- 1Centre for Ecological Sciences, Indian Institute of Science, Bangalore, India
- 2Department of Biology and Psychology, Ashoka University, Sonipat, India
Females of the pseudophylline bushcricket species Onomarchus uninotatus respond to a conspecific acoustic call with bouts of tremulation, followed by phonotaxis in some cases. This tremulation sends out a vibratory signal that propagates along the branch of the jackfruit trees where these animals are almost always found, and the male is able to localize the signal and perform vibrotaxis toward the female. Males are unable to localize the signal if it emanates from a branch unconnected to their perch, and therefore, female tremulation might not be a productive response when the nearest male is on an adjacent, disconnected tree. We hypothesized that female behavioral response choice between tremulation and phonotaxis might vary with distance from the caller. A semi-naturalistic experiment indicates that if the male and female are 4 m apart on a connected perch, females tremulate, and never perform phonotaxis while males perform vibrotaxis. However, at a distance of 9 m, 4 out of 10 females begin phonotaxis after a period of tremulation. We then hypothesized that features of the male call that indicate caller distance, such as call sound pressure level (SPL), might be responsible for this distance-dependent variation in the choice between phonotaxis and tremulation However, we found that at all SPLs, the female tremulates in response to male calls before attempting phonotaxis and that the probability of phonotaxis and tremulation both increased with calling song SPL. We conclude that our first hypothesis is upheld and that females do behave differently with respect to distance from the male, but that the cue affecting the distance-dependent increase in the probability of initiation of phonotaxis in female response choice is not the SPL of the male's advertisement call.
Introduction
Among acoustically communicating Ensiferans, duetting species depart from the standard Ensiferan paradigm of female phonotaxis to male calls. Among duetting Ensiferans as well as in various other lineages of duetting insects, females produce a signal in response to male calls, and male taxis to the female response call is the most common form of localization for acoustically duetting cicadas, visually duetting fireflies, and vibrationally duetting lacewings and stoneflies (Bailey, 2003).
However, other response modes exist and the relative prevalence of male and female phonotaxis varies across and even within acoustically duetting Ensiferan species (Bailey, 2003). In some species such as Elephantodeta nobilis (Bailey and Field, 2000), Amblycorypha parvipennis (Galliart and Shaw, 1996), Amblycorypha rotundifolia, Montezumina modesta, (Spooner, 1995), Microcentrum rhombifolium, Scudderia texensis, (Spooner, 1968), Steropleurus stali and Steropleurus nobrei (Hartley, 1993), Isophya rossica, Isophya stepposa, and Isophya taurica (Zhantiev and Dubrovin, 1977; Zhantiev and Korsunovskaya, 1986), the sexes can approach each other by performing mutual phonotaxis to each other's calls.
Mutual phonotaxis can occur simultaneously or in a particular order, varying with respect to distance and call sound pressure levels (SPL). For example, in Microcentrum rhombifolium, Amblycorypha oblongifolia, and Scudderia texensis, females perform phonotaxis only to low SPL calls indicative of distant males (Spooner, 1968).
In many species the male calling songs are complex, with a particular part of the call eliciting a temporally specific female acoustic response (Heller and von Helversen, 1986; Bailey and Hammond, 2003). The male call may also vary over the time course of the duetting and localization process, and with respect to the female's response. In Amblycorypha floridana and to some extent Montezumina modesta, the male produces two types of sounds, a short lisp that elicits a high SPL acoustic response from the female toward which he partially moves (Spooner, 1995), and then he produces another longer high SPL lisp which elicits a phonotactic response from the female (Spooner, 1968). This can occur in the reverse order in some species such as Scudderia texensis and Microcentrum rhombifolium, where the female partially approaches the source of the male call, by when he begins the second call type to which she produces (low intensity) acoustic responses, which then attract him to move the rest of the way toward her (Spooner, 1968). In these cases, the male and female both effectively share the burden of localization.
On the other hand, in the duetting phaneropterine Poecilimon ornatus, the male moves toward the female while she stays stationary and produces responses to the male call, and males reduce their call SPL as they approach females (Helversen et al., 2001). Likewise, Scudderia curvicauda males produce high SPL songs when low SPL answering calls are broadcast to them, and reduce their calling SPL as they do phonotaxis (Spooner, 1968). In these cases, call SPL would not be indicative of male distance.
Males of the ephippigerine species Steropleurus stali (Bateman, 2001) and Platystolus obvius (Hartley et al., 1974) increase their calling rate once the female engages in a duet, and similarly Scudderia texensis, S. furcate, and S. cuneata males increase the number of successive pulses in their songs. There is some indication that some aspects of the acoustic call may be used as a cue for distance. Male Leptophyes punctatissima only move toward females (Hartley and Robinson, 1976) whose answering call SPL is over 50 dB SPL (Zimmermann et al., 1989), and whose overall response latency falls within a narrow window of 20–50 ms relative to the male call (Robinson et al., 1986). Both the calling SPL and the overall response latency vary with respect to the distance between the duetting pair, and successful phonotaxis also varies sharply with respect to distance (Zimmermann et al., 1989). The latency of the female responses is remarkably low and reliable for each individual Leptophyes female, but the general phenomenon of species-specific latencies of female replies being necessary for male phonotaxis holds for many acoustically duetting Ensiferans (Bailey, 2003). With longer latencies, there is potential for silent satellite males to insert their own brief trigger pulses that can elicit a female acoustic response into the intervals between chirps of the male call, as is seen in Elephantodeta nobilis (Bailey and Field, 2000).
The multimodal duetting communication system of the pseudophylline bushcricket Onomarchus uninotatus involves male acoustic and female vibratory signals (Rajaraman et al., 2015). The male's acoustic call elicits bouts of tremulation from the females that have a specific temporal relationship of alternation with the acoustic chirps. This female tremulation transmits a vibrational signal along the substrate, which the male can detect and use to localize the female by performing vibrotaxis. The male performs vibrotaxis by tracking the vibrational component of the duet, but does not move toward the same vibrational signal if the acoustic component of the duet is missing (Rajaraman et al., 2015). This pattern of male vibrotaxis to the female vibrational signal is also seen in lebinthine crickets described by Ter Hofstede et al. (2015). However, the female O. uninotatus can also perform phonotaxis to the male call, after a period of tremulation (Rajaraman et al., 2015). Two possible forms of localization might therefore operate in O. uninotatus: female phonotaxis to the male acoustic call, or male vibrotaxis to the female's tremulation in response to his acoustic call, depending on the female response choice between tremulation and phonotaxis. Since a vibrational signal would not transmit well across trees and O. uninotatus is a canopy dweller, we hypothesized that the female response mode might vary with distance from the male. We also predicted that females would preferentially respond with phonotaxis to low SPL male calls (indicative of a distant male) and that tremulation would be the preferred response to high SPL calls. We first investigated the spatiotemporal dynamics of duetting in a semi-natural setup with the duetting pair initially separated by different distances, and then examined female responses to played back calls of varying SPLs.
