- 1Plant Evolutionary Ecology, Institute of Evolution and Ecology, University of Tübingen, Tübingen, Germany
- 2Biodiversity Research/Systematic Botany, Institute of Biochemistry and Biology, University of Potsdam, Potsdam, Germany
- 3Berlin-Brandenburg Institute of Advanced Biodiversity Research (BBIB), Berlin, Germany
- 4Institute for Landscape and Open Space, Hochschule für Technik HSR Rapperswil, Rapperswil, Switzerland
Soil biota involved in plant-soil feedbacks (PSFs) have an impact on the growth of plant individuals. So far, studies investigating the role of soil-biota mediated PSFs in plant performance were mostly performed in greenhouses and focused predominantly on species differences, whereas the contribution of PSFs to plant performance under field conditions and intraspecific variation in PSFs among plant populations remain poorly investigated. Here, we performed a PSF pot experiment under field conditions to study intraspecific variation in plant responses to soil biota. We used seeds from multiple seed families of Plantago lanceolata L. together with Plantago-conditioned soils from contrasting habitats (three non-fertilized pastures vs. three fertilized mown pastures) to test whether plants show a positive or negative response to their parental soil biota. We furthermore tested whether these PSFs depend on abiotic habitat factors and insect herbivory. To this end, we reciprocally transplanted plants and their soil biota between the two habitat types and excluded aboveground herbivores from half of the plants, respectively. When grown without herbivores, plants from both habitat types showed similar and neutral PSFs independently of the transplant site. In contrast, in the presence of herbivores, PSFs for plants from non-fertilized pastures were negative in both habitats (i.e., plants performed better when they grew with foreign soil biota), whereas PSFs for plants from fertilized mown-pastures remained neutral. Our results suggest that soil biota alone might only play a minor role for performance of P. lanceolata and that the outcome of soil-biota mediated PSFs is modulated by effects of herbivores in different habitats.
Introduction
For a better understanding of the interplay between ecosystem dynamics and evolution, we not only need to understand how species interact, but also how populations evolve in response to environmental drivers (Van Nuland et al., 2016; Ware et al., 2019a). The concept of eco-evolutionary feedbacks looks at the interaction and adaptation between plants and their biotic and abiotic environment (Bailey et al., 2009). So-called plant-soil feedbacks (PSFs; Bever et al., 1997) are an ideal system to investigate such eco-evolutionary dynamics, as soil microbiota have short life-cycles and can quickly adapt while plant fitness is very closely linked to its interaction with soil biota (TerHorst and Zee, 2016). PSFs influence plant biomass production and performance and thus competitiveness and coexistence of species in plant communities (Klironomos, 2002; Van der Putten et al., 2013; Heinze et al., 2015a). On top of that, other environmental players, such as herbivory or habitat, can alter PSF responses (Heinze and Joshi, 2018; Pfennigwerth et al., 2018; Ware et al., 2019b). Simultaneously, PSFs may depend on the plant genotype, and different plant populations may evolve diverging PSF responses (Bukowski and Petermann, 2014; Wagg et al., 2015; Luo et al., 2016; Allen et al., 2018; Hawkins and Crawford, 2018). Given the ecological impact of PSFs on plant communities and the substantial intraspecific variation in PSFs, there is a need for an integrated approach that considers evolutionary- and ecological-scale processes simultaneously in order to improve our understanding of PSFs.
To measure PSF effects, experiments typically compare plant growth on “home” soils (i.e., self-cultivated) to plant growth on “away” soils (i.e., non-self-cultivated; Bever et al., 1997; Brinkman et al., 2010; Van der Putten et al., 2013). PSFs are positive when plant growth is greater on home than on away soils and negative when plant growth is greater on away than on home soils (Bever, 1994). Soil biota play an important role in PSFs (Ehrenfeld et al., 2005; Brinkman et al., 2010). Negative PSFs mostly result from the harmful effects of soil pathogens and herbivores such as fungal pathogens, insect larvae or nematodes, whereas positive PSFs follow from symbioses with mycorrhizal fungi and other microorganisms such as decomposers involved in nutrient cycling (Van der Putten et al., 2016). Across the literature PSFs are predominantly negative (e.g., Kulmatiski et al., 2008) suggesting that pathogenic or parasitic soil biota accumulate over time in “home” soils. Subsequently, these negative PSFs would lead to a decrease in competitive ability (Kardol et al., 2007) and would cause local rarity (Klironomos, 2002) or even extinction in local communities when competing with species that gain positive PSFs from soil biota. Similar to species in a community, populations within a species can respond differently to their soil biota as a result of evolutionary processes (Bukowski and Petermann, 2014; Wagg et al., 2015).
Agriculturally used grasslands provide an ideal system to study intraspecific variation in PSFs. Agricultural land use affects environmental conditions belowground by influencing abiotic soil properties (Alt et al., 2011; Birkhofer et al., 2012), which in turn influences the composition of soil biota (Herold et al., 2014). Plant roots, representing a large part of a plant's biomass (Yang et al., 2010), are exposed to these soil biota and interact with them. Research on such interactions has shown that land-use mediated changes in soil biota affect plant performance and coexistence (Heinze et al., 2015a,b). Plants also alter abiotic soil properties and soil biota through litter production, nutrient-uptake and exudation processes and hence create soil-legacy effects (Ehrenfeld et al., 2005; Van der Putten et al., 2013). Such initial plant-induced changes of soil properties can then influence the establishment and growth of subsequently establishing plants (Bever, 1994; Bever et al., 1997; Kardol et al., 2007). Interestingly, long-term land-use management may elicit evolutionary responses in soil biota, plants and their interaction, which has been well investigated for plants (Turesson, 1922; Warwick and Briggs, 1979; Silvertown et al., 2006). Moreover, additional factors, such as herbivores and habitat, can be influenced by land use as well (Gardiner and Hassall, 2009; Gossner et al., 2014; Simons et al., 2014) and may have evolutionary implications for PSFs. Therefore, including multiple environmental variables may help to understand the eco-evolutionary dynamics of PSFs under realistic environmental conditions.
The majority of PSF experiments have been performed in greenhouse conditions that fail to place PSFs in the context of environmental conditions that are likely to affect the role of plant growth in communities (Heinze et al., 2016). Relative to PSFs measured in greenhouse experiments, measurements of PSFs under field conditions have been found to vary mainly due to the diverse abiotic and biotic interactions that plants and soils receive under natural field conditions (Casper et al., 2008; Heinze et al., 2016). Hence, there is a need to perform PSF experiments under field conditions (Van der Putten et al., 2016), where biotic interactions, such as herbivory, are present. Under such natural conditions recent research found, for example, that aboveground insect herbivory modulated the outcome of PSFs (Heinze and Joshi, 2018) and that the PSF response increased with the intensity of herbivory (Heinze et al., 2019).
We performed a reciprocal pot transplant experiment in the field and investigated intraspecific variation in biotic PSFs (i.e., using inoculated standardized soils) in the wide-spread plant species, Plantago lanceolata L. We included six P. lanceolata populations, three each from two contrasting habitats with contrasting land-use intensity (non-fertilized pastures vs. fertilized mown pastures). In this experiment we also tested the effect of abiotic habitat factors by reciprocally transplanting plants into the two habitat types, and the effect of herbivore presence versus absence, using a herbivory-exclosure treatment, on the outcome of intraspecific biotic PSFs.
