- 1Department of Zoology, Edward Grey Institute, University of Oxford, Oxford, UK
- 2Department of Life Sciences, Imperial College London, Ascot, UK
- 3School of Biological Sciences, University of Reading, Reading, UK
- 4Bristol Zoological Society, Bristol Zoo Gardens, Bristol, UK
- 5Department of Zoology, University of Otago, Dunedin, New Zealand
- 6Evolution and Ecology Research Centre and School of Biological, Earth and Environmental Sciences, University of New South Wales, Sydney, NSW, Australia
Communal signaling—wherein males and females collaborate to produce joint visual or acoustic displays—is perhaps the most complex and least understood form of communication in social animals. Although many communal signals appear to mediate competitive interactions within and between coalitions of individuals, previous studies have highlighted a confusing array of social and environmental factors that may explain the evolution of these displays, and we still lack the global synthesis needed to understand why communal signals are distributed so unevenly across large taxonomic and geographic scales. Here, we use Bayesian phylogenetic models to test whether acoustic communal signals (duets and choruses) are explained by a range of life-history and environmental variables across 10328 bird species worldwide. We estimate that duets and choruses occur in 1830 (18%) species in our sample and are thus considerably more widespread than previously thought. We then show that global patterns in duetting and chorusing, including evolutionary transitions between communal signaling and solo signaling, are not explained by latitude, migration, climate, or habitat, and only weakly correlated with cooperative breeding. Instead, they are most strongly associated with year-round territoriality, typically in conjunction with stable social bonds. Our results suggest that the evolution of communal signals is associated with the coordinated defense of ecological resources by stable coalitions of males and females, and that other widely reported associations are largely by-products of this underlying trend.
Introduction
Communal signals are joint visual or acoustic displays produced when two or more individuals coordinate their signaling behavior, a communication strategy widespread in social animals, including crustaceans (Tóth and Duffy, 2005), primates (Müller and Anzenberger, 2002), and birds (Hall, 2009). A key feature of these signals is that male and female animals both contribute to a combined signal, often with extreme temporal precision, as in the case of many avian duets and choruses (Mann et al., 2006; Hall and Magrath, 2007). Duetting and chorusing are perhaps not ancestral, but nonetheless an ancient traits in birds (Logue and Hall, 2014; Odom et al., 2014), and a common feature of species in which both sexes sing (Slater and Mann, 2004). Thus, the question of why birds signal communally is central to understanding the factors driving song evolution in females and maintaining song as a trait in both sexes.
Numerous hypotheses have been proposed for the function of communal signals (Hall, 2009), generally based on the concept of competition for either ecological resources, or mates and mating opportunities (Farabaugh, 1982). Ecological hypotheses highlight the importance of cooperative defense of home ranges and foraging territories, with duets and choruses signaling the relative competitive ability of coalitions in terms of quality, stability, or numerical advantage (McComb et al., 1994; Seddon and Tobias, 2003; Radford and du Plessis, 2004; Hall and Magrath, 2007). Social hypotheses focus instead on pair or group bonds, suggesting a role for communal signals in guarding against extra-pair or extra-group fertilization (Sonnenschein and Reyer, 1983; Seddon and Tobias, 2006; Tobias and Seddon, 2009), defending positions in partnerships or groups (Rogers et al., 2007) or signaling commitment between breeding partners (Wickler, 1980). Of course, these factors are not mutually exclusive as territorial behavior and social bonding have partially overlapping functions, perhaps explaining why previous studies have found mixed support for both sets of ideas (Hall, 2000; Marshall-Ball et al., 2006; Rogers et al., 2007; Mennill and Vehrencamp, 2008).
Another obstacle to disentangling the drivers of communal signal evolution is that several alternative proximate explanations have been proposed. For example, duets and choruses are more often reported in the tropics, and thus may simply be associated with latitude, temperature, or climatic stability (Slater and Mann, 2004; Logue and Hall, 2014). Similarly, communal signals are often a feature of social and sedentary species, perhaps because they are a by-product of cooperative breeding (Seddon and Tobias, 2003; Radford and du Plessis, 2004), or otherwise associated with long-term monogamy (Benedict, 2008) and stable social bonds in general (Logue and Hall, 2014). The same pattern viewed in reverse may explain the rarity of duetting in migratory lineages, where social bonds are more likely to break down (Logue and Hall, 2014). Habitat may also play a role, particularly as pair or group members are thought to maintain contact using acoustic communal signals in habitats where visual signals are ineffective, such as dense forests (Slater, 1997; Slater and Mann, 2004; Mennill and Vehrencamp, 2008).
Many of these social and environmental factors are closely interrelated, making it difficult to interpret experimental results in single species studies (Hall, 2000; Seddon and Tobias, 2006; Rogers et al., 2007; Tobias and Seddon, 2009), and creating a severe challenge for comparative analyses (Benedict, 2008; Logue and Hall, 2014). Furthermore, previous analyses have only used incomplete sets of predictors related to key hypotheses, with poor coverage of territorial behavior and social bonds. Thus, our understanding of communal signaling remains patchy and the extent to which we can generalize from previous results is unclear (Odom et al., 2015).
We addressed these issues by compiling information on territoriality, sociality, and the occurrence of duets and choruses across 10328 bird species (99% of extant species richness; see Appendix B in Supplementary Material). For each species, we estimated the standard duration of territory defense and social bonds, as well as the density of their primary habitat. All species were scored for migration and cooperative breeding, and we also used geographical range polygons to quantify environmental predictors, including latitude and climatic stability. These datasets offer a useful perspective on the relative roles of social and environmental factors in regulating song evolution in both sexes, particularly as the taxonomic sampling is so comprehensive.
Most studies investigating the function of communal signaling in birds have focused either on single species or single clades (e.g., Odom et al., 2015). The most extensive analyses to date have dealt with a regional passerine avifauna (300 North American species; Benedict, 2008), or subsets (<5 %) of the global avifauna with high quality data (Logue and Hall, 2014). While these studies have highlighted potential mechanisms, they are sensitive to regional or taxonomic biases in the availability or quality of data, along with other sampling effects (e.g., tropical species are absent from the North American sample). Our approach is designed to assess general patterns while minimizing sampling effects by revising and updating data from all birds, the largest terrestrial vertebrate radiation. We further account for variation in data quality by assigning all species to one of four categories of uncertainty (see Materials and Methods).
We conducted two analyses. First, we combined all intrinsic and extrinsic traits, along with the evolutionary relationships among lineages, into a Bayesian mixed model (Hadfield, 2010; Hadfield and Nakagawa, 2010). This method can be used to identify key correlations but is much less informative about the sequence of evolutionary events giving rise to duets and choruses over time. Thus, we used a second Bayesian analysis of correlated evolution (Pagel and Meade, 2006) to estimate transition rates to and from communal signaling under a range of different character states. We used these analyses to clarify the extent to which ecological, social, and environmental drivers provide the most general explanation for global patterns in communal signaling, and whether the evolution of intrinsic life history traits facilitates the evolution of communal signaling (or vice versa).
Materials and Methods
Definitions
We define communal signaling as an acoustic display involving two or more members of a social unit, including both males and females. Their contribution to the display must include long-range acoustic signals that are coordinated or stereotyped in some way, whether they be loosely synchronous, regularly alternating, or precisely interwoven. In many cases, the primary long-range acoustic signal in birds is termed the “song,” but because we are interested in the underlying processes giving rise to communal signals, our definition extends beyond songs to include other long-range vocalizations with song-like functions, including non-vocal signals. Thus, for example, coordinated calling by pair-members in some seabirds is considered duetting (Bretagnolle, 1996), as is joint drumming by male and female woodpeckers (Picidae). Our definition includes all classic duets and choruses (Farabaugh, 1982), but excludes various multi-individual vocalizations, including contact calls, alarm calls, and flight calls. Further, details on discriminating these categories are given below.
In line with convention, we defined duets as communal signals involving two individuals, whereas choruses involve three or more individuals. Following previous studies (e.g., Logue and Hall, 2014), we pooled duetting and chorusing species together in our main analyses because (1) the division between them is very imprecise with many chorusing species occasionally duetting, and vice versa, causing much confusion in the literature, and (2) both forms of behavior are likely to evolve through similar mechanisms (Seddon, 2002; Seddon and Tobias, 2003).
We also collated data on key intrinsic and extrinsic factors hypothesized to play a role in the evolution of communal signaling (Hall, 2009). Specifically, following previous studies (Jetz and Rubenstein, 2011; Salisbury et al., 2012; Pigot and Tobias, 2015), we classified species according to their degree of territoriality (non-territorial, weakly territorial, or year-round territorial); social bond stability (solitary, short-term bond, or long-term bond); type of mating system (cooperative or non-cooperative); movement (non-migratory, partially migratory, or migratory); and habitat (open, semi-open, and dense).