Materials and Methods
Onomarchus uninotatus were caught as nymphs from plantations of Artocarpus sp. in Kaddari village, Karnataka, India (latitude 13°13'N, longitude 75°5'E), between December 2011 and May 2012 and then again between November 2016 and May 2018. The nymphs were reared on Artocarpus heterophyllus leaves and water in cylindrical plastic boxes (diameter 15 cm, height 17 cm) in the laboratory at the Indian Institute of Science, Bangalore at room temperature (18–24 deg C) and a natural 12 h:12 h light:dark cycle as described in Rajaraman et al. (2015). Experiments were carried out on virgin males at least 1 week after the final molt and females at least 10–15 days after the final molt, at which point they became responsive to male calling song.
The SPLs reported for the male call were measured in the wild with a handheld SPL meter (Brüel and Kjær Observer 2260, Denmark) with a 1/2 inch microphone (Brüel and Kjær 4189, Denmark, frequency response range 6 Hz−20 kHz), 0.5 m from below and behind the calling male.
Semi-natural Experiment on Duetting
An experiment on the distance dependent dynamics of duetting behavior was conducted in a semi-natural setup, with the female and male separated by a distance of either 4 or 9 m. For the 4 m setup, 7 of the 11 trials were conducted in a 4 m*3 m*3.5 m cage that was built outdoors around a 4 m long live Artocarpus heterophyllus branch. For the 9 m treatment, a 10 m*3 m*4 m cage was built outdoors around a cut Artocarpus heterophyllus branch of length 9.2 m. The branch was stripped of side-branches, and the branch split into two about 1 m from its tip. The same 9.2 m long cut branch was used in a 4 m treatment for 4 trials. After checking that there were no significant differences in the latency of female tremulation, male vibrotaxis and localization between the cut and the intact branches at 4 m across the cut and live branch trials (Supplementary Figures 1–3), these data were pooled.
The experiment was conducted in the night between 1930 and 0030 h between January 2017 and May 2018. The males and females were kept separated in the experimental arenas at least 2 h before each experimental trial in order to acclimatize them to the conditions in the arena. For each trial the males were released beforehand on the branch and the females were released at the appropriate distance after the male started calling. The sequence of events was recorded with male and female behavior separately monitored using a Canon XA-10 Professional Camcorder and Sony Handycam HDR-XR 500 in the night shot mode. The end of the trial was marked by the mutual co-localization of the pair. Only one trial was done per night and animals were not repeated across nights.
The behavior of the duetting couple was then coded by the combination of behavioral states exhibited by males and females. The combined behavioral state of the pair was assessed by analyzing the videos of each individual of the communicating pair, and marking the behavioral state demonstrated by the duetting pair during each 1 s bin. The transition probability between behavioral states was then calculated using a first order Markov model in R (Markovchain package, v. 0.6.9.10), which assumes that every state is determined only by the state preceding it, and given an input of a series of states, calculates the transition probability between behavioral states.
In order to assess the normality of data in the two groups (4 and 9 m), a Shapiro-Wilk test was performed and the data in the two groups were found to be non-normally distributed. Comparisons between responses at different distances were checked with a Wilcoxon rank sum test.
Female Responses to Varied Call SPL
An experiment investigating the effect of changing song SPL on female response choice was carried out in an anechoic chamber, on whose floor lay a T shaped structure consisting of a 1 m jackfruit branch of relatively uniform diameter nailed to the center of another 2 m long branch, with all 3 ends placed on blocks of black acoustic foam. In each trial, a randomly chosen female was placed on the end of the jackfruit branch leading to the T junction, such that either turn would lead to a 1 m long walk toward an X-mini speaker (v1.1, XMI Pvt. Ltd, Singapore, frequency range 120 Hz to 20 KHz) placed at either end of the 2 m long branch. At any given trial only one randomly selected speaker would be used to play the male call, as described in Rajaraman et al. (2015). The experiment was conducted between 2100 and 0300 h from February to May.
Females were subjected to a randomly ordered series of 8 trials, each with a different stimulus: the conspecific natural pre-recorded Onomarchus uninotatus call played back at one of the following sound pressure levels (SPL): 36, 40, 46, 56, 66, or 76 dB SPL (re. 2*10−5 N/m2); a silent control, or a heterospecific call control (Gryllacropsis call, frequency 1.7 kHz) played back at 66 dB SPL. Onomarchus uninotatus and Gryllacropsis sp. calls were sourced from those made by Diwakar and Balakrishnan (2007). The single recorded male call played back to all females in the SPL experiment (Supplementary Figure 4) was the same as that used in Rajaraman et al. (2015) and had a mean calling period of 1.203 ± 0.01 s (mean ± s.d., n = 6 chirps played on repeat), while the calling period typical of all male calls described by Diwakar and Balakrishnan (2007) was 1.17 ± 0.15 s (mean ± s.e., averaged across n = 6 animals). The number of syllables per chirp in our call was 3, which was typical of animals we heard calling in the wild, and recordings made in the wild by Diwakar and Balakrishnan (2007) indicate a mean of 2.32 ± 0.47 syllables per chirp (mean ± s.e., averaged across n = 6 animals); accordingly the average chirp duration they report is 0.15 ± 0.04 s. This is similar to the duration of 2 syllables of the chirp in the male call we played back; but with the third syllable included, the average duration of our call was 0.210 ± 0.001 s (mean ± s.d., n = 7 chirps). The syllable duration in the male call we played was 0.044 ± 0.001 s (mean ± s.d., n = 21 syllables), comparable to the duration of 0.044 ± 0.004 s (mean ± s.e., n = 6 animals) measured by Diwakar and Balakrishnan (2007). The peak calling frequency of our played back call was 3.36 kHz, and the average dominant calling frequency reported by Diwakar and Balakrishnan (2007) was 3.23 ±0. 0.1 kHz, with a bandwidth of 0.4 ± 0.06 kHz (mean ± s.e., n = 6 animals). The SPL reported in the lab experiment was measured at the female's initial location by a handheld sound level meter (Brüel and Kjær Observer 2260, Denmark) with a 1/2 inch microphone (Brüel and Kjær 4189, Denmark, frequency response range 6 Hz to 20 kHz). The call was relayed to the speaker from a laptop (Acer Aspire S3, Acer, Taiwan), and played out through Audacity free software (v. 2.1.2, GNU GPL) at a sampling rate of 44.1 kHz. The response of the animal to each stimulus was recorded for 5 min with a videocamera (Sony Handycam DCR-HC96E, Japan) in the night shot mode. Videos were digitized using Microsoft Windows Movie Maker software (v. 5.1, Microsoft Corporation, USA) onto a HP laptop (Compaq nx6320, Hewlett-Packard, USA) on which the videos remain available for reference. Consecutive trials were separated by a minimum of 5 minutes. The female was classified as having performed phonotaxis if she walked all the way to a speaker.