Specifically, we asked:
1. Do P. lanceolata populations differ in biotic PSFs between the two contrasting plant origins?
2. Are biotic PSFs of P. lanceolata affected by aboveground-insect herbivory and habitat?
3. Does herbivory damage to plants vary with respect to plant and soil origin?
4. What is the relative impact of soil biota (i.e., biotic PSFs) compared to aboveground-insect herbivory and habitat on the overall biomass variation?
Materials and Methods
Study Site and Species
To test whether populations of P. lanceolata from two habitat types of contrasting land use show PSFs to local soil biota and how PSFs are affected by herbivory and abiotic habitat factors, we performed an experiment under field conditions in the UNESCO Biosphere Reserve Schorfheide-Chorin embedded in a glacial landscape in the lowlands of north-eastern Germany (Figure S1A). The experiment was conducted within the framework of the Biodiversity Exploratories, a large-scale and long-term project investigating the effects of land use on biodiversity in Germany (Fischer et al., 2010). The two contrasting habitat types—non-fertilized pastures and fertilized mown pastures—were chosen, because the factors fertilization and mowing were shown to affect the composition of soil biota (Herold et al., 2014) as well as aboveground phenotypic trait differentiation of plants (Völler et al., 2013) on the sites of the Biodiversity Exploratories. For each habitat type, three sites were chosen resulting in three pairs of non-fertilized pasture vs. fertilized mown pasture (mean geographic distance between pairs: 1.3 km, Figure S1B).
The perennial herbaceous plant species, Plantago lanceolata L., was chosen as a model organism because it is widespread in both habitat types (Joshi et al., 2001). P. lanceolata is an outbreeding (Ross, 1973) and mostly wind-pollinated (Clifford, 1962) grassland species. In previous studies P. lanceolata has been shown to exhibit strong trait differentiation and adaptation to specific land use practices (e.g. Wolff and Delden, 1987; Van Tienderen and van der Toorn, 1991; Joshi et al., 2001). For instance, in a reciprocal transplant experiment using three populations from sites with contrasting land use, fitness was always higher in the home site and fitness-related traits such as flowering time varied (Van Tienderen and van der Toorn, 1991).
Seed and Soil Collection and Preparation
Seeds of P. lanceolata were collected in summer 2017 in all six sites from five randomly chosen individual plants, which were growing at least 1 m apart from each other. Seeds collected from a single plant individual were considered a seed family. To avoid microbial contaminations, seeds were surface-sterilized using 7% sodium hypochlorite (NaClO) solution (Heinze et al., 2017). Afterwards seeds were washed with autoclaved water (20 min, 121°C), germinated in sterilized (5-times in 24 h; 20 min, 121°C) sand (grain size: 2 mm; Brun and Böhm, Potsdam, Germany) in petri dishes (diameter: 9 cm) and placed in sterile plastic chambers (32 cm × 50 cm × 14 cm; Meyer; Germany) in a greenhouse at the University of Potsdam.
To investigate the effect of local soil biota on plants from different origins we used species-specific field-conditioned soils of P. lanceolata in accordance with the “natural experiment” approach (Kulmatiski and Kardol, 2008). Previous results from the Biodiversity Exploratories have shown that the land-use treatments affected abiotic soil properties (Alt et al., 2011; Birkhofer et al., 2012) and the composition of soil biota (Herold et al., 2014) on the chosen sites. In spring 2018 on the respective sites, similar to Heinze and Joshi (2018), soil material was collected from below P. lanceolata individuals located in the center of larger patches (diameter >20 cm) of P. lanceolata to make sure that the soil was conditioned by our target species and over longer time periods in both habitats. Following Brandt et al. (2014) we collected rhizosphere soil and soil directly adjacent to the rhizosphere from 20 individuals per site. After sampling, the soil was stored for 3 weeks at 4°C in the dark until use in the experiment.
PSF Experiment
Seeds and soils of plants from six study sites were used in an experiment to investigate intraspecific variation in PSFs and how effects of land use modulate PSF responses. Within the three habitat pairs (i.e., a non-fertilized pasture and a fertilized mown pasture), rhizosphere soils from the same habitat served as “home” soil, whereas soils from the contrasting habitat served as “away” soil. As we were interested in site-specific PSFs and not within-site variation in PSFs we mixed the 20 individual soil samples to one bulk sample per site. Although this mixing procedure has been criticized for its potential to increase the likelihood of falsely detecting PSFs by decreasing variance in plant responses among individual soil samples (Reinhart and Rinella, 2016) this procedure was appropriate for our specific research question. Furthermore, several studies reported that soil mixtures produce similar PSFs compared to independent soil samples and suggest that soil handling methods should be dependent on specific research questions and feasibility (e.g., Cahill et al., 2016; Kulmatiski, 2016; Gundale et al., 2019; Teste et al., 2019). To reduce potential differences in soil nutrient availability among soils and to focus on effects of soil biota we used the collected rhizosphere soils as inoculum (10%) into an autoclaved soil:sand mixture (Brinkman et al., 2010). The soil:sand mixture consisted of a 1:1 mixture of sieved (mesh size: 7 mm) field soil collected from a meadow at the field site of the University of Potsdam (N52° 24′ 29.76″, E13° 1′ 13.74″, Brandenburg, Germany) and purchased sand (grain size: 2 mm; Brun and Böhm; Potsdam, Germany).
Pots (Deepots D25: volume 0.41 L; height 25 cm; diameter 5 cm; Stuewe and Sons; USA) were prepared with an autoclaved fleece strip (6 cm × 25 cm) covering 10 cm of the pots' inside and extending 15 cm below the pot to enable continuous watering from below. The pots were subsequently filled with the inoculated soils. To limit cross-contamination of soil biota between the pots, each pot was placed in a separate plastic cup (volume 0.3 L; height 15.2 cm; diameter 5.9 cm) and received an additional layer (1 cm) of sterilized sand on top.
In May 2018, 1-week old seedlings were transplanted into the prepared pots. The planting scheme followed a reciprocal transplant design: per habitat pair, seedlings of both habitat types were planted in their home and away soils. Each plant origin × soil origin × herbivory treatment (see below and Figure 1) combination was replicated 10 times in each site, by including two offspring each from the five randomly chosen seed families per plant origin.
