (2010). Which birds adjust the frequency of vocalizations in urban noise? Anim. Behav. 79, 863–867. doi: 10.1016/j.anbehav.2009.12.036
Hutton, P., Ligon, R. A., McGraw, K. J., Seymoure, B. M., and Simpson, R. K. (2015). Dynamic color communication. Curr. Opin. Behav. Sci. 6, 41–49. doi: 10.1016/j.cobeha.2015.08.007
Isaksson, C. (2009). The chemical pathway of carotenoids: from plants to birds. Ardea 97, 125–128. doi: 10.5253/078.097.0116
Isaksson, C. (2010). Pollution and its impact on wild animals: a meta-analysis on oxidative stress. EcoHealth 7, 342–350. doi: 10.1007/s10393-010-0345-7
Isaksson, C. (2015). Urbanization, oxidative stress and inflammation: a question of evolving, acclimatizing or coping with urban environmental stress. Funct. Ecol. 29, 913–923. doi: 10.1111/1365-2435.12477
Isaksson, C., and Andersson, S. (2007). Carotenoid diet and nestling provisioning in urban and rural great tits Parus major. J. Avian Biol. 38, 564–572. doi: 10.1111/j.2007.0908-8857.04030.x
Isaksson, C., Johansson, A., and Andersson, S. (2008). Egg yolk carotenoids in relation to habitat and reproductive investment in the great tit Parus major. Physiol. Biochem. Zool. 81, 112–118. doi: 10.1086/522650
Isaksson, C., McLaughlin, P., Monaghan, P., and Andersson, S. (2007a). Carotenoid pigmentation does not reflect total non-enzymatic antioxidant activity in plasma of adult and nestling great tits, Parus major. Funct. Ecol. 21, 1123–1129. doi: 10.1111/j.1365-2435.2007.01317.x
Isaksson, C., Örnborg, J., Stephensen, E., and Andersson, S. (2005). Plasma glutathione and carotenoid coloration as potential biomarkers of environmental stress in great tits. EcoHealth 2, 138–146. doi: 10.1007/s10393-005-3869-5
Isaksson, C., Von Post, M., and Andersson, S. (2007b). Sexual, seasonal, and environmental variation in plasma carotenoids in great tits, Parus major. Biol. J. Linn. Soc. 92, 521–527. doi: 10.1111/j.1095-8312.2007.00852.x
Jacquin, L., Lenouvel, P., Haussy, C., Ducatez, S., and Gasparini, J. (2011). Melanin-based coloration is related to parasite intensity and cellular immune response in an urban free living bird: the feral pigeon Columba livia. J. Avian Biol. 42, 11–15. doi: 10.1111/j.1600-048X.2010.05120.x
Janssens, L., and Stoks, R. (2013). Predation risk causes oxidative damage in prey. Biol. Lett. 9:20130350. doi: 10.1098/rsbl.2013.0350
Jasinska, E. J., Goss, G. G., Gillis, P. L., Van Der Kraak, G. J., Matsumoto, J., de Souza Machado, A. A., et al. (2015). Assessment of biomarkers for contaminants of emerging concern on aquatic organisms downstream of a municipal wastewater discharge. Sci. Total Environ. 530–531, 140–153. doi: 10.1016/j.scitotenv.2015.05.080
Jones, T. M., Rodewald, A. D., and Shustack, D. P. (2010). Variation in plumage coloration of northern cardinals in urbanizing landscapes. Wilson J. Ornithol. 122, 326–333. doi: 10.1676/09-082.1
Kight, C. R., and Swaddle, J. P. (2015). Eastern bluebirds alter their song in response to anthropogenic changes in the acoustic environment. Integr. Comp. Biol. 55:icv070. doi: 10.1093/icb/icv070
Kramarenko, S. S., Khokhutkin, I. M., and Grebennikov, M. E. (2007). Specific features of phenetic structure of the terrestrial snail Cepaea vindobonensis (Pulmonata; Helicidae) in urbanized and natural populations. Russ. J. Ecol. 38, 39–45. doi: 10.1134/S1067413607010079
Kulczykowska, E., Kasprzak, M., Kalamarz, H., Kuriata, M., Nietrzeba, M., Jerzak, L., et al. (2007). Melatonin and thyroxine response to pollution in white stork nestlings (Ciconia ciconia): aspects of rhythmicity and age. Comp. Biochem. Physiol. C 146, 392–397. doi: 10.1016/j.cbpc.2007.04.012
Lampe, U., Reinhold, K., and Schmoll, T. (2014). How grasshoppers respond to road noise: developmental plasticity and population differentiation in acoustic signalling. Funct. Ecol. 28, 660–668. doi: 10.1111/1365-2435.12215