Differences between the numbers of animals responding to different treatments were tested with a McNemar's test, applying the Yates correction, the null hypothesis being that the females responded equally to all stimulus types. The latency of onset and the duration of tremulation and phonotaxis were noted for each treatment and comparisons were made using Welch's paired t-tests after the normal distribution of differences between treatments was checked using a Shapiro-Wilk test. To compare the number of tremulations at 40 dB SPL vs. the silent control, since the differences were not normally distributed, a Wilcoxon paired signed-rank test was performed.
Results
Female Response Choice Varies With Distance From Duetting Male Partner in Semi-natural Conditions
The results of the semi-natural experiment are depicted in an ethogram (Figure 1). The behavioral state at the beginning of the experiment always involved the female being released onto a branch, at a set distance away from the already calling male. The female would sit for a brief period of time without tremulation while the male sang, and soon after this female tremulation began. This multimodal duet was occasionally interrupted, with either pauses in the female's tremulatory response, or pauses in male call. When the male call stopped, females would sometimes stop tremulating; sometimes the female would continue to tremulate in silence (states A-C, Figures 1A,B). As with the initial experiments on female O. uninotatus (Rajaraman et al., 2015), tremulation was always her first response to the male call.
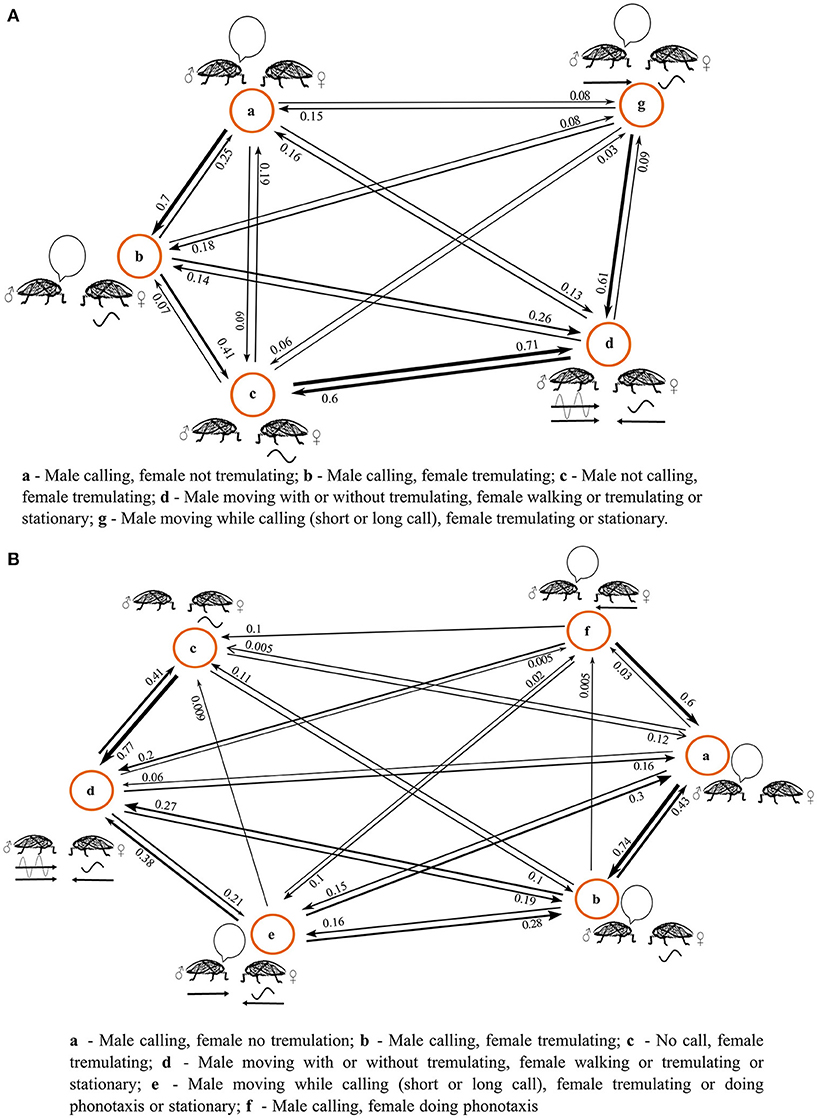
Figure 1. Ethograms of the behavior of the duetting pair at initial separation distances of (A) 4 m (n = 11) and (B) 9 m (n = 10), constructed using a First Order Markov Model. The numbers next to the arrows are probability values which give (NOT gives) the probability of transitioning from one behavior to the other. The thicknesses of the arrows are in accordance with the probability values they represent.
At a separation of 4 m, the state of male calling and female tremulation transitioned into a state where the male began vibrotaxis (n = 11) (state D, Figure 1A). In some cases, the male began vibrotaxis while continuing to call, but the call structure changed to one with a shorter number of syllables, usually just 1 syllable (Supplementary Figure 5), eventually always leading to the state of silent vibrotaxis by the male (state G, Figure 1A). The female continued to stay stationary during male vibrotaxis, sometimes tremulating when the male called, and sometimes tremulating even in the absence of the acoustic call. While the female occasionally walked around, it was never in response to the male call, and so at 4 m, females never performed phonotaxis.
The females' first response is tremulation in both the live and cut branch setups at 4 m, and no phonotaxis seen in both. Mate-search is solely performed by males in both setups. No statistically significant differences were seen in the latency of female tremulation (Supplementary Figure 1), male vibrotaxis (Supplementary Figure 2) or the latency of localization by males in the cut vs. live branch (Supplementary Figure 3), although there is a lot more variation seen in the localisation latency in the cut branch.
At a separation of 9 m, however, 4 out of 10 females performed phonotaxis. The initial part of the behavioral sequence is similar to that at 4 m, with the male calling, and the female commencing tremulation, with occasional interruptions in the duet (states A–C, Figure 1B). Males sometimes performed vibrotaxis as happened with the 4 m treatment of the semi-natural experiment, sometimes calling on the way, usually with an abbreviated call structure (states D, G, Figure 1B; Supplementary Figure 5). However, in 2 cases the females ended their tremulation and began phonotaxis while the male called, before the male commenced moving (state F, Figure 1B). In the 2 other cases, the female began phonotaxis while the male was performing vibrotaxis and, while walking, he produced the changed call (Supplementary Figure 5). In all cases where the female performed phonotaxis at 9 m, she did not walk all the way toward the male, and instead paused and tremulated in response to his call if it arrived when she was stationary.
At both 4 and 9 m, the transition probability matrix of observed behavior was found to be non random and significantly different from the expected transition probability matrix if the sequence of behavior were to be random and all transitions were equally likely, as tested using a chi square goodness of fit-test (at 4 m, χ2 = 503.1, df = 16; at 9 m χ2 = 780.3, df = 25).