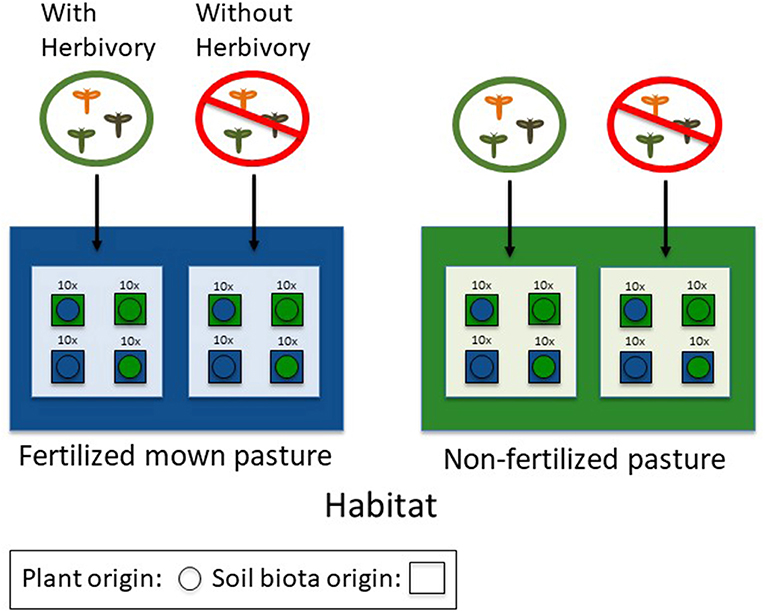
Figure 1. Conceptual diagram of the experimental design. In three pairs of contrasting habitats (non-fertilized pastures vs. fertilized mown pastures) plants (circles) of different origin were reciprocally transplanted and grown in their home and away soils (squares) in the presence and absence of aboveground insect herbivores.
After planting, seedlings were transported to a protected outdoor location on the field site of the University of Potsdam and were allowed to acclimatize for 2 weeks.
Herbivory Treatment
To investigate the impact of aboveground insect herbivory on PSFs of the different plant origins we performed a herbivory-exclusion treatment in accordance with Heinze and Joshi (2018) and Heinze et al. (2019). This herbivore-exclusion treatment was applied on all six experimental sites. In each site we established two plots (120 cm × 160 cm) that were spaced 80 cm apart. Each plot was equipped with a cage (length 160 cm × width 120 cm × height 100 cm) that was either completely covered with fly mesh (mesh size: 1.3 mm; Meyer; Germany) or only shaded by fly mesh on top. The fully covered cages excluded herbivorous insects (MacDonald and Kotanen, 2010), whereas the shaded cages allowed aboveground herbivorous insects to reach the experimental plants while providing the same levels of shade and precipitation as the cage treatment (Heinze and Joshi, 2018). In the fully covered plots, we removed the aboveground vegetation with the top 5 cm of the soil to exclude non-developed aboveground-herbivorous insects whose eggs might be attached to plants or buried in the soil. Furthermore, in the fully covered plots the fly screen was buried into the soil and one side was prepared as a door to water the plants. The fully covered plots that excluded aboveground herbivorous insects (>1.3 mm) are referred to as “without herbivory,” whereas the shaded plots are referred to as “with herbivory” treatment throughout the manuscript.
Experimental Set-Up and Measurements
After 2 weeks of acclimatization in early-June, the prepared pots were brought to the six prepared sites. At each site, plants from both habitat types were grown in their home and away soil in the presence vs. absence of aboveground herbivory (Figure 1) resulting in a total of 480 experimental plants (3 habitat pairs × 2 sites × 2 plant origins × 2 soil origins × 2 herbivory treatments × 5 seed families × 2 replicates).
As we wanted to exclude direct competition between experimental plants and neighboring plants and as we were interested in the effects of aboveground invertebrates (excluding slugs) the experimental plants were placed in boxes (78 cm × 50 cm × 30 cm). The two replicates per treatment combination were divided between the two boxes at each plot and placed at random positions within those boxes. In that way each box contained one replicate for every treatment combination, either in the with herbivory treatment or the without herbivory treatment. At all sites the experimental set-up was fenced (3 m × 3 m) to prevent damages by cattle.
During the experiment, plants were watered every third week. After 12 weeks in the field, damage by aboveground chewing insects without further discrimination of feeding guilds was visually estimated for whole plants. Afterwards shoot biomass was harvested and roots were washed. Root and shoot biomass were dried to constant weight (minimum 48 h at 80°C) and kept in the drying oven until it was weighed.
Statistical Analysis
To compare plant performance on their home vs. away soil, PSFs were calculated for each seed family individually. PSF values were calculated using total plant biomass (shoot + root). To obtain a quantitative measure of PSFs, where positive values show positive PSF and negative values negative PSF, respectively, the log ratio of home vs. away biomass was calculated (Brinkman et al., 2010). Home biomass was the biomass of a certain seed family on its own soil and away biomass was the biomass of the same seed family on the soil from the contrasting site.
Due to the random distribution of the pots in the boxes a block-wise analysis was not appropriate. Therefore, in accordance with Heinze et al. (2016) we calculated PSFs using all possible combinations of seed family-specific biomass production of one seed family in home soil in comparison to biomass production of this seed family in away soils (Equation 1).
For each replicate of the six plant origins, the home/away ratio in multiple comparisons with all 10 replicates in the same away group was calculated.
The PSF values were used in a linear mixed-effects model, testing for effects of the experimental treatments. Since the factor soil origin was already incorporated in our calculation of the PSFs, the factors plant origin (non-fertilized pasture vs. fertilized mown pasture), habitat (idem) and herbivory (with vs. without) and all possible interactions were included as fixed factors whereas habitat pair was included as a random factor.
To test for the effect of the herbivory treatment, a linear mixed-effects model was performed using the estimated percentage of feeding damage on aboveground biomass as response variable against all the factors included in our design, i.e., plant origin, habitat, soil origin and herbivory, and all possible two-way interactions. We included habitat pair and seed family as random factors in this model.
To determine the amount of variance in total plant biomass explained by the experimental treatments, a variance component analysis was performed using a linear mixed-effects model in which the same explanatory factors were included as in the model for feeding damage, but now all factors were treated as random factors.
The residuals of all models were checked for normality of distribution and homogeneity of variance. Data analysis was performed using R version 3.4.2 (R Core Team, 2017) and the lme4 package (Bates et al., 2014).
Results
Biotic PSFs Between Contrasting Plant Origins in the Absence or Presence of Aboveground Insect Herbivory
In our PSF model, plant origin as well as its interaction with the herbivory treatment had a significant influence on PSFs, while other factors remained non-significant (Table 1). To explore the environmental interactions in more detail, we performed two separate models, one using data for plants excluded from herbivory and the other on data of plants exposed to natural herbivory.
When grown without herbivores, biotic PSFs of plants from both habitat types were neutral, independently of transplant site (Table 2; Figure 2A). In the presence of aboveground insect herbivores, however, the plants from non-fertilized pastures showed negative biotic PSFs in both habitat types, whereas the PSFs for plants from fertilized mown pastures remained neutral (Table 2; Figure 2B).
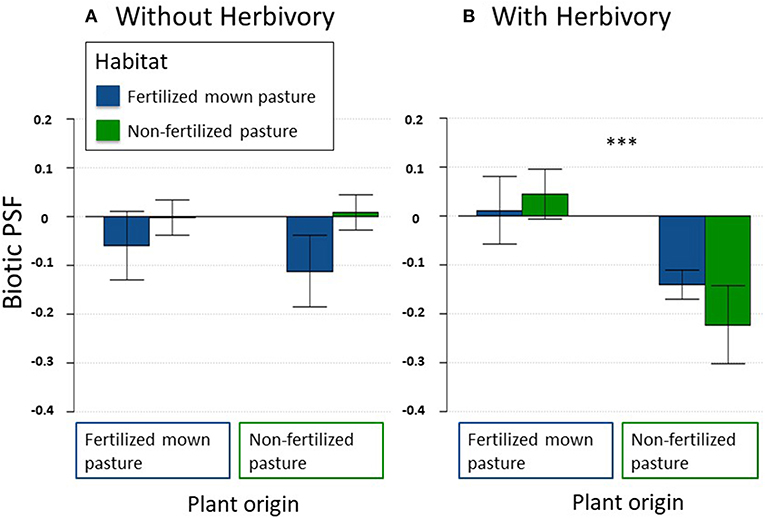
Figure 2. Biotic plant-soil feedback [PSF; log(biomass on home soil/biomass on away soil)] of P. lanceolata grown without (A) and with (B) aboveground herbivorous insects. Within graphs, left bars represent plants that originated from fertilized mown pastures, whereas right bars represent plants from non-fertilized pastures. The habitat the plants grew in during the experiment is indicated by colors (blue: fertilized mown pastures, green: non-fertilized pastures). Data represent mean ± SE (n = 30). Asteriks between bars represent signifigance, ***P < 0.001.