We defined year-round territoriality as territory defense lasting throughout the year rather than residency within a restricted area. For example, species that are vocal and aggressive (responsive to playbacks) for part of the year, and then remain in the same general area silently and unobtrusively for the rest of the year, are classified as seasonally rather than year-round territorial. We defined long-term social bonds as pair or group bonds extending beyond a single year, although this does not necessarily imply that they are year-round. Thus, migratory species in which pair members habitually reunite in subsequent breeding seasons are treated as having long-term social bonds. A detailed rationale, with explanation of our assignment of species to all these categories, is given in Appendix A of Supplementary Material, and summarized in Table 1.
Data Collection
We compiled data from field observations, feedback from regional experts, published literature, sound archives, and other online sources of information. Details of signaling behavior, social system, territorial behavior, and movements in birds were compiled in a global database through direct observations by JAT and NS. Observations of >4000 breeding bird species spanned a 20-year period including fieldwork in Europe, the Middle East and North Africa, sub-Saharan Africa, Madagascar, South-east Asia, Australasia and the South Pacific, and extensively in North, Central and South America, with a focus on female song, duetting behavior, social systems and year-round movements (see, e.g., Tobias and Williams, 1996; Tobias and Seddon, 2000, 2003a,b; Seddon et al., 2002, 2003; Tobias, 2003b; Tobias et al., 2008, 2011). Throughout, playbacks were routinely used to assess the strength and seasonality of territory defense, and the contribution of males and females to territorial interactions, at different seasons when possible. To augment these observations, we solicited feedback from field biologists and ornithologists with experience of particular regions or clades. This included a number of professional birding guides who together observe >5000 bird species per annum, often using playback to show rare species to clients.
In addition to information generated from fieldwork, we conducted a thorough review of published literature and online resources. A major source of information was The Handbook of the Birds of the World (HBW) series (del Hoyo et al., 1992-2013), comprising 16 edited volumes of species and family accounts for all known bird species. The referenced species accounts, including sections dedicated to vocalizations and movements, are compiled by experts in focal taxa. This information was supplemented by family monographs, regional handbooks and key digital resources, such as Birds of America Online. We also conducted extensive searches for information on communal signaling through primary electronic databases (e.g., ISI Web of Knowledge, Scopus), and the meta-search engine Google Scholar. We used standardized search terms to identify sources of information for communal signaling (birds, cooperative signal*/song/singing, communal signal*/song/singing, chorus, chorusing, duet, duetting), territoriality (birds, territor*, year-round territor*, long-term territor*, stable territor*, breeding territor*, flock territor*, non-territor*), and social bonding (birds, social bond*, pair bond*, group bond*, mate retention, mate fidelity, monogamy, divorce; where asterisks (*) denote multiple possible suffixes). Relevant secondary articles were identified from the references cited in books or articles found using these approaches.
Finally, we extended recent efforts (Logue and Hall, 2014) to extract information from sound recordings, images, and videos stored in public and private sound archives to compile evidence of communal signaling, with a focus on Macaulay Library (http://www.macaulaylibrary.org), Xeno-canto (http://www.xeno-canto.org), and the Internet Bird Collection (ibc.lynxeds.com). Together, these sources contain material for almost all the world's birds. We listened to sound files to verify putative cases of communal signaling and to survey signaling behavior in poorly known species. In addition, we checked accompanying metadata compiled by field recordists for textual confirmation of communal signaling and other details.
Details of methods for assigning species to categories of territory and social bond duration are provided in Appendix A of Supplementary Material. We classified signals as communal with due caution, bearing in mind several alternative possibilities. Distinguishing other multi-individual signals (e.g., contact calls, flock calls, alarm calls, flight calls) was generally straightforward, both in the field and using sound recordings, because these types of acoustic signal are relatively simple, uncoordinated among individuals, and often phylogenetically conserved variations on a theme. We interpreted multi-individual acoustic signals as evidence of communal signaling when they were coordinated or stereotyped. Typical examples include call-and-answer duets, where the gap between songs is shorter and more consistent than in contests between territorial rivals. Similarly, concurrent bursts of acoustic signals from multiple individuals in group-living species often provided a distinctive signature of communal signaling. In practice, assignment to categories was often simplified by the behavior al context of signaling, either directly observed in the field, or reported in sound file metadata. When the context was unclear, we did not necessarily assume communal signaling was occurring when two or more individuals were audible producing long-range acoustic signals, as in many cases it was difficult to rule out counter-singing by individuals in neighboring territories (i.e., different social units).
A separate challenge involves confirming that both males and females are contributing to communal signals. However, in practice this problem was largely irrelevant to socially monogamous species where we assume pairs contain one individual of both sexes. Although scoring species for female song can be challenging when the sexes are monomorphic (Odom et al., 2014), communal signaling is often easier to detect and verify in such cases because both pair members signal in unison. Conversely, it is sometimes difficult to be sure that females are contributing to choruses in group-living species. However, both sexes are known to contribute to choruses in color-marked populations of several species, and the same message emerges from observations of many monomorphic group-living species in which all individuals can be observed signaling together. Indeed, after considerable attention to this issue, we are not aware of any case of chorusing species where signaling is limited to one sex.
With respect to duetting species, we revised and updated some previous classifications with new information when it was clear that the original reports were based on misinterpretation. Our survey suggested that some species previously listed as duetters should be delisted for the purpose of our analyses because (1) the evidence for communal signaling clearly involved rare or unusual behavior, and (2) literature reports of communal signaling are sometimes based on different definitions of duets and choruses, with these terms often used loosely. For example, some authors use the terms “duet” or “chorus” to refer to counter-calling between neighboring territorial males, or to acoustic signals used in alarm and agitation. One such case is the American Rock-wren Salpinctes obsoletus, in which males can produce simple calls during close-quarters agonistic interactions with neighbors, sometimes accompanied by the female, leading to this species being listed as a duetter (Odom et al., 2015). We re-classify the species as a non-duetter because the calls are short-range signals given in agitation, whereas females do not sing or produce any other long-range signals, either alone or with the male. This distinction is important because males and females of all pair- or group-living bird species occasionally produce short-range acoustic signals at the same time. Counting all such cases as duets will obscure the underlying distribution of conventional duetting, potentially biasing the results of comparative studies, as recently shown in a similar dataset for avian cooperative breeding (Griesser and Suzuki, 2016). Where switches in classification were less certain, we simply assigned a lower score for data quality (see below).
Classifying the world's birds to behavioral and life history categories is challenging, not least because direct information is scarce for many species. We also acknowledge that the boundary between categorical variables is unavoidably blurred, making assignments subjective in some cases. For example, it can be difficult to judge whether a poorly known tropical species is territorial year-round or only during the breeding season, or whether its social bonds endure for a short breeding season or for multiple years (Stutchbury and Morton, 2001). However, for most species there is a growing body of information about local movements and whether particular pairs/groups remain spatially fixed over time. We followed simple rules-of-thumb to classify all cases. When evidence suggested that pairs or groups are highly sedentary, we assumed that pair/group bonds lasted more than a year on average (<50% divorce rate per annum). Our assumption is based on the general pattern established in field studies of color-marked bird populations: we are not aware of any bird species which lives in sedentary pairs or groups year-round and which also has a >50% annual divorce rate. When pair/group bonds break down seasonally (e.g., in migratory species, waterbirds), the uncertainty over divorce rates increases. We assumed that bonds lasted <1 year on average (i.e., >50% pairs or groups that reform in subsequent seasons contain new combinations of individuals) when there is evidence of this outcome in phylogenetically or ecologically related lineages (e.g., many migrant passerines). Conversely, when there was strong evidence that monogamous pairings extended beyond a single breeding season in phylogenetically or ecologically related lineages (e.g., seabirds and many migrant non-passerines), we assumed that >50% pairs reforming over subsequent seasons were likely to contain the same individuals. These procedures may result in some degree of misclassification but we argue that our categories provide an accurate general reflection of variation in social bond duration across the world's birds. Moreover, uncertain cases are reflected in scores of data quality and thus our conservative analyses are restricted to more objective cases.
Finally, assignment to categories may be uncertain when information is drawn from a single locality, or when species vary in a particular trait across their range. Whenever possible, we selected categories on the basis of their predominance in terms of behavior, or their prevalence across the global range of a species. Thus, for example, we classified species as year-round territorial only if such populations made up more than 50% of the global breeding range. In variable or wide-ranging species, we ensured that predictors and response variables were drawn from the same (or geographically closest) population.
Spatial and Climatic Data
Because communal signaling and the underlying degree of cooperation among individuals may be influenced by latitude and climatic conditions (Rubenstein and Lovette, 2007; Jetz and Rubenstein, 2011; Odom et al., 2014), we used the geographical range polygon for each species to extract median midpoint latitude and environmental data (mean annual temperature, temperature range, annual precipitation, and precipitation range) from the Worldclim database (http://www.worldclim.org), following standard methods (Pigot et al., 2010). Species lacking adequate data were excluded for the relevant analyses, leaving a sample of 9230 species for nested taxonomic models. After further excluding species for which no published genetic data yet exist, we retained a sample of 5505 species for phylogenetic mixed models.
For further details of hypotheses and data collection methods, see Appendix A in Supplementary Material; for a complete list of species and sources of information, see Appendices B, C in Supplementary Material.