Female Response Mode Does Not Change With Acoustic Call SPL
Since females showed varied responses to the male call depending on their distance from the male, female responses were tested to male acoustic calls played back at a range of sound pressure levels (SPLs). A robust tremulation response to the conspecific call was seen within the first minute of onset of the male call for all individuals at 66 dB SPL. The number of animals that respond to the conspecific call within the first minute is lower at other tested SPLs, reducing monotonically with reducing stimulus SPL. The relationship between the proportion of females out of the total number of 16 who tremulate within the first minute of the onset of the male acoustic call, and SPL, can be fit by a saturating inverse exponential relationship (Figure 2A).
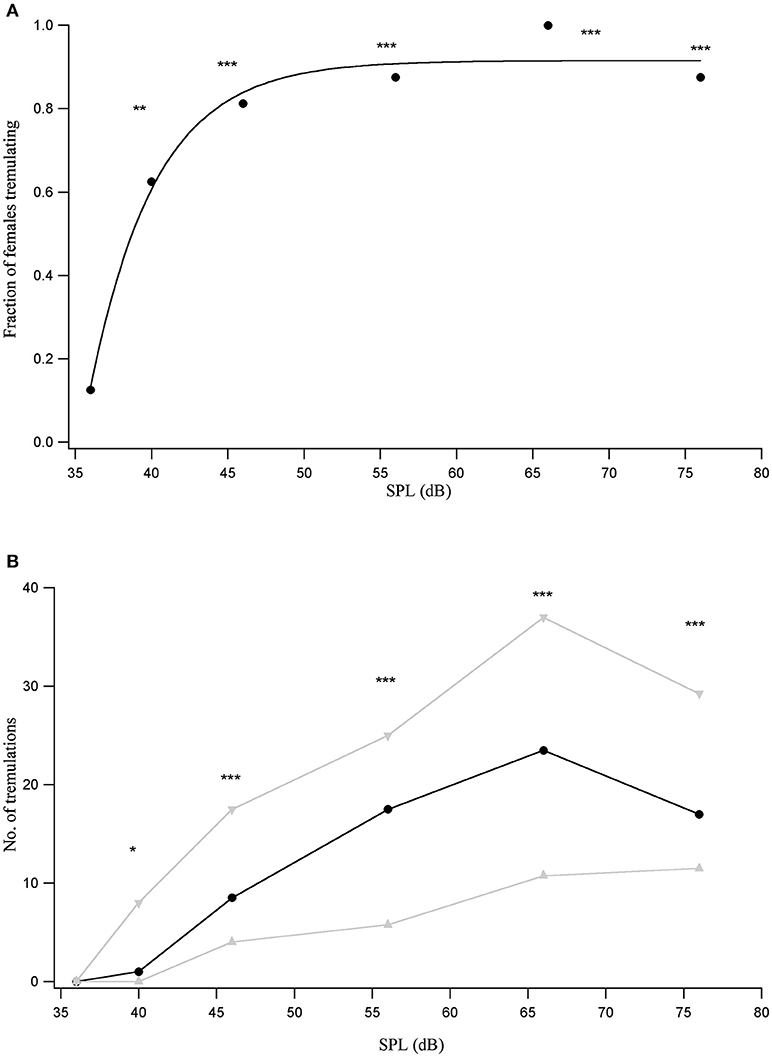
Figure 2. (A) Proportion of animals that tremulate in response to the conspecific call played back at different SPLs (n = 16). **p < 0.01, ***p < 0.001 for comparisons of response at a given SPL with the response to silent controls. (B) Median number of tremulation events (black trace) observed across animals in the first minute following the onset of the conspecific male acoustic call, played back at different SPLs, with the interquartile range indicated in gray. *p < 0.05, ***p < 0.001 for pairwise comparisons with the response to a silent control.
Females never tremulated in silence prior to being exposed to the male call. The number of females that tremulate to the 40 dB SPL playback of the conspecific call is significant compared to the number responding to silence (χ2 = 8.1, df = 1, p = 0.0044). This is also true for the number of females tremulating at all higher SPLs in comparison to the number tremulating in silence (in all cases p < 0.001). However, the number of females that tremulate at 35 dB SPL is not significantly different from the number tremulating in silence, suggesting that the threshold of the response lies between 35 and 40 dB SPL.
The median number of female tremulatory responses observed during the first minute following the onset of playback of the conspecific male acoustic call rises monotonically with conspecific stimulus SPL upto 66 dB SPL. At 76 dB SPL, the highest tested SPL, we see a decrease in the number of tremulation events, although this decrease is not significant (Figure 2B).
The number of tremulatory responses at 40 dB SPL is significant relative to the response to silence (Wilcoxon signed-rank V = 50, p = 0.01), as are the responses for 46 dB SPL and above (in all cases p < 0.001), while the response at 35 dB SPL is not significantly different from the response to silence. This corroborates the estimate of a tremulation threshold lying between 35 and 40 dB SPL.
The proportion of females out of a total of 16 that show a phonotactic response to the conspecific call is lower at each SPL than the proportion of animals that show a tremulation response. While the proportion of animals responding with phonotaxis to the conspecific call does not rise monotonically with call SPL even up to 66 dB SPL, the relationship can be fit by a saturating inverse exponential (Figure 3). The number of animals showing a phonotactic response to the conspecific call is only significantly higher than the number walking during a silent control, when the call is played back at 66 dB SPL (χ2 = 8.1, df = 1, p = 0.0044) and 76 dB SPL (χ2 = 8.1, df = 1, p = 0.0044).
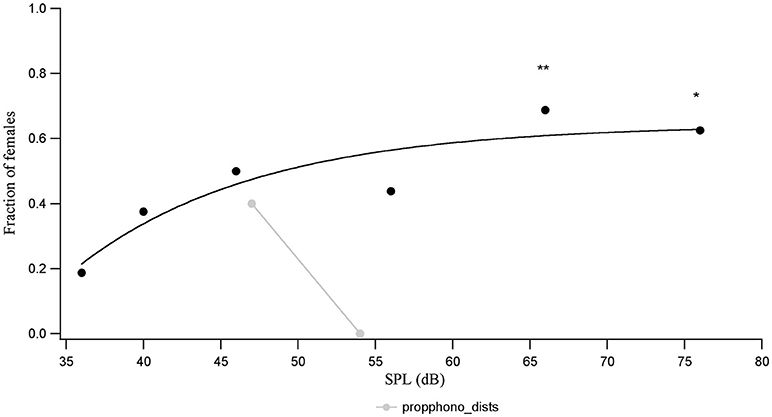
Figure 3. The proportion of animals (n = 16) who complete phonotaxis vs. conspecific call SPL, compared to the proportion of animals seen to complete phonotaxis in the semi-natural experiments at 4 and 9 m distances (corresponding to acoustic call SPLs of 54 and 47 dB SPL, respectively). *p < 0.05 and **p < 0.01 for comparisons of response at a given SPL with the response to silent controls.