Herbivory Damage
In general, damage by aboveground herbivorous insects on experimental plants was low (1.02% ± 0.07, Figure 3) and was unaffected by soil biota [F(1, 340) = 0.07; P > 0.5; Table S1]. Although some feeding damage was observed in the without-herbivory plots, the percentage of aboveground feeding damage was significantly higher in the with-herbivory plots [F(340, 7) = 13.79; P = 0.0002; Table S1; Figure 3], but only in the fertilized mown pastures (Figure 3). Damage in the with- and without-herbivory plots was similar in the non-fertilized pastures (Figure 3). These different herbivory effects had, however, no significant effect on plant biomass in this experiment (see below and Table 3).
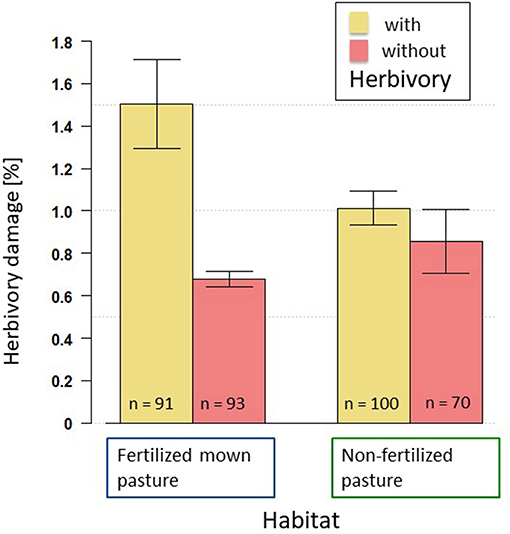
Figure 3. Visually estimated damage by aboveground insect herbivores on experimental plants in the with-herbivory plots (yellow) and without-herbivory plots (orange) grown in fertilized mown pastures (left bars) and non-fertilized pastures (right bars).
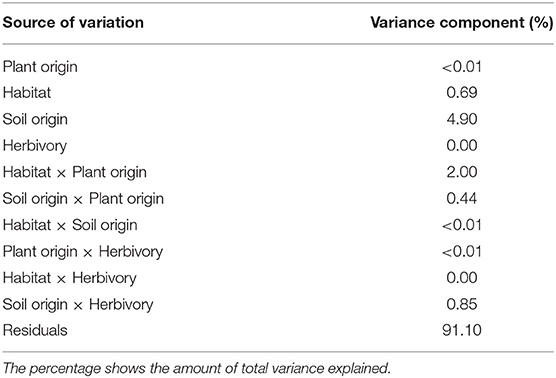
Table 3. Results of a variance component analysis for total plant biomass using a linear mixed-effects model, testing all factors included in our study design (plant origin, habitat, soil origin, and herbivory) and all possible two-way interactions.
Relative Impact of Treatments on Plant Performance
Overall, the factors –and their interactions– included in the study design together explained less than 10% of the variation in biomass of the experimental plants (Table 3). Out of these factors the origin of soil biota accounted for most of the variance in biomass production (4.9%). The interaction between plant origin and habitat accounted for 2.0% of the variance whereas all other factors and their interactions explained less than 1% (Table 3).
Discussion
PSFs Without Aboveground Herbivores
We found a main effect of plant origin on PSF as well as an interaction between plant origin and herbivory. When focusing on the results when natural aboveground insect herbivory was excluded, i.e., the sole effect of soil biota, no differences in intraspecific PSFs for populations of P. lanceolata were observed. Although only scarcely treated in the literature, there are examples of studies that found intraspecific variation in PSFs (Wagg et al., 2015; Luo et al., 2016; Eck et al., 2019). For example, Smith et al. (2012) found seedling survival of Populus angustifolia to be 2.5 times higher in soil biota from the rhizosphere of their mother tree, but neutral PSFs as well have been found (Bukowski and Petermann, 2014; Allen et al., 2018). However, our study did not test for individual effects of maternal soil, but for land-use effects (i.e., fertilization etc.) on soil biota and how different intensities of land use might influence PSFs in a population.
Fertilization is known to influence the composition of soil biota (Herold et al., 2014) and thus the relative abundance of fungi and bacteria in soils (van der Heijden et al., 2008). Although we used soils from non-fertilized vs. fertilized sites in our experiment, we did not observe differences in PSFs when aboveground insect herbivores were excluded. Either the contrasting land-use regimes did not create as much differentiation in local soil biota as expected or there were indeed differences in local soil biota, but the plants respond neutrally to them. We do not know the effects of soil biota per se, since a soil inoculum was used without an unconditioned or sterilized control soil to test against the effect of soil biota. Although the inoculation technique is optimal to investigate effects of soil biota (e.g., biotic PSFs; Brinkman et al., 2010) there are also several disadvantages. For example, inoculation into sterilized background soil might dilute the soil biota to an extent where effects, actually present in nature, are not detected anymore (Brinkman et al., 2010). Furthermore, since populations of bacteria grow much faster than those of fungi, the latter would decrease in their ratio when adding the inoculum to standardized soil. This effect could further minimize the effect of fungi in our experimental set-up. However, in contrast to other studies working on intraspecific PSFs using experimental conditioning, we used field-conditioned soil according to the “natural experiment” approach (Kulmatiski and Kardol, 2008) that is suggested to better reflect the soil communities in the field (Brinkman et al., 2010). Furthermore, with regard to our questions, the approach appeared favorable, as land use was shown to create substantial and long-lasting changes to the microbial soil community (Jangid et al., 2011; Dupouey et al., 2013) and thus soil legacies that cannot be changed via short-term conditioning with the targeted plants under artificial experimental conditions (Kulmatiski and Beard, 2011).
In general, experimental growth conditions –controlled conditions in greenhouses or climate chambers vs. field conditions– were shown to affect plant physiology (Ferreira et al., 1995) and growth (Mishra et al., 2012) as well as the development and composition of soil biota (Ge et al., 2011). In the field, harsh abiotic conditions (e.g., wind; Whitehead and Luti, 1962; Nicoll and Ray, 1996), fluctuating light regimes (Hunt and Burnett, 1973) as well as temperature (thermoperiodicity; Went, 1974) may increase biomass allocation to roots which are suggested to modulate interactions between plants and soil biota (Bardgett et al., 1998). Hence, our field experiment testing for intraspecific PSF effects fulfilled the need to consider PSFs under more natural conditions (Van der Putten et al., 2016).