Data Limitations, Inference, and Uncertainty
In this study, we provide the first global assessment of communal signaling, territoriality, and social bond duration across the world's birds. The scale of this assessment raises a number of challenges, not least because a large proportion of bird species remain poorly known. Nonetheless, we argue that sufficient information is now available to assign almost all species to a useful classification system. To achieve this goal, we used multiple strands of evidence, including direct observations and extensive unpublished information from sound archives and expert field ornithologists. Given the rapid pace of recent ornithological exploration in remote regions, most bird species–aside from a handful of extreme rarities–are now familiar to fieldworkers or birding guides at particular localities where information gathered on repeated visits can provide insight into territorial and social behavior through time. This influx of information is not readily available in published literature, but allows many species previously considered data deficient to be categorized with greater confidence. For example, Cacicus koepckeae is excluded from previous literature-based analyses of communal signaling (Odom et al., 2015) but included here as a territorial duetting species on the basis of field observations (Tobias, 2003a) and sound files archived online (see http://www.xeno-canto.org/species/Cacicus-koepckeae).
Where evidence was inconclusive, classifications were inferred partly from information relating to multiple close relatives, following standard procedures (Wilman et al., 2014). For communal signals, this type of inference was only used when there were strong grounds for doing so–for instance, when behavior was consistent across close relatives, backed up by circumstantial evidence such as field reports, sound recordings or videos. A similar approach was taken for life history attributes, with estimates of the duration of territory defense or social bonds often representing a best-guess when sufficient evidence was available from field observations, literature, and related species (see Appendix A in Supplementary Material for full details and rationale). Inferences were never drawn on the basis of phylogenetic relationships alone. Nonetheless, given the scale of our dataset, some lineages are almost certainly misclassified. A detailed summary of possible sources of error is provided in Appendix A of Supplementary Material.
To provide more information about variation in uncertainty, we assigned classifications of all species to four categories of data quality: A, high quality data based on published sources or strongly supported evidence from direct observations; B, medium quality data, including cases where the classification is very likely correct but largely based on field observations and reports; C, low quality data based on few observations, or unsubstantiated literature reports; D, absence of direct evidence. Henceforth, we refer to A as the conservative dataset, B as the medium quality dataset, and C and D together as poor quality data. The degree of inference from congeners is reflected in these categories, from very low inference in A, and minor, supporting inference in B, to larger levels of inference in C. Classifications of data-deficient species (D) were entirely based on inference. Where we found a strong consensus from all strands of evidence, we scored data quality higher than where evidence was in conflict. For example, golden whistlers Pachycephala pectoralis are reported to duet in captivity (Brown and Brown, 1994), but this behavior has not been detected in the field. Although this report may use a different definition of duetting to that employed in this study, it nonetheless increases the level of doubt about the lack of duetting observed in congeners, and thus we score most other Pachycephala species with an increased level of uncertainty. Finally, because levels of uncertainty often differ for information on communal signaling and general ecology, we scored data quality for both signaling and ecological data separately. Inclusion of species in analyses depended on both signaling and ecological data meeting minimum standards. Species were included (1) in our taxonomic analyses only if they scored A/B for song data quality and A/B/C for life history data quality; (2) in our main (medium quality data) analyses only if they were scored A for song data quality, and A/B for life history data quality; and (3) in our conservative analyses only if they scored A for both signaling and life history data quality. Like all datasets of global scale, ours will undoubtedly benefit from further quality control and curation, and we hope to facilitate this process by archiving all data online in association with this article.
Comparative Analyses
Our analyses included a range of (categorical) behavior and life history variables, and (continuous) climatic variables extracted from geographical ranges. We assessed the effects of these factors on the occurrence and evolution of communal signaling using Bayesian binary-response mixed-effect models with logit link, implemented in the R package, MCMCglmm (Hadfield, 2010; Hadfield and Nakagawa, 2010). To account for the potential effects of phylogenetic inertia, we adopted two complementary modeling approaches: (1) Bayesian taxonomic mixed models (BTMM) in which Order, Family and Genus were entered as nested random factors for all species, and (2) Bayesian phylogenetic mixed models (BPMM), in which phylogenetic relationships were entered as a random factor, assuming a Brownian model of evolution. This random term translates into phylogenetic variance equivalent to Pagel's λ (Pagel, 1999). We included BTMM as this allowed us to include all species with sufficient data (n = 9230), whereas BPMM were run on trees obtained from a published multilocus phylogeny, pruned to species with molecular data (n = 5505 for the medium dataset; and n = 1665 for the conservative dataset; Jetz et al., 2012). We first performed models (BTMM/BPMM) with 11 predictors (10 input variables: 4 intrinsic/life-history and 6 extrinsic/environmental; Table S2). We then re-ran the same models including significant predictors (i.e., those that were statistically significant in both full models) and their second-order interactions. Only interactions with strong effects were included, following (Gelman and Hill, 2007) (see electronic Supplementary Material, Table S3).
For all BTMMs and BPMMs, we used a Gelman prior for random effects (in MCMCglmm (Hadfield, 2010) using the command “gelman.prior” (Gelman et al., 2008) with V = 10−6, nu = −1). We ran three independent runs of MCMCglmm for all models models, each run for 1.5 × 106 iterations. After discarding a burn-in of 106 and a thinning of 5000, the remaining 1000 samples constituted our posterior distribution for each chain. We checked convergence of model parameters (fixed effects and random effects) using the Gelman-Rubin statistic (the potential scale reduction, PSR, factor should be <1.1 among chains (Gelman and Rubin, 1992); all PSR factors met this criterion). We only used posterior distributions from the first of three chains for reporting our parameter estimates (models and 95% credible limits, CLs). Note that in binary models (BTMM/BPMM) a dispersion parameter (akin to residual variance) is unidentifiable (zero). To run the models in MCMCglmm, we fixed the parameter > 0, but then rescaled estimates in the results tables (Table 1, and electronic Supplementary Material Table S1) so that the parameter = 0.
Regression analysis such as BTMM or BPMM are informative about the ecological and social conditions favoring the evolution of communal signaling, but not about the direction of causality. To address this question, we used Pagel's Discrete algorithm implemented in BayesTraits (Pagel and Meade, 2006) to test whether and how key traits have evolved in tandem across the same phylogenetic tree described above. We defined key traits as those significantly correlated with communal signaling in mixed models (BTMM and BPMM). The sample size (n = 5669 species) is slightly larger than for BPMMs because fewer species lacked relevant variables. The BayesTraits method uses a likelihood ratio test to compare a model in which the traits evolve independently (independent model) with one in which they evolve in tandem (dependent model). It also estimates the likelihood of evolutionary transitions among traits, assuming correlated evolution. These transition rates provide information about the relative stability of communal signaling with or without a particular life-history trait (and vice versa).
We used this approach to model how communal signaling was associated with territoriality, social bonds and mating system (independent and dependent models in each case, 6 models in total). As the method can only be applied to binary traits, we dichotomized variables initially classified into three categories (see Table S1). We grouped territoriality into: 1 = species with year-round territoriality, 0 = weak/seasonal territoriality or non-territorial. Similarly, we dichotomized social bond duration into: 1 = long-term (>1 year) pair/group bonds, 0 = short-term pair/group bonds or non-sociality. We grouped traits in this way for two main reasons. First, it produces the most balanced sampling in a dichotomous framework because relatively few species are non-territorial or lack social bonds (Figure 3). Second, this division most closely reflects existing hypotheses for communal signaling, which point to the importance of year-round territoriality (Benedict, 2008) and social stability (Logue and Hall, 2014).
We ran each BayesTraits model for 1.1 × 107 iterations, discarding an initial burn-in of 106 and sampling the chain every 10,000 iterations, resulting in a sample of 1000 per model/per tree. We ran two independent chains on each tree in the sample and combined samples resulting from all the runs, which constituted our posterior distributions for all parameter estimates. In all cases, a hyper prior of an exponential distribution (seeding from a uniform distribution on the interval 0–100) for a reversible jump procedure (see http://www.evolution.rdg.ac.uk/BayesTraits.html). The trees were scaled by 0.05, as the rates are proportional to the branch lengths. This places the transition rates on a more usable scale and does not alter their relative values. For each chain, the marginal likelihood was calculated using a stepping stone sampler (Xie et al., 2011): 100 stones were distributed according to a beta distribution (shape 0.400000, scale 1.000000) and each stone was run for 25,000 iterations.
Results
Prevalence and Distribution of Communal Signaling
We found evidence of communal signaling in 1830 species (18%) in the total list of 10328 species (see Appendix A in Supplementary Material). Excluding species with poor signaling data (category C and D) produced a smaller total of 1812 species with communal signaling (17%); of these, duetting occurs in 1627 (~16 %) species, a total that includes chorusing species which occasionally duet. Duetting was previously thought to be present in only 222 (or ~2–3%) of species (Thorpe, 1972; Kunkel, 1974; Farabaugh, 1982; Hall, 2004), with the estimate recently revised to 420 species (~4%; Hall, 2009). Even excluding species with poor quality data (categories C and D), our results indicate that communal signaling is taxonomically widespread, evolving multiple times across the avian tree of life (Figure 1), occurring in 26/39 orders (67%) and 110/225 families (49%), with roughly equal prevalence in the passerines (1102/6049, 18%) and non-passerines (710/3522, 20%).