The calling SPL of the males is fairly similar across individuals, measured to be about around 71.9 ± 0.5 dB SPL (n = 5) measured 50 cm from behind the animal in the field. We interpolate the call SPL that the females would hear in the semi-natural experiment using an attenuation of 6 dB SPL per doubling of distance, to find that at a 4 m horizontal distance the female would hear the male acoustic call at 54 dB SPL, while at 9 m she would hear the call at 47 dB SPL. The proportion of females doing phonotaxis at 46 dB SPL (lab experiment) and at 47 db SPL (9 m treatment, semi-natural experiment) are similar. Interestingly, however, the proportion of females doing phonotaxis at a distance of 4 m and 54 dB SPL in the semi-natural experiment is zero, unlike the proportion of females who perform phonotaxis in the lab during playback of the acoustic call at 56 dB SPL (Figure 3). This suggests that SPL of the advertising call is not the cue mediating distance dependent variations in female response choice in the semi-natural experiment.
We examined the latency of the onset of both tremulatory (Figure 4A) and phonotactic (Figure 4B) responses after song onset at different SPLs of conspecific call playback, to see whether male acoustic call SPL affected the duration for which females tremulated before beginning phonotaxis. We found no significant differences across SPLs above threshold. However at all SPLs, the latency of the first tremulation event was an order of magnitude lower than the latency of phonotaxis (Figure 4).
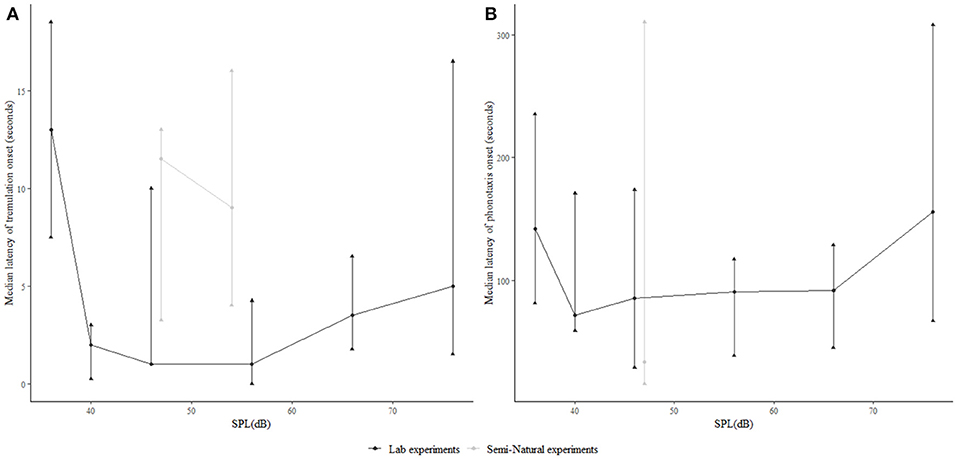
Figure 4. Relationship of the latency of (A) onset of female tremulation and (B) the onset of female phonotaxis after song onset, in the laboratory as well as semi-natural settings, relative to stimulus SPL.
Since tremulation always preceded phonotaxis, we proceeded to investigate whether vibrotaxis similarly was likely to precede phonotaxis. The time taken for a calling male to initiate vibrotaxis was assessed in the semi-natural experiment, since vibrotaxis was initiated in response to female tremulation, whereas the time taken to initiate phonotaxis was assessed in the laboratory experiment where calls were played back continuously. All vibrotaxis latencies are calculated from the onset of female tremulation, while phonotaxis latencies are calculated from the onset of the male acoustic call. At 54 dB SPL, corresponding to a 4 m distance, the time taken to initiate vibrotaxis in the semi-natural experiment was significantly shorter than the time taken to initiate phonotaxis at 56 dB SPL in lab experiments, whereas at 9 m, vibrotaxis at 47 dB SPL did not commence significantly more quickly than phonotaxis as assessed in the laboratory playback experiment at 46 dB SPL. When the semi-natural experimental trials where the female began phonotaxis were separated from trials where she did not do phonotaxis (in order to check that at all SPLs, comparisons of latency were made under conditions where the origin of the signals was stationary), there was still no significant difference in the latency of onset of male vibrotaxis and female phonotaxis at 9 m (Figure 5).
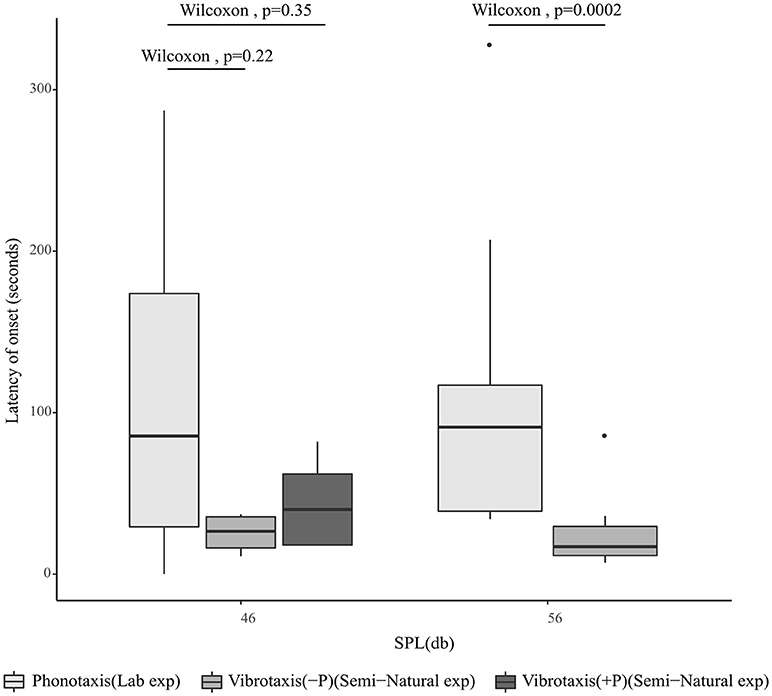
Figure 5. Median latency of onset of female phonotaxis assessed in the laboratory experiment at 46 and 56 dB SPL where the position of the sound emitting speaker does not move (n = 13), compared to time taken for the onset of male vibrotaxis as assessed in the semi-natural experiment at 4 m (54 dB SPL) (n = 11) and 9 m (47) dB SPL (n = 10). For 4 m, the source of the vibrational signal was always stationary; for 9 m, vibrotaxis data are segregated by whether the female did (+P) (n = 4) or did not perform phonotaxis (–P) (n = 6). All vibrotaxis latencies for this plot are calculated from the onset of female tremulation, while phonotaxis latencies are calculated from the onset of the male acoustic call.
Vibrotaxis is the only form of localization seen in the semi-natural experiment at a separation of 4 m, while it is the dominant form of localization at a separation of 9 m. The time taken for the completion of vibrotaxis by the male and localization of the female in the semi-natural experiment, however, is significantly higher than the latency of onset of female phonotaxis in the laboratory experiment, at both distances and SPLs (Figure 6). This suggests that at 4 m, while vibrotaxis typically begins more swiftly than phonotaxis to sound played back at an equivalent SPL, it is not completed quickly enough to explain the failure of the female to begin phonotaxis at that SPL. When vibrotaxis and phonotaxis both happen, colocalization is significantly quicker (p = 0.02) than when only one form of localization takes place.