The absence of a response to local soil biota under natural conditions –but without aboveground insect herbivory– does not imply that other environmental factors do not affect the performance of plants (see below). Further studies on PSFs might incorporate other important factors affecting plant growth and soil biota such as abiotic soil conditions that are influenced by land use (Alt et al., 2011; Birkhofer et al., 2012). However, we included one important environmental factor—aboveground insect herbivory—in our experiment that was shown to affect the outcome of PSFs under field conditions (Heinze and Joshi, 2018), depending on the intensity of herbivory (Heinze et al., 2019).
PSFs With Aboveground Insect Herbivory
When experimental plants were exposed to natural aboveground insect herbivory, PSFs became negative for plants from the non-fertilized pastures but remained neutral for plants from the fertilized mown pastures. Such neutral to negative PSFs were often found in grasslands (Ehrenfeld et al., 2005; Kulmatiski et al., 2008; Van der Putten et al., 2016). In our study on intraspecific variation in PSF, the performance of P. lanceolata from non-fertilized pastures increased when growing on away soils, but only when insect herbivores were present. This result indicates that P. lanceolata harbors intraspecific variation in PSF between populations with contrasting land use. This is a remarkable result given that the six study sites were located in a geographically small area and that gene flow is thus likely to be substantial among the study populations of this wind-pollinated outcrossing species. We thus propose that natural selection for differential PSF effects in the respective sites is stronger than the counteracting effects of gene flow. Simultaneously, it may be that gene flow allows the plant populations to evolve resistance against rapidly evolving antagonistic soil organisms. Both processes suggest a strong ecological significance for the observed intraspecific differences in PSF and implies that eco-evolutionary feedbacks play a role in shaping these grassland ecosystems.
The mechanism behind the negative PSF effects remains unclear. Since aboveground insect herbivory obviously has a direct effect on shoot biomass, it is important to assess whether our results could be driven purely by shoot biomass loss. When testing for an effect of soil origin under the presence of insect herbivory on shoot and root biomass separately, we found that root biomass was more strongly affected than shoot biomass (Figure S2). Moreover, aboveground herbivory itself significantly affected root biomass but not shoot biomass (Table S2). Since root biomass can only be indirectly influenced by aboveground herbivory, the observed negative PSFs on total biomass under the influence of herbivory is thus—at least in part—indirectly caused by aboveground herbivory and seems to involve changes in biomass allocation by the plant as the integrative result of both soil biota and insect herbivory.
Similarly, Mursinoff and Tack (2017) found that the response of P. lanceolata to local vs. foreign soil differed under the presence of a specialist leaf pathogen. Also Zhu et al. (2018) found similar effects in these plant-soil-herbivore interactions in P. lanceolata. Interestingly, some studies revealed that herbivory can change plant-soil-fungi interactions (Bardgett and Wardle, 2003; Bennett et al., 2009; Kostenko et al., 2012), while other studies demonstrated effects of plant-soil fungi interactions on herbivory (Fontana et al., 2009). From our results we cannot infer the exact mechanisms acting here, i.e., how the three parties are influencing each other. Further experiments are needed to elucidate the causal directions between herbivory and soil biota through P. lanceolata in our system and to understand the evolutionary drivers shaping this intraspecific variation in PSF. We speculate that the negative relationship between insect abundance and land-use intensity (Simons et al., 2017) as well as the negative relationship between symbiotic soil fungi and land-use intensity (de Vries et al., 2006) may act as selection pressures causing the observed intraspecific variation in PSF.
An important result is that insect herbivory was needed to elicit differential responses to soil origin between plants from different habitats. Although strictly speaking PSF solely involves plants and their soil biota and is ideally measured under controlled experimental conditions such as a greenhouse, it is clear that such approaches take plants and their associated soil biota out of their natural ecological context. By conducting PSF experiments under more natural conditions, we may be better able to assess the significance of various ecological drivers and their interactions, although the complexity of such experiments increases as well.
Relative Effect of Treatments on Plant Performance
With 4.9%, soil differences explained a small, but nevertheless the biggest part of total variance in our variance component analysis. Interestingly, differences in habitat only accounted for 0.7% of explained intraspecific variation in P. lanceolata. Assuming that land use is the primary driver of the differences in soil biota, this would imply that results of reciprocal transplant experiments may be driven substantially by soil biota rather than abiotic habitat effects. This is interesting given that data on the importance of climate vs. soil in plant local adaptation is scarce (Macel et al., 2007). However, it should be noted that the six study sites were located in a geographically small area and variation in microclimate among the habitats is therefore presumably small and solely influenced by land-use effects on biotic factors such as vegetation height. The interaction between habitat and plant origin accounted for 2% of the explained variation. Although such an interaction indicates variable responses of plants from different origins when transplanted to different habitats and may suggest local (mal-)adaptation (Kawecki and Ebert, 2004), this factor was not significant in our PSF model (Table 1) and does not reflect a consistent pattern among habitat pairs.
Conclusion
Our experiment investigated the effects of soil biota on intraspecific variation among plant populations under natural conditions. We did not consider plants and their soils in isolation, but included their interaction with other biotic and abiotic factors. Our study therefore contributes to the growing literature considering eco-evolutionary feedbacks to explain how complex interactions between multiple parties influence ecological and evolutionary dynamics (Van Nuland et al., 2016; Ware et al., 2019a).
The most interesting finding of our study is that intraspecific differences between populations of P. lanceolata from sites with contrasting land-use intensity only became apparent when plants were exposed to insect herbivory. Thus, our study offers support to the theory that interactions between plants and soil-microbiota can be mediated through aboveground-herbivores and the responses they induce in plants. Additionally, our study is among the first to show such complex interactions under field conditions.
Data Availability Statement
The datasets generated for this study are available on request to the corresponding author.
Author Contributions
AK, JJ, JS, and JH designed the study. LK, AK, JS, and JH collected seeds and soils, performed the experiment, and measured the data. LK, JS, and JH analyzed the data and wrote the first draft of the manuscript. LK, AK, JJ, OB, JS, and JH contributed to later versions of the manuscript.
Funding
This work was funded by the DFG Priority Program 1374 Infrastructure-Biodiversity-Exploratories (JJ and JH: JO 777/9-1; LK, AK, OB, and JS: SCHE 1899/1-1).
Conflict of Interest
The authors declare that the research was conducted in the absence of any commercial or financial relationships that could be construed as a potential conflict of interest.
The handling editor declared a past co-authorship with one of the authors JH.
Acknowledgments
We would like to thank Nils Landmann from the ABP Landwirtschaft Neugrimnitz and colleagues as well as Torsten Meene, Markus Rubenbauer and Uta Schuhmacher for help in the field and organization. We also thank the manager of the Exploratory Schorfheide-Chorin, Miriam Teuscher, and all former managers for their work in maintaining the plot and project infrastructure, Christiane Fischer for giving support through the central office, Andreas Ostrowski for managing the central data base, and Markus Fischer, Eduard Linsenmair, Dominik Hessenmöller, Daniel Prati, Ingo Schöning, François Buscot, Ernst-Detlef Schulze, Wolfgang W. Weisser and the late Elisabeth Kalko for their role in setting up the Biodiversity Exploratories project. Field work permits were issued by the responsible state environmental offices of Brandenburg.