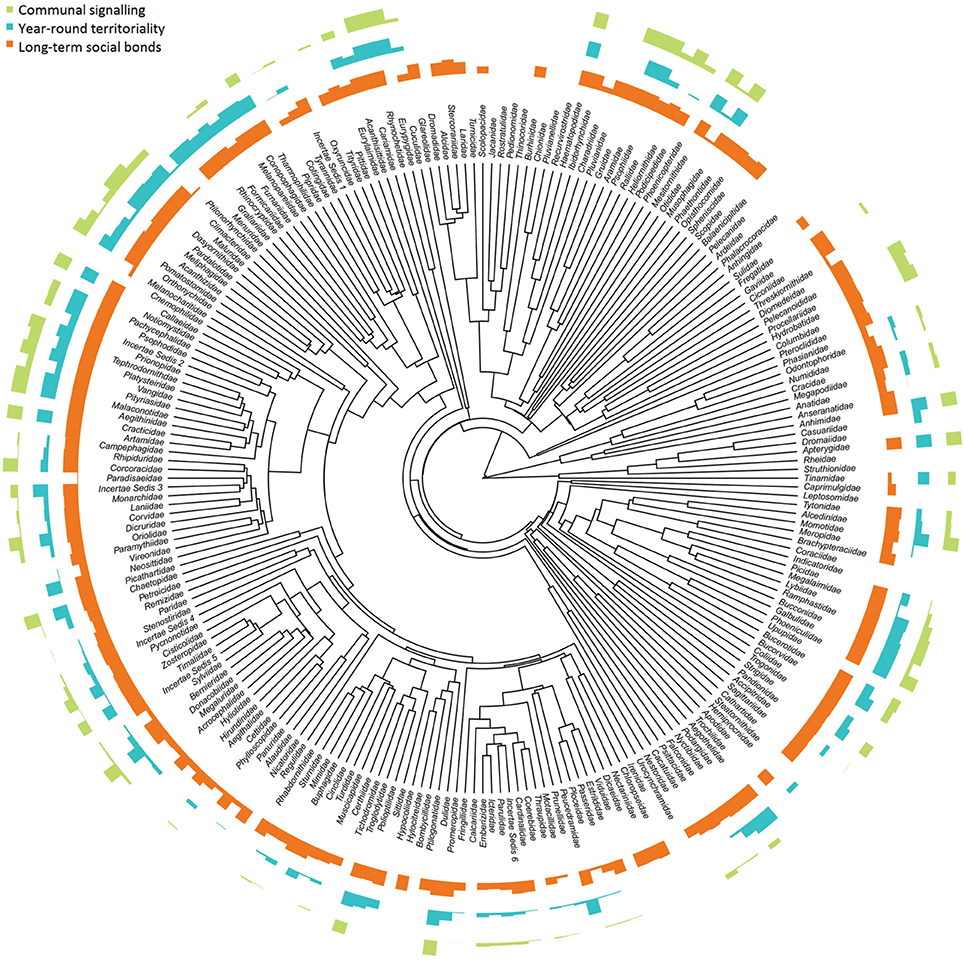
Figure 1. Proportion of species with communal signaling, long-term (> 1 year) social bonds, and year-round territoriality across avian families. Data are aggregated from 5505 species within 224 bird families and plotted at the tips of a maximum clade credibility phylogenetic tree. Species with high uncertainty were removed prior to calculating family totals; data presented are therefore the same as our main analyses (medium certainty); patterns based on more conservative data are very similar (see Figure S1). Bars are scaled to the proportion of species in each family expressing a particular trait: tallest bars = all species; shortest bars = zero species.
Our data confirmed that the geographical distribution of communal signaling is uneven, with greatest prevalence in western Amazonia, western and central Africa, Indo-Malaya, and northern Australia (Figure 2A). This distribution remains essentially unchanged when focusing on duetting species (Figure 2B) and conservative data (Figure S2). In general, more duetting and chorusing species occur in the tropics (Figures 2, 3A). However, this pattern is largely driven by greater species richness in the tropics, and after correcting for the gradient in overall diversity we find that communal signaling peaks in the southern hemisphere (Figure 3A). Across the world's terrestrial biomes (Olson et al., 2001), the highest proportions of species with communal signaling (18–20%) occur in tropical and subtropical habitats (grasslands, savannas, shrublands, and both moist and dry broadleaf forests), while the smallest proportions (~6%) occur in tundra and boreal forests (Table S1).
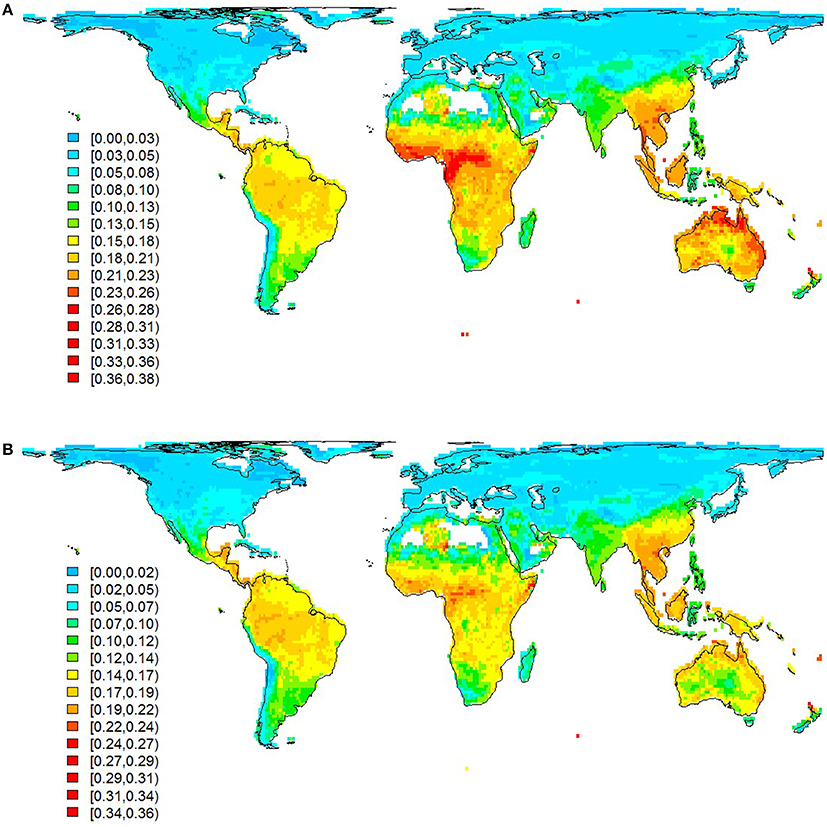
Figure 2. Global patterns in the distribution of communal signaling. Prevalence of species with (A) communal signaling and (B) duetting (subset of A), calculated as the proportion of total species occurring in 110 × 110 km grid cells. Legend gives lower and upper values for each color. Grid cells with <10 species were removed (e.g., Sahara). Species with high uncertainty were removed prior to calculating community totals; data presented are therefore the same as our main analyses (medium certainty) although a slightly smaller sample size (n = 5018) because some species lacked accurate maps; patterns based on more conservative data are very similar (see Figure S2).
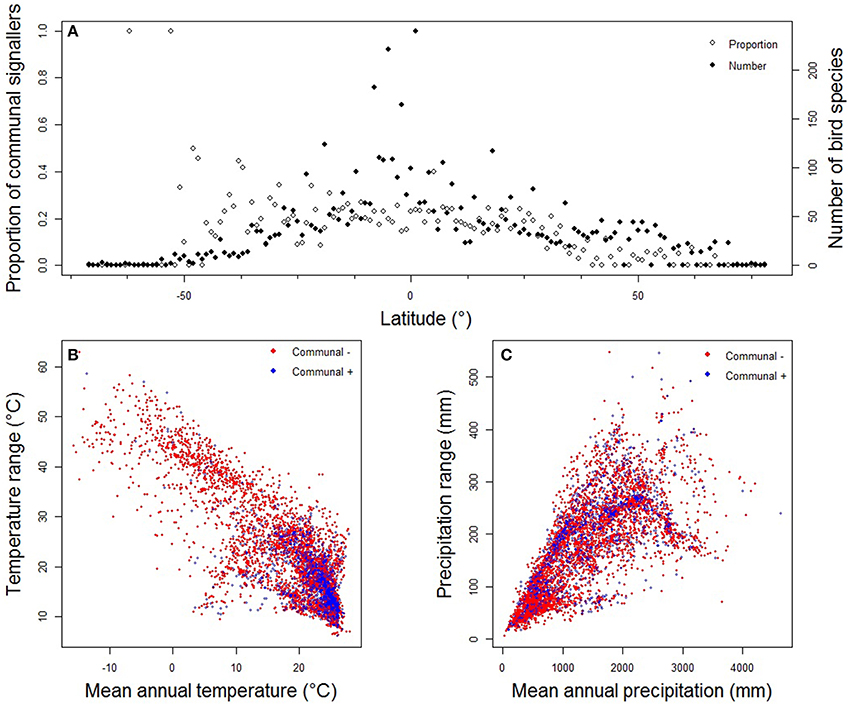
Figure 3. Spatial and environmental correlates of communal signaling in birds. Panels show the relationship between (A) communal signaling and midpoint latitude of species geographic ranges; (B) communal signaling (= Communal +) and temperature; and (C) communal signaling (= Communal +) and precipitation. Points in (A) are the proportion or number of species occurring within each 1° band of latitude; points in (B) and (C) represent data from a single species. Species with high uncertainty were removed and thus data presented are the same as our main analyses (medium certainty; n = 5505); patterns based on more conservative data are very similar (see Figure S3).