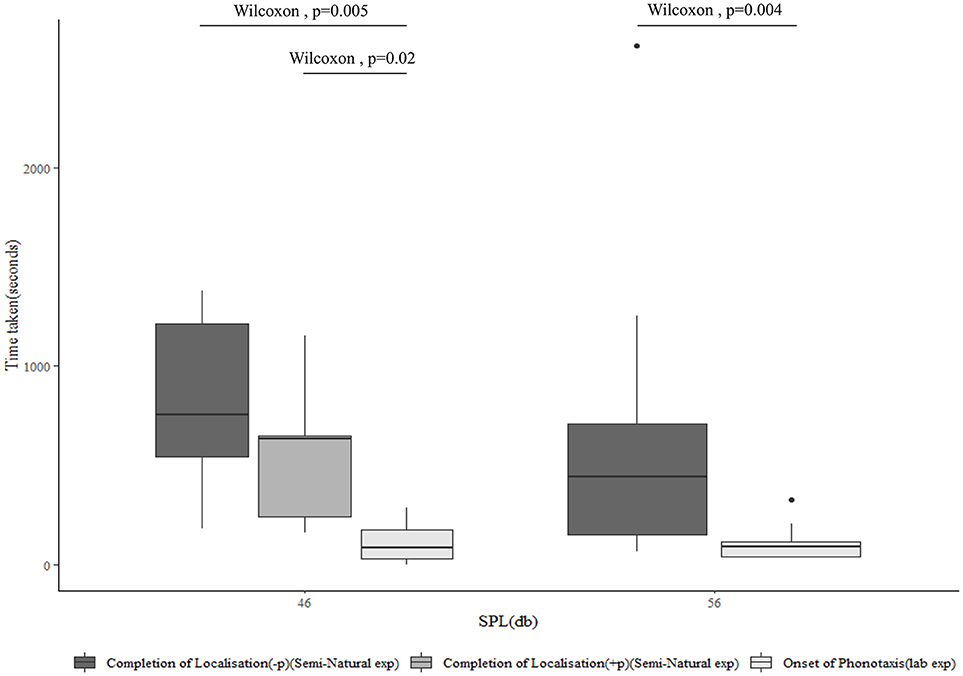
Figure 6. The median latency of onset of phonotaxis as assessed in the laboratory experiment at 46 and 56 dB SPL (n = 13), compared to time taken for the completion of vibrotaxis and localization of the female by the male as separately assessed in those treatments of the semi-natural experiment where the female does not perform phonotaxis, at 4 m (54 dB SPL) (n = 11) and 9 m (47) dB SPL (n = 10), and where the female does perform phonotaxis at 9 m (n = 4). All vibrotaxis latencies for this plot are calculated from the onset of female tremulation, while phonotaxis latencies are calculated from the onset of the male acoustic call.
Discussion
Female Response Choice Varies With Distance From Duetting Male Partner
The results of the semi-natural experiment support our hypothesis that the mode of female response and the sequence of behaviors involved in mutual localization are affected by the initial distance separating the duetting pair. In a choice between responding to the male acoustic call with tremulation and phonotaxis, it is clear that tremulation is the more likely and more immediate female behavioral response, in both the semi-natural and laboratory conditions. Only a subset of the females who tremulate perform phonotaxis. In the semi-natural condition phonotaxis occurs only at a separation of 9 m. At a separation of 4 m, however, not a single female does phonotaxis. This clearly indicates that the female employs different strategies and modes of response at different initial separation distances from the male.
Female Response Mode Does Not Vary With the SPL of the Male's Acoustic Call of Advertisement
Our second hypothesis looked at SPL. In investigating how the choice between phonotaxis and tremulation varied with stimulus SPL, we were testing the hypothesis that louder calls would preferentially elicit a tremulation response and no phonotaxis, of the kind seen at 4 m distances, while lower SPL calls would more likely elicit a phonotactic response.
We do not find support for the hypothesis that SPL affected the choice between tremulatory and phonotactic behavior because the timing of onset of both tremulation and phonotaxis did not vary significantly with SPL. Phonotaxis always followed a period of tremulation and the latency of onset did not decrease with decreasing SPL. The time taken to complete phonotaxis did not vary significantly with SPL either.
The probability of phonotaxis and the probability of tremulation both increased with SPL up to 66 dB SPL. Therefore, at high SPL calls indicating proximal male callers, a female is more likely to tremulate, and more likely to do phonotaxis. When she tremulates, the number of tremulations she performs within the first minute of the onset of the male acoustic call goes up with increasing call SPL. This is not surprising at a neurophysiological level, because all responses could be expected to increase with sensory stimulus levels. Phonotaxis in particular has been shown to generally improve with increasing stimulus levels (Ulagaraj and Walker, 1975; Walker and Forrest, 1989; Forrest and Green, 1991). But at all SPLs above threshold, the probability of female tremulation was higher than the probability of phonotaxis, suggesting that these two behaviors do not trade-off in terms of probability along a range of advertisement male acoustic calling song SPLs.
Response Threshold and Overload
An exception to the increase in probability and frequency of tremulation, and the probability of phonotaxis with SPL, is the decrease in these parameters going from 66 dB SPL to 76 dB (Figures 2, 3). A call played back at 76 dB SPL may therefore represent an example of unnatural sensory overload. Ulagaraj and Walker (1975), however, found that calls as much as 6 dB louder than the natural calling SPL of 100 dB attracted more mole cricket females of the species Neoscapteriscus borellii in sound traps than the called played at the natural SPL of 100 dB. Beyond 106 dB, the number of females attracted to the sound trap plateaued. Sensory overload may unsurprisingly differ in its attractive or aversive value between various Orthopterans.
The threshold of the tremulation response was estimated from the SPL at which both the frequency and probability of tremulation in response to the conspecific call differ significantly from behavior under the silent control condition (Figure 2). For both parameters the threshold estimated this way lay between 35 dB SPL where the response was not significantly different from the response under silence, and 40 dB SPL where the response was significantly different.
Phonotactic Suppression at Short Distances in Semi-natural Conditions
In the semi-natural experiment, as opposed to the laboratory experiment, phonotaxis is not in fact more likely as call SPL increases. Phonotaxis was never observed in the semi-natural experiment at a separation of 4 m, but was observed at a separation of 9 m. In the laboratory experiments where we manipulate acoustic call SPL, we do not see a reduced probability of phonotaxis for high-SPL calls that might indicate a nearby male. This is in contradiction with the reduced probability of phonotaxis seen in the semi-natural experiment at a shorter distance and high call SPL. The difference between these results and those showing increasing phonotaxis probability with calling SPL in the laboratory experiment suggests that the SPL of the advertisement call is not the cue mediating the difference in female response modes at different distances.