Supplementary Material
The Supplementary Material for this article can be found online at: https://www.frontiersin.org/articles/10.3389/fevo.2019.00422/full#supplementary-material
References
Allen, W. J., Meyerson, L. A., Flick, A. J., and Cronin, J. T. (2018). Intraspecific variation in indirect plant-soil feedbacks influences a wetland plant invasion. Ecology 99, 1430–1440. doi: 10.1002/ecy.2344
Alt, F., Oelmann, Y., Herold, N., Schrumpf, M., and Wilcke, W. (2011). Phosphorus partitioning in grassland and forest soils of Germany as related to land-use type, management intensity, and land use-related pH. J. Plant Nutr. Soil Sci. 174, 195–209. doi: 10.1002/jpln.201000142
Bailey, J. K., Schweitzer, J. A., Úbeda, F., Koricheva, J., LeRoy, C. J., Madritch, M. D., et al. (2009). From genes to ecosystems: a synthesis of the effects of plant genetic factors across levels of organization. Philos. Trans. R. Soc. B Biol. Sci. 364, 1607–1616. doi: 10.1098/rstb.2008.0336
Bardgett, R. D., and Wardle, D. A. (2003). Herbivore-mediated linkages between aboveground and belowground communities. Ecology 84, 2258–2268. doi: 10.1890/02-0274
Bardgett, R. D., Wardle, D. A., and Yeates, G. W. (1998). Linking above-ground and below-ground interactions: How plant responses to foliar herbivory influence soil organisms. Soil Biol. Biochem. 30, 1867–1878. doi: 10.1016/S0038-0717(98)00069-8
Bates, D., Mächler, M., Bolker, B., and Walker, S. (2014). Fitting Linear Mixed-Effects Models using lme4. J. Stat. Softw. 67, 1–48. doi: 10.18637/jss.v067.i01
Bennett, A. E., Bever, J. D., and Deane Bowers, M. (2009). Arbuscular mycorrhizal fungal species suppress inducible plant responses and alter defensive strategies following herbivory. Oecologia 160, 771–779. doi: 10.1007/s00442-009-1338-5
Bever, J. D. (1994). Feeback between plants and their soil communities in an old field community. Ecology 75, 1965–1977. doi: 10.2307/1941601
Bever, J. D., Westover, K. M., and Antonovics, J. (1997). Incorporating the soil community into plant population dynamics : the utility of the feedback approach. Society 85, 561–573. doi: 10.2307/2960528
Birkhofer, K., Schöning, I., Alt, F., Herold, N., Klarner, B., Maraun, M., et al. (2012). General relationships between abiotic soil properties and soil biota across spatial scales and different land-use types. PLoS ONE 7:e43292. doi: 10.1371/journal.pone.0043292
Brandt, A. J., del Pino, G. A., and Burns, J. H. (2014). Experimental protocol for manipulating plant-induced soil heterogeneity. J. Visual. Exp. 85:e51580. doi: 10.3791/51580
Brinkman, P. E., Van der Putten, W. H., Bakker, E. J., and Verhoeven, K. J. F. (2010). Plant-soil feedback: experimental approaches, statistical analyses and ecological interpretations. J. Ecol. 98, 1063–1073. doi: 10.1111/j.1365-2745.2010.01695.x
Bukowski, A. R., and Petermann, J. S. (2014). Intraspecific plant-soil feedback and intraspecific overyielding in Arabidopsis thaliana. Ecol. Evol. 4, 2533–2545. doi: 10.1002/ece3.1077
Cahill, J. F., Cale, J. A., Karst, J., Bao, T., Pec, G. J., and Erbilgin, N. (2016). No silver bullet: different soil handling techniques are useful for different research questions, exhibit differential type I and II error rates, and are sensitive to sampling intensity. New Phytologist. 216, 11–14. doi: 10.1111/nph.14141
Casper, B. B., Bentivenga, S. P., Ji, B., Doherty, J. H., Edenborn, H. M., and Gustafson, D. J. (2008). Plant soil feedback: testing the generality with the same grasses in serpentine and prairie soils. Ecology 89, 2154–2164. doi: 10.1890/07-1277.1
Clifford, H. T. (1962). Insect pollination of Plantago lanceolata L. Nature 193, 196. doi: 10.1038/193196a0
de Vries, F. T., Hoffland, E., van Eekeren, N., Brussaard, L., and Bloem, J. (2006). Fungal/bacterial ratios in grasslands with contrasting nitrogen management. Soil Biol. Biochem. 38, 2092–2103. doi: 10.1016/j.soilbio.2006.01.008
Dupouey, A. J. L., Dambrine, E., Laffite, J. D., and Moares, C. (2013). Irreversible impact of past land use on forest soils and biodiversity. Ecology 83, 2978–2984. doi: 10.1890/0012-9658(2002)083[2978:IIOPLU]2.0.CO;2
Eck, J. L., Stump, S. M., Delavaux, C. S., Mangan, S. A., and Comita, L. S. (2019). Evidence of within-species specialization by soil microbes and the implications for plant community diversity. Proc. Natl. Acad. Sci. U.S.A. 116, 7371–7376. doi: 10.1073/pnas.1810767116
Ehrenfeld, J. G., Ravit, B., and Elgersma, K. (2005). Feedback in the plant-soil system. Annu. Rev. Environ. Resour. 30, 75–115. doi: 10.1146/annurev.energy.30.050504.144212
Ferreira, J. F., Simon, J. E., and Janick, J. (1995). Developmental studies of Artemisia annua: flowering and artemisinin production under greenhouse and field conditions. Planta Med. 61, 167–170. doi: 10.1055/s-2006-958040
Fischer, M., Bossdorf, O., Gockel, S., Hänsel, F., Hemp, A., Hessenmöller, D., et al. (2010). Implementing large-scale and long-term functional biodiversity research: the Biodiversity Exploratories. Basic Appl. Ecol. 11, 473–485. doi: 10.1016/j.baae.2010.07.009
Fontana, A., Reichelt, M., Hempel, S., Gershenzon, J., and Unsicker, S. B. (2009). The effects of arbuscular mycorrhizal fungi on direct and indirect defense metabolites of Plantago lanceolata L. J. Chem. Ecol. 35, 833–843. doi: 10.1007/s10886-009-9654-0
Gardiner, T., and Hassall, M. (2009). Does microclimate affect grasshopper populations after cutting of hay in improved grassland? J. Insect Conserv. 13, 97–102. doi: 10.1007/s10841-007-9129-y
Ge, T., Nie, S., Wu, J., Shen, J., Xiao, H., Tong, C., et al. (2011). Chemical properties, microbial biomass, and activity differ between soils of organic and conventional horticultural systems under greenhouse and open field management: a case study. J. Soils Sediments 11, 25–36. doi: 10.1007/s11368-010-0293-4
Gossner, M. M., Weisser, W. W., and Meyer, S. T. (2014). Invertebrate herbivory decreases along a gradient of increasing land-use intensity in German grasslands. Basic Appl. Ecol. 15, 347–352. doi: 10.1016/j.baae.2014.03.003