Predictors of Communal Signaling
We found that there is a strong phylogenetic signal in the occurrence of duetting and chorusing (Figure 1), with evolutionary history a dominant predictor of these traits in our combined full (Table S2), and final models (Table S3). In the BTMM, taxonomy (Order, Family, Genus) explained 16–39% of the variance in communal acoustic signaling, and in the BPMM, phylogeny explained ~96% of variance (at both levels of data certainty we used in analyses; see below). This result is not surprising given that communal signaling is widespread in some clades (e.g., antbirds Thamnophilidae) but absent in others (e.g., hummingbirds Trochilidae). However, the strength of phylogenetic signal may be inflated because we sometimes inferred shared character states among close relatives. We note that (1) even a much weaker phylogenetic signal supports our assumption of a Brownian motion model of evolution in subsequent analyses, and (2) inference of shared character states among relatives does not affect our main results because we use both taxonomic (BTMM) and phylogenetic (BPMM) models to correct for phylogenetic non-independence when testing for associations with communal signaling.
We found that territoriality, social bonds, cooperative breeding, latitude, and temperature range were all significant predictors of communal signaling in BTMMs (Tables S2, S3). No such association was found between habitat density or migration and communal signaling. However, the results of this hierarchical model should be treated with some caution because the BTMM contains only basic evolutionary information and may therefore fail to account adequately for phylogenetic non-independence (pseudoreplication).
Table S4 When we re-analyzed our data using BPMM, thus controlling for phylogeny, we found that communal signaling was significantly associated with territoriality and social bond stability, and that cooperative breeding was the only other significant (but weaker) correlate. We note that territoriality and cooperative breeding are strongly correlated: a model predicting cooperative breeding as a function of territoriality has an overall estimated R2 of 0.956, with an estimated partial R2 of phylogeny of 0.954; the coefficient estimate for the scaled territoriality value is −1.849 (CI: −2.677, −1.172). In contrast, we found no evidence that latitude, habitat density, migration, or climatic variability were associated with communal signaling (Tables S2, S3). Thus, although species with duets and choruses appear to be more prevalent in relatively stable tropical habitats (Figure 2A) with low annual variation in temperature (Figure 3B) and rainfall (Figure 3C), these associations disappeared when we accounted for evolutionary relationships and life-history traits. Running BPMMs on conservative data produced very similar results, except that the relationship between cooperative breeding and communal signaling then becomes non-significant (Table S2).
The fact that year-round territoriality and long-term social stability emerge as the most important predictors of communal signaling seems to make sense because many duetting or chorusing species share both these life history traits (Figure 4). However, the underlying correlation between territoriality and sociality is accounted for by our mixed modeling approach, and in any case the relationship between them was highly asymmetric: 3010 species with both strong territoriality and long-term social bonds made up 97% of the 3096 species with strong territoriality, but only 40% of the 7556 species with long-term social bonds. We also detected a significant interaction between territoriality and sociality (Table S3). Specifically, our results suggest that having one or other of year-round territoriality or social stability has a very large effect on the probability of communal signaling, particularly in the case of year-round territoriality, but that it's less important to have both (Table S3).
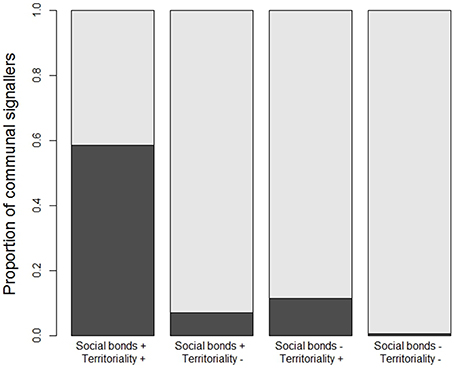
Figure 4. Associations between communal signaling and the stability of territoriality and social bonds. White bars show the proportion of species with communal signaling, black bars show the proportion with non-communal signaling, partitioned among species that have (+) or do not have (−) long-term social bonds and year-round territories (see Table 1). High uncertainty data were removed so that patterns are based on the medium certainty data (n = 5505 species) used in our main analyses; proportions calculated using conservative data are similar (see Figure S4).
Co-Evolution of Communal Signaling with Life-History Traits
When we used BayesTraits analyses to examine evolutionary transitions between states, we again found strong evidence that communal signaling evolved together with year-round territoriality (average log Bayes Factor 824.66), stable social bonds (average log Bayes Factor 310.70) and, to a lesser extent, cooperative breeding (average log Bayes Factor 26.23; Tables S4, S5). A log Bayes Factor above two can be viewed as significant (Kass and Raftery, 1995). Re-running these analyses on conservative data produced similar results (Tables S4, S6). The associations were slightly weaker (although still very strong) between communal signaling and both year-round territoriality (average log Bayes Factor 528.69) and stable social bonds (average log Bayes Factor 229.81). However, the significant association between communal signaling and cooperative breeding in the conservative dataset was much lower (average log Bayes Factor 6.29) and not significant in every tree (91 out of 100 had a Bayes Factor > 2).
Figure 5 illustrates the flow between evolutionary states detected in BayesTraits analyses. The arrows depicting this flow provide information about the stability of evolutionary states, with a low transition rate toward and a high transition rate away from a particular state indicating low stability of that state. For example, in (C) State 3 (communal signals and weak social bonds) is highly unstable, readily transitioning to State 1 (solo signals and weak social bonds) or State 4 (communal signals and strong social bonds). Similarly, the co-occurrence of communal signaling with cooperative breeding is unstable, readily transitioning to state 3, where breeding is non-cooperative but signaling is communal (Figure 5D). Conversely, in (B), State 4 (communal signals and strong territoriality) is stable, with balanced transitions to and from State 2 (solo signals and strong territoriality), and State 3 (communal signals and weak territoriality). The key points to take from Figure 5 are that q24 (evolving communal signals with territoriality) occurs 20 times faster than q13 (evolving communal signals without territoriality; Figure 5B), and that q34 (evolving communal signals with social bonds) occurs 23 times faster than q12 (evolving social bonds without communal signals; Figure 5C; Table S5).
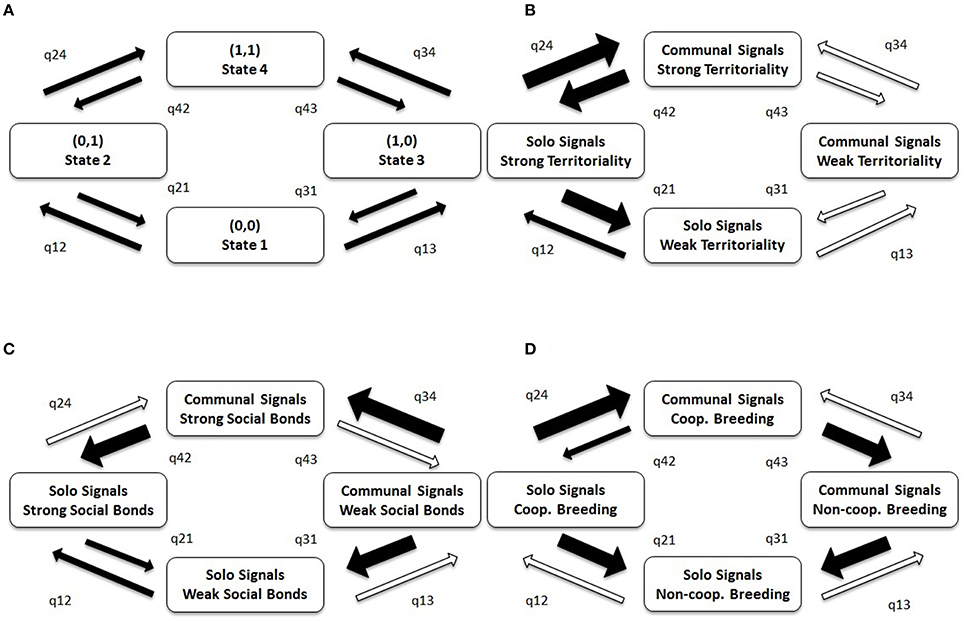
Figure 5. The co-evolution of communal signaling with life-history traits in birds. (A) Model illustrating four possible evolutionary states (1–4) between two traits and eight possible transition paths (q). (B–D) Results of BayesTraits analyses testing the relative stability of communal signaling in relation to three other life-history traits: (B) territoriality, (C) social bonds, and (D) cooperative breeding. Strong territoriality = year-round territory defense; Strong social bonds = estimated duration of pair or group bonds >1 year. Broad arrows indicate high transition rates (>40 transitions per lineage per billion years); thin arrows indicate medium transition rates (10–40 transitions per lineage per billion years); open arrows indicate low transition rates (<10 transitions per lineage per billion years); transitions are labeled such that qXY indicates the evolutionary transition from State X to Y. Actual values are provided in Table S5. Species with poor quality trait or phylogenetic data were removed from analyses (leaving a sample of n = 5669 species); the results based on conservative data are similar (see Figure S5, Table S6).