Lampe, U., Schmoll, T., Franzke, A., and Reinhold, K. (2012). Staying tuned: grasshoppers from noisy roadside habitats produce courtship signals with elevated frequency components. Funct. Ecol. 26, 1348–1354. doi: 10.1111/1365-2435.12000
Lazerte, S. E., Otter, K. A., and Slabbekoorn, H. (2015). Relative effects of ambient noise and habitat openness on signal transfer for chickadee vocalizations in rural and urban green-spaces. Bioacoustics 24, 233–252. doi: 10.1080/09524622.2015.1060531
Leon, E., Beltzer, A., and Quiroga, M. (2014). The Saffron finch (Sicalis flaveola) modifies its vocalizations to adapt to urban habitats. Rev. Mex. Biodivers. 85, 546–552. doi: 10.7550/rmb.32123
Lionetto, M. G., Caricato, R., Giordano, M. E., Pascariello, M. F., Marinosci, L., and Schettino, T. (2003). Integrated use of biomarkers (acetylcholinesterase and antioxidant enzymes activities) in Mytilus galloprovincialis and Mullus barbatus in an Italian coastal marine area. Mar. Pollut. Bull. 46, 324–330. doi: 10.1016/S0025-326X(02)00403-4
Lowry, H., Lill, A., and Wong, B. B. M. (2012). How noisy does a noisy miner have to be? Amplitude adjustments of alarm calls in an avian urban adapter. PLoS ONE 7:e29960. doi: 10.1371/journal.pone.0029960
Lucas, L. D., and French, S. S. (2012). Stress-induced tradeoffs in a free-living lizard across a variable landscape: consequences for individuals and populations. PLoS ONE 7:e49895. doi: 10.1371/journal.pone.0049895
Massaro, M., Davis, L. S., and Darby, J. T. (2003). Carotenoid-derived ornaments reflect parental quality in male and female yellow-eyed penguins (Megadyptes antipodes). Behav. Ecol. Sociobiol. 55, 169–175. doi: 10.1007/s00265-003-0683-3
McDonnell, M. J., and Hahs, A. K. (2015). Adaptation and adaptedness of organisms to urban environments. Annu. Rev. Ecol. Evol. Syst. 46, 261–280. doi: 10.1146/annurev-ecolsys-112414-054258
Mockford, E. J., and Marshall, R. C. (2009). Effects of urban noise on song and response behaviour in great tits. Proc. R. Soc. B 276, 2979–2985. doi: 10.1098/rspb.2009.0586
Møller, A. P., Erritzøe, J., and Karadas, F. (2010). Levels of antioxidants in rural and urban birds and their consequences. Oecologia 163, 35–45. doi: 10.1007/s00442-009-1525-4
Narango, D. L., and Rodewald, A. D. (2016). Urban-associated drivers of song variation along a rural–urban gradient. Behav. Ecol. 27, 608–616. doi: 10.1093/beheco/arv197
Navara, K. J., and Nelson, R. J. (2007). The dark side of light at night: physiological, epidemiological, and ecological consequences. J. Pineal Res. 43, 215–224. doi: 10.1111/j.1600-079X.2007.00473.x
Nowicki, S., Searcy, W. A., and Peters, S. (2002). Brain development, song learning and mate choice in birds: a review and experimental test of the nutritional stress hypothesis. J. Comp. Physiol. A 188, 1003–1014. doi: 10.1007/s00359-002-0361-3
Obukhova, N. Y. (2007). Polymorphism and phene geography of the blue rock pigeon in Europe. Russ. J. Genet. 43, 492–501. doi: 10.1134/S1022795407050031
Obukhova, N. Y. (2011). Dynamics of balanced polymorphism morphs in blue rock pigeon Columbia livia. Russ. J. Genet. 47, 83–89. doi: 10.1134/S1022795411010078
Parris, K. M., Velik-Lord, M., and North, J. M. A. (2009). Frogs call at a higher pitch in traffic noise. Ecol. Soc. 14, 25.
Potvin, D. A., and Mulder, R. A. (2013). Immediate, independent adjustment of call pitch and amplitude in response to varying background noise by silvereyes (Zosterops lateralis). Behav. Ecol. 24, 1363–1368. doi: 10.1093/beheco/art075
Potvin, D. A., Mulder, R. A., and Parris, K. M. (2014). Silvereyes decrease acoustic frequency but increase efficacy of alarm calls in urban noise. Anim. Behav. 98, 27–33. doi: 10.1016/j.anbehav.2014.09.026
Potvin, D. A., and Parris, K. M. (2012). Song convergence in multiple urban populations of silvereyes (Zosterops lateralis). Ecol. Evol. 2, 1977–1984. doi: 10.1002/ece3.320
Prakesh, I., Idris, M., and Kumari, S. (1998). Scent marking behaviour of three desert rodents. Proc. Indian Natl. Acad. Sci. 64, 319–334.