Gundale, M. J., Wardle, D. A., Kardol, P., and Nilsson, M. C. (2019). Comparison of plant–soil feedback experimental approaches for testing soil biotic interactions among ecosystems. New Phytol. 221, 577–587. doi: 10.1111/nph.15367
Hawkins, A. P., and Crawford, K. M. (2018). Interactions between plants and soil microbes may alter the relative importance of intraspecific and interspecific plant competition in a changing climate. AoB Plants 10:ply039. doi: 10.1093/aobpla/ply039
Heinze, J., Bergmann, J., Rillig, M. C., and Joshi, J. (2015a). Negative biotic soil-effects enhance biodiversity by restricting potentially dominant plant species in grasslands. Perspect. Plant Ecol. Evol. Syst. 17, 227–235. doi: 10.1016/j.ppees.2015.03.002
Heinze, J., Gensch, S., Weber, E., and Joshi, J. (2017). Soil temperature modifies effects of soil biota on plant growth. J. Plant Ecol. 10, 808–821. doi: 10.1093/jpe/rtw097
Heinze, J., and Joshi, J. (2018). Plant–soil feedback effects can be masked by aboveground herbivory under natural field conditions. Oecologia 186, 235–246. doi: 10.1007/s00442-017-3997-y
Heinze, J., Simons, N. K., Seibold, S., Wacker, A., Weithoff, G., Gossner, M. M., et al. (2019). The relative importance of plant-soil feedbacks for plant-species performance increases with decreasing intensity of herbivory. Oecologia 190, 651–664. doi: 10.1007/s00442-019-04442-9
Heinze, J., Sitte, M., Schindhelm, A., Wright, J., and Joshi, J. (2016). Plant-soil feedbacks: a comparative study on the relative importance of soil feedbacks in the greenhouse versus the field. Oecologia 181, 559–569. doi: 10.1007/s00442-016-3591-8
Heinze, J., Werner, T., Weber, E., Rillig, M. C., and Joshi, J. (2015b). Soil biota effects on local abundances of three grass species along a land-use gradient. Oecologia, 179, 249–259. doi: 10.1007/s00442-015-3336-0
Herold, N., Schöning, I., Gutknecht, J., Alt, F., Boch, S., Müller, J., et al. (2014). Soil property and management effects on grassland microbial communities across a latitudinal gradient in Germany. Appl. Soil Ecol. 73, 41–50. doi: 10.1016/j.apsoil.2013.07.009
Hunt, R., and Burnett, J. A. (1973). The effects of light intensity and external potassium level on root/shoot ratio and rates of potassium uptake in perennial ryegrass (Lolium perenne L.). Ann. Bot. 37, 519–537. doi: 10.1093/oxfordjournals.aob.a084718
Jangid, K., Williams, M. A., Franzluebbers, A. J., Schmidt, T. M., Coleman, D. C., and Whitman, W. B. (2011). Land-use history has a stronger impact on soil microbial community composition than aboveground vegetation and soil properties. Soil Biol. Biochem. 43, 2184–2193. doi: 10.1016/j.soilbio.2011.06.022
Joshi, J., Schmid, B., Caldeira, M. C., Dimitrakopoulos, P. G., Good, J., Harris, R., et al. (2001). Local adaptation enhances performance of common plant species. Ecol. Lett. 4, 536–544. doi: 10.1046/j.1461-0248.2001.00262.x
Kardol, P., Cornips, N. J., Van Kempen, M. M. L., Bakx-Schotman, J. M. T., and Van Der Putten, W. H. (2007). Microbe-mediated plant-soil feedback causes historical contingency effects in plant community assembly. Ecol. Monogr. 77, 147–162. doi: 10.1890/06-0502
Kawecki, T. J., and Ebert, D. (2004). Conceptual issues in local adaptation. Ecol. Lett. 7, 1225–1241. doi: 10.1111/j.1461-0248.2004.00684.x
Klironomos, J. N. (2002). Feedback with soil biota contributes to plant rarity and invasiveness in communities. Nature 417, 67–70. doi: 10.1038/417067a
Kostenko, O., van de Voorde, T. F., Mulder, P. P. J., van der Putten, W. H., and Martijn Bezemer, T. (2012). Legacy effects of aboveground-belowground interactions. Ecol. Lett. 15, 813–821. doi: 10.1111/j.1461-0248.2012.01801.x
Kulmatiski, A. (2016). Factorial and ‘self vs. other' plant soil feedback experiments produce similar predictions of plant growth in communities. Plant Soil 408, 485–492. doi: 10.1007/s11104-016-2946-6
Kulmatiski, A., and Beard, K. H. (2011). Long-term plant growth legacies overwhelm short-term plant growth effects on soil microbial community structure. Soil Biol. Biochem. 43, 823–830. doi: 10.1016/j.soilbio.2010.12.018
Kulmatiski, A., Beard, K. H., Stevens, J. R., and Cobbold, S. M. (2008). Plant-soil feedbacks: a meta-analytical review. Ecol. Lett. 11, 980–992. doi: 10.1111/j.1461-0248.2008.01209.x
Kulmatiski, A., and Kardol, P. (2008). Getting plant–soil feedbacks out of the greenhouse: experimental and conceptual approaches. Progr. Bot. doi: 10.1007/978-3-540-72954-9_18
Luo, W., Callaway, R. M., and Atwater, D. Z. (2016). Intraspecific diversity buffers the inhibitory effects of soil biota. Ecology 97, 1913–1918. doi: 10.1002/ecy.1469
MacDonald, A. A. M., and Kotanen, P. M. (2010). The effects of disturbance and enemy exclusion on performance of an invasive species, common ragweed, in its native range. Oecologia 162, 977–986. doi: 10.1007/s00442-009-1557-9
Macel, M., Lawson, C. S., Mortimer, S. R., Šmilauerova, M., Bischoff, A., Crémieux, L., et al. (2007). Climate vs. soil factors in local adaptation of two common plant species. Ecology 88, 424–433. doi: 10.1890/0012-9658(2007)88[424:CVSFIL]2.0.CO;2
Mishra, Y., Johansson Jänkänpää, H., Kiss, A. Z., Funk, C., Schröder, W. P., and Jansson, S. (2012). Arabidopsis plants grown in the field and climate chambers significantly differ in leaf morphology and photosystem components. BMC Plant Biol. 12:6. doi: 10.1186/1471-2229-12-6
Mursinoff, S., and Tack, A. J. M. (2017). Spatial variation in soil biota mediates plant adaptation to a foliar pathogen. New Phytol. 214, 644–654. doi: 10.1111/nph.14402
Nicoll, B. C., and Ray, D. (1996). Adaptive growth of tree root systems in response to wind action and site conditions. Tree Physiol. 16, 891–898. doi: 10.1093/treephys/16.11-12.891
Pfennigwerth, A. A., Van Nuland, M. E., Bailey, J. K., and Schweitzer, J. A. (2018). Plant–soil feedbacks mediate shrub expansion in declining forests, but only in the right light. J. Ecolo. 106, 179–194. doi: 10.1111/1365-2745.12833
Reinhart, K. O., and Rinella, M. J. (2016). A common soil handling technique can generate incorrect estimates of soil biota effects on plants. New Phytol. 210, 786–789. doi: 10.1111/nph.13822