Discussion
Our comparative analyses reveal that avian duets and choruses are significantly linked to both year-round territory defense and long-term social bonds, and only weakly associated with cooperative breeding. Furthermore, once we accounted for these relationships, as well as for shared ancestry, we found no evidence that latitude, climatic variability, habitat, or migration predicted the occurrence of communal signals. These findings are corroborated by patterns of co-evolution among key life-history traits, which indicate that the presence of duets and choruses is most stable in association with territoriality and sociality. Thus, our results suggest that social factors predominate over environmental factors in driving communal signal evolution, and that the intensity and duration of ecological resource defense coupled with social stability provides the most general explanation for communal signal evolution.
The advantage of our broad-scale approach is that it offers sufficient statistical power to compare the effects of multiple factors. Our results shift the emphasis away from previously identified correlations with latitude, habitat density, migration, and climatic variability, perhaps because earlier studies were based on relatively restricted datasets sampled inconsistently across latitudes, climates, or major clades (e.g., passerines vs. non-passerine). This patchy sampling may generate different outcomes because associations vary across clades and contexts. For instance, while it is clear that for some species duets function partly in maintaining contact between pair members in dense habitats (Mennill and Vehrencamp, 2008), many duetting species occur in open environments, implying that habitat density does not provide a general explanation for communal signaling.
By sampling across the full span of environmental and life history variation in the world's birds, we have shown that correlations between communal signaling and environmental (extrinsic) factors are consistently subordinate to correlations with life-history (intrinsic) factors. The importance of species ecology over environmental conditions in promoting communal signaling has not previously been reported, but fits the observation that duets are well known in temperate zone species with year-round territoriality (e.g., tawny owl Strix aluco) or long-term social bonds (numerous seabirds), as well as in tropical species with the same underlying traits. Rather than latitude or climate explaining patterns in signaling behavior, our results suggest that the uneven geographical distribution of communal signaling shown in Figure 2 arises simply because extended forms of territoriality and sociality are biased toward the tropics and southern hemisphere. Indeed, this effect has been reported within evolutionary lineages: in the house wren Troglodytes aedon complex, for example, communal signals are common in the tropics where territories are defended year-round, but rare in the temperate zone where territoriality is seasonal (Stutchbury and Morton, 2001).
Selection is likely to favor long-term territoriality and social bonds at low and southern latitudes for a number of reasons (Jankowski et al., 2012; Tobias et al., 2013). First, the climate is generally more stable than in the northern temperate zone (Ghalambor et al., 2006), promoting sedentary lifestyles and stable social bonds. Second, the year-round availability of many ecological resources (Huston and Wolverton, 2009) means that the territories of land-birds are worth defending over longer time-periods. Third, avian populations in the tropics often approach carrying capacity owing to reduced mortality and increased longevity (Wiersma et al., 2007; Williams et al., 2010). Together, these factors place a high premium on the collaborative defense of ecological resources and group membership in the tropics, as territory or group vacancies are theoretically scarce and difficult to regain if lost. In this context, individuals may signal communally to protect their positions in long-term coalitions, which in turn cooperate over signal production to deter rival pairs or groups.
Disentangling the role of territoriality and sociality is challenging because communal signaling frequently occurs in conjunction with both year-round territoriality and long-term social bonds, which often occur together (Figure 4). This connection between long-term territoriality and social cohesion suggests that competition for ecological resources increases in parallel with competition over membership of partnerships or coalitions of individuals, perhaps helping to explain why avian duets appear to mediate both cooperation (i.e., joint territory defense; Seddon and Tobias, 2003; Hall and Magrath, 2007) and conflict (i.e., mate- defense; Sonnenschein and Reyer, 1983; Rogers et al., 2007; Tobias and Seddon, 2009). Nonetheless, phylogenetic mixed models revealed that the effect of territoriality was more than twice as strong as that of social bonds (Tables S2, S3), whereas cooperative breeding was only weakly associated, with an effect approximately one quarter that of social bonds.
Similarly, the evidence from evolutionary transitions suggests that the combination of year-round territoriality and communal signaling is a more stable state, and far more likely to co-evolve, than long-term social bonds coupled with communal signaling (Figure 5, Table S5). Furthermore, the BayesTraits analyses provide a clue that territoriality may be crucially important as a precursor to communal signaling, whereas long-term social bonds in pairs or groups may actually arise after communal signaling evolves–that is, pair and group bonds may result from selection for defending resources as a coalition, rather than vice versa. Although the pattern of evolutionary transitions in our dataset is most consistent with this interpretation, we do not specifically reconstruct ancestral states, and so the question of evolutionary pathways to (and from) communal signaling requires further investigation.
Many cooperatively breeding birds appear to signal as a group, and thus our finding that cooperative breeding is only weakly associated with communal signaling is perhaps surprising. The reason for this outcome becomes clearer when considering the correlation between cooperative breeding and territoriality, which is both strong and largely explained by phylogeny. Of these two associated variables, our results indicate that cooperative breeding is a much weaker predictor of communal signaling, and thus when territoriality is accounted for in phylogenetic models, cooperative breeding has very little additional explanatory power. This is particularly evident in our conservative analyses, where the association between cooperative breeding and communal signaling is removed altogether.
Cooperative breeding is only one form of cooperation in birds, and almost all avian duets and choruses function at least partly in cooperative contexts (Dahlin and Benedict, 2014), suggesting that global patterns of communal signaling can shed light on the evolution of cooperation (Logue and Hall, 2014). In highlighting the importance of long-term social bonds, our findings echo those of previous studies on duetting (Benedict, 2008; Logue and Hall, 2014). Moreover, as pointed out by Logue and Hall (2014), this pattern aligns with theoretical (Trivers, 1971) and empirical studies (Heide and Miner, 1992; Bó, 2005) suggesting that cooperation among individuals is most likely to arise when they associate over prolonged periods. Previous explanations for this effect are mainly based around the concepts of trust, reciprocity or kin selection (Heide and Miner, 1992). However, while it is difficult to rule out the influence of these factors in our study, the abundance of communal signals in species that are either socially monogamous or group-territorial with low intra-group relatedness suggests a prominent role for the simpler theory of interdependence (Roberts, 2005). Under this view, individuals cooperate over signal production because of the direct fitness benefits of collaboration–that is, individuals are more likely to maintain positions in pairs or groups and to defend adequate resources to reproduce if they coordinate signaling as a team.
Challenges and Opportunities
Synthesizing current information on territorial behavior, social stability and communal signaling across the world's birds is difficult given the lack of published studies for most species. Nonetheless, by incorporating multiple strands of evidence, including direct observations, experiments, and expert knowledge, we believe our dataset provides the most robust and comprehensive estimate of current knowledge to date. Our classification of species into broad categories means that, although some error is unavoidable, the majority of lineages are very likely assigned correctly. Moreover, the sample size is large enough to absorb considerable noise and we suspect that the key patterns reported are so striking that future adjustments will have little influence on the main results. This conclusion is strongly supported by sensitivity analyses showing that our results are robust to variation in data quality.
In effect, we have followed the model adopted by many prominent studies of climate change, or the IUCN Red List categories of conservation status (IUCN, 2001). The Red List uses arbitrary thresholds to assign species to threat categories in all but the most data-poor scenarios, often on the basis of expert opinion. Despite the drawbacks and early criticisms of this approach, it has been shown to be largely accurate, and has proved to be an extremely valuable tool for a prolific field of research (Rodrigues et al., 2006). While further revisions and corrections are inevitable, we hope the classifications presented here provide a similar template for further study, both to refine the dataset and to underpin broad-scale tests of evolutionary theory, in line with previously published datasets of similar scope (Cockburn, 2006; Jetz and Rubenstein, 2011; Wilman et al., 2014).
Conclusions
Based on our global survey, we estimate that communal signaling occurs in at least 1830 (~18 %) bird species, and is thus far more widespread than often assumed. Our analyses confirm that the occurrence of this behavior across the world's birds is correlated with a suite of environmental variables, including climatic variability and latitude, as well as migratory behavior and cooperative breeding. However, all these associations appear to be secondary because they are largely or entirely explained by a combination of long-term territory ownership and social bonds. We propose that the value and defendability of ecological resources, and the fluctuation of their value and defendability over time, are key factors driving the evolution of communal signaling. Competition for defendable resources may promote the formation of stable coalitions, theoretically increasing the degree of interdependence and collaboration among individuals. Given that communal signals are by definition produced by females as well as males, it seems likely that similar processes also play a prominent role in maintaining songs in females. However, further studies are required focusing more explicitly on patterns of female song in birds, including species where females sing independently from males.