Qvarnström, A., Pärt, T., and Sheldon, B. C. (2000). Adaptive plasticity in mate preference linked to differences in reproductive effort. Nature 405, 344–347. doi: 10.1038/35012605
Redondo, P., Barrantes, G., and Sandoval, L. (2013). Urban noise influences vocalization structure in the House Wren Troglodytes aedon. Ibis 155, 621–625. doi: 10.1111/ibi.12053
Ríos-Chelén, A. A., Lee, G. C., and Patricelli, G. L. (2015). Anthropogenic noise is associated with changes in acoustic but not visual signals in red-winged blackbirds. Behav. Ecol. Sociobiol. 69, 1139–1151. doi: 10.1007/s00265-015-1928-7
Ríos-Chelén, A. A., Quirós-Guerrero, E., Gil, D., and Macías Garcia, C. (2013). Dealing with urban noise: vermilion flycatchers sing longer songs in noisier territories. Behav. Ecol. Sociobiol. 67, 145–152. doi: 10.1007/s00265-012-1434-0
Ripmeester, E. A. P., Mulder, M., and Slabbekoorn, H. (2010). Habitat-dependent acoustic divergence affects playback response in urban and forest populations of the European blackbird. Behav. Ecol. 21, 876–883. doi: 10.1093/beheco/arq075
Robertson, B. A., Rehage, J. S., and Sih, A. (2013). Ecological novelty and the emergence of evolutionary traps. Trends Ecol. Evol. 28, 552–560. doi: 10.1016/j.tree.2013.04.004
Robinson, M. R., Pilkington, J. G., Clutton-Brock, T. H., Pemberton, J. M., and Kruuk, L. E. B. (2008). Environmental heterogeneity generates fluctuating selection on a secondary sexual trait. Curr. Biol. 18, 751–757. doi: 10.1016/j.cub.2008.04.059
Rodewald, A. D., Shustack, D. P., and Jones, T. M. (2011). Dynamic selective environments and evolutionary traps in human-dominated landscapes. Ecology 92, 1781–1788. doi: 10.1890/11-0022.1
Seger-Fullam, K., Rodewald, A., and Soha, J. (2011). Urban noise predicts song frequency in northern cardinals and American robins. Bioacoustics 20, 267–276. doi: 10.1080/09524622.2011.9753650
Seifried, H. E., Anderson, D. E., Fisher, E. I., and Milner, J. A. (2007). A review of the interaction among dietary antioxidants and reactive oxygen species. J. Nutr. Biochem. 18, 567–579. doi: 10.1016/j.jnutbio.2006.10.007
Senar, J. C., Conroy, M. J., Quesada, J., and Mateos-Gonzalez, F. (2014). Selection based on the size of the black tie of the great tit may be reversed in urban habitats. Ecol. Evol. 4, 2625–2632. doi: 10.1002/ece3.999
Shieh, B., Sen, L.iang, S. H., Chen, C. C., Loa, H. H., and Liao, C. Y. (2012). Acoustic adaptations to anthropogenic noise in the cicada Cryptotympana takasagona Kato (Hemiptera: Cicadidae). Acta Ethol. 15, 33–38. doi: 10.1007/s10211-011-0105-x
Skarphedinsdottir, H., Hallgrimsson, G. T., Hansson, T., Hägerroth, P.-A. Å., Liewenborg, B., Tjärnlund, U., et al. (2010). Genotoxicity in herring gulls (Larus argentatus) in Sweden and Iceland. Mutat. Res. 702, 24–31. doi: 10.1016/j.mrgentox.2010.07.002
Slabbekoorn, H., Yeh, P., and Hunt, K. (2007). Sound transmission and song divergence: a comparison of urban and forest acoustics. Condor 109, 67–78. doi: 10.1650/0010-5422(2007)109
Stewart, A. J. A., and Lees, D. R. (1996). The Colour/Pattern Polymorphism of Philaenus spumarius (L.) (Homoptera: Cercopidae) in England and Wales. Philos. Trans. R. Soc. B 351, 69–89. doi: 10.1098/rstb.1996.0005
Stoddard, P. K. (2002). The evolutionary origins of electric signal complexity. J. Physiol. 96, 485–491. doi: 10.1016/S0928-4257(03)00004-4
Suárez-Rodríguez, M., and Macías Garcia, C. (2014). There is no such a thing as a free cigarette; lining nests with discarded butts brings short-term benefits, but causes toxic damage. J. Evol. Biol. 27, 2719–2726. doi: 10.1111/jeb.12531
Svensson, P. A., and Wong, B. B. M. (2011). Carotenoid-based signals in behavioural ecology: a review. Behaviour 148, 131–189. doi: 10.1163/000579510X548673
Tinghitella, R. M., and Zuk, M. (2009). Asymmetric mating preferences accommodated the rapid evolutionary loss of a sexual signal. Evolution 63, 2087–2098. doi: 10.1111/j.1558-5646.2009.00698.x
Tomášek, O., Gabrielová, B., Kačer, P., Maršík, P., Svobodová, J., Syslová, K., et al. (2016). Opposing effects of oxidative challenge and carotenoids on antioxidant status and condition-dependent sexual signalling. Sci. Rep. 6:23546. doi: 10.1038/srep23546
Tringali, A., and Bowman, R. (2015). Suburban immigrants to wildlands disrupt honest signaling in ultra-violet plumage. Avian Conserv. Ecol. 10:9. doi: 10.5751/ACE-00746-100109
Troïanowski, M., Dumet, A., Condette, C., Lengagne, T., and Mondy, N. (2015). Traffic noise affects colouration but not calls in the European treefrog (Hyla arborea). Behaviour 152, 821–836. doi: 10.1163/1568539X-00003255
van der Vliet, A., and Bast, A. (1992). Effect of oxidative stress on receptors and signal transmission. Chem. Biol. Interact. 85, 95–116.
Venancio, L. P. R., Silva, M. I. A., da Silva, T. L., Moschetta, V. A. G., de Campos Zuccari, D. A. P., Almeida, E. A., et al. (2013). Pollution-induced metabolic responses in hypoxia-tolerant freshwater turtles. Ecotoxicol. Environ. Saf. 97, 1–9. doi: 10.1016/j.ecoenv.2013.06.035
von Schantz, T., Bensch, S., Grahn, M., Hasselquist, D., and Wittzell, H. (1999). Good genes, oxidative stress and condition-dependent sexual signals. Proc. R. Soc. B 266, 1–12. doi: 10.1098/rspb.1999.0597
Walker, L. K., Stevens, M., Karadaş, F., Kilner, R. M., and Ewen, J. G. (2013). A window on the past: male ornamental plumage reveals the quality of their early-life environment. Proc. Biol. Sci. 280:20122852. doi: 10.1098/rspb.2012.2852
Whittaker, D. J., Soini, H. A., Atwell, J. W., Hollars, C., Novotny, M. V., and Ketterson, E. D. (2010). Songbird chemosignals: volatile compounds in preen gland secretions vary among individuals, sexes, and populations. Behav. Ecol. 21, 608–614. doi: 10.1093/beheco/arq033
Wood, W. E., and Yezerinac, S. M. (2006). Song sparrow (Melospiza melodia) song varies with urban noise. Auk 123, 650–659. doi: 10.1642/0004-8038(2006)123[650:SSMMSV]2.0.CO;2
Yang, X., and Slabbekoorn, H. (2014). Timing vocal behaviour: lack of temporal overlap avoidance to fluctuating noise levels in singing Eurasian wrens. Behav. Process. 108, 131–137. doi: 10.1016/j.beproc.2014.10.002
Yeh, P. J. (2004). Rapid evolution of a sexually selected trait following population establishment in a novel habitat. Evolution 58, 166–174. doi: 10.1554/03-182
Keywords: antioxidants, animal communication, dishonest signals, oxidative stress, pro-oxidants, signal honesty, urbanization
Citation: Hutton P and McGraw KJ (2016) Urban Impacts on Oxidative Balance and Animal Signals. Front. Ecol. Evol. 4:54. doi: 10.3389/fevo.2016.00054
Received: 30 January 2016; Accepted: 26 April 2016;
Published: 19 May 2016.
Edited by:
David Costantini, University of Antwerp, BelgiumReviewed by:
Stefania Casagrande, Max Planck Institute for Ornithology, GermanyAmparo Herrera-Dueñas, Complutense University of Madrid, Spain
Copyright © 2016 Hutton and McGraw. This is an open-access article distributed under the terms of the Creative Commons Attribution License (CC BY). The use, distribution or reproduction in other forums is permitted, provided the original author(s) or licensor are credited and that the original publication in this journal is cited, in accordance with accepted academic practice. No use, distribution or reproduction is permitted which does not comply with these terms.
*Correspondence: Pierce Hutton, cGllcmNlLmh1dHRvbkBhc3UuZWR1