Specifically, the similarity in the probability of phonotaxis seen in natural and laboratory experimental results at 46–47 dB SPL (Figure 3), and the divergence at higher SPLs suggests that at higher SPLs, other cues indicating the proximity of the pair might reduce the probability of female phonotaxis. One such cue might be the cessation of the male call in response to female tremulation, prior to beginning vibrotaxis. While this cue differs between the laboratory and semi-natural experiments, we would expect such a cue to suppress phonotaxis both at 4 and 9 m separations. Likewise, outdoor conditions of background noise, temperature and humidity differ between the semi-natural and laboratory experiments, but would not be expected to vary between the 4 and 9 m treatments of the semi-natural experiment.
A simple possibility is that the female does not perform phonotaxis in the 4 m treatment of the semi-natural experiment because the male reaches her before she begins to move. But in two cases out of four examples of phonotaxis at a 9 m separation in the semi-natural experiment, the onset of female phonotaxis was well after the onset of male vibrotaxis. The other two examples show the onset of female phonotaxis prior to the onset of male vibrotaxis, suggesting that phonotaxis is not simply a delayed response relative to vibrotaxis, commencing only if the male fails to arrive. Furthermore, the average time taken by the male to locate the female 4 m away is significantly longer than the time it would have taken her to begin phonotaxis in the lab experiment where we played back the male calling song (Figure 5). The absence of phonotaxis at 4 m can therefore not be explained by the female waiting for the male to actually find her. This adds weight to the possibility that phonotaxis in semi-natural conditions is suppressed by cues at short distances, rather than the possibility that females simply do not commence phonotaxis at short distances because the male arrives before she would.
In all cases in the 4 m treatment of the semi-natural experiment, male vibrotaxis was likely to commence earlier than female phonotaxis latency as measured in the lab playback experiment (Figure 6). Therefore, other cues from the walking male might exert a distance dependent effect. Vibrational cues from the male, or the changes in the male acoustic calling structure while he walks, might provide cues to the female that suppress the probability of her commencing phonotaxis. All these cues are present only in the semi-natural experiment and not in the lab experiment. Since both of these decay with transmission distance, they might be of lower intensity at 9 m and exert a suppressive effect only at 4 m.
Another possibility is that olfactory or visual cues mediate male taxis toward the female at short distances. Our occasional observations of males walking toward females even before commencing a broadcasting call at short distances (1–4 m) suggests that non-auditory cues might play a role. The semi-natural experiment was conducted outdoors and so light was not completely controlled as a factor and neither was smell. However, in the laboratory playback experiment, both light and smell were controlled for. Dim light is known to affect phonotaxis in Ensiferans (Bohm et al., 1991; von Helversen and Wendler, 2000), as well as courtship song in grasshoppers (Riede, 1986).
As the male walks toward the female, the call structure changes, but it is also possible that call SPL varies—it could become louder as the male moves closer, or softer. While we have video recordings of the experiment that include an audio component, these data cannot provide a proper calibrated quantification of the change in SPL as the male moves. Our study was also limited by the relatively difficult comparison between the complexity of signaling in semi-natural settings, which is clear from the ethograms (Figure 1) and the laboratory experiments involving the manipulation of acoustic call SPL. Controlling for non-acoustic cues in the semi-natural experiment also proved to be difficult, whereas these could be controlled in the laboratory. Any vibrational non-acoustic cues from the male's walking fell below the threshold of measurement of a Polytec Laser Doppler Vibrometer Polytec Scanning Vibrometer data acquisition unit (VIB-E-220, Polytec GmbH, Waldbronn, Germany) controlled by the vibrometer software (Vibsoft version 4.8, Polytec GmbH, Waldbronn, Germany), and therefore, these could not be quantified. It must be noted that this vibrometer also fails to detect tremulations of an amplitude at which the males respond, and so the detection threshold of the males is lower and more sensitive than this vibrometer. The question of whether non-acoustic cues mediate phonotactic suppression, and if so, which cues could be investigated more precisely in further studies with more sensitive equipment that manipulate the presence or absence of each of these cues.
We conclude that female O. uninotatus show different modes of response to duetting male partners at different distances, but that acoustic call SPL is not the cue mediating this difference.
Data Availability Statement
All reported behavior has been videorecorded and a copy of these raw data in the form of videos as well as analysis is with the authors, as well as stored at the Center for Ecological Sciences, Indian Institute of Science. The raw data supporting the conclusions of this manuscript will be made available by the authors, without undue reservation, to any researcher.
Ethics Statement
This study was carried out in accordance with the regulations of the Government of India, and under the guidance of the Institutional Animal Ethics Committee of the Indian Institute of Science. The protocol was approved by the Institutional Research Ethics Committee of the Indian Institute of Science.
Author Contributions
RB, KR, and AN designed the experiments, analyzed the results, and wrote the paper together. KR performed the laboratory experiment. AN and AD performed the semi-natural experiment. All authors gave final approval for publication.
Funding
We thank the DST-SERB (through individual grant to RB) and DBT-IISc partnership program (Govt. of India) for funding the research and DST-FIST for some of the equipment used. We thank the Ministry of Human Resource development, Govt. of India for the student fellowship to AN. KR was funded by the Dr. D.S. Kothari Postdoctoral Fellowship and the DST-INSPIRE fellowship [IFA12-LSBM-43].
Conflict of Interest Statement
The authors declare that the research was conducted in the absence of any commercial or financial relationships that could be construed as a potential conflict of interest.
Acknowledgments
We thank Sudhakar Malekudiya Gowda for his help in obtaining animals for this study. We thank Ayush Verma, Chirag Chittar, Deekshith Kumar, Harin Aiyanna, Meghana Binraj, Pavan C, Nivedithaa Diwakar, Subhasmita Patro, Syed Abrar, Tanveen Randhawa, and Vinayaka Hegde for helping with the video recordings for the semi-natural experiments.
Supplementary Material
The Supplementary Material for this article can be found online at: https://www.frontiersin.org/articles/10.3389/fevo.2018.00172/full#supplementary-material
Supplementary Figure 1. The latency of female tremulation at a separation of 4 m from the male in the semi-natural experiment, using the cut vs. live branch.
Supplementary Figure 2. The latency of male vibrotaxis, at a separation of 4 m from the female in the semi-natural experiment, with the cut vs. live branch.
Supplementary Figure 3. The time taken for localization by the male of the female, from an initial separation of 4 m in the semi-natural experiment, on the cut vs. live branch.
Supplementary Figure 4. The sample recorded male call which was played back on repeat to females in the playback experiment.
Supplementary Figure 5. A sample spectrogram of the change in the male call over the course of a minute, with respect to the onset of tremulation, vibrotaxis, and phonotaxis, in the 9 m treatment of the semi-natural experiment.