Ross, M. D. (1973). Inheritance of self-incompatibility in Plantago lanceolata. Heredity 30, 169–176. doi: 10.1038/hdy.1973.19
Silvertown, J., Poulton, P., Johnston, E., Edwards, G., Heard, M., and Biss, P. M. (2006). The park grass experiment 1856-2006: its contribution to ecology. J. Ecol. 94, 801–814. doi: 10.1111/j.1365-2745.2006.01145.x
Simons, N. K., Gossner, M. M., Lewinsohn, T. M., Boch, S., Lange, M., Müller, J., et al. (2014). Resource-mediated indirect effects of grassland management on arthropod diversity. PLoS ONE 9:e107033. doi: 10.1371/journal.pone.0107033
Simons, N. K., Lewinsohn, T., Blüthgen, N., Buscot, F., Boch, S., Daniel, R., et al. (2017). Contrasting effects of grassland management modes on species-abundance distributions of multiple groups. Agric. Ecosyst. Environ. 237, 143–153. doi: 10.1016/j.agee.2016.12.022
Smith, D. S., Schweitzer, J. A., Turk, P., Bailey, J. K., Hart, S. C., Shuster, S. M., et al. (2012). Soil-mediated local adaptation alters seedling survival and performance. Plant Soil 352, 243–251. doi: 10.1007/s11104-011-0992-7
TerHorst, C. P., and Zee, P. C. (2016). Eco-evolutionary dynamics in plant–soil feedbacks. Funct. Ecol. 30, 1062–1072. doi: 10.1111/1365-2435.12671
Teste, F. P., Kardol, P., Turner, B. L., Wardle, D. A., Zemunik, G., Renton, M., et al. (2019). Toward more robust plant-soil feedback research: comment. Ecology. 100:e02590. doi: 10.1002/ecy.2590
Turesson, G. (1922). The genotypical response of the plant species to the habitat. Hereditas 3, 211–350. doi: 10.1111/j.1601-5223.1922.tb02734.x
van der Heijden, M. G., Bardgett, R. D., and van Straalen, N. M. (2008). The unseen majority: soil microbes as drivers of plant diversity and productivity in terrestrial ecosystems. Ecol. Lett. 11, 296–310. doi: 10.1111/j.1461-0248.2007.01139.x
Van der Putten, W. H., Bardgett, R. D., Bever, J. D., Bezemer, T. M., Casper, B. B., Fukami, T., et al. (2013). Plant-soil feedbacks: The past, the present and future challenges. J. Ecol. 101, 265–276. doi: 10.1111/1365-2745.12054
Van der Putten, W. H., Bradford, M. A., Pernilla Brinkman, E., van de Voorde, T. F. J., and Veen, G. F. (2016). Where, when and how plant–soil feedback matters in a changing world. Funct. Ecol. 30, 1109–1121. doi: 10.1111/1365-2435.12657
Van Nuland, M. E., Wooliver, R. C., Pfennigwerth, A. A., Read, Q. D., Ware, I. M., Mueller, L., et al. (2016). Plant-soil feedbacks: connecting ecosystem ecology and evolution. Funct. Ecol. 30, 1032–1042. doi: 10.1111/1365-2435.12690
Van Tienderen, P. H., and van der Toorn, J. (1991). Genetic differentiation between populations of Plantago lanceolata. I. Local adaptation in three contrasting habitats. J. Ecol. 79, 27–42. doi: 10.2307/2260782
Völler, E., Auge, H., Bossdorf, O., and Prati, D. (2013). Land use causes genetic differentiation of life-history traits in Bromus hordeaceus. Glob. Chang. Biol. 19, 892–899. doi: 10.1111/gcb.12087
Wagg, C., Schneider, S., Widmer, F., and Van Der Heijden, M. G. A. (2015). Intraspecific and intergenerational differences in plant-soil feedbacks. Oikos 124, 994–1004. doi: 10.1111/oik.01743
Ware, I. M., Fitzpatrick, C. R., Senthilnathan, A., Bayliss, S. L. J., Beals, K. K., Mueller, L. O., et al. (2019a). Feedbacks link ecosystem ecology and evolution across spatial and temporal scales: Empirical evidence and future directions. Funct. Ecol. 33, 31–42. doi: 10.1111/1365-2435.13267
Ware, I. M., Van Nuland, M. E., Schweitzer, J. A., Yang, Z., Schadt, C. W., Sidak-Loftis, L. C., et al. (2019b). Climate-driven reduction of genetic variation in plant phenology alters soil communities and nutrient pools. Glob. Chang. Biol. 25, 1514–1528. doi: 10.1111/gcb.14553
Warwick, S. I., and Briggs, D. (1979). The Genecology of Lawn Weeds. New Phytol. 83, 509–536. doi: 10.1111/j.1469-8137.1979.tb07616.x
Went, F. W. (1974). Reflections and speculations. Annu. Rev. Plant Physiol. 25, 1–26. doi: 10.1146/annurev.pp.25.060174.000245
Whitehead, F. H., and Luti, R. (1962). Experimental studies of the effect of wind on plant growth and anatomy. New Phytol. 61, 56–58. doi: 10.1111/j.1469-8137.1962.tb06273.x
Wolff, K., and Delden, W. Van. (1987). Genetic analysis of ecological relevant morphological variability in Plantago lanceolata L. I population characteristics. Heredity 58, 183–192. doi: 10.1007/BF00289397
Yang, Y., Fang, J., Ma, W., Guo, D., and Mohammat, A. (2010). Large-scale pattern of biomass partitioning across China's grasslands. Global Ecol. Biogeogr. 19, 268–277. doi: 10.1111/j.1466-8238.2009.00502.x
Keywords: aboveground-insect herbivory, eco-evolutionary feedback, intraspecific variation, land use, plant-soil feedback, reciprocal transplant experiment, soil biota
Citation: Kirchhoff L, Kirschbaum A, Joshi J, Bossdorf O, Scheepens JF and Heinze J (2019) Plant-Soil Feedbacks of Plantago lanceolata in the Field Depend on Plant Origin and Herbivory. Front. Ecol. Evol. 7:422. doi: 10.3389/fevo.2019.00422
Received: 14 June 2019; Accepted: 21 October 2019;
Published: 07 November 2019.
Edited by:
Justin Wright, Duke University, United StatesReviewed by:
Joe Bailey, The University of Tennessee, Knoxville, United StatesKerri Crawford, University of Houston, United States
Copyright © 2019 Kirchhoff, Kirschbaum, Joshi, Bossdorf, Scheepens and Heinze. This is an open-access article distributed under the terms of the Creative Commons Attribution License (CC BY). The use, distribution or reproduction in other forums is permitted, provided the original author(s) and the copyright owner(s) are credited and that the original publication in this journal is cited, in accordance with accepted academic practice. No use, distribution or reproduction is permitted which does not comply with these terms.
*Correspondence: Leah Kirchhoff, bGVhaC5raXJjaGhvZmZAZ214LmRl
†These authors share last authorship
‡ORCID: Leah Kirchhoff orcid.org/0000-0002-5411-8480
Anna Kirschbaum orcid.org/0000-0002-9379-8765
Jasmin Joshi orcid.org/0000-0002-4210-2465
Oliver Bossdorf orcid.org/0000-0001-7504-6511
J. F. Scheepens orcid.org/0000-0003-1650-2008
Johannes Heinze orcid.org/0000-0002-3556-5883