Author Contributions
JT and NS conceived, designed, and coordinated the study, and wrote the manuscript. JT and AC compiled and curated data. CS, AM, and SN designed and carried out statistical analyses.
Funding
JT was supported by the John Fell Fund and the Natural Environment Research Council. NS was funded by a Royal Society University Research Fellowship. SN was supported by a Royal Society of New Zealand Rutherford Discovery Fellowship and an Australian Research Council Future Fellowship.
Conflict of Interest Statement
The authors declare that the research was conducted in the absence of any commercial or financial relationships that could be construed as a potential conflict of interest.
Acknowledgments
We thank Walter Jetz, David Orme, and Alex Pigot for technical support, access to data and comments on an earlier draft. We are also grateful to Moudud Hussain, Robin Lucas, Hannah MacGregor, and Monte Neate-Clegg for assistance with literature reviews and data management. Data collection was facilitated by many field researchers and curators of museums and sound archives (see Appendixes A,B in Supplementary Material for details).
Supplementary Material
The Supplementary Material for this article can be found online at: http://journal.frontiersin.org/article/10.3389/fevo.2016.00074
References
Bó, P. D. (2005). Cooperation under the shadow of the future: experimental evidence from infinitely repeated games. Am. Econ. Rev. 95, 1591–1604. doi: 10.1257/000282805775014434
Benedict, L. (2008). Occurrence and life history correlates of vocal duetting in North American passerines. J. Avian Biol. 39, 57–65. doi: 10.1111/j.0908-8857.2008.04103.x
Bretagnolle, V. (1996). “Acoustic communicationin a group of non-passerine birds, the Petrels,” in Ecology and Evolution of Acoustic Communication in Birds, eds E. Kroodsma and E. H. Miller (New York, NY: Cornell University Press Ithaca), 160–178.
Brown, R. J., and Brown, M. N. (1994). Matched song and duetting by a breeding pair of golden whistlers Pachycephala pectoralis. Emu 94, 58–59. doi: 10.1071/MU9940058
Cockburn, A. (2006). Prevalence of different modes of parental care in birds. Proc. R. Soc. Lond. B 273, 1375–1383. doi: 10.1098/rspb.2005.3458
Dahlin, C. R., and Benedict, L. (2014). Angry birds need not apply: a perspective on the flexible form and multifunctionality of avian vocal duets. Ethology 120, 1–10. doi: 10.1111/eth.12182
del Hoyo, J., Elliott, A., and Christie, D. (1992-2013). Handbook of the Birds of the World, Vol. 1, Barcelona: Lynx Edicions.
Farabaugh, S. M. (1982). “The ecological and social significance of duetting,” in Acoustic Communication in Birds, eds D. E. Kroodsma and E. H. Miller (New York, NY: Academic Press), 85–124.
Gelman, A., and Hill, J. (2007). Data Analysis Using Regression and Multilevel/Hierarchical Models. Cambridge: Cambridge University Press.
Gelman, A., Jakulin, A., Pittau, M. G., and Su, Y. S. (2008). A weakly informative default prior distribution for logistic and other regression models. Ann. Appl. Stat. 2, 1360–1383. doi: 10.1214/08-AOAS191
Gelman, A., and Rubin, D. B. (1992). Inference from iterative simulation using multiple sequences (with discussion). Stat. Sci. 7, 457–511. doi: 10.1214/ss/1177011136
Ghalambor, C. K., Huey, R. B., Martin, P. R., Tewksbury, J. J., and Wang, G. (2006). Are mountain passes higher in the tropics? Janzen's hypothesis revisited. Comp. Biol. 46, 5–17. doi: 10.1093/icb/icj003
Griesser, M., and Suzuki, T. N. (2016). Occasional cooperative breeding in birds and the robustness of comparative analyses concerning the evolution of cooperative breeding. Zool. Lett. 2, 7. doi: 10.1186/s40851-016-0041-8
Hadfield, J. D. (2010). MCMC methods for multi-response Generalised Linear Mixed Models: the MCM Cglmm R package. J. Stat. Soft. 33, 1–22.
Hadfield, J. D., and Nakagawa, S. (2010). General quantitative genetic methods for comparative biology: phylogenies, taxonomies and multi-trait models for continuous and categorical characters. J. Evo. Biol. 23, 494–508. doi: 10.1111/j.1420-9101.2009.01915.x
Hall, M. L. (2000). The function of duetting in magpie-larks: conflict, cooperation, or commitment? Anim. Behav. 60, 667–677. doi: 10.1006/anbe.2000.1517
Hall, M. L. (2004). A review of hypotheses for the functions of avian duetting. Behav. Ecol. Sociobiol. 55, 415–430. doi: 10.1007/s00265-003-0741-x
Hall, M. L. (2009). A review of vocal duetting in birds. Adv. Stud. Behav. 40, 67–121. doi: 10.1016/S0065-3454(09)40003-2
Hall, M. L., and Magrath, R. D. (2007). Temporal coordination signals coalition quality. Curr. Biol. 17, R406–R407. doi: 10.1016/j.cub.2007.04.022
Heide, J. B., and Miner, A. S. (1992). The shadow of the future: effects of anticipated interaction and frequency of contact on buyer-seller cooperation. Acad. Manage. 35, 265–291. doi: 10.2307/256374
Huston, M. A., and Wolverton, S. (2009). The global distribution of net primary production: resolving the paradox. Ecol. Monogr. 79, 343–377. doi: 10.1890/08-0588.1
IUCN (2001). Species Survival Commission. IUCN Red List Categories and Criteria: Version 3.1. Gland, Switzerland and Cambridge, UK: IUCN. Available online at: http://www.iucnredlist.org/static/categories_criteria_3_1
Jankowski, J. E., Graham, C. H., Parra, J. L., Robinson, S. K., Seddon, N., Touchton, J. M., et al. (2012). The role of competition in structuring tropical bird communities. Ornitol. Neotrop. 23, 115–124.
Jetz, W., and Rubenstein, D. R. (2011). Environmental uncertainty and the global biogeography of cooperative breeding in birds. Curr. Biol. 21, 72–78. doi: 10.1016/j.cub.2010.11.075
Jetz, W., Thomas, G. H., Joy, J. B., Hartmann, K., and Mooers, A. O. (2012). The global diversity of birds in space and time. Nature 491, 444–448. doi: 10.1038/nature11631
Kass, R. E., and Raftery, A. E. (1995). Bayes factors. J. Amer. Stat. Assoc. 90, 773–795. doi: 10.1080/01621459.1995.10476572
Kunkel, P. (1974). Mating systems of tropical birds: the effects of weakness or absence of external reproduction-timing factors, with special reference to prolonged pair bonds. Z. Tierpsychol. 34, 265–307.
Logue, D. M., and Hall, M. L. (2014). Migration and the evolution of duetting in songbirds. Proc. R. Soc. Lond. B. 281, 20140103. doi: 10.1098/rspb.2014.0103
Mann, N. I., Dingess, K. A., and Slater, P. J. B. (2006). Antiphonal four-part synchronized chorusing in a Neotropical wren. Biol. Lett. 2, 1–4. doi: 10.1098/rsbl.2005.0373
Marshall-Ball, L., Mann, N., and Slater, P. J. B. (2006). Multiple functions to duet singing: hidden conflicts and apparent cooperation. Anim. Behav. 71, 823–831. doi: 10.1016/j.anbehav.2005.05.021
McComb, K., Packer, C., and Pusey, A. (1994). Roaring and numerical assessment in contests between groups of female lions, Panthera leo. Anim. Behav. 47, 379–387. doi: 10.1006/anbe.1994.1052
Mennill, D. J., and Vehrencamp, S. L. (2008). Context-dependent functions of avian duets revealed by microphone-array recordings and multispeaker playback. Curr. Biol. 18, 1314–1319. doi: 10.1016/j.cub.2008.07.073
Müller, A. E., and Anzenberger, G. (2002). Duetting in the titi monkey Callicebus cupreus: structure, pair specificity and development of duets. Folia Primatol. 73, 104–115. doi: 10.1159/000064788
Odom, K. J., Hall, M. L., Riebel, K., Omland, K. E., and Langmore, N. E. (2014). Female song is widespread and ancestral in songbirds. Nat. Comms. 5, 3379. doi: 10.1038/ncomms4379
Odom, K. J., Omland, K. E., and Price, J. J. (2015). Differentiating the evolution of female song and male–female duets in the New World blackbirds: can tropical natural history traits explain duet evolution? Evolution 69, 839–847. doi: 10.1111/evo.12588
Olson, D. M., Dinerstein, E., Wikramanayake, E. D., Burgess, N. D., Powell, G. V. N., Underwood, E. C., et al. (2001). Terrestrial Ecoregions of the World: a new map of life on earth. BioScience 51, 933–938. doi: 10.1641/0006-3568(2001)051[0933:TEOTWA]2.0.CO;2
Pagel, M. (1999). Inferring the historical patterns of biological evolution. Nature 401, 877–884. doi: 10.1038/44766
Pagel, M., and Meade, A. (2006). Bayesian analysis of correlated evolution of discrete characters by reversible-jump Markov chain Monte Carlo. Am. Nat. 167, 808–825. doi: 10.1086/503444
Pigot, A. L., Owens, I. P. F., and Orme, C. D. L. (2010). The environmental limits to geographic range expansion in birds. Ecol. Lett. 13, 705–715. doi: 10.1111/j.1461-0248.2010.01462.x
Pigot, A. L., and Tobias, J. A. (2015). Dispersal and the transition to sympatry in vertebrates. Proc. R. Soc. B. 282, 20141929. doi: 10.1098/rspb.2014.1929
Radford, A. N., and du Plessis, M. A. (2004). Territorial vocal rallying in the green woodhoopoe: factors affecting the contest length and outcome. Anim. Behav. 68, 803–810. doi: 10.1016/j.anbehav.2004.01.010
Roberts, G. (2005). Cooperation through interdependence. Anim. Behav. 70, 901–908. doi: 10.1016/j.anbehav.2005.02.006
Rodrigues, A. S. L., Pilgrim, J. D., Lamoreux, J. F., Hoffmann, M., and Brooks, T. M. (2006). The value of the IUCN Red List for conservation. Trends Ecol. Evol. 21, 71–76. doi: 10.1016/j.tree.2005.10.010
Rogers, A. C., Langmore, N. E., and Mulder, R. A. (2007). Function of pair duets in the eastern whipbird: cooperative defense or sexual conflict? Behav. Ecol. 18, 182–188. doi: 10.1093/beheco/arl070
Rubenstein, D. R., and Lovette, I. J. (2007). Temporal environmental variability drives the evolution of cooperative breeding in birds. Curr. Biol. 17, 1414–1419. doi: 10.1016/j.cub.2007.07.032
Salisbury, C. L., Seddon, N., Cooney, C. R., and Tobias, J. A. (2012). The latitudinal gradient in dispersal constraints: ecological specialisation drives diversification in tropical birds. Ecol. Lett. 15, 847–855. doi: 10.1111/j.1461-0248.2012.01806.x
Seddon, N. (2002). Structure context and possible function of solos, duets and choruses in the subdesert mesite (Monias benschi). Behaviour 139, 645−676. doi: 10.1163/15685390260136753
Seddon, N., and Tobias, J. A. (2003). Communal singing in the cooperatively breeding subdesert mesite Monias benschi: evidence of numerical assessment? J. Avian Biol. 34, 72–80. doi: 10.1034/j.1600-048X.2003.03067.x
Seddon, N., and Tobias, J. A. (2006). Duets defend mates in a suboscine passerine, the warbling antbird (Hypocnemis cantator). Behav. Ecol. 17, 73–83. doi: 10.1093/beheco/ari096
Seddon, N., Tobias, J. A., and Alvarez, A. (2002). Vocal communication in the Pale-winged Trumpeter Psophia leucoptera: repertoire, context and functional reference. Behav. 139, 1331–1359. doi: 10.1163/156853902321104190
Seddon, N., Tobias, J. A., and Butchart, S. H. M. (2003). Group living, territoriality and breeding behaviour in the Subdesert Mesite Monias benschi. IBIS 145, 277–294. doi: 10.1046/j.1474-919X.2003.00150.x
Slater, P. J. B. (1997). Singing in the rain forest: the duets of bay wrens. Trends Ecol. Evol. 12, 207–208. doi: 10.1016/S0169-5347(97)01075-6
Slater, P. J. B., and Mann, N. (2004). Why do the females of many bird species sing in the tropics? J. Avian Biol. 35, 289–294. doi: 10.1111/j.0908-8857.2004.03392.x
Sonnenschein, E., and Reyer, H. U. (1983). Mate-guarding and other functions of antiphonal duets in the slate-coloured boubou (Laniarius funebris) Z. Tierpsychol. 63, 112–140. doi: 10.1111/j.1439-0310.1983.tb00083.x
Stutchbury, B. J. M., and Morton, E. S. (2001). Behavioral Ecology of Tropical Birds. San Diego, CA: Academic Press.
Thorpe, W. H. (1972). Duetting and antiphonal song in birds: its extent and significance. Behaviour 18, 1–197.
Tobias, J. A. (2003a). Further sightings of Selva Cacique Cacicus koepckeae in Manu National Park, Peru. Cotinga 19, 79–80.
Tobias, J. A. (2003b). Notes on breeding behaviour in Black-faced Cotinga Conioptilon mcilhennyi. Cotinga 19, 80–81.
Tobias, J. A., Şekercioğlu, Ç. H., and Vargas, F. H. (2013). “Bird conservation in tropical ecosystems: challenges and opportunities,” in Key Topics in Conservation Biology 2, eds D. W. Macdonald and K. J. Willis (London: John Wiley and Sons), 258–276.
Tobias, J. A., Gamarra-Toledo, V., Garcia-Olaechea, D., Pulgarin, P. C., and Seddon, N. (2011). Year-round resource defence and the evolution of male and female song in suboscine birds: social armaments are mutual ornaments. J. Evol. Biol. 24, 2118–2138. doi: 10.1111/j.1420-9101.2011.02345.x
Tobias, J. A., Lebbin, D. J., Aleixo, A., Andersen, M. J., Guilherme, E., Hosner, P. A., et al. (2008). Distribution, behavior and conservation status of the Rufous Twistwing Cnipodectes superrufus. Wilson J. Ornithol. 120, 38–49. doi: 10.1676/06-088.1
Tobias, J. A., and Seddon, N. (2000). Territoriality as a paternity guard in the European robin Erithacus rubecula. Anim. Behav. 60, 165–173. doi: 10.1006/anbe.2000.1442
Tobias, J. A., and Seddon, N. (2003a). Breeding, foraging and vocal behavior in the White-throated Jacamar Brachygalba albogularis. Wilson J. Ornithol. 115, 237–240. doi: 10.1676/02-074
Tobias, J. A., and Seddon, N. (2003b). Vocalization and display in the Long-tailed Ground-roller Uratelornis chimaera. Wilson J. Ornithol. 115, 193–196. doi: 10.1676/02-063
Tobias, J. A., and Seddon, N. (2009). Signal jamming mediates sexual conflict in a duetting bird. Curr. Biol. 19, 577–582. doi: 10.1016/j.cub.2009.02.036
Tobias, J. A., and Williams, R. S. R. (1996). Notes on the behaviour of the Masked Saltator in southern Ecuador. Auk 113, 942–944. doi: 10.2307/4088876
Tóth, E., and Duffy, J. E. (2005). Coordinated group response to nest intruders in social shrimp. Biol. Letts. 1, 49–52. doi: 10.1098/rsbl.2004.0237
Trivers, R. (1971). The evolution of reciprocal altruism. Q. Rev. Biol. 46, 35–57. doi: 10.1086/406755
Wickler, W. (1980). Vocal duetting and the pair bond. 1. Coyness and partner commitment—a hypothesis. Z. Tierpsychol. 52, 201–209. doi: 10.1111/j.1439-0310.1980.tb00711.x
Wiersma, P., Muñoz-Garcia, A., Walker, A., and Williams, J. B. (2007). Tropical birds have a slow pace of life. Proc. Natl. Acad. Sci. U.S.A. 104, 9340–9345. doi: 10.1073/pnas.0702212104
Williams, J. B., Miller, R. A., Harper, J. M., and Wiersma, P. (2010). Functional linkages for the pace of life, life-history, and environment in birds. Integ. Comp. Biol. 50, 855–868. doi: 10.1093/icb/icq024
Wilman, H., Belmaker, J., Simpson, J., de la Rosa, C., Rivadeneira, M. M., and Jetz, W. (2014). EltonTraits 1.0: species-level foraging attributes of the world's birds and mammals. Ecology 95, 2027. doi: 10.1890/13-1917.1
Keywords: birdsong, chorusing, cooperation, duetting, ecological competition, resource defense, sociality
Citation: Tobias JA, Sheard C, Seddon N, Meade A, Cotton AJ and Nakagawa S (2016) Territoriality, Social Bonds, and the Evolution of Communal Signaling in Birds. Front. Ecol. Evol. 4:74. doi: 10.3389/fevo.2016.00074
Received: 02 November 2015; Accepted: 08 June 2016;
Published: 24 June 2016.
Edited by:
Michelle L. Hall, University of Melbourne, AustraliaReviewed by:
Dustin R. Rubenstein, Columbia University, USAMatthew R. E. Symonds, Deakin University, Australia
Copyright © 2016 Tobias, Sheard, Seddon, Meade, Cotton and Nakagawa. This is an open-access article distributed under the terms of the Creative Commons Attribution License (CC BY). The use, distribution or reproduction in other forums is permitted, provided the original author(s) or licensor are credited and that the original publication in this journal is cited, in accordance with accepted academic practice. No use, distribution or reproduction is permitted which does not comply with these terms.
*Correspondence: Joseph A. Tobias, ai50b2JpYXNAaW1wZXJpYWwuYWMudWs=