References
Bailey, W. J. (2003). Insect duets: their mechanisms and underlying evolution. Physiol. Entomol. 28, 157–174. doi: 10.1046/j.1365-3032.2003.00337.x
Bailey, W. J., and Field, G. (2000). Acoustic satellite behaviour in the Australian bushcricket Elephantodeta nobilis (Phaneropterinae, Tettigoniidae, Orthoptera). Animal Behav. 59, 361–369. doi: 10.1006/anbe.1999.1325
Bailey, W. J., and Hammond, T. J. (2003). Duetting in insects – does call length influence reply latency? J. Zool. 260, 267–274. doi: 10.1017/S0952836903003728
Bateman, P. W. (2001). Changes in phonotactic behavior of a bushcricket with mating history. J. Ins. Behav. 14, 333–343. doi: 10.1023/A:1011167128430
Bohm, H., Schildberger, K., and Huber, F. (1991). Visual and acoustic course controling the cricket Gryllus bimaculatus. J. Exp. Biol. 159, 235–248.
Diwakar, S., and Balakrishnan, R. (2007). The assemblage of acoustically communicating crickets of a tropical evergreen forest in Southern India: call diversity and diel calling patterns. Bioacoustics 16, 113–135. doi: 10.1080/09524622.2007.9753571
Forrest, T. G., and Green, D. M. (1991). Sexual selection and female choice in mole crickets (Scapteriscus: Gryllotalpidae): modelling the effects of intensity and male spacing. Bioacoustics 3, 93–109. doi: 10.1080/09524622.1991.9753166
Galliart, P. L., and Shaw, K. C. (1996). The effect of variation on parameters of the male calling song of the katydid, Amblycorypha parvipennis (Orthoptera: Tettigoniidae), on female phonotaxis and phonoresponse. J. Insect Behav. 9, 841–855. doi: 10.1007/BF02208973
Hartley, J. C. (1993). Acoustic behaviour and phonotaxis in the duetting ephippigerines, Steropleurus nobrei and Steropleurus stali (Tettigoniidae). Zool. J. Linn. Soc. 107, 155–167. doi: 10.1111/j.1096-3642.1993.tb00219.x
Hartley, J. C., and Robinson, D. J. (1976). Acoustic behavior of both sexes of the speckled bushcricket Leptophyes punctatissima. Physiol. Entomol. 1, 21–25. doi: 10.1111/j.1365-3032.1976.tb00882.x
Hartley, J. C., Robinson, D. J., and Warne, A. C. (1974). Female response song in the ephippigerines Steropleurus stali and Platystolus obvius (Orthoptera: Tettigoniidae). An. Behav. 22, 382–389. doi: 10.1016/S0003-3472(74)80035-7
Heller, K. G., and von Helversen, D. (1986). Acoustic communication in phaneropterine bushcrickets: species-specific delay of female stridulatory response and matching male sensory time window. Behav. Ecol. Sociobiol. 18, 189–198. doi: 10.1007/BF00290822
Helversen, D. V., Schul, J., and Kleindienst, H. U. (2001). Male recognition mechanism for female responses implies a dilemma for their localisation in a phaneropterine bushcricket. J. Comp. Physiol. A 186, 1153–1158. doi: 10.1007/s003590000167
Rajaraman, K., Godthi, V., Pratap, R., and Balakrishnan, R. (2015). A novel acoustic-vibratory multimodal duet. J. Exp. Biol. 218, 3042–3050. doi: 10.1242/jeb.122911
Riede, K. (1986). Modification of the courtship song by visual stimuli in the grasshopper Gomphocerus rufus (Acrididae). Physiol. Entomol. 11, 61–74. doi: 10.1111/j.1365-3032.1986.tb00391.x
Robinson, D., Rheinlaender, J., and Hartley, J. C. (1986). Temporal parameters of male–female sound communication in Leptophyes punctatissima. Physiol. Entomol. 11, 317–323. doi: 10.1111/j.1365-3032.1986.tb00419.x
Spooner, J. D. (1968). Pair-forming acoustic systems of phaneropterine katydids (Orthoptera, Tettigoniidae). Animal Behav. 16, 197–212. doi: 10.1016/0003-3472(68)90001-8
Spooner, J. D. (1995). Pair-forming phonotaxic strategies of phaneropterine katydids (Tettigoniidae: Phaneropterinae). J. Orthopteran Res. 4, 127–129. doi: 10.2307/3503467
Ter Hofstede, H. M., Schöneich, S., Robillard, T., and Hedwig, B. (2015). Evolution of a communication system by sensory exploitation of startle behavior. Curr. Biol. 25, 1–8. doi: 10.1016/j.cub.2015.10.064
Ulagaraj, S. M., and Walker, T. J. (1975). Response of flying mole crickets to three parameters of synthetic songs broadcast outdoors. Nature 253, 530–531. doi: 10.1038/253530a0
von Helversen, D., and Wendler, G. (2000). Coupling of visual to auditory cues during phonotactic approach in the phaneropterine bushcricket Poecilimon affinis. J. Comp. Physiol. A 186, 729–736. doi: 10.1007/s003590000126
Walker, T. J., and Forrest, T. G. (1989). Mole cricket phonotaxis: effects of intensity to synthetic calling song (Orthoptera: Gryllotalpida: Scapteriscus acletus). Florida Entomol. 72, 655–659. doi: 10.2307/3495043
Zhantiev, R. D., and Dubrovin, N. N. (1977). Sound communication in the genus Isophya (Orthoptera, Tettigoniidae). Zool. Zh. 56, 38–51.
Zhantiev, R. D., and Korsunovskaya, O. S. (1986). Sound communication in bush crickets (Tettigoniidae, Phaneropterinae) of the European Part of the USSR. Zool. Zh. 65, 1151–1163.
Keywords: multimodal, duet, acoustic, bushcricket, katydid, Ensifera
Citation: Rajaraman K, Nair A, Dey A and Balakrishnan R (2018) Response Mode Choice in a Multimodally Duetting Paleotropical Pseudophylline Bushcricket. Front. Ecol. Evol. 6:172. doi: 10.3389/fevo.2018.00172
Received: 30 June 2018; Accepted: 08 October 2018;
Published: 30 October 2018.
Edited by:
Varvara Yu. Vedenina, Institute for Information Transmission Problems (RAS), RussiaReviewed by:
Hannah Marie Ter Hofstede, Dartmouth University, United StatesCiara Kernan, Dartmouth University, United States, in collaboration with reviewer HMTH
Klaus-Gerhard Heller, Humboldt-Universität zu Berlin, Germany
Copyright © 2018 Rajaraman, Nair, Dey and Balakrishnan. This is an open-access article distributed under the terms of the Creative Commons Attribution License (CC BY). The use, distribution or reproduction in other forums is permitted, provided the original author(s) and the copyright owner(s) are credited and that the original publication in this journal is cited, in accordance with accepted academic practice. No use, distribution or reproduction is permitted which does not comply with these terms.
*Correspondence: Kaveri Rajaraman, Yml0dHVAYXNob2thLmVkdS5pbg==
†Present Address: Kaveri Rajaraman, Ashoka University, Rajiv Gandhi Education City, P. S. Rai, Sonipat, India
‡These authors have contributed equally